- Department of Gastrointestinal Surgery, Peking University Shougang Hospital, Beijing, China
Introduction: Colon cancer is one of the most prevalent malignancies and causes of cancer-related deaths worldwide. Thus, further research is required to explicate the latent molecular mechanisms and look for novel biomarkers. E2F3 has been confirmed to be an oncogene in a variety of cancers. However, the particular regulation of E2F3 in colon cancer needs further investigation.
Methods: The self-renewal ability was detected through a sphere formation assay. The tumorigenic ability was measured through nude mice in vivo assay. The protein expression of genes was examined through a Western blot. The expression of E2F3 in tumor tissues was detected through an IHC assay. The resistance to cisplatin was assessed through the CCK-8 assay. The cell migration and invasion abilities were measured after upregulating or suppressing E2F3 through the Transwell assay.
Results: Results uncovered that E2F3 was upregulated in spheroid cells. In addition, E2F3 facilitates stemness in colon cancer. Moreover, E2F3 facilitated colon cancer cell migration and invasion. Finally, it was revealed that E2F3 affected the STAT3 pathway to modulate stemness in colon cancer. E2F3 served as a promoter regulator in colon cancer, aggravating tumorigenesis and stemness in colon cancer progression through the STAT3 pathway.
Conclusion: E2F3 may be a useful biomarker for anticancer treatment in colon cancer.
Introduction
Colon cancer is one of the most prevalent tumors and results in cancer-interrelated deaths globally (1, 2). Early surgical operations obviously ameliorate the 5-year survival rate of colon cancer patients, but most advanced colon cancer patients are found to have metastasis and are not suited for surgery (3, 4). Unluckily, treatment options with useful effects for advanced colon cancer patients were few (5, 6). Hence, comprehending the relevant molecular regulatory mechanism of colon cancer progression and looking for accurate and serviceable biomarkers were imperative.
More and more factors (lncRNAs, circRNAs, miRNAs, and proteins) as molecular targets have been disclosed to join in the regulation of cancer’s progression, including colon cancer. For example, lncRNA LINC01578 modulates the NF-κB/YY1 axis to strengthen metastasis in colon cancer (7). CircRNA circCSPP1 combines with miR-431 to regulate ROCK1 and ZEB1, then facilitates the tumorigenesis of colon cancer (8). HOXD13 enhances PTPRN2 expression to aggravate colon cancer progression (9). RNA binding protein PUM1 accelerates tumor growth in colon cancer (10).
E2F transcription factor 3 (E2F3) is one transcription factor that modulates a variety of biological processes, such as DNA synthesis and restoration, centrosome copy, and so on (11, 12). It exhibits dysregulated expression in diversified cancers. For instance, E2F3, modulated by circ-PRKAR1B, facilitates the progression of liver cancer (13). E2F3 aggravates breast cancer tumor metastasis (14). H3K27ac-triggered FOXC2-AS1 enhances E2F3 to strengthen tongue squamous cell carcinoma (15). In addition, miR-34a targets E2F1 and E2F3 to modulate breast cancer tumorigenesis (16). QKI-6 reduces E2F3 to retard the progression of bladder cancer by regulating NF-κB signaling (17). Certainly, E2F3 has also been probed in colon cancer. For example, miR-449b downregulates CCND1 and E2F3 expression to suppress the progression of colon cancer (18). In colon cancer, dysregulation of miR-34a targets Sirt1 and E2F3 to result in drug resistance to 5-FU (19). Apigenin modulates miR-215-5p to reduce E2F1/3 and retards colorectal cancer tumorigenesis (20). However, the regulatory function of E2F3 needs more research in colon cancer.
The signal transducer and activator of the transcription 3 (STAT3) pathway has also been discovered to be a key regulatory pathway that participates in diversified cancers’ progression (21–23). Gene set enrichment analysis has discovered that the critical oncogenic pathway (STAT3) is tightly associated with E2F7/8 (24). In addition, E2F3 and STAT3 are both overexpressed in breast cancer (25). However, the influences of E2F3 on the STAT3 pathway are unknown in colon cancer.
The purpose of our work is to probe the regulatory effects of E2F3 on the tumorigenesis of colon cancer. Our findings demonstrated that E2F3 accelerated stemness in colon cancer by activating the STAT3 pathway. This discovery may provide a valid target for colon cancer’s targeted therapy and mitigate the suffering of colon cancer patients.
Materials and methods
Cell line and cell culture
The colon cancer cell line HCT116 was gained from the Cell Bank of the Chinese Academy of Sciences (Shanghai, China) and cultured in RPMI-1640 medium (Gibco, Carlsbad, CA, USA) with 10% fetal bovine serum (FBS), and then maintained at 37°C with 5% CO2 in a humidified incubator. Spheroid cells are a small number of tumor cells with stem cell characteristics that are enriched by sphere culture. To verify the function of the STAT3 pathway, colon cancer cells were treated with epidermal growth factor (EGF, 10 ng/ml, Sino Biological, Beijing, China) to stimulate STAT3 activation and selective STAT3 inhibitor S3I-201 (100 μM, MeilunBio, Dalian, China) to suppress STAT3 DNA-binding activity for 24 h.
Sphere formation assay
RPMI-1640 medium containing 1% antibiotic mixture (100 mg/ml streptomycin and 100 U/ml penicillin G, Gibco), 1% N2 supplement (Invitrogen, Carlsbad, California, USA), 2% B27 supplement (Invitrogen, Carlsbad, California, USA), 20 ng/ml FGF2 (Chemicon, Temecula, CA, USA), and 20 ng/ml EGF (Chemicon, Temecula, CA, USA) was applied to incubate cells in the 24-well plate. After 2 weeks, the spheroids were observed under a microscope (Olympus, Tokyo, Japan) at ×100 magnification.
