- 1Faculty of Chinese Medicine, Macau University of Science and Technology, Macao, Macao SAR, China
- 2School of Second Clinical Medicine, Guangzhou University of Chinese Medicine, Guangzhou, China
- 3Guangxi Key Laboratory of Applied Fundamental Research of Zhuang Medicine, Guangxi University of Chinese Medicine, Nanning, China
- 4State Key Laboratory of Quality Research in Chinese Medicines, Macau University of Science and Technology Zhuhai MUST Science and Technology Research Institute, Macau University of Science and Technology, Macao, Macao SAR, China
The incidence of gastric cancer is increasing year by year. Most gastric cancers are already in the advanced stage with poor prognosis when diagnosed, which means the current treatment is not satisfactory. Angiogenesis is an important link in the occurrence and development of tumors, and there are multiple anti-angiogenesis targeted therapies. To comprehensively evaluate the efficacy and safety of anti-angiogenic targeted drugs alone and in combination against gastric cancer, we systematically searched and sorted out relevant literature. In this review, we summarized the efficacy and safety of Ramucirumab, Bevacizumab, Apatinib, Fruquintinib, Sorafenib, Sunitinib, Pazopanib on gastric cancer when used alone or in combination based on prospective clinical trials reported in the literature, and sorted response biomarkers. We also summarized the challenges faced by anti-angiogenesis therapy for gastric cancer and available solutions. Finally, the characteristics of the current clinical research are summarized and suggestions and prospects are raised. This review will serve as a good reference for the clinical research of anti-angiogenic targeted drugs in the treatment of gastric cancer.
1 Introduction
Gastric cancer (GC) is one of the most common causes of cancer death and ranks third in cancer-related death worldwide (1, 2). The survival rate of GC has improved in recent years, but its mortality rate still accounts for 23.4% of malignant tumors (3), and the 5-year survival rate is less than 10% (4). For the lack of effective screening methods, most GC patients are diagnosed at advanced stage, resulting in poor prognosis with mPFS less than 12 months (5).
The treatment of GC mainly includes surgery, radiotherapy, chemotherapy and biological targeted therapy. Endoscopic mucosal dissection is the main treatment for early GC (6), while surgical treatment (e.g. total gastrectomy, distal gastrectomy, proximal gastrectomy) is the first choice for GC (7) and complete resection is the only curative treatment that may cure GC (8). However, despite aggressive surgical intervention, more than 50% of patients with curative resection experienced disease recurrence in the form of metastatic disease (9). The development of metastatic disease is almost fatal. Despite the advances in chemotherapy regimens for GC, the efficacy is still unsatisfactory and the drug resistance of patients is extremely high (10). Therefore, the research focus is to develop more effective and personalized treatment regimens to prolong survival time and improve quality of life of advanced GC patients.
Angiogenesis is the formation of blood vessels form existing ones and it is also the basis of tumor proliferation, invasion, and metastasis in advanced GC (11). Previous studies have shown that serum VEGF levels of advanced GC patients are higher than those of healthy individuals (12). In GC, tumor cells and stromal cells produce various angiogenic factors, such as vascular endothelial growth factor (VEGF), interleukin-8 (IL-8), and platelet-derived endothelial cell growth factor (PD-ECGF) (13). These factors stimulate the proliferation and migration of endothelial cells, which induces the formation of new capillaries in the tumor microenvironment (14). Angiogenesis is co-regulated by pro-angiogenic and anti-angiogenic factors (15). MicroRNAs (miRNAs) are small non-coding RNAs and they bind to mRNAs to regulate the expression of genes involved in angiogenesis (16). It has been verified that some miRNAs play a role in GC angiogenesis by targeting different angiogenic factors or pathways (17). For example, miR-126 can inhibit GC angiogenesis by suppressing VEGF and its receptor VEGFR2 (18). MiR-34a can inhibit GC angiogenesis by targeting PD-ECGF and ANG2 (19). MiR-221 can promote GC angiogenesis by inhibiting the anti-angiogenic factor thrombospondin-1 (TSP-1) (20). The microenvironment in the gastric mucosa may also affect the angiogenic phenotype of GC as chronic inflammation, hypoxia, and acidosis can upregulate the expression of angiogenic factors and receptors (21). Angiogenesis is an important hallmark of malignancy, thus inhibition of this process has become a hallmark of biological anticancer therapies for solid tumors in the contemporary world (22). Angiogenesis inhibitors have entered various stages of clinical trials and are widely used in the clinic, but a summary of the successes and problems encountered in current clinical studies is lacking.
