- 1Basic and Molecular Epidemiology of Gastrointestinal Disorders Research Centre, Research Institute for Gastroenterology and Liver Diseases, Shahid Beheshti University of Medical Sciences, Tehran, Iran
- 2Department of Laboratory Medicine, Faculty of Paramedical, Kurdistan University of Medical Sciences, Sanandaj, Iran
- 3School of Medicine, Tehran University of Medical Sciences, Tehran, Iran
- 4Department of Molecular Cell Biology, Microbiology Trend, Faculty of Basic Sciences, Islamic Azad University, Central Tehran Branch, Tehran, Iran
- 5Hematology-Oncology and Stem Cell Transplantation Research Center, Tehran University of Medical Sciences, Tehran, Iran
Introduction: Colorectal cancer (CRC) is a devastating disease that affects millions of people worldwide. Recent research has highlighted the crucial role of the guanylate cyclase-C (GC-C) signaling axis in CRC, from the early stages of tumorigenesis to disease progression. GC-C is activated by endogenous peptides guanylin (GU) and uroguanylin (UG), which are critical in maintaining intestinal fluid homeostasis. However, it has been found that these peptides may also contribute to the development of CRC. This systematic review focuses on the latest research on the GC-C signaling axis in CRC.
Methods: According to the aim of the study, a systematic literature search was conducted on Medline and PubMed databases. Ultimately, a total of 40 articles were gathered for the systematic review.
Results: Our systematic literature search revealed that alterations in GC-C signaling compartments in CRC tissue have demonstrated potential as diagnostic, prognostic, and therapeutic markers. This research highlights a potential treatment for CRC by targeting the GC-C signaling axis. Promising results from recent studies have explored the use of this signaling axis to develop new vaccines and chimeric antigen receptors that may be used in future clinical trials.
Conclusion: The findings presented in this review provide compelling evidence that targeting the GC-C signaling axis may be an advantageous approach for treating CRC.
Highlights
● 1. The progression of colorectal cancer (CRC) is attributed to GC-C, GN, and UG peptides.
● 2. In CRC, the GN and GU expression is lost due to abnormal APC-β-catenin-TCF transcriptional regulation in the early phase of tumor formation. This impairs the GC-C signaling axis and disrupts intestinal homeostasis, contributing to tumor initiation.
● 3. When the GC-C axis is blocked, there is excessive cell growth, increased crypt size, and reduced cell differentiation in the secretory lineage.
● 4. Targeting GC-C with CAR-T cells could be an innovative and practical approach to treating advanced stages of CRC or those resistant to traditional therapies.
● 5. The repurposing of GC-C signaling axis-targeted treatments developed for other diseases is a promising strategy for CRC treatment. Further research is needed to investigate their safety and efficacy.
1 Introduction
Globally, colorectal cancer (CRC) is the third most prevalent form of cancer and has the second-highest mortality rate among all cancers. The CRC mortality rate has been decreasing at a rate of around 2% each year during the last decade. Projections indicate that by the end of 2023, there will have been over 150,000 new cases and about 53,000 fatalities (1). Population-wide shifts toward better lifestyles (e.g., less consumption of red and processed meat) and increased participation in the cancer screening program have been attributed to a decline in colorectal cancer incidence (2, 3). However, this improvement has been made since the early 2000s, primarily because of increased colonoscopy screening methods and the removal of precursor lesions. The primary prevention of colorectal cancer continues to be the most efficient way of reducing the rising global burden of this disease (4–7).
Symptoms of CRC in its early stages are unspecific, which might lead to missed diagnoses or mistaken assessments. Therefore, most cases of CRC are detected in the late stages (8). Surgical resection, radiation therapy before surgery (for rectal cancer), chemotherapy after surgery (for stages III/IV and high-risk stage II colon cancer), and targeted therapy are the most common therapies for CRC (9, 10). Although development in anticancer therapy, particularly immune checkpoint inhibitors (ICIs), has revolutionized CRC treatment, only a fraction of patients respond to these treatments appropriately, and the reason for failure in other patients has not yet been known properly (7, 11). Thus, understanding the molecular mechanism in cancers is crucial for creating reliable diagnostic and prognostic biomarkers in both practice and research (12). Significant advancements in microarray and high-efficiency sequencing technologies like next-generation sequencing have accelerated the exploration and identification of the essential genetic or epigenetic modifications in carcinogenesis, tumor growth, disease recurrence, and metastasis in CRC as well as the discovery of cancer biomarkers with the potential for the development of novel diagnostic, prognostic, and therapeutic techniques (13, 14). Over the past few decades, key driver and passenger genes in CRC have been recognized (15). Auspiciously, apart from the main oncogenes leading to CRC, numerous other genes implicated in CRC development and progression are being discovered and may be exploited as new biomarkers in clinical settings to predict prognosis or therapy response in the future (16).
The transmembrane receptor GC-C is selectively expressed from duodenal to rectal intestinal epithelial cells (17). GC-C is activated by endogenous peptides GN and UG, as well as exogenous ligands such as heat-stable enterotoxins (STs), which are secreted by diarrhea-producing enterotoxigenic E. coli (ETEC) (18).
GN and UG are very close in structure and biological functions. The GC-C signal transduction controls the equilibrium of liquid and electrolyte transport and secretion in the digestive tract (19, 20). However, new findings indicate that these novel peptides have diverse physiological roles alongside those previously documented for the control of homeostasis and might contribute to the tumorigenesis of colorectal adenocarcinoma (21–25). The coincidence of the emergence and development of CRC with the presence of GC-C, GN, and UG proteins, along with their encoding genes GUCY2C, GUCA2A, and GUCA2B, respectively, has piqued the interest of researchers. Particularly, GUCY2C has been found to play a regulatory role in intestinal inflammation and inflammatory bowel disease pathology. Studies of intestinal inflammation in Gucy2c knockout mice have reported the impairment of the epithelial barrier, increased invasiveness of pathogenic bacteria, and alterations in the intestinal microbiota (26, 27). Overall, these data suggest that IBD susceptibility may be mediated by alterations in the intestinal microbiota caused by changes in intestinal ion transportation regulated by GC-C. As a result, we have undertaken a comprehensive review of the literature to explore the relationship between these genes and CRC. Our systematic review not only examines how these genes contribute to CRC development but also emphasizes their potential uses in diagnosing and treating the disease, illustrated as schematic abstract (Figure 1).
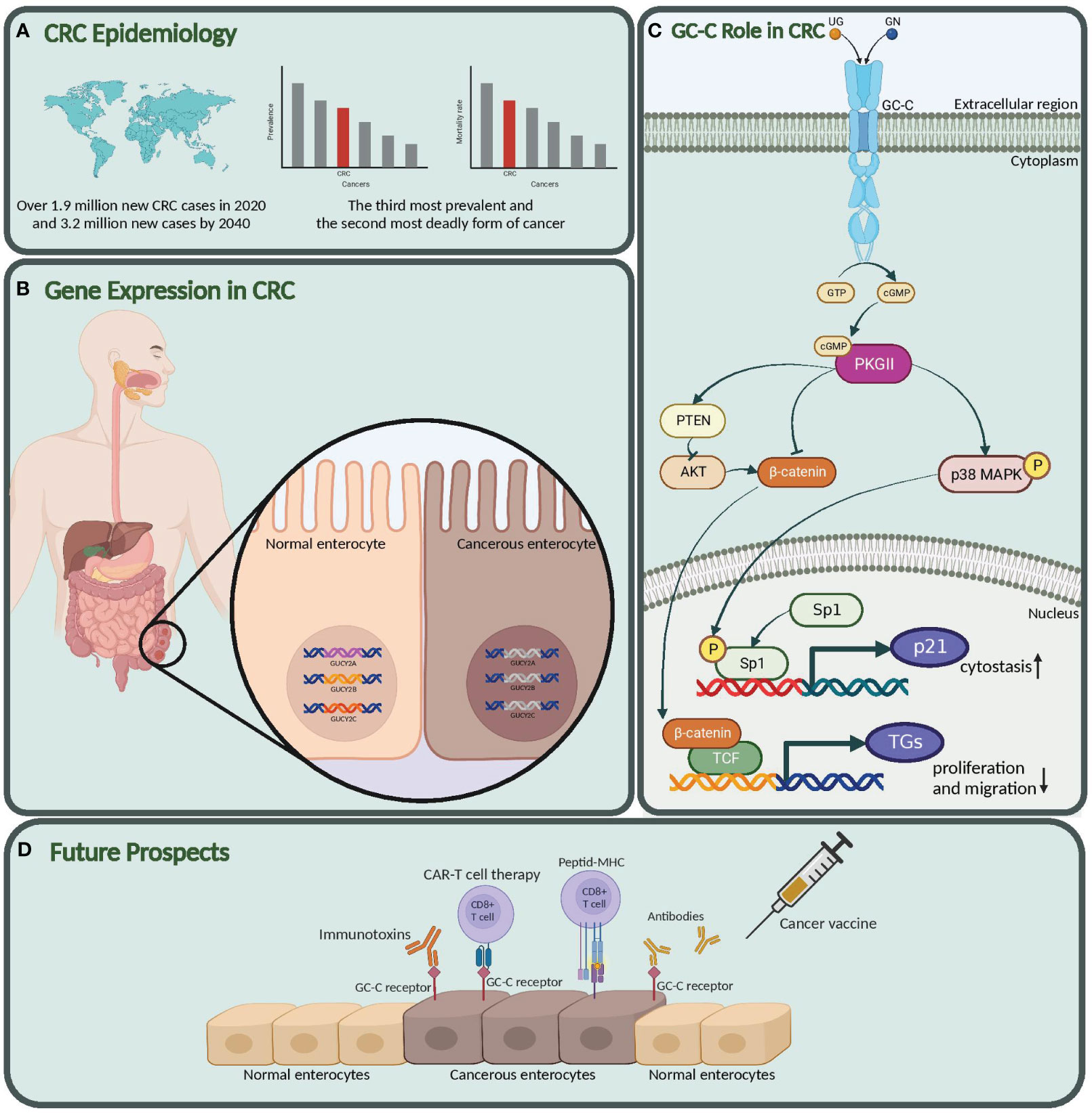
Figure 1 Graphical abstract. (A) CRC epidemiology. Global impact of colorectal cancer. (B) Gene expression in CRC. suppressed expression in GUCY2C, GUCA2A, and GUCA2B in Cancerous vs. Normal Enterocytes. (C) GC-C role in CRC. Here, we also explored the molecular mechanism and role of this pathway in the pathogenesis of colon cancer. (D) Future prospects. Our study highlights the potential of the Guanylate cyclase-c (GC-C) signaling pathway as a diagnostic biomarker, prognostic indicator, and target for new therapeutic strategies in colorectal cancer. GUCY2C, Guanylate Cyclase 2C; GUCA2A, Guanylyl cyclase-activating protein 2A; GUCA2B, Guanylate Cyclase Activator 2B; GC-C, Guanylate Cyclase-C; CRC, Colorectal Cancer. Created with BioRender.com.
2 Materials and methods
2.1 Search strategy and data extraction
This study presents a systematic review focusing on the role of the Guanylate cyclase-C Signaling Axis CRC. The search for relevant articles was conducted until May 27, 2023, extensively utilizing the Medline and PubMed databases. The search algorithm incorporated key terms related to the Guanylate cyclase-C Signaling Axis, including Guanylyl cyclase C, Guanylin, uroguanylin, their associated genes, and colorectal cancer. Meta-analyses, reviews, case reports, correspondences, and personal opinions studies were excluded (Table 1).
2.2 Inclusion and exclusion criteria, population, intervention, and outcomes
During the initial screening process, duplicate articles and those published before 2018 were excluded. Additionally, only articles written in English were included to mitigate potential language and publication biases. Two experts (MEP and AA) independently evaluated article content, demographics, research methodologies, and outcomes to identify potentially eligible studies. Inclusion criteria encompassed studies involving human, animal, in vivo, and in vitro colorectal cancer samples, focusing on applying the guanylate cyclase-c signaling axis for diagnostic, prognostic, therapeutic targeting, and survival assessment purposes. Studieswith inadequate research designs or misaligned outcomes were excluded from consideration. The search terms and methodologies applied (including database searches, screening, selection, and inclusion criteria) were consistently employed to ensure comprehensive coverage of relevant articles, adhering to the PRISMA statement of 2020 (28).
