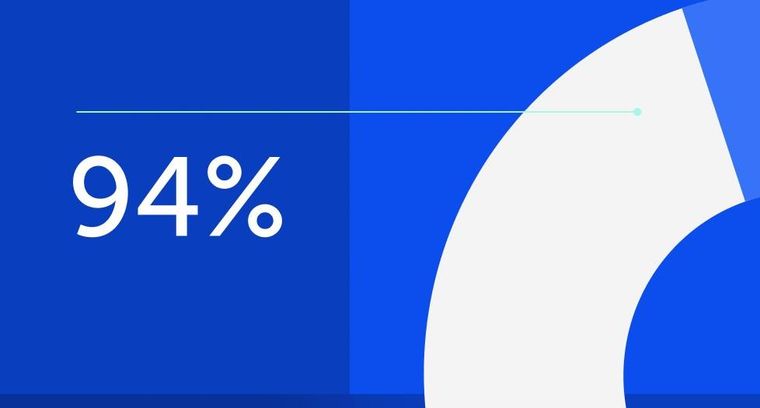
94% of researchers rate our articles as excellent or good
Learn more about the work of our research integrity team to safeguard the quality of each article we publish.
Find out more
ORIGINAL RESEARCH article
Front. Oncol., 20 October 2023
Sec. Thoracic Oncology
Volume 13 - 2023 | https://doi.org/10.3389/fonc.2023.1225646
Introduction: Next-generation sequencing (NGS) is currently widely used for biomarker studies and molecular profiling to identify concurrent alterations that can lead to the better characterization of a tumor’s molecular landscape. However, further evaluation of technical aspects related to the detection of gene rearrangements and copy number alterations is warranted.
Methods: There were 12 ALK rearrangement-positive tumor specimens from patients with non-small cell lung cancer (NSCLC) previously detected via fluorescence in situ hybridization (FISH), immunohistochemistry (IHC), and an RNA-based NGS assay, and 26 MET high gene copy number (GCN) cases detected by FISH, selected for this retrospective study. All 38 pre-characterized cases were reassessed utilizing the PGDx™ elio™ tissue complete assay, a 505 gene targeted NGS panel, to evaluate concordance with these conventional diagnostic techniques.
Results: The detection of ALK rearrangements using the DNA-based NGS assay demonstrated excellent sensitivity with the added benefit of characterizing gene fusion partners and genomic breakpoints. MET copy number alterations were also detected; however, some discordances were observed likely attributed to differences in algorithm, reporting thresholds and gene copy number state. TMB was also assessed by the assay and correlated to the presence of NSCLC driver alterations and was found to be significantly lower in cases with NGS-confirmed canonical driver mutations compared with those without (p=0.0019).
Discussion: Overall, this study validates NGS as an accurate approach for detecting structural variants while also highlighting the need for further optimization to enable harmonization across methodologies for amplifications.
Lung cancer is one of the leading causes of cancer-related deaths in the world. (1) In the current era of precision medicine, non-small cell lung cancer (NSCLC) has a wide assortment of targeted therapies linked to genomic biomarkers and signatures. (2, 3) Progress in genomic analysis using next-generation sequencing (NGS) has enabled the comprehensive detection of multiple targetable alterations simultaneously from limited tumor tissue. (4) Despite this, single-variant and -gene approaches are still employed as standard procedure in many settings to detect clinically relevant driver mutations resulting in tissue exhaustion and, potentially, incomplete tumor characterization. Apparent discrepancies between NGS and these conventional approaches, particularly regarding gene rearrangements and amplifications, however, make the interpretation of some of these alterations by NGS technologies challenging (5–8).
ALK gene fusions are important predictive biomarkers for targeted tyrosine kinase inhibitor (TKI) efficacy in NSCLC (9, 10). ALK rearrangements were initially assessed by fluorescent in situ hybridization (FISH); however, the approach is laborious, costly, and highly subjective (5, 6, 11). Additionally, because this methodology relies on the detection of merged or discrete signals from fluorescent probes, certain ALK gene fusions may be undetected or false-positive patterns could also be reported (12). Increasingly, immunohistochemistry (IHC) has been proposed as an improved and simplified alternative to FISH in the detection of ALK fusions (11, 13). However, both techniques are cell-based, microscopic approaches, making the process inherently subjective, with additional uncertainty arising from borderline or inconclusive cases. Moreover, these approaches are heavily affected by tumor heterogeneity, depending on the tissue sections used for analysis, particularly due to the scarce material usually obtained for lung cancer diagnosis. Consequently, FISH or IHC can be discordant and inconclusive for ALK fusions (6, 7, 14).
MET amplification represents a therapeutic target in NSCLC, though the clinical consensus and standardization of its definition are still subject to controversy (15, 16). Currently, TKIs of MET, such as crizotinib initially, and more recently capmatinib and tepotinib, have demonstrated clinical efficacy and safety in lung cancer patients with MET exon 14 skipping mutations (17, 18) and MET gene amplification (19). Due to the availability of targeted therapies, molecular testing of MET is now included in routine clinical workup in the NSCLC setting with comprehensive NGS panels including analysis for both exon 14 skipping mutations and MET amplifications (20). Reasons for equivocal outcomes for MET amplification may be attributed to a lack of clinically defined cutoffs for MET copy number or fold change (21–23). When using FISH, MET amplifications can be measured by comparing the ratio of MET copies to chromosome enumeration probe 7 (CEP7) (19, 23, 24). FISH also enables differentiation of focal amplifications and polysomy; however, the clinical significance for each of these patterns remains unclear. Regarding NGS, the assessment of MET amplification depends on the platform and remains to be validated in a broad clinical context including associations with response to MET inhibitors (25, 26).
The comprehensive nature of NGS has also facilitated the creation of novel cancer signatures, previously not possible with conventional techniques, such as tumor mutation burden (TMB) (20). TMB acts as a composite genomic score, often reported as mutations per exome or mutations per megabase (muts/Mb) of DNA sequenced, which highlights the overall mutational load of a tumor (27, 28). Clinical observations in NSCLC show that TMB-high (TMB-H) tumors are more responsive to immune checkpoint inhibition (29, 30). This observation has been confirmed in other solid tumor types and has led to FDA approval of pembrolizumab in TMB-H cases, making TMB a new pan-solid tumor biomarker for immunotherapy (31).