In this review, in order to provide more personalized treatment plans for GC patients and provide a reliable theoretical basis for the treatment of GC with anti-angiogenic drugs, we systematically expounded the mechanism of angiogenesis on GC and summarized the results of registered clinical trials of anti-angiogenic targeted drugs for GC, as well as the response biomarkers of these drugs. Finally, based on the review, we also discussed the problems in the current research and the direction of future clinical research.
2 Angiogenesis mechanism of gastric cancer
The occurrence and development of tumors depend on angiogenesis, and new blood vessels promote tumor invasion and metastasis (23). In the process of angiogenesis, a variety of factors are involved in the regulation. Vascular endothelial growth factor (VEGF) is considered to be the strongest pro-angiogenic growth factor (24), and its receptor (vascular endothelial growth factor receptor, VEGFR) has become a hotspot for research in recent years and great progress has been made (25).
The VEGF family includes vascular endothelial growth factor A (VEGF-A), vascular endothelial growth factor B (VEGF-B), vascular endothelial growth factor C (VEGF-C), vascular endothelial growth factor D (VEGF-D), vascular endothelial growth factor E (VEGF-E) and placental growth factor (PIGF), whose receptors include 3 tyrosine kinase receptors vascular endothelial growth factor receptor 1 (VEGFR-1, also known as Flt-1), vascular endothelial growth factor receptor 2 (VEGFR-2, also known as KDR/Flk-1), vascular endothelial growth factor receptor 3 (VEGFR-3, also known as Flt-4), whose receptors bind to VEGF with high affinity (26).
VEGF receptors are transmembrane tyrosine kinases that activate various angiogenic pathways upon ligand binding and receptor dimerization (27). VEGF-A is the member of the VEGF family that is most closely related to angiogenesis (28). Different isoforms of VEGF-A have different functions depending on their binding affinity and specificity for the receptors (29). For example, both VEGF-A121 and VEGF-A165 bind to VEGFR-1 and VEGFR-2, but interestingly, VEGF-A121 has a higher affinity for VEGFR-2 and is more potent in inducing endothelial cell proliferation and migration than VEGF-A165 (30). On the other hand, VEGF-A189 and VEGF-A206 mainly bind to VEGFR-1 with a lower angiogenic activity than VEGF-A121 and VEGF-A165 (31). Ligand-receptor binding is the basic step for receptor activation and subsequent signal transduction (32). VEGF-A binds to both VEGFR-1 and VEGFR-2, but mainly signals through VEGFR-2 (33). The receptor most closely related to tumor angiogenesis is VEGFR-2 (13). VEGF-B and PlGF only bind to VEGFR-1 and regulate its activity (34). VEGF-C and VEGF-D bind to both VEGFR-2 and VEGFR-3, but mainly signal through VEGFR-3 (35). VEGFR-3 is the specific receptor for lymphatic growth factors VEGF-C and VEGF-D, which regulates the function of both vascular and lymphatic endothelial cells during embryonic development (36).
The function of VEGF in tumor angiogenesis mainly lies in three aspects. First, VEGF is a homodimeric glycoprotein encoded by a single gene, which can directly stimulate the movement, proliferation and division of vascular endothelial cells, increase the permeability of micro-vessels to promote the assimilation of cadherin and reduce the intercellular adhesion (37). VEGF is closely related to nitric oxide (NO) and can reduce endothelial nitric oxide synthetase (NOS) activity (38), reduce vascular tension to increase microvascular permeability, which is conducive to the extravasation of fibrinogen and other plasma proteins, and become the basis of tumor neovascularization network formation and accelerate tumor hematogenous metastasis (39). Second, VEGF changes the activation of endothelial cells, and induces the expression of a series of endothelial cell genes from different sources under hypoxic conditions (40), including the expression of procoagulant factor, plasminogen activator inhibitor-1 (PAI-1), matrix metalloproteinase (MMP), interstitial collagenase and tissue factor, to degrade the extracellular matrix around blood vessels (41) to promote the release of pro-angiogenic factors stored in the extracellular matrix (42) thus inducing vascularization. Third, VEGF is a mitogen of endothelial cells, which activates the MAPK signaling pathway to stimulate the mitosis of endothelial cells and promote the proliferation and deformation (43); VEGF binds to VEGFR-1 (also known as Flt1) and phosphorylates Flt1 (33). Phosphorylated Flt1 cannot significantly promote the proliferation of endothelial cells, but it can activate the actin reorganization induced by P38-MAPK of the mitogen-activated protein kinase (MAPK) family and promote the migration of endothelial cells (44), while PIGF binds to Flt-1 to increase endothelial cell proliferation by activating p38 MAPK (45); VEGF is rapidly phosphorylated upon binding to VEGFR-2, and the phosphorylation activates multiple signal transduction molecules (46), including P38 - MAPK, PI3K, Akt/PKB, PKC, Ras GAP, Raf -1, MEK, ERK. Phosphorylated VEGFR-2 promotes mitosis and proliferation of endothelial cells by activating the MAPK pathway and PKC-MAPK bypass (47). VEGF is phosphorylated after binding to VEGFR-3 and this activates p42/p44 MAPK transduction through the Ras-independent pathway and promotes the proliferation of lymphatic endothelial cells (48). Phosphorylation of VEGFR-3 can also activate PI3K/Akt, thereby transducing survival signals in lymphatic endothelial cells and vascular endothelial cells to prevent cell apoptosis (49).
