- 1Guangdong Provincial Key Laboratory of Colorectal and Pelvic Floor Diseases, Guangdong Institute of Gastroenterology, The Sixth Affiliated Hospital, Sun Yat-sen University, Guangzhou, China
- 2Department of General Surgery (Colorectal Surgery), The Sixth Affiliated Hospital, Sun Yat-sen University, Guangzhou, China
- 3Biomedical Innovation Center, The Sixth Affiliated Hospital, Sun Yat-sen University, Guangzhou, China
- 4Institute of Basic Medical Sciences, School of Life Sciences and Biopharmaceuticals, Guangdong Pharmaceutical University, Guangzhou, China
- 5State Key Laboratory for Liver Research, The University of Hong Kong, Hong Kong, Hong Kong SAR, China
Hepatocellular carcinoma (HCC) is a heterogeneous and aggressive liver cancer that presents limited treatment options. Despite being the standard therapy for advanced HCC, sorafenib frequently encounters resistance, emphasizing the need to uncover the underlying mechanisms and develop effective treatments. This comprehensive review highlights the crucial interplay between the tumor microenvironment, cancer stem cells (CSCs), and epithelial-mesenchymal transition (EMT) in the context of sorafenib resistance. The tumor microenvironment, encompassing hypoxia, immune cells, stromal cells, and exosomes, exerts a significant impact on HCC progression and therapy response. Hypoxic conditions and immune cell infiltration create an immunosuppressive milieu, shielding tumor cells from immune surveillance and hindering therapeutic efficacy. Additionally, the presence of CSCs emerges as a prominent contributor to sorafenib resistance, with CD133+ CSCs implicated in drug resistance and tumor initiation. Moreover, CSCs undergo EMT, a process intimately linked to tumor progression, CSC activation, and further promotion of sorafenib resistance, metastasis, and tumor-initiating capacity. Elucidating the correlation between the tumor microenvironment, CSCs, and sorafenib resistance holds paramount importance in the quest to develop reliable biomarkers capable of predicting therapeutic response. Novel therapeutic strategies must consider the influence of the tumor microenvironment and CSC activation to effectively overcome sorafenib resistance in HCC.
Introduction
Primary liver cancer was identified as the sixth most commonly diagnosed cancer and the third leading cause of cancer-related deaths worldwide in 2020, ranking only below lung and colorectal cancer in terms of mortality rates (1). The predominant form of primary liver cancer is hepatocellular carcinoma (HCC), accounting for 75% to 85% of all cases (1). Despite significant advancements in HCC treatment, clinical outcomes remain unsatisfactory, with over half of patients experiencing relapse within five years (2).
For patients with advanced-stage HCC, the first-line treatment since 2008 has been the multi-kinase inhibitor sorafenib, which has demonstrated efficacy in extending patients’ survival time (3). However, the development of drug resistance typically occurs within six months of treatment (4), necessitating the urgent exploration of novel therapeutic regimens to overcome sorafenib resistance.
Sorafenib, a multi-target tyrosine kinase inhibitor (TKI), has exhibited potent anti-angiogenic and anti-proliferative effects on tumor cells. Notably, sorafenib achieves tumor angiogenesis inhibition by targeting the hepatocyte cytokine receptor, platelet-derived growth factor receptor, and vascular endothelial growth factor receptor 2 (VEGFR-2) (5). Furthermore, it hinders tumor proliferation by focusing on RAF-1, b-Raf, and kinase activity in the Ras/Raf/MEK/ERK signaling pathway (6). Despite the introduction of alternative treatment strategies such as lenvatinib, regorafenib, and immune checkpoint inhibitors, sorafenib retains a prominent position in clinical settings, supported by substantial evidence.
Elucidating the factors contributing to sorafenib resistance holds immense significance in improving treatment outcomes for hepatocellular carcinoma (HCC) patients. Recent studies have emphasized the crucial roles played by the tumor microenvironment (TME) and cancer stem cells (CSCs) in drug resistance development. In this review, we provide an overview of recent findings regarding hypoxia, the immune microenvironment, viral reactivation, and exosomes within the TME, alongside the distinctive features of CSCs that contribute to sorafenib resistance. This comprehensive understanding facilitates the advancement of novel clinical implementations and treatment selection (Figure 1).