Given the multitude of clinically relevant genomic biomarkers present in NSCLC, we sought to analytically evaluate the level of concordance between conventional molecular pathology approaches and a DNA-based NGS assay. Furthermore, we aimed to elucidate any associations between concomitant driver mutations, co-alterations, and explore correlations to clinical outcomes in specific cases.
As a referral testing center for lung cancer biomarkers, Hospital del Mar has tested over 3,950 NSCLC samples from 2012 to 2017 for ALK, ROS1, and MET alterations (32, 33). Samples that presented sufficient residual material after diagnosis were deposited in the biobank collection of our Institution (MARBiobanc integrated in the Xarxa de Bancs de Tumors de Catalunya). Only initial diagnostic samples with remaining FFPE tissue from NSCLC patients were selected for this study. There were 12 samples with known ALK fusion events previously identified by FISH (Abbott Molecular, Des Plaines, IL, USA) reviewed to determine the tumor area and screened by IHC (D5F3, Ventana, Tucson, AZ, USA) and RNA-based NGS (Oncomine™ Focus Assay, Thermo Fisher Scientific, Waltham, MA, USA). Furthermore, 26 advanced NSCLC cases screened for MET amplification by FISH using the Cappuzzo score system (MET high gene copy number (GCN) defined as those with a mean of ≥5 copies per cell) (24) were recategorized into the currently accepted FISH criteria as the following: high-amplification (MET/CEP7 ratio ≥4.0), medium-amplification (MET/CEP7 ratio >2.2–<4.0), low-amplification (MET/CEP7 ratio ≥1.8–≤2.2), and non-amplification (MET/CEP7 ratio <1.8) (19) (Supplementary Figure 1). Using these FISH criteria, NSCLC samples considered positive for the presence of MET amplification are those with a MET/CEP7 >2.2 (high- and medium-amplification), classifying low-amplified together with non-amplified as negative as no actionability was seen when using MET inhibitors (19). This project was approved by the local ethics committee (CEIC-PSMAR: 2015/6336/I), and all patients provided written informed consent.
All 38 samples were further evaluated by the Personal Genome Diagnostics (PGDx™) elio™ tissue complete, a research use only (RUO) comprehensive genomic profiling NGS assay (PGDx, Baltimore, MD, USA). This NGS panel assesses 505 genes using a minimum starting input of 50 ng (100 ng recommended) DNA from FFPE tumor tissue and identifies key genomic alterations (single-nucleotide variants (SNVs), insertion and deletions (indels), gene rearrangements, and amplifications) and complex genomic signatures including TMB, reported as muts/Mb (exome equivalent), and microsatellite instability status. ERBB2 amplifications were called when >2.5-fold change on normalized read depth was detected, and all other gene amplifications represented on the panel were called when >3-fold change was observed. Quality control metrics evaluated by PGDx elio tissue complete are shown in Supplementary Table 1. Sample processing from FFPE tissue, including library preparation, hybrid capture, sequencing, and analysis was performed at PGDx. DNA from FFPE was extracted using the QIAamp® DNA FFPE Tissue Kit (QIAGEN, Hilden, Germany) according to the manufacturer’s protocol. Captured libraries were sequenced on the NextSeq® platform (Illumina, San Diego, CA, USA). Somatic variant identification was performed using the PGDx elio tissue complete bioinformatics software, which incorporates a machine learning-based variant calling algorithm to differentiate between true somatic mutations and artifacts or false positive signal (34). The resultant NGS calls were compared with available data from the orthogonal approaches and with patient clinical data. TMB and microsatellite status were also assessed by the PGDx elio tissue complete assay using previously described methods (35). Finally, cases with driver alterations were analyzed for concomitant aberrations and correlated with clinical features. The Mann–Whitney U test was used for continuous parameters. Statistical tests were conducted at the two-sided 0.05 alpha level of significance, carried out with SPSS Statistics software (SPSS Inc., Chicago, IL, USA).
There were 38 specimens from patients with NSCLC utilized in this study, composed of 27 males and 11 females (Table 1). The mean age of the population at the time of biopsy was 61 years (range, 36–86 years). 71% of the samples were from current smokers (n=27), 18% from former smokers (n=7), and 11% from never smokers (n=4). The study included early- and advanced-stage NSCLC patients consisting of 18% (n=7) with stage I, 5% (n=2) with stage II, 24% (n=9) with stage III, and 53% (n=20) with stage IV disease.
There was 100% concordance between the PGDx elio tissue complete assay and the orthogonal approaches which included IHC, FISH, and the Oncomine Focus Assay (Table 2). EML4::ALK (E13:A20) was found to be the most prevalent ALK fusion comprising 58% of the cases (n=7). Notably, in addition to providing the exact genomic breakpoints for these rearrangements, the PGDx elio tissue complete assay reported the rearrangement types including nine inversions, one duplication within chromosome 2, and two translocations (IRF2BP2 in chromosome 1 and KIF5B in chromosome 10). Notably, the PGDx elio tissue complete identified a novel translocation, IRF2BP2::ALK (I1:A20), which has not been previously described (Table 2; Supplementary Figure 2). This sample from Pt. 11 had positive IHC and FISH for the presence of ALK rearrangement and an ALK imbalance positive expression result when analyzed using the RNA-based NGS Oncomine Focus Assay. The result indicates the presence of a fusion event due to expression imbalance between the 3′ and 5′ ends of the gene, but this NGS approach is not capable of specifically determining the novel gene partner. The DNA-based NGS PGDx elio tissue complete assay allowed the detection of novel fusion genes due to both exonic and intronic probe tiling that captures variants that are not detected through the other methods.
Table 2 Detection of ALK gene rearrangements in 12 patient cases using IHC, FISH, the Thermo Fisher Oncomine Focus Assay, and the PGDx elio tissue complete assay.
