- Department of Oncology and Radiotherapy, Medical University of Gdańsk, Gdańsk, Poland
Breast cancer brain metastasis (BCBM) has a devastating impact on patient survival, cognitive function and quality of life. Radiotherapy remains the standard management of BM but may result in considerable neurotoxicity. Herein, we describe the current knowledge on methods for reducing radiation-induced cognitive dysfunction in patients with BCBM. A better understanding of the biology and molecular underpinnings of BCBM, as well as more sophisticated prognostic models and individualized treatment approaches, have appeared to enable more effective neuroprotection. The therapeutic armamentarium has expanded from surgery and whole-brain radiotherapy to stereotactic radiosurgery, targeted therapies and immunotherapies, used sequentially or in combination. Advances in neuroimaging have allowed more accurate screening for intracranial metastases, precise targeting of intracranial lesions and the differentiation of the effects of treatment from disease progression. The availability of numerous treatment options for patients with BCBM and multidisciplinary approaches have led to personalized treatment and improved therapeutic outcomes. Ongoing studies may define the optimal sequencing of available and emerging treatment options for patients with BCBM.
Simple summary
Up to 10-30% of breast cancer patients will develop metastases to the brain or meninges. Radiotherapy remains one of the main treatments for intracranial metastases. Although radiotherapy prolongs the survival of breast cancer patients, it is associated with considerable toxicity, which is particularly manifested in cognitive function deterioration. Animal studies suggest that these sequelae result mainly from radiation damage to the hippocampus. Avoiding this structure during brain radiotherapy and its pharmacological protection have been the subjects of multiple trials. Another brain-sparing approach that has emerged in the standard management of countable brain metastases is stereotactic radiotherapy. We present current knowledge on the efficacy and safety of these strategies in breast cancer patients with brain metastases.
1 Introduction
Breast cancer (BC) has surpassed lung cancer as the most commonly diagnosed malignancy among women (1). It is the second most common cause of malignant central nervous system (CNS) dissemination after lung cancer (2). According to the Surveillance, Epidemiology, and End Results Program, 10-30% of patients with BC develop brain or leptomeningeal metastases (BMs), making BMs the most common cause of BC-related deaths (3). New and more effective treatment methods, including anti-HER2 antibodies, CDK4/6 inhibitors, antibody−drug conjugates and immunotherapy, substantially prolong patients’ overall survival (OS), while the availability of precise CNS imaging increases the detection rate of asymptomatic BM (4). Currently, three options in BCBM treatment, i.e., systemic therapy, surgery and radiotherapy (RT), prolong OS by several months, mostly in patients with HER2-positive breast cancer (5). Owing to poor blood−brain barrier (BBB) penetration, chemotherapy has a limited role in the management of BM (6–8), but a better understanding of BM biology has facilitated the development of novel targeted therapies (9). In oligometastatic disease with fewer than five metastatic lesions, surgical excision and/or stereotactic radiosurgery (SRS) or stereotactic radiotherapy (SRT) are the treatments of choice (10–12). In patients with multiple or large BMs and uncontrolled extracranial disease, either whole-brain RT (WBRT) or partial-brain RT (PBRT) is used (13, 14). Although RT has significantly improved the survival of BCBM patients, a relatively large proportion of treated patients will develop radiation-related deterioration of cognitive functions (CFs), including deficits in memory, spatial information processing abilities, and learning difficulties that significantly affect their quality of life (15, 16). The incidence of radiation-induced brain damage is probably underestimated, but the use of novel magnetic resonance imaging (MRI) techniques, such as diffusion-weighted imaging, proton magnetic resonance spectroscopy, and perfusion MRI, has improved the ability to better characterize treatment-related changes. The frequency and severity of cognitive impairment following brain RT are affected by the patient’s age and education, tumor type, RT volume (WBRT, PBRT, SRS, and SRT), RT dose, time elapsed since treatment, definition of neurocognitive impairment, baseline neurocognitive function (NCF), and use of concurrent or previous chemotherapy, targeted agents or immunotherapy (17). We describe the current knowledge on the possibilities of neuroprotection in patients with BCBM receiving radiotherapy.
2 Local treatment for breast cancer brain metastases
For a long time, WBRT has been the standard of care for patients with BM since it improves the median OS from 1 to 6 months compared with best supportive care (7, 18, 19). Clinical studies have shown no difference in OS among various fractionation regimens. Radiosensitizers were also found to fail to improve the prognosis of patients with BM treated with RT (20–22). Therefore, WBRT of 30 Gy in 10 fractions or 20 Gy in 5 fractions has remained the standard of treatment, regardless of BM histology (13, 14, 23–25).
In the case of a single BM from multiple primaries, surgical excision plus WBRT was compared with WBRT alone in randomized trials (26–28), and the results were inconclusive. Combined therapy was shown to be better than WBRT alone (median survival 9-10 months versus 3-6 months) in two studies (26, 27), especially in patients without active systemic disease. Patients with BCBM constituted only 7.5-12% of the studies population and the only histological stratification was the division into patients with NSCLC vs. other sites of cancer and no statistical difference was found. Wroński et. al (29) reported retrospective series of 70 BCBM patients (10% of patients operated due to brain metastases between 1974 and 1993 in their hospitals) treated with neurosurgery with mOS=16.2 months from the time of diagnosis of BM and mOS=14 months from the time of surgery. Among the favorable prognostic factors, the authors mentioned younger age of patients, smaller size of metastases (but not their number) and hormonal status with mOS=21.9 months for ER+ vs. 12.5 months for ER- BC. However, in multivariate analysis, only the use of WBRT after surgery and the absence of meningeal involvement had good prognostic significance. In another retrospective series of 198 patients with BCBM, mOS=14.9 months was also found, but only in the group of 28% of patients with single BM treated surgically or with the use of a gamma knife (SRS), while patients undergoing WBRT had mOS=5.4 months (30). In a more recent series of 53 patients with BCBM from 1994-2010 treated with resection and in 2/3 cases with radiotherapy (SRS-gamma knife, WBRT or both), mOS was 16 months, even though 30% were patients with TNBC and 45% patients with HER2+, probably due to the greater use of effective systemic therapies (31).
On the one hand, the better prognosis of patients with a limited number of BMs has led to interest in more aggressive local treatment. In the RTOG 9508 randomized trial, in patients with 1-3 BMs, a stereotactic boost added to WBRT improved not only the survival of those with a single unresectable brain metastasis (mOS: 6.5 vs. 4.9 months, p=0.0393) but also the functional autonomy of all patients (stable or improved Karnofsky Performance Status [KPS] score at 6 months in 43% vs. 27% in the WBRT group, p=0.03) (32). On the other hand, in the European Organisation for Research and Treatment of Cancer EORTC 22952-26001 study, OS and functionally independent survival were similar, regardless of the addition of WBRT to SRS in the treatment of 1-3 BM (33). These results have changed the paradigm of adding localized treatment of BM to WBRT by using SRS without WBRT, with a single dose of radiation precisely delivered to the BM, maximizing the chance for local control, and sparing the normal brain tissue by omitting WBRT. Although BCBM patients constituted only 12% in the EORTC study, retrospective data confirm very good results of SRS in this group of patients with mOS=15.7 months (34). In the analysis of 91 patients with BCBM who received SRS, a statistically significant prognostic factors were: receptor status, BCBM volume and stable extracranial disease. Patients with ER+/HER2- had mOS=13.8 months, the best results were obtained in patients ER+/HER2+ with mOS=21.4 months and ER-/HER2+ with mOS=20.4 months, and the worst prognosis was reported in patients with TNBC with mOS=8.5 months. These results were better than overall survival reported in other retrospective series of patients with BCBM (35, 36). BCBM volume > 10 cm3 was associated with a worse prognosis with mOS=9.2 months. The authors suggested the possibility of selecting this group of patients for combined treatment with SRS + WBRT, although in the light of the latest knowledge, these patients may also be candidates for FSRT, but the value of both approaches requires validation. Patients with stable extracranial disease undergoing SRS for BCBM had a better prognosis (mOS=20.1 months) compared to patients with progressive extracerebral lesions (mOS=11.4 months). Interestingly, patients without extracranial disease had an mOS of 13.4 months, but this is probably due to the significant proportion of TNBC patients in this group, in whom BMs are often the first manifestation of dissemination and the prognosis remains unfavorable.
In the lack of randomized trials comparing the outcomes of SRS with or without WBRT in BCBM patients, we summarized phase III randomized trials including patients with different numbers (single, up to three, and up to four), sizes and pathological types of BM, as well as resected single BM (Table 1). Most of these studies have shown less cognitive impairment in patients with BM after SRS than after WBRT but at the cost of a higher risk of further intracranial progression. On the other hand, WBRT significantly decreased in-brain recurrences but increased the risk of death due to neurotoxicity in the setting of intact as well as resected BM (37, 38, 40, 41). Knowing that the mere presence of brain metastases causes cognitive disorders in patients with BM (42) and that the use of SRS/FSRT increases the risk of further brain metastases that aggravate these disorders and require further local treatment, the effects of which overlap with existing cognitive problems, it seems that the several months of observations in the cited studies (maximum 6 months) may be insufficient to assess long-term radiation-induced neurotoxicity.
