- 1Key Laboratory of Carcinogenesis and Translational Research (Ministry of Education/Beijing), Department of Nuclear Medicine, Peking University Cancer Hospital & Institute, Beijing, China
- 2Key Laboratory for Research and Evaluation of Radiopharmaceuticals (National Medical Products Administration), Department of Nuclear Medicine, Peking University Cancer Hospital & Institute, Beijing, China
- 3Department of Thoracic Surgery, Aerospace 731 Hospital, Beijing, China
- 4Department of Thoracic Surgery, Peking University People’s Hospital, Beijing, China
- 5Thoracic Oncology Institute, Peking University People’s Hospital, Beijing, China
- 6Breax Laboratory, PCAB Research Center of Breath and Metabolism, Beijing, China
With the application of low-dose computed tomography in lung cancer screening, pulmonary nodules have become increasingly detected. Accurate discrimination between primary lung cancer and benign nodules poses a significant clinical challenge. This study aimed to investigate the viability of exhaled breath as a diagnostic tool for pulmonary nodules and compare the breath test with 18F-fluorodeoxyglucose (18F-FDG) positron emission tomography (PET)–computed tomography (CT). Exhaled breath was collected by Tedlar bags and analyzed by high-pressure photon ionization time-of-flight mass spectrometry (HPPI-TOFMS). A retrospective cohort (n = 100) and a prospective cohort (n = 63) of patients with pulmonary nodules were established. In the validation cohort, the breath test achieved an area under the receiver operating characteristic curve (AUC) of 0.872 (95% CI 0.760–0.983) and a combination of 16 volatile organic compounds achieved an AUC of 0.744 (95% CI 0.7586–0.901). For PET-CT, the SUVmax alone had an AUC of 0.608 (95% CI 0.433–0.784) while after combining with CT image features, 18F-FDG PET-CT had an AUC of 0.821 (95% CI 0.662–0.979). Overall, the study demonstrated the efficacy of a breath test utilizing HPPI-TOFMS for discriminating lung cancer from benign pulmonary nodules. Furthermore, the accuracy achieved by the exhaled breath test was comparable with 18F-FDG PET-CT.
Introduction
The National Lung Screening Trial (NLST) has confirmed that low-dose computed tomography (LDCT) lung cancer screening can decrease lung cancer mortality by 20% in high-risk populations compared to X-ray (1). Since then, LDCT has been recommended for lung cancer screening and many pulmonary nodules have been detected along with lung cancer screening (2–4). Pulmonary nodules are defined as pulmonary lesions less than 3 cm in CT images. The pathology of pulmonary nodules includes malignant diseases, such as primary lung cancer, distant metastases, or rarer lymphoma, as well as benign causes, such as tuberculosis, pneumonia, fungi infections, and primary benign tumors (hamartoma, angioma, etc.) (5). Discriminating between primary lung cancer and benign nodules is a clinical challenge for radiologists, thoracic surgeons, and physicians practicing with LDCT-based lung cancer screening (6, 7).
Several clinical associations have made guidelines or recommendations to manage pulmonary nodules, such as the American College of Chest Physicians and The Fleischner Society in 2013 and 2017, respectively (3, 8). Rather than biopsy or surgery, high-risk pulmonary nodules are often recommended for positron emission tomography (PET) first, since it is non-invasive and can provide information for differential diagnosis and staging at the same time (9, 10). However, PET-CT is very expensive and not sensitive enough for small pulmonary nodules.
Human breath includes thousands of volatile organic compounds (VOCs) (11, 12), and mounting evidence has proved that testing VOCs of exhaled breath can precisely detect lung cancer (13–15). Cancer cells have altered metabolism and generate a variety of aberrant metabolites, and some of these aberrant metabolites could be exhaled outside and detected by mass spectrometry or nano-sensors. Breath test is totally non-invasive and easy to collect, which is a promising tool for lung cancer early detection and screening. It has been reported that VOCs in exhaled breath can discriminate lung cancer from benign nodules (16). Gas chromatography–mass spectrometer (GC-MS) has been considered as the gold standard of exhaled breath VOC analysis, but it is not applicable in clinical practice because it requires complex sample pretreatment and time- consuming detecting processes (17). Several researchers have tried to diagnose pulmonary nodules by breath test, and they used different methods (18) (19, 20). High-pressure photon ionization time-of-flight mass spectrometry (HPPI-TOFMS) is a direct mass spectrometry that does not require sample pretreatment and only takes 1 min to analyze one sample; thus, HPPI-TOFMS is a suitable tool for clinical application (13, 17). HPPI-TOFMS has been reported to be effective for detection of lung cancer (13), esophageal cancer (21), and tuberculosis (22), but it is unknown whether HPPI-TOFMS could discriminate lung cancer from benign pulmonary nodules.
In this study, we first performed the breath test by HPPI-TOFMS and trained a model in a retrospective cohort and then tested whether this model could discriminate lung cancer from benign pulmonary nodules and compared its diagnostic accuracy with PET-CT in a prospective cohort.
