- 1The Second School of Clinical Medicine, Southern Medical University, Guangzhou, China
- 2The Second School of Clinical Medical, Shanxi Medical University, Taiyuan, China
- 3Department of General Surgery, Affiliated Drum Tower Hospital of Nanjing University Medical School, Nanjing, China
- 4Medical Department of General Surgery, The 1st Medical Center, Chinese PLA General Hospital, Beijing, China
- 5Department of General Surgery, The 7th Medical Center, Chinese PLA General Hospital, Beijing, China
Neutrophil extracellular traps (NETs) are web-like structures derived from neutrophils, which typically consist of DNA, released from the nucleus or mitochondria, and decorated with histones and granule proteins. They are well known as an important structure in innate immunity to eliminate pathogenic bacteria, similar to neutrophils. Initially, NETs are reported to take part in the progression of inflammatory diseases; now, they have also been implicated in the progression of sterile inflammation such as autoimmune disease, diabetes, and cancer. In this review, we will describe the recent studies which have investigated the role of NETs in the development of cancer, especially metastasis. We also prescribe the strategies for targeting NETs in the multiple cancer types, which suggest that NETs are a promising treatment for cancer patients.
1 Introduction
There are many ways to cure cancer, including surgery, chemotherapy, radiotherapy, and immunotherapy, however, recurrence and metastasis are still the main reason for the low survival rate of patients (1). The tumor microenvironment (TME) comprises all the non-cancerous host cells in the tumor, including fibroblasts, endothelial cells, neurons, adipocytes, adaptive, and innate immune cells, as well as its non-cellular components, including the extracellular matrix (ECM), and soluble products such as chemokines, cytokines, growth factors, and extracellular vesicles. The constant interactions between tumor cells and the TME play a decisive role in tumor initiation, progression, metastasis, and response to therapies (2, 3).
Neutrophils which take part in the pathogenesis of numerous diseases are the essential players in the early response against pathogens and during acute inflammation and play an important role in the regulation of innate and adaptive immune responses (4, 5). Recently, cancer-associated inflammation has been recognized as a hallmark of tumor biology (6). An inflammatory response to a tumor will contribute to cancer initiation and progression, allowing tumor cells to escape elimination by the immune system. Recent studies showed that neutrophils are an important component of the TME and have highlighted their importance in tumor progression and therapy (7–9). Due to the heterogeneity and plasticity of neutrophils, when receiving different external incentives, tumor-associated neutrophils (TAN) are polarized into antitumor and pro-tumor populations, which are named TAN-N1 and TAN-N2 (10). In 2004, Brinkmann et al. (11) first described that neutrophil extracellular traps (NETs), extracellular fibers released from neutrophils, consist of granule proteins and chromatin, bind Gram-positive and -negative bacteria, and are vital components of the innate response. The pathway of NET production has been described as a new form of cell death, NETosis, distinct from apoptosis and necrosis (12). In addition to their important role in defense capability, NETs also play an important role in the TME (13). In this review, we will not only introduce how the NETs are produced by neutrophils but describe the crosstalk between NETs and tumor cells and the prognostic significance of NETs on cancer patients intensively.
2 An overview of nets
Previous research on neutrophils and their product, NETs, have mainly focused on inflammatory diseases, including sepsis and wound. In recent years, as studies involving neutrophils have intensified, it has been discovered that neutrophils and NETs are implicated in the progression of sterile inflammation including autoimmune disease, diabetes, and cancer (14–16).
NETs are unique net-like structure in the organism that originated from neutrophils. Like neutrophils, they act as the first defense of the organism against external stress, playing a part in removing foreign pathogens. The progression of NET formation was first described in 2004 (11); neutrophils are activated by external factors such as lipopolysaccharide (LPS) and phorbol myristate acetate (PMA) and then release intracellular DNA, histones, and granule proteins such as myeloperoxidase (MPO) and neutrophil elastase (NE) (17). These substances constitute the NETs in the extracellular compartment, and this special structure can be observed under the electron microscope. They play an important role in regulating the biological behavior of the tumor, especially tumor metastasis (18, 19).
The process of NET formation is known as “NETosis,” and there are two forms of NETosis based on whether the neutrophils lyse and die after the generation of NETs (Figure 1). The first is lytic NETosis, in which the neutrophil plasma membrane is cleaved and dies after the formation of NETs, and it lasts around 2–4 h. The other form is non-lytic NETosis, a new way different from lytic NETosis, described by Yipp et al. (20). During the pathway, neutrophils do not lyse and die after generating NETs; instead, they preserve the phagocytic function of normal neutrophils, and it starts within 1 h after stimulated by Staphylococcus aureus (21) and Candida albicans (22).
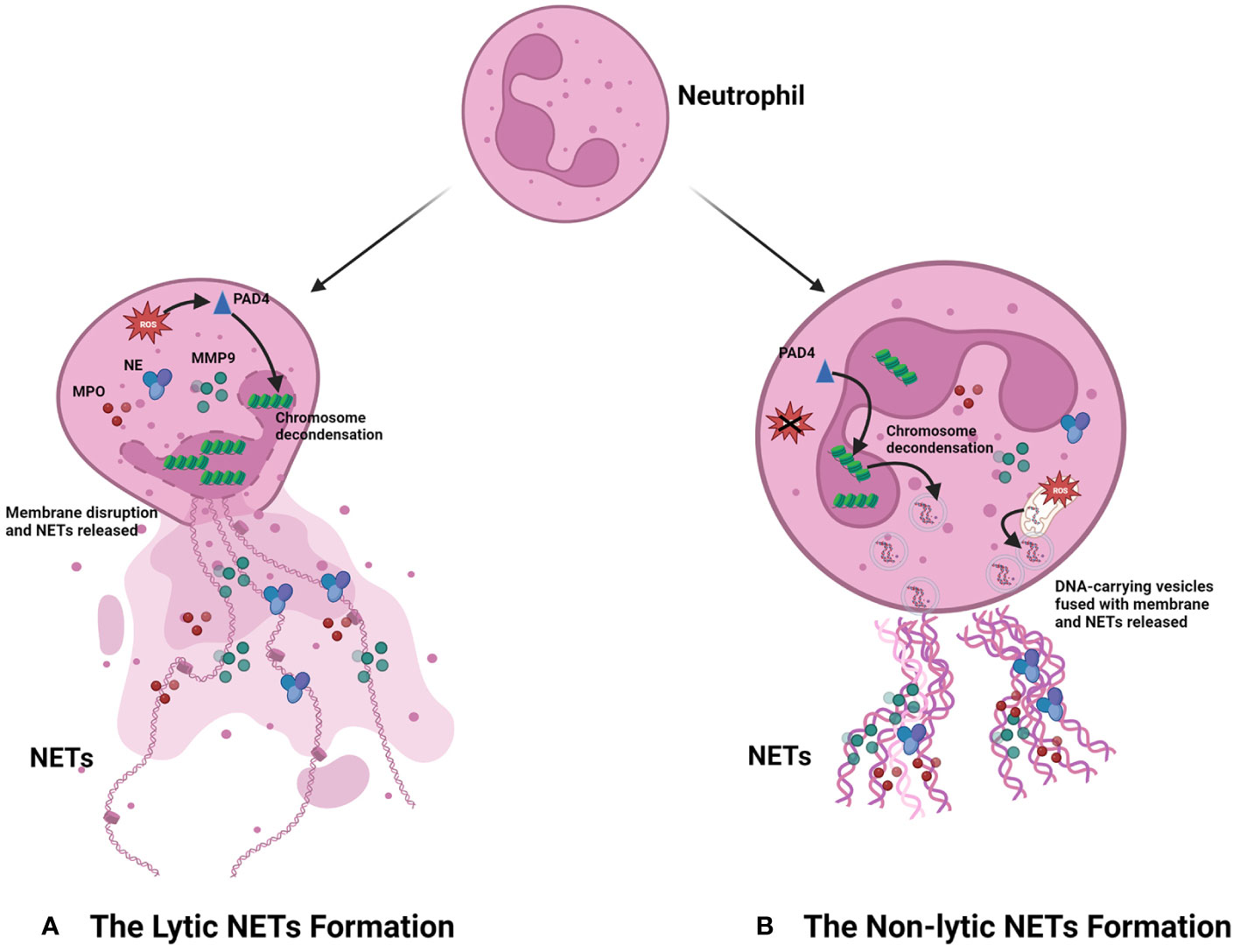
Figure 1 Progression of NET formation. When neutrophils are stimulated by stimulus, they can produce NETs in two main ways according to the different destinies. (A) The lytic NET formation. When stimuli including LPS, PMA, and IL-8 bind to the receptors of neutrophils, NADPH-oxidase is active, which can increase the level of ROS. Then, the increased ROS promotes chromosome decondensation by activating PAD4. The DNA derived from the chromosome is decorated with granule proteins and forms NETs, which are released from the dead neutrophil. (B) The non-lytic NET formation. The stimulus including damage-associated molecular patterns (DAMPs), bacteria, and injury can promote NET formation in neutrophils by forming vesicles. Different from the destiny of neutrophils in the lytic NET formation, these neutrophils still preserve intact membranes and the phagocytic function. Notably, the DNA in NETs derived from the chromosome of the nucleus is ROS-independent; however, the formation of NETs comprising mitochondrial DNA is ROS-dependent.
