- 1Department of Clinical Biochemistry, School of Pharmacy and Pharmaceutical Sciences, Isfahan University of Medical Sciences, Isfahan, Iran
- 2Nutrition and Food Security Research Center, Isfahan University of Medical Sciences, Isfahan, Iran
- 3School of Medicine, Shahid Beheshti University of Medical Sciences, Tehran, Iran
- 4School of Medicine, Iran University of Medical Science, Tehran, Iran
- 5Research Committee, Department of Immunology, School of Medicine, Shahid Beheshti, University of Medical Sciences, Tehran, Iran
- 6Department of Pharmacology, School of Medicine, Shahid Beheshti University of Medical Sciences, Tehran, Iran
- 7Department of Clinical Biochemistry, Faculty of Medicine, Ahvaz Jundishapur University of Medical Sciences, Ahvaz, Iran
- 8Department of Immunology, School of Medicine, Isfahan University of Medical Sciences, Isfahan, Iran
- 9School of Medicine, Kashan University of Medical Sciences, Kashan, Iran
- 10Research Center for Biochemistry and Nutrition in Metabolic Diseases, Institute for Basic Sciences, Kashan University of Medical Sciences, Kashan, Iran
- 11Student Research Committee, Department of Immunology, School of Medicine, Shahid Beheshti University of Medical Sciences, Tehran, Iran
Hepatocellular carcinoma (HCC) is one of the deadliest cancers due to multifocal development and distant metastasis resulting from late diagnosis. Consequently, new approaches to HCC diagnosis and treatment are required to reduce mortality rates. A large body of evidence suggests that non-coding RNAs (ncRNAs) are important in cancer initiation and progression. Cancer cells release many of these ncRNAs into the blood or urine, enabling their use as a diagnostic tool. Circular RNAs (CircRNAs) are as a members of the ncRNAs that regulate cancer cell expansion, migration, metastasis, and chemoresistance through different mechanisms such as the Wnt/β-catenin Signaling pathway. The Wnt/β-catenin pathway plays prominent roles in several biological processes including organogenesis, stem cell regeneration, and cell survival. Aberrant signaling of both pathways mentioned above could affect the progression and metastasis of many cancers, including HCC. Based on several studies investigated in the current review, circRNAs have an effect on HCC formation and progression by sponging miRNAs and RNA-binding proteins (RBPs) and regulating the Wnt/β-catenin signaling pathway. Therefore, circRNAs/miRNAs or RBPs/Wnt/β-catenin signaling pathway could be considered promising prognostic and therapeutic targets in HCC.
1 Introduction
Hepatocellular carcinoma (HCC) is an invasive type of cancer that leads to over 700,000 deaths annually worldwide (1). This tragedy occurs due to lack of effective diagnostic tools as well as efficient therapeutic approaches (2, 3). Although surgical removal, liver transplants, and chemotherapeutic agents are the most commonly used treatments for HCC, they are ineffective in many cases due to multifocal development and distant metastasis caused by late diagnosis (4, 5). Approximately 70% of patients with HCC are diagnosed late, therefore, even if these therapeutic strategies are used in early stages, they are unable to improve the 5-year overall survival rate over 70% (6, 7). As a result, advances in diagnostic and therapeutic approaches must be expanded to reduce HCC mortality.
Non-coding RNAs (ncRNAs) are RNAs derived from the human genome but not translated into protein (8). NcRNAs regulate gene expression through several pathways, such as interactions with epigenetic factors to silence genes and interactions with transcription factors to inhibit or promote target gene expression (9). Based on abundant evidence, ncRNAs play an important role in the initiation and progression of various cancers (10, 11). Cancer cells release many of these ncRNAs into the blood or urine, so they can be used as a diagnostic factor. These ncRNAs are divided into four main types, including microRNA (miRNA), long non-coding RNA (lncRNA), circular RNA (circRNA) and PIWI interacting RNA (piRNA) (12). CircRNA have a unique and stable structure that comprises covalently closed loops (13). They are crucial in the development of several malignancies, including colorectal cancer (14), cholangiocarcinoma (15), bladder cancer (16), and HCC (17), through the regulation of cancer cell proliferation, migration and metastasis, and chemoresistance (18). Most important functions of circRNA for regulating target gene expression are miRNA regulation and effect on the signaling pathways such as Wnt/β-catenin (19).
The Wnt family includes a group of proteins that play important roles in a various cellular functions, such as organ development, stem cell renewal, and the cell viability (20). The Wnt Cascade is divided into different branches, including the canonical Wnt/β-catenin (Wnt/β-catenin dependent pathway) and the non-canonical Wnt/β-catenin pathway (β-catenin-independent pathway). The latter is further divided into the Wnt/calcium and planar cell polarity (PCP) pathways that have an effect on cancer progression and propagation (21). Wnt/β-catenin is involved in numerous physiological mechanisms, such as cell expansion and differentiation, cell death, migration, and tissue homeostasis (22). Abnormalities in Wnt signaling have been linked to several cancers, including gastrointestinal cancers, especially colorectal cancer, leukemia, melanoma, breast cancer and also in a significant subset of HCC (23, 24).
Several studies have found that circRNAs have the potential to be used as biomarkers in tissue samples for cancers diagnosis, with noticeable clinical applications for predicting HCC prognosis. This could potentially lead to improved therapeutic strategies (25, 26). For instance, Peisi kou et al, revealed that expression of Circular RNA hsa_circ_0078602 was meaningfully decreased in 79 samples of HCC patients when compared to controlled non-tumoral tissues (P=0.015). Kaplan-Meier survival analysis indicated that lower expression of hsa_circ_0078602 is associated with poor prognosis in HCC cases (27). In contrast, another study on HCC tissue samples from 59 patients found that circ_0000267 is associated with poor prognosis and promotes proliferation, migration and infiltration of cancer cells through sponging miR‐646 (28).
Our theory is that circRNAs could become a novel approach for detecting and treating HCC in the future. To support this idea, we conducted a review of recent studies that investigate the impact of circRNAs on HCC development, with a particular focus on how they affect miRNA regulation and the Wnt/β-catenin signaling pathway.
2 Wnt pathway: canonical and non-canonical signaling
The Wnt pathway comprises two types of signal transduction pathways (STPs): canonical (Wnt/β-catenin) and non-canonical (planar cell pole (PCP) and Wnt/calcium (Wnt/Ca2+)) (29, 30) as shown in (Figure 1). Many studies have investigated the role of Wnt signaling in the tumorigenesis, with an focus on signaling that relies on β-catenin (31). In the absence of Wnt, a complex of β-catenin degradation proteins, including Axis inhibition protein (AXIN), adenomatous polyposis coli (APC), and glycogen synthase kinase 3-beta (GSK-3-beta), is formed. This complex breaks down β-catenin through phosphorylation at both serine and threonine residues. When Wnt is present, it binds to one of the ten Frizzled (FZD) receptors, leading to the formation of a receptor complex containing Wnt, FZD, lipoprotein receptor-related protein (LRP), Disheveled (DVL), and AXIN. Inside this dynamic complex, DVL is phosphorylated and eventually blocks GSK-3-beta, which makes β-catenin less phosphorylated and prevents its proteolytic degradation (32). β-catenin is then stored in the cytoplasm and can be translocated to the nucleus, where it interacts with members of the TCF/LEF family of gene-activating transcription factors, such as CREB-binding protein (CBP) and p300. c-Myc and cyclin D1 among the many target genes (33). Receptor tyrosine kinase-like orphan receptor 2 (ROR2), a transmembrane orphan receptor tyrosine kinase required for non-canonical Wnt signaling, can also function as canonical signaling through FZD2 interaction (34). Therefore, ROR2 and other Wnt-binding receptors like the receptor tyrosine kinase RYK may play a regulatory role in β-catenin-independent Wnt signaling. Wnt ligands that activate the two non-canonical Wnt pathways include Wnt4, Wnt5a, Wnt7a, Wnt11, and Wnt16. Wnt11. For instance, stimulates the PCP signaling pathway, which in turn activates the small GTPases RAS homologue gene-family member A (RhoA) and Ras-related C3 botulinum toxin substrate 1 (RAC1) (35). This causes the stress kinases Jun N-terminal kinase (JNK) and Rho-associated coiled-coil-containing protein kinase 1 (ROCK) to be activated, altering cell adherence and migration. Wnt5a is the most well-known Ca2+-dependent Wnt signaling inducer, triggering STP via DVL-3 and phospholipases (36). When this pathway is activated, there is a temporary increase in cytoplasmic free Ca2+, which can stimulate the PKC family, calcium calmodulin mediated kinase II (CaMKII), and the calcineurin phosphatase. In addition to Ca2+-dependent Wnt STP, tumor cells have been found to have a novel non-canonical Wnt STP mediated by FYN tyrosine kinase and STAT3 (37). Wnt proteins are controversial in that they may interact with receptors, control the Wnt STP and Wnt-related elements. For instance, non-canonical Wnt signaling inhibits canonical Wnt activity via a variety of pathways, including PKC-alpha, CaMKII-Transforming growth factor β-Activated Kinase (TAK)1, Nemo-like Kinase (NLK), Siah2 E3 ubiquitin ligase, and calcineurin-NFAT. Non-canonical Wnt ligands have also been shown to activate the canonical pathway and further complicate Wnt signaling, making modification in cancer therapies challenging (38).
