- Department of Physiology and Pathophysiology, School of Basic Medical Sciences, Cheeloo College of Medicine, Shandong University, Jinan, Shandong, China
Extrahepatic cholangiocarcinoma (eCCA) contains perihilar cholangiocarcinoma and distal cholangiocarcinoma both of which can arise at any point of the biliary tree and originate from disparate anatomical sites. Generally, the incidence of eCCA is increasing globally. Though surgical resection is the principal treatment of choice for the early stages of eCCA, optimal survival remains restricted by the high risk of recurrence when most patients are present with unresectable disease or distant metastasis. Furthermore, both intra- and intertumoral heterogeneity make it laborious to determine molecularly targeted therapies. In this review, we mainly focused on current findings in the field of eCCA, mostly including epidemiology, genomic abnormalities, molecular pathogenesis, tumor microenvironment, and other details while a summary of the biological mechanisms driving eCCA may shed light on intricate tumorigenesis and feasible treatment strategies.
1 Introduction
Cholangiocarcinoma (CCA) usually refers to a range of invasive adenocarcinomas including intrahepatic cholangiocarcinoma (iCCA), perihilar cholangiocarcinoma (pCCA) and distal cholangiocarcinoma (dCCA) based on dissimilarly anatomical locations while the latter two are also collectively termed as extrahepatic cholangiocarcinoma (eCCA). Anatomically, pCCA and dCCA can be discriminated by whether the tumor originates between the second-order ducts and the insertion of the cystic duct or from epithelium distal to the insertion of the cystic duct whereas dCCA implicates the common bile duct typically (1). Moreover, pCCA and dCCA also diverge in pathogenesis, cells of origin, genome aberrations, molecular profiles, and risk factors. Although distinct from iCCA, eCCA should be cautiously termed to cover pCCA and dCCA due to the ambiguous origins of pCCA (2). Histologically, pCCA and dCCA are mainly common mucin-producing adenocarcinomas or papillary tumors, unlike more heterogeneous iCCA which can be classified into perihilar large duct type and peripheral small duct type with S100P and SPP1 expressed, respectively, in term of the size or level of the bile duct affected by malignant cells (3–5). Interestingly, the perihilar large duct type of iCCA is more similar to pCCA and dCCA whereas those subtypes can derive from columnar mucin-producing cholangiocytes or peribiliary glands (4). In term of patterns of growth, iCCA tends to be mass-forming while its large duct type and eCCA can be periductal infiltrating or intraductal growing. Besides, several precancerous lesions including mucinous, cystic neoplasm, biliary epithelial neoplasia, intraductal tubulopapillary neoplasm and intraductal papillary neoplasm of the bile duct can be related to iCCA large duct type and eCCA, not iCCA small duct type (4). Furthermore, viral and cirrhosis are usually underlying in iCCA whereas cholangitis and liver flukes are more common in eCCA. Regarding frequent mutations, IDH1 mutations and FGFR2 fusions with targeted drugs are more frequent in iCCA but nearly absent in eCCA which may be inclined to ERBB alterations (4). eCCA is a rare cancer, but its incidence and mortality have been increasing which menace human health severely (6). Regarding the treatment of eCCA, surgical resection with negative margins is the curative and available treatment strategy for patients present with the early-stage or resectable disease when recurrence is still prevalent (7). Moreover, multidisciplinary treatment of advanced eCCA is also crucial. For instance, adjuvant therapy with S-1 encompassing a mixture of tegafu, gimeracil, and oteracil potassium could improve survival among patients with CCA resected according to a phase 3 randomized clinical trial (8). However, effective molecularly targeted therapy for eCCA is still an urgent enigma to be unveiled.
Here, we summarize current advances in the oncogenic mechanisms and treatment strategies of eCCA, mainly concerning epidemiology, genomic abnormalities, molecular pathogenesis, tumor microenvironment, and other pertinent details to provide a comprehensive panorama of eCCA and highlight the importance of personalized and multidisciplinary considerations.
2 Epidemiology and risk factors, past and current
The global Incidence of eCCA increased worldwide during the period 1993-2012 spanning two decades according to the CI5plus database for 33 inclusive countries (9). More accurately, the age-standardized incidence for eCCA indeed increased with geographical variation and most evidently in Thailand and Colombia in the 20 years examined. Mortality rates for eCCA have also increased, but more slowly than iCCA in Western countries (6). Summarizing gallbladder carcinoma and other biliary carcinomas including eCCA, an estimated 12,130 new cases, and 4,400 deaths were reported in the United States, in 2022 with a minute difference by gender (10). However, it was also reported that the age-standardized incidence of eCCA has been descending over the past few decades (11, 12). Of note, these trends need conservative assessment given that International Classification of Diseases (ICD) codes for cholangiocarcinoma have been updated several times. Separate codes for iCCA, pCCA, and dCCA were not available until the new ICD-11 classification came into effect which may influence epidemiological estimation (13). Thus, epidemiological trends reported for eCCA need to be evaluated meticulously whereas data is more reliable when ample and new. In addition, pCCA and dCCA have different prognoses and distinctive epidemiological trends. Surveillance, Epidemiology, and End Results (SEER) database have shown better survival in dCCA when compared with pCCA from 2000 to 2018 (14). Regarding dCCA, a recent Swedish cohort study disclosed that incidence rates elevated principally among those patients aged more than 55 during the consecutive calendar periods. Contrastively, the increase in both intrahepatic and perihilar cholangiocarcinoma was more evident in younger adults (15).
In general, several common risk factors including obesity, alcohol consumption, and cigarette smoking could be linked to eCCA (16). Furthermore, metabolic diseases, such as type 2 diabetes, nonalcoholic fatty liver disease, and hypertension are also risk factors for eCCA which are also shared by iCCA (17, 18). Remarkably, dose-dependent alcohol consumption increased the risk of CCA for patients with prediabetes and diabetes, but not normoglycemic, which indicated a synergistic effect, and alcohol abstinence might humiliate the risk of CCA for those patients (19). A large pan-European cohort showed that pCCA was featured with primary sclerosing cholangitis (PSC) and dCCA with choledocholithiasis (20). Though viral infections including hepatitis B virus and hepatitis C virus have been associated with incremental CCA risk previously, they seem to influence iCCA mainly, not eCCA in Europe while a similar situation could be adequate for primary biliary cholangitis (16, 20). Several studies also evaluated the role of drugs such as statins and aspirin in the prevention of eCCA. Statin usage has been noticed to be associated with a reduced risk for eCCA whose users with dCCA had better overall survival than statin-free patients (HR=0.53) (21). Notably, multiple cohorts have revealed that aspirin was associated with a decreased risk of CCA (22, 23). Even so, low-dose aspirin was not associated with eCCA risk significantly but non-steroidal anti-inflammatory drugs with aspirin excluded could increase the risk of eCCA (HR=1.32) as reported by Marcano-Bonilla L et al. (24). Besides, proton pump inhibitors with extended duration may also increase eCCA risk (25). Those evidence indicated that drug usage should be cautious for patients with eCCA.
3 Clinical symptoms and diagnosis, early to arise
eCCA can be asymptomatic or non-specific during early stages which makes it tough to diagnose early. The most common symptom of eCCA is obstructive jaundice whereas it is less frequent in iCCA (26). Besides, some constitutional symptoms such as fatigue, anorexia, weight loss, and abdominal pain could be noticed in patients with either benign or malignant diseases (27). Generally, diagnosis of eCCA can benefit from imaging, endoscopy and histology. Imaging techniques including CT and MRI are important for diagnosis and staging of CCA. Owing to direct compression, dCCA shows abrupt biliary tree cutoff from CT scanning while pCCA can be obvious only when dilated segmental bile ducts emerge (28). MRI can delineate the biliary tree with its lesions in detail and allow accurate ducts depicted by magnetic resonance cholangiopancreatography (MRCP) which is critical for the diagnosis, staging, and treatment planning of pCCA (29). MRI illustrates CCA as hypointense lesions and heterogeneously hyperintense lesions on T1-weighted images and T2-weighted images, respectively (30). Remarkably, Endoscopic retrograde cholangiopancreatography (ERCP) is a robust mode for the biliary tree assessment and acquirement of brush cytology and biopsies with high specificity but low sensitivity (31). In addition to the primary modalities including MRCP and ERCP, endoscopic ultrasound (EUS) can be complemental and helpful for the evaluation of biliary strictures and assessment of eCCA or regional lymph nodes (32). It also allows tissue acquisition via needle aspiration and may detect small bile duct masses (33). Furthermore, cholangioscopy covering bile duct mucosa and targeted biopsies could enhance the diagnostic accuracy of malignant biliary strictures (34). Recently, Ishii T et al. reported that cholangioscopy enhanced by image systems is very useful for diagnosing eCCA (35). Histologically, eCCA can be flat, nodular sclerosing, or intraductal papillary type whose growth patterns are periductal infiltrating or intraductal growing. eCCA derives from mucous cells and/or columnar cholangiocytes which also concern precancerous lesions including intraductal epithelial neoplasia. Several tissue markers such as MUC5AC, MUC6, S100P, and BAP1 contribute to differentiating eCCA from diverse CCA types (4). In total, early diagnosis is still challenging for eCCA and a combination of clinical, imaging, endoscopy and histologic data is usually necessary.
4 Surgical resection and adjuvant therapy, two rocks and one bird
Surgical resection maintains a momentous tactic for pCCA and dCCA therapy while adequate assessment and preoperative consideration are necessary to be priorly executed which restricts candidates for curative-intent surgical resection therapy (36, 37).
Generally speaking, pancreaticoduodenectomy and lymphadenectomy are involved in surgery for dCCA (Table 1). Achieving a margin negative (R0) resection is crucial for dCCA and pCCA management while negative margin assessment and complete resection may benefit from the intraoperative frozen section (43). Curative and eligible surgical resections for eCCA patients depend on multiple clinical conditions. A study based on a cohort in the Netherlands determined an overall survival predictive model for patients after pancreatoduodenectomy for dCCA. Five independent prognostic factors covering age at diagnosis, pT category, pN category, resection margin status, and tumor differentiation were included in the model which was also robust for inferring prognosis (44). Furthermore, both tumor budding and tumor invasive thickness were associated with adverse postoperative prognosis in eCCA (45, 46). Interestingly, nerve fiber density invaded by tumors could be related to unfavorable outcomes of pCCA patients undergoing curative-intent surgery (47). Regarding preoperative evaluation, preoperative biliary drainage is still debated but needed when obstructive symptoms are present for eCCA patients whereas endoscopic biliary drainage seems to be more suitable for dCCA than percutaneous transhepatic biliary drainage which had lower rates of complications for pCCA (48–50). Moreover, laboratory assessment on peripheral blood revealed that neutrophil count, fibrinogen-to-lymphocyte ratio (FLR), and FLR-neutrophil score could predict the prognosis of patients with resected eCCA (51).
Historically, adjuvant therapy after curative resection of biliary tract cancer is commendatory whose decisions need to be based on adequate and robust data from clinical trials. Previously, no difference was settled between the gemcitabine adjuvant chemotherapy group and the control group in eCCA patients who underwent curative resection from a randomized phase 3 trial (52). Recently, another randomized phase 3 trial confirmed adjuvant therapy with S-1 (a mixture of tegafu, gimeracil, and oteracil) could improve survival among patients with resected eCCA, iCCA, gallbladder carcinoma (GBC), and ampullary carcinoma involved versus surgery alone (8). A prospective study (SWOG 0809) focusing on adjuvant chemotherapy (gemcitabine and capecitabine) followed by chemoradiation in patients with eCCA and GBC showed that adjuvant therapy could benefit patients with lymph node-positive (53). Similarly, adjuvant therapy could improve the long-term survival of patients with perineural invasion and lymph node metastasis after curative-intent resection for dCCA (38). Although phase 3 studies evaluating adjuvant radiotherapy are lacking, there are shreds of evidence that adjuvant radiotherapy should be considered for patients after resection of dCCA (39). To sum up, the role of neoadjuvant and adjuvant therapies for eCCA should be optimized with more comprehensive investigations (Table 2).
