- 1Department of Molecular Medicine, University of Southern Denmark, Odense, Denmark
- 2Immunology Research Center, Tabriz University of Medical Sciences, Tabriz, Iran
- 3Department of Pathology, Odense University Hospital, Odense, Denmark
- 4Department of Clinical Research, University of Southern Denmark, Odense, Denmark
- 5Danish Institute for Advanced Study, University of Southern Denmark, Odense, Denmark
Background: Colorectal cancer (CRC) ranks as the third most prevalent cancer globally, highlighting the pressing need to address its development. Inflammation plays a crucial role in augmenting the risk of CRC and actively contributes to all stages of tumorigenesis. Consequently, targeting early inflammatory responses in the intestinal tract to restore homeostasis holds significant potential for preventing and treating CRC. Fibrinogen C domain-containing 1 (FIBCD1), a chitin-binding transmembrane protein predominantly found on human intestinal epithelial cells (IECs), has garnered attention in previous research for its ability to effectively suppress inflammatory responses and promote tissue homeostasis at mucosal barriers.
Methods: In this study, we investigated the role of FIBCD1 in CRC development using transgenic mice that mimic human expression of FIBCD1 at the intestinal mucosal barrier. To model aspects of CRC, we employed the azoxymethane/dextran sodium sulfate (AOM/DSS) mouse model. Additionally, we examined the expression pattern of FIBCD1 in surgical specimens obtained from human CRC patients by immunohistochemical methods. By accessing public data repositories, we further evaluated FIBCD1 expression in colon adenocarcinoma and explored survival outcomes associated with FIBCD1 expression.
Results: Here, we demonstrate that FIBCD1 substantially impacts CRC development by significantly reducing intestinal inflammation and suppressing colorectal tumorigenesis in mice. Furthermore, we identify a soluble variant of FIBCD1 that is significantly increased in feces during acute inflammation. Finally, we demonstrate increased expression of FIBCD1 by immunohistochemistry in human CRC specimens at more developed tumor stages. These results are further supported by bioinformatic analyses of publicly available repositories, indicating increased FIBCD1 expression in tumor tissues, where higher expression is associated with unfavorable prognosis.
Conclusion: Collectively, these findings suggest that FIBCD1 influences early inflammatory responses in the AOM/DSS model, leading to a reduction in tumor size and burden. The increased expression of FIBCD1 in human CRC samples raises intriguing questions regarding its role in CRC, positioning it as a compelling candidate and novel molecular target for future research.
1 Introduction
Colorectal cancer (CRC) is the third leading cause of cancer-related deaths with an incidence of more than 1.8 million annual cases (1). It is well established that inflammation plays a prominent role in tumorigenesis and the progression of several different types of cancers, including CRC (2–4). Prolonged intestinal inflammation observed in patients with inflammatory bowel disease (IBD) greatly increases the risk of CRC development, yet the molecular pathogenesis of colitis-associated colorectal cancer (CAC) remains poorly defined (5). It has been suggested that repeated cycles of cell damage as well as sustained production of reactive nitrogen and oxygen species at the site of inflammation can damage cellular DNA, and thereby favor the formation of malignant cells (6). Furthermore, the inflammatory microenvironment exhibits tumor-promoting effects through the production of inflammatory mediators that can drive cancer cell proliferation, aid metastasis, and influence the efficiency of chemotherapeutic drugs (2). In contrast to CRC, which generally arises from adenomatous polyps and mainly occurs in older patients, CAC is more frequently diagnosed in younger patients and arises from flat dysplastic mucosa as a consequence of chronic inflammation (7, 8).
Animal models of CAC, such as the azoxymethane (AOM)/dextran sodium sulfate (DSS) mouse model, have emerged as important tools that mimic aspects of the human disease and improve our understanding of the underlying pathological mechanisms (9). The AOM/DSS model is a two-step tumor model of CAC in which administration of AOM, a pro-carcinogen that causes DNA damage in colonic epithelial cells, is followed by repeated exposure to DSS that inflicts mucosal damage leading to chronic colitis as well as the development of distally located invasive adenocarcinomas (9–11). The AOM/DSS model effectively recapitulates the CAC development from inflammation to malignancy, allowing the investigation of pathways and novel target molecules that can lead to improved treatment strategies.
Fibrinogen C domain containing 1 (FIBCD1) is a membrane protein expressed in human epithelial cells at mucosal barrier surfaces, such as the gastrointestinal and respiratory tract (12). Acting as a pattern recognition receptor, FIBCD1 specifically recognizes acetylated compounds such as chitin, the structural component of e.g., invertebrates, insects, and fungi (13). Previous studies have shown that FIBCD1 expression in epithelial cells can ameliorate fungal-driven intestinal inflammation and promote tissue homeostasis (14, 15). Moreover, studies have demonstrated that FIBCD1 expression in lung epithelium can influence inflammatory responses to fungal pathogens (16, 17). Based on FIBCD1’s ability to dampen inflammatory responses in mucosal tissues, we hypothesized that enhanced FIBCD1 expression would lead to decreased intestinal inflammation and reduce the development of colitis-associated colon cancer. By employing transgenic mice that specifically express FIBCD1 in the intestinal epithelium and subjecting them to AOM/DSS treatment, we demonstrate that increased FIBCD1 expression ameliorates the development of CAC.
2 Materials and methods
2.1 Mice
Fibcd1-transgenic mice (TG), specifically overexpressing Fibcd1 in intestinal epithelial cells (IEC) via the Villin promotor (14), and wild-type littermates (WT) C57BL/6 mice were generated and maintained under standard conditions (12-h light/dark cycle, 21–24°C, and 55% relative humidity) at the Biomedical Laboratory, University of Southern Denmark. All experiments were performed using co-housed, sex- and age-matched littermates and conducted in accordance with the national ethical committee (Animal Experiments Inspectorate under Danish Ministry of Food, Agriculture and Fisheries, The Danish Veterinary and Food Administration, approval identification number: 2019-15-0201-00349).
2.2 AOM/DSS model
Colitis-associated colorectal cancer (CAC) was induced in mice by combinatory treatment with azoxymethane (AOM) and dextran sulfate sodium (DSS) as previously described (2). In brief, TG and WT littermates were randomized to receive a single intraperitoneal (i.p.) injection with AOM (10 mg/kg body weight, Sigma Aldrich). 3 days later, chronic colitis was induced by three cycles of 1.5% DSS (molecular weight 36,000–50,000 Da, Colitis Grade, MP Biomedicals) in drinking water for 5-7 days followed by two weeks of recovery with normal drinking water. Mice were monitored daily for weight and general health. Due to ethical considerations, mice were sacrificed if weight loss exceeded 20% of the initial body weight or if they showed significant signs of pain or distress.
2.3 Tissue collection and processing
Mice were euthanized by cervical dislocation 15 days after the final DSS treatment. The entire large intestines were harvested and measured. Afterward, the colons were separated, flushed with phosphate-buffered saline (PBS), cut open longitudinally, and spread on a filter paper with the intestinal lining facing up. The tissue specimens were stained with methylene blue (2% in PBS) for 1 minute, washed in PBS, and photographed for tumor counting and measuring. A piece of the tumor and the adjacent tissue were taken from each colon for RNA extraction. For histopathological analysis, the remaining tissues were destained with fresh PBS, swiss-rolled, and fixed in 10% buffered formalin (Sigma) for 48 h. The tissues were then embedded in paraffin, sectioned into 4 μm thick slides, and stained with hematoxylin and eosin (H&E).
2.4 RNA isolation and cDNA synthesis
RNA was extracted from colon tissue samples using TRI Reagent® (Sigma-Aldrich) according to the manufacturer’s instructions. For tissues isolated from DSS-treated mice, a lithium chloride (8 M LiCl) precipitation step was performed to remove DSS contamination from the RNA (18). RNA concentration was determined using a NanoDrop™ One spectrophotometer (Thermo Fisher Scientific) and the total RNA was converted into cDNA using the High-Capacity cDNA Reverse Transcription kit (Thermo Fisher Scientific) according to the manufacturer’s instructions.
2.5 Fecal DNA purification
Fecal samples were collected from WT and TG littermates at eight different time points: Before AOM treatment (day -3), at the initiation of DSS treatment (day 0), and on days 10, 22, 32, 44, 54, and 65 after AOM treatment. One or two fecal pellets from each mouse were collected, snap-frozen in liquid nitrogen, and stored at −80°C until further processing. Before DNA extraction, the samples were treated with lyticase (Sigma-Aldrich) for 1 h at 30°C, followed by bead-beating, and isolation using the QIAamp® Fast DNA Stool kit (Qiagen) as described previously (14).
2.6 RT-qPCR and qPCR
Quantification of gene expression from colonic and tumor tissues was performed using the TaqMan Gene Expression Assays (Applied Biosystems) and the SYBR green primers listed in Table 1. The data were generated on a StepOnePlus™ Real-Time PCR (Thermo Fisher Scientific) and the results were normalized to Hprt1 using the 2–ΔCt method. For fecal microbiota analysis, the SYBR green primers listed in Table 2 were used. Relative abundance was standardized to input DNA using 16S as a reference. To counteract DSS inhibition of PCRs, the samples were supplemented with 0.01 g/l spermine (Sigma-Aldrich) right before analysis (19).
2.7 Fecal inflammatory biomarkers
Fecal samples were suspended in PBS containing 2 mM EDTA to a final concentration of 200 mg/ml. Subsequently, the samples were homogenized, vortexed vigorously, and the supernatants were collected by centrifugation at 10.000 g for 10 min. Fecal lipocalin (LCN2) and calprotectin (CAL) levels were determined using enzyme-linked immunosorbent assays (ELISAs): Mouse Lipocalin-2/NGAL DuoSet® ELISA kit (Cat no. DY1857-05) and Calprotectin (Cat no. DY8598-05) (R&D systems) according to the manufacturer’s recommendations. For the detection of soluble FIBCD1 in fecal specimens a sandwich ELISA was developed using monoclonal antibodies raised against the fibrinogen-related domain of FIBCD1 previously produced (14). In brief, MaxiSorp 96-well plates (NUNC) were coated with 1µg/ml of monoclonal anti-FIBCD1 antibody (clone 11-14-40) in standard ELISA coating buffer (pH 9.5), followed by blocking in 5% BSA in PBS. PBS supplemented with 0.05% (vol/vol) Tween20 was used for all washing steps. Samples were loaded in diluent buffer (10 to 1,000-fold, diluted in PBS/1% BSA) and compared to a recombinant FIBCD1 standard. Soluble FIBCD1 and standards were detected using biotinylated 1 µg/ml of monoclonal anti-FIBCD1 antibody (clone 11-14-25) and quantified using streptavidin/HRP combined with TMB substrate according to conventional ELISA methods.
