- 1Institute of Pharmaceutical Research, GLA University, Mathura, India
- 2Department of Nursing, Al-Maarif University College, Ramadi, Anbar, Iraq
- 3Department of Physics, College of Education, University of Al-Qadisiyah, Al-Diwaniyah, Iraq
- 4Medical Laboratories Techniques Department, Al-Mustaqbal University College, Babylon, Hilla, Iraq
- 5National University of Science and Technology, Dhi Qar, Iraq
- 6Department of Public Health and Healthcare management, Samarkand State Medical University, Samarkand, Uzbekistan
- 7Department of Scientific Affairs, Tashkent State Dental Institute, Tashkent, Uzbekistan
- 8College of Pharmacy, Prince Sattam Bin Abdulaziz University, Alkharj, Saudi Arabia
- 9Azogues Campus Nursing Career, Health and Behavior Research Group (HBR), Psychometry and Ethology Laboratory, Catholic University of Cuenca, Cuenca, Ecuador
- 10Epidemiology and Biostatistics Research Group, CES University, Medellín, Colombia
- 11Educational Statistics Research Group (GIEE), National University of Education, Azogues, Ecuador
- 12Faculty of Nursing, Umm al-Qura University, Makkah, Saudi Arabia
- 13Department of Medical Physics and Radiology, Faculty of Paramedical Sciences, Kashan University of Medical Sciences, Kashan, Iran
Cancer is caused by defects in coding and non-coding RNAs. In addition, duplicated biological pathways diminish the efficacy of mono target cancer drugs. MicroRNAs (miRNAs) are short, endogenous, non-coding RNAs that regulate many target genes and play a crucial role in physiological processes such as cell division, differentiation, cell cycle, proliferation, and apoptosis, which are frequently disrupted in diseases such as cancer. MiR-766, one of the most adaptable and highly conserved microRNAs, is notably overexpressed in several diseases, including malignant tumors. Variations in miR-766 expression are linked to various pathological and physiological processes. Additionally, miR-766 promotes therapeutic resistance pathways in various types of tumors. Here, we present and discuss evidence implicating miR-766 in the development of cancer and treatment resistance. In addition, we discuss the potential applications of miR-766 as a therapeutic cancer target, diagnostic biomarker, and prognostic indicator. This may shed light on the development of novel therapeutic strategies for cancer therapy.
1 Introduction
The significance of post-transcriptional gene regulation in the development and spread of cancer has recently become much clearer. Although messenger RNA (mRNA) levels in cells are significantly influenced by transcriptional activation and repression, posttranscriptional processes ultimately decide whether or not mRNA will be translated into proteins. These would include microRNAs’ negative regulation and RNA-binding proteins’ control of RNA longevity (miRNAs) (1–8). Noncoding RNAs known as miRNAs are a conserved family that is named for their short size (18–22 nucleotides on average) (9–17). According to experts, miRNAs can control most (minimum of 2/3) of protein-coding genes (18). circular RNAs and Long non-coding RNAs are two examples of non-protein-coding transcripts that may be associated with miRNAs (19). They are found in almost all tissues (18). The RNA-induced silencing complex (RISC) is produced when specialised protein factors, including Argonaute proteins, bind to microRNAs to exert a negative regulation on certain target genes. The RISC detects target transcripts by a direct link between miRNA and mRNA molecules, often based on patterns in the 3’ untranslated regions (3’ UTR) of the mRNA. By encouraging mRNA instability, preventing translation, or directly cleaving mRNA, expression is suppressed (20). RNA polymerase II frequently transcripts miRNAs from distinct genomic components (21). But introns that are in protein-coding genes contain hairpin structures that can also produce miRNAs (22, 23).
Every single miRNA may simultaneously target dozens or even hundreds of genes seed sequence refers to the first eight nucleotides of the miRNA, which are essential for target identification (20). MiRNAs regulate gene expression, leading to dysregulated expression and abnormal activity (24–29). MiRNAs regulate a variety of biological processes, such as cell development, differentiation, proliferation, apoptosis, tumorigenesis, cancer progression, and signal transduction, based on the targeted genes, such as tumour inhibitor genes and oncogenes (30–35). Additionally, miRNAs could be therapeutic in addition to diagnostic biomarkers (36, 37). Ever since the finding of circulating miRNAs in 2008, >79 miRNAs have been identified as serum or plasma biomarkers of a variety of malignancies, such as gastric, lung, breast, esophageal, colon, melanoma, ovarian, and prostate cancer (38–45). One important miRNA, miR-766, is found in an intron that exists in the Septin-6 (SEP6) gene and promotes the course of cancer (46). MiR-766 expression is linked to carcinogenesis, malignant behavior, and tumor suppressive and/or oncogene genes (47–53). In addition to hematological malignancies, miR-766 is implicated in several types of cancers, such as colon, colorectal, papillary thyroid, prostate, cervical, breast, osteosarcoma, lung, and gastric cancer. MiR-766 is an oncogene or tumour-inhibiting gene, and its function in the genesis of malignancies is discussed.
2 MicroRNA biogenesis
RNA polymerase II transcribes microRNAs to create a double-stranded hairpin primary transcript. Pre-miRNA is broken down by Drosha and converted into mature miRNA duplex strands by RNase III endonuclease Dicer (54–57). Strands are oriented in either 5’ or 3’ orientation. Argonaute can be loaded with either RNA strand to form RISC, with the ratio of -5p to -3p dependent on thermodynamic endurance (58). But, depending on the kind of cell, the ratio of -5p to -3p may favor one strand to the other or be equal. RISC can be tailored to different gene targets depending on the number or presence of different strands (59, 60). When miRNAs attach to their mRNA targets’ 3’-UTRs by complementary base pairing, they subsequently regulate gene expression by either targeting their destruction or repressing translation. Up to sixty percent of the human genome’s genes are controlled by microRNAs, which have been discovered to impact a number of cellular processes and the onset of illness (61–68). Additionally, miRNAs and pre-miRNAs are highly firm in the extracellular environment and can be produced from the cell through into bloodstream. Because they are carried inside exosomes, circulating microvesicles, protein complexes, or high-density lipoproteins, they may be taken up by cells in tissues through cell-to-cell communication (69). They are becoming clinical biomarkers and therapeutic targets for individualised medicine in sophisticated diseases, and they continue to stimulate a wide range of research (24, 25, 29, 70, 71).
3 miRNA acts as OncomiR or tumor suppressor
MicroRNAs can inhibit or promote the cancer phenotype by inhibiting the production of oncogenes or tumour suppressors (25, 72–77). Typically, tumor-suppressive miRNAs are expressed less than it should while oncogenic miRNAs (oncomiRs) are expressed more than enough in malignancies (71, 78–85). Based on the kind of cancer and the particular miRNA that is impacted, cancer progression, metastasis, and/or survival could be drastically decreased whenever these oncomiRs or miRNAs suppress tumors are silenced or activated, respectively. It is also conceivable for malignancies to become entirely dependent on, or “addicted” to, an oncomiR, in which case suppressing the oncomiR causes the tumour to fully regress (86). Therefore, miRNAs may be classed as either tumor-promoting or tumor-suppressing, and the regulation of their synthesis for therapeutic purposes is a fiercely disputed subject (87–91).
Yet, there are arguments in favor of caution in the therapeutic approaches. There are a lot of contradictory reports in the literature about whether or not particular miRNAs are tumor-suppressing or carcinogenic. MiRNAs can be both tumor suppressive and carcinogenic. For example, miR-766 can act as both a tumor suppressor (Figure 1) and oncomiR in osteosarcoma (51, 92). Given the vast array of genes that a certain miRNA affects, the variety of consequences is not surprising. It implies that any designation of a miRNA as oncogenic or tumor suppressive must be carefully examined because it may constitute an oversimplification (93).
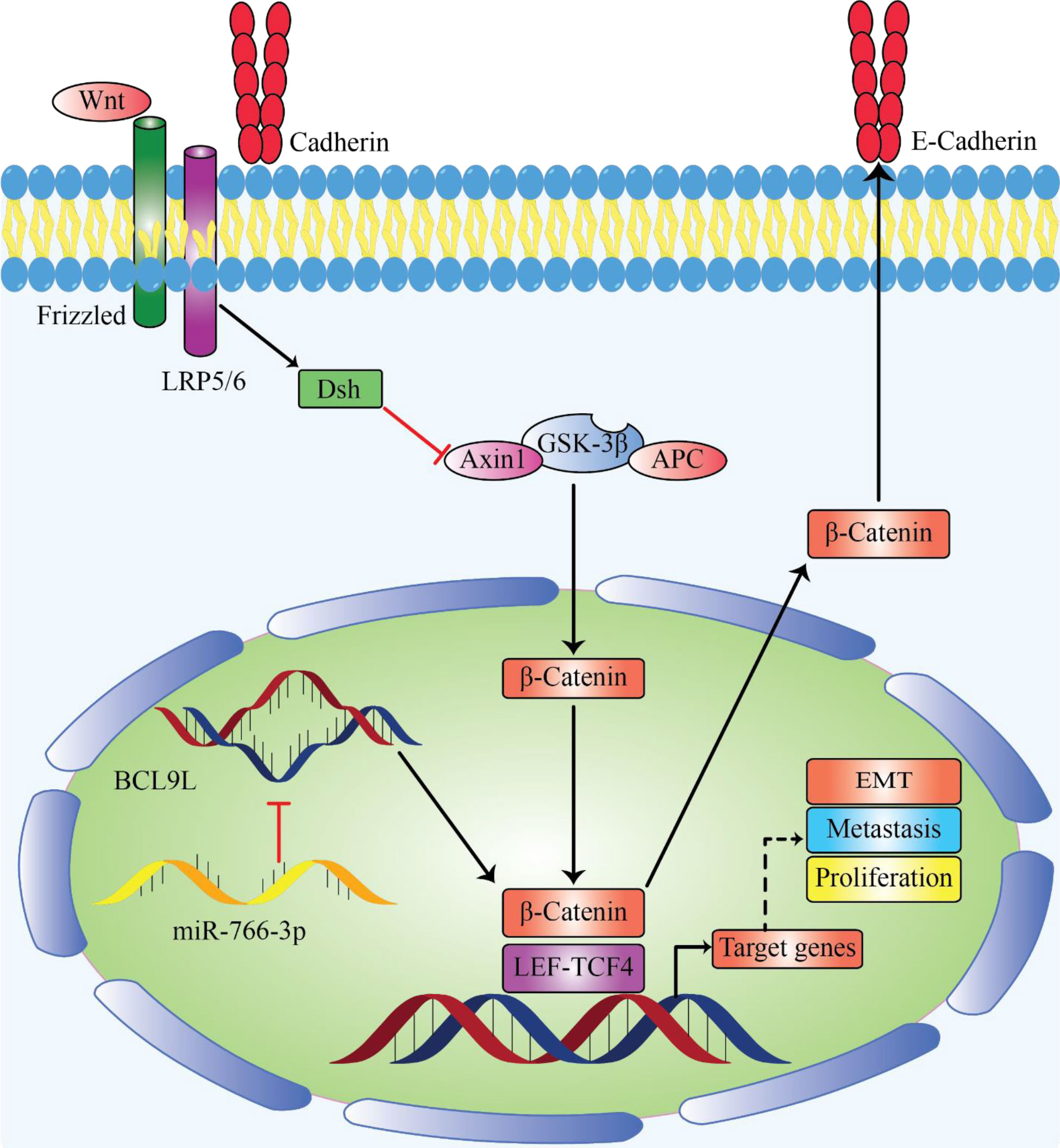
Figure 1 MiR-766 can be sererved as tumor suppressor miRNA by decreasing cancer development. Enforced expression of miR-766-3p lead to suppression of metastasis by inhibiting EMT, migration and invasion in osteosarcoma by regulating the β-Catenin/TCF-4 signal pathway and targeting BCL9L (92).
4 miR-766
4.1 miR-766 as tumor suppressor miRNA in cancer cells
4.1.1 Colorectal cancer
Colorectal cancer (CRC) is the third most prevalent malignancy and the third leading cause of cancer-related death worldwide (94, 95). CRC affects 4.4% of persons in affluent nations and 1.4% of people in underdeveloped nations (96). Despite improvements in care over the past few decades, almost 50% of CRC patients pass away within 5 years. Surgical excision causes a high mortality rate due to tumour reappearance and metastasis (97, 98). Therefore, continued research further into underlying molecular pathways of CRC is very important from a therapeutic standpoint.
A group of transcription factors known as nuclear receptors (NRs) controls homeostasis, metabolism and differentiation by spatiotemporally regulating gene expression. Humans only have 48 NRs that have been found, 25 of them are orphan NRs that have unknown endogenous biological roles and ligands (99, 100). Numerous studies have demonstrated the tight connection between orphan NRs and specific forms of cancer (101). The orphan nuclear receptor hepatocyte nuclear factor 4 gamma (HNF4G) is substantially elevated in CRC tissues (102). HNF4 function is still not entirely understood, yet. Although, dysregulation of miR-766-3p level in CRC has been documented (Table 1), which suggests it may be crucial in CRC pathogenesis.
Recently, He et al. (111) HNF4G expression is noticeably elevated in CRC tissues. In addition, there was a definite association between a high degree of tumor-node metastasis and a poor prognosis for CRC, as well as an increased expression of HNF4G. Additionally, HNF4G overexpression strongly induced CRC cell proliferation in vitro. In addition, they identified that HNF4G targets G protein gamma 12 (GNG12) and protein tyrosine kinase 2 to activate the phosphatidylinositol 3-kinase/protein kinase B (PI3K/AKT) pathway and enhance cell proliferation in colorectal cancer (CRC) (PTK2). MiR-766-3p expression was significantly downregulated and elevated in CRC. Both of these changes were associated with miR-766-3p ability’s to target HNF4G in vivo and in vitro and prevent CRC cell proliferation. Collectively, their findings demonstrated that miR-766-3p suppresses the phosphatidylinositol 3−kinase (PI3K)/protein kinase B (AKT) pathway by targeting the expression of HNF4G, which in turn limits the growth of CRC cells (111). As a result, CRC treatment may benefit from the development of medicines that specifically aim at the miR-766-3p/HNF4G axis (Figure 2).