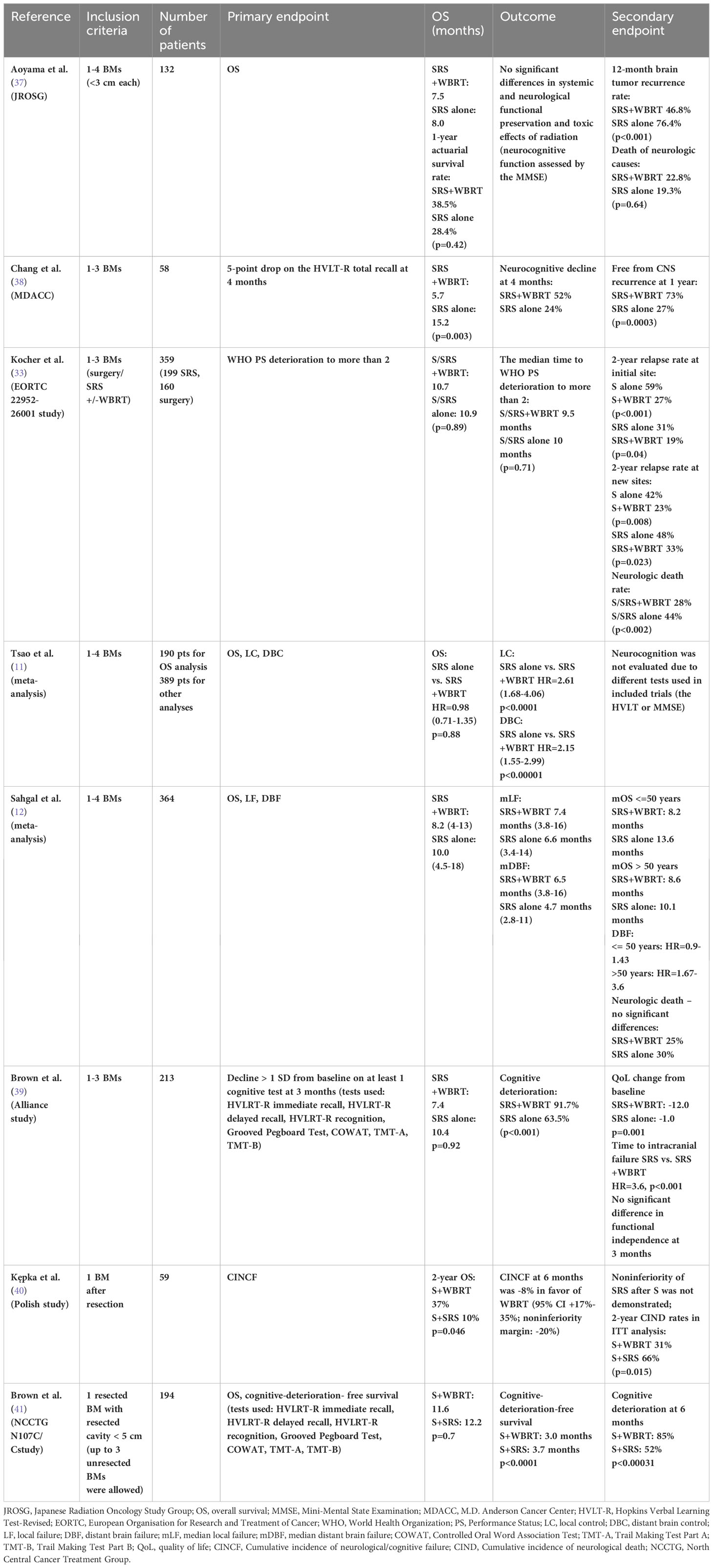
Table 1 Phase III trials and meta-analyses of S/SRS+/-WBRT for the treatment of brain metastases (BMs) with cognitive endpoints.
However, CF was prospectively assessed in only a minority of those trials using various tools and methods, such as the Hopkins Verbal Learning Test-Revised [HVLT-R] Immediate Recall, Delayed Recall and Recognition; Grooved Pegboard Test; Controlled Oral Word Association Test [COWAT]; Trail Making Test Part A [TMT-A]; and/or Trail Making Test Part B [TMT-B]. Owing to the heterogeneity of the patient populations, it is very difficult to compare these results and draw conclusions. The Mini-Mental State Examination (MMSE) showed very low sensitivity to detect RT-induced cognitive impairment (37). Using the Hopkins Verbal Learning Test-Revised (HVLT-R), Chang et al. (38) proved that combined SRS+WBRT vs. SRS alone in patients with 1-3 BMs improved the treatment outcomes at the cost of significant deterioration of learning and memory function as early as at 4 months.
Despite the doubts about SRS/FSRT, the lack of long-term survival benefit of adding WBRT established the role of SRS/FSRT in the treatment of oligometastatic brain metastases, especially in cancers such as breast cancer where metastasectomy/stereotactic radiotherapy of a limited number of metastases to other organs was already standard.
Promising results of SRS in oligometastatic BMs were extrapolated to polymetastatic BM treatment. The noninferiority of stereotactic radiotherapy in 5-10 vs. 2-4 BMs has been demonstrated in a nonrandomized study (HR=0.97, 95% CI, 0.81-1.18; p=0.78) (43, 44).
The development of molecular diagnostics and further research on the prognosis of patients with BM depending on prognostic factors led to the creation of the graded prognostic assessment (GPA) for estimating the survival of patients with brain metastases, including a separate breast GPA, the use of which shows the longer survival of patients with BCBM in relation to other patients with BM.
The prolonged survival of patients with BC due to advances in systemic treatment has led to an increased risk of late complications following WBRT, especially in those with the HER2-positive subtype, in whom intracranial spreading is particularly frequent (45, 46). Because of the lack of a survival advantage of WBRT added to stereotactic radiotherapy, SRS/FSRT alone is recommended for most patients, even in patients with multiple metastases, especially if their total volume is ≤15 ml (11, 43, 44, 47–49). The SRS dose and BM volume did not correlate with posttherapy CF (44, 46–49). Thanks to new technical solutions, SRS/FSRT for BM therapy has become more accessible and less time-consuming. Modern arc RT planning systems enable the creation of a single plan for several focal lesions in the brain (mono-isocenter technique), which, compared with the conventional multi-isocenter technique, not only shortens the treatment planning time but also decreases neurotoxicity by reducing the dose to the healthy brain (50).
3 Radiation-induced neurotoxicity
During standard radiation treatment (PBRT, WBRT, SRT, and SRS), healthy brain tissue is inevitably exposed to radiation. The exact mechanism underlying radiation-induced brain damage is not fully understood. For many years, the brain has been regarded as a highly radioresistant organ. Acute neurological symptoms were observed early after a single dose of 30 Gy, whereas white matter necrosis occurred after a conventionally fractionated dose of 60 Gy (51). With modern techniques, focal neurological deficits, epilepsy, and increased intracranial pressure have become less common (51, 52).
Early and early-delayed side effects, e.g., somnolence, headaches, drowsiness, attention deficits, and short-term memory loss, are caused by brain edema and transient demyelination, respectively. In contrast, late-delayed neurotoxicity is irreversible owing to white matter necrosis, vascular fibrosis, permanent demyelination, or gliosis (51). Biologically, these processes are associated with the proliferative capacity of glial and vascular endothelial cells. White matter necrosis is currently uncommon; however, the dynamic interplay among astrocytes, microglia, oligodendrocytes, endothelial cells, and neurons leads to radiation-induced neurocognitive dysfunction (16, 53). Most data on the mechanism of radiation-induced brain damage are obtained from studies on animal models, including rodents and nonhuman primates, and have suggested that the loss of hippocampal neurogenesis is a main problem (54, 55). Another hypothesis indicates that cognitive impairment after irradiation is caused by a neuroinflammatory cascade, including the disruption of the BBB, neural progenitor cell (NPC) death, hippocampal dysfunction, and direct activation of glia, causing the senescence-associated secretory phenotype (56).
3.1 Hippocampus
The hippocampus is the central player in memory and neurogenesis. Damage to this structure affects reasoning skills, learning difficulties, and memory and attention deficits and can progress to dementia. A preclinical study has shown that normal cognitive function (CF) is associated with neurogenesis in neural stem cells located in the subgranular zone of the hippocampal dentate gyrus (57). Transcriptomic analyses have shown that although hippocampal neurogenesis is decreased, it is preserved and possible in adulthood (57–59). Hippocampal glutamate receptor 1 and protein kinase C-gamma are likely responsible for synaptic plasticity in working memory (60). In mice, NPC maturation is suppressed or incorrect after ionizing radiation even at very low doses. Moreover, individuals exposed to irradiation did not pass the maze test, which is a hallmark of cognitive deterioration (52). Clinical observations have suggested similar effects in humans. Bilateral hippocampal irradiation with doses of >7.3 Gy was significantly correlated with cognitive dysfunction, with a 30% mean relative CF decrease at 4 months after RT (61).
3.2 Blood−brain barrier
For years, the BBB has been thought to be the most essential factor for BM resistance to systemic treatment. Abnormal tumor blood vessels facilitate the penetration of various molecules. As shown in an animal model, radiation destabilizes the plasma membrane of cells of the BBB and damages endothelial cells, increasing BBB permeability. The upregulation of proinflammatory genes and intracellular adhesion molecules leads to hypoxia, which amplifies the toxic changes in the irradiated brain microenvironment (62–64). Animal studies have shown that apoptosis observed 24 hours after WBRT leads to a 15% loss of cerebral microvascular endothelial cells (65).
3.3 Radiation-induced senescence
Radiation-induced DNA damage occurs directly from a high energy beam or indirectly via free radicals and reactive oxygen species. Single-strand DNA breaks may be repaired, while double-strand breaks are irreversible, causing cell apoptosis, senescence, mutations and genomic instability in astrocytes or neurons, endothelial cells, and fibroblasts. Thus, a complicated cascade of inflammatory processes in astrocytes involving cytokines, such as IL-6 and IL-1beta, changes the brain phenotype into a senescence-associated secretory phenotype (17, 66). The isoforms of p53 play a crucial role in promoting or restoring astrocyte senescence (17, 66).
3.4 Histological characteristics of radiation-induced brain injury
Every patient with neurological or neurocognitive changes requires evaluation to exclude the presence of a new BM, irradiated tumor progression, necrosis or other postradiation neurodegenerative changes. Despite progress in radiological imaging, no specific features allowing unequivocal differential diagnosis of degenerative versus cancer-related changes in the brain have been defined (67). Diagnosis in such cases may be achieved by neurosurgical excision, which is not possible in most patients. Tissue specimens from patients eligible for surgical excision usually contain residual tumor, necrotic tissue or both. Occasionally, viable normal brain tissue in a biopsy sample shows radiation-related changes, including astrogliosis, vascular alterations, tissue rarefaction, chronic inflammation, and atypia of glia and neurons (68, 69).
3.5 Chemotherapy-induced cognitive impairment
BM usually occurs late during metastatic BC. Therefore, patients with breast cancer brain metastases may receive up to several lines of chemotherapy before radiotherapy in the brain area. It is known that chemotherapy also impairs cognitive functions, and breast cancer patients are more sensitive to the symptoms of so-called “chemobrain”. One of the reasons often mentioned for this is the lack of other symptoms of the disease in patients with breast cancer and greater focus on cognitive disorders. Processing speed and attention span were shown to decrease in patients with stage I-IIIa breast cancer during chemotherapy and did not return to normal 2 months after systemic treatment (70). Breast cancer patients treated with intracranial radiotherapy for brain metastases are at risk for the cumulative adverse effects of several treatments on cognition.