In vivo assay
Animal studies were conducted in accordance with the institutional guidelines of Beijing Viewsolid Biotechnology Co. Ltd. (Beijing, China). (VS212601450). The male BALB/c nude mice (6 weeks; n = 5 in each group) were obtained from Weitong Lihua Experimental Animal Technology (Beijing, China). The 1 × 106 cells were subcutaneously injected into the right flank of nude mice. At 1 month postinjection, the mice were killed, tumors were collected, and pictures of tumors were taken for examining their volumes.
Western blot
The isolated proteins in RIPA lysis buffer (Beyotime Institute of Biotechnology, Shanghai, China) were electrophoresed with SDS-PAGE followed by moving onto the polyvinylidene fluoride (PVDF) membranes (Amersham Pharmacia Biotech, Sweden). After being blocked, the membrane was probed with primary antibodies including E2F3 (ab152126; dilution 1:500; rabbit; Abcam, Shanghai, China), A2B5 (ab53521; dilution 1 µg/ml; mouse), CD133 (ab222782; dilution 1:2,000; rabbit), Nestin (ab105389; dilution 1:100; rabbit), Sox2 (ab97959; dilution 1 µg/ml; rabbit), and GAPDH (ab8245; dilution 1:500; mouse). Next, HRP-anti-rabbit IgG (ab6721; dilution 1:2,000) was mixed with the membranes. At last, the bands were assessed with the enhanced chemiluminescence system (ECL, Thermo Fisher Scientific Inc, Waltham, MA, USA).
IHC assay
After being deparaffinized and rehydrated, paraffin sections of tumor tissue from nude mice were mixed with primary anti-E2F3 (ab152126; dilution 1:500; rabbit; Abcam, Shanghai, China) overnight at 4°C. Following washing, appropriate second antibodies (ab6721; dilution 1:1,000; HRP-anti-rabbit) were cultivated with the sections. Next, sections were dyed through 3,3′-diaminobenzidine (DAB) and counterstained with hematoxylin. The images were then captured on a Carl Zeiss LSM880 AiryscanTM confocal microscope (magnification ×100) using ZEN software.
CCK-8 assay
The cells were grown in 96-well plates and treated with cisplatin (DDP, 40 μM) for 48 h. CCK8 reagent (10 μl, Takara, Dalian, China) was mixed into the well for another 2 h. The cell viability was tested at 450 nm under the Microplate Reader (Bio-Rad, Hercules, California, USA).
Transwell assay
The Matrigel-coated (Becton Dickinson, Franklin Lakes, NJ, USA) (or not) Transwell chambers (Corning Life Sciences, Corning, NY, USA) were employed for invasion (or migration) assays. The upper chamber was set with cells (1 × 104) and RPMI-1640 medium without FBS, and the lower chamber was set with RPMI-1640 medium with 10% FBS. Next, the invaded or migrated cells were fixed through 90% ethanol and dyed with 0.1% crystal violet. The invaded or migrated cells were examined through the inverted microscope (IX71, magnification ×200, Olympus, Tokyo, Japan). Images were analyzed using ImageJ (National Institutes of Health, NIH, Bethesda, Maryland, USA).
Statistical analysis
