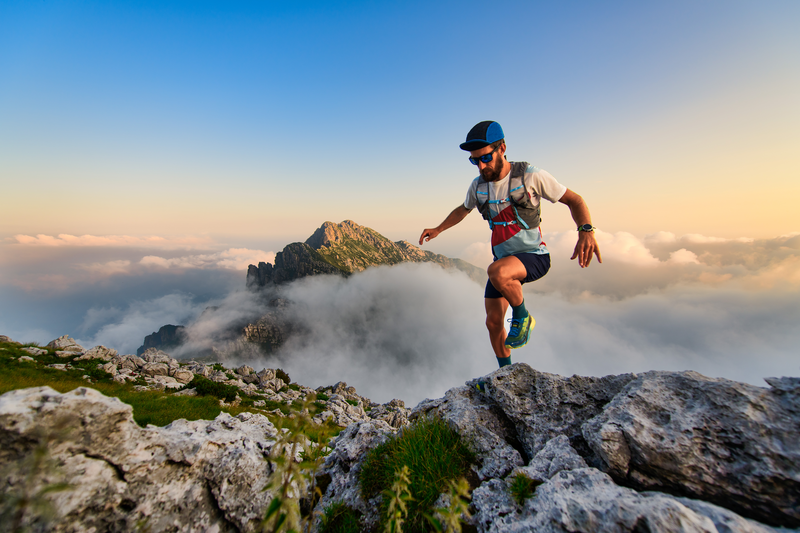
95% of researchers rate our articles as excellent or good
Learn more about the work of our research integrity team to safeguard the quality of each article we publish.
Find out more
REVIEW article
Front. Oncol. , 06 September 2023
Sec. Cancer Molecular Targets and Therapeutics
Volume 13 - 2023 | https://doi.org/10.3389/fonc.2023.1227657
Due to predictions of increasing incidences and deaths from ovarian cancer, this neoplasm is a challenge for modern health care. The advent of NGS technology has made it possible to understand the molecular characteristics of many cancers, including ovarian cancer. The data obtained in research became the basis for the development of molecularly targeted therapies thus leading to the entry of NGS analysis into the diagnostic process of oncological patients. This review presents targeted therapies currently in preclinical or clinical trials, whose promising results offer hope for their use in clinical practice in the future. As more therapeutic options emerge, it will be necessary to modify molecular diagnostic regimens to select the best treatment for a given patient. New biomarkers are needed to predict the success of planned therapy. An important aspect of public health is molecular testing in women with a familial predisposition to ovarian cancer enabling patients to be included in prevention programs. NGS technology, despite its high throughput, poses many challenges, from the quality of the diagnostic material used for testing to the interpretation of results and classification of sequence variants. The article highlights the role of molecular testing in ongoing research and also its role in the diagnostic and therapeutic process in the era of personalized medicine. The spread of genetic testing in high-risk groups, the introduction of more targeted therapies and also the possibility of agnostic therapies could significantly improve the health situation for many women worldwide.
Ovarian cancer (OC) is diagnosed in a large number of women around the world annually - in 2020, 313,959 new cases were reported - significantly reducing their comfort level and also taking the lives of many of them (207,252 deaths) (1, 2). OC ranks seventh in cancer incidence and is fifth leading cause of cancer-related death in women worldwide (1), in addition is considered to be the most lethal gynecological cancer (3). Statistical studies have shown a general downward trend in the incidence and mortality of ovarian cancer over the past decade linked, no doubt, to advances in medicine. However, the significant growth in incidence in younger women is worrisome (2).
It is known that the increased risk of developing ovarian cancer is related to genetic predisposition and family history (Hereditary Ovarian Cancer - HOC). Hereditary cancers, i.e. those caused by inherited mutations in genes that predispose to cancer development, account for as many as 20-25% of epithelial ovarian cancer cases (4). It is worth mentioning that breast and ovarian cancers show some common features at the molecular level (5). The distinct disease syndrome is Hereditary Breast and Ovarian Cancer (HBOC) in which the risk of breast and ovarian cancer is higher than in the general population (6). Breast cancer cases due to HBOC are estimated to account for 2 to 7%; for ovarian cancer, the range is 15 to 18% (4).
Whether spontaneously occurring or associated with a genetic predisposition, OC represents a major global problem and a challenge of modern medicine. Undoubtedly, the development of next-generation sequencing (NGS) technology has contributed to a better knowledge and understanding of cancer biology and, moreover, in the detection of high-risk groups (7). This is crucial in the early diagnosis of cancer and also in the selection of targeted therapy (8). NGS panels approved for use in patient diagnosis allow assessment of both somatic and germline variants, as well as single or global genomic changes (7). Such broad capabilities of NGS technology make it widely used in scientific research and patient diagnostics, including oncology, and an important step towards personalized medicine. However, many factors influence the success and improvement of NGS research, such as sequencing chemistry, sequencing technology, bioinformatics and data analysis (9).
High-grade serous ovarian cancer (HGSOC) is the most common histological type of ovarian cancer and accounts for about 75% of ovarian cancers of epithelial origin (10). Despite the common histological features identified by pathologists, HGSOC tumors have significant differences in the clinical course of the disease, including response to treatment (11). HGSOCs exhibit significant heterogeneity due to differences at the molecular level (12).
The TP53 somatic mutation occurs as the first molecular alteration involved in HGSOC carcinogenesis and is referred to as a driver mutation. This condition was identified in 96% of HGSOC cases (3). The protein encoded by TP53, called p53, is a transcription factor that activates genes engaged in DNA repair, cell cycle and apoptosis after nonreversible DNA damage (13). Loss of p53 function promotes tumorigenesis, but does not itself cause a malignant phenotype, and at least one more genotoxic event is required. Most frequently, this is a BRCA1/2 mutation. BRCA1/2 can lose its functionality through somatic mutation, germline mutation or through promoter hypermethylation, which belongs to epigenetic modifications. It is worth noting that mutations are mutually exclusive with epigenetic silencing of the BRCA1/2 gene (3). BRCA1 and BRCA2 proteins are involved in DNA repair through homologous recombination (homologous recombination repair (HRR)) (14). Defects in HRR are estimated to occur in 50% of HGSOC cases. Besides changes in BRCA1/2, it can be caused by mutations in ATM, BRIP1, CHEK2, NBN, PALB2, RAD51B (3, 14). However, an HGSOC tumor can acquire genomic instability through disruption in another pathway. BRCA1/2 loss of function is mutually exclusive with CCNE1 amplification and RB1 inactivation. Both CCNE1 and RB1 are involved in the Rb cell cycle regulation pathway (3). The protein cyclin E1 forms a complex with cyclin-dependent kinase 2 (CDK2) to promote cell cycle progression from G1 to S phase (15). Cyclin E1 expression relies on E2F transcription factors that are bound to retinoblastoma protein (Rb) (16). Amplification of CCNE1 and deletion of RB1 hasten the cell cycle, leading to defective S-phase progression and an elevated number of chromosome breaks, resulting in genomic instability. CCNE1 amplification appears in about 20% of HGSOC cases while RB1 inactivation occurs in 10% of HGSOC (3).