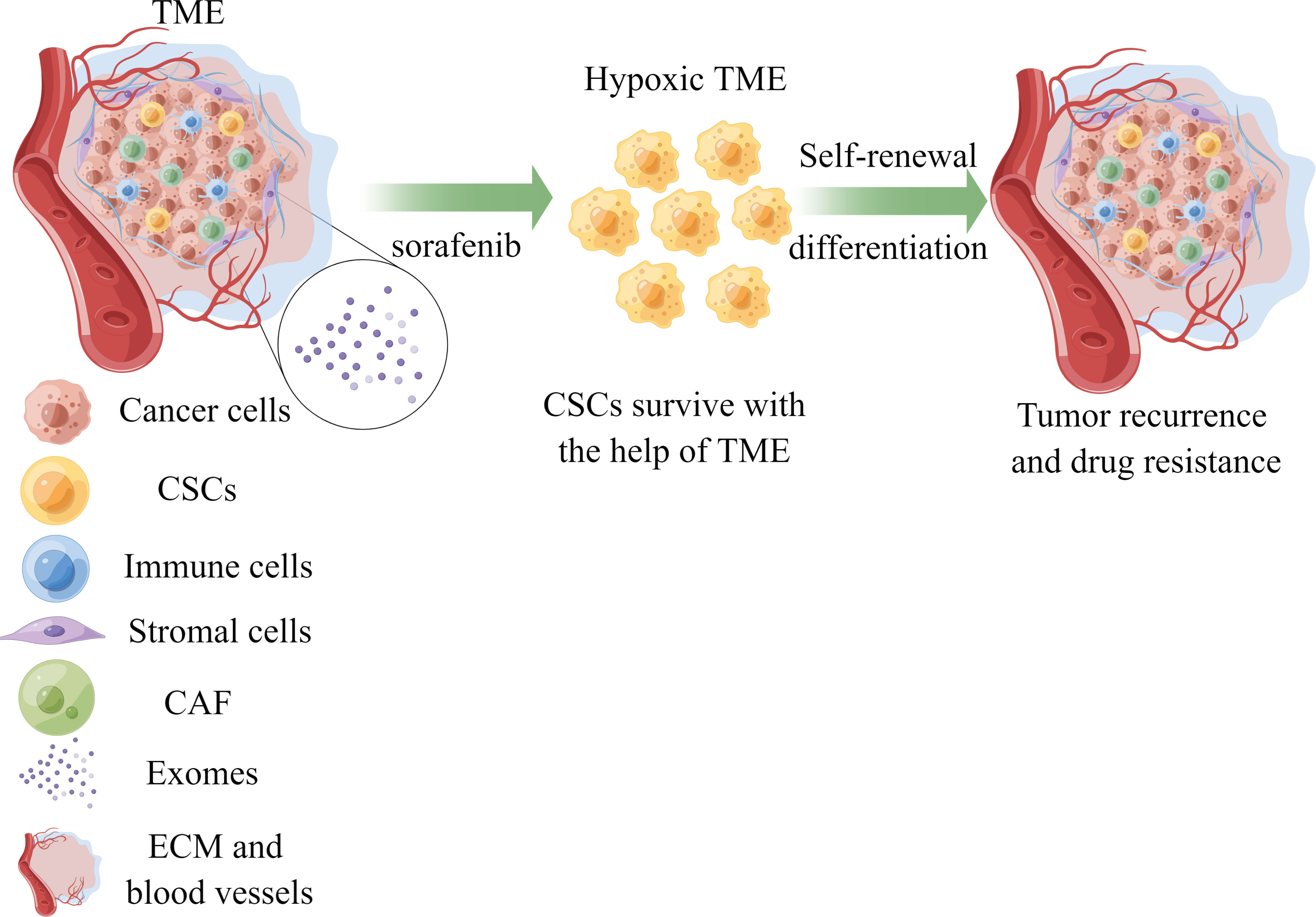
Figure 1 The role of TME and CSCs in sorafenib resistance of HCC. Cancer stem cells could survive with the help of components of TME under the treatment of sorafenib, leading to drug resistance and tumor recurrence. TME, tumor microenvironment; CSCs, cancer stem cells; CAF, cancer-associated fibroblasts; ECM, extracellular matrix; HCC, hepatocellular carcinoma.
Tumor microenvironment and sorafenib resistance of HCC
The tumor microenvironment (TME) comprises tumor cells, cancer-associated stromal cells, immune cells, secreted products (such as cytokines and chemokines) from these cells, and non-cellular components of the extracellular matrix (ECM) (7). The TME significantly influences tumor initiation and progression. Recent studies have underscored the critical role of interactions between tumor and non-tumor cells in hepatocellular carcinoma (HCC) metastasis (3). Furthermore, non-cellular proteins or molecules within the TME have been implicated in profoundly affecting tumor cell behavior (2). The major effects and molecular signaling of TME components on sorafenib resistance in HCC are summarized in Table 1.
Hypoxia
The hypoxic environment in hepatocellular carcinoma (HCC) contributes to angiogenesis, invasiveness, and chemoresistance by upregulating the expression of vascular endothelial growth factor (VEGF) and hypoxia-inducible factor 1α (HIF-1α) (8). HIFs are transcription factors (TFs) composed of key members such as HIF-1α, HIF-2α, HIF-3α, and HIF-β, which regulate tumor proliferation, angiogenesis, and metastasis through hypoxia-induced signaling pathways. Previous studies have demonstrated the crucial roles of both HIF-1α and HIF-2α in HCC initiation (9). Overexpression of HIF-1α has been observed in HCC patients and correlates with poor clinical outcomes (9).
A causal loop exists between acquired sorafenib resistance and the hypoxic tumor microenvironment. Multiple studies have indicated that continuous sorafenib treatment induces tumor hypoxia, leading to the selection of tumor clones that adapt to a hypoxic environment by activating HIF-1α and NF-κB, thereby diminishing the efficacy of sorafenib (10). Nuclear translocation of HIF-1α and HIF-2α is indispensable for HCC cells to develop sorafenib resistance and regain angiogenesis and proliferation capabilities (11, 12). Other studies have found that sorafenib triggers a switch from HIF-1α-dependent to HIF-2α-dependent pathways, promoting the expression of VEGF and cyclin D1, thus enhancing sorafenib resistance and tumor growth (13, 14). Increased expression of HIF-2α induced by sorafenib activates the TGF-α/EGFR pathway, leading to drug resistance (15). Given that HIF proteins play a significant role in sorafenib resistance, various approaches have been explored to target HIF proteins using small molecules or microRNAs (28). For example, Xu et al. reported that Mir-338-3p sensitized HCC cells to sorafenib by targeting HIF-1α (16). Additionally, the HIF-2α inhibitor PT-2385 was found to significantly improve sorafenib efficacy by upregulating the androgen receptor and suppressing downstream pSTAT3/pAKT/pERK signaling (17). Collectively, these findings highlight the tight association between abnormal expression of HIF proteins and sorafenib resistance in HCC, suggesting a causal relationship between hypoxia and the therapeutic efficacy of sorafenib. On the other hand, clinical sorafenib treatment can activate the C-X-C receptor type 4 (CXCR4)/stromal-derived factor-1α (SDF-1α) axis, aggravate intratumoral hypoxia in hepatocellular carcinoma (HCC), and further lead to hepatocellular carcinoma. Therefore, a multifunctional oxygen delivery nanoplatform was rationally constructed based on oxygen-saturated perfluorohexane (PFH) core liposomes, with the CXCR4 antagonist LFC131 peptide modified on the surface to simultaneously deliver sorafenib and the CSF1/CSF1R inhibitor PLX3397 (named PFH@LSLP) for the treatment of HCC resistant to sorafenib (18). Targeting these molecules could offer an effective therapeutic strategy to overcome sorafenib resistance in HCC.
