- 1Department of Gynaecology and Obstetrics, The Second Affiliated Hospital of Fujian Medical University, Quanzhou, Fujian, China
- 2Centre of Neurological and Metabolic Research, The Second Affiliated Hospital of Fujian Medical University, Quanzhou, Fujian, China
- 3Group of Neuroendocrinology, Garvan Institute of Medical Research, Sydney, NSW, Australia
Endometrial cancer (EC) is one of the most common gynecological cancers, and its risk factors include obesity and metabolic, genetic, and other factors. Recently, the circadian rhythm has also been shown to be associated with EC, as the severity of EC was found to be related to night work and rhythm disorders. Therefore, circadian rhythm disorders (CRDs) may be one of the metabolic diseases underlying EC. Changes in the circadian rhythm are regulated by clock genes (CGs), which in turn are regulated by non-coding RNAs (ncRNAs). More importantly, the mechanism of EC caused by ncRNA-mediated CRDs is gradually being unraveled. Here, we review existing studies and reports and explore the relationship between EC, CRDs, and ncRNAs.
1 Introduction
Endometrial cancer (EC) is a malignancy of the endometrial epithelium. The annual incidence of EC is very high. Worldwide, 417,367 cases of EC were reported in 2020, making it the sixth most common cancer among women (1). Continuous exposure to exogenous or endogenous estrogen without progesterone antagonism is a risk factor for endometrial cancer. Other risk factors, such as obesity, tamoxifen use, insulin resistance, type 2 diabetes, and polycystic ovary syndrome, can increase the risk of EC (2). The main symptom of EC is abnormal uterine bleeding, which can be accompanied by vaginal secretions and uterine infection (3). When a patient presents with any of these symptoms, abdominal and pelvic examinations should be considered (4). The primary clinical treatment for EC is surgery, including total hysterectomy, bilateral salpingo-oophorectomy, and adjuvant therapy. However, the 5-year survival rates of patients with stage IVA and IVB EC are only 17% and 15%, respectively, although 67% of these patients display early signs of the disease (5). Therefore, finding a new treatment strategy is urgent.
The circadian rhythm is a stable regulatory system in the human body and is regulated by several hormones, particularly melatonin (MLT). Some studies have shown that MLT is involved in the regulation of epithelial-mesenchymal transformation and subsequent tumor invasion (6–10) as well as in inhibiting osteosarcoma (6) and ovarian cancer stem cells (7). At the molecular level, circadian rhythms are regulated by clock genes (CGs). Many diseases are caused by the abnormal expression of these genes, including cancer, endocrine, cardiovascular, and psychological diseases (11, 12). As a genetic disease, cancer is caused by uncontrolled growth and one reason for this is changes in circadian pathway genes (13). Furthermore, non-coding RNAs (ncRNAs), including microRNAs (miRNAs), long non-coding RNAs (lncRNAs), and circular RNAs (circRNAs), are involved in the regulation of CGs, such as miR-576-5p (14), miR-126-5p (15).
In this paper, we reviewed the latest progress in EC caused by CRDs and mediated by ncRNAs. Additionally, we attempted to summarize the relationship between ncRNAs, circadian rhythms, and EC.
2 Endometrial cancer
Historically, proliferative lesions that occur in glands without cytological atypia are called “hyperplasia” and have a 2% cancer risk, while those with cytological atypia are called “atypical hyperplasia” and have a 23% cancer risk (16). Endometrial intraepithelial neoplasia (EIN) is now recognized to precede atypical endometrial hyperplasia and is considered a precursor lesion of endometrial cancer (17).
The etiology of EC is not completely clear; however, it includes a variety of risk factors, such as BMI, as analyzed by Aune et al. (18). Using data from 22,320 cases, high BMI at 18–25 years of age, waist and hip circumferences, waist-to-hip ratio, height, and weight gain (over 10kg) were associated with an increased risk of EC. In other words, there is a positive correlation between body fat, weight gain, height, and the risk of EC. Renehan et al. (19) also reported that every 5 kg/m2 increase in BMI raises the risk of developing EC. Fisher et al. (20) reported that although tamoxifen reduced the incidence of breast cancer, it increased the risk of EC. Crosbie et al. (2) reported that insulin resistance, hyperinsulinemia, type 2 diabetes, and polycystic ovary syndrome (PCOS) could promote endometrial hyperplasia, which might be associated with EC.
Estrogen promotes endometrial hyperplasia; periodic menstruation and estrogen-antagonistic progesterone work together to maintain endometrial health. In obese women, the adipose tissue converts adrenal androgen to estrogen, forming a hyperestrogenic state (2). This state may interfere with the normal proliferation of the endometrium and increase the risk of EC. Additionally, Modugno et al. (21) showed that obesity is a chronic pro-inflammatory state that promotes the development of an inflammatory microenvironment and is accompanied by high levels of circulating c-reactive protein (CRP), interleukin-6 (IL-6), and tumor necrosis factor-alpha (TNF-α). These inflammatory markers may mediate the changes in the endometrial immune microenvironment.
Tamoxifen can stimulate endometrial hyperplasia. The stimulating effect of the long-term use of tamoxifen may be the mechanism that increases the risk of EC (20). Similarly, insulin resistance, hyperinsulinemia, type 2 diabetes, and PCOS can reduce the circulation levels of estrogen-binding proteins, insulin-like growth factor (IGF)-1, sex hormone-binding globulin, and IGF-binding protein, and improve their efficiency to stimulate endometrial growth (2) (Figure 1).