3 CircRNAs: biogenesis and biological functions
According to the circular structure and lack of 3′-end and 5′-end of circRNAs, they have higher stability than linear RNAs and resist degradation by RNAase R (39, 40). CircRNAs play an essential role in various cellular processes and gene expression via regulating RNA polymerase II function and participating in mRNA processing (41). Thousands of genes have been found to produce circRNAs in mammals. Unlike linear RNAs, circRNAs are generated through the non-sequential back-splicing (or exon skipping) of protein-coding RNA precursors catalyzed by spliceosomes or ribozymes (42). CircRNAs may arise from different gene parts such as exons, introns, intergenic sites, antisense, and untranslated regions (UTRs) (43) and are divided into five major types based on the origin of their gene: Exonic circRNA (the most abundant type), intronic circular RNA, Exon-intron circular RNA, Intragenic circular RNAs, and Antisense circular RNAs (39). The two most widely accepted models for exonic-circRNA biogenesis are lariat-induced circularization and intron pairings (44). Non-adjacent exons of a pre-mRNA become closer together in the lariat-driven circularization model by developing an intermediate lariat structure containing multiple exons and introns. Exonic circRNA is produced after intron separation by connecting the downstream exon (splice donor) and upstream exon (splice receptor) (45). In some cases, during lariat-driven circularization, introns are not spliced out, resulting in the formation of exon-intron circRNA (46). The intron pairing-driven circularization is based on the complementary pairing of Alu repeats on the intron ends, followed by pre-mRNA reverse splicing, which results in the formation of exonic and exon-intron circRNA (43). The functions of circRNA generally are: regulating gene splicing and transcription, regulating protein translation and function, sponging miRNA, and interacting with RNA-binding proteins (RBP) (47, 48). Localization of circRNA can also determine its function, as nuclear circRNAs mainly regulate transcriptional processes, and cytoplasmic circRNA mainly regulates the functions of miRNA and protein. Even though most circRNA are ncRNAs, recent studies revealed that some cytoplasmic circRNA can be translated to peptides (49). Expression of circRNA is different in various cell types and tissues; for instance, circRNA is widely distributed in the brain and is involved in brain development and function (50, 51). As circRNAs play a pivotal role in regulating many cellular processes, aberrant expression of circRNA leads to impaired cellular function. Several investigations have revealed that circRNA is overexpressed in a variety of human diseases, including cardiovascular disease, neurologic disease, autoimmune disease, and cancers (52). CircRNA can exert tumorigenesis or tumor suppressor effects through various mechanisms, such as regulating cell proliferation and apoptosis. Therefore, circRNA can be considered a diagnostic marker and therapeutic target in several diseases, including different types of cancers.
4 CircRNAs in HCC: prognostic and diagnostic value
Given the severity and high recurrence rate of HCC, the prognosis remains poor. As a result, effective diagnostic and prognostic biomarkers are required. Several biomarkers have been suggested for HCC diagnosis and prognosis, including alpha-fetoprotein (AFP), Lens culinaris-agglutinin-reactive fraction of AFP (AFP-L3), protein induced by vitamin K absence orantagonist-II (PIVKA-II), vascular endothelial growth factor (VEGF), hypoxia-inducible factor (HIF), and others (53). On the other hand, circRNAs are increasingly linked to HCC progression and migration, despite their unknown function (54). Shang et al. discovered that hsa_circ_0005075 is overexpressed in HCC tissues and correlates with tumor size (55). Similarly, Qin et al. discovered that hsa_circ_0001649 was down expressed in HCC cells, indicating that it could be used as a prognostic and diagnostic biomarker (56). Recent studies have linked high ciRS-7 and AFP levels in HCC to hepatic microvascular invasion (57). CircRNAs could also communicate with miRNAs and act as miRNA sponges, influencing HCC progression by controlling downstream of a desired gene (58). Hsa_circ_0001649 and hsa_circ_0005075 contain potential miR-182 and miR-93 binding sites, respectively (59). MiR-182 and miR-93 have been identified as possible biomarkers for HCC prognosis and diagnosis. Furthermore, mounting evidence suggests that Wnt-related circRNAs have diagnostic biomarker potential and are linked to tumor development (60). In the following sections, we discuss about Wnt signaling pathways and their relationship with HCC as well as the role of the circRNA/Wnt axis in the HCC progression.
5 Interactions between circRNAs and Wnt signaling pathway in HCC
CircRNAs have been demonstrated to modulate cell proliferation, migration, and apoptosis by regulating gene transcription or by activating or inactivating signaling pathways associated with cancer (60). The most circRNAs function as miRNA sponges, activating or inhibiting the Wnt signaling pathway (61). As research advances, more circRNA-Wnt pathway interactions will be discovered. CircRNAs can influence cancer development and advancement through direct or indirect interactions with the Wnt pathway. Wnt-related circRNA abnormal activation has been linked to cancer cell proliferation, progression, invasion, and metastasis in tumors of the gastrointestinal and respiratory tracts, the central nervous system, the musculoskeletal system, and the endocrine system (60). The relevant studies suggest that this abnormal expression could be used as prognostic and diagnostic biomarkers, as well as treatment targets of cancer. These findings add to our knowledge of the fundamental processes that lead to cancer initiation and development. As a result, an overview of the most recent research on the circRNA-Wnt/β-catenin pathway in HCC and its regulatory framework for developing new treatments was conducted (Figure 2 and Table 1). The next section will discuss the expression, characteristics, activities, and processes of circRNAs/Wnt axis in HCC.
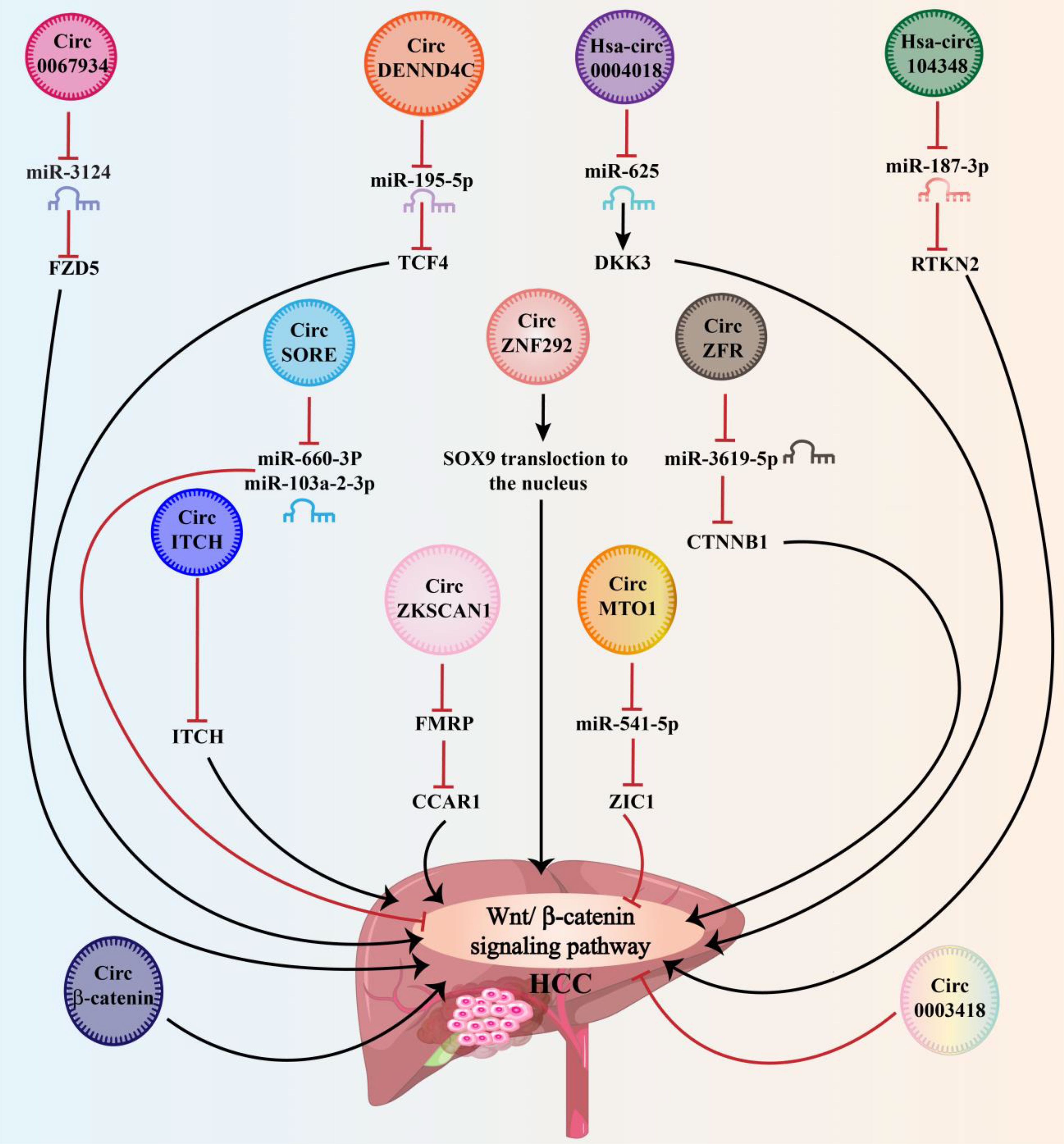
Figure 2 By sponging miRNAs, circRNA molecules regulate the Wnt signaling pathway for further modulation of HCC development in a positive and/or negative manner.