Immune microenvironment
The immune microenvironment plays a crucial role in the malignant transformation and progression of hepatocellular carcinoma (HCC), and significant advancements have been made in immunotherapy strategies in recent years. Emerging evidence suggests a close correlation between the tumor immune microenvironment and drug resistance. For instance, the infiltration of tumor-associated macrophages (TAMs) has been reported to be involved in regulating the sensitivity of HCC to sorafenib (29–31). Studies have shown that TAM infiltration occurs concomitantly with the tumor epithelial-to-mesenchymal transition (EMT) status (19). This phenomenon may be attributed to the ability of TAMs to induce EMT and enhance stemness features in tumor cells, contributing to sorafenib resistance (20). However, the underlying mechanisms of TAM-mediated tumor cell progression remain largely unknown. Cytokines or chemokines secreted by TAMs are often the key mediators of communication between TAMs and cancer cells. For instance, hepatocyte growth factor (HGF) produced by M2 macrophages has been shown to promote tumor growth, migration, and invasion (21). Infiltration levels of M2 TAMs and HGF expression were found to be significantly increased in drug-resistant tumor cells compared to sorafenib-sensitive tumors (21). Mechanistic studies have revealed that HGF mediates drug resistance in HCC through the activation of the HGF/C-Met, ERK1/2/MAPK, and PI3K/AKT pathways (21). Considering the pivotal role of TAMs in supporting cancer cell survival and progression, therapeutic agents targeting TAMs are being rapidly developed, with some clinical trials demonstrating promising results and improved clinical outcomes (32).
Neutrophils, another component of the immune microenvironment, exert significant influence on the behavior of cancer cells. The impact of neutrophils on cancer cell proliferation and metastasis can vary depending on the specific tumor microenvironment (31). Zhou and colleagues investigated the role of tumor-associated neutrophils (TANs) in HCC progression and found that patients who received preoperative sorafenib treatment exhibited significantly higher levels of TAN infiltration compared to those who did not receive sorafenib. This finding was further validated in xenograft mouse models, where sorafenib treatment led to increased TAN infiltration along with enhanced expression of CCL2 and CCL17. Depletion of TANs combined with sorafenib administration achieved the greatest suppression of tumor growth in mice compared to using sorafenib alone (31).
Over more, cancer-associated fibroblasts (CAFs) play a significant role in chemo-resistance induction, promoting tumor cell proliferation, invasiveness, and metastatic potential in various cancers, including HCC (33, 34). CAF-derived SPP1 was identified to enhance TKI resistance in HCC by bypassing oncogenic signaling activation and promoting EMT. Targeting CAF-derived SPP1 represents a promising therapeutic approach against TKI resistance in HCC (35).
Furthermore, the immune microenvironment is regulated by hypoxia. Studies have demonstrated that sorafenib-induced hypoxia can upregulate the expression of CXCL5 and IL-1β in HCC cells in a HIF-dependent manner (22, 31). This, in turn, recruits peripheral blood neutrophils to the tumor site, where they differentiate into TANs. Under hypoxic conditions, TAMs and regulatory T cells (Tregs) are also activated, collectively promoting tumor angiogenesis (31). Gao et al. revealed that targeting intratumoral stem-like CCR4+ regulatory T cells (Tregs) enhances antitumor immunity, overcomes sorafenib resistance, and sensitizes tumors to PD-1 checkpoint blockade. Inhibiting Treg infiltration into the tumor microenvironment using a CCR4 antagonist or N-CCR4-Fc effectively reduces Treg accumulation. These findings suggest that targeting intratumoral CCR4+ Tregs holds promise as a therapeutic strategy to improve immune responses and overcome sorafenib resistance (24). Continued sorafenib treatment has been observed to cause tumor immunosuppression characterized by increased expression of programmed death ligand 1 (PD-L1) and accumulation of Treg cells, leading to tumor recurrence (36, 37). In light of these observations, the combination of immune checkpoint blockade with sorafenib holds great promise for the treatment of HCC.
