- 1Wuxi School of Medicine, Jiangnan University, Wuxi, China
- 2Department of Nuclear Medicine, Affiliated Hospital of Jiangnan University, Wuxi, China
Background: In the past 5 years, ferroptosis-associated cancer immunity has been attracted significant research interest.
Objective: This study was performed to identify and analyze the global output trend for ferroptosis in cancer immunity.
Methods: Relevant studies were retrieved from the Web of Science Core Collection on Feb 10th, 2023. The VOSviewer and Histcite softwares were utilized to perform the visual bibliometric and deep mining analyses.
Results: A total of 694 studies (530 articles (76.4%) and 164 (23.6%) review articles) were retrieved from the Web of Science Core Collection for visualization analyses. The top 3 key keywords were ferroptosis, prognosis and immunotherapy. The top 30 local citation score (LCS) authors were all collaborators of Zou Weiping. Deep mining of 51 nanoparticle-related articles showed that BIOMATERIALS was the most popular journal. The primary goal of gene signatures related to ferroptosis and cancer immunity was to establish prognostic predictions.
Conclusion: There has been a significant increase in ferroptosis-associated immune publications in the recent 3 years. The key research hotspots include mechanisms, prediction and therapeutic outcomes. The most influential article was from the Zou Weiping’s group, which proposed that system xc-mediated ferroptosis is induced by CD8(+) T cell-secreted IFNγ after PD-L1 blockage for immunotherapy. The frontier of research in the field of ferroptosis-associated immune is the study on nanoparticle and gene signature The limitation of this bibliometric study is that publications on this topic are few.
1 Introduction
Cancer is a malignant tumor. People are more likely to die from malignant tumors as their incidences have risen significantly in recent years. According to the latest cancer epidemiological data, nearly 10 million people died of cancer in 2020, accounting for nearly one in six deaths, which highlights the serious threat the disease imposes on public health (https://www.who.int/news-room/fact-sheets/detail/cancer). Cancer can be treated in various ways, and current strategies involve a combination of surgery, radiotherapy, chemotherapy, immunotherapy, and other methods (1). Among these strategies, immunotherapies are the most promising. Major immunotherapy approaches include inhibitory immune checkpoints (ICB) blockade, antigen-specific peptide vaccination, oncolytic virotherapy, and adoptive cell therapies, and they are based on the key role of tumor-specific T cell activation (2). Immunogenic cell death (ICD) caused by immunotherapy results in long-lasting and effective immunological memory compared to other types of cell death (3–5).
However, many cancer cells enable to develop immune escape and relapse pathways, so some cancer types cannot respond to effective primary immunotherapy (6). Besides immunotherapy triggered ICD, ferroptosis is another type of immunogenic cell death (7). A study by Dmitri V Krysko et al. showed that early ferroptotic tumor cells stimulated tumor immunity, produced immune memory, and persistently killed cancer cells (8). Ferroptosis was first proposed and named by Stockwell BR’s group, which pioneered studies on ferroptosis (9). Canonical ferroptosis involves the suppression of system xc−, glutathione (GSH), and glutathione peroxidase 4 (GPX4), which accumulates reactive oxygen species (ROS), phospholipid peroxidation (PUFA-PL), and iron (10, 11). Additionally, Acyl-CoA Synthetase Long-Chain Family Member 4 (ACSL4) is a promoter that executes ferroptosis. High ACSL4 expression or activity sensitize and promote cell ferroptosis (12, 13). In addition, targeting ferroptosis is considered to be an effective cancer treatment strategy. Most of the treatment strategies are based on the mechanism of ferroptosis are aimed at identifying sensitive signature panels of ferroptosis and improve the efficacy of anti-cancer therapies. Numerous studies have reported that ferroptosis inducers are sensitive to many cancers such as glioma, triple negative breast cancer (14, 15) and provide more opportunities for the treatment of cancer (16).
Early in 2018, Jennifer Tsoi et al. first showed that ferroptosis-inducing agents are sensitive to reduced dedifferentiated melanoma cells after induction of Interferon Gamma (IFNγ) (17). Subsequently, Zou’s group found that the ovarian cancer cells killed by immunotherapy PD-L1 blockage were ferroptotic, and the mechanism underlying this process involved the function of IFNγ released by PD-L1 blockage-activated CD8 (+) T cells. IFNγ was able to target cancer cells by suppressing system Xc- and lead to ferroptosis as a result (18). In addition, combining immunotherapy and radiotherapy followed the same process (19). However, combining IFNγ and AA triggered ACSL4-induced intrinsic ferroptosis instead (20).
In this study, original articles and review articles on ferroptosis in cancer immunity were identified from the Web of Science Core Collection using VOSviewer. Histcite was used to visualize and analyze the collected articles. This bibliometric study identifies and analyzes the global trend on ferroptosis in cancer immunity. The graphic abstract is shown in Figure 1. Our analysis is expected to inspire researchers and provide reference data to improve future management of scientific work.
2 Materials and methods
2.1 Search strategy and data collections
Bibliometric data for this study were collected from the Web of Science Core Collection, including Science Citation Index Expanded (SCI-EXPANDED), Social Sciences Citation Index (SSCI), Arts & Humanities Citation Index (AHCI), Emerging Sources Citation Index (ESCI) from, Current Chemical Reactions (CCR-EXPANDED), and Index Chemicus (IC). The terms ‘ferroptosis,’ ‘immunity,’ and ‘cancer’ were searched in the MeSH (https://www.ncbi.nlm.nih.gov/mesh). The words or phrases, such as anti-tumor immunity, immunity, tumor immunotherapy, anti-tumor immunotherapies, anti-tumor immune therapy, immune therapy, immunology therapy, anti-tumor immunology therapy, radioimmunotherapy, immunomodulation, and Neoplasm were used in the documents of cancer immunity field. Ferroptotic, cancer(s), and Neoplasm(s) were also applied in some documents. Truncators were adopted to avoid missing documents during searching and to obtain more comprehensive data. *” represented an infinite truncated word, and $ represented a finite truncated word. To obtain a systematic and comprehensive analysis of ferroptosis in the cancer immunity field, the following search strategy was applied; #1 represented (((((((((TS = (anti-tumor immunity)) OR TS = (immunity)) OR TS = (cancer immunotherapy*)) OR TS = (anti-tumor immunotherapy*)) OR TS = (immune therapy*)) OR TS = (anti-tumor immune therapy*)) OR TS = (immunology therapy*)) OR TS = (anti-tumor immunology therapy*)) OR TS = (Radioimmunotherapy*)) OR TS = (Immunomodulation), #2 represented TS = (Neoplasm* or cancer$), and #3 represented TS = (ferropto*). The final search strategy is TS = #1 AND #2 AND #3. Only articles published in English and full articles and reviews were considered. Other document types, including early access, editorial materials, meeting abstracts, corrections, and book chapters, were excluded. The search flow chart is shown in Figure 2. The search was completed on Feb 10th, 2023.
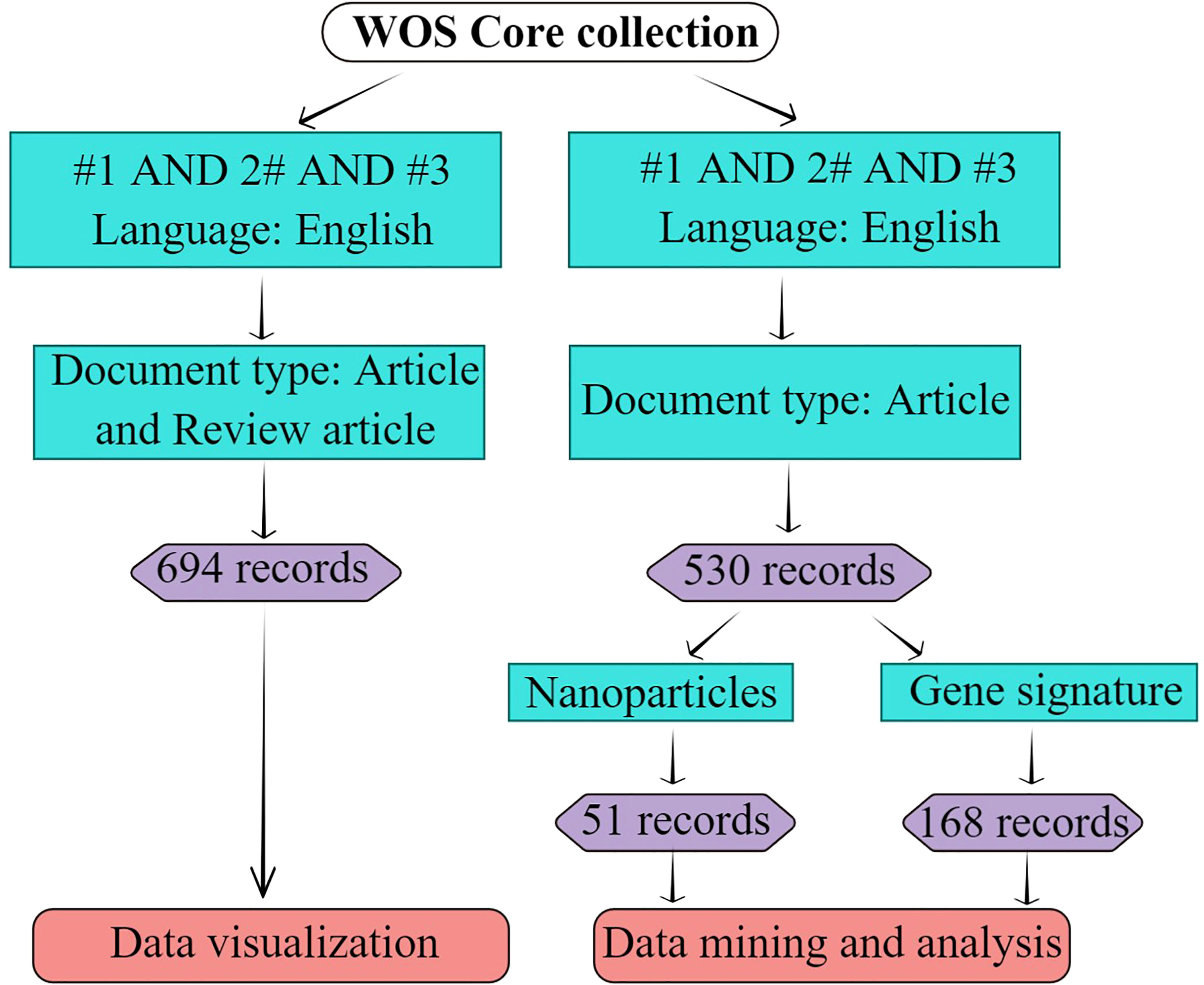
Figure 2 Search strategy. #1 represents “(((((((((TS=(anti-tumor immunity)) OR TS=(immunity)) OR TS=(cancer immunotherapy*)) OR TS=(anti-tumor immunotherapy*)) OR TS=(immune therapy*)) OR TS=(anti-tumor immune therapy*)) OR TS=(immunology therapy*)) OR TS=(anti-tumor immunology therapy*)) OR TS=(Radioimmunotherapy*)) OR TS=(Immunomodulation)”; #2 represents “TS=(Neoplasm* or cancer$)”; #3 represents “TS=(ferropto*)”; “*” represents infinite truncator; “$” represents finite truncator; the retrieval date was Feb 10th, 2023. This figure was drawn by Figdraw of HOME for Researchers.
