- 1Laboratori i Servei d’Urologia, Hospital Clínic de Barcelona, Barcelona, Spain
- 2Genètica i tumors urològics, Fundació de Recerca Clínic Barcelona-Institut d’Investigacions Biomèdiques August Pi i Sunyer (FRCB-IDIBAPS), Barcelona, Spain
- 3Departament de Biomedicina, Facultat de Medicina i Ciències de la Salut, Universitat de Barcelona (UB), Barcelona, Spain
- 4Servei d’Anatomia Patològica, Hospital Clínic de Barcelona, Barcelona, Spain
- 5Servei d’Oncologia Mèdica, Hospital Clínic de Barcelona, Barcelona, Spain
Background and aims: The spatial and temporal genetic heterogeneity of bladder cancer (BC) makes challenging to find specific drivers of metastatic disease, thus preventing to determine those BC patients at high risk of tumor progression. Our aim was to identify DNA mutations providing aggressive behavior to bladder tumors and analyze them in patients’ cell-free DNA (cfDNA) during their follow-up after radical cystectomy (RC) in order to monitor tumor evolution.
Methods: Six BC patients who underwent RC and presented disease progression during their follow-up were included. Next-generation sequencing was used to determine somatic mutations in several primary tumor and metastatic specimens from each patient. Shared DNA mutations between primary bladder tumor and metastatic sites were identified in cfDNA samples through droplet digital PCR.
Results: Besides BC genetic heterogeneity, specific mutations in at least one of these genes —TERT, ATM, RB1, and FGFR3— were found in primary tumors and their metastases in all patients. These mutations were also identified in the patients’ cfDNA at different follow-up time points. Additionally, the dynamic changes of these mutations in cfDNA allowed us to determine tumor evolution in response to treatment.
Conclusion: The analysis of BC mutations associated with poor prognosis in plasma cfDNA could be a valuable tool to monitor tumor evolution, thus improving the clinical management of BC patients.
1 Introduction
Approximately 25% of bladder cancers (BC) patients are diagnosed as muscle-invasive bladder cancer (MIBC). Radical cystectomy (RC) with lymphadenectomy, preceded by neoadjuvant chemotherapy in eligible patients, is the standard treatment for localized MIBC. However, despite undergoing radical surgery, up to 50% of MIBC patients will develop local relapse or distant metastasis within the first two years of RC (1). Metastatic BC patients have a poor prognosis with a five-year relative survival of 6% (2). These patients are generally offered platinum-based chemotherapy; however, the response rate achieved is around 50% (3). An improved understanding of the underlying biology of BC would lead to advances in the treatment and clinical outcomes of this tumor.
The rapid evolution of sequencing technologies has revealed the great complexity of the genomic abnormalities of individual cancer cells and substantial molecular intratumor heterogeneity (ITH), which may evolve over the course of disease and treatment exposure (4). These findings may explain the failure of some approaches toward predictive biomarker development that are exacerbated by tumor sampling bias driven by ITH while also indicating the way forward (5). Examining tumor clonal architecture and its evolution throughout treatment and disease progression may enable the identification of common tumor driver events and individual heterogeneous somatic events present in each tumor that contribute to drug resistance and treatment failure.
Liquid biopsy has emerged to eliminate tumor molecular profile limitations: it is a non-invasive technique, detects tumor clonal evolution in real-time, and reveals ITH (6). Notably, cell-free DNA (cfDNA)-based liquid biopsy has multiple clinical applications and has been studied in different types of tumors, including BC, as a biomarker for detecting molecular relapse or minimal residual disease, monitoring treatment response, or identifying therapy resistance mechanisms (7–11). However, cfDNA-based liquid biopsy has not been implemented in clinical urological practice yet. This is most likely because of the high BC genetic intra- and inter-lesion heterogeneity that makes a challenge to identify those biomarkers predicting which patients are at high risk of metastatic relapse and consequently guiding treatment decisions. In this study, we aimed to determine DNA mutations providing aggressive behavior to bladder tumors and analyze them in patients’ cfDNA during their follow up after RC. The identification of these mutations in bloodstream could be helpful to monitor tumor evolution in response to treatments, thus improving clinical management of bladder cancer patients.
2 Materials and methods
2.1 Patients and samples
Six BC patients, who underwent RC and lymphadenectomy between 2011 and 2019 at our center and showed disease progression during their follow-up, were included in this study. The exclusion criterion was the presence of another active neoplasm. The clinicopathological features and treatments received by the patients enrolled are summarized in Supplementary Table S1. None of the patients received neoadjuvant chemotherapy. Tumor dissemination was controlled postoperatively via a computed tomography scan at three-month intervals during the first year, six-month intervals for the next two years, and annually thereafter. Tumors were considered progressing when relapse or distant metastasis developed during follow-up.
Primary tumor specimens were obtained from cystectomy (N=5) and transurethral resection of bladder tumor (TURBT) (N=1). Metastatic tissue specimens were obtained from locoregional (N=2) and distant (N=3) metastases from the IDIBAPS biobank, except in one patient (Pt#6), whose metastatic biopsy was not available. One to four biopsies were obtained from primary tumors and locoregional/distant metastases from each patient (Figure 1; Supplementary Figure S1). Tissue sections were stained using hematoxylin and eosin (H&E) staining to differentiate cellular components, according to standard histopathological laboratory protocol.
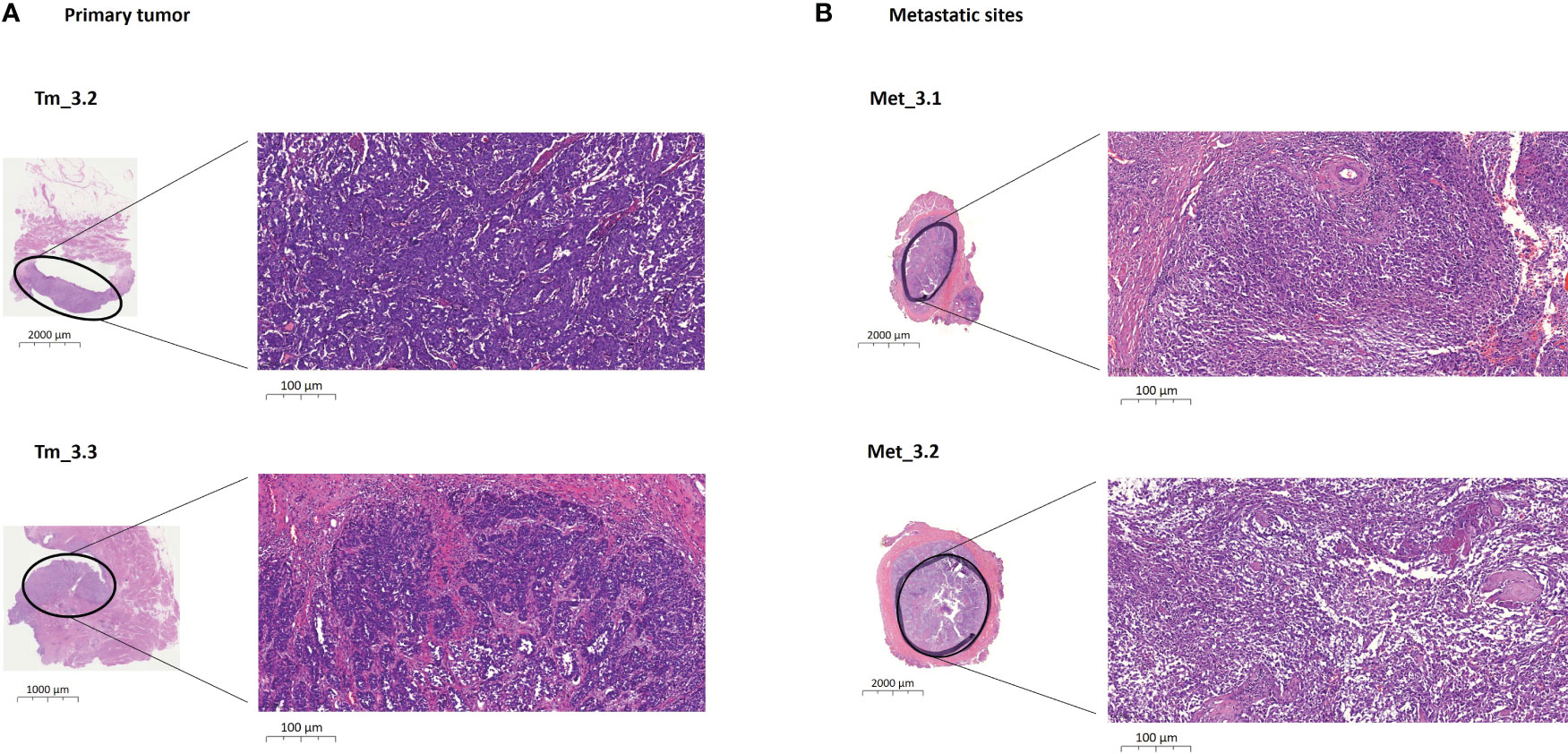
Figure 1 Example of biopsies obtained from a primary bladder tumor and distant metastases from one patient (Pt#3). (A) Images of histological regions (Tm_3.2 and Tm_3.3) stained with H&E from the RC specimen at different scales. These regions present a morphology of high-grade conventional urothelial carcinoma that invades the subepithelial connective tissue, developing solid nests and pseudoglandular structures. (B) Images of histological regions(Met_3.1 and Met_3.2) stained with H&E from the phallectomy specimen at different scales. These regions show tumoral invasion, presenting a sarcomatoid morphology that affects cavernous bodies. H&E, hematoxylin and eosin; Met, metastatic specimen; Pt, patient; RC, radical cystectomy; Tm, primary tumor specimen.