VEGF is closely related to GC. Studies have confirmed that the expression level of VEGF in cancer tissue and serum of patients with GC can be a reliable indicator of GC occurrence, development, metastasis and prognosis. Some studies (50–52) compared the expression level of VEGF in GC tissue and normal gastric mucosal tissue, and the results indicated that the expression level of VEGF in GC tissue was higher than that in normal gastric mucosal tissue and was related to pathological type, TNM system, and lymph node metastases (53), which suggests that VEGF may be a potential diagnosis and treatment indicator for the occurrence and development of GC. The VEGF in the serum of patients with GC mainly comes from the primary lesion, while VEGF also recruits effector cells such as endothelial cells, hematopoietic stem cells, osteoblasts and osteoclasts in the bone marrow to the site of neovascularization, thereby forming the embryonic form of new blood vessels, increasing VEGF expression in peripheral blood (54). The high expression of VEGF is an important turning point of angiogenesis in GC. The level of serum VEGF correlates with tumor type and infiltration depth (55), and also lymph node metastasis (56), hematogenous metastasis (57, 58) and early recurrence (59). VEGF promotes the maturation and stability of the neovascular bed, and it not only provides key nutrients for tumor growth, maintains and promotes tumor growth, but also facilitates tumor metastasis as a tubular channel (60) (See Figure 1 for more details).
3 Current monoclonal antibodies for angiogenesis treatment in gastric cancer
3.1 Ramucirumab
Ramucirumab is an intravenously administered fully human IgG1 monoclonal antibody derived from phage display technology (61). Ramucirumab inhibits angiogenesis, and it binds to the end of the extracellular domain with high affinity, which induces spatial overlap and conformational changes in the receptor that ultimately prevents ligand binding to VEGFR-2, thereby inhibiting downstream signaling (62). VEGFR-2 is the primary receptor responsible for the spectrum of VEGF -induced biological changes that drive many cancers, including changes in vascular structure and function, proliferation and migration (63). Unlike clinically approved angiogenesis inhibitors, Ramucirumab has specificity and potently inhibits VEGFR-2 (64).
Ramucirumab is the first molecular-targeted drug (65) for clinical single intervention. Its clinical application for GC treatment is shown in Table 1. The earliest study was a phase 3 REGARD trial (73) conducted in 2009, which involved 355 patients with GC or gastroesophageal junction adenocarcinoma who underwent first-line platinum- or fluoropyrimidine-containing chemotherapy. The patients were divided into 2 groups and given ramucirumab and placebo interventions and the results showed that the ramucirumab group had a longer survival period. It is worth noting that the incidence of hypertension in the ramucirumab group was higher than that of the placebo group. An open-label, non-random phase 2 clinical trial in Japan showed (74) that the median overall survival (mOS) of the ramucirumab group was 8.6 months; median progression-free survival (mPFS) was 6.6 months; 12-week progression-free survival rate (12-week PFS rate) was 23.8%, and diarrhea, loss of appetite, high blood pressure, gastric bleeding and protein urine and other adverse events were reported. Taking the REGARD trial as a reference, ramucirumab showed clinical activity and controllable safety in this study.
As Ramucirumab alone showed great efficacy in the second-line treatment of GC or gastroesophageal junction adenocarcinoma, the US Food and Drug Administration (FDA) approved ramucirumab for the treatment of GC or gastroesophageal junction adenocarcinoma in 2014 (61). A US multicenter, double-blind, randomized phase 3 RAINBOW trial was conducted in 170 centers in 27 countries in North America, South America, Asia and Australia (66), and it also recruited patients with GC or gastroesophageal junction adenocarcinoma. Patients in the test group (330/665) received ramucirumab plus paclitaxel, and patients in the control group (335/665) received placebo plus paclitaxel. The results showed that the median overall survival and median progression-free survival of the ramucirumab paclitaxel group were better than those of the placebo paclitaxel group (mOS 9.6 months vs 7.4 months; mPFS 4.4 months vs 2.9 months) (68). Another RAMIRIS trial compared the safety and efficacy of FOLFIRI plus ramucirumab with paclitaxel plus ramucirumab for the same patients, and the results showed that FOLFIRI plus ramucirumab has a relative advantage in terms of the objective response rate (ORR), mPFS, and mOS (69). Ramucirumab has become the world’s first molecularly targeted drug proven to be effective in the second-line treatment of GC or gastroesophageal junction adenocarcinoma combined with chemotherapy, providing a new option for such patients (75).