Currently, the standard of care for patients with stage II-IV ovarian cancer is platinum-based chemotherapy and surgical cytoreduction (16). Ovarian tumors with HRD are characterized by higher sensitivity to platinum and poly(ADP-ribose) polymerase inhibitor (PARPi) therapies. When RB1 function is lost, the tumor is also sensitive to platinum based therapies. In contrast, ovarian cancer with CCNE1 overexpression shows greater resistance to platinum compound therapies (17). Identifying the pathogenesis of the tumor at the molecular level allows the choice of targeted therapy.
For the treatment of breast and ovarian cancer, the Food and Drug Administration (FDA) has approved several PARPi. These drugs are effective for cancer patients with mutated BRCA1/2 and for treating recurrent platinum-sensitive ovarian cancer (18, 19). A meta-analysis by Staropoli et al. of 1,839 epithelial ovarian cancer patients showed a statistically significant benefit from PARPi. A prolongation of survival time was confirmed, with no significant differences in progression-free survival (PFS) for the individual pharmaceuticals in this group (20). PARPi acts through synthetic lethality with loss of BRCA1/2 functionality. This occurs due to the destabilization of replication forks by PARPi, homologous recombination does not function properly in these cells and consequently DNA double-strand breaks (DSBs) occur, which cause cell death (21, 22). Initially, PARPi was used only in patients with an identified BRCA1/2 mutation. However, the treatment is known to be effective for homologous recombination deficiency (HRD), which can be also caused by mutations in other genes. This approach has allowed a significant expansion of the group of patients in whom targeted therapy can be used (23). The use of PARPi in clinical practice has resulted in improved PFS. Despite its high efficacy, not all patients with tumors with mutations in BRCA1/2 or HRD respond to treatment, and moreover, most patients eventually develop resistance (24).
Strategy that appears to be very promising in clinical practice is combination therapy involving PARP inhibitors and antiangiogenic drugs. The combination of these medications has been shown to result in a significant prolongation of PFS in both women with newly diagnosed advanced OC and with recurrent disease (25). Combinations with PI3K/AKT (phosphoinositide 3-kinase/protein kinase B), EGFR (epidermal growth factor receptor) and BET (bromodomain and extraterminal domain) inhibitors are proposed as other therapies that can overcome PARPi resistance (24). Noteworthy are the findings of Gasimla et al. The researchers analyzed the molecular profiles of BRCA2-deficient HGSOC tumors resistant to PARPi. They concluded that in these cases KRAS amplification is responsible for the resistance. The researchers suggest that inhibition of PLK1 (Polo-Like Kinase 1), which plays a role in regulating cell division, restores PARPi sensitivity (19). Immunogenicity plays an important role in the response to treatment. Kraya et al. showed differences in the immunogenicity of ovarian tumors with mutations in BRCA1/2 and distinguished two groups: tumors with PTEN loss and BRCA1 promoter hypermethylation, and tumors with wild-type PTEN. Cancers in the first group were characterized by significantly reduced CD3+, CD8+, and FOXP3+ T cell composition and higher HRD scores. The researchers suggest that combination therapy involving PARPi and immune checkpoint blockade (ICB) may be effective in patients with BRCA1/2 deficiency and wild-type PTEN (26). Shein et al. suggest that assessing the expression of immune-related genes may be an effective biomarker for estimating OS in women with ovarian cancer (27). The incorporation of epigenetic regulation into therapy was explored by Pulliam et al. Researchers have shown that inhibition of DNMT1 (an enzyme involved in DNA repair) sensitizes breast and ovarian cancers resistant to PARP inhibitor therapy. Moreover, DNMTi and PARPi therapy is effective regardless of BRCA status (18). Gupta et al. reported synergistic effects of histone deacetylase inhibitors (HDACi) and PARPi in vitro in ovarian cancer cells with an efficient homologous recombination mechanism. HDACi induces defective DNA repair, leading to an increase in the number of DSBs and, by repressing HR, enhances the action of PARPi. The researchers indicate that this therapy could have significant effects in poor-prognosis tumors with CCNE1 amplification (28). The challenge is to develop a scheme to classify patients for monotherapy or combination therapy. Necessary for this is the search for biomarkers, especially at the molecular level (25, 26).
Poly(ADP-ribose) glycohydrolase (PARG) inhibitors, similarly to PARPi, destabilize replication forks and induce DNA damage (21). However, PARG inhibitors show activity in BRCA wild-type cells, making them an effective alternative for patients with primary or acquired PARPi resistance (29). Successful completion of clinical trials on the efficacy of PARG inhibitors and a deeper understanding of their mechanism of action, as well as finding markers to predict susceptibility to therapy, is essential for the development of a new targeted drug effective in many types of cancer, not limited to ovarian cancer (21, 29).
CCNE1 amplification is common in many types of cancer. Tumors with this molecular alteration are characterized by relative resistance to chemotherapy and, in addition, van Wagensveld et al. showed a lower content of immune cells which may result in a worse response of patients to immunotherapy (30). Therefore, finding targeted therapies for this genetic change has become a challenge for many researchers. Along with CCNE1 amplification, TP53 mutation is often present. In this group, significantly longer overall survival (OS) was observed in patients who received antiangiogenic therapy (31). One other strategy in cancers with CCNE1 amplification is CDK2 inhibition. The resulting CDK2-cyclin-E1 complex plays a role in chromosomal instability. Therefore, CDK2 inhibitors could inhibit tumorigenesis. CCNE1 amplification could be considered a biomarker of susceptibility to CDK2 inhibition. However, the oncogenic effect of cyclin E1 independent of CDK2 should be considered (32). Gallo et al. conducted studies in cell models and showed that CCNE1 overexpression results in susceptibility to PKMYT1 kinase inhibition. PKMYT1 kinase is a negative regulator of CDK1 and the selective inhibitor developed by the researchers appears to be an effective therapeutic strategy. The drug is intended for oral use, and clinical trials are currently ongoing for use as monotherapy or in combination with gemcitabine (33). High levels of cyclin E1 cause upregulation of the ATR axis, which induces the ATR/Chk1/WEE1 phosphorylation cascade (34). Xu et al. conducted studies on cell lines and xenografts with CCNE1 overexpression. The researchers showed that this genetic alteration causes sensitivity to WEE1 inhibition and ataxia telangiectasia-associated Rad3 (ATR) inhibition (WEE1i-ATRi). Preclinical studies on a number of such drugs are currently underway and four ATRi and one WEE1i are in phase I - II clinical trials for efficacy in cancer therapy (35, 36). Xu et al. suggest that by combining the two inhibitors, it will be possible to use lower doses of the drug, thereby reducing toxicity. However, the authors emphasize that treatment with WEE1i-ATRi, despite good tolerance in mice, requires in vivo studies before use in clinical practice (15).