Materials and methods
Participant’s recruitment and study design
This study was reported according to the Standards for Reporting Diagnostic Accuracy, and a checklist was attached (23). The prospective specimen collection, retrospective blinded evaluation design (24) was utilized. This study was approved by the Ethics Committee Board of Peking University People’s Hospital (2021PHB349), and informed consent was obtained from all participants.
For the retrospective cohort, patients who received thoracic surgery or endobronchial ultrasound–guided transbronchial needle aspirate were consecutively recruited at the Department of Thoracic Surgery, Peking University People’s Hospital from September 2021 to October 2021. The inclusion criteria were 1) age > 18 years, 2) pulmonary lesions <3 cm in CT images, 3) the pathological diagnoses were primary lung cancer or benign lung diseases, and 4) no history of cancer and no anticancer treatment before.
For the prospective cohort, patients with pulmonary lesions planning to receive 18F-FDG PET-CT were prospectively recruited at the Department of Nuclear Medicine, Cancer Hospital of Peking University from November 2021 to January 2022. Patients were consecutively recruited with the following criteria: 1) age > 18 years, 2) with pulmonary lesions and plan to have 18F-FDG PET-CT scanning, and 3) no history of cancer within 5 years. After PET-CT scanning, we followed up pathologic diagnoses of all eligible participants. Patients who met the following criteria were excluded: 1) the lung lesions were metastasized from other organs; 2) lung lesions were larger than 3 cm in CT scans; and 3) no pathological diagnosis. For all participants, the clinical data and demographic data were collected from medical records and questionnaires.
Exhaled breath collection
Exhaled breath samples were collected by trained investigators following the same protocol according to our previous studies (13). Exhaled breath was collected before PET-CT scanning and the morning before surgery. All participants fasted for at least 6 h before sample collection. To reduce potential confounding factors, all participants were asked not to ingest spicy food, alcohol, or coffee the night before exhaled breath collection. Disposable face masks and Tedlar bags were used to collect exhaled breath. A disposable face mask was replaced before each collection to avoid cross- contamination. Briefly, participants first gargled with pure water and then performed a single deep nasal inhalation followed by complete exhalation via their mouth into a Tedlar air bag. At both clinical centers, breath samples were collected in a fixed room and the room air was also collected before and after sample collection of participants. All air bags were delivered to lab and detected within 4 h.
High-pressure photon ionization time-of-flight mass spectrometry detection
Exhaled breath was detected by HPPI-TOFMS as previously described (13). The pressure in the HPPI ion source was set at 500 Pa, and two capillaries were arranged in the ion source. The gas-phase exhaled breath sample was directly introduced into the ionization region through a 250- μm i.d. 0.60- m- long stainless- steel capillary from the Tedlar bag. In order to eliminate condensation of exhaled VOCs and minimize possible surface adsorption, the stainless steel capillary was heated to 100°C and the HPPI ion source was heated to 60°C. The TOF signals were recorded by a 400ps time-to-digital converter rate at 25 kHz, and all the mass spectra were accumulated for 60s. Mass spectrum peaks detected by HPPI-TOFMS with m/z <500 were recorded and 32,500 features were extracted from the HPPI-TOFMS data of each exhaled breath sample.
Positron emission tomography–computed tomography imaging
18F-FDG PET-CT was performed as previously reported (25). The patients were instructed to fast for 6 h before 18F-FDG injection. The 18F was manufactured by HM-20 medical cyclotron (Sumitomo Corporation, Japan), and the 18F-FDG was administrated intravenously according to the patient’s body weight (3.0–3.7 MBq/kg). Imaging was performed using a PET/CT scanner (Biograph64, SIEMENS, Erlangen, Germany) operated in 3D Flow Motion (bed entry speed 1 mm/s) from the apex of the skull to the midthigh, with a PET axial field of view of 21.6 cm. The PET images were reconstructed by the TrueX + TOF method offered by the vendor. The injected activity was 3.7 MBq/kg, and the time from injection to scan was approximately 60 ± 10 min. Diagnoses of 18F-FDG PET-CT were made by two authors independently based on SUVmax and CT images. Discernments between two authors were solved by discussion.
Statistical analyses
Machine learning models with mass spectrometry data of exhaled breath as input were constructed with the caret package (https://cran.r-project.org/web/packages/caret/). Then, the diagnostic capacity of the breath model and 16 VOCs model was evaluated with the area under the receiver operating characteristic (ROC) curve (AUC) and accuracy through the R packages pROC (https://cran.r-project.org/web/packages/pROC/index.html) in discriminating lung cancer from benign nodules.
Sensitivity, specificity, accuracy, the positive predict value, and the negative predictive value were calculated to evaluate the diagnostic performance of PET-CT and the breath test. The ROC was performed and the AUC was calculated to evaluate the diagnostic performance of PET-CT and the breath test. Baseline characteristics were analyzed with the independent t test or Fisher’s exact test. Two-sided P values less than 0.05 were considered statistically significant. All statistical analyses were performed using SPSS software (version 24.0).