All data were expressed as the mean ± standard deviation (SD). All experiments were conducted in triplicate. SPSS 20.0 software was employed to carry out the statistical analysis. The comparisons were estimated through the Student’s t-test (two groups) or one-way ANOVA (multiple groups). p < 0.05 was set as a significant difference.
Results
E2F3 was upregulated in spheroid cells
At first, it was discovered that the increased number of spheres was greater in the spheroid cells than in the HCT116 cells (Figure 1A). Furthermore, the tumorigenic ability of spheroid cells was stronger than that of HCT116 cells (Figure 1B). In addition, it was discovered that A2B5, CD133, Nestin, and Sox2 were all higher in spheroid cells (Figure 1C). Importantly, the expression of E2F3 was upregulated in spheroid cells (Figure 1D).
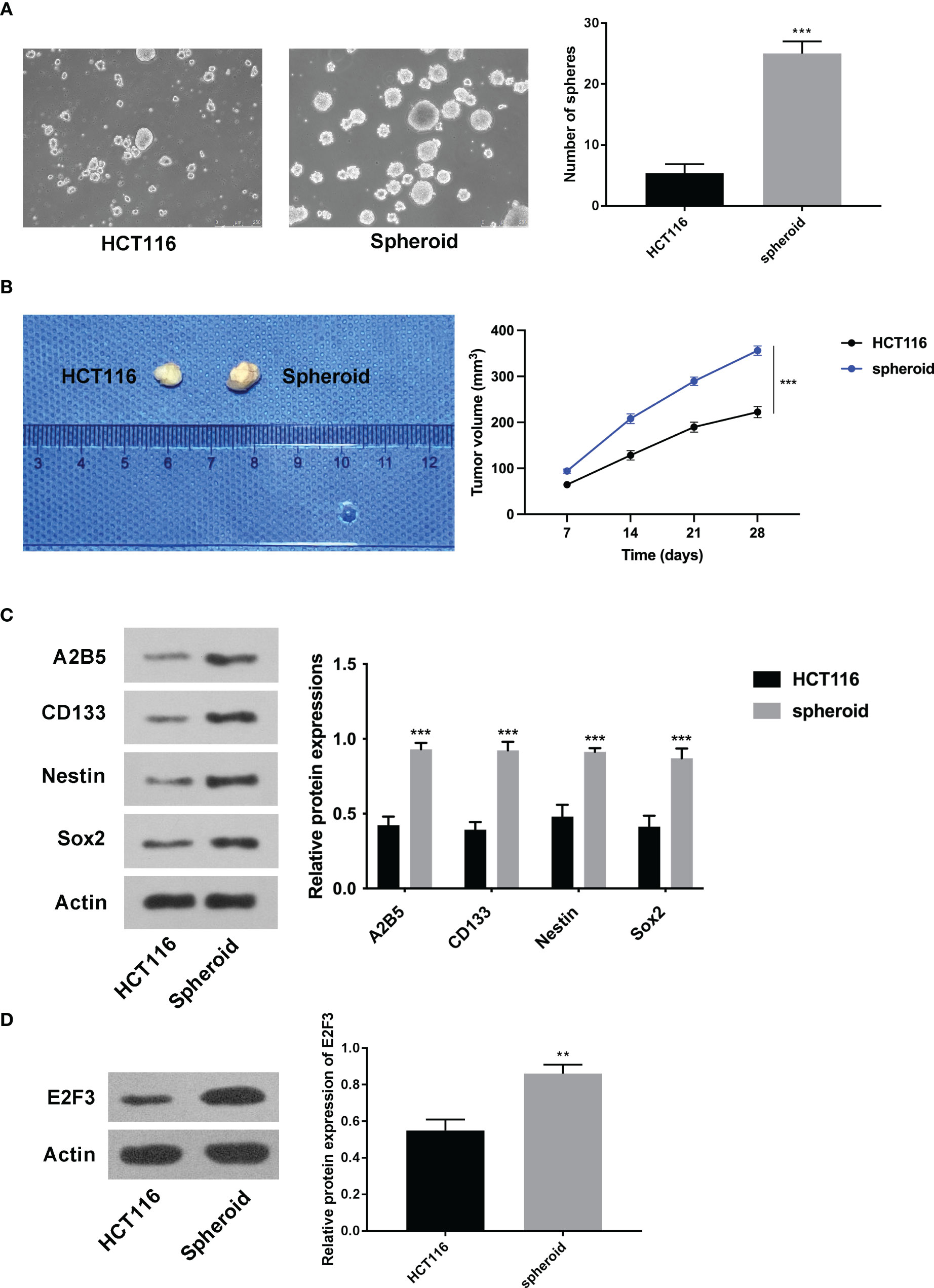
Figure 1 E2F3 was upregulated in spheroid cells. (A) The self-renewal ability of spheroid cells and HCT-116 cells was detected through the sphere formation assay. (B) The tumorigenic ability of spheroid cells and HCT-116 cells was measured through nude mice in vivo assay. (C) The protein expression of A2B5, CD133, Nestin, and Sox2 in spheroid cells and HCT-116 cells was assessed through Western blot. (D) The expression of E2F3 in spheroid cells and HCT-116 cells was examined through Western blot. **p < 0.01; ***p < 0.001.
E2F3 facilitated stemness in colon cancer
As displayed in Figure 2A, the overexpression and knockdown efficiencies of E2F3 were confirmed. Next, we discovered that the formation ability of spheres was enhanced after E2F3 overexpression and reduced after E2F3 inhibition (Figure 2B). Moreover, tumor growth was increased after overexpressing E2F3 and decreased after suppressing E2F3 (Figure 2C). Through the IHC assay, E2F3 expression was enhanced after E2F3 overexpression and reduced after E2F3 knockdown (Figure 2D). Additionally, a Western blot was performed to measure the stemness-related proteins (A2B5, CD133, Nestin, Sox2) (26, 27). Results from Western blot revealed that the protein levels of A2B5, CD133, Nestin, and Sox2 were upregulated through E2F3 overexpression and downregulated through E2F3 knockdown (Figure 2E). Taken together, E2F3 facilitated stemness in colon cancer.
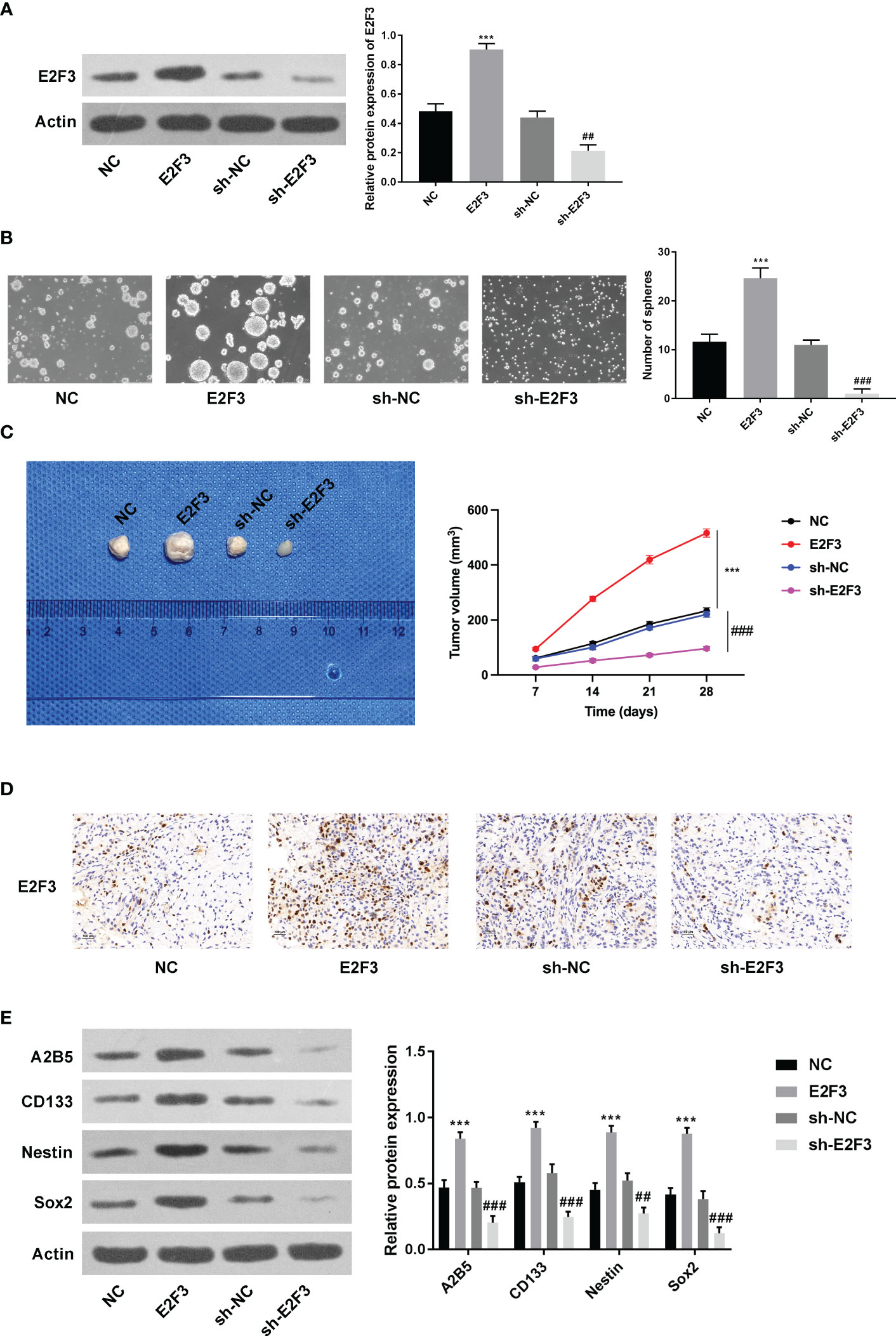
Figure 2 E2F3 facilitates stemness in colon cancer. (A) The overexpression and knockdown efficiencies were measured through Western blotting. (B) The self-renewal ability was tested after overexpressing or silencing E2F3 through sphere formation assay. Scale bar: 250 μm. (C) The tumorigenic ability was examined after up- or downregulating E2F3 through nude mice in vivo assay. (D) The expression of E2F3 in tumor tissues was detected through an IHC assay. Scale bar: 100 μm. (E) The protein expression of A2B5, CD133, Nestin, and Sox2 was inspected through Western blot. ***p < 0.001 vs. the NC group; ##p < 0.01; ###p < 0.001 vs. the sh-NC group.