An immunohistochemistry (IHC) panel composed of three markers was performed to determine the tumor’s molecular subtype. Formalin-fixed paraffin-embedded (FFPE) tissue samples were cut and stained on BenchMark ULTRA VENTANA (Roche Diagnostics, Tucson, AZ) with CK20 (VENTANA CONFIRM anti-Cytokeratin20, SP33 clone), GATA3 (CELL MARQUE GATA3 L50-823 clone), and CK5 (VENTANA anti-cytokeratin 5/6 D5/16B4 clone) antibodies. Neoplasms expressing intense CK20 and/or GATA3 were classified as luminal subtypes. CK5-positive neoplasms with loss of GATA3 or weak positivity were categorized as basal subtypes.
Genomic DNA was isolated from 26 FFPE sections of 20 µm using the RecoverAll Total Nucleic Acid Isolation kit (Ambion, Inc. Austin, TX, USA), according to the manufacturer’s instructions. Then, DNA was quantified by spectrophotometric analysis at 260 nm (NanoDrop Technologies, Wilmington, DE, USA).
One 10 mL EDTA tube of peripheral blood was collected before RC and at one, four, 12, and 24 months after surgery, except for one patient (Pt#5), from whom only one blood sample was collected seven years after RC. Blood samples were stored at 4°C until processed within the following 24 h.
2.2 Next-generation sequencing of primary tissue and metastasis
A total of 15 primary tumor and 11 metastasis biopsies were analyzed by NGS using the Oncomine Bladder Panel (Thermo Fisher Scientific, Massachusetts, USA) with six additional genes. The 31 genes involved in this panel are shown in Supplementary Table S2. Ten ng of DNA were used for library preparation according to the manufacturer’s instructions. Sequencing was performed on an Ion S5 System using the Ion 540 Chip (Thermo Fisher Scientific). The mean depth of coverage was 1,104X (range 58-2,000X). NGS data were analyzed with Ion Reporter Software v5.12 (Thermo Fisher Scientific) using an Oncomine Extended (5.18) filter to select for pathogenic variants. The NGS data are available in https://www.ncbi.nlm.nih.gov/clinvar/submitters/509290/.
The clonal evolution of the sequenced tumors was estimated with PHYLOViZ v.2.0. The pathogenic variants identified in each tumor region for each patient were used to develop a phylogenetic tree showing tumor evolution.
2.3 Blood sample procedures
Blood samples were centrifuged at 3,500 rpm for 15 min at 4°C to separate plasma, followed by plasma centrifugation at 16,000 x g for 10 min at 4°C to remove any remaining cells. Plasma samples were stored at -80°C until cfDNA extraction.
cfDNA was extracted from 2-5 ml of plasma (depending on availability) using the QIAamp Circulating Nucleic Acid kit (Qiagen, Hilden, Germany), according to the manufacturer’s instructions. For two patients (Pt#1 and Pt#2), cfDNA samples at the time of cystectomy and one month later were missed due to technical failures.
2.4 Droplet digital PCR
Tumor mutations shared between primary tumors and their metastatic sites were detected in cfDNA samples by droplet digital PCR (ddPCR) using the QX200 Droplet Digital PCR system (Bio-Rad, Watford, UK), following the manufacturer’s instructions. A mutation is considered that has a potential aggressive behavior when is shared between primary tumors and metastatic sites. For each patient, only mutations identified in their tissue (primary tumor and/or metastasis) were subsequently analyzed in their plasma cfDNA (Supplementary Table S3).
Assays to detect and quantify the fractional abundance of point mutations and corresponding wild-type alleles were custom-designed using Primer3 Input (version 4.1.0) (Supplementary Table S4). The optimal annealing temperature and limit of detection (LOD) were determined for each assay (Supplementary Methods). The mutant allele fraction (MAF) was calculated as the number of droplets positive with the mutant amplicon divided by the total droplets positive with the amplicon (wild-type and mutant). The presence of a mutation was considered detectable if MAF > LOD. Data analysis was carried out using QuantaSoft Analysis Pro Software, version 1.0 (Bio-Rad).
3 Results
3.1 Clinicopathological features of patients
The six patients enrolled in this study underwent radical cystectomy and lymphadenectomy, five due to MIBC and one (Pt#5) due to a T1 extensive tumor (TURBT). Two patients presented positive lymph nodes (LN+) at the time of cystectomy (Pt#1 and Pt#2). Clinical variables and treatments received during patient follow-up are summarized in Figure 2. IHC analysis determined that tumors from Pt#2, Pt#3, Pt#4, and Pt#6 presented basal differentiation, the tumor from Pt#5 had luminal differentiation, and the tumor from Pt#1 presented both luminal and basal subtypes. All six patients developed locoregional (Pt#1 and Pt#2) or distant metastases (Pt#3, Pt#4, Pt#5, and Pt#6). The median time to progression was seven months (range 1-22 months). One patient (Pt#3) received adjuvant chemotherapy, and all six patients received treatment upon tumor progression. Five of the six (83%) patients died due to BC; the median survival time was 16 months (range 5-93 months).