For patients with unresectable disease, the FDA has approved pembrolizumab for patients with unresectable or metastatic microsatellite instability-high or mismatch repair deficient solid tumors (including CCA) (37). However, as shown in results from the KEYNOTE-158 and KEYNOTE-028 studies, pembrolizumab treatment achieved a low objective response rate of 6–13% and an inferior survival of less than 2 months in patients (61). Remarkably, liver transplantation (LT) is a therapeutic option in patients with unresectable malignant tumors including CCA (37). However, early experience showed high recurrence rates with transplant (64). Despite poor outcomes after LT for CCA, recent studies have fluctuated this premise since neoadjuvant therapy including chemotherapy and/or radiotherapy followed by liver transplantation offers a potentially curative strategy for patients with unresectable disease (65). For instance, a recent meta-analysis indicated that LT with neoadjuvant chemoradiation completed achieved higher overall survival rates than LT alone in patients with unresectable pCCA (82.8%, 65.5%, and 65.7% at 1 year, 3 years, and 5 years, respectively, vs. 71.2%, 48%, and 31.6%, respectively; p < 0.001) (66). It further supports the curative possibility of neoadjuvant chemoradiation therapy followed by liver transplantation for unresectable CCA patients.
Regarding the management of complications including obstructive jaundice and biliary infection for unresectable eCCA, endoscopic biliary stent placement is effective partially, but limited in improving the overall survival of patients (67). Endoscopic radiofrequency ablation (RFA) has been an accessible technique for alleviating malignant biliary stenosis since first reported (40), although it may be inclined to treat patients without distant metastasis (68, 69) (Table 1). Several randomized controlled trials showed that additional endoscopic RFA could improve the overall survival of patients with unresectable eCCA than those with stent placement alone (54, 55). Furthermore, endoscopic RFA combined with S-1 administered orally for unresectable eCCA patients achieved significantly longer survival (16 months vs. 11 months, p<0.01) and stent patency time (6.6 months vs. 5.6 months, p=0.014) than RFA sole (56). Evidence from retrospective studies also indicated that patients with locally advanced eCCA could benefit from the combination of endobiliary RFA and gemcitabine plus cisplatin treatments (70, 71).
5 Tumor microenvironment of eCCA, no cell is alone
The tumor microenvironment (TME) is composed of diverse cellular types and extracellular components, supporting and maintaining tumor progression while deciphering the complexity of TME is more feasible in the single-cell era (72).
Among innate immune cells, activated M2 macrophages induce tumor progression with anti-inflammatory and immunosuppressive effects which could stimulate the canonical Wnt/b-catenin pathway driving CCA growth (73). A high density of tumor-associated macrophages was associated with incremental recurrence of pCCA in a retrospective study (74). Furthermore, elevated PD-L1+ M2 tumor-associated macrophages (CD45+ CSF1R+ CD68+ CD163+) also correlated with inferior outcomes in dCCA and higher expression of IL6, IL10, and ARG1, contributing to effector T cell suppression (Figure 1) (75). Though natural killer (NK) cells may comprise a considerable proportion across immune ingredients of eCCA and seem to be lower in tumors compared to para-tumor tissues and peripheral blood (76), they were insufficiently studied in eCCA. A previous study reported that a mouse xenograft model induced by HuCCT-1 cells, an iCCA cell line, and then infused with ex vivo expanded human NK cells showed significant tumor inhibition (77). Tumor-associated neutrophils (TANs, CD66b+) could predict poor prognosis in eCCA patients (78). Similarly, the systemic immune-inflammation index calculated by neutrophil, platelet, and lymphocyte counts from serum was an independent prognostic factor for patients under resection of eCCA (79). Interestingly, neutrophils recruited by tumor-cell-derived microvesicles loading methotrexate and subsequent macrophage repolarization could alleviate biliary obstructions of patients with eCCA and execute tumor cells with reactive oxygen species and nitric oxide levels elevated, displaying an antitumor N1 phenotype (80). However, neutrophils heterogeneity in eCCA is still poorly understood. Remarkably, immunosuppressive functions including recruiting macrophages and suppressing T cell cytotoxicity of TANs have been elucidated adequately in liver cancer at the single cell resolution recently (81).
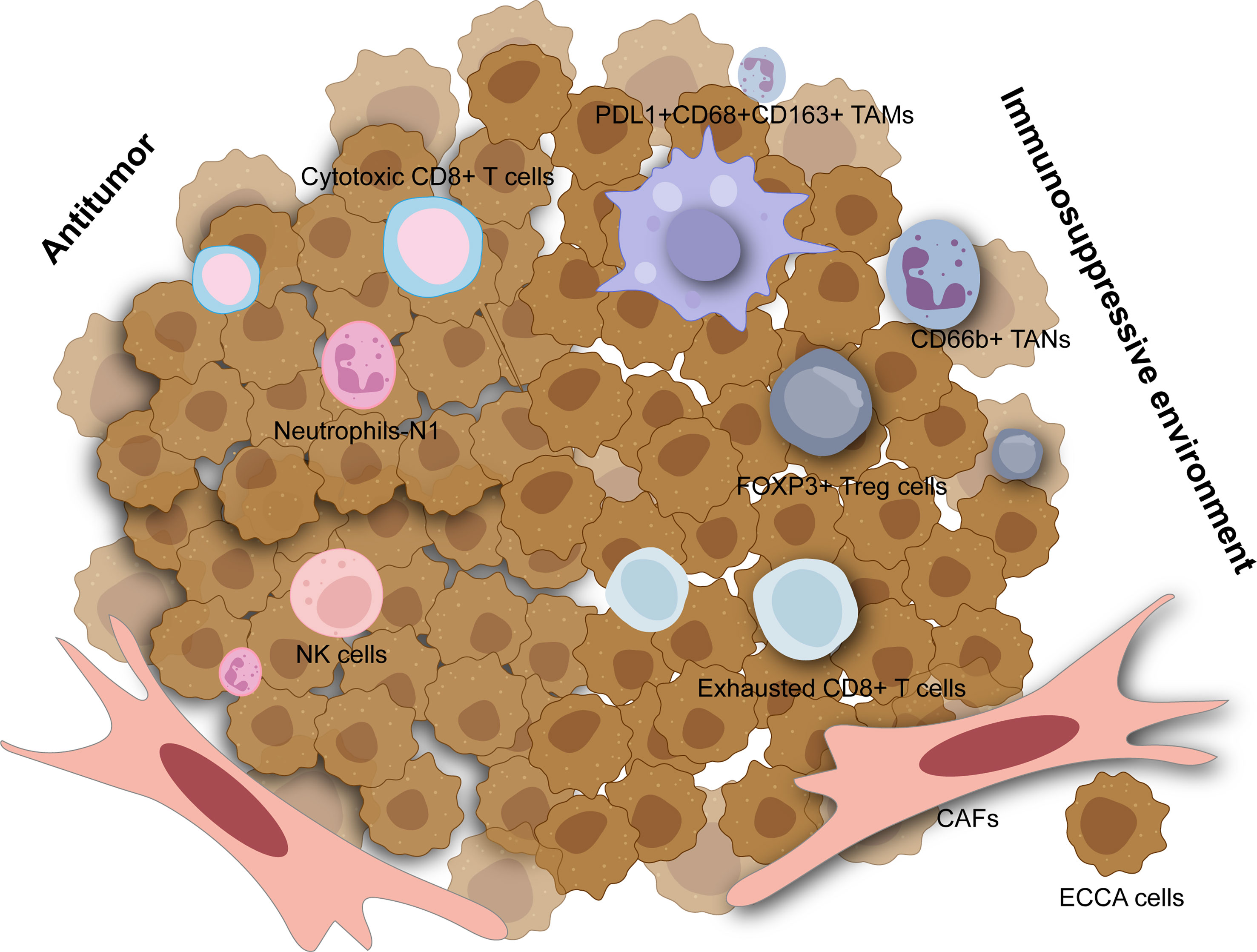
Figure 1 The tumor microenvironment in extrahepatic cholangiocarcinoma. The immunosuppressive environment is mainly composed of tumor-associated macrophage (TAMs), tumor-associated neutrophils (TANs), regulatory T cells (Tregs), exhausted CD8+ T cells and cancer-associated fibroblasts (CAFs) which are all associated with worse outcomes. Cytotoxic CD8+ T cells, neutrophils with N1 phenotype, and natural killer (NK) cells can have antitumor effects in extrahepatic cholangiocarcinoma.
Regarding the adaptive immune system, tumor-infiltrating lymphocytes (TILs) mainly include CD4+ T lymphocytes and CD8+ T lymphocytes which consist of diverse subsets in eCCA (82). FOXP3+ regulatory T cells (Tregs) characterized by TGF-β and IL-10 secretion are noticed to infiltrate into the tumors with an immunosuppressive profile. Several studies have elevated Tregs in eCCA based on immunohistochemical results for FOXP3 while increased Tregs are significantly associated with worse OS in patients with p/dCCA (78, 83, 84). Experiments in vitro showed that FOXP3+ Tregs could be recruited by FOXM1 which bound to the FOXP3 promoter region and thus promoted its transcription in pCCA cell lines (85). Similarly, single-cell RNA sequencing on tissues derived from patients with dCCA also revealed that tumor infiltrating Tregs were abundant in dCCA tumors with immunosuppressive genes such as TIGIT, CTLA4, and TNFRSF18 highly expressed (Figure 1) (86). Furthermore, several genes related to immunotherapy including ACP5, MAGEH1, TNFRSF9, and CCR8 could be specially expressed in tumor infiltrating Tregs in eCCA as shown in the single-cell landscape from another research (76). For CD8+ T cells, some studies concluded that higher numbers of them were associated with better OS for eCCA (78, 82) while heterogeneity of CD8+ T cells may be neglected. As reported recently, cytotoxic CD8+ T cells could function as effectors in dCCA while exhausted CD8+ T cells were also enriched with PDCD1, CTLA4, LAG3, and HAVCR2 expressed (76, 86). Notably, mucosal-associated invariant T cells possessing cytotoxicity and innateness were absent in the pCCA tumor microenvironment (87). Histologically, canonical tertiary lymphoid structures were associated with favorable survival in pCCA (88).
Cancer-associated fibroblasts (CAFs) are a heterogeneous cell population of fibroblasts and myofibroblast-like cells and constitute CCA stroma chiefly with typical phenotypic markers such as α-SMA, PDGFRβ, FAP, and so on (89). In CCA, CAFs likely derive from a variety of cell types including hepatic stellate cells, portal fibroblasts, fibrocytes, or epithelial mesenchymal transition (EMT) (90). CAFs can mediate crosstalk with CCA cells or TME which pave the road for tumorigenesis. Extrahepatic TFK-1 cells co-cultured with CAFs showed incremental activation of STAT3, JNK, ERK, and AKT pathways (91). Admittedly, recent studies focused on CAFs and iCCA more while some evidence was also not special for eCCA (92, 93).
6 Genomic landscape of eCCA, common and maverick
Molecular heterogeneity across eCCA has been unveiled at the genomic level whereas pCCA and dCCA do bear dissimilar genomic alterations (94). DNA mismatch repair (MMR) deficiency could be found in about 5% of pCCA and dCCA, lower than iCCA as reported previously (95). Conventional mutations in TP53, KRAS, ARID1A, SMAD4, and GNAS were commonly shared in eCCA whereas CCA subtypes do carry diverse genomic profiles (96, 97). PRKACA and PRKACB fusions and ELF3 mutations could be inclined to occur in pCCA/dCCA (98). According to Simbolo M et al, KRAS mutations may be more prevalent in dCCA when compared to pCCA (99). Paradoxically, KRAS mutations were more common in pCCA than dCCA in another cohort (94). Furthermore, ERBB2 amplifications could occur more frequently in eCCA (100). ERBB2 mutations or amplifications were also linked to a proliferation class of eCCA where patients with dCCA predominate (96). Several driver genes involved in post-transcriptional modification such as RBM10 and METTL14 mutation were more enriched in pCCA than iCCA. Conversely, both tumor mutation burden and copy number alteration burden of pCCA were lower than iCCA (101). Intriguingly, aristolochic acid exposure which could induce TP53 mutation in iCCA was superior to eCCA in a Chinese cohort where high mutational frequencies of THAP9, SEC24B, and CAND1 were noticed in eCCA (102). Actually, canonical FGFR2 fusion events were nearly absent in eCCA whereas AXL-HNRNPUL1 gene fusions could be detected in a few cases with eCCA (98, 100). Of note, cell-free DNA (cfDNA) analysis excels at shedding light on tumor heterogeneity and provides an unbiased genomic profiling for patients. cfDNA analysis on advanced cholangiocarcinoma (both iCCA and eCAA, subtype was not specified) revealed that three targetable alterations including FGFR2 fusion, IDH1 mutations, and BRAF V600E were clonal in the generality of the cohort. Besides, discordance and concordance between cfDNA and tissue for mutation detection could be noticed in the former one and the latter two, respectively (103). The high heterogeneity of eCCA can be likely attributed to genomics aberrations, highlighting the demand for characterizing the molecular basis of sensitivity and resistance to available treatments (Figure 2).