E2F3 facilitated cell migration and invasion in colon cancer
Further investigation demonstrated that the migration and invasion abilities were strengthened after upregulating E2F3 and weakened after silencing E2F3 (Figures 3A, B). Moreover, the E-cadherin expression was enhanced, and the N-cadherin and vimentin were reduced after E2F3 overexpression. The E-cadherin expression was reduced, and the N-cadherin and vimentin were enhanced after E2F3 inhibition (Figure 3C). In addition, the IC50 of HCT116 cells transfected with NC, E2F3, sh-NC, or sh-E2F3 plasmids was increased after E2F3 overexpression and decreased after E2F3 repression (Figure 3D). These data revealed that E2F3 facilitated cell migration and invasion in colon cancer.
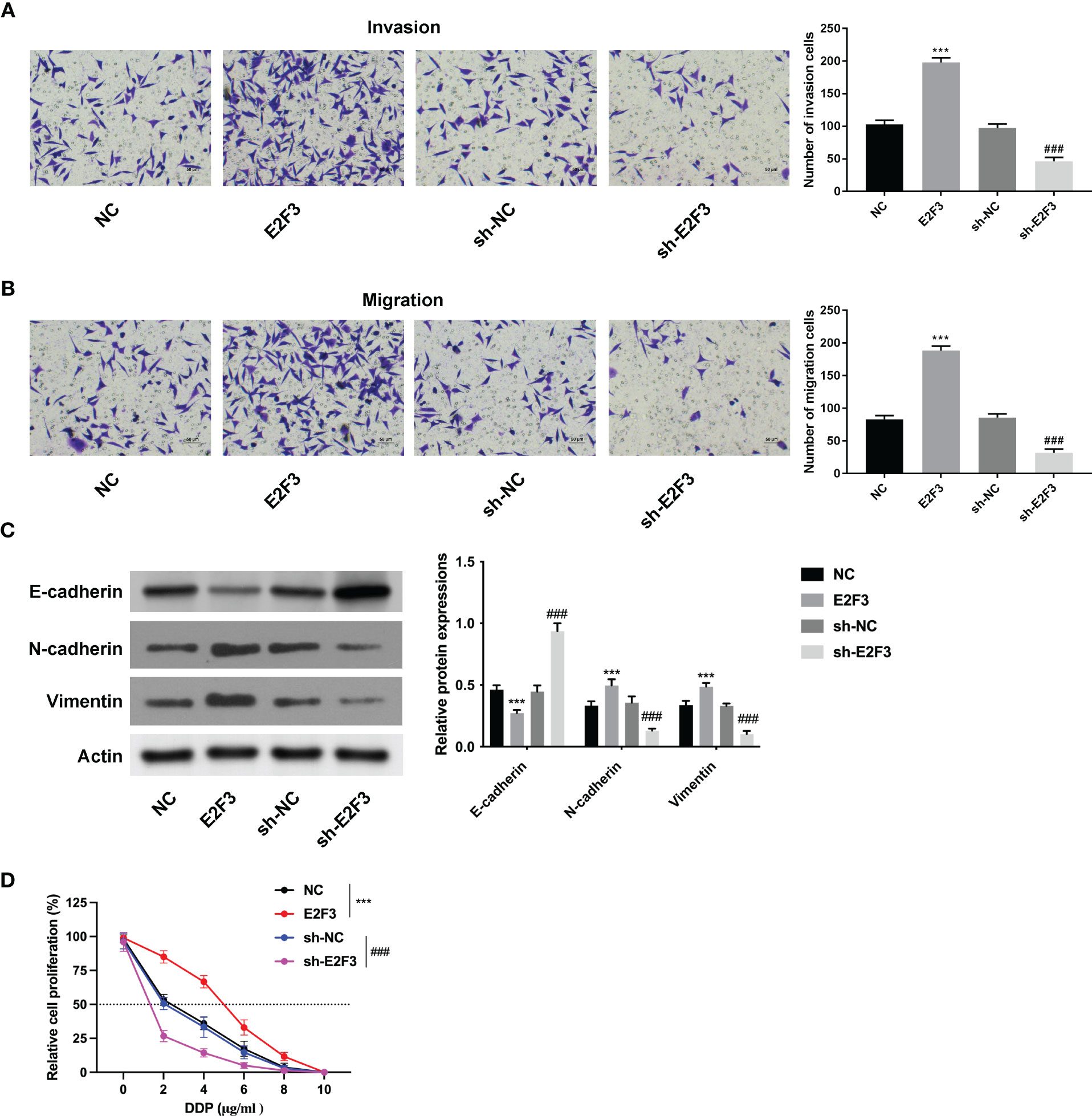
Figure 3 E2F3 facilitates cell migration and invasion in colon cancer. (A, B) The cell migration and invasion abilities were measured after upregulating or suppressing E2F3 through Transwell assay. Scale bar: 50 μm. (C) The protein expression of E-cadherin, N-cadherin, and vimentin was evaluated after E2F3 overexpression or suppression through Western blot. (D) The resistance to cisplatin was assessed after overexpressing or inhibiting E2F3 through CCK-8 assay. ***p < 0.001 vs. the NC group; ###p < 0.001 vs. the sh-NC group.
