- 1Department of Biology, University of Pisa, Pisa, Italy
- 2Pancreato-Biliary Endoscopy and Endosonography Division, Pancreas Translational and Clinical Research Center, Istituti di Ricovero e Cura a Carattere Scientifico (IRCCS) San Raffaele Scientific Institute, Milan, Italy
- 3Unit of Pancreatic Surgery, Pancreas Translational and Clinical Research Center, San Raffaele Scientific Institute, Vita-Salute University, Milan, Italy
- 4Department of Translational Research and of New Technologies in Medicine and Surgery, University of Pisa, Pisa, Italy
- 5Genomic Epidemiology Group, German Cancer Research Center (DKFZ), Heidelberg, Germany
- 6General Surgery Unit, Department of Translational Research and New Technologies in Medicine and Surgery, University of Pisa, Pisa, Italy
- 7Digestive and Liver Disease Unit, Sant’Andrea University Hospital, Faculty of Medicine and Psychology, Sapienza University of Rome, Rome, Italy
Introduction: Pancreatic ductal adenocarcinoma (PDAC) is lethal due to its late diagnosis and lack of successful treatments. A possible strategy to reduce its death burden is prevention. Intraductal papillary mucinous neoplasms (IPMNs) are precursors of PDAC. It is difficult to estimate the incidence of IPMNs because they are asymptomatic. Two recent studies reported pancreatic cysts in 3% and 13% of scanned subjects. The possibility of identifying a subgroup of IPMN patients with a higher probability of progression into cancer could be instrumental in increasing the survival rate. In this study, genetic and non-genetic PDAC risk factors were tested in a group of IPMN patients under surveillance.
Methods: A retrospective study was conducted on 354 IPMN patients enrolled in two Italian centres with an average follow-up of 64 months. With the use of DNA extracted from blood, collected at IPMN diagnosis, all patients were genotyped for 30 known PDAC risk loci. The polymorphisms were analysed individually and grouped in an unweighted polygenic score (PGS) in relation to IPMN progression. The ABO blood group and non-genetic PDAC risk factors were also analysed. IPMN progression was defined based on the development of worrisome features and/or high-risk stigmata during follow-up.
Results: Two genetic variants (rs1517037 and rs10094872) showed suggestive associations with an increment of IPMN progression. After correction for multiple testing, using the Bonferroni correction, none of the variants showed a statistically significant association. However, associations were observed for the non-genetic variables, such as smoking status, comparing heavy smokers with light smokers (HR = 3.81, 95% 1.43–10.09, p = 0.007), and obesity (HR = 2.46, 95% CI 1.22–4.95, p = 0.012).
Conclusion: In conclusion, this study is the first attempt to investigate the presence of shared genetic background between PDAC risk and IPMN progression; however, the results suggest that the 30 established PDAC susceptibility polymorphisms are not associated with clinical IPMN progression in a sample of 354 patients. However, we observed indications of cigarette smoking and body mass index (BMI) involvement in IPMN progression. The biological mechanism that could link these two risk factors to progression could be chronic inflammation, of which both smoking and obesity are strong promoters.
1 Introduction
Pancreatic ductal adenocarcinoma (PDAC) is a hard-to-treat cancer (HTTC), and it is projected to become the second most common cancer in terms of mortality by 2030 in the United States (1). In the past decade, only modest improvement has been obtained in terms of survival time, which, only recently, approached 11% at 5 years after diagnosis in the United States (2). This meagre survival is principally due to the late diagnosis when, usually, the tumour is in an advanced stage. Early diagnosis is challenging for the lack of specific symptoms and a comprehensive understanding of the aetiology of the disease (3). Compared to more common cancers, only a small number of environmental/lifestyle factors have been established (4, 5). Our knowledge of the genetics of PDAC is also relatively limited, with approximately 30 susceptibility loci identified (6–14). Considering that there is no successful treatment apart from surgery, prevention remains the best option to decrease the death toll for this disease. Up to 8%–10% of the patients undergoing abdominal imaging harbour unsuspected pancreatic cysts in the general population, the majority of which are intraductal papillary mucinous neoplasms (IPMNs), well-known precursors of PDAC (15, 16). The precise incidence of IPMN is challenging to estimate due to the asymptomatic nature of this condition, which makes the diagnosis difficult, leading to an underestimation of the incidence. Two studies that carried out a series of computed tomography scans in subjects without history or symptoms associated with pancreatic diseases have reported pancreatic cysts in 3% and 13% of scanned subjects (17, 18). The diagnosis of high-risk IPMNs offers the unique opportunity to treat a direct precursor of PDAC. IPMNs are common, with an estimated prevalence of 3%–6% in the general population that rises to 10% in the elderly (19–21). Surgery is the only curative approach for IPMN with suspicion of malignancy, but pancreatectomy is still associated with high morbidity and mortality rates (22). Moreover, a considerable fraction of IPMN patients do not progress to invasive cancer (23, 24). There are significant differences in the incidence rate of pancreatic malignancy among the different IPMN forms. Branch-duct (BD) IPMNs have a progression rate to pancreatic cancer of 3.3% after 5 years from initial diagnosis, which rises to 15% after 15 years. Compared to BD, main-duct (MD) and mixed-type (MT) IPMNs have higher progression rates of 6%–45% and 60%–90%, respectively (25–29). Current guidelines identify high-risk stigmata (HRSs) and worrisome features (WFs) as indications for surgical resection (30). Although WFs and HRSs are useful indicators of clinical progression, they are not necessarily markers of progression towards cancer. Nevertheless, a recent study reported that patients with at least a WF at diagnosis are more prone to progress to malignancy if they develop additional WFs or HRSs during surveillance (31). The identification of endogenous (e.g., genetic variability) and exogenous (e.g., lifestyle factors such as smoking and alcohol consumption) markers of progression is of the uttermost importance since it could improve the stratification of patients according to their risk of developing PDAC. Several lines of evidence suggest that lifestyle and genetic factors play a role in transforming IPMN into PDAC (32–36). Since carcinogenesis is a complex and multifactorial process, the only viable way to predict individuals’ risks is to analyse them with a comprehensive approach (33, 34). Additionally, it has been proposed that PDAC risk factors may play a role in IPMN progression. For example, non-O blood group carriers have an increased risk of developing PDAC and have been suggested to have a higher chance of acquiring WFs and/or HRSs (34). Therefore, in this study, with the aim of finding progression markers, we have tested 30 single-nucleotide polymorphisms (SNPs), the ABO blood group, and five non-genetic PDAC risk factors in a group of 354 IPMN patients under follow-up, recruited in two high-volume Italian centres.