2.2 Methodology
Bibliometric visualization and deep mining analysis were performed using VOSviewer (VOSviewer version 1.6.18) and HistCite (HistCite Pro 2.1). The distribution of publication years, the ratio of article and review articles, and the statistics of cancer-type were determined using Microsoft Excel 2016.
3 Results
3.1 Years and cancer-type distribution involved in the collected publication documents
A total of 530 (76.4%) articles (after removing one duplicate from 531) and 124 (23.6%) reviews were retrieved (Figure 3A, Tables S1, S2). The chronological distribution of published documents is presented in Figure 3B. Based on the trend line, it was observed that the number of publications on ferroptosis and cancer immunity increased rapidly from 2021 (n = 210, 30.3%) to 2022 (n = 398, 57.3%). As of Feb 10th, 2023, 14 documents have been published, and it is likely that more articles will be published in 2023. Among the 530 articles retrieved, 26 types of cancers were mentioned. Among them, lung cancer, hepatocellular carcinoma and breast cancer were the top three most researched cancer types in research associated with ferroptosis and cancer immunity (Figure 3C).
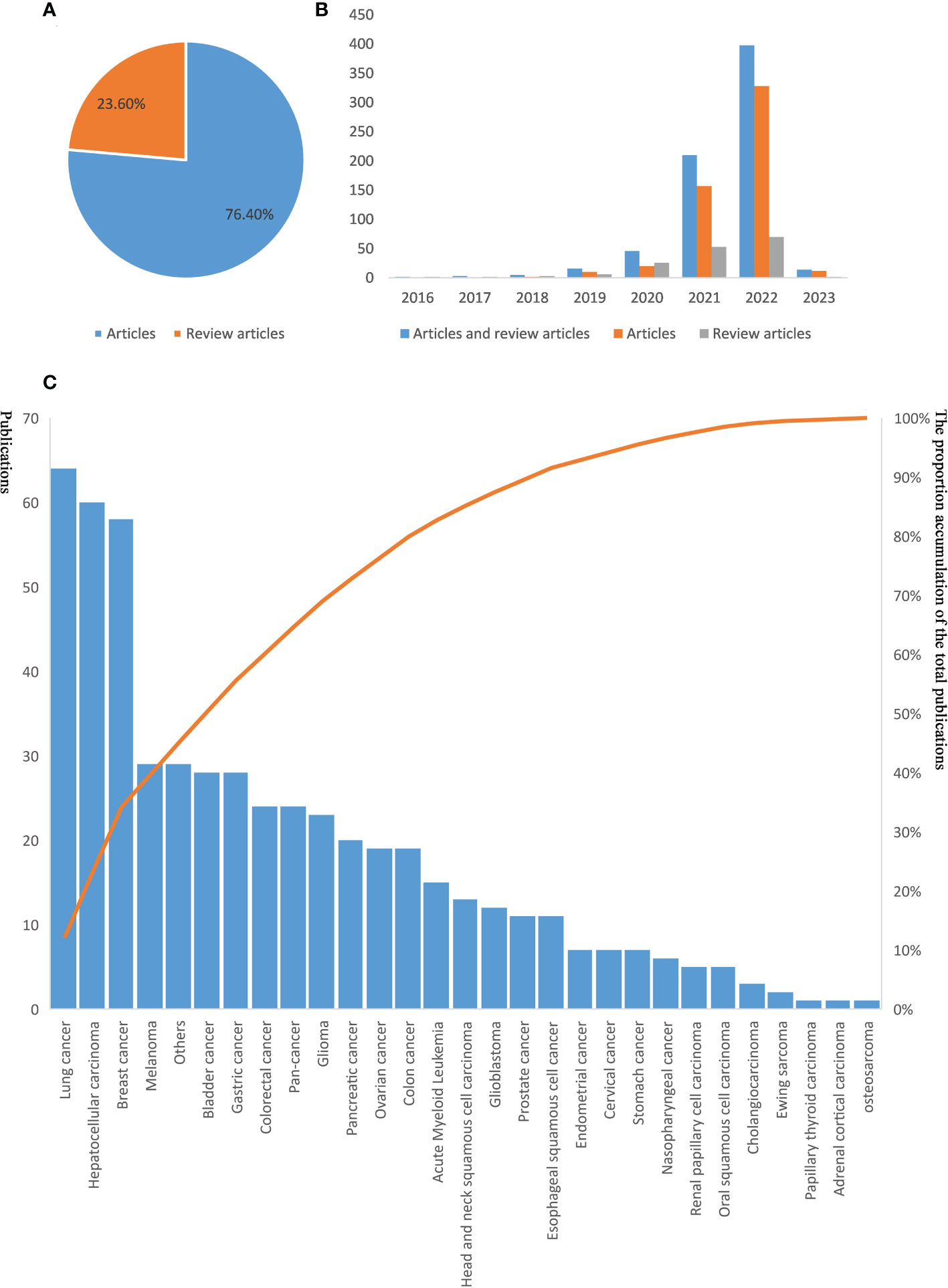
Figure 3 The distribution among years, article type, and cancer type. (A) the proportion of articles and reviews. (B) abscissa axis on the publication year. The vertical coordinate represents the number of published documents, the blue column represents the sum of the article and reviews article documents, the orange column represents article documents, the gray column represents review article documents. (C) distribution of involved cancer type.
3.2 Citation and co-citation analysis of the documents
Histcite and VOSviewer were used to analyze the citation and co-citations of 694 documents. The top 10 global citation score (GCS) and local citation score (LCS) documents are shown in Tables S3, S4. The GCS was determined by analyzing 694 documents using the Histcite software. The GCS ranged from 1,448 to 281, while LCS ranged from 321 to 37. Wang W.M., 2019” ranked first in LCS among the articles, and “Xie Y., 2016” ranked first in GCS among the reviews. The connection network of citation documents analyzed by Histcite is shown in Figure 4A. An article “Wang W.M., 2019” had the highest centrality, indicating that it was the most influential article in the field. Similarly, the connection network visualization through VOSviewer also showed the highest citations of the article “Wang WM, 2019” in article type documents (Figure 4B). To analyze the cited references of documents, a co-citation analysis of cited references was performed. As shown in Figure 4C, the top 10 most cited articles are listed in Table 1. An article by Dixon S.J., 2012 was the most cited publication and was the first article in which ferroptosis was defined.
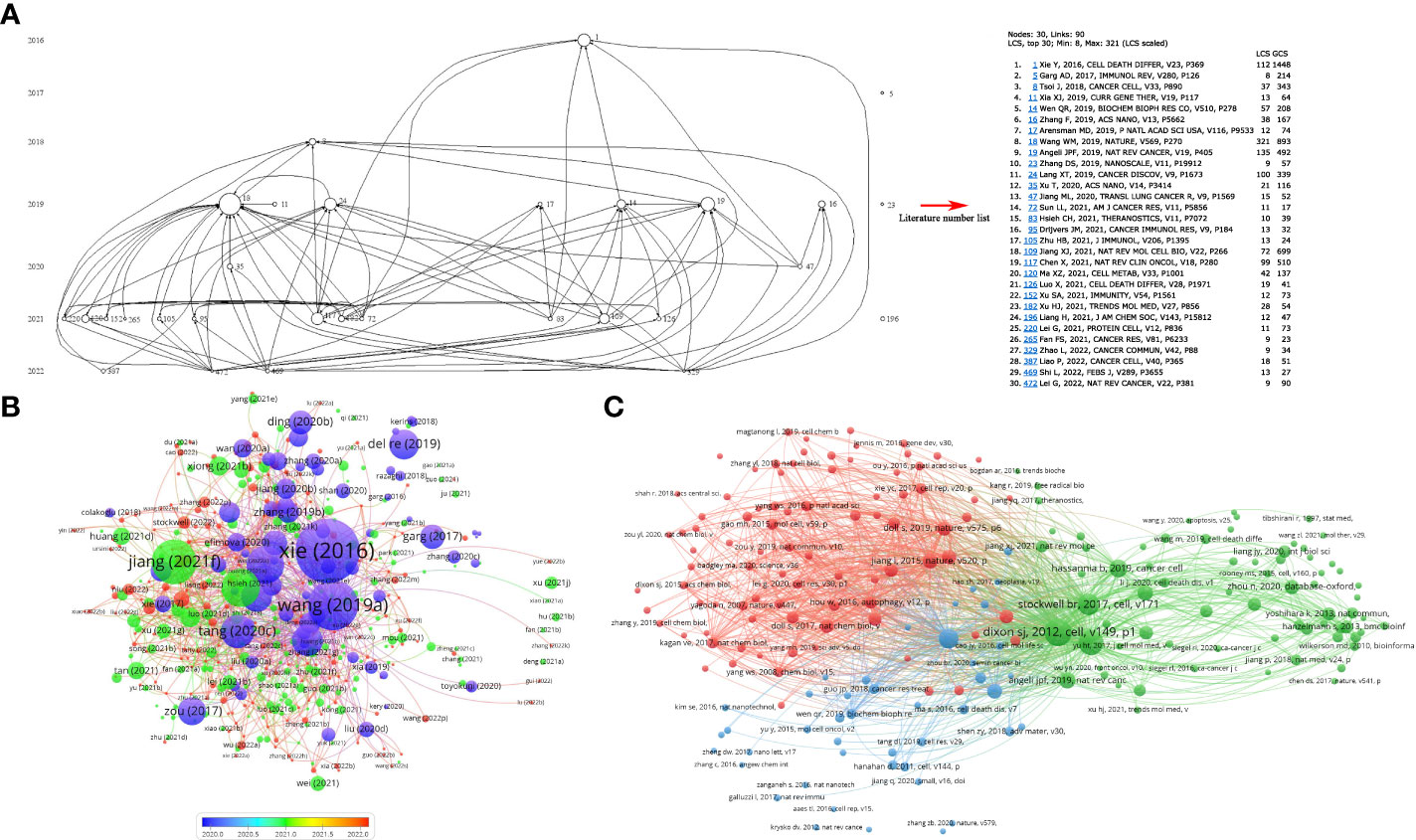
Figure 4 Citation and co-citation of documents. (A) the connection of top 30 LCS documents. The analysis was performed by HistCite; (B) citation of documents analyzed by VOSviewer; (C) co-citation of cited documents analyzed by VOSviewer.