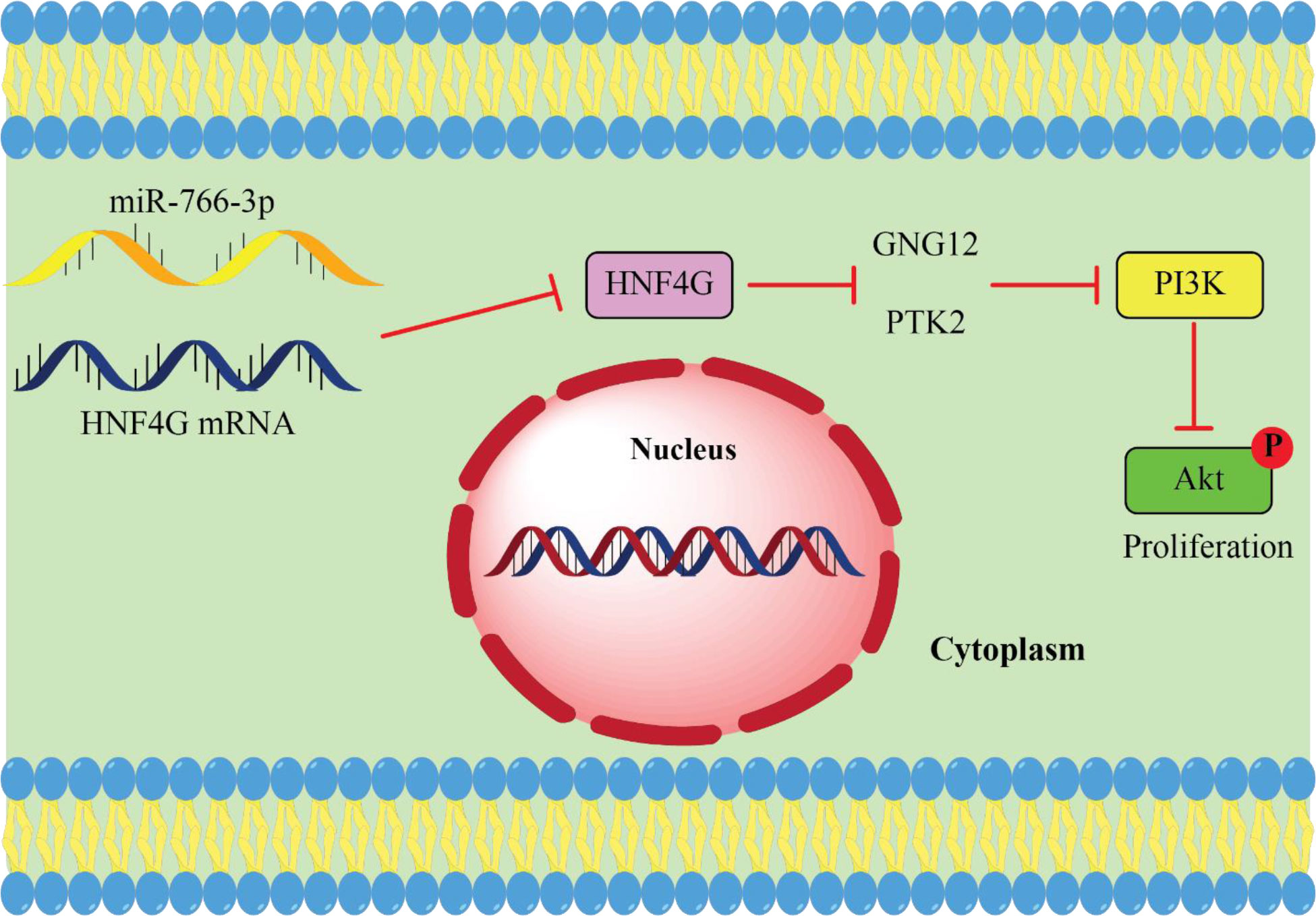
Figure 2 miR-766-3p acts as tumor suppressor miRNA in CRC. upregulated HNF4G leads to cancer cell proliferation by activating the PI3K/AKT pathway in return miR-766 suppresses CRC cell proliferation by targeting HNF4G and inhibits PI3K/AKT pathway (111).
4.1.2 Colon cancer
With more than 1,800,000 cases reported and more than 881,000 deaths in 2018, colon cancer ranks third among the most common malignancies globally, accounting for about 10 percent of all cancer cases and deaths (128). Due to the fact that patients are often identified at a late stage, the recovery rate is poor. Despite ongoing advancements in colon cancer diagnosis and treatment methods, approximately 50% of patients still experience metastasis and recurrence (129, 130). Therefore, understanding the process of colon cancer cells is essential for creating novel tools for both diagnosis and treatment.
Transforming growth factor beta induced (TGFBI) takes involved in some of physiological processes, for example growth of the tumor, differentiation, and metastasis (131). The necessity of TGFBI in the development of cancer has been approved by numerous researchs. TGFBI is associated with a bad prognosis for patients and promotes the proliferation of oral squamous cell carcinoma cells. Overexpression of TGFBI promotes glioma cell proliferation and migration (132, 133). TGFBI may act as a pro- or anti-oncogenic molecule in ovarian cancer (134). Colon cancer tissues had higher levels of TGFBI mRNA, which is linked to extravasation and invasiveness (135, 136). For this reason, Gao and colleagues (53) analyzed how TGFBI affects colon cancer cells and whether miRNAs target the TGFBI gene. Using in vitro and bioinformatics experiments, it was determined that miR-766-3p can specifically aim TGFBI and that, in colon cancer, its level was downregulated while TGFBI was elevated. Additionally, they discovered that expression of miR-766-3p is forcefully suppressed cancerous cell activities and caused cell apoptosis in colon cancer. Overexpression of miR-766-3p inhibits cancer growth by targeting TGFBI (53). According to another research, it is intriguing to note that miR-766 may function as a microRNA that inhibits tumour growth in colon cancer cells. MiR-766 promotes the death of colon cancer cells via altering the p53/Bax signalling pathway (137). Consequently, miR-766 could be the new therapeutic aim for colon cancer.
Several tumor suppressor genes’ promoters are methylated, which directly inhibits transcription factor binding and halts gene expression (138). Elevated DNMT3B enzyme expression leads to aberrant de nevo methylation and CpG island methylation (CIMP) in colon cancer (139). MiR-339 and miR-766 target DNA Methyltransferase 3 Beta (DNMT3B) to alter methylation pattern in tumour suppressor genes (109). Ectopic production of miR-339 and specifically miR-766 has been shown to limit the growth of colon cancer by reducing the methylation that is in the tumor suppressor genes like Dickkopf-related protein 2 (DKK2), WNT inhibitory factor 1 (WIF1), secreted frizzled-related protein 1 (SFRP1), and SFRP2 (109).
4.1.3 Breast cancer
Breast cancer is the most common type of cancer in women, leading to cancer-related death (128, 140). Individual particular targeted therapy has become more popular in therapeutic settings. Therefore, it is believed that identifying viable prognostic biomarkers and attractive targets is a necessary first step in achieving this goal. A tumor suppressor gene called P53 that frequently develops mutations in several different types of malignancies, for instance breast cancer. Changes in the gene impact the expression of several genes that are either directly or indirectly regulated by p53 (141) One of p53’s key roles is cell cycle regulation. Cell cycle arrest is predominantly caused by p53’s transactivation of its downstream targets, such as Stratifin (SFN), growth arrest and DNA damage-inducible alpha (GADD45A), and G2 and S phase-expressed-1 (GTSE1), at the G1/S and G2/M checkpoints (142). Previous research has shown that microRNAs have a function in p53-mediated control of the cell cycle. More and more microRNAs are being discovered as actors in the post-transcriptional regulation of p53 and the cell cycle regulation associated with p53 (143). Recently, Wang et al. (52), revealed that miR-766 expression levels were abnormally high in breast cancer and that miR-766 overexpression led to an increase of wild-type kind p53 protein in a number of cancer cell lines. In addition, miR-766 inhibits proliferation and engraftment in a variety of cancer cell lines and induces G2 cell cycle arrest, demonstrating its role in the p53 signalling pathway. Additionally, they discovered that the oncogene and negative regulator of p53 context-dependent roles of MDMX (MDM4) is specifically targeted by miR-766, which promotes p53 signaling (52). Overall, their research showed that miR-766 slows the spread of breast cancer through increasing p53 signaling by targeting MDM4.
Breast cancer that returns after therapy is referred to as a recurrence. Thirty-three percent of all patients with breast cancer will experience a distant metastatic relapse even after the initial disease remission. The primary factor in breast cancer patients’ deaths and a condition that is largely incurable is breast cancer metastasis (144, 145). Complex processes that makeup cancer metastasis is typically beyond the reach of a single molecular target’s intervention. A microRNA that regulates metastasis may be a promising option for a disease target since it may impact the aggressiveness of breast cancer cells by concurrently modulating many pathway effectors (146). By comparing a metastasis model with a patient-derived xenograft primary tumour model, Oh et al. (112) were able to evaluate the functional potential of a recently reported human metastasis-related miRNA, miR-766, which had been found before. In the orthotopic xenograft model, triple-negative breast cancer cells overexpressing miR-766 developed the primary tumour at the same pace as vector-transfected control cells. In contrast to control cancer cells, breast cancer cells that overexpressed miR-766 significantly inhibited the formation of tumour spheres and Matrigel invasion. In addition, compared to control cells, lung metastasis was significantly decreased in breast cancer cells overexpressing miR-766. They discovered that miR-766 upregulation dramatically lowers lung metastases in breast cancer (112). Therefore, miR-766 had a greater effect on distant metastasis than on cancer genesis and early tumour growth, and it may be a future therapeutic target for the effective treatment of lethal breast cancer metastasis.
4.1.4 Renal cell carcinoma
Renal cell carcinoma (RCC), which accounts for 2% to 3% of adult malignancies, is the most lethal form of genitourinary cancer in humans (147). In around 30% of RCC patients, metastatic lesions are present at the time of first diagnosis (148–150). Deaths and morbidity from RCC have continued to grow in recent years. Surgical excision is still the only curative option for RCC because it is resistant to radiation, chemotherapy, and immunotherapy (151, 152). Although tyrosine kinase inhibitor (TKI)-based antiangiogenic therapy is the standard treatment for advanced or metastatic RCC, its efficacy is restricted owing to drug resistance (153, 154). Therefore, it is becoming more important to enhance novel RCC diagnostic and treatment procedures. Numerous miRNAs have been implicated in anti-tumor development in RCC patients, according to research done to date (155, 156). There is growing evidence that miR-766 either promotes or inhibits tumor growth in different group types of human malignancies. (Table 1). It’s intriguing to note that miR-766-3p is the miRNA that is downregulated the most in RCC, according to a genome-wide microRNA expression study (157). MiR-766-role 3p’s in RCC remains unknown. Alternate splicing regulators have acted as new participants in tumour regulating the activities of several tumour suppressors or oncogenes during the course of evolution (158, 159). The serine/arginine-rich (SR) proteins are crucial for alternative splicing regulation. Canonical component of the SR proteins, splicing factor SF2 is also known as SRSF1 (160, 161). If we observe SF2, it has bad prognosis and upregulation in a number of human malignancies (162, 163), It is still mostly unknown how SF2 and miRNAs interact. According to studies, RCC tissues had higher levels of methylation in the miR-766-3p promoter than non-tumorous tissues (116). In addition, it is evident that a decreased miR-766-3p level is associated with a poorer prognosis and clinical stage in RCC patients. A high level of SF2, a new oncoprotein in RCC, is highly associated with a poor prognosis in a large cohort of RCC specimens, and SF2 may stimulate the proliferation of RCC cells by boosting the expression of P-AKT and phosphorylated ERK (P-ERK). According to experimental findings, miR-766-3p may be specifically aiming SF2 in RCC cells (116). In addition, the expression of miR-766-3p ectopic inhibits cell division via targeting SF2 expression and other SF2/P-AKT/P-ERK signalling pathways. By modulating SF2/P-AKT/P-ERK signalling, miR-766-3p acted as an inhibitory noncoding RNA to restrict the progression of RCC (116). By focusing on this signaling pathway and miRNA, we may be able to better control the course of RCC and open a treatment path for additional RCC progression.
4.1.5 Pancreatic cancer
Pancreatic cancer is one of the most lethal malignant tumours in the world. The most prevalent type of pancreatic cancer, is pancreatic ductal adenocarcinoma (PDAC), accounting for around 96% of all cases (164). The therapeutic results of PDAC patients remain poor despite the significant advancements achieved in therapeutic methods, such as surgery and radiochemotherapy (165). The lack of an effective early detection approach and the aggressive characteristics of PDAC, such as fast development and early metastasis, are mostly to blame for the poor prognosis of these individuals (166). PDAC patients generally have five-year survival rates of 5%, whereas those of patients who undergo surgical excision range from 10% to 25% (167, 168). Understanding PDAC processes is essential for developing novel biomarkers and therapeutic methods. According to accumulating evidence, several miRNAs are altered in PDAC, and these modifications have a significant effect on the malignant behavior of PDAC (169, 170). As a result, miRNAs are prospective therapeutic targets and diagnostic indicators for PDAC patients, and the relationship among miRNAs and PDAC has to be clarified. Recently, Li et al. (121) evaluated miR-766 expression in PDAC cell lines and tissues and looked into the mechanisms behind its functions in PDAC. They discovered that PDAC cell lines and tissues had considerably lower levels of miR-766. Additionally, PDAC cell invasion and proliferation were constrained by increased miR-766. Additionally, it was discovered that miR766 directly targeted E26 transformation specific 1 (ETS1) in PDAC cells. In addition, ETS1 overexpression negated the beneficial effects of miR-766 restoration on PDAC cells, while ETS1 downregulation mimicked the inhibitory impact of miR-766 overexpression on PDAC cells (121). MiR-766 has the potential to be a therapeutic target for PDAC patients. More studies are shown in Table 1.
4.2 miR-766 acts as oncomiRNA in cancer cells
According to several studies, miR-766 functioned as an oncomir that promoted cell migration and proliferation while inhibiting apoptosis by suppressing a number of targets. It was also found to be elevated in a number of malignancies (Table 1). MiR-766 was identified as an oncomir in the tissues of CRC patients with chronic myeloid, cutaneous squamous cell carcinoma (CSCC), leukemia, hepatocellular carcinoma (HCC), acute promyelocytic leukemia (APL), osteosarcoma, cervical cancer, breast cancer, and gastric cancer, as will be discussed further on.
4.2.1 Cutaneous squamous cell carcinoma
Cutaneous squamous cell carcinoma is the second-most common kind of skin cancer. Despite tremendous advances in treatment approaches, the 5-year survival rate for cscc patients is insufficient due to the aggressiveness of the tumour and distant metastases (171). Consequently, it is essential to identify likely molecular pathways of CSCC development and to investigate potential therapeutic targets. PDCD5 is essential for cell proliferation, apoptosis, and metastasis (172, 173). On the other hand, miR-766 has been found to be significantly expressed in the CSCC patient’s cells and might be crucial to the disease’s molecular pathogenesis, according to a microarray analysis. On the CSCC, the precise regulatory impact of miR-766 is still unknown. Recently, Liu et al. (104), suggested that miR-766 might function in CSCC cells as an oncomiRNA. They discovered that in CSCC cells and tissues, miR-766 expression was elevated whereas PDCD5 expression was downregulated. MiR-766 sped up A431 and SCL-1 cells’ motility, invasion, and proliferation, while also boosting MMP-2 and MMP-9. As a result, miR-766 can increase proliferation migration and invasion, of CSCC cells by reducing the level of PDCD5 expression (104). Additionally, through increasing HOXA9, miR766-3p downregulation inhibited CSCC cell growth, metastasis, and glycolysis (174).