Results
Clinical characteristics of enrolled patients
The overall study design is shown in Figure 1. We first retrospectively selected 49 lung cancer patients and 51 benign pulmonary nodules from Peking University People’s Hospital as the discovery cohort (PKUPH cohort). Then, 119 patients with pulmonary nodules and received 18F-FDG PET-CT were prospectively recruited from the Cancer Hospital of Peking University. Following this, 119 patients with pulmonary nodules who underwent 18F-FDG PET-CT were prospectively recruited from the Cancer Hospital of Peking University (CAPKU cohort) (Figure 1A, CAPKU cohort). Out of the 119 patients, 56 were excluded based on the exclusion criteria. A total of 63 patients were included as the validation set (Figure 1A, CAPKU enrollment diagram).
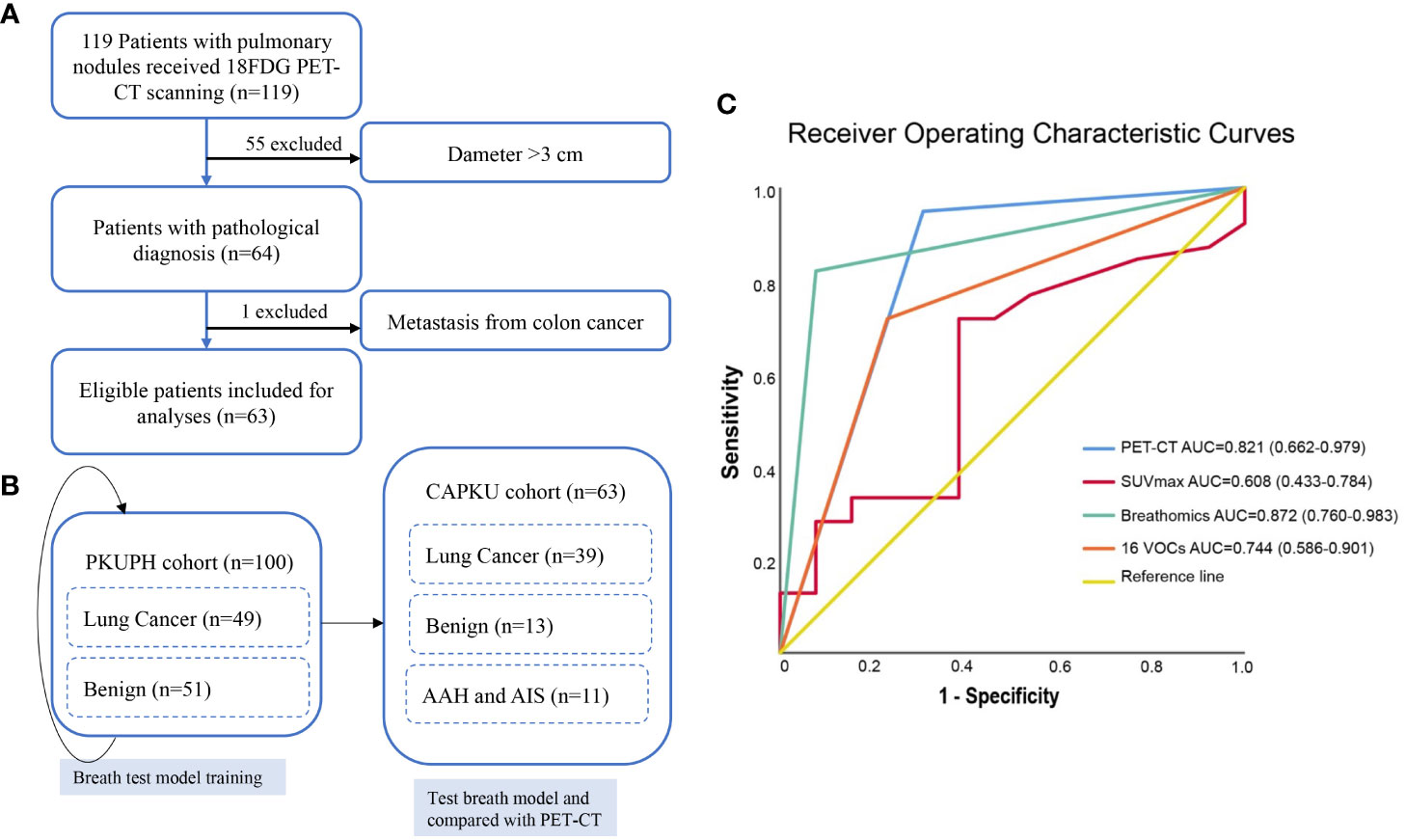
Figure 1 Flow diagram of participant recruitment in the Cancer Hospital of Peking University (CAPKU) cohort (A) and data analyses process (B). Receiver operating characteristics of the breath test and positron emission tomography–computed tomography in the validation CAPKU cohort (C). AAH, atypical adenomatous hyperplasia; AIS, adenocarcinoma in situ.