The concurrent alterations identified by expanded genomic analysis are shown in Figure 1. Of the 12 samples processed using the PGDx elio tissue complete, 58.3% were found to harbor concomitant TP53 alterations, which were found to be predominantly missense mutations. Overall, the majority of ALK-rearranged cases were not found to have concomitant gene amplification events, with 83% of cases (n=10) having no gene amplifications present. However, two ALK-positive cases were found to have gene amplifications: one with amplifications in both FGFR3 and FGFR4 and one with a MYC amplification.
Figure 1 PGDx elio tissue complete comprehensive genomic profiling results of 12 ALK fusion-positive patients with demographics correlates. Co-occurring amplifications, TMB scores, and SNVs and indels with the fraction (%) of patient with mutations in each gene are presented.
Of the 26 MET high GCN samples selected for the PGDx elio tissue complete assay analysis, only eight cases were recategorized as high-amplified (n=2) and medium-amplified (n=6) following the currently accepted FISH criteria. Five out of these eight cases were confirmed as being MET-amplified by the PGDx elio tissue complete. Therefore, we found three discordant cases between FISH and NGS amplification calls (Table 3). Interestingly, in these cases, increased MET copies were detected by the NGS approach but did not meet the PGDx amplification calling criteria, specifically ≥3-fold amplification and at least 25% of the queried regions of MET amplified (Table 3, numbers denoted in red), and accordingly were not deemed to be genuine amplification events. FISH results from these three cases were reviewed and stated as highly heterogeneous by FISH with focal MET amplifications, partially explaining the results observed by NGS, which is based on a bulk tumor analysis (Supplementary Figure 3).
Table 3 Detection of MET amplification in 26 patient cases using FISH and the PGDx elio tissue complete assay.
In a correlative analysis, the five MET-amplified cases by both FISH and the PGDx elio tissue complete assay were found not to carry any concurrent targetable driver alterations (Pts. 15, 16, 27, 28, and 32; Figure 2). Interestingly, one low-amplified MET case by FISH, which was classified as not amplified by the PGDx elio tissue complete assay (Pt. 18), was found to have a concomitant MET exon 14 skipping mutation (Figure 3A). Five non-amplified cases were found to harbor key NSCLC alternative driver mutations (two KRAS exon 2 mutations and three EGFR sensitizing mutations) (Figures 2, 3A).
Figure 2 PGDx elio tissue complete comprehensive genomic profiling results of 26 previously characterized MET high gene copy number patients with demographic correlates. Amplifications, TMB scores, and SNVs and indels with the fraction (%) of patient with mutations in each gene are presented.
Figure 3 PGDx elio tissue complete TMB results. (A) Comparison of TMB scores and canonical driver mutations in all 38 cases. (B) A statistically significant inverse relationship between TMB scores and incidence of canonical driver mutations was observed (p = 0.0019).
TMB scores in this NSCLC cohort ranged from 0.7 to 50.5 muts/Mb, with a mean TMB of 8.6 and median of 5.2 muts/Mb (Figure 3A). Interestingly, there appears to be an inverse relationship between the incidence of canonical NSCLC driver mutations and TMB scores, wherein those cases with identified driver mutations have a significantly lower TMB scores than those with no identified drivers (p=0.0019, Figure 3B). Regarding microsatellite status, all samples assessed by the PGDx elio tissue complete were found to be microsatellite stable (data not shown).
Clinical outcome data from specific cases of interest were investigated to assess associations with response to targeted therapy. Patient 11, harboring the newly described IRF2BP2::ALK translocation, was a 65-year-old man, a 30-pack-year smoker diagnosed with stage IV disease. He received first-line platinum-pemetrexed therapy with progression at first assessment after 2 months. He then initiated crizotinib at standard doses that was efficacious for 4 months when he had central nervous system progression with rapid deterioration, with no further treatment. Two patients with MET alterations received anti-MET TKI through clinical trial enrollment. Patient 24 was a 55-year-old man, a 50-pack-year smoker who was classified as MET amplified by FISH, although not confirmed by the PGDx elio tissue complete assay. This patient received SAR125844, a selective MET TKI, with stable disease as best response that lasted 4 months. The second was patient 28, classified by FISH as MET high GCN and confirmed as amplified by the PGDx elio tissue complete assay. He entered a clinical trial with capmatinib and obtained a partial response that lasted 18 months.
The use of reliable screening methods for detection of molecular alterations in lung cancer is crucial for selecting the appropriate targeted treatment for eligible patients. Our study demonstrates an excellent concordance between all orthogonal methods and the PGDx elio tissue complete NGS assay for ALK rearrangements. Although IHC and FISH assays can detect ALK fusion events, these methods do not determine ALK fusion partners that may predict clinical benefit of selective TKI more accurately (14, 36). Diversity of fusions and how these may affect response to therapies makes comprehensive NGS analysis increasingly necessary for precision oncology.
EML4::ALK is a canonical structural variant with fusion of exon 13 of EML4 with exon 20 of ALK reported to occur in ~30% of NSCLC cases (37, 38). Interestingly, in studies evaluating the clinical consequence of other ALK fusions, many reports have seen no significant difference between EML4::ALK and non-EML4::ALK variants following treatment with targeted ALK inhibitors (39–41). Differences in clinical outcomes, however, have been reported within distinct EML4::ALK fusion variants, where patients with the canonical EML4::ALK (E13:A20) fusion respond better to crizotinib compared with other EML4::ALK fusion variants (41). More recent studies confirm the prognostic value of different variants, although second- and third-generation ALK inhibitors show better results compared with crizotinib regardless the variant (42). The IRF2BP2 gene identified as a new translocation partner fused with ALK exon 20 codifies for an IFN regulatory factor binding protein that interacts with IFN regulatory factor 2 (IRF‐2). The IRF2BP2 gene has been described as a fusion partner in acute promyelocytic leukemia when fused to RARA gene and in NSCLC is found to be fused to NTRK1 (43, 44). The patient with the IRF2BP2::ALK fusion (Pt. 11) received crizotinib as second-line treatment with lower than expected benefit, although progression was at the central nervous system, confirming the limitation of crizotinib at this anatomical site (10). The information provided by comprehensive NGS, evaluated in the context of clinical response to the different ALK inhibitors, may aid in the selection of the optimal treatment strategy for each patient.