3.3 Association analysis of Keywords
During the articles search, 1,585 keywords were retrieved from 530 articles. The minimum number of occurrences of a keyword was 5, and 178 keywords met the threshold. The network visualization map consisted of 173 keywords that were analyzed using VOSviewer. The map shows the co-occurrence relations of keywords by excluding cancer, expression, cells, death, and cell death, which are unrelated to the topic (Figure 5A). The size of the circle indicates the occurrence of keywords. As shown in Figures 5B–D, the top 3 keywords were ferroptosis, immunotherapy, and prognosis.
Furthermore, some representative keywords were selected based on their close relationship with ferroptosis, immunotherapy, and prognosis, such as resistance, gene signature, and nanoparticles (Figures 5E–G). The keyword “resistance” reflects the common problems in clinical treatment. The keyword “gene signature” is a comprehensive biomarker that can predict disease prognosis, and nanoparticles represent a novel way of treating diseases. The above keywords imply that the clinical problem guided the identification of prediction biomarkers and novel treatment options, and the two are the main focus in the field of ferroptosis and cancer immunity.
3.4 Author connection analysis
The analysis by Histcite software showed that 4,400 authors contributed to 530 articles. The top 30 authors with the highest total global citation score (TGCS) and total local citation score (TLCS) are shown in Table 2. By analyzing the signature of their articles, the 30 authors were correlated with Zou Weiping directly or indirectly. The citation network is shown in Figure 6A. Meanwhile, co-authorship analysis was conducted for the 4,400 authors by VOSviewer. A total of 103 authors had published ≥ 2 articles and were cited more than 100 times. The connection network of the above authors is shown in Figure 6B. Among them, the largest set of author clusters enriched was Zou Weiping and his colleagues, who headed a group of 21 authors (Figure 6C). Furthermore, all articles on ferroptosis and tumor immunity by Zou’s laboratory were retrieved, and four articles have been published so far (Table S5). The four articles were read carefully. Among them, one was a review article on IFNγ signaling in tumor immunity in Jan 2022 (21), and the remaining three articles were published in May 2019, Dec 2019, and Apr 2022 respectively. First, they found that IFNγ released by CD8(+) T cells after PD-L1 blockage induced cancer cells ferroptosis via suppressing system Xc- (18). Then, their second article showed that the combination of immunotherapy and radiotherapy also triggered system xc- mediated ferroptosis via IFNγ released by PD-L1 activated CD8(+) T cells and radiotherapy activated Ataxia- Telangiectasia mutated gene (ATM) respectively (19). Based on the above findings, IFNγ and arachidonic acid (AA) were combined further to induce ferroptosis of cancer cells directly. ACSL4, but not system xc-, was discovered to mediate ferroptosis in the third article (20) (Figure 6D). Another big cluster of authors comprised Krysko, Dmitri, V, and colleagues, who published three articles, one each in 2019, 2021, and 2022 (Table S6, Figure 6B). They first discovered that photosens (PS) or photodithazine (PD)-photodynamic therapy (PDT) (PS-PDT or PD-PDT) induced the death of cancer cells. The death could be reversed by ferroptosis inhibitors. The cancer cells are engulfed by bone marrow-derived dendritic cells (BMDC), in which the BMDCs matured and produced IL6 (22, 23). Then they demonstrated that early ferroptotic tumor cells were immunogenic and promoted the phenotypic maturation of BMDCs by acting as vaccines (8).
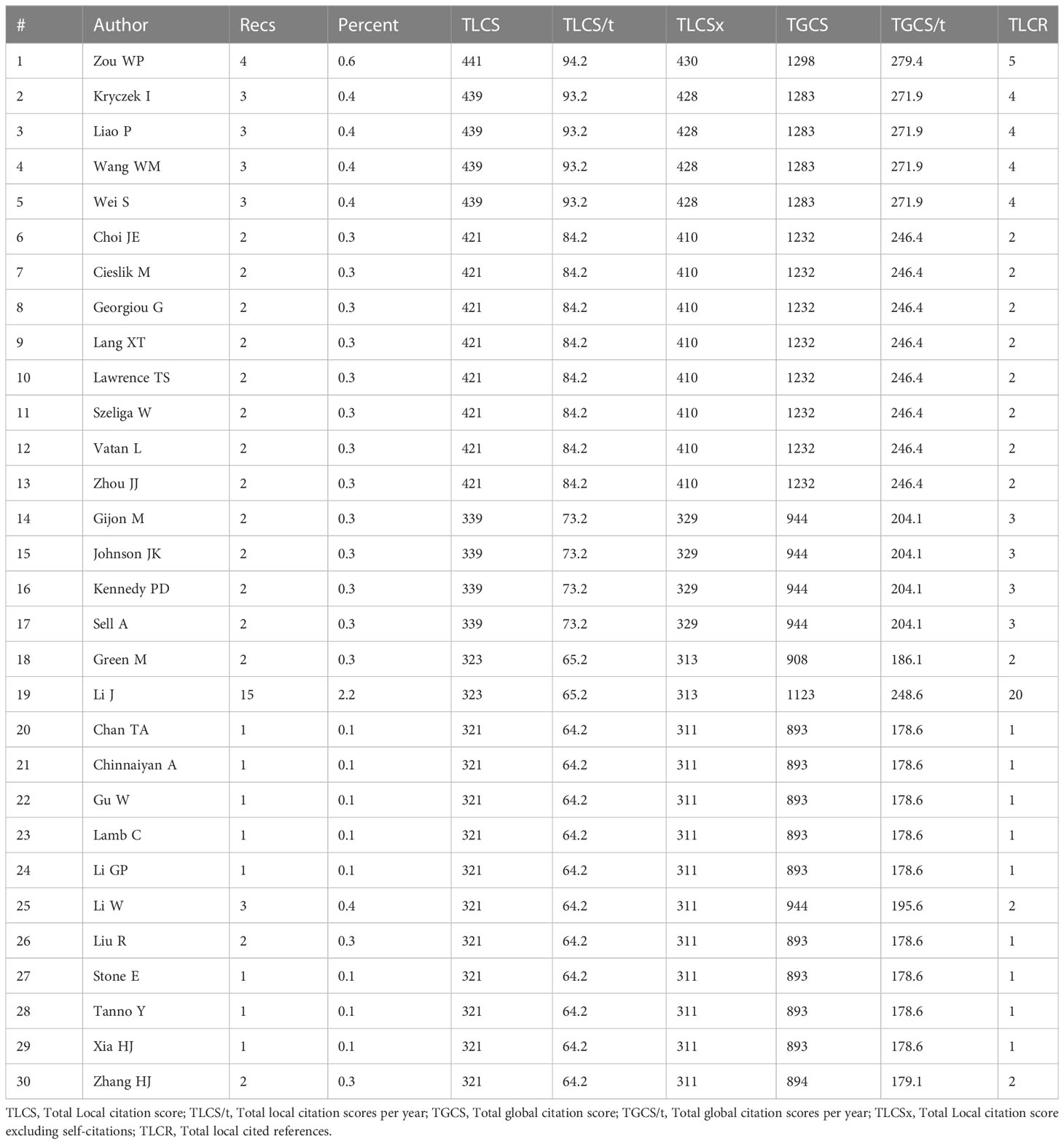
Table 2 Top 30 authors with the highest total global citation score (TGCS) and total local citation score (TLCS).
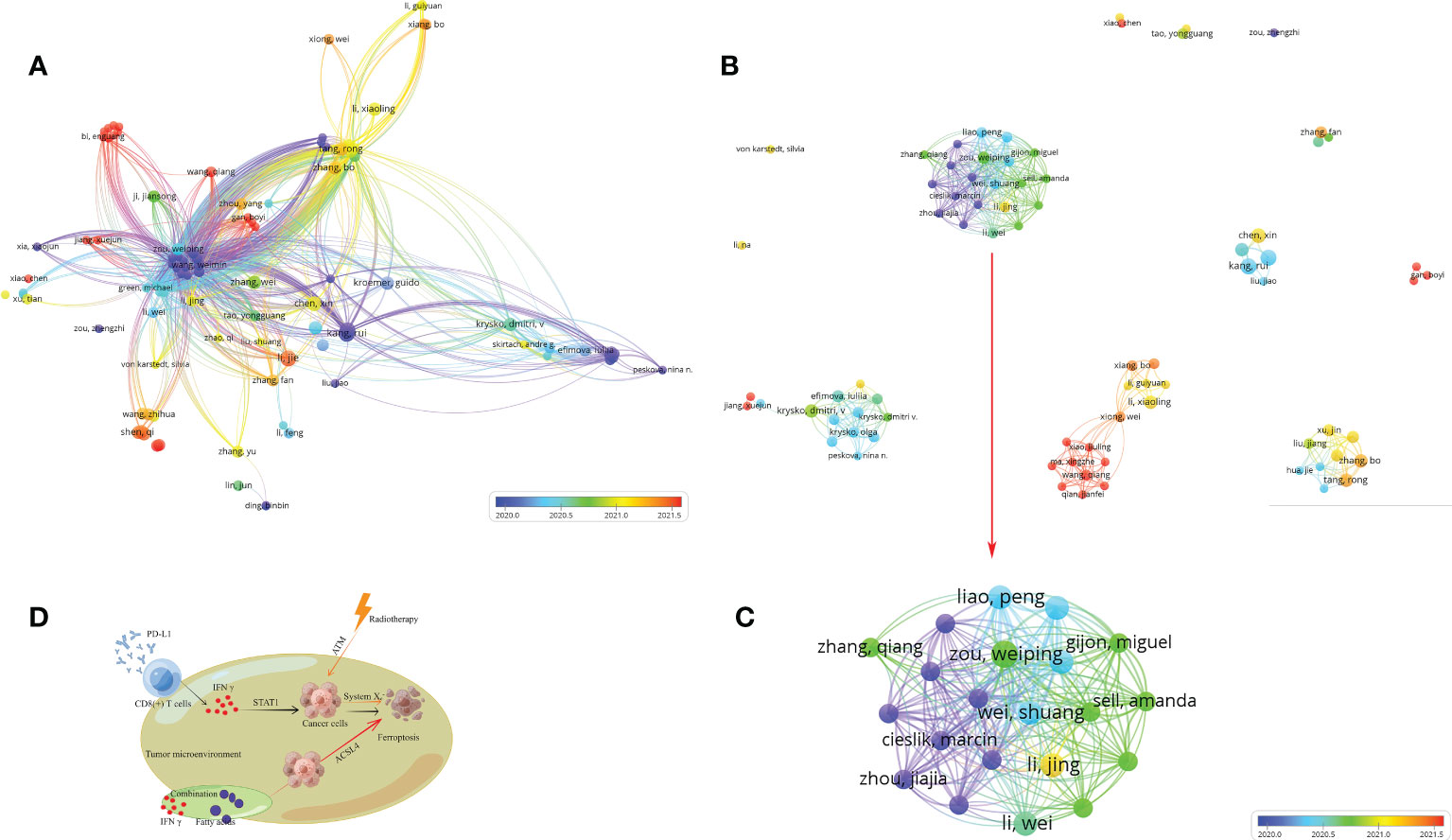
Figure 6 Author and their published articles. (A) citation of authors; (B, C) co-authorship of authors, (C) the red arrow indicates cluster in (B); (D) the mechanism of ferroptosis induction and immunotherapy combination. This figure was drawn by Figdraw of HOME for Researchers.