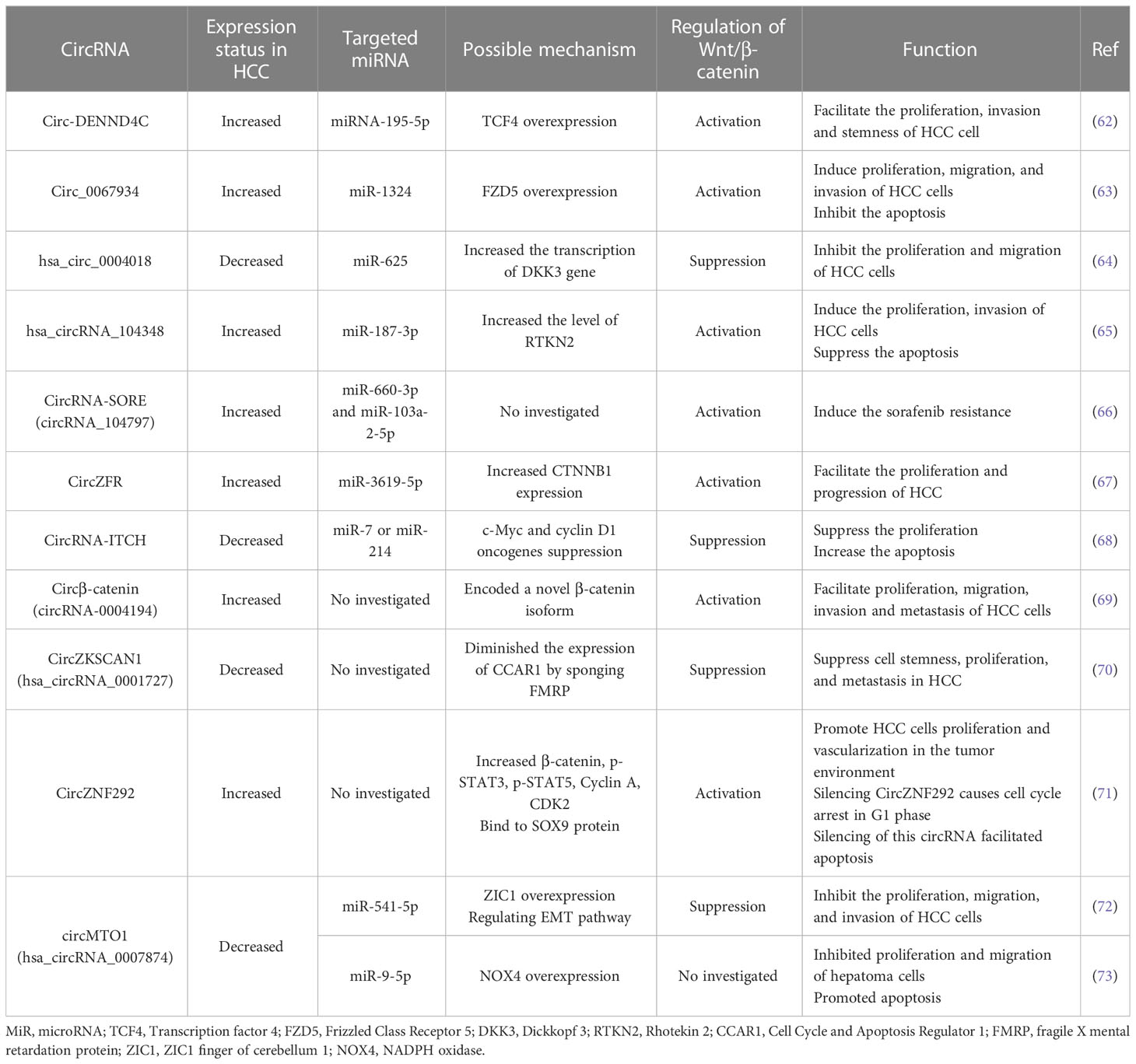
Table 1 CircRNAs which are involved in the progression of the HCC through regulating Wnt signaling pathway.
5.1 Circ_0067934
Circ_0067934 is a one type of circRNAs which has been indicated that participated in the HCC, esophageal squamous cell carcinoma, thyroid tumors, and lung cancer (74). Circ_0067934 overexpression is positively linked to the low overall survival of HCC. Zhu et al. discovered that silencing circ_0067934 reduced the cancer cells expansion, invasion, and metastasis, while enhancing the apoptosis both in vitro and in vivo (63). MiRNAs are generally known as regulators of various gene expression through targeting the mRNAs (75). It has been seen that silencing circ_0067934 considerably elevated miR-1324 levels in HCC cells. MiR-1324 overexpression suppresses the production of FZD5 protein which positively activates the Wnt/β-catenin signaling pathway. This pathway has a critical role in the HCC formation and progression (63, 76–78). In result, circ_0067934 could enhance FZD5 protein by inhibition of miR-1324 that leads to Wnt/β-catenin axis activation in HCC.
5.2 Circ_0003418
Circ_0003418 expression was discovered to be inversely related to tumor size, TNM stage, and HBsAg level in HCC cell lines. Circ_0003418 may reduce the ability of HCC cells to proliferate, migrate, and invade. Moreover, circ_0003418 reduced resistance to cisplatin in HCC cells by inhibiting the Wnt/β-catenin signaling pathway (79, 80)
5.3 CircRNA-ITCH
ITCH, an E3 ubiquitin protein ligase, is a protein which inhibits the Wnt/β-catenin signaling pathway. CircRNA-ITCH could increase the ITCH gene expression, indirectly inhibiting the Wnt/β-catenin signaling pathway (81, 82). CircRNA-ITCH played as an inhibitory factor for esophageal squamous cell carcinoma, lung cancer and triple-negative breast cancer by suppressing Wnt pathway (83–85). Furthermore, overexpression of circRNA-ITCH in HCC cell lines could substantially decrease HCC cell growth and enhance the cell death. CircRNA-ITCH could hinder the Wnt/β-catenin signaling pathway, resulting in a decrease in c-Myc and cyclin D1 oncogenes as Wnt/β-catenin pathway target genes (68, 86).
5.4 Circ−DENND4C
Circ-DENND4C (DENN domain containing 4C) has been shown to be overexpressed in breast cancer and glioma (87, 88). Liu et al., indicated that circ-DENND4C was significantly upregulated in HCC. They observed that silencing circ-DENND4C decreased the cell cycle-related proteins (Cyclin D1, CDK4) and Bcl-2, while enhancing Bax expression (62, 89). Moreover, they revealed that circ-DENND4C lowered the expression of miRNA-195-5p in HCC, which contributes to the overexpression of transcription factor 4 (TCF4). TCF4 overexpression resulted in the accumulation of β-catenin by activating the Wnt/β-catenin signaling pathway (62).
5.5 Has circ0004018
The expression of hsa_circ_0004018 was decreased in HCC compared with non-tumor tissue (90). According to reports, hsa_circ_0004018 serves as a sponge for miR-625. MiR-625 could target Dickkopf-3 (DKK3), a crucial gene in the hindering Wnt/β-catenin signaling pathway, and thus inhibit DKK3 gene expression. Hsa_circ_0004018 could suppress the HCC cell growth and invasion by diminishing miR-625 function, enhancing DKK3 gene expression and inactivating Wnt/β-catenin signaling pathway (64).
5.6 CircZNF292
Previous studies have indicated that circRNA zinc finger protein 292 (circZNF292) was upregulated in the hypoxic environment in the solid tumors, including HCC. CircZNF292 has proangiogenic function in the hypoxic condition of tumor environment in vitro (91–93). It has been demonstrated that circZNF292 knocking-down could decrease the downstream gene expression involved in Wnt/β-catenin signaling pathway including β-catenin, cyclin D1, and c-Myc. So, circZNF292 could promote the proliferation of HCC cells via Wnt/β-catenin signaling pathway activity (94). It has been shown that Wnt/β-catenin signaling pathway contributes to the vascularization in the tumor environment through upregulation of β-catenin which directly associated with the expression of the epithelial mesenchymal transition (EMT) factors, such as Twist1 and VE-cadherin (95, 96). CircZNF292 silencing significantly decreased β-catenin, Twist1 and VE-cadherin levels in the HCC cells through reducing Wnt/β-catenin signaling pathway activity. CircZNF292 could bind to SOX9 protein, a nuclear transcription factor which negatively regulates the activity of Wnt/β-catenin signaling pathway, in the cytoplasm and inhibited its translocation to the nucleus, resulting in the enhancement of Wnt/β-catenin signaling pathway activity and induction of vascularization (94). Thus, circZNF292 silencing could be investigated as a novel therapeutic strategy in HCC patients. In addition, knocking down circZNF292 caused cell cycle arrest in G1 phase, induced apoptosis and inactivated the Wnt/β-catenin signaling pathway of HCC cells, making circRNA ZNF292 as a target in HCC (71).
5.7 Hsa_circRNA_104348
It has been revealed that hsa_circRNA_104348 expression increased in the HCC and correlated with the poor prognosis. Overexpression of hsa_circRNA_104348 induced proliferation, whereas inhibiting apoptosis of the HCC cells (65). Rhotekin 2 (RTKN2) is a protein which is expressed in various tissues, but it has been reported that RTKN2 is upregulated in some cancers such as ovarian cancer, bladder cancer and HCC (97–100). The expression of RTKN2 can be regulated by miR-187-3p. Hsa_circRNA_104348 could act as a sponge for miR-187-3p and sequestered miR-187-3p, which contributed to RTKN2 overexpression in HCC. In addition, hsa_circRNA_104348 activated the Wnt/β-catenin signaling pathway and facilitated HCC cell proliferation, invasion, and metastasis (65).