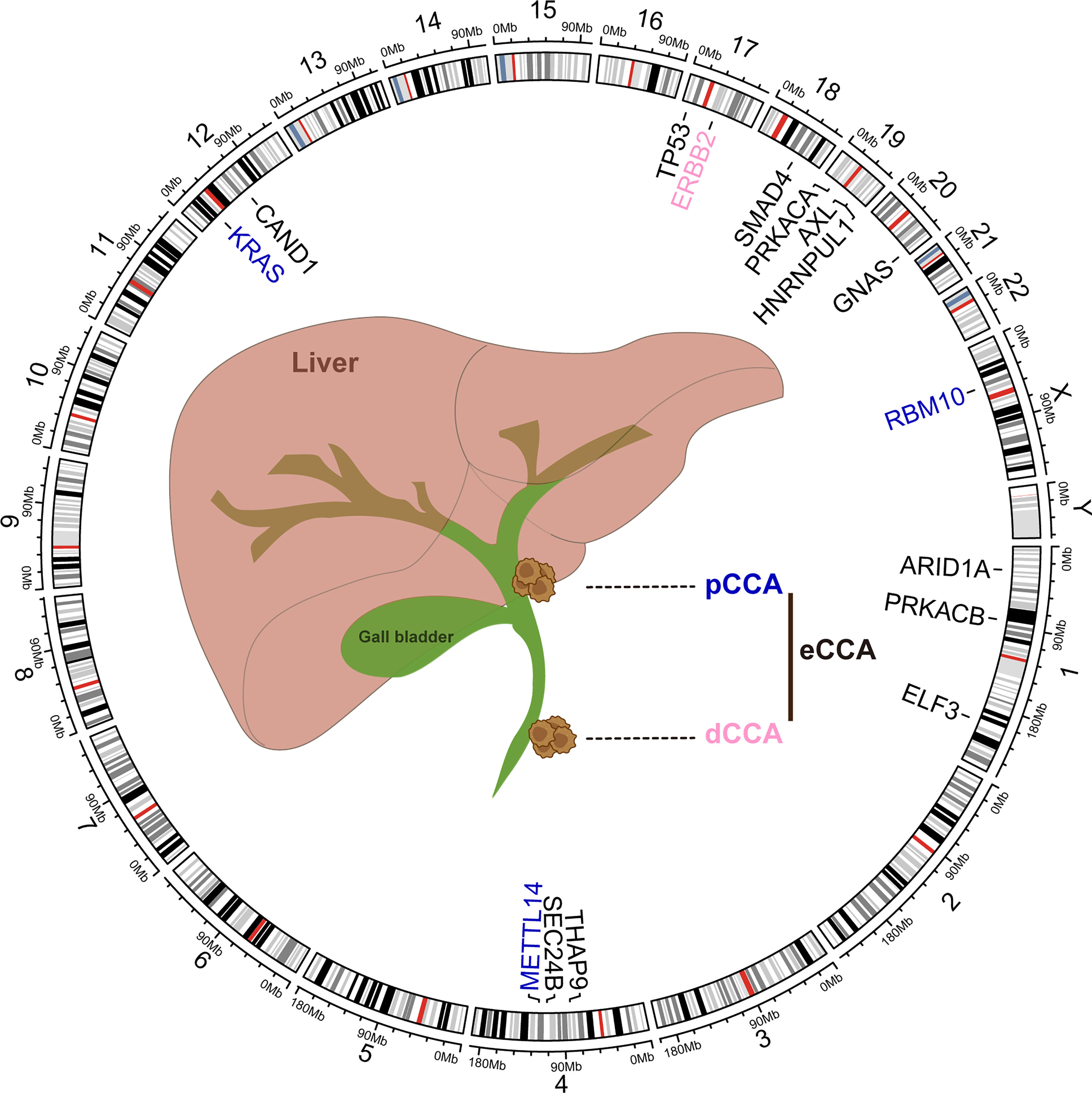
Figure 2 Common genomic alterations in extrahepatic cholangiocarcinoma. The genetic alterations and locations in human chromosomes (hg38) were depicted in the circos plot executed by R language package criclize.
7 Pathogenesis of eCCA, classical but complex
To elaborate pathogenesis of eCCA is insurmountable while it is challenging to catch the “Achilles’ Heel” of eCCA which can be related to signaling pathways to some extent. According to bulk transcriptomic profiles, ‘metabolic’, ‘proliferation’, ‘mesenchymal’, and ‘immune’ subtypes of eCCA were previously identified with disparate oncogenic pathways activated respectively (96). Indeed, several developmental pathways can be linked to eCCA (Figure 3). The Notch signaling pathway counts on ligands attaching to Notch receptors and subsequent release of Notch intracellular domain 1 (NICD1) which is then shifted to the nucleus where target genes regulating cell proliferation, migration, and invasion are activated (2). Although recent research mainly focused on the mechanism of the Notch pathway and iCCA (104), the Notch receptors were indeed overexpressed in pCCA and dCCA (105). The Wnt/β-catenin pathway is commonly activated in CCA and partially mutated in dCCA (73, 106). The Wnt/β-catenin pathway could be inhibited through ClC-5 inhibition in eCCA cells (107). TTYH3 could facilitate cell proliferation, migration, and invasion via the Wnt/β-catenin pathway in the QBC939 cell line (108). lncRNA PCAT1 was also involved in the positive regulation of pCCA and dCCA progression through miR-122 (109). Remarkably, SOX17 which is the WNT/β-catenin pathway promoter inhibitor was hypermethylated and thus repressed in patients with CCA (110). Apart from its seeming tumor suppression effect, SOX17 could sensitize tumors to chemotherapy with MRP3 suppressed in EGI-1 and TFK-1 cell lines (111). Alteration of classic oncogenic pathways is also involved in the pathogenesis of eCCA with genomic instability (96). For instance, transcription factor HOXA5 could augment MXD1 expression by binding to its promoter region directly which then activated the p53 signaling pathway, thus inhibiting eCCA cell proliferation (112). Notably, the MYC-oncogene pathways can drive tumorigenesis and be related to immune evasion in cancer (Figure 3) (113). HMGA1 inducing TRIP13 expression which suppressed FBXW7 transcription could stabilize c-Myc which expedited their transcription in a positive feedback, thus promoting EMT and stemness of pCCA (114). TCF7 inducing c-Myc transcription could impel pCCA progression (115). Besides, WDR5 could boost HIF-1α accumulation and then drive EMT and metastasis of eCCA in a Myc-dependent way (116). Interestingly, the depletion of glutamine could offset hypoxia-induced chemoresistance in eCCA cells with c-Myc restraint (117). Regarding metabolism pathways, lipid metabolism, and fatty acid oxidation were strikingly enhanced in the EGI1 cell line with intracellular lipids accumulation and increased cell stemness (118). Compared with iCCA, FABP5 functioning as a fatty acid transport protein is highly expressed and associated with poor survival in eCCA (119). Moreover, JNK/c-Jun pathways could also be associated with both iCCA and eCCA (120, 121). Proinflammatory cytokines, such as IL-6, IL-8. can be involved in augmenting tumorigenesis of eCCA. IL-6 in serum was a prognostic factor in eCCA patients (122). Likewise, the Genetic variant of CXCR1 (also termed IL-8RA) could predict inferior outcomes for pCCA patients (123). Angiogenesis is also essential for eCCA. High levels of VEGF have been noticed in eCCA cell lines and tissues previously (124). Recently, Li T et al. reported that VEGF was regulated by Gab1 via SHP2/ERK1/2 which could be inhibited by apatinibin in pCCA cells (125).
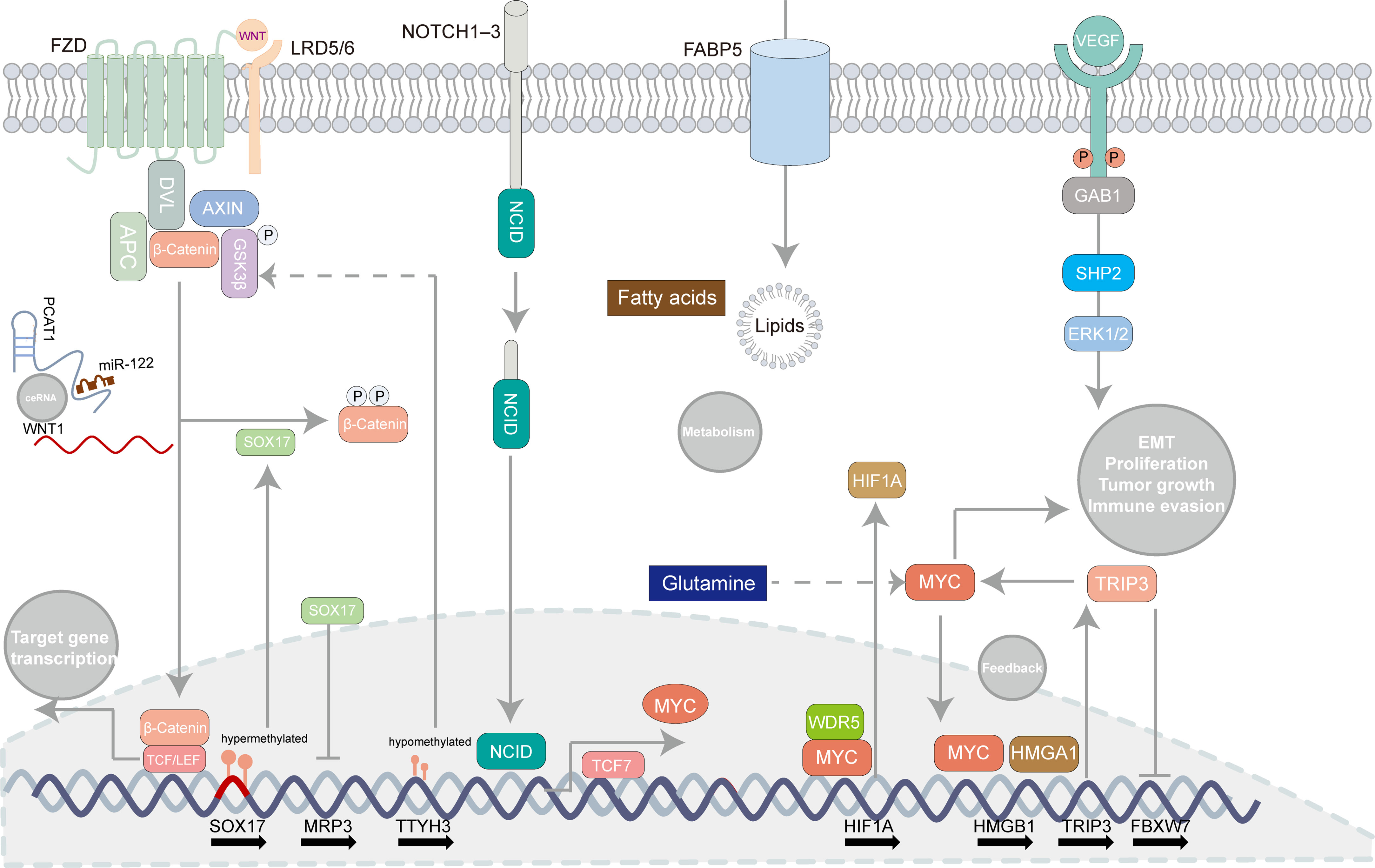
Figure 3 Oncogenic pathways involved in extrahepatic cholangiocarcinoma. Some canonical pathways can be related to tumorigenesis in extrahepatic cholangiocarcinoma such as the Notch signaling pathway, the WNT/β-catenin pathway, the MYC-oncogene pathways, lipid metabolism, and angiogenesis. Several regulatory mechanisms concerning those pathways are recapitulated in the illustrator.
Cancer stem cells (CSCs) are a characteristic subpopulation of tumor cells and harbor the ability to maintain renewal which can be involved in recurrence, metastasis, and drug resistance (126). As shown in accumulative shreds of evidence, CSCs are interrelated with EMT intimately (4). Not only does TGFβ contribute to EMT, but it also facilitates stemness in extrahepatic TFK-1 cells in vitro (127). Besides, CSCs from iCCA and eCCA can be identified with ALDH expressed (127). Remarkably, though cell proliferation and invasion were more increased in iCCA than in eCCA cell lines, stem cell surface markers (CD13, CD24, CD44, CD90, and EPCAM) were similarly expressed for both sides (128).