3.5 Nanoparticle therapy-related articles
According to the connection between ferroptosis and anti-tumor immunity, a series of nanomedicine articles have emerged. The 530 articles were refined by tapping the keyword “nanoparticles”, and 53 documents were collected (Table S7). Next, the source of 53 articles was investigated, and there were 28 journals in total (Table 3). The top four publications were occupied by BIOMATERIALS (6 records), ACS NANO (4 records), SMALL (4 records), and THERANOSTICS (4 records). The top ten most cited articles are listed in Table 4. Furthermore, the top 30 LCS were analyzed by Histcite software. Article by Zhang F, Li F, Lu GH, Nie WD, Zhang LJ, et al. Engineering Magnetosomes for Ferroptosis/Immunomodulation Synergism in Cancer. ACS NANO. 2019 MAY; 13 (5): 5662-5673” had the best center direction and was the most cited (Figure 7A). This article was the first nanomedicine report on ferroptosis and immunotherapy. The article was published in May 2019, almost simultaneously with an influential article by Wang W.M. Wang W.M. (2019) was submitted on Apr 2018 from the USA, and Zhang F. (2019) was submitted on Jan 2019 from China. A literature coupling analysis was conducted, and the total link strength of the 53 articles was ranked from 156 to 23. The top three most linked articles were Du (2022), Jiang (2020), and Sepand (2020) (Figure 7B). 51 articles (excluding one hypothesis and one review from 53 records) on nanoparticles are selected to summarize the details of nanoparticles in fighting cancer. The statistics about the name, composition, and function of the nanoparticles are listed in Table 5. Most of the nanoparticles mentioned above were constructed based on the synergistic effect of ferroptosis and immunotherapy.
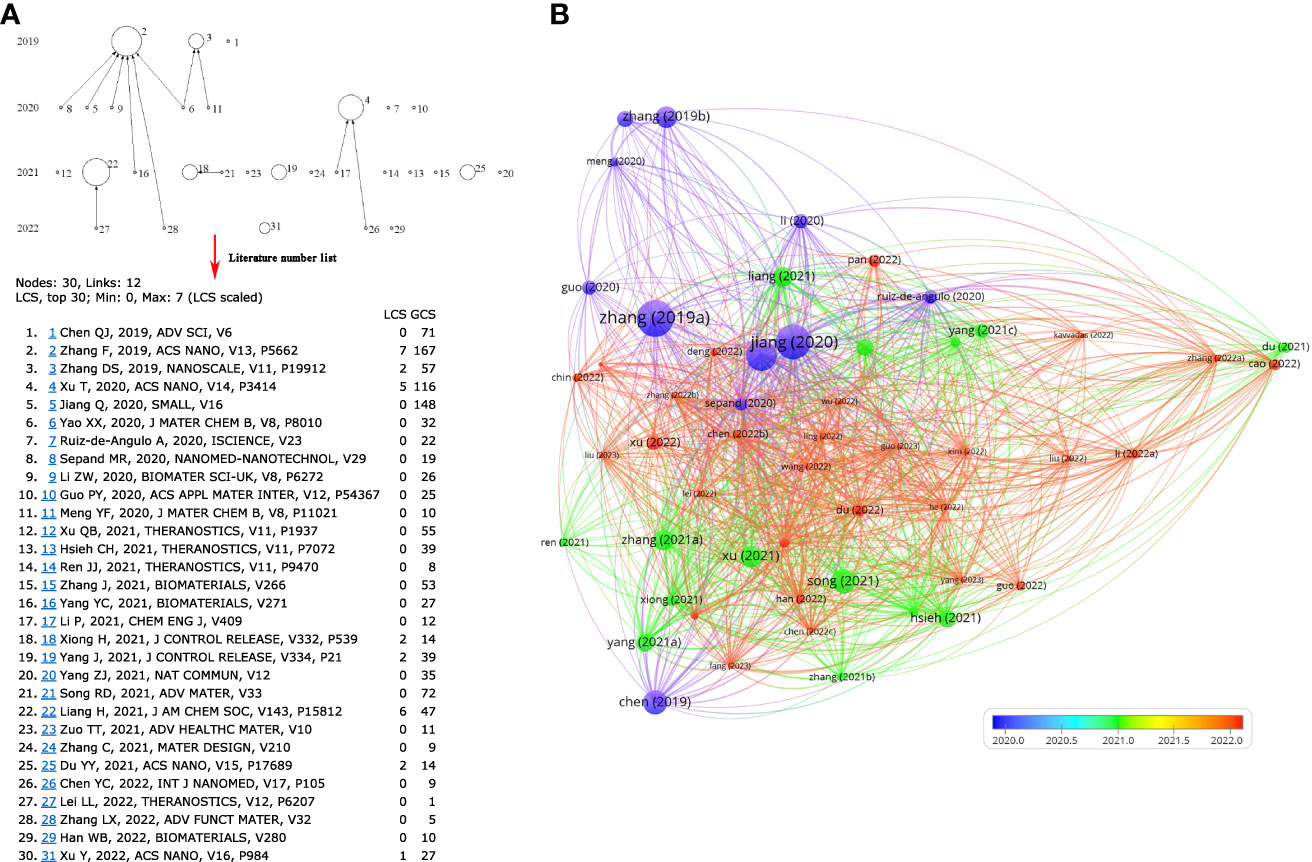
Figure 7 Data mining in nanoparticle-related articles. (A) Top 30 LCS of 53 documents. The analysis was performed by HistCite, the note of Top 30 LCS literature; (B) Literature coupling analysis by VOSviewer.
3.6 Gene signature-related articles
A total of 168 articles out of 530 articles contained the keywords “gene signature” (Table S8). The publication period ranged from 2020 to 2023. As shown in Figure 8A, only one article was published in 2020, 67 articles in 2021, and 97 articles in 2022, three articles in 2023. These articles were screened by reading the title and abstract, and the articles were divided into 10 clusters according to the directions of a gene signature in ferroptosis and cancer immunity (Figure 8B). These articles mainly focused on five aspects of gene signature in the field of ferroptosis and cancer immunity, including diagnosis, sensitivity, therapy, prognosis, and mechanism. The number of articles on the above directions either alone or in combinations, is shown in Figure 8B. Most of these gene signature-related articles were focused on the direction of prognosis, including single and combined directions.
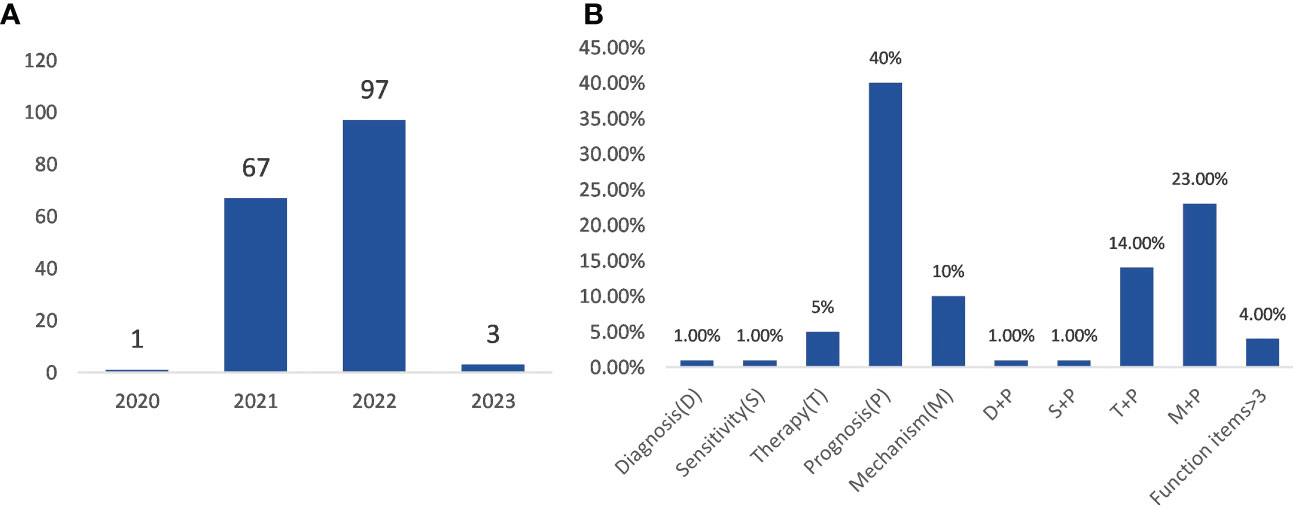
Figure 8 Analysis of gene signature-related articles. (A) the time distribution of gene signature-related article publications; (B) the ratio of research direction to gene signature.
4 Discussion
Since its first discovery, ferroptosis has become popular worldwide. In the last ten years, there has been a sharp increase in the number of documents on ferroptosis and tumor immunity, and these two interrelated fields have gained significant attention in the recent three years (Figure 3B). The increase in the number of articles on cancer and ferroptosis was still observed until the end of 2022. Among these publications, the documents on lung cancer are the most published, maybe because lung cancer has high morbidity and attracts more attention from researchers. Overall, our findings imply that more researchers might focus on ferroptosis and cancer immunity. Keywords analysis in this study presents the hotspots in the field of ferroptosis and cancer immunity, such as prognosis, gene signature, and nanoparticles, which have the potential for prediction and therapy strategy. Gene signature for drug sensitivity or prognosis prediction is popular for big data application in cancer research development based on real data analysis and model validation (75, 76). Overall, the attention on drug sensitivity and treatment outcomes of ferroptosis and cancer immune has increased. In the next few years, more articles on ferroptosis and cancer immunity are anticipated to be published.
Dixon (2012) and Wang (2019) were the most co-cited references. Wang W.M. et al. reported that IFNγ released by CD8(+) T cells after PD-L1 blockage triggered ferroptosis mediated by system xc-, indicating the connection of immunotherapy and ferroptosis and the influence of tumor microenvironment. Wang’s colleagues (Zou’s lab) focused on the synergistic effect of immunotherapy and ferroptosis. Zou’s group contributed a lot to the synergy theory of ferroptosis and immunotherapy. Screening of their articles published in recent years revealed that they mainly focused on cancer immunotherapy. Apart from ferroptosis, they also researched on autophagy and how it may work in synergy with immunotherapy to treat cancer (77). Moreover, T cells and macrophages played a significant role in cancer immunotherapy, which was evident in their published papers (78). From 2019 to 2022, they published more than 20 articles on the mechanism of immunotherapy and some combination treatments, suggesting their persistent efforts in cancer research (Table S9).