E2F3 affected the STAT3 pathway in colon cancer
The protein expression of STAT3 was upregulated after overexpressing E2F3 and downregulated after silencing E2F3 (Figure 4A). Moreover, the increased spheres mediated by E2F3 overexpression were reversed after STAT3 inhibitor (S3I-201) (Figure 4B). Additionally, the decreased spheres mediated by E2F3 knockdown were rescued after the STAT3 activator (STAT3C) (Figure 4C). To sum up, E2F3 affected the STAT3 pathway in colon cancer.
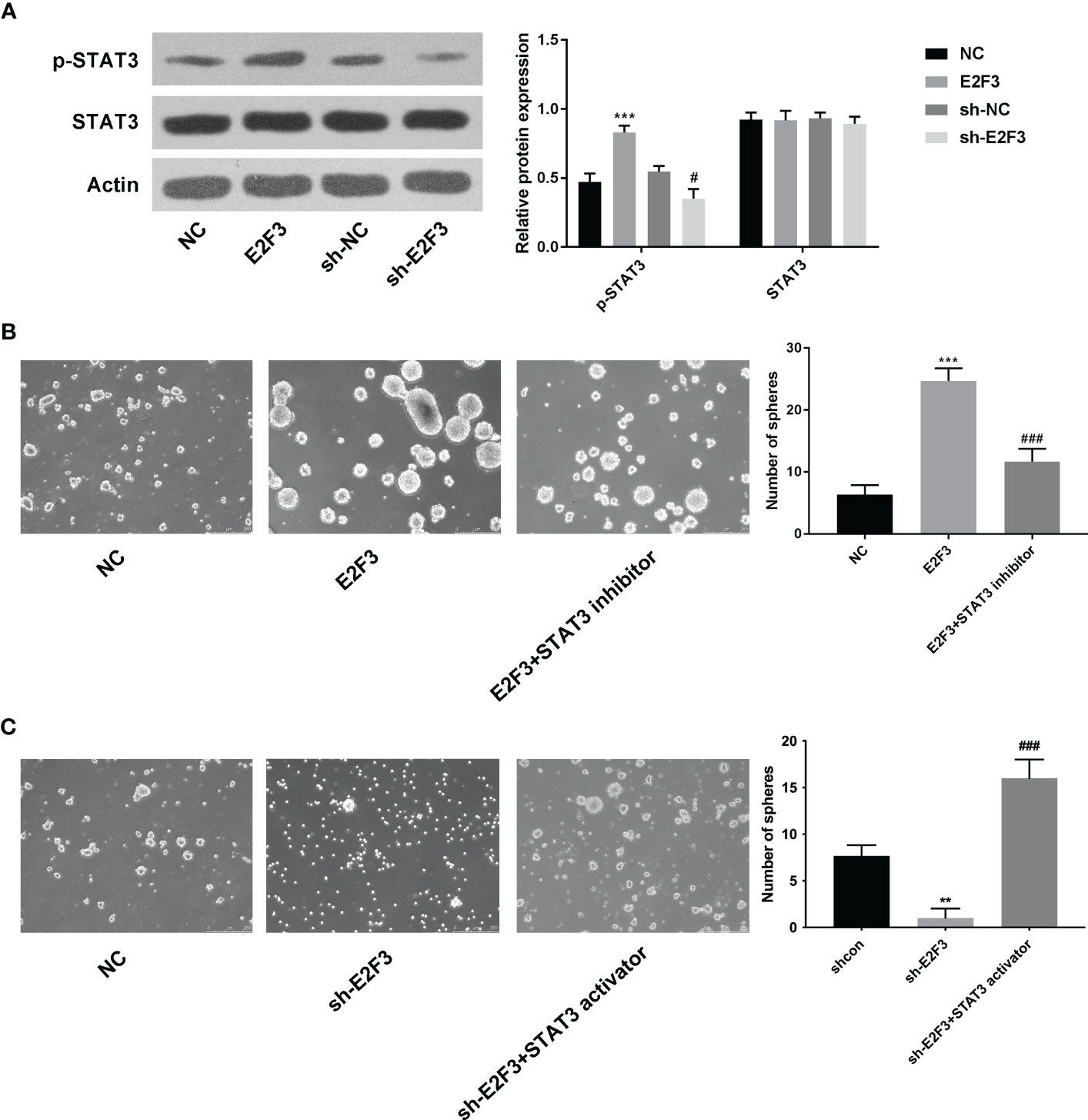
Figure 4 E2F3 affects the STAT3 pathway in colon cancer. (A) The p-STAT3 and STAT3 protein expressions were determined after overexpressing or repressing E2F3 through Western blotting. (B) The self-renewal ability was tested in the NC, E2F3, and E2F3+STAT3 inhibitor groups through sphere formation assay. Scale bar: 250 μm. (C) The self-renewal ability was measured in the shcon, shE2F3, and shE2F3+STAT3 activator groups through a sphere formation assay. Scale bar: 250 μm. **p < 0.01; ***p < 0.001 vs. the NC group; #p<0.05 vs the sh-NC group; ###p < 0.001 vs. the E2F3 group.