In the detection of MET copy number status via FISH, the fluorescent signal of MET is compared with a CEP7 control and the resultant ratio is reported as a specific level of amplification (19). Through the PGDx elio tissue complete assay, the detected read density for MET is compared with the targeted regions overall through a bulk tumor analysis and interpreted bioinformatically in a more quantitative manner. The discrepancies in what constitutes a genuine MET amplification arise from the lack of clinically defined and meaningful cutoffs in assessing MET amplifications, thereby impeding the adoption of MET assessment in standard-of-care workups (15, 16, 45). After approval of MET targeting therapies, a cutoff of 10-fold change detected by NGS has been accepted as the threshold for prescription. However, this remains controversial as assays typically set cutoffs and thresholds for MET amplifications respective to their test and system, and therefore, may be problematic as results of MET positivity do not translate well across platforms (19, 46).
In our series, of 26 MET high GCN cases reclassified according to current FISH criteria, (19) only eight were considered MET amplified (two high-amplified and six medium-amplified). Analyzing by NGS with the PGDx elio tissue complete assay, we observed that five of these eight were also considered amplified, with only three discordant cases (discussed later). The other 22 MET high GCN cases analyzed in this study were divided by current FISH criteria into 4 low-amplified and 15 non-amplified. The agreement with the NGS panel in these 22 cases was 100% since MET amplification was not detected in any of them. Even so, the copy number values resulting from NGS of Pt. 29 and Pt. 39 stand out, with a MET copy number adjusted to the tumor of 2.6% and 37.8% of regions amplified, and Pt. 39, with a MET copy number of 4.2% and 15.6% of regions amplified, respectively. Both cases displayed copy number variation but did not reach the calling threshold to indicate an amplification by the PGDx elio tissue complete assay (≥3-fold copy number in at least 25% of the queried regions), which is consistent with heterogeneous copy number events such as MET gene gains or chromosome 7 polysomy (47–49). As opposed to gains or polysomy, amplification is thought to represent a state of true biologic selection for MET activation as an oncogenic driver (16). In published results of clinical trials in response to MET inhibitors, clinical benefit was only observed in patients with the so-called “true” amplification (18, 19, 50).
Consistent with this, the prevalence rate of MET amplifications in NSCLC can range from 1% to 5%, with estimates varying depending on the assay used and the fold amplification thresholds implemented. Notably, MET-amplified NSCLC patients have been observed to benefit from certain targeted inhibitors, such as crizotinib; however, the magnitude of benefit is below what is observed with other driver alterations, highlighting the relevance of defining and harmonizing the cutoff (51–55). Interestingly, from the two patients treated with MET inhibitors in our series, the one with the discordant results with PGDx elio tissue complete showed a poor (4-month) performance of TKI treatment compared with the patient with confirmed MET amplification by both techniques.
Whereas detecting rearrangement events via FISH and NGS shows high concordance, detecting copy numbers for genomic amplifications remains more ambiguous. Recent studies are addressing this clinical gap. In the study of capmatinib, patients whose tumors harbored MET GCN of at least 10 exhibited greater therapeutic benefit; however, the response rates were relatively low (40% for treatment naïve vs. 29% for previously treated) compared with patients with exon 14 skipping mutations in MET in the same scenarios (18). In that study, MET GCN alterations were defined by FISH and retrospectively assessed by NGS using the FoundationOne CDx panel. No correlation of both assessments has been reported to our knowledge. In another study with tepotinib, MET amplification assessed by Guardant 360® technology with a cutoff of CN ≥2.5 in liquid biopsy in the absence of MET mutations, tepotinib showed encouraging results (ORR 71% in treatment naïve vs. 30% in second- and third-line settings) (25). These results might better reflect a more biologically relevant cutoff defined by NGS in this study.
Regarding the inverse relationship between TMB score and the presence of canonical driver alterations, it has been previously described that EGFR mutant lung cancers featured lower overall TMB as compared with EGFR wild‐type cancers (56). Also, potential clinical implications have been seen between EGFR-positive patients with low/high TMB when treated with TKIs (57). One limitation of our study is that we do not have sufficient statistical power to compare the parameters of response to treatment and overall survival because both ALK-fused and MET-amplified cases were pooled together in the driver alteration-detected group. However, this inverse association between high TMB and the presence of an ALK fusion was also observed previously, pointing out its potential interest when designing a precision therapy strategy for these patients (58, 59).
The discordant MET results observed in our study might derive from different technical aspects. The PGDx elio tissue complete assay considers factors such as tumor purity, percent of the sequenced genomic region amplified, and minimum coverage depths in determining whether the observed increase in copy number constitutes a genuine amplification event. Such metrics are not taken into consideration with FISH, instead relying on the subjectivity of the observer when deciding on which areas to assess in identifying copy number changes. Bias generated by FISH count due to tissue heterogeneity could potentially differ between tissue sections as reported to occur in the context of other biomarkers and indication, for example, HER2 assessment in breast cancer (60). Despite this, NGS approaches do not solve this biological phenomenon and MET focal amplifications could require further assessment by FISH, as these might not be captured by NGS if the majority of cells do not harbor the amplification. There is no specific information of clinical responses in these particular cases.
The PGDx elio tissue complete assay demonstrated high overall concordance with conventional diagnostic approaches for ALK gene rearrangements. Additionally, the DNA-based NGS approach provides far greater insight into the underlying genomic events with the ability to identify exact breakpoints of gene fusions and report the specific loci associated with gene amplifications. Moreover, it provides broader information regarding potentially relevant genomic co-alterations that may impact clinical outcomes. The optimal cutoff point for defining MET amplifications remains to be defined and might be specific to the NGS platform. Further studies with clinical outcome data are needed to further refine MET amplification as a predictive biomarker.