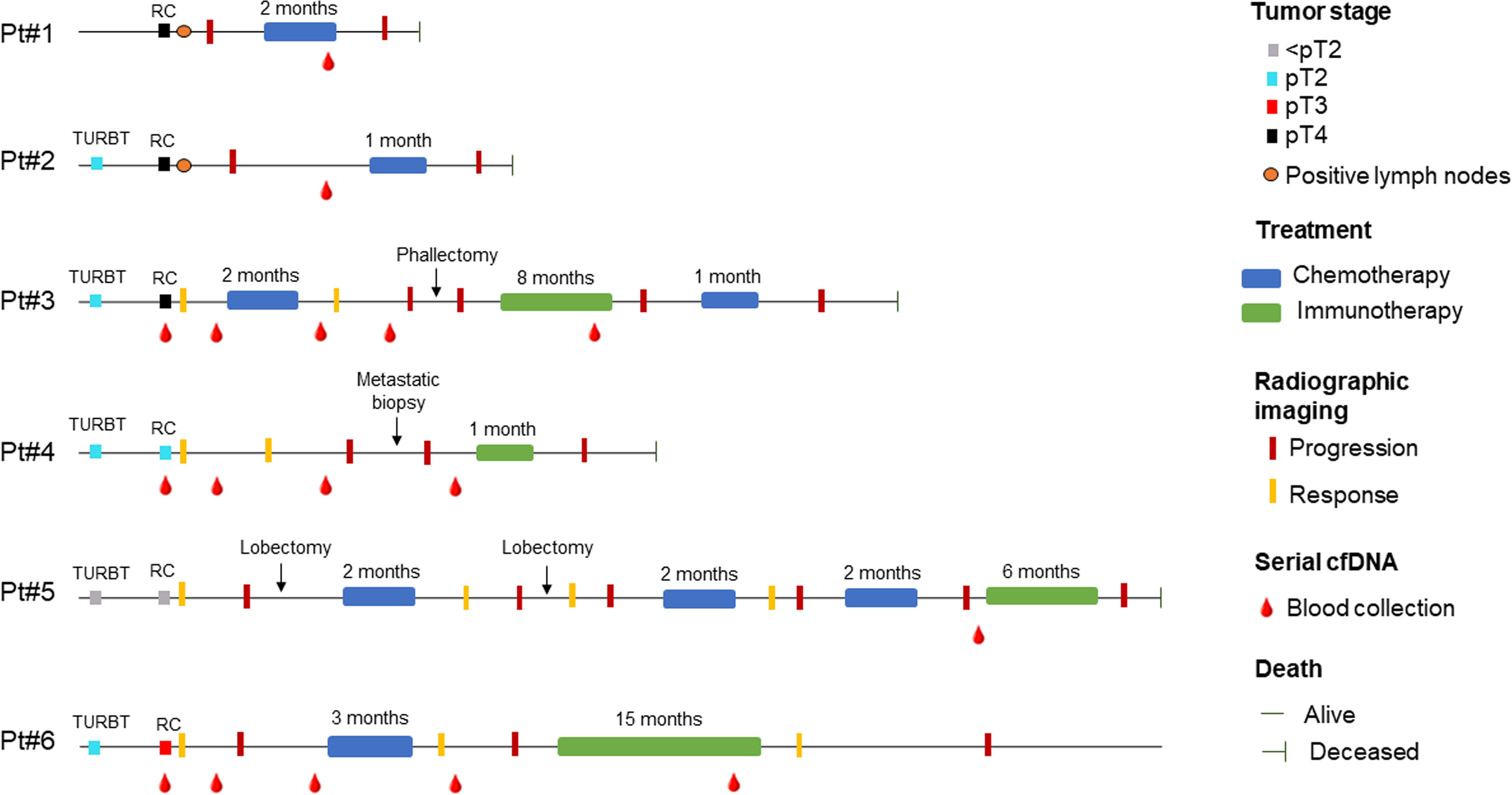
Figure 2 Clinicopathological variables and treatments received for the six patients included in the study at different follow-up time points. Clinical controls, treatments, and blood draws are indicated in chronological order; however, the temporal proportion between these events is not proportional along the chronological line. RC, radical cystectomy; TURBT, transurethral resection of bladder tumor.
3.2 Mutations in primary tumor and metastatic samples
The spatial molecular heterogeneity of BC was determined by examining several different morphologically tumor regions from the primary tumor and metastatic sites in each patient (Figure 1; Supplementary Figure S1). Each region harbored private as well as shared genetic alterations with the other different morphologically tumor regions (Supplementary Table S5), showing high BC intra- and inter-lesion heterogeneity but also intra- and inter-lesion shared genetic features. Figure 3 depicts evolutionary trees displaying the evolution of somatic mutations in each patient. As shown, metastatic sites harbored additional mutations to the primary tumors, indicating an accumulation of somatic mutations over time (Supplementary Table S5).
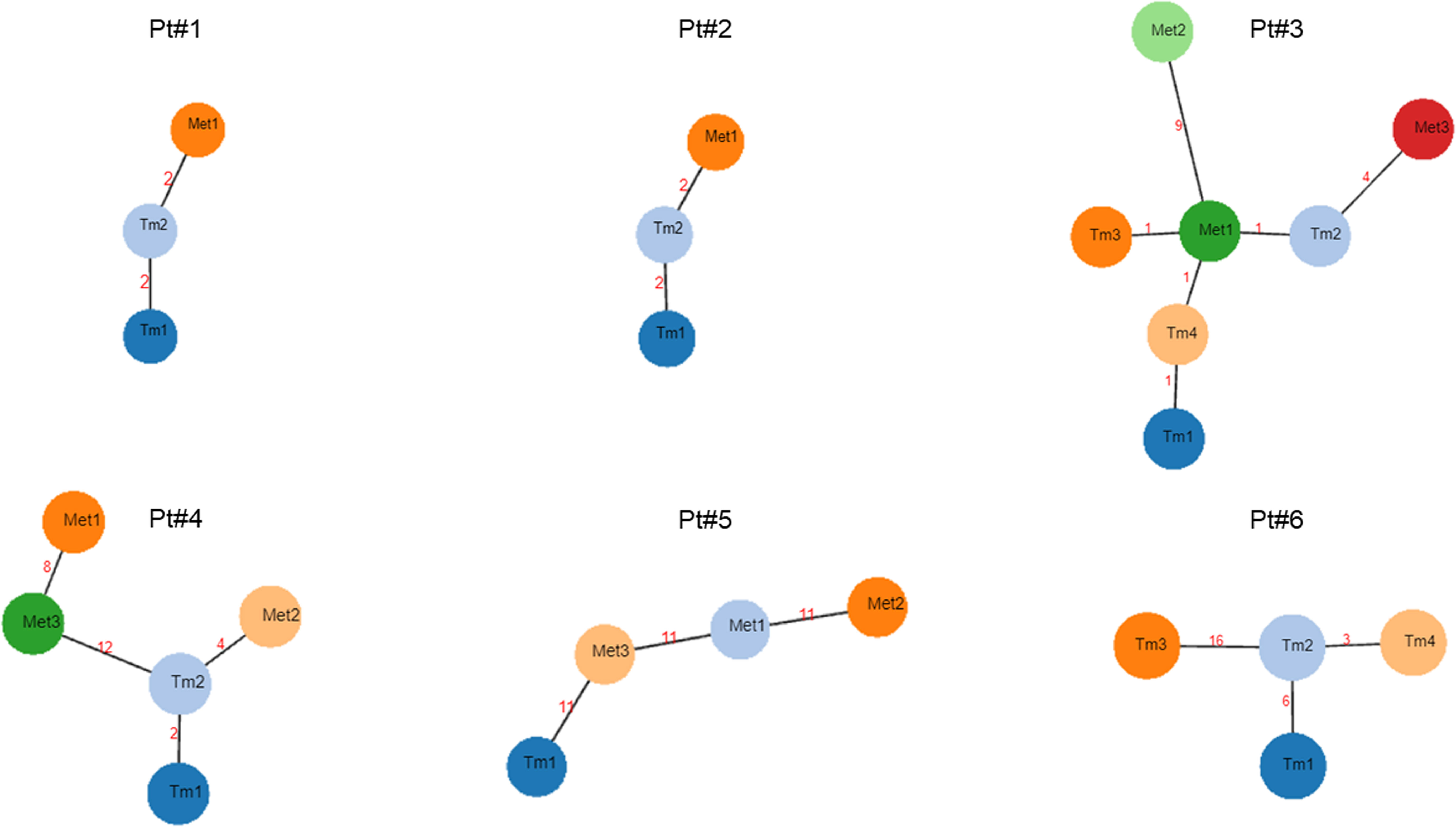
Figure 3 Phylogenetic trees showing tumor evolution for each metastatic bladder cancer patient. Each node represents a single primary tumor specimen or metastatic biopsy. The numbers between two nodes correspond to genetic changes between them. Met, metastatic biopsy; Pt, Patient; Tm, primary tumor biopsy.