5.8 CircRNA-SORE (circRNA_104797)
Sorafenib is a kinase inhibitor drug which is used in the advanced HCC (101). CircRNA-SORE has been shown to significantly increase the Sorafenib resistance by hindering the tumor cell apoptosis. circRNA-SORE play a role in sponging miR-660-3p and miR-103a-2-5p. MiR-660-3p and miR-103a-2-5p could significantly inhibit Wnt/β-catenin signaling pathway. Therefore, Sorafenib resistance is maintained by circRNA-SORE, which regulates the Wnt/β-catenin signaling pathway (66).
5.9 CircZFR
Circular RNA circZFR has been linked to the progression of HCC, breast cancer, papillary thyroid carcinoma, and bladder cancer (102–105). CircZFR functions as an oncogene in HCC, is significantly upregulated in HCC, and is associated with a poor prognosis in HCC patients. CircZFR has been shown to downregulate miR-3619-5p, while catenin Beta 1 (CTNNB1) expression is positively correlated with circZFR overexpression (106). CTNNB1 mutations were found in 18% to 40% of HCC patients and were associated with activation of the Wnt/β-catenin signaling pathway (62, 90). Thus, by regulating the miR-3619-5p/CTNNB1 axis, circZFR activated the Wnt/β-catenin signaling pathway, allowing circZFR silencing as a novel therapeutic target for HCC (106).
5.10 Circβ-catenin (circRNA-0004194)
CircRNA-0004194, also known as circβ-catenin, was overexpressed in the HCC. Liang et al., observed that circβ-catenin silencing in vivo and in vitro could repress the progression, migration, and invasion of HCC cells. Interestingly, circβ-catenin is a translatable protein that has resulted in an isoform known as β-catenin-370aa. This isoform could attenuate glycogen synthase kinase 3β (GSK3β) function through prevention of GSK3β binding to fill-length β-catenin. GSK3β inhibits β-catenin activity by β-catenin degradation. Thus, circβ-catenin could enhance β-catenin level in the tumor microenvironment by preventing β-catenin destruction, inducing Wnt/β-catenin signaling pathway (69, 107).
5.11 CircZKSCAN1 (hsa_circRNA_0001727)
The studies have shown that the expression of circZKSCAN1 was decreased in the HCC cell lines in comparison to the non-tumor tissue (70). Previous research reported that Fragile X mental retardation Protein (FMRP), a protein which mainly functions in the nervous system by targeting mRNAs, is overexpressed in HCC cells (108). CircZKSCAN1 and FMRP play opposing roles in HCC cells, with circZKSCAN1 suppressing stemness and FMRP increasing stemness through regulation of cell cycle and apoptosis regulator 1 (CCAR1) expression (70). CCAR1 is a β-catenin-binding protein that facilitates β-catenin in triggering Wnt target gene transcription (109–111). Moreover, CCAR1 overexpression in HCC is correlated to low survival rate (112). CircZKSCAN1 served as an RBP sponge in competition with target gene of FMRP, CCAR1, suppressing the Wnt/β-catenin signaling pathway, inhibiting cell stemness, expansion, and invasion in HCC and could be considered a therapeutic agent for HCC (70).
5.12 CircMTO1 (hsa_circRNA_0007874)
Previous studies were stated that circMTO1 play a role as a tumor suppressor (113) and inhibit the development of several cancers such as glioblastoma (114), gallbladder cancer (115), lung adenocarcinoma (116). The expression of circMTO1 was found to be downregulated in HCC. CircMTO1 could serve as a sponge for miR-541-5p and suppress its regulatory function. It has been demonstrated that ZIC1 is a direct target of miR-541-5p, as miR-541-5p expression correlated negatively with ZIC1 expression. Evidence has demonstrated that ZIC1 downregulation led to the significantly enhancement in β-catenin, cyclin D1, and c-Myc levels. In addition, an in vivo model, intratumoral injection of a miR-541-5p inhibitor reduced tumor size. As a result, the circMTO1/miR-541-5p/ZIC1 axis may have therapeutic potential for HCC by suppressing HCC cell growth, invasion, and metastasis through regulation of the Wnt/β-catenin signaling pathway (72). CircMTO1 is also a molecular sponge for miR-9-5p and it has been shown that overexpression of circMTO1 significantly reduced miR-9-5p expression. MiR-9-5p downregulation contributed to the upregulation of NOX4, a protein involved in the hepatocytes proliferation during physiological condition and tumorigenesis (73). Downregulation of NOX4 is associated with the tumorigenic potential and HCC formation (117, 118) and the activation of circMTO1/miR-9 5p/NOX4 axis promoted the apoptosis of HCC cells (73).
6 Wnt-related circRNA, a potential biomarker for cancer prognosis and treatment
Clinicians have believed that treatment choices require early prognostic data. A growing body of evidence suggests that Wnt-related circRNAs could be valuable for prognosis (60). In several malignancies, these circRNAs have a strong correlation with 5-year overall survival, disease-, recurrence-, and progression-free survival. According to Li et al., there was a negative correlation between the overexpression of circCCT3 and the colorectal cancer patient’s chance of disease-free survival (119). It has also been demonstrated that lower recurrence-free survival is associated with reduced circZKSCAN1 expression in HCC patients (70). Furthermore, increased level of circ_0109046 in endometrial cancer patients can predict a worse 5-year overall survival (120).Based on studies, it has been determined that circ_0067934 and circZFR are potential prognostic biomarkers in patients with HCC (63, 106). Such research has crucial consequences for determining prognosis and selecting treatments. Wnt-related circRNAs are also linked to other important prognostic variables. For instance, low levels of circMTO1 can predict late TNM stage and lymphatic metastases in colorectal cancer (CRC) (121). Thus, targeting Wnt-related circRNA expression could be a beneficial cancer therapeutic strategy. The most circRNAs function as miRNA sponges, activating or inactivating the Wnt pathway. It is also possible to control the target miRNAs of Wnt-related circRNAs. In CRC, for example, injection of MiR-582 significantly reversed the cellular mechanisms governed by circ_0009361 (122). CircRNA_NEK6 acts as a sponge for miR-370-3p, stimulating the FZD8/Wnt axis to promote the development of thyroid carcinoma (123). Circ_0121582 acts as a sponge of miR-224 in AML to overexpress GSK3β and trigger the Wnt/β-catenin pathway (124). Circ-SFMBT2 regulates the miR-1276/CTNNB1/Wnt/β-catenin axis, contributing to gastric cancer formation and progression. Knocking down circ_SMAD4 could inhibit the development of gastric cancer by decreasing cell proliferation (125). In addition, circ-ZNF124 silence has been shown to suppress NSCLC phenotypes (125). However, the current challenge is to identify targeted therapeutics capable of continually modulating circRNA expression and delivering its impact. This necessitates an in-depth knowledge of the biology and role of Wnt-related circRNAs.
7 Conclusions and future perspectives
NcRNAs are play a promising role in regulating different types of cancers includes HCC through the regulation of gene expression and modulation of biological pathways. In this context, targeting ncRNAs, include circRNAs, represents a novel method in cancer treatment and control drug resistance with advantages compared to conventional therapeutic strategies, such as specificity and potential reduction in toxicity (126, 127). For instance, Yi Li et al. proposed a potential approach to target circ_0000098 in HCC through a targeted drug delivery strategy using platelet-coated particles entrapped with doxorubicin and short hairpin RNA against circ_0000098. This was injected into mouse HCC models, and the results demonstrated remarkable sensitivity of HCC cells to doxorubicin and a reduction in drug resistance (127).
The Wnt signaling pathway is required for several physiological processes, including cell differentiation, motility, expansion, and muscular tissue formation. Both circRNA and the Wnt signaling pathway play important roles in cancer initiation and development. Developing evidence suggests that the circRNA/Wnt axis regulates tumor advancement, invasion, and metastasis by modulating the expression of cancer-associated genes. CircRNAs that are involved in the Wnt pathway typically perform their function by sponging miRNAs, which can be used as diagnostic markers. Wnt-related circRNAs may potentially be useful as biomarkers in the therapy of HCC. To manage tumor growth, researchers are attempting to either upregulate tumor-promoting circRNAs or downregulate tumor-suppressor circRNAs. Further research is needed to confirm the interactions and related mechanisms between suppressive miRNAs and corresponding circRNAs involved in the Wnt pathway for the diagnosis, prognosis, and treatment of HCC.
Author contributions
The authors confirm contributions to this paper as follows,
AM, conceptualization, investigation, database searching, writing, and review manuscript. FZ, ST, HR, MM, YG, NH, SH, SG, RM, BG, and ZA, data collection, writing, draft preparation, and figure design. MA and HM contributed in the project administration, editing, validation, coordinated the study, and accurately evaluated the manuscript. All authors contributed to the article and approved the submitted version.
Conflict of interest
The authors declare that the research was conducted in the absence of any commercial or financial relationships that could be construed as a potential conflict of interest.
Publisher’s note
All claims expressed in this article are solely those of the authors and do not necessarily represent those of their affiliated organizations, or those of the publisher, the editors and the reviewers. Any product that may be evaluated in this article, or claim that may be made by its manufacturer, is not guaranteed or endorsed by the publisher.
Abbreviations
CircRNAs, Circular RNAs; EMT, Epithelial to mesenchymal transition; HCC, Hepatocellular carcinoma, NcRNAs, Non-coding RNAs; MiRNAs, MicroRNAs; RBP, RNA-binding proteins; GSK-3-beta, glycogen synthase kinase 3-beta; Fzd, Frizzled; LRP, lipoprotein receptor-related protein; DVL, Disheveled; CBP, CREB-binding protein; ROR2, Receptor tyrosine kinase-like orphan receptor 2; VEGF, vascular endothelial growth factor.