4.2.2 Hepatocellular carcinoma
Hepatocellular carcinoma (HCC) is a leading cause of cancer mortality worldwide, with improved diagnosis and therapy. However, a substantial proportion of HCC patients are diagnosed at an advanced stage of the disease, and HCC recurrence or metastasis is still prevalent after liver resection (175). Therefore, a study on the abnormal expression and biological roles of miRNAs in HCC might lead to the creation of novel therapeutic targets and diagnostic biomarkers. Numerous research has examined the connections between miRNAs and the development of HCC illness in recent years (24, 176). However, only a few miRNAs were investigated in these studies, and it is necessary to evaluate the role of other miRNAs in the incidence of HCC. As was previously reported, miR-766 was linked to cell metastasis, proliferation, and death by targeting many down regulatory signaling pathways and genes in HCC cells (Table 1). However, more study is required to determine the molecular mechanisms and functions and of miR-766 in the development of HCC. Recently, Yang et al. (50) miR-766’s expression, functions, and processes in the in vivo and in vitro carcinogenesis of HCC were examined. They observed that miR-766 was higher in HCC tissues and that an increase in miR-766 directly reduces the expression of nuclear receptor subfamily 3 group c member 2 (NR3C2), hence promoting the spread and proliferation of HCC cells. In addition, they demonstrated that NR3C2 is one of the key targets of miR-766 and that it contributes to HCC carcinogenesis via altering the -catenin signalling pathway. This work established the importance of the miR766-NR3C2-catenin signalling pathway in the development of HCC and indicated that miR766 may play a role in the clinical treatment and diagnosis of HCC patients.
4.2.3 Cervical cancer
Cervical cancer (CC) is a disease that affects the cervix and can lead to cancer (32, 96). The previous study predicted that CC annually results in about 509,590 new cases and 311,365 fatalities (128). Surgery and chemotherapy are effective for early-stage CC, but some patients experience metastasis and recurrence (177). An innovative method for treating CC and investigating its control mechanism (31, 178, 179).
A brand-new protein that is highly conserved, called SCAI (suppressor of cancer cell invasion) mediates metastasis by acting as a 3-dimensional matrix (180). According to research, SCAI serves as a target DNA for a number of miRNAs in human malignancies (181, 182). In many different types of cancer, SCAI is down-regulated and functions as an anti-tumor gene (183, 184). Cai et al. studied the miR-766-5p expression and its impact on CC initiation and progression (107). The research revealed that the expression levels of miR-766-5p and SCAI were significantly upregulated and downregulated, respectively, in CC tissues and cell lines. The receiver operating characteristic (ROC) curve suggests that miR-5p may be a biomarker for the diagnosis of CC, as it inhibits migration, proliferation, and invasion of CC cells while inducing apoptosis. By inhibiting SCAI, the inhibitory effects on the migration, invasion, and proliferation of CC cells in vitro were eliminated, and the inhibitory impact of SCAI on tumour xenograft growth in vivo was also eliminated (107).
5 Interactions among lncRNA/circRNA, with miR-766 in cancer
Noncoding RNAs regulate invasion, proliferation, apoptosis, and metastasis in cancer cells (185–187). Noncoding RNAs regulate the development of cancer in several ways. lncRNAs and miRNAs, for instance, alter target gene expression (mRNA), which is crucial for the biological activity of cancer cells (188). As opposing endogenous RNAs, lncRNAs and circRNAs have the binding affinity of miRNAs and interact with them, hence enhancing the translation of miRNA-targeted genes. MiRNAs, for example, inhibit the translation of their target genes by mRNA degradation or inhibition of mRNA translation. The biological basis of tumors is connected to the ceRNA regulatory network (189, 190). Additionally, circRNAs and lncRNAs can interact directly with proteins to influence gene transcription and so take part in regulation (191). The circRNA-lncRNA-miRNA-gene modification network in the apoptosis pathway of cancer has been the subject of several studies, which we sum up in this section. This research provides new insights into the pathogenic processes of cancer as well as novel views on the design of less invasive early detection and treatment methods.
5.1 CircularRNA and miR-766
CircRNAs are single-stranded, closed circular RNAs with poly (A) tails and 5′-3′ ends (192). Salzman et al. (193) More than 1/10 of genes that are have the potential to create circRNAs, according to research that quantified the quantity of circRNA types in both healthy and mammalian cells. Circular transcripts of CDR1 as act as miRNA sponges for miR-7 (194, 195). These studies made circRNAs the focus of scholarly investigation and the next big thing in the noncoding RNA area.
Nearly 184,615 new instances of laryngeal cancer were identified worldwide in 2018, and 99,840 people will pass away from the condition in 2020 (196). Laryngeal squamous cell carcinoma (LSCC), a prevalent kind of laryngeal cancer, imposes a substantial cost on individuals and the healthcare system (197). Similar to other cancers, the progression of LSCC is a complicated process including alterations to the metabolism, genes, and pathways (198, 199). However, the pathogenesis of LSCC hasn’t been precisely outlined. Consequently, it is essential to elucidate the molecular mechanism of LSCC. CircRNAs have crucial roles in the development of several malignancies, including LSCC (200) and circRNA-associated-ceRNA networks have discovered to be involved in LSCC development. CircRNA-associated-ceRNA networks have discovered to be involved in LSCC development (201, 202).
Recently, Chen et al. (120) LSCC progression was looked at to see if the circularRNA-associated ceRNA network involving circSHKBP1 and miR-766-5p was involved. They claimed that LSCC cell lines and specimens and showed up- and down-regulation of the genes circSHKBP1 and miR-766-5p, respectively. The expression of circSHKBP1 was associated with poor prognosis and clinical markers in LSCC, but decreased miR-766-5p expression improved the overall survival rate and could positively influence the high mobility group A2 (HMGA2). Importantly, circSHKBP1’s si-effects on the development of LSCC were abolished by HMGA2 overexpression. These findings revealed that circSHKBP1, which targets miR-766-5p, promoted LSCC carcinogenesis by increasing HMGA2 levels (Figure 3) (120).
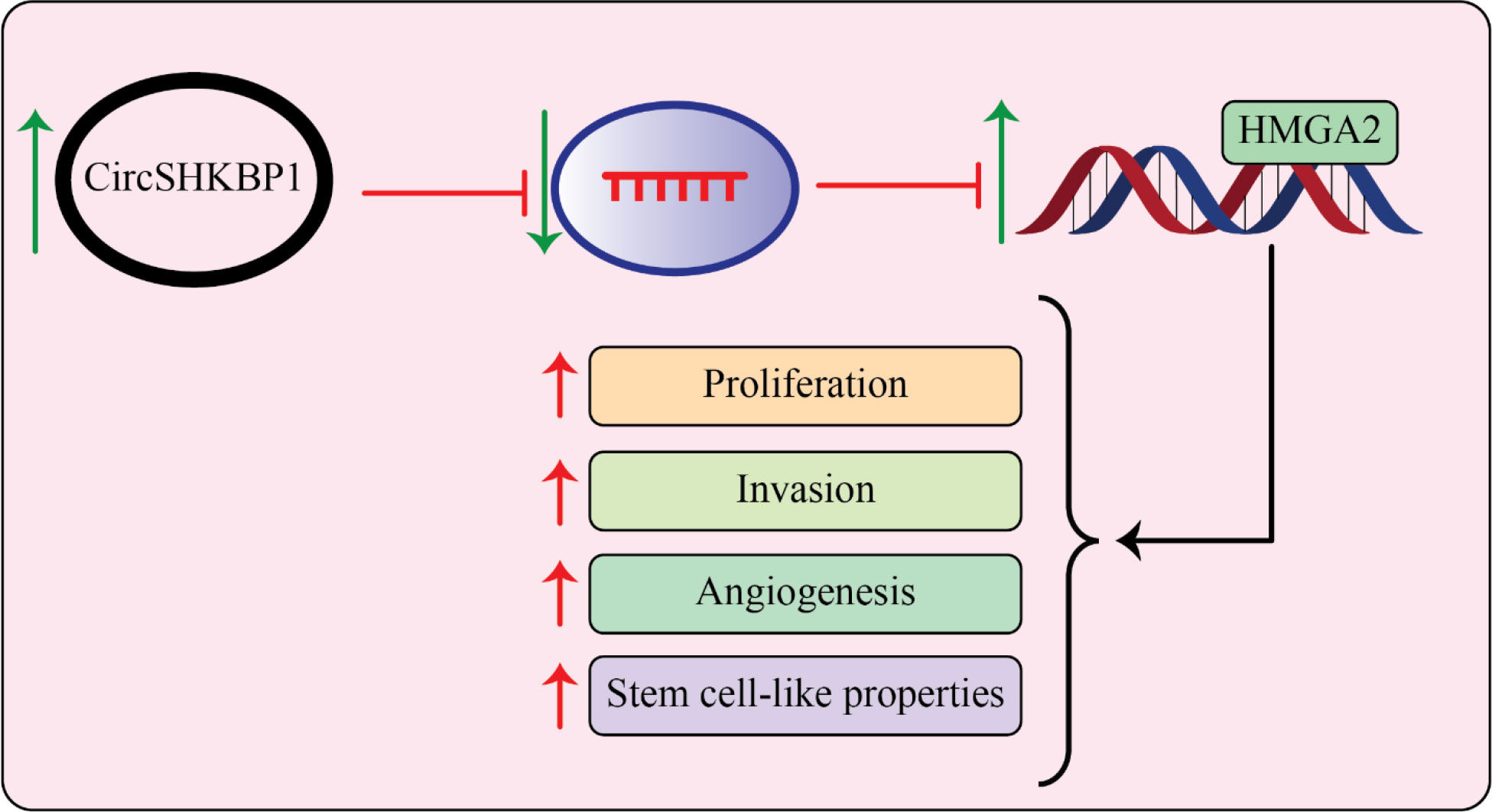
Figure 3 circSHKBP1 induces LSCC progression by upregulating HMGA2 levels by inhibiting miR-766-5p. The expression level of circSHKBP1 and miR-766-5p is upregulated and downregulated, respectively, in LSCC specimens and cell lines. High mobility group AT-hook 2 (HMGA2) promotes cancer development and miR-766-5p as tumor suppressor miRNA inhibits tumorigenesis of LSCC by targeting HMGA2. On another side, circSHKBP1 induces tumor development of LSCC by overexpression of HMGA2 through sponging miR-766-5p (120).
Radiation therapy is a common and vigorous treatment for glioma, although its effectiveness is significantly hampered by the glioma cells’ innate and acquired resistance (203). Extracellular vesicles (EVs) contain circulating RNAs that are directly associated to the radioresistance of cancer cells (30, 74, 204, 205). In a study by Zhao et al. (206), to create radioresistant glioma U215 cells, glioma U251 cells were subjected to serial 5 Gy radiation treatments with a 60Co source (RR-U215). Following the separation of EVs as from RR-U125 cell culture media, an RT-qPCR study revealed that ciR-ATP8B4 expression was considerably higher in EVs than in non-EVs. The circular ATP8B4/miR-766-5p axis in RR-EVs may have a role in glioma radioresistance (206). Exosome-derived molecule makes HCT116 CRC cells more sensitive to chemotherapy drugs (207). MiR-766-5p is a sponge used for circ 0094343 and TRIM67 (tripartite motif-containing 67). It functions as an oncomiR in CRC tissues and has a negative correlation with TRIM67 expression. circ-0094343 enhances the chemosensitivity of CRC cells and inhibits their proliferation and glycolysis (207). Therefore, the circ 0094343/miR-766-5p/TRIM67 axis could provide novel insights into CRC treatment.
Cells’ stress response, known as autophagy, can shield them from harm (71). Incorrect autophagy has been seen in several diseases, including cancers and viral infections (71, 208). Moderate autophagy promotes invasion and development of pancreatic cancer cells, while excessive autophagy damages organelles and degrades pro-oncogenes, leading to apoptosis (209). Autophagy related 7 (ATG7) activation inhibits autophagy, reducing pancreatic cancer cell proliferation and metastasis (210, 211). Despite this, it is unknown how ATG7 governs pancreatic cancer.
Autophagy related 7 activation inhibits autophagy, reducing pancreatic cancer cell proliferation and metastasis (123). CircATG7 overexpression increased pancreatic cancer cell motility, proliferation, and autophagy, while ATG7 inhibition diminished its effects. MiR-766-5p works as a tumour suppressor to counter the effects of circATG7 (123). ATG7, a miR-766-5p target gene, was expressed greater, while circATG7 sponging miR-766-5p decreased its expression. As a conclusion, circATG7 speeds up the development of pancreatic cancer via miR-766-5p/ATG7 (123). circATG7 could therefore be a possible therapeutic approach for PC.
5.2 LncRNA and miR-766
Long non-coding RNAs play a significant role in the start, development, and metastasis of malignancies, and are emerging as potential biomarkers for cancer patients (70, 212). Additionally, the variety of cancers may have interesting therapeutic targets in lncRNAs and also the pathways which they affect. LncRNA nucleus enriched abundant transcript 1 (NEAT1), a crucial structural component of the subnuclear structure paraspeckle, has a close association with both innate immunity and malignant malignancies (213, 214). NEAT1 is involved in lymphoblastic, lymphoid, promyelocytic, and chronic myeloid leukemia (CML) cancers (213, 215, 216). However, its role in CML is not known. A large number of immature white blood cells accumulate in the bone marrow owing to CML, a malignant clonal proliferative disease that impairs the normal hematopoiesis of the bone marrow (217). Clinically, tyrosine kinase inhibitors (TKIs) are utilized to treat CML, despite their poor efficacy in patients with relapsed CML (218). Therefore, it is crucial to find new treatment targets for CML. In CML cell lines and PBMCs of CML patients, there is a considerable downregulation in the expression level of the lncRNA-NEAT1 (105). In addition, ectopic expression of NEAT1 reduces cell viability and enhances apoptosis in CML cells. In addition, NEAT1 overexpression decreased miR-766-5p expression in K562 and KCL22 cells, but NEAT1 knockdown increased it. MiR-766-5p levels are significantly elevated in CML patients. In addition, miR-766-5p reverses the effects of NEAT1 on CML cell growth and death, functioning as a tumour suppressor in these cells. Additionally, CDKN1A was identified as miR-766-target 5p’s gene and was inhibited in CML PBMCs. Overall, lncRNA-NEAT1 suppresses the growth of CML cells and stimulates apoptosis through sponging miR-766-5p. Upregulated miR-766-5p in response counteracted NEAT1’s impacts on apoptosis and survival rate in CML cells (105). A distinct finding was reported by Zhao et al. in a different study they conducted on the impact of the lncRNA-NEAT1/miRNA-766-5p axis in prostate cancer (PCa) cells (121). According to their findings, overexpressed miR-766-5p inhibits PCa cells’ ability to invade, migrate, and proliferate via aiming E2F transcription factor 3 (E2F3). In exchange, lncRNA-NEAT1 encourages the growth of PCa by sponging miR-766-5p (122). More research is therefore required on the NEAT1/miRNA-766-5p axis function in various malignancies.