8 Biomarkers of eCCA, novel or clinical
Non-invasive and robust biomarkers of eCCA with diagnostic and prognostic significance have been urgent for execution. Novel biomarkers of eCCA have been emerging with advanced test tools and abundant specimens available (Figure 4). Squamous cell carcinoma antigen (SCCA) detected in bile samples was found to be increased in patients with eCCA and could be a special biomarker for eCCA (129). Similarly, microRNA (miR-31-5p, miR-378d, miR-182-5p, and miR-92a-3p) derived from bile cytologic samples were also increased in eCCA cases compared with control cases (130). Anti-apoptotic protein Bcl-xL encoded by BCL2L1 was identified as a prognostic marker in cholangiocarcinoma depending on anatomical subtypes when it indicated beneficial prognosis, especially for pCCA (131). The preoperative serum is also an accessible and robust source for biomarkers in biofluids. Preoperative serum carbohydrate antigen 19-9 could be related to regional lymph node metastases and the prognosis of both pCCA and dCCA with a cutoff of 37 U/ml (132–134). A recent study reported that elevated serum CA242 (>20 IU/ml) was associated with vascular invasion and lymph node metastasis of pCCA (135). Furthermore, inflammatory markers including neutrophils, fibrinogen-to-prealbumin ratio, and fibrinogen-to-lymphocyte-to-neutrophil ratio from preoperative peripheral blood were all independent factors for overall survival of eCCA according to the recent multivariate Cox analyses (51).
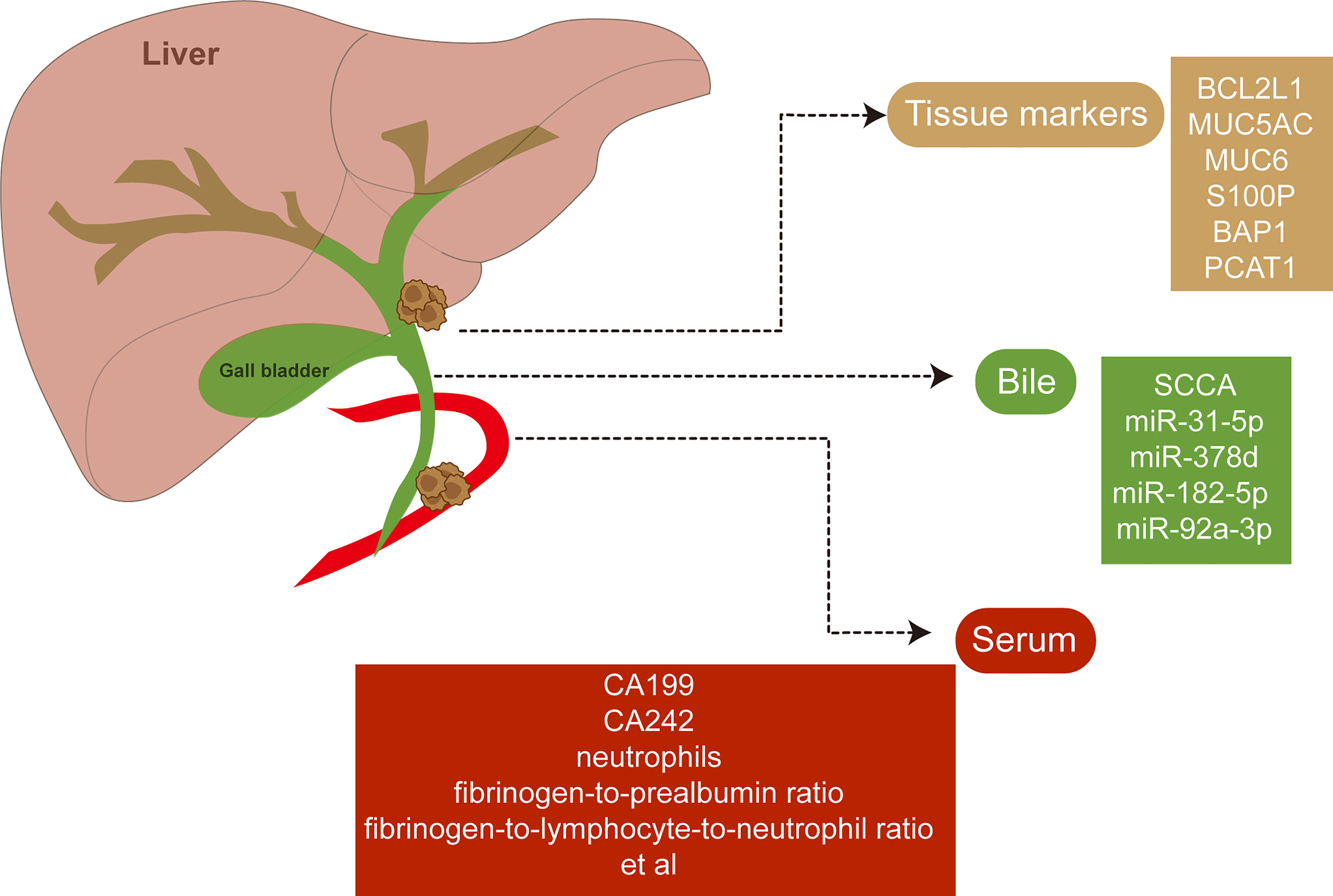
Figure 4 Potential biomarkers of extrahepatic cholangiocarcinoma. Novel biomarkers of extrahepatic cholangiocarcinoma have been discovered with abundant specimens including tissues, bile, and serum available.
Moreover, long non-coding RNAs (lncRNAs) are characterized as non-coding RNAs whose transcripts are longer than 200 nucleotides lacking the ability to code for proteins but influencing tumorigenesis, which are also implicated in the molecular biomarkers of CCA (136, 137). For instance, lncRNA PCAT1 was remarkably upregulated in both eCCA tissues and cell lines (109). MALAT1 could be involved in the pathogenesis of pCCA and predict poor overall survival (138). Some studies have also evaluated the role of lncRNAs in eCCA cell lines. AFAP1-AS1 was relevant to cell growth and metastasis in TFK-1 cell line (139). LINC00184 increased cell growth in QBC939 cell line (140). However, effective tissue markers related to lncRNA for identify eCCA are yet to be discovered.
9 Rare histological subtypes related to eCCA, less is more
Histologically, pCCA and dCCA mainly cover mucinous adenocarcinomas or papillary tumors beyond which several additional histological subtypes could be also noticed in eCCA, rarely but factually (141). Adenosquamous carcinoma featured with concomitant adenocarcinoma and squamous carcinoma accounts for 2% of eCCA as previously reported (142). Though it occurs predominantly among the rare subtypes of eCCA, adenosquamous carcinoma can carry different molecular profiles (143). A recent case report showed that an adenosquamous carcinoma patient with distant lymph node metastases carried Her-2 amplification and preserved a stable state after receiving several lines of trastuzumab treatment combined with chemotherapy and radiotherapy (144). Besides, another rare type related to eCCA is signet ring cell carcinoma. Signet ring cell carcinoma is characterized by abundant mucus in the cytoplasm extruding nucleus from center to margin of cell. Generally, a few cases with signet ring cell carcinoma of eCCA were reported up to now (145). Previous studies also described two separable types containing intestinal type and pancreatobiliary type with CK7 negative plus CK20/MUC2 positive and CK7 positive plus CK20/MUC2 negative, respectively (146, 147). That signet ring cell carcinoma of eCCA with distant lymph node metastasis has also been noticed recently (145). Distant metastases always lead to a poor prognosis in eCCA patients. A SEER-based study reported that the liver and distant lymph were the most common sites for metastases and multiple sites (at least two) occurred in some cases (148). Particularly, patients with unresectable advanced eCCA and liver metastases may benefit from chemotherapy combining gemcitabine and cisplatin or pembrolizumab and nab-paclitaxel (149, 150). About the gastrointestinal tracts, several studies also reported colonic metastasis of eCCA (151, 152). Rarely, distal skeletal muscle metastasis could appear in a few eCCA patients as reported (153). Those evidence suggested that adequate follow-up periods should be considered for eCCA since sporadic metastasis could occur.
10 Targeted therapies and immunotherapy
Molecularly in-depth understanding of CCA contributes to confirming achievable drug targets. Although IDH1 mutations and FGFR2 fusions do provide new treatment tactics, they are more frequent in iCCA and nearly absent in eCCA (100, 154, 155). Moreover, several randomized controlled trials concerning the epidermal growth factor receptor (EGFR) inhibitors (erlotinib, cetuximab, lapatinib, or panitumumab) did not achieve effective outcomes in advanced CCA (57–60, 156) (Table 2). A previous meta-analysis has also shown that first-line chemotherapy with the addition of anti-EGFR monoclonal antibodies does not improve the overall and progression-free survival of patients with advanced CCA (157). Alternatively, targeting abnormal ERBB2 which is more common in eCCA may be a favorable approach. A case report suggested a combination of Trastuzumab and pertuzumab was curative for the patient with ERBB2-amplified eCCA (41). Immune checkpoint blockade can reinforce antitumor immunity by hindering intrinsic suppressors (e.g. CTLA4, PD1, or PDL1) from the immunosuppressive microenvironment where the tumor locates while several checkpoint inhibitors have been approved for clinical application (158). Regarding eCCA, four novel transcriptome-based subtypes have been suggested (96). Tumors in the “immune” class not only overexpressed PD-1/PD-L1 but also had a higher lymphocyte infiltration which implies a better response to immune checkpoint inhibitors. Furthermore, the ratio of PD1 positive to CD8 + TILs could be linked to worse outcomes for eCCA patients (159). A subset of CD8+RORγt+ T cells with PD1 expressed lowly was noticed to be associated with reduced survival in dCCA as reported previously (160). Actually, pembrolizumab seems to be more effective in CCA patients with microsatellite instability (MSI) or mismatch repair deficiency (dMMR) whose incidence is low in CCA while it is also reported that the number of ECC patients with PDL1 positive could be small (161, 162). Indeed, the TOPAZ-1 trial has improved our understanding of CCA and immunotherapy (42).
Several immunotherapy agents such as Pembrolizumab, Nivolumab and Atezolizumab have shown low response rates in patients with advanced stages of CCA (61–63).
Up to now, more clinical trials are still requisite for eCCA.
11 Conclusion
CCA is heterogeneous and comprised of diverse subtypes. Not only do those subtypes arise from different locations, but iCCA and eCCA also carry disparate risk factors, diverse cells of origin, and individual genome aberrations. Sophisticated interactions between eCCA cells or CSCs, and the TME make it laborious to elaborate the biological mechanisms underpinning tumorigenesis where high-resolution single cell multi-omics may shed light on. Now, there is still a lack of therapeutic approaches for eCCA since not all patients with eCCA can benefit from accessible treatments including surgery, adjuvant therapy, targeted therapies, and immunotherapy, emphasizing the importance of personalized and multidisciplinary considerations. However, improved understanding of the specific TME and pathogenesis in eCCA, along with accumulating data from single cell resolution will indisputably bring more efficient therapeutic options for patients in the future. Furthermore, considering that several benign diseases are risk factors of eCCA, it is also crucial for patients with eCCA to prevent early, diagnose accurately, and treat timely. That is, better to batten down the hatches before the storm comes.
Author contributions
YY: Visualization, data curation, writing - original draft. XZ: Project administration, supervision, writing - review & editing. Both authors contributed to the article and approved the submitted version.
Funding
This work was supported by grants from the National Natural Science Foundation of China (no. 8210100902) to XZ.
Conflict of interest
The authors declare that the research was conducted in the absence of any commercial or financial relationships that could be construed as a potential conflict of interest.
Publisher’s note
All claims expressed in this article are solely those of the authors and do not necessarily represent those of their affiliated organizations, or those of the publisher, the editors and the reviewers. Any product that may be evaluated in this article, or claim that may be made by its manufacturer, is not guaranteed or endorsed by the publisher.
Abbreviations
CAFs, Cancer-associated fibroblasts; CCA, cholangiocarcinoma; CfDNA, cell-free DNA; CSCs, cancer stem cells; dCCA, distal cholangiocarcinoma; eCCA, extrahepatic cholangiocarcinoma; EGFR, epidermal growth factor receptor; EMT, epithelial mesenchymal transition; ERCP, endoscopic retrograde cholangiopancreatography; EUS, endoscopic ultrasound; FDA, Food and Drug Administration; FLR, fibrinogen-to-lymphocyte ratio; HR, hazard ratio; iCCA, intrahepatic cholangiocarcinoma; ICD, International Classification of Diseases; LT, liver transplantation; LncRNAs, long non-coding RNAs; MMR, DNA mismatch repair; MRCP, magnetic resonance cholangiopancreatography; MSI, microsatellite instability; NICD1, notch intracellular domain 1; NK, natural killer; pCCA, perihilar cholangiocarcinoma; PSC, primary sclerosing cholangitis; SEER, Surveillance, Epidemiology, and End Results; TANs, tumor-associated neutrophils; TILs, tumor-infiltrating lymphocytes; TME, tumor microenvironment; Tregs, regulatory T cells.