In addition to the most common amplification found in HGSOC which is CCNE1 amplification, somatic copy number alterations (SCNAs) also affect MYC, PIK3CA, KRAS and TERT and may also become targets for personalized therapy. Martins et al. show that MYC copy number correlates with the in vitro response to mTORC1/2 inhibition. Moreover, the researchers showed that activation of the mTOR survival pathway in ovarian tumors with MYC amplification is associated with a higher frequency of SCNAs in PI3K pathway genes. The coexistence of these two SCNAs also occurs in squamous cell lung cancer and triple-negative breast cancer (37). Meanwhile, Martins et al. demonstrated the efficacy of combination therapy involving mTOR pathway inhibition and paclitaxel in HGSOC tumors with MYC amplification (38). Transcription of the MYC oncogene depends on CDK7, CDK12, and CDK13, among others. Zeng et al. propose as a therapeutic strategy the use of THZ-1, which inhibits the aforementioned cyclin-dependent kinases which leads to a decrease in MYC expression levels and thus inhibition of tumor growth (39).
RB1 mutations are found in several cancer types including OC, which are further characterized by resistance to available therapies. Gong et al. tested 36 cell cycle inhibitors, of which the Aurora kinase inhibitors AURKA and AURKB showed the strongest synthetic lethality against small cell lung cancer (SCLC) cell lines. AURKA showed significantly higher selectivity compared to AURKB and was also well tolerated by mice (40). While Oser et al. demonstrated the efficacy of Aurora kinase B on SCLC cell lines with RB1 loss of function, they also point to good tolerance in experimental animals and suggest the possibility of using it in other types of cancer with this genetic alteration (41).
Figure 1 and Table 1 summarize the treatment options for women with HGSOC that may be possible in the near future.
Figure 1 Targeted treatment options for HGSOC approved by the FDA and under study described in the text.
Most cases of HOC and HBOC are determined by germline mutations in BRCA1 or BRCA2 (6, 42). It is estimated that about 1 in 400-800 individuals carry the germline pathogenic variant of the BRCA1 and/or BRCA2 genes (43). Patients are placed under medical supervision if diagnosed as carrying a BRCA mutation. Chemoprevention or risk-reducing surgical treatment: risk‐reducing mastectomy and risk‐reduction salpingo‐oophorectomy may be used to reduce the risk of developing breast and ovarian cancer (44).
As for familial or hereditary ovarian cancer syndromes, they are caused by inherited mutations in tumor suppressor genes. In addition to BRCA1 and BRCA2 genes belonging to the Fanconi anemia pathway, mutations in PALB2, ATM, RAD51C/D and BRIP1 are also known to increase the risk of ovarian cancer. Another important pathway is the mismatched DNA repair pathway and genes such as MLH1, MSH2 and MSH6 (42).
Beyond the increased risk of developing breast and ovarian cancer, individuals in the HBOC group are at increased risk of developing other cancers, such as melanoma, pancreatic cancer and, in the case of men, prostate cancer (6). The risk of developing a specific cancer is related to the type of mutation. Women who have inherited the pathogenic BRCA1 variant tend to suffer from ovarian cancer at a younger age. In contrast, patients with a mutation in BRCA2 have a greater predisposition to develop male breast cancer, prostate cancer, pancreatic cancer and melanoma (45). Other HBOC-related genes include ATM, BRIP1, CDH1, PALB2, PTEN, RAD51C, and TP53 (46). Velázquez et al. used a panel of 35 genes to study hereditary cancers. In the case of HBOC, pathogenic variants were noted in the following genes: BLM, BRCA2, BRIP1, CHEK2. The researchers underline that by using the expanded panel, they were able to detect pathogenic variants that would not have been detected with routinely used diagnostic panels (47). Resch et al. also demonstrated the benefits of using wider gene panels in their study. Most of the pathogenic variants identified were BRCA1, BRCA2. However, they also detected variants in CHEK2 and RAD51C and also in ATM, BARD1, MUTYCH and SMARCA4 which would not have been possible with a standard panel (48).
It should also be taken into account that the etiology of HBOC may be related to constitutional mosaic variants. These variants can involve somatic and also germinal cells, and can therefore be passed onto offspring. In their work, Hidalgo Mayoral et al. described a clinical case of a breast cancer patient in whom they detected a mosaic variant c.9648 + 1G>A in the BRCA2 gene. The authors point to other cases reported in the literature and emphasize the role of BRCA1/2 mosaic variants in HBOC. Due to the low allele frequency (VAF), many of these may have been missed in genetic analyses. A separate diagnostic algorithm is needed to detect potential mosaic variants using NGS sequencing (49).
Despite the significant genomic similarities between ovarian and breast tumors and the existence of the HBOC disease entity, these tumors show some distinguishing features. Weber-Lassalle et al. subjected samples of HBOC patients to genetic analysis. The researchers identified germline variants in BARD1 in breast cancer patients. Moreover, the presence of these variants was associated with an earlier onset of cancer (42.3 years) compared to the overall study group (48.6 years). BARD1 variants were not detected in ovarian cancer (50).
It is extremely important that testing is not limited to BRCA1/2. The benefit of expanding molecular analyses was demonstrated by Tao et al. 25% of HGSOC patients had genetic alterations other than disorders leading to BRCA loss of function. This gives patients a chance to receive off-label treatment or other therapies described in this review as part of their participation in clinical trials (51).
Currently available tests to assess HRD include: analysis of genes associated with homologous recombination repair, genomic “scars” and/or mutational signatures, and real-time functional assessment of HRD. It should also be remembered that the HRD phenotype is very complex and thus extremely difficult to assess reliably and unequivocally in a single analysis. These validated tests can be used to make treatment decisions for PARPi. However, they are not effective in predicting the group of patients who will not benefit from PARPi, i.e. they have no negative predictive value (23, 52).
Considering the mutually exclusive nature of the occurrence of BRCA mutations and CNNE1 amplification, the detection of high-level amplification in combination with CCNE1 overexpression can be used as a criterion for predicting PARPi resistance (53). However, there are reports that genetic changes primarily thought to be mutually exclusive can co-occur. This is particularly true for loss of RB function, which appears to co-occur in tumors with BRCA1/2 mutations or other disorders of homologous recombination pathway genes (54). Perhaps understanding this aspect will become possible through the use of spatial genomic, transcriptomic and proteomic technology. Spatial biology offers the possibility to analyze different cellular subclones and also complex interactions with the tumor microenvironment (TME), which together determine the process of carcinogenesis in an individual (55).