In clinical, only a small percentage of patients responded to immune checkpoint inhibitors (ICIs) such as PD-L1. Resistance to checkpoint inhibitors was significant (79). Coincidently, immunotherapy and ferroptosis work in synergy to overcome the resistance of single cancer treatment especially immunotherapy. Apart from ferroptosis, necroptosis and apoptosis also act as important role in killing cancer, However, Krysko, Dmitri et al. showed that ferroptosis had unique immunogenic characteristics. In his research, early ferroptotic tumor cell death stimulated tumor immunity and enhanced immune memory (8). Ferroptotic cancer cells released three DAMPs, including HMGB1, ATP, and CRT, promoting immunogenicity that aided in overcoming resistance to the cancer drug. Furthermore, various lipids released by ferroptotic cancer cells were shown to target immune cells such as DCs and CD8+ T cells to exert the anti-tumor immunity effects (8, 80).
1183405However, conflicting opinions still exist. Research by Peter Vandenabeele‘s group showed that ferroptotic cancer cells impair the function of DCs and cannot stimulate the immune response in cancer patients (81). In addition, another research by this group also showed that ferroptosis induction impairs the recruitment of immune cells and may act as a biomarker to predict the poor outcome of cancer patients (82). In cancer immunity, T lymphocytes are the major regulators of anti-cancer immunity. Antigen-presenting cells (APCs) including dendritic cells (DCs) and macrophages present antigenic peptides of cancer cells to T cells, the peptide binding major histocompatibility complex molecules (MHCs) is exposed on the cell surface to be recognized by T cell receptors (TCRs) and form TCR-peptide-MHC complex that activates the T cell (83). Therefore, the activation of APC cells and T cells plays a major role in anti-cancer immunity. Initially, the cytokine IFNγ was identified to be primarily secreted by CD8+ T cells and known to affect the dedifferentiation degree of melanomas cells. The dedifferentiation subtype of melanomas cells is promoted by IFNγ in response to ferroptosis inducers. It is therefore speculated that ferroptosis inducers in combination with cytokine stimulators may promote anti-tumor immunity (17). Subsequently, a research published on the nature journal demonstrated that the IFNγ secretion of CD8+ T cells activated by PD-L1 blockage promoted ferroptosis of CD45- ID8 cells, and combination of cyst(e)inase and PD-L1 blockage significantly increased the anti-cancer efficacy (18). Subsequently, Iuliia Efimova and her colleagues suggested that when early ferroptotic cancer cells (specifically MCA205 cells) are taken up by BMDCs, they can trigger the activation of BMDCs by inducing the expression of CD80, CD86, and MHCII on BMDCs, potentially serving as a cancer treatment vaccine. They confirmed the strong immunogenic potential of early ferroptotic cancer cells (8). Nevertheless, conflicting findings emerged from Peter Vandenabeele’s laboratory, wherein they replicated the experimental conditions used by Iuliia Efimova’s team but discovered that early ferroptotic cancer cells are non-immunogenic and do not trigger anti-tumor immunity. Moreover, they discovered that the corpses of ferroptotic cancer cells were negatively correlated with the activation and proliferation of CD8+ T cells (81). A recent study revealed that pathologically activated neutrophils and myeloid-derived suppressor cells (PMN-MDSCs) in human tumors, exhibiting elevated ferroptosis levels, are particularly susceptible to ferroptosis via the regulation of FATP2. Consequently, the functionality and proliferation of T cells within the tumor microenvironment are impeded, with the sensitivity of ferroptosis in PMNs being contingent on hypoxia. Notably, the combination of ferroptosis inhibition and PD-1 antibody has been found to augment antitumor immunity (84). The cells used in the study were obtained from tumor-bearing animals not directly from cell lines, and the hypoxia condition of tumor microrenvironment (TME) was developed in vitro. Thus, the results of the study may more accurately match clinical findings. An alternative hypothetical explanation for the conflicting viewpoints is the immune cell status in response to ferroptotic signals. Specifically, if T cells or DC cells are already activated by immunotherapy within the tumor microenvironment, then the induction of ferroptosis may potentially synergize with the anti-tumor immunity. Conversely, if the antitumor immunity is unresponsive, the hierarchy of cell death within the tumor microenvironment is determined by the varying sensitivity of cell types to ferroptosis. Under such circumstances, if immune cells are more susceptible to ferroptosis, then Rina Kim’s findings may be more comprehensible. Further evidence from scholars is necessary to validate the veracity of these findings. Overall, research into the intersection of ferroptosis and tumor immunity has made significant progress in recent years, as evidenced by the milestones highlighted in Figure 9.
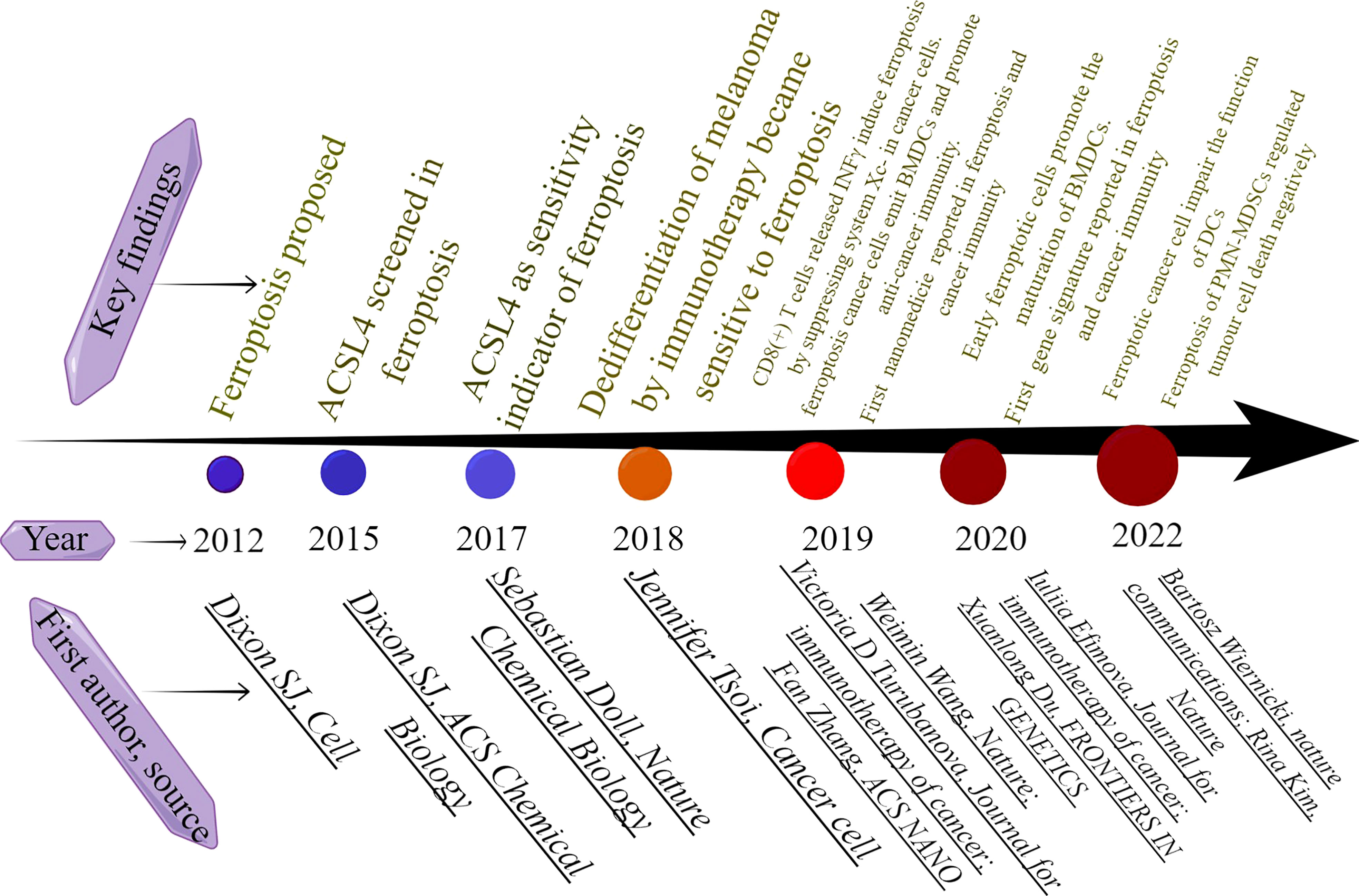
Figure 9 The milestones in ferroptosis and cancer immunity. This figure was drawn by Figdraw of HOME for Researchers.
With the development of the synergy theory of ferroptosis and cancer immunity, a series of applications that show great potential, such as nano-medicine and gene signature-based prediction, have emerged. Nanoparticles are applied in combination with ferroptosis, and immunotherapies. In three years, 51 articles about nanoparticle designs based on ferroptosis and immunotherapies targets had already been published. This number of publications indicated that researchers are confident in potentially inducing synergistic effects on ferroptosis and immunotherapies. The design of nanoparticles for inducing ferroptosis primarily focuses on targeting ROS, iron overload, GSH, and certain ferroptosis-regulated molecules, including system xc-, GPX4, and ACSL4 (85–90). ROS is one of the most important mechanisms that is applied during the formulation of nano-medicines. On one hand, physiological ROS accumulation can promote the death of cancer cells. Therefore, the nanoparticles such as PPS designed by Xianwen Wang and his colleagues aimed to increase the accumulation of H2O2, decrease the amounts of glutathione (GSH) and cause death by targeting cancer cells (91). As such, PPS may serve as an ideal complement to ferroptosis inducers and/or immunotherapies, thereby improving the effectiveness of cancer treatment. However, the rapid buildup of ROS in cancer cells over a short period can result in a severe inflammatory response. To resolve the excess ROS problems caused by PPT, Xianwen Wang and his colleagues designed ultrasmall ZrC NDs, which eliminates ROS to decrease inflammation caused by PTT treatment for glioma (92). Subsequently, ultrasmall ZrC–PVP nanodots (NDs) (ZrC–PVP NDs) based on the combination of PTT–RT photothermal therapy (PTT) -Radiation therapy (RT) was designed for glioma treatment (93). Furthermore, they attempted to disrupt the respiration process and modify the oxidation state of cancer cells by continuously generating H2 through the transfer of MgG rods into the cancer cells. This approach has the potential to trigger a robust synergistic effect with ferroptosis inducers and immunotherapies (94). As an increasing number of nano-medicines for cancer treatment based on ROS, GSH, and iron are being explored, the long-term accumulation and biodegradability of these medicines may prove critical to their clinical translation (95). So far, nanoparticles combining ferroptosis induction and immunotherapies are very diverse. However, there is still a long way to go for patients to benefit, considering the transformation, clinic trial, production volume, and economy.