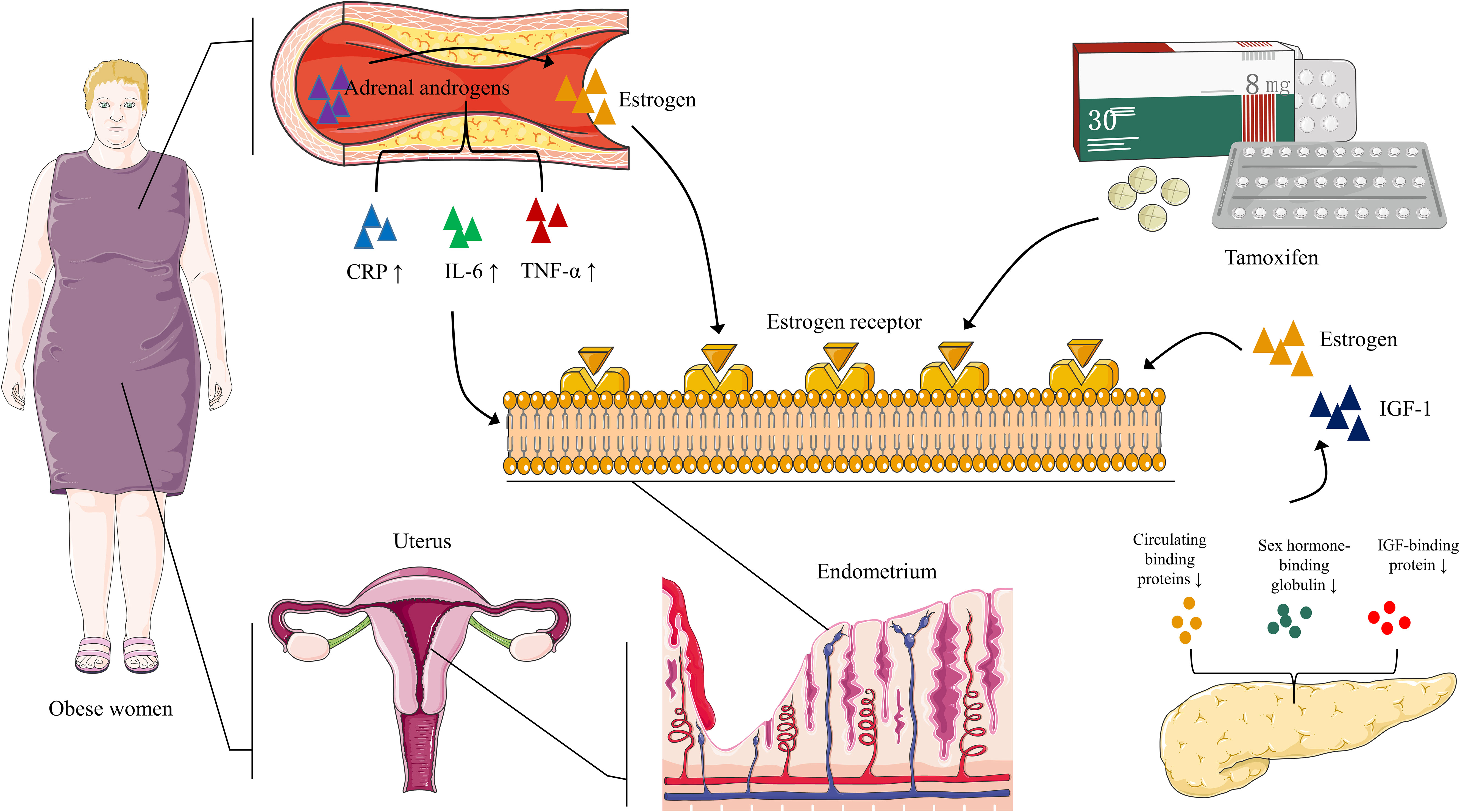
Figure 1 EC: pathways to carcinogenesis In obese women, adipose tissue aromatizes adrenal androgens into estrogen, leading to a hyperestrogenic state. Obesity also promotes high circulating CRP, IL-6, and TNF-α levels, which may be associated with changes in the endometrial immune microenvironment. Prolonged tamoxifen use promotes estrogen binding to its receptor, further enhancing the hyperestrogenic state. Insulin resistance, hyperinsulinemia, type 2 diabetes, and PCOS inhibit circulating binding proteins of estrogen and insulin-like growth factor (IGF)-1, sex hormone-binding globulin, and IGF-binding protein. As a result, estrogen and IGF-1 bioavailability increases, stimulating the endometrium.
Total hysterectomy and bilateral salpingo-oophorectomy are cornerstones of EC treatment and can be performed using open or minimally invasive techniques. Minimally invasive surgery is the first treatment choice for early-stage EC when the uterus is completely resectable. Minimally invasive surgery has the advantages of a short hospital stay, less blood loss, less pain, and low perioperative incidence (22–25).
3 Circadian rhythm disorders
Sleep and wakefulness have distinct circadian rhythms and are two bodily functional states. Sleep restores vitality, strengthens the immune system, maintains brain and heart health, consolidates memory (26). The circadian rhythm regulates the sleep cycle, and an appropriate circadian rhythm results in normal cell growth and survival. Various factors regulate circadian rhythms, one of the most important of which is MLT. Light-generated neural signals regulate MLT metabolism via the retinohypothalamic tract (RHT)-suprachiasmatic nucleus (SCN)-paraventricular nucleus (PVN)-brainstem-spinal cord (levels T1–T3)-superior cervical ganglion (SCG)-pineal gland pathway (27). Since MLT promotes sleep and reduces daily activity performance, inappropriate light stimulation can produce circadian rhythm disorders (CRDs) and disrupt MLT metabolism in the pineal gland (27).
Sun et al. demonstrated an increase in the number of macrophages in tissues and organs during circadian rhythm disturbances. Several studies have suggested a potential link between circadian rhythms and the cell division cycle (28–30) as well as malignancy (31). This occurs when the timing of daily activities is out of sync with an individual’s innate chronotype (32). CRDs may cause defects in the regulation of cell proliferation (33). For example, disturbances in circadian rhythms caused by night work may increase the risk of breast and prostate cancer (34–36) as well as EC (32, 37).
3.1 Clock genes
The first clock gene discovered in Drosophila is called the “period” gene (38, 39). There are at least 12 known CGs in mammals: Period1 (PER1), Period2 (PER2), Period3 (PER3), Cryptochrome 1 (CRY1), Cryptochrome 2 (CRY2), Circadian Locomotor Output Cycles Kaput (CLOCK), the transcription factor Aryl Hydrocarbon Receptor Nuclear Translocator-Like (ARNTL), Timeless (TIM) (13), Retinoic acid-related Orphan Nuclear Receptor (ROR) (40–42), Neuronal PAS domain protein-2 (NPAS2) (42, 43), Nuclear Receptor Subfamily 1 Group D members 1 and 2 (NR1D1 and NR1D2, respectively, also known as REV-ERB alpha and beta, respectively) (42, 44), and Casein Kinase I Epsilon (CSNK1E) (42, 45). Among these, PER1, PER2, and NPAS2 are associated with EC (46, 47). AT-rich interaction domain 1A (ARID1A) may be involved in the progression of EC by regulating the CGs BHLHE41 and ARNTL (48). The following sections discuss the relationship between CGs and EC.
3.1.1 Period1 and period2
PER1 and PER2 may be involved in mechanisms underlying EC onset and progression. Wang et al. (46) found that a high expression of PER1 and PER2 was associated with a better prognosis in EC. In their study, western blotting showed that the expression of PER1 and PER2 decreased in the rhythm group, whereas the expression of breast carcinoma amplified sequence 4 (BCAS4), tubulin beta-2B chain (TUBB2B), and Roof Plate-Specific Spondin-4 (RSPO4) increased in the breast cancer group. The high expression of PER1 indicates that the survival time of patients with EC is longer, while the high expression of TUBB2B indicates a lower survival rate. TUBB2B is related to diffuse and symmetrical aberrations in cerebral cortex development, and its importance in the central nervous system reveals its potential role in regulating circadian rhythms. In addition, they transfected Ishikawa cells with overexpressed PER1 plasmid and found that the apoptosis rate was significantly increased after 24h, and cell invasion was disturbed after 24h and 48h (46). A loss of PER expression suppresses the diurnal oscillation of decidualized human endometrial stromal cells (49). It is reported that BCAS4, TUBB2B, and RSPO4 regulate cancer development by interacting with other proteins (50–52). All in all, the severity of EC is associated with CRDs, and factors such as the CGs PER1 and PER2 may regulate the mechanisms of EC onset and development.
3.1.2 AT-rich interaction domain 1A
The switch/sucrose non-fermentable (SWI/SNF) complex is a nucleosome-remodeling factor found in both eukaryotes and prokaryotes (53). Through transcriptional control, it participates in the regulation of gene expression and is essential for cancer growth (54). SWI/SNF is a multi-subunit complex that includes AT-rich interaction domain 1A (ARID1A). ARID1A is one of the most commonly mutated genes in human cancers, such as colorectal cancer (55, 56), gastric cancer (57, 58), pancreatic cancer (59), esophageal adenocarcinoma (60), liver cancer (61), ovarian clear cell carcinoma (62), and endometrioid carcinoma (63–65). As reported by Hanyang Hu et al. (48), ARID1A regulates the binding of ER to clock gene enhancers in EC. ARID1A depletion affects chromatin accessibility and ER binding in enhancers, leading for the downregulation of CGs ARNTL and BHLHE41, eventually favoring attenuation of endometrial cancer cell proliferation and metastasis. In addition, a decreased ARID1A expression was linked to shorter progression-free survival in patients with endometrial-associated cancer. In summary, ARID1A and circadian rhythm genes can be regarded as novel diagnostic markers and potential targets for the treatment of EC (53).