It should be noted that CCNE1 overexpression can occur without copy number amplification. Clinical trials have demonstrated the efficacy of WEE1i in both patients with CCNE1 amplification and overexpression. Therefore, future studies will probably evaluate the possibility of using CCNE1 expression instead of amplification of this gene as a marker of susceptibility to WEE1i therapy (56).
It is also worth mentioning that the use of PARPi prolonged PFS, but not OS. Therefore, it is important to consider the use of another therapeutic option. Unfortunately, immunotherapies in ovarian cancer have been less successful than in non-small cell lung cancer and melanoma. In order to improve the efficacy of this therapy, patient selection based on immunological profiling including assessment of high-grade microsatellite instability (MSIH), mismatch repair deficiencies (dMMR) and HRD is proposed (57).
In order for a patient to qualify for genetic testing, a woman must meet criteria outlined in national guidelines. These include age, hormone receptor status in BC, OC histology, and history of cancers that may be related to HBOC in the family. Although, these guidelines in different countries cover the same aspects however, the cutoff points may vary. There is a need to refine consistent recommendations between countries for monitoring mutation carriers specifically in genes other than BRCA1/2 (58).
The decision to use a multigene panel is up to the clinician. The physician, after analyzing the patient’s phenotype and taking a health history, can choose the most appropriate molecular diagnostic option to identify HBOC, or to exclude it with higher probability. In addition to commercially available panel tests with a wide range, some laboratories also offer custom-designed panels after listening to the clinician’s suggestions (45).
Despite improvements in diagnosis through the use of multigene panels, the issue of variants of uncertain significance (VUS) remains crucial. There is a need for algorithms as well as improved data flow and monitoring the situation globally to identify variants on which further research should be conducted to determine their clinical significance (59). These issues are discussed in more detail in the next section of the article.
Another important aspect is that HBOC tests should be tailored to different ethnic groups, as each has characteristic variants that occur with increased frequency compared to other groups. This approach can contribute to a better understanding of the molecular characteristics of specific communities of people and lead to the development of genetic tests dedicated to a specific community which will markedly improve the sensitivity and specificity of the test (60).
With the advent of sequencing technologies, we have seen their continued evolution. Sanger sequencing, referred to as first-generation sequencing, is limited to regions of interest within the genes of interest. The development of next-generation sequencing technologies has enabled comprehensive sequencing of larger regions of the genome. In the case of gene panels, gene fragments whose genetic variation is responsible for the occurrence of a defined phenotype are sequenced. It is also possible to sequence targeted protein-coding regions of all genes - whole exome sequencing (WES) and even whole genome sequencing (WGS), i.e. coding and non-coding sequences. NGS allows for more extensive analyses and is consequently more efficient due to the time and cost savings compared to Sanger sequencing (61).
Using advances in technology such as NGS, it is possible to make treatment decisions (62). Currently, biopsy material archived in the form of formalin-fixed paraffin-embedded (FFPE) blocks is used for molecular diagnosis of solid tumors (63). In this material, due to the fixation process, there may be changes in nucleic acids, their degradation and also modification of nitrogenous bases, which will be misread as a sequence variant in NGS analysis. To prevent these errors, researchers are developing new and more efficient protocols for nucleic acid isolation and library preparation (64). Another aspect of crucial importance in the fight against ovarian cancer worldwide is early detection and also monitor disease progression based on molecular testing. Many studies are looking into the possibility of using liquid biopsy, which has the undoubted advantage of being non-invasive (62). Analysis of cell-free tumor DNA (ctDNA) circulating in the blood, which can be performed using the NGS technique, has many potential applications such as early detection of both primary disease and recurrence, predicting prognosis, characterization of tumor heterogeneity and monitoring of treatment over time (7, 65). However, the researchers point out that protocols for analyzing material obtained by liquid biopsy need to be refined, ctDNA enrichment methods need to be improved and the sensitivity of analytical techniques for detecting at low allele frequencies needs to be optimized. Further research is needed before liquid biopsy can be used in clinical practice (66).
The discovery of mutually exclusive pathways and also their interactions has become the basis for the development of new targeted therapies and, in the future, diagnostic regimens that qualify patients for treatment (67). Also in the case of approved drugs - PARPi, whose action is based on the concept of synthetic lethality, incoming data from studies show that it is essential to know the genetic context of the pathogenic change (68). For better insight and understanding of the clinical course of the disease, it is necessary to take into account not only individual genomic changes but their complex interrelationships, which together make up the characteristics of a given patient and thus determine the success of the planned therapeutic strategy (69). Consequently, there is a need to develop new biomarkers, the combined use of which will enable a better understanding of the patient’s clinical condition and allow the selection of the optimal treatment pathway (68).
NGS sequencing has provided a wealth of data regarding the molecular biology of cancers, including HGSOC. However, it is now known that the use of bioinformatics analysis and tools is essential. It is through these that the pathways of carcinogenesis can be analyzed in depth (67). To detect homologous recombination deficiency, research uses methods based on whole-exome or whole-genome sequencing. However, in the practice of molecular diagnostic oncology, targeted sequencing panels are most often used. Therefore, the number of identifiable mutations is too low for HRD signature analysis, and as a result, many cases with HRD are not identified and patients do not receive PARPi treatment. Gulhan et al. propose a computational tool called Signature Multivariate Analysis (SigMA), which enables detection of mutation signatures from panel sequencing data. The researchers’ impressive results indicate that the use of SigMA could significantly increase the number of patients who could be considered for targeted therapy (70). Schouten et al. developed a classifier that detects HRD-associated mutations based on evaluation of sequence variants in a panel of genes, epigenetic changes, and copy number alteration. The classifier proved to be an effective tool identifying most cancers with BRCA loss-of-function and BRCA-like phenotype, as well as all analyzed tumors with the RAD51C germline mutation (71). In contrast, Li et al. created a signature predictive of initial platinum resistance (IPR) not dependent on HRD but based on the differential expression of 11 genes. The researchers also identified significant differences in the characteristics of tumor infiltrating lymphocytes. The results suggest that patients with a high-IPR signature should show a better response to ICB (72).
In order for knowledge of the molecular profiles of tumors to be realistically reflected in clinical practice, that is, for treatment to be applied to a patient, it is important to keep in mind the significant heterogeneity of OC tumors, both temporally and spatially. For this reason, it seems reasonable that sequencing should also be performed after treatment and in relapse (73). This involves more research and thus cost generation. In silico data can be used to validate NGS assays instead of physical samples. In addition to high throughput and reliability, the use of in silico data makes it possible not to generate incremental costs associated with sequencing additional samples. These data can be successfully used to simulate hard-to-find gene variants, low-frequency variants or specific classes of variants (e.g., medium-sized insertions) (74).