2.8 Histopathological score
Intestinal inflammation was assessed in a blinded fashion using a previously established protocol (20). Briefly, colonic sections were stained with H&E and scanned using the slide scanner NanoZoomer XR (HAMAMATSU, Japan). The sections were evaluated for disease severity based on scoring of inflammatory cell infiltration (graded 0-3) and overall colonic architecture (graded 0-3). The sum of the two values gave a total colitis severity score between 0 and 6. The analysis was performed using the NDP.view2 software (HAMAMATSU, Japan). Tumor burden and volume were analyzed using ImageJ v1.49 software.
2.9 Human surgical CRC specimens and FIBCD1 immunoscore
35 formalin-fixed paraffin-embedded tissue specimens prepared from surgically removed CRC patient material, as well as information about disease stage, were obtained from the Immunology Research Center, Tabriz University of Medical Sciences (Supplementary Table 1). Staining for FIBCD1 was performed on 4 µm tissue sections using the monoclonal anti-FIBCD1 antibody HG-HybB-12-2. The stainings were performed in parallel with validation controls using CHO cells expressing recombinant full-length FIBCD1 (13) and representative non-cancerous human colon specimens using an optimized protocol described previously (12). The semiquantitative immune score for FIBCD1 expression was performed on specimens obtained from 35 patients clinically diagnosed with CRC stage I (n=8), II (n=19), and III (n=8) using a scoring system consisting of a distribution score and an intensity score, as described previously (21). The FIBCD1 positive epithelial cell distribution was scored from 0 to 4 as follows: 0, less than 10% of the epithelial cells were positive for FIBCD1; 1, 10%–30% of the epithelial cells were positive for FIBCD1; 2, 31%–60%, 3, 61-90%, and 4, >90% of the epithelial cells were positive for FIBCD1. The intensity score was graded as 0, no expression; 1, weak expression; 2, moderate expression; and 3, strong expression. The sum of the distribution score and the intensity score composed the FIBCD1 immunoscore (0-7). All immunostainings were evaluated by a trained gastrointestinal pathologist blinded to clinical data.
2.10 Sample preparation and immunofluorescence microscopy
Representative paraffin-embedded colonic sections, each 4 μm thick, underwent deparaffinization followed by antigen retrieval by boiling for 20 minutes using citrate buffer at pH 6.0. The sections were left at room temperature for 1.5 hours in the citrate buffer before blocking with 3% BSA. Permeabilization and staining were performed in PBS containing 0.2% Triton X100 and 3% BSA using the monoclonal antibodies: anti-CD45-BV480 (clone 30-F11), anti-CD326-APC (clone G8.8), and anti-CD3-FITC (clone 17A2). The sections were counterstained with DAPI and mounted using Fluorescent Mounting Medium (Dako). Representative fluorescent images were captured and visualized with an Olympus APX100 Imaging System.
2.11 Validation of FIBCD1 gene expression in public data repositories
To assess the differential expression of FIBCD1 in colon adenocarcinoma (COAD) we analyzed tumor samples (n=274) from The Cancer Genome Atlas (TCGA) and compared them to normal samples from both TCGA and Genotype-Tissue Expression (GTEx) datasets (n=349), utilizing the Gene Expression Profiling Interactive Analysis (GEPIA) platform (http://www.gepia.cancer.pku.cn) (22). To investigate the impact of FIBCD1 expression on the survival outcomes of individuals diagnosed with colon cancer, we employed the Kaplan–Meier plotter survival analysis platform. The online tool KM plotter (http://www.kmplot.com) (23) comprises information from 1130 cancer patients, curated from 12 GEO gene expression datasets. The KM plotter generated survival plots, which included hazard ratios, 95% confidence intervals, and log-rank p-values for the selected gene probe. For analysis the optimal JetSet gene probe was used.
2.12 Statistical analysis
Statistical analysis was performed using GraphPad Prism v9.4.0 (GraphPad Software Inc, San Diego, California, USA). Student’s t-test with Welch’s correction for unequal SD was used to compare single parameters between two datasets. Single parameters with more than two datasets were analyzed using one-way ANOVA followed by the Tukey multiple comparisons test. Weight curves were analyzed by a mixed-effects model (REML) of two-way repeated measures ANOVA followed by Holm-Šídák’s multiple comparisons test. Correlation analysis was conducted using Spearman’s r-test. Simple Survival Analysis (Kaplan–Meier) was performed and the Log-rank (Mantel-Cox) test was used for comparison between the two groups. The results are presented as means + standard error of mean (SEM) and p values < 0.05 were considered statistically significant.
3 Results
3.1 FIBCD1 is expressed in human CRC tissues, is increased with disease progression and is associated with a poor prognosis.
To determine whether FIBCD1 is expressed in human colorectal cancer tissue and to evaluate the extent of FIBCD1 expression in relation to disease progression, we performed immunohistochemical staining of colonic tumor specimens isolated from patients diagnosed with CRC stage I-III (Figures 1A, B). Validation of antibody-specificity is depicted in Figure 1C, while representative stainings of FIBCD1 in non-cancerous human colon tissues are depicted in Figure 1D. In general, we found that FIBCD1 expression and distribution were highly variable across all three stages of disease severity assessed, not unlike what is also observed in non-cancerous control specimens. However, direct assessment of the tissue specimens based on disease progression revealed a significant increase in FIBCD1 expression and distribution at more advanced tumor staging (p < 0.0398). To build upon these findings, we evaluated publicly available resources/platforms to explore FIBCD1 in the context of colon cancer. Utilizing the Gene Expression Profiling Interactive Analysis (GEPIA) platform (www.gepia.cancer-pku.cn) (22), we assessed the differential expression of FIBCD1 in colon adenocarcinoma (COAD) by analyzing tumor samples (n=274) from The Cancer Genome Atlas (TCGA) and comparing them to normal samples from both TCGA and Genotype-Tissue Expression (GTEx) datasets (n=349). Consistent with our IHC data, the expression data show a highly variable but significant increase in FIBCD1 expression in tumor tissues compared to normal controls (p=0.01) (Figure 1E). Using the Kaplan–Meier plotter survival analysis platform (www.kmplot.com) (23), we investigated the impact of FIBCD1 expression on the survival outcomes of individuals diagnosed with colon cancer. The data curated from 12 GEO gene expression datasets showed that high FIBCD1 expression is associated with a poor prognosis (p=0.00018) (Figure 1F).
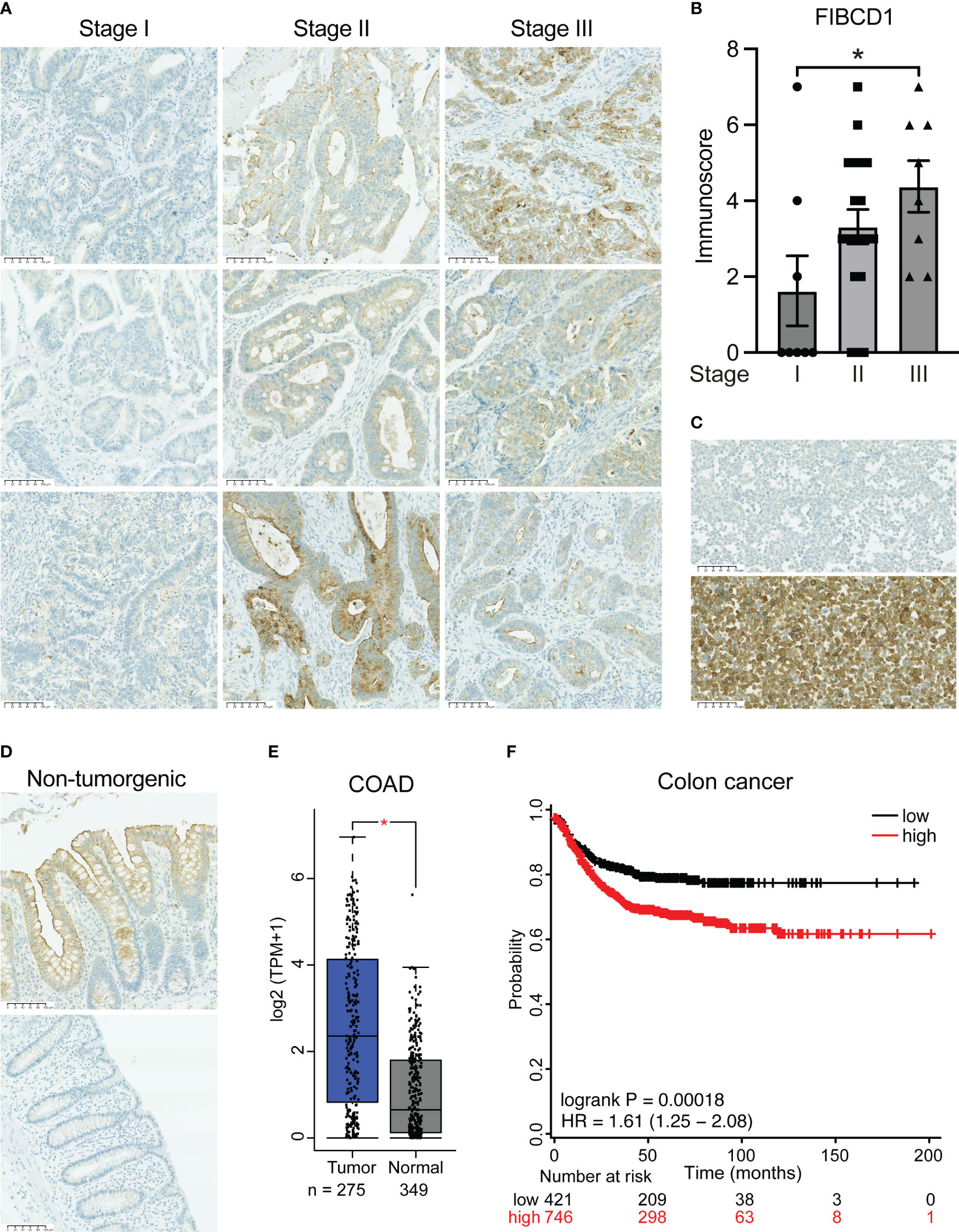
Figure 1 FIBCD1 expression in human colorectal cancer correlates with disease severity. (A) Representative immunohistochemical images of tumor tissues from patients with stage I-III CAC. Scalebar, 100 µm. (B) Statistical evaluation of FIBCD1 expression according to colorectal cancer stage I (n=8), II (n=19), and III (n=8). Data are presented as mean ± SEM, where dots represent individual specimens. Statistics: one-way ANOVA followed by post hoc Tukey test for multiple comparisons. *P < 0.05. (C) Validation of staining procedure employed using anti-FIBCD1 monoclonal antibody clone HG-Hyb-12-2. The representative images depict CHO cells (upper panel) and CHO cells expressing recombinant full-length FIBCD1 (lower panel). (D) Representative immunohistochemical images of non-tumorgenic human colon tissues. (E) Box plot diagram depicting gene expression of FIBCD1 in colon adenocarcinoma (COAD) and normal tissues analyzed via the GEPIA platform (www.gepia.cancer.pku.cn). Tumor n=274, normal n=349. Statistics, dots represent individual specimens, P = 0.01. (F) Kaplan-Meier survival curves showing the correlation between FIBCD1 gene expression and recurrence-free survival in 1130 colon cancer patients curated from 12 GEO gene expression datasets. The plot was prepared using the online analysis platform KM Plotter (www.kmplot.com). Statistics, P = 0.00018.