2.3 Risk of bias
Two assessors (MEP and AA) conducted independent assessments to evaluate potential bias. In cases of disagreement, a third assessor (PJ) was consulted. The evaluation of bias employed the Risk Of Bias In Non-randomized Studies - of Interventions (ROBINS-I) tool, which encompassed eight domains: bias arising from confounding; bias in participant selection; bias in classification; bias due to deviations in intended interventions; bias resulting from missing data; bias due to outcome measurement; bias in the selection of reported results; and overall bias. This rigorous approach ensured a comprehensive assessment of potential biases in the included studies (29).
3 Results
Figure 2 is a flowchart of the searching, screening, and process of the references we selected from the literature. According to the search strategy, 138 published papers were found in each database. After omitting 69 papers as duplicate articles, two separate team members screened 69 titles and abstracts. Twenty-nine articles were eliminated after the title and abstract screening (Review n=20) (Not CRC n=1) (Not Guanylate cyclase axis n=8), and the remaining 40 articles were evaluated for eligibility. All full-text original articles are obtained via open access or institutional subscription. All papers were in English, and none were excluded during the full-text screening stage. Ultimately, a total of 40 articles were gathered for the systematic review. There were 21 papers focused on GUCY2C (Table 2), eight papers on GUCA2A (Table 3), eight more papers on GUCA2B (Table 4), and finally, three papers that covered both GUCA2A and GUCA2B.
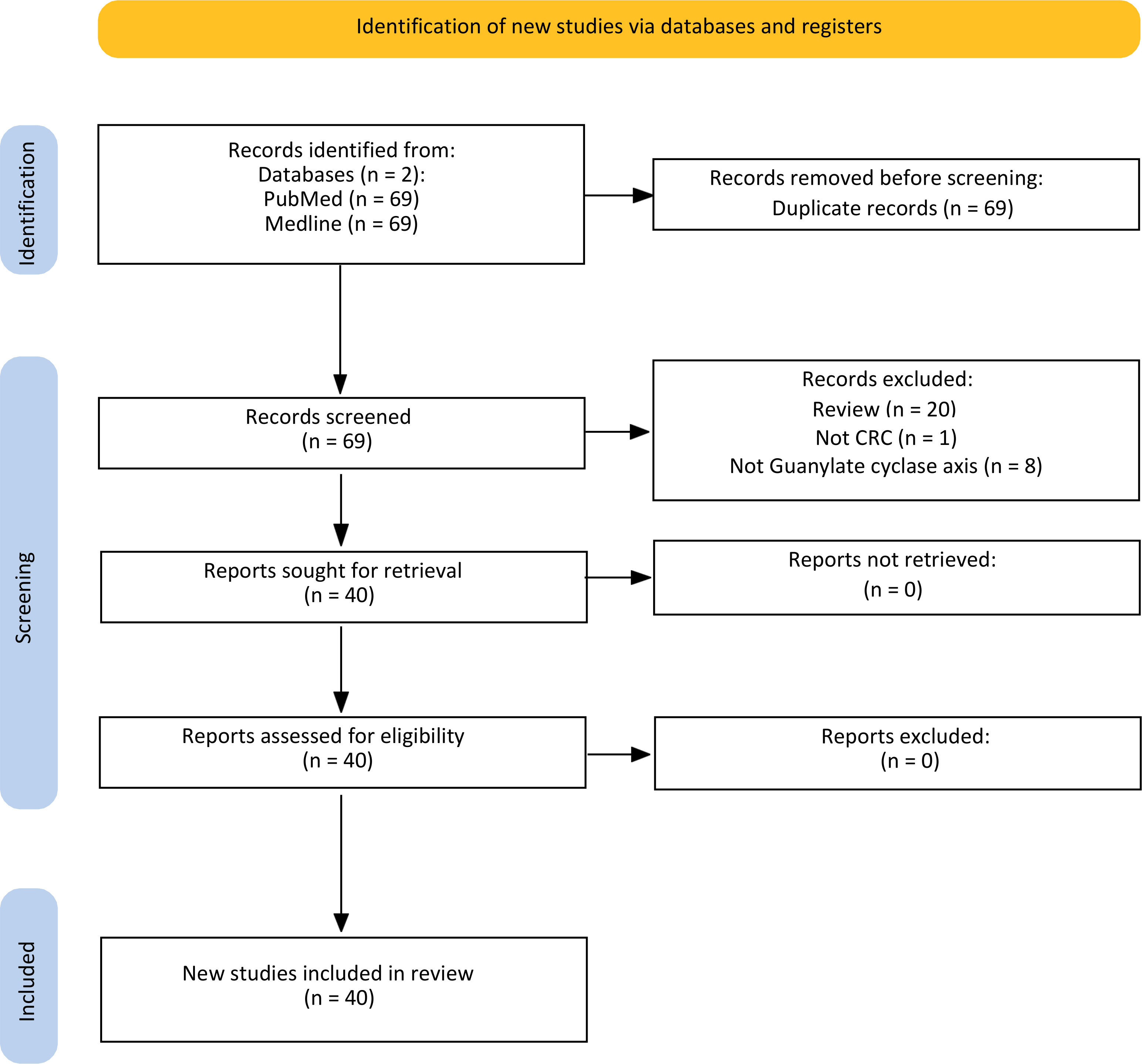
Figure 2 Flow chart of the literature search for Guanylate cyclase-C signaling pathway related to colorectal cancer.
3.1 GUCY2C
The GUCY2C gene encodes the GC-C receptor, a membrane protein composed of various domains, including extracellular binding, transmembrane, juxta-membrane, kinase homology, linker, guanylyl cyclase, and C-terminal domains (Figure 3) (69, 70). GC-C is triggered by the guanylin family of peptides, which consists of endogenous peptides, including GN, UG, and lymphoguanylin, and an exogenous peptide toxin produced by enteric bacteria (71, 72).
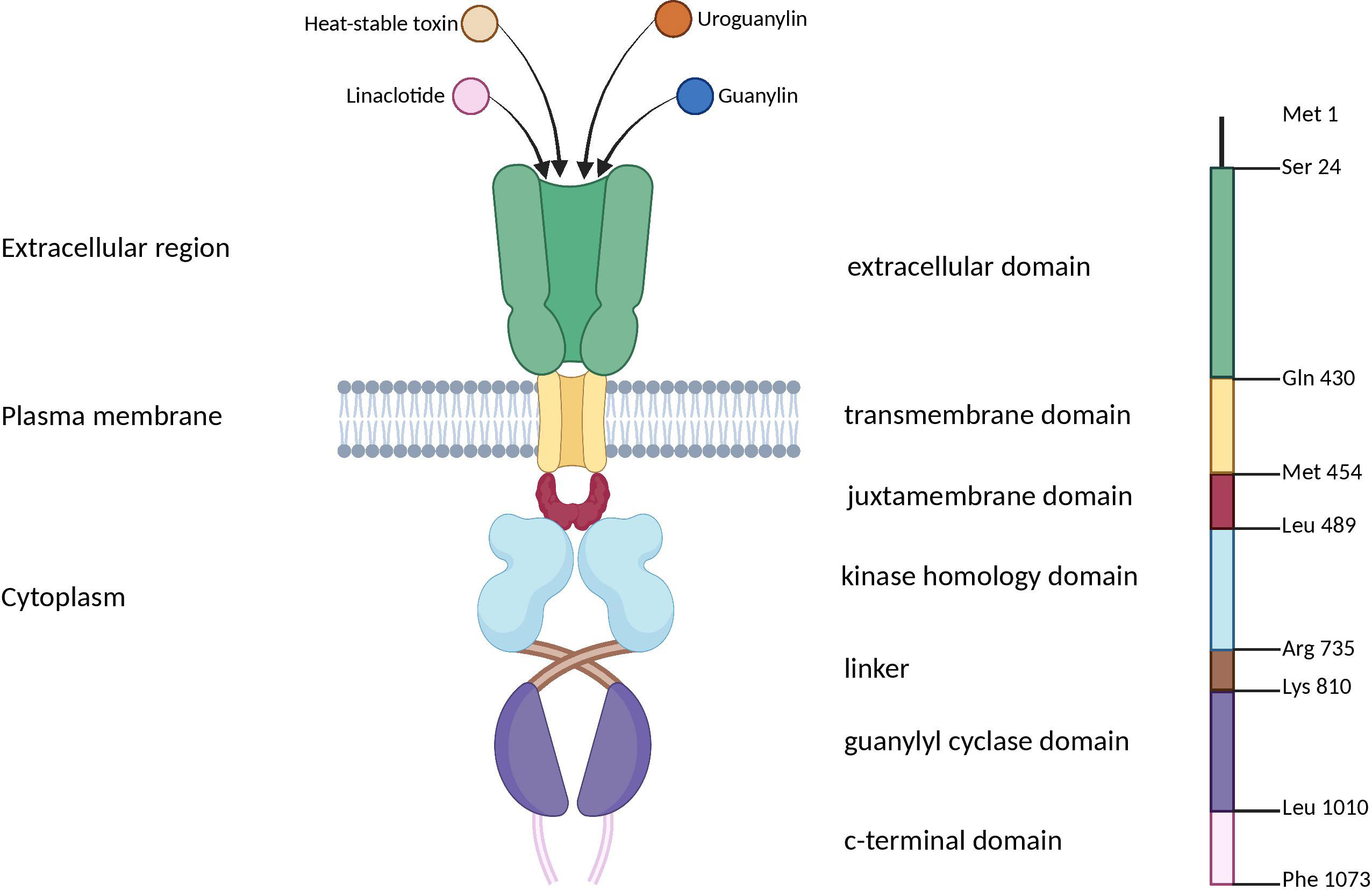
Figure 3 Schematic representation of domain organization of the guanylyl cyclase GC-C receptor. GC-C is predicted to be a transmembrane receptor homodimer with seven functional domains. These domains include the extracellular domain responsible for binding peptide ligands such as STs, guanylin, uroguanylin, and the FDA-approved ST analog, Linaclotide. Additionally, there is a transmembrane domain, a juxta membrane domain, a kinase-homology domain, and a linker region that may facilitate catalytic subunit dimerization and regulate its function. GC-C, Guanylate Cyclase-C; ST, heat-stable enterotoxins. Created with BioRender.com.
Growing evidence suggests a close association between CRC and dysbiosis of gut microbiota. Recent studies have shed light on the specific roles of intestinal microorganisms in initiating and facilitating the development of CRC. Notably, our review demonstrates that ETEC produces a substance called ST, which binds to the GC-C receptor with high affinity. ETEC is an important cause of diarrheal disease, particularly in low- and middle-income countries (73). Conversely, the incidence of CRC in developed countries is up to ten times higher than in underdeveloped countries (74). Intriguingly, an inverse epidemiological correlation exists between diarrheal diseases caused by ST-producing ETEC and colorectal cancer. This inverse correlation may be attributed to the involvement of GUCY2C as a tumor suppressor in CRC pathophysiology, particularly in developing countries. A recent study in mice has revealed that chronic colonization with ST-producing Escherichia coli counters the development of colorectal tumors. These findings suggest that bacterially produced ST reinstates GUYC2C signaling, which in turn opposes the tumor transformation (75). Additionally, more research is needed to investigate the potential of using ST as a possible prophylactic or therapeutic approach for preventing or treating CRC. These studies could pave the way for developing novel strategies for CRC prevention and treatment.
Moreover, the activation of the GC-C signaling axis is pH-dependent, meaning that different parts of the gastrointestinal (GI) tract have different regulatory effects (28, 29). The distribution of GC-C receptors throughout the GI tract is widespread, but their activation patterns depend on the location and type of activator. In the upper small intestine, GC-C receptors are more strongly activated at lower pH levels (~ pH 5.5) by UG. On the other hand, in the lower small intestine and colorectum, these receptors are activated at higher pH levels (~ pH 8.0) by GN (Figure 4) (76, 77).