The clinical course of the disease varies depending on the gene in which the pathogenic variant is present, whether the variant is germline, somatic, or an epigenetic modification, and the location of the change, i.e. which codon is affected (75). Therefore, correct and clear reporting and classify of detected changes is crucial. Somatic sequence variants are classified based on their clinical significance: tier I - variants of strong clinical significance; tier II - variants of potential clinical significance; tier III - variants of unknown clinical significance (VUS); and tier IV - variants of known non-significant significance (considered benign or probably benign). According to current guidelines, variants from tiers I-III must be included on the NGS analysis report, while it is not recommended to include lesions classified as tier IV. Tiers I and II have therapeutic, prognostic and diagnostic significance. VUS variants are the most challenging, and it is essential to report them which will enable researchers to decide later on their reclassification (76). Whereas germline variants are classified in a five-tier system: class 1 – non-pathogenic; class 2 - likely not pathogenic; class 3 – uncertain; class 4 - likely pathogenic; and class 5 - definitely pathogenic (77). Due to the distinctiveness of the scale used in the classification of somatic and germline sequential variants, it is helpful to include a verbal category name that indicates the pathogenicity of the variant to avoid potential confusion and misunderstanding.
Also extremely useful in the classification of variants are informatics tools that allow for the efficient flow of incoming data from NGS analyses. Hirotsu et al. demonstrated the utility of using MH BRCA and MH Guide developed by Molecular Health in determining the significance of VUS. The authors show significant value in confirming or exclusion of HBOC and also in predicting susceptibility to PARPi application when a variant of unknown clinical significance is detected (78).
Modern medicine has a great knowledge of the molecular-level changes involved in carcinogenesis, resulting in breakthroughs in patient diagnosis and treatment. Approaching cancer as if it were a disease determined by genetic changes and using NGS technology can significantly improve the prognosis of women with HGSOC. The use and utility of NGS testing in OC is summarized in Figure 2. However, despite its high efficiency, performance, NGS poses many challenges. Bioinformatics tools and the use of algorithms to obtain comprehensive and reliable molecular characterization of a patient’s sample are helpful. It is crucial to properly interpret the test results and relate them to the patient’s clinical condition. There is also a need to search for new therapeutic targets and conduct clinical trials of further targeted therapies. Before introducing new drugs into clinical practice, it is necessary to evaluate their efficacy, selectivity against tumor cells and also possible side effects. Clinical trials on the efficacy of new molecularly targeted drugs are being conducted around the world and Table 2 collects examples of such trials. A great opportunity may be the possibility of tumor-agnostic therapy, i.e. the use of targeted drugs regardless of the type of cancer just on the basis of finding the presence of a specific genetic change. Biomarkers should be sought to predict the response to the planned therapy. A very important aspect is the identification of women with a hereditary predisposition to ovarian cancer. As the range of therapeutic options grows, it is necessary to develop a laboratory diagnostic pathway that allows the best classification of patients for specific therapies. Pursuing these goals will contribute to more efficient diagnosis and treatment from an economic perspective and, invaluably, to improving the quality and prolonging the lives of patients. This can only be achieved through continuous improvement of skills and cooperation between clinicians and laboratory diagnosticians.
Figure 2 Possibilities of using NGS technology in the diagnostic and therapeutic process of ovarian cancer.
PB: Conceptualization, Data collection, Writing. JK: Supervision. JN: Supervision. All authors contributed to the article and approved the submitted version.
The authors declare that the research was conducted in the absence of any commercial or financial relationships that could be construed as a potential conflict of interest.
All claims expressed in this article are solely those of the authors and do not necessarily represent those of their affiliated organizations, or those of the publisher, the editors and the reviewers. Any product that may be evaluated in this article, or claim that may be made by its manufacturer, is not guaranteed or endorsed by the publisher.
1. Khanlarkhani N, Azizi E, Amidi F, Khodarahmian M, Salehi E, Pazhohan A, et al. Metabolic risk factors of ovarian cancer: a review. JBRA Assisted Reprod (2022) 26(2):335–47. doi: 10.5935/1518-0557.20210067
2. Huang J, Chan WC, Ngai CH, Lok V, Zhang L, Lucero-Prisno DE III, et al. Worldwide burden, risk factors, and temporal trends of ovarian cancer: A global study. Cancers (2022) 14:2230. doi: 10.3390/cancers14092230
3. Otsuka I. Mechanisms of high-grade serous carcinogenesis in the fallopian tube and ovary: current hypotheses, etiologic factors, and molecular alterations. Int J Mol Sci (2021) 22:4409. doi: 10.3390/ijms22094409
4. Bellcross CA. Hereditary breast and ovarian cancer. An updated primer for OB/GYNs. Obstet Gynecol Clin N Am (2022) 49:117–47. doi: 10.1016/j.ogc.2021.11.005
5. Serio PAdMP, de Lima Pereira GF, Katayama MLH, Roela RA, Maistro S, Folgueira MAAK. Somatic mutational profile of high-grade serous ovarian carcinoma and triple-negative breast carcinoma in young and elderly patients: similarities and divergences. Cells (2021) 10:3585. doi: 10.3390/cells10123586
6. Yoshida R. Hereditary breast and ovarian cancer (HBOC): review of its molecular characteristics, screening, treatment, and prognosis. Breast Cancer (2021) 28:1167–80. doi: 10.1007/s12282-020-01148-2