The original contributions presented in the study are publicly available. This data can be found here: https://ega-archive.org/datasets/EGAD50000000016. Accession number - EGA50000000010.
Ethical review and approval was not required for the study on human participants in accordance with the local legislation and institutional requirements. Written informed consent from the patients/participants or patients/participants’ legal guardian/next of kin was not required to participate in this study in accordance with the national legislation and the institutional requirements.
SC, JJ, MS, EK, EW, DN, JS, BB, and EA contributed to conception and design of the study. JJ, JK, KG, EV, EK, EW, JH, GC, DN, and JS organized the database. JJ, EK, and JG performed the statistical analysis. SC and EK wrote the first draft of the manuscript. JJ, MS, JK, KG, EV, LP, BB, and EA wrote sections of the manuscript. MH-W, PR, XR, and ET participate in image acquisition and technical support in immunohistochemistry, fluorescence in situ hybridization and next-generation sequencing. All authors contributed to the article and approved the submitted version.
The submitted manuscript has been read and approved by all contributing authors. This work was partially supported with an unrestricted research grant from Pfizer S.L.U. The funder was not involved in the study design, collection, analysis, interpretation of data, the writing of this article, or the decision to submit it for publication. This work was also supported by grants from Agència de Gestió d’Ajuts Universitaris i de Recerca (2021-SGR-776 and 2021-SGR-628) and Fondo de Investigación Sanitaria-Instituto de Salud Carlos III co-funded by the European Union (CB16/12/00241 and PI22/00105).
Authors JJ, JK, KG, EV, EK, EW, JH, GC, DN, and JS were or are currently employed by Personal Genome Diagnostics (PGDx/Labcorp).
The authors declare that the research was conducted in the absence of any commercial or financial relationships that could be construed as a potential conflict of interest.
All claims expressed in this article are solely those of the authors and do not necessarily represent those of their affiliated organizations, or those of the publisher, the editors and the reviewers. Any product that may be evaluated in this article, or claim that may be made by its manufacturer, is not guaranteed or endorsed by the publisher.
The Supplementary Material for this article can be found online at: https://www.frontiersin.org/articles/10.3389/fonc.2023.1225646/full#supplementary-material
Supplementary Figure 1 | Representative H&E and FISH images of cases illustrating the currently accepted FISH criteria for MET copy number classification. Samples considered with high MET GCN were reclassified according to the current FISH-criteria as (A) high-amplification (MET/CEP7 ratio ≥4.0), (B) medium-amplification (MET/CEP7 ratio >2.2-<4.0), and (C) low-amplification (MET/CEP7 ratio ≥1.8-≤2.2).
Supplementary Figure 2 | Representative microscopy images of Patient 11, an ALK-positive case with the IRF2BP2::ALK (I1:A20) novel fusion partner. (A) Patient was a 65-year-old man diagnosed of a stage IV lung adenocarcinoma showing an acinar predominant pattern with signed ring cells (10X). (B) ALK D5F3 (Ventana) positive expression (20X). (C) FISH positive pattern showing isolated 3’ALK signals (100X).
Supplementary Figure 3 | Representative H&E and FISH images of one MET discordant case (Patient 26) between FISH and NGS. (A) Non-amplified case with chromosome 7 polysomy and classified as MET high copy number gain according to Cappuzzo score (MET GCN ≥5.0). Case presented a highly heterogeneous FISH counting with areas with (B) focal MET amplifications with a high CEP7 enumeration and areas (C) without MET amplification and presenting chromosome 7 polysomy.
Supplementary Table 1 | Quality control parameters for NGS using the PGDx elio tissue complete assay.
PGDx, Personal Genome Diagnostics; NGS, next-generation sequencing; FISH, fluorescence in situ hybridization; IHC, immunohistochemistry; NSCLC, non-small cell lung cancer; TKI, tyrosine kinase inhibitor; TMB, tumor mutation burden; TMB-H, TMB-high; muts/Mb, mutations per megabase; GCN, gene copy number; SNVs, single-nucleotide variants; indels,insertion/deletions.
1. Siegel RL, Miller KD, Wagle NS, Jemal A. Cancer statistics, 2023. CA Cancer J Clin (2023) 73:17–48. doi: 10.3322/caac.21763
2. Chan BA, Hughes BGM. Targeted therapy for non-small cell lung cancer: Current standards and the promise of the future. Transl Lung Cancer Res (2015) 4:36–54. doi: 10.3978/j.issn.2218-6751.2014.05
3. Bernicker EH, Allen TC, Cagle PT. Update on emerging biomarkers in lung cancer. J Thorac Dis (2019) 11:S81–8. doi: 10.21037/jtd.2019.01.46
4. Pennell NA, Arcila ME, Gandara DR, West H. LUNG CANCER biomarker testing for patients with advanced non-small cell lung cancer: real-world issues and tough choices. Am Soc Clin Oncol Educ Book (2019) 39:531–42. doi: 10.1200/EDBK_237863
5. Uguen A, Talagas M, Marcorelles P, Guéguen P, Costa S, Andrieu-Key S, et al. Next-generation sequencing and immunohistochemistry as future gold standard of ALK testing in lung cancer? Oncologist (2015) 20:e24–4. doi: 10.1634/theoncologist.2015-0123