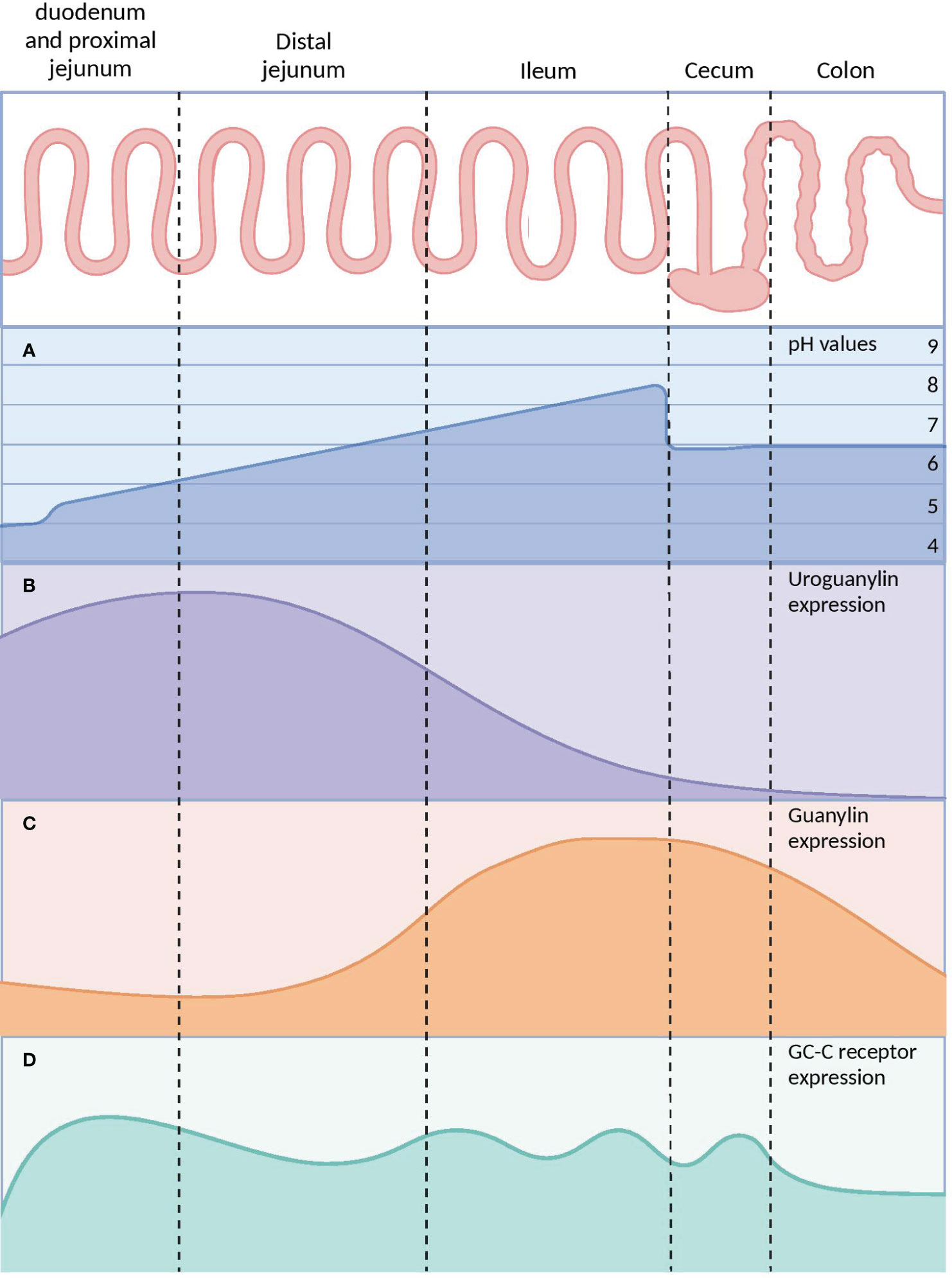
Figure 4 The differences in pH and GC-C signaling axis parts throughout the gastrointestinal tract. (A) The pH of the small intestine increases gradually while the pH of the caecum decreases due to microorganism populations. (B) UG expression is at its maximum in the distal jejunum and at its lowest in the colon. (C) GN concentrations rise along the distal small intestine, peak in the caecum, and then drop rapidly in the distal colon. (D) The expression of GC-C receptors is constant throughout the intestine. GC-C, Guanylate Cyclase-C; UG, Uroguanylin; GN, Guanylin. Created with BioRender.com.
Upon binding of agonist peptides to the extracellular domain of the GC-C receptor, the intracellular catalytic domain converts guanosine triphosphate into cyclic guanosine monophosphate (cGMP). This second messenger then activates cGMP-dependent protein kinases G (PKG), cyclic-nucleotide-gated (CNG) channels, and cGMP-regulated cyclic-nucleotide phosphodiesterase (78). In intestinal cells, protein kinase G II (PKGII) phosphorylates the cystic fibrosis transmembrane conductance regulator (CFTR), leading to increased efflux of chloride (Cl−) and bicarbonate (HCO−) ions from intestinal cells into the lumen. The resulting anion efflux causes a net osmotic increase, driving water into the GI tract and promoting fluid secretion (Figure 5A) (78, 79).
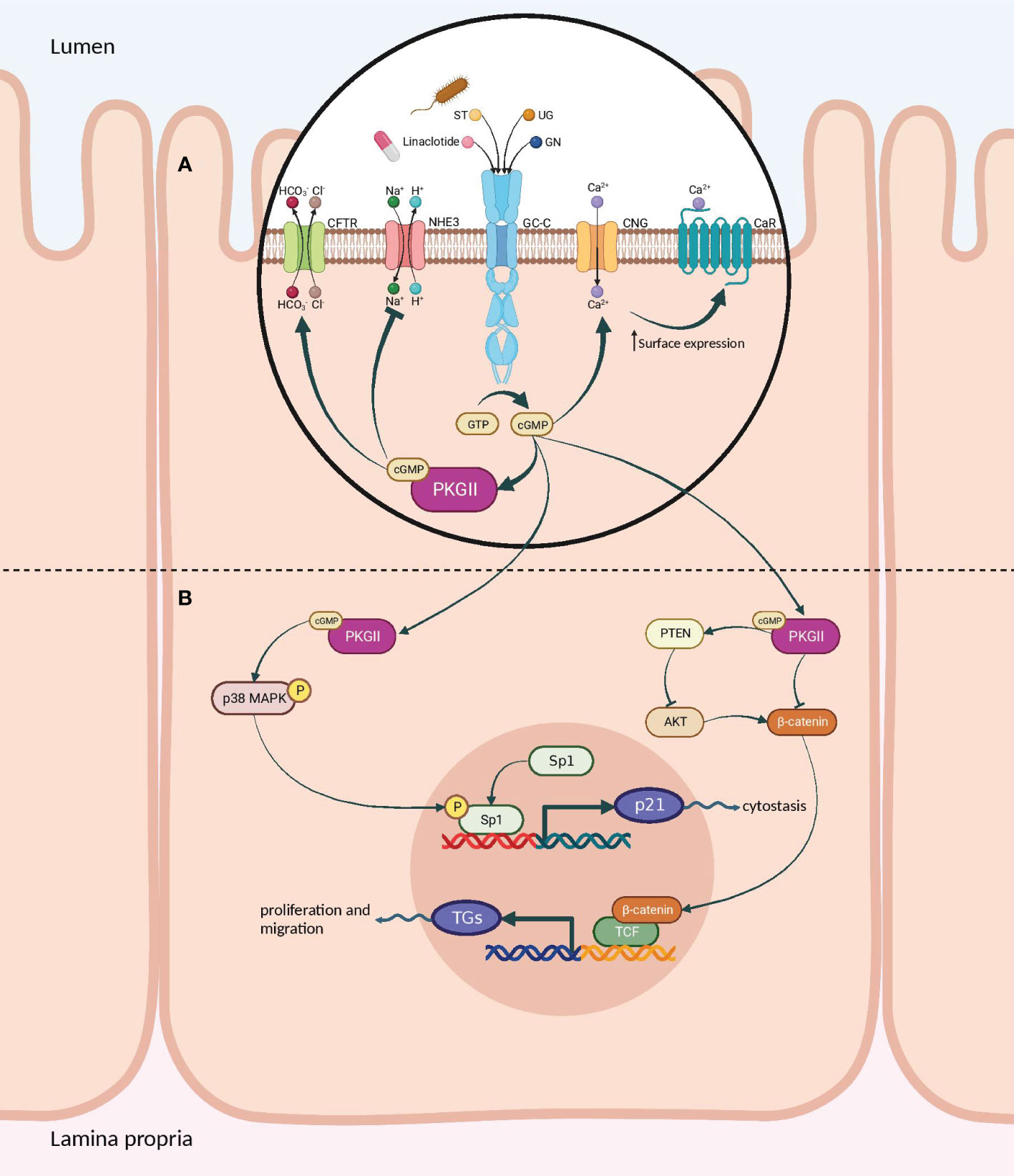
Figure 5 Signaling pathways of the GC-C/cGMP axis that regulate fluid-ion homeostasis and cellular proliferation in the intestine. (A) The binding of ligands to GC-C catalyzes the formation of cGMP from GTP. Increased intracellular cGMP levels result in the activation of cGMP-dependent PKGII. Reduced intestinal sodium absorption is caused by PKGII-mediated inhibitory phosphorylation of NHE3. PKGII phosphorylated and activated the CFTR anion channel, increasing intestinal chloride and water secretion. Increased cGMP activates CNG ion channels, promoting Ca2+influx, which recruits CaR to the plasma membrane. (B) Cyclic GMP production activates PKGII and p38 MAPK, resulting in phosphorylation of the Sp1 transcription factor. Sp1 upregulates the expression of p21 and mediates cytostasis. PKGII-mediated signaling opposes pro-survival and pro-proliferative phenotypes mediated by the β-catenin/TCF and Akt pathways. GC-C, Guanylate Cyclase-C; PKGII, Protein kinase G II; Protein kinase; NHE3, Na+/H+ exchanger isoform 3; CFTR, Cystic fibrosis transmembrane conductance regulator; CNG, Cyclic nucleotide-gated; CaR, calcium-sensing G-protein coupled receptors; MAPK, Mitogen-activated protein kinases; TCF, T cell factor. Created with BioRender.com.
GC-C also controls the balance between proliferation and differentiation by increasing p21 expression under normal physiological circumstances (77, 80). It has been shown that GC-C mediates antitumorigenic processes in addition to p21-mediated cytostasis. One prominent instance is the GC-C signaling-mediated attenuation of β-catenin-mediated TCF transcriptional activity. PKGII-mediated signaling opposes β-catenin/TCF-mediated proliferative and promigratory phenotypes (81, 82). β-catenin/TCF signaling, in turn, suppresses the GC-C axis by inhibiting the transcription of its ligands, GN and UG. Additionally, GC-C blocks PTEN-mediated pro-tumorigenic Akt signaling (Figure 5B) (78, 83–85). As a tumor suppressor, GC-C regulates the migration and differentiation of stem cells at the base of intestinal crypts into enterocytes and other cell types (23). In fact, inhibiting the GC-C axis results in hyper-proliferation, hyperplasia of proliferating crypts, accelerated migration, diminished differentiation along the secretory lineage, and decreased apoptosis (77).
Another noteworthy aspect of GC-C is its potential as a tumor biomarker for CRC detection. While guanylyl cyclase family members are typically only expressed in normal intestinal cells, GC-C is found in approximately 95% of colorectal and some other gastrointestinal cancers, such as pancreatic tumors. In contrast, GC-C expression is rare in non-intestinal tissues and tumors. This suggests that GC-C could be a valuable and novel biomarker for identifying CRC (17, 86, 87).