References
1. Chang W-T, Bow Y-D, Fu P-J, Li C-Y, Wu C-Y, Chang Y-H, et al. A marine terpenoid, heteronemin, induces both the apoptosis and ferroptosis of hepatocellular carcinoma cells and involves the ROS and MAPK pathways. Oxid Med Cell Longevity (2021) 2021:7689045. doi: 10.1155/2021/7689045
2. Rossi L, Zoratto F, Papa A, Iodice F, Minozzi M, Frati L, et al. Current approach in the treatment of hepatocellular carcinoma. World J Gastrointest Oncol (2010) 2(9):348–59. doi: 10.4251/wjgo.v2.i9.348
3. Moeinabadi-Bidgoli K, Rezaee M, Rismanchi H, Mohammadi MM, Babajani A. Mesenchymal stem cell-derived antimicrobial peptides as potential anti-neoplastic agents: new insight into anticancer mechanisms of stem cells and exosomes. Front Cell Dev Biol (2022) 10:900418. doi: 10.3389/fcell.2022.900418
4. Dasari S, Tchounwou PB. Cisplatin in cancer therapy: molecular mechanisms of action. Eur J Pharmacol (2014) 740:364–78. doi: 10.1016/j.ejphar.2014.07.025
5. Wang W, Huang Z, Guo B, Liu S, Xiao W, Liang J. Short- and long-term outcomes of laparoscopic hepatectomy in elderly patients with hepatocellular carcinoma. J BUON (2018) 23(4):971–8.
6. Grandhi MS, Kim AK, Ronnekleiv-Kelly SM, Kamel IR, Ghasebeh MA, Pawlik TM. Hepatocellular carcinoma: from diagnosis to treatment. Surg Oncol (2016) 25(2):74–85. doi: 10.1016/j.suronc.2016.03.002
7. Chen SH, Wan QS, Zhou D, Wang T, Hu J, He YT, et al. A simple-to-Use nomogram for predicting the survival of early hepatocellular carcinoma patients. Front Oncol (2019) 9:584. doi: 10.3389/fonc.2019.00584
8. Kimura T. [Non-coding natural antisense RNA: mechanisms of action in the regulation of target gene expression and its clinical implications]. Yakugaku Zasshi (2020) 140(5):687–700. doi: 10.1248/yakushi.20-00002
9. Kumar S, Gonzalez EA, Rameshwar P, Etchegaray JP. Non-coding RNAs as mediators of epigenetic changes in malignancies. Cancers (Basel) (2020) 12(12). doi: 10.3390/cancers12123657
10. Mafi A, Keshavarzmotamed A, Hedayati N, Yeganeh Boroujeni Z, Reiter RJ, Mousavi Dehmordi R, et al. Melatonin targeting non-coding RNAs in cancer: focus on mechanisms and potential therapeutic targets. Eur J Pharmacol (2023) 950:175755. doi: 10.1016/j.ejphar.2023.175755
11. Rezaee M, Mohammadi F, Keshavarzmotamed A, Yahyazadeh S, Vakili O, Milasi YE, et al. The landscape of exosomal non-coding RNAs in breast cancer drug resistance, focusing on underlying molecular mechanisms. Front Pharmacol (2023) 14:1152672. doi: 10.3389/fphar.2023.1152672
12. Mafi A, Rahmati A, Babaei Aghdam Z, Salami R, Salami M, Vakili O, et al. Recent insights into the microRNA-dependent modulation of gliomas from pathogenesis to diagnosis and treatment. Cell Mol Biol Lett (2022) 27(1):65. doi: 10.1186/s11658-022-00354-4
13. Chen LL, Yang L. Regulation of circRNA biogenesis. RNA Biol (2015) 12(4):381–8. doi: 10.1080/15476286.2015.1020271
14. Zhang R, Xu J, Zhao J, Wang X. Silencing of hsa_circ_0007534 suppresses proliferation and induces apoptosis in colorectal cancer cells. Eur Rev Med Pharmacol Sci (2018) 22(1):118–26. doi: 10.26355/eurrev_201801_14108
15. Xu Y, Yao Y, Zhong X, Leng K, Qin W, Qu L, et al. Downregulated circular RNA hsa_circ_0001649 regulates proliferation, migration and invasion in cholangiocarcinoma cells. Biochem Biophys Res Commun (2018) 496(2):455–61. doi: 10.1016/j.bbrc.2018.01.077
16. Li B, Xie F, Zheng FX, Jiang GS, Zeng FQ, Xiao XY. Overexpression of CircRNA BCRC4 regulates cell apoptosis and MicroRNA-101/EZH2 signaling in bladder cancer. J Huazhong Univ Sci Technolog Med Sci (2017) 37(6):886–90. doi: 10.1007/s11596-017-1822-9
17. Shi L, Yan P, Liang Y, Sun Y, Shen J, Zhou S, et al. Circular RNA expression is suppressed by androgen receptor (AR)-regulated adenosine deaminase that acts on RNA (ADAR1) in human hepatocellular carcinoma. Cell Death Dis (2017) 8(11):e3171. doi: 10.1038/cddis.2017.556
18. Dou D, Ren X, Han M, Xu X, Ge X, Gu Y, et al. CircUBE2D2 (hsa_circ_0005728) promotes cell proliferation, metastasis and chemoresistance in triple-negative breast cancer by regulating miR-512-3p/CDCA3 axis. Cancer Cell Int (2020) 20:454. doi: 10.1186/s12935-020-01547-7
19. Panda AC. Circular RNAs act as miRNA sponges. Adv Exp Med Biol (2018) 1087:67–79. doi: 10.1007/978-981-13-1426-1_6
20. Croce JC, McClay DR. Evolution of the wnt pathways. Methods Mol Biol (2008) 469:3–18. doi: 10.1007/978-1-60327-469-2_1
21. Komiya Y, Habas R. Wnt signal transduction pathways. Organogenesis (2008) 4(2):68–75. doi: 10.4161/org.4.2.5851
22. Zhang Y, Wang X. Targeting the wnt/β-catenin signaling pathway in cancer. J Hematol Oncol (2020) 13(1):165. doi: 10.1186/s13045-020-00990-3
23. Vermeulen L, De Sousa EMF, van der Heijden M, Cameron K, de Jong JH, Borovski T, et al. Wnt activity defines colon cancer stem cells and is regulated by the microenvironment. Nat Cell Biol (2010) 12(5):468–76. doi: 10.1038/ncb2048
24. Nejak-Bowen KN, Monga SP. Beta-catenin signaling, liver regeneration and hepatocellular cancer: sorting the good from the bad. Semin Cancer Biol (2011) 21(1):44–58. doi: 10.1016/j.semcancer.2010.12.010
25. Wang F, Nazarali AJ, Ji S. Circular RNAs as potential biomarkers for cancer diagnosis and therapy. Am J Cancer Res (2016) 6(6):1167–76.