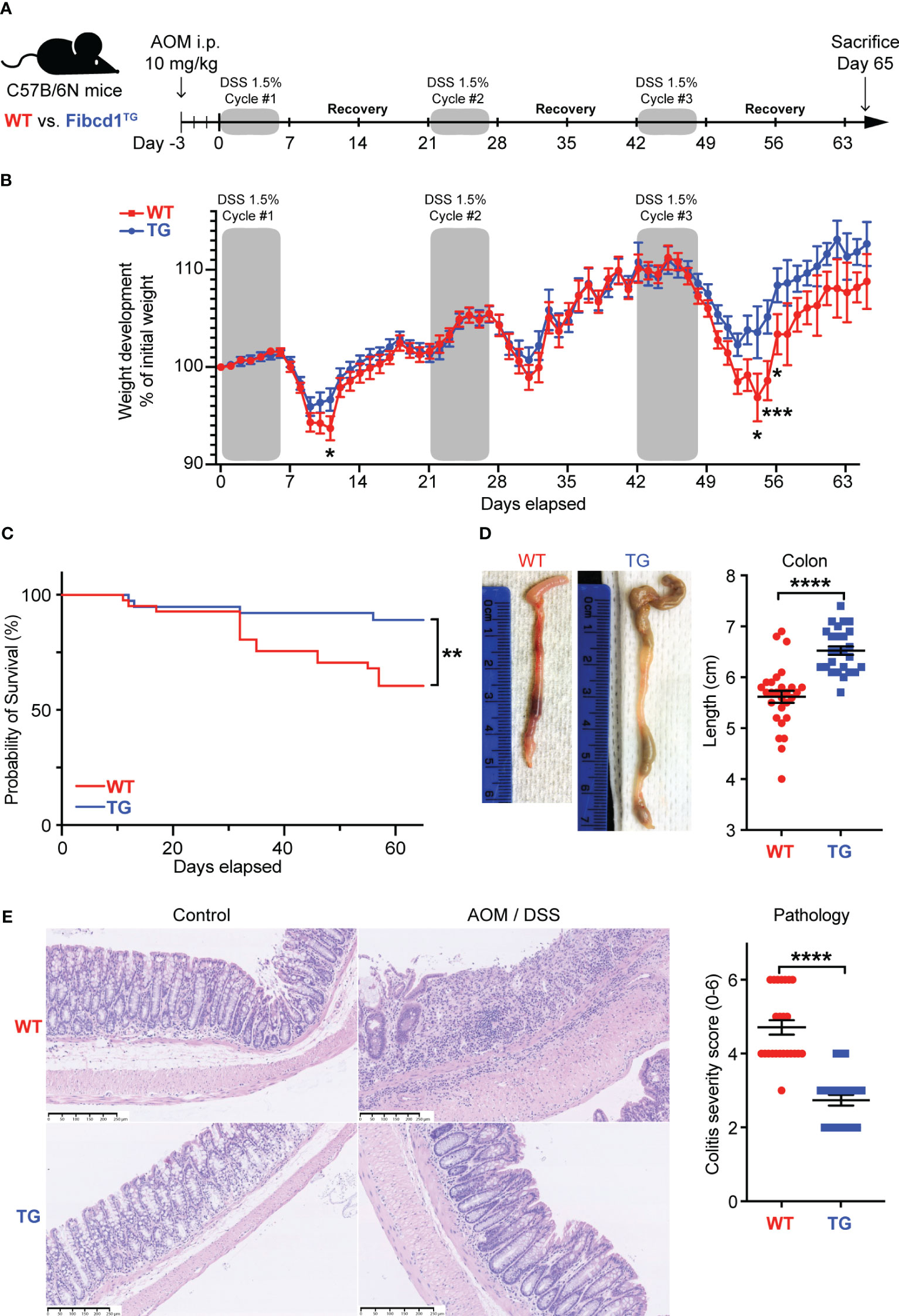
Figure 2 FIBCD1 overexpression attenuates colonic inflammation in a mouse model of colorectal cancer. (A) Schematic representation illustrating the experimental layout: Wildtype and Fibcd1-transgenic (TG) littermates received a single dose of AOM i.p. injection followed by three cycles of 1.5% DSS in drinking water. Mice were sacrificed 65 days after the experiment start. (B) Changes in the body weight of the AOM/DSS treated mice over the course of the experiment (n = 26-29 mice per group). (C) Kaplan-Meyer survival analysis of the WT and TG littermates exposed to AOM/DSS. (D) Representative images and statistics of colon lengths of WT and TG littermates at day 65 (n = 23-24 mice per group). Scalebar, 250 µm. (E) Representative H&E-stained images and evaluation of histological colitis severity (score for infiltration of inflammatory cells and colonic architecture) in colon tissue specimens from WT and TG littermates treated with AOM/DSS (n = 23-24 mice per group). Scale bar 250μm. Statistics: Results are pooled from three independent experiments and data are presented as mean ± SEM where dots represent individual mice. Mixed-effects model (REML) of Two-Way ANOVA repeated measures were performed for weight curves data in B, followed by post hoc Holm-Šídák’s multiple comparisons test. Simple Survival Analysis (Kaplan–Meier) was performed and the Log-rank (Mantel-Cox) test was used for the comparison of data in (C) Unpaired Student’s t-test was performed to analyze data in (D, E). *P < 0.05, **P < 0.01, ***P < 0.001, and ****,P < 0.0001.
3.2 Intestinal overexpression of FIBCD1 attenuates colonic inflammation in a mouse model for CAC
To investigate the potential significance of FIBCD1 on the development of CAC, we used Fibcd1-transgenic mice (TG), specifically overexpressing FIBCD1 in the intestinal epithelium as demonstrated in (14), and WT littermates in an AOM/DSS mouse model of CAC (Figure 2A). Validation of Fibcd1 expression in tumor and non-tumorigenic adjacent tissues from TG and WT littermates at termination is shown in Supplementary Figure 1. A hallmark of experimental colitis is a notable body weight loss and shortening of the colon (24). DSS-induced weight loss was significantly reduced following DSS treatments in TG mice compared to WT littermates (Figure 2B). In addition, Kaplan–Meier survival analysis revealed that TG mice had a higher survival rate compared to WT mice (p=0.006) (Figure 2C). At the time of the sacrifice, TG mice also showed significantly increased colon length compared to WT mice (p = 0.0007) (Figure 2D). Consistent with less severe disease symptoms, histopathological examination of the H&E-stained colon tissues revealed reduced intestinal colitis severity in TG mice, characterized by decreased infiltration of inflammatory cells and more normal-appearing overall mucosal architecture (p<0.0001) (Figure 2E).
3.3 Overexpression of FIBCD1 ameliorates the progression of CAC
It is well-recognized that chronic inflammation of the intestinal mucosa can play a fundamental role in the initiation and progression of CRC (25). To assess whether FIBCD1 expression influences tumor formation and development, the colons from WT and TG littermates sacrificed at day 65 in the CAC model were dissected longitudinally and stained with methylene blue. In these tissues, we observed a striking reduction in the number of visible tumors (p<0.0001) in TG mice in comparison to WT mice (Figures 3A, B). Representative H&E stainings of well-developed adenocarcinomas in WT and TG littermates are visualized in Figure 3C. In addition, the total tumor volume was significantly reduced in TG mice in comparison to WT mice (p = 0.0019) (Figure 3D). Moreover, correlation analyses demonstrated a significant positive association between tumor load and histopathological colitis severity score (R2 = 0.342, p<0.0001), which was further supported by significant negative correlations between colon length vs. colitis severity (R2 = 0.471, p<0.0001) as well as tumor load (R2 = - 0.146, p=0.0068) (Figures 3E–G).