Apart from nanoparticles, the gene signatures for prognosis prediction in ferroptosis and cancer immunotherapies are hotspots that attract the attention of so many researchers. The traction on gene signature indicates that it may be promising for evaluating patients in the future. The gene signature research is based on big data and data mining, which reflects the principles of gene presentation and paves the way for treatment based on translation research. Apart from prediction, prompt treatment based on the combination of ferroptosis induction and immunotherapy is urgent, but currently, the strategy is full of challenges.
In summary, publications on ferroptosis and tumor immunotherapy have increased rapidly in the last 3 years, and most studies were on lung cancer. Zou Weiping’s laboratory contributed a lot and published the most influential articles. The mechanism, prediction, and therapy directions of this topic are hotspots in the research trends. Particularly, the development of nanomedicines based on the benefit of combining ferroptosis and immunotherapy has been rapid, and it presents promising hotspots in future research.
4.1 Limitation
Nevertheless, this bibliometric study has limitations that should be mentioned. The search date in this study in the Web of Science Core Collection was Feb 10th, 2023. Since the data is constantly updated, some parts of documents in this field, from the search date to the publication date, are not included. Another limitation is that the keywords tapped are TS and are mostly present in the title or the abstract. Therefore, some useful keywords in the main text could be missed. The third reason is that the database selected for the study may not be comprehensive enough to cover all documents in this field. Last but not least, there are conflicting views on whether ferroptosis is immunogenic or not and its role in affecting immune cells. No other group provides external evidence to support or disprove their hypothesis. Maybe it will be revealed by more research in the near future. Other limitations in this study have been previously described (96, 97).
Data availability statement
The original contributions presented in the study are included in the article/Supplementary Material. Further inquiries can be directed to the corresponding author.
Author contributions
ZW and CY designed the search strategy and prepared the manuscript. HZ, LW, ZM, Y’aC, and HF analyzed the data, read the manuscript, and advised on method development. All authors have approved the final version of the manuscript. All authors contributed to the article.
Funding
This study was supported by the National Natural Science Foundation of China (No: 31900528), Natural Science Foundation of Jiangsu Province (BK20190601), Research Project of Public Health Research Center of Jiangnan University (grant number: JUPH201822), and Youth Fund for Basic Research Project of Jiangnan University (grant number: JUSRP11953). This research was supported by the Wuxi school of medicine and its affiliated hospital of Jiangnan University.
Acknowledgments
We thank Professor Kun Xiong from Central South University for his instruction to this research.
Conflict of interest
The authors declare that the research was conducted in the absence of any commercial or financial relationships that could be construed as a potential conflict of interest.
Publisher’s note
All claims expressed in this article are solely those of the authors and do not necessarily represent those of their affiliated organizations, or those of the publisher, the editors and the reviewers. Any product that may be evaluated in this article, or claim that may be made by its manufacturer, is not guaranteed or endorsed by the publisher.
Supplementary material
The Supplementary Material for this article can be found online at: https://www.frontiersin.org/articles/10.3389/fonc.2023.1183405/full#supplementary-material
References
1. Qu X, Zhou D, Lu J, Qin D, Zhou J, Liu HJ. Cancer nanomedicine in preoperative therapeutics: nanotechnology-enabled neoadjuvant chemotherapy, radiotherapy, immunotherapy, and phototherapy. Bioact Mater (2023) 24:136–52. doi: 10.1016/j.bioactmat.2022.12.010
2. Iorgulescu JB, Braun D, Oliveira G, Keskin DB, Wu CJ. Acquired mechanisms of immune escape in cancer following immunotherapy. Genome Med (2018) 10(1):87. doi: 10.1186/s13073-018-0598-2
3. Yan WT, Lu S, Yang YD, Ning WY, Cai Y, Hu XM, et al. Research trends, hot spots and prospects for necroptosis in the field of neuroscience. Neural Regener Res (2021) 16(8):1628–37. doi: 10.4103/1673-5374.303032
4. Niu X, Chen L, Li Y, Hu Z, He F. Ferroptosis, necroptosis, and pyroptosis in the tumor microenvironment: perspectives for immunotherapy of SCLC. Semin Cancer Biol (2022) 86(Pt 3):273–85. doi: 10.1016/j.semcancer.2022.03.009
5. Yan WT, Zhao WJ, Hu XM, Ban XX, Ning WY, Wan H, et al. PANoptosis-like cell death in ischemia/reperfusion injury of retinal neurons. Neural Regener Res (2023) 18(2):357–63. doi: 10.4103/1673-5374.346545
6. O'Donnell JS, Teng MWL, Smyth MJ. Cancer immunoediting and resistance to T cell-based immunotherapy. Nat Rev Clin Oncol (2019) 16(3):151–67. doi: 10.1038/s41571-018-0142-8
7. Friedmann Angeli JP, Krysko DV, Conrad M. Ferroptosis at the crossroads of cancer-acquired drug resistance and immune evasion. Nat Rev Cancer (2019) 19(7):405–14. doi: 10.1038/s41568-019-0149-1
8. Efimova I, Catanzaro E, van der Meeren L, Turubanova VD, Hammad H, Mishchenko TA, et al. Vaccination with early ferroptotic cancer cells induces efficient antitumor immunity. J Immunother Cancer (2020) 8(2). doi: 10.1136/jitc-2020-001369
9. Dixon SJ, Lemberg KM, Lamprecht MR, Skouta R, Zaitsev EM, Gleason CE, et al. Ferroptosis: an iron-dependent form of nonapoptotic cell death. Cell (2012) 149(5):1060–72. doi: 10.1016/j.cell.2012.03.042
10. Jiang X, Stockwell BR, Conrad M. Ferroptosis: mechanisms, biology and role in disease. Nat Rev Mol Cell Biol (2021) 22(4):266–82. doi: 10.1038/s41580-020-00324-8
11. Chen J, Wang Y, Li M, Zhu X, Liu Z, Chen Q, et al. Netrin-1 alleviates early brain injury by regulating ferroptosis via the PPARgamma/Nrf2/GPX4 signaling pathway following subarachnoid hemorrhage. Transl Stroke Res (2023). doi: 10.1007/s12975-022-01122-4
12. Yuan H, Li X, Zhang X, Kang R, Tang D. Identification of ACSL4 as a biomarker and contributor of ferroptosis. Biochem Biophys Res Commun (2016) 478(3):1338–43. doi: 10.1016/j.bbrc.2016.08.124
13. Doll S, Proneth B, Tyurina YY, Panzilius E, Kobayashi S, Ingold I, et al. ACSL4 dictates ferroptosis sensitivity by shaping cellular lipid composition. Nat Chem Biol (2017) 13(1):91–8. doi: 10.1038/nchembio.2239
14. Luo Y, Tian G, Fang X, Bai S, Yuan G, Pan Y. Ferroptosis and its potential role in glioma: from molecular mechanisms to therapeutic opportunities. Antioxidants (Basel) (2022) 11(11). doi: 10.3390/antiox11112123
15. Yang F, Xiao Y, Ding JH, Jin X, Ma D, Li DQ, et al. Ferroptosis heterogeneity in triple-negative breast cancer reveals an innovative immunotherapy combination strategy. Cell Metab (2023) 35(1):84–100 e8. doi: 10.1016/j.cmet.2022.09.021
16. Motooka Y, Toyokuni S. Ferroptosis as ultimate target of cancer therapy. Antioxid Redox Signal (2023). doi: 10.1089/ars.2022.0048
17. Tsoi J, Robert L, Paraiso K, Galvan C, Sheu KM, Lay J, et al. Multi-stage differentiation defines melanoma subtypes with differential vulnerability to drug-induced iron-dependent oxidative stress. Cancer Cell (2018) 33(5):890–904 e5. doi: 10.1016/j.ccell.2018.03.017
18. Wang W, Green M, Choi JE, Gijon M, Kennedy PD, Johnson JK, et al. CD8(+) T cells regulate tumour ferroptosis during cancer immunotherapy. Nature (2019) 569(7755):270–4. doi: 10.1038/s41586-019-1170-y
19. Lang X, Green MD, Wang W, Yu J, Choi JE, Jiang L, et al. Radiotherapy and immunotherapy promote tumoral lipid oxidation and ferroptosis via synergistic repression of SLC7A11. Cancer Discov (2019) 9(12):1673–85. doi: 10.1158/2159-8290.CD-19-0338
20. Liao P, Wang W, Wang W, Kryczek I, Li X, Bian Y, et al. CD8(+) T cells and fatty acids orchestrate tumor ferroptosis and immunity via ACSL4. Cancer Cell (2022) 40(4):365–378 e6. doi: 10.1016/j.ccell.2022.02.003
21. Du W, Frankel TL, Green M, Zou W. IFNgamma signaling integrity in colorectal cancer immunity and immunotherapy. Cell Mol Immunol (2022) 19(1):23–32. doi: 10.1038/s41423-021-00735-3
22. Turubanova VD, Balalaeva IV, Mishchenko TA, Catanzaro E, Alzeibak R, Peskova NN, et al. Immunogenic cell death induced by a new photodynamic therapy based on photosens and photodithazine. J Immunother Cancer (2019) 7(1):350. doi: 10.1186/s40425-019-0826-3
23. Turubanova VD, Mishchenko TA, Balalaeva IV, Efimova I, Peskova NN, Klapshina LG, et al. Novel porphyrazine-based photodynamic anti-cancer therapy induces immunogenic cell death. Sci Rep (2021) 11(1):7205. doi: 10.1038/s41598-021-86354-4
24. Yang Q, Liu T, Zheng H, Zhou Z, Huang Y, Jia H, et al. A nanoformulation for immunosuppression reversal and broad-spectrum self-amplifying antitumor ferroptosis-immunotherapy. Biomaterials (2023) 292:121936. doi: 10.1016/j.biomaterials.2022.121936