26. De Palma FDE, Salvatore F, Pol JG, Kroemer G, Maiuri MC. Circular RNAs as potential biomarkers in breast cancer. Biomedicines (2022) 10(3). doi: 10.3390/biomedicines10030725
27. Kou P, Zhang C, Lin J, Wang H. Circular RNA hsa_circ_0078602 may have potential as a prognostic biomarker for patients with hepatocellular carcinoma. Oncol Lett (2019) 17(2):2091–8. doi: 10.3892/ol.2018.9863
28. Pan H, Tang L, Jiang H, Li X, Wang R, Gao J, et al. Enhanced expression of circ_0000267 in hepatocellular carcinoma indicates poor prognosis and facilitates cell progression by sponging miR-646. J Cell Biochem (2019) 120(7):11350–7. doi: 10.1002/jcb.28411
29. Nayak L, Bhattacharyya NP, De RK. Wnt signal transduction pathways: modules, development and evolution. BMC Syst Biol (2016) 10 Suppl 2(Suppl 2):44. doi: 10.1186/s12918-016-0299-7
30. Taciak B, Pruszynska I, Kiraga L, Bialasek M, Krol M. Wnt signaling pathway in development and cancer. J Physiol Pharmacol (2018) 69(2). doi: 10.26402/jpp.2018.2.07
31. Wen X, Wu Y, Awadasseid A, Tanaka Y, Zhang W. New advances in canonical wnt/β-catenin signaling in cancer. Cancer Manage Res (2020) 12:6987. doi: 10.2147/CMAR.S258645
32. Liu J, Xiao Q, Xiao J, Niu C, Li Y, Zhang X, et al. Wnt/β-catenin signalling: function, biological mechanisms, and therapeutic opportunities. Signal Transduct Target Ther (2022) 7(1):3. doi: 10.1038/s41392-021-00762-6
33. You H, Li Q, Kong D, Liu X, Kong F, Zheng K, et al. The interaction of canonical wnt/β-catenin signaling with protein lysine acetylation. Cell Mol Biol Letters (2022) 27(1):7. doi: 10.1186/s11658-021-00305-5
34. Min JK, Park H-S, Lee Y-B, Kim J-G, Kim J-I, Park J-B. Cross-talk between wnt signaling and src tyrosine kinase. Biomedicines (2022) 10(5):1112. doi: 10.3390/biomedicines10051112
35. Qin K, Yu M, Fan J, Wang H, Zhao P, Zhao G, et al. Canonical and noncanonical wnt signaling: a comprehensive review of multilayered mediators, signaling mechanisms and crosstalk with major signaling pathways. Genes Diseases (2023). doi: 10.1016/j.gendis.2023.01.030
36. Hadi F, Akrami H, Shahpasand K, Fattahi MR. Wnt signalling pathway and tau phosphorylation: a comprehensive study on known connections. Cell Biochem Funct (2020) 38(6):686–94. doi: 10.1002/cbf.3530
37. Li X, Ortiz MA, Kotula L. The physiological role of wnt pathway in normal development and cancer. Exp Biol Med (2020) 245(5):411–26. doi: 10.1177/1535370220901683
38. Rapp J, Jaromi L, Kvell K, Miskei G, Pongracz JE. WNT signaling–lung cancer is no exception. Respir Res (2017) 18:1–16. doi: 10.1186/s12931-017-0650-6
39. Venkatesh T, Thankachan S, Kabekkodu SP, Chakraborti S, Suresh PS. Emerging patterns and implications of breast cancer epigenetics: an update of the current knowledge. Epigenet Reprod Health: Elsevier (2021) p:295–324. doi: 10.1016/B978-0-12-819753-0.00015-5
40. Wang Y, Huang S. Circular RNA: a novel noncoding RNA in cancer. Cancer noncoding RNAs: Elsevier (2018), 187–202. doi: 10.1016/B978-0-12-811022-5.00011-5
41. Mafi A, Yadegar N, Salami M, Salami R, Vakili O, Aghadavod E. Circular RNAs; powerful microRNA sponges to overcome diabetic nephropathy. Pathol Res Pract (2021) 227:153618. doi: 10.1016/j.prp.2021.153618
42. Greene J, Baird AM, Brady L, Lim M, Gray SG, McDermott R, et al. Circular RNAs: biogenesis, function and role in human diseases. Front Mol biosciences (2017) 4:38. doi: 10.3389/fmolb.2017.00038
43. Wang M, Yu F, Li P. Circular RNAs: characteristics, function and clinical significance in hepatocellular carcinoma. Cancers (Basel) (2018) 10(8). doi: 10.3390/cancers10080258
44. Jeck WR, Sorrentino JA, Wang K, Slevin MK, Burd CE, Liu J, et al. Circular RNAs are abundant, conserved, and associated with ALU repeats. RNA (New York NY) (2013) 19(2):141–57. doi: 10.1261/rna.035667.112
45. Vakili O, Asili P, Babaei Z, Mirahmad M, Keshavarzmotamed A, Asemi Z, et al. Circular RNAs in alzheimer's disease: a new perspective of diagnostic and therapeutic targets. CNS Neurol Disord Drug Targets (2022) 22(9):1335–1354. doi: 10.2174/1871527321666220829164211
46. Li Z, Huang C, Bao C, Chen L, Lin M, Wang X, et al. Exon-intron circular RNAs regulate transcription in the nucleus. Nat Struct Mol Biol (2015) 22(3):256–64. doi: 10.1038/nsmb.2959
47. Kristensen LS, Andersen MS, Stagsted LVW, Ebbesen KK, Hansen TB, Kjems J. The biogenesis, biology and characterization of circular RNAs. Nat Rev Genet (2019) 20(11):675–91. doi: 10.1038/s41576-019-0158-7
48. Zhao X, Cai Y, Xu J. Circular RNAs: biogenesis, mechanism, and function in human cancers. Int J Mol Sci (2019) 20(16). doi: 10.3390/ijms20163926
49. Zhang G, Hou J, Mei C, Wang X, Wang Y, Wang K. Effect of circular RNAs and N6-methyladenosine (m6A) modification on cancer biology. Biomed Pharmacother (2023) 159:114260. doi: 10.1016/j.biopha.2023.114260
50. Ma Y, Zheng L, Gao Y, Zhang W, Zhang Q, Xu Y. A comprehensive overview of circRNAs: emerging biomarkers and potential therapeutics in gynecological cancers. Front Cell Dev Biol (2021) 9:709512. doi: 10.3389/fcell.2021.709512
51. Rybak-Wolf A, Stottmeister C, Glažar P, Jens M, Pino N, Giusti S, et al. Circular RNAs in the mammalian brain are highly abundant, conserved, and dynamically expressed. Mol Cell (2015) 58(5):870–85. doi: 10.1016/j.molcel.2015.03.027
52. Xia X, Tang X, Wang S. Roles of CircRNAs in autoimmune diseases. Front Immunol (2019) 10:639. doi: 10.3389/fimmu.2019.00639
53. Ahn KS, O’Brien DR, Kim YH, Kim T-S, Yamada H, Park J-W, et al. Associations of serum tumor biomarkers with integrated genomic and clinical characteristics of hepatocellular carcinoma. Liver Cancer (2021) 10(6):593–605. doi: 10.1159/000516957
54. Xiong D, He R, Dang Y, Wu H, Feng Z, Chen G. The latest overview of circRNA in the progression, diagnosis, prognosis, treatment, and drug resistance of hepatocellular carcinoma. Front Oncol (2021) 10:608257. doi: 10.3389/fonc.2020.608257
55. Shang X, Li G, Liu H, Li T, Liu J, Zhao Q, et al. Comprehensive circular RNA profiling reveals that hsa_circ_0005075, a new circular RNA biomarker, is involved in hepatocellular crcinoma development. Medicine. (2016) 95(22):e3811. doi: 10.1097/MD.0000000000003811
56. Qin M, Liu G, Huo X, Tao X, Sun X, Ge Z, et al. Hsa_circ_0001649: a circular RNA and potential novel biomarker for hepatocellular carcinoma. Cancer Biomarkers (2016) 16(1):161–9. doi: 10.3233/CBM-150552
57. Xu L, Zhang M, Zheng X, Yi P, Lan C, Xu M. The circular RNA ciRS-7 (Cdr1as) acts as a risk factor of hepatic microvascular invasion in hepatocellular carcinoma. J Cancer Res Clin Oncol (2017) 143:17–27. doi: 10.1007/s00432-016-2256-7
58. Li D, Zhang J, Li J. Role of miRNA sponges in hepatocellular carcinoma. Clinica Chimica Acta (2020) 500:10–9. doi: 10.1016/j.cca.2019.09.013
59. Hu J, Li P, Song Y, Yx Ge, Xm M, Huang C, et al. Progress and prospects of circular RNAs in hepatocellular carcinoma: novel insights into their function. J Cell Physiol (2018) 233(6):4408–22. doi: 10.1002/jcp.26154
60. Xue C, Li G, Zheng Q, Gu X, Bao Z, Lu J, et al. The functional roles of the circRNA/Wnt axis in cancer. Mol Cancer (2022) 21(1):1–24. doi: 10.1186/s12943-022-01582-0
61. Singh D, Kesharwani P, Alhakamy NA, Siddique HR. Accentuating CircRNA-miRNA-transcription factors axis: a conundrum in cancer research. Front Pharmacol (2022) 12:784801. doi: 10.3389/fphar.2021.784801
62. Liu X, Yang L, Jiang D, Lu W, Zhang Y. Circ-DENND4C up-regulates TCF4 expression to modulate hepatocellular carcinoma cell proliferation and apoptosis via activating wnt/β-catenin signal pathway. Cancer Cell Int (2020) 20:295. doi: 10.1186/s12935-020-01346-0
63. Zhu Q, Lu G, Luo Z, Gui F, Wu J, Zhang D, et al. CircRNA circ_0067934 promotes tumor growth and metastasis in hepatocellular carcinoma through regulation of miR-1324/FZD5/Wnt/β-catenin axis. Biochem Biophys Res Commun (2018) 497(2):626–32. doi: 10.1016/j.bbrc.2018.02.119
64. Zhu P, Liang H, Huang X, Zeng Q, Liu Y, Lv J, et al. Circular RNA Hsa_circ_0004018 inhibits wnt/β-catenin signaling pathway by targeting microRNA-626/DKK3 in hepatocellular carcinoma. Onco Targets Ther (2020) 13:9351–64. doi: 10.2147/OTT.S254997
65. Huang G, Liang M, Liu H, Huang J, Li P, Wang C, et al. CircRNA hsa_circRNA_104348 promotes hepatocellular carcinoma progression through modulating miR-187-3p/RTKN2 axis and activating wnt/β-catenin pathway. Cell Death Dis (2020) 11(12):1065. doi: 10.1038/s41419-020-03276-1
66. Xu J, Wan Z, Tang M, Lin Z, Jiang S, Ji L, et al. N(6)-methyladenosine-modified CircRNA-SORE sustains sorafenib resistance in hepatocellular carcinoma by regulating β-catenin signaling. Mol Cancer (2020) 19(1):163. doi: 10.1186/s12943-020-01281-8
67. Tan A, Li Q, Chen L. CircZFR promotes hepatocellular carcinoma progression through regulating miR-3619–5p/CTNNB1 axis and activating wnt/β-catenin pathway. Arch Biochem biophysics (2019) 661:196–202. doi: 10.1016/j.abb.2018.11.020
68. Yang B, Zhao J, Huo T, Zhang M, Wu X. Effects of CircRNA-ITCH on proliferation and apoptosis of hepatocellular carcinoma cells through inhibiting wnt/β-catenin signaling pathway. J buon (2020) 25(3):1368–74.