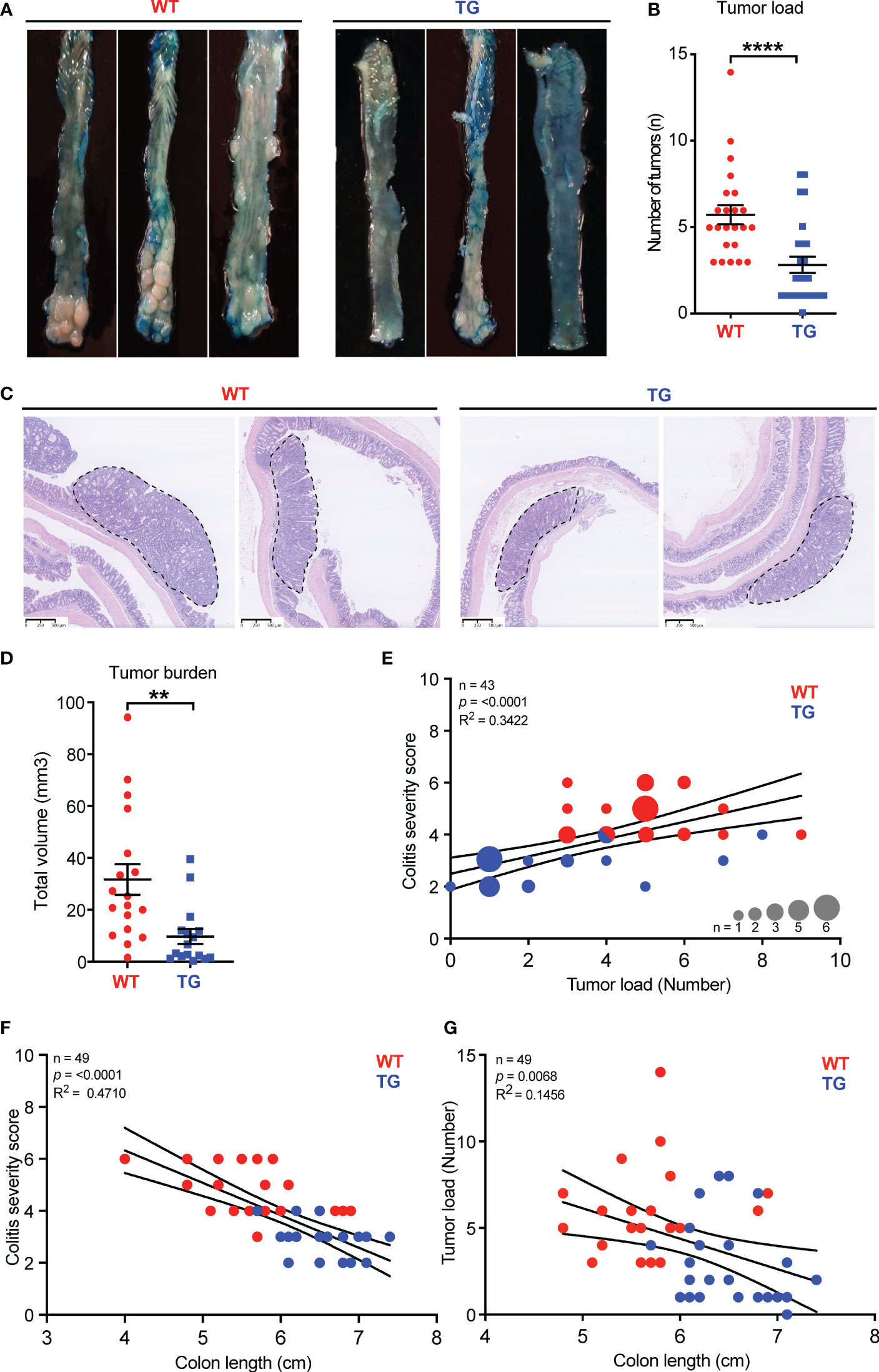
Figure 3 FIBCD1 overexpression ameliorates the development and progression of tumors in a mouse model of colorectal cancer. (A) Representative images of colonic tumors stained with methylene blue from WT and TG littermates at day 65. (B) Number of tumors in WT and TG littermates at the end of the experiment (n = 20-23 mice per group). (C) Representative H&E stainings of well-developed adenocarcinoma from WT and TG littermates, dotted lines indicate circumference. (D) Total volume of tumors in WT and TG littermates at the end of the experiment (n = 20-23 mice per group). (E) Correlation analysis between colitis severity score and tumor load, (F) between colitis severity score and colon length, and (G) between tumor load and colon length in WT and TG littermates (n = 43-49). Statistics: Results are pooled from two independent experiments and data are presented as mean ± SEM. The differences in dot size indicate the number of animals at that particular level. Unpaired Student’s t-test was performed to analyze data in (B, D) nonparametric Spearman r test was performed to analyze data in (E–G). **P < 0.01, and ****,P < 0.0001.
3.4 FIBCD1 reduces the levels of biomarkers associated with intestinal disease and inflammation
Fecal biomarkers such as calprotectin (CAL) and lipocalin-2 (LCN2) have been identified as important diagnostic tools to evaluate inflammatory activity in the intestinal mucosa (26). To further examine the impact of FIBCD1 on disease severity, fecal samples were collected from WT and TG mice over the course of the experiment. We found that TG mice had significantly lower levels of LCN2 and CAL in the feces compared to WT littermates at several time points (Figure 4A, B). To examine the impact of FIBCD1 at the transcriptional level, RNA was isolated from the dissected colonic tumors and the tumor-adjacent tissue for RT-qPCR analysis. In the tumor tissues, we observed reduced expression of inflammatory genes in the TG mice in comparison to WT littermates. Expression levels of Il1b, one of the major mediators of inflammatory responses, as well as the pleiotropic cytokine Il6, were both significantly reduced in the tumors of TG mice compared to WT mice. Likewise, mRNA levels for inflammatory markers such as Il17, Tnfa, and Ccl2 as well as the anti-microbial peptide Reg3g, were significantly downregulated in TG mice (Figure 4C). To examine the possible presence of infiltrating immune cells in the developed tumors, we performed an analysis by immunofluorescence microscopy of paraffin-embedded sections containing well-developed tumors from WT and TG littermates. In these tissues, we identified distinct subsets of infiltrating CD45+ immune cells, including CD3+ T cells in representative tumors from WT and TG littermates (Supplementary Figure 2). Although not quantitative, we observed an apparent reduction in staining for CD45 and CD3 in TG tumors compared to WT in a limited number of specimens assessed. Finally, we also investigated the gene expression profile in the tumor-adjacent tissue and observed a similar response profile to the tumor tissues, characterized by a general decrease in pro-inflammatory signature, with downregulation of transcripts for Il1b, Il6, Il17, Ccl2, as well as reduced expression of Il2 in the TG mice (Supplementary Figure 3).
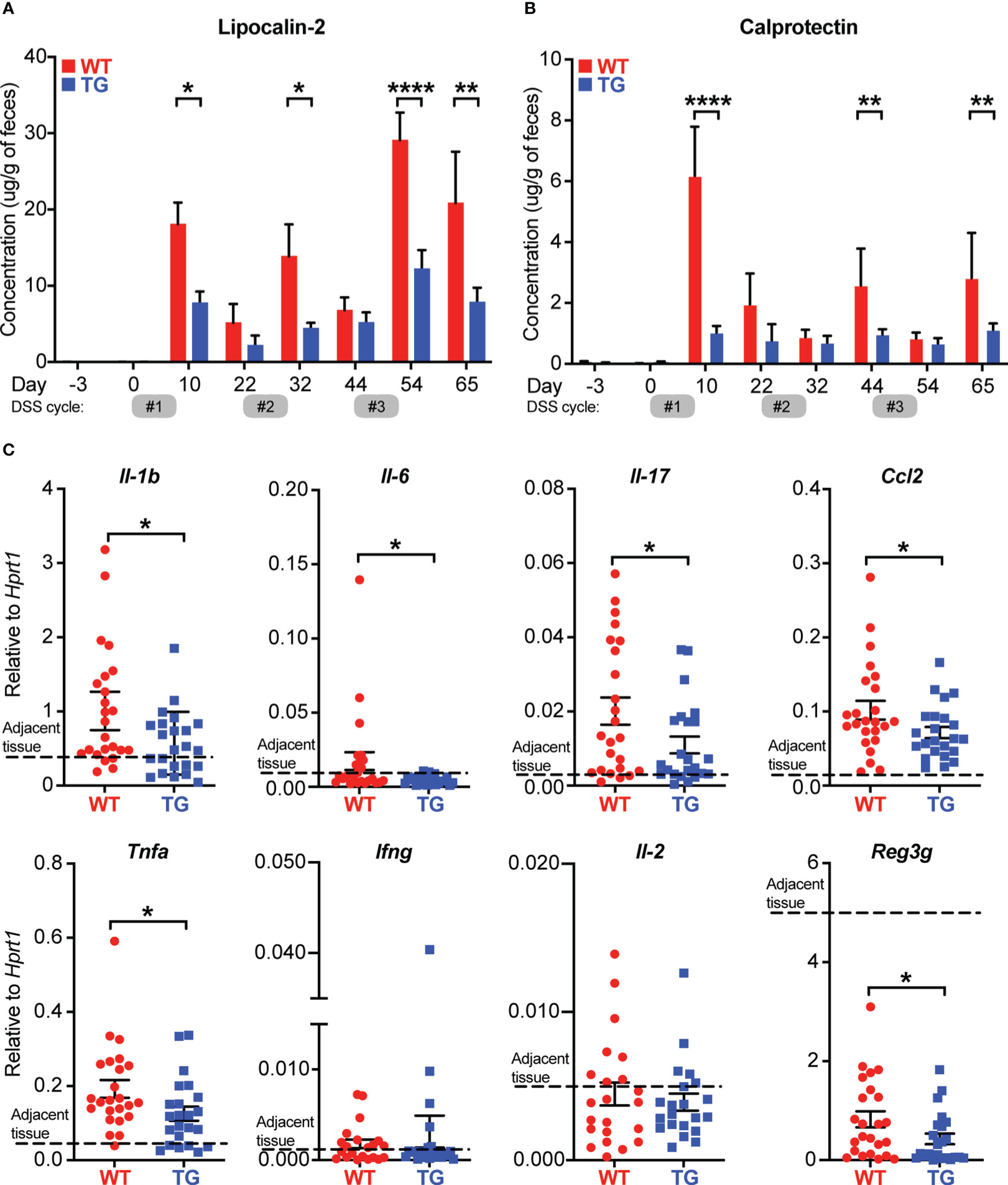
Figure 4 FIBCD1 influences biomarkers associated with disease and inflammation. (A, B) LCN2 and Calprotectin (inflammatory biomarkers) were quantified in fecal specimens before disease induction and at different time points throughout the experiment (n = 12 samples per group). (C) Relative expression of the indicated genes in tumor tissues isolated from WT and TG littermates (n=23-24 mice per group). The dotted line represents the average level of expression in adjacent tissues (WT and TG combined). Statistics: Results are pooled from two independent experiments and data are presented as mean ± SEM where dots represent individual mice. Mixed-effects model (REML) of Two-Way ANOVA repeated measures were performed for data in A and B, followed by post hoc Holm-Šídák’s multiple comparisons test. Unpaired Student’s t-test was performed to analyze data in (C). *p < 0.05, **p < 0.01, and ****,p < 0.0001.