7. Morganti S, Tarantino P, Ferraro E, D’Amico P, Viale G, Trapani D, et al. Complexity of genome sequencing and reporting: Next generation sequencing (NGS) technologies and implementation of precision medicine in real life. Crit Rev Oncol/Hematol (2019) 133:171–82. doi: 10.1016/j.critrevonc.2018.11.008
8. Hussen BM, Abdullah ST, Salihi A, Sabir DK, Sidiq KR, Rasul MF, et al. The emerging roles of NGS in clinical oncology and personalized medicine. Pathol - Res Pract (2022) 230:153760. doi: 10.1016/j.prp.2022.153760
9. Zhong Y, Xu F, Wu J, Schubert J, Li MM. Application of next generation sequencing in laboratory medicine. Ann Lab Med (2021) 41:25–43. doi: 10.3343/alm.2021.41.1.25
10. Lheureux S, Gourley C, Vergote I, Oza AM. Epithelial ovarian cancer. Lancet (2019) 393:1240–53. doi: 10.1016/S0140-6736(18)32552-2
11. Handley KF, Sims TT, Bateman NW, Glassman D, Foster KI, Lee S, et al. Classification of high-grade serous ovarian cancer using tumor morphologic characteristics. JAMA Network Open (2022) 5:e2236626. doi: 10.1001/jamanetworkopen.2022.36626
12. Khashaba M, Fawzy M, Abdel-Aziz A, Eladawei G, Nagib R. Subtyping of high grade serous ovarian carcinoma: histopathological and immunohistochemical approach. J Egyptian Natl Cancer Inst (2022) 34(6). doi: 10.1186/s43046-022-00104-9
13. Lheureux S, Braunstein M, Oza AM. Epithelial ovarian cancer: evolution of management in the era of precision medicine. CA Cancer J Clin (2019) 69:280–304. doi: 10.3322/caac.21559
14. Harbin LM, Gallion HH, Allison DB, Kolesar JM. Next generation sequencing and molecular biomarkers in ovarian cancer—An opportunity for targeted therapy. Diagnostics (2022) 12:842. doi: 10.3390/diagnostics12040842
15. Xu H, George E, Kinose Y, Kim H, Shah JB, Peake JD, et al. CCNE1 copy number is a biomarker for response to combination WEE1-ATR inhibition in ovarian and endometrial cancer models. Cell Rep Med (2021) 2:100394. doi: 10.1016/j.xcrm.2021.100394
16. Gorski JW, Ueland FR, Kolesar JM. CCNE1 amplification as a predictive biomarker of chemotherapy resistance in epithelial ovarian cancer. Diagnostics (2020) 10:279. doi: 10.3390/diagnostics10050279
17. da Costa AABA, do Canto LM, Larsen SJ, Ribeiro ARG, Stecca CE, Petersen AH, et al. Genomic profiling in ovarian cancer retreated with platinum based chemotherapy presented homologous recombination deficiency and copy number imbalances of CCNE1 and RB1 genes. BMC Cancer (2019) 19:422. doi: 10.1186/s12885-019-5622-4
18. Pulliam N, Fang F, Ozes AR, Tang J, Adewuyi A, Keer H, et al. An effective epigenetic-PARP inhibitor combination therapy for breast and ovarian cancers independent of BRCA-mutations. Clin Cancer Res (2018) 24:3163–75. doi: 10.1158/1078-0432.CCR-18-0204
19. Gasimli K, Raab M, Rad MT, Kurunci-Csacsko E, Becker S, Strebhardt K, et al. Sequential targeting of PLK1 and PARP1 reverses the resistance to PARP inhibitors and enhances platin-based chemotherapy in BRCA-deficient high-grade serous ovarian cancer with KRAS amplification int. J Mol Sci (2022) 23:10892. doi: 10.3390/ijms231810892
20. Staropoli N, Ciliberto D, Giudice TD, Iuliano E, Cucè M, Grillone F, et al. The Era of PARP inhibitors in ovarian cancer: “Class Action” or not? A systematic review and meta-analysis. Crit Rev Oncol/Hematol (2018) 131:83–9. doi: 10.1016/j.critrevonc.2018.08.011
21. Slade D. PARP and PARG inhibitors in cancer treatment. Genes Dev (2020) 34:360–94. doi: 10.1101/gad.334516.119
22. Cong K, Peng M, Kousholt AN, Lee WTC, Lee S, Nayak S, et al. Replication gaps are a key determinant of PARP inhibitor synthetic lethality with BRCA deficiency. Mol Cell (2021) 81:3128–3144.e7. doi: 10.1016/j.molcel.2021.06.011
23. Miller RE, Elyashiv O, El-Shakankery KH, Ledermann JA. Ovarian cancer therapy: homologous recombination deficiency as a predictive biomarker of response to PARP inhibitors. OncoTargets Ther (2022) 15:1105–17. doi: 10.2147/OTT.S272199
24. Miller RE. Poly(ADP-ribose) polymerase inhibitor combination therapy. Cancer J (2021) 27(6):506–10. doi: 10.1097/PPO.0000000000000565
25. Secord AA, O’Malley DM, Sood AK, Westin SN, Liu JF. Rationale for combination PARP inhibitor and antiangiogenic treatment in advanced epithelial ovarian cancer: A review. Gynecol Oncol (2021) 162:482–95. doi: 10.1016/j.ygyno.2021.05.018
26. Kraya AA, Maxwell KN, Eiva MA, Wubbenhorst B, Pluta J, Feldman M, et al. PTEN loss and BRCA1 promoter hypermethylation negatively predict for immunogenicity in BRCA-deficient ovarian cancer. JCO Precis Oncol (2022) 6:e2100159. doi: 10.1200/PO.21.00159
27. Shen S, Wang G, Zhang R, Zhao Y, Yu H, Wei Y, et al. Development and validation of an immune gene-set based Prognostic signature in ovarian cancer. EBioMedicine (2019) 40:318–26. doi: 10.1016/j.ebiom.2018.12.054
28. Gupta VG, Hirst J, Petersen S, Roby KF, Kusch M, Zhou H, et al. Entinostat, a selective HDAC1/2 inhibitor, potentiates the effects of olaparib in homologous recombination proficient ovarian cancer. Gynecol Oncol (2021) 162:163–72. doi: 10.1016/j.ygyno.2021.04.015
29. Pillay N, Brady RM, Dey M, Morgan RD, Taylor SS. DNA replication stress and emerging prospects for PARG inhibitors in ovarian cancer therapy. Prog Biophys Mol Biol (2021) 163:160–70. doi: 10.1016/j.pbiomolbio.2021.01.004
30. van Wagensveld L, van Baal JOAM, Timmermans M, Gaillard D, Borghuis L, Coffelt SB, et al. Homologous recombination deficiency and cyclin E1 amplification are correlated with immune cell infiltration and survival in high-grade serous ovarian cancer. Cancers (2022) 14:5965. doi: 10.3390/cancers14235965
31. ShuyangYao F, Meric-Bernstam, Hong D, Janku F, Naing A, Piha-Paul S, et al. Clinical characteristics and outcomes of phase I cancer patients with CCNE1 amplifcation: MD Anderson experiences. Scic Rep (2022) 12:8701. doi: 10.1038/s41598-022-12669-5
32. Tadesse S, Anshabo AT, Portman N, Lim E, Tilley W, Caldon CE, et al. Targeting CDK2 in cancer: challenges and opportunities for therapy. Drug Discov Today (2020) 25:406–13. doi: 10.1016/j.drudis.2019.12.001