Overall, a total of 6, 5, 9, 4, 21, and 42 mutations (mean of 15 mutations) were identified in the primary tumor samples from each of the six patients, respectively. Interestingly, primary tumors with basal differentiation (Pt#2, Pt#3, Pt#4, and Pt#6) harbored mutations in the key basal genes RB1 and/or TP53. The tumor from Pt#5 had luminal differentiation and harbored FGFR3 mutations. However, Pt#1, who presented a tumor with both molecular subtypes, did not harbor mutations in FGFR3, RB1, or TP53; instead, they harbored KRAS mutations (Supplementary Table S5).
Globally, a total of 7, 4, 25, 43, and 61 mutations (mean of 29 mutations) were identified in the metastatic sites of the first five patients, respectively. Of note, patients with distant metastases (Pt#3, Pt#4, and Pt#5) harbored a higher number of mutations than patients with locoregional metastases (Pt#1 and Pt#2) (mean of 43 vs. 6 mutations).
The most frequently mutated genes in primary tumors were ATM (12%), ARID1A (9%), NF1 (8%), and KDM6A (8%); in metastases, the genes were ATM (20%), ARID1A (16%), BRCA2 (13%), NF1 (11%), and TP53 (11%) (Figure 4). Four mutations were identified in common between primary tumors and metastatic sites in the six BC patients (Table 1). Overall, the c.1-124C>T hotspot mutation in the TERT promoter was found in 60% (9/15) of primary tumor specimens and 55% (6/11) of metastatic sites. The RB1 c.13delA mutation was found in 33% (5/15) of primary tumor regions and 36% (4/11) of metastatic regions. The ATM c.1236-2A>T mutation was also identified in 33% (5/15) of primary tumor samples but in only 9% (1/11) of metastatic samples. Finally, the FGFR3 c.742C>T mutation was found in only 7% of primary tumor specimens and 27% of metastatic sites. Notably, these specific mutations were not found in all tumor regions from the same patient, confirming the spatial molecular heterogeneity of BC (Table 1).
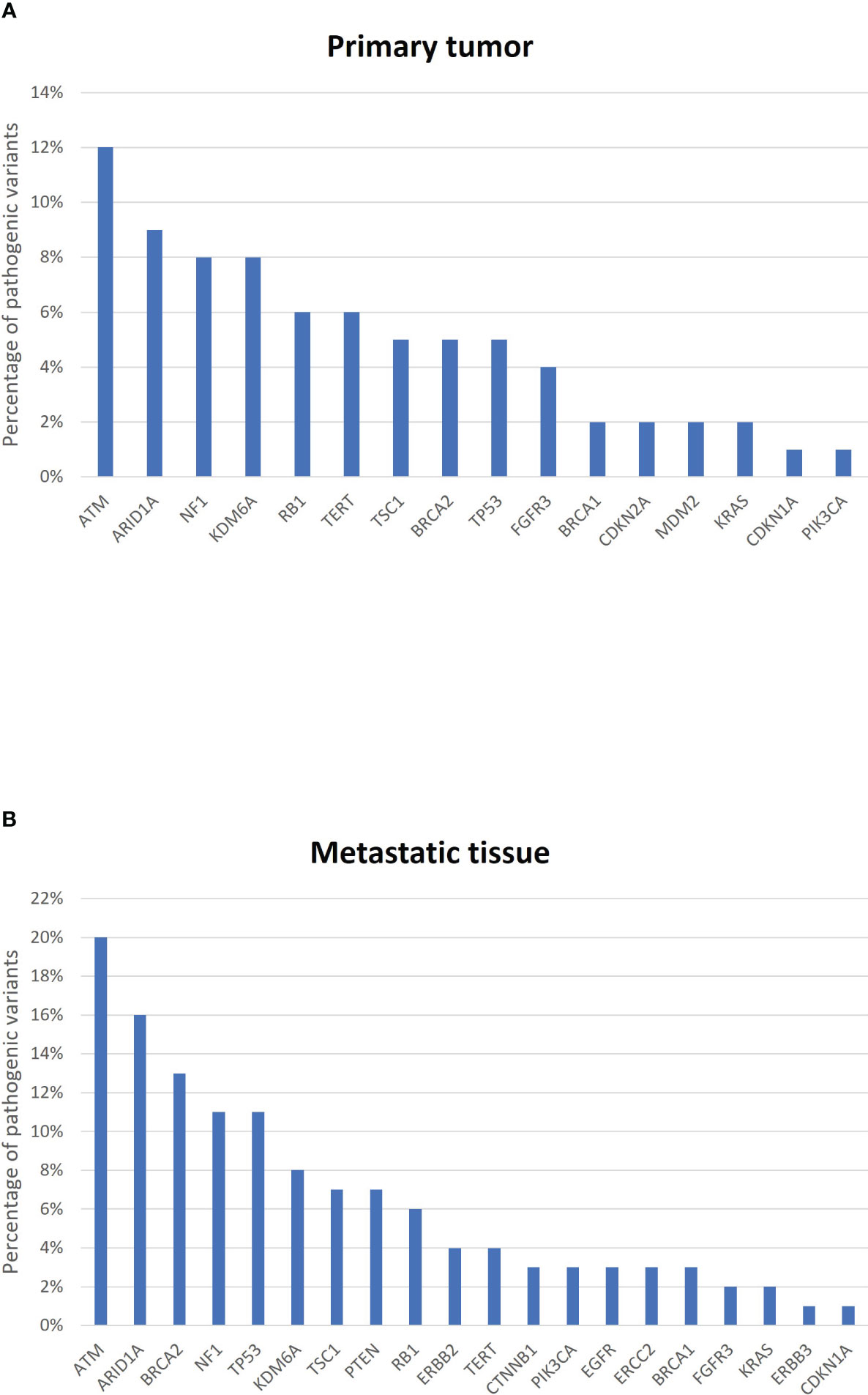
Figure 4 Most frequently mutated genes in (A) primary tumors and (B) metastatic sites from the six bladder cancer patients. The graph represents the percentage of pathogenic variants per gene.