25. He Z, Zhou H, Zhang Y, Du X, Liu S, Ji J, et al. Oxygen-boosted biomimetic nanoplatform for synergetic phototherapy/ferroptosis activation and reversal of immune-suppressed tumor microenvironment. Biomaterials (2022) 290:121832. doi: 10.1016/j.biomaterials.2022.121832
26. Zhang M, Fang Z, Cui M, Liu K. Multifunctional metal complex-based gene delivery for tumour immune checkpoint blockade combination therapy. J Drug Target (2022) 30(7):753–66. doi: 10.1080/1061186X.2022.2056186
27. Hsieh CH, Hsieh HC, Shih FS, Wang PW, Yang LX, Shieh DB, et al. An innovative NRF2 nano-modulator induces lung cancer ferroptosis and elicits an immunostimulatory tumor microenvironment. Theranostics (2021) 11(14):7072–91. doi: 10.7150/thno.57803
28. Yang J, Ma S, Xu R, Wei Y, Zhang J, Zuo T, et al. Smart biomimetic metal organic frameworks based on ROS-ferroptosis-glycolysis regulation for enhanced tumor chemo-immunotherapy. J Control Release (2021) 334:21–33. doi: 10.1016/j.jconrel.2021.04.013
29. Yang Z, Zhu Y, Dong Z, Li W, Yang N, Wang X, et al. Tumor-killing nanoreactors fueled by tumor debris can enhance radiofrequency ablation therapy and boost antitumor immune responses. Nat Commun (2021) 12(1):4299. doi: 10.1038/s41467-021-24604-9
30. Zuo T, Fang T, Zhang J, Yang J, Xu R, Wang Z, et al. pH-sensitive molecular-Switch-Containing polymer nanoparticle for breast cancer therapy with ferritinophagy-cascade ferroptosis and tumor immune activation. Adv Healthc Mater (2021) 10(21):e2100683. doi: 10.1002/adhm.202100683
31. Guo WH, Chen Z, Li ZH, Huang HL, Ren YX, Li ZY, et al. Cancer cell membrane biomimetic mesoporous silica nanotheranostics for enhanced ferroptosis-mediated immuogenic cell death on gastric cancer. Chem Eng J (2023) 455. doi: 10.1016/j.cej.2022.140868
32. Wei YW, Wang ZH, Yang J, Xu R, Deng HZ, Ma SY, et al. Reactive oxygen species/photothermal therapy dual-triggered biomimetic gold nanocages nanoplatform for combination cancer therapy via ferroptosis and tumor-associated macrophage repolarization mechanism. J Colloid Interface Sci (2022) 606:1950–65. doi: 10.1016/j.jcis.2021.09.160
33. Zhang J, Yang J, Zuo T, Ma S, Xokrat N, Hu Z, et al. Heparanase-driven sequential released nanoparticles for ferroptosis and tumor microenvironment modulations synergism in breast cancer therapy. Biomaterials (2021) 266:120429. doi: 10.1016/j.biomaterials.2020.120429
34. Kim KS, Choi B, Choi H, Ko MJ, Kim DH, Kim DH. Enhanced natural killer cell anti-tumor activity with nanoparticles mediated ferroptosis and potential therapeutic application in prostate cancer. J Nanobiotechnol (2022) 20(1):428. doi: 10.1186/s12951-022-01635-y
35. Zhang C, Liu HF, Gong M, Yang M, Yang ZQ, Xie YL, et al. Biomimetic gold nanocages for overcoming chemoresistance of osteosarcoma by ferroptosis and immunogenic cell death. Materials Design (2021) 210. doi: 10.1016/j.matdes.2021.110087
36. Li Z, Rong L. Cascade reaction-mediated efficient ferroptosis synergizes with immunomodulation for high-performance cancer therapy. Biomater Sci (2020) 8(22):6272–85. doi: 10.1039/d0bm01168a
37. Yao X, Yang B, Wang S, Dai Z, Zhang D, Zheng X, et al. A novel multifunctional FePt/BP nanoplatform for synergistic photothermal/photodynamic/chemodynamic cancer therapies and photothermally-enhanced immunotherapy. J Mater Chem B (2020) 8(35):8010–21. doi: 10.1039/d0tb00411a
38. Lei L, Dong Z, Xu L, Yang F, Yin B, Wang Y, et al. Metal-fluorouracil networks with disruption of mitochondrion enhanced ferroptosis for synergistic immune activation. Theranostics (2022) 12(14):6207–22. doi: 10.7150/thno.75323
39. Jiang Q, Wang K, Zhang X, Ouyang B, Liu H, Pang Z, et al. Platelet membrane-camouflaged magnetic nanoparticles for ferroptosis-enhanced cancer immunotherapy. Small (2020) 16(22):e2001704. doi: 10.1002/smll.202001704
40. Chen C, Du W, Jing WQ, Sun P, Shi CD, Zhang SC, et al. Leveraging tumor cell ferroptosis for colorectal cancer treatment via nanoelicitor-activated tumoricidal immunity. Chem Eng J (2022) 430:132983. doi: 10.1016/j.cej.2021.132983
41. Guo P, Wang L, Shang W, Chen J, Chen Z, Xiong F, et al. Intravesical In Situ immunostimulatory gel for triple therapy of bladder cancer. ACS Appl Mater Interfaces (2020) 12(49):54367–77. doi: 10.1021/acsami.0c15176
42. Zhang F, Li F, Lu GH, Nie W, Zhang L, Lv Y, et al. Engineering magnetosomes for Ferroptosis/Immunomodulation synergism in cancer. ACS Nano (2019) 13(5):5662–73. doi: 10.1021/acsnano.9b00892
43. Li Q, Su R, Bao X, Cao K, Du Y, Wang N, et al. Glycyrrhetinic acid nanoparticles combined with ferrotherapy for improved cancer immunotherapy. Acta Biomater (2022) 144:109–20. doi: 10.1016/j.actbio.2022.03.030
44. Ling YY, Wang WJ, Hao L, Wu XW, Liang JH, Zhang H, et al. Self-amplifying Iridium(III) photosensitizer for ferroptosis-mediated immunotherapy against transferrin receptor-overexpressing cancer. Small (2022) 18(49):e2203659. doi: 10.1002/smll.202203659
45. Chen Q, Liu L, Lu Y, Chen X, Zhang Y, Zhou W, et al. Tumor microenvironment-triggered aggregated magnetic nanoparticles for reinforced image-guided immunogenic chemotherapy. Adv Sci (Weinh) (2019) 6(6):1802134. doi: 10.1002/advs.201802134
46. Han W, Duan X, Ni K, Li Y, Chan C, Lin W. Co-Delivery of dihydroartemisinin and pyropheophorbide-iron elicits ferroptosis to potentiate cancer immunotherapy. Biomaterials (2022) 280:121315. doi: 10.1016/j.biomaterials.2021.121315
47. Ruiz-de-Angulo A, Bilbao-Asensio M, Cronin J, Evans SJ, Clift MJD, Llop J, et al. Chemically programmed vaccines: iron catalysis in nanoparticles enhances combination immunotherapy and immunotherapy-promoted tumor ferroptosis. iScience (2020) 23(9):101499. doi: 10.1016/j.isci.2020.101499
48. Li J, Zhou Y, Liu J, Yang X, Zhang K, Lei L, et al. Metal-phenolic networks with ferroptosis to deliver NIR-responsive CO for synergistic therapy. J Control Release (2022) 352:313–27. doi: 10.1016/j.jconrel.2022.10.025
49. Wang Y, Chen Q, Song H, Zhang Y, Chen H, Liu P, et al. A triple therapeutic strategy with antiexosomal iron efflux for enhanced ferroptosis therapy and immunotherapy. Small (2022) 18(41):e2201704. doi: 10.1002/smll.202201704
50. Song R, Li T, Ye J, Sun F, Hou B, Saeed M, et al. Acidity-activatable dynamic nanoparticles boosting ferroptotic cell death for immunotherapy of cancer. Adv Mater (2021) 33(31):e2101155. doi: 10.1002/adma.202101155
51. Zhang LX, Song Y, Cao KX, Du YY, Han MD, Shi Z, et al. Hepcidin-based nanocomposites for enhanced cancer immunotherapy by modulating iron export-mediated N6-methyladenosine RNA transcript. Advanced Funct Materials (2022) 32(2):2107195. doi: 10.1002/adfm.202107195
52. Chen C, Wang Z, Jia S, Zhang Y, Ji S, Zhao Z, et al. Evoking highly immunogenic ferroptosis aided by intramolecular motion-induced photo-hyperthermia for cancer therapy. Adv Sci (Weinh) (2022) 9(10):e2104885. doi: 10.1002/advs.202104885
53. Fang T, Ma S, Wei Y, Yang J, Zhang J, Shen Q. Catalytic immunotherapy-photothermal therapy combination for melanoma by ferroptosis-activating vaccine based on artificial nanoenzyme. Materials Today Chem (2023) 27:101308. doi: 10.1016/j.mtchem.2022.101308
54. Chin YC, Yang LX, Hsu FT, Hsu CW, Chang TW, Chen HY, et al. Iron oxide@chlorophyll clustered nanoparticles eliminate bladder cancer by photodynamic immunotherapy-initiated ferroptosis and immunostimulation. J Nanobiotechnol (2022) 20(1):373. doi: 10.1186/s12951-022-01575-7
55. Du YQ, Zhang R, Yang JN, Liu SK, Zhou JL, Zhao RX, et al. A "Closed-loop" therapeutic strategy based on mutually reinforced ferroptosis and immunotherapy. Advanced Funct Materials (2022) 32(13):2111784. doi: 10.1002/adfm.202111784
56. Liang H, Wu X, Zhao G, Feng K, Ni K, Sun X. Renal clearable ultrasmall single-crystal fe nanoparticles for highly selective and effective ferroptosis therapy and immunotherapy. J Am Chem Soc (2021) 143(38):15812–23. doi: 10.1021/jacs.1c07471
57. Xu Q, Zhan G, Zhang Z, Yong T, Yang X, Gan L. Manganese porphyrin-based metal-organic framework for synergistic sonodynamic therapy and ferroptosis in hypoxic tumors. Theranostics (2021) 11(4):1937–52. doi: 10.7150/thno.45511
58. Ren J, Zhou J, Liu H, Jiao X, Cao Y, Xu Z, et al. Ultrasound (US)-activated redox dyshomeostasis therapy reinforced by immunogenic cell death (ICD) through a mitochondrial targeting liposomal nanosystem. Theranostics (2021) 11(19):9470–91. doi: 10.7150/thno.62984
59. Wu F, Chen H, Liu R, Suo Y, Li Q, Zhang Y, et al. Modulation of the tumor immune microenvironment by Bi(2) Te(3) -Au/Pd-Based theranostic nanocatalysts enables efficient cancer therapy. Adv Healthc Mater (2022) 11(19):e2200809. doi: 10.1002/adhm.202200809
60. Liu Y, Quan X, Li J, Huo J, Li X, Zhao Z, et al. Liposomes embedded with PEGylated iron oxide nanoparticles enable ferroptosis and combination therapy in cancer. Natl Sci Rev (2023) 10(1):nwac167. doi: 10.1093/nsr/nwac167
61. Du Y, Han M, Cao K, Li Q, Pang J, Dou L, et al. Gold nanorods exhibit intrinsic therapeutic activity via controlling N6-Methyladenosine-Based epitranscriptomics in acute myeloid leukemia. ACS Nano (2021) 15(11):17689–704. doi: 10.1021/acsnano.1c05547