2 Materials and methods
2.1 Study population
The retrospective study population consisted of 354 subjects with a diagnosis of IPMNs enrolled by two Italian centres: 273 individuals from San Raffaele Research Hospital (Milan, Italy) and 81 from Ospedale Cisanello-University of Pisa (Pisa, Italy). The diagnosis of IPMN was ascertained by the observation of magnetic resonance imaging with magnetic resonance cholangiopancreatography and/or endoscopic ultrasonography. The inclusion criteria were as follows: a) age of >18 years, b) will and can cooperate, and c) a certain diagnosis of IPMN, with a follow-up of at least 12 months. Exclusion criteria from the study were the absence of follow-up and indication for surgery at diagnosis. WFs were defined as a) cyst size ≥3 cm, b) enhancing mural nodule <5 mm, c) thickened enhanced cyst walls, d) main pancreatic duct (MPD) size between 5 and 9 mm, e) abrupt change in MPD diameter with distal pancreatic atrophy, f) lymphadenopathy, g) increased serum level of CA19-9, h) cyst growth rate >5 mm/2 years, and i) acute pancreatitis. HRS was defined as a) obstructive jaundice caused by the IPMN, b) enhancing mural nodule ≥5 mm, and c) MPD ≥ 10 mm. WFs and HRSs at diagnosis were defined according to the International Association of Pancreatology guidelines (30). A small subgroup (46 from San Raffaele and 10 from Pisa) already showed at least one WF at diagnosis and was followed up like all the other enrolled subjects.
An aliquot of whole peripheral blood was collected at IPMN diagnosis to conduct genetic analyses. The study obtained institutional review board (IRB) approval in both the enrolling centres: San Raffaele Research Hospital, protocol number 133/2016 (for recording clinical information) protocol BIOGASTRO 2011, amendment 6/11/2017 (for blood sample biobanking and biomarker investigation); Cisanello Hospital-University of Pisa, the ethical committee was the “Comitato Etico Regionale per la Sperimentazione Clinica della Toscana sezione Area Vasta Nord Ovest”, protocol code “IPMN-PDAC transition”. The study population details are shown in Table 1.
2.2 Study outcomes
Four outcomes of interest were defined: I) development of the first WF and/or HRS for patients who did not present any at diagnosis, II) development of an additional WF or HRS for patients showing one at diagnosis, III) development of more than two WFs or HRSs for patients who did not present any at diagnosis, and IV) development of HRSs in naïve patients.
2.3 SNP selection
The 30 known PDAC risk SNPs identified so far were selected to generate a polygenic score (PGS) representative of the risk of developing PDAC. In detail, 28 SNPs, identified through genome-wide association studies (GWASs), were selected (12, 37–41). Furthermore, two SNPs identified in two candidate gene association studies were added (42, 43). In addition, two SNPs were used to infer the ABO blood group, specifically rs505922, which discriminates O from non-O, and rs8176746, which distinguishes between A and B alleles (41, 44). The selected SNPs and their association with PDAC risk are shown in Supplementary Table 1. This specific PGS was designed and validated in two studies on PDAC susceptibility (45, 46).
2.4 Non-genetic PDAC risk factors
At diagnosis, in addition to sex and age, data on smoking expressed as smoking status (smokers/non-smokers) and divided into classes based on the number of cigarettes smoked per day (light smoker, a smoker who reports consuming between 1 and 10 cigarettes per day; moderate smoker, 11–20 cigarettes per day; heavy smoker, 21 cigarettes or more per day), diabetes status, (yes/no), body mass index (BMI) (usual BMI before diagnosis) were collected. BMI was also categorised into four classes: underweight (BMI < 18.5), healthy weight (BMI >18.5 to <25), overweight (BMI 25 to <30), and obese (BMI 30 or higher). Finally, a family history of pancreatic cancer was reported as a binary variable, considering the presence of pancreatic cancer cases in at least one first-degree relative.
2.5 Genotyping
Genomic DNA of IPMN patients was extracted from whole blood using the QIAamp DNA Kit (QIAGEN, CA, USA) and genotyped in 384-well plates with TaqMan technology (Thermo Fisher Applied Biosystems, Waltham, MA, USA). In addition to the samples, no-template controls and duplicated samples (8%), used for quality control purposes, were included on each plate. The endpoint fluorescence reading of the plates and the assignment of the genotype were performed using QuantStudio 5 Real-Time PCR system (Thermo Fisher Applied Biosystems, Waltham, MA, USA) and QuantStudio software. The frequencies of the 32 SNPs genotyped in IPMN were tested for deviation from the Hardy–Weinberg equilibrium using Pearson’s chi-square test.
2.6 Statistical analysis and score computation
Association with progression was calculated as hazard ratio (HR) using the Cox regression multivariate analysis adjusted for age and sex. The same analyses were performed for the four defined outcomes. The different types of IPMN were compared, using branch-duct IPMNs as reference. The PGS was calculated for all the subjects with a genotyping call rate (CR) higher than 80%, summing the number of PDAC risk alleles, thus generating a score with a value within 0 to 60 and adding the value associated with the ABO blood group, with a value of 0 for the OO group and 1 for the other blood groups. To compensate for the missing genotypes in the subjects with a CR lower than 100%, the PGS was scaled by dividing PGS by the subject CR. The PGS computation is described in detail elsewhere (45). Applying the Bonferroni correction (dividing the threshold value of 0.05 by the number of tests: 30 SNPs and 10 non-genetic factors, for a total of 40 independent tests), we considered the p-value = 0.0013 as the threshold for statistical significance.
3 Results
Of 354 patients with a median age of 67 years (IQR 63.0–70.0 years), during an average follow-up time of 64 months, 91 patients developed the first WF and/or HRS, while 29 patients developed an additional one. Of the 354 patients, 205 were female, and 149 were male. BD IPMNs were the most common (314), followed by MT (31) and MD IPMNs (9). The information on BMI was collected for 342 subjects. Among these, 197 (58%) had normal weight, 96 (28%) were overweight, 30 (9%) were obese, and 19 (6%) were underweight. A total of 225 patients (64%) were never smokers, while 129 (36%) were current smokers. In addition, data on alcohol consumption were collected for 354 patients; of these, 201 (57%) were non-drinkers. The study population characteristics are summarised in Table 1.
3.1 Genetic association analysis
A total of 30 SNPs were analysed individually and combined in a PGS in relation to IPMN clinical progression. All SNPs were in Hardy–Weinberg equilibrium, and the average genotyping call rate was 99%.
A statistically significant association (p ≤ 0.05) was observed in the model that included only the patient without WFs at diagnosis, with the T allele of 18q21.32-rs1517037 increasing the probability of IPMN progression (HR = 1.55, 95% CI 1.06–2.29, p = 0.026). A similar result was observed in the analyses carried out on patients without WFs at diagnosis who developed more than two WFs or HRSs during follow-up. The results are shown in Supplementary Table 1.
Moreover, in the model that included the patients who showed WFs at diagnosis, the A allele of the 8q24.21-rs10094872 was associated with increased progression probability (HR = 1.39, 95% CI 1.02–1.89, p = 0.043). After correction for multiple testing (using the Bonferroni correction), none of the variants showed a statistically significant association.
The PGS was not associated with IPMN clinical progression (p > 0.05). Also, the individual blood group was not associated with progression. The results of the association analysis of SNPs and blood groups are shown in Table 2.