33. Gallo D, Young JTF, Fourtounis J, Martino G, Álvarez-Quilón A, Bernier C, et al. CCNE1 amplification is synthetic lethal with PKMYT1 kinase inhibition. Nature (2022) 604:749–56. doi: 10.1038/s41586-022-04638-9
34. Goehring L, Huang TT. WEE1i-ATRi combination therapy: a promising low-dose treatment for CCNE1-amplified gynecologic cancers. Cell Rep Med (2021) 2:100402. doi: 10.1016/j.xcrm.2021.100402
35. Li S, Wang T, Fei X, Zhang M. ATR inhibitors in platinum-resistant ovarian cancer. Cancers (2022) 14:5902. doi: 10.3390/cancers14235902
36. Du X, Li J, Luo X, Li R, Li F, Zhang Y, et al. Structure-activity relationships of Wee1 inhibitors: A review. Eur J Med Chem (2020) 203:112524. doi: 10.1016/j.ejmech.2020.112524
37. Martins FC, Couturier D-L, de Santiago I, Sauer CM, Vias M, Angelova M, et al. Clonal somatic copy number altered driver events inform drug sensitivity in high-grade serous ovarian cancer. Nat Commun (2022) 13:6360. doi: 10.1038/s41467-022-33870-0
38. Martins FC, Couturier D-L, d. Santiago I, Vias M, Sanders D, Piskorz A, et al. Combination of mTOR inhibition and paclitaxel as a personalised strategy in the context of MYC-amplified high-grade serous ovarian cancer. Ann Oncol (2019) 30:vii10–1. doi: 10.1093/annonc/mdz413.038
39. Zeng M, Kwiatkowski NP, Zhang T, Nabet B, Xu M, Liang Y, et al. Targeting MYC dependency in ovarian cancer through inhibition of CDK7 and CDK12/13. eLife (2018) 7:e39030. doi: 10.7554/eLife.39030
40. Gong X, Du J, Parsons SH, Merzoug FF, Webster Y, Iversen PW, et al. Aurora A kinase inhibition is synthetic lethal with loss of the RB1 tumor suppressor gene. Cancer Discov (2019) 9:248–63. doi: 10.1158/2159-8290.CD-18-0469
41. Oser MG, Fonseca R, Chakraborty AA, Brough R, Spektor A, Jennings RB, et al. Cells lacking the RB1 tumor suppressor gene are hyperdependent on aurora B kinase for survival. Cancer Discov (2019) 9(2):230–47. doi: 10.1158/2159-8290.CD-18-0389
42. Samuel D, Diaz-Barbe A, Pinto A, Schlumbrecht M, George S. Hereditary ovarian carcinoma: cancer pathogenesis looking beyond BRCA1 and BRCA2. Cells (2022) 11(539). doi: 10.3390/cells11030539
43. Schettini F, Giudici F, Bernocchi O, Sirico M, Corona SP, Giuliano M, et al. Poly (ADP-ribose) polymerase inhibitors in solid tumours: Systematic review and meta-analysis. Eur J Cancer (2021) 149:134–52. doi: 10.1016/j.ejca.2021.02.035
44. Yamauchi H, Takei J. Management of hereditary breast and ovarian cancer. Int J Clin Oncol (2018) 23:45–51. doi: 10.1007/s10147-017-1208-9
45. Petrucelli N, Daly MB, Pal T. BRCA1- and BRCA2-associated hereditary breast and ovarian cancer. GeneReviews (1998).
46. Doddato G, Valentino F, Giliberti A, Papa FT, Tita R, Bruno LP, et al. Exome sequencing in BRCA1-2 candidate familias: the contribution of other cancer susceptibility genes. Front Oncol (2021) 11:649435. doi: 10.3389/fonc.2021.649435
47. Velázquez C, Lastra E, Cobos FA, Abella L, de la Cruz V, Hernando BA, et al. A comprehensive custom panel evaluation for routine hereditary cancer testing: improving the yield of germline mutation detection. J Transl Med (2020) 18(232). doi: 10.1186/s12967-020-02391-z
48. Resch LD, Hotz A, Zimmer AD, Komlosi K, Singh N, Tzschach A, et al. The importance of extended analysis using current molecular genetic methods based on the example of a cohort of 228 patients with hereditary breast and ovarian cancer syndrome. Genes (2021) 12:1483. doi: 10.3390/genes12101483
49. Mayoral IH, Santiago AA, Sánchez-Zapardiel JM, Calero BH, de la Hoya M, Gómez-Sanz A, et al. Unexpected findings in hereditary breast and ovarian cancer syndrome: low-level constitutional mosaicism in BRCA2. Genes (2023) 14:502. doi: 10.3390/genes14020502
50. Weber-Lassalle N, Borde J, Weber-Lassalle K, Horváth J, Niederacher D, Arnold N, et al. Germline loss-of-function variants in the BARD1 gene are associated with early-onset familial breast cancer but not ovarian cancer. Breast Cancer Res (2019) 21:55. doi: 10.1186/s13058-019-1137-9
51. Tao J, Sun D, Zhou H, Zhu J, Zhang X, Hou H. Next-generation sequencing identifies potential novel therapeutic targets in Chinese HGSOC patients. Pathol - Res Pract (2022) 238:154074. doi: 10.1016/j.prp.2022.154074
52. Miller RE, Leary A, Scott CL, Serra V, Lord CJ, Bowtell D, et al. ESMO recommendations on predictive biomarker testing for homologous recombination deficiency and PARP inhibitor benefit in ovarian cancer. Ann Oncol (2020) 31(12):1606–22. doi: 10.1016/j.annonc.2020.08.2102
53. Chan AM, Enwere E, McIntyre JB, Wilson H, Nwaroh C, Wiebe N, et al. Combined CCNE1 high-level amplification and overexpression is associated with unfavourable outcome in tubo-ovarian high-grade serous carcinoma. J Pathol Clin Res (2020) 6:252–62. doi: 10.1002/cjp2.168
54. Hollis RL. Molecular characteristics and clinical behaviour of epithelial ovarian cancers. Cancer Lett (2023) 555:216057. doi: 10.1016/j.canlet.2023.216057
55. Seferbekova Z, Lomakin A, Yates LR, Gerstung M. Spatial biology of cancer evolution. Nat Rev Genet (2023) 24:295–313. doi: 10.1038/s41576-022-00553-x
56. Takebe N, Naqash AR, Coyne GOS, Kummar S, Do K, Bruns A, et al. Safety, anti-tumor activity, and biomarker analysis in a phase 1 trial of the once-daily Wee1 inhibitor adavosertib (AZD1775) in patients with advanced solid tumors. Clin Cancer Res (2021) 27:3834–44. doi: 10.1158/1078-0432.CCR-21-0329
57. Morand S, Devanaboyina M, Staats H, Stanbery L, Nemunaitis J. Ovarian cancer immunotherapy and personalized medicine. Int J Mol Sci (2021) 22:6532. doi: 10.3390/ijms22126532
58. Marmolejo DH, Wong MYZ, Bajalica-Lagercrantz S, Tischkowitz M, Balmaña J, e. E.-G. T. G. 3. Overview of hereditary breast and ovarian cancer (HBOC) guidelines across Europe. Eur J Med Genet (2021) 64:104350. doi: 10.1016/j.ejmg.2021.104350
59. Castillo-Guardiola V, Rosado-Jimenez L, Sarabia-Meseguer MD, Marín-Vera M, Macías-Cerrolaza JA, García-Hernandez R, et al. Next step in molecular genetics of hereditary breast/ovarian cancer: Multigene panel testing in clinical actionably genes and prioritization algorithms in the study of variants of uncertain significance. Eur J Med Genet (2022) 65:104468. doi: 10.1016/j.ejmg.2022.104468