Detailed clinical characteristics of eligible participants are shown in Table 1. As shown, lung cancer patients in the PKUPH cohort were all at stage I, and in the CAPKU cohort 76.9% (30/39, Figure 1B), lung cancer patients were at stage I.
Accuracy of high-pressure photon ionization time-of-flight mass spectrometry
HPPI-TOFMS has been proved to be accurate for lung cancer detection in a proof-of-concept study. In this study, we first trained a model to discriminate lung cancer from benign pulmonary nodules in the PKUPH cohort. A total of 100 patients with pulmonary nodules were included. In the discovery cohort, the random forest model reached the best AUC and accuracy. By constructing a model with all features of mass spectrometry peaks, the random forest model reached 82.1% sensitivity, 92.3% specificity, 84.6% accuracy, 97.0% positive predictive value, 63.2% negative predictive value, and 0.872 AUC (95% CI 0.760–0.983) in the CAPKU cohort (Figure 1C).
With perioperative sampling, 16 VOCs have been identified as potential lung cancer–specific biomarkers (26). Using the 16 VOCs, the model reached 71.8% sensitivity, 76.9% specificity, 73.1% accuracy, 90.3% positive predictive value, 47.6% negative predictive value, and 0.744 AUC (95% CI 0.586–0.901) in the validation cohort (Figure 1C).
Accuracy of 18F-FDG positron emission tomography–computed tomography
We first analyzed the diagnostic accuracy of SUVmax in the CAPKU cohort. As shown in Figure 1C, SUVmax reached the best AUC of 0.608 (95% CI 0.433–0.784) when the threshold was set as 1.35. SUVmax achieved 71.8% sensitivity, 61.5% specificity, 69.2% accuracy, 84.8% positive predictive value, and 42.1% negative predictive value in the validation cohort.