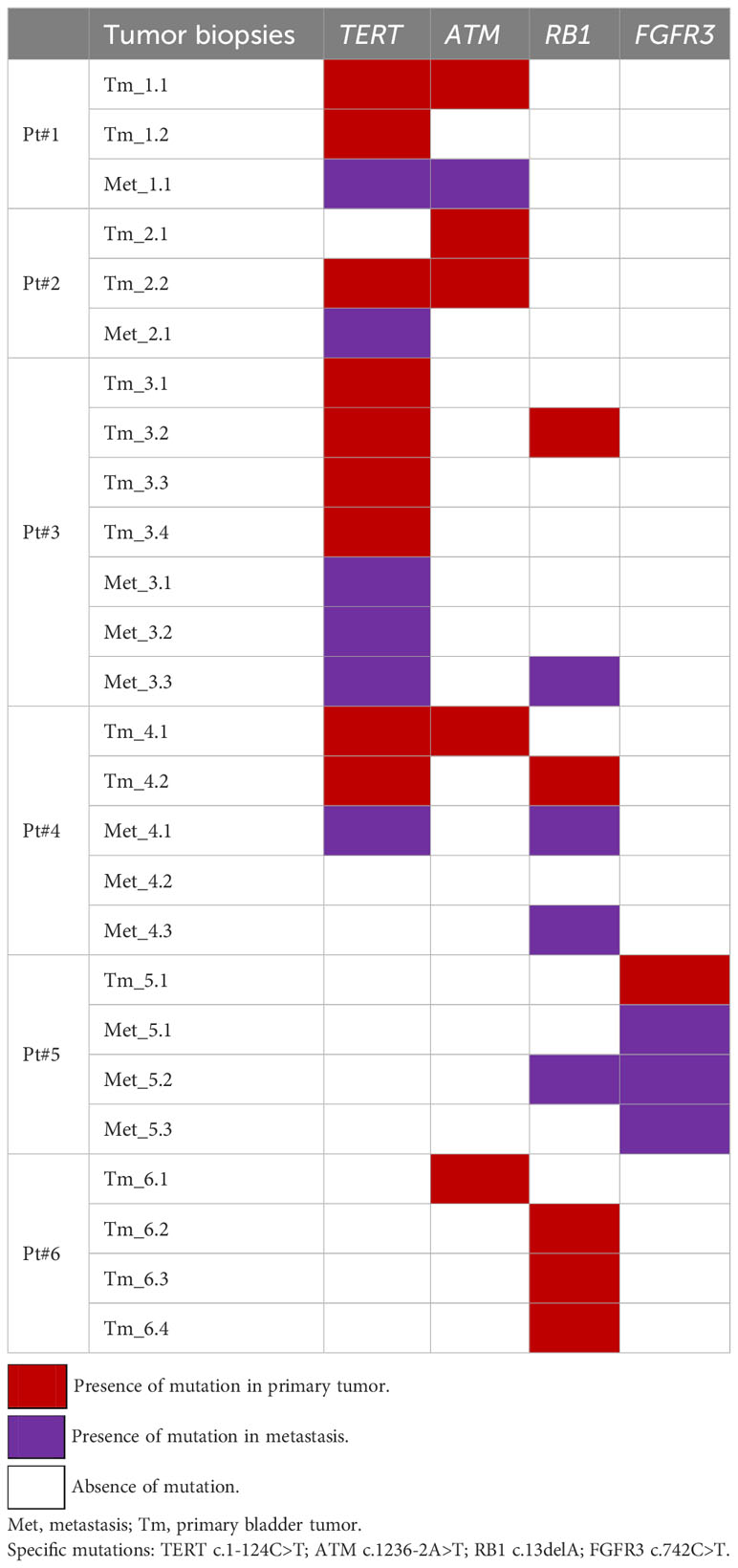
Table 1 Distribution of the most frequent gene mutations according to different regions analyzed from the primary tumor and metastasis in each bladder cancer patient.
3.3 Mutation analysis in cfDNA and correlation with tumor tissue
ddPCR was used to determine the four mutations (TERT c.1-124C>T, RB1 c.13delA, ATM c.1236-2A>T, and FGFR3 c.742C>T) shared between the primary tumors and their respective metastases in plasma cfDNA samples. Table 2 depicts mutations identified in tumor tissue (primary and metastasis) and cfDNA at different follow-up time points in the six BC patients. As shown, mutations in metastatic tissue and cfDNA samples during patient follow-up had a higher concordance than mutations in primary tumors and cfDNA samples at the time of cystectomy (89% vs. 29%). Furthermore, mutation evolution is evidenced by their dynamic changes during patient follow-up. For instance, Pt#3 was treated with three cycles of adjuvant gemcitabine-cisplatin chemotherapy after RC. Both TERT and RB1 mutations were present in their cfDNA at the time of RC, but the RB1 mutation was not detected after chemotherapy (4 and 12 months after RC). In Pt#6, the RB1 mutation was identified prior to four cycles of salvage gemcitabine-cisplatin chemotherapy (four months after RC), but was absent after treatment (12 months after RC) (Figure 2; Table 2).
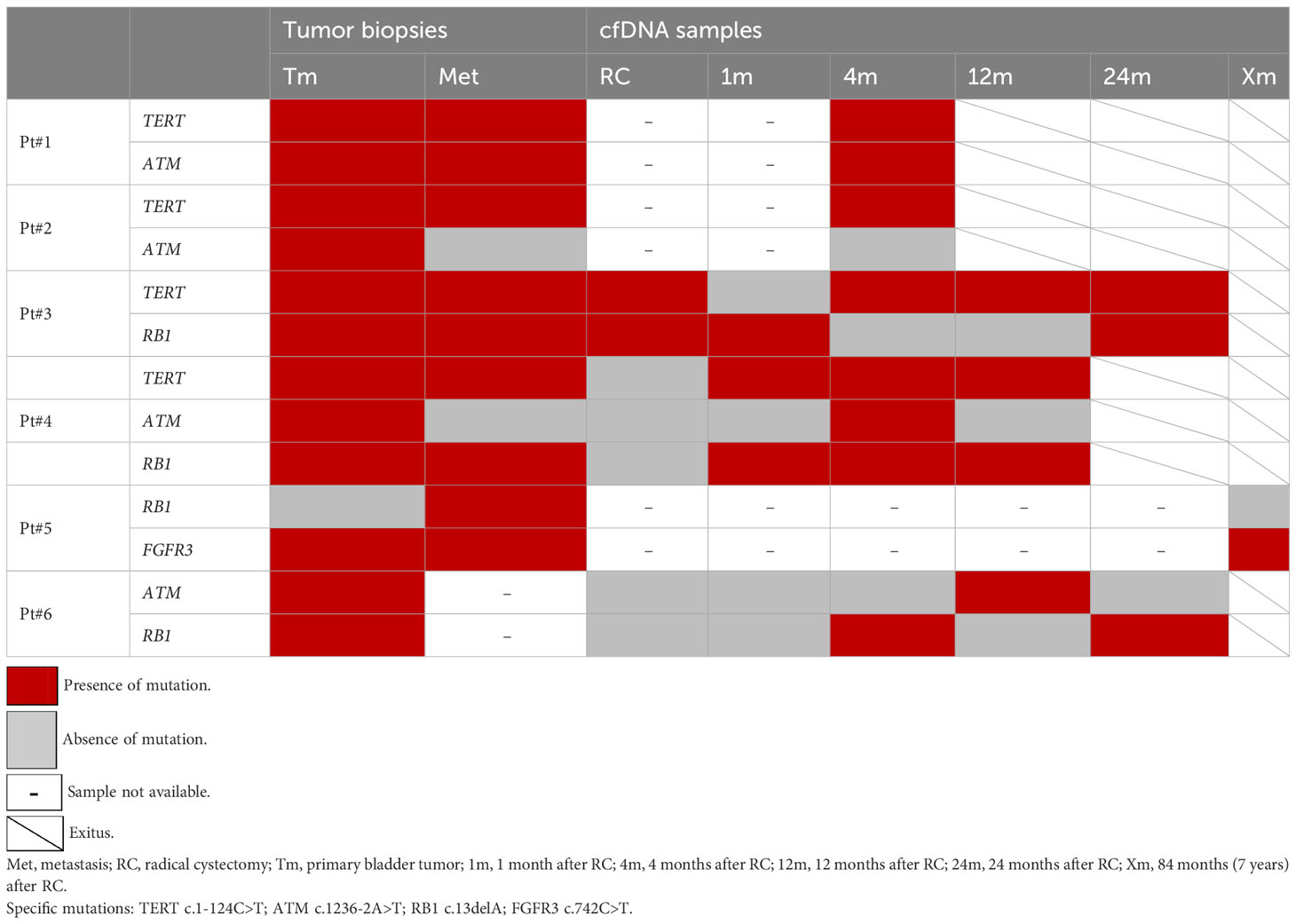
Table 2 Detection of specific gene mutations in tumor biopsies and cell-free DNA (cfDNA) samples during patient follow-up.