69. Liang WC, Wong CW, Liang PP, Shi M, Cao Y, Rao ST, et al. Translation of the circular RNA circβ-catenin promotes liver cancer cell growth through activation of the wnt pathway. Genome Biol (2019) 20(1):84. doi: 10.1186/s13059-019-1685-4
70. Zhu YJ, Zheng B, Luo GJ, Ma XK, Lu XY, Lin XM, et al. Circular RNAs negatively regulate cancer stem cells by physically binding FMRP against CCAR1 complex in hepatocellular carcinoma. Theranostics (2019) 9(12):3526–40. doi: 10.7150/thno.32796
71. Chen CH, Su YJ, Ding H, Duan J, Wang J. Circular RNA ZNF292 affects proliferation and apoptosis of hepatocellular carcinoma cells by regulating wnt/β-catenin pathway. Eur Rev Med Pharmacol Sci (2020) 24(23):12124–30. doi: 10.26355/eurrev_202012_24001
72. Li D, Zhang J, Yang J, Wang J, Zhang R, Li J, et al. CircMTO1 suppresses hepatocellular carcinoma progression via the miR-541-5p/ZIC1 axis by regulating wnt/β-catenin signaling pathway and epithelial-to-mesenchymal transition. Cell Death Dis (2021) 13(1):12. doi: 10.1038/s41419-021-04464-3
73. Wang J, Tan Q, Wang W, Yu J. Mechanism of the regulatory effect of overexpression of circMTO1 on proliferation and apoptosis of hepatoma cells via miR-9-5p/NOX4 axis. Cancer Manag Res (2020) 12:3915–25. doi: 10.2147/CMAR.S240719
74. Wang H, Yan X, Zhang H, Zhan X. CircRNA circ_0067934 overexpression correlates with poor prognosis and promotes thyroid carcinoma progression. Med Sci Monit (2019) 25:1342–9. doi: 10.12659/MSM.913463
75. Michlewski G, Cáceres JF. Post-transcriptional control of miRNA biogenesis. RNA (New York NY) (2019) 25(1):1–16. doi: 10.1261/rna.068692.118
76. Liu X, Qin J, Gao T, Li C, He B, Pan B, et al. YTHDF1 facilitates the progression of hepatocellular carcinoma by promoting FZD5 mRNA translation in an m6A-dependent manner. Mol Ther Nucleic Acids (2020) 22:750–65. doi: 10.1016/j.omtn.2020.09.036
77. Hu Z, Wang P, Lin J, Zheng X, Yang F, Zhang G, et al. MicroRNA-197 promotes metastasis of hepatocellular carcinoma by activating wnt/β-catenin signaling. Cell Physiol Biochem (2018) 51(1):470–86. doi: 10.1159/000495242
78. Debebe A, Medina V, Chen CY, Mahajan IM, Jia C, Fu D, et al. Wnt/β-catenin activation and macrophage induction during liver cancer development following steatosis. Oncogene (2017) 36(43):6020–9. doi: 10.1038/onc.2017.207
79. Chen H, Liu S, Li M, Huang P, Li X. circ_0003418 inhibits tumorigenesis and cisplatin chemoresistance through wnt/β-catenin pathway in hepatocellular carcinoma. Onco Targets Ther (2019) 12:9539–49. doi: 10.2147/OTT.S229507
80. Liao R, Liu L, Zhou J, Wei X, Huang P. Current molecular biology and therapeutic strategy status and prospects for circRNAs in HBV-associated hepatocellular carcinoma. Front Oncol (2021) 11. doi: 10.3389/fonc.2021.697747
81. Peng Y, Wang HH. Cir-ITCH inhibits gastric cancer migration, invasion and proliferation by regulating the wnt/β-catenin pathway. Sci Rep (2020) 10(1):1–13. doi: 10.1038/s41598-020-74452-8
82. Wei W, Li M, Wang J, Nie F, Li L. The E3 ubiquitin ligase ITCH negatively regulates canonical wnt signaling by targeting dishevelled protein. Mol Cell Biol (2012) 32(19):3903–12. doi: 10.1128/MCB.00251-12
83. Li F, Zhang L, Li W, Deng J, Zheng J, An M, et al. Circular RNA ITCH has inhibitory effect on ESCC by suppressing the wnt/β-catenin pathway. Oncotarget (2015) 6(8):6001–13. doi: 10.18632/oncotarget.3469
84. Wan L, Zhang L, Fan K, Cheng ZX, Sun QC, Wang JJ. Circular RNA-ITCH suppresses lung cancer proliferation via inhibiting the wnt/β-catenin pathway. BioMed Res Int (2016) 2016:1579490. doi: 10.1155/2016/1579490
85. Wang ST, Liu LB, Li XM, Wang YF, Xie PJ, Li Q, et al. Circ-ITCH regulates triple-negative breast cancer progression through the Wnt/β-catenin pathway. Neoplasma (2019) 66(2):232–239. doi: 10.4149/neo_2018_180710N460
86. Guo W, Zhang J, Zhang D, Cao S, Li G, Zhang S, et al. Polymorphisms and expression pattern of circular RNA circ-ITCH contributes to the carcinogenesis of hepatocellular carcinoma. Oncotarget (2017) 8(29):48169–77. doi: 10.18632/oncotarget.18327
87. Wu P, Gao Y, Shen S, Xue Y, Liu X, Ruan X, et al. KHDRBS3 regulates the permeability of blood-tumor barrier via cDENND4C/miR-577 axis. Cell Death Dis (2019) 10(7):536. doi: 10.1038/s41419-019-1771-2
88. Liang G, Liu Z, Tan L, Su AN, Jiang WG, Gong C. HIF1α-associated circDENND4C promotes proliferation of breast cancer cells in hypoxic environment. Anticancer Res (2017) 37(8):4337–43. doi: 10.21873/anticanres.11827
89. Luo X, O'Neill KL, Huang K. The third model of Bax/Bak activation: a bcl-2 family feud finally resolved? F1000Res (2020) 9. doi: 10.12688/f1000research.25607.1
90. Fu L, Yao T, Chen Q, Mo X, Hu Y, Guo J. Screening differential circular RNA expression profiles reveals hsa_circ_0004018 is associated with hepatocellular carcinoma. Oncotarget (2017) 8(35):58405. doi: 10.18632/oncotarget.16881
91. Wilde L, Roche M, Domingo-Vidal M, Tanson K, Philp N, Curry J, et al. Metabolic coupling and the reverse warburg effect in cancer: implications for novel biomarker and anticancer agent development. Semin Oncol (2017) 44(3):198–203. doi: 10.1053/j.seminoncol.2017.10.004
92. Adrian LH. Hypoxia–a key regulatory factor in tumour growth. Nat Rev Cancer (2002) 2(1):38–47. doi: 10.1038/nrc704
93. Boeckel J-N, Jaé N, Heumüller AW, Chen W, Boon RA, Stellos K, et al. Identification and characterization of hypoxia-regulated endothelial circular RNA. Circ Res (2015) 117(10):884–90. doi: 10.1161/CIRCRESAHA.115.306319
94. Yang W, Liu Y, Gao R, Xiu Z, Sun T. Knockdown of cZNF292 suppressed hypoxic human hepatoma SMMC7721 cell proliferation, vasculogenic mimicry, and radioresistance. Cell Signal (2019) 60:122–35. doi: 10.1016/j.cellsig.2019.04.011
95. Qi L, Song W, Liu Z, Zhao X, Cao W, Sun B. Wnt3a promotes the vasculogenic mimicry formation of colon cancer via wnt/β-catenin signaling. Int J Mol Sci (2015) 16(8):18564–79. doi: 10.3390/ijms160818564
96. Qi L, Sun B, Liu Z, Cheng R, Li Y, Zhao X. Wnt3a expression is associated with epithelial-mesenchymal transition and promotes colon cancer progression. J Exp Clin Cancer Res (2014) 33(1):1–11. doi: 10.1186/s13046-014-0107-4
97. Collier FM, Loving A, Baker AJ, McLeod J, Walder K, Kirkland MA. RTKN2 induces NF-KappaB dependent resistance to intrinsic apoptosis in HEK cells and regulates BCL-2 genes in human CD4(+) lymphocytes. J Cell Death (2009) 2:9–23. doi: 10.4137/JCD.S2891
98. Lin Z, Li D, Cheng W, Wu J, Wang K, Hu Y. MicroRNA-181 functions as an antioncogene and mediates NF-κB pathway by targeting RTKN2 in ovarian cancers. Reprod Sci (2019) 26(8):1071–81. doi: 10.1177/1933719118805865
99. Liao YX, Zeng JM, Zhou JJ, Yang GH, Ding K, Zhang XJ. Silencing of RTKN2 by siRNA suppresses proliferation, and induces G1 arrest and apoptosis in human bladder cancer cells. Mol Med Rep (2016) 13(6):4872–8. doi: 10.3892/mmr.2016.5127
100. Wei W, Chen H, Liu S. Knockdown of rhotekin 2 expression suppresses proliferation and invasion and induces apoptosis in hepatocellular carcinoma cells. Mol Med Rep (2016) 13(6):4865–71. doi: 10.3892/mmr.2016.5113
101. Liu L, Cao Y, Chen C, Zhang X, McNabola A, Wilkie D, et al. Sorafenib blocks the RAF/MEK/ERK pathway, inhibits tumor angiogenesis, and induces tumor cell apoptosis in hepatocellular carcinoma model PLC/PRF/5. Cancer Res (2006) 66(24):11851–8. doi: 10.1158/0008-5472.CAN-06-1377
102. Yang X, Liu L, Zou H, Zheng Y-W, Wang K-P. circZFR promotes cell proliferation and migration by regulating miR-511/AKT1 axis in hepatocellular carcinoma. Digestive Liver Disease (2019) 51(10):1446–55. doi: 10.1016/j.dld.2019.04.012