In a study conducted by Jimenez-Luna et al. (43), the potential of GUCY2C, PTGS2, JAG1, and PGF circulating RNAs as biomarkers in metastatic CRC (mCRC) was investigated. The researchers collected 59 serum and blood samples from mCRC patients, divided into two groups: one receiving chemotherapy plus antiangiogenic treatment and the other receiving only chemotherapy. Additionally, 47 healthy control samples were included. The samples were then analyzed using digital polymerase chain reaction (PCR). The study revealed a significant correlation between GUCY2C and GUCY2C/PTGS2 expression in the bloodstream and the response to anti-angiogenic agents. These results suggest that evaluating genes involved in the process of angiogenesis could serve as a promising non-invasive diagnostic tool for metastatic colorectal cancer and predict its response to anti-angiogenic therapy (43) (Table 2).
Blomain et al. (38) contributed significantly to understanding intestinal tumorigenesis by uncovering the crucial role of the GN hormone and GC-C receptor signaling in tumor initiation and progression. Specifically, their study revealed that the loss of GN hormone expression, but not the GC-C receptor, occurs at the earliest stages of adenomatous polyposis coli (APC)-dependent tumor transformation in both humans and mice. This loss of GN expression results from mutant APC-β-catenin-TCF transcriptional regulation, which suppresses GC-C signaling and perturbs intestinal homeostatic mechanisms, contributing to tumor progression. The authors proposed that the replacement and reconstitution of GC-C signaling could prevent tumorigenesis by restoring the GN hormone expression (38). This finding provides a potential therapeutic target for preventing the development and progression of intestinal tumors.
Recent studies have focused on the extensive utilization of GUCY2C biology in experimental cancer immunotherapy, including developing vaccines, immunotoxins, and chimeric antigen receptor (CAR) T cells (88). Developing cancer vaccines that can activate the immune system to identify and destroy cancer cells holds immense potential (89). One approach that has recently piqued interest is targeting the GC-C receptor to create effective cancer vaccines. For example, the promising results in preclinical and clinical trials suggest that GC-C-based therapies could effectively treat cancer. In the study by Flickinger et al. (48), a prime-boost strategy was investigated that involved the use of a chimeric adenoviral vector (Ad5.F35) that is resistant to pre-existing immunity, followed by recombinant Listeria monocytogenes (Lm) to amplify immunity to the GI cancer antigen GUCY2C. It was found that the combination of Ad5.F35 and Lm-GUCY2C enhanced the quantity, avidity, polyfunctionality, and antitumor efficacy of GUCY2C-specific effector CD8+ T cells in mice. The results suggest that Lm-GUCY2C could be used to increase GUCY2C-specific immunity in patients who are given adenovirus-based GUCY2C vaccines that are currently being tested in clinical trials to prevent or treat recurrent GI cancer (48).
In another study, Lin et al. (32) demonstrated the potential of non-thermal, atmospheric pressure plasma (NTP) in inducing immunogenic cell death in an animal model of CRC. The study included assessments of cell viability, anti-tumor vaccination assays, and ELISpot analysis. The findings suggest that NTP treatment can enhance T-cell responses targeting the CRC-specific antigen GC-C, potentially enhancing the immune system’s ability to recognize and eliminate cancer cells. The study sheds light on the possible role of non-thermal plasma in stimulating immunogenic cell death for future clinical applications in cancer immunotherapy and vaccine manufacturing, particularly for CRC.
CAR T-cell therapy is a promising treatment method by engineering T cells to express CARs that can target cancer cells. By doing this, the immune system can selectively attack and eliminate tumors (90). A highly optimistic target for this therapy is the GC-C receptor. In an investigation, Magee et al. (33) engineered a human-specific single-chain variable fragment (scFv) directed toward GC-C to engineer CAR-T cells for the treatment of colorectal cancer metastasis. The CAR-T cells were tested in preclinical murine models and provided long-term protection against murine colorectal cancer cells expressing human GUCY2C lung metastases. The study also demonstrated the ability of the CAR-T cells to recognize and kill human colorectal cancer cells expressing GUCY2C in a xenograft model in immunodeficient mice. These findings suggest the potential of human GUCY2C-specific CAR-T cell therapy for the treatment of metastatic colorectal cancer expressing GUCY2C (33). GUCY2C-targeted CAR T cells could offer a novel and effective strategy for treating CRC, particularly those in advanced stages of cancer or refractory to conventional therapies.
3.2 GUCA2A
The Guanylate Cyclase Activator 2A (GUCA2A) gene encodes Guanylin (GN), which is a bioactive peptide synthesized in the intestinal mucosa and acts as an endocrine ligand for GC-C. GN serves crucial functions in maintaining intestinal fluid homeostasis and preserving gut physiology (91). The mature form of guanylin consists of 15 amino acids derived from the C-terminus of a longer pre-proguanylin peptide. This longer peptide includes a signal sequence and a proguanylin sequence span residues 1-21 and 22-115, respectively (92). Although some studies indicate that proguanylin is the primary form of the peptide that is secreted, the specific enzymatic pathway that processes proguanylin into its bioactive GN form has not been entirely understood yet (93). The GN peptide comprises four cysteine residues, enabling the assembly of two intramolecular disulfide bonds. The disulfide bonds in the peptide are essential for keeping the peptide in its proper shape, which is required for binding to the GC-C receptor (Figure 6A) (94). The GC-C receptor is additionally stimulated by the enteric bacterial ST peptides, which comprise 19 amino acids and three disulfide bonds and are homologs of GN (93, 95). The 3-disulfide structure of ST peptides significantly enhances their potency at the GC-C receptor, surpassing that of endogenous GC-C agonists (94). GN exists in two isoforms, a right-handed and a left-handed spiral form, which exhibits different biological activities and has varying affinities for binding to GC-C (95). The bioactive isomer of GN binds to the extracellular domain of GC-C, leading to the activation of the receptor’s intracellular domain. The biological relevance and mechanism of the interconversion of these isomers remain unknown (95). Research efforts have aimed to identify the cellular source of guanylin, with early studies proposing that enteroendocrine cells, such as goblet cells, paneth cells, tuft cells, and enterocytes, were responsible for producing these peptides due to their proposed hormonal function. However, discrepancies between studies and techniques have made it difficult to determine a definitive source (93, 96, 97). Although the stimuli that trigger proguanylin secretion remain poorly characterized, evidence suggests a strong association with the salt consumption (98).
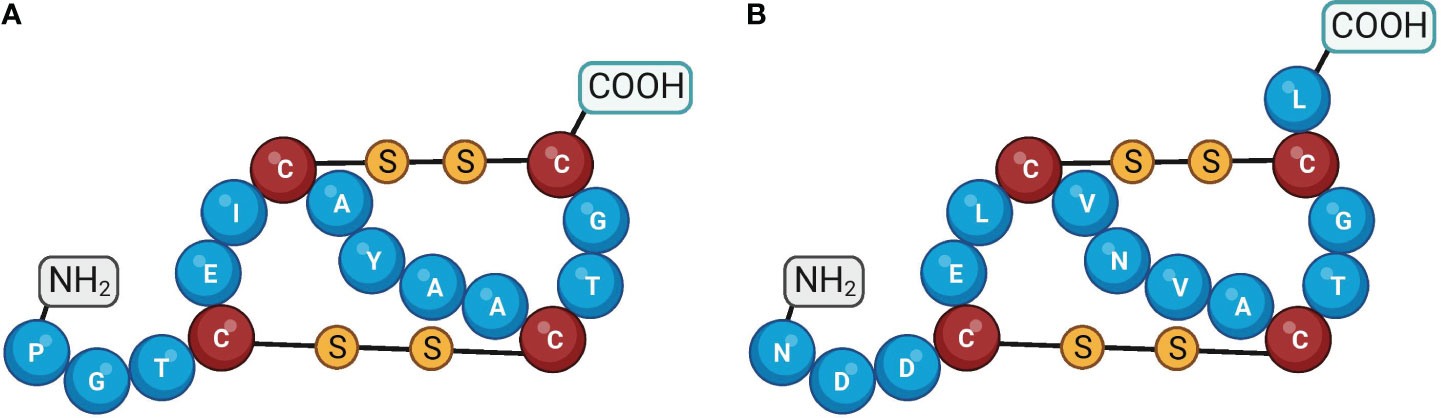
Figure 6 Amino acid structures of guanylin and uroguanylin. Guanylin (A) and uroguanylin (B) The cysteine residues are shown by the different colors of the amino acid and by the disulfide bonds. Created with BioRender.com.
The loss of GN has been shown to have detrimental effects on the intestinal epithelial cells that produce GC-C, disrupting the homeostatic mechanisms necessary for organizing the crypt-villus axis. The GC-C expression remains consistent across the crypt-to-villus axis. However, the endogenous ligands GN and UG are secreted in an ascending gradient, with the highest concentration in the differentiated villus compartment and the lowest in the proliferating crypt compartment. This gradient restricts proliferation and reprograms metabolism in crypts, contributing to maintaining a healthy intestinal epithelium (99) (Figure 7). The perturbation of GN production leads to deficiencies in cellular proliferation, sensing and repair of DNA damage, and metabolic programming, collectively contributing to the development of tumors (85, 100). GN stands out as a frequently absent gene product in the context of colorectal tumorigenesis and is regarded as one of the initial occurrences in the progression of intestinal tissue transformation (101, 102). In an investigation involving a cohort of patients diagnosed with stage I-III CRC, it was observed that both GUCA2A mRNA and peptides exhibited a loss or substantial reduction in cancerous tissues when compared to adjacent healthy tissues in more than 85% of the cases (102). Moreover, lower circulating levels of proguanylin were observed in individuals with obesity, and levels increased following Roux-en-Y gastric bypass surgery, suggesting potential links to metabolism or food intake (103). Consistent with these results, GN expression and peptide levels were reduced in mice fed a high-fat diet. Conversely, the risk of developing colon cancer due to obesity was decreased when GN was forcibly re-expressed (100).
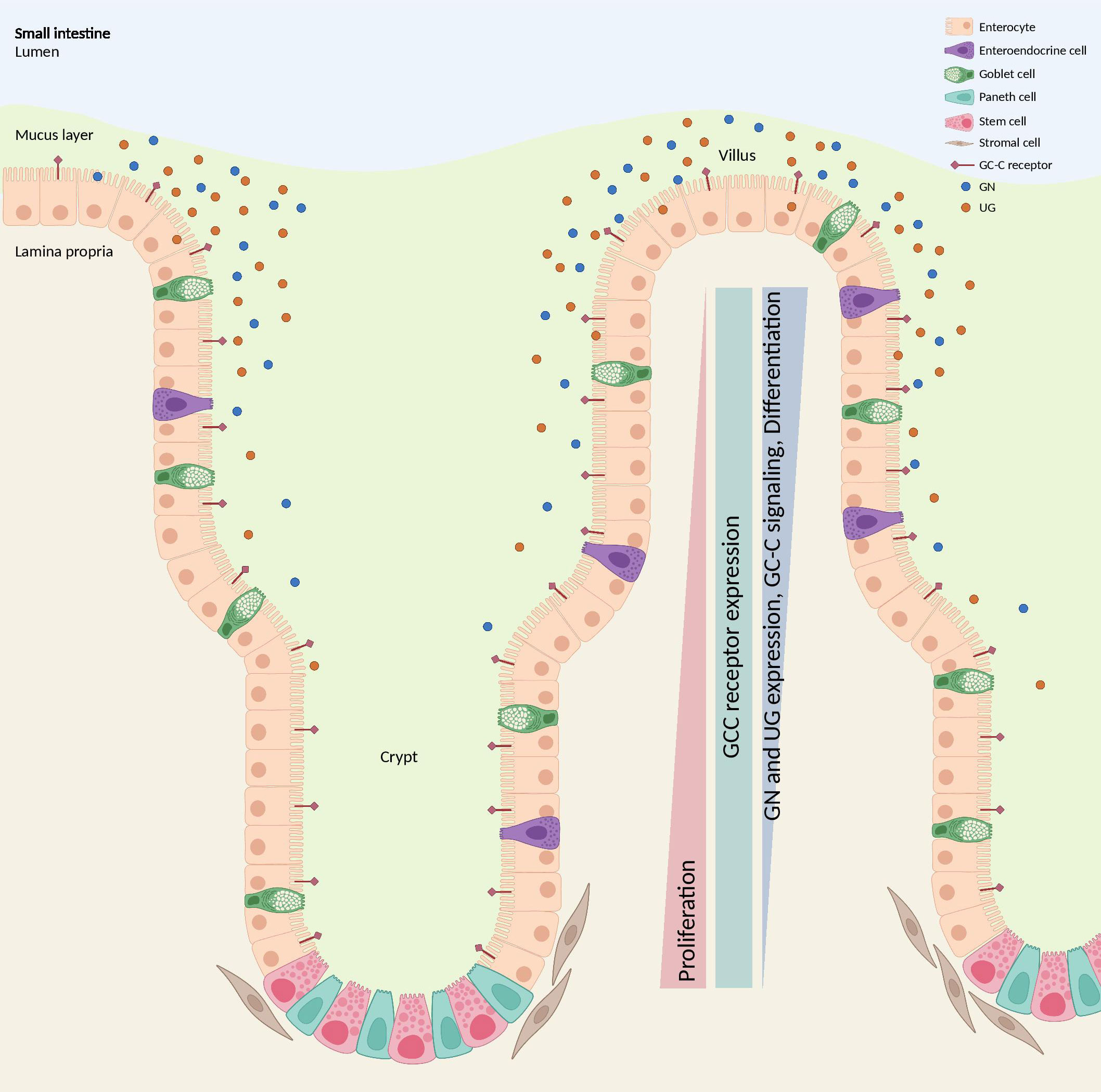
Figure 7 GC-C signaling regulates crypt-villus proliferation. While the GC-C receptors are expressed along the crypt-surface axes, the endogenous ligands GN and UG are produced by differentiated cells bordering the lumen and are absent in crypts. GC-C signaling opposes the proliferative gradient along that vertical axis by restricting cell growth in the crypts and promoting the maturation of differentiated cells. GC-C, guanylate cyclase c; GN, Guanylin; UG, Uroguanylin. Created with BioRender.com.