6. Cabillic F, Hofman P, Ilie M, Peled N, Hochmair M, Dietel M, et al. ALK IHC and FISH discordant results in patients with NSCLC and treatment response: For discussion of the question - To treat or not to treat? ESMO Open (2018) 3:e000419. doi: 10.1136/esmoopen-2018-000419
7. Scattone A, Catino A, Schirosi L, Caldarola L, Tommasi S, Lacalamita R, et al. Discordance between FISH, IHC, and NGS analysis of ALK status in advanced non–small cell lung cancer (NSCLC): a brief report of 7 cases. Transl Oncol (2019) 12:389–95. doi: 10.1016/j.tranon.2018.11.006
8. Vollbrecht C, Lenze D, Hummel M, Lehmann A, Moebs M, Frost N, et al. RNA-based analysis of ALK fusions in non-small cell lung cancer cases showing IHC/FISH discordance. BMC Cancer (2018) 18:1158. doi: 10.1186/s12885-018-5070-6
9. Soda M, Choi YL, Enomoto M, Takada S, Yamashita Y, Ishikawa S, et al. Identification of the transforming EML4-ALK fusion gene in non-small-cell lung cancer. Nature (2007) 448:561–6. doi: 10.1038/nature05945
10. Peters S, Camidge DR, Shaw AT, Gadgeel S, Ahn JS, Kim D-W, et al. Alectinib versus crizotinib in untreated ALK -positive non–small-cell lung cancer. New Engl J Med (2017) 377:829–38. doi: 10.1056/NEJMoa1704795
11. Niu X, Chuang JC, Berry GJ, Wakelee HA. Anaplastic Lymphoma Kinase Testing: IHC vs. FISH vs. NGS. Curr Treat Opt Oncol (2017) 18:71. doi: 10.1007/s11864-017-0513-x
12. Yatabe Y. ALK FISH. and IHC: You cannot have one without the other. J Thorac Oncol (2015) 10:548–50. doi: 10.1097/JTO.0000000000000461
13. Mino-Kenudson M. Immunohistochemistry for predictive biomarkers in non-small cell lung cancer. Transl Lung Cancer Res (2017) 6:570–87. doi: 10.21037/tlcr.2017.07.06
14. Clavé S, Rodon N, Pijuan L, Díaz O, Lorenzo M, Rocha P, et al. Next-generation sequencing for ALK and ROS1 rearrangement detection in patients with non–small-cell lung cancer: implications of FISH-positive patterns. Clin Lung Cancer (2019) 20:e421–9. doi: 10.1016/j.cllc.2019.02.008
15. Kawakami H, Okamoto I, Okamoto W, Tanizaki J, Nakagawa K, Nishio K. Targeting MET amplification as a new oncogenic driver. Cancers (Basel) (2014) 6:1540–52. doi: 10.3390/cancers6031540
16. Drilon A, Cappuzzo F, Ou SHI, Camidge DR. Targeting MET in lung cancer: will expectations finally be MET? J Thorac Oncol (2017) 12:15–26. doi: 10.1016/j.jtho.2016.10.014
17. Paik PK, Felip E, Veillon R, Sakai H, Cortot AB, Garassino MC, et al. Tepotinib in non–small-cell lung cancer with MET exon 14 skipping mutations. New Engl J Med (2020) 383:931–43. doi: 10.1056/NEJMoa2004407
18. Wolf J, Seto T, Han J-Y, Reguart N, Garon EB, Groen HJM, et al. Capmatinib in MET exon 14–mutated or MET -amplified non–small-cell lung cancer. New Engl J Med (2020) 383:944–57. doi: 10.1056/NEJMoa2002787
19. Camidge DR, Otterson GA, Clark JW, Ignatius Ou SH, Weiss J, Ades S, et al. Crizotinib in patients with MET-amplified NSCLC. J Thorac Oncol (2021) 16:1017–29. doi: 10.1016/j.jtho.2021.02.010
20. Lindeman NI, Cagle PT, Aisner DL, Arcila ME, Beasley MB, Bernicker EH, et al. Updated molecular testing guideline for the selection of lung cancer patients for treatment with targeted tyrosine kinase inhibitors: guideline from the college of american pathologists, the international association for the study of lung cancer, and the association for molecular pathology. J Mol Diagnost (2018) 20:129–59. doi: 10.1016/j.jmoldx.2017.11.004
21. Go H, Jeon YK, Park HJ, Sung SW, Seo JW, Chung DH. High MET gene copy number leads to shorter survival in patients with non-small cell lung cancer. J Thorac Oncol (2010) 5:305–13. doi: 10.1097/JTO.0b013e3181ce3d1d
22. Dziadziuszko R, Wynes MW, Singh S, Asuncion BR, Ranger-Moore J, Konopa K, et al. Correlation between MET gene copy number by silver in situ hybridization and protein expression by immunohistochemistry in non-small cell lung cancer. J Thorac Oncol (2012) 7:340–7. doi: 10.1097/JTO.0b013e318240ca0d
23. Noonan SA, Berry L, Lu X, Gao D, Barón AE, Chesnut P, et al. Identifying the appropriate FISH criteria for defining MET copy number-driven lung adenocarcinoma through oncogene overlap analysis. J Thorac Oncol (2016) 11:1293–304. doi: 10.1016/j.jtho.2016.04.033
24. Cappuzzo F, Marchetti A, Skokan M, Rossi E, Gajapathy S, Felicioni L, et al. Increased MET gene copy number negatively affects survival of surgically resected non-small-cell lung cancer patients. J Clin Oncol (2009) 27:1667–74. doi: 10.1200/JCO.2008.19.1635
25. Le X, Paz-Ares LG, Van Meerbeeck J, Viteri S, Cabrera Galvez C, Vicente Baz D, et al. Tepotinib in patients (pts) with advanced non-small cell lung cancer (NSCLC) with MET amplification (METamp). J Clin Oncol (2021) 39:9021. doi: 10.1200/JCO.2021.39.15_suppl.9021
26. Schubart C, Stöhr R, Tögel L, Fuchs F, Sirbu H, Seitz G, et al. Met amplification in non-small cell lung cancer (NSCLC)-A consecutive evaluation using next-generation sequencing (NGS) in a real-world setting. Cancers (Basel) (2021) 13:5023. doi: 10.3390/cancers13195023