References
1. Valle JW, Kelley RK, Nervi B, Oh DY, Zhu AX. Biliary tract cancer. Lancet (2021) 397(10272):428–44. doi: 10.1016/S0140-6736(21)00153-7
2. Rodrigues PM, Olaizola P, Paiva NA, Olaizola I, Agirre-Lizaso A, Landa A, et al. Pathogenesis of cholangiocarcinoma. Annu Rev Pathol (2021) 16:433–63. doi: 10.1146/annurev-pathol-030220-020455
3. Kendall T, Verheij J, Gaudio E, Evert M, Guido M, Goeppert B, et al. Anatomical, histomorphological and molecular classification of cholangiocarcinoma. Liver Int (2019) 39 Suppl 1:7–18. doi: 10.1111/liv.14093
4. Banales JM, Marin JJG, Lamarca A, Rodrigues PM, Khan SA, Roberts LR, et al. Cholangiocarcinoma 2020: the next horizon in mechanisms and management. Nat Rev Gastroenterol Hepatol (2020) 17(9):557–88. doi: 10.1038/s41575-020-0310-z
5. Song G, Shi Y, Meng L, Ma J, Huang S, Zhang J, et al. Single-cell transcriptomic analysis suggests two molecularly subtypes of intrahepatic cholangiocarcinoma. Nat Commun (2022) 13(1):1642. doi: 10.1038/s41467-022-29164-0
6. Vithayathil M, Khan SA. Current epidemiology of cholangiocarcinoma in Western countries. J Hepatol (2022) 77(6):1690–8. doi: 10.1016/j.jhep.2022.07.022
7. Komaya K, Ebata T, Shirai K, Ohira S, Morofuji N, Akutagawa A, et al. Recurrence after resection with curative intent for distal cholangiocarcinoma. Br J Surg (2017) 104(4):426–33. doi: 10.1002/bjs.10452
8. Nakachi K, Ikeda M, Konishi M, Nomura S, Katayama H, Kataoka T, et al. Adjuvant s-1 compared with observation in resected biliary tract cancer (Jcog1202, ascot): a multicentre, open-label, randomised, controlled, phase 3 trial. Lancet (2023) 401(10372):195–203. doi: 10.1016/S0140-6736(22)02038-4
9. Florio AA, Ferlay J, Znaor A, Ruggieri D, Alvarez CS, Laversanne M, et al. Global trends in intrahepatic and extrahepatic cholangiocarcinoma incidence from 1993 to 2012. Cancer (2020) 126(11):2666–78. doi: 10.1002/cncr.32803
10. Siegel RL, Miller KD, Fuchs HE, Jemal A. Cancer statistics, 2022. CA Cancer J Clin (2022) 72(1):7–33. doi: 10.3322/caac.21708
11. Rizvi S, Khan SA, Hallemeier CL, Kelley RK, Gores GJ. Cholangiocarcinoma - evolving concepts and therapeutic strategies. Nat Rev Clin Oncol (2018) 15(2):95–111. doi: 10.1038/nrclinonc.2017.157
12. Khan SA, Tavolari S, Brandi G. Cholangiocarcinoma: epidemiology and risk factors. Liver Int (2019) 39 Suppl 1:19–31. doi: 10.1111/liv.14095
13. Piegari G. The who's global clinical practice network and the icd-11 implementation. World Psychiatry (2021) 20(2):313–4. doi: 10.1002/wps.20866
14. Ali H, Zweigle J, Patel P, Tedder B, Khan R, Agrawal S. Survival analysis of extrahepatic cholangiocarcinoma based on surveillance, epidemiology, and end results database. Ann Hepatobiliary Pancreat Surg (2023). doi: 10.14701/ahbps.22-090
15. Rahman R, Ludvigsson JF, von Seth E, Lagergren J, Bergquist A, Radkiewicz C. Age trends in biliary tract cancer incidence by anatomical subtype: a Swedish cohort study. Eur J Cancer (2022) 175:291–8. doi: 10.1016/j.ejca.2022.08.032
16. Clements O, Eliahoo J, Kim JU, Taylor-Robinson SD, Khan SA. Risk factors for intrahepatic and extrahepatic cholangiocarcinoma: a systematic review and meta-analysis. J Hepatol (2020) 72(1):95–103. doi: 10.1016/j.jhep.2019.09.007
17. Park JH, Hong JY, Park YS, Kang G, Han K, Park JO. Association of prediabetes, diabetes, and diabetes duration with biliary tract cancer risk: a nationwide cohort study. Metabolism (2021) 123:154848. doi: 10.1016/j.metabol.2021.154848
18. Park JH, Hong JY, Kwon M, Lee J, Han K, Han IW, et al. Association between non-alcoholic fatty liver disease and the risk of biliary tract cancers: a south Korean nationwide cohort study. Eur J Cancer (2021) 150:73–82. doi: 10.1016/j.ejca.2021.03.024
19. Park JH, Hong JY, Han K, Park YS, Park JO. Light-to-Moderate alcohol consumption increases the risk of biliary tract cancer in prediabetes and diabetes, but not in normoglycemic status: a nationwide cohort study. J Clin Oncol (2022) 40(31):3623–32. doi: 10.1200/JCO.22.00145
20. Izquierdo-Sanchez L, Lamarca A, La Casta A, Buettner S, Utpatel K, Klumpen HJ, et al. Cholangiocarcinoma landscape in Europe: diagnostic, prognostic and therapeutic insights from the enscca registry. J Hepatol (2022) 76(5):1109–21. doi: 10.1016/j.jhep.2021.12.010
21. Lavu S, Therneau TM, Harmsen WS, Mara KC, Wongjarupong N, Hassan M, et al. Effect of statins on the risk of extrahepatic cholangiocarcinoma. Hepatology (2020) 72(4):1298–309. doi: 10.1002/hep.31146
22. Liao SF, Koshiol J, Huang YH, Jackson SS, Huang YH, Chan C, et al. Postdiagnosis aspirin use associated with decreased biliary tract cancer-specific mortality in a Large nationwide cohort. Hepatology (2021) 74(4):1994–2006. doi: 10.1002/hep.31879
23. Kim MH, Park SM, Chang J, Hwang IC. Association between aspirin and cholangiocarcinoma in a Large Asian cohort. JAMA Oncol (2020) 6(12):1974–6. doi: 10.1001/jamaoncol.2020.5018
24. Marcano-Bonilla L, Schleck CD, Harmsen WS, Sadr-Azodi O, Borad MJ, Patel T, et al. Aspirin, statins, non-aspirin nsaids, metformin, and the risk of biliary cancer: a Swedish population-based cohort study. Cancer Epidemiol Biomarkers Prev (2022) 31(4):804–10. doi: 10.1158/1055-9965.EPI-20-1322
25. Kamal H, Sadr-Azodi O, Engstrand L, Brusselaers N. Association between proton pump inhibitor use and biliary tract cancer risk: a Swedish population-based cohort study. Hepatology (2021) 74(4):2021–31. doi: 10.1002/hep.31914
26. Bowlus CL, Olson KA, Gershwin ME. Evaluation of indeterminate biliary strictures. Nat Rev Gastroenterol Hepatol (2017) 14(12):749. doi: 10.1038/nrgastro.2017.154
27. Blechacz B, Komuta M, Roskams T, Gores GJ. Clinical diagnosis and staging of cholangiocarcinoma. Nat Rev Gastroenterol Hepatol (2011) 8(9):512–22. doi: 10.1038/nrgastro.2011.131
28. Joo I, Lee JM, Yoon JH. Imaging diagnosis of intrahepatic and perihilar cholangiocarcinoma: recent advances and challenges. Radiology (2018) 288(1):7–13. doi: 10.1148/radiol.2018171187
29. Beyer G, Kasprowicz F, Hannemann A, Aghdassi A, Thamm P, Volzke H, et al. Definition of age-dependent reference values for the diameter of the common bile duct and pancreatic duct on mrcp: a population-based, cross-sectional cohort study. Gut (2023). doi: 10.1136/gutjnl-2021-326106
30. Cadamuro M, Al-Taee A, Gonda TA. Advanced endoscopy meets molecular diagnosis of cholangiocarcinoma. J Hepatol (2023). doi: 10.1016/j.jhep.2023.01.027
31. Park MS, Kim TK, Kim KW, Park SW, Lee JK, Kim JS, et al. Differentiation of extrahepatic bile duct cholangiocarcinoma from benign stricture: findings at mrcp versus ercp. Radiology (2004) 233(1):234–40. doi: 10.1148/radiol.2331031446
32. Coronel M, Lee JH, Coronel E. Endoscopic ultrasound for the diagnosis and staging of biliary malignancy. Clin Liver Dis (2022) 26(1):115–25. doi: 10.1016/j.cld.2021.08.010
33. Sadeghi A, Mohamadnejad M, Islami F, Keshtkar A, Biglari M, Malekzadeh R, et al. Diagnostic yield of eus-guided fna for malignant biliary stricture: a systematic review and meta-analysis. Gastrointest Endosc (2016) 83(2):290–8 e1. doi: 10.1016/j.gie.2015.09.024
34. Draganov PV, Chauhan S, Wagh MS, Gupte AR, Lin T, Hou W, et al. Diagnostic accuracy of conventional and cholangioscopy-guided sampling of indeterminate biliary lesions at the time of ercp: a prospective, long-term follow-up study. Gastrointest Endosc (2012) 75(2):347–53. doi: 10.1016/j.gie.2011.09.020
35. Ishii T, Kaneko T, Murakami A, Ueda M, Sugimori K, Kawana I, et al. New image-enhanced cholangioscopy for the diagnosis of cholangiocarcinoma. Endoscopy (2023) 55(S 01):E139–E40. doi: 10.1055/a-1956-0967
36. Gorji L, Beal EW. Surgical treatment of distal cholangiocarcinoma. Curr Oncol (2022) 29(9):6674–87. doi: 10.3390/curroncol29090524
37. Hewitt DB, Brown ZJ, Pawlik TM. Current perspectives on the surgical management of perihilar cholangiocarcinoma. Cancers (Basel) (2022) 14(9):2208. doi: 10.3390/cancers14092208
38. Hou JJ, Maithel SK, Weber SM, Poultsides G, Wolfgang CL, Fields RC, et al. Impact of adjuvant therapy on outcomes after curative-intent resection for distal cholangiocarcinoma. J Surg Oncol (2023) 127(4):607–15. doi: 10.1002/jso.27146
39. Kamarajah SK, Bednar F, Cho CS, Nathan H. Survival benefit with adjuvant radiotherapy after resection of distal cholangiocarcinoma: a propensity-matched national cancer database analysis. Cancer (2021) 127(8):1266–74. doi: 10.1002/cncr.33356
40. Steel AW, Postgate AJ, Khorsandi S, Nicholls J, Jiao L, Vlavianos P, et al. Endoscopically applied radiofrequency ablation appears to be safe in the treatment of malignant biliary obstruction. Gastrointest Endosc (2011) 73(1):149–53. doi: 10.1016/j.gie.2010.09.031
41. Yarlagadda B, Kamatham V, Ritter A, Shahjehan F, Kasi PM. Trastuzumab and pertuzumab in circulating tumor DNA Erbb2-amplified Her2-positive refractory cholangiocarcinoma. NPJ Precis Oncol (2019) 3:19. doi: 10.1038/s41698-019-0091-4
42. Greten TF, Schwabe R, Bardeesy N, Ma L, Goyal L, Kelley RK, et al. Immunology and immunotherapy of cholangiocarcinoma. Nat Rev Gastroenterol Hepatol (2023). doi: 10.1038/s41575-022-00741-4
43. Chen Z, Yu B, Bai J, Li Q, Xu B, Dong Z, et al. The impact of intraoperative frozen section on resection margin status and survival of patients underwent pancreatoduodenectomy for distal cholangiocarcinoma. Front Oncol (2021) 11:650585. doi: 10.3389/fonc.2021.650585
44. Belkouz A, Van Roessel S, Strijker M, van Dam JL, Daamen L, van der Geest LG, et al. Development and external validation of a prediction model for overall survival after resection of distal cholangiocarcinoma. Br J Cancer (2022) 126(9):1280–8. doi: 10.1038/s41416-021-01687-1
45. Ogino M, Nakanishi Y, Mitsuhashi T, Hatanaka Y, Amano T, Marukawa K, et al. Impact of tumour budding grade in 310 patients who underwent surgical resection for extrahepatic cholangiocarcinoma. Histopathology (2019) 74(6):861–72. doi: 10.1111/his.13827
46. Zhao Y, Nakanishi Y, Ogino M, Oba M, Okamura K, Tsuchikawa T, et al. Validation study of tumor invasive thickness for postoperative prognosis in 110 patients who underwent pancreatoduodenectomy for distal cholangiocarcinoma at a single institution. Am J Surg Pathol (2019) 43(5):717–23. doi: 10.1097/PAS.0000000000001244