Lung adenocarcinoma (LUAD), a subtype of lung cancer, has been recognised as a malignant lung tumour (147, 219). Numerous studies have shown that the development of LUAD includes a variety of complex biological processes, including numerous epigenetic and genetic alterations (220, 221). Despite substantial efforts in LUAD treatment, including chemotherapy, radiation, surgery, and molecular targeted therapy, the prognosis for individuals with severe LUAD remains bleak (222, 223). lncRNAs are associated with a number of biological processes in LUAD, including cell, invasion, metastasis, and proliferation, according to many investigations. LncRNA cancer susceptibility candidate 9.5 (CASC9.5), for example, stimulates the development and metastasis of LUAD cells (224). A new lncRNA called PRKCZ antisense RNA 1 (PRKCZ-AS1) has not been investigated in the growth of cancer. Wang et al. recently looked into the function of PRKCZ-AS1 in LUAD cells. In this work, it was observed that PRKCZ-AS1 expression was significantly upregulated in LUAD cell lines and tissues. Moreover, the inhibition of PRKCZ-AS1 reduced the migration and proliferation of LUAD cells while increasing death. Additionally, the expression of PRKCZ-AS1 in LUAD cells had a negative effect on the expression of miR-766-5p. In addition, overexpression of miR-766-5p in LUAD cells decreased cell migration and proliferation by targeting MAPK1 (119). In other words, PRKCZ-AS1 as onco-lncRNA promotes the development of LUAD by sponging miR-766-5p to boost MAPK1 expression, which improves the prospect of using PRKCZ-AS1 as a biomarker and therapeutic targets in LUAD research.
Recently, it has been observed that LINC01503 as an onco-lncRNA lead to inducing resistance of ovarian carcinoma to carboplatin by overexpressing PD-L1 through sponging miR-766-5p (87), which which can be used as potential target to overcome chemotherapeutic barriers in ovarian cancer hterapy (Figure 4).
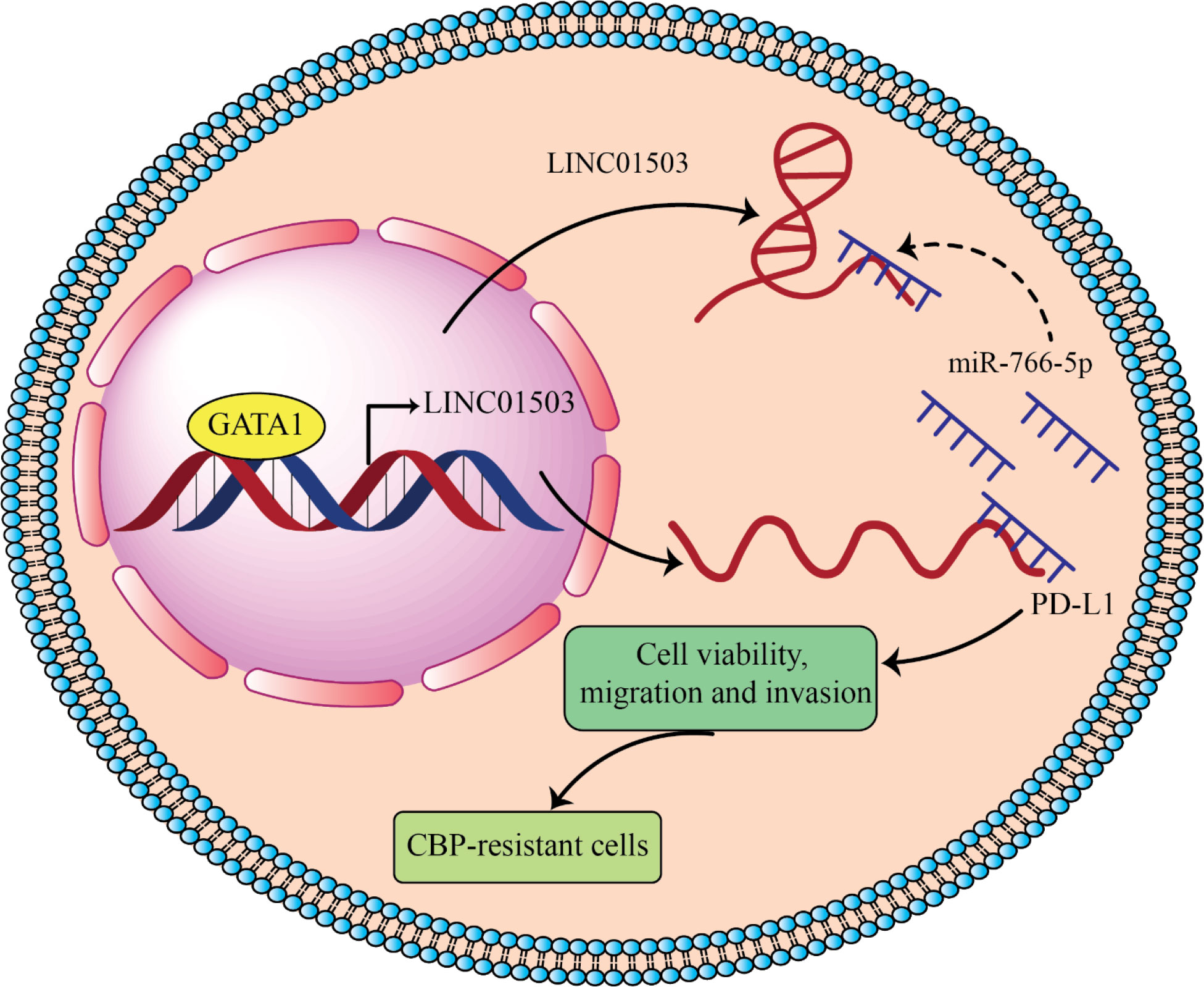
Figure 4 LINC01503 acts as onco-lncRNA in ovarian cancer and can induces cancer development by overexpressing PD-L1 through inhibiting miR-766 (87). LINC01503 and miR-766-5p expression levels significantly increase and decrease, respectively, in carboplatin (CBP) resistant ovarian carcinoma. Downregulation of LINC01503 decreases CBP resistance of ovarian carcinoma and in return overexpression of miR-766-5p increased CBP sensitivity by targeting PD-L1. GATA-binding protein 1 (GATA1) upregulated LINC01503 level and this lncRNA by sponging miR-766-5p leads to PD-L1 overexpression and promotes CBP resistance in OCa cells.
6 MicroRNA Therapeutics in Cancer and limitation of miR-766
The miRNA replacement-based therapeutic strategy is directed at pathological cells that decrease the expression of onco-suppressor miRNAs that play a function in controlling the expression of mRNAs that encode crucial oncoproteins, using the development of anticancer treatments as a sample field of inquiry (225). It is obvious that important stage in oncogene upregulation leading to tumour initiation and advancement is the reduction of certain miRNAs that target oncogenes. MiRNA inhibitors and oligomers, such as small molecule inhibitors, miRNA sponges, miRNA masking, RNA, DNA, and DNA analogues (miRNA antisense treatment), can easily be used to suppress miRNA activity. On the other hand, miRNA mimetics that have been modified, like plasmid or lentiviral vectors expressing miRNA sequences, can be used to improve miRNA function (miRNA replacement treatment) (226).
For instance, Li et al. (207), who demonstrated that exosome-delivered circ 0094343 hinders the proliferation, clone formation, as well as glycolysis of colorectal cancer cells and improves chemosensitivity to different chemotherapeutic medications through the overexpression of TRIM67 through sponging miR-766-5p, described the inhibition of miRNA activity by miRNA sponges (207). In addition, it has been discovered that lenti-miR-766 transduction reduces cell proliferation and the population of colorectal cancer cell lines in the G1 phase in vitro (109). OS cells transfected with an inhibitor or mimic of miR-766-3p were injected subcutaneously into nude mice to examine miR-766-effects 3p’s in vivo. It was discovered that miR-766-3p knockdown increased OS cell development, resulting in larger and heavier tumors. Yet, high levels of miR-766-3p expression significantly slow tumor growth as compared to controls, resulting in a lighter and smaller tumor (227). In a different study, breast cancer cells that overexpress miR-766 (MB231 miR-766-GFP) formed less tumor spheres in vivo than control cells that had only been transfected with the vector (MB231 VectorGFP). In addition, compared to control cells, breast cancer cells with lung metastasis overexpressing miR-766 has been significantly decreased. Hence, miR-766 had a stronger impact on distant metastasis than Proliferation of cancer cells and early tumour growth, suggesting that tt might be a possible it could be a potential therapeutic target for the successful management of deadly breast cancer metastasis in the future (112).
On the basis of the observations that 1) the administration of various antagomiR molecules in combination causes stronger anti-tumor effects and 2) some anti-miR molecules can make drug-resistant tumour cell lines more susceptible to therapeutic drugs, combination strategies have recently been developed. A miRNA replacement treatment that combines antagomir and miRNA, and 3) miRNA replacement treatment combined with medication administration. The use of miR-766-based cancer therapy has not yet been studied, and more research is required.
There are certain restrictions on miR-766 that can be more carefully considered in further research. Although restoring normal miR-766 expression is advantageous, it is challenging to directly use miR-766 as a treatment due to the lack of information on the transcriptional and processing regulation of miR-766 during biogenesis and its precise function in tumorigenesis.
To reduce these adverse effects in an efficient treatment plan, specifics of the immunogenic and cytotoxic effects of miR-766 delivered in vivo must be investigated. Similar to how we suggested that miR-766 may be a part of the transcription factor-like gene regulatory network for mirnas, which is still unknown. As a result, it is not currently viable to knockdown inhibitory mirnas of miR-766 using anti-mirna oligos.
Limitations on Delivery Systems and Methods, a nother downside of a potential treatment is the lack of a suitable, secure, and efficient method of miR-766 distribution. Targeted delivery may employ biological vectors like aav and lentivirus, however uniformity of the procedure is necessary to avoid non-targeted site introduction. To treat brain and neuronal tumours, efficient neuron-specific delivery methods must be developed as delivery of mirna that targets the brain is not yet successful.
7 Conclusion
MiRNAs have received increasing attention as a result of their discovery, and one research discovered that they regulate a variety of cellular biological activities and contribute to the aetiology of disorders, including cancer (51). Additionally, the quantities of circulating miRNA are helpful for assessing or diagnosing human disease activities (51). This study investigated the role of miR-766 as a tumour suppressor or oncogene gene in relevant solid tumours, as well as its potential to be a tumour biomarker, targeted therapeutic method, and prognostic indicator in diverse cancer types. A number of studies have been conducted on the mechanisms underlying its activity as a cancer-inhibiting gene, but it is still unclear why it serves as both an onco-miRNA in cancer cells. This may be influenced by the pathogenic kind, histological origin, cellular microenvironment, and location of the relevant tumour.
In addition, we describe how lncRNAs and circRNAs act as sponges in cancer cells to regulate miR-766 and its target genes. We highlight examples of lncRNAs and circRNAs that interact with miR-766 in network modification and explore their impact on cancer malignancy. However, little is known about the mechanism by which the lncRNA-miR-766-mRNA ceRNA network controls the ncRNA field in cancer. Therefore, more research is required to clarify the activities and processes of cancer-related ceRNA. The circRNA, lncRNA, miRNA, and miRNA targeting mRNAs of the ceRNA network may potentially serve as potential therapeutic diagnostic markers and cancer targets. Future study will concentrate on clarifying previously reached controversial results.
Author contributions
BF involved in the conception, design, and drafting of the manuscript. JG, HA-H, MA, AJ, MD, ZA, FA, AR-C and PR contributed to data collection and manuscript drafting. All authors approved the final version for submission.
Conflict of interest
The authors declare that the research was conducted in the absence of any commercial or financial relationships that could be construed as a potential conflict of interest.
Publisher’s note
All claims expressed in this article are solely those of the authors and do not necessarily represent those of their affiliated organizations, or those of the publisher, the editors and the reviewers. Any product that may be evaluated in this article, or claim that may be made by its manufacturer, is not guaranteed or endorsed by the publisher.