Exosomes
Exosomes, which are small extracellular vesicles (EVs) derived from stromal cells or tumor cells, play a critical role in cell-to-cell communication within the tumor microenvironment (TME). Numerous studies have highlighted the involvement of exosomes in the regulation of sorafenib sensitivity both in vitro and in vivo (23). For instance, exosomes secreted by adipose tissue mesenchymal stem cells (AMSCs) were found to carry and deliver Mir-122, a microRNA that mediates the communication between AMSCs and HCC cells. Mir-122, upon transport into tumor cells, regulates the sensitivity of HCC to sorafenib by modulating the expression of target genes. Intratumoral injection of Mir-122-loaded exosomes significantly enhanced the anti-tumor effect of sorafenib in HCC mouse models, underscoring the therapeutic potential of exosomes in cancer treatment (25). In addition to microRNAs, long non-coding RNAs (lncRNAs) have also been implicated in exosome-mediated drug resistance. Studies have revealed that sorafenib treatment increases the expression of lncRNA-VLDLR in tumor cells, which subsequently leads to the release of EVs. Incubation of tumor cells with EVs containing lncRNA-VLDLR significantly reduces their sensitivity to sorafenib, confirming the involvement of exosomes in drug resistance (26).
Given the involvement of exosomes in various aspects of tumor behavior, exosomes loaded with specific functional molecules hold promise as an effective therapeutic strategy. For instance, Li et al. demonstrated that exosomes modified with Si-GRP78, which targets the oncogene GRP78, inhibit HCC cell proliferation and invasion while enhancing sensitivity to sorafenib treatment (27). These findings collectively suggest that exosome-mediated intercellular communication can be harnessed to develop innovative strategies for overcoming drug resistance in cancer treatment.
Cancer stem cell and sorafenib resistance of HCC
Cancer stem cells (CSCs) represent a distinct subset of tumor cells that possess stem cell-like properties, including self-renewal and the ability to differentiate (38). The presence of CSCs contributes to tumor heterogeneity, therapy resistance, and tumor recurrence (39). The origin of CSCs is a subject of ongoing debate. Some studies suggest that CSCs may arise from tissue stem cells, while others propose that they originate from dedifferentiation or cell fate transition of tumor cells (38). Emerging evidence indicates that the epithelial-mesenchymal transition (EMT) process plays a role in the generation of CSCs associated with drug resistance (39). In this review, we focus on the potential mechanisms underlying sorafenib resistance mediated by CSCs, taking into account the characteristics of EMT and tumor heterogeneity. Furthermore, we discuss how this knowledge may contribute to the development of novel therapeutic approaches to overcome sorafenib resistance in advanced HCC.
CSCs contribute to tumor heterogenicity
Hepatocellular carcinoma (HCC) exhibits high genomic and transcriptomic heterogeneity, contributing to its ability for unlimited proliferation, distant metastasis, and therapy resistance (40). This heterogeneity is driven by the self-renewal and differentiation capabilities of cancer stem cells (CSCs), which give rise to distinct subpopulations within the tumor with varying sensitivity to treatment (41). Single-cell RNA sequencing studies have revealed that CSC populations differ among HCC patients, underscoring the heterogeneity of CSCs (42, 43). Key transcription factors such as NANOG, SOX2, OCT4, KLF4, and c-MYC regulate the stemness features of CSCs, enabling tumors to sustain self-renewal (44). Various surface markers, including CD133, CD90, CD24, and CD44, are utilized to identify and isolate CSCs from the bulk tumor. CD133+ CSCs have been associated with increased drug resistance and tumor initiation potential (44).
Developmental signaling pathways, such as Hedgehog, Hippo, Notch, TGF-β, and Wnt/β-catenin, play crucial roles in regulating CSCs and driving tumor heterogeneity, therapy resistance, and cancer progression (45). For instance, Notch signaling components, including Notch and Jagged proteins, are significantly upregulated in CD133+ CSCs of HCC (46). Researchers have identified an expanding repertoire of biomarkers and driver genes involved in the activation of CSCs. Targeting these drivers may hold promise for eliminating CSCs and reversing therapeutic resistance in HCC. Recently, a research find the overexpression of SERPINA12 in hepatocellular carcinoma (HCC) is associated with aggressive clinical features and stemness characteristics. This upregulation is mediated by TCF7L2 binding to the SERPINA12 promoter, resulting in enhanced transcriptional activity (47). SERPINA12 interacts with GRP78, activating the AKT/GSK3β/β-catenin pathway and forming a positive feedback loop. In an immunocompetent HCC mouse model, intravenous administration of rAAV8-shSERPINA12 sensitized HCC cells to sorafenib and suppressed the cancer stem cell subset. Targeting SERPINA12 holds promise for improving sorafenib efficacy and inhibiting HCC stemness (47). Meanwhile, Luo et al. found that GLI1 editing promotes metabolic shift to oxidative phosphorylation (OXPHOS) to control stress and stem cell-like state through PINK1-Parkin-mediated mitophagy in HCC, thereby endowing it with unique metastatic and sola Fenil resistance (48).
CSCs undergo EMT
Indeed, the epithelial-mesenchymal transition (EMT) process plays a critical role in the regulation of cancer stem cell (CSC) features and contributes to tumor aggressiveness and therapy resistance. EMT is a complex cellular reprogramming process in which epithelial cells lose their differentiated characteristics and acquire mesenchymal traits (49). Tumor cells undergoing EMT exhibit enhanced migratory, invasive, and anti-apoptotic capabilities (50). Growing evidence suggests that EMT is closely intertwined with CSCs and influences their behavior (51).
During EMT, tumor cells can undergo a reversible transition to a mesenchymal phenotype, leading to the acquisition of CSC-like properties, such as increased metastatic potential and tumor-initiating capacity. For example, liver CSCs characterized by the expression of the RALY RNA binding protein like (RALYL) exhibit reversible EMT states and demonstrate enhanced metastasis and tumor initiation abilities (52). Poorly differentiated HCC with stem cell characteristics is strongly associated with an EMT phenotype, which is often associated with a lower response to chemotherapy and poorer survival outcomes (39).