4 Neurotoxicity-sparing strategies
The current understanding of BM biology and molecular underpinnings will facilitate the implementation of neuroprotective treatment protocols. The widespread use of brain RT has prompted the development of brain-sparing approaches. Available data were obtained from the studies performed in patients with various primary tumors. The most appealing treatment strategies include metastasis-directed RT (SRS/FSRT as previously described), hippocampus-avoiding (HA) radiation, and the use of memantine (12, 71–73). Effective systemic treatment of BCBM, which would allow clinicians to delay the use of intracranial radiotherapy, is still being researched, as well as completely novel approaches changing the paradigms of BCBM therapy.
4.1 Hippocampal-avoiding radiotherapy
The hippocampus is essential in memory function. Neuronal progenitor cells of the hippocampal dentate gyrus, which are responsible for neurogenesis, are extremely vulnerable and radiosensitive. Neurogenesis is essential for the recovery of radiation-related loss memory and CF deterioration after WBRT (74–76). Interestingly, BM distribution correlates with the primary tumor diagnosis: BMs due to pulmonary and gastrointestinal cancers are usually located in the infratentorial area, BMs due to skin cancer and sarcoma are usually located in the supratentorial space, whereas BCBMs are located in structures supplied by the posterior circulation areas, such as the cerebellum (77). Several studies have assessed patterns of failure after SRS, showing 0-4.1% recurrences in the hippocampus (Table 2), justifying the HA approach in RT for BM (74, 78–84). The avoidance of the hippocampus during WBRT is considered to be safe, with approximately 7.6% and 12.1% perihippocampal disease progression after HA-WBRT (85–88). High-quality MRI is required to prepare the HA-SRS plan (Figure 1), limiting the doses within the hippocampus to Dmean <5 Gy for SRS and <7 Gy for FSRT (50) (Figure 2). The RTOG trial, the first phase II randomized study to prospectively assess an HA approach, showed a significantly lower incidence of verbal memory decline with an HA approach than without an HA approach (7% vs. 30%; p<0.001) (75), but BCBM patients accounted for only 15% of the study group, and cognitive function was assessed only for 6 months after the completion of radiotherapy. With an HA approach, it was possible to restrict the dose to the hippocampus to 9 Gy in 98% of the volume and to 17 Gy in 2% of the volume with no deterioration of treatment efficacy (89). Recently, the HA concept was evaluated in patients with small cell lung cancer (SCLC) receiving 25 Gy in 10 fractions as standard prophylactic cranial irradiation (PCI). In that phase III trial, 150 patients with SCLC (71.3% with limited disease) were randomized to standard PCI or HA-PCI (90). Data including delayed free recall (DFR) on the Free and Cued Selective Reminding Test (FCSRT), quality of life, the incidence and location of BM, and OS were collected at baseline and at 3, 6, 12, and 24 months after RT. The decline in DFR from baseline to 3 months was lower in the HA-PCI arm (5.8%) than in the PCI arm (23.5%; odds ratio, 5; 95% CI, 1.57-15.86; p=0.003). The analysis of all FCSRT scores showed a decline in the total recall (TR) (TR: 8.7% vs. 20.6%) at 3 months; DFR (11.1% vs. 33.3%), TR (20.3% vs. 38.9%), and total free recall (14.8% vs. 31.5%) at 6 months, and TR (14.2% vs. 47.6%) at 24 months. The incidence of BMs, OS, and quality of life were not significantly different between the groups. The researchers concluded that sparing the hippocampus is possible and facilitates CF preservation without deteriorating the outcomes of patients with SCLC. It is known that the clinical and molecular characteristics of BCBM patients are significantly different from those of SCLC patients receiving PCI. Clinical studies have not confirmed the benefit of PCI in patients with BC and have reported no evidence of cognitive dysfunction in PCI patients (91). Considering these reports and the fact that patients with BCBM may have increased symptoms of “chemobrain” after previous treatment, cognitive impairment associated with current brain metastases, and possible systemic treatment options active in brain metastases, translating the results of SCLC patients’ treatment to patients with BCBM seems difficult or impossible.
Nevertheless, due to the lack of research results on the use of HA-WBRT in the BCBM group, considering the results of the cited studies, the increasing availability of MRI and the relatively small group of patients with BCBM not eligible for SRS/FSRT, we often use the HA-WBRT technique in everyday clinical practice as we describe in detail later in the work.
4.2 Memantine
In a randomized, double-blind, placebo-controlled RTOG 0614 trial, adult patients with a BM outside the 5 mm margin around the hippocampus and a KPS score >70 were randomly allocated to WBRT or WBRT with the addition of memantine (92). Memantine has been used as an uncompetitive antagonist of the N-methyl-D-aspartate (NMDA) receptor, which is crucial for synaptic plasticity, a cellular mechanism of learning and memory. Memantine administered concomitantly with WBRT was shown to be beneficial. Cognitive decline was delayed (HR=0.78, 95% CI, 0.62-0.99, p=0.01), while memory decline was less at 24 weeks, but it was not statistically significant (p=0.059). Superior results were also seen for memantine in executive function at 8 weeks (p=0.008), processing speed at 24 weeks (p=0.0137) and delayed recognition at 24 weeks (p=0.0149). Overall, the probability of cognitive function failure at 24 weeks in the memantine arm was 53.8%, while 64.9% in the placebo arm. In NRG COO1, HA-WBRT combined with memantine significantly improved CF (HR=0.74, 95% CI, 0.58–0.95; p=0.020) over WBRT plus memantine, showing promising results for combined treatment (71). However, patients with BCBM constituted less than 15% in the RTOG 0614 study and 18.5% in the NRG CC001 trial, and the observation period was only 6-8 months after radiotherapy, which is a limitation of these trials. Apart from the heterogeneous histology of BM, other limitations of these studies include the lack of information on the systemic treatment used and the number and initial volume of BM, as well as on comorbidities or cigarette smoking, which could have influenced cognitive functions. There are several reports showing that despite the favorable results of clinical trials and the potentially simple implementation of memantine treatment into clinical practice, few radiotherapists recommend its use (93–95). A recent update of the NRG CC001 trial was published with a median follow-up of 12.1 months, showing sustained preservation of cognitive function in the HA-WBRT + memantine arm (adjusted hazard ratio, 0.74, P = .016) (96). Patients who received HA-WBRT + memantine experienced less symptom burden at 6 (P <.001 using imputed data) and 12 months (P = .026 using complete-case data; P <.001 using imputed data), less symptom interference at 6 (P = .003 using complete-case data; P = .0016 using imputed data) and 12 months (P = .0027 using complete-case data; P = .0014 using imputed data), and fewer cognitive symptoms over time (P = .043 using imputed data). There were no differences in overall survival, intracranial progression-free survival, or toxicity between treatment arms.
Thus, confirmation of the beneficial neuroprotective effect of memantine in BCBM is needed, but considering the availability of the therapy, its relatively low cost and low toxicity, treatment with memantine may be offered to patients with BCBM who cannot avoid WBRT, and especially to those who are determined to undergo neurotoxicity-sparing treatment.
4.3 FLASH radiotherapy (FLASH-RT)
FLASH-RT is a new option in external-beam therapy. It appeared in the literature in 1960-1970, but it was rediscovered in 2014 (97). In FLASH-RT, single short radiotherapy pulses are delivered at ultrahigh dose rates >40 Gy/s as opposed to conventionally used rates of 0,07-0,1 Gy/s. This technique has gained interest due to unexpected normal tissue tolerance with efficacy similar to conventional radiotherapy. Montay-Gruel et al. (98) have demonstrated the sparing of memory in mice after whole brain irradiation of a single dose of 10 Gy when dose rates above 100 Gy/s were achieved. This effect (called the FLASH effect) was significantly lower in the range of 30-100 Gy/s and disappeared completely with dose rates <30 Gy/s. The researchers revealed the preservation of memory and neurogenesis in the hippocampus 2 months after FLASH radiotherapy, but these results were also found after 6 months of observation. Additional experiments showed lower levels of reactive oxygen species (ROS), a lack of neuroinflammation and dendritic complexity and a synaptic landscape similar to the nonirradiated brain tissue (99).
In the context of breast cancer treatment, FLASH radiotherapy is also being studied, but mainly in the context of adjuvant radiotherapy after breast cancer surgery, and most of these studies involve animal models or feasibility studies in humans (100–102).
Although FLASH radiotherapy has been shown to improve the therapeutic index, especially in brain irradiation, most of the knowledge was obtained from animal studies, and a better understanding of the radiobiology of this new therapy is still needed. Clinical implementation will also require solutions to technical challenges, but the investigators think that progress from achievements made during the last decade is just a matter of time.
4.4 Systemic treatment of BCBM
Anaplastic lymphoma kinase (ALK)-mutated or epidermal growth factor receptor (EGFR)-mutated lung adenocarcinoma patients with brain metastases are primarily treated with systemic molecular therapies instead of upfront brain irradiation, especially when there are few and small lesions in the CNS. This therapeutic change became possible due to high BBB penetration by new anti-ALK and anti-EGFR drugs. It is still uncertain whether primary systemic treatment in the case of BCBM will delay the application of intracranial radiotherapy and thus delay its toxicity.
As mentioned above, BCBM patients are usually excluded from clinical trials or are a small group in the treated population, making it impossible to draw conclusions about recommended therapy. Currently, according to the growing population of surviving advanced breast cancer patients, there are multiple ongoing clinical trials in disseminated BC investigating new agents alone or in combination with already well-established treatments (such as trastuzumab, lapatinib, and capecitabine). Of the most studied drugs are inhibitors of the PI3K/AKT/mTOR (phosphatidylinositol-3 kinase/AKT/mammalian target of rapamycin) signaling pathway, HER2 (human epidermal growth factor receptor 2) and pan-HER inhibitors, immunotherapy, CDK4/6 (cyclin-dependent kinases 4/6) inhibitors and PARP (polyadenosine diphosphate-ribose polymerase) inhibitors.
The PI3K/AKT/mTOR pathway is activated in 30-40% of BCs and even in 43-75% of BCBMs, making this molecular disorder a potential therapeutic target (103). The PI3K/AKT/mTOR inhibiting regiment of buparlisib plus capecitabine is being tested in an ongoing clinical trial (NCT02000882).
Trastuzumab, an anti-HER2 antibody, is too large to penetrate the BBB but is very effective extracranially and prolongs the survival of BC patients; however, it increases the risk of metastasis to the CNS as the first relapse location (104). The subsequently developed anti-HER2 antibody pertuzumab showed disappointing results against brain metastases (105). The regimen of lapatinib (dual tyrosine-kinase inhibitor [TKI] of HER1 and HER2) combined with capecitabine is effective in HER2+ BCBM (106), with a median time to WBRT of 8.5 months in untreated low volume BM (107). Trastuzumab conjugates (trastuzumab-emtansyna [TDM-1] and trastuzumab-deruxtecan [T-Dxd]) showed promising activity in the CNS, especially T-Dxd (42, 108–110). Ultimately, in search of small molecules that easily penetrate the blood−brain barrier, novel TKIs inhibiting the human epidermal growth factor receptor family have been developed (tucatinib, neratinib, pyrotinib), showing promising effectiveness in HER2+ BCBM (111–113). Nevertheless, there are many ongoing trials to establish their role in clinical practice, also in the context of brain irradiation, as it is uncertain if they should be used before, after or even with CNS radiotherapy as radiosensitizers (114).