As the most extensively studied procedure for the formation of NETs, the stimuli of the lytic NETosis mainly include PMA, LPS, and interleukin-8 (IL-8), which, upon contact with neutrophils, initiate intracellular generation of reactive oxygen species (ROS) in an NADPH-dependent way, followed by activation of the peptidyl arginine deiminase 4 (PAD4), an essential enzyme in the NETosis process. PAD4 can facilitate chromatin decondensation, which allows DNA and histones to be excreted outside the cell and constitute the framework of NETs. It also activates a diverse range of granule proteins in neutrophils, such as NE and MPO, which bind to DNA extracellularly and collectively form the NETs (15). However, different from lytic NETosis, when external stimuli provoke non-lytic NETosis, neutrophils can form DNA-carrying vesicles independent of ROS. These vesicles can then merge with the cytosolic membrane and deliver DNA to the extracellular space, after which it is combined with granule proteins from the neutrophils to form NETs (23, 24). In addition, the researchers have also discovered that the DNA within NETs can come to be originated not only from the nucleus but also from the mitochondria, an organelle containing low amounts of DNA (25–27). Between the two pathways, the most studied is the lytic NET formation, which is also mainly discussed in this review.
3 How nets promote tumor growth and metastasis
3.1 Crosstalk between tumor cells and NETs
The interaction between tumor cells and NETs includes several different ways (Figure 2). First, NETs can activate a variety of receptors and signal pathways associated with growth, and metastasis to shape the characteristics of the tumor. High mobility group box 1 (HMGB1), a protein widely distributed in the body, has been discovered to have the pro-inflammatory function and becomes in recent years one of the popular targets of research in critical care medicine, and NETs also seem to enhance the malignancy of cancer (28), for the possible reason, just like Zhang et al. reported, that HMGB1 activates the nuclear factor-kappa B (NF-κB) signaling pathway upon binding to the receptor for advanced glycation end products (RAGE) on the tumor cell surface and promotes tumor secretion of IL-8 (29, 30). In contrast, IL-8 recruits neutrophils and promotes the production of NETs, thereby creating a positive feedback, which also promotes colorectal cancer liver metastasis (31). The study by Tohme et al. (32) has indicated that NETs can promote HMGB1 production within tumor cells and activate TLR9-dependent pathways to promote tumor cell growth, metastasis, and invasive ability. Furthermore, the binding of NETs to tumor cells can also induce tumor cells to acquire resistance to death as well as enhanced invasiveness by activating the TLR4/9-COX2 pathway, and the use of DNase I in combination with the anti-inflammatory drugs can effectively reduce hepatocellular carcinoma metastasis (33). According to Albrengues et al. (34), NETs can also “wake up” dormant tumor cells through metalloproteinase (MMP) and NE, facilitating metastasis and recurrence.
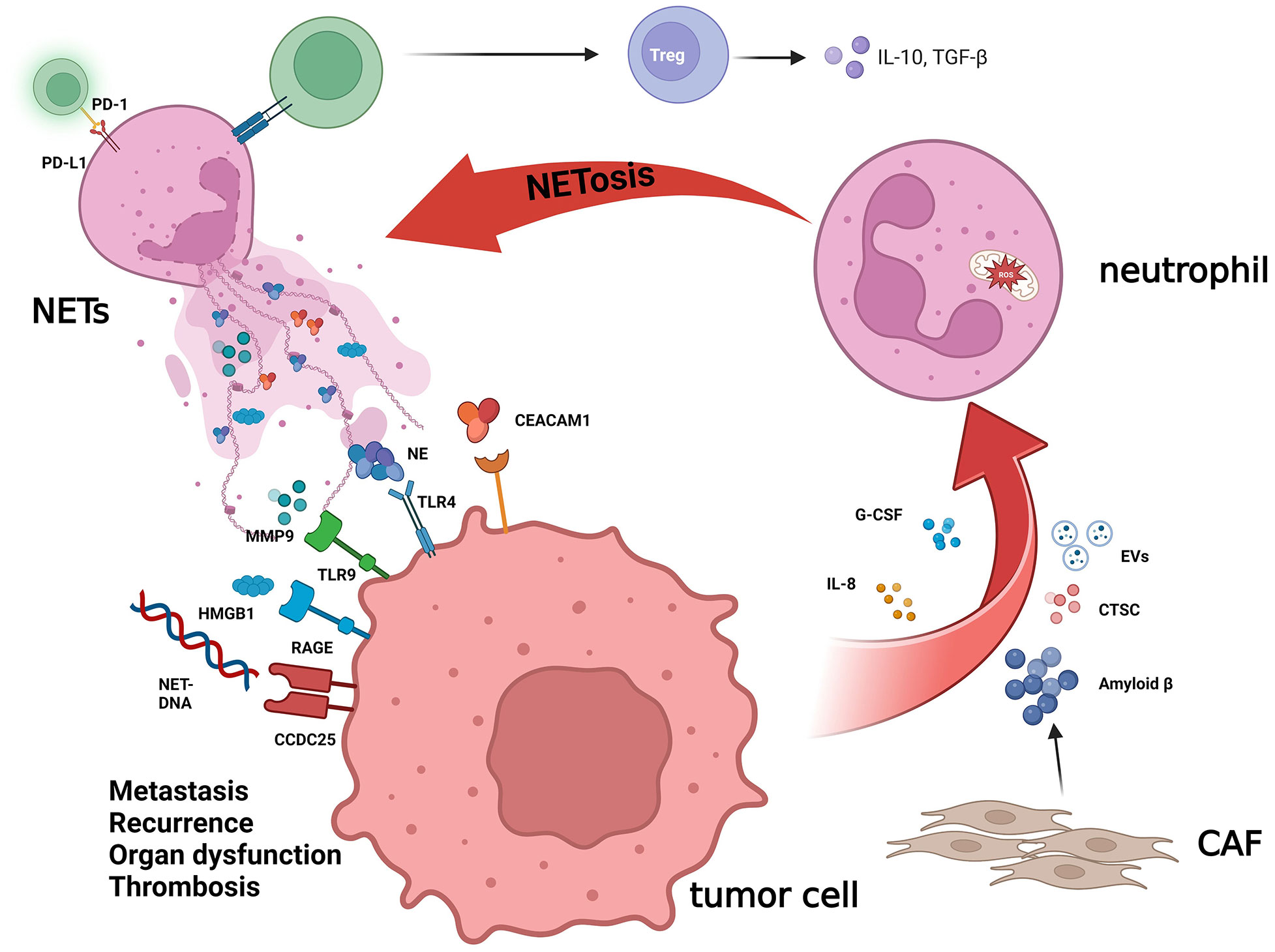
Figure 2 The crucial role of NETs in cancer biology. NETs, a net-like structure produced by neutrophils, play an important role in TME, which can influence cancer biology by cross-talking with tumor cells. NET-DNA, which is the main component of NETs, can interact with CCDC25 on the cytomembrane of tumor cells and then activate the ILK-β-Parvin pathway to promote cell motility. NETs are decorated with HMGB1, and RAGE is the major receptor for HMGB1 in mediating sterile inflammation. The NE, MMP9, and CEACAM1 released by NETs trigger the TLR-4 and TLR-9 receptors on cancer cells, accelerating the growth, metastasis, and recurrence of the tumor by altering the metabolism and “waking up” dormant tumor cells. Other components in the TME also have a mutual effect with NETs. The amyloid β derived from CAF and other factors produced by tumor cells, including IL-8, G-CSF, CTSC, and EVs, will increase the level of NETs. NETs can also promote the differentiation of Treg cells and affect the immune-modulating function of T cells.
Similarly, tumor cells can also impact the formation of NETs by secreting some cytokines and proteins, the most investigated of which are IL-8 and granulocyte colony-stimulating factor (G-CSF) (30, 35–37). Xiao et al. (38) reported that cathepsin C (CTSC), the protease produced by tumor cells, can activate proteinase 3 (PR3) on the neutrophil membrane, to promote interleukin-1β (IL-1β) to process and NF-κB to activate, which can upregulate interleukin-6 (IL-6) and CCL3, recruit neutrophils, and promote the production of ROS in neutrophils to induce NET formation. The extracellular vesicles (EVs) derived from the tumor are deemed to associate with the growth of cancer and modulate the TME and immune function (39, 40). The construction of a mouse model of breast cancer using 4T1 breast cancer cells reveals that 4T1-derived EVs promote the emergence of NETs and accelerate cancer-associated thrombosis in veins (41). Moreover, EVs derived from KRAS-mutated colorectal tumor cells can induce neutrophil recruitment and promote NET formation through IL-8 activation (42). Recently, Guimarães-Bastos et al. (43) revealed that EVs derived from melanoma cells can induce neutrophil chemotaxis, promote TAN polarization to TAN-N2, a pro-tumor population, and facilitate NET formation, thus contributing to tumor progression.