3.5 FIBCD1 alters the expression of apoptosis-related genes in colonic tumors
To further assess the effects of FIBCD1 on CAC tumor progression, we analyzed the isolated tumor tissues from AOM/DSS-treated WT and TG littermates for the expression of CAC-related oncogenes and tumor suppressor genes as well as genes involved in cell proliferation and apoptosis. When quantified we found no significant difference in the expression of well-known oncogenes like Kras, Erbb2, Sox9, Ctnnb1, Stat3, and Pik3ca between the tumor tissues isolated from WT and TG littermates (Figure 5A). Moreover, we found no difference in the expression of genes related to tumor suppression like Apc, Tp53, Tgfbr2, and Smad4 (Figure 5B). In contrast, genes associated with apoptosis like Bcl2, Xiap, and Casp1 were found to be marginally yet significantly upregulated in TG when compared to WT littermates (Figure 5C). Additionally, the expression of these cancer-related genes was also assessed in adjacent tissues, where no changes between WT and TG littermates were observed (Supplementary Figure 4).
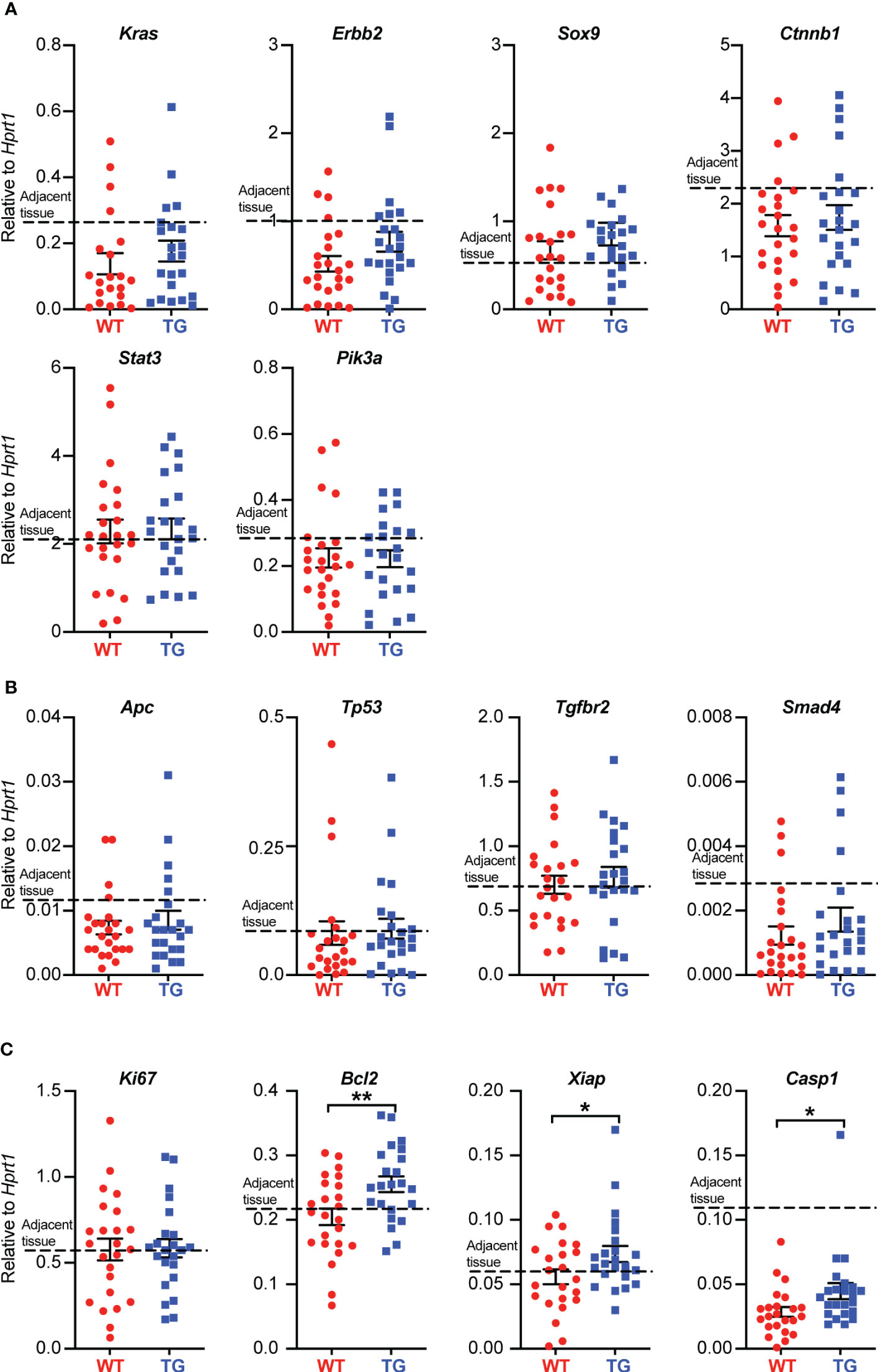
Figure 5 FIBCD1 alters the expression of genes associated with apoptosis in colonic tumor tissues. (A) Relative expression of the indicated oncogenes, (B) tumor suppressor genes, and (C) genes associated with cell proliferation and apoptosis in tumor tissues isolated from WT and TG littermates (n=23-24 mice per group). The dotted line represents the average level of expression in adjacent tissues (WT and TG combined). Statistics: Results are pooled from two independent experiments and data are presented as mean ± SEM where dots represent individual mice. Unpaired Student’s t test was performed to analyze data. *P < 0.05 and **P < 0.01.
3.6 FIBCD1’s protective effect against CAC is not mediated by compositional changes in the intestinal microbiota
FIBCD1 is a chitin-binding receptor that has previously been shown to limit and control fungal dysbiosis in the intestines (14). Following, we asked whether the protective effect of FIBCD1 on the development of CAC could be partially mediated by compositional changes to the intestinal microbiota. To investigate this, we purified fecal DNA from WT and TG mice and evaluated changes in the microbiota by qPCR analysis. In general, we observed a very limited presence of fungal DNA in the collected fecal samples (fungal Ct values were consistently above 32 or non-detected) in all sample material assessed using well-established quantitative procedures (14, 27). Moreover, we found no significant differences in the overall fungal burden between co-housed TG and WT littermates regardless of disease progression (Figure 6A). Similarly, we did not detect significant changes in the abundance of the biggest bacterial phyla including Firmicutes and Bacteroides at any time point (Figures 6B, C).
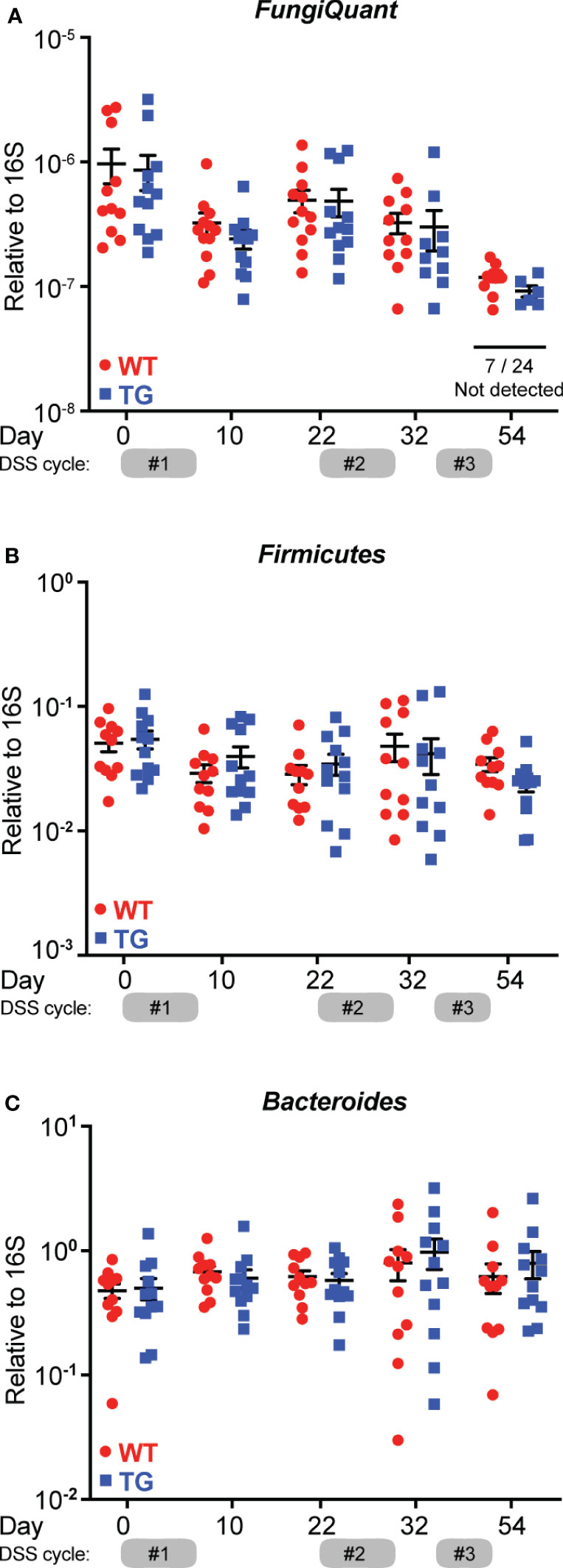
Figure 6 FIBCD1 protective effects are independent of the intestinal microbiota composition. (A) Quantification of total fungal presence in feces from co-housed WT and TG littermates using broad coverage primers (FungiQuant) at different time points throughout the experiment (n = 12 samples per group). (B, C) Quantification of relative abundance of Firmicutes and Bacteroides relative to total 16S in feces from co-housed WT and TG littermates at different time points throughout the experiment (n = 12 samples per group). Statistics: Results are pooled from two independent experiments and data are presented as mean ± SEM where dots represent individual mice. No significant differences were observed when data were analyzed using a mixed-effects model (REML) of Two-Way ANOVA repeated measures followed by post hoc Holm-Šídák’s multiple comparisons test.
3.7 Soluble FIBCD1 is detected in feces from transgenic mice and increases during acute inflammation
Recently, Graca et al. have identified FIBCD1 as a possible myokine that is shed from the surface of cells expressing full-length transmembrane FIBCD1 (28). To investigate FIBCD1 as a potential shedded marker of inflammation in the context of CRC, we developed a sandwich ELISA that recognizes epitopes contained in a shedded variant of FIBCD1 (Figure 7A). After validation and optimization of the assay, we assessed for the presence of shedded FIBCD1 in fecal sample supernatants collected from WT and TG littermates in the AOM/DSS mouse model of CAC. In accordance with previous reports of very low expression of Fibcd1 in mouse intestinal tissues (14), our assay did not detect any presence of shedded FIBCD1 protein in the sample material collected from WT mice. However, in sample material collected from TG littermates, we observed a high concentration of shedded FIBCD1 across all samples evaluated (Figure 7B). Moreover, we observed a highly significant increase in shedded FIBCD1 in samples collected from TG littermates during the acute phase (day 10) of the initial inflammation induced by DSS treatment (p<0.0001).