3.1.3 Neuronal PAS domain protein-2
NPAS2, the longest CG in humans, is a mammalian transcription factor with a length of 176.68 kb (47). The PAS domain of NPAS2 binds to heme as a prosthetic group, making heme-based signal transduction possible, thereby playing a key role in generating circadian rhythms (66). NPAS2 participates in the cell cycle and the DNA damage response (67). A recent study showed that high NPAS2 expression is associated with poor survival in patients with EC (47). The data from that study show that NPAS2 is positively correlated with poor prognosis in EC. In addition, overexpression of NPAS2 significantly induces the proliferation of Ishikawa cells, while silenced NPAS2 inhibits the growth of AN3CA cells, and these situations are likely due to the influence of NPAS2 expression on the G1 and S phases of the cell cycle. This suggests that NPAS2 can be used as an indicator for the diagnosis and treatment of EC. Moreover, the researchers analyzed and predicted the expression correlation between miRNAs and NPAS2 in UCEC using the starBase database and found that NPAS2 was negatively correlated with miR-17-5p (R=-0.119, p=2.09E-02) and miR-93-5p (R=-0.091, p=7.96E-02), and positively correlated with miR-106a-5p (R=0.111, p=3.21E-02) and miR-381-3p (R=0.198, p=1.11E-04) (47).
To sum up, PER1 and PER2 may regulate EC pathogenesis and progression, ARID1A affects EC cell growth and metastasis, and NPAS2 affects EC cell proliferation and apoptosis. Focusing on these CGs and exploring corresponding targeted therapy may lead to a potential tool for improving the effectiveness of EC therapy (Table 1).
4 Endometrial cancer and circadian rhythm disorders
A study showed that age, education, smoking, type of work, marital status, fertility, menopause, gynecological history, hypertension, and shift time were all related to the severity of EC (46). When uncontrollable factors (such as age and menopause) were excluded, the correlation between rhythm-related factors and EC was the strongest (R ≈ 0.1). In order to control the diurnal functioning of the whole body, the circadian rhythm makes the behavior pattern consistent with ambient light and darkness, supporting body function by predicting and coordinating the necessary metabolic procedures (68). Disorders of the circadian rhythm disrupt the metabolic balance in the body. Endometrial proliferation, secretion, and shedding occur periodically, and the disruption of this cycle elevates the risk of disease. Working at night is one such case. Viswanathan et al. (69) reported that night shift work might increase the risk of EC. Besides, Von Behren et al. (32) explored the relationship between EC and chronotypes and found that post-menopausal women with evening chronotypes were more likely to develop EC, especially those with a body mass index (BMI) of 30 or higher. According to a study of sleep/night shift characteristics of patients with EC conducted by Wang et al. (46), the severity of EC is associated with night shift and rhythm disorders. In addition, people who work at night are exposed to inappropriately timed light, causing cortisol, body temperature, and MLT rhythms to be out of sync (70). As discussed above, the effect of CRDs on EC is ultimately mediated by CGs.
5 Non-coding RNAs
NcRNAs affect circadian rhythms through the gene-effector protein-circadian rhythm axis. NcRNAs are mainly composed of miRNAs, lncRNAs, and circRNAs (71, 72), and they play an important role in tumor development (73, 74). Here, we discuss the influence of miRNAs and lncRNAs on CRDs. MiRNAs are small ncRNAs, 19–24 nucleotides long, whereas lncRNAs are longer than 200 nucleotides. LncRNAs control gene expression by altering the function of transcription, splicing, translation, or miRNAs (75). Ray et al. (13) found that a subset of ncRNAs changes in cancer tissue, with target sites on certain CG mRNAs that can directly influence the abundance of these clock genes; another subset of ncRNAs targets specific oncogenes or tumor suppressor genes and is directly regulated by CGs. The potential use of ncRNAs in disease diagnosis has become widespread. Gharib et al. (76) examined the levels of miR-31 in 100 patients with breast cancer and their adjacent normal breast tissues using RT-PCR and concluded that miR-31 is expressed at low levels in breast cancer. Similarly, Zhao et al. (77) concluded that serum miR-205-5p is a valuable biomarker for lung cancer diagnosis because it promotes the proliferation and metastasis of lung cancer cells by regulating TP53INP1. Herein, we summarize the information that has become available in recent years.
By selectively targeting the ZBT4/Sp1 axis, miR-576-5p may affect the ability of EC cells to proliferate, migrate, and invade (14). Circ_0002577 downregulates miR-126-5p in concert with MACC1 to promote EC invasion and metastasis (15). MiR-1271-5p overexpression prevents EC cell proliferation, migration, and invasion by targeting its downstream target, CTNND1, and induces cell death (78). MiR-202-5p (79), miR-197 (80), miR-298 (81), and miR-105 (82) have similar effects to miR-1271-5p and can target downstream genes to prevent the proliferation, migration, and invasion of EC cells. CLOCK-controlled miR-455-5p regulates circadian rhythms by accelerating the degradation of clock mRNA (83). In short, the above-mentioned miRNAs related to CGs are usually stable in normal tissues but are irregularly expressed in abnormal tissues, especially in tumors (84). Nonetheless, we did not observe many EC-related miRNAs in the data we collected. Future studies should explore additional miRNAs related to EC and CRDs.
LncRNAs also play a important role in EC, as lncRNA binding to miRNAs promotes or inhibits the proliferation of EC cells. For example, the lncRNA OIP5-AS1 inhibits the proliferation and invasion of EC cells by suppressing miR-200C-3p, which in turn regulates PTEN/AKT (85). Thus, lncRNAs, like miRNAs, play an indispensable role in the development of EC. Again, from the information collected, we did not observe many lncRNAs associated with EC. Further exploration of the interaction of lncRNAs and miRNAs with EC will help discover new therapeutic options for treating EC. To summarize the information we collected, we have compiled Figure 2.