3.2 NETs chat with other components
In addition, as part of the tumor microenvironment, NETs can also interact with other tumor components. Compared with the normal, Zhang et al. (44) revealed that Th17 and interleukin-17 (IL-17) levels were significantly increased in pancreatic cancer and further found that the elevation of IL-17 induced neutrophil recruitment and NET production, which in turn had a suppressive effect on CD8+ T cells. Similarly, Kaltenmeier et al. (45) observed that the immune function of T cells is significantly suppressed function in the TME with a high density of NETs. In vitro, they found that NETs also contained programmed cell death-ligand 1 (PD-L1), which inhibited T-cell function by combining with programmed cell death protein 1 (PD-1) on the T-cell surface, resulting in T-cell dysfunction and metabolic failure, and hence promoting tumor growth.
Cancer-associated fibroblasts (CAFs) are a common composition within the stroma, affecting tumor angiogenesis, stromal remodeling, and antitumor immunity and promoting tumor invasion (46–48). A recent study revealed the impact of CAFs on the formation of NETs (49); the amyloid-β produced by CAFs is involved in the induction of NETs by tumor cells through promoting intra-neutrophil ROS production and supports cancer progression, whereas NETs can also promote liver metastasis of pancreatic tumors by enhancing the migration ability of hepatic stellate cells and forming CAFs (50). DNA derived from NETs (NET-DNA) can also activate the stellate cells in the pancreas then forming fibrous stroma, promoting and enabling tumor proliferation by activating RAGE (51). CCDC25, a transmembrane protein, is a DNA receptor (52–54) and enhances cell motility by activating the ILK-β-Parvin pathway after binding to NET-DNA. Notably, they suggested that metastasis to the liver, but not other organs, was related to a higher level of NET-DNA in breast and colon cancer patients, implying that tumor metastases could be predicted by detecting NET-DNA content in the blood. In addition, Rayes et al. (55) prevented the metastasis of colon cancer by blocking carcinoembryonic Ag cell adhesion molecule 1 (CEACAM1), a component protein of NETs.
3.3 NETs promote metabolic reprogramming
Tumor cells can escape the immune clearance of the body through metabolic reprogramming. Variations in tumor metabolic pathways, including those favoring mitochondrial metabolism as well as oxidative phosphorylation, may allow tumor cells in the TME to cope better with stress (56). New studies show that NETs have an effect on the metabolism of tumor cells (57). NE in NETs can activate TLR-4 on the surface of tumor cells, leading to an accumulation of intracellular PGC1a levels, which enhances the function of mitochondria and accelerates the growth of cancer.
In addition to directly affecting tumor cells’ metabolism, NETs can also change the metabolism of immune cells. Non-alcoholic steatohepatitis (NASH) can be developed into hepatocellular carcinoma (HCC) with or without cirrhosis, Tsung et al. (58) found that those mice whose non-alcoholic steatohepatitis (NASH) was induced by a high-fat diet had greater NETs in the liver at an early stage, which could recruit the macrophages and promote the evolution of liver cancer. Inhibiting NET formation would not influence the development of a fatty liver but decrease the evolution of HCC. Subsequently, Wang et al. (59) used the FoxP3-DTR mouse model to simulate Treg cell clearance and discovered that NETs could induce the development of NASH to HCC by promoting the oxidative phosphorylation of mitochondria within naïve CD4+ T cells and promoting their conversion to Treg cells, which also indicated that NETs could promote the connection between the innate and adaptive immune. Zenlander et al. (60), however, found no significant difference in the levels of NETs in patients with liver cancer that developed from cirrhosis compared with those with only cirrhosis. Also, tumor cells can promote NET production in a ROS-dependent pathway by inducing a transition from TAN to glycolytic and pentose phosphate metabolic pathways (61).
4 Clinical significance of nets
4.1 NETs in the tumor microenvironment
Recently, there has been growing research suggesting that NETs are important part of the tumor microenvironment after discharging from neutrophils and can influence not only the progression of the tumor but also the metastasis and therapy, especially the metastasis of cancer.
It was found that, compared with the normal tissues, the density of NETs was significantly higher in patients with breast, gastric, and lung cancers (62–64). Yang et al. (52) also found that NETs were abundant within liver metastases in cancer patients and the NET levels could be used for early prediction of liver metastases in breast cancer patients. Similarly, it has been reported that the peripheral blood neutrophil-to-lymphocyte ratio (NLR) correlates obviously with NETs in peripheral blood and the density of NETs tends to be higher in patients with lymph node metastases (65). Remarkably, the distribution of NETs was inconsistent in the tumor and its adjacent paraneoplastic tissues, with the highest density of NETs in the center of the tumor and the tendency for both the density of NETs and neutrophils to decrease from the center of the tumor to the stroma (65). It may be since neutrophils within the tumor are more likely to develop NETs (66); this suggests that intra-tumor NETs may be more capable of influencing the tumor. Nevertheless, it was found that cervical cancer with a high density of NETs in the stroma had a better prognosis. At the same time, the level of NET intratumor did not affect the patient’s prognosis (67). Surendran et al. (68) developed a new three-dimensional (3D) tumor-immune microenvironment (TIME)-on-Chip device, which can simulate the TME in vitro to observe the neutrophil response during tumor cell proliferation and invasion. As a result, it was observed that NETs were formed when neutrophils came into contact with tumor cells and that NETs promoted tumor cell clustering and invasion into the stroma, which was more evident with NETs in the stroma. Therefore, it would be a promising field to explore the prognostic effects of NETs at different locations of the tumor.
Tumor metastasis is often the cause of poor prognosis for patients, including lymphatic metastasis and distant organ metastasis; the recurrence and metastasis of tumors can also be associated with NETs. First, epithelial–mesenchymal transition (EMT), a common cause of metastasis, has been demonstrated to be modulated by NETs. Metastasis is always under the regulation of TME changes like inflammation, intravasation of angiogenesis, and cancerous cells, which is described as EMT. EMT enables epithelial cells to obtain a mesenchymal cell phenotype, accelerating the entry of tumor cells into the vascular system and leading to distant metastases (69, 70). Using purified NETs cocultured with colorectal cancer cell lines, Stehr et al. found that NETs could promote the cell motility of CRC cells (71), which was correlated with more mesenchymal biomarkers, and EMT increased the transcription factors while reducing the level of the epithelial biomarkers, such as E-cadherin (CDH1) and epithelial cell adhesion molecule (EPCAM). Similarly, the same results were observed in gastric cancer (63), pancreatic cancer (28), and lung cancer (72). NETs can promote EMT and metastasis in non-small cell lung cancer by inhibiting long non-coding RNA (lncRNA) MIR503HG expression and activating the NF-κB pathway. NETs have also been shown to promote tumor cell entry into the circulatory system by downregulating intercellular tight-junction molecules (73–75). As an important component of the innate immune system, the complement system is significant in the process of tumor growth. Liu et al. (76) found that complement factor 5a (C5a), the downstream product of the C3b-catalyzed cleavage of C5, can recruit neutrophils, and membrane attack complex (MAC), a multiprotein containing several complement compositions, can promote NET formation by activating neutrophils that have contact with vascular endothelium. Then, NETs destroy the endothelial barrier and enhance vascular leakage, facilitating the entry of tumor cells into the blood and causing distant metastasis. Depleting the neutrophils or inhibiting the formation of MAC gives protection to the vascular endothelium and prevents the metastasis.
The alteration of the microenvironment in distant metastatic organs before tumor metastasis is known as pre-metastatic niche (PMN), and it helps to attract circulating tumor cells (CTCs) (77), thus promoting metastasis. There are six characteristics of PMN, namely, immunosuppression, angiogenesis/vascular permeability, inflammation, lymphangiogenesis, organotropism, and metabolic reprogramming. Zeng et al. (78) demonstrated that in situ breast cancer cells can enhance the level of hydroxy acid oxidase 1 (HAO1) in the lung, the rate-limiting enzyme of oxalate metabolism, and promote oxalate generation by activating the TLR3-IRF3 signaling pathway. Oxalate not only promotes the growth of metastatic tumor cells through the MAPK pathway but also activates NADPH oxidase, leading to increased ROS production, thus inducing the production of NETs and promoting the formation of PMN in the lung, making breast cancer more prone to metastasis to the lung. In addition, mesenchymal stem cells (MSCs) in the lung have potent pro-metastatic properties (79). Th2 cells in the lung induce C3 synthesis by MSCs through STAT6, which can induce neutrophil recruitment and NET formation to promote metastasis. By blocking the Th2-STAT6-C3-NET pathway, lung metastasis driven by MSCs was also attenuated. A particular type of neutrophil population was recently identified (80), tumor-associated aged neutrophils, whose cell marker is CXCR4hiCD62Llo. In a constructed tumor metastasis model, tumor cells lead to the accumulation of aged neutrophils by disrupting neutrophil homeostasis and directly stimulating neutrophil aging regulated by angiotensin II. The aged neutrophils can release multiple metastasis-promoting factors like NETs and MMP9, and aged neutrophil permutation can significantly increase liver metastasis of breast cancer and melanoma, which are mediated mainly by NETs. It has been observed that these cells are present not only early in the pre-metastatic microenvironment of the lung but also in the peripheral blood of patients (81). Aged neutrophils induce mitochondrial DNA release by sirtuin 1 (SIRT1), thereby inducing the formation of NETs, rather than the traditional Cit-Histone H3-dependent lytic NET formation, promoting breast cancer lung metastasis. Earlier research also found that the presence of NETs in the peritoneum as well as in the omentum contributes to PMN formation and promotes tumor metastasis (82, 83).