Discussion
E2F3 has been affirmed to be a pivotal facilitator in diverse cancers (13–17), including colon cancer, but its role associated with stemness in colon cancer needs more investigations. In this study, E2F3 expression was upregulated in colon cancer spheroid cells.
Cancer stem cells (CSCs) with invigorative self-renewal and infinite replication capacity are a kind of cell subset that can aggravate the development of malignant tumors (28, 29). CSCs are the major reason for tumor recurrence relapse (30). A growing number of studies are attempted to search for valid bio-targets on stemness in various cancers. For example, CDK1 interacts with Sox2in lung cancer to facilitate stemness (31). Furthermore, HET0016 targets CYP4Z1 in breast cancer to relieve stemness (32). Tanshinone IIA stimulates ferroptosis to suppress stemness in gastric cancer (33). ALG3 modulates the glycosylation of TGF-β receptor II to aggravate stemness and radioresistance in breast cancer (34). Of course, these regulatory effects related to stemness take part in colon cancer. For instance, NF-κB reduces miR-195-5p/497-5p and enhances MCM2 in colon cancer to affect stemness (35). Moreover, miR-139-5p targets E2-2 to attenuate stemness and metastasis in colon cancer (36). In colon cancer, cysteinyl leukotriene receptor 1 contributes to 5-fluorouracil resistance and stemness (37).GATA6 heightens LRH-1 expression in colon cancer to facilitate stemness (38). In this study, we uncovered that E2F3 overexpression facilitated and E2F3 knockdown reduced stemness in colon cancer. Moreover, E2F3 was discovered to aggravate colon cancer cell migration and invasion. Cisplatin is a common drug for colon cancer (39, 40), so a CCK-8 assay was done to investigate cisplatin resistance in colon cancer. It was uncovered that the IC50 of DDP was strengthened after E2F3 overexpression and reduced after E2F3 suppression.
The STAT3 pathway is a critical regulatory pathway in diversified cancers, including colon cancer. For instance, HDGF induces the STAT3 signaling pathway in breast cancer to strengthen radioresistance (21). Additionally, NR1D1 retards the JAK/STAT3 signaling pathway to relieve the growth of ovarian cancer (41). Insulin-like growth factor 1 modulates the STAT3 pathway to facilitate cell proliferation and invasion in papillary thyroid cancer (42). P4HB knockdown suppresses the STAT3 signaling to enhance cell apoptosis in colon cancer (43). Resveratrol targets the AKT/STAT3 signaling pathway to relieve colon cancer tumorigenesis (44). Shikonin modulates ADAM17 and the IL-6/STAT3 signaling pathway to attenuate the tumor growth of colon cancer cells and exert synergistic effects by regulating (45). However, the relationship between the STAT3 pathway and E2F3 in colon cancer remains unknown. In the end, it was revealed from this study that E2F3 affected the STAT3 pathway to enhance stemness in colon cancer.
To sum up, it was the first time to uncover that E2F3 acted as a facilitator in colon cancer, strengthening tumor growth and stemness in colon cancer progression by affecting the STAT3 pathway. However, our results regarding the functions of E2F3 in colon cancer remain restrained. In the future, more experiments will be done to further manifest the other regulatory effects of E2F3.
Data availability statement
The original contributions presented in the study are included in the article/Supplementary Material. Further inquiries can be directed to the corresponding author.
Ethics statement
The animal study was reviewed and approved by Beijing Viewsolid Biotechnology Co. LTD.
Author contributions
QG and WH designed the study. KA and ZL collected the data. CD and WH analyzed the data. QG wrote the manuscript. All authors contributed to the article and approved the submitted version.
Funding
This work was supported by the Peking University Shougang Hospital Shouyi Medical Technology Development Fund (SGYYQ202010).
Conflict of interest
The authors declare that the research was conducted in the absence of any commercial or financial relationships that could be construed as a potential conflict of interest.
Publisher’s note
All claims expressed in this article are solely those of the authors and do not necessarily represent those of their affiliated organizations, or those of the publisher, the editors and the reviewers. Any product that may be evaluated in this article, or claim that may be made by its manufacturer, is not guaranteed or endorsed by the publisher.