Several studies have demonstrated that CSC enrichment and activation of EMT signaling pathways are associated with sorafenib resistance in HCC (53–55). Furthermore, the induction of EMT and CSC signaling has been identified as key mechanisms contributing to tumor recurrence in HCC patients treated with radiofrequency ablation (RFA) for local control of the disease (56, 57). Moreover, the researchers shed light on the presence of a small subset of quiescent stem-like cells within HCC cell populations, which exhibit intrinsic resistance to sorafenib. Through comprehensive investigations utilizing single-cell sequencing and in vitro experiments, the study provides compelling evidence elucidating the pivotal role of stemness and EMT in driving sorafenib resistance at the single-cell level. The findings underscore the importance of considering the unique characteristics of these quiescent stem-like cells and their association with EMT in the development of targeted therapeutic strategies to overcome sorafenib resistance in HCC (58). Understanding the intricate relationship between CSCs and EMT is crucial for the development of novel treatment approaches to overcome sorafenib resistance in HCC. By targeting the molecular pathways involved in CSCs and EMT, researchers may uncover potential therapeutic strategies to improve the outcomes of HCC patients and reduce tumor recurrence.
Conclusions
Indeed, the tumor microenvironment, including factors such as hypoxia, immune cells, stromal cells, and exosomes, plays a crucial role in promoting sorafenib resistance in HCC. Additionally, CSCs and EMT activation contribute to drug resistance, tumor metastasis, and relapse. In conclusion, future studies should focus on elucidating the correlation between the tumor microenvironment, cancer stemness, and sorafenib sensitivity, as well as identifying reliable biomarkers for predicting the response to sorafenib. Additionally, novel therapeutic methods targeting sorafenib-resistant HCC should be developed, considering the influence of the tumor microenvironment and CSC activation. By addressing these challenges, we can advance our understanding and develop effective strategies to overcome sorafenib resistance in HCC.
Author contributions
SQC wrote a paper, including manuscripts, images, and tables. QY and XYG provided guidance and suggestions for the paper, and made revisions to the paper. YQD helped modify part of the content.
Funding
This work was supported by the National Natural Science Foundation of China (Grant No. 81903049 and 82273345), the Natural Science Foundation of Guangdong Province (No. 2023A1515010563), Science and Technology Program of Guangzhou (No.2023A04J1818).
Conflict of interest
The authors declare that the research was conducted in the absence of any commercial or financial relationships that could be construed as a potential conflict of interest.
Publisher’s note
All claims expressed in this article are solely those of the authors and do not necessarily represent those of their affiliated organizations, or those of the publisher, the editors and the reviewers. Any product that may be evaluated in this article, or claim that may be made by its manufacturer, is not guaranteed or endorsed by the publisher.
Abbreviations
HCC, hepatocellular carcinoma; TME, tumor microenvironment; CSCs, cancer stem cells; TKI, tyrosine kinase inhibitor; VEGFR, vascular endothelial growth factor receptor; PDGFR-β, platelet-derived growth factor receptor; ECM, extracellular matrix; VEGF, vascular endothelial growth factor; HIF-1α, hypoxia-inducible factor 1α; TFs, transcription factors; TAMs, tumor-associated macrophages; EMT, epithelial-to-mesenchymal transition; HGF, hepatocyte growth factor; TANs, tumor-associated neutrophils; Treg, regulatory T cells; PD-L1, programmed death ligand 1; MSCs, mesenchymal stem cells; CAF, cancer-associated fibroblasts; LAMA5, laminin 5; EVs, extracellular vesicles; AMSCs, adipose tissue mesenchymal stem cells; lncRNA, long non-coding RNA; DCs, dendritic cells.
References
1. Sung H, Ferlay J, Siegel RL, Laversanne M, Soerjomataram I, Jemal A, et al. Global cancer statistics 2020: GLOBOCAN estimates of incidence and mortality worldwide for 36 cancers in 185 countries. CA Cancer J Clin (2021) 71(3):209–49. doi: 10.3322/caac.21660
2. Forner A, Reig M, Bruix J. Hepatocellular carcinoma. Lancet (2018) 391(10127):1301–14. doi: 10.1016/S0140-6736(18)30010-2
3. Villanueva A. Hepatocellular carcinoma. N Engl J Med (2019) 380(15):1450–62. doi: 10.1056/NEJMra1713263