The role of immunotherapy in the setting of BCBM is less certain, as it shows positive results only in metastatic TNBC; however, no benefit has been found in the subgroup of patients with BCBM (115). The knowledge in this subject is limited, but the results of a few trials are on the way, including those of studies combining immunotherapy with brain radiotherapy (NCT03449238 and NCT03483012).
More optimistic results have been seen in HR+ HER2- BCBM patients treated with CDK4/6 inhibitors, particularly abemaciclib, which showed good penetration through the BBB (116). Prospective trials are underway (NCT02308020, NCT02896335, and NCT04334330).
PARP inhibitors (olaparib and talazoparib) already approved by the FDA for the treatment of germline BRCA-mutated BC have been investigated in BCBM. To date, talazoparib (117) and veliparib (118) have shown activity in the brain, interestingly in the case of veliparib in combination with WBRT, but further studies are expected to elucidate their role in BCBM and the optimal sequence of treatment modalities.
4.5 Secondary prevention of BCBM
SRS/FSRT is an effective method of local treatment for BCBM, with fewer local failures than surgical treatment and lower neurotoxicity than WBRT due to the reduction in the irradiated brain volume, including the uninvolved hippocampus. At the same time, limiting treatment to existing metastases carries the risk of metastasis development in the rest of the brain. WBRT reduces this risk at the expense of neurotoxicity without prolonging survival (Table 1). A new idea is to prevent the development of secondary BCBM (after initial local therapy) with systemic treatment. In preclinical studies in mice, at low metronomic doses, temozolomide (TMZ), a BBB-crossing alkylating agent routinely used in the treatment of glioblastoma, has been shown to reduce the risk of developing brain metastases, despite its lack of efficacy in treating existing metastases. In a phase 1 study, metronomic use of TMZ in combination with T-DM1 showed low toxicity and potential activity in the secondary prevention of BCBM in HER2(+) BC patients (119). A phase II study evaluating this treatment is ongoing (NCT03190967).
4.6 Other options
There are many other methods of treating BCBM under investigation that avoid radiotherapy and its toxicities. Many of them are incorporating new approaches to omit the problem with the BBB. One of them is intrathecal administration of drugs, such as trastuzumab (120). The second emerging technology is nanotherapy (121), which uses nanoparticles carrying anticancer agents to deliver drugs, but further research on its role in BCBM is needed. The other option includes brain radiotherapy to improve the penetrability of the BBB for systemic treatment, but its role in this capacity has not been well proven.
Another interesting possibility is liquid biopsy of cerebrospinal fluid to identify the exact molecular alterations of BCBM cells (122), as it is well known that metastatic breast cancer cells can change their molecular features during invasion and metastasis formation, for instance, in the case of HER2 amplification. Establishing the molecular status of brain metastases can be used to tailor treatment more precisely and effectively.
5 Practical implications
The group of patients with BCBM is not homogeneous. The choice of BM treatment method is determined by the patient’s age, performance status, number, size and location of brain metastases and control of extracranial metastases.
Traditionally patients with a single brain metastasis, especially over 3 cm, with neurosurgical symptoms, severe edema and mass effect and/or located in the posterior cranial fossa with hydrocephalus, were eligible for neurosurgical treatment, if the extracranial metastases were stable and systemic treatment options were available.
In everyday practice, we increasingly qualify patients with BCBM for primary BM radiotherapy due to the increased risk of dissemination to the meninges after primary neurosurgical treatment, which in patients with TNBC may be as high as 24%. The qualification criteria for SRS/FSRT include the potential possibility of further systemic treatment, the slow dynamics of the disease, previous intracranial radiotherapy, and the patient’s general condition. If at least 2 out of 4 factors favor SRS/FSRT, we qualify patients for this method, even in the presence of >4 metastatic foci if they are countable. Traditionally, we qualified patients with a single BCBM <= 2 cm for SRS; currently, the final choice of irradiation technique is determined by the parameters of the radiotherapy plan. To diagnose intracranial spread, we use 3-Tesla magnetic resonance imaging using a SPACE sequence, thanks to which we detect more small lesions. If, when preparing the radiotherapy plan, the measured volume of metastases exceeds 25 cm3, it will not be possible to safely and effectively perform SRS and we qualify the patient for FSRT. In case of BCBM volume <25cm3, we prepare SRS. If the irradiation plan meets the radiosurgical criteria, i.e. with a dose of approximately 20Gy in 1 fraction for metastatic lesions, the volume of the healthy brain receiving a dose of 12Gy is less than 5 cm3 (V12<5 cm3) and the plan conformity index is <1.4 (CI<1, 4), we qualify the patient for SRS. If the radiotherapy plan does not meet the above parameters, we qualify the patient for FSRT, usually 27Gy in 3 fractions or 30/35Gy in 5 fractions, depending on meeting the remaining constraints for intracranial radiotherapy, including the average dose to the hippocampus below 7 Gy (Dmean<7Gy). Patients with poor general condition, short expected survival, lack of cooperation with medical staff and uncontrolled epilepsy are not eligible for SRS/FSRT.
For patients with BCBM who are not eligible for SRS/FSRT, e.g. due to uncountable brain metastases, or in the case of BCBM from TNBC undergoing neurosurgery, remaining in good general condition, cooperating with medical staff, in whom we expect > 3-month survival, we use WBRT. In each case in which we have available brain MRI, we use HA-WBRT, using 30Gy in 10 fractions (12 days of treatment), with the maximum dose (the highest dose in 0.03 cm3 of both hippocampi) limited to 16Gy [Dmax <=16Gy], and doses in the entire hippocampal volume up to 9 Gy [D100% <=9Gy]. Approximately 3 days before starting HA-WBRT, we start memantine. In the first week at a dose of 1 x 5mg orally in the morning, followed by the addition of a 5-mg dose in the evening during week 2. In week 3, we increase the morning dose to 10 mg. In the fourth week we reach a dose of 2 x 10 mg per day and continue this treatment for up to 24 weeks in total. In case of creatinine clearance below 30 mL/min the maximum dose is 5 mg orally twice daily and the treatment should not be administered if the creatinine clearance is less than 5 mL/min.
According to the previously cited clinical trial data, we know that both the metastatic changes themselves and oncological treatment, both with radiotherapy and systemic therapy, have a significant impact on the cognitive functions of a patient with BCBM. Basic screening tests such as MMSE have limited sensitivity in detecting these disorders, and oncologists and radiation oncologists in their daily practice do not have enough time or knowledge to perform more advanced tests. Due to the popularization of Breast Units, which comprehensively deal with the treatment of patients with breast cancer and bring together doctors of various specialties, senology nurses, dieticians and psychologists in one place, it seems reasonable that patients with BCBM should also be provided with care by neuropsychologists. This would allow us to obtain real-world data about the frequency, depth and persistence of cognitive disorders in BCBM patients and their dependence on oncological treatment.
6 Future directions
HA-WBRT +/- memantine is not routine practice in radiotherapy facilities, despite phase III studies showing neurocognitive benefits and safety of such therapy. One of the reasons is the heterogeneity of the patient population included in these studies. Another criticism was short follow-up, but the recently published update of the NRG CC001 study demonstrated that the benefits persisted at one-year follow-up. Another reason is the mediocre benefit of the intervention - in the group of patients treated with HA-WBRT, 60% still have cognitive disorders after 6 months. There are also technical and economic aspects. WBRT can be planned and implemented easily in any radiotherapy facility, even within 5 days. HA-WBRT requires the use of MRI to contour the hippocampi and the use of intensity-modulated beam technique (IMRT) by physicists, and the usual regimen is 30 Gy in 10 fractions, which extends the procedure time to 2-3 weeks and significantly increases workload on par with SRS/FSRT planning. In some countries, despite the same amount of work, the HA-WBRT procedure may be less cost-effective than SRS/FSRT. For this reason, the results of a study comparing SRS with HA-WBRT + memantine in less than 5 BM may provide a solution for everyday practice (NCT03550391). We expect an increase in the use of stereotactic techniques in the treatment of BCBM, which will replace surgical treatment associated with a higher risk of meningeal metastases and allow for dose reduction not only in the hippocampus, but also in other parts of the healthy brain related to cognitive functions, such as the corpus callosum. Technological development now allows for safe and effective irradiation of patients with > 10 BM with FSRT.
Secondly, the radiotherapy community also expects the results of studies assessing the effectiveness of systemic treatment in BCBM, especially in HER2+ breast cancer, because small molecules with good penetration of the BBB may change the paradigm and replace intracranial radiotherapy or significantly delay its use. Old radiosensitizers have failed in the treatment of BM, but we expect that intracranial radiotherapy combined with modern hormone therapy, immunotherapy or PARP inhibitors will change the standards of management of BCBM.
7 Conclusions
Despite the significant incidence rate, patients with brain metastases from breast cancer (BCBMs) remain underrepresented in randomized clinical trials (RCTs) that investigate diverse strategies for minimizing cognitive deficits after intracranial radiotherapy. The bulk of available data originates from studies involving lung cancer patients, particularly those with non-small cell lung cancer (NSCLC). Given the disparities in clinical and molecular attributes along with variations in prognosis between NSCLC and distinct subtypes of breast cancer it becomes imperative to validate these findings within a cohort of BCBM (123, 124). This validation should consider the long-term repercussions of approaches aimed at mitigating radiation-induced neurotoxicity, in contrast to the prevailing trend of evaluating outcomes only at 6-month juncture, as observed in prior trials.
The augmented survival rate among breast cancer patients with well-regulated systemic control presents an opportunity to employ an efficacious and safe method for managing intracranial disease. Emerging systemic therapies, particularly those tailored for HER2-positive breast cancer, exhibit enhanced potency in managing brain metastases. This development may potentially defer the need for immediate radiotherapy in BCBMs (111, 125). However, for those with triple-negative breast cancer (TNBC) BCBMs, the optimal synergy between systemic treatment and intracranial radiotherapy - both in terms of efficacy and safety – remains uncertain. The anticipation of utilizing immunotherapies and PARP inhibitors for this indication is on the horizon.