47. Bednarsch J, Kather J, Tan X, Sivakumar S, Cacchi C, Wiltberger G, et al. Nerve fibers in the tumor microenvironment as a novel biomarker for oncological outcome in patients undergoing surgery for perihilar cholangiocarcinoma. Liver Cancer (2021) 10(3):260–74. doi: 10.1159/000515303
48. Mori S, Aoki T, Park KH, Shiraki T, Sakuraoka Y, Iso Y, et al. Impact of preoperative percutaneous transhepatic biliary drainage on post-operative survival in patients with distal cholangiocarcinoma. ANZ J Surg (2019) 89(9):E363–E7. doi: 10.1111/ans.15329
49. Coelen RJS, Roos E, Wiggers JK, Besselink MG, Buis CI, Busch ORC, et al. Endoscopic versus percutaneous biliary drainage in patients with resectable perihilar cholangiocarcinoma: a multicentre, randomised controlled trial. Lancet Gastroenterol Hepatol (2018) 3(10):681–90. doi: 10.1016/S2468-1253(18)30234-6
50. Al Mahjoub A, Menahem B, Fohlen A, Dupont B, Alves A, Launoy G, et al. Preoperative biliary drainage in patients with resectable perihilar cholangiocarcinoma: is percutaneous transhepatic biliary drainage safer and more effective than endoscopic biliary drainage? a meta-analysis. J Vasc Interv Radiol (2017) 28(4):576–82. doi: 10.1016/j.jvir.2016.12.1218
51. Li S, Zhang X, Lou C, Gu Y, Zhao J. Preoperative peripheral blood inflammatory markers especially the fibrinogen-to-Lymphocyte ratio and novel flr-n score predict the prognosis of patients with early-stage resectable extrahepatic cholangiocarcinoma. Front Oncol (2022) 12:1003845. doi: 10.3389/fonc.2022.1003845
52. Ebata T, Hirano S, Konishi M, Uesaka K, Tsuchiya Y, Ohtsuka M, et al. Randomized clinical trial of adjuvant gemcitabine chemotherapy versus observation in resected bile duct cancer. Br J Surg (2018) 105(3):192–202. doi: 10.1002/bjs.10776
53. Gholami S, Colby S, Horowitz DP, Guthrie KA, Ben-Josef E, El-Khoueiry AB, et al. Adjuvant chemoradiation in patients with lymph node-positive biliary tract cancers: secondary analysis of a single-arm clinical trial (Swog 0809). Ann Surg Oncol (2023) 30(3):1354–63. doi: 10.1245/s10434-022-12863-9
54. Yang J, Wang J, Zhou H, Zhou Y, Wang Y, Jin H, et al. Efficacy and safety of endoscopic radiofrequency ablation for unresectable extrahepatic cholangiocarcinoma: a randomized trial. Endoscopy (2018) 50(8):751–60. doi: 10.1055/s-0043-124870
55. Gao DJ, Yang JF, Ma SR, Wu J, Wang TT, Jin HB, et al. Endoscopic radiofrequency ablation plus plastic stent placement versus stent placement alone for unresectable extrahepatic biliary cancer: a multicenter randomized controlled trial. Gastrointest Endosc (2021) 94(1):91–100 e2. doi: 10.1016/j.gie.2020.12.016
56. Yang J, Wang J, Zhou H, Wang Y, Huang H, Jin H, et al. Endoscopic radiofrequency ablation plus a novel oral 5-fluorouracil compound versus radiofrequency ablation alone for unresectable extrahepatic cholangiocarcinoma. Gastrointest Endosc (2020) 92(6):1204–12 e1. doi: 10.1016/j.gie.2020.04.075
57. Lee J, Park SH, Chang HM, Kim JS, Choi HJ, Lee MA, et al. Gemcitabine and oxaliplatin with or without erlotinib in advanced biliary-tract cancer: a multicentre, open-label, randomised, phase 3 study. Lancet Oncol (2012) 13(2):181–8. doi: 10.1016/S1470-2045(11)70301-1
58. Chen JS, Hsu C, Chiang NJ, Tsai CS, Tsou HH, Huang SF, et al. A kras mutation status-stratified randomized phase ii trial of gemcitabine and oxaliplatin alone or in combination with cetuximab in advanced biliary tract cancer. Ann Oncol (2015) 26(5):943–9. doi: 10.1093/annonc/mdv035
59. Vogel A, Kasper S, Bitzer M, Block A, Sinn M, Schulze-Bergkamen H, et al. Picca study: panitumumab in combination with Cisplatin/Gemcitabine chemotherapy in kras wild-type patients with biliary cancer-a randomised biomarker-driven clinical phase ii aio study. Eur J Cancer (2018) 92:11–9. doi: 10.1016/j.ejca.2017.12.028
60. Leone F, Marino D, Cereda S, Filippi R, Belli C, Spadi R, et al. Panitumumab in combination with gemcitabine and oxaliplatin does not prolong survival in wild-type kras advanced biliary tract cancer: a randomized phase 2 trial (Vecti-bil study). Cancer (2016) 122(4):574–81. doi: 10.1002/cncr.29778
61. Piha-Paul SA, Oh DY, Ueno M, Malka D, Chung HC, Nagrial A, et al. Efficacy and safety of pembrolizumab for the treatment of advanced biliary cancer: results from the keynote-158 and keynote-028 studies. Int J Cancer (2020) 147(8):2190–8. doi: 10.1002/ijc.33013
62. Kim RD, Chung V, Alese OB, El-Rayes BF, Li D, Al-Toubah TE, et al. A phase 2 multi-institutional study of nivolumab for patients with advanced refractory biliary tract cancer. JAMA Oncol (2020) 6(6):888–94. doi: 10.1001/jamaoncol.2020.0930
63. Yarchoan M, Cope L, Ruggieri AN, Anders RA, Noonan AM, Goff LW, et al. Multicenter randomized phase ii trial of atezolizumab with or without cobimetinib in biliary tract cancers. J Clin Invest (2021) 131(24). doi: 10.1172/JCI152670
64. Meyer CG, Penn I, James L. Liver transplantation for cholangiocarcinoma: results in 207 patients. Transplantation (2000) 69(8):1633–7. doi: 10.1097/00007890-200004270-00019
65. Rizzo A, Brandi G. Neoadjuvant therapy for cholangiocarcinoma: a comprehensive literature review. Cancer Treat Res Commun (2021) 27:100354. doi: 10.1016/j.ctarc.2021.100354
66. Cambridge WA, Fairfield C, Powell JJ, Harrison EM, Soreide K, Wigmore SJ, et al. Meta-analysis and meta-regression of survival after liver transplantation for unresectable perihilar cholangiocarcinoma. Ann Surg (2021) 273(2):240–50. doi: 10.1097/SLA.0000000000003801
67. Xia MX, Cai XB, Pan YL, Wu J, Gao DJ, Ye X, et al. Optimal stent placement strategy for malignant hilar biliary obstruction: a Large multicenter parallel study. Gastrointest Endosc (2020) 91(5):1117–28 e9. doi: 10.1016/j.gie.2019.12.023
68. Xia MX, Wang SP, Yuan JG, Gao DJ, Ye X, Wang TT, et al. Effect of endoscopic radiofrequency ablation on the survival of patients with inoperable malignant biliary strictures: a Large cohort study. J Hepatobiliary Pancreat Sci (2022) 29(6):693–702. doi: 10.1002/jhbp.960
69. Xia M, Qin W, Hu B. Endobiliary radiofrequency ablation for unresectable malignant biliary strictures: a survival benefit perspective. Dig Endosc (2023). doi: 10.1111/den.14542
70. Inoue T, Naitoh I, Kitano R, Ibusuki M, Kobayashi Y, Sumida Y, et al. Endobiliary radiofrequency ablation combined with gemcitabine and cisplatin in patients with unresectable extrahepatic cholangiocarcinoma. Curr Oncol (2022) 29(4):2240–51. doi: 10.3390/curroncol29040182
71. Gonzalez-Carmona MA, Mohring C, Mahn R, Zhou T, Bartels A, Sadeghlar F, et al. Impact of regular additional endobiliary radiofrequency ablation on survival of patients with advanced extrahepatic cholangiocarcinoma under systemic chemotherapy. Sci Rep (2022) 12(1):1011. doi: 10.1038/s41598-021-04297-2
72. Kang B, Camps J, Fan B, Jiang H, Ibrahim MM, Hu X, et al. Parallel single-cell and bulk transcriptome analyses reveal key features of the gastric tumor microenvironment. Genome Biol (2022) 23(1):265. doi: 10.1186/s13059-022-02828-2
73. Boulter L, Guest RV, Kendall TJ, Wilson DH, Wojtacha D, Robson AJ, et al. Wnt signaling drives cholangiocarcinoma growth and can be pharmacologically inhibited. J Clin Invest (2015) 125(3):1269–85. doi: 10.1172/JCI76452
74. Atanasov G, Hau HM, Dietel C, Benzing C, Krenzien F, Brandl A, et al. Prognostic significance of macrophage invasion in hilar cholangiocarcinoma. BMC Cancer (2015) 15:790. doi: 10.1186/s12885-015-1795-7
75. Xia T, Li K, Niu N, Shao Y, Ding D, Thomas DL, et al. Immune cell atlas of cholangiocarcinomas reveals distinct tumor microenvironments and associated prognoses. J Hematol Oncol (2022) 15(1):37. doi: 10.1186/s13045-022-01253-z
76. Xu L, Lu Y, Deng Z, Li X, Shi Y, Zhao K, et al. Single-cell landscape of immunocytes in patients with extrahepatic cholangiocarcinoma. J Transl Med (2022) 20(1):210. doi: 10.1186/s12967-022-03424-5
77. Jung IH, Kim DH, Yoo DK, Baek SY, Jeong SH, Jung DE, et al. In Vivo Study of natural killer (Nk) cell cytotoxicity against cholangiocarcinoma in a nude mouse model. In Vivo (2018) 32(4):771–81. doi: 10.21873/invivo.11307
78. Kitano Y, Okabe H, Yamashita YI, Nakagawa S, Saito Y, Umezaki N, et al. Tumour-infiltrating inflammatory and immune cells in patients with extrahepatic cholangiocarcinoma. Br J Cancer (2018) 118(2):171–80. doi: 10.1038/bjc.2017.401
79. Toyoda J, Sahara K, Maithel SK, Abbott DE, Poultsides GA, Wolfgang C, et al. Prognostic utility of systemic immune-inflammation index after resection of extrahepatic cholangiocarcinoma: results from the U.S. extrahepatic biliary malignancy consortium. Ann Surg Oncol (2022) 29(12):7605–14. doi: 10.1245/s10434-022-12058-2
80. Gao Y, Zhang H, Zhou N, Xu P, Wang J, Gao Y, et al. Methotrexate-loaded tumour-Cell-Derived microvesicles can relieve biliary obstruction in patients with extrahepatic cholangiocarcinoma. Nat BioMed Eng (2020) 4(7):743–53. doi: 10.1038/s41551-020-0583-0
81. Xue R, Zhang Q, Cao Q, Kong R, Xiang X, Liu H, et al. Liver tumour immune microenvironment subtypes and neutrophil heterogeneity. Nature (2022) 612(7938):141–7. doi: 10.1038/s41586-022-05400-x
82. Liu D, Heij LR, Czigany Z, Dahl E, Lang SA, Ulmer TF, et al. The role of tumor-infiltrating lymphocytes in cholangiocarcinoma. J Exp Clin Cancer Res (2022) 41(1):127. doi: 10.1186/s13046-022-02340-2
83. Goeppert B, Frauenschuh L, Zucknick M, Stenzinger A, Andrulis M, Klauschen F, et al. Prognostic impact of tumour-infiltrating immune cells on biliary tract cancer. Br J Cancer (2013) 109(10):2665–74. doi: 10.1038/bjc.2013.610
84. Ueno T, Tsuchikawa T, Hatanaka KC, Hatanaka Y, Mitsuhashi T, Nakanishi Y, et al. Prognostic impact of programmed cell death ligand 1 (Pd-L1) expression and its association with epithelial-mesenchymal transition in extrahepatic cholangiocarcinoma. Oncotarget (2018) 9(28):20034–47. doi: 10.18632/oncotarget.25050