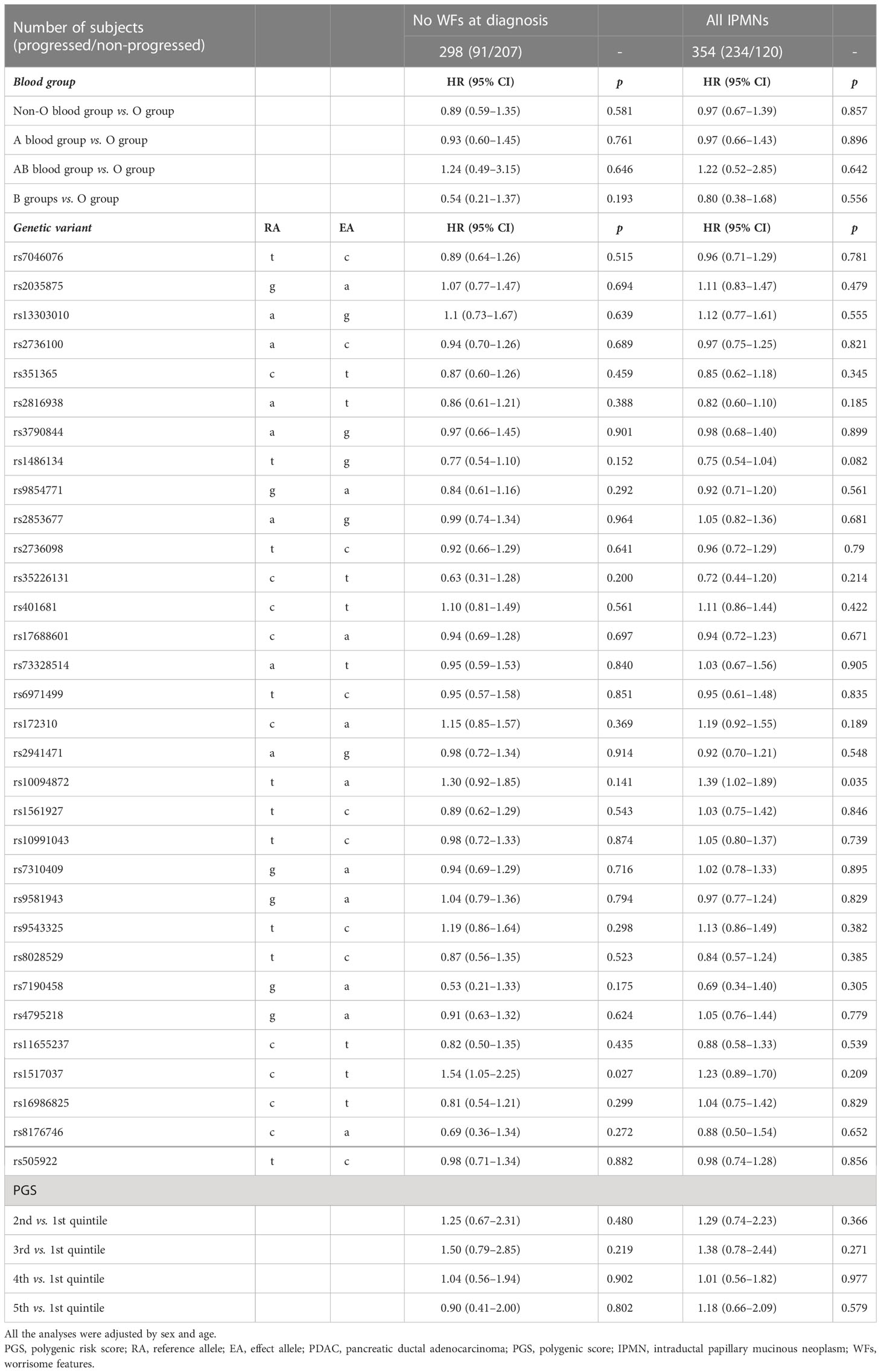
Table 2 Results of the association analysis between ABO blood group, PDAC susceptibility loci analysed individually and combined in a PGS, and the risk of IPMN progression.
3.2 Non-genetic factors
Several statistically significant associations (p ≤ 0.05) were observed for the non-genetic variables. The number of cigarettes smoked per day, analysed by dividing the smokers into light, moderate, and heavy smokers, was associated with IPMN progression, HR = 3.81 (95% 1.43–10.09, p = 0.007), in the model that included IPMN patients without WFs at diagnosis, and HR = 2.98 (95% CI 1.26–7.04, p = 0.013) analysing all the IPMN patients. Instead, the smoking status (ever vs. never) was not associated with progression.
Furthermore, obese patients (BMI > 30 kg/m2) showed an increased risk of clinical progression compared to healthy weight patients (BMI 18–25 kg/m2), with an HR = 2.46 (95% CI 1.22–4.95, p = 0.01) in the model with the patients without WFs at diagnosis, and HR = 2.03 (95% CI 1.17–3.52, p = 0.012) in the model that included all the patients.
Analysing the risk of clinical progression of branch-duct, mixed-type, and main-duct IPMNs, the MD, compared to the BD, showed an increased probability of progression, in particular in the model that included just the patients without WFs at diagnosis, HR = 10.17 (95% CI 1.34–77.13, p = 0.025), a trend confirmed in the more inclusive model, HR = 3.52 (95% CI 1.60–7.74, p = 0.002). The mixed-type IPMN also showed an increment of the probability of progression but was less evident than main-duct IPMN, HR = 2.37 (95% CI 1.24–4.55, p = 0.009) and HR = 1.69 (95% CI 1.04–2.76, p = 0.035), in the model with all patients.
Finally, diabetes and a family history of pancreatic cancer were not associated with clinical IPMN progression. Sex (HR = 1.27, 95% CI 1.01–2.38, p = 0.035), age (HR = 1.03, 95% CI 1.01–1.06, p = 0.011), the size of the largest cyst (HR = 1.07, 95% CI 1.04–1.10, p = 3 × 10−5), and pancreatitis (HR = 3.02, 95% CI 1.40–6.53, p = 0.005) were associated with IPMN progression (Table 3). After correction for multiple testing, none of the variables showed a statistically significant association, except for the size of the largest cyst. In the analyses conducted in patients without WFs at diagnosis who developed more than two WFs or HRSs during follow-up, the association with the size of the cyst was confirmed, HR = 1.20 (95% CI 1.11–1.31, p = 1.53 × 10−5). In this analysis, an association with chronic pancreatitis was also observed, HR = 3.40 (95% CI 1.06–10.88, p = 0.039). Results are shown in Supplementary Table 2.