Bashir et al. (51) found that GUCA2A expression was lost in both tubular and serrated adenomas compared to their corresponding normal colon tissues. The study also suggested that this loss may silence GUCY2C, which could lead to the development of microsatellite instability tumors (51) (Table 3). Blomain et al. (38) reported a similar finding, demonstrating that the loss of GN expression occurs early in APC-dependent tumors in both humans and mice, whereas the GC-C receptor remains intact. In 2022, Liu et al. (60) conducted an integrated bioinformatics analysis to identify hub genes associated with colorectal adenocarcinoma and assess their prognostic significance. They collected colon adenocarcinoma (COAD)/rectum adenocarcinoma (READ) data from the Cancer Genome Atlas (TCGA) database (https://www.cancer.gov/ccg/access-data). Additionally, they utilized the gene expression profile of GSE25070 from the Gene Expression Omnibus (GEO) database (https://www.ncbi.nlm.nih.gov/geo/). This data collection aimed to explore differentially expressed genes between colorectal adenocarcinoma and normal tissues. Their analysis led to the identification of a GUCA2A-CLCA1-CLCA4 gene signature that could accurately predict the prognosis of CRC patients (60). Another study by Ming Li et al. (59) utilized three microarray datasets (GSE23878, GSE33113, and GSE41328) to investigate the relationship between gene expression and patient outcomes in CRC. They identified four differentially expressed genes (DEGs), including GUCA2A, ADH1C, CLCA4, and CXCL8, all of which were associated with significantly lower overall survival in CRC patients (59). Chen et al. (16) analyzed 437 mutation data from colon cancer samples and discovered a positive correlation between GUCA2A expression and patient survival. In a separate study, Hases et al. (55) used data from TCGA database analyzed clinical samples of colorectal tumors, and matched noncancerous adjacent tissue from CRC patients to identify sex-specific biomarkers for CRC. GUCA2A was identified as a prognostic biomarker for CRC, specifically in males (55). Zhang et al. (52) identified GUCA2A as a hub gene significantly correlating with patients’ overall survival (OS). Furthermore, they conducted qPCR analysis and found that GUCA2A is significantly downregulated in tumor and metastatic tissues when compared to adjacent normal tissues (52).
3.3 GUCA2B
The human gene encoding uroguanylin (UG) termed Guanylate Cyclase Activator 2B (GUCA2B), is located on chromosome 1 in humans (1p34.2), which consists of 3 exons. The expression of GUCA2B mRNA resembled that of GUCA2A expression, indicating that GUCA2A promoter-proximal and upstream super-enhancer elements synchronize the expression of both genes (49). UG peptide comprises 16 amino acids and, equal to GN, consists of two disulfide bonds between positions seven and 15 (Figure 6B) (93). This peptide is produced and expressed in enterochromaffin cells predominantly in the proximal part of the small intestine (76, 104). Plasma UG circulates as both propeptide (proUG) and active forms, while plasma GN circulates mainly as proGN (105). Renal tubular brush border membrane-associated enzymes convert the inactive proUG through a proteolytic process into the bioactive UG, resulting in high amounts of UG in the urine (106). Of significance, the expression of UG is invariably lost early during neoplastic transformation in the intestine (107, 108). By the oral administration of human UG, Shailubhai et al. (22) demonstrated that the number of polyps was reduced by 50% in ApcMin/+ mice (mice carrying mutations in the APC gene) as well as the progression of polyps into adenocarcinoma was decreased. In agreement with this finding, Basu et al. (80) showed that oral administration of UG prevented ApcMin/+ mice from forming adenomas, their progression to colon tumors, and the development of inflammation-induced colonic tumors in ApcMin/+ mice. As a bioinformatics research sample, Chu et al. (109) used prediction analysis of microarray (PAM), artificial neural network (ANN), classification and regression trees (CART), and C5.0 to identify gene expression profiles of CRC and normal mucosa. They pooled 16 datasets containing 88 normal mucosal tissues and 1186 CRCs and identified the top eight differential genes in CRCs, including suppressor genes GUCA2B, CA7, IL6R SPIB, CWH43, and AQP8; and oncogenes TCN1 and SPP1 (109).
Recently, Yang et al. (67) performed a comprehensive in silico analysis and discovered that patients with DNAH7 missense mutations might benefit more from ICIs (Table 4). Through establishing the protein-protein interaction (PPI), they identified the top key genes associated with the DNAH7 mutation, including GUCA2B, AQP8, MS4A12, and ZG16 (67). These results may shed light on the possible role of the GUCA2B gene as a predictor of ICIs response in CRC patients, which requires further studies in this regard. Ebadfardzadeh et al. (56) conducted a comprehensive analysis of gene expression patterns in four microarray datasets (GSE113513, GSE10950, GSE25070, and GSE37182) available in GEO, as well as miRNA expression profiles. After analyzing the data, the researchers identified a total of 43 DEGs, including ten hub genes; GUCA2B, GUCA2A, CLCA4, SLC26A3, KRT20, CLCA1, MAOA, MS4A12, AQP8, and ADH1A. Additionally, the team identified four differentially expressed miRNAs that compromise miR-502-3p, miR-552, miR-490-5p, and miR-423-5p. Based on their bioinformatics analysis, the DEGs identified in this study could serve as significant biomarkers in the molecular mechanisms of CRC development, potentially aiding in developing novel strategies for predicting, screening, and diagnosing CRC patients (56). In another study, the GSE41258 and GSE81558 microarray datasets were analyzed by Han et al. to search for specific molecular targets for diagnosis and prognosis in CRC patients (63). A total of 53 DEGs were identified between CRC and normal colorectal tissues. The list was narrowed to ten hub genes, including GUCA2A, GUCA2B, GCG, SST, MS4A12, PLP1, CHGA, PYY, VIP, and CLCA4. However, just CLCA4 and MS4A12 expression levels had a statistically significant effect on CRC patients’ OS (63). In the same vein, the GSE50760 and GSE104178 datasets were further mined to identify potential target genes correlated with CRC pathogenesis. There were 53 overlapped DEGs in these three datasets. The researchers utilized the String-db online tool to assess the possible interaction of the shared DEGs (110). Ultimately, they identified a significant sub-network of ten genes, including GUCA2B, GUCA2A, GCG, BEST4, UCN3, SST, NPY, PYY, OTOP2, and TMEM82. However, expression levels of GUCA2B and GUCA2A have no correlation with the OS of patients with CRC in this study either (58). Nomiri et al. (66) sought to identify a potential target for CRC therapy using Weighted Gene Co-expression Network Analysis (WGCNA) to investigate key modules, hub genes, and mRNA-miRNA regulatory networks correlated with CRC. The study found 372 genes to be considerably positively associated with CRC (r = 0.98, P-value = 9e-07) and determined 22 hub genes through survival and differential expression analyses. Among these hub genes, GUCA2B, C2ORF88, and CCDC68 were found to play crucial roles in the overall survival rate of CRC patients. The expression analysis was consistent with RT-qPCR results, demonstrating a significant reduction in the expression of GUCA2B in CRC tissues. In addition, the study identified top microRNAs correlated with GUCA2B, and receiver operating characteristic (ROC) analyses indicated that GUCA2B has a high diagnostic performance for CRC (66). In 2022, Rappaport et al. (49) examined a GUCY2C ligand transcriptional silencing mechanism by β-catenin/TCF signaling. In this regard, they performed RNA sequencing analysis of conditional human colon cancer cell lines of β-catenin/TCF signaling to map the core Wnt-transcriptional program. As an important result of this investigation, a novel locus control region regulated by APC-β-catenin-TCF silences GUCA2A and GUCA2B transcription was discerned. With this discovery, they introduced a unique opportunity to reverse GUCY2C ligand silencing and oppose tumorigenesis in the context of mutant Wnt signaling (49). A former study by Pucci et al. (111) has established that higher B4GALNT2 gene expression in CRC patients strongly predicts a good prognosis for their cancer outcome. In another study, to further illustrate the biological importance of this gene in CRC, Pucci and her group obtained gene expression data of 626 COAD/READ samples from the TCGA database and divided CRC patients based on B4GALNT2 expression into two different groups, including higher and lower expressers. Patient stratification revealed that the higher expression cohort displayed a concomitantly high level of other genes associated with a favorable prognosis, such as GUCA2B, ZG16, ITLN1, and BEST2 (64).
4 Discussion and future prospects
Early detection of colorectal cancer is crucial, as it enables access to a broader range of therapeutic interventions and significantly impacts patient survival. Our review study highlights the critical involvement of the guanylate cyclase-c signaling pathway in the early stages of colon carcinogenesis. Investigating the signaling pathway expression in individuals with high-risk colon polyps or early stages of colon carcinoma may be beneficial. This could assess the GC-C axis as a diagnostic marker in a cohort study.
To our knowledge, no studies have yet measured the levels of guanylin and uroguanylin in the serum of patients with CRC. Further investigation is needed to fill this gap by examining the serum levels of these proteins in diverse patient cohorts, as well as their correlation with the cancer stage. This information could provide valuable insights into the severity of CRC and help predict disease progression.
In light of the promising advancements in immunotherapy for cancer treatment, investigating the immune roles of GUCA2A, GUCA2B, and GUCY2C genes could provide valuable insights into their potential as targets for immunotherapy. Specifically, GUCY2C has been found to play a regulatory role in intestinal inflammation and inflammatory bowel disease pathology. At the same time, GUCA2A has been associated with the immune signature of CRC. Therefore, further investigations into the immune-related functions of these genes could pave the way for developing more effective immunotherapies for treating CRC.
Recent studies have suggested that targeting GUCA2A, GUCA2B, and GUCY2C genes could be a promising approach for treating CRC. For instance, linaclotide (a GC-C receptor agonist) has been used in previous studies for the prevention of CRC (112), and is currently being evaluated in phase I (NCT01950403) and phase II (NCT03796884) clinical trials for the treatment of CRC. Further research into the use of drugs targeting the GC-C receptor or those that affect these genes could lead to developing more effective and targeted treatments for CRC patients. Many studies have examined the effects of GC-C receptor agonists in treating CRC. However, there is a shortage of drugs specifically targeting the expression of GUCA2A and GUCA2B genes related to CRC. Although research on GN and UG has mainly been done through bioinformatics, it is crucial to carry out in vivo, in vitro, and human studies on GUCA2A and GUCA2B to better comprehend how these genes contribute to CRC growth and advancement.