60. Ansari FZE, Jouali F, Marchoudi N, Bennani MM, Ghailani NN, Barakat A, et al. Screening of BRCA1/2 genes mutations and copy number variations in patients with high risk for hereditary breast and ovarian cancer syndrome (HBOC). BMC Cancer (2020) 20:747. doi: 10.1186/s12885-020-07250-0
61. Kumar KR, Cowley MJ, Davis RL. Next-generation sequencing and emerging technologies. Semin Thromb Hemost (2019) 45:661–73. doi: 10.1055/s-0039-1688446
62. Punzón-Jiménez P, Lago V, Domingo S, Simón C, Mas A. Molecular management of high-grade serous ovarian carcinoma. Int J Mol Sci (2022) 23:13777. doi: 10.3390/ijms232213777
63. Chougule A, Jagtap V, Nikam A, Kale S, Nambiar K, Bagayatkar P, et al. Comprehensive development and implementation of good laboratory practice for NGS based targeted panel on solid tumor FFPE tissues in diagnostics. Diagnostics (2020) 12:1291. doi: 10.3390/diagnostics12051291
64. Cazzato G, Caporusso C, Arezzo F, Cimmino A, Colagrande A, Loizzi V, et al. ForMalin-fixed and paraffin-embedded samples for next generation sequencing: problems and solutions. Genes (2021) 12:1472. doi: 10.3390/genes12101472
65. Asante D-B, Calapre L, Ziman M, Meniawy TM, Gray ES. Liquid biopsy in ovarian cancer using circulating tumor DNA and cells: Ready for prime time? Cancer Lett (2020) 468:59–71. doi: 10.1016/j.canlet.2019.10.014
66. Zhu JW, Charkhchi P, Akbari MR. Potential clinical utility of liquid biopsies in ovarian cancer. Mol Cancer (2022) 21:114. doi: 10.1186/s12943-022-01588-8
67. Liany H, Lin Y, Jeyasekharan A, Rajan V. Mining pathway associations from networks of mutual exclusivity interactions. bioRxiv (2020). doi: 10.1101/2020.02.20.957241
68. Setton J, Zinda M, Riaz N, Durocher D, Zimmermann M, Koehler M, et al. Synthetic lethality in cancer therapeutics: the next generation. Cancer Discov (2021) 11:1626–35. doi: 10.1158/2159-8290.CD-20-1503
69. da Costa AABA, Baiocchi G. Genomic profiling of platinum-resistant ovarian cancer: The road into druggable targets. Semin Cancer Biol (2021) 77:29–41. doi: 10.1016/j.semcancer.2020.10.016
70. Gulhan DC, Lee JJ-K, Melloni GEM, Cortés-Ciriano I, Park PJ. Detecting the mutational signature of homologous recombination deficiency in clinical samples. Nat Genet (2019) 51:912–9. doi: 10.1038/s41588-019-0390-2
71. Schouten PC, Richters L, Vis DJ, Kommoss S, van Dijk E, Ernst C, et al. Ovarian cancer–specific BRCA-like copy-number aberration classifiers detect mutations associated with homologous recombination deficiency in the AGO-TR1 trial. Clin Cancer Res (2021) 27:6559–69. doi: 10.1158/1078-0432.CCR-21-1673
72. Li Y, Nie Y, Guo H, Guo H, Ha C, Li Y. Establish of an initial platinum- resistance predictor in high-grade serous ovarian cancer patients regardless of homologous recombination deficiency status. Front Oncol (2022) 12:847085. doi: 10.3389/fonc.2022.847085
73. Lino-Silva LS. Ovarian carcinoma: pathology review with an emphasis in their molecular characteristics. Chin Clin Oncol (2020) 9:45. doi: 10.21037/cco-20-31
74. Duncavage EJ, Coleman JF, de Baca ME, Kadri S, Leon A, Routbort M, et al. Recommendations for the use of in silico approaches for next-generation sequencing bioinformatic pipeline validation. A joint report of the association for molecular pathology, association for pathology informatics, and college of american pathologists. J Mol Diagn (2023) 25:3–16. doi: 10.1016/j.jmoldx.2022.09.007
75. Creeden JF, Nanavaty NS, Einloth KR, Gillman CE, Stanbery L, Hamouda DM, et al. Homologous recombination proficiency in ovarian and breast cancer patients. BMC Cancer (2021) 21:1154. doi: 10.1186/s12885-021-08863-9
76. Li MM, Datto M, Duncavage EJ, Kulkarni S, Lindeman NI, Roy S, et al. Standards and guidelines for the interpretation and reporting of sequence variants in cancer. A joint consensus recommendation of the association for molecular pathology, american society of clinical oncology, and college of american pathologists. J Mol Diagn (2017) 19:4–23. doi: 10.1016/j.jmoldx.2016.10.002
77. Golubeva VA, Nepomuceno TC, Monteiro ANA. Germline missense variants in BRCA1: new trends and challenges for clinical annotation. Cancers (2019) 11, 522. doi: 10.3390/cancers11040522
Keywords: ovarian cancer, HGSOC, targeted therapy, cancer screening, HBOC (hereditary breast and ovarian cancer), cancer precision medicine
Citation: Bukłaho PA, Kiśluk J and Nikliński J (2023) Diagnostics and treatment of ovarian cancer in the era of precision medicine - opportunities and challenges. Front. Oncol. 13:1227657. doi: 10.3389/fonc.2023.1227657
Received: 23 May 2023; Accepted: 21 August 2023;
Published: 06 September 2023.
Edited by:
Carole Sourbier, United States Food and Drug Administration, United StatesReviewed by:
Hashem Obaid Alsaab, Taif University, Saudi ArabiaCopyright © 2023 Bukłaho, Kiśluk and Nikliński. This is an open-access article distributed under the terms of the Creative Commons Attribution License (CC BY). The use, distribution or reproduction in other forums is permitted, provided the original author(s) and the copyright owner(s) are credited and that the original publication in this journal is cited, in accordance with accepted academic practice. No use, distribution or reproduction is permitted which does not comply with these terms.
*Correspondence: Patrycja Aleksandra Bukłaho, cGF0cnljamEuYnVrbGFob0BzZC51bWIuZWR1LnBs
Disclaimer: All claims expressed in this article are solely those of the authors and do not necessarily represent those of their affiliated organizations, or those of the publisher, the editors and the reviewers. Any product that may be evaluated in this article or claim that may be made by its manufacturer is not guaranteed or endorsed by the publisher.
Research integrity at Frontiers
Learn more about the work of our research integrity team to safeguard the quality of each article we publish.