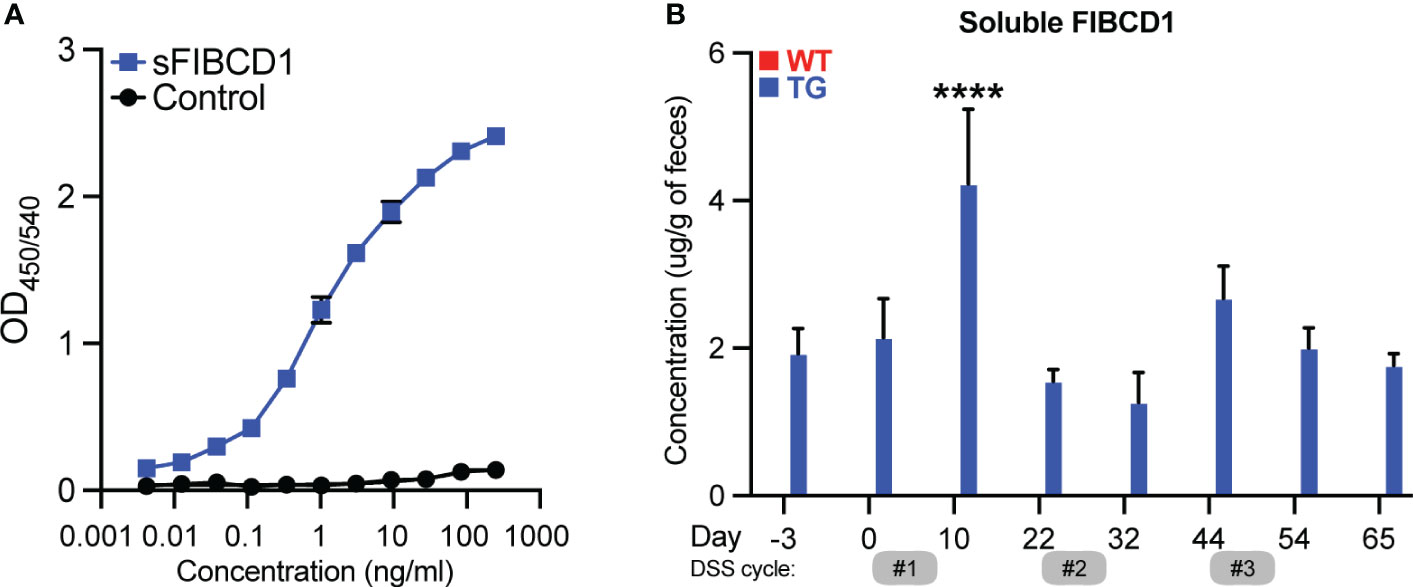
Figure 7 Soluble FIBCD1 is detectable in feces at increased levels during acute inflammation. (A) Representative binding curves demonstrating the ELISA developed for detection and quantification of soluble FIBCD1. Recombinant FIBCD1 was used as a standard (0-250 ng) and resuspended in a fixed amount of fecal sample suspension (10mg/ml). (B) Quantification of soluble FIBCD1 in feces isolated from WT and TG littermates at different time points throughout the experiment (n = 12 samples per group). Statistics: Results are pooled from two independent experiments and data are presented as mean ± SEM. All samples from WT mice were below detection. Mixed-effects model (REML) of Two-Way ANOVA repeated measures were performed for TG sample groups, followed by post hoc Holm-Šídák’s multiple comparisons test. ****,P < 0.0001.
4 Discussion
In the present study, we sought to examine the role of intestinal FIBCD1 in CRC. In human material from CRC patients, we identified expression of FIBCD1 in the tumor tissues where expression and distribution were found to correlate with the disease stage, supporting a possible role for FIBCD1 in human CRC. Utilizing transgenic mice overexpressing FIBCD1 in the intestinal tissues, thereby mimicking its expression in the human gut, we found that expression of FIBCD1 significantly alleviated intestinal inflammation, increased survival, and decreased tumor formation as well as burden in a mouse model of inflammation-driven colorectal cancer. Moreover, we observed that the reduction of disease severity was associated with decreased expression of several canonical inflammatory mediators implicated in the development and progression of CAC (29, 30). On the other hand, overexpression of FIBCD1 did not exhibit a notable impact on the expression of genes associated with tumor development or suppression. Additionally, FIBCD1 did not appear to mediate disease protection by inducing significant fungal or bacterial alterations in the gut microbiota. Interestingly, we observed the presence of soluble FIBCD1 protein in the feces of TG mice, with a significant increase in the initial phases of intestinal inflammation. These findings suggest the potential use of shedded FIBCD1 as a biomarker of inflammation and/or disease progression in future applications.
By employing the AOM/DSS mouse model, we present novel evidence that increased expression of FIBCD1 in the intestines significantly attenuated the development of CAC. This attenuation was characterized by a decrease in the number of tumors and overall tumor burden. Chronic inflammation often precedes cancer development and plays a decisive role in various steps involved in cancer initiation and progression (31, 32). It is well established that aberrant inflammatory responses in the tumor microenvironment can promote cancer cell survival, neoangiogenesis, and metastatic spread as well as interfere with anti-cancer therapy (31). FIBCD1 overexpression in IECs resulted in attenuated intestinal inflammation characterized by reduced weight loss, increased colon length, and decreased epithelial damage. Furthermore, we observed reduced levels of calprotectin and lipocalin-2 in FIBCD1-overexpressing mice. Both calprotectin and lipocalin-2 are important fecal biomarkers associated with intestinal disease and inflammation (26). Previous studies have shown that fecal lipocalin-2 is elevated in patients with CRC and associated with tumor progression (33, 34). Similarly, high levels of fecal calprotectin have been shown to be a pre-diagnostic factor for patients with CRC and positively correlate with tumor growth (35).
The observed reduction in tumor burden and tumor size in TG mice was associated with decreased expression of inflammatory cytokines such as Il-1b, Il-6, Il-17, and Ccl2 in the tumor and tumor-adjacent tissue, as well as a likely reduction in tumor-infiltrating immune cells. Inflammatory mediators in the tumor microenvironment can enhance tumor development via the recruitment of additional immune cells that, in turn, provide survival signals and growth factors for cancer cells (4, 31). For example, IL-6 expression has been shown to drive early tumorigenesis in CAC via activation of the STAT3 pathway that increases the survival of pre-malignant IECs (36). IL-17 has been shown to have a pro-tumorigenic effect in CRC development as well as promote resistance to anti-cancer therapy (37). As inflammation is instrumental in tumor development in CAC, the ability of FIBCD1 to modulate and dampen inflammatory responses in the intestinal tract may be one of its primary anti-tumorigenic actions observed in the AOM/DSS model. On the other hand, high levels of FIBCD1 expression in tissue samples from patients with gastric and hepatic cancer have been associated with increased tumor size and invasiveness as well as overall poorer prognosis and survival (38, 39). Our analysis of publicly available resources indicates that FIBCD1 expression is increased in colon cancer compared to normal tissues, and elevated FIBCD1 expression is linked to a poorer survival prognosis. In agreement with this, our immunohistochemical analysis of colorectal cancer tissue showed increased expression of FIBCD1 at later tumor stages. A possible explanation for this discrepancy may be that during tumor progression, as a means of immune escape mechanism, carcinogenic epithelial cells increase expression of FIBCD1 to induce a pro-tumor microenvironment characterized by increased local immune suppression that favors cancer growth and metastasis (3). Hence, FIBCD1 might be an emerging novel molecular target and/or a prognostic biomarker of several different gastrointestinal malignancies.
The anti-inflammatory properties of FIBCD1 reported in previous studies have been attributed to direct fungal recognition and reduced fungal outgrowth (14, 16, 40). Additionally, mycobiota sequence analyses showed compositional changes in the fungal microbiota in FIBCD1-overexpressing mice compared to WT littermates (14). Emerging research has shown an association between fungal dysbiosis and CRC development and progression (41–43). Increased burden of Candida spp. as well as altered composition of various fungal communities have been identified in CRC patients compared to healthy controls (44). In this study, we generally observed a very limited fungal presence in co-housed WT and TG littermates maintained in our animal facility. Thus, we were not able to detect significant changes in the total abundance of fungal DNA or prevalent species like Candida spp., suggesting that the reduced intestinal inflammation observed in TG mice was not linked to decreased fungal burden or changes in fungal composition observed in previous studies (14).
Nevertheless, the anti-tumor properties of FIBCD1 were evident in the AOM/DSS model, suggesting an alternative mechanism for the protective effect of FIBCD1 in inflammation-associated carcinogenesis. In our study, we found no evidence of FIBCD1 expression directly influencing the expression of well-known oncogenes or tumor suppressor genes within the tumor tissues. On the other hand, we observed a slight but significant difference in apoptosis-related gene expression, which could be contributed to various reasons including changes in the tumor-associated immune cell composition. However, Graca et al. have recently demonstrated that FIBCD1 can be cleaved from the cell surface, generating a secreted form of FIBCD1 protein containing the fibrinogen-related domain that participates in ligand binding (28). Soluble FIBCD1 has been shown to interact with and regulate integrin subunits as well as reduce expression of inflammatory genes in skeletal muscle cells during cancer-induced myofiber atrophy (28). Building upon these findings, we developed a sandwich ELISA to evaluate the potential presence of FIBCD1 in feces due to the shedding of epithelial-derived membrane-bound FIBCD1 in response to inflammation and the development of CAC. While we were not able to detect and quantify endogenous soluble FIBCD1 in feces from WT mice, our assay proved sensitive for the detection and quantification of soluble FIBCD1 in fecal specimens from TG mice. Proteolytic cleavage of membrane proteins is a fundamental molecular process that serves as a functional switch of hundreds of target proteins involved in most physiological processes as well as cancer and inflammation (45, 46). In our animal model, we observed a highly significant increase of soluble FIBCD1 in feces during the initial phase of inflammation and disease development. Although transgenic and thereby highly artificial by nature, the discovery of a soluble variant of FIBCD1 derived from membrane-bound protein may be highly relevant for the understanding of FIBCD1 function in relation to human inflammation and CAC development. Regulatory T-cells and macrophages with suppressive phenotypes can exhibit integrin profiles distinct from those of inflammatory infiltrates (47–49). As a result, cleaved FIBCD1 may bind to specific integrins present on cell types involved in immunosuppression and tissue repair. Identifying binding partners of soluble FIBCD1, as well as conducting a comprehensive analysis of immune cell composition in transgenic animals, could provide valuable insights into the immunomodulatory functions of FIBCD1 in CAC. Moreover, the data provoke the question of whether soluble FIBCD1 might serve as a novel noninvasive biomarker of disease development in human pathologies like IBD or CAC.