In addition to the above factors contributing to tumor metastasis, surgical operation and postoperative infection have been reported as risk factors for recurrence and metastasis in postoperative patients. For the majority of solid tumors, surgery is the preferred treatment to improve the prognosis of patients (84). However, the study found that whereas the operation removes the primary tumor, it is also deemed to promote the eruption of undetected microscopic lesions, increasing the possibility of recurrence and metastasis after surgery (85), and NETs also participated in the process (86). During the operation, with the destruction of the tumor and its associated blood vessels, some of the tumor cells can flow into the circulation system to form the CTCs, which act as “seeds” in the process of cancer recurrence and metastasis (87). In addition, tissue damage caused by surgery activates the immune and coagulation systems of the body, in which neutrophils, NETs (88), and platelets (89–91) can promote the tissue healing process, but they may also contribute to the spreading and metastasis of tumor. When CTCs enter the peripheral blood, they are rapidly coated by platelets to protect them from external stress and destruction by NK cells. Ren et al. (89) simulated the effects of surgery on the organism by constructing a model of liver ischemia–reperfusion injury (I/R) and showed that platelets were activated by local inflammation caused in I/R through the TLR4-ERK5 pathway and then bound to CTCs to form platelet-tumor cell clusters and that integrins could facilitate the connection of clusters and NETs and promote metastasis (92). In addition to the inflammatory response to tissue damage caused by surgery, postoperative infection, one of the common complications of the surgery, also promotes tumor recurrence and metastasis to some extent (93, 94). Postoperative peritoneal infection in gastric cancer induces NET formation and promotes gastric cancer invasion and metastasis by activating the TGF-β signaling pathway (95). Wang et al. have certificated that elevated LPS levels caused by a postoperative infection in colorectal cancer can induce NET production through the activation of TLR-9 and MAPK signaling pathways, which are closely associated with increased postoperative recurrence rates (96).
The link between cancer and thrombosis has been discovered for decades (97, 98), whereas increased levels of blood clotting factors, tissue factor, and activation of fibrous protein has been described as the mechanism. However, the exact mechanism causing this change is not known. Fuchs et al. (99) revealed that NETs can promote platelet adhesion, aggregation, and activation in the vasculature and induce thrombosis. In the research of Demers et al. (100) in 2012, it was first described that induction of NETs by G-CSF, which was derived from tumor cells, could promote coagulation in tumor patients, leading to cancer-associated thrombosis formation. Subsequent studies (101–104) also demonstrated that neutrophils and NETs contribute to platelet activation and tissue factor synthesis, leading to the formation of venous thrombosis in cancer patients. However, it has been shown in other investigations that NETs only affect the formation of atherothrombosis in tumor patients and do not affect venous thrombosis (41, 105). Therefore, whether NETs affect venous thrombosis in cancer patients and the specific mechanisms involved need further investigation. Other than promoting the formation of cancer-associated thrombosis, some researchers have recently suggested that NETs may also affect the myocardium (106). Using mice with breast cancer, they found a correlation between myocardial dysfunction and NETs in mice, and inhibiting the formation of NETs improved the inflammatory response of the myocardium and decreased the level of biomarkers.
4.2 NETs in cancer therapy
NETs have been previously investigated in sepsis and other inflammatory diseases and can be a more promising target for cancer therapy based on their proliferation-promoting and metastatic effects on tumors as well as their impact on the TME. The current therapeutic approach to NETs consists of two main aspects, inhibition of NET formation or destruction of formed NETs. NET-DNA from the nucleus and mitochondria, which is the vital composition of NETs, can be hydrolyzed by DNase I. NET-DNA can accelerate the growth and metastasis of cancer after binding to CCDC25, and destroying NETs by using DNase I is frequently used in current trials (107, 108). Many recent studies proved that heparin can promote the degradation of NETs by detaching histones from the NET-DNA skeleton (99); the use of low molecular heparin can hinder the formation of NETs induced by PMA (109). However, a recent study found that heparin can induce NET formation in vitro (110). This suggests that further research is expected to confirm whether low molecular heparin can be used to degrade NETs and by what mechanism.
Interfering with the formation of NETs can be a positive strategy instead of degrading the formed NETs by suppressing the compositions crucial for the NETs, such as PAD4, NE, or MPO (Table 1). Chromatin densification is the most pivotal process in the formation of NETs, and it is dependent on the existence of PAD4 (57). Lewis et al. (114) have recommended two inhibitors of the PAD4, especially GSK484, which can suppress disease by destroying NETs. NE and MPO are the critical compositions of NETs, and mouse models lacking NE have been utilized to study the effects of decreased NETs on cancer metastasis (18, 86) and sepsis (123). With regard to MPO, which is usually viewed as a marker of NETs, it can also influence the NETs. Mice treated with MPO inhibitors could not form NETs and are always utilized to observe the impacts of NETs on cancer. Based on the increasing research on NETs and tumors, specific blockade of the interplay has become a new therapeutic option. Blocking NETs or tumor cell-derived factors, including IL-8, IL-17, and their receptors, has been shown to affect the biological behavior of cancer (44, 124–126).
Furthermore, NETs have been demonstrated to play a role in tumor treatment resistance, including chemotherapy resistance (127), immunotherapy resistance (44, 128, 129), and radiation therapy resistance (130). NETs were observed in radiation-resistant bladder cancer patients compared with the radiation-sensitive patients, and inhibiting HMGB1 and NETs significantly improved the outcome of radiation therapy (130). The TME has a vital role in cancer immunity and probably helps to inhibit the effects of immune checkpoint inhibitors and other new immunotherapies in terminal cancer patients. Zhang et al. (129) used DNase I to degrades NETs that could decrease the resistance to anti-PD-1 therapy in a CRC model. However, a study by Liu et al. (131) indicated that NETs show a novel immunomodulatory role in Bacillus Calmette-Guerin (BCG) immunotherapy. Tumor cells activated by BCG can induce NETs through their production of IL-8 and TNFα, and these NETs help to recruit T cells and macrophages and repair damaged tissue, inducing tumor cell apoptosis and cell cycle arrest.
5 Conclusion
Despite the multiple treatment options available for cancer, recurrence, and metastasis are currently still the most common cause of patient death. With intensive research in recent years, the TME has been recognized as the main influencing factor of tumor behavior. The cellular debris produced by apoptosis and necrosis of tumor cells leads to local inflammatory reactions, activating the innate immune response and recruiting neutrophils. NETosis is a vital way by which neutrophils function, but research on the impacts of NETs on tumor cells is still in its infancy. Although a few studies have suggested that NETs have some antitumor effects, it is clear that NETs play a role in promoting the proliferation, invasion, and metastasis of tumors, and even the effectiveness of chemotherapy, radiotherapy, and immunotherapy on cancer patients is related to NETs.
There are mounts of mechanisms underlying NET-dependent tumor progression and metastasis. As reviewed herein, NETs and tumor cells in the TME have been shown to interact through the production of multiple factors, proteins, and their receptors. All results of different studies listed in this review imply the need for further studies about the interaction between NETs and tumors. Although there are some indications that NETs are associated with prognosis in cancer patients, there is still a lack of relevant clinical trials. In addition, it should be noted that neutrophils and NETs are important components of innate immunity, and inhibition of NET formation or destruction of formed NETs may affect neutrophils and reduce pathogenic clearance. Hence, the development of therapies that can accurately target NETs within the tumor without adversely affecting immune function is necessary. Taken together, the emerging role of NETs in cancer diagnosis, growth, invasion, metastasis, and therapy should attract enough attention.
Author contributions
WZ designed and wrote the manuscript and drafted the figures, QW searched relevant literature. XS and JD supervised this review. All authors have read and agreed to the published version of the manuscript.
Funding
This work was supported by the National Natural Science Foundation of China (81870393, JD and No.81970500, XS).
Conflict of interest
The authors declare that the research was conducted in the absence of any commercial or financial relationships that could be construed as a potential conflict of interest.
Publisher’s note
All claims expressed in this article are solely those of the authors and do not necessarily represent those of their affiliated organizations, or those of the publisher, the editors and the reviewers. Any product that may be evaluated in this article, or claim that may be made by its manufacturer, is not guaranteed or endorsed by the publisher.