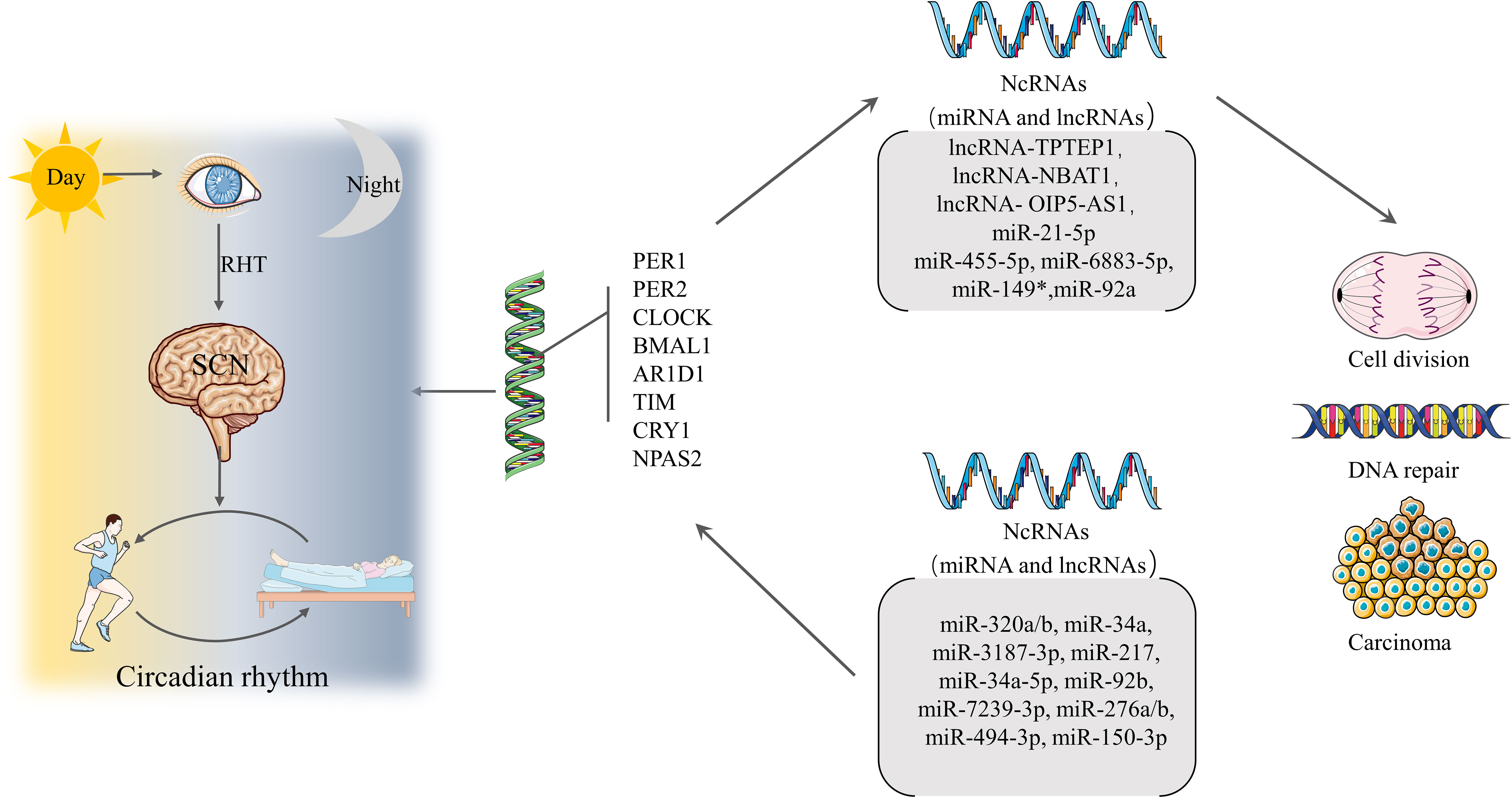
Figure 2 Formation of circadian rhythms and associated regulation Light travels through the retina and through the RHT to the SCN, forming the circadian rhythm that guides the mode of operation of daytime activity and nighttime rest. Circadian rhythms are regulated by CGs. A properly functioning CGs can direct ncRNAs to regulate cell division and DNA repair, while dysregulation can lead to cancer. Conversely, ncRNAs can affect the expression of CGs leading to CRDs and a range of diseases.
6 Discussion
The biological clock is a 24-h self-service oscillator controlled by CGs. Every cell in the human body has a day-night oscillator controlled by a master clock. This oscillator provides rhythmicity to specific cells and organs through rate-limiting metabolic program stages (68). The breakdown of the circadian rhythm disrupts the rhythmic nature of every cell and organ, resulting in a wide range of diseases. The circadian rhythm is regulated by CGs. The disorder of circadian rhythm is usually accompanied by abnormal expression of CGs, which also involves ncRNAs. This review summarizes recent perspectives on EC and CRDs, and collects relevant CGs and ncRNAs, including PER1, PER2, NAPS2, and ARID1A. However, based on our review, the only ncRNAs associated with CGs and EC are miR-17-5p, miR-93-5p, and lncRNA SNHG14. MiR-17-5p expression is negatively correlated with NAPS2 expression (47). Gao et al. (86) showed that miR-17-5p inhibited CLOCK translation, downregulated NAPS2 levels, and increased CRY1 expression. Furthermore, miR-17-5p directly targeted p21 to affect the migration and invasion of EC cells (87). Zhang et al. (88) showed that the lncRNA SNHG14 inhibited EC migration and invasion via the miR-93-5p/ZBTB7A axis. In other words, SNHG14 expression negatively correlated with miR-93-5p, and high SNHG14 expression inhibited EC development (Figure 3).
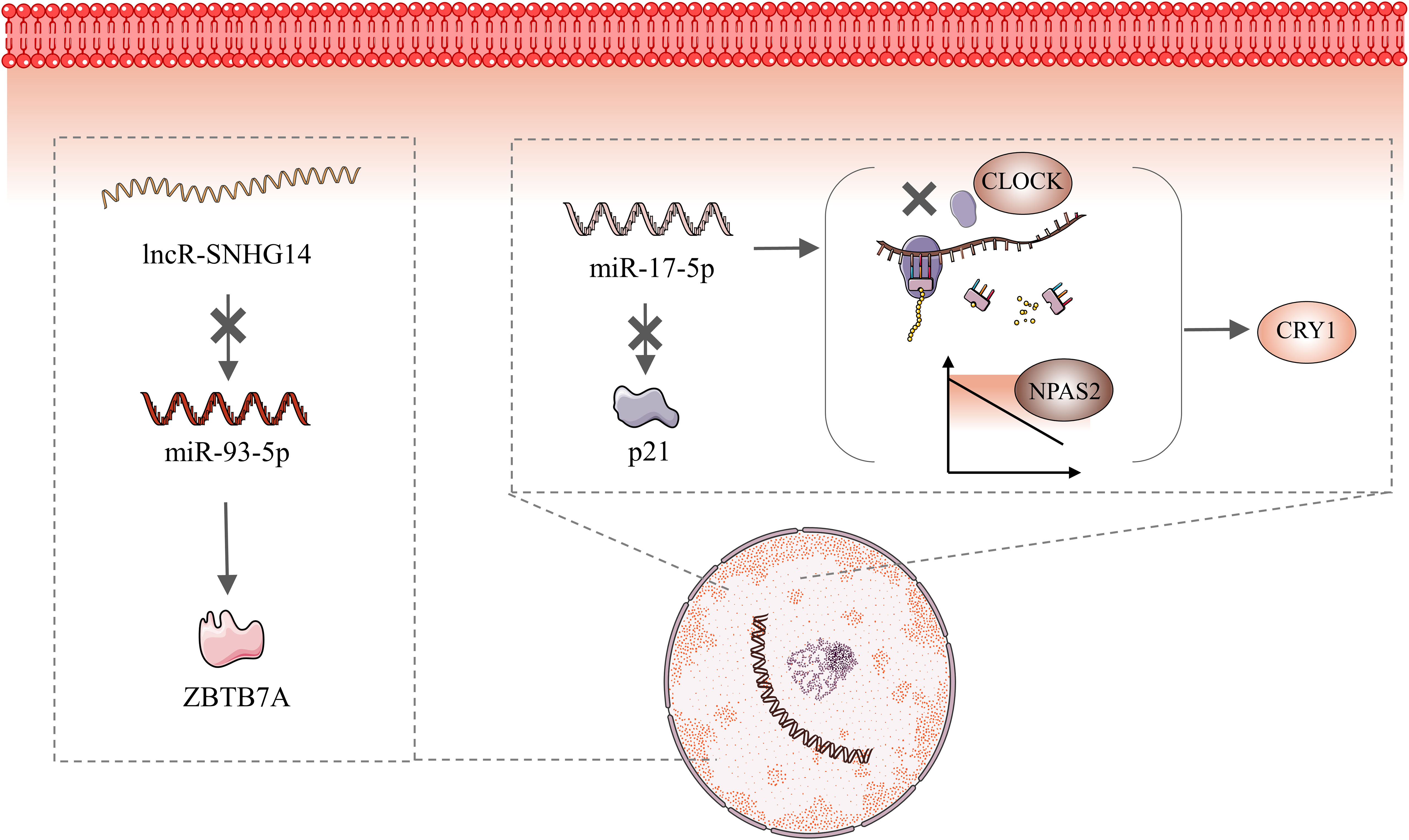
Figure 3 Molecular Mechanisms of MiR-17-5P and miR-93-5 PMiR-17-5P maintains circadian stability via inhibiting CLOCK translation, downregulating NAPS2 levels, and increasing CRY1 expression. In addition, miR-17-5p directly targets p21 to affect the migration and invasion of endometrial cancer cells. Elevated SNHG14 expression inhibits miR-93-5P, which then directly targets ZBTB7A.