In a recent study by Fell et al., FIBCD1 was shown to function as an endocytic receptor for glycosaminoglycans, thereby regulating signaling within the brain’s extracellular matrix and playing a pivotal role in the structure and function of the nervous system (50). Notably, mice lacking Fibcd1 exhibited impaired hippocampal-dependent learning and diminished synaptic remodeling, underscoring the significant involvement of FIBCD1 in shaping the structural and functional aspects of the nervous system. Furthermore, single-cell and spatial transcriptomic sequencing of hippocampal tissue from mice with Parkinson’s disease identified Fibcd1 as one of the highly differentially regulated genes in the CA3 subregion, involved in memory and cognitive functions (51, 52). These studies further illustrate that FIBCD1 exhibits pleiotropic functions, with emerging novel roles in various diseases and physiological functions extending beyond chitin recognition.
Taken together, the present study using an AOM/DSS-induced CAC mouse model found that overexpression of FIBCD1 in the intestinal epithelium resulted in decreased inflammation and tumor formation. The presence of soluble FIBCD1 during the initial stages of intestinal inflammation introduces intriguing possibilities concerning its potential as an immune response regulator during inflammation. It is conceivable that shedded FIBCD1 contributes to the intricate regulation of the inflammatory milieu by facilitating immune cell recruitment and migration through integrin binding or by selectively binding to specific cell types and influencing their transcriptomic profile, thus mitigating inflammatory responses. Understanding the precise role and mechanisms of action of soluble FIBCD1 has potential to pave the way for innovative therapeutic approaches targeting inflammation-related diseases. Given the well-established connection between inflammation and cancer development, it is plausible that FIBCD1 may hold anti-carcinogenic properties that stem from its ability to influence early inflammatory responses in the intestinal tract. Although the mechanistic basis underlying this protective effect remains to be determined, our observations suggest that FIBCD1 might be a novel molecular target in CAC and an attractive candidate for future research.
Data availability statement
The original contributions presented in the study are included in the article/Supplementary Material. Further inquiries can be directed to the corresponding author.
Ethics statement
The studies involving human material were approved by The Ethics Committee of Tabriz University of Medical Sciences, Tabriz, Iran. The studies were conducted in accordance with the local legislation and institutional requirements. The animal studies were approved by The Animal Experiments Inspectorate under the Danish Ministry of Food, Agriculture and Fisheries, The Danish Veterinary and Food Administration. The studies were conducted in accordance with the local legislation and institutional requirements.
Author contributions
VS: Conceptualization, Formal analysis, Funding acquisition, Investigation, Methodology, Writing – review & editing, Writing – original draft. MD: Data curation, Formal analysis, Funding acquisition, Investigation, Methodology, Validation, Writing – original draft, Writing – review & editing. BP: Investigation, Writing – review & editing. SS: Investigation, Writing – review & editing. SS: Investigation, Writing – review & editing. SD: Investigation, Methodology, Validation, Writing – review & editing. GS: Conceptualization, Resources, Supervision, Writing – review & editing. UH: Conceptualization, Resources, Supervision, Writing – review & editing. BB: Conceptualization, Resources, Supervision, Writing – review & editing. JM: Conceptualization, Funding acquisition, Investigation, Methodology, Project administration, Resources, Supervision, Visualization, Writing – original draft, Writing – review & editing.
Funding
The author(s) declare financial support was received for the research, authorship, and/or publication of this article. This work was supported by grants from the Novo Nordic Foundation (grant NNF19OC0058349 to JM), Frimodt-Heineke Fonden (grant to JM), Hørslev Fonden (grant 203866 to JM), Louis-Hansens Fond (grant 22-2B-10882 to JM), Beckett fonden (grant 22-2-8795 to JM), Fonden til lægevidenskabens Fremme (grant 20-L-0219 to VS), Brødrene Hartmanns Fond (grant A38338 to MD), Torben og Alice Frimodts Fond (grant 10204 to MD).
Acknowledgments
We thank Seyda Ünsal for her valuable assistance with sectioning paraffin-embedded tissues. We thank all members of the Moeller lab for discussions and critical reading of the manuscript.
Conflict of interest
The authors declare that the research was conducted in the absence of any commercial or financial relationships that could be construed as a potential conflict of interest.
Publisher’s note
All claims expressed in this article are solely those of the authors and do not necessarily represent those of their affiliated organizations, or those of the publisher, the editors and the reviewers. Any product that may be evaluated in this article, or claim that may be made by its manufacturer, is not guaranteed or endorsed by the publisher.
Supplementary material
The Supplementary Material for this article can be found online at: https://www.frontiersin.org/articles/10.3389/fonc.2023.1280891/full#supplementary-material
References
1. Rawla P, Sunkara T, Barsouk A. Epidemiology of colorectal cancer: incidence, mortality, survival, and risk factors. Prz Gastroenterol (2019) 14(2):89–103. doi: 10.5114/pg.2018.81072
2. Mantovani A, Allavena P, Sica A, Balkwill F. Cancer-related inflammation. Nature (2008) 454(7203):436–44. doi: 10.1038/nature07205
3. Zhao H, Wu L, Yan G, Chen Y, Zhou M, Wu Y, et al. Inflammation and tumor progression: signaling pathways and targeted intervention. Signal Transduct Target Ther (2021) 6(1):263. doi: 10.1038/s41392-021-00658-5
4. Greten FR, Grivennikov SI. Inflammation and cancer: triggers, mechanisms, and consequences. Immunity (2019) 51(1):27–41. doi: 10.1016/j.immuni.2019.06.025
5. Porter RJ, Arends MJ, Churchhouse AMD, Din S. Inflammatory bowel disease-associated colorectal cancer: translational risks from mechanisms to medicines. J Crohns Colitis (2021) 15(12):2131–41. doi: 10.1093/ecco-jcc/jjab102
6. Kruk J, Aboul-Enein HY. Reactive oxygen and nitrogen species in carcinogenesis: implications of oxidative stress on the progression and development of several cancer types. Mini Rev Med Chem (2017) 17(11):904–19. doi: 10.2174/1389557517666170228115324
7. Vetter LE, Merkel S, Bénard A, Krautz C, Brunner M, Mittelstädt A, et al. Colorectal cancer in Crohn's colitis is associated with advanced tumor invasion and a poorer survival compared with ulcerative colitis: a retrospective dual-center study. Int J Colorectal Dis (2021) 36(1):141–50. doi: 10.1007/s00384-020-03726-4
8. Soh JS, Jo SI, Lee H, Do EJ, Hwang SW, Park SH, et al. Immunoprofiling of colitis-associated and sporadic colorectal cancer and its clinical significance. Sci Rep (2019) 9(1):6833. doi: 10.1038/s41598-019-42986-1
9. Parang B, Barrett CW, Williams CS. AOM/DSS model of colitis-associated cancer. Methods Mol Biol (2016) 1422:297–307. doi: 10.1007/978-1-4939-3603-8_26
10. De Robertis M, Massi E, Poeta ML, Carotti S, Morini S, Cecchetelli L, et al. The AOM/DSS murine model for the study of colon carcinogenesis: From pathways to diagnosis and therapy studies. J Carcinog (2011) 10:9. doi: 10.4103/1477-3163.78279
11. Thaker AI, Shaker A, Rao MS, Ciorba MA. Modeling colitis-associated cancer with azoxymethane (AOM) and dextran sulfate sodium (DSS). J Vis Exp (2012) 67). doi: 10.3791/4100-v
12. von Huth S, Moeller JB, Schlosser A, Marcussen N, Nielsen O, Nielsen V, et al. Immunohistochemical localization of fibrinogen C domain containing 1 on epithelial and mucosal surfaces in human tissues. J Histochem Cytochem (2018) 66(2):85–97. doi: 10.1369/0022155417743694
13. Schlosser A, Thomsen T, Moeller JB, Nielsen O, Tornøe I, Mollenhauer J, et al. Characterization of FIBCD1 as an acetyl group-binding receptor that binds chitin. J Immunol (2009) 183(6):3800–9. doi: 10.4049/jimmunol.0901526
14. Moeller JB, Leonardi I, Schlosser A, Flamar AL, Bessman NJ, Putzel GG, et al. Modulation of the fungal mycobiome is regulated by the chitin-binding receptor FIBCD1. J Exp Med (2019) 216(12):2689–700. doi: 10.1084/jem.20182244
15. Andersen MCE, Johansen MW, Nissen T, Nexoe AB, Madsen GI, Sorensen GL, et al. FIBCD1 ameliorates weight loss in chemotherapy-induced murine mucositis. Support Care Cancer (2021) 29(5):2415–21. doi: 10.1007/s00520-020-05762-w
16. Jepsen CS, Dubey LK, Colmorten KB, Moeller JB, Hammond MA, Nielsen O, et al. FIBCD1 binds aspergillus fumigatus and regulates lung epithelial response to cell wall components. Front Immunol (2018) 9:1967. doi: 10.3389/fimmu.2018.01967
17. Bhattacharya S, Amarsaikhan N, Maupin AJ, Schlosser A, Füchtbauer EM, Holmskov U, et al. FIBCD1 deficiency decreases disease severity in a murine model of invasive pulmonary aspergillosis. Immunohorizons (2021) 5(12):983–93. doi: 10.4049/immunohorizons.2100092
18. Viennois E, Chen F, Laroui H, Baker MT, Merlin D. Dextran sodium sulfate inhibits the activities of both polymerase and reverse transcriptase: lithium chloride purification, a rapid and efficient technique to purify RNA. BMC Res Notes (2013) 6:360. doi: 10.1186/1756-0500-6-360
19. Krych Ł, Kot W, Bendtsen KMB, Hansen AK, Vogensen FK, Nielsen DS. Have you tried spermine? A rapid and cost-effective method to eliminate dextran sodium sulfate inhibition of PCR and RT-PCR. J Microbiol Methods (2018) 144:1–7. doi: 10.1016/j.mimet.2017.10.015
20. Erben U, Loddenkemper C, Doerfel K, Spieckermann S, Haller D, Heimesaat MM, et al. A guide to histomorphological evaluation of intestinal inflammation in mouse models. Int J Clin Exp Pathol (2014) 7(8):4557–76.