References
1. Mirzaei H, Gholamin S, Shahidsales S, Sahebkar A, Jaafari MR, Mirzaei HR, et al. MicroRNAs as potential diagnostic and prognostic biomarkers in melanoma. Eur J Cancer (Oxford Engl 1990). (2016) 53:25–32. doi: 10.1016/j.ejca.2015.10.009
2. Salarinia R, Sahebkar A, Peyvandi M, Mirzaei HR, Jaafari MR, Riahi MM, et al. Epi-drugs and epi-miRs: moving beyond current cancer therapies. Curr Cancer Drug targets. (2016) 16(9):773–88. doi: 10.2174/1568009616666151207110143
3. Saadatpour L, Fadaee E, Fadaei S, Nassiri Mansour R, Mohammadi M, Mousavi SM, et al. Glioblastoma: exosome and microRNA as novel diagnosis biomarkers. Cancer Gene Ther (2016) 23(12):415–8. doi: 10.1038/cgt.2016.48
4. Mirzaei H, Momeni F, Saadatpour L, Sahebkar A, Goodarzi M, Masoudifar A, et al. MicroRNA: relevance to stroke diagnosis, prognosis, and therapy. J Cell Physiol (2018) 233(2):856–65. doi: 10.1002/jcp.25787
5. Mirzaei H, Fathullahzadeh S, Khanmohammadi R, Darijani M, Momeni F, Masoudifar A, et al. State of the art in microRNA as diagnostic and therapeutic biomarkers in chronic lymphocytic leukemia. J Cell Physiol (2018) 233(2):888–900. doi: 10.1002/jcp.25799
6. Moridikia A, Mirzaei H, Sahebkar A, Salimian J. MicroRNAs: potential candidates for diagnosis and treatment of colorectal cancer. J Cell Physiol (2018) 233(2):901–13. doi: 10.1002/jcp.25801
7. Gholamin S, Mirzaei H, Razavi SM, Hassanian SM, Saadatpour L, Masoudifar A, et al. GD2-targeted immunotherapy and potential value of circulating microRNAs in neuroblastoma. J Cell Physiol (2018) 233(2):866–79. doi: 10.1002/jcp.25793
8. Keshavarzi M, Sorayayi S, Jafar Rezaei M, Mohammadi M, Ghaderi A, Rostamzadeh A, et al. MicroRNAs-based imaging techniques in cancer diagnosis and therapy. J Cell Biochem (2017) 118(12):4121–8. doi: 10.1002/jcb.26012
9. Mahjoubin-Tehran M, Rezaei S, Jesmani A, Birang N, Morshedi K, Khanbabaei H, et al. New epigenetic players in stroke pathogenesis: from non-coding RNAs to exosomal non-coding RNAs. Biomed pharmacother (2021) 140:111753. doi: 10.1016/j.biopha.2021.111753
10. Saadat S, Noureddini M, Mahjoubin-Tehran M, Nazemi S, Shojaie L, Aschner M, et al. Pivotal role of TGF-β/Smad signaling in cardiac fibrosis: non-coding RNAs as effectual players. Front Cardiovasc Med (2020) 7:588347. doi: 10.3389/fcvm.2020.588347
11. Asgarpour K, Shojaei Z, Amiri F, Ai J, Mahjoubin-Tehran M, Ghasemi F, et al. Exosomal microRNAs derived from mesenchymal stem cells: cell-to-cell messages. Cell communication Signaling CCS. (2020) 18(1):149. doi: 10.1186/s12964-020-00650-6
12. Ghaemmaghami AB, Mahjoubin-Tehran M, Movahedpour A, Morshedi K, Sheida A, Taghavi SP, et al. Role of exosomes in malignant glioma: microRNAs and proteins in pathogenesis and diagnosis. Cell communication Signaling CCS. (2020) 18(1):120. doi: 10.1186/s12964-020-00623-9
13. Rezaei S, Mahjoubin-Tehran M, Aghaee-Bakhtiari SH, Jalili A, Movahedpour A, Khan H, et al. Autophagy-related MicroRNAs in chronic lung diseases and lung cancer. Crit Rev oncology/hematol (2020) 153:103063. doi: 10.1016/j.critrevonc.2020.103063
14. Ahangar Davoodi N, Najafi S, Naderi Ghale-Noie Z, Piranviseh A, Mollazadeh S, Ahmadi Asouri S, et al. Role of non-coding RNAs and exosomal non-coding RNAs in retinoblastoma progression. Front Cell Dev Biol (2022) 10:1065837. doi: 10.3389/fcell.2022.1065837
15. Yousefi F, Shabaninejad Z, Vakili S, Derakhshan M, Movahedpour A, Dabiri H, et al. TGF-β and WNT signaling pathways in cardiac fibrosis: non-coding RNAs come into focus. Cell communication Signaling CCS. (2020) 18(1):87. doi: 10.1186/s12964-020-00555-4
16. Hashemian SM, Pourhanifeh MH, Fadaei S, Velayati AA, Mirzaei H, Hamblin MR. Non-coding RNAs and exosomes: their role in the pathogenesis of sepsis. Mol Ther Nucleic Acids (2020) 21:51–74. doi: 10.1016/j.omtn.2020.05.012
17. Amiri A, Mahjoubin-Tehran M, Asemi Z, Shafiee A, Hajighadimi S, Moradizarmehri S, et al. Role of resveratrol in modulating microRNAs in human diseases: from cancer to inflammatory disorder. Curr medicinal Chem (2021) 28(2):360–76. doi: 10.2174/1875533XMTAyiOTQi2
18. Friedman RC, Farh KK-H, Burge CB, Bartel DP. Most mammalian mRNAs are conserved targets of microRNAs. Genome Res (2009) 19(1):92–105. doi: 10.1101/gr.082701.108
19. Li C, Wang Z, Zhang J, Zhao X, Xu P, Liu X, et al. Crosstalk of mRNA, miRNA, lncRNA, and circRNA and their regulatory pattern in pulmonary fibrosis. Mol Therapy-Nucleic Acids (2019) 18:204–18. doi: 10.1016/j.omtn.2019.08.018
20. Bartel DP. MicroRNAs: target recognition and regulatory functions. cell (2009) 136(2):215–33. doi: 10.1016/j.cell.2009.01.002
21. Lee Y, Kim M, Han J, Yeom KH, Lee S, Baek SH, et al. MicroRNA genes are transcribed by RNA polymerase II. EMBO J (2004) 23(20):4051–60. doi: 10.1038/sj.emboj.7600385
22. Westholm JO, Lai EC. Mirtrons: microRNA biogenesis via splicing. Biochimie (2011) 93(11):1897–904. doi: 10.1016/j.biochi.2011.06.017
23. Iacona JR, Lutz CS. miR-146a-5p: expression, regulation, and functions in cancer. Wiley Interdiscip Reviews: RNA. (2019) 10(4):e1533. doi: 10.1002/wrna.1533
24. Yousefpouran S, Mostafaei S, Manesh PV, Iranifar E, Bokharaei-Salim F, Nahand JS, et al. The assessment of selected MiRNAs profile in HIV, HBV, HCV, HIV/HCV, HIV/HBV Co-infection and elite controllers for determination of biomarker. Microbial Pathogenesis. (2020) 147:104355. doi: 10.1016/j.micpath.2020.104355
25. Letafati A, Najafi S, Mottahedi M, Karimzadeh M, Shahini A, Garousi S, et al. MicroRNA let-7 and viral infections: focus on mechanisms of action. Cell Mol Biol letters. (2022) 27(1):1–47. doi: 10.1186/s11658-022-00317-9
26. Nahand JS, Shojaie L, Akhlagh SA, Ebrahimi MS, Mirzaei HR, Baghi HB, et al. Cell death pathways and viruses: role of microRNAs. Mol Therapy-Nucleic Acids (2021) 24:487–511. doi: 10.1016/j.omtn.2021.03.011
27. Aghbash PS, Hemmat N, Nahand JS, Shamekh A, Memar MY, Babaei A, et al. The role of Th17 cells in viral infections. Int Immunopharmacol (2021) 91:107331. doi: 10.1016/j.intimp.2020.107331
28. Jamshidi S, Bokharaei-Salim F, Nahand JS, Monavari SH, Moghoofei M, Garshasbi S, et al. Evaluation of the expression pattern of 4 microRNAs and their correlation with cellular/viral factors in PBMCs of long term non-progressors and HIV infected naïve individuals. Curr HIV Res (2022) 20(1):42–53.
29. Hussen BM, Ahmadi G, Marzban H, Azar MEF, Sorayyayi S, Karampour R, et al. The role of HPV gene expression and selected cellular MiRNAs in lung cancer development. Microbial pathogenesis. (2021) 150:104692. doi: 10.1016/j.micpath.2020.104692
30. Mirzaei H, Rahimian N, Mirzaei HR, Nahand JS, Hamblin MR. MicroRNAs in cancer. In: Exosomes and MicroRNAs in biomedical science. Springer (2022). p. 11–40.
31. Nahand JS, Taghizadeh-boroujeni S, Karimzadeh M, Borran S, Pourhanifeh MH, Moghoofei M, et al. microRNAs: new prognostic, diagnostic, and therapeutic biomarkers in cervical cancer. J Cell Physiol (2019) 234(10):17064–99. doi: 10.1002/jcp.28457
32. Sadri Nahand J, Moghoofei M, Salmaninejad A, Bahmanpour Z, Karimzadeh M, Nasiri M, et al. Pathogenic role of exosomes and microRNAs in HPV-mediated inflammation and cervical cancer: a review. Int J cancer. (2020) 146(2):305–20. doi: 10.1002/ijc.32688
33. Javandoost E, Firoozi-Majd E, Rostamian H, Khakpoor-Koosheh M, Mirzaei HR. Role of microRNAs in chronic lymphocytic leukemia pathogenesis. Curr medicinal Chem (2020) 27(2):282–97. doi: 10.2174/0929867326666190911114842
34. Shabaninejad Z, Yousefi F, Movahedpour A, Ghasemi Y, Dokanehiifard S, Rezaei S, et al. Electrochemical-based biosensors for microRNA detection: nanotechnology comes into view. Analytical Biochem (2019) 581:113349. doi: 10.1016/j.ab.2019.113349
35. Saeedi Borujeni MJ, Esfandiary E, Baradaran A, Valiani A, Ghanadian M, Codoñer-Franch P, et al. Molecular aspects of pancreatic β-cell dysfunction: oxidative stress, microRNA, and long noncoding RNA. J Cell Physiol (2019) 234(6):8411–25. doi: 10.1002/jcp.27755
36. Mulrane L, Klinger R, McGee SF, Gallagher WM, O’Connor DP. microRNAs: a new class of breast cancer biomarkers. Expert Rev Mol diagnostics. (2014) 14(3):347–63. doi: 10.1586/14737159.2014.901153
37. Markou A, Liang Y, Lianidou E. Prognostic, therapeutic and diagnostic potential of microRNAs in non-small cell lung cancer. Clin Chem Lab Med (2011) 49(10):1591–603. doi: 10.1515/CCLM.2011.661
38. Schwarzenbach H, Nishida N, Calin GA, Pantel K. Clinical relevance of circulating cell-free microRNAs in cancer. Nat Rev Clin Oncol (2014) 11(3):145–56. doi: 10.1038/nrclinonc.2014.5
39. Pritchard CC, Kroh E, Wood B, Arroyo JD, Dougherty KJ, Miyaji MM, et al. Blood cell origin of circulating MicroRNAs: a cautionary note for cancer biomarker StudiesCirculating MicroRNA biomarkers and blood cells. Cancer Prev Res (2012) 5(3):492–7. doi: 10.1158/1940-6207.CAPR-11-0370
40. Simonian M, Mosallayi M, Mirzaei H. Circulating miR-21 as novel biomarker in gastric cancer: diagnostic and prognostic biomarker. J Cancer Res Ther (2018) 14(2):475.
41. Fathullahzadeh S, Mirzaei H, Honardoost MA, Sahebkar A, Salehi M. Circulating microRNA-192 as a diagnostic biomarker in human chronic lymphocytic leukemia. Cancer Gene Ther (2016) 23(10):327–32. doi: 10.1038/cgt.2016.34
42. Mohammadi M, Goodarzi M, Jaafari MR, Mirzaei HR, Mirzaei H. Circulating microRNA: a new candidate for diagnostic biomarker in neuroblastoma. Cancer Gene Ther (2016) 23(11):371–2. doi: 10.1038/cgt.2016.45
43. Mirzaei H, Khataminfar S, Mohammadparast S, Sales SS, Maftouh M, Mohammadi M, et al. Circulating microRNAs as potential diagnostic biomarkers and therapeutic targets in gastric cancer: current status and future perspectives. Curr medicinal Chem (2016) 23(36):4135–50. doi: 10.2174/0929867323666160818093854
44. Mirzaei HR, Sahebkar A, Mohammadi M, Yari R, Salehi H, Jafari MH, et al. Circulating microRNAs in hepatocellular carcinoma: potential diagnostic and prognostic biomarkers. Curr Pharm design. (2016) 22(34):5257–69. doi: 10.2174/1381612822666160303110838
45. Gholamin S, Pasdar A, Khorrami MS, Mirzaei H, Mirzaei HR, Salehi R, et al. The potential for circulating microRNAs in the diagnosis of myocardial infarction: a novel approach to disease diagnosis and treatment. Curr Pharm design. (2016) 22(3):397–403.
46. Vasilatou D, Papageorgiou S, Pappa V, Papageorgiou E, Dervenoulas J. The role of microRNAs in normal and malignant hematopoiesis. Eur J haematol (2010) 84(1):1–16. doi: 10.1111/j.1600-0609.2009.01348.x
47. Li YC, Li CF, Chen LB, Li DD, Yang L, Jin JP, et al. MicroRNA-766 targeting regulation of SOX6 expression promoted cell proliferation of human colorectal cancer. OncoTargets Ther (2015) 8:2981–8. doi: 10.2147/OTT.S89459
48. Duan X, Liu X, Cao Y, Li Y, Silayiding A, Zhang L, et al. Effect of MicroRNA-766 promotes proliferation, chemoresistance, migration, and invasion of breast cancer cells. Clin Breast Cancer. (2021) 21(1):e1–e17. doi: 10.1016/j.clbc.2019.10.006
49. Zhang Z, Sun H, Hou J, Li L, Wu L. Circular RNA circFADS2 inhibits the progression of cutaneous squamous cell carcinoma by regulating miR-766-3p/HOXA9 axis. Histol Histopathol (2021).
50. Yang C, Ma X, Guan G, Liu H, Yang Y, Niu Q, et al. MicroRNA-766 promotes cancer progression by targeting NR3C2 in hepatocellular carcinoma. FASEB J (2019) 33(1):1456–67. doi: 10.1096/fj.201801151R
51. Yang J, Han Q, Li C, Yang H, Chen X, Wang X. Circular RNA circ_0001105 inhibits progression and metastasis of osteosarcoma by sponging miR-766 and activating YTHDF2 expression. OncoTargets Ther (2020) 13:1723–36. doi: 10.2147/OTT.S234668
52. Wang Q, Selth LA, Callen DF. MiR-766 induces p53 accumulation and G2/M arrest by directly targeting MDM4. Oncotarget (2017) 8(18):29914–24. doi: 10.18632/oncotarget.15530
53. Gao J, Fei L, Wu X, Li H. MiR-766-3p suppresses malignant behaviors and stimulates apoptosis of colon cancer cells via targeting TGFBI. Can J Gastroenterol Hepatol (2022) 2022:7234704. doi: 10.1155/2022/7234704
54. O'Brien J, Hayder H, Zayed Y, Peng C. Overview of microRNA biogenesis, mechanisms of actions, and circulation. Front endocrinol (2018) 9:402. doi: 10.3389/fendo.2018.00402
55. Rarani FZ, Rashidi B, Jafari Najaf Abadi MH, Hamblin MR, Reza Hashemian SM, Mirzaei H. Cytokines and microRNAs in SARS-CoV-2: what do we know? Mol Ther Nucleic Acids (2022) 29:219–42. doi: 10.1016/j.omtn.2022.06.017
56. Mohammadi AH, Seyedmoalemi S, Moghanlou M, Akhlagh SA, Talaei Zavareh SA, Hamblin MR, et al. MicroRNAs and synaptic plasticity: from their molecular roles to response to therapy. Mol neurobiol (2022) 59(8):5084–102. doi: 10.1007/s12035-022-02907-2
57. Jafarzadeh A, Marzban H, Nemati M, Jafarzadeh S, Mahjoubin-Tehran M, Hamblin MR, et al. Dysregulated expression of miRNAs in immune thrombocytopenia. Epigenomics (2021) 13(16):1315–25. doi: 10.2217/epi-2021-0092
58. Khvorova A, Reynolds A, Jayasena SD. Functional siRNAs and miRNAs exhibit strand bias. Cell (2003) 115(2):209–16. doi: 10.1016/S0092-8674(03)00801-8
59. Almeida MI, Nicoloso MS, Zeng L, Ivan C, Spizzo R, Gafà R, et al. Strand-specific miR-28-5p and miR-28-3p have distinct effects in colorectal cancer cells. Gastroenterology (2012) 142(4):886–96. e9. doi: 10.1053/j.gastro.2011.12.047
60. Jiang L, Huang Q, Zhang S, Zhang Q, Chang J, Qiu X, et al. Hsa-miR-125a-3p and hsa-miR-125a-5p are downregulated in non-small cell lung cancer and have inverse effects on invasion and migration of lung cancer cells. BMC cancer. (2010) 10(1):1–13. doi: 10.1186/1471-2407-10-318
61. Mirzaei H. Stroke in women: risk factors and clinical biomarkers. J Cell Biochem (2017) 118(12):4191–202. doi: 10.1002/jcb.26130
62. Mirzaei H, Masoudifar A, Sahebkar A, Zare N, Sadri Nahand J, Rashidi B, et al. MicroRNA: a novel target of curcumin in cancer therapy. J Cell Physiol (2018) 233(4):3004–15. doi: 10.1002/jcp.26055
63. Golabchi K, Soleimani-Jelodar R, Aghadoost N, Momeni F, Moridikia A, Nahand JS, et al. MicroRNAs in retinoblastoma: potential diagnostic and therapeutic biomarkers. J Cell Physiol (2018) 233(4):3016–23. doi: 10.1002/jcp.26070
64. Banikazemi Z, Haji HA, Mohammadi M, Taheripak G, Iranifar E, Poursadeghiyan M, et al. Diet and cancer prevention: dietary compounds, dietary MicroRNAs, and dietary exosomes. J Cell Biochem (2018) 119(1):185–96. doi: 10.1002/jcb.26244
65. Saeedi Borujeni MJ, Esfandiary E, Taheripak G, Codoñer-Franch P, Alonso-Iglesias E, Mirzaei H. Molecular aspects of diabetes mellitus: resistin, microRNA, and exosome. J Cell Biochem (2018) 119(2):1257–72. doi: 10.1002/jcb.26271
66. Masoudi MS, Mehrabian E, Mirzaei H. MiR-21: a key player in glioblastoma pathogenesis. J Cell Biochem (2018) 119(2):1285–90. doi: 10.1002/jcb.26300
67. Mirzaei H, Ferns GA, Avan A, Mobarhan MG. Cytokines and MicroRNA in coronary artery disease. Adv Clin Chem (2017) 82:47–70. doi: 10.1016/bs.acc.2017.06.004
68. Tavakolizadeh J, Roshanaei K, Salmaninejad A, Yari R, Nahand JS, Sarkarizi HK, et al. MicroRNAs and exosomes in depression: potential diagnostic biomarkers. J Cell Biochem (2018) 119(5):3783–97. doi: 10.1002/jcb.26599
69. Yilmaz SG, Isbir S, Kunt AT, Isbir T. Circulating microRNAs as novel biomarkers for atherosclerosis. vivo. (2018) 32(3):561–5.