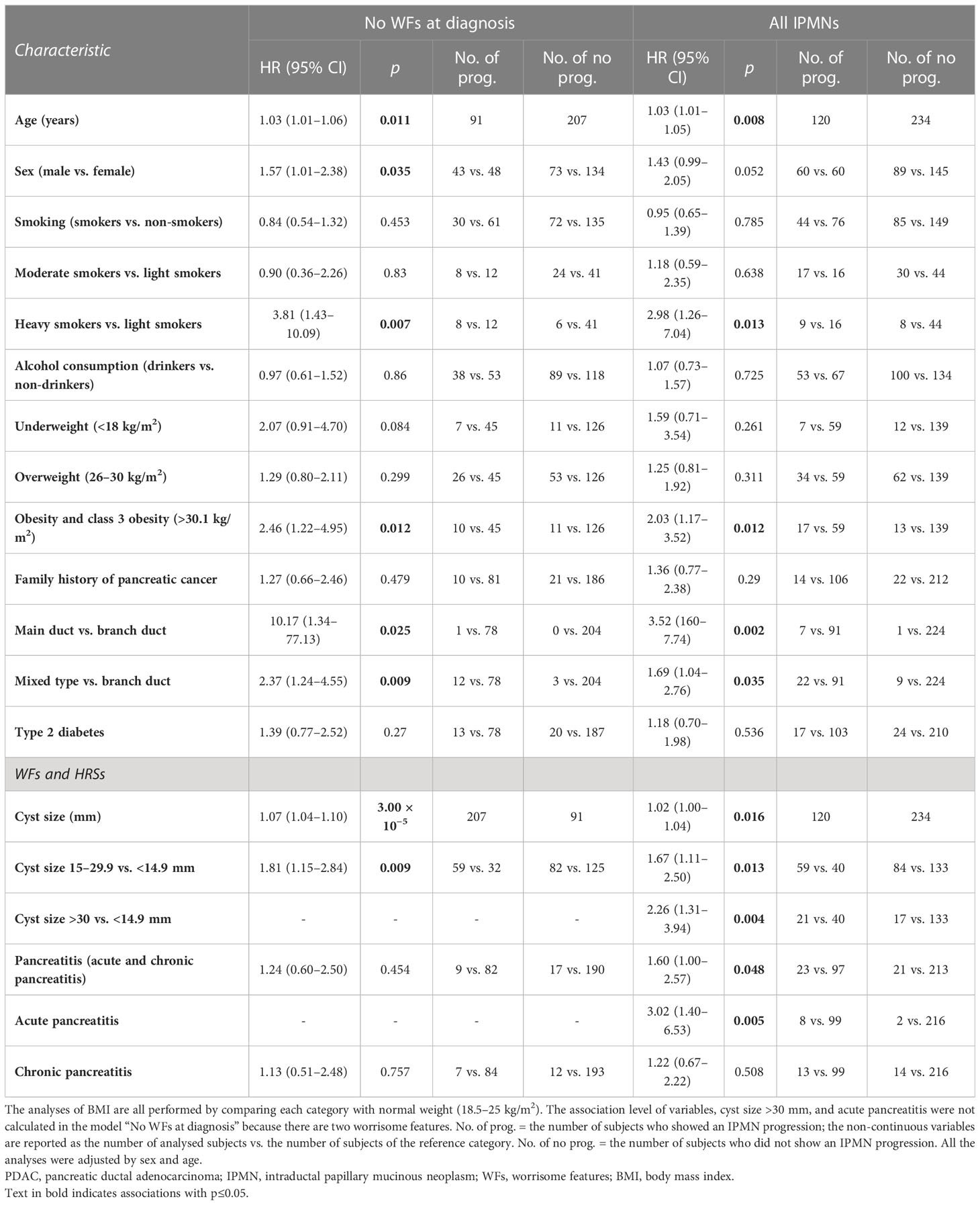
Table 3 Results of the association analysis between the known PDAC risk factors and the IPMN progression.
4 Discussion
Considering that PDAC is an HTTC, detection at non-malignant stages is, at the moment, the best strategy to lower the death burden associated with the disease. A viable approach is to focus on high-risk individuals, among which IPMN patients are a large proportion. The estimated IPMN progression rate to PDAC ranges from 3%–15% for BD IPMNs to 40%–90% for MD IPMNs (25–28, 47–50). Therefore, identifying IPMNs that will evolve into PDAC will be instrumental for detecting PDAC cases during the early stages and will also limit the overtreatment of patients who do not require surgery. Since some PDAC risk factors such as BMI and heavy cigarette smoking have been suggested to play a role in the development of WFs and HRSs in IPMN patients (34), in this study, we analysed several PDAC risk factors (30 PDAC susceptibility SNPs, the ABO blood group, and five non-genetic PDAC risk factors) in relation to IPMN progression.
According to the current knowledge, our data confirmed the greater tendency to clinical progression of MD IPMN when compared with BD IPMN. Furthermore, we observed that the MD has 10 times more possibility to progress than BD, HR = 10.17 (1.34–77.33, p = 0.025).
4.1 Genetic background and IPMN progression
We observed two SNPs associated with IPMN progression with a p-value lower than 0.05. The T allele of the 18q21.32-rs1517037 and the A allele of 8q24.21-rs10094872 were associated with IPMN progression. rs1517037 showed similar results in the model that considered patients without WFs at diagnosis who developed more than two WFs or HRSs during follow-up, making rs1517037 a potential candidate for future studies on IPMN progression. This SNP is an intergenic variant near the gastrin-releasing peptide (GRP) gene, and the C allele is associated with PDAC risk. This variant is also associated with several traits related to PDAC risks, such as BMI, diabetes, and pancreas volume (51–54). The T allele of rs10094872 is associated with PDAC risk; this variant is situated near the MYC proto-oncogene. MYC plays a role in cell cycle progression, apoptosis, and cellular transformation, and mutations in this gene are frequently observed in numerous human cancers (55–60). Although these associations are intriguing, they are not statistically significant when applying multiple testing corrections. Additionally, the alleles associated with increased PDAC risk are associated with a lower probability of IPMN progression in our study. Therefore, these associations are probably due to statistical fluctuation. Thus, the results suggest the absence of a shared genetic background between PDAC risk and IPMN progression. This conclusion agrees with our previous results, which identified a germline variant associated with clinical IPMN progression that is not associated with PDAC risk (33).
We did not observe a statistically significant association between the ABO blood group and IPMN progression, which further supports the different genetic predispositions for IPMN progression and PDAC risk. The role of the non-O blood group in IPMN progression in non-resected IPMN patients was investigated only in one study by Capurso and colleagues, which observed an increased risk of progression in the A blood group compared to O subjects (34). A possible explanation for the difference between the results of the two studies could be ascribed to the different sample sizes since the present study includes four times more patients than the other. Three studies investigating the role of the ABO blood group in IPMN-resected patients reported heterogeneous results (61–63). Zelga and colleagues, analysing 819 resected IPMN patients, reported a significant predominance of the non-O blood group compared with the O blood group in patients with malignant IPMNs (63). Instead, Poruk and colleagues reported that O blood group individuals had a higher probability of having high-grade dysplasia in a sample of 777 resected IPMNs (61). Finally, Hamada and colleagues analysed 1,815 Japanese patients and found that A or B blood group individuals have a higher risk of developing PDAC when compared with those in the O blood group (62). Therefore, the role of the ABO blood group in IPMN progression remains controversial also for resected patients.