Supplementary material
The Supplementary Material for this article can be found online at: https://www.frontiersin.org/articles/10.3389/fonc.2023.1203712/full#supplementary-material
References
1. Labianca R, Beretta GD, Kildani B, Milesi L, Merlin F, Mosconi S, et al. Colon cancer. Crit Rev Oncol Hematol (2010) 74(2):106–33. doi: 10.1016/j.critrevonc.2010.01.010
2. Shawki S, Ashburn J, Signs SA, Huang E. Colon cancer: inflammation-associated cancer. Surg Oncol Clin N Am (2018) 27(2):269–87. doi: 10.1016/j.soc.2017.11.003
3. Freeman HJ. Early stage colon cancer. World J Gastroenterol (2013) 19(46):8468–73. doi: 10.3748/wjg.v19.i46.8468
4. Arredondo J, Pastor E, Simó V, Beltrán M, Castañón C, Magdaleno MC, et al. Neoadjuvant chemotherapy in locally advanced colon cancer: a systematic review. Tech Coloproctol (2020) 24(10):1001–15. doi: 10.1007/s10151-020-02289-4
5. Wu C. Systemic therapy for colon cancer. Surg Oncol Clin N Am (2018) 27(2):235–42. doi: 10.1016/j.soc.2017.11.001
6. Lannagan TR, Jackstadt R, Leedham SJ, Sansom OJ. Advances in colon cancer research: in vitro and animal models. Curr Opin Genet Dev (2021) 66:50–6. doi: 10.1016/j.gde.2020.12.003
7. Liu J, Zhan Y, Wang J, Wang J, Guo J, Kong D. Long noncoding RNA LINC01578 drives colon cancer metastasis through a positive feedback loop with the NF-κB/YY1 axis. Mol Oncol (2020) 14(12):3211–33. doi: 10.1002/1878-0261.12819
8. Wang J, Zhou L, Chen B, Yu Z, Zhang J, Zhang Z, et al. Circular RNA circCSPP1 promotes the occurrence and development of colon cancer by sponging miR-431 and regulating ROCK1 and ZEB1. J Transl Med (2022) 20(1):58. doi: 10.1186/s12967-022-03240-x
9. Yin J, Guo Y. HOXD13 promotes the malignant progression of colon cancer by upregulating PTPRN2. Cancer Med (2021) 10(16):5524–33. doi: 10.1002/cam4.4078
10. Gor R, Sampath SS, Lazer LM, Ramalingam S. RNA Binding protein PUM1 promotes colon cancer cell proliferation and migration. Int J Biol Macromol (2021) 174:549–61. doi: 10.1016/j.ijbiomac.2021.01.154
11. Balasubramanian S, Ahmad N, Mukhtar H. Upregulation of E2F transcription factors in chemically induced mouse skin tumors. Int J Oncol (1999) 15(2):387–90. doi: 10.3892/ijo.15.2.387
12. Gao Y, Feng B, Lu L, Han S, Chu X, Chen L, et al. MiRNAs and E2F3: a complex network of reciprocal regulations in human cancers. Oncotarget (2017) 8(36):60624–39. doi: 10.18632/oncotarget.17364
13. Liu G, Ouyang X, Gong L, Yao L, Liu S, Li J, et al. E2F3 promotes liver cancer progression under the regulation of circ-PRKAR1B. Mol Ther Nucleic Acids (2021) 26:104–13. doi: 10.1016/j.omtn.2021.07.002
14. Jusino S, Rivera-Rivera Y, Chardón-Colón C, Ruiz-Justiz AJ, Vélez-Velázquez J, Isidro A, et al. E2F3 drives the epithelial-to-mesenchymal transition, cell invasion, and metastasis in breast cancer. Exp Biol Med (Maywood) (2021) 246(19):2057–71. doi: 10.1177/15353702211035693
15. Chen C, Chen Q, Wu J, Zou H. H3K27ac-induced FOXC2-AS1 accelerates tongue squamous cell carcinoma by upregulating E2F3. J Oral Pathol Med (2021) 50(10):1018–30. doi: 10.1111/jop.13232
16. Han R, Zhao J, Lu L. MicroRNA−34a expression affects breast cancer invasion in vitro and patient survival via downregulation of E2F1 and E2F3 expression. Oncol Rep (2020) 43(6):2062–72. doi: 10.3892/or.2020.7549
17. Shi F, Deng Z, Zhou Z, Jiang CY, Zhao RZ, Sun F, et al. QKI-6 inhibits bladder cancer malignant behaviours through down-regulating E2F3 and NF-κB signalling. J Cell Mol Med (2019) 23(10):6578–94. doi: 10.1111/jcmm.14481
18. Fang Y, Gu X, Li Z, Xiang J, Chen Z. miR-449b inhibits the proliferation of SW1116 colon cancer stem cells through downregulation of CCND1 and E2F3 expression. Oncol Rep (2013) 30(1):399–406. doi: 10.3892/or.2013.2465
19. Akao Y, Noguchi S, Iio A, Kojima K, Takagi T, Naoe T. Dysregulation of microRNA-34a expression causes drug-resistance to 5-FU in human colon cancer DLD-1 cells. Cancer Lett (2011) 300(2):197–204. doi: 10.1016/j.canlet.2010.10.006
20. Cheng Y, Han X, Mo F, Zeng H, Zhao Y, Wang H, et al. Apigenin inhibits the growth of colorectal cancer through down-regulation of E2F1/3 by miRNA-215-5p. Phytomedicine (2021) 89:153603. doi: 10.1016/j.phymed.2021.153603
21. Qiu L, Ma Y, Chen X, Zhou L, Zhang H, Zhong G, et al. Heparin-binding growth factor (HDGF) drives radioresistance in breast cancer by activating the STAT3 signaling pathway. J Transl Med (2021) 19(1):344. doi: 10.1186/s12967-021-03021-y
22. Mohan CD, Rangappa S, Nayak SC, Sethi G, Rangappa KS. Paradoxical functions of long noncoding RNAs in modulating STAT3 signaling pathway in hepatocellular carcinoma. Biochim Biophys Acta Rev Cancer (2021) 1876(1):188574. doi: 10.1016/j.bbcan.2021.188574
23. Yang F, Wang H, Yan B, Li T, Min L, Chen E, et al. Decreased level of miR-1301 promotes colorectal cancer progression via activation of STAT3 pathway. Biol Chem (2021) 402(7):805–13. doi: 10.1515/hsz-2020-0301
24. Yu H, Li Z, Wang M. Expression and prognostic role of E2F transcription factors in high-grade glioma. CNS Neurosci Ther (2020) 26(7):741–53. doi: 10.1111/cns.13295
25. Mirzaei S, Paskeh MDA, Entezari M, Bidooki SH, Ghaleh VJ, Rezaei S, et al. siRNA and targeted delivery systems in breast cancer therapy. Clin Transl Oncol (2023) 25(5):1167–88. doi: 10.1007/s12094-022-03043-y
26. Song K, Chen J, Ding J, Xu H, Xu H, Qin Z. Hyperbaric oxygen suppresses stemness-associated properties and nanog and oncostatin m expression, but upregulates β-catenin in orthotopic glioma models. J Int Med Res (2020) 48(3):300060519872898. doi: 10.1177/0300060519872898
27. Lojewski X, Hermann A, Wegner F, Araúzo-Bravo MJ, Hallmeyer-Elgner S, Kirsch M, et al. Human adult white matter progenitor cells are multipotent neuroprogenitors similar to adult hippocampal progenitors. Stem Cells Transl Med (2014) 3(4):458–69. doi: 10.5966/sctm.2013-0117
28. Dawood S, Austin L, Cristofanilli M. Cancer stem cells: implications for cancer therapy. Oncol (Williston Park) (2014) 28(12):1101–7, 1110.