21. Nexoe AB, Pilecki B, Von Huth S, Husby S, Pedersen AA, Detlefsen S, et al. Colonic epithelial surfactant protein D expression correlates with inflammation in clinical colonic inflammatory bowel disease. Inflammation Bowel Dis (2019) 25(8):1349–56. doi: 10.1093/ibd/izz009
22. Tang Z, Li C, Kang B, Gao G, Li C, Zhang Z. GEPIA: a web server for cancer and normal gene expression profiling and interactive analyses. Nucleic Acids Res (2017) 45(W1):W98–w102. doi: 10.1093/nar/gkx247
23. Kovács SA, Fekete JT, Győrffy B. Predictive biomarkers of immunotherapy response with pharmacological applications in solid tumors. Acta Pharmacol Sin (2023) 44(9):1879–89. doi: 10.1038/s41401-023-01079-6
24. Chassaing B, Aitken JD, Malleshappa M, Vijay-Kumar M. Dextran sulfate sodium (DSS)-induced colitis in mice. Curr Protoc Immunol (2014) 104:15.25.1–15.25.14. doi: 10.1002/0471142735.im1525s104
25. Schmitt M, Greten FR. The inflammatory pathogenesis of colorectal cancer. Nat Rev Immunol (2021) 21(10):653–67. doi: 10.1038/s41577-021-00534-x
26. Zollner A, Schmiderer A, Reider SJ, Oberhuber G, Pfister A, Texler B, et al. Faecal biomarkers in inflammatory bowel diseases: calprotectin versus lipocalin-2-a comparative study. J Crohns Colitis (2021) 15(1):43–54. doi: 10.1093/ecco-jcc/jjaa124
27. Liu CM, Kachur S, Dwan MG, Abraham AG, Aziz M, Hsueh PR, et al. FungiQuant: a broad-coverage fungal quantitative real-time PCR assay. BMC Microbiol (2012) 12:255. doi: 10.1186/1471-2180-12-255
28. Graca FA, Rai M, Hunt LC, Stephan A, Wang YD, Gordon B, et al. The myokine Fibcd1 is an endogenous determinant of myofiber size and mitigates cancer-induced myofiber atrophy. Nat Commun (2022) 13(1):2370. doi: 10.1038/s41467-022-30120-1
29. Knüpfer H, Preiss R. Serum interleukin-6 levels in colorectal cancer patients–a summary of published results. Int J Colorectal Dis (2010) 25(2):135–40. doi: 10.1007/s00384-009-0818-8
30. Francescone R, Hou V, Grivennikov SI. Cytokines, IBD, and colitis-associated cancer. Inflammation Bowel Dis (2015) 21(2):409–18. doi: 10.1097/MIB.0000000000000236
31. Grivennikov SI, Greten FR, Karin M. Immunity, inflammation, and cancer. Cell (2010) 140(6):883–99. doi: 10.1016/j.cell.2010.01.025
32. Afify SM, Hassan G, Seno A, Seno M. Cancer-inducing niche: the force of chronic inflammation. Br J Cancer (2022) 127(2):193–201. doi: 10.1038/s41416-022-01775-w
33. McLean MH, Thomson AJ, Murray GI, Fyfe N, Hold GL, El-Omar EM. Expression of neutrophil gelatinase-associated lipocalin in colorectal neoplastic progression: a marker of Malignant potential? Br J Cancer (2013) 108(12):2537–41. doi: 10.1038/bjc.2013.264
34. Chaudhary N, Choudhary BS, Shah SG, Khapare N, Dwivedi N, Gaikwad A, et al. Lipocalin 2 expression promotes tumor progression and therapy resistance by inhibiting ferroptosis in colorectal cancer. Int J Cancer (2021) 149(7):1495–511. doi: 10.1002/ijc.33711
35. Blad N, Palmqvist R, Karling P. Pre-diagnostic faecal calprotectin levels in patients with colorectal cancer: a retrospective study. BMC Cancer (2022) 22(1):315. doi: 10.1186/s12885-022-09440-4
36. Grivennikov S, Karin E, Terzic J, Mucida D, Yu GY, Vallabhapurapu S, et al. IL-6 and Stat3 are required for survival of intestinal epithelial cells and development of colitis-associated cancer. Cancer Cell (2009) 15(2):103–13. doi: 10.1016/j.ccr.2009.01.001
37. Wang K, Kim MK, Di Caro G, Wong J, Shalapour S, Wan J, et al. Interleukin-17 receptor a signaling in transformed enterocytes promotes early colorectal tumorigenesis. Immunity (2014) 41(6):1052–63. doi: 10.1016/j.immuni.2014.11.009
38. Jiang C, Zhu J, Zhou P, Zhu H, Wang W, Jin Q, et al. Overexpression of FIBCD1 is predictive of poor prognosis in gastric cancer. Am J Clin Pathol (2018) 149(6):474–83. doi: 10.1093/ajcp/aqy013
39. Wang Y, Sun M, Liu J, Liu Y, Jiang C, Zhu H, et al. FIBCD1 overexpression predicts poor prognosis in patients with hepatocellular carcinoma. Oncol Lett (2020) 19(1):795–804. doi: 10.3892/ol.2019.11183
40. Pilecki B, Moeller JB. Fungal recognition by mammalian fibrinogen-related proteins. Scand J Immunol (2020) 92(4):e12925. doi: 10.1111/sji.12925
41. Wang Y, Ren Y, Huang Y, Yu X, Yang Y, Wang D, et al. Fungal dysbiosis of the gut microbiota is associated with colorectal cancer in Chinese patients. Am J Transl Res (2021) 13(10):11287–301.
42. Zhang Z, Zheng Y, Chen Y, Yin Y, Chen Y, Chen Q, et al. Gut fungi enhances immunosuppressive function of myeloid-derived suppressor cells by activating PKM2-dependent glycolysis to promote colorectal tumorigenesis. Exp Hematol Oncol (2022) 11(1):88. doi: 10.1186/s40164-022-00334-6
43. Luan C, Xie L, Yang X, Miao H, Lv N, Zhang R, et al. Dysbiosis of fungal microbiota in the intestinal mucosa of patients with colorectal adenomas. Sci Rep (2015) 5:7980. doi: 10.1038/srep07980
44. Coker OO, Nakatsu G, Dai RZ, Wu WKK, Wong SH, Ng SC, et al. Enteric fungal microbiota dysbiosis and ecological alterations in colorectal cancer. Gut (2019) 68(4):654–62. doi: 10.1136/gutjnl-2018-317178
45. Lichtenthaler SF, Lemberg MK, Fluhrer R. Proteolytic ectodomain shedding of membrane proteins in mammals-hardware, concepts, and recent developments. EMBO J (2018) 37(15). doi: 10.15252/embj.201899456
46. Düsterhöft S, Jung S, Hung CW, Tholey A, Sönnichsen FD, Grötzinger J, et al. Membrane-proximal domain of a disintegrin and metalloprotease-17 represents the putative molecular switch of its shedding activity operated by protein-disulfide isomerase. J Am Chem Soc (2013) 135(15):5776–81. doi: 10.1021/ja400340u
47. Sun H, Lagarrigue F, Wang H, Fan Z, Lopez-Ramirez MA, Chang JT, et al. Distinct integrin activation pathways for effector and regulatory T cell trafficking and function. J Exp Med (2021) 218(2). doi: 10.1084/jem.20201524
48. Klann JE, Kim SH, Remedios KA, He Z, Metz PJ, Lopez J, et al. Integrin activation controls regulatory T cell-mediated peripheral tolerance. J Immunol (2018) 200(12):4012–23. doi: 10.4049/jimmunol.1800112
49. Dash SP, Chakraborty P, Sarangi PP. Inflammatory monocytes and subsets of macrophages with distinct surface phenotype correlate with specific integrin expression profile during murine sepsis. J Immunol (2021) 207(11):2841–55. doi: 10.4049/jimmunol.2000821
50. Fell CW, Hagelkruys A, Cicvaric A, Horrer M, Liu L, Li JSS, et al. FIBCD1 is an endocytic GAG receptor associated with a novel neurodevelopmental disorder. EMBO Mol Med (2022) 14(9):e15829. doi: 10.15252/emmm.202215829
51. Jia E, Sheng Y, Shi H, Wang Y, Zhou Y, Liu Z, et al. Spatial transcriptome profiling of mouse hippocampal single cell microzone in parkinson's disease. Int J Mol Sci (2023) 24(3). doi: 10.3390/ijms24031810
Keywords: Fibcd1, intestinal epithelial cells, AOM/DSS, CRC, inflammation
Citation: Shahgoli VK, Dubik M, Pilecki B, Skallerup S, Schmidt SG, Detlefsen S, Sorensen GL, Holmskov U, Baradaran B and Moeller JB (2023) Expression of FIBCD1 by intestinal epithelial cells alleviates inflammation-driven tumorigenesis in a mouse model of colorectal cancer. Front. Oncol. 13:1280891. doi: 10.3389/fonc.2023.1280891
Received: 21 August 2023; Accepted: 06 November 2023;
Published: 28 November 2023.
Edited by:
Shuyun Rao, Feinstein Institute for Medical Research, United StatesReviewed by:
Haizhu Song, Medical School of Nanjing University, ChinaSokchea Khou, Oregon Health and Science University, United States
Copyright © 2023 Shahgoli, Dubik, Pilecki, Skallerup, Schmidt, Detlefsen, Sorensen, Holmskov, Baradaran and Moeller. This is an open-access article distributed under the terms of the Creative Commons Attribution License (CC BY). The use, distribution or reproduction in other forums is permitted, provided the original author(s) and the copyright owner(s) are credited and that the original publication in this journal is cited, in accordance with accepted academic practice. No use, distribution or reproduction is permitted which does not comply with these terms.
*Correspondence: Jesper B. Moeller, jbmoeller@health.sdu.dk
†These authors have contributed equally to this work