4.2 Non-genetic factors and IPMN progression
Our results suggest that clinical IPMN progression and PDAC risk share, at least partially, the same non-genetic risk factors. We observed an association between two established PDAC risk factors, cigarette smoking and BMI, with IPMN progression. Cigarette smoking was already observed in association with IPMN progression; Carr et al. reported that smoking may accelerate the development of IPMN and the subsequent transformation into invasive cancer (64). The role of smoking was also reported in a Japanese population, in agreement with our observation in Europeans (65). In pancreatic cancer, the molecular mechanisms of cigarette smoking are currently unknown; however, a synergic role of nicotine metabolites and rare mutations (i.e., KRAS mutations) has been proposed. Higher nicotine retention was observed in some specific organs, including the pancreas, with high nicotine concentrations and its derivatives in pancreatic tissue and pancreatic juice (66–68). In a study by Prokopczyk and colleagues, smokers and non-smokers were compared, reporting clear evidence of higher nicotine derivative accumulation in the pancreatic juice of smokers, which was up to seven times higher than in non-smokers (67). Therefore, the mechanism that induces pancreatic cancer could also be involved in IPMN progression. Exposure to smoking causes the activation of immune cells, which induces the production of proinflammatory factors with consequent recruitment of immune cells, including T cells, macrophages, dendritic cells, and neutrophils. The cascade of immune cell production promoted by cigarette smoking may result in chronic inflammation.
We also observed an increased progression rate in obese patients compared with healthy-weight patients, in agreement with a previous study, which suggests the association of obesity with an increased frequency of malignancy in BD IPMN (69). The association was partially confirmed by the analyses conducted considering the patients who develop new HRSs.
Conversely, the analyses on the patients who developed two or more WFs did not support the association of cigarette smoking and BMI with IPMN progression. These latter analyses might represent the group more likely to progress towards cancer but were conducted on a limited number of subjects, reducing the power to detect an association. The accumulation of body fat has been associated with several diseases, including pancreatic cancer (70). In obese individuals, adipose cells around the pancreas produce an inflammatory environment (71), and the adipocytes associated with pancreatic cancer cells secrete higher than normal adipokines, chemokines, proinflammatory cytokines, and growth factor levels, which may accelerate the neoplastic process (72). All these observations suggest a central role of the chronic inflammatory process, driven by the accumulation of body fat, in the evolution of IPMNs towards pancreatic cancer. Chronic inflammation results in an immunosuppressive tumour microenvironment, which may promote tumour development through dysregulated cell division, fast proliferation, and prolonged cell survival (73, 74).
4.3 Study limitations
A possible limitation of the study is the fact that all patients are under surveillance with no final diagnosis following surgery, and thus malignancy (high grade and invasive) is unknown for most of the individuals. Therefore, the associations that we observed are with clinical progression, and a validation in a set of IPMN patients who underwent resection and for which final pathology has been determined is warranted. Nevertheless, Marchegiani and colleagues showed that patients with at least a WF at diagnosis have an increased probability to evolve into high-grade dysplasia if they acquire additional WFs or HRSs (31). This evidence highlights the importance of identifying markers of the progression of new WFs and HRSs.
4.4 Conclusion
In conclusion, the results of our study show that the 30 established PDAC susceptibility polymorphisms are not associated with clinical IPMN progression in a sample of 354 patients either individually or combined in a score. However, the results suggest proinflammatory habits such as smoking or patient characteristics such as obesity as common factors among PDAC risk and IPMN progression.
Data availability statement
The datasets presented in this article are not readily available because of legal and privacy restrictions. Requests to access the datasets should be directed to the corresponding author.
Ethics statement
The study obtained IRB approval in both the enrolling centres. S. Raffaele Research Hospital: protocol number 133/2016 (for recording clinical information) protocol BIOGASTRO 2011, amendment 6/11/2017 (for blood samples biobanking and for biomarkers investigation). Cisanello Hospital-University of Pisa: the ethical committee was the “Comitato Etico Regionale per la Sperimentazione Clinica della Toscana sezione Area Vasta Nord Ovest”, protocol code “IPMN-PDAC transition”. The patients/participants provided their written informed consent to participate in this study.
Author contributions
DC and GC conceived the study. MG and CC performed experimental work. MG and CC performed data analysis. All other authors contributed to the collection of samples and data. MG and DC drafted the manuscript, and all other authors took part in its critical revision. GC and DC share the last authorship. All authors contributed to the article and approved the submitted version.
Funding
The research leading to these results has received funding from AIRC under IG 2019-ID. 23672 project – P.I. DC, and from AIRC under IG 2021 ID 26201 project - P.I. GC.
Conflict of interest
The authors declare that the research was conducted in the absence of any commercial or financial relationships that could be construed as a potential conflict of interest.
Publisher’s note
All claims expressed in this article are solely those of the authors and do not necessarily represent those of their affiliated organizations, or those of the publisher, the editors and the reviewers. Any product that may be evaluated in this article, or claim that may be made by its manufacturer, is not guaranteed or endorsed by the publisher.
Supplementary material