When combined with CT features, 18F-FDG PET-CT had a better AUC (0.821, 95% CI 0.662–0.979) for discriminating lung cancer from benign nodules (Figure 1C). 18F-FDG PET-CT achieved 94.9% sensitivity, 69.2% specificity, 88.5% accuracy, 90.2% positive predictive value, 81.5% negative predictive value, and 0.744 AUC (95% CI 0.586-0.901) in the validation cohort.
Breath test and 18F-FDG positron emission tomography–computed tomography for detection of atypical adenomatous hyperplasia/adenocarcinoma in situ
According to the current WHO classification, AAH and AIS have been classified as benign. However, AAH and AIS are the very early stage of lung adenocarcinoma; they may slowly progress and become invasive adenocarcinoma. Thus, in the validation cohort, we compared diagnostic accuracy of breath test and 18F-FDG PET-CT among pulmonary nodules diagnosed with AAH and AIS. As shown in Table 2, the mean SUVmax of the 11 nodules were 0.95 ranging from 0.4 to 3.2, with only one patient having nodules with SUVmax > 1.35. A representative image was shown in Figure 2. Among 11 patients with AAH/AIS, 4 patients were correctly detected by the breath test and 7 patients were classified as lung cancer according to SUVmax.
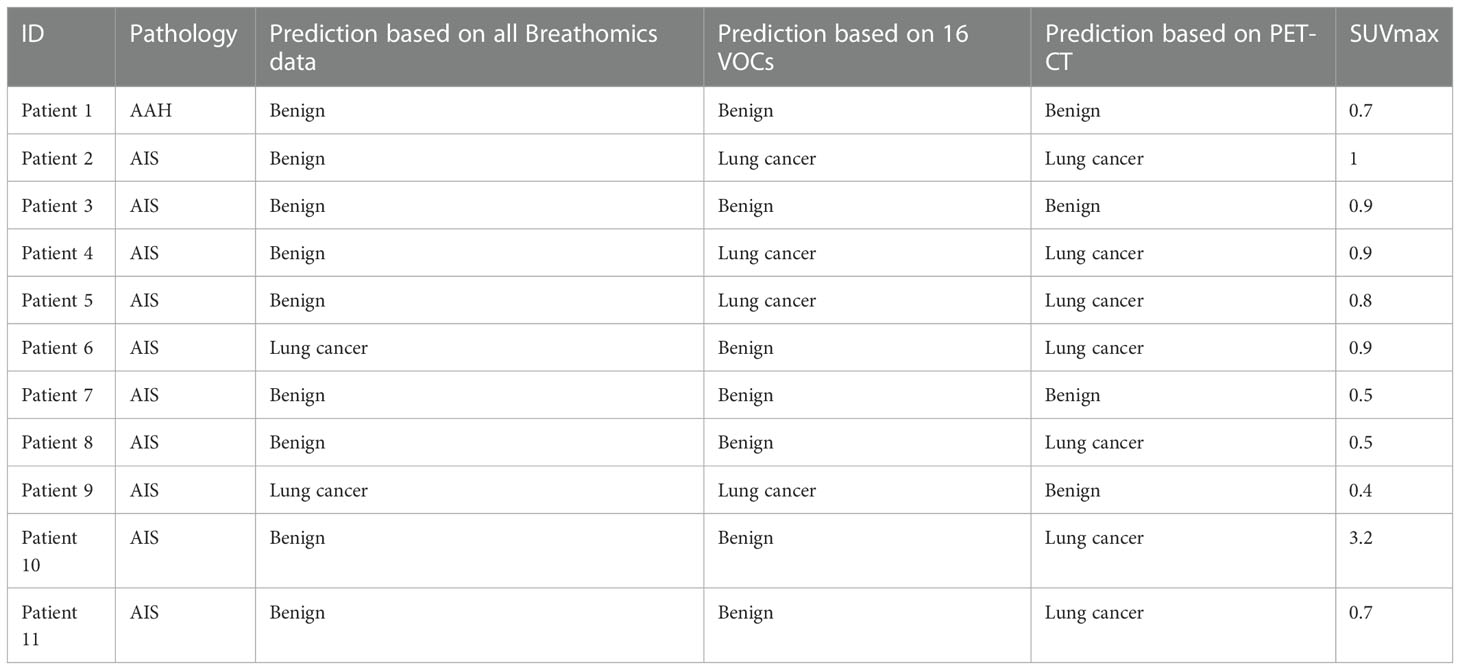
Table 2 Detailed diagnoses by breath test and PET-CT among 11 participants with atypical adenomatous hyperplasia or adenocarcinoma in situ.
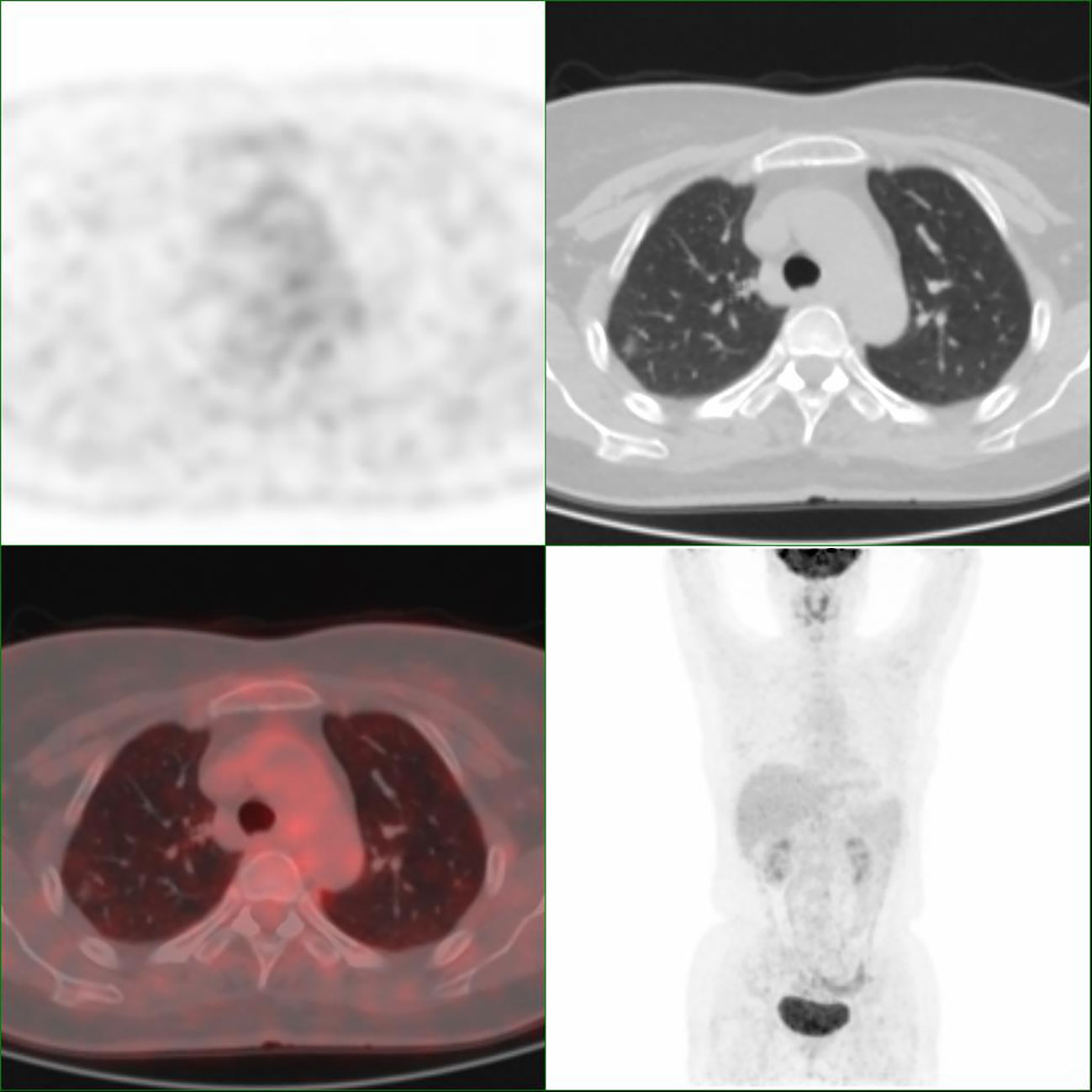
Figure 2 Representative images of Patient 4 with adenocarcinoma in situ. The nodule has an SUVmax of 0.9.