From existing references, it can be seen that ncRNAs are upstream molecules of CGs, which means that CGs are regulated by ncRNAs. In addition, lncRNAs regulate miRNAs. Notably, this result is usually achieved by lncRNAs acting as molecular sponges. When CRDs occur, the human body activates ncRNAs in a uncertain way, thereby regulating the expression level of CGs. Abnormal levels of CGs expression ultimately lead to the occurrence of EC. From a macro perspective, long-term CRDs can cause EC. It is noteworthy that NAPS2 may be regulated by mi-93-5p and is negatively correlated, while lncRNA SNHG14 is also negatively correlated with mi-93-5p. This implies that NAPS2 is positively correlated with lncRNA SNHG14. Then, according to Zhang et al. (88), lncRNA SNHG14 is lowly expressed in EC patients, which means that NPAS2 should also be at low expression levels. However, the study by Zheng et al. (47). demonstrated that elevated levels of NAPS2 in EC patients. This conclusion is contradictory to their relationship. So far, we cannot explain this result, and we speculate that it may be the result of the action of multiple molecular pathways.
Interestingly, data reported by Costas et al. (89) did not support the carcinogenic role of CRDs in EC. This result is contrary to the findings of our previously mentioned study by Viswanathan et al. (69): the Nurses’ Health Study I found a significant elevated risk (RR=1.47) of endometrial cancer among long-term rotating night workers (>20 years). We observed that the study by Costas et al. (89). included only 180 cases while the study by Viswanathan et al. (69). included 53,487 women. The number of samples may have had an impact on the results of the study, and we believe that the results of a study with a large sample may be more convincing. What’s more, night work was defined as a working schedule that involved partly or entirely working between 00:00 and 06:00, while the latter defined night work as working at least three nights per month, in addition to daytime or evening shifts in that month. It can be seen that the target populations of these two studies are fundamentally different. It is possible that this is one of the reasons for the inconsistency of these two results. Although it is unclear why similar studies have reached inconsistent conclusions, the mechanism underlying the occurrence and development of EC needs to be further explored to resolve the conflicting evidence.
7 Conclusion and prospects
Circadian rhythms enable organisms to move regularly and maintain the balance between action and recovery, as disrupting this balance may lead to disease progression. We attempted to synthesize existing information on the role of CGs, miRNAs, and lncRNAs in developing EC to enhance our understanding of their participation in disease biology. This review summarizes the carcinogenic pathways associated with circadian gene ncRNAs. Deepening our understanding of these pathways is crucial for future EC research and can be extended to studies of other tumors. In addition, the carcinogenic pathway of CGs and ncRNAs provides a new direction for exploring new therapeutic targets. We believe that this area should be explored in more detail in the future.
Author contributions
LZ: Data curation, Investigation, Methodology, Supervision, Writing – original draft, Writing – review & editing. SC: Supervision, Writing – review & editing. LZ: Writing – review & editing. QH: Supervision, Writing – review & editing. JC: Supervision, Writing – review & editing. WC: Supervision, Writing – review & editing. SL: Funding acquisition, Investigation, Methodology, Supervision, Writing – review & editing. QS: Funding acquisition, Methodology, Supervision, Writing – review & editing.
Funding
The author(s) declare financial support was received for the research, authorship, and/or publication of this article. This work was supported by the Science and Technology Bureau of Quanzhou (grant number 2020CT003), the Science and Technology Bureau of Quanzhou (grant number 2020NO31s) and the Science and technology project of Fujian Provincial Health Commission (grant number 2020CXB027).
Acknowledgments
We are thankful to The Second Affiliated Hospital of Fujian Medical University for providing infrastructure facilities. We would like to thank Editage (www.editage.cn) for English language editing.
Conflict of interest
The authors declare that the research was conducted in the absence of any commercial or financial relationships that could be construed as a potential conflict of interest.
Publisher’s note
All claims expressed in this article are solely those of the authors and do not necessarily represent those of their affiliated organizations, or those of the publisher, the editors and the reviewers. Any product that may be evaluated in this article, or claim that may be made by its manufacturer, is not guaranteed or endorsed by the publisher.
References
1. Sung H, Ferlay J, Siegel RL, Laversanne M, Soerjomataram I, Jemal A. Global cancer statistics 2020: GLOBOCAN estimates of incidence and mortality worldwide for 36 cancers in 185 countries. CA: Cancer J Clin (2021) 71:209–49. doi: 10.3322/caac.21660
2. Crosbie EJ, Kitson SJ, McAlpine JN, Mukhopadhyay A, Powell ME, Singh N, et al. Endometrial cancer. Lancet (London England) (2022) 399:1412–28. doi: 10.1016/s0140-6736(22)00323-3
3. Makker V, MacKay H, Ray-Coquard I, Levine DA, Westin SN, Aoki D, et al. Endometrial cancer. Nat Rev Dis Primers (2021) 7:88. doi: 10.1038/s41572-021-00324-8
4. Suspected cancer: recognition and referral. National Institute for Health and Care Excellence (NICE) Copyright © NICE 2021 (2021) Available at: https://www.nice.org.uk/guidance/ng12/resources/suspected-cancer-recognition-and-referral-pdf-1837268071621 (Accessed March 12, 2022).