The Supplementary Material for this article can be found online at: https://www.frontiersin.org/articles/10.3389/fonc.2023.1172606/full#supplementary-material
References
1. Rahib L, Smith BD, Aizenberg R, Rosenzweig AB, Fleshman JM, Matrisian LM. Projecting cancer incidence and deaths to 2030: the unexpected burden of thyroid, liver, and pancreas cancers in the united states. Cancer Res (2014) 74:2913–21. doi: 10.1158/0008-5472.CAN-14-0155
2. Siegel RL, Miller KD, Fuchs HE, Jemal A. Cancer statistics, 2022. CA Cancer J Clin (2022) 72:7–33. doi: 10.3322/caac.21708
3. Kleeff J, Korc M, Apte M, La Vecchia C, Johnson CD, Biankin AV, et al. Pancreatic cancer. Nat Rev Dis Prim (2016) 2:16022. doi: 10.1038/nrdp.2016.22
4. Klein AP. Pancreatic cancer epidemiology: understanding the role of lifestyle and inherited risk factors. Nat Rev Gastroenterol Hepatol (2021) 18:493–502. doi: 10.1038/s41575-021-00457-x
5. Ke T, Lophatananon A, Nieroda M, Kyriazis D, Manias G, Wajid U, et al. Risk factors of pancreatic cancer: a literature review. doi: 10.15761/CRR.1000241
6. Gentiluomo M, Canzian F, Nicolini A, Gemignani F, Landi S, Campa D. Germline genetic variability in pancreatic cancer risk and prognosis. Semin Cancer Biol (2022) 79:105–31. doi: 10.1016/j.semcancer.2020.08.003
7. Corradi C, Lencioni G, Gentiluomo M, Felici A, Latiano A, Kiudelis G, et al. Polymorphic variants involved in methylation regulation: a strategy to discover risk loci for pancreatic ductal adenocarcinoma. J Med Genet (2023) jmg–2022-108910. doi: 10.1136/jmg-2022-108910
8. Giaccherini M, Farinella R, Gentiluomo M, Mohelnikova-Duchonova B, Kauffmann EF, Palmeri M, et al. Association between a polymorphic variant in the CDKN2B-AS1/ANRIL gene and pancreatic cancer risk. Int J Cancer (2022) 153:373–9. doi: 10.1002/ijc.34383
9. Lu Y, Gentiluomo M, Macauda A, Gioffreda D, Gazouli M, Petrone MC, et al. Identification of recessively inherited genetic variants potentially linked to pancreatic cancer risk. Front Oncol (2021) 11:771312. doi: 10.3389/FONC.2021.771312
10. Lu Y, Corradi C, Gentiluomo M, López de Maturana E, Theodoropoulos GE, Roth S, et al. Association of genetic variants affecting microRNAs and pancreatic cancer risk. Front Genet (2021) 12:693933. doi: 10.3389/fgene.2021.693933
11. Campa D, Rizzato C, Stolzenberg-Solomon R, Pacetti P, Vodicka P, Cleary SP, et al. TERT gene harbors multiple variants associated with pancreatic cancer susceptibility. Int J Cancer (2015) 137:2175–83. doi: 10.1002/ijc.29590
12. Childs EJ, Mocci E, Campa D, Bracci PM, Gallinger S, Goggins M, et al. Common variation at 2p13.3, 3q29, 7p13 and 17q25.1 associated with susceptibility to pancreatic cancer. Nat Genet (2015) 47:911–6. doi: 10.1038/ng.3341
13. Campa D, Gentiluomo M, Stein A, Aoki MN, Oliverius M, Vodičková L, et al. The PANcreatic disease ReseArch (PANDoRA) consortium: ten years’ experience of association studies to understand the genetic architecture of pancreatic cancer. Crit Rev Oncol Hematol (2023), 104020. doi: 10.1016/J.CRITREVONC.2023.104020
14. Campa D, Rizzato C, Capurso G, Giese N, Funel N, Greenhalf W, et al. Genetic susceptibility to pancreatic cancer and its functional characterisation: the PANcreatic disease ReseArch (PANDoRA) consortium. Dig Liver Dis (2013) 45:95–9. doi: 10.1016/j.dld.2012.09.014
15. Crippa S, Arcidiacono PG, De Cobelli F, Falconi M. Review of the diagnosis and management of intraductal papillary mucinous neoplasms. United Eur Gastroenterol J (2020) 8:249–55. doi: 10.1177/2050640619894767
16. Kent TS. Intraductal papillary mucinous neoplasm and the pancreatic incidentaloma. World J Gastrointest Surg (2010) 2:319. doi: 10.4240/wjgs.v2.i10.319
17. Lee KS, Sekhar A, Rofsky NM, Pedrosa I. Prevalence of incidental pancreatic cysts in the adult population on MR imaging. Am J Gastroenterol (2010) 105:2079–84. doi: 10.1038/AJG.2010.122
18. Laffan TA, Horton KM, Klein AP, Berlanstein B, Siegelman SS, Kawamoto S, et al. Prevalence of unsuspected pancreatic cysts on MDCT. AJR Am J Roentgenol (2008) 191:802. doi: 10.2214/AJR.07.3340
19. Baiocchi GL, Portolani N, Grazioli L, Mazza G, Gheza F, Bartoli M, et al. Management of pancreatic intraductal papillary mucinous neoplasm in an academic hospital (2005-2010): what follow-up for unoperated patients? Pancreas (2013) 42:696–700. doi: 10.1097/MPA.0B013E318270B98B
20. Laurent L, Vullierme MP, Rebours V, Maire F, Hentic O, Francoz C, et al. Estimation of the prevalence of intraductal papillary mucinous neoplasm of the pancreas in the French population through patients waiting for liver transplantation. United Eur Gastroenterol J (2017) 5:499–503. doi: 10.1177/2050640616664842
21. Ricci C, Migliori M, Imbrogno A, Mazzotta E, Felicani C, Serra C, et al. Prevalence of asymptomatic intraductal papillary mucinous neoplasms in healthy and ill populations detected by ultrasonography: a single-center study of 6353 outpatients. Pancreas (2019) 48:113–20. doi: 10.1097/MPA.0000000000001205
22. Latenstein AEJ, Mackay TM, Beane JD, Busch OR, van Dieren S, Gleeson EM, et al. The use and clinical outcome of total pancreatectomy in the united states, Germany, the Netherlands, and Sweden. Surgery (2021) 170:563–70. doi: 10.1016/j.surg.2021.02.001
23. Lewis R, Drebin JA, Callery MP, Fraker D, Kent TS, Gates J, et al. A contemporary analysis of survival for resected pancreatic ductal adenocarcinoma. HPB (2013) 15:49–60. doi: 10.1111/J.1477-2574.2012.00571.X
24. Waters JA, Schnelldorfer T, Aguilar-Saavedra JR, Chen JH, Yiannoutsos CT, Lillemoe KD, et al. Survival after resection for invasive intraductal papillary mucinous neoplasm and for pancreatic adenocarcinoma: a multi-institutional comparison according to American joint committee on cancer stage. J Am Coll Surg (2011) 213:275–83. doi: 10.1016/J.JAMCOLLSURG.2011.04.003
25. Balduzzi A, Marchegiani G, Pollini T, Biancotto M, Caravati A, Stigliani E, et al. Systematic review and meta-analysis of observational studies on BD-IPMNS progression to malignancy. Pancreatology (2021) 21:1135–45. doi: 10.1016/j.pan.2021.04.009
26. Sahora K, Mino-Kenudson M, Brugge W, Thayer SP, Ferrone CR, Sahani D, et al. Branch duct intraductal papillary mucinous neoplasms: does cyst size change the tip of the scale? a critical analysis of the revised international consensus guidelines in a large single-institutional series. Ann Surg (2013) 258:466–74. doi: 10.1097/SLA.0B013E3182A18F48