85. Ma K, Sun Z, Li X, Guo J, Wang Q, Teng M. Forkhead box M1 recruits Foxp3(+) treg cells to induce immune escape in hilar cholangiocarcinoma. Immun Inflammation Dis (2022) 10(11):e727. doi: 10.1002/iid3.727
86. Li H, Qu L, Yang Y, Zhang H, Li X, Zhang X. Single-cell transcriptomic architecture unraveling the complexity of tumor heterogeneity in distal cholangiocarcinoma. Cell Mol Gastroenterol Hepatol (2022) 13(5):1592–609 e9. doi: 10.1016/j.jcmgh.2022.02.014
87. Zimmer CL, Filipovic I, Cornillet M, O'Rourke CJ, Berglin L, Jansson H, et al. Mucosal-associated invariant T-cell tumor infiltration predicts long-term survival in cholangiocarcinoma. Hepatology (2022) 75(5):1154–68. doi: 10.1002/hep.32222
88. Zhang FP, Zhu K, Zhu TF, Liu CQ, Zhang HH, Xu LB, et al. Intra-tumoral secondary follicle-like tertiary lymphoid structures are associated with a superior prognosis of overall survival of perihilar cholangiocarcinoma. Cancers (Basel) (2022) 14(24):6107. doi: 10.3390/cancers14246107
89. Montori M, Scorzoni C, Argenziano ME, Balducci D, De Blasio F, Martini F, et al. Cancer-associated fibroblasts in cholangiocarcinoma: current knowledge and possible implications for therapy. J Clin Med (2022) 11(21):6498. doi: 10.3390/jcm11216498
90. Ying F, Man Chan MS, Wah Lee TK. Cancer-associated fibroblasts in hepatocellular carcinoma and cholangiocarcinoma. Cell Mol Gastroenterol Hepatol (2023) 15(4):985–99. doi: 10.1016/j.jcmgh.2023.01.006
91. Gundlach JP, Kerber J, Hendricks A, Bernsmeier A, Halske C, Roder C, et al. Paracrine interaction of cholangiocellular carcinoma with cancer-associated fibroblasts and schwann cells impact cell migration. J Clin Med (2022) 11(10):2785. doi: 10.3390/jcm11102785
92. Sun L, Wang Y, Wang X, Navarro-Corcuera A, Ilyas S, Jalan-Sakrikar N, et al. Pd-L1 promotes myofibroblastic activation of hepatic stellate cells by distinct mechanisms selective for tgf-beta receptor I versus ii. Cell Rep (2022) 38(6):110349. doi: 10.1016/j.celrep.2022.110349
93. Olaizola P, Lee-Law PY, Fernandez-Barrena MG, Alvarez L, Cadamuro M, Azkargorta M, et al. Targeting Nae1-mediated protein hyper-neddylation halts cholangiocarcinogenesis and impacts on tumor-stroma crosstalk in experimental models. J Hepatol (2022) 77(1):177–90. doi: 10.1016/j.jhep.2022.02.007
94. Simbolo M, Bersani S, Vicentini C, Taormina SV, Ciaparrone C, Bagante F, et al. Molecular characterization of extrahepatic cholangiocarcinoma: perihilar and distal tumors display divergent genomic and transcriptomic profiles. Expert Opin Ther Targets (2021) 25(12):1095–105. doi: 10.1080/14728222.2021.2013801
95. Silva VW, Askan G, Daniel TD, Lowery M, Klimstra DS, Abou-Alfa GK, et al. Biliary carcinomas: pathology and the role of DNA mismatch repair deficiency. Chin Clin Oncol (2016) 5(5):62. doi: 10.21037/cco.2016.10.04
96. Montal R, Sia D, Montironi C, Leow WQ, Esteban-Fabro R, Pinyol R, et al. Molecular classification and therapeutic targets in extrahepatic cholangiocarcinoma. J Hepatol (2020) 73(2):315–27. doi: 10.1016/j.jhep.2020.03.008
97. Lowery MA, Ptashkin R, Jordan E, Berger MF, Zehir A, Capanu M, et al. Comprehensive molecular profiling of intrahepatic and extrahepatic cholangiocarcinomas: potential targets for intervention. Clin Cancer Res (2018) 24(17):4154–61. doi: 10.1158/1078-0432.CCR-18-0078
98. Nakamura H, Arai Y, Totoki Y, Shirota T, Elzawahry A, Kato M, et al. Genomic spectra of biliary tract cancer. Nat Genet (2015) 47(9):1003–10. doi: 10.1038/ng.3375
99. Simbolo M, Fassan M, Ruzzenente A, Mafficini A, Wood LD, Corbo V, et al. Multigene mutational profiling of cholangiocarcinomas identifies actionable molecular subgroups. Oncotarget (2014) 5(9):2839–52. doi: 10.18632/oncotarget.1943
100. Rizzato M, Brignola S, Munari G, Gatti M, Dadduzio V, Borga C, et al. Prognostic impact of Fgfr2/3 alterations in patients with biliary tract cancers receiving systemic chemotherapy: the bitcoin study. Eur J Cancer (2022) 166:165–75. doi: 10.1016/j.ejca.2022.02.013
101. Zhang Y, Ma Z, Li C, Wang C, Jiang W, Chang J, et al. The genomic landscape of cholangiocarcinoma reveals the disruption of post-transcriptional modifiers. Nat Commun (2022) 13(1):3061. doi: 10.1038/s41467-022-30708-7
102. Deng M, Ran P, Chen L, Wang Y, Yu Z, Cai K, et al. Proteogenomic characterization of cholangiocarcinoma. Hepatology (2023) 77(2):411–29. doi: 10.1002/hep.32624
103. Berchuck JE, Facchinetti F, DiToro DF, Baiev I, Majeed U, Reyes S, et al. The clinical landscape of cell-free DNA alterations in 1671 patients with advanced biliary tract cancer. Ann Oncol (2022) 33(12):1269–83. doi: 10.1016/j.annonc.2022.09.150
104. Hu S, Molina L, Tao J, Liu S, Hassan M, Singh S, et al. Notch-Yap1/Tead-Dnmt1 axis drives hepatocyte reprogramming into intrahepatic cholangiocarcinoma. Gastroenterology (2022) 163(2):449–65. doi: 10.1053/j.gastro.2022.05.007
105. Aoki S, Mizuma M, Takahashi Y, Haji Y, Okada R, Abe T, et al. Aberrant activation of notch signaling in extrahepatic cholangiocarcinoma: clinicopathological features and therapeutic potential for cancer stem cell-like properties. BMC Cancer (2016) 16(1):854. doi: 10.1186/s12885-016-2919-4
106. Gingras MC, Covington KR, Chang DK, Donehower LA, Gill AJ, Ittmann MM, et al. Ampullary cancers harbor Elf3 tumor suppressor gene mutations and exhibit frequent wnt dysregulation. Cell Rep (2016) 14(4):907–19. doi: 10.1016/j.celrep.2015.12.005
107. Shi Z, Zhou L, Zhou Y, Jia X, Yu X, An X, et al. Inhibition of clc-5 suppresses proliferation and induces apoptosis in cholangiocarcinoma cells through the Wnt/Beta-catenin signaling pathway. BMB Rep (2022) 55(6):299–304. doi: 10.5483/BMBRep.2022.55.6.044
108. Xue W, Dong B, Zhao Y, Wang Y, Yang C, Xie Y, et al. Upregulation of Ttyh3 promotes epithelial-to-Mesenchymal transition through Wnt/Beta-catenin signaling and inhibits apoptosis in cholangiocarcinoma. Cell Oncol (Dordr) (2021) 44(6):1351–61. doi: 10.1007/s13402-021-00642-9
109. Zhang F, Wan M, Xu Y, Li Z, Leng K, Kang P, et al. Long noncoding rna Pcat1 regulates extrahepatic cholangiocarcinoma progression Via the Wnt/Beta-Catenin-Signaling pathway. BioMed Pharmacother (2017) 94:55–62. doi: 10.1016/j.biopha.2017.07.025
110. Merino-Azpitarte M, Lozano E, Perugorria MJ, Esparza-Baquer A, Erice O, Santos-Laso A, et al. Sox17 regulates cholangiocyte differentiation and acts as a tumor suppressor in cholangiocarcinoma. J Hepatol (2017) 67(1):72–83. doi: 10.1016/j.jhep.2017.02.017
111. Lozano E, Asensio M, Perez-Silva L, Banales JM, Briz O, Marin JJG. Mrp3-mediated chemoresistance in cholangiocarcinoma: target for chemosensitization through restoring Sox17 expression. Hepatology (2020) 72(3):949–64. doi: 10.1002/hep.31088
112. Xiong F, Liu W, Wang X, Wu G, Wang Q, Guo T, et al. Hoxa5 inhibits the proliferation of extrahepatic cholangiocarcinoma cells by enhancing Mxd1 expression and activating the P53 pathway. Cell Death Dis (2022) 13(9):829. doi: 10.1038/s41419-022-05279-6
113. Dhanasekaran R, Deutzmann A, Mahauad-Fernandez WD, Hansen AS, Gouw AM, Felsher DW. The myc oncogene - the grand orchestrator of cancer growth and immune evasion. Nat Rev Clin Oncol (2022) 19(1):23–36. doi: 10.1038/s41571-021-00549-2
114. Li Z, Liu J, Chen T, Sun R, Liu Z, Qiu B, et al. Hmga1-Trip13 axis promotes stemness and epithelial mesenchymal transition of perihilar cholangiocarcinoma in a positive feedback loop dependent on c-myc. J Exp Clin Cancer Res (2021) 40(1):86. doi: 10.1186/s13046-021-01890-1
115. Liu Z, Sun R, Zhang X, Qiu B, Chen T, Li Z, et al. Transcription factor 7 promotes the progression of perihilar cholangiocarcinoma by inducing the transcription of c-myc and fos-like antigen 1. EBioMedicine (2019) 45:181–91. doi: 10.1016/j.ebiom.2019.06.023
116. Chen T, Li K, Liu Z, Liu J, Wang Y, Sun R, et al. Wdr5 facilitates emt and metastasis of cca by increasing hif-1alpha accumulation in myc-dependent and independent pathways. Mol Ther (2021) 29(6):2134–50. doi: 10.1016/j.ymthe.2021.02.017
117. Wappler J, Arts M, Roth A, Heeren RMA, Peter Neumann U, Olde Damink SW, et al. Glutamine deprivation counteracts hypoxia-induced chemoresistance. Neoplasia (2020) 22(1):22–32. doi: 10.1016/j.neo.2019.10.004
118. Ruiz de Gauna M, Biancaniello F, Gonzalez-Romero F, Rodrigues PM, Lapitz A, Gomez-Santos B, et al. Cholangiocarcinoma progression depends on the uptake and metabolization of extracellular lipids. Hepatology (2022) 76(6):1617–33. doi: 10.1002/hep.32344
119. Nakagawa R, Hiep NC, Ouchi H, Sato Y, Harada K. Expression of fatty-Acid-Binding protein 5 in intrahepatic and extrahepatic cholangiocarcinoma: the possibility of different energy metabolisms in anatomical location. Med Mol Morphol (2020) 53(1):42–9. doi: 10.1007/s00795-019-00230-9
120. Jiao CY, Feng QC, Li CX, Wang D, Han S, Zhang YD, et al. Bub1b promotes extrahepatic cholangiocarcinoma progression Via Jnk/C-jun pathways. Cell Death Dis (2021) 12(1):63. doi: 10.1038/s41419-020-03234-x
121. Chen XX, Yin Y, Cheng JW, Huang A, Hu B, Zhang X, et al. Bap1 acts as a tumor suppressor in intrahepatic cholangiocarcinoma by modulating the Erk1/2 and Jnk/C-jun pathways. Cell Death Dis (2018) 9(10):1036. doi: 10.1038/s41419-018-1087-7
122. Shimura T, Shibata M, Gonda K, Kofunato Y, Okada R, Ishigame T, et al. Clinical significance of soluble intercellular adhesion molecule-1 and interleukin-6 in patients with extrahepatic cholangiocarcinoma. J Invest Surg (2018) 31(6):475–82. doi: 10.1080/08941939.2017.1358310
123. Lurje I, Czigany Z, Bednarsch J, Gaisa NT, Dahl E, Knuchel R, et al. Genetic variant of Cxcr1 (Rs2234671) associates with clinical outcome in perihilar cholangiocarcinoma. Liver Cancer (2022) 11(2):162–73. doi: 10.1159/000521613
124. Yoshikawa D, Ojima H, Iwasaki M, Hiraoka N, Kosuge T, Kasai S, et al. Clinicopathological and prognostic significance of egfr, vegf, and Her2 expression in cholangiocarcinoma. Br J Cancer (2008) 98(2):418–25. doi: 10.1038/sj.bjc.6604129
125. Li T, Tian Y, Ren W, Chen P, Luo M, Sang H. Gab1 regulates invadopodia and autocrine vegf through Shp2/Erk1/2 in hilar cholangiocarcinoma cells. Am J Transl Res (2022) 14(12):8934–46.