5. Siegel RL, Miller KD, Jemal A. Cancer statistics, 2018. CA: Cancer J Clin (2018) 68:7–30. doi: 10.3322/caac.21442
6. Qu H, Xue Y, Lian W, Wang C, He J, Fu Q, et al. Melatonin inhibits osteosarcoma stem cells by suppressing SOX9-mediated signaling. Life Sci (2018) 207:253–64. doi: 10.1016/j.lfs.2018.04.030
7. Akbarzadeh M, Akbar Movassaghpour A, Ghanbari H, Kheirandish M, Fathi Maroufi N, Rahbarghazi R, et al. The potential therapeutic effect of melatonin on human ovarian cancer by inhibition of invasion and migration of cancer stem cells. Sci Rep (2017) 7(1):17062. doi: 10.1038/s41598-017-16940-y
8. Chen X, Wang Z, Ma H, Zhang S, Yang H, Wang H, et al. Melatonin attenuates hypoxia-induced epithelial-mesenchymal transition and cell aggressive via Smad7/CCL20 in glioma. Oncotarget (2017) 8(55):93580–92. doi: 10.18632/oncotarget.20525
9. Mao L, Summers W, Xiang S, Yuan L, Dauchy RT, Reynolds A, et al. Melatonin represses metastasis in her2-postive human breast cancer cells by suppressing RSK2 expression. Mol Cancer Res: MCR (2016) 14:1159–69. doi: 10.1158/1541-7786.Mcr-16-0158
10. Wu SM, Lin W-Y, Shen C-C, Pan H-C, Keh-Bin W, Chen Y-C, et al. Melatonin set out to ER stress signaling thwarts epithelial mesenchymal transition and peritoneal dissemination via calpain-mediated C/EBPβ and NFκB cleavage. J Pineal Res (2016) 60:142–54. doi: 10.1111/jpi.12295
11. Greene MW. Circadian rhythms and tumor growth. Cancer Lett (2012) 318:115–23. doi: 10.1016/j.canlet.2012.01.001
12. Baker FC, Driver HS. Circadian rhythms, sleep, and the menstrual cycle. Sleep Med (2007) 8:613–22. doi: 10.1016/j.sleep.2006.09.011
13. Ray I, Goswami S. Circadian rhythm genes in cancer: insight into their functions and regulation involving noncoding RNAs. Chronobiol Int (2021) 38:1231–43. doi: 10.1080/07420528.2021.1928157
14. Chen C, Zhang Q, Kong B. miRNA-576-5p promotes endometrial cancer cell growth and metastasis by targeting ZBTB4. Clin Trans Oncol (2022) 25(3):706–20. doi: 10.1007/s12094-022-02976-8
15. Wang W, Jin W, Liu X, Zheng L. Circ_0002577/miR-126-5p/MACC1 axis promotes endometrial carcinoma progression by regulation of proliferation, migration, invasion, and apoptosis of endometrial carcinoma cells. Arch Gynecol Obstetrics (2022) 306:481–91. doi: 10.1007/s00404-022-06412-8
16. Kurman RJ, Kaminski PF, Norris HJ. The behavior of endometrial hyperplasia. A long-term study of “untreated” hyperplasia in 170 patients. Cancer (1985) 56:403–12. doi: 10.1002/1097-0142(19850715)56:2<403::AID-CNCR2820560233>3.0.CO;2-X
17. Ring KL, Mills AM, Modesitt SC. Endometrial hyperplasia. Obstetrics Gynecol (2022) 140:1061–75. doi: 10.1097/aog.0000000000004989
18. Aune D, Navarro Rosenblatt DA, Chan DSM, Vingeliene S, Abar L, Vieira AR, et al. Anthropometric factors and endometrial cancer risk: a systematic review and dose-response meta-analysis of prospective studies. Ann Oncol (2015) 26:1635–48. doi: 10.1093/annonc/mdv142
19. Renehan AG, Soerjomataram I, Tyson M, Egger M, Zwahlen M, Coebergh JW, et al. Incident cancer burden attributable to excess body mass index in 30 European countries. Int J Cancer (2010) 126:692–702. doi: 10.1002/ijc.24803
20. Fisher B, Costantino JP, Wickerham DL, Cecchini RS, Cronin WM, Robidoux A, et al. Tamoxifen for the prevention of breast cancer: current status of the National Surgical Adjuvant Breast and Bowel Project P-1 study. J Natl Cancer Institute (2005) 97:1652–62. doi: 10.1093/jnci/dji372
21. Modugno F, Ness RB, Chen C, Weiss NS. Inflammation and endometrial cancer: a hypothesis. Cancer Epidemiol Biomarkers Prev (2005) 14:2840–7. doi: 10.1158/1055-9965.Epi-05-0493
22. Mourits MJ, Bijen CB, Arts HJ, ter Brugge HG, van der Sijde R, Paulsen L, et al. Safety of laparoscopy versus laparotomy in early-stage endometrial cancer: a randomised trial. Lancet Oncol (2010) 11:763–71. doi: 10.1016/s1470-2045(10)70143-1
23. Walker JL, Piedmonte MR, Spirtos NM, Eisenkop SM, Schlaerth JB, Mannel RS, et al. Recurrence and survival after random assignment to laparoscopy versus laparotomy for comprehensive surgical staging of uterine cancer: Gynecologic Oncology Group LAP2 Study. J Clin Oncol: Off J Am Soc Clin Oncol (2012) 30:695–700. doi: 10.1200/jco.2011.38.8645
24. Janda M, Gebski V, Davies LC, Forder P, Brand A, Hogg R, et al. Effect of total laparoscopic hysterectomy vs total abdominal hysterectomy on disease-free survival among women with stage I endometrial cancer: A randomized clinical trial. Jama (2017) 317:1224–33. doi: 10.1001/jama.2017.2068
25. Galaal K, Donkers H, Bryant A, Lopes AD. Laparoscopy versus laparotomy for the management of early stage endometrial cancer. Cochrane Database Systematic Rev (2018) 10:Cd006655. doi: 10.1002/14651858.CD006655.pub3
26. Zisapel N. New perspectives on the role of melatonin in human sleep, circadian rhythms and their regulation. Br J Pharmacol (2018) 175:3190–9. doi: 10.1111/bph.14116
27. Vasey C, McBride J, Penta K. Circadian rhythm dysregulation and restoration: the role of melatonin. Nutrients (2021) 13(10):3480. doi: 10.3390/nu13103480
28. Bjarnason GA, Jordan R. Circadian variation of cell proliferation and cell cycle protein expression in man: clinical implications. Prog Cell Cycle Res (2000) 4:193–206. doi: 10.1007/978-1-4615-4253-7_17
29. Matsuo T, Yamaguchi S, Mitsui S, Emi A, Shimoda F, Okamura H, et al. Control mechanism of the circadian clock for timing of cell division. Vivo Sci (New York NY) (2003) 302:255–9. doi: 10.1126/science.1086271
30. Panda S, Antoch MP, Miller BH, Su AI, Schook AB, Straume M, et al. Coordinated transcription of key pathways in the mouse by the circadian clock. Cell (2002) 109:307–20. doi: 10.1016/s0092-8674(02)00722-5
31. Hansen J. Increased breast cancer risk among women who work predominantly at night. Epidemiol (Cambridge Mass) (2001) 12:74–7. doi: 10.1097/00001648-200101000-00013
32. Von Behren J, Hurley S, Goldberg D, Clague DeHart J, Wang SS, Reynolds P, et al. Chronotype and risk of post-menopausal endometrial cancer in the California Teachers Study. Chronobiol Int (2021) 38:1151–61. doi: 10.1080/07420528.2021.1912073