In tandem with these advancements, novel treatment modalities like FLASH radiotherapy, nanoparticles facilitating drug delivery to the brain, and TMZ as the secondary prevention for HER2+ BCBM following stereotactic radiosurgery (SRS) and/or surgery, are subjects of intensive research.
These innovations have the potential to reshape the fundamental approaches to treat BCBMs. It is crucial to underscore that expecting comprehensive human data encompassing anatomopathological and molecular changes linked to radiotherapy is an impracticable endeavor. To synthesize drawing upon existing insights derived from animal studies concerning radiation-induced brain damage, along with strategies aimed at alleviating neurotoxicity in human cases, the suggestion is to prioritize stereotactic radiosurgery (SRS) or fractionated stereotactic radiotherapy (FSRT) over whole-brain radiotherapy (WBRT) when addressing patients with oligometastatic brain metastases from breast cancer (BCBMs). Even in cases of polymetastatic BCBMs, that can be enumerated, FSRT stands as the preferred approach. In scenarios when WBRT is unavoidable, such as when facing uncountable number of BCBMs or in cases of surgically resected triple-negative BCBMs, hippocampal-avoidant WBRT (HA-WBRT) in conjunction with memantine administration may be considered.
Author contributions
All authors listed have made a substantial, direct, and intellectual contribution to the work, wrote the manuscript and approved it for publication.
Funding
The author(s) declare financial support was received for the research, authorship, and/or publication of this article. This work was supported by statutory funds of the Medical University of Gdansk (01-10024/0006042).
Conflict of interest
The authors declare that the research was conducted in the absence of any commercial or financial relationships that could be construed as a potential conflict of interest.
Publisher’s note
All claims expressed in this article are solely those of the authors and do not necessarily represent those of their affiliated organizations, or those of the publisher, the editors and the reviewers. Any product that may be evaluated in this article, or claim that may be made by its manufacturer, is not guaranteed or endorsed by the publisher.
References
1. Sung H, Ferlay J, Siegel RL, Laversanne M, Soerjomataram I, Jemal A, et al. Global cancer statistics 2020: GLOBOCAN estimates of incidence and mortality worldwide for 36 cancers in 185 countries. CA Cancer J Clin (2021) 71:209–49. doi: 10.3322/caac.21660
2. Lin NU, Gaspar LE, Soffietti R. Breast cancer in the central nervous system: multidisciplinary considerations and management. Am Soc Clin Oncol Educ B (2017) 37:45–56. doi: 10.1200/EDBK_175338
3. Wu Q, Li J, Zhu S, Wu J, Chen C, Liu Q, et al. Breast cancer subtypes predict the preferential site of distant metastases: A SEER based study. Oncotarget (2017) 8:27990–6. doi: 10.18632/oncotarget.15856
4. Popp I, Rau A, Kellner E, Reisert M, Fennell JT, Rothe T, et al. Hippocampus-avoidance whole-brain radiation therapy is efficient in the long-term preservation of hippocampal volume. Front Oncol (2021) 11:714709. doi: 10.3389/fonc.2021.714709
5. Sperduto PW, Kased N, Roberge D, Chao ST, Shanley R, Luo X, et al. The effect of tumor subtype on the time from primary diagnosis to development of brain metastases and survival in patients with breast cancer. J Neuro-Oncol (2013) 112:467–72. doi: 10.1007/S11060-013-1083-9
6. Chen G, Huynh M, Chen A, Fehrenbacher L, Gandara D, Lau D. Chemotherapy for brain metastases in small-cell lung cancer. Clin Lung Cancer (2008) 9:35–8. doi: 10.3816/CLC.2008.n.006
7. Soffietti R, Costanza A, Laguzzi E, Nobile M, Rudà R. Radiotherapy and chemotherapy of brain metastases. J Neurooncol (2005) 75:31–42. doi: 10.1007/s11060-004-8096-3
8. Lee SH. Role of chemotherapy on brain metastasis. Prog Neurol Surg (2012) 25:110–4. doi: 10.1159/000331183
9. Plant-Fox AS, O’Halloran K, Goldman S. Pediatric brain tumors: the era of molecular diagnostics, targeted and immune-based therapeutics, and a focus on long term neurologic sequelae. Curr Probl Cancer (2021) 45:100777. doi: 10.1016/j.currproblcancer.2021.100777
10. Mintz A, Perry J, Spithoff K, Chambers A, Laperriere N. Management of single brain metastasis: A practice guideline. Curr Oncol (2007) 14:131–43. doi: 10.3747/co.2007.129
11. Tsao M, Xu W, Sahgal AA. Meta-analysis evaluating stereotactic radiosurgery, whole-brain radiotherapy, or both for patients presenting with a limited number of brain metastases. Cancer (2012) 118:2486–93. doi: 10.1002/CNCR.26515
12. Sahgal A, Aoyama H, Kocher M, Neupane B, Collette S, Tago M, et al. Phase 3 trials of stereotactic radiosurgery with or without whole-brain radiation therapy for 1 to 4 brain metastases: individual patient data meta-analysis. Int J Radiat Oncol Biol Phys (2015) 91:710–7. doi: 10.1016/J.IJROBP.2014.10.024
13. Harwood AR, John Simpson W. Radiation therapy of cerebral metastases: A randomized prospective clinical trial. Int J Radiat Oncol Biol Phys (1977) 2:1091–4. doi: 10.1016/0360-3016(77)90114-6
14. Borgelt B, Gelber R, Kramer S, Brady LW, Chang CH, Davis LW, et al. The palliation of brain metastases: final results of the first two studies by the radiation therapy oncology group. Int J Radiat Oncol Biol Phys (1980) 6:1–9. doi: 10.1016/0360-3016(80)90195-9
15. Saad S, Wang TJC. Neurocognitive deficits after radiation therapy for brain Malignancies. Am J Clin Oncol Cancer Clin Trials (2015) 38:634–40. doi: 10.1097/COC.0000000000000158
16. Turnquist C, Harris BT, Harris CC. Radiation-induced brain injury: current concepts and therapeutic strategies targeting neuroinflammation. Neuro-Oncol Adv (2020) 2(1):vdaa057. doi: 10.1093/noajnl/vdaa057
17. Lee D-S, Yu M, Jang H-S, Kim Y-S, Choi B-O, Kang Y-N, et al. Radiation-induced brain injury: retrospective analysis of twelve pathologically proven cases. Radiat Oncol J (2011) 29:147. doi: 10.3857/roj.2011.29.3.147
18. Horton J, Baxter DH, Olson KB. The management of metastases to the brain by irradiation and corticosteroids. Am J Roentgenol Radium Ther Nucl Med (1971) 111:334–6. doi: 10.2214/ajr.111.2.334
19. Zimm S, Wampler GL, Stablein D, Hazra T, Young HF. Intracerebral metastases in solid-tumor patients: natural history and results of treatment. Cancer (1981) 48:384–394. doi: 10.1002/1097-0142(19810715)48:2<384::AID-CNCR2820480227>3.0.CO;2-8
20. T.Komarnicky L, Phillips TL, Martz K, Asbell S, Isaacson S, Urtasun RA. Randomized phase III protocol for the evaluation of misonidazole combined with radiation in the treatment of patients with brain metastases (RTOG-7916). Int J Radiat Oncol Biol Phys (1991) 20:53–8. doi: 10.1016/0360-3016(91)90137-S
21. Knisely JPS, Berkey B, Chakravarti A, Yung AWK, Curran WJ, Robins HI, et al. Phase III study of conventional radiation therapy plus thalidomide versus conventional radiation therapy for multiple brain metastases (RTOG 0118). Int J Radiat Oncol Biol Phys (2008) 71:79–86. doi: 10.1016/J.IJROBP.2007.09.016
22. Suh JH, Stea B, Nabid A, Kresl JJ, Fortin A, Mercier JP, et al. Phase III study of efaproxiral as an adjunct to whole-brain radiation therapy for brain metastases. J Clin Oncol (2006) 24:106–14. doi: 10.1200/JCO.2004.00.1768
23. Borgelt B, Gelber R, Larson M, Hendrickson F, Griffin T, Roth R. Ultra-rapid high dose irradiation schedules for the palliation of brain metastases: final results of the first two studies by the radiation therapy oncology group. Int J Radiat Oncol Biol Phys (1981) 7:1633–8. doi: 10.1016/0360-3016(81)90184-X
24. Haie-Meder C, Pellae-Cosset B, Laplanche A, Lagrange JL, Tuchais C, Nogues C, et al. Results of a randomized clinical trial comparing two radiation schedules in the palliative treatment of brain metastases. Radiother Oncol (1993) 26:111–6. doi: 10.1016/0167-8140(93)90091-L
25. Murray KJ, Scott C, Greenberg HM, Emami B, Seider M, Vora NL, et al. Randomized phase III study of accelerated hyperfractionation versus standard in patients with unresected brain metastases: A report of the radiation therapy oncology group (RTOG) 9104. Int J Radiat Oncol Biol Phys (1997) 39:571–4. doi: 10.1016/S0360-3016(97)00341-6
26. Patchell RA, Tibbs PA, Walsh JW, Dempsey RJ, Maruyama Y, Kryscio RJ, et al. Randomized trial of surgery in the treatment of single metastases to the brain. N Engl J Med (1990) 322:494–500. doi: 10.1056/NEJM199002223220802
27. Vecht CJ, Haaxma-Reiche H, Noordijk EM, Padberg GW, Voormolen JHC, Hoekstra FH, et al. Treatment of single brain metastasis: radiotherapy alone or combined with neurosurgery. Ann Neurol (1993) 33:583–90. doi: 10.1002/ANA.410330605
28. Mink AH, Kestle J, Rathbone MP, Gaspar L, Hugenholtz H, Fisher B, et al. Randomized trial to assess the efficacy of surgery in addition to radiotherapy in patients with a single cerebral metastasis. Cancer (1996) 78, 1470–6. doi: 10.1002/(SICI)1097-0142(19961001)78:7<1470::AID-CNCR14>3.0.CO;2-X
29. Wrónski M, Arbit E, McCormick B. Surgical treatment of 70 patients with brain metastases from breast carcinoma. Cancer (1997) 80:1746–54. doi: 10.1002/(SICI)1097-0142(19971101)80:9<1746::AID-CNCR8>3.0.CO;2-C
30. Lee SS, Ahn JH, Kim MK, Sym SJ, Gong G, Do Ahn S, et al. Brain metastases in breast cancer: prognostic factors and management. Breast Cancer Res Treat (2008) 111:523–30. doi: 10.1007/s10549-007-9806-2