4. Bejjani AC, Finn RS. Hepatocellular carcinoma: pick the winner-tyrosine kinase inhibitor versus immuno-oncology agent-based combinations. J Clin Oncol (2022) 40(24):2763–73. doi: 10.1200/JCO.21.02605
5. Vogel A, Meyer T, Sapisochin G, Salem R, Saborowski A. Hepatocellular carcinoma. Lancet (2022) 400(10360):1345–62. doi: 10.1016/S0140-6736(22)01200-4
6. Akula SM, Abrams SL, Steelman LS, Emma MR, Augello G, Cusimano A, et al. RAS/RAF/MEK/ERK, PI3K/PTEN/AKT/mTORC1 and TP53 pathways and regulatory miRs as therapeutic targets in hepatocellular carcinoma. Expert Opin Ther Targets (2019) 23(11):915–29. doi: 10.1080/14728222.2019.1685501
7. Xiao Y, Yu D. Tumor microenvironment as a therapeutic target in cancer. Pharmacol Ther (2021) 221:107753. doi: 10.1016/j.pharmthera.2020.107753
8. Liu LP, Ho RL, Chen GG, Lai PB. Sorafenib inhibits hypoxia-inducible factor-1α synthesis: implications for antiangiogenic activity in hepatocellular carcinoma. Clin Cancer Res (2012) 18(20):5662–71. doi: 10.1158/1078-0432.CCR-12-0552
9. Xia S, Pan Y, Liang Y, Xu J, Cai X. The microenvironmental and metabolic aspects of sorafenib resistance in hepatocellular carcinoma. EBioMedicine (2020) 51:102610. doi: 10.1016/j.ebiom.2019.102610
10. Liang Y, Zheng T, Song R, Wang J, Yin D, Wang L, et al. Hypoxia-mediated sorafenib resistance can be overcome by EF24 through Von hippel-lindau tumor suppressor-dependent HIF-1α inhibition in hepatocellular carcinoma. Hepatology (2013) 57(5):1847–57. doi: 10.1002/hep.26224
11. Song Z, Liu T, Chen J, Ge C, Zhao F, Zhu M, et al. HIF-1α-induced RIT1 promotes liver cancer growth and metastasis and its deficiency increases sensitivity to sorafenib. Cancer Lett (2019) 460:96–107. doi: 10.1016/j.canlet.2019.06.016
12. Dong XF, Liu TQ, Zhi XT, Zou J, Zhong JT, Li T, et al. COX-2/PGE2 axis regulates HIF2α activity to promote hepatocellular carcinoma hypoxic response and reduce the sensitivity of sorafenib treatment. Clin Cancer Res (2018) 24(13):3204–16. doi: 10.1158/1078-0432.CCR-17-2725
13. Tang W, Chen Z, Zhang W, Cheng Y, Zhang B, Wu F, et al. The mechanisms of sorafenib resistance in hepatocellular carcinoma: theoretical basis and therapeutic aspects. Signal Transduct Target Ther (2020) 5(1):87. doi: 10.1038/s41392-020-0187-x
14. Méndez-Blanco C, Fondevila F, García-Palomo A, González-Gallego J, Mauriz JL. Sorafenib resistance in hepatocarcinoma: role of hypoxia-inducible factors. Exp Mol Med (2018) 50(10):1–9. doi: 10.1038/s12276-018-0159-1
15. Zhao D, Zhai B, He C, Tan G, Jiang X, Pan S, et al. Upregulation of HIF-2α induced by sorafenib contributes to the resistance by activating the TGF-α/EGFR pathway in hepatocellular carcinoma cells. Cell Signal (2014) 26(5):1030–9. doi: 10.1016/j.cellsig.2014.01.026
16. Xu H, Zhao L, Fang Q, Sun J, Zhang S, Zhan C, et al. MiR-338-3p inhibits hepatocarcinoma cells and sensitizes these cells to sorafenib by targeting hypoxia-induced factor 1α. PloS One (2014) 9(12):e115565. doi: 10.1371/journal.pone.0115565
17. Xu J, Zheng L, Chen J, Sun Y, Lin H, Jin RA, et al. Increasing AR by HIF-2α inhibitor (PT-2385) overcomes the side-effects of sorafenib by suppressing hepatocellular carcinoma invasion via alteration of pSTAT3, pAKT and pERK signals. Cell Death Dis (2017) 8(10):e3095. doi: 10.1038/cddis.2017.411
18. Wang Y, Wang Z, Jia F, Xu Q, Shu Z, Deng J, et al. CXCR4-guided liposomes regulating hypoxic and immunosuppressive microenvironment for sorafenib-resistant tumor treatment. Bioact Mater (2022) 17:147–61. doi: 10.1016/j.bioactmat.2022.01.003
19. Pastushenko I, Brisebarre A, Sifrim A, Fioramonti M, Revenco T, Boumahdi S, et al. Identification of the tumour transition states occurring during EMT. Nature (2018) 556(7702):463–8. doi: 10.1038/s41586-018-0040-3
20. Fan QM, Jing YY, Yu GF, Kou XR, Ye F, Gao L, et al. Tumor-associated macrophages promote cancer stem cell-like properties via transforming growth factor-beta1-induced epithelial-mesenchymal transition in hepatocellular carcinoma. Cancer Lett (2014) 352(2):160–8. doi: 10.1016/j.canlet.2014.05.008
21. Dong N, Shi X, Wang S, Gao Y, Kuang Z, Xie Q, et al. M2 macrophages mediate sorafenib resistance by secreting HGF in a feed-forward manner in hepatocellular carcinoma. Br J Cancer (2019) 121(1):22–33. doi: 10.1038/s41416-019-0482-x
22. Zhang J, Zhang Q, Lou Y, Fu Q, Chen Q, Wei T, et al. Hypoxia-inducible factor-1α/interleukin-1β signaling enhances hepatoma epithelial-mesenchymal transition through macrophages in a hypoxic-inflammatory microenvironment. Hepatology (2018) 67(5):1872–89. doi: 10.1002/hep.29681