Discussion
In this study, we found that SUVmax had the best diagnostic performance when the cutoff value was set as 1.35, which is much lower than the previously reported 2.5 (27, 28). It has been reported that 18F-FDG PET-CT could detect small pulmonary nodules less than 1 cm, lower cutoff may provide accurate diagnosis of benign and malignant pulmonary nodules (29). However, it should be noted that lung cancer risk among patients with PET-negative pulmonary nodules cannot be neglected. Akpoviroro et al. followed up 191 LungRADS-4 patients, and they found that 22.4% (15/67) patients were diagnosed with lung cancer in the PET-negative group (30).
In this study, SUVmax alone achieved an AUC of 0.608; however, the AUC increased to 0.821 when CT image features were added, indicating an improvement in diagnostic accuracy. Among 11 patients with AAH or AIS, 7 were correctly diagnosed as lung cancer by 18F-FDG PET-CT. As the mean SUVmax was 0.95 and only one nodule had SUVmax > 1.35, SUVmax alone is not sufficient for the detection of AIS/AAH. Furthermore, the inclusion of CT images significantly enhanced the accuracy of diagnosis in 18F-FDG PET-CT. These data suggested that SUVmax alone is not enough to discriminate lung cancer from benign pulmonary nodules and CT images should be an indispensable part of pulmonary nodule follow-up.
Compared with PET-CT, the breath test showed better diagnostic accuracy in the CAPKU cohort. The 16 VOCs achieved 71.8% sensitivity and 76.9% specificity, while all exhale breath mass spectrometry features achieved 82.1% sensitivity and 92.3% specificity. Although the diagnostic accuracy decreased slightly, the 16 VOCs still achieved accuracy >70%. These data suggest that the breath test may be used for diagnosis of pulmonary nodules or follow-up, especially for PET-negative pulmonary nodules.
Discriminating lung cancer from benign pulmonary nodules is very challenging, and many methods have been tried, such as circulating cell-free DNA, metabolomics, and exhaled breath. Thus, this study provides new insights into the current lung cancer screening strategy. Exhaled breath is easy and non-invasive to collect, and the breath test by HPPI-TOFMS is fast and feasible, which is very useful to help identify high-risk populations. A well-designed study is warned to investigate how to integrate the breath test into the current LDCT lung cancer screening model.
It is crucial to note the limitations of the study when interpreting the results. While over 90% of incidentally detected pulmonary nodules are generally benign, participants in this study were highly selected before they were recruited, which resulted in a lower proportion of benign nodules. On the other hand, although we have identified 16 lung cancer– specific VOCs in a previous study (26), we did not compare results from HPPI-TOFMS with the current gold- standard GC-MS as reported by Markar et al. (31).
Conclusions
In summary, the study demonstrated the efficacy of a breath test utilizing HPPI-TOFMS for discriminating lung cancer from benign pulmonary nodules, and the accuracy achieved by the exhaled breath test was comparable with 18F-FDG PET-CT.
Data availability statement
The original contributions presented in the study are included in the article/supplementary material. Further inquiries can be directed to the corresponding authors.
Ethics statement
The studies involving human participants were reviewed and approved by Ethics Committee Board of Peking University People’s Hospital. The patients/participants provided their written informed consent to participate in this study.
Author contributions
Conceptualization, XD, NL, ZY, and MQ; methodology, XD, PW, and GL; software, HC; formal analysis, XD; investigation, XD, HC, NL, and MQ; writing—original draft preparation, XD and MQ; writing—review and editing, XD, NL, ZY, and MQ; visualization, XD and MQ; supervision, NL, ZY, and MQ; project administration, NL, ZY, and MQ; funding acquisition, MQ. All authors have read and agreed to the published version of the manuscript.
Funding
This research was funded by National Natural Science Foundation of China, grant number 82173386.
Acknowledgments
Language polishing was partially assisted by ChatGPT3.5 via HIPLOT (https://hiplot.com.cn).
Conflict of interest
The authors declare that the research was conducted in the absence of any commercial or financial relationships that could be construed as a potential conflict of interest.
Publisher’s note
All claims expressed in this article are solely those of the authors and do not necessarily represent those of their affiliated organizations, or those of the publisher, the editors and the reviewers. Any product that may be evaluated in this article, or claim that may be made by its manufacturer, is not guaranteed or endorsed by the publisher.