31. Leone JP, Lee AV, Brufsky AM. Prognostic factors and survival of patients with brain metastasis from breast cancer who underwent craniotomy. Cancer Med (2015) 4:989–94. doi: 10.1002/cam4.439
32. Andrews DW, Scott CB, Sperduto PW, Flanders AE, Gaspar LE, Schell MC, et al. Whole brain radiation therapy with or without stereotactic radiosurgery boost for patients with one to three brain metastases: phase III results of the RTOG 9508 randomised trial. Lancet (2004) 363:1665–72. doi: 10.1016/S0140-6736(04)16250-8
33. Kocher M, Soffietti R, Abacioglu U, Villà S, Fauchon F, Baumert BG, et al. Adjuvant Whole-Brain Radiotherapy versus Observation after Radiosurgery or Surgical Resection of One to Three Cerebral Metastases: Results of the EORTC 22952-26001 Study. J Clin Oncol (2011) 29:134–41. doi: 10.1200/JCO.2010.30.1655
34. Wilson TG, Robinson T, MacFarlane C, Spencer T, Herbert C, Wade L, et al. Treating brain metastases from breast cancer: outcomes after stereotactic radiosurgery. Clin Oncol (2020) 32:390–6. doi: 10.1016/j.clon.2020.02.007
35. Aversa C, Rossi V, Geuna E, Martinello R, Milani A, Redana S, et al. Metastatic breast cancer subtypes and central nervous system metastases. Breast (2014) 23:623–8. doi: 10.1016/j.breast.2014.06.009
36. Anders CK, Deal AM, Miller CR, Khorram C, Meng H, Burrows E, et al. The prognostic contribution of clinical breast cancer subtype, age, and race among patients with breast cancer brain metastases. Cancer (2011) 117:1602–11. doi: 10.1002/cncr.25746
37. Aoyama H, Shirato H, Tago M, Nakagawa K, Toyoda T, Hatano K, et al. Stereotactic radiosurgery plus whole-brain radiation therapy vs stereotactic radiosurgery alone for treatment of brain metastases: A randomized controlled trial. JAMA (2006) 295:2483–91. doi: 10.1001/JAMA.295.21.2483
38. Chang EL, Wefel JS, Hess KR, Allen PK, Lang FF, Kornguth DG, et al. Neurocognition in patients with brain metastases treated with radiosurgery or radiosurgery plus whole-brain irradiation: A randomised controlled trial. Lancet Oncol (2009) 10:1037–44. doi: 10.1016/S1470-2045(09)70263-3
39. Brown PD, Jaeckle K, Ballman KV, Farace E, Cerhan JH, Keith Anderson S, et al. Effect of radiosurgery alone vs radiosurgery with whole brain radiation therapy on cognitive function in patients with 1 to 3 brain metastases: A randomized clinical trial. JAMA (2016) 316:401–9. doi: 10.1001/JAMA.2016.9839
40. Kępka L, Tyc-Szczepaniak D, Bujko K, Olszyna-Serementa M, Michalski W, Sprawka A, et al. Stereotactic radiotherapy of the tumor bed compared to whole brain radiotherapy after surgery of single brain metastasis: results from a randomized trial. Radiother Oncol (2016) 121:217–24. doi: 10.1016/j.radonc.2016.10.005
41. Brown PD, Ballman KV, Cerhan JH, Anderson SK, Carrero XW, Whitton AC, et al. Postoperative stereotactic radiosurgery compared with whole brain radiotherapy for resected metastatic brain disease (NCCTG N107C/CEC·3): A multicentre, randomised, controlled, phase 3 trial. Lancet Oncol (2017) 18:1049–60. doi: 10.1016/S1470-2045(17)30441-2
42. Pérez-García JM, Vaz Batista M, Cortez P, Ruiz-Borrego M, Cejalvo JM, de la Haba-Rodriguez J, et al. Trastuzumab deruxtecan in patients with central nervous system involvement from HER2-positive breast cancer: the DEBBRAH trial. Neuro Oncol (2023) 25:157–66. doi: 10.1093/neuonc/noac144
43. Yamamoto M, Serizawa T, Shuto T, Akabane A, Higuchi Y, Kawagishi J, et al. Stereotactic radiosurgery for patients with multiple brain metastases (JLGK0901): A multi-institutional prospective observational study. Lancet Oncol (2014) 15:387–95. doi: 10.1016/S1470-2045(14)70061-0
44. Yamamoto M, Serizawa T, Higuchi Y, Sato Y, Kawagishi J, Yamanaka K, et al. A multi-institutional prospective observational study of stereotactic radiosurgery for patients with multiple brain metastases (JLGK0901 study update): irradiation-related complications and long-term maintenance of mini-mental state examination scores. Int J Radiat Oncol Biol Phys (2017) 99:31–40. doi: 10.1016/j.ijrobp.2017.04.037
45. Garcia-Alvarez A, Papakonstantinou A, Oliveira M. Brain metastases in her2-positive breast cancer: current and novel treatment strategies. Cancers (Basel) (2021) 13:2927. doi: 10.3390/cancers13122927
46. Sperduto PW, Mesko S, Li J, Cagney D, Aizer A, Lin NU, et al. Beyond an updated graded prognostic assessment (Breast GPA): A prognostic index and trends in treatment and survival in breast cancer brain metastases from 1985 to today, in: Proceedings of the International Journal of Radiation Oncology Biology Physics, June 1 2020, Vol. 107, pp. 334–43. Amsterdam, Netherlands: Elsevier Inc.
47. Churilla TM, Ballman KV, Brown PD, Twohy EL, Jaeckle K, Farace E, et al. Stereotactic radiosurgery with or without whole-brain radiation therapy for limited brain metastases: A secondary analysis of the north central cancer treatment group N0574 (Alliance) randomized controlled trial. Int J Radiat Oncol Biol Phys (2017) 99:1173–8. doi: 10.1016/J.IJROBP.2017.07.045
48. Churilla TM, Handorf E, Collette S, Collette L, Dong Y, Aizer AA, et al. Whole Brain Radiotherapy after Stereotactic Radiosurgery or Surgical Resection among Patients with One to Three Brain Metastases and Favorable Prognoses: A Secondary Analysis of EORTC 22952-26001. Ann Oncol (2017) 28:2588–94. doi: 10.1093/ANNONC/MDX332
49. Aoyama H, Tago M, Shirato H. Investigators, for the J.R.O.S.G. 99-1 (JROSG 99-1) stereotactic radiosurgery with or without whole-brain radiotherapy for brain metastases: secondary analysis of the JROSG 99-1 randomized clinical trial. JAMA Oncol (2015) 1:457–64. doi: 10.1001/JAMAONCOL.2015.1145
50. Ruggieri R, Naccarato S, Mazzola R, Ricchetti F, Corradini S, Fiorentino A, et al. Linac-based VMAT radiosurgery for multiple brain lesions: comparison between a conventional multi-isocenter approach and a new dedicated mono-isocenter technique. Radiat Oncol (2018) 13:1–9. doi: 10.1186/S13014-018-0985-2/FIGURES/3
51. Tang Y, Li Y, Luo D, Rong X, Ye J, Peng Y. Epilepsy related to radiotherapy in patients with nasopharyngeal carcinoma. Epilepsy Res (2011) 96:24–8. doi: 10.1016/j.eplepsyres.2011.04.010
52. Haldbo-Classen L, Amidi A, Wu LM, Lukacova S, von Oettingen G, Gottrup H, et al. Long-term cognitive dysfunction after radiation therapy for primary brain tumors. Acta Oncol (Madr) (2019) 58:745–52. doi: 10.1080/0284186X.2018.1557786
53. Robbins ME, Bourland JD, Cline JM, Wheeler KT, Deadwyler SAA. Model for assessing cognitive impairment after fractionated whole-brain irradiation in nonhuman primates. Radiat Res (2011) 175:519–25. doi: 10.1667/RR2497.1
54. Warrington JP, Csiszar A, Johnson DA, Herman TS, Ahmad S, Lee YW, et al. Cerebral microvascular rarefaction induced by whole brain radiation is reversible by systemic hypoxia in mice. Am J Physiol - Hear Circ Physiol (2011) 300(3):H736–44. doi: 10.1152/ajpheart.01024.2010
55. Chakraborti A, Allen A, Allen B, Rosi S, Fike JR. Cranial irradiation alters dendritic spine density and morphology in the hippocampus. PLoS One (2012) 7(7):e40844. doi: 10.1371/journal.pone.0040844
56. Gutierrez-Quintana R, Walker DJ, Williams KJ, Forster DM, Chalmers AJ. Radiation-induced neuroinflammation: A potential protective role for poly(ADP-ribose) polymerase inhibitors? Neuro-Oncol Adv (2022) 4(1):vdab190. doi: 10.1093/noajnl/vdab190
57. Boldrini M, Fulmore CA, Tartt AN, Simeon LR, Pavlova I, Poposka V, et al. Human hippocampal neurogenesis persists throughout aging. Cell Stem Cell (2018) 22:589–599.e5. doi: 10.1016/j.stem.2018.03.015
58. Eriksson PS, Perfilieva E, Björk-Eriksson T, Alborn AM, Nordborg C, Peterson DA, et al. Neurogenesis in the adult human hippocampus. Nat Med (1998) 4:1313–7. doi: 10.1038/3305
59. Kumar A, Pareek V, Faiq MA, Kumar P, Kumari C, Singh HN, et al. Transcriptomic analysis of the neurogenesis signature suggests continued but minimal neurogenesis in the adult human hippocampus. bioRxiv (2019) 6:664995. doi: 10.1101/664995
60. Moore ED, Kooshki M, Wheeler KT, Metheny-Barlow LJ, Robbins ME. Differential expression of homer1a in the hippocampus and cortex likely plays a role in radiation-induced brain injury. Radiat Res (2014) 181:21–32. doi: 10.1667/RR13475.1
61. Gondi V, Hermann BP, Mehta MP, Tomé WA. Hippocampal dosimetry predicts neurocognitive function impairment after fractionated stereotactic radiotherapy for benign or low-grade adult brain tumors. Int J Radiat Oncol Biol Phys (2013) 85:345–54. doi: 10.1016/j.ijrobp.2012.11.031
62. Hoorelbeke D, Decrock E, De Smet M, De Bock M, Descamps B, Van Haver V, et al. Cx43 channels and signaling via IP3/Ca2+, ATP, and ROS/NO propagate radiation-induced DNA damage to non-irradiated brain microvascular endothelial cells. Cell Death Dis (2020) 11(3):194. doi: 10.1038/s41419-020-2392-5
63. Moretti R, Caruso P. An iatrogenic model of brain small-vessel disease: post-radiation encephalopathy. Int J Mol Sci (2020) 21:1–21. doi: 10.3390/ijms21186506
64. Gorbunov NV, Kiang JG. Brain damage and patterns of neurovascular disorder after ionizing irradiation. Complications in radiotherapy and radiation combined injury. Radiat Res (2021) 196:1–16. doi: 10.1667/RADE-20-00147.1
65. Peña LA, Fuks Z, Kolesnick RN. Radiation-induced apoptosis of endothelial cells in the murine central nervous system: protection by fibroblast growth factor and sphingomyelinase deficiency1. Cancer Res (2000) 60:321–7. Available at: https://pubmed.ncbi.nlm.nih.gov/10667583/.