23. Qu Z, Wu J, Wu J, Luo D, Jiang C, Ding Y. Exosomes derived from HCC cells induce sorafenib resistance in hepatocellular carcinoma both in vivo and in vitro. J Exp Clin Cancer Res (2016) 35(1):159. doi: 10.1186/s13046-016-0430-z
24. Gao Y, You M, Fu J, Tian M, Zhong X, Du C, et al. Intratumoral stem-like CCR4+ regulatory T cells orchestrate the immunosuppressive microenvironment in HCC associated with hepatitis b. J Hepatol (2022) 76(1):148–59. doi: 10.1016/j.jhep.2021.08.029
25. Lou G, Song X, Yang F, Wu S, Wang J, Chen Z, et al. Exosomes derived from miR-122-modified adipose tissue-derived MSCs increase chemosensitivity of hepatocellular carcinoma. J Hematol Oncol (2015) 8:122. doi: 10.1186/s13045-015-0220-7
26. Takahashi K, Yan IK, Wood J, Haga H, Patel T. Involvement of extracellular vesicle long noncoding RNA (linc-VLDLR) in tumor cell responses to chemotherapy. Mol Cancer Res (2014) 12(10):1377–87. doi: 10.1158/1541-7786.MCR-13-0636
27. Li H, Yang C, Shi Y, Zhao L. Exosomes derived from siRNA against GRP78 modified bone-marrow-derived mesenchymal stem cells suppress sorafenib resistance in hepatocellular carcinoma. J Nanobiotechnol (2018) 16(1):103. doi: 10.1186/s12951-018-0429-z
28. Wu FQ, Fang T, Yu LX, Lv GS, Lv HW, Liang D, et al. ADRB2 signaling promotes HCC progression and sorafenib resistance by inhibiting autophagic degradation of HIF1α. J Hepatol (2016) 65(2):314–24. doi: 10.1016/j.jhep.2016.04.019
29. Chen Y, Huang Y, Reiberger T, Duyverman AM, Huang P, Samuel R, et al. Differential effects of sorafenib on liver versus tumor fibrosis mediated by stromal-derived factor 1 alpha/C-X-C receptor type 4 axis and myeloid differentiation antigen-positive myeloid cell infiltration in mice. Hepatology (2014) 59(4):1435–47. doi: 10.1002/hep.26790
30. Cheng K, Cai N, Zhu J, Yang X, Liang H, Zhang W. Tumor-associated macrophages in liver cancer: from mechanisms to therapy. Cancer Commun (Lond) (2022) 42(11):1112–40. doi: 10.1002/cac2.12345
31. Zhou SL, Zhou ZJ, Hu ZQ, Huang XW, Wang Z, Chen EB, et al. Tumor-associated neutrophils recruit macrophages and T-regulatory cells to promote progression of hepatocellular carcinoma and resistance to sorafenib. Gastroenterology (2016) 150(7):1646–58.e17. doi: 10.1053/j.gastro.2016.02.040
32. Nakamura K, Smyth MJ. Myeloid immunosuppression and immune checkpoints in the tumor microenvironment. Cell Mol Immunol (2020) 17(1):1–12. doi: 10.1038/s41423-019-0306-1
33. Peng H, Xue R, Ju Z, Qiu J, Wang J, Yan W, et al. Cancer-associated fibroblasts enhance the chemoresistance of CD73+ hepatocellular carcinoma cancer cells via HGF-Met-ERK1/2 pathway. Ann Transl Med (2020) 8(14):856. doi: 10.21037/atm-20-1038
34. Kubo N, Araki K, Kuwano H, Shirabe K. Cancer-associated fibroblasts in hepatocellular carcinoma. World J Gastroenterol (2016) 22(30):6841–50. doi: 10.3748/wjg.v22.i30.6841
35. Eun JW, Yoon JH, Ahn HR, Kim S, Kim YB, Lim SB, et al. Cancer-associated fibroblast-derived secreted phosphoprotein 1 contributes to resistance of hepatocellular carcinoma to sorafenib and lenvatinib. Cancer Commun (Lond) (2023) 43(4):455–79. doi: 10.1002/cac2.12414
36. Chen Y, Ramjiawan RR, Reiberger T, Ng MR, Hato T, Huang Y, et al. CXCR4 inhibition in tumor microenvironment facilitates anti-programmed death receptor-1 immunotherapy in sorafenib-treated hepatocellular carcinoma in mice. Hepatology (2015) 61(5):1591–602. doi: 10.1002/hep.27665
37. Xu GL, Ni CF, Liang HS, Xu YH, Wang WS, Shen J, et al. Upregulation of PD-L1 expression promotes epithelial-to-mesenchymal transition in sorafenib-resistant hepatocellular carcinoma cells. Gastroenterol Rep (Oxf) (2020) 8(5):390–8. doi: 10.1093/gastro/goaa049
38. Clara JA, Monge C, Yang Y, Takebe N. Targeting signalling pathways and the immune microenvironment of cancer stem cells - a clinical update. Nat Rev Clin Oncol (2020) 17(4):204–32. doi: 10.1038/s41571-019-0293-2
39. Lee TK, Guan XY, Ma S. Cancer stem cells in hepatocellular carcinoma - from origin to clinical implications. Nat Rev Gastroenterol Hepatol (2022) 19(1):26–44. doi: 10.1038/s41575-021-00508-3
40. Zhang Q, Lou Y, Yang J, Wang J, Feng J, Zhao Y, et al. Integrated multiomic analysis reveals comprehensive tumour heterogeneity and novel immunophenotypic classification in hepatocellular carcinomas. Gut (2019) 68(11):2019–31. doi: 10.1136/gutjnl-2019-318912
41. Losic B, Craig AJ, Villacorta-Martin C, Martins-Filho SN, Akers N, Chen X, et al. Intratumoral heterogeneity and clonal evolution in liver cancer. Nat Commun (2020) 11(1):291. doi: 10.1038/s41467-019-14050-z
42. Zheng H, Pomyen Y, Hernandez MO, Li C, Livak F, Tang W, et al. Single-cell analysis reveals cancer stem cell heterogeneity in hepatocellular carcinoma. Hepatology (2018) 68(1):127–40. doi: 10.1002/hep.29778