4 Discussion
Bladder cancer is one of the tumors with the highest genetic heterogeneity (4), presenting a challenge to identify a set of recurrent cfDNA biomarkers for use in the clinical setting to monitor tumor evolution in response to treatment (12).
Here, we have addressed the challenge of BC heterogeneity by analyzing genetic features of several different morphologically tumor regions from primary bladder tumor and locoregional/distant metastases from six BC patients. Furthermore, we have identified genetic alterations shared between primary bladder tumors and their metastases in different BC patients and we have been able to determine them in plasma cfDNA from these patients during their follow-up. The assessment of these BC genetic alterations providing aggressive behavior in plasma cfDNA would allow real-time monitoring of tumor evolution in a real clinical scenario.
Since some of the MIBC molecular subtypes have been associated with bladder tumor aggressivity, we first classified bladder tumors included in this study according to basal or luminal differentiation. As expected, the four MIBC tumors from our cohort that presented basal differentiation harbored mutations in the TP53 and/or RB1 genes. This subtype has been associated with aggressive tumors and a poor prognosis (13, 14) and indeed, these patients did have a poor prognosis, presenting a two-year mortality rate of 75%. The tumor from Pt#5 with luminal differentiation harbored an FGFR3 mutation, as expected for this molecular subtype. This is the only non-muscle-invasive BC patient in our series and the one with the longest progression-free survival (seven years). In fact, BC patients with luminal tumors have been found to have the best prognosis compared with all other molecular subtypes (13, 14). Finally, BC tumors with both basal and luminal subtypes have been suggested to present aggressive behavior since they are considered to have a similar prognosis to basal subtype (13, 14) and actually Pt#1 showed a short cancer-specific survival. This tumor did not harbor mutations in the TP53, RB1, or FGFR3 genes. However, we found genomic alterations in KRAS, promoting the activation of the RAS pathway in this tumor, which has been suggested to be associated with luminal differentiation (14, 15).
Germane to the mutations present in the distinct tumor regions of primary bladder tumors as well as locoregional/distant metastases, we were able to confirm previously reported ITH of bladder tumors, as well as lesion-to-lesion genetic heterogeneity (12, 16, 17). In fact, phylogenetic trees showing branched tumor evolution in each patient demonstrated the existence of more aggressive clones in primary tumors that would originate the metastasis. Additionally, we found that distant metastases were genetically more altered than locoregional ones, and both metastatic regions were genetically more altered than primary tumors, most probably due to the emergence of resistance mutations during disease progression (18). Interestingly, although both high genetic intra- and inter-lesion heterogeneity were identified, we were able to detect several mutations in common between primary tumors and metastatic lesions. All six patients harbored at least one of these four mutations in primary tumors and metastatic lesions: TERT c.1-124C>T, ATM c.1236-2A>T, RB1 c.13delA, and FGFR3 c.742C>T. These inter-lesion-shared mutations were analyzed in cfDNA samples during patient follow-up for real-time monitoring of disease relapse. Remarkably, a higher DNA mutation concordance was found between metastatic lesions and cfDNA samples during follow-up than between the primary tumor and cfDNA at the time of cystectomy. Multiple factors can account for this DNA mutation discordance between tumor and cfDNA at surgery, such as preanalytical methodological factors (amount of plasma analyzed, assay sensitivity, etc.) (10) or low cfDNA levels that could prevent variant detection (false negative). In fact, other authors previously reported better concordance between cfDNA and tissue samples when high cfDNA levels were present (19).
Substantial evidence suggests that the detection of circulating tumor DNA (ctDNA; the variable fraction of plasma cfDNA derived from tumor) in the bloodstream following potential curative treatment is associated with a high risk of tumor relapse (7, 11, 20–22). However, the identification of specific mutations in cfDNA can also be an advantage for applying targeted therapy. For instance, those patients with advanced or metastatic BC harboring FGFR3 mutations in their primary tumor are already being treated with the Food and Drug Administration (FDA)-approved drug Erdafitinib® (NCT05316155), a fibroblast growth factor receptor (FGFR) kinase inhibitor (23). Interestingly, an FGFR3 missense mutation (c.742C>T; p.Arg248Cys), a gain-of-function mutation that promotes cell proliferation and migration (24, 25), was found in Pt#5’s cfDNA samples, suggesting that liquid biopsy could be a useful tool to identify potential therapeutic targets during patient follow-up. In fact, this specific FGFR3 mutation was also identified in cfDNA samples from a series of BC patients who were candidates for Erdafitinib (12), emphasizing that the detection of the FGFR3 mutation in plasma cfDNA could be helpful to guide targeted treatment in a non-invasive way. FGFR3 alterations occur mainly in non-muscle-invasive BC (25), and it was mutated in the only patient of our series (Pt#5) with non-invasive disease at the time of RC. However, this patient did not present an RB1 mutation in their primary bladder tumor; however, they did in their metastatic tissue. This is most likely due to RB1 mutations being commonly found in higher BC stages and associated with poor patient outcomes (26).
As regards somatic mutations in DNA repair genes, such as ATM and RB1, these have been associated with better responses to cisplatin-based chemotherapy in MIBC (27–29). Interestingly, three patients from our cohort (Pt#3, Pt#4, and Pt#5) harbored an RB1 mutation both in primary tumors/metastatic sites and plasma cfDNA. This mutation was undetectable in cfDNA from Pt#3 and Pt#5 after treatment with gemcitabine-cisplatin chemotherapy. The remaining patient (Pt#4) did not receive cisplatin-based chemotherapy, and the RB1 mutation was present in their cfDNA during the entire follow-up. RB1 is a tumor suppressor gene that has been associated with DNA repair as well as cell proliferation and division (27). Several studies have demonstrated that mutations in RB1 are frequently found in bladder cancer (26, 27), although, as far as we know, the specific mutation (c.13delA; loss-of-function mutation) found in our cohort had not been described before. On the other hand, ATM is a core component of the DNA repair system, and its signaling pathway is known to play a role in the development of breast cancer and other tumors (30). Several mutations in ATM have been previously described in BC (26, 31), however, interestingly, the specific ATM mutation found in our cohort (c.1236-2A>T) was first described in our previous study analyzing bladder tumors (32). Finally, the TERT promoter mutation (c.1-124C>T, gain-of-function mutation) has been widely reported in several solid tumors, including bladder cancer (33–36), where it plays an important role in tumorigenesis. In a previous study (22), which included both progressive and non-progressive BC patients, we showed that none of the non-progressive patients harboring ATM and TERT mutations in their primary tumors, present these mutations in their cfDNA during their follow-up after RC. Furthermore, in another previous study, we described that the presence of the mutation TERT c.1-124C>T could be considered a biomarker of aggressivity in MIBC (32). This data suggests that the presence of mutation in ATM and TERT in plasma cfDNA after treatment is related to tumor dissemination and confers a poor prognosis to patients.