70. Abbasi-Kolli M, Sadri Nahand J, Kiani SJ, Khanaliha K, Khatami A, Taghizadieh M, et al. The expression patterns of MALAT-1, NEAT-1, THRIL, and miR-155-5p in the acute to the post-acute phase of COVID-19 disease. Braz J Infect Dis (2022) 26(3):102354. doi: 10.1016/j.bjid.2022.102354
71. Sadri Nahand J, Salmaninejad A, Mollazadeh S, Tamehri Zadeh SS, Rezaee M, Sheida AH, et al. Virus, exosome, and MicroRNA: new insights into autophagy. Adv Exp Med Biol (2022) 1401:97–162. doi: 10.1007/5584_2022_715
72. Balandeh E, Mohammadshafie K, Mahmoudi Y, Hossein Pourhanifeh M, Rajabi A, Bahabadi ZR, et al. Roles of non-coding RNAs and angiogenesis in glioblastoma. Front Cell Dev Biol (2021) 9:716462. doi: 10.3389/fcell.2021.716462
73. Mousavi SM, Derakhshan M, Baharloii F, Dashti F, Mirazimi SMA, Mahjoubin-Tehran M, et al. Non-coding RNAs and glioblastoma: insight into their roles in metastasis. Mol Ther oncolytics. (2022) 24:262–87. doi: 10.1016/j.omto.2021.12.015
74. Rahimian N, Nahand JS, Hamblin MR, Mirzaei H. Exosomal MicroRNA profiling. Methods Mol Biol (Clifton NJ). (2023) 2595:13–47. doi: 10.1007/978-1-0716-2823-2_2
75. Fadaei S, Zarepour F, Parvaresh M, Motamedzadeh A, Tamehri Zadeh SS, Sheida A, et al. Epigenetic regulation in myocardial infarction: non-coding RNAs and exosomal non-coding RNAs. Front Cardiovasc Med (2022) 9:1014961. doi: 10.3389/fcvm.2022.1014961
76. Jafarzadeh A, Noori M, Sarrafzadeh S, Tamehri Zadeh SS, Nemati M, Chatrabnous N, et al. MicroRNA-383: a tumor suppressor miRNA in human cancer. Front Cell Dev Biol (2022) 10:955486. doi: 10.3389/fcell.2022.955486
77. Rafiyan M, Abadi M, Zadeh SST, Hamblin MR, Mousavi M, Mirzaei H. Lysophosphatidic acid signaling and microRNAs: new roles in various cancers. Front Oncol (2022) 12:917471. doi: 10.3389/fonc.2022.917471
78. Jafarzadeh A, Seyedmoalemi S, Dashti A, Nemati M, Jafarzadeh S, Aminizadeh N, et al. Interplays between non-coding RNAs and chemokines in digestive system cancers. Biomed pharmacother (2022) 152:113237. doi: 10.1016/j.biopha.2022.113237
79. Mousavi SM, Amin Mahdian SM, Ebrahimi MS, Taghizadieh M, Vosough M, Sadri Nahand J, et al. Microfluidics for detection of exosomes and microRNAs in cancer: state of the art. Mol Ther Nucleic Acids (2022) 28:758–91. doi: 10.1016/j.omtn.2022.04.011
80. Ahmadpour S, Taghavi T, Sheida A, Tamehri Zadeh SS, Hamblin MR, Mirzaei H. Effects of microRNAs and long non-coding RNAs on chemotherapy response in glioma. Epigenomics (2022) 14(9):549–63. doi: 10.2217/epi-2021-0439
81. Tamtaji OR, Derakhshan M, Rashidi Noshabad FZ, Razaviyan J, Hadavi R, Jafarpour H, et al. Non-coding RNAs and brain tumors: insights into their roles in apoptosis. Front Cell Dev Biol (2021) 9:792185. doi: 10.3389/fcell.2021.792185
82. Jafarzadeh A, Paknahad MH, Nemati M, Jafarzadeh S, Mahjoubin-Tehran M, Rajabi A, et al. Dysregulated expression and functions of microRNA-330 in cancers: a potential therapeutic target. Biomed pharmacother (2022) 146:112600. doi: 10.1016/j.biopha.2021.112600
83. Dorraki N, Ghale-Noie ZN, Ahmadi NS, Keyvani V, Bahadori RA, Nejad AS, et al. miRNA-148b and its role in various cancers. Epigenomics (2021) 13(24):1939–60. doi: 10.2217/epi-2021-0155
84. Jafarzadeh A, Naseri A, Shojaie L, Nemati M, Jafarzadeh S, Bannazadeh Baghi H, et al. MicroRNA-155 and antiviral immune responses. Int Immunopharmacol (2021) 101(Pt A):108188. doi: 10.1016/j.intimp.2021.108188
85. Razavi ZS, Asgarpour K, Mahjoubin-Tehran M, Rasouli S, Khan H, Shahrzad MK, et al. Angiogenesis-related non-coding RNAs and gastrointestinal cancer. Mol Ther oncolytics. (2021) 21:220–41. doi: 10.1016/j.omto.2021.04.002
86. Medina PP, Nolde M, Slack FJ. OncomiR addiction in an in vivo model of microRNA-21-induced pre-b-cell lymphoma. Nature (2010) 467(7311):86–90. doi: 10.1038/nature09284
87. Davoodvandi A, Marzban H, Goleij P, Sahebkar A, Morshedi K, Rezaei S, et al. Effects of therapeutic probiotics on modulation of microRNAs. Cell communication Signaling CCS. (2021) 19(1):4. doi: 10.1186/s12964-020-00668-w
88. Razavi ZS, Tajiknia V, Majidi S, Ghandali M, Mirzaei HR, Rahimian N, et al. Gynecologic cancers and non-coding RNAs: epigenetic regulators with emerging roles. Crit Rev oncology/hematol (2021) 157:103192. doi: 10.1016/j.critrevonc.2020.103192
89. Mirzaei H, Hamblin MR. Regulation of glycolysis by non-coding RNAs in cancer: switching on the warburg effect. Mol Ther oncolytics. (2020) 19:218–39. doi: 10.1016/j.omto.2020.10.003
90. Ashrafizadeh M, Zarrabi A, Hashemipour M, Vosough M, Najafi M, Shahinozzaman M, et al. Sensing the scent of death: modulation of microRNAs by curcumin in gastrointestinal cancers. Pharmacol Res (2020) 160:105199. doi: 10.1016/j.phrs.2020.105199
91. Pourhanifeh MH, Vosough M, Mahjoubin-Tehran M, Hashemipour M, Nejati M, Abbasi-Kolli M, et al. Autophagy-related microRNAs: possible regulatory roles and therapeutic potential in and gastrointestinal cancers. Pharmacol Res (2020) 161:105133. doi: 10.1016/j.phrs.2020.105133
92. Zhang S, Chen H, Liu W, Fang L, Qian Z, Kong R, et al. miR-766-3p suppressed tumorigenesis, epithelial-mesenchymal transition, and metastasis by targeting BCL9L via β-catenin signaling pathway in osteosarcoma cells. (2020).
93. Svoronos AA, Engelman DM, Slack FJ. OncomiR or tumor suppressor? the duplicity of microRNAs in cancer. Cancer Res (2016) 76(13):3666–70.
94. Xi Y, Xu P. Global colorectal cancer burden in 2020 and projections to 2040. Trans Oncol (2021) 14(10):101174.
95. Rahimian N, Miraei HR, Amiri A, Ebrahimi MS, Nahand JS, Tarrahimofrad H, et al. Plant-based vaccines and cancer therapy: where are we now and where are we going? Pharmacol Res (2021) 169:105655.
96. Jemal A, Bray F, Center MM, Ferlay J, Ward E, Forman D. Global cancer statistics. CA Cancer J Clin (2011) 61(2):69–90.
97. Chaffer CL, Weinberg RA. A perspective on cancer cell metastasis. Science (2011) 331(6024):1559–64. doi: 10.1126/science.1203543
98. Weitz J, Koch M, Debus J, Höhler T, Galle PR, Büchler MW. Colorectal cancer. Lancet (London England). (2005) 365(9454):153–65. doi: 10.1016/S0140-6736(05)17706-X
99. Benoit G, Cooney A, Giguere V, Ingraham H, Lazar M, Muscat G, et al. International union of pharmacology. LXVI. Orphan Nucl receptors. Pharmacol Rev (2006) 58(4):798–836.
100. Germain P, Staels B, Dacquet C, Spedding M, Laudet V. Overview of nomenclature of nuclear receptors. Pharmacol Rev (2006) 58(4):685–704. doi: 10.1124/pr.58.4.2
101. Dhiman VK, Bolt MJ, White KP. Nuclear receptors in cancer - uncovering new and evolving roles through genomic analysis. Nat Rev Genet (2018) 19(3):160–74. doi: 10.1038/nrg.2017.102
102. Gerdin AK, Surve VV, Jönsson M, Bjursell M, Björkman M, Edenro A, et al. Phenotypic screening of hepatocyte nuclear factor (HNF) 4-gamma receptor knockout mice. Biochem Biophys Res Commun (2006) 349(2):825–32. doi: 10.1016/j.bbrc.2006.08.103
103. Jia B, Xia L, Cao F. The role of miR-766-5p in cell migration and invasion in colorectal cancer. Exp Ther Med (2018) 15(3):2569–74. doi: 10.3892/etm.2018.5716
104. Liu P, Shi L, Ding Y, Luan J, Shan X, Li Q, et al. MicroRNA-766 promotes the proliferation, migration and invasion, and inhibits the apoptosis of cutaneous squamous cell carcinoma cells by targeting PDCD5. OncoTargets Ther (2020) 13:4099–110. doi: 10.2147/OTT.S222821
105. Yao F-Y, Zhao C, Zhong F-M, Qin T-Y, Wen F, Li M-Y, et al. M (6) a modification of lncRNA NEAT1 regulates chronic myelocytic leukemia progression via miR-766-5p/CDKN1A axis. Front Oncol (2021) 11:679634. doi: 10.3389/fonc.2021.679634
106. Liang H, Li X, Wang L, Yu S, Xu Z, Gu Y, et al. MicroRNAs contribute to promyelocyte apoptosis in As2O3-treated APL cells. Cell Physiol Biochem (2013) 32(6):1818–29. doi: 10.1159/000356615
107. Cai Y, Zhang K, Cao L, Sun H, Wang H. Inhibition of microrna-766-5p attenuates the development of cervical cancer through regulating SCAI. Technol Cancer Res Treat (2020) 19:1533033820980081. doi: 10.1177/1533033820980081
108. Xue Y, Xue D, Yao B, Hu C, Liu J. Correlation between microRNA-766 expression in patients with advanced gastric cancer and the efficacy of platinum-containing chemotherapy. Eur Rev Med Pharmacol Sci (2020) 24(17):8822–9.
109. Afgar A, Fard-Esfahani P, Mehrtash A, Azadmanesh K, Khodarahmi F, Ghadir M, et al. MiR-339 and especially miR-766 reactivate the expression of tumor suppressor genes in colorectal cancer cell lines through DNA methyltransferase 3B gene inhibition. Cancer Biol Ther (2016) 17(11):1126–38. doi: 10.1080/15384047.2016.1235657
110. Chen W, Cai G, Liao Z, Lin K, Li G, Li Y. miRNA−766 induces apoptosis of human colon cancer cells through the p53/Bax signaling pathway by MDM4. Exp Ther Med (2019) 17(5):4100–8.
111. He XX, Luo SS, Qin HQ, Mo XW. MicroRNA-766-3p-mediated downregulation of HNF4G inhibits proliferation in colorectal cancer cells through the PI3K/AKT pathway. Cancer Gene Ther (2022) 29(6):803–13. doi: 10.1038/s41417-021-00362-0
112. Oh K, Lee DS. In vivo Validation of metastasis-regulating microRNA-766 in human triple-negative breast cancer cells. Lab Anim Res (2017) 33(3):256–63. doi: 10.5625/lar.2017.33.3.256
113. You Y, Que K, Zhou Y, Zhang Z, Zhao X, Gong J, et al. MicroRNA-766-3p inhibits tumour progression by targeting Wnt3a in hepatocellular carcinoma. Molecules Cells (2018) 41(9):830–41.