Finally, the future prospects for the Guanylate cyclase-C signaling pathway in oncology are hopeful, with potential applications in the diagnosis, prognosis, and treatment of CRC. Further research and clinical trials are demanded to fully realize these peptides’ potential and develop novel drug delivery systems that enhance their therapeutic efficacy.
Although research studies have unveiled the significance of the GC-C signaling pathway in colorectal cancer, its practical application in clinical oncology remains constrained. To address this limitation, we propose some future directions for integrating this pathway into clinical settings. Achieving a comprehensive understanding of the GC-C signaling pathway in gastrointestinal cancer, particularly colon cancer, necessitates a comprehensive evaluation of all its constituent components (GUCY2C, GUCA2A, and GUCA2B). Recognizing the transformative impact of systems biology approaches in elucidating complex biological phenomena, we advocate for a multi-layered assessment encompassing this pathway’s transcriptomics, proteomics, metabolomics, and metagenomics, employing high-resolution methods.
5 Conclusion
To sum up, this systematic review provides a comprehensive summary of the current state of knowledge on the potential role of the guanylyl cyclase-c receptor (GC-C) and its endogenous ligands, Guanylin and Uroguanylin, in the development and progression of colorectal cancer (CRC). Clinical studies have shown that GC-C expression and its activators are associated with patient outcomes, suggesting their potential as prognostic tools. The review also underscores the therapeutic potential of GC-C targeted therapies in CRC treatment. The findings suggest that combining GC-C targeted therapies with other treatment modalities could enhance their effectiveness and overcome drug resistance. Hopefully, these results will serve as a valuable resource for researchers and clinicians developing new and effective therapies for CRC patients, ultimately leading to improved patient outcomes. Further research is warranted to determine the efficacy and safety of GC-C targeted therapies, and the reviewed literature provides a foundation for future studies in this field.
Data availability statement
The original contributions presented in the study are included in the article/supplementary material. Further inquiries can be directed to the corresponding author.
Author contributions
MoeinP: Methodology, Writing – original draft, Writing – review & editing. AA: Methodology, Writing – original draft. PJ: Writing – original draft. AS: Writing – original draft. MobinP: Writing – original draft. YT: Writing – original draft. ZS: Conceptualization, Supervision, Writing – review & editing.
Funding
The author(s) declare that no financial support was received for the research, authorship, and/or publication of this article.
Acknowledgments
We would like to thank BioRender.com for providing the high-quality figures used in this manuscript. Further, the Research Institute of Gastroenterology and Liver Disease is gratefully acknowledged for their support and provision of resources throughout the duration of this research.
Conflict of interest
The authors declare that the research was conducted in the absence of any commercial or financial relationships that could be construed as a potential conflict of interest.
Publisher’s note
All claims expressed in this article are solely those of the authors and do not necessarily represent those of their affiliated organizations, or those of the publisher, the editors and the reviewers. Any product that may be evaluated in this article, or claim that may be made by its manufacturer, is not guaranteed or endorsed by the publisher.
References
1. Siegel R, Miller K, Wagle NS, Jemal A. Cancer statistic. CA: A Cancer J Clin (2023) 73(1):17–48. doi: 10.3322/caac.21763
2. Edwards BK, Ward E, Kohler BA, Eheman C, Zauber AG, Anderson RN, et al. Annual report to the nation on the status of cancer 1975-2006, featuring colorectal cancer trends and impact of interventions (risk factors, screening, and treatment) to reduce future rates. Cancer (2010) 116:544–73. doi: 10.1002/cncr.24760
3. Arnold M, Abnet C, Neale R, Vignat J, Giovannucci E, Mcglynn K, et al. Global burden of 5 major types of gastrointestinal cancer. Gastroenterology (2020) 159(1):335–49.e15. doi: 10.1053/j.gastro.2020.02.068
4. Siegel RL, Ward EM, Jemal A. Trends in colorectal cancer incidence rates in the United States by tumor location and stage 1992-2008. Cancer Epidemiol Biomarkers Prev (2012) 21:411–6. doi: 10.1158/1055-9965.EPI-11-1020
5. Schreuders EH, Ruco A, Rabeneck L, Schoen RE, Sung JJ, Young GP, et al. Colorectal cancer screening: a global overview of existing programmes. Gut (2015) 64:1637–49. doi: 10.1136/gutjnl-2014-309086
6. Keum N, Giovannucci E. Global burden of colorectal cancer: emerging trends, risk factors and prevention strategies. Nat Rev Gastroenterol Hepatol (2019) 16:713–32. doi: 10.1038/s41575-019-0189-8
7. Sung H, Ferlay J, Siegel RL, Laversanne M, Soerjomataram I, Jemal A, et al. Global cancer statistics 2020: GLOBOCAN estimates of incidence and mortality worldwide for 36 cancers in 185 countries. CA Cancer J Clin (2021) 71:209–49. doi: 10.3322/caac.21660
8. Kijima S, Sasaki T, Nagata K, Utano K, Lefor AT, Sugimoto H. Preoperative evaluation of colorectal cancer using CT colonography, MRI, and PET/CT. World J Gastroenterol (2014) 20:16964–75. doi: 10.3748/wjg.v20.i45.16964
9. Brenner H, Kloor M, Pox CP. Colorectal cancer. Lancet (2014) 383:1490–502. doi: 10.1016/S0140-6736(13)61649-9
10. Ohhara Y, Fukuda N, Takeuchi S, Honma R, Shimizu Y, Kinoshita I, et al. Role of targeted therapy in metastatic colorectal cancer. World J Gastrointestinal Oncol (2016) 8:642. doi: 10.4251/wjgo.v8.i9.642
11. Hinoue T, Weisenberger DJ, Lange CP, Shen H, Byun HM, Van Den Berg D, et al. Genome-scale analysis of aberrant DNA methylation in colorectal cancer. Genome Res (2012) 22:271–82. doi: 10.1101/gr.117523.110
12. Eynali S, Khoei S, Khoee S, Esmaelbeygi E. Evaluation of the cytotoxic effects of hyperthermia and 5-fluorouracil-loaded magnetic nanoparticles on human colon cancer cell line HT-29. Int J Hyperthermia (2017) 33:327–35. doi: 10.1080/02656736.2016.1243260
13. Nannini M, Pantaleo MA, Maleddu A, Astolfi A, Formica S, Biasco G. Gene expression profiling in colorectal cancer using microarray technologies: results and perspectives. Cancer Treat Rev (2009) 35:201–9. doi: 10.1016/j.ctrv.2008.10.006
14. Muzny DM, Bainbridge MN, Chang K, Dinh HH, Drummond JA, Fowler G, et al. Comprehensive molecular characterization of human colon and rectal cancer. Nature (2012) 487:330–7. doi: 10.1038/nature11252
15. Huang D, Sun W, Zhou Y, Li P, Chen F, Chen H, et al. Mutations of key driver genes in colorectal cancer progression and metastasis. Cancer Metastasis Rev (2018) 37:173–87. doi: 10.1007/s10555-017-9726-5
16. Xu H, Ma Y, Zhang J, Gu J, Jing X, Lu S, et al. Identification and verification of core genes in colorectal cancer. BioMed Res Int (2020) 2020:8082697. doi: 10.1155/2020/8082697
17. Blomain ES, Merlino DJ, Pattison AM, Snook AE, Waldman SA. Guanylyl cyclase C hormone axis at the intersection of obesity and colorectal cancer. Mol Pharmacol (2016) 90:199–204. doi: 10.1124/mol.115.103192
18. Entezari AA, Snook AE, Waldman SA. Guanylyl cyclase 2C (GUCY2C) in gastrointestinal cancers: recent innovations and therapeutic potential. Expert Opin Ther Targets (2021) 25:335–46. doi: 10.1080/14728222.2021.1937124
19. Forte LR. Guanylin regulatory peptides: structures, biological activities mediated by cyclic GMP and pathobiology. Regul Pept (1999) 81:25–39. doi: 10.1016/S0167-0115(99)00033-6
20. Li P, Lin JE, Marszlowicz GP, Valentino MA, Chang C, Schulz S, et al. GCC signaling in colorectal cancer: Is colorectal cancer a paracrine deficiency syndrome? Drug News Perspect (2009) 22:313–8. doi: 10.1358/dnp.2009.22.6.1395254
21. Cohen MB, Hawkins JA, Witte DP. Guanylin mRNA expression in human intestine and colorectal adenocarcinoma. Lab Invest (1998) 78:101–8.
22. Shailubhai K, Yu HH, Karunanandaa K, Wang JY, Eber SL, Wang Y, et al. Uroguanylin treatment suppresses polyp formation in the Apc(Min/+) mouse and induces apoptosis in human colon adenocarcinoma cells via cyclic GMP. Cancer Res (2000) 60:5151–7.