103. Luo L, Miao P, Ming Y, Tao J, Shen H. Circ-ZFR promotes progression of bladder cancer by upregulating WNT5A via sponging miR-545 and miR-1270. Front Oncol (2021) 10:596623. doi: 10.3389/fonc.2020.596623
104. Chen Z, Wang F, Xiong Y, Wang N, Gu Y, Qiu X. CircZFR functions as a sponge of miR-578 to promote breast cancer progression by regulating HIF1A expression. Cancer Cell Int (2020) 20(1):1–13. doi: 10.1186/s12935-020-01492-5
105. Wei H, Pan L, Tao D, Li R. Circular RNA circZFR contributes to papillary thyroid cancer cell proliferation and invasion by sponging miR-1261 and facilitating C8orf4 expression. Biochem Biophys Res Commun (2018) 503(1):56–61. doi: 10.1016/j.bbrc.2018.05.174
106. Tan A, Li Q, Chen L. CircZFR promotes hepatocellular carcinoma progression through regulating miR-3619-5p/CTNNB1 axis and activating wnt/β-catenin pathway. Arch Biochem Biophys (2019) 661:196–202. doi: 10.1016/j.abb.2018.11.020
107. Vecchio E, Nisticò N, Golino G, Iaccino E, Maisano D, Mimmi S, et al. IBtkα activates the β-Catenin-Dependent transcription of MYC through ubiquitylation and proteasomal degradation of GSK3β in cancerous b cells. Int J Mol Sci (2022) 23(4). doi: 10.3390/ijms23042044
108. Xing Z, Zeng M, Hu H, Zhang H, Hao Z, Long Y, et al. Fragile X mental retardation protein promotes astrocytoma proliferation via the MEK/ERK signaling pathway. Oncotarget (2016) 7(46):75394. doi: 10.18632/oncotarget.12215
109. Chang T-S, Wei K-L, Lu C-K, Chen Y-H, Cheng Y-T, Tung S-Y, et al. Inhibition of CCAR1, a coactivator of β-catenin, suppresses the proliferation and migration of gastric cancer cells. Int J Mol Sci (2017) 18(2):460. doi: 10.3390/ijms18020460
110. Rishi AK, Zhang L, Boyanapalli M, Wali A, Mohammad RM, Yu Y, et al. Identification and characterization of a cell cycle and apoptosis regulatory protein-1 as a novel mediator of apoptosis signaling by retinoid CD437. J Biol Chem (2003) 278(35):33422–35. doi: 10.1074/jbc.M303173200
111. Ou C-Y, Kim JH, Yang CK, Stallcup MR. Requirement of cell cycle and apoptosis regulator 1 for target gene activation by wnt and β-catenin and for anchorage-independent growth of human colon carcinoma cells. J Biol Chem (2009) 284(31):20629–37. doi: 10.1074/jbc.M109.014332
112. Ha SY, Kim JH, Yang JW, Kim J, Kim B, Park C-K. The overexpression of CCAR1 in hepatocellular carcinoma associates with poor prognosis. Cancer Res treatment: Off J Korean Cancer Assoc (2016) 48(3):1065–73. doi: 10.4143/crt.2015.302
113. Ghafouri-Fard S, Khoshbakht T, Bahranian A, Taheri M, Hallajnejad M. CircMTO1: a circular RNA with roles in the carcinogenesis. Biomedicine pharmacotherapy = Biomedecine pharmacotherapie (2021) 142:112025. doi: 10.1016/j.biopha.2021.112025
114. Zhang X, Zhong B, Zhang W, Wu J, Wang Y. Circular RNA CircMTO1 inhibits proliferation of glioblastoma cells via miR-92/WWOX signaling pathway. Med Sci Monit (2019) 25:6454–61. doi: 10.12659/MSM.918676
115. Wang X, Lin YK, Lu ZL, Li J. Circular RNA circ-MTO1 serves as a novel potential diagnostic and prognostic biomarker for gallbladder cancer. Eur Rev Med Pharmacol Sci (2020) 24(16):8359–66. doi: 10.26355/eurrev_202008_22632
116. Zhang B, Chen M, Jiang N, Shi K, Qian R. A regulatory circuit of circ-MTO1/miR-17/QKI-5 inhibits the proliferation of lung adenocarcinoma. Cancer Biol Ther (2019) 20(8):1127–35. doi: 10.1080/15384047.2019.1598762
117. Crosas-Molist E, Bertran E, Sancho P, López-Luque J, Fernando J, Sánchez A, et al. The NADPH oxidase NOX4 inhibits hepatocyte proliferation and liver cancer progression. Free Radic Biol Med (2014) 69:338–47. doi: 10.1016/j.freeradbiomed.2014.01.040
118. Ha SY, Paik YH, Yang JW, Lee MJ, Bae H, Park CK. NADPH oxidase 1 and NADPH oxidase 4 have opposite prognostic effects for patients with hepatocellular carcinoma after hepatectomy. Gut Liver (2016) 10(5):826–35. doi: 10.5009/gnl15543
119. Li W, Xu Y, Wang X, Cao G, Bu W, Wang X, et al. circCCT3 modulates vascular endothelial growth factor a and wnt signaling to enhance colorectal cancer metastasis through sponging miR-613. DNA Cell Biol (2020) 39(1):118–25. doi: 10.1089/dna.2019.5139
120. Li Y, Liu J, Piao J, Ou J, Zhu X. Circ_0109046 promotes the malignancy of endometrial carcinoma cells through the microRNA-105/SOX9/Wnt/β-catenin axis. IUBMB Life (2021) 73(1):159–76. doi: 10.1002/iub.2415
121. Ge Z, Li L, Wang C, Wang Y, Ma W. CircMTO1 inhibits cell proliferation and invasion by regulating wnt/beta-catenin signaling pathway in colorectal cancer. Eur Rev Med Pharmacol Sci (2018) 22(23):8203–9. doi: 10.26355/eurrev_201812_16513
122. Geng Y, Zheng X, Hu W, Wang Q, Xu Y, He W, et al. Hsa_circ_0009361 acts as the sponge of miR-582 to suppress colorectal cancer progression by regulating APC2 expression. Clin Science (2019) 133(10):1197–213. doi: 10.1042/CS20190286
123. Chen F, Feng Z, Zhu J, Liu P, Yang C, Huang R, et al. Emerging roles of circRNA_NEK6 targeting miR-370-3p in the proliferation and invasion of thyroid cancer via wnt signaling pathway. Cancer Biol Ther (2018) 19(12):1139–52. doi: 10.1080/15384047.2018.1480888
124. Chen J, Lei P, Zhou M. hsa_circ_0121582 inhibits leukemia growth by dampening wnt/β-catenin signaling. Clin Trans Oncol (2020) 22:2293–302. doi: 10.1007/s12094-020-02377-9
125. Wang L, Li B, Yi X, Xiao X, Zheng Q, Ma L. Circ_SMAD4 promotes gastric carcinogenesis by activating wnt/β-catenin pathway. Cell Proliferation (2021) 54(3):e12981. doi: 10.1111/cpr.12981
126. Yaghoobi A, Nazerian Y, Meymand AZ, Ansari A, Nazerian A, Niknejad H. Hypoxia-sensitive miRNA regulation via CRISPR/dCas9 loaded in hybrid exosomes: a novel strategy to improve embryo implantation and prevent placental insufficiency during pregnancy. Front Cell Dev Biol (2022) 10:1082657. doi: 10.3389/fcell.2022.1082657
Keywords: Wnt signaling, circRNA, hepatocellular carcinoma, mechanism, non-coding RNA
Citation: Mafi A, Rismanchi H, Malek Mohammadi M, Hedayati N, Ghorbanhosseini SS, Hosseini SA, Gholinezhad Y, Mousavi Dehmordi R, Ghezelbash B, Zarepour F, Taghavi SP, Asemi Z, Alimohammadi M and Mirzaei H (2023) A spotlight on the interplay between Wnt/β-catenin signaling and circular RNAs in hepatocellular carcinoma progression. Front. Oncol. 13:1224138. doi: 10.3389/fonc.2023.1224138
Received: 17 May 2023; Accepted: 15 June 2023;
Published: 21 July 2023.
Edited by:
Tatsuo Shimura, Maki Hospital, JapanReviewed by:
Jianye Cai, Third Affiliated Hospital of Sun Yat-sen University, ChinaYuen Gao, Michigan State University, United States
Copyright © 2023 Mafi, Rismanchi, Malek Mohammadi, Hedayati, Ghorbanhosseini, Hosseini, Gholinezhad, Mousavi Dehmordi, Ghezelbash, Zarepour, Taghavi, Asemi, Alimohammadi and Mirzaei. This is an open-access article distributed under the terms of the Creative Commons Attribution License (CC BY). The use, distribution or reproduction in other forums is permitted, provided the original author(s) and the copyright owner(s) are credited and that the original publication in this journal is cited, in accordance with accepted academic practice. No use, distribution or reproduction is permitted which does not comply with these terms.
*Correspondence: Mina Alimohammadi, bWluYS5hbGltb2hhbW1hZGlAc2JtdS5hYy5pcg==; bWluYS5hbGltb2hhbW1hZGkxMUBnbWFpbC5jb20=; Hamed Mirzaei, aC5taXJ6YWVpMjAwMkBnbWFpbC5jb20=; Zatollah Asemi, QXNlbWlfckB5YWhvby5jb20=
†These authors have contributed equally to this work and share first authorship
‡ORCID: Zatollah Asemi, orcid.org/0000-0001-5265-4792
Mina Alimohammadi, orcid.org/0000-0002-5953-3894