To the best of our knowledge, this is the first work to evaluate in plasma cfDNA a set of genetic alterations associated with aggressive behavior in bladder cancer, initially identified in primary and metastatic sites from BC patients. Nonetheless, we must acknowledge some study limitations. First, some tumors might not have shed ctDNA into the plasma at the time of cystectomy, even if the tumor had a targetable mutation, with the consequent risk of a false-negative result. In addition, this study includes only six patients. However, it must be considered the difficulty of obtaining metastatic biopsies from these patients. In fact, our cohort of metastatic patients is one of the largest in the literature considering the type of samples analyzed from each patient (different morphologically tumor regions from primary and metastatic sites). Nonetheless, more patients need be investigated to determine the final role of these four genes as biomarkers for monitoring tumor evolution and assessment of treatment response in advanced, metastatic BC patients.
5 Conclusion
A higher number of mutations was identified in distant metastases compared with locoregional metastases. Moreover, mutations in metastatic tissue had a high concordance with mutations in cfDNA samples. Despite BC genetic intra- and inter-lesion heterogeneity, specific mutations in TERT, ATM, RB1, and FGFR3 were found to be shared between primary and metastatic lesions in different BC patients. These shared mutations have been identified in cfDNA samples during patient follow-up, allowing real-time monitoring of these patients. Consequently, the analysis of BC mutations associated with poor prognosis in plasma cfDNA could be a valuable tool to monitor tumor evolution in response to treatments, thus improving the clinical management of BC patients.
Data availability statement
The datasets presented in this study can be found in online repositories. The names of the repository/repositories and accession number(s) can be found in the article/Supplementary Material.
Ethics statement
The studies involving humans were approved by Clinical Research Ethics Committee of the Hospital Clinic of Barcelona (HCB/2018/0026). The studies were conducted in accordance with the local legislation and institutional requirements. The participants provided their written informed consent to participate in this study.
Author contributions
RC: Investigation, Methodology, Writing – original draft. MI-T: Resources, Writing – review & editing. JO: Resources, Writing – review & editing. FR: Writing – review & editing. LR-C: Methodology, Writing – review & editing. SH: Writing – review & editing. BM: Data curation, Writing – review & editing. AA: Writing – review & editing. LI: Writing – review & editing. LM: Funding acquisition, Investigation, Supervision, Writing – review & editing.
Funding
The author(s) declare financial support was received for the research, authorship, and/or publication of this article. This work was supported by the Instituto de Salud Carlos III (ISCIII) through the Plan Estatal de Investigación Científica y Técnica y de Innovación 2018-2020 and 2021-2023 (project reference numbers PI17/01343 and PI20/00409) and co-funded by the European Regional Development Fund (FEDER).
Acknowledgments
We thank all the patients who participated in this study and all the staff and nurses of the Urology Department of the Hospital Clinic for collaborating with sample collection. We are indebted to the IDIBAPS biobank for tissue sample procurement. We thank Dr. Ramón Trullas for helping us with the ddPCR experiments. We thank Helena Kruyer for the English correction of the manuscript. Funding from the CERCA Programme/Generalitat de Catalunya is gratefully acknowledged. This work was developed at the Centre de Recerca Biomèdica Cellex, Barcelona.
Conflict of interest
The authors declare that the research was conducted in the absence of any commercial or financial relationships that could be construed as a potential conflict of interest.
Publisher’s note
All claims expressed in this article are solely those of the authors and do not necessarily represent those of their affiliated organizations, or those of the publisher, the editors and the reviewers. Any product that may be evaluated in this article, or claim that may be made by its manufacturer, is not guaranteed or endorsed by the publisher.
Supplementary material
The Supplementary Material for this article can be found online at: https://www.frontiersin.org/articles/10.3389/fonc.2023.1270962/full#supplementary-material
References
1. Witjes JA, Bruins HM, Cathomas R, Compérat E, Cowan NC, Efstathiou JA, et al. European association of urology guidelines on muscle invasive and metastatic bladder cancer: summary of the 2020 guidelines. Eur Urol (2021) 79:82–104. doi: 10.1016/j.eururo.2020.03.055
2. Bladder Cancer: Statistics. Cancer.Net. Available at: https://www.cancer.net/cancer-types/bladder-cancer/statistics (Accessed January 31, 2023).
3. von der Maase H. Gemcitabine in transitional cell carcinoma of the urothelium. Expert Rev Anticancer Ther (2003) 3:11–9. doi: 10.1586/14737140.3.1.11
4. Knowles MA, Hurst CD. Molecular biology of bladder cancer: New insights into pathogenesis and clinical diversity. Nat Rev Cancer (2015) 15:25–41. doi: 10.1038/nrc3817
5. McGranahan N, Swanton C. Clonal heterogeneity and tumor evolution: past, present, and the future. Cell (2017) 168:613–28. doi: 10.1016/J.CELL.2017.01.018
6. Mannelli C. Tissue vs liquid biopsies for cancer detection: ethical issues. J Bioeth Inq (2019) 16:551–7. doi: 10.1007/S11673-019-09944-Y/METRICS
7. Vandeputte C, Kehagias P, El Housni H, Ameye L, Laes JF, Desmedt C, et al. Circulating tumor DNA in early response assessment and monitoring of advanced colorectal cancer treated with a multi-kinase inhibitor. Oncotarget (2018) 9:17756–69. doi: 10.18632/ONCOTARGET.24879
8. Syeda MM, Wiggins JM, Corless BC, Long GV, Flaherty KT, SChadendorf D, et al. Circulating tumour DNA in patients with advanced melanoma treated with dabrafenib or dabrafenib plus trametinib: a clinical validation study. Lancet Oncol (2021) 22:370–80. doi: 10.1016/S1470-2045(20)30726-9
9. Birkenkamp-Demtröder K, Christensen E, Nordentoft I, Knudsen M, Taber A, Høyer S, et al. Monitoring treatment response and metastatic relapse in advanced bladder cancer by liquid biopsy analysis. Eur Urol (2018) 73:535–40. doi: 10.1016/J.EURURO.2017.09.011
10. Pascual J, Attard G, Bidard FC, Curigliano G, De Mattos-Arruda L, Diehn M, et al. ESMO recommendations on the use of circulating tumour DNA assays for patients with cancer: a report from the ESMO Precision Medicine Working Group. Ann Oncol (2022) 33:750–68. doi: 10.1016/J.ANNONC.2022.05.520