33. Canaple L, Kakizawa T, Laudet V. The days and nights of cancer cells. Cancer Res (2003) 63:7545–52.
34. Schernhammer ES, Laden F, Speizer FE, Willett WC, Hunter DJ, Kawachi I, et al. Rotating night shifts and risk of breast cancer in women participating in the nurses’ health study. J Natl Cancer Institute (2001) 93:1563–8. doi: 10.1093/jnci/93.20.1563
35. Lie JA, Roessink J, Kjaerheim K. Breast cancer and night work among Norwegian nurses. Cancer Causes Control: CCC (2006) 17:39–44. doi: 10.1007/s10552-005-3639-2
36. Papantoniou K, Castaño-Vinyals G, Espinosa A, Aragonés N, Pérez-Gómez B, Burgos J, et al. Night shift work, chronotype and prostate cancer risk in the MCC-Spain case-control study. Int J Cancer (2015) 137:1147–57. doi: 10.1002/ijc.29400
37. Rumble ME, Rose SL, Hanley White K, Moore AH, Gehrman P, Benca RM, et al. Circadian actigraphic rest-activity rhythms following surgery for endometrial cancer: A prospective, longitudinal study. Gynecol Oncol (2015) 137:448–55. doi: 10.1016/j.ygyno.2015.04.001
38. Cox KH, Takahashi JS. Circadian clock genes and the transcriptional architecture of the clock mechanism. J Mol Endocrinol (2019) 63:R93–r102. doi: 10.1530/jme-19-0153
39. Konopka RJ, Benzer S. Clock mutants of Drosophila melanogaster. Proc Natl Acad Sci United States America (1971) 68:2112–6. doi: 10.1073/pnas.68.9.2112
40. Shearman LP, Zylka MJ, Weaver DR, Kolakowski LF Jr., Reppert SM. Two period homologs: circadian expression and photic regulation in the suprachiasmatic nuclei. Neuron (1997) 19:1261–9. doi: 10.1016/s0896-6273(00)80417-1
41. Liu F, Chang HC. Physiological links of circadian clock and biological clock of aging. Protein Cell (2017) 8:477–88. doi: 10.1007/s13238-016-0366-2
42. Angelousi A, Kassi E, Ansari-Nasiri N, Randeva H, Kaltsas G, Chrousos G, et al. Clock genes and cancer development in particular in endocrine tissues. Endocrine-related Cancer (2019) 26:R305–r317. doi: 10.1530/erc-19-0094
43. Reick M, Garcia JA, Dudley C, McKnight SL. NPAS2: an analog of clock operative in the mammalian forebrain. Sci (New York NY) (2001) 293:506–9. doi: 10.1126/science.1060699
44. Preitner N, Damiola F, Lopez-Molina L, Zakany J, Duboule D, Albrecht U, et al. The orphan nuclear receptor REV-ERBalpha controls circadian transcription within the positive limb of the mammalian circadian oscillator. Cell (2002) 110:251–60. doi: 10.1016/s0092-8674(02)00825-5
45. Bugge A, Feng D, Everett LJ, Briggs ER, Mullican SE, Wang F, et al. Rev-erbα and Rev-erbβ coordinately protect the circadian clock and normal metabolic function. Genes Dev (2012) 26:657–67. doi: 10.1101/gad.186858.112
46. Wang Z, Wang H, Wang Z, He S, Jiang Z, Yan C, et al. Associated analysis of PER1/TUBB2B with endometrial cancer development caused by circadian rhythm disorders. Med Oncol (Northwood London England) (2020) 37:90. doi: 10.1007/s12032-020-01415-4
47. Zheng X, Lv X, Zhu L, Xu K, Shi C, Cui L, et al. The circadian gene NPAS2 act as a putative tumor stimulative factor for uterine corpus endometrial carcinoma. Cancer Manage Res (2021) 13:9329–43. doi: 10.2147/cmar.S343097
48. Hu H, Chen Z, Ji L, Wang Y, Yang M, Lai R, et al. ARID1A-dependent permissive chromatin accessibility licenses estrogen-receptor signaling to regulate circadian rhythms genes in endometrial cancer. Cancer Lett (2020) 492:162–73. doi: 10.1016/j.canlet.2020.08.034
49. Muter J, Lucas ES, Chan Y-W, Brighton PJ, Moore JD, Lacey L, et al. The clock protein period 2 synchronizes mitotic expansion and decidual transformation of human endometrial stromal cells. FASEB J: Off Publ Fed Am Societies Exp Biol (2015) 29:1603–14. doi: 10.1096/fj.14-267195
50. Coussy F, Lallemand F, Vacher S, Schnitzler A, Chemlali W, Caly M, et al. Clinical value of R-spondins in triple-negative and metaplastic breast cancers. Br J Cancer (2017) 116:1595–603. doi: 10.1038/bjc.2017.131
51. Liu H, Zhou Y, Tan F, Wang Y, Pei H. Expression of regulatory factor R-spondin family in Wnt signaling pathway in colorectal cancer and its clinical significance. J Cent South University Med Sci (2017) 42:501–6. doi: 10.11817/j.issn.1672-7347.2017.05.003
52. Nami B, Wang Z. Genetics and expression profile of the tubulin gene superfamily in breast cancer subtypes and its relation to taxane resistance. Cancers (2018) 10(8):274. doi: 10.3390/cancers10080274
53. Toumpeki C, Liberis A, Tsirkas I, Tsirka T, Kalagasidou S, Inagamova L, et al. The role of ARID1A in endometrial cancer and the molecular pathways associated with pathogenesis and cancer progression. In Vivo (Athens Greece) (2019) 33:659–67. doi: 10.21873/invivo.11524
54. Pulice JL, Kadoch C. Composition and function of mammalian SWI/SNF chromatin remodeling complexes in human disease. Cold Spring Harbor Symp Quantitative Biol (2016) 81:53–60. doi: 10.1101/sqb.2016.81.031021
55. Cerami E, Gao J, Dogrusoz U, Gross BE, Onur Sumer S, Arman Aksoy B, et al. The cBio cancer genomics portal: an open platform for exploring multidimensional cancer genomics data. Cancer Discovery (2012) 2:401–4. doi: 10.1158/2159-8290.Cd-12-0095
56. Mathur R, Alver BH, Roman San AK, Wilson BG, Wang X, Agoston AT, et al. ARID1A loss impairs enhancer-mediated gene regulation and drives colon cancer in mice. Nat Genet (2017) 49:296–302. doi: 10.1038/ng.3744
57. Abe H, Maeda D, Hino R, Otake Y, Isogai M, Shinozaki Ushiku A, et al. ARID1A expression loss in gastric cancer: pathway-dependent roles with and without Epstein-Barr virus infection and microsatellite instability. Virchows Archiv: Int J Pathol (2012) 461:367–77. doi: 10.1007/s00428-012-1303-2
58. Wang K, Yuen ST, Xu J, Lee SP, Yan HHN, Shi ST, et al. Whole-genome sequencing and comprehensive molecular profiling identify new driver mutations in gastric cancer. Nat Genet (2014) 46:573–82. doi: 10.1038/ng.2983
59. Waddell N, Pajic M, Patch A-M, Chang DK, Kassahn KS, Bailey P, et al. Whole genomes redefine the mutational landscape of pancreatic cancer. Nature (2015) 518:495–501. doi: 10.1038/nature14169
60. Streppel MM, Lata S, DelaBastide M, Montgomery EA, Wang JS, Canto MI, et al. Next-generation sequencing of endoscopic biopsies identifies ARID1A as a tumor-suppressor gene in Barrett’s esophagus. Oncogene (2014) 33:347–57. doi: 10.1038/onc.2012.586
61. Fujimoto A, Totoki Y, Abe T, Boroevich KA, Hosoda F, Nguyen HH, et al. Whole-genome sequencing of liver cancers identifies etiological influences on mutation patterns and recurrent mutations in chromatin regulators. Nat Genet (2012) 44:760–4. doi: 10.1038/ng.2291
62. Chandler RL, Damrauer JS, Raab JR, Schisler JC, Wilkerson MD, Didion JP, et al. Coexistent ARID1A-PIK3CA mutations promote ovarian clear-cell tumorigenesis through pro-tumorigenic inflammatory cytokine signalling. Nat Commun (2015) 6:6118. doi: 10.1038/ncomms7118