27. Chan TA, Yarchoan M, Jaffee E, Swanton C, Quezada SA, Stenzinger A, et al. Development of tumor mutation burden as an immunotherapy biomarker: Utility for the oncology clinic. Ann Oncol (2019) 30:44–56. doi: 10.1093/annonc/mdy495
28. Allgäuer M, Budczies J, Christopoulos P, Endris V, Lier A, Rempel E, et al. Implementing tumor mutational burden (TMB) analysis in routine diagnostics—a primer for molecular pathologists and clinicians. Transl Lung Cancer Res (2018) 7:703–15. doi: 10.21037/tlcr.2018.08.14
29. Hellmann MD, Ciuleanu T-E, Pluzanski A, Lee JS, Otterson GA, Audigier-Valette C, et al. Nivolumab plus ipilimumab in lung cancer with a high tumor mutational burden. New Engl J Med (2018) 378:2093–104. doi: 10.1056/NEJMoa1801946
30. Samstein RM, Lee CH, Shoushtari AN, Hellmann MD, Shen R, Janjigian YY, et al. Tumor mutational load predicts survival after immunotherapy across multiple cancer types. Nat Genet (2019) 51:202–6. doi: 10.1038/s41588-018-0312-8
31. Marabelle A, Fakih M, Lopez J, Shah M, Shapira-Frommer R, Nakagawa K, et al. Association of tumour mutational burden with outcomes in patients with advanced solid tumours treated with pembrolizumab: prospective biomarker analysis of the multicohort, open-label, phase 2 KEYNOTE-158 study. Lancet Oncol (2020) 21:1353–65. doi: 10.1016/S1470-2045(20)30445-9
32. Vidal J, Clavé S, De Muga S, González I, Pijuan L, Gimeno J, et al. Assessment of ALK status by FISH on 1000 Spanish non-small cell lung cancer patients. J Thorac Oncol (2014) 9:1816–20. doi: 10.1097/JTO.0000000000000361
33. Clavé S, Gimeno J, Muñoz-Mármol AM, Vidal J, Reguart N, Carcereny E, et al. ROS1 copy number alterations are frequent in non-small cell lung cancer. Oncotarget (2016) 7:8019–28. doi: 10.18632/oncotarget.6921
34. Wood DE, White JR, Georgiadis A, Van Emburgh B, Parpart-Li S, Mitchell J, et al. A machine learning approach for somatic mutation discovery. Sci Transl Med (2018) 10:eaar7939. doi: 10.1126/scitranslmed.aar7939
35. Keefer LA, White JR, Wood DE, Gerding KMR, Valkenburg KC, Riley D, et al. Automated next-generation profiling of genomic alterations in human cancers. Nat Commun (2022) 13:2830. doi: 10.1038/s41467-022-30380-x
36. Lin C, Shi X, Yang S, Zhao J, He Q, Jin Y, et al. Comparison of ALK detection by FISH, IHC and NGS to predict benefit from crizotinib in advanced non-small-cell lung cancer. Lung Cancer (2019) 131:62–8. doi: 10.1016/j.lungcan.2019.03.018
37. Chia PL, Dobrovic A, Dobrovic A, John T. Prevalence and natural history of ALK positive non-small-cell lung cancer and the clinical impact of targeted therapy with ALK inhibitors. Clin Epidemiol (2014) 6:423–32. doi: 10.2147/CLEP.S69718
38. Yoshida T, Oya Y, Tanaka K, Shimizu J, Horio Y, Kuroda H, et al. Differential crizotinib response duration among ALK fusion variants in ALK-positive non-small-cell lung cancer. J Clin Oncol (2016) 34:3383–9. doi: 10.1200/JCO.2015.65.8732
39. Cha YJ, Kim HR, Shim HS. Clinical outcomes in ALK-rearranged lung adenocarcinomas according to ALK fusion variants. J Transl Med (2016) 14:296. doi: 10.1186/s12967-016-1061-z
40. Kobayashi T, Fujimoto H, Gabazza EC. Efficacy of crizotinib in ALK fusion variants. J Thorac Dis (2016) 8:E1381–3. doi: 10.21037/jtd.2016.10.62
41. Su Y, Long X, Song Y, Chen P, Li S, Yang H, et al. Distribution of ALK fusion variants and correlation with clinical outcomes in chinese patients with non-small cell lung cancer treated with crizotinib. Target Oncol (2019) 14:159–68. doi: 10.1007/s11523-019-00631-x
42. Orlov SV, Iyevleva AG, Filippova EA, Lozhkina AM, Odintsova SV, Sokolova TN, et al. Efficacy of lorlatinib in lung carcinomas carrying distinct ALK translocation variants: The results of a single-center study. Transl Oncol (2021) 14:101121. doi: 10.1016/j.tranon.2021.101121
43. Yin CC, Jain N, Mehrotra M, Zhang J, Protopopov A, Zuo Z, et al. Identification of a cryptic IRF2BP2-RARA fusion in acute promyelocytic leukemia. J Natl Compr Canc Netw (2015) 13:19–22. doi: 10.6004/jnccn.2015.0005
44. Ryosuke O, Amélie B, Shumei K, Sicklick JK, Bazhenova L, Kurzrock R. Analysis of NTRK alterations in pan-cancer adult and pediatric Malignancies: implications for NTRK-targeted therapeutics. JCO Precis Oncol (2018) 2018:00183. doi: 10.1200/PO.18.00183
45. Salgia R. MET in lung cancer: Biomarker selection based on scientific rationale. Mol Cancer Ther (2017) 16:555–65. doi: 10.1158/1535-7163.MCT-16-0472
46. Peng LX, Jie GL, Li AN, Liu SY, Sun H, Zheng MM, et al. MET amplification identified by next-generation sequencing and its clinical relevance for MET inhibitors. Exp Hematol Oncol (2021) 10:52. doi: 10.1186/s40164-021-00245-y
47. Solomon JP, Yang SR, Choudhury NJ, Ptashkin RN, Eslamdoost N, Falcon CJ, et al. Bioinformatically expanded next-generation sequencing analysis optimizes identification of therapeutically relevant MET copy number alterations in >50,000 tumors. Clin Cancer Res (2022) 28:4649–59. doi: 10.1158/1078-0432.CCR-22-1321
48. Kron A, Scheffler M, Heydt C, Ruge L, Schaepers C, Eisert AK, et al. Genetic heterogeneity of MET-aberrant NSCLC and its impact on the outcome of immunotherapy. J Thorac Oncol (2021) 16:572–82. doi: 10.1016/j.jtho.2020.11.017