66. Turnquist C, Beck JA, Horikawa I, Obiorah IE, Von Muhlinen N, Vojtesek B, et al. Radiation-induced astrocyte senescence is rescued by Δ133p53. Neuro Oncol (2019) 21:474–85. doi: 10.1093/neuonc/noz001
67. Pospisil P, Kazda T, Hynkova L, Bulik M, Dobiaskova M, Burkon P, et al. Post-WBRT cognitive impairment and hippocampal neuronal depletion measured by in vivo metabolic MR spectroscopy: results of prospective investigational study. Radiother Oncol (2017) 122:373–9. doi: 10.1016/j.radonc.2016.12.013
68. Belka C, Budach W, Kortmann RD, Bamberg M. Radiation induced CNS toxicity - molecular and cellular mechanisms. Br J Cancer (2001) 85:1233–9. doi: 10.1054/bjoc.2001.2100
69. Perry A, Schmidt RE. Cancer therapy-associated CNS neuropathology: an update and review of the literature. Acta Neuropathol (2006) 111:197–212. doi: 10.1007/s00401-005-0023-y
70. Martín BR, Rodríguez EJF, Galve MIR, Hernández JJC. Study of chemotherapy-induced cognitive impairment in women with breast cancer. Int J Environ Res Public Health (2020) 17:1–13. doi: 10.3390/ijerph17238896
71. Brown PD, Gondi V, Pugh S, Tome WA, Wefel JS, Armstrong TS, et al. Hippocampal Avoidance during Whole-Brain Radiotherapy plus Memantine for Patients with Brain Metastases: Phase III Trial NRG Oncology CC001. J Clin Oncol (2020) 38:1019–29. doi: 10.1200/JCO.19.02767
72. Brown PD, Shook S, Laack NN, Wefel JS, Choucair A, Suh JH, et al. Memantine for the prevention of cognitive dysfunction in patients receiving whole-brain radiation therapy (WBRT): first report of RTOG 0614, a placebo-controlled, double-blind, randomized trial. Int J Radiat Oncol (2012) 84:S1–2. doi: 10.1016/j.ijrobp.2012.07.017
73. Jafari A, Siavashpour Z, Houshyari M. An updated review on memantine efficacy in reducing cognitive dysfunction of whole-brain irradiation for adult patients with brain metastasis. Int J Cancer Manag (2021) 14:e111966. doi: 10.5812/ijcm.111966
74. Gondi V, Tome WA, Marsh J, Struck A, Ghia A, Turian JV, et al. Estimated Risk of Perihippocampal Disease Progression after Hippocampal Avoidance during Whole-Brain Radiotherapy: Safety Profile for RTOG 0933. Radiother Oncol (2010) 95:327–31. doi: 10.1016/j.radonc.2010.02.030
75. Gondi V, Pugh SL, Tome WA, Caine C, Corn B, Kanner A, et al. Preservation of memory with conformal avoidance of the hippocampal neural stem-cell compartment during whole-brain radiotherapy for brain metastases (RTOG 0933): A phase II multi-institutional trial. J Clin Oncol (2014) 32:3810–6. doi: 10.1200/JCO.2014.57.2909
76. Monje ML, Mizumatsu S, Fike JR, Palmer TD. Irradiation induces neural precursor-cell dysfunction. Nat Med (2002) 8:955–62. doi: 10.1038/nm749
77. Schroeder T, Bittrich P, Kuhne JF, Noebel C, Leischner H, Fiehler J, et al. Mapping distribution of brain metastases: does the primary tumor matter? J Neurooncol (2020) 147:229–35. doi: 10.1007/s11060-020-03419-6
78. Hong AM, Suo C, Valenzuela M, Haydu LE, Jacobsen KD, Reisse CH, et al. Low incidence of melanoma brain metastasis in the hippocampus. Radiother Oncol (2014) 111:59–62. doi: 10.1016/j.radonc.2014.01.012
79. Harth S, Abo-Madyan Y, Zheng L, Siebenlist K, Herskind C, Wenz F, et al. Estimation of intracranial failure risk following hippocampal-sparing whole brain radiotherapy. Radiother Oncol (2013) 109:152–8. doi: 10.1016/j.radonc.2013.09.009
80. Ghia A, Tomé WA, Thomas S, Cannon G, Khuntia D, Kuo JS, et al. Distribution of brain metastases in relation to the hippocampus: implications for neurocognitive functional preservation. Int J Radiat Oncol Biol Phys (2007) 68:971–7. doi: 10.1016/j.ijrobp.2007.02.016
81. Wan JF, Zhang SJ, Wang L, Zhao K. Le implications for preserving neural stem cells in whole brain radiotherapy and prophylactic cranial irradiation: A review of 2270 metastases in 488 patients. J Radiat Res (2013) 54:285–91. doi: 10.1093/jrr/rrs085
82. Sun B, Huang Z, Wu S, Shen G, Cha L, Meng X, et al. Incidence and relapse risk of intracranial metastases within the perihippocampal region in 314 patients with breast cancer. Radiother Oncol (2016) 118:181–6. doi: 10.1016/j.radonc.2015.11.010
83. Lin SY, Tsan DL, Chuang CC, Yang CC, Pai PC, Wang CL, et al. Oncological outcomes after hippocampus-sparing whole-brain radiotherapy in cancer patients with newly diagnosed brain oligometastases: A single-arm prospective observational cohort study in Taiwan. Front Oncol (2022) 11:784635. doi: 10.3389/fonc.2021.784635
84. Diwanji T, Snider JW, Koroulakis A, Feigenberg SJ, Mohindra P, Kwok Y, et al. Interobserver variability in atlas-based, manual segmentation of the hippocampus in patients with brain metastases. Int J Radiat Oncol (2016) 96:E130. doi: 10.1016/j.ijrobp.2016.06.918
85. Grosu A. SP-0693 whole brain irradiation with hippocampal avoidance. Radiother Oncol (2019) 133:S359–60. doi: 10.1016/s0167-8140(19)31113-2
86. Popp I, Rau S, Hintz M, Schneider J, Bilger A, Fennell JT, et al. Hippocampus-avoidance whole-brain radiation therapy with a simultaneous integrated boost for multiple brain metastases. Cancer (2020) 126:2694–703. doi: 10.1002/cncr.32787
87. Westover KD, Travis Mendel J, Dan T, Kumar K, Gao A, Pulipparacharuv S, et al. Phase II trial of hippocampal-sparing whole brain irradiation with simultaneous integrated boost for metastatic cancer. Neuro Oncol (2020) 22:1831–9. doi: 10.1093/neuonc/noaa092
88. Oehlke O, Wucherpfennig D, Fels F, Frings L, Egger K, Weyerbrock A, et al. Whole brain irradiation with hippocampal sparing and dose escalation on multiple brain metastases: local tumour control and survival. Strahlentherapie und Onkol (2015) 191:461–9. doi: 10.1007/s00066-014-0808-9
89. Grosu AL, Frings L, Bentsalo I, Oehlke O, Brenner F, Bilger A, et al. Whole-brain irradiation with hippocampal sparing and dose escalation on metastases: neurocognitive testing and biological imaging (HIPPORAD) - A phase II prospective randomized multicenter trial (NOA-14, ARO 2015-3, DKTK-ROG). BMC Cancer (2020) 20:532. doi: 10.1186/s12885-020-07011-z
90. de Dios NR, Couñago F, Murcia-Mejía M, Rico-Oses M, Calvo-Crespo P, Samper P, et al. Randomized phase III trial of prophylactic cranial irradiation with or without hippocampal avoidance for small-cell lung cancer (PREMER): A GICOR-GOECP-SEOR study. J Clin Oncol (2021) 39:3118–27. doi: 10.1200/JCO.21.00639
91. Canney P, Murray E, Dixon-Hughes J, Lewsley LA, Paul JA. Prospective randomised phase III clinical trial testing the role of prophylactic cranial radiotherapy in patients treated with trastuzumab for metastatic breast cancer - Anglo Celtic VII. Clin Oncol (2015) 27:460–4. doi: 10.1016/j.clon.2015.04.033
92. Brown PD, Pugh S, Laack NN, Wefel JS, Khuntia D, Meyers C, et al. Memantine for the prevention of cognitive dysfunction in patients receiving whole-brain radiotherapy: A randomized, double-blind, placebo-controlled trial. Neuro Oncol (2013) 15:1429–37. doi: 10.1093/neuonc/not114
93. Lamba N, Mehanna E, Kearney RB, Catalano PJ, Brown PD, Haas-Kogan DA, et al. Prescription of Memantine during Non-Stereotactic, Brain-Directed Radiation among Patients with Brain Metastases: A Population-Based Study. J Neurooncol (2020) 148:509–17. doi: 10.1007/s11060-020-03542-4
94. Keit E, Lee SF, Woodward M, Rembielak A, Shiue K, Desideri I, et al. Palliative whole brain radiation therapy: an international state of practice. Ann Palliat Med (2023) 0:0–0. doi: 10.21037/apm-23-448
95. Slade AN, Stanic S. The impact of RTOG 0614 and RTOG 0933 trials in routine clinical practice: the US survey of utilization of memantine and IMRT planning for hippocampus sparing in patients receiving whole brain radiotherapy for brain metastases. Contemp Clin Trials (2016) 47:74–7. doi: 10.1016/j.cct.2015.12.013
96. Gondi V, Deshmukh S, Brown PD, Wefel JS, Armstrong TS, Tome WA, et al. Sustained preservation of cognition and prevention of patient-reported symptoms with hippocampal avoidance during whole-brain radiation therapy for brain metastases: final results of NRG oncology CC001. Int J Radiat Oncol Biol Phys (2023) 117:571–80. doi: 10.1016/j.ijrobp.2023.04.030
97. Favaudon V, Caplier L, Monceau V, Pouzoulet F, Sayarath M, Fouillade C, et al. Ultrahigh dose-rate FLASH irradiation increases the differential response between normal and tumor tissue in mice. Sci Transl Med (2014) 6(245):245ra93. doi: 10.1126/scitranslmed.3008973