27. Sahora K, Fern Andez-Del Castillo C, Dong F, Marchegiani G, Thayer SP, Ferrone CR, et al. Not all mixed-type intraductal papillary mucinous neoplasms behave like main-duct lesions: implications of minimal involvement of the main pancreatic duct. Surg (United States) (2014) 156:661–21. doi: 10.1016/j.surg.2014.04.023
28. Sugiyama M, Suzuki Y. “Natural History of IPMN: Adenoma-Carcinoma Sequence in IPMN.,” Pancreatic Cancer, Cystic Neoplasms and Endocrine Tumors: Diagnosis and Management. John Wiley & Sons, Ltd (2015). p. 225–8. doi: 10.1002/9781118307816.ch30
29. Oyama H, Tada M, Takagi K, Tateishi K, Hamada T, Nakai Y, et al. Long-term risk of malignancy in branch-duct intraductal papillary mucinous neoplasms. Gastroenterology (2020) 158:226–237.e5. doi: 10.1053/J.GASTRO.2019.08.032
30. Tanaka M, Fernández-Del Castillo C, Kamisawa T, Jang JY, Levy P, Ohtsuka T, et al. Revisions of international consensus Fukuoka guidelines for the management of IPMN of the pancreas. Pancreatology (2017) 17:738–53. doi: 10.1016/j.pan.2017.07.007
31. Marchegiani G, Pollini T, Andrianello S, Tomasoni G, Biancotto M, Javed AA, et al. Progression vs cyst stability of branch-duct intraductal papillary mucinous neoplasms after observation and surgery. JAMA Surg (2021) 156:654–61. doi: 10.1001/JAMASURG.2021.1802
32. Ardeshna DR, Rangwani S, Cao T, Pawlik TM, Stanich PP, Krishna SG. Intraductal papillary mucinous neoplasms in hereditary cancer syndromes. Biomedicines (2022) 10(7):1475. doi: 10.3390/biomedicines10071475
33. Giaccherini M, Gentiluomo M, Arcidiacono PG, Falconi M, Testoni SGG, Apadula L, et al. A polymorphic variant in telomere maintenance is associated with worrisome features and high-risk stigmata development in IPMNs. Carcinogenesis (2022) 43:728–35. doi: 10.1093/carcin/bgac051
34. Capurso G, Crippa S, Vanella G, Traini M, Zerboni G, Zaccari P, et al. Factors associated with the risk of progression of low-risk branch-duct intraductal papillary mucinous neoplasms. JAMA Netw Open (2020) 3:e2022933. doi: 10.1001/JAMANETWORKOPEN.2020.22933
35. Overbeek KA, van Leeuwen N, Tacelli M, Anwar MS, Yousaf MN, Chhoda A, et al. International external validation of a stratification tool to identify branch-duct intraductal papillary mucinous neoplasms at lowest risk of progression. United Eur Gastroenterol J (2022) 10:169–78. doi: 10.1002/UEG2.12207
36. Capurso G, Boccia S, Salvia R, Del Chiaro M, Frulloni L, Arcidiacono PG, et al. Risk factors for intraductal papillary mucinous neoplasm (ipmn) of the pancreas: a multicentre case-control study. Am J Gastroenterol (2013) 108:1003–9. doi: 10.1038/ajg.2013.42
37. Klein AP, Wolpin BM, Risch HA, Stolzenberg-Solomon RZ, Mocci E, Zhang M, et al. Genome-wide meta-analysis identifies five new susceptibility loci for pancreatic cancer. Nat Commun (2018) 9:556. doi: 10.1038/s41467-018-02942-5
38. Zhang M, Wang Z, Obazee O, Jia J, Childs EJ, Hoskins J, et al. Three new pancreatic cancer susceptibility signals identified on chromosomes 1q32.1, 5p15.33 and 8q24.21. Oncotarget (2016) 7:66328–43. doi: 10.18632/oncotarget.11041
39. Wolpin BM, Rizzato C, Kraft P, Kooperberg C, Petersen GM, Wang Z, et al. Genome-wide association study identifies multiple susceptibility loci for pancreatic cancer. Nat Genet (2014) 46:994–1000. doi: 10.1038/ng.3052
40. Petersen GM, Amundadottir L, Fuchs CS, Kraft P, Stolzenberg-Solomon RZ, Jacobs KB, et al. A genome-wide association study identifies pancreatic cancer susceptibility loci on chromosomes 13q22.1, 1q32.1 and 5p15.33. Nat Genet (2010) 42:224–8. doi: 10.1038/ng.522
41. Amundadottir L, Kraft P, Stolzenberg-Solomon RZ, Fuchs CS, Petersen GM, Arslan AA, et al. Genome-wide association study identifies variants in the ABO locus associated with susceptibility to pancreatic cancer. Nat Genet (2009) 41:986–90. doi: 10.1038/ng.429
42. Pistoni L, Gentiluomo M, Lu Y, López de Maturana E, Hlavac V, Vanella G, et al. Associations between pancreatic expression quantitative traits and risk of pancreatic ductal adenocarcinoma. Carcinogenesis (2021) 42:1037–45. doi: 10.1093/carcin/bgab057
43. Corradi C, Gentiluomo M, Gajdán L, Cavestro GM, Kreivenaite E, Di Franco G, et al. Genome-wide scan of long noncoding RNA single nucleotide polymorphisms and pancreatic cancer susceptibility. Int J Cancer (2021) 148:2779–88. doi: 10.1002/ijc.33475
44. Rizzato C, Campa D, Pezzilli R, Soucek P, Greenhalf W, Capurso G, et al. ABO blood groups and pancreatic cancer risk and survival: results from the PANcreatic disease ReseArch (PANDoRA) consortium. Oncol Rep (2013) 29:1637–44. doi: 10.3892/OR.2013.2285
45. Galeotti AA, Gentiluomo M, Rizzato C, Obazee O, Neoptolemos JP, Pasquali C, et al. Polygenic and multifactorial scores for pancreatic ductal adenocarcinoma risk prediction. J Med Genet (2021) 58:369–77. doi: 10.1136/jmedgenet-2020-106961
46. Nodari Y, Gentiluomo M, Mohelnikova-Duchonova B, Kreivenaite E, Milanetto AC, Skieceviciene J, et al. Genetic and non-genetic risk factors for early-onset pancreatic cancer. Dig Liver Dis (2023). doi: 10.1016/j.dld.2023.02.023
47. Rodriguez JR, Salvia R, Crippa S, Warshaw AL, Bassi C, Falconi M, et al. Branch-duct intraductal papillary mucinous neoplasms: observations in 145 patients who underwent resection. Gastroenterology (2007) 133:72–9. doi: 10.1053/j.gastro.2007.05.010
48. Sohn TA, Yeo CJ, Cameron JL, Hruban RH, Fukushima N, Campbell KA, et al. Intraductal papillary mucinous neoplasms of the pancreas: an updated experience. Ann Surg (2004) 239:788–97. doi: 10.1097/01.sla.0000128306.90650.aa
49. Salvia R, Fernández-del Castillo C, Bassi C, Thayer SP, Falconi M, Mantovani W, et al. Main-duct intraductal papillary mucinous neoplasms of the pancreas: clinical predictors of malignancy and long-term survival following resection. Ann Surg (2004) 239:678–85. doi: 10.1097/01.sla.0000124386.54496.15
50. Schmidt CM, White PB, Waters JA, Yiannoutsos CT, Cummings OW, Baker M, et al. Intraductal papillary mucinous neoplasms: predictors of malignant and invasive pathology. Ann Surg (2007) 246:644–51. doi: 10.1097/SLA.0b013e318155a9e5
51. Xue A, Wu Y, Zhu Z, Zhang F, Kemper KE, Zheng Z, et al. Genome-wide association analyses identify 143 risk variants and putative regulatory mechanisms for type 2 diabetes. Nat Commun (2018) 9:2941. doi: 10.1038/s41467-018-04951-w