References
1. T. National Lung Screening Trial Research, Aberle DR, Adams AM, Berg CD, Black WC, Clapp JD, et al. Reduced lung-cancer mortality with low-dose computed tomographic screening. N Engl J Med (2011) 365(5):395–409. doi: 10.1056/NEJMoa1102873
2. Callister ME, Baldwin DR, Akram AR, Barnard S, Cane P, Draffan J, et al. British Thoracic society standards of care: British thoracic society guidelines for the investigation and management of pulmonary nodules. Thorax (2015) 70(Suppl 2):ii1–ii54. doi: 10.1136/thoraxjnl-2015-207168
3. MacMahon H, Naidich DP, Goo JM, Lee KS, Leung ANC, Mayo JR, et al. Guidelines for management of incidental pulmonary nodules detected on CT images: from the fleischner society 2017. Radiology (2017) 284(1):228–43. doi: 10.1148/radiol.2017161659
4. Naidich DP, Bankier AA, MacMahon H, Schaefer-Prokop CM, Pistolesi M, Goo JM, et al. Recommendations for the management of subsolid pulmonary nodules detected at CT: a statement from the fleischner society. Radiology (2013) 266(1):304–17. doi: 10.1148/radiol.12120628
5. Nasim F, Ost DE. Management of the solitary pulmonary nodule. Curr Opin Pulm Med (2019) 25(4):344–53. doi: 10.1097/MCP.0000000000000586
6. van Klaveren RJ, Oudkerk M, Prokop M, Scholten ET, Nackaerts K, Vernhout R, et al. Management of lung nodules detected by volume CT scanning. N Engl J Med (2009) 361(23):2221–9. doi: 10.1056/NEJMoa0906085
7. McWilliams A, Tammemagi MC, Mayo JR, Roberts H, Liu G, Soghrati K, et al. Probability of cancer in pulmonary nodules detected on first screening CT. N Engl J Med (2013) 369(10):910–9. doi: 10.1056/NEJMoa1214726
8. Gould MK, Donington J, Lynch WR, Mazzone PJ, Midthun DE, Naidich DP, et al. Evaluation of individuals with pulmonary nodules: when is it lung cancer? diagnosis and management of lung cancer, 3rd ed: American college of chest physicians evidence-based clinical practice guidelines. Chest (2013) 143(5 Suppl):e93S–e120S. doi: 10.1378/chest.12-2351
9. Garcia-Velloso MJ, Bastarrika G, de-Torres JP, Lozano MD, Sanchez-Salcedo P, Sancho L, et al. Assessment of indeterminate pulmonary nodules detected in lung cancer screening: diagnostic accuracy of FDG PET/CT. Lung Cancer (2016) 97:81–6. doi: 10.1016/j.lungcan.2016.04.025
10. Mazzone PJ, Lam L. Evaluating the patient with a pulmonary nodule: a review. JAMA (2022) 327(3):264–73. doi: 10.1001/jama.2021.24287
11. Horvath I, Lazar Z, Gyulai N, Kollai M, Losonczy G. Exhaled biomarkers in lung cancer. Eur Respir J (2009) 34(1):261–75. doi: 10.1183/09031936.00142508
12. Marzorati D, Mainardi L, Sedda G, Gasparri R, Spaggiari L, Cerveri P. A review of exhaled breath: a key role in lung cancer diagnosis. J Breath Res (2019) 13(3):034001. doi: 10.1088/1752-7163/ab0684
13. Meng S, Li Q, Zhou Z, Li H, Liu X, Pan S, et al. Assessment of an exhaled breath test using high-pressure photon ionization time-of-Flight mass spectrometry to detect lung cancer. JAMA Netw Open (2021) 4(3):e213486. doi: 10.1001/jamanetworkopen.2021.3486
14. Ibrahim W, Carr L, Cordell R, Wilde MJ, Salman D, Monks PS, et al. Breathomics for the clinician: the use of volatile organic compounds in respiratory diseases. Thorax (2021) 76(5):514–21. doi: 10.1136/thoraxjnl-2020-215667
15. van de Goor R, van Hooren M, Dingemans AM, Kremer B, Kross K. Training and validating a portable electronic nose for lung cancer screening. J Thorac Oncol (2018) 13(5):676–81. doi: 10.1016/j.jtho.2018.01.024
16. Chen X, Muhammad KG, Madeeha C, Fu W, Xu L, Hu Y, et al. Calculated indices of volatile organic compounds (VOCs) in exhalation for lung cancer screening and early detection. Lung Cancer (2021) 154:197–205. doi: 10.1016/j.lungcan.2021.02.006
17. Wang DH, Lee HS, Yoon D, Berry G, Wheeler TM, Sugarbaker DJ, et al. Progression of EGFR-mutant lung adenocarcinoma is driven by alveolar macrophages. Clin Cancer Res (2017) 23(3):778–88. doi: 10.1158/1078-0432.CCR-15-2597