63. Guan B, Wang TL, Shih Ie M. ARID1A, a factor that promotes formation of SWI/SNF-mediated chromatin remodeling, is a tumor suppressor in gynecologic cancers. Cancer Res (2011) 71:6718–27. doi: 10.1158/0008-5472.Can-11-1562
64. Mao TL, Ardighieri L, Ayhan A, Kuo K-T, Wu C-H, Wang T-L, et al. Loss of ARID1A expression correlates with stages of tumor progression in uterine endometrioid carcinoma. Am J Surg Pathol (2013) 37:1342–8. doi: 10.1097/PAS.0b013e3182889dc3
65. Levine DA, The Cancer Genome Atlas Research Network. Integrated genomic characterization of endometrial carcinoma. Nature (2013) 497:67–73. doi: 10.1038/nature12113
66. Dioum EM, Rutter J, Tuckerman JR, Gonzalez G, Gilles-Gonzalez M-A, McKnight SL, et al. NPAS2: a gas-responsive transcription factor. Sci (New York NY) (2002) 298:2385–7. doi: 10.1126/science.1078456
67. Hoffman AE, Zheng T, Ba Y, Zhu Y. The circadian gene NPAS2, a putative tumor suppressor, is involved in DNA damage response. Mol Cancer Res: MCR (2008) 6:1461–8. doi: 10.1158/1541-7786.Mcr-07-2094
68. Reinke H, Asher G. Crosstalk between metabolism and circadian clocks. Nat Rev Mol Cell Biol (2019) 20:227–41. doi: 10.1038/s41580-018-0096-9
69. Viswanathan AN, Hankinson SE, Schernhammer ES. Night shift work and the risk of endometrial cancer. Cancer Res (2007) 67:10618–22. doi: 10.1158/0008-5472.Can-07-2485
70. Razavi P, Devore EE, Bajaj A, Lockley SW, Figueiro MG, Ricchiuti V, et al. Shift work, chronotype, and melatonin rhythm in nurses. Cancer Epidemiol Biomarkers Prevention: Publ Am Assoc Cancer Res Cosponsored Am Soc Prev Oncol (2019) 28:1177–86. doi: 10.1158/1055-9965.Epi-18-1018
71. Quinn JJ, Chang HY. Unique features of long non-coding RNA biogenesis and function. Nat Rev Genet (2016) 17:47–62. doi: 10.1038/nrg.2015.10
72. Guttman M, Rinn JL. Modular regulatory principles of large non-coding RNAs. Nature (2012) 482:339–46. doi: 10.1038/nature10887
73. Cesarini V, Silvestris DA, Tassinari V, Tomaselli S, Alon S, Eisenberg E, et al. ADAR2/miR-589-3p axis controls glioblastoma cell migration/invasion. Nucleic Acids Res (2018) 46:2045–59. doi: 10.1093/nar/gkx1257
74. Luo H, Zhu G, Xu J, Lai Q, Yan B, Guo Y, et al. HOTTIP lncRNA promotes hematopoietic stem cell self-renewal leading to AML-like disease in mice. Cancer Cell (2019) 36:645–659.e648. doi: 10.1016/j.ccell.2019.10.011
75. Marchese FP, Raimondi I, Huarte M. The multidimensional mechanisms of long noncoding RNA function. Genome Biol (2017) 18:206. doi: 10.1186/s13059-017-1348-2
76. Gharib AF, Khalifa AS, Mohamed Eed E, Banjer HJ, Ali Shami A, Askary El A, et al. Role of microRNA-31 (miR-31) in breast carcinoma diagnosis and prognosis. In Vivo (Athens Greece) (2022) 36:1497–502. doi: 10.21873/invivo.12857
77. Zhao YL, Zhang J-X, Yang J-J, Wei Y-B, Peng J-F, Fu C-J, et al. MiR-205-5p promotes lung cancer progression and is valuable for the diagnosis of lung cancer. Thorac Cancer (2022) 13:832–43. doi: 10.1111/1759-7714.14331
78. Wei D, Tian M, Fan W, Zhong X, Wang S, Chen Y, et al. Circular RNA circ_0000043 promotes endometrial carcinoma progression by regulating miR-1271-5p/CTNND1 axis. Arch Gynecol Obstetrics (2021) 303:1075–87. doi: 10.1007/s00404-020-05849-z
79. Nian Y, Li X, Ma J, Gao T, Liu D. Circ_0075960 targets the miR-202-5p/CTNND1 axis to promote the growth and migration of endometrial carcinoma cells via regulating Wnt/β-catenin signaling activity. J Gynecol Oncol (2023) 34:e11. doi: 10.3802/jgo.2023.34.e11
80. Shen Q, He T, Yuan H. Hsa_circ_0002577 promotes endometrial carcinoma progression via regulating miR-197/CTNND1 axis and activating Wnt/β-catenin pathway. Cell Cycle (Georgetown Tex) (2019) 18:1229–40. doi: 10.1080/15384101.2019.1617004
81. Liu Y, Yuan H, He T. Downregulated circular RNA hsa_circ_0005797 inhibits endometrial cancer by modulating microRNA-298/Catenin delta 1 signaling. Bioengineered (2022) 13:4634–45. doi: 10.1080/21655979.2021.2013113
82. Li Y, Liu J, Piao J, Ou J, Zhu X. Circ_0109046 promotes the Malignancy of endometrial carcinoma cells through the microRNA-105/SOX9/Wnt/β-catenin axis. IUBMB Life (2021) 73:159–76. doi: 10.1002/iub.2415
83. Cheng Q, Fan X, Liu Y, Xu L, Dong P, Song L, et al. miR-455-5p regulates circadian rhythms by accelerating the degradation of Clock mRNA. IUBMB Life (2022) 74:245–58. doi: 10.1002/iub.2587
84. Kochan DZ, Kovalchuk O. Circadian disruption and breast cancer: an epigenetic link? Oncotarget (2015) 6:16866–82. doi: 10.18632/oncotarget.4343
85. Liu Y, Cai X, Cai Y, Chang Y. lncRNA OIP5-AS1 Suppresses Cell Proliferation and Invasion of Endometrial Cancer by Regulating PTEN/AKT via Sponging miR-200c-3p. J Immunol Res (2021) 2021:4861749. doi: 10.1155/2021/4861749
86. Gao Q, Zhou L, Yang SY, Cao JM. A novel role of microRNA 17-5p in the modulation of circadian rhythm. Sci Rep (2016) 6:30070. doi: 10.1038/srep30070
87. Shen Y, Lu L, Xu J, Meng W, Qing Y, Liu Y, et al. Bortezomib induces apoptosis of endometrial cancer cells through microRNA-17-5p by targeting p21. Cell Biol Int (2013) 37:1114–21. doi: 10.1002/cbin.10139
88. Zhang K, Cai Y, Zhou Q, Sun H, Wei J. Long non-coding RNA SNHG14 impedes viability, migration and invasion of endometrial carcinoma cells through modulating miR-93-5p/ZBTB7A axis. Cancer Manage Res (2020) 12:9515–25. doi: 10.2147/cmar.S257419
Keywords: endometrial cancer, circadian rhythm disorders, non-coding RNAs, clock genes, miRNA, lncRNA
Citation: Zheng L-t, Chen S-r, Zhou L-y, Huang Q-y, Chen J-m, Chen W-h, Lin S and Shi Q-y (2023) Latest advances in the study of non-coding RNA-mediated circadian rhythm disorders causing endometrial cancer. Front. Oncol. 13:1277543. doi: 10.3389/fonc.2023.1277543
Received: 14 August 2023; Accepted: 07 November 2023;
Published: 22 November 2023.
Edited by:
Elizabeth Cash, University of Louisville, United StatesReviewed by:
Mario Cioce, Campus Bio-Medico University, ItalyPaolo Martini, University of Brescia, Italy
Copyright © 2023 Zheng, Chen, Zhou, Huang, Chen, Chen, Lin and Shi. This is an open-access article distributed under the terms of the Creative Commons Attribution License (CC BY). The use, distribution or reproduction in other forums is permitted, provided the original author(s) and the copyright owner(s) are credited and that the original publication in this journal is cited, in accordance with accepted academic practice. No use, distribution or reproduction is permitted which does not comply with these terms.
*Correspondence: Qi-yang Shi, d3NxeTIxNEAxNjMuY29t; Shu Lin, c2h1bGluMTk1NkAxMjYuY29t
†These authors have contributed equally to this work