98. Montay-Gruel P, Petersson K, Jaccard M, Boivin G, Germond JF, Petit B, et al. Irradiation in a Flash: Unique Sparing of Memory in Mice after Whole Brain Irradiation with Dose Rates above 100 Gy/S. Radiother Oncol (2017) 124:365–9. doi: 10.1016/j.radonc.2017.05.003
99. Montay-Gruel P, Acharya MM, Petersson K, Alikhani L, Yakkala C, Allen BD, et al. Long-term neurocognitive benefits of FLASH radiotherapy driven by reduced reactive oxygen species. Proc Natl Acad Sci U S A (2019) 166:10943–51. doi: 10.1073/pnas.1901777116
100. van Marlen P, van de Water S, Dahele M, Slotman BJ, Verbakel WFAR. Single ultra-high dose rate proton transmission beam for whole breast FLASH-irradiation: quantification of FLASH-dose and relation with beam parameters. Cancers (Basel) (2023) 15:2579. doi: 10.3390/cancers15092579
101. Zhu H, Xie D, Wang Y, Huang R, Chen X, Yang Y, et al. Comparison of intratumor and local immune response between MV X-ray FLASH and conventional radiotherapies. Clin Transl Radiat Oncol (2023) 38:138–46. doi: 10.1016/j.ctro.2022.11.005
102. Lattery G, Kaulfers T, Cheng C, Zhao X, Selvaraj B, Lin H, et al. Pencil beam scanning bragg peak FLASH technique for ultra-high dose rate intensity-modulated proton therapy in early-stage breast cancer treatment. Cancers (Basel) (2023) 15:4560. doi: 10.3390/cancers15184560
103. Adamo B, Deal AM, Burrows E, Geradts J, Hamilton E, Blackwell KL, et al. Phosphatidylinositol 3-kinase pathway activation in breast cancer brain metastases. Breast Cancer Res (2011) 13(6):R125. doi: 10.1186/bcr3071
104. Olson EM, Abdel-Rasoul M, Maly J, Wu CS, Lin NU, Shapiro CL. Incidence and risk of central nervous system metastases as site of first recurrence in patients with HER2-positive breast cancer treated with adjuvant trastuzumab. Ann Oncol (2013) 24:1526–33. doi: 10.1093/annonc/mdt036
105. Lin NU, Pegram M, Sahebjam S, Ibrahim N, Fung A, Cheng A, et al. Pertuzumab plus high-dose trastuzumab in patients with progressive brain metastases and HER2-positive metastatic breast cancer: primary analysis of a phase II study. J Clin Oncol (2021) 39:2667–75. doi: 10.1200/JCO.20.02822
106. Metro G, Foglietta J, Russillo M, Stocchi L, Vidiri A, Giannarelli D, et al. Clinical outcome of patients with brain metastases from HER2-positive breast cancer treated with lapatinib and capecitabine. Ann Oncol (2011) 22:625–30. doi: 10.1093/annonc/mdq434
107. Bachelot T, Romieu G, Campone M, Diéras V, Cropet C, Dalenc F, et al. Lapatinib plus capecitabine in patients with previously untreated brain metastases from HER2-positive metastatic breast cancer (LANDSCAPE): A single-group phase 2 study. Lancet Oncol (2013) 14:64–71. doi: 10.1016/S1470-2045(12)70432-1
108. Krop IE, Lin NU, Blackwell K, Guardino E, Huober J, Lu M, et al. Trastuzumab Emtansine (T-DM1) versus Lapatinib plus Capecitabine in Patients with HER2-Positive Metastatic Breast Cancer and Central Nervous System Metastases: A Retrospective, Exploratory Analysis in EMILIA. Ann Oncol (2015) 26:113–9. doi: 10.1093/annonc/mdu486
109. Montemurro F, Delaloge S, Barrios CH, Wuerstlein R, Anton A, Brain E, et al. Trastuzumab emtansine (T-DM1) in patients with HER2-positive metastatic breast cancer and brain metastases: exploratory final analysis of cohort 1 from KAMILLA, a single-arm phase IIIb clinical trial☆. Ann Oncol (2020) 31:1350–8. doi: 10.1016/j.annonc.2020.06.020
110. Bartsch R, Berghoff AS, Furtner J, Marhold M, Bergen ES, Roider-Schur S, et al. Trastuzumab deruxtecan in HER2-positive breast cancer with brain metastases: A single-arm, phase 2 trial. Nat Med (2022) 28:1840–7. doi: 10.1038/s41591-022-01935-8
111. Murthy RK, Loi S, Okines A, Paplomata E, Hamilton E, Hurvitz SA, et al. Tucatinib, trastuzumab, and capecitabine for HER2-positive metastatic breast cancer. N Engl J Med (2020) 382:597–609. doi: 10.1056/nejmoa1914609
112. Hurvitz SA, Saura C, Oliveira M, Trudeau ME, Moy B, Delaloge S, et al. Efficacy of neratinib plus capecitabine in the subgroup of patients with central nervous system involvement from the NALA trial. Oncologist (2021) 26:e1327–38. doi: 10.1002/onco.13830
113. Lin Y, Lin M, Zhang J, Wang B, Tao Z, Du Y, et al. Real-world data of pyrotinib-based therapy in metastatic HER2-positive breast cancer: promising efficacy in lapatinib-treated patients and in brain metastasis. Cancer Res Treat (2020) 52:1059–66. doi: 10.4143/crt.2019.633
114. Tian W, Hao S, Wang L, Chen Y, Li Z, Luo D. Pyrotinib treatment enhances the radiosensitivity in HER2-positive brain metastatic breast cancer patients. Anticancer Drugs (2022) 33:e622–7. doi: 10.1097/CAD.0000000000001199
115. Schmid P, Adams S, Rugo HS, Schneeweiss A, Barrios CH, Iwata H, et al. Atezolizumab and nab-paclitaxel in advanced triple-negative breast cancer. N Engl J Med (2018) 379:2108–21. doi: 10.1056/nejmoa1809615
116. Tolaney SM, Sahebjam S, Le Rhun E, Bachelot T, Kabos P, Awada A, et al. A phase II study of abemaciclib in patients with brain metastases secondary to hormone receptor-positive breast cancer. Clin Cancer Res (2020) 26:5310–9. doi: 10.1158/1078-0432.CCR-20-1764
117. Litton JK, Rugo HS, Ettl J, Hurvitz SA, Gonçalves A, Lee K-H, et al. Talazoparib in patients with advanced breast cancer and a germline BRCA mutation. N Engl J Med (2018) 379:753–63. doi: 10.1056/nejmoa1802905
118. Mehta MP, Wang D, Wang F, Kleinberg L, Brade A, Robins HI, et al. Veliparib in combination with whole brain radiation therapy in patients with brain metastases: results of a phase 1 study. J Neurooncol (2015) 122:409–17. doi: 10.1007/s11060-015-1733-1
119. Jenkins S, Zhang W, Steinberg SM, Nousome D, Houston N, Wu X, et al. Phase I study and cell-free DNA analysis of T-DM1 and metronomic temozolomide for secondary prevention of HER2-positive breast cancer brain metastases. Clin Cancer Res (2023) 29:OF1–OF10. doi: 10.1158/1078-0432.ccr-22-0855
120. Platini C, Long J, Walter S. Meningeal carcinomatosis from breast cancer treated with intrathecal trastuzumab. Lancet Oncol (2006) 7:778–80. doi: 10.1016/S1470-2045(06)70864-6
121. He C, Cai P, Li J, Zhang T, Lin L, Abbasi AZ, et al. Blood-brain barrier-penetrating amphiphilic polymer nanoparticles deliver docetaxel for the treatment of brain metastases of triple negative breast cancer. J Control Release (2017) 246:98–109. doi: 10.1016/j.jconrel.2016.12.019
122. De Mattos-Arruda L. Liquid biopsy for HER2-positive breast cancer brain metastasis: the role of the cerebrospinal fluid. ESMO Open (2017) 2(4):e000270. doi: 10.1136/esmoopen-2017-000270
123. Wang Y, Ye F, Liang Y, Yang Q. Breast cancer brain metastasis: insight into molecular mechanisms and therapeutic strategies. Br J Cancer (2021) 125:1056–67. doi: 10.1038s41416-021-01424-8
124. Sun H, Xu J, Dai S, Ma Y, Sun T. Breast cancer brain metastasis: current evidence and future directions. Cancer Med (2023) 12:1007–24. doi: 10.1002/cam4.5021
125. Lin NU, Borges V, Anders C, Murthy RK, Paplomata E, Hamilton E, et al. Intracranial efficacy and survival with tucatinib plus trastuzumab and capecitabine for previously treated HER2-positive breast cancer with brain metastases in the HER2CLIMB trial. J Clin Oncol (2020) 38:2610–9. doi: 10.1200/JCO.20.00775
Keywords: brain metastases, radiation-related neurotoxicity, hippocampus-avoiding radiotherapy, radiosurgery, cognitive function
Citation: Buczek D, Zaucha R and Jassem J (2024) Neurotoxicity-sparing radiotherapy for brain metastases in breast cancer: a narrative review. Front. Oncol. 13:1215426. doi: 10.3389/fonc.2023.1215426
Received: 01 May 2023; Accepted: 19 December 2023;
Published: 02 February 2024.
Edited by:
Mattia Falchetto Osti, Sapienza University of Rome, ItalyReviewed by:
DeeDee Smart, National Cancer Institute (NIH), United StatesRaees Tonse, Baptist Hospital of Miami, United States
Copyright © 2024 Buczek, Zaucha and Jassem. This is an open-access article distributed under the terms of the Creative Commons Attribution License (CC BY). The use, distribution or reproduction in other forums is permitted, provided the original author(s) and the copyright owner(s) are credited and that the original publication in this journal is cited, in accordance with accepted academic practice. No use, distribution or reproduction is permitted which does not comply with these terms.
*Correspondence: Renata Zaucha, cnphdWNoYUBndW1lZC5lZHUucGw=
†These authors have contributed equally to this work
‡ORCID: Dagmara Buczek, orcid.org/0000-0002-3588-3400
Renata Zaucha, orcid.org/0000-0001-8503-1559
Jacek Jassem, orcid.org/0000-0002-8875-6747