114. Liu L, Qi X, Gui Y, Huo H, Yang X, Yang L. Overexpression of circ_0021093 circular RNA forecasts an unfavorable prognosis and facilitates cell progression by targeting the miR-766-3p/MTA3 pathway in hepatocellular carcinoma. Gene (2019) 714:143992. doi: 10.1016/j.gene.2019.143992
115. Li Z, Liu Y, Yan J, Zeng Q, Hu Y, Wang H, et al. Circular RNA hsa_circ_0056836 functions an oncogenic gene in hepatocellular carcinoma through modulating miR-766-3p/FOSL2 axis. Aging (2020) 12(3):2485–97. doi: 10.18632/aging.102756
116. Chen C, Xue S, Zhang J, Chen W, Gong D, Zheng J, et al. DNA-Methylation-mediated repression of miR-766-3p promotes cell proliferation via targeting SF2 expression in renal cell carcinoma. Int J Cancer. (2017) 141(9):1867–78. doi: 10.1002/ijc.30853
117. Hong X, Chen Y, Zhang D. CircZNF652 accelerates the proliferation and migration of primary lung carcinoma cells by downregulating miR-766. Trop J Pharm Res (2021) 20(11):2255–60. doi: 10.4314/tjpr.v20i11.3
118. Bai Y, Zhang G, Cheng R, Yang R, Chu H. CASC15 contributes to proliferation and invasion through regulating miR-766-5p/KLK12 axis in lung cancer. Cell Cycle (2019) 18(18):2323–31. doi: 10.1080/15384101.2019.1646562
119. Wang M, Liao Q, Zou P. PRKCZ-AS1 promotes the tumorigenesis of lung adenocarcinoma via sponging miR-766-5p to modulate MAPK1. Cancer Biol Ther (2020) 21(4):364–71. doi: 10.1080/15384047.2019.1702402
120. Chen F, Zhang H, Wang J. Circular RNA CircSHKBP1 accelerates the proliferation, invasion, angiogenesis, and stem cell-like properties via modulation of microR-766-5p/high mobility group AT-hook 2 axis in laryngeal squamous cell carcinoma. Bioengineered (2022) 13(5):11551–63. doi: 10.1080/21655979.2022.2068922
121. Li S, Yan G, Yue M, Kang Z, Wang L. MicroRNA−766 inhibits the malignant biological behaviours of pancreatic ductal adenocarcinoma by directly targeting ETS1. Mol Med Rep (2019) 19(2):1380–7.
122. Zhao W, Zhu X, Jin Q, Lin B, Ji R. The lncRNA NEAT1/miRNA-766-5p/E2F3 regulatory axis promotes prostate cancer progression. J Oncol (2022) 2022. doi: 10.1155/2022/1866972
123. He Z, Cai K, Zeng Z, Lei S, Cao W, Li X. Autophagy-associated circRNA circATG7 facilitates autophagy and promotes pancreatic cancer progression. Cell Death disease. (2022) 13(3):1–15. doi: 10.1038/s41419-022-04677-0
124. Zhou N, Zhu X, Man L. LINC00963 functions as an oncogene in bladder cancer by regulating the miR-766-3p/MTA1 axis. Cancer Manage Res (2020) 12:3353–61. doi: 10.2147/CMAR.S249979
125. Gen Y, Muramatsu T, Inoue J, Inazawa J. miR-766-5p targets super-enhancers by downregulating CBP and BRD4miR-766-5p targets super-enhancers in cancer. Cancer Res (2021) 81(20):5190–201. doi: 10.1158/0008-5472.CAN-21-0649
126. Huang C, Su X, Zhou D-L, Xu B-H, Liu Q, Zhang X, et al. A diagnostic and predictive lncRNA lnc-MPEG1-1 promotes the proliferation and metastasis of papillary thyroid cancer cells by occupying miR-766-5p. Mol Therapy-Nucleic Acids (2022) 28:408–22. doi: 10.1016/j.omtn.2022.03.023
127. Wang T, Zhu X, Wang K. CircMIIP contributes to non-small cell lung cancer progression by binding miR-766-5p to upregulate FAM83A expression. Lung (2022) 200(1):107–17.
128. Bray F, Ferlay J, Soerjomataram I, Siegel RL, Torre LA, Jemal A. Global cancer statistics 2018: GLOBOCAN estimates of incidence and mortality worldwide for 36 cancers in 185 countries. CA: Cancer J Clin (2018) 68(6):394–424.
129. Van Cutsem E, Nordlinger B, Cervantes A. Advanced colorectal cancer: ESMO clinical practice guidelines for treatment. Ann Oncol (2010) 21 Suppl 5:v93–7.
130. Kim H, Yu Y, Choi S, Lee H, Yu J, Lee JH, et al. Evodiamine eliminates colon cancer stem cells via suppressing notch and wnt signaling. Molecules (Basel Switzerland). (2019) 24(24).
131. Lang K, Kahveci S, Bonberg N, Wichert K, Behrens T, Hovanec J, et al. TGFBI protein is increased in the urine of patients with high-grade urothelial carcinomas, and promotes cell proliferation and migration. Int J Mol Sci (2019) 20(18).
132. Guo SK, Shen MF, Yao HW, Liu YS. Enhanced expression of TGFBI promotes the proliferation and migration of glioma cells. Cell Physiol Biochem (2018) 49(3):1097–109. doi: 10.1159/000493293
133. Wang BJ, Chi KP, Shen RL, Zheng SW, Guo Y, Li JF, et al. TGFBI promotes tumor growth and is associated with poor prognosis in oral squamous cell carcinoma. J Cancer. (2019) 10(20):4902–12. doi: 10.7150/jca.29958
134. Ween MP, Oehler MK, Ricciardelli C. Transforming growth factor-Beta-Induced protein (TGFBI)/(βig-H3): a matrix protein with dual functions in ovarian cancer. Int J Mol Sci (2012) 13(8):10461–77. doi: 10.3390/ijms130810461
135. Ma C, Rong Y, Radiloff DR, Datto MB, Centeno B, Bao S, et al. Extracellular matrix protein betaig-h3/TGFBI promotes metastasis of colon cancer by enhancing cell extravasation. Genes Dev (2008) 22(3):308–21. doi: 10.1101/gad.1632008
136. Buckhaults P, Rago C, St Croix B, Romans KE, Saha S, Zhang L, et al. Secreted and cell surface genes expressed in benign and malignant colorectal tumors. Cancer Res (2001) 61(19):6996–7001.
137. Chen W, Cai G, Liao Z, Lin K, Li G, Li Y. miRNA-766 induces apoptosis of human colon cancer cells through the p53/Bax signaling pathway by MDM4. Exp Ther Med (2019) 17(5):4100–8. doi: 10.3892/etm.2019.7436
138. Curradi M, Izzo A, Badaracco G, Landsberger N. Molecular mechanisms of gene silencing mediated by DNA methylation. Mol Cell Biol (2002) 22(9):3157–73. doi: 10.1128/MCB.22.9.3157-3173.2002
139. Kanai Y, Hirohashi S. Alterations of DNA methylation associated with abnormalities of DNA methyltransferases in human cancers during transition from a precancerous to a malignant state. Carcinogenesis (2007) 28(12):2434–42. doi: 10.1093/carcin/bgm206
140. DeSantis CE, Ma J, Gaudet MM, Newman LA, Miller KD, Goding Sauer A, et al. Breast cancer statistics, 2019. CA Cancer J Clin (2019) 69(6):438–51. doi: 10.3322/caac.21583
141. Kaur RP, Vasudeva K, Kumar R, Munshi A. Role of p53 gene in breast cancer: focus on mutation spectrum and therapeutic strategies. Curr Pharm design. (2018) 24(30):3566–75. doi: 10.2174/1381612824666180926095709
142. Taylor WR, Stark GR. Regulation of the G2/M transition by p53. Oncogene (2001) 20(15):1803–15. doi: 10.1038/sj.onc.1204252
143. Liu J, Zhang C, Zhao Y, Feng Z. MicroRNA control of p53. J Cell Biochem (2017) 118(1):7–14. doi: 10.1002/jcb.25609
144. Feiten S, Dünnebacke J, Heymanns J, Köppler H, Thomalla J, van Roye C, et al. Breast cancer morbidity: questionnaire survey of patients on the long term effects of disease and adjuvant therapy. Deutsches Arzteblatt Int (2014) 111(31-32):537–44.
145. Harbeck N, Penault-Llorca F, Cortes J, Gnant M, Houssami N, Poortmans P, et al. Breast cancer. Nat Rev Dis Primers. (2019) 5(1):66. doi: 10.1038/s41572-019-0111-2
146. Chan SH, Wang LH. Regulation of cancer metastasis by microRNAs. J Biomed science. (2015) 22(1):9. doi: 10.1186/s12929-015-0113-7
147. Siegel RL, Miller KD, Jemal A. Cancer statistics, 2019. CA Cancer J Clin (2019) 69(1):7–34. doi: 10.3322/caac.21551
148. Lam JS, Leppert JT, Figlin RA, Belldegrun AS. Surveillance following radical or partial nephrectomy for renal cell carcinoma. Curr Urol Rep (2005) 6(1):7–18. doi: 10.1007/s11934-005-0062-x
149. Bukowski RM. Natural history and therapy of metastatic renal cell carcinoma: the role of interleukin-2. Cancer (1997) 80(7):1198–220. doi: 10.1002/(SICI)1097-0142(19971001)80:7<1198::AID-CNCR3>3.0.CO;2-H
150. Hollingsworth JM, Miller DC, Daignault S, Hollenbeck BK. Five-year survival after surgical treatment for kidney cancer: a population-based competing risk analysis. Cancer (2007) 109(9):1763–8. doi: 10.1002/cncr.22600
151. Feldman DR, Motzer RJ. Novel targets and therapies for metastatic renal cell carcinoma. Oncol (Williston Park NY). (2006) 20(14):1745–53.
152. Najjar YG, Rini BI. Novel agents in renal carcinoma: a reality check. Ther Adv Med Oncol (2012) 4(4):183–94. doi: 10.1177/1758834012443725
153. Cho IC, Chung J. Current status of targeted therapy for advanced renal cell carcinoma. Korean J urology. (2012) 53(4):217–28. doi: 10.4111/kju.2012.53.4.217
154. Motzer RJ, Michaelson MD, Redman BG, Hudes GR, Wilding G, Figlin RA, et al. Activity of SU11248, a multitargeted inhibitor of vascular endothelial growth factor receptor and platelet-derived growth factor receptor, in patients with metastatic renal cell carcinoma. J Clin Oncol (2006) 24(1):16–24. doi: 10.1200/JCO.2005.02.2574
155. Ghafouri-Fard S, Shirvani-Farsani Z, Branicki W, Taheri M. MicroRNA signature in renal cell carcinoma. Front Oncol (2020) 10:596359. doi: 10.3389/fonc.2020.596359
156. Redova M, Svoboda M, Slaby O. MicroRNAs and their target gene networks in renal cell carcinoma. Biochem Biophys Res Commun (2011) 405(2):153–6. doi: 10.1016/j.bbrc.2011.01.019
157. Osanto S, Qin Y, Buermans HP, Berkers J, Lerut E, Goeman JJ, et al. Genome-wide microRNA expression analysis of clear cell renal cell carcinoma by next generation deep sequencing. PloS One (2012) 7(6):e38298. doi: 10.1371/journal.pone.0038298
158. Keren H, Lev-Maor G, Ast G. Alternative splicing and evolution: diversification, exon definition and function. Nat Rev Genet (2010) 11(5):345–55. doi: 10.1038/nrg2776
159. Venables JP. Aberrant and alternative splicing in cancer. Cancer Res (2004) 64(21):7647–54. doi: 10.1158/0008-5472.CAN-04-1910
160. Krainer AR, Conway GC, Kozak D. Purification and characterization of pre-mRNA splicing factor SF2 from HeLa cells. Genes Dev (1990) 4(7):1158–71. doi: 10.1101/gad.4.7.1158
161. Cartegni L, Chew SL, Krainer AR. Listening to silence and understanding nonsense: exonic mutations that affect splicing. Nat Rev Genet (2002) 3(4):285–98. doi: 10.1038/nrg775
162. Anczuków O, Rosenberg AZ, Akerman M, Das S, Zhan L, Karni R, et al. The splicing factor SRSF1 regulates apoptosis and proliferation to promote mammary epithelial cell transformation. Nat Struct Mol Biol (2012) 19(2):220–8. doi: 10.1038/nsmb.2207
163. Karni R, de Stanchina E, Lowe SW, Sinha R, Mu D, Krainer AR. The gene encoding the splicing factor SF2/ASF is a proto-oncogene. Nat Struct Mol Biol (2007) 14(3):185–93. doi: 10.1038/nsmb1209
164. Orth M, Metzger P, Gerum S, Mayerle J, Schneider G, Belka C, et al. Pancreatic ductal adenocarcinoma: biological hallmarks, current status, and future perspectives of combined modality treatment approaches. Radiat Oncol (London England). (2019) 14(1):141. doi: 10.1186/s13014-019-1345-6
165. Wolpin BM. Pancreatic cancer. Hematol/oncol Clinics North America. (2015) 29(4):xiii–xiv. doi: 10.1016/j.hoc.2015.06.002
166. Winter JM, Brennan MF, Tang LH, D'Angelica MI, Dematteo RP, Fong Y, et al. Survival after resection of pancreatic adenocarcinoma: results from a single institution over three decades. Ann Surg Oncol (2012) 19(1):169–75. doi: 10.1245/s10434-011-1900-3
167. Cleary SP, Gryfe R, Guindi M, Greig P, Smith L, Mackenzie R, et al. Prognostic factors in resected pancreatic adenocarcinoma: analysis of actual 5-year survivors. J Am Coll Surgeons. (2004) 198(5):722–31. doi: 10.1016/j.jamcollsurg.2004.01.008
168. Cho K, Ishiwata T, Uchida E, Nakazawa N, Korc M, Naito Z, et al. Enhanced expression of keratinocyte growth factor and its receptor correlates with venous invasion in pancreatic cancer. Am J Pathol (2007) 170(6):1964–74. doi: 10.2353/ajpath.2007.060935
169. Tesfaye AA, Azmi AS, Philip PA. miRNA and gene expression in pancreatic ductal adenocarcinoma. Am J Pathol (2019) 189(1):58–70. doi: 10.1016/j.ajpath.2018.10.005
170. Hernandez YG, Lucas AL. MicroRNA in pancreatic ductal adenocarcinoma and its precursor lesions. World J gastrointestinal Oncol (2016) 8(1):18–29. doi: 10.4251/wjgo.v8.i1.18
171. Waldman A, Schmults C. Cutaneous squamous cell carcinoma. Hematol/Oncol Clinics. (2019) 33(1):1–12. doi: 10.1016/j.hoc.2018.08.001
172. Li P, Fei H, Wang L, Xu H, Zhang H, Zheng L. PDCD5 regulates cell proliferation, cell cycle progression and apoptosis. Oncol Letters. (2018) 15(1):1177–83.
173. Peng C, Zhao H, Song Y, Chen W, Wang X, Liu X, et al. SHCBP1 promotes synovial sarcoma cell metastasis via targeting TGF-β1/Smad signaling pathway and is associated with poor prognosis. J Exp Clin Cancer Res (2017) 36(1):1–12. doi: 10.1186/s13046-017-0616-z
174. Zhang Z, Sun H, Hou J, Li L, Wu L. Circular RNA circFADS2 inhibits the progression of cutaneous squamous cell carcinoma by regulating miR-766-3p/HOXA9 axis. Histol histopathology. (2022) 37(4):335–48.