126. Batlle E, Clevers H. Cancer stem cells revisited. Nat Med (2017) 23(10):1124–34. doi: 10.1038/nm.4409
127. Shuang ZY, Wu WC, Xu J, Lin G, Liu YC, Lao XM, et al. Transforming growth factor-Beta1-Induced epithelial-mesenchymal transition generates aldh-positive cells with stem cell properties in cholangiocarcinoma. Cancer Lett (2014) 354(2):320–8. doi: 10.1016/j.canlet.2014.08.030
128. Yang J, Sontag DP, Burczynski FJ, Xi S, Gong Y, Minuk GY. Comparison of growth features and cancer stem cell prevalence in intrahepatic and extrahepatic cholangiocarcinoma cell lines. Clin Exp Hepatol (2022) 8(1):60–9. doi: 10.5114/ceh.2022.114192
129. Gringeri E, Biasiolo A, Di Giunta M, Mescoli C, Guzzardo V, Sartori A, et al. Bile detection of squamous cell carcinoma antigen (Scca) in extrahepatic cholangiocarcinoma. Dig Liver Dis (2022) 55(4):534–40. doi: 10.1016/j.dld.2022.10.010
130. Uchihata Y, Arihiro K, Kaneko Y, Shimizu T, Marubashi Y, Aoki C, et al. Analysis of microrna in bile cytologic samples is useful for detection and diagnosis of extrahepatic cholangiocarcinoma. Am J Clin Pathol (2022) 158(1):122–31. doi: 10.1093/ajcp/aqac015
131. Hoffmeister-Wittmann P, Mock A, Nichetti F, Korell F, Heilig CE, Scherr AL, et al. Bcl-X(L) as prognostic marker and potential therapeutic target in cholangiocarcinoma. Liver Int (2022) 42(12):2855–70. doi: 10.1111/liv.15392
132. Yamamoto R, Sugiura T, Ashida R, Ohgi K, Yamada M, Otsuka S, et al. Prognostic value of carbohydrate antigen 19-9 and the surgical margin in extrahepatic cholangiocarcinoma. Ann Gastroenterol Surg (2022) 6(2):307–15. doi: 10.1002/ags3.12525
133. Bolm L, Petrova E, Weitz J, Ruckert F, Wittel UA, Makowiec F, et al. Prognostic relevance of preoperative bilirubin-adjusted serum carbohydrate antigen 19-9 in a multicenter subset analysis of 179 patients with distal cholangiocarcinoma. HPB (Oxford) (2019) 21(11):1513–9. doi: 10.1016/j.hpb.2019.03.363
134. Lee JW, Lee JH, Park Y, Kwon J, Lee W, Song KB, et al. Prognostic impact of perioperative Ca19-9 levels in patients with resected perihilar cholangiocarcinoma. J Clin Med (2021) 10(7):1345. doi: 10.3390/jcm10071345
135. Heng G, Huang B, Shen Y, Wang D, Lan Z, Yao Y, et al. Vascular invasion and lymph node metastasis mediate the effect of Ca242 on prognosis in hilar cholangiocarcinoma patients after radical resection. Front Oncol (2022) 12:1071439. doi: 10.3389/fonc.2022.1071439
136. Ghafouri-Fard S, Safarzadeh A, Hussen BM, Taheri M, Samsami M. A review on the role of ncrnas in the pathogenesis of cholangiocarcinoma. Int J Biol Macromol (2023) 225:809–21. doi: 10.1016/j.ijbiomac.2022.11.144
137. Wu Y, Hayat K, Hu Y, Yang J. Long non-coding rnas as molecular biomarkers in cholangiocarcinoma. Front Cell Dev Biol (2022) 10:890605. doi: 10.3389/fcell.2022.890605
138. Tan X, Huang Z, Li X. Long non-coding rna Malat1 interacts with mir-204 to modulate human hilar cholangiocarcinoma proliferation, migration, and invasion by targeting Cxcr4. J Cell Biochem (2017) 118(11):3643–53. doi: 10.1002/jcb.25862
139. Shi X, Zhang H, Wang M, Xu X, Zhao Y, He R, et al. Lncrna Afap1-As1 promotes growth and metastasis of cholangiocarcinoma cells. Oncotarget (2017) 8(35):58394–404. doi: 10.18632/oncotarget.16880
140. Sun HB, Zhang GC, Liu J, Nie CS. Long noncoding rna Linc00184 facilitates the proliferation, metastasis, and adenine metabolism of cholangiocarcinoma Via modulating hsa-Mir-23b-3p/Anxa2 axis. Environ Toxicol (2021) 36(8):1576–90. doi: 10.1002/tox.23154
141. Sapuppo E, Brunetti O, Tessitore D, Brandi G, Di Giovanni N, Fadda G, et al. Rare histotypes of epithelial biliary tract tumors: a literature review. Crit Rev Oncol Hematol (2023) 181:103892. doi: 10.1016/j.critrevonc.2022.103892
142. Hoshimoto S, Hoshi S, Hishinuma S, Tomikawa M, Shirakawa H, Ozawa I, et al. Adenosquamous carcinoma in the biliary tract: association of the proliferative ability of the squamous component with its proportion and tumor progression. Scand J Gastroenterol (2017) 52(4):425–30. doi: 10.1080/00365521.2016.1273383
143. Kanagasabapathy S, Subasinghe D, Sivaganesh S, Wijesinghe H. Adenosquamous carcinoma of the distal common bile duct: a case of a rare type of cholangiocarcinoma. Clin Pathol (2022) 15:2632010X221099884. doi: 10.1177/2632010X221099884
144. Hong Y, Li X, Cao D. Case report: trastuzumab treatment in adenosquamous carcinoma of the extrahepatic biliary tract with her-2 amplification. Front Oncol (2021) 11:538328. doi: 10.3389/fonc.2021.538328
145. Xie CB, Wu Y, Li F, Zhao KF, Shi RS, Huang Q, et al. Primary signet-ring cell carcinoma of the extrahepatic bile duct: a case report. World J Gastrointest Oncol (2022) 14(7):1356–62. doi: 10.4251/wjgo.v14.i7.1356
146. Kita E, Tsujimoto A, Nakamura K, Sudo K, Hara T, Kainuma O, et al. Signet ring cell carcinoma of the extrahepatic bile duct diagnosed by preoperative biopsy: a case report. Case Rep Gastroenterol (2014) 8(3):353–7. doi: 10.1159/000369249
147. Fischer HP, Zhou H. Pathogenesis of carcinoma of the papilla of vater. J Hepatobiliary Pancreat Surg (2004) 11(5):301–9. doi: 10.1007/s00534-004-0898-3
148. Wang X, Yu GY, Chen M, Wei R, Chen J, Wang Z. Pattern of distant metastases in primary extrahepatic bile-duct cancer: a seer-based study. Cancer Med (2018) 7(10):5006–14. doi: 10.1002/cam4.1772
149. Adachi K, Okuwaki K, Nishiyama R, Kida M, Imaizumi H, Iwai T, et al. A case of extrahepatic bile duct cancer with distant metastases showing pathological complete response to treatment combining gemcitabine and cisplatin. Clin J Gastroenterol (2019) 12(5):466–72. doi: 10.1007/s12328-019-00972-0
150. Tan S, Yu J, Huang Q, Zhou N, Xiong X, Gou H. Durable response to the combination of pembrolizumab and nab-paclitaxel in a metastatic extrahepatic cholangiocarcinoma: a case report and literature review. Front Pharmacol (2022) 13:1037646. doi: 10.3389/fphar.2022.1037646
151. Zheng X, Zhang WT, Hu J, Chen XF. A colonic metastasis from extrahepatic cholangiocarcinoma after removal of primary tumor: a case report and literature review. Chin Clin Oncol (2020) 9(2):21. doi: 10.21037/cco.2020.01.08
152. Vabi BW, Carter J, Rong R, Wang M, Corasanti JG, Gibbs JF. Metastatic colon cancer from extrahepatic cholangiocarcinoma presenting as painless jaundice: case report and literature review. J Gastrointest Oncol (2016) 7(2):E25–30. doi: 10.3978/j.issn.2078-6891.2015.119
153. Qian H, Huang Z, Sun X, Ren P. Skeletal muscle metastasis from extrahepatic cholangiocarcinoma: a case report and literature review. Front Surg (2022) 9:922834. doi: 10.3389/fsurg.2022.922834
154. Boscoe AN, Rolland C, Kelley RK. Frequency and prognostic significance of isocitrate dehydrogenase 1 mutations in cholangiocarcinoma: a systematic literature review. J Gastrointest Oncol (2019) 10(4):751–65. doi: 10.21037/jgo.2019.03.10
155. Javle M, Bekaii-Saab T, Jain A, Wang Y, Kelley RK, Wang K, et al. Biliary cancer: utility of next-generation sequencing for clinical management. Cancer (2016) 122(24):3838–47. doi: 10.1002/cncr.30254
156. Peck J, Wei L, Zalupski M, O'Neil B, Villalona Calero M, Bekaii-Saab T. Her2/Neu may not be an interesting target in biliary cancers: results of an early phase ii study with lapatinib. Oncology (2012) 82(3):175–9. doi: 10.1159/000336488
157. Rizzo A, Frega G, Ricci AD, Palloni A, Abbati F, DEL S, et al. Anti-egfr monoclonal antibodies in advanced biliary tract cancer: a systematic review and meta-analysis. In Vivo (2020) 34(2):479–88. doi: 10.21873/invivo.11798
158. Morad G, Helmink BA, Sharma P, Wargo JA. Hallmarks of response, resistance, and toxicity to immune checkpoint blockade. Cell (2022) 185(3):576. doi: 10.1016/j.cell.2022.01.008
159. Lim YJ, Koh J, Kim K, Chie EK, Kim B, Lee KB, et al. High ratio of programmed cell death protein 1 (Pd-1)(+)/Cd8(+) tumor-infiltrating lymphocytes identifies a poor prognostic subset of extrahepatic bile duct cancer undergoing surgery plus adjuvant chemoradiotherapy. Radiother Oncol (2015) 117(1):165–70. doi: 10.1016/j.radonc.2015.07.003
160. Chellappa S, Hugenschmidt H, Hagness M, Subramani S, Melum E, Line PD, et al. Cd8+ T cells that coexpress rorgammat and T-bet are functionally impaired and expand in patients with distal bile duct cancer. J Immunol (2017) 198(4):1729–39. doi: 10.4049/jimmunol.1600061
161. O'Rourke CJ, Munoz-Garrido P, Andersen JB. Molecular targets in cholangiocarcinoma. Hepatology (2021) 73 (Suppl 1):62–74. doi: 10.1002/hep.31278
Keywords: extrahepatic cholangiocarcinoma, pathogenesis, tumorigenesis, genomics, tumor microenvironment
Citation: Yang Y and Zhang X (2023) An overview of extrahepatic cholangiocarcinoma: from here to where? Front. Oncol. 13:1171098. doi: 10.3389/fonc.2023.1171098
Received: 21 February 2023; Accepted: 11 April 2023;
Published: 01 May 2023.
Edited by:
Maria Lina Tornesello, G. Pascale National Cancer Institute Foundation (IRCCS), ItalyReviewed by:
Massimiliano Cadamuro, University of Padua, ItalyJianfeng Yang, Hangzhou First People's Hospital, China
Copyright © 2023 Yang and Zhang. This is an open-access article distributed under the terms of the Creative Commons Attribution License (CC BY). The use, distribution or reproduction in other forums is permitted, provided the original author(s) and the copyright owner(s) are credited and that the original publication in this journal is cited, in accordance with accepted academic practice. No use, distribution or reproduction is permitted which does not comply with these terms.
*Correspondence: Xiaolu Zhang, xiaolu.zhang@sdu.edu.cn