62. Xu Y, Guo Y, Zhang C, Zhan M, Jia L, Song S, et al. Fibronectin-coated metal-phenolic networks for cooperative tumor chemo-/Chemodynamic/Immune therapy via enhanced ferroptosis-mediated immunogenic cell death. ACS Nano (2022) 16(1):984–96. doi: 10.1021/acsnano.1c08585
63. Pan WL, Tan Y, Meng W, Huang NH, Zhao YB, Yu ZQ, et al. Microenvironment-driven sequential ferroptosis, photodynamic therapy, and chemotherapy for targeted breast cancer therapy by a cancer-cell-membrane-coated nanoscale metal-organic framework. Biomaterials (2022) 283:121449. doi: 10.1016/j.biomaterials.2022.121449
64. Li P, Gao MQ, Hu ZJ, Xu T, Chen JR, Ma YX, et al. Synergistic ferroptosis and macrophage re-polarization using engineering exosome-mimic M1 nanovesicles for cancer metastasis suppression. Chem Eng J (2021) 409:128217. doi: 10.1016/j.cej.2020.128217
65. Yang Y, Tian Q, Wu S, Li Y, Yang K, Yan Y, et al. Blue light-triggered Fe(2+)-release from monodispersed ferrihydrite nanoparticles for cancer iron therapy. Biomaterials (2021) 271:120739. doi: 10.1016/j.biomaterials.2021.120739
66. Zhang D, Cui P, Dai Z, Yang B, Yao X, Liu Q, et al. Tumor microenvironment responsive FePt/MoS(2) nanocomposites with chemotherapy and photothermal therapy for enhancing cancer immunotherapy. Nanoscale (2019) 11(42):19912–22. doi: 10.1039/c9nr05684j
67. Xu T, Ma Y, Yuan Q, Hu H, Hu X, Qian Z, et al. Enhanced ferroptosis by oxygen-boosted phototherapy based on a 2-in-1 nanoplatform of ferrous hemoglobin for tumor synergistic therapy. ACS Nano (2020) 14(3):3414–25. doi: 10.1021/acsnano.9b09426
68. Meng Y, Zhang D, Sun Y, Dai Z, Zhang T, Yu D, et al. Core-shell FePt-cube@covalent organic polymer nanocomposites: a multifunctional nanocatalytic agent for primary and metastatic tumor treatment. J Mater Chem B (2020) 8(48):11021–32. doi: 10.1039/d0tb01981j
69. Liu B, Ji Q, Cheng Y, Liu M, Zhang B, Mei Q, et al. Biomimetic GBM-targeted drug delivery system boosting ferroptosis for immunotherapy of orthotopic drug-resistant GBM. J Nanobiotechnol (2022) 20(1):161. doi: 10.1186/s12951-022-01360-6
70. Deng H, Zhang J, Yang Y, Yang J, Wei Y, Ma S, et al. Chemodynamic and photothermal combination therapy based on dual-modified metal-organic framework for inducing tumor Ferroptosis/Pyroptosis. ACS Appl Mater Interfaces (2022) 14(21):24089–101. doi: 10.1021/acsami.2c00574
71. Cao K, Du Y, Bao X, Han M, Su R, Pang J, et al. Glutathione-bioimprinted nanoparticles targeting of N6-methyladenosine FTO demethylase as a strategy against leukemic stem cells. Small (2022) 18(13):e2106558. doi: 10.1002/smll.202106558
72. Chen Y, Shang H, Wang C, Zeng J, Zhang S, Wu B, et al. RNA-Seq explores the mechanism of oxygen-boosted sonodynamic therapy based on all-in-One nanobubbles to enhance ferroptosis for the treatment of HCC. Int J Nanomed (2022) 17:105–23. doi: 10.2147/IJN.S343361
73. Xiong H, Wang C, Wang Z, Lu H, Yao J. Self-assembled nano-activator constructed ferroptosis-immunotherapy through hijacking endogenous iron to intracellular positive feedback loop. J Control Release (2021) 332:539–52. doi: 10.1016/j.jconrel.2021.03.007
74. Guo W, Wu Z, Chen J, Guo S, You W, Wang S, et al. Nanoparticle delivery of miR-21-3p sensitizes melanoma to anti-PD-1 immunotherapy by promoting ferroptosis. J Immunother Cancer (2022) 10(6):e004381. doi: 10.1136/jitc-2021-004381
75. Kwon MJ. Emerging immune gene signatures as prognostic or predictive biomarkers in breast cancer. Arch Pharm Res (2019) 42(11):947–61. doi: 10.1007/s12272-019-01189-y
76. Goker M, Denys H, van de Vijver K, Braems G. Genomic assays for lobular breast carcinoma. J Clin Transl Res (2022) 8(6):523–31. doi: 10.18053/jctres.08.202206.009
77. Qiao Y, Choi JE, Tien JC, Simko SA, Rajendiran T, Vo JN, et al. Autophagy inhibition by targeting PIKfyve potentiates response to immune checkpoint blockade in prostate cancer. Nat Cancer (2021) 2:978–93. doi: 10.1038/s43018-021-00237-1
78. Chow A, Schad S, Green MD, Hellmann MD, Allaj V, Ceglia N, et al. Tim-4(+) cavity-resident macrophages impair anti-tumor CD8(+) T cell immunity. Cancer Cell (2021) 39(7):973–988 e9. doi: 10.1016/j.ccell.2021.05.006
79. Wang Z, Wang Y, Gao P, Ding J. Immune checkpoint inhibitor resistance in hepatocellular carcinoma. Cancer Lett (2023) 555:216038. doi: 10.1016/j.canlet.2022.216038
80. Demuynck R, Efimova I, Naessens F, Krysko DV. Immunogenic ferroptosis and where to find it?". J Immunother Cancer (2021) 9(12):e003430. doi: 10.1136/jitc-2021-003430
81. Wiernicki B, Maschalidi S, Pinney J, Adjemian S, Vanden Berghe T, Ravichandran KS, et al. Cancer cells dying from ferroptosis impede dendritic cell-mediated anti-tumor immunity. Nat Commun (2022) 13(1):3676. doi: 10.1038/s41467-022-31218-2
82. Oltean T, Lippens L, Lemeire K, De Tender C, Vuylsteke M, Denys H, et al. Association of cell death markers with tumor immune cell infiltrates after chemo-radiation in cervical cancer. Front Oncol (2022) 12:892813. doi: 10.3389/fonc.2022.892813
83. Escors D, Gato-Canas M, Zuazo M, Arasanz H, Garcia-Granda MJ, Vera R, et al. The intracellular signalosome of PD-L1 in cancer cells. Signal Transduct Target Ther (2018) 3:26. doi: 10.1038/s41392-018-0022-9
84. Kim R, Hashimoto A, Markosyan N, Tyurin VA, Tyurina YY, Kar G, et al. Ferroptosis of tumour neutrophils causes immune suppression in cancer. Nature (2022) 612(7939):338–46. doi: 10.1038/s41586-022-05443-0
85. Han JJ, Kang H, Li XS, Kwon N, Li HD, Park S, et al. Photo-fenozyme nanoparticles based on Fe(II)-Coordination-Driven cyanine-based amino acid assembly for photodynamic ferrotherapy. ACS Appl Nano Materials (2021) 4(6):5954–62. doi: 10.1021/acsanm.1c00843
86. Niu BY, Liao KX, Zhou YX, Wen T, Quan GL, Pan X, et al. Application of glutathione depletion in cancer therapy: enhanced ROS-based therapy, ferroptosis, and chemotherapy. Biomaterials (2021) 277:121110. doi: 10.1016/j.biomaterials.2021.121110
87. Zheng H, Jiang J, Xu S, Liu W, Xie Q, Cai X, et al. Nanoparticle-induced ferroptosis: detection methods, mechanisms and applications. Nanoscale (2021) 13(4):2266–85. doi: 10.1039/d0nr08478f
88. Shi ZY, Zheng JZ, Tang WB, Bai Y, Zhang L, Xuan ZD, et al. Multifunctional nanomaterials for ferroptotic cancer therapy. Front Chem (2022) 10:868630. doi: 10.3389/fchem.2022.868630
89. Zhang SC, Li ZQ, Xu ZM, Tang YX, Duan CC, Dai HY, et al. Reactive oxygen species-based nanotherapeutics for head and neck squamous cell carcinoma. Materials Design (2022) 223:111194. doi: 10.1016/j.matdes.2022.111194
90. Sun FW, Peng YY, Li YP, Xu MH, Cai T. Fenton-reaction-triggered metabolism of acetaminophen for enhanced cancer therapy. Chin Chem Lett (2023) 34(2):107507. doi: 10.1016/j.cclet.2022.05.021
91. Zhu DM, Ling RY, Chen H, Lyu M, Qian HS, Wu KL, et al. Biomimetic copper single-atom nanozyme system for self-enhanced nanocatalytic tumor therapy. Nano Res (2022) 15(8):7320–8. doi: 10.1007/s12274-022-4359-6
92. Liu D, Dai X, Zhang W, Zhu X, Zha Z, Qian H, et al. Liquid exfoliation of ultrasmall zirconium carbide nanodots as a noninflammatory photothermal agent in the treatment of glioma. Biomaterials (2023) 292:121917. doi: 10.1016/j.biomaterials.2022.121917
93. Yin M, Chen X, Guo Q, Xiao L, Gao P, Zang D, et al. Ultrasmall zirconium carbide nanodots for synergistic photothermal-radiotherapy of glioma. Nanoscale (2022) 14(40):14935–49. doi: 10.1039/d2nr04239h
94. Yang N, Gong F, Liu B, Hao Y, Chao Y, Lei H, et al. Magnesium galvanic cells produce hydrogen and modulate the tumor microenvironment to inhibit cancer growth. Nat Commun (2022) 13(1):2336. doi: 10.1038/s41467-022-29938-6
95. Wang XW, Zhong XY, Li JX, Liu Z, Cheng L. Inorganic nanomaterials with rapid clearance for biomedical applications. Chem Soc Rev (2021) 50(15):8669–742. doi: 10.1039/d0cs00461h
96. Chen Y, Li Y, Guo L, Hong J, Zhao W, Hu X, et al. Bibliometric analysis of the inflammasome and pyroptosis in brain. Front Pharmacol (2020) 11:626502. doi: 10.3389/fphar.2020.626502
Keywords: ferroptosis, immunotherapy, anti-tumor immunity, nanoparticles, gene signature, bibliometric analysis
Citation: Wang Z, Zhang H, Wang L, Ma Z, Cui Y, Fu H and Yu C (2023) Bibliometric analysis of ferroptosis: a comprehensive evaluation of its contribution to cancer immunity and immunotherapy. Front. Oncol. 13:1183405. doi: 10.3389/fonc.2023.1183405
Received: 10 March 2023; Accepted: 06 April 2023;
Published: 27 April 2023.
Edited by:
Huiyan Zeng, Beth Israel Deaconess Medical Center and Harvard Medical School, United StatesCopyright © 2023 Wang, Zhang, Wang, Ma, Cui, Fu and Yu. This is an open-access article distributed under the terms of the Creative Commons Attribution License (CC BY). The use, distribution or reproduction in other forums is permitted, provided the original author(s) and the copyright owner(s) are credited and that the original publication in this journal is cited, in accordance with accepted academic practice. No use, distribution or reproduction is permitted which does not comply with these terms.
*Correspondence: Chunjing Yu, eWNqd3hkMTk3OEBqaWFuZ25hbi5lZHUuY24=