18. Chen K, Nie Y, Park S, Zhang K, Zhang Y, Liu Y, et al. Development and validation of machine learning-based model for the prediction of malignancy in multiple pulmonary nodules: analysis from multicentric cohorts. Clin Cancer Res (2021) 27(8):2255–65. doi: 10.1158/1078-0432.Ccr-20-4007
19. Rai SN, Das S, Pan J, Mishra DC, Fu XA. Multigroup prediction in lung cancer patients and comparative controls using signature of volatile organic compounds in breath samples. PloS One (2022) 17(11):e0277431. doi: 10.1371/journal.pone.0277431
20. Guirao A, Molins L, Ramon I, Sunyer G, Vinolas N, Marrades R, et al. Trained dogs can identify malignant solitary pulmonary nodules in exhaled gas. Lung Cancer (2019) 135:230–3. doi: 10.1016/j.lungcan.2019.06.008
21. Huang Q, Wang S, Li Q, Wang P, Li J, Meng S, et al. Assessment of breathomics testing using high-pressure photon ionization time-of-Flight mass spectrometry to detect esophageal cancer. JAMA Netw Open (2021) 4(10):e2127042. doi: 10.1001/jamanetworkopen.2021.27042
22. Fu L, Feng Y, Ren T, Yang M, Yang Q, Lin Y, et al. Detecting latent tuberculosis infection with a breath test using mass spectrometer: a pilot cross-sectional study. Biosci Trends (2023) 17(1):73–7. doi: 10.5582/bst.2022.01476
23. Bossuyt PM, Reitsma JB, Bruns DE, Gatsonis CA, Glasziou PP, Irwig L, et al. STARD 2015: an updated list of essential items for reporting diagnostic accuracy studies. BMJ (2015) 351:h5527. doi: 10.1136/bmj.h5527
24. Ransohoff DF. The process to discover and develop biomarkers for cancer: a work in progress. J Natl Cancer Inst (2008) 100(20):1419–20. doi: 10.1093/jnci/djn339
25. Zhou N, Zhang A, Su H, Zhao W, Li N, Yang Z. (18)F-FDG distribution pattern improves the diagnostic accuracy of single pulmonary solid nodule. Front Oncol (2022) 12:983833. doi: 10.3389/fonc.2022.983833
26. Wang P, Huang Q, Meng S, Mu T, Liu Z, He M, et al. Identification of lung cancer breath biomarkers based on perioperative breathomics testing: a prospective observational study. EClinicalMedicine (2022) 47:101384. doi: 10.1016/j.eclinm.2022.101384
27. Truong MT, Ko JP, Rossi SE, Rossi I, Viswanathan C, Bruzzi JF, et al. Update in the evaluation of the solitary pulmonary nodule. Radiographics (2014) 34(6):1658–79. doi: 10.1148/rg.346130092
28. Chen J, Mehta V, Chowdhary V, Chaya N, Lowry JW. Outcome of PET-negative solid pulmonary nodules: a retrospective study. Acad Radiol (2021) 28(5):628–33. doi: 10.1016/j.acra.2020.03.016
29. Khalaf M, Abdel-Nabi H, Baker J, Shao Y, Lamonica D, Gona J. Relation between nodule size and 18F-FDG-PET SUV for malignant and benign pulmonary nodules. J Hematol Oncol (2008) 1:13. doi: 10.1186/1756-8722-1-13
30. Akpoviroro O, Asokan S, Steiling K, Rebello A, Burks EJ, Litle VR, et al. Lung cancer risk in suspicious lung nodules with negative positron emission tomography. Ann Thorac Surg (2022) 113(6):1821–6. doi: 10.1016/j.athoracsur.2021.06.041
Keywords: lung cancer, breath test, pulmonary nodule, PET-CT, early detection
Citation: Ding X, Lin G, Wang P, Chen H, Li N, Yang Z and Qiu M (2023) Diagnosis of primary lung cancer and benign pulmonary nodules: a comparison of the breath test and 18F-FDG PET-CT. Front. Oncol. 13:1204435. doi: 10.3389/fonc.2023.1204435
Received: 12 April 2023; Accepted: 17 May 2023;
Published: 02 June 2023.
Edited by:
Qingqing Zhu, The First Affiliated Hospital of Soochow University, ChinaReviewed by:
Xi Yang, Fudan University, ChinaMarion Tonneau, University of Montreal Hospital Centre (CRCHUM), Canada
Copyright © 2023 Ding, Lin, Wang, Chen, Li, Yang and Qiu. This is an open-access article distributed under the terms of the Creative Commons Attribution License (CC BY). The use, distribution or reproduction in other forums is permitted, provided the original author(s) and the copyright owner(s) are credited and that the original publication in this journal is cited, in accordance with accepted academic practice. No use, distribution or reproduction is permitted which does not comply with these terms.
*Correspondence: Mantang Qiu, qiumantang@163.com; Nan Li, rainbow6283@sina.com; Zhi Yang, pekyz@163.com