23. Li P, Schulz S, Bombonati A, Palazzo JP, Hyslop TM, Xu Y, et al. Guanylyl cyclase C suppresses intestinal tumorigenesis by restricting proliferation and maintaining genomic integrity. Gastroenterology (2007) 133:599–607. doi: 10.1053/j.gastro.2007.05.052
24. Pitari GM, Li P, Lin JE, Zuzga D, Gibbons AV, Snook AE, et al. The paracrine hormone hypothesis of colorectal cancer. Clin Pharmacol Ther (2007) 82:441–7. doi: 10.1038/sj.clpt.6100325
25. Waldman SA, Hyslop T, Schulz S, Barkun A, Nielsen K, Haaf J, et al. Association of GUCY2C expression in lymph nodes with time to recurrence and disease-free survival in pN0 colorectal cancer. Jama (2009) 301:745–52. doi: 10.1001/jama.2009.141
26. Steinbrecher KA, Harmel-Laws E, Garin-Laflam MP, Mann EA, Bezerra LD, Hogan SP, et al. Murine guanylate cyclase C regulates colonic injury and inflammation. J Immunol (2011) 186:7205–14. doi: 10.4049/jimmunol.1002469
27. Mann EA, Harmel-Laws E, Cohen MB, Steinbrecher KA. Guanylate cyclase C limits systemic dissemination of a murine enteric pathogen. BMC Gastroenterol (2013) 13:135. doi: 10.1186/1471-230X-13-135
28. Page MJ, Mckenzie JE, Bossuyt PM, Boutron I, Hoffmann TC, Mulrow CD, et al. The PRISMA 2020 statement: an updated guideline for reporting systematic reviews. Bmj (2021) 372:n71. doi: 10.1016/j.rec.2021.07.010
29. Sterne JA, Hernán MA, Reeves BC, Savović J, Berkman ND, Viswanathan M, et al. ROBINS-I: a tool for assessing risk of bias in non-randomised studies of interventions. Bmj (2016) 355:i4919. doi: 10.1136/bmj.i4919
30. Chervoneva I, Freydin B, Hyslop T, Waldman SA. Modeling qRT-PCR dynamics with application to cancer biomarker quantification. Stat Methods Med Res (2018) 27:2581–95. doi: 10.1177/0962280216683204
31. Ghai A, Singh B, Li M, Daniels TA, Coelho R, Orcutt K, et al. Optimizing the radiosynthesis of [(68)Ga]DOTA-MLN6907 peptide containing three disulfide cyclization bonds - a GCC specific chelate for clinical radiopharmaceuticals. Appl Radiat Isot (2018) 140:333–41. doi: 10.1016/j.apradiso.2018.08.006
32. Lin AG, Xiang B, Merlino DJ, Baybutt TR, Sahu J, Fridman A, et al. Non-thermal plasma induces immunogenic cell death in vivo in murine CT26 colorectal tumors. Oncoimmunology (2018) 7:e1484978. doi: 10.1080/2162402X.2018.1484978
33. Magee MS, Abraham TS, Baybutt TR, Flickinger JC Jr., Ridge NA, Marszalowicz GP, et al. Human GUCY2C-targeted chimeric antigen receptor (CAR)-expressing T cells eliminate colorectal cancer metastases. Cancer Immunol Res (2018) 6:509–16. doi: 10.1158/2326-6066.CIR-16-0362
34. Sharman SK, Islam BN, Hou Y, Singh N, Berger FG, Sridhar S, et al. Cyclic-GMP-elevating agents suppress polyposis in apc(Min) mice by targeting the preneoplastic epithelium. Cancer Prev Res (Phila) (2018) 11:81–92. doi: 10.1158/1940-6207.CAPR-17-0267
35. Bang YJ, Takano T, Lin CC, Fasanmade A, Yang H, Danaee H, et al. TAK-264 (MLN0264) in previously treated asian patients with advanced gastrointestinal carcinoma expressing guanylyl cyclase C: results from an open-label, non-randomized phase 1 study. Cancer Res Treat (2018) 50:398–404. doi: 10.4143/crt.2017.074
36. Abraham TS, Flickinger JC Jr., Waldman SA, Snook AE. TCR retrogenic mice as a model to map self-tolerance mechanisms to the cancer mucosa antigen GUCY2C. J Immunol (2019) 202:1301–10. doi: 10.4049/jimmunol.1801206
37. Abu-Yousif AO, Cvet D, Gallery M, Bannerman BM, Ganno ML, Smith MD, et al. Preclinical antitumor activity and biodistribution of a novel anti-GCC antibody-drug conjugate in patient-derived xenografts. Mol Cancer Ther (2020) 19:2079–88. doi: 10.1158/1535-7163.MCT-19-1102
38. Blomain ES, Rappaport JA, Pattison AM, Bashir B, Caparosa E, Stem J, et al. APC-β-catenin-TCF signaling silences the intestinal guanylin-GUCY2C tumor suppressor axis. Cancer Biol Ther (2020) 21:441–51. doi: 10.1080/15384047.2020.1721262
39. Flickinger JC Jr., Singh J, Carlson R, Leong E, Baybutt TR, Barton J, et al. Chimeric Ad5.F35 vector evades anti-adenovirus serotype 5 neutralization opposing GUCY2C-targeted antitumor immunity. J Immunother Cancer (2020) 8(2):e001046. doi: 10.1136/jitc-2020-001046
40. Jiang L, Feng JG, Wang G, Zhu YP, Ju HX, Li DC, et al. Circulating guanylyl cyclase C (GCC) mRNA is a reliable metastatic predictor and prognostic index of colorectal cancer. Transl Cancer Res (2020) 9:1843–50. doi: 10.21037/tcr.2020.02.34
41. Mathur D, Root AR, Bugaj-Gaweda B, Bisulco S, Tan X, Fang W, et al. A novel GUCY2C-CD3 T-cell engaging bispecific construct (PF-07062119) for the treatment of gastrointestinal cancers. Clin Cancer Res (2020) 26:2188–202. doi: 10.1158/1078-0432.CCR-19-3275
42. Pattison AM, Barton JR, Entezari AA, Zalewski A, Rappaport JA, Snook AE, et al. Silencing the intestinal GUCY2C tumor suppressor axis requires APC loss of heterozygosity. Cancer Biol Ther (2020) 21:799–805. doi: 10.1080/15384047.2020.1779005
43. Jimenez-Luna C, González-Flores E, Ortiz R, Martínez-González LJ, Antúnez-Rodríguez A, Expósito-Ruiz M, et al. Circulating PTGS2, JAG1, GUCY2C and PGF mRNA in peripheral blood and serum as potential biomarkers for patients with metastatic colon cancer. J Clin Med (2021) 10(11):2248. doi: 10.3390/jcm10112248
44. Maresca KP, Chen J, Mathur D, Giddabasappa A, Root A, Narula J, et al. Preclinical evaluation of (89)Zr-df-iab22m2c pet as an imaging biomarker for the development of the gucy2c-Cd3 bispecific Pf-07062119 as A T cell engaging therapy. Mol Imaging Biol (2021) 23:941–51. doi: 10.1007/s11307-021-01621-0
45. Weinberg DS, Foster NR, Della’zanna G, Mcmurray RP, Kraft WK, Pallotto A, et al. Phase I double-blind, placebo-controlled trial of dolcanatide (SP-333) 27 mg to explore colorectal bioactivity in healthy volunteers. Cancer Biol Ther (2021) 22:544–53. doi: 10.1080/15384047.2021.1967036
46. Asghari Alashti F, Goliaei B, Minuchehr Z. Analyzing large scale gene expression data in colorectal cancer reveals important clues; CLCA1 and SELENBP1 downregulated in CRC not in normal and not in adenoma. Am J Cancer Res (2022) 12:371–80.
47. Flickinger JC Jr., Singh J, Yarman Y, Carlson RD, Barton JR, Waldman SA, et al. T-cell responses to immunodominant listeria epitopes limit vaccine-directed responses to the colorectal cancer antigen, guanylyl cyclase C. Front Immunol (2022) 13:855759. doi: 10.3389/fimmu.2022.855759
48. Flickinger JC Jr., Staudt RE, Singh J, Carlson RD, Barton JR, Baybutt TR, et al. Chimeric adenoviral (Ad5.F35) and listeria vector prime-boost immunization is safe and effective for cancer immunotherapy. NPJ Vaccines (2022) 7:61. doi: 10.1038/s41541-022-00483-z
49. Rappaport JA, Entezari AA, Caspi A, Caksa S, Jhaveri AV, Stanek TJ, et al. A β-catenin-TCF-sensitive locus control region mediates GUCY2C ligand loss in colorectal cancer. Cell Mol Gastroenterol Hepatol (2022) 13:1276–96. doi: 10.1016/j.jcmgh.2021.12.014
50. Kim R, Leal AD, Parikh A, Ryan DP, Wang S, Bahamon B, et al. A phase I, first-in-human study of TAK-164, an antibody-drug conjugate, in patients with advanced gastrointestinal cancers expressing guanylyl cyclase C. Cancer Chemother Pharmacol (2023) 91:291–300. doi: 10.1007/s00280-023-04507-w
51. Bashir B, Merlino DJ, Rappaport JA, Gnass E, Palazzo JP, Feng Y, et al. Silencing the GUCA2A-GUCY2C tumor suppressor axis in CIN, serrated, and MSI colorectal neoplasia. Hum Pathol (2019) 87:103–14. doi: 10.1016/j.humpath.2018.11.032
52. Zhang H, Du Y, Wang Z, Lou R, Wu J, Feng J. Integrated analysis of oncogenic networks in colorectal cancer identifies GUCA2A as a molecular marker. Biochem Res Int (2019) 2019:6469420. doi: 10.1155/2019/6469420
53. Zheng ZG, Ma BQ, Xiao Y, Wang TX, Yu T, Huo YH, et al. Identification of biomarkers for the diagnosis and treatment of primary colorectal cancer based on microarray technology. Trans Cancer Res (2020) 9:3453. doi: 10.21037/tcr-19-2290
54. Chen J, Apizi A, Wang L, Wu G, Zhu Z, Yao H, et al. TCGA database analysis of the tumor mutation burden and its clinical significance in colon cancer. J Gastrointestinal Oncol (2021) 12:2244. doi: 10.21037/jgo-21-661
55. Hases L, Ibrahim A, Chen X, Liu Y, Hartman J, Williams C. The importance of sex in the discovery of colorectal cancer prognostic biomarkers. Int J Mol Sci (2021) 22(3):1354. doi: 10.3390/ijms22031354
56. Ebadfardzadeh J, Kazemi M, Aghazadeh A, Rezaei M, Shirvaliloo M, Sheervalilou R. Employing bioinformatics analysis to identify hub genes and microRNAs involved in colorectal cancer. Med Oncol (2021) 38:114. doi: 10.1007/s12032-021-01543-5
57. Chodary Khameneh S, Razi S, Shamdani S, Uzan G, Naserian S. Weighted correlation network analysis revealed novel long non-coding RNAs for colorectal cancer. Sci Rep (2022) 12:2990. doi: 10.1038/s41598-022-06934-w
58. Guo S, Sun Y. OTOP2, inversely modulated by miR-3148, inhibits CRC cell migration, proliferation and epithelial-mesenchymal transition: evidence from bioinformatics data mining and experimental verification. Cancer Manag Res (2022) 14:1371–84. doi: 10.2147/CMAR.S345299
59. Li M, Liu Z, Song J, Wang T, Wang H, Wang Y, et al. Identification of down-regulated ADH1C is associated with poor prognosis in colorectal cancer using bioinformatics analysis. Front Mol Biosci (2022) 9:791249. doi: 10.3389/fmolb.2022.791249
60. Liu Y, Chen L, Meng X, Ye S, Ma L. Identification of hub genes in colorectal adenocarcinoma by integrated bioinformatics. Front Cell Dev Biol (2022) 10:897568. doi: 10.3389/fcell.2022.897568
61. Feodorova Y, Tashkova D, Koev I, Todorov A, Kostov G, Simitchiev K, et al. Novel insights into transcriptional dysregulation in colorectal cancer. Neoplasma (2018) 65:415–24. doi: 10.4149/neo_2018_170707N467
62. Parikh K, Antanaviciute A, Fawkner-Corbett D, Jagielowicz M, Aulicino A, Lagerholm C, et al. Colonic epithelial cell diversity in health and inflammatory bowel disease. Nature (2019) 567:49–55. doi: 10.1038/s41586-019-0992-y
63. Han J, Zhang X, Liu Y, Jing L, Liu YB, Feng L. CLCA4 and MS4A12 as the significant gene biomarkers of primary colorectal cancer. Biosci Rep (2020) 40(8):BSR20200963. doi: 10.1042/BSR20200963
64. Pucci M, Malagolini N, Dall’olio F. Glycosyltransferase B4GALNT2 as a predictor of good prognosis in colon cancer: lessons from databases. Int J Mol Sci (2021) 22(9):4331. doi: 10.3390/ijms22094331
65. Bouzo BL, Lores S, Jatal R, Alijas S, Alonso MJ, Conejos-Sánchez I, et al. Sphingomyelin nanosystems loaded with uroguanylin and etoposide for treating metastatic colorectal cancer. Sci Rep (2021) 11:17213. doi: 10.1038/s41598-021-96578-z
66. Nomiri S, Hoshyar R, Chamani E, Rezaei Z, Salmani F, Larki P, et al. Prediction and validation of GUCA2B as the hub-gene in colorectal cancer based on co-expression network analysis: In-silico and in-vivo study. BioMed Pharmacother (2022) 147:112691. doi: 10.1016/j.biopha.2022.112691
67. Yang W, Shen Z, Yang T, Wu M. DNAH7 mutations benefit colorectal cancer patients receiving immune checkpoint inhibitors. Ann Transl Med (2022) 10:1335. doi: 10.21037/atm-22-6166
68. Horaira MA, Islam MA, Kibria MK, Alam MJ, Kabir SR, Mollah MNH. Bioinformatics screening of colorectal-cancer causing molecular signatures through gene expression profiles to discover therapeutic targets and candidate agents. BMC Med Genomics (2023) 16:64. doi: 10.1186/s12920-023-01488-w
69. Horst BG, Yokom AL, Rosenberg DJ, Morris KL, Hammel M, Hurley JH, et al. Allosteric activation of the nitric oxide receptor soluble guanylate cyclase mapped by cryo-electron microscopy. Elife (2019) 8:e50634. doi: 10.7554/eLife.50634
70. Kang Y, Liu R, Wu JX, Chen L. Structural insights into the mechanism of human soluble guanylate cyclase. Nature (2019) 574:206–10. doi: 10.1038/s41586-019-1584-6
71. Yarla NS, Gali H, Pathuri G, Smriti S, Farooqui M, Panneerselvam J, et al. Targeting the paracrine hormone-dependent guanylate cyclase/cGMP/phosphodiesterases signaling pathway for colorectal cancer prevention. Semin Cancer Biol (2019) 56:168–74. doi: 10.1016/j.semcancer.2018.08.011
72. Bose A, Visweswariah SS. The pseudokinase domain in receptor guanylyl cyclases. Methods Enzymol (2022) 667:535–74. doi: 10.1016/bs.mie.2022.03.046
73. Khalil I, Walker R, Porter CK, Muhib F, Chilengi R, Cravioto A, et al. Enterotoxigenic Escherichia coli (ETEC) vaccines: Priority activities to enable product development, licensure, and global access. Vaccine (2021) 39:4266–77. doi: 10.1016/j.vaccine.2021.04.018
74. Hossain MS, Karuniawati H, Jairoun AA, Urbi Z, Ooi J, John A, et al. Colorectal cancer: A review of carcinogenesis, global epidemiology, current challenges, risk factors, preventive and treatment strategies. Cancers (Basel) (2022) 14(7):1732. doi: 10.3390/cancers14071732
75. Li P, Lin JE, Snook AE, Waldman SA. ST-producing E. coli oppose carcinogen-induced colorectal tumorigenesis in mice. Toxins (Basel) (2017) 9(9):279. doi: 10.3390/toxins9090279
76. Rahbi H, Narayan H, Jones DJ, Ng LL. The uroguanylin system and human disease. Clin Sci (Lond) (2012) 123:659–68. doi: 10.1042/CS20120021
77. Waldman SA, Camilleri M. Guanylate cyclase-C as a therapeutic target in gastrointestinal disorders. Gut (2018) 67:1543–52. doi: 10.1136/gutjnl-2018-316029
78. Prasad H, Mathew JKK, Visweswariah SS. Receptor guanylyl cyclase C and cyclic GMP in health and disease: perspectives and therapeutic opportunities. Front Endocrinol (Lausanne) (2022) 13:911459. doi: 10.3389/fendo.2022.911459
79. Bose A, Banerjee S, Visweswariah SS. Mutational landscape of receptor guanylyl cyclase C: Functional analysis and disease-related mutations. IUBMB Life (2020) 72:1145–59. doi: 10.1002/iub.2283
80. Basu N, Saha S, Khan I, Ramachandra SG, Visweswariah SS. Intestinal cell proliferation and senescence are regulated by receptor guanylyl cyclase C and p21. J Biol Chem (2014) 289:581–93. doi: 10.1074/jbc.M113.511311
81. Thompson WJ, Piazza GA, Li H, Liu L, Fetter J, Zhu B, et al. Exisulind induction of apoptosis involves guanosine 3’,5’-cyclic monophosphate phosphodiesterase inhibition, protein kinase G activation, and attenuated beta-catenin. Cancer Res (2000) 60:3338–42.