References
2. Quail DF, Joyce JA. Microenvironmental regulation of tumor progression and metastasis. Nat Med (2013) 19:1423–37. doi: 10.1038/nm.3394
3. Joyce JA, Pollard JW. Microenvironmental regulation of metastasis. Nat Rev Cancer (2009) 9:239–52. doi: 10.1038/nrc2618
4. Kolaczkowska E, Kubes P. Neutrophil recruitment and function in health and inflammation. Nat Rev Immunol (2013) 13:159–75. doi: 10.1038/nri3399
5. Mantovani A, Cassatella MA, Costantini C, Jaillon S. Neutrophils in the activation and regulation of innate and adaptive immunity. Nat Rev Immunol (2011) 11:519–31. doi: 10.1038/nri3024
6. Hanahan D, Weinberg RA. Hallmarks of cancer: the next generation. Cell (2011) 144:646–74. doi: 10.1016/j.cell.2011.02.013
7. Coffelt SB, Wellenstein MD, de Visser KE. Neutrophils in cancer: neutral no more. Nat Rev Cancer (2016) 16:431–46. doi: 10.1038/nrc.2016.52
8. Coffelt SB, Kersten K, Doornebal CW, Weiden J, Vrijland K, Hau C-S, et al. IL-17-producing γδ T cells and neutrophils conspire to promote breast cancer metastasis. Nature (2015) 522:345–8. doi: 10.1038/nature14282
9. Jaillon S, Ponzetta A, Di Mitri D, Santoni A, Bonecchi R, Mantovani A. Neutrophil diversity and plasticity in tumour progression and therapy. Nat Rev Cancer (2020) 20:485–503. doi: 10.1038/s41568-020-0281-y
10. Fridlender ZG, Sun J, Kim S, Kapoor V, Cheng G, Ling L, et al. Polarization of tumor-associated neutrophil phenotype by TGF-beta: “N1” versus “N2” TAN. Cancer Cell (2009) 16:183–94. doi: 10.1016/j.ccr.2009.06.017
11. Brinkmann V, Reichard U, Goosmann C, Fauler B, Uhlemann Y, Weiss DS, et al. Neutrophil extracellular traps kill bacteria. Science (2004) 303:1532–5. doi: 10.1126/science.1092385
12. Fuchs TA, Abed U, Goosmann C, Hurwitz R, Schulze I, Wahn V, et al. Novel cell death program leads to neutrophil extracellular traps. J Cell Biol (2007) 176:231–41. doi: 10.1083/jcb.200606027
13. Ronchetti L, Boubaker NS, Barba M, Vici P, Gurtner A, Piaggio G. Neutrophil extracellular traps in cancer: not only catching microbes. J Exp Clin Cancer Res CR (2021) 40:231. doi: 10.1186/s13046-021-02036-z
14. Honda M, Kubes P. Neutrophils and neutrophil extracellular traps in the liver and gastrointestinal system. Nat Rev Gastroenterol Hepatol (2018) 15:206–21. doi: 10.1038/nrgastro.2017.183
15. Papayannopoulos V. Neutrophil extracellular traps in immunity and disease. Nat Rev Immunol (2018) 18:134–47. doi: 10.1038/nri.2017.105
16. Wong SL, Demers M, Martinod K, Gallant M, Wang Y, Goldfine AB, et al. Diabetes primes neutrophils to undergo NETosis, which impairs wound healing. Nat Med (2015) 21:815–9. doi: 10.1038/nm.3887
17. Papayannopoulos V, Metzler KD, Hakkim A, Zychlinsky A. Neutrophil elastase and myeloperoxidase regulate the formation of neutrophil extracellular traps. J Cell Biol (2010) 191:677–91. doi: 10.1083/jcb.201006052
18. Rayes RF, Mouhanna JG, Nicolau I, Bourdeau F, Giannias B, Rousseau S, et al. Primary tumors induce neutrophil extracellular traps with targetable metastasis-promoting effects. JCI Insight (2019) 4:e128008. doi: 10.1172/jci.insight.128008
19. Guan X, Lu Y, Zhu H, Yu S, Zhao W, Chi X, et al. The crosstalk between cancer cells and neutrophils enhances hepatocellular carcinoma metastasis via neutrophil extracellular traps-associated cathepsin G component: a potential therapeutic target. J Hepatocell Carcinoma (2021) 8:451–65. doi: 10.2147/JHC.S303588
20. Yipp BG, Petri B, Salina D, Jenne CN, Scott BNV, Zbytnuik LD, et al. Infection-induced NETosis is a dynamic process involving neutrophil multitasking. vivo Nat Med (2012) 18:1386–93. doi: 10.1038/nm.2847
21. Pilsczek FH, Salina D, Poon KKH, Fahey C, Yipp BG, Sibley CD, et al. A novel mechanism of rapid nuclear neutrophil extracellular trap formation in response to staphylococcus aureus. J Immunol Baltim Md 1950 (2010) 185:7413–25. doi: 10.4049/jimmunol.1000675
22. Byrd AS, O’Brien XM, Johnson CM, Lavigne LM, Reichner JS. An extracellular matrix-based mechanism of rapid neutrophil extracellular trap formation in response to candida albicans. J Immunol Baltim Md 1950 (2013) 190:4136–48. doi: 10.4049/jimmunol.1202671
23. Yipp BG, Kubes P. NETosis: how vital is it? Blood (2013) 122:2784–94. doi: 10.1182/blood-2013-04-457671
24. de Buhr N, von Köckritz-Blickwede M. How neutrophil extracellular traps become visible. J Immunol Res (2016) 2016:4604713. doi: 10.1155/2016/4604713
25. Yousefi S, Mihalache C, Kozlowski E, Schmid I, Simon HU. Viable neutrophils release mitochondrial DNA to form neutrophil extracellular traps. Cell Death Differ (2009) 16:1438–44. doi: 10.1038/cdd.2009.96
26. Itagaki K, Kaczmarek E, Lee YT, Tang IT, Isal B, Adibnia Y, et al. Mitochondrial DNA released by trauma induces neutrophil extracellular traps. PloS One (2015) 10:e0120549. doi: 10.1371/journal.pone.0120549
27. McIlroy DJ, Jarnicki AG, Au GG, Lott N, Smith DW, Hansbro PM, et al. Mitochondrial DNA neutrophil extracellular traps are formed after trauma and subsequent surgery. J Crit Care (2014) 29:1133.e1–1133.e5. doi: 10.1016/j.jcrc.2014.07.013
28. Kajioka H, Kagawa S, Ito A, Yoshimoto M, Sakamoto S, Kikuchi S, et al. Targeting neutrophil extracellular traps with thrombomodulin prevents pancreatic cancer metastasis. Cancer Lett (2021) 497:1–13. doi: 10.1016/j.canlet.2020.10.015
29. Zha C, Meng X, Li L, Mi S, Qian D, Li Z, et al. Neutrophil extracellular traps mediate the crosstalk between glioma progression and the tumor microenvironment via the HMGB1/RAGE/IL-8 axis. Cancer Biol Med (2020) 17:154–68. doi: 10.20892/j.issn.2095-3941.2019.0353
30. Zhu B, Zhang X, Sun S, Fu Y, Xie L, Ai P. NF-κB and neutrophil extracellular traps cooperate to promote breast cancer progression and metastasis. Exp Cell Res (2021) 405:112707. doi: 10.1016/j.yexcr.2021.112707
31. Yang L, Liu L, Zhang R, Hong J, Wang Y, Wang J, et al. IL-8 mediates a positive loop connecting increased neutrophil extracellular traps (NETs) and colorectal cancer liver metastasis. J Cancer (2020) 11:4384–96. doi: 10.7150/jca.44215
32. Tohme S, Yazdani HO, Al-Khafaji AB, Chidi AP, Loughran P, Mowen K, et al. Neutrophil extracellular traps promote the development and progression of liver metastases after surgical stress. Cancer Res (2016) 76:1367–80. doi: 10.1158/0008-5472.CAN-15-1591
33. Yang L-Y, Luo Q, Lu L, Zhu W-W, Sun H-T, Wei R, et al. Increased neutrophil extracellular traps promote metastasis potential of hepatocellular carcinoma via provoking tumorous inflammatory response. J Hematol OncolJ Hematol Oncol (2020) 13:3. doi: 10.1186/s13045-019-0836-0
34. Albrengues J, Shields MA, Ng D, Park CG, Ambrico A, Poindexter ME, et al. Neutrophil extracellular traps produced during inflammation awaken dormant cancer cells in mice. Science (2018) 361:eaao4227. doi: 10.1126/science.aao4227
35. Li Y, Yuan R, Ren T, Yang B, Miao H, Liu L, et al. Role of sciellin in gallbladder cancer proliferation and formation of neutrophil extracellular traps. Cell Death Dis (2021) 12:30. doi: 10.1038/s41419-020-03286-z
36. de Andrea CE, Ochoa MC, Villalba-Esparza M, Teijeira Á, Schalper KA, Abengozar-Muela M, et al. Heterogenous presence of neutrophil extracellular traps in human solid tumours is partially dependent on IL-8. J Pathol (2021) 255:190–201. doi: 10.1002/path.5753
37. Gomes T, Várady CBS, Lourenço AL, Mizurini DM, Rondon AMR, Leal AC, et al. IL-1β blockade attenuates thrombosis in a neutrophil extracellular trap-dependent breast cancer model. Front Immunol (2019) 10:2088. doi: 10.3389/fimmu.2019.02088
38. Xiao Y, Cong M, Li J, He D, Wu Q, Tian P, et al. Cathepsin c promotes breast cancer lung metastasis by modulating neutrophil infiltration and neutrophil extracellular trap formation. Cancer Cell (2021) 39:423–437.e7. doi: 10.1016/j.ccell.2020.12.012