175. Chidambaranathan-Reghupaty S, Fisher PB, Sarkar D. Hepatocellular carcinoma (HCC): epidemiology, etiology and molecular classification. Adv Cancer Res (2021) 149:1–61. doi: 10.1016/bs.acr.2020.10.001
176. Sadri Nahand J, Bokharaei-Salim F, Salmaninejad A, Nesaei A, Mohajeri F, Moshtzan A, et al. microRNAs: key players in virus-associated hepatocellular carcinoma. J Cell Physiol (2019) 234(8):12188–225. doi: 10.1002/jcp.27956
177. Sagae S, Monk BJ, Pujade-Lauraine E, Gaffney DK, Narayan K, Ryu SY, et al. Advances and concepts in cervical cancer trials: a road map for the future. Int J gynecological Cancer (2016) 26(1):199–207. doi: 10.1097/IGC.0000000000000587
178. Nahand JS, Vandchali NR, Darabi H, Doroudian M, Banafshe HR, Moghoofei M, et al. Exosomal microRNAs: novel players in cervical cancer. Epigenomics (2020) 12(18):1651–60. doi: 10.2217/epi-2020-0026
179. Sadri Nahand J, Salmaninejad A, Mollazadeh S, Tamehri Zadeh SS, Rezaee M, Sheida AH, et al. Virus, exosome, and MicroRNA: new insights into autophagy. In: Cell biology and translational medicine, vol. 17. Springer (2022). p. 97–162.
180. Brandt DT, Baarlink C, Kitzing TM, Kremmer E, Ivaska J, Nollau P, et al. SCAI acts as a suppressor of cancer cell invasion through the transcriptional control of beta1-integrin. Nat Cell Biol (2009) 11(5):557–68. doi: 10.1038/ncb1862
181. Yi T, Zhou X, Sang K, Zhou J, Ge L. MicroRNA-1270 modulates papillary thyroid cancer cell development by regulating SCAI. Biomed pharmacother = Biomedecine pharmacotherapie. (2019) 109:2357–64. doi: 10.1016/j.biopha.2018.08.150
182. Lin L, Liu D, Liang H, Xue L, Su C, Liu M. MiR-1228 promotes breast cancer cell growth and metastasis through targeting SCAI protein. Int J Clin Exp pathology. (2015) 8(6):6646–55.
183. Fintha A, Gasparics Á, Fang L, Erdei Z, Hamar P, Mózes MM, et al. Characterization and role of SCAI during renal fibrosis and epithelial-to-mesenchymal transition. Am J Pathol (2013) 182(2):388–400. doi: 10.1016/j.ajpath.2012.10.009
184. Gasparics Á, Kökény G, Fintha A, Bencs R, Mózes MM, Ágoston EI, et al. Alterations in SCAI expression during cell plasticity, fibrosis and cancer. Pathol Oncol Res POR. (2018) 24(3):641–51. doi: 10.1007/s12253-017-0293-4
185. Jiang M-C, Ni J-J, Cui W-Y, Wang B-Y, Zhuo W. Emerging roles of lncRNA in cancer and therapeutic opportunities. Am J Cancer Res (2019) 9(7):1354.
186. Liu SJ, Dang HX, Lim DA, Feng FY, Maher CA. Long noncoding RNAs in cancer metastasis. Nat Rev Cancer. (2021) 21(7):446–60. doi: 10.1038/s41568-021-00353-1
187. Nahand JS, Jamshidi S, Hamblin MR, Mahjoubin-Tehran M, Vosough M, Jamali M, et al. Circular RNAs: new epigenetic signatures in viral infections. Front Microbiol (2020) 11:1853. doi: 10.3389/fmicb.2020.01853
188. Hao N-B, He Y-F, Li X-Q, Wang K, Wang R-L. The role of miRNA and lncRNA in gastric cancer. Oncotarget (2017) 8(46):81572. doi: 10.18632/oncotarget.19197
189. Yu Y, Gao F, He Q, Li G, Ding G. lncRNA UCA1 functions as a ceRNA to promote prostate cancer progression via sponging miR143. Mol Therapy-Nucleic Acids (2020) 19:751–8. doi: 10.1016/j.omtn.2019.11.021
190. Wang ZY, Duan Y, Wang P. SP1-mediated upregulation of lncRNA SNHG4 functions as a ceRNA for miR-377 to facilitate prostate cancer progression through regulation of ZIC5. J Cell Physiol (2020) 235(4):3916–27. doi: 10.1002/jcp.29285
191. Zeng X, Xiao J, Bai X, Liu Y, Zhang M, Liu J, et al. Research progress on the circRNA/lncRNA–miRNA–mRNA axis in gastric cancer. Pathology-Research Pract (2022) 154030. doi: 10.1016/j.prp.2022.154030
192. Chen L-L, Yang L. Regulation of circRNA biogenesis. RNA Biol (2015) 12(4):381–8. doi: 10.1080/15476286.2015.1020271
193. Salzman J, Gawad C, Wang PL, Lacayo N, Brown PO. Circular RNAs are the predominant transcript isoform from hundreds of human genes in diverse cell types. PloS One (2012) 7(2):e30733. doi: 10.1371/journal.pone.0030733
194. Memczak S, Jens M, Elefsinioti A, Torti F, Krueger J, Rybak A, et al. Circular RNAs are a large class of animal RNAs with regulatory potency. Nature (2013) 495(7441):333–8. doi: 10.1038/nature11928
195. Hansen TB, Jensen TI, Clausen BH, Bramsen JB, Finsen B, Damgaard CK, et al. Natural RNA circles function as efficient microRNA sponges. Nature (2013) 495(7441):384–8. doi: 10.1038/nature11993
196. Sung H, Ferlay J, Siegel RL, Laversanne M, Soerjomataram I, Jemal A, et al. Global cancer statistics 2020: GLOBOCAN estimates of incidence and mortality worldwide for 36 cancers in 185 countries. CA: Cancer J Clin (2021) 71(3):209–49. doi: 10.3322/caac.21660
197. Bradford CR, Ferlito A, Devaney KO, Mäkitie AA, Rinaldo A. Prognostic factors in laryngeal squamous cell carcinoma. Laryngoscope Invest Otolaryngology. (2020) 5(1):74–81. doi: 10.1002/lio2.353
198. Bodnar M, Szylberg Ł, Kazmierczak W, Marszalek A. Tumor progression driven by pathways activating matrix metalloproteinases and their inhibitors. J Oral Pathol Med (2015) 44(6):437–43. doi: 10.1111/jop.12270
199. Faubert B, Solmonson A, DeBerardinis RJ. Metabolic reprogramming and cancer progression. Science (2020) 368(6487):eaaw5473. doi: 10.1126/science.aaw5473
200. Bach D-H, Lee SK, Sood AK. Circular RNAs in cancer. Mol Therapy-Nucleic Acids (2019) 16:118–29. doi: 10.1016/j.omtn.2019.02.005
201. Shuang Y, Liu J, Niu J, Guo W, Li C. A novel circular RNA circPPFIA1 promotes laryngeal squamous cell carcinoma progression through sponging miR-340-3p and regulating ELK1 expression. Bioengineered (2021) 12(1):5220–30. doi: 10.1080/21655979.2021.1959866
202. Wei Z, Chang K, Fan C. Hsa_circ_0042666 inhibits proliferation and invasion via regulating miR-223/TGFBR3 axis in laryngeal squamous cell carcinoma. Biomed Pharmacotherapy. (2019) 119:109365. doi: 10.1016/j.biopha.2019.109365
203. Choi SH, Yoon HI, Yi S, Park JW, Cho J, Shin DA, et al. Treatment outcomes of radiotherapy for primary spinal cord glioma. Strahlentherapie und Onkologie. (2019) 195(2):164–74. doi: 10.1007/s00066-018-1366-3
204. Hon KW, Ab-Mutalib NS, Abdullah NMA, Jamal R, Abu N. Extracellular vesicle-derived circular RNAs confers chemoresistance in colorectal cancer. Sci Rep (2019) 9(1):1–13. doi: 10.1038/s41598-019-53063-y
205. Mirzaei H, Rahimian N, Mirzaei HR, Nahand JS, Hamblin MR. Exosomes and MicroRNAs in biomedical science. Synthesis Lectures Biomed Engineering. (2022) 17(1):1–175. doi: 10.1007/978-3-031-79177-2
206. Zhao M, Xu J, Zhong S, Liu Y, Xiao H, Geng L, et al. Expression profiles and potential functions of circular RNAs in extracellular vesicles isolated from radioresistant glioma cells. Oncol Rep (2019) 41(3):1893–900. doi: 10.3892/or.2019.6972
207. Li C, Li X. Exosome-derived Circ_0094343 promotes chemosensitivity of colorectal cancer cells by regulating glycolysis via the miR-766-5p/TRIM67 axis. Contrast Media Mol Imaging. (2022) 2022. doi: 10.1155/2022/2878557
209. Lim SM, Mohamad Hanif EA, Chin S-F. Is targeting autophagy mechanism in cancer a good approach? the possible double-edge sword effect. Cell bioscience. (2021) 11(1):1–13. doi: 10.1186/s13578-021-00570-z
210. Fu Z, Cheng X, Kuang J, Feng H, Chen L, Liang J, et al. CQ sensitizes human pancreatic cancer cells to gemcitabine through the lysosomal apoptotic pathway via reactive oxygen species. Mol Oncol (2018) 12(4):529–44. doi: 10.1002/1878-0261.12179
211. Qiang L, Sample A, Shea CR, Soltani K, Macleod KF, He Y-Y. Autophagy gene ATG7 regulates ultraviolet radiation-induced inflammation and skin tumorigenesis. Autophagy (2017) 13(12):2086–103. doi: 10.1080/15548627.2017.1380757
212. Gao N, Li Y, Li J, Gao Z, Yang Z, Li Y, et al. Long non-coding RNAs: the regulatory mechanisms, research strategies, and future directions in cancers. Front Oncol (2020) 10:598817. doi: 10.3389/fonc.2020.598817
213. Feng S, Liu N, Chen X, Liu Y, An J. Long non-coding RNA NEAT1/miR-338-3p axis impedes the progression of acute myeloid leukemia via regulating CREBRF. Cancer Cell Int (2020) 20(1):1–11. doi: 10.1186/s12935-020-01182-2
214. Gao C, Zhang J, Wang Q, Ren C. Overexpression of lncRNA NEAT1 mitigates multidrug resistance by inhibiting ABCG2 in leukemia. Oncol letters. (2016) 12(2):1051–7. doi: 10.3892/ol.2016.4738
215. Zeng C, Liu S, Lu S, Yu X, Lai J, Wu Y, et al. The c-myc-regulated lncRNA NEAT1 and paraspeckles modulate imatinib-induced apoptosis in CML cells. Mol cancer. (2018) 17(1):1–6. doi: 10.1186/s12943-018-0884-z
216. Zeng C, Xu Y, Xu L, Yu X, Cheng J, Yang L, et al. Inhibition of long non-coding RNA NEAT1 impairs myeloid differentiation in acute promyelocytic leukemia cells. BMC cancer. (2014) 14(1):1–7. doi: 10.1186/1471-2407-14-693
217. Li Z, Luo J. Epigenetic regulation of HOTAIR in advanced chronic myeloid leukemia. Cancer Manage Res (2018) 10:5349. doi: 10.2147/CMAR.S166859
218. Dieter C, Lourenco ED, Lemos NE. Association of long non-coding RNA and leukemia: a systematic review. Gene (2020) 735:144405. doi: 10.1016/j.gene.2020.144405
219. Ettinger DS, Akerley W, Bepler G, Blum MG, Chang A, Cheney RT, et al. Non–small cell lung cancer. J Natl Compr Cancer network. (2010) 8(7):740–801. doi: 10.6004/jnccn.2010.0056
220. Saji H, Tsuboi M, Shimada Y, Kato Y, Hamanaka W, Kudo Y, et al. Gene expression profiling and molecular pathway analysis for the identification of early-stage lung adenocarcinoma patients at risk for early recurrence. Oncol Rep (2013) 29(5):1902–6. doi: 10.3892/or.2013.2332
221. Kabbout M, Garcia MM, Fujimoto J, Liu DD, Woods D, Chow C-W, et al. ETS2 mediated tumor suppressive function and MET oncogene inhibition in human non–small cell lung CancerETS2 tumor suppressor in human NSCLC. Clin Cancer Res (2013) 19(13):3383–95. doi: 10.1158/1078-0432.CCR-13-0341
222. Li Z, Yamada S, Wu Y, Wang K-Y, Liu Y-P, Uramoto H, et al. Polypeptide n-acetylgalactosaminyltransferase-6 expression independently predicts poor overall survival in patients with lung adenocarcinoma after curative resection. Oncotarget (2016) 7(34):54463. doi: 10.18632/oncotarget.9810
223. Behera M, Owonikoko TK, Gal AA, Steuer CE, Kim S, Pillai RN, et al. Lung adenocarcinoma staging using the 2011 IASLC/ATS/ERS classification: a pooled analysis of adenocarcinoma in situ and minimally invasive adenocarcinoma. Clin Lung cancer. (2016) 17(5):e57–64. doi: 10.1016/j.cllc.2016.03.009
224. Zhou J, Xiao H, Yang X, Tian H, Xu Z, Zhong Y, et al. Long noncoding RNA CASC9. 5 promotes the proliferation and metastasis of lung adenocarcinoma. Sci Rep (2018) 8(1):1–11.
225. Wang V, Wu W. MicroRNA-based therapeutics for cancer. BioDrugs Clin immunotherapeutics biopharmaceuticals Gene Ther (2009) 23(1):15–23. doi: 10.2165/00063030-200923010-00002
226. Gambari R, Brognara E, Spandidos DA, Fabbri E. Targeting oncomiRNAs and mimicking tumor suppressor miRNAs: New trends in the development of miRNA therapeutic strategies in oncology (Review). Int J Oncol (2016) 49(1):5–32. doi: 10.3892/ijo.2016.3503
Keywords: cancer, microRNA, miR-766, biomarker, drug resistance
Citation: Gupta J, Kareem Al-Hetty HRA, Aswood MS, Turki Jalil A, Azeez MD, Aminov Z, Alsaikhan F, Ramírez-Coronel AA, Ramaiah P and Farhood B (2023) The key role of microRNA-766 in the cancer development. Front. Oncol. 13:1173827. doi: 10.3389/fonc.2023.1173827
Received: 25 February 2023; Accepted: 18 April 2023;
Published: 02 May 2023.
Edited by:
José Díaz-Chávez, Instituto Nacional de Cancerología (INCAN), MexicoReviewed by:
Jian Pu, Affiliated Hospital of Youjiang Medical University for Nationalities, ChinaJiwei Zhang, Shanghai University of Traditional Chinese Medicine, China
Copyright © 2023 Gupta, Kareem Al-Hetty, Aswood, Turki Jalil, Azeez, Aminov, Alsaikhan, Ramírez-Coronel, Ramaiah and Farhood. This is an open-access article distributed under the terms of the Creative Commons Attribution License (CC BY). The use, distribution or reproduction in other forums is permitted, provided the original author(s) and the copyright owner(s) are credited and that the original publication in this journal is cited, in accordance with accepted academic practice. No use, distribution or reproduction is permitted which does not comply with these terms.
*Correspondence: Hussein Riyadh Abdul Kareem Al-Hetty, hussain.riyad@uoa.edu.iq; Abduladheem Turki Jalil, abedalazeem799@gmail.com; Bagher Farhood, bffarhood@gmail.com, farhood-b@kaums.ac.ir
‡ORCID: Pushpamala Ramaiah, orcid.org/0000-0001-6784-613X