82. Li N, Lee K, Xi Y, Zhu B, Gary BD, Ramírez-Alcántara V, et al. Phosphodiesterase 10A: a novel target for selective inhibition of colon tumor cell growth and β-catenin-dependent TCF transcriptional activity. Oncogene (2015) 34:1499–509. doi: 10.1038/onc.2014.94
83. Deguchi A, Thompson WJ, Weinstein IB. Activation of protein kinase G is sufficient to induce apoptosis and inhibit cell migration in colon cancer cells. Cancer Res (2004) 64:3966–73. doi: 10.1158/0008-5472.CAN-03-3740
84. Basu N, Bhandari R, Natarajan VT, Visweswariah SS. Cross talk between receptor guanylyl cyclase C and c-src tyrosine kinase regulates colon cancer cell cytostasis. Mol Cell Biol (2009) 29:5277–89. doi: 10.1128/MCB.00001-09
85. Lin JE, Li P, Snook AE, Schulz S, Dasgupta A, Hyslop TM, et al. The hormone receptor GUCY2C suppresses intestinal tumor formation by inhibiting AKT signaling. Gastroenterology (2010) 138:241–54. doi: 10.1053/j.gastro.2009.08.064
86. Pattison AM, Merlino DJ, Blomain ES, Waldman SA. Guanylyl cyclase C signaling axis and colon cancer prevention. World J Gastroenterol (2016) 22:8070–7. doi: 10.3748/wjg.v22.i36.8070
87. Lisby AN, Flickinger JC Jr., Bashir B, Weindorfer M, Shelukar S, Crutcher M, et al. GUCY2C as a biomarker to target precision therapies for patients with colorectal cancer. Expert Rev Precis Med Drug Dev (2021) 6:117–29. doi: 10.1080/23808993.2021.1876518
88. Baybutt TR, Aka AA, Snook AE. The heat-stable enterotoxin receptor, guanylyl cyclase C, as a pharmacological target in colorectal cancer immunotherapy: A bench-to-bedside current report. Toxins (Basel) (2017) 9(9):282. doi: 10.3390/toxins9090282
89. Morse MA, Gwin WR, Mitchell DA. Vaccine therapies for cancer: then and now. Target Oncol (2021) 16:121–52. doi: 10.1007/s11523-020-00788-w
90. Hong M, Clubb JD, Chen YY. Engineering CAR-T cells for next-generation cancer therapy. Cancer Cell (2020) 38:473–88. doi: 10.1016/j.ccell.2020.07.005
91. Caspi A, Entezari AA, Crutcher M, Snook AE, Waldman SA. Guanylyl cyclase C as a diagnostic and therapeutic target in colorectal cancer. Personalized Med (2022) 19:457–72. doi: 10.2217/pme-2022-0026
92. Porto WF, Franco OL, Alencar SA. Computational analyses and prediction of guanylin deleterious SNPs. Peptides (2015) 69:92–102. doi: 10.1016/j.peptides.2015.04.013
93. De Sauvage FJ, Keshav S, Kuang WJ, Gillett N, Henzel W, Goeddel DV. Precursor structure, expression, and tissue distribution of human guanylin. Proc Natl Acad Sci U.S.A. (1992) 89:9089–93. doi: 10.1073/pnas.89.19.9089
94. Camilleri M. Guanylate cyclase C agonists: emerging gastrointestinal therapies and actions. Gastroenterology (2015) 148:483–7. doi: 10.1053/j.gastro.2015.01.003
95. Skelton NJ, Garcia KC, Goeddel DV, Quan C, Burnier JP. Determination of the solution structure of the peptide hormone guanylin: observation of a novel form of topological stereoisomerism. Biochemistry (1994) 33:13581–92. doi: 10.1021/bi00250a010
96. Ikpa PT, Sleddens HF, Steinbrecher KA, Peppelenbosch MP, De Jonge HR, Smits R, et al. Guanylin and uroguanylin are produced by mouse intestinal epithelial cells of columnar and secretory lineage. Histochem Cell Biol (2016) 146:445–55. doi: 10.1007/s00418-016-1453-4
97. Dye FS, Larraufie P, Kay R, Darwish T, Rievaj J, Goldspink DA, et al. Characterisation of proguanylin expressing cells in the intestine - evidence for constitutive luminal secretion. Sci Rep (2019) 9:15574. doi: 10.1038/s41598-019-52049-0
98. Kita T, Kitamura K, Sakata J, Eto T. Marked increase of guanylin secretion in response to salt loading in the rat small intestine. Am J Physiol (1999) 277:G960–6. doi: 10.1152/ajpgi.1999.277.5.G960
99. Li P, Lin JE, Schulz S, Pitari GM, Waldman SA. Can colorectal cancer be prevented or treated by oral hormone replacement therapy? Curr Mol Pharmacol (2009) 2:285–92. doi: 10.2174/1874-470210902030285
100. Lin JE, Colon-Gonzalez F, Blomain E, Kim GW, Aing A, Stoecker B, et al. Obesity-induced colorectal cancer is driven by caloric silencing of the guanylin-GUCY2C paracrine signaling axis. Cancer Res (2016) 76:339–46. doi: 10.1158/0008-5472.CAN-15-1467-T
101. Notterman DA, Alon U, Sierk AJ, Levine AJ. Transcriptional gene expression profiles of colorectal adenoma, adenocarcinoma, and normal tissue examined by oligonucleotide arrays. Cancer Res (2001) 61:3124–30.
102. Wilson C, Lin JE, Li P, Snook AE, Gong J, Sato T, et al. The paracrine hormone for the GUCY2C tumor suppressor, guanylin, is universally lost in colorectal cancer. Cancer Epidemiol Biomarkers Prev (2014) 23:2328–37. doi: 10.1158/1055-9965.EPI-14-0440
103. Fernandez-Cachon ML, Pedersen SL, Rigbolt KT, Zhang C, Fabricius K, Hansen HH, et al. Guanylin and uroguanylin mRNA expression is increased following Roux-en-Y gastric bypass, but guanylins do not play a significant role in body weight regulation and glycemic control. Peptides (2018) 101:32–43. doi: 10.1016/j.peptides.2017.12.024
104. Qian X, Prabhakar S, Nandi A, Visweswariah SS, Goy MF. Expression of GC-C, a receptor-guanylate cyclase, and its endogenous ligands uroguanylin and guanylin along the rostrocaudal axis of the intestine. Endocrinology (2000) 141:3210–24. doi: 10.1210/endo.141.9.7644
105. Moss NG, Fellner RC, Qian X, Yu SJ, Li Z, Nakazato M, et al. Uroguanylin, an intestinal natriuretic peptide, is delivered to the kidney as an unprocessed propeptide. Endocrinology (2008) 149:4486–98. doi: 10.1210/en.2007-1725
106. Qian X, Moss NG, Fellner RC, Goy MF. Circulating prouroguanylin is processed to its active natriuretic form exclusively within the renal tubules. Endocrinology (2008) 149:4499–509. doi: 10.1210/en.2007-1724
107. Pitari GM, Baksh RI, Harris DM, Li P, Kazerounian S, Waldman SA. Interruption of homologous desensitization in cyclic guanosine 3’,5’-monophosphate signaling restores colon cancer cytostasis by bacterial enterotoxins. Cancer Res (2005) 65:11129–35. doi: 10.1158/0008-5472.CAN-05-2381
108. Lin JE, Snook AE, Li P, Stoecker BA, Kim GW, Magee MS, et al. GUCY2C opposes systemic genotoxic tumorigenesis by regulating AKT-dependent intestinal barrier integrity. PloS One (2012) 7:e31686. doi: 10.1371/journal.pone.0031686
109. Chu CM, Yao CT, Chang YT, Chou HL, Chou YC, Chen KH, et al. Gene expression profiling of colorectal tumors and normal mucosa by microarrays meta-analysis using prediction analysis of microarray, artificial neural network, classification, and regression trees. Dis Markers (2014) 2014:634123. doi: 10.1155/2014/634123
110. Szklarczyk D, Franceschini A, Wyder S, Forslund K, Heller D, Huerta-Cepas J, et al. STRING v10: protein–protein interaction networks, integrated over the tree of life. Nucleic Acids Res (2015) 43:D447–52. doi: 10.1093/nar/gku1003
111. Pucci M, Gomes Ferreira I, Orlandani M, Malagolini N, Ferracin M, Dall’olio F. High expression of the sd(a) synthase B4GALNT2 associates with good prognosis and attenuates stemness in colon cancer. Cells (2020) 9(4):948. doi: 10.3390/cells9040948
Keywords: Guanylate cyclase-C Signaling Axis, Guanylyl cyclase C, guanylin, uroguanylin, colorectal cancer, therapeutic target
Citation: Piroozkhah M, Aghajani A, Jalali P, Shahmoradi A, Piroozkhah M, Tadlili Y and Salehi Z (2023) Guanylate cyclase-C Signaling Axis as a theragnostic target in colorectal cancer: a systematic review of literature. Front. Oncol. 13:1277265. doi: 10.3389/fonc.2023.1277265
Received: 14 August 2023; Accepted: 28 September 2023;
Published: 20 October 2023.
Edited by:
Zsolt Kovács, George Emil Palade University of Medicine, Pharmacy, Sciences and Technology of Târgu Mureş, RomaniaReviewed by:
Li Zhang, University of Minnesota Twin Cities, United StatesShinobu Ohnuma, Tohoku University, Japan
Copyright © 2023 Piroozkhah, Aghajani, Jalali, Shahmoradi, Piroozkhah, Tadlili and Salehi. This is an open-access article distributed under the terms of the Creative Commons Attribution License (CC BY). The use, distribution or reproduction in other forums is permitted, provided the original author(s) and the copyright owner(s) are credited and that the original publication in this journal is cited, in accordance with accepted academic practice. No use, distribution or reproduction is permitted which does not comply with these terms.
*Correspondence: Zahra Salehi, WmFocmEuc2FsZWhpNjQ2M0B5YWhvby5jb20=
†These authors have contributed equally to this work and share first authorship