43. Ho DW, Tsui YM, Sze KM, Chan LK, Cheung TT, Lee E, et al. Single-cell transcriptomics reveals the landscape of intra-tumoral heterogeneity and stemness-related subpopulations in liver cancer. Cancer Lett (2019) 459:176–85. doi: 10.1016/j.canlet.2019.06.002
44. Liu Z, Dai X, Wang T, Zhang C, Zhang W, Zhang W, et al. Hepatitis b virus PreS1 facilitates hepatocellular carcinoma development by promoting appearance and self-renewal of liver cancer stem cells. Cancer Lett (2017) 400:149–60. doi: 10.1016/j.canlet.2017.04.017
45. Yang L, Shi P, Zhao G, Xu J, Peng W, Zhang J, et al. Targeting cancer stem cell pathways for cancer therapy. Signal Transduct Target Ther (2020) 5(1):8. doi: 10.1038/s41392-020-0110-5
46. Song Y, Jang J, Shin TH, Bae SM, Kim JS, Kim KM, et al. Sulfasalazine attenuates evading anticancer response of CD133-positive hepatocellular carcinoma cells. J Exp Clin Cancer Res (2017) 36(1):38. doi: 10.1186/s13046-017-0511-7
47. Yu H, Zhou L, Loong JHC, Lam KH, Wong TL, Ng KY, et al. SERPINA12 promotes the tumorigenic capacity of HCC stem cells through hyperactivation of AKT/β-catenin signaling. Hepatology (2023). doi: 10.1097/HEP.0000000000000269
48. Luo J, Gong L, Yang Y, Zhang Y, Liu Q, Bai L, et al. Enhanced mitophagy driven by ADAR1-GLI1 editing supports the self-renewal of cancer stem cells in hepatocellular carcinoma. Hepatology (2023). doi: 10.1097/HEP.0000000000000299
49. Lambert AW, Weinberg RA. Linking EMT programmes to normal and neoplastic epithelial stem cells. Nat Rev Cancer (2021) 21(5):325–38. doi: 10.1038/s41568-021-00332-6
50. Huang T, Song X, Xu D, Tiek D, Goenka A, Wu B, et al. Stem cell programs in cancer initiation, progression, and therapy resistance. Theranostics (2020) 10(19):8721–43. doi: 10.7150/thno.41648
51. Bakir B, Chiarella AM, Pitarresi JR, Rustgi AK. EMT. MET, plasticity, and tumor metastasis. Trends Cell Biol (2020) 30(10):764–76. doi: 10.1016/j.tcb.2020.07.003
52. Wang X, Wang J, Tsui YM, Shi C, Wang Y, Zhang X, et al. RALYL increases hepatocellular carcinoma stemness by sustaining the mRNA stability of TGF-β2. Nat Commun (2021) 12(1):1518. doi: 10.1038/s41467-021-21828-7
53. Chen J, Jin R, Zhao J, Liu J, Ying H, Yan H, et al. Potential molecular, cellular and microenvironmental mechanism of sorafenib resistance in hepatocellular carcinoma. Cancer Lett (2015) 367(1):1–11. doi: 10.1016/j.canlet.2015.06.019
54. Chow AK, Ng L, Lam CS, Wong SK, Wan TM, Cheng NS, et al. The enhanced metastatic potential of hepatocellular carcinoma (HCC) cells with sorafenib resistance. PloS One (2013) 8(11):e78675. doi: 10.1371/journal.pone.0078675
55. Jeng KS, Chang CF, Sheen IS, Jeng CJ, Wang CH. Cellular and molecular biology of cancer stem cells of hepatocellular carcinoma. Int J Mol Sci (2023) 24(2):1417. doi: 10.3390/ijms24021417
56. Ikemoto T, Shimada M, Yamada S. Pathophysiology of recurrent hepatocellular carcinoma after radiofrequency ablation. Hepatol Res (2017) 47(1):23–30. doi: 10.1111/hepr.12705
57. Xu XL, Liu XD, Liang M, Luo BM. Radiofrequency ablation versus hepatic resection for small hepatocellular carcinoma: systematic review of randomized controlled trials with meta-analysis and trial sequential analysis. Radiology (2018) 287(2):461–72. doi: 10.1148/radiol.2017162756
Keywords: hepatocellular carcinoma, sorafenib resistance, tumor microenvironment, cancer stem cells, hypoxia, immune microenvironment, EMT, exosomes
Citation: Chen S, Du Y, Guan X-Y and Yan Q (2023) The current status of tumor microenvironment and cancer stem cells in sorafenib resistance of hepatocellular carcinoma. Front. Oncol. 13:1204513. doi: 10.3389/fonc.2023.1204513
Received: 12 April 2023; Accepted: 03 July 2023;
Published: 27 July 2023.
Edited by:
Jiang Chen, Sir Run Run Shaw Hospital, Zhejiang University, ChinaReviewed by:
Yuen Gao, Michigan State University, United StatesVinay Kumar Sharma, Eunice Kennedy Shriver National Institute of Child Health and Human Development (NIH), United States
Copyright © 2023 Chen, Du, Guan and Yan. This is an open-access article distributed under the terms of the Creative Commons Attribution License (CC BY). The use, distribution or reproduction in other forums is permitted, provided the original author(s) and the copyright owner(s) are credited and that the original publication in this journal is cited, in accordance with accepted academic practice. No use, distribution or reproduction is permitted which does not comply with these terms.
*Correspondence: Qian Yan, yanq23@mail.sysu.edu.cn