39. van der Pol E, Böing AN, Harrison P, Sturk A, Nieuwland R. Classification, functions, and clinical relevance of extracellular vesicles. Pharmacol Rev (2012) 64:676–705. doi: 10.1124/pr.112.005983
40. Colombo M, Raposo G, Théry C. Biogenesis, secretion, and intercellular interactions of exosomes and other extracellular vesicles. Annu Rev Cell Dev Biol (2014) 30:255–89. doi: 10.1146/annurev-cellbio-101512-122326
41. Leal A, Mizurini D, Gomes T, Rochael N, Saraiva E, Dias M, et al. Tumor-derived exosomes induce the formation of neutrophil extracellular traps: implications for the establishment of cancer-associated thrombosis. Sci Rep (2017) 7:6438. doi: 10.1038/s41598-017-06893-7
42. Shang A, Gu C, Zhou C, Yang Y, Chen C, Zeng B, et al. Exosomal KRAS mutation promotes the formation of tumor-associated neutrophil extracellular traps and causes deterioration of colorectal cancer by inducing IL-8 expression. Cell Commun Signal CCS (2020) 18:52. doi: 10.1186/s12964-020-0517-1
43. Guimarães-Bastos D, Frony AC, Barja-Fidalgo C, Moraes JA. Melanoma-derived extracellular vesicles skew neutrophils into a pro-tumor phenotype. J Leukoc Biol (2022) 111:585–96. doi: 10.1002/JLB.3A0120-050RR
44. Zhang Y, Chandra V, Riquelme Sanchez E, Dutta P, Quesada PR, Rakoski A, et al. Interleukin-17-induced neutrophil extracellular traps mediate resistance to checkpoint blockade in pancreatic cancer. J Exp Med (2020) 217:e20190354. doi: 10.1084/jem.20190354
45. Kaltenmeier C, Yazdani HO, Morder K, Geller DA, Simmons RL, Tohme S. Neutrophil extracellular traps promote T cell exhaustion in the tumor microenvironment. Front Immunol (2021) 12:785222. doi: 10.3389/fimmu.2021.785222
46. Harper J, Sainson RCA. Regulation of the anti-tumour immune response by cancer-associated fibroblasts. Semin Cancer Biol (2014) 25:69–77. doi: 10.1016/j.semcancer.2013.12.005
47. Valkenburg KC, de Groot AE, Pienta KJ. Targeting the tumour stroma to improve cancer therapy. Nat Rev Clin Oncol (2018) 15:366–81. doi: 10.1038/s41571-018-0007-1
48. Wang Q, Shen X, Chen G, Du J. Drug resistance in colorectal cancer: from mechanism to clinic. Cancers (2022) 14:2928. doi: 10.3390/cancers14122928
49. Munir H, Jones JO, Janowitz T, Hoffmann M, Euler M, Martins CP, et al. Stromal-driven and amyloid β-dependent induction of neutrophil extracellular traps modulates tumor growth. Nat Commun (2021) 12:683. doi: 10.1038/s41467-021-20982-2
50. Takesue S, Ohuchida K, Shinkawa T, Otsubo Y, Matsumoto S, Sagara A, et al. Neutrophil extracellular traps promote liver micrometastasis in pancreatic ductal adenocarcinoma via the activation of cancer−associated fibroblasts. Int J Oncol (2020) 56:596–605. doi: 10.3892/ijo.2019.4951
51. Miller-Ocuin JL, Liang X, Boone BA, Doerfler WR, Singhi AD, Tang D, et al. DNA Released from neutrophil extracellular traps (NETs) activates pancreatic stellate cells and enhances pancreatic tumor growth. Oncoimmunology (2019) 8:e1605822. doi: 10.1080/2162402X.2019.1605822
52. Yang L, Liu Q, Zhang X, Liu X, Zhou B, Chen J, et al. DNA Of neutrophil extracellular traps promotes cancer metastasis via CCDC25. Nature (2020) 583:133–8. doi: 10.1038/s41586-020-2394-6
53. Liu R, Zhao E, Wang F, Cui H. CCDC25: precise navigator for neutrophil extracellular traps on the prometastatic road. Signal Transduct Target Ther (2020) 5:162. doi: 10.1038/s41392-020-00285-6
54. Tang Q, Liang B, Zhang L, Li X, Li H, Jing W, et al. Enhanced CHOLESTEROL biosynthesis promotes breast cancer metastasis via modulating CCDC25 expression and neutrophil extracellular traps formation. Sci Rep (2022) 12:17350. doi: 10.1038/s41598-022-22410-x
55. Rayes RF, Vourtzoumis P, Bou Rjeily M, Seth R, Bourdeau F, Giannias B, et al. Neutrophil extracellular trap-associated CEACAM1 as a putative therapeutic target to prevent metastatic progression of colon carcinoma. J Immunol Baltim Md 1950 (2020) 204:2285–94. doi: 10.4049/jimmunol.1900240
56. Savchenko AS, Borissoff JI, Martinod K, De Meyer SF, Gallant M, Erpenbeck L, et al. VWF-mediated leukocyte recruitment with chromatin decondensation by PAD4 increases myocardial ischemia/reperfusion injury in mice. Blood (2014) 123:141–8. doi: 10.1182/blood-2013-07-514992
57. Yazdani HO, Roy E, Comerci AJ, van der Windt DJ, Zhang H, Huang H, et al. Neutrophil extracellular traps drive mitochondrial homeostasis in tumors to augment growth. Cancer Res (2019) 79:5626–39. doi: 10.1158/0008-5472.CAN-19-0800
58. van der Windt DJ, Sud V, Zhang H, Varley PR, Goswami J, Yazdani HO, et al. Neutrophil extracellular traps promote inflammation and development of hepatocellular carcinoma in nonalcoholic steatohepatitis. Hepatology (2018) 68:1347–60. doi: 10.1002/hep.29914
59. Wang H, Zhang H, Wang Y, Brown ZJ, Xia Y, Huang Z, et al. Regulatory T-cell and neutrophil extracellular trap interaction contributes to carcinogenesis in non-alcoholic steatohepatitis. J Hepatol (2021) 75:1271–83. doi: 10.1016/j.jhep.2021.07.032
60. Zenlander R, Havervall S, Magnusson M, Engstrand J, Ågren A, Thålin C, et al. Neutrophil extracellular traps in patients with liver cirrhosis and hepatocellular carcinoma. Sci Rep (2021) 11:18025. doi: 10.1038/s41598-021-97233-3
61. Jiang Z-Z, Peng Z-P, Liu X-C, Guo H-F, Zhou M-M, Jiang D, et al. Neutrophil extracellular traps induce tumor metastasis through dual effects on cancer and endothelial cells. Oncoimmunology (2022) 11:2052418. doi: 10.1080/2162402X.2022.2052418
62. Rivera-Franco MM, Leon-Rodriguez E, Torres-Ruiz JJ, Gómez-Martín D, Angles-Cano E, de la Luz Sevilla-González M. Neutrophil extracellular traps associate with clinical stages in breast cancer. Pathol Oncol Res POR (2020) 26:1781–5. doi: 10.1007/s12253-019-00763-5
63. Zhu T, Zou X, Yang C, Li L, Wang B, Li R, et al. Neutrophil extracellular traps promote gastric cancer metastasis by inducing epithelial−mesenchymal transition. Int J Mol Med (2021) 48:127. doi: 10.3892/ijmm.2021.4960
64. Zhang L, Yi H, Chen J, Li H, Luo Y, Cheng T, et al. Neutrophil extracellular traps facilitate A549 cell invasion and migration in a macrophage-maintained inflammatory microenvironment. BioMed Res Int (2022) 2022:8316525. doi: 10.1155/2022/8316525
65. Zhang Y, Hu Y, Ma C, Sun H, Wei X, Li M, et al. Diagnostic, therapeutic predictive, and prognostic value of neutrophil extracellular traps in patients with gastric adenocarcinoma. Front Oncol (2020) 10:1036. doi: 10.3389/fonc.2020.01036
66. Li M, Lin C, Leso A, Nefedova Y. Quantification of citrullinated histone H3 bound DNA for detection of neutrophil extracellular traps. Cancers (2020) 12:E3424. doi: 10.3390/cancers12113424
67. Yan B, Dai X, Ma Q, Wu X. Stromal neutrophil extracellular trap density is an independent prognostic factor for cervical cancer recurrence. Front Oncol (2021) 11:659445. doi: 10.3389/fonc.2021.659445
68. Surendran V, Rutledge D, Colmon R, Chandrasekaran A. A novel tumor-immune microenvironment (TIME)-on-Chip mimics three dimensional neutrophil-tumor dynamics and neutrophil extracellular traps (NETs)-mediated collective tumor invasion. Biofabrication (2021) 13:035029. doi: 10.1088/1758-5090/abe1cf
69. Gupta GP, Massagué J. Cancer metastasis: building a framework. Cell (2006) 127:679–95. doi: 10.1016/j.cell.2006.11.001
70. Pastushenko I, Blanpain C. EMT transition states during tumor progression and metastasis. Trends Cell Biol (2019) 29:212–26. doi: 10.1016/j.tcb.2018.12.001
71. Stehr AM, Wang G, Demmler R, Stemmler MP, Krug J, Tripal P, et al. Neutrophil extracellular traps drive epithelial-mesenchymal transition of human colon cancer. J Pathol (2022) 256:455–67. doi: 10.1002/path.5860