29. Nassar D, Blanpain C. Cancer stem cells: basic concepts and therapeutic implications. Annu Rev Pathol (2016) 11:47–76. doi: 10.1146/annurev-pathol-012615-044438
30. Atashzar MR, Baharlou R, Karami J, Abdollahi H, Rezaei R, Pourramezan F, et al. Cancer stem cells: a review from origin to therapeutic implications. J Cell Physiol (2020) 235(2):790–803. doi: 10.1002/jcp.29044
31. Huang Z, Shen G, Gao J. CDK1 promotes the stemness of lung cancer cells through interacting with Sox2. Clin Transl Oncol (2021) 23(9):1743–51. doi: 10.1007/s12094-021-02575-z
32. Liu H, Qin H, Zhou Y, Yuan Y, Liu Y, Chen Y, et al. HET0016 attenuates the stemness of breast cancer cells through targeting CYP4Z1. Mol Carcinog (2021) 60(6):413–26. doi: 10.1002/mc.23302
33. Ni H, Ruan G, Sun C, Yang X, Miao Z, Li J, et al. Tanshinone IIA inhibits gastric cancer cell stemness through inducing ferroptosis. Environ Toxicol (2022) 37(2):192–200. doi: 10.1002/tox.23388
34. Sun X, He Z, Guo L, Wang C, Lin C, Ye L, et al. ALG3 contributes to stemness and radioresistance through regulating glycosylation of TGF-β receptor II in breast cancer. J Exp Clin Cancer Res (2021) 40(1):149. doi: 10.1186/s13046-021-01932-8
35. Wang L, Guo J, Zhou J, Wang D, Kang X, Zhou L. NF-κB maintains the stemness of colon cancer cells by downregulating miR-195-5p/497-5p and upregulating MCM2. J Exp Clin Cancer Res (2020) 39(1):225. doi: 10.1186/s13046-020-1523-2
36. Ma X, Liu J, Li J, Li Y, Le VM, Li S, et al. miR-139-5p reverses stemness maintenance and metastasis of colon cancer stem-like cells by targeting E2-2. J Cell Physiol (2019) 234(12):22703–18. doi: 10.1002/jcp.28836
37. Satapathy SR, Sjölander A. Cysteinyl leukotriene receptor 1 promotes 5-fluorouracil resistance and resistance-derived stemness in colon cancer cells. Cancer Lett (2020) 488:50–62. doi: 10.1016/j.canlet.2020.05.023
38. Lai HT, Chiang CT, Tseng WK, Chao TC, Su Y. GATA6 enhances the stemness of human colon cancer cells by creating a metabolic symbiosis through upregulating LRH-1 expression. Mol Oncol (2020) 14(6):1327–47. doi: 10.1002/1878-0261.12647
39. Manogaran P, Somasundaram B, Viswanadha VP. Reversal of cisplatin resistance by neferine/isoliensinine and their combinatorial regimens with cisplatin-induced apoptosis in cisplatin-resistant colon cancer stem cells (CSCs). J Biochem Mol Toxicol (2022) 36(3):e22967. doi: 10.1002/jbt.22967
40. Hong H, Cao W, Wang Q, Liu C, Huang C. Synergistic antitumor effect of andrographolide and cisplatin through ROS-mediated ER stress and STAT3 inhibition in colon cancer. Med Oncol (2022) 39(5):101. doi: 10.1007/s12032-022-01691-2
41. Wang H, Fu Y. NR1D1 suppressed the growth of ovarian cancer by abrogating the JAK/STAT3 signaling pathway. BMC Cancer (2021) 21(1):871. doi: 10.1186/s12885-021-08597-8
42. Yang L, Tan Z, Li Y, Zhang X, Wu Y, Xu B, et al. Insulin-like growth factor 1 promotes proliferation and invasion of papillary thyroid cancer through the STAT3 pathway. J Clin Lab Anal (2020) 34(12):e23531. doi: 10.1002/jcla.23531
43. Zhou Y, Yang J, Zhang Q, Xu Q, Lu L, Wang J, et al. P4HB knockdown induces human HT29 colon cancer cell apoptosis through the generation of reactive oxygen species and inactivation of STAT3 signaling. Mol Med Rep (2019) 19(1):231–7. doi: 10.3892/mmr.2018.9660
44. Li D, Wang G, Jin G, Yao K, Zhao Z, Bie L, et al. Resveratrol suppresses colon cancer growth by targeting the AKT/STAT3 signaling pathway. Int J Mol Med (2019) 43(1):630–40. doi: 10.3892/ijmm.2018.3969
Keywords: E2F3, stemness, STAT3 pathway, colon cancer, cancer
Citation: Gao Q, An K, Lv Z, Wang Y, Ding C and Huang W (2023) E2F3 accelerates the stemness of colon cancer cells by activating the STAT3 pathway. Front. Oncol. 13:1203712. doi: 10.3389/fonc.2023.1203712
Received: 11 April 2023; Accepted: 07 June 2023;
Published: 29 June 2023.
Edited by:
Nan-Shan Chang, National Cheng Kung University, TaiwanReviewed by:
Kenneth K. W. To, The Chinese University of Hong Kong, ChinaYi-Jia Li, Beckman Research Institute, United States
Copyright © 2023 Gao, An, Lv, Wang, Ding and Huang. This is an open-access article distributed under the terms of the Creative Commons Attribution License (CC BY). The use, distribution or reproduction in other forums is permitted, provided the original author(s) and the copyright owner(s) are credited and that the original publication in this journal is cited, in accordance with accepted academic practice. No use, distribution or reproduction is permitted which does not comply with these terms.
*Correspondence: Wensheng Huang, hwswwbhyx@sina.com