49. Casadevall D, Gimeno J, Clavé S, Taus A, Pijuan L, Arumí M, et al. MET expression and copy number heterogeneity in nonsquamous non-small cell lung cancer (nsNSCLC). Oncotarget (2015) 6:16215–26. doi: 10.18632/oncotarget.3976
50. Albers J, Friese-Hamim M, Clark A, SChadt O, Walter-Bausch G, Stroh C, et al. The preclinical pharmacology of tepotinib – a highly selective MET inhibitor with activity in tumors harboring MET alterations. Mol Cancer Ther (2023) 22:833–43. doi: 10.1158/1535-7163.MCT-22-0537
51. Camidge DR, Otterson GA, Clark JW, Ou S-HI, Weiss J, Ades S, et al. Crizotinib in patients (pts) with MET-amplified non-small cell lung cancer (NSCLC): Updated safety and efficacy findings from a phase 1 trial. J Clin Oncol (2018) 36:9062. doi: 10.1200/JCO.2018.36.15_suppl.9062
52. Caparica R, Yen CT, Coudry R, Ou SHI, Varella-Garcia M, Camidge DR, et al. Responses to crizotinib can occur in high-level MET-amplified non–small cell lung cancer independent of MET exon 14 alterations. J Thorac Oncol (2017) 12:141–4. doi: 10.1016/j.jtho.2016.09.116
53. Zhang Y, Wang WY, Wang Y, Xu Y, Tian Y, Huang MJ, et al. Response to crizotinib observed in lung adenocarcinoma with MET copy number gain but without a high-level MET/CEP7 ratio, MET overexpression, or exon 14 splicing mutations. J Thorac Oncol (2016) 11:e59–62. doi: 10.1016/j.jtho.2015.12.102
54. Schildhaus HU, Schultheis AM, Rüuschoff J, Binot E, Merkelbach-Bruse S, Fassunke J, et al. Met amplification status in therapy-naïve adeno- And squamous cell carcinomas of the lung. Clin Cancer Res (2015) 21:907–15. doi: 10.1158/1078-0432.CCR-14-0450
55. Camidge DR, Ou S-HI, Shapiro G, Otterson GA, Villaruz LC, Villalona-Calero MA, et al. Efficacy and safety of crizotinib in patients with advanced c-MET-amplified non-small cell lung cancer (NSCLC). J Clin Oncol (2014) 32:8001. doi: 10.1200/jco.2014.32.15_suppl.8001
56. Offin M, Rizvi H, Tenet M, Ni A, Sanchez-Vega F, Li BT, et al. Tumor mutation burden and efficacy of EGFR-tyrosine kinase inhibitors in patients with EGFR-mutant lung cancers. Clin Cancer Res (2019) 25:1063–9. doi: 10.1158/1078-0432.CCR-18-1102
57. Lin C, Shi X, Zhao J, He Q, Fan Y, Xu W, et al. Tumor mutation burden correlates with efficacy of chemotherapy/targeted therapy in advanced non–small cell lung cancer. Front Oncol (2020) 10:480. doi: 10.3389/fonc.2020.00480
58. Liu S, Huang T, Liu M, He W, Zhao YS, Yang L, et al. The genomic characteristics of ALK fusion positive tumors in chinese NSCLC patients. Front Oncol (2020) 10:726. doi: 10.3389/fonc.2020.00726
59. Ross JS, Ali SM, Fasan O, Block J, Pal S, Elvin JA, et al. ALK fusions in a wide variety of tumor types respond to anti-ALK targeted therapy. Oncologist (2017) 22:1444–50. doi: 10.1634/theoncologist.2016-0488
60. Ross DS, Zehir A, Cheng DT, Benayed R, Nafa K, Hechtman JF, et al. Next-generation assessment of human growth factor receptor 2 (ERBB2) amplification status: clinical validation in the context of a hybrid capture-based, comprehensive solid tumor genomic profiling assay. J Mol Diagnost (2017) 19:244–54. doi: 10.1016/j.jmoldx.2016.09.010
Keywords: next generation sequencing (NGS), non-small cell lung cancer (NSCLC), MET amplification, ALK rearrangement, fluorescence in situ hybridization (FISH), biomarkers, molecular testing
Citation: Clavé S, Jackson JB, Salido M, Kames J, Gerding KMR, Verner EL, Kong EF, Weingartner E, Gibert J, Hardy-Werbin M, Rocha P, Riera X, Torres E, Hernandez J, Cerqueira G, Nichol D, Simmons J, Taus Á, Pijuan L, Bellosillo B and Arriola E (2023) Comprehensive NGS profiling to enable detection of ALK gene rearrangements and MET amplifications in non-small cell lung cancer. Front. Oncol. 13:1225646. doi: 10.3389/fonc.2023.1225646
Received: 19 May 2023; Accepted: 28 August 2023;
Published: 20 October 2023.
Edited by:
Francesco Pepe, University of Naples Federico II, ItalyReviewed by:
Caterina De Luca, University of Naples Federico II, ItalyCopyright © 2023 Clavé, Jackson, Salido, Kames, Gerding, Verner, Kong, Weingartner, Gibert, Hardy-Werbin, Rocha, Riera, Torres, Hernandez, Cerqueira, Nichol, Simmons, Taus, Pijuan, Bellosillo and Arriola. This is an open-access article distributed under the terms of the Creative Commons Attribution License (CC BY). The use, distribution or reproduction in other forums is permitted, provided the original author(s) and the copyright owner(s) are credited and that the original publication in this journal is cited, in accordance with accepted academic practice. No use, distribution or reproduction is permitted which does not comply with these terms.
*Correspondence: Edurne Arriola, ZWFycmlvbGFAcHNtYXIuY2F0
Disclaimer: All claims expressed in this article are solely those of the authors and do not necessarily represent those of their affiliated organizations, or those of the publisher, the editors and the reviewers. Any product that may be evaluated in this article or claim that may be made by its manufacturer is not guaranteed or endorsed by the publisher.
Research integrity at Frontiers
Learn more about the work of our research integrity team to safeguard the quality of each article we publish.