72. Wang Y, Liu F, Chen L, Fang C, Li S, Yuan S, et al. Neutrophil extracellular traps (NETs) promote non-small cell lung cancer metastasis by suppressing lncRNA MIR503HG to activate the NF-κB/NLRP3 inflammasome pathway. Front Immunol (2022) 13:867516. doi: 10.3389/fimmu.2022.867516
73. Yu M, Li T, Li B, Liu Y, Wang L, Zhang J, et al. Phosphatidylserine-exposing blood cells, microparticles and neutrophil extracellular traps increase procoagulant activity in patients with pancreatic cancer. Thromb Res (2020) 188:5–16. doi: 10.1016/j.thromres.2020.01.025
74. Li Y, Yang Y, Gan T, Zhou J, Hu F, Hao N, et al. Extracellular RNAs from lung cancer cells activate epithelial cells and induce neutrophil extracellular traps. Int J Oncol (2019) 55:69–80. doi: 10.3892/ijo.2019.4808
75. McDowell SAC, Luo RBE, Arabzadeh A, Doré S, Bennett NC, Breton V, et al. Neutrophil oxidative stress mediates obesity-associated vascular dysfunction and metastatic transmigration. Nat Cancer (2021) 2:545–62. doi: 10.1038/s43018-021-00194-9
76. Liu X, Wang Y, Bauer AT, Kirschfink M, Ding P, Gebhardt C, et al. Neutrophils activated by membrane attack complexes increase the permeability of melanoma blood vessels. Proc Natl Acad Sci U.S.A. (2022) 119:e2122716119. doi: 10.1073/pnas.2122716119
77. Liu Y, Cao X. Characteristics and significance of the pre-metastatic niche. Cancer Cell (2016) 30:668–81. doi: 10.1016/j.ccell.2016.09.011
78. Zeng Z, Xu S, Wang F, Peng X, Zhang W, Zhan Y, et al. HAO1-mediated oxalate metabolism promotes lung pre-metastatic niche formation by inducing neutrophil extracellular traps. Oncogene (2022) 41:3719–31. doi: 10.1038/s41388-022-02248-3
79. Zheng Z, Li Y-N, Jia S, Zhu M, Cao L, Tao M, et al. Lung mesenchymal stromal cells influenced by Th2 cytokines mobilize neutrophils and facilitate metastasis by producing complement C3. Nat Commun (2021) 12:6202. doi: 10.1038/s41467-021-26460-z
80. Peng Z, Liu C, Victor AR, Cao D-Y, Veiras LC, Bernstein EA, et al. Tumors exploit CXCR4(hi)CD62L(lo) aged neutrophils to facilitate metastatic spread. Oncoimmunology (2021) 10:1870811. doi: 10.1080/2162402X.2020.1870811
81. Yang C, Wang Z, Li L, Zhang Z, Jin X, Wu P, et al. Aged neutrophils form mitochondria-dependent vital NETs to promote breast cancer lung metastasis. J Immunother Cancer (2021) 9:e002875. doi: 10.1136/jitc-2021-002875
82. Lee W, Ko SY, Mohamed MS, Kenny HA, Lengyel E, Naora H. Neutrophils facilitate ovarian cancer premetastatic niche formation in the omentum. J Exp Med (2019) 216:176–94. doi: 10.1084/jem.20181170
83. Kanamaru R, Ohzawa H, Miyato H, Matsumoto S, Haruta H, Kurashina K, et al. Low density neutrophils (LDN) in postoperative abdominal cavity assist the peritoneal recurrence through the production of neutrophil extracellular traps (NETs). Sci Rep (2018) 8:632. doi: 10.1038/s41598-017-19091-2
84. Bleicher RJ, Ruth K, Sigurdson ER, Beck JR, Ross E, Wong Y-N, et al. Time to surgery and breast cancer survival in the united states. JAMA Oncol (2016) 2:330–9. doi: 10.1001/jamaoncol.2015.4508
85. Neeman E, Ben-Eliyahu S. Surgery and stress promote cancer metastasis: new outlooks on perioperative mediating mechanisms and immune involvement. Brain Behav Immun (2013) 30 Suppl:S32–40. doi: 10.1016/j.bbi.2012.03.006
86. Cools-Lartigue J, Spicer J, McDonald B, Gowing S, Chow S, Giannias B, et al. Neutrophil extracellular traps sequester circulating tumor cells and promote metastasis. J Clin Invest (2013) 123:67484. doi: 10.1172/JCI67484
87. Martin OA, Anderson RL, Narayan K, MacManus MP. Does the mobilization of circulating tumour cells during cancer therapy cause metastasis? Nat Rev Clin Oncol (2017) 14:32–44. doi: 10.1038/nrclinonc.2016.128
88. Zhang W, Qin T, Yang Z, Yin L, Zhao C, Feng L, et al. Telomerase-positive circulating tumor cells are associated with poor prognosis via a neutrophil-mediated inflammatory immune environment in glioma. BMC Med (2021) 19:277. doi: 10.1186/s12916-021-02138-7
89. Ren J, He J, Zhang H, Xia Y, Hu Z, Loughran P, et al. Platelet TLR4-ERK5 axis facilitates NET-mediated capturing of circulating tumor cells and distant metastasis after surgical stress. Cancer Res (2021) 81:2373–85. doi: 10.1158/0008-5472.CAN-20-3222
90. Gay LJ, Felding-Habermann B. Contribution of platelets to tumour metastasis. Nat Rev Cancer (2011) 11:123–34. doi: 10.1038/nrc3004
91. Schlesinger M. Role of platelets and platelet receptors in cancer metastasis. J Hematol OncolJ Hematol Oncol (2018) 11:125. doi: 10.1186/s13045-018-0669-2
92. Najmeh S, Cools-Lartigue J, Rayes RF, Gowing S, Vourtzoumis P, Bourdeau F, et al. Neutrophil extracellular traps sequester circulating tumor cells via β1-integrin mediated interactions. Int J Cancer (2017) 140:2321–30. doi: 10.1002/ijc.30635
93. Kubota T, Hiki N, Sano T, Nomura S, Nunobe S, Kumagai K, et al. Prognostic significance of complications after curative surgery for gastric cancer. Ann Surg Oncol (2014) 21:891–8. doi: 10.1245/s10434-013-3384-9
94. Kanda M, Ito S, Mochizuki Y, Teramoto H, Ishigure K, Murai T, et al. Multi-institutional analysis of the prognostic significance of postoperative complications after curative resection for gastric cancer. Cancer Med (2019) 8:5194–201. doi: 10.1002/cam4.2439
95. Xia X, Zhang Z, Zhu C, Ni B, Wang S, Yang S, et al. Neutrophil extracellular traps promote metastasis in gastric cancer patients with postoperative abdominal infectious complications. Nat Commun (2022) 13:1017. doi: 10.1038/s41467-022-28492-5
96. Wang W-W, Wu L, Lu W, Chen W, Yan W, Qi C, et al. Lipopolysaccharides increase the risk of colorectal cancer recurrence and metastasis due to the induction of neutrophil extracellular traps after curative resection. J Cancer Res Clin Oncol (2021) 147:2609–19. doi: 10.1007/s00432-021-03682-8
97. Zwicker JI, Furie BC, Furie B. Cancer-associated thrombosis. Crit Rev Oncol Hematol (2007) 62:126–36. doi: 10.1016/j.critrevonc.2007.01.001
98. Connolly GC, Khorana AA. Emerging risk stratification approaches to cancer-associated thrombosis: risk factors, biomarkers and a risk score. Thromb Res (2010) 125 Suppl 2:S1–7. doi: 10.1016/S0049-3848(10)00227-6
99. Fuchs TA, Brill A, Duerschmied D, Schatzberg D, Monestier M, Myers DD, et al. Extracellular DNA traps promote thrombosis. Proc Natl Acad Sci (2010) 107:15880–5. doi: 10.1073/pnas.1005743107
100. Demers M, Krause DS, Schatzberg D, Martinod K, Voorhees JR, Fuchs TA, et al. Cancers predispose neutrophils to release extracellular DNA traps that contribute to cancer-associated thrombosis. Proc Natl Acad Sci U.S.A. (2012) 109:13076–81. doi: 10.1073/pnas.1200419109
101. Hisada Y, Grover SP, Maqsood A, Houston R, Ay C, Noubouossie DF, et al. Neutrophils and neutrophil extracellular traps enhance venous thrombosis in mice bearing human pancreatic tumors. Haematologica (2020) 105:218–25. doi: 10.3324/haematol.2019.217083
102. Boone BA, Murthy P, Miller-Ocuin J, Doerfler WR, Ellis JT, Liang X, et al. Chloroquine reduces hypercoagulability in pancreatic cancer through inhibition of neutrophil extracellular traps. BMC Cancer (2018) 18:678. doi: 10.1186/s12885-018-4584-2
103. Cedervall J, Zhang Y, Huang H, Zhang L, Femel J, Dimberg A, et al. Neutrophil extracellular traps accumulate in peripheral blood vessels and compromise organ function in tumor-bearing animals. Cancer Res (2015) 75:2653–62. doi: 10.1158/0008-5472.CAN-14-3299
104. Carminita E, Crescence L, Brouilly N, Altié A, Panicot-Dubois L, Dubois C. DNAse-dependent, NET-independent pathway of thrombus formation. vivo Proc Natl Acad Sci (2021) 118:e2100561118. doi: 10.1073/pnas.2100561118