11. Christensen E, Birkenkamp-Demtröder K, Sethi H, Shchegrova S, Salari R, Nordentoft I, et al. Early detection of metastatic relapse and monitoring of therapeutic efficacy by ultra-deep sequencing of plasma cell-free DNA in patients with urothelial bladder carcinoma. J Clin Oncol (2019) 37:1547–57. doi: 10.1200/JCO.18.02052
12. Clinton TN, Chen Z, Wise H, Lenis AT, Chavan S, Donoghue MTA, et al. Genomic heterogeneity as a barrier to precision oncology in urothelial cancer. Cell Rep (2022) 41:11859. doi: 10.1016/j.celrep.2022.111859
13. Kamoun A, de Reyniès A, Allory Y, Sjödahl G, Robertson AG, Seiler R, et al. A consensus molecular classification of muscle-invasive bladder cancer. Eur Urol (2020) 77:420–33. doi: 10.1016/J.EURURO.2019.09.006
14. Robertson AG, Kim J, Al-Ahmadie H, Bellmunt J, Guo G, Cherniack AD, et al. Comprehensive molecular characterization of muscle invasive bladder cancer. Cell (2017) 171:540. doi: 10.1016/J.CELL.2017.09.007
15. Inamura K. Bladder cancer: new insights into its molecular pathology. Cancers (Basel) (2018) 10:100. doi: 10.3390/CANCERS10040100
16. Thomsen MBH, Nordentoft I, Lamy P, Høyer S, Vang S, Hedegaard J, et al. Spatial and temporal clonal evolution during development of metastatic urothelial carcinoma. Mol Oncol (2016) 10:1450–60. doi: 10.1016/j.molonc.2016.08.003
17. Thomsen MBH, Nordentoft I, Lamy P, Vang S, Reinert L, Mapendano CK, et al. Comprehensive multiregional analysis of molecular heterogeneity in bladder cancer. Sci Rep (2017) 7:11702. doi: 10.1038/s41598-017-11291-0
18. Priestley P, Baber J, Lolkema MP, Steeghs N, de Bruijn E, Shale C, et al. Pan-cancer whole-genome analyses of metastatic solid tumours. Nature (2019) 575:210–6. doi: 10.1038/S41586-019-1689-Y
19. Yang N, Li Y, Liu Z, Qin H, Du D, Cao X, et al. The characteristics of ctDNA reveal the high complexity in matching the corresponding tumor tissues. BMC Cancer (2018) 18:319. doi: 10.1186/s12885-018-4199-7
20. Powles T, Assaf ZJ, Davarpanah N, Banchereau R, Szabados BE, Yuen KC, et al. ctDNA guiding adjuvant immunotherapy in urothelial carcinoma. Nature (2021) 595:432–7. doi: 10.1038/S41586-021-03642-9
21. Tie J, Wang Y, Tomasetti C, Li L, Springer S, Kinde I, et al. Circulating tumor DNA analysis detects minimal residual disease and predicts recurrence in patients with stage II colon cancer. Sci Transl Med (2016) 8:346ra92. doi: 10.1126/SCITRANSLMED.AAF6219
22. Carrasco R, Ingelmo-Torres M, Gómez A, Trullas R, Roldán FL, Ajami T, et al. Cell-free DNA as a prognostic biomarker for monitoring muscle-invasive bladder cancer. Int J Mol Sci (2022) 23:11732. doi: 10.3390/ijms231911732
23. Roubal K, Myint ZW, Kolesar JM. Erdafitinib: A novel therapy for FGFR-mutated urothelial cancer. Am J Health Syst Pharm (2020) 77:346–51. doi: 10.1093/AJHP/ZXZ329
24. Dodurga Y, Tataroglu C, Kesen Z, Satiroglu-Tufan NL. Incidence of fibroblast growth factor receptor 3 gene (FGFR3) A248C, S249C, G372C, and T375C mutations in bladder cancer. Genet Mol Res (2011) 10:86–95. doi: 10.4238/VOL10-1GMR923
25. Iyer G, Milowsky MI. Fibroblast growth factor receptor-3 in urothelial tumorigenesis. Urol Oncol (2013) 31:303–11. doi: 10.1016/J.UROLONC.2011.12.001
26. Yin M, Grivas P, Emamekhoo H, Mendiratta P, Ali S, Hsu J, et al. ATM/RB1 mutations predict shorter overall survival in urothelial cancer. Oncotarget (2018) 9:16891–8. doi: 10.18632/oncotarget.24738
27. Zhang D, Tian J, Xia Q, Yang Z, Gu B. Significance and mechanisms analyses of RB1 mutation in bladder cancer disease progression and drug selection by bioinformatics analysis. Bladder Cancer (2021) 7:133–42. doi: 10.3233/BLC-200368
28. Plimack ER, Dunbrack RL, Brennan TA, Andrake MD, Zhou Y, Serebriiskii IG, et al. Defects in DNA repair genes predict response to neoadjuvant cisplatin-based chemotherapy in muscle-invasive bladder cancer. Eur Urol (2015) 68:959. doi: 10.1016/J.EURURO.2015.07.009
29. Miron B, Hoffman-Censits JH, Anari F, O’Neill J, Geynisman DM, Zibelman MR, et al. Defects in DNA repair genes confer improved long-term survival after cisplatin-based neoadjuvant chemotherapy for muscle invasive bladder cancer. Eur Urol Oncol (2020) 3:544. doi: 10.1016/J.EUO.2020.02.003
30. Jin MH, Oh DY. ATM in DNA repair in cancer. Pharmacol Ther (2019) 203:107391. doi: 10.1016/J.PHARMTHERA.2019.07.002
31. Pan YH, Zhang JX, Chen X, Liu F, Cao JZ, Chen Y, et al. Predictive value of the TP53/PIK3CA/ATM mutation classifier for patients with bladder cancer responding to immune checkpoint inhibitor therapy. Front Immunol (2021) 12:643282. doi: 10.3389/fimmu.2021.643282
32. Carrasco R, Ingelmo-Torres M, Gómez A, Roldán FL, Segura N, Ribal MJ, et al. Prognostic implication of TERT promoter mutation and circulating tumor cells in muscle-invasive bladder cancer. World J Urol (2022) 40:2033–9. doi: 10.1007/S00345-022-04061-9
33. Barthel FP, Wei W, Tang M, Martinez-Ledesma E, Hu X, Amin SB, et al. Systematic analysis of telomere length and somatic alterations in 31 cancer types. Nat Genet (2017) 49:349. doi: 10.1038/NG.3781
34. Borah S, Xi L, Zaug AJ, Powell NM, Dancik GM, Cohen SB, et al. TERT promoter mutations and telomerase reactivation in urothelial cancer. Sci (1979) (2015) 347:1006–10. doi: 10.1126/science.1260200
35. Günes C, Wezel F, Southgate J, Bolenz C. Implications of TERT promoter mutations and telomerase activity in urothelial carcinogenesis. Nat Rev Urol (2018) 15:386–93. doi: 10.1038/S41585-018-0001-5
Keywords: bladder cancer, cell-free DNA, metastasis, mutation, tumor heterogeneity
Citation: Carrasco R, Ingelmo-Torres M, Oriola J, Roldán FL, Rodríguez-Carunchio L, Herranz S, Mellado B, Alcaraz A, Izquierdo L and Mengual L (2023) Assessment of aggressive bladder cancer mutations in plasma cell-free DNA. Front. Oncol. 13:1270962. doi: 10.3389/fonc.2023.1270962
Received: 01 August 2023; Accepted: 13 November 2023;
Published: 30 November 2023.
Edited by:
Hua Tan, National Human Genome Research Institute (NIH), United StatesReviewed by:
Georgi Guruli, Virginia Commonwealth University, United StatesYibo Li, Southern Methodist University, United States
Copyright © 2023 Carrasco, Ingelmo-Torres, Oriola, Roldán, Rodríguez-Carunchio, Herranz, Mellado, Alcaraz, Izquierdo and Mengual. This is an open-access article distributed under the terms of the Creative Commons Attribution License (CC BY). The use, distribution or reproduction in other forums is permitted, provided the original author(s) and the copyright owner(s) are credited and that the original publication in this journal is cited, in accordance with accepted academic practice. No use, distribution or reproduction is permitted which does not comply with these terms.
*Correspondence: Lourdes Mengual, bG1lbmd1YWxAdWIuZWR1
†These authors share senior authorship