52. Dönertaş HM, Fabian DK, Fuentealba M, Partridge L, Thornton JM. Common genetic associations between age-related diseases. Nat Aging (2021) 1:400–12. doi: 10.1038/s43587-021-00051-5
53. Locke AE, Kahali B, Berndt SI, Justice AE, Pers TH, Day FR, et al. Genetic studies of body mass index yield new insights for obesity biology. Nature (2015) 518:197–206. doi: 10.1038/nature14177
54. Liu Y, Basty N, Whitcher B, Bell JD, Sorokin EP, van Bruggen N, et al. Genetic architecture of 11 organ traits derived from abdominal MRI using deep learning. Elife (2021) 10:e65554. doi: 10.7554/eLife.65554
55. Schulze A, Oshi M, Endo I, Takabe K. Myc targets scores are associated with cancer aggressiveness and poor survival in er-positive primary and metastatic breast cancer. Int J Mol Sci (2020) 21:1–13. doi: 10.3390/ijms21218127
56. Marinkovic T, Marinkovic D. Obscure involvement of MYC in neurodegenerative diseases and neuronal repair. Mol Neurobiol (2021) 58:4169–77. doi: 10.1007/s12035-021-02406-w
57. Gajzer D, Logothetis CN, Sallman DA, Calon G, Babu A, Chan O, et al. MYC overexpression is associated with an early disease progression from MDS to AML. Leuk Res (2021) 111:106733. doi: 10.1016/j.leukres.2021.106733
58. Pallotta MM, Di Nardo M, Sarogni P, Krantz ID, Musio A. Disease-associated c-MYC downregulation in human disorders of transcriptional regulation. Hum Mol Genet (2022) 31:1599–609. doi: 10.1093/hmg/ddab348
59. Hasenan N, Isa SAM, Hussain FA. Association between c-myc expression with clinicopathological features in T and NK cell lymphomas. Asian Pacific J Cancer Prev (2021) 22:4011–6. doi: 10.31557/APJCP.2021.22.12.4011
60. Cao L, Ren C, Zhang G, Li X, Chen B, Li K, et al. Characteristics of MYC amplification and their association with clinicopathological and molecular factors in patients with breast cancer. DNA Cell Biol (2022) 41:521–38. doi: 10.1089/dna.2020.6487
61. Poruk KE, Griffin J, Makary MA, He J, Cameron JL, Weiss MJ, et al. Blood type as a predictor of high-grade dysplasia and associated malignancy in patients with intraductal papillary mucinous neoplasms. J Gastrointest Surg (2019) 23:477–83. doi: 10.1007/S11605-018-3795-9
62. Hamada T, Oyama H, Nakai Y, Tada M, Koh H, Tateishi K, et al. ABO blood group and risk of pancreatic carcinogenesis in intraductal papillary mucinous neoplasms. Cancer Epidemiol Biomarkers Prev (2021) 30:102–1028. doi: 10.1158/1055-9965.EPI-20-1581
63. Zelga P, Hernández-Barco YG, Qadan M, Ferrone CR, Baba T, Bolm L, et al. ABO blood group distribution and risk of malignancy in patients undergoing resection for intraductal papillary mucinous neoplasm (IPMN). Pancreatology (2022) 22:264–9. doi: 10.1016/J.PAN.2021.12.012
64. Carr RA, Roch AM, Shaffer K, Aboudi S, Schmidt CM, DeWitt J, et al. Smoking and IPMN malignant progression. Am J Surg (2017) 213:494–7. doi: 10.1016/j.amjsurg.2016.10.033
65. Fukushima G, Abe K, Kitago M, Iwasaki E, Hirata A, Takemura R, et al. Association between clinical backgrounds and malignant progression of suspected intraductal papillary mucinous neoplasm. Pancreas (2022) 51:617–23. doi: 10.1097/MPA.0000000000002064
66. Chowdhury P, Doi R, Chang LW, Rayford PL. Tissue distribution of [3H]-nicotine in rats. BioMed Environ Sci (1993) 6:59–64.
67. Prokopczyk B, Hoffmann D, Bologna M, Cunningham AJ, Trushin N, Akerkar S, et al. Identification of tobacco-derived compounds in human pancreatic juice. Chem Res Toxicol (2002) 15:677–85. doi: 10.1021/TX0101088/ASSET/IMAGES/LARGE/TX0101088F00005.JPEG
68. Pandol SJ, Apte MV, Wilson JS, Gukovskaya AS, Edderkaoui M. The burning question: why is smoking a risk factor for pancreatic cancer? Pancreatology (2012) 12:344–9. doi: 10.1016/j.pan.2012.06.002
69. Schmidt CM, Sturm EC, Roch AM, Shaffer KM, Schmidt CM, Lee SJ, et al. Obesity increases malignant risk in patients with branch-duct intraductal papillary mucinous neoplasm. Surgery (2013) 154:803–9. doi: 10.1016/J.SURG.2013.07.011
70. Zhou B, Wu D, Liu H, Du L, Wang Y, Xu J, et al. Obesity and pancreatic cancer: an update of epidemiological evidence and molecular mechanisms. Pancreatology (2019) 19:941–50. doi: 10.1016/j.pan.2019.08.008
71. Incio J, Liu H, Suboj P, Chin SM, Chen IX, Pinter M, et al. Obesity-induced inflammation and desmoplasia promote pancreatic cancer progression and resistance to chemotherapy. Cancer Discovery (2016) 6:852–69. doi: 10.1158/2159-8290.CD-15-1177
72. Hertzer KM, Xu M, Moro A, Dawson DW, Du L, Li G, et al. Robust early inflammation of the peripancreatic visceral adipose tissue during diet-induced obesity in the KrasG12D model of pancreatic cancer. Pancreas (2016) 45:458–65. doi: 10.1097/MPA.0000000000000497
73. Mantovani A, Allavena P, Sica A, Balkwill F. Cancer-related inflammation. J Exp Med (2008) 454:435–44. doi: 10.1038/nature07205
Keywords: pancreatic ductal adenocarcinoma (PADC), intraductal papillary mucinous neoplasms (IPMN), single nucleotide polymorphism (SNP), association study, polygenic score (PGS)
Citation: Gentiluomo M, Corradi C, Arcidiacono PG, Crippa S, Falconi M, Belfiori G, Farinella R, Apadula L, Lauri G, Bina N, Rizzato C, Canzian F, Morelli L, Capurso G and Campa D (2023) Role of pancreatic ductal adenocarcinoma risk factors in intraductal papillary mucinous neoplasm progression. Front. Oncol. 13:1172606. doi: 10.3389/fonc.2023.1172606
Received: 23 February 2023; Accepted: 16 May 2023;
Published: 06 June 2023.
Edited by:
Michele Yip-Schneider, Indiana University Bloomington, United StatesReviewed by:
Rick Jansen, University of Minnesota Twin Cities, United StatesKazumichi Kawakubo, Hokkaido University Hospital, Japan
Copyright © 2023 Gentiluomo, Corradi, Arcidiacono, Crippa, Falconi, Belfiori, Farinella, Apadula, Lauri, Bina, Rizzato, Canzian, Morelli, Capurso and Campa. This is an open-access article distributed under the terms of the Creative Commons Attribution License (CC BY). The use, distribution or reproduction in other forums is permitted, provided the original author(s) and the copyright owner(s) are credited and that the original publication in this journal is cited, in accordance with accepted academic practice. No use, distribution or reproduction is permitted which does not comply with these terms.
*Correspondence: Daniele Campa, ZGFuaWVsZS5jYW1wYUB1bmlwaS5pdA==
†These authors share first authorship
‡These authors share last authorship