105. Oklu R, Sheth RA, Wong KHK, Jahromi AH, Albadawi H. Neutrophil extracellular traps are increased in cancer patients but does not associate with venous thrombosis. Cardiovasc Diagn Ther (2017) 7:S140–9. doi: 10.21037/cdt.2017.08.01
106. Cedervall J, Herre M, Dragomir A, Rabelo-Melo F, Svensson A, Thålin C, et al. Neutrophil extracellular traps promote cancer-associated inflammation and myocardial stress. Oncoimmunology (2022) 11:2049487. doi: 10.1080/2162402X.2022.2049487
107. Park J, Wysocki RW, Amoozgar Z, Maiorino L, Fein MR, Jorns J, et al. Cancer cells induce metastasis-supporting neutrophil extracellular DNA traps. Sci Transl Med (2016) 8:361ra138. doi: 10.1126/scitranslmed.aag1711
108. Shi L, Yao H, Liu Z, Xu M, Tsung A, Wang Y. Endogenous PAD4 in breast cancer cells mediates cancer extracellular chromatin network formation and promotes lung metastasis. Mol Cancer Res MCR (2020) 18:735–47. doi: 10.1158/1541-7786.MCR-19-0018
109. Manfredi AA, Rovere-Querini P, D’Angelo A, Maugeri N. Low molecular weight heparins prevent the induction of autophagy of activated neutrophils and the formation of neutrophil extracellular traps. Pharmacol Res (2017) 123:146–56. doi: 10.1016/j.phrs.2016.08.008
110. Lelliott PM, Momota M, Shibahara T, Lee MSJ, Smith NI, Ishii KJ, et al. Heparin induces neutrophil elastase-dependent vital and lytic NET formation. Int Immunol (2020) 32:359–68. doi: 10.1093/intimm/dxz084
111. Zeng J, Xu H, Fan P-Z, Xie J, He J, Yu J, et al. Kaempferol blocks neutrophil extracellular traps formation and reduces tumour metastasis by inhibiting ROS-PAD4 pathway. J Cell Mol Med (2020) 24:7590–9. doi: 10.1111/jcmm.15394
112. Li M, Lin C, Deng H, Strnad J, Bernabei L, Vogl DT, et al. A novel peptidylarginine deiminase 4 (PAD4) inhibitor BMS-P5 blocks formation of neutrophil extracellular traps and delays progression of multiple myeloma. Mol Cancer Ther (2020) 19:1530–8. doi: 10.1158/1535-7163.MCT-19-1020
113. Telerman A, Granot G, Leibovitch C, Yarchovsky-Dolberg O, Shacham-Abulafia A, Partouche S, et al. Neutrophil extracellular traps are increased in chronic myeloid leukemia and are differentially affected by tyrosine kinase inhibitors. Cancers (2021) 14:119. doi: 10.3390/cancers14010119
114. Lewis HD, Liddle J, Coote JE, Atkinson SJ, Barker MD, Bax BD, et al. Inhibition of PAD4 activity is sufficient to disrupt mouse and human NET formation. Nat Chem Biol (2015) 11:189–91. doi: 10.1038/nchembio.1735
115. Cedervall J, Dragomir A, Saupe F, Zhang Y, Ärnlöv J, Larsson E, et al. Pharmacological targeting of peptidylarginine deiminase 4 prevents cancer-associated kidney injury in mice. OncoImmunology (2017) 6:e1320009. doi: 10.1080/2162402X.2017.1320009
116. Deng H, Lin C, Garcia-Gerique L, Fu S, Cruz Z, Bonner EE, et al. A novel selective inhibitor JBI-589 targets PAD4-mediated neutrophil migration to suppress tumor progression. Cancer Res (2022) 82:3561–72. doi: 10.1158/0008-5472.CAN-21-4045
117. Chen J, Hou S, Liang Q, He W, Li R, Wang H, et al. Localized degradation of neutrophil extracellular traps by photoregulated enzyme delivery for cancer immunotherapy and metastasis suppression. ACS Nano (2022) 16:2585–97. doi: 10.1021/acsnano.1c09318
118. Zhan X, Wu R, Kong X-H, You Y, He K, Sun X-Y, et al. Elevated neutrophil extracellular traps by HBV-mediated S100A9-TLR4/RAGE-ROS cascade facilitate the growth and metastasis of hepatocellular carcinoma. Cancer Commun 43:225–45. doi: 10.1002/cac2.12388
119. Zhang H, Xu X, Xu R, Ye T. Drug repurposing of ivermectin abrogates neutrophil extracellular traps and prevents melanoma metastasis. Front Oncol (2022) 12:989167. doi: 10.3389/fonc.2022.989167
120. Li JC, Zou XM, Yang SF, Jin JQ, Zhu L, Li CJ, et al. Neutrophil extracellular traps participate in the development of cancer-associated thrombosis in patients with gastric cancer. World J Gastroenterol (2022) 28:3132–49. doi: 10.3748/wjg.v28.i26.3132
121. Nie M, Yang L, Bi X, Wang Y, Sun P, Yang H, et al. Neutrophil extracellular traps induced by IL8 promote diffuse Large b-cell lymphoma progression via the TLR9 signaling. Clin Cancer Res Off J Am Assoc Cancer Res (2019) 25:1867–79. doi: 10.1158/1078-0432.CCR-18-1226
122. Deng J, Kang Y, Cheng C-C, Li X, Dai B, Katz MH, et al. DDR1-induced neutrophil extracellular traps drive pancreatic cancer metastasis. JCI Insight (2021) 6:146133. doi: 10.1172/jci.insight.146133
123. Silva CMS, Wanderley CWS, Veras FP, Sonego F, Nascimento DC, Gonçalves AV, et al. Gasdermin d inhibition prevents multiple organ dysfunction during sepsis by blocking NET formation. Blood (2021) 138:2702–13. doi: 10.1182/blood.2021011525
124. Skov L, Beurskens FJ, Zachariae COC, Reitamo S, Teeling J, Satijn D, et al. IL-8 as antibody therapeutic target in inflammatory diseases: reduction of clinical activity in palmoplantar Pustulosis1. J Immunol (2008) 181:669–79. doi: 10.4049/jimmunol.181.1.669
125. Schott AF, Goldstein LJ, Cristofanilli M, Ruffini PA, McCanna S, Reuben JM, et al. Phase ib pilot study to evaluate reparixin in combination with weekly paclitaxel in patients with HER-2–negative metastatic breast cancer. Clin Cancer Res (2017) 23:5358–65. doi: 10.1158/1078-0432.CCR-16-2748
126. Greene S, Robbins Y, Mydlarz WK, Huynh AP, Schmitt NC, Friedman J, et al. Inhibition of MDSC trafficking with SX-682, a CXCR1/2 inhibitor, enhances NK-cell immunotherapy in head and neck cancer models. Clin Cancer Res (2020) 26:1420–31. doi: 10.1158/1078-0432.CCR-19-2625
127. Ramachandran IR, Condamine T, Lin C, Herlihy SE, Garfall A, Vogl DT, et al. Bone marrow PMN-MDSCs and neutrophils are functionally similar in protection of multiple myeloma from chemotherapy. Cancer Lett (2016) 371:117–24. doi: 10.1016/j.canlet.2015.10.040
128. Teijeira Á, Garasa S, Gato M, Alfaro C, Migueliz I, Cirella A, et al. CXCR1 and CXCR2 chemokine receptor agonists produced by tumors induce neutrophil extracellular traps that interfere with immune cytotoxicity. Immunity (2020) 52:856–871.e8. doi: 10.1016/j.immuni.2020.03.001
129. Zhang H, Wang Y, Onuma A, He J, Wang H, Xia Y, et al. Neutrophils extracellular traps inhibition improves PD-1 blockade immunotherapy in colorectal cancer. Cancers (2021) 13:5333. doi: 10.3390/cancers13215333
130. Shinde-Jadhav S, Mansure JJ, Rayes RF, Marcq G, Ayoub M, Skowronski R, et al. Role of neutrophil extracellular traps in radiation resistance of invasive bladder cancer. Nat Commun (2021) 12:2776. doi: 10.1038/s41467-021-23086-z
Keywords: cancer, therapeutics, tumor microenvironment, neutrophil extracellular traps, metastasis (cancer metastasis)
Citation: Zhong W, Wang Q, Shen X and Du J (2023) The emerging role of neutrophil extracellular traps in cancer: from lab to ward. Front. Oncol. 13:1163802. doi: 10.3389/fonc.2023.1163802
Received: 16 February 2023; Accepted: 13 April 2023;
Published: 28 April 2023.
Edited by:
Robson Q. Monteiro, Federal University of Rio de Janeiro, BrazilReviewed by:
Araci M R Rondon, Leiden University Medical Center (LUMC), NetherlandsSoraya Mezouar, Aix-Marseille University, France
Copyright © 2023 Zhong, Wang, Shen and Du. This is an open-access article distributed under the terms of the Creative Commons Attribution License (CC BY). The use, distribution or reproduction in other forums is permitted, provided the original author(s) and the copyright owner(s) are credited and that the original publication in this journal is cited, in accordance with accepted academic practice. No use, distribution or reproduction is permitted which does not comply with these terms.
*Correspondence: Junfeng Du, ZHVqZjY2QDEyNi5jb20=; Xiaofei Shen, ZGcxNTM1MDU4QHNtYWlsLm5qdS5lZHUuY24=