By comparison, in the RAINBOW trial, both Japanese and Western patients had improved mPFS and ORR after ramucirumab combined with paclitaxel and had similar safety profiles. However, in the evaluation of adverse reactions, the incidence of neutropenia in Japanese patients was higher than that in Western patients (76, 77) Thus, a randomized, multicentre, double-blind phase 3 RAINBOW-Asia trial (78, 79) (a bridging study similar to RAINBOW) was initiated, in which patients were randomized to receive ramucirumab plus paclitaxel (n=294) or placebo plus paclitaxel (n=146), the primary outcome was (mPFS 4.14 months vs 3.15 months, mOS 8.71 months vs 7.92 months), and the most common adverse reaction was also decreased neutrophil count (54% vs 39%). In 2021, Eli Lilly and Company announced that the RAINBOW-Asia trial had reached its pre-specified research priority, and the results, together with the RAINBOW results, supported ramucirumab in combination with paclitaxel as a second-line therapy for GC or gastroesophageal junction adenocarcinoma (80).
Based on the efficacy and safety of ramucirumab, researchers began to explore the efficacy and safety of ramucirumab combined with different chemotherapy drugs for the patients. A phase 3 RAINFALL study of ramucirumab plus a fluoropyrimidine and cisplatin or placebo plus a fluoropyrimidine and cisplatin (67) showed statistical significance in the primary analysis of mPFS, but the results were not confirmed in a sensitivity analysis of progression-free survival by central independent review because the results of mOS were not statistically significant. Therefore, it is not recommended to add ramucirumab to cisplatin plus fluoropyrimidine chemotherapy as first-line therapy. An East Asian multicenter, double-blind, randomized, phase 2 RAINSTORM trial of mPFS (70) suggested that the addition of ramucirumab into fluoropyrimidine and cisplatin did not prolong mPFS or mOS, which means not all chemotherapy regimens combined with ramucirumab can increase the efficacy.
3.2 Bevacizumab
Bevacizumab is the first VEGF monoclonal antibody (81). Bevacizumab is a recombinant humanized monoclonal IgG1 antibody that can bind to VEGF. It can bind to VEGF-A to inhibit the activity of VEGF and block its interaction with VEGFR-1 and VEGFR-2 (82), including endothelial cell enhanced vascular permeability activity, mitogenic activity and other pro-angiogenic activities, to inhibit tumor angiogenesis (81). Bevacizumab regresses tumor vessels and normalizes remaining tumor vessels while inhibiting neovascularization or recurrent angiogenesis (83). As one of the earliest therapies targeting the tumor microenvironment (84), bevacizumab has been used as a targeted therapy drug for various cancers (85). Several studies have demonstrated that bevacizumab exhibits modest antitumor activity in a wide range of malignancies when used in combination with chemotherapy (86).
In recent years, progress has been made in the treatment of metastatic colorectal cancer, NSCL, metastatic breast cancer, ovarian cancer, cervical cancer and other cancers with bevacizumab (87). Bevacizumab was originally approved for the treatment of metastatic colorectal cancer in the United States (US) and the European Union (EU) in 2004 and 2005, respectively (88). Many clinical studies proved the efficacy of bevacizumab (See Table 1 for more details).
GC treatment with bevacizumab has been researched recently (89). Some studies suggested that bevacizumab was not recommended as a perioperative treatment for patients with resectable GC (90). However, there is no unified conclusion on the predictive indicators of the efficacy of bevacizumab at present, and it is still impossible to confirm which group of people is suitable for bevacizumab treatment. A multicenter trial with small samples evaluating bevacizumab combined with chemotherapy in the treatment of GC and gastroesophageal junction adenocarcinoma was conducted in the United States. The combined regimen (72) had an ORR of 65%, a median time to disease progression (mTTP) of 8.3 months, and an mOS of 12.3 months. Compared with historical controls, mTTP improved by 75%. The toxicity profile included hypertension, gastrointestinal perforation, gastrointestinal bleeding, and thromboembolic events, and was not different from other bevacizumab-containing regimens. It is suggested that we optimize the use of bevacizumab in the treatment of GC (91).
The following AVAGAST was an international clinical study involving patients from Europe, the United States, Korea, and Japan. Chemotherapy in combination with or without bevacizumab was taken as first-line treatment for patients with GC (71). The included patients received subsequent chemotherapy after disease progression, and the results showed that the ORR, mOS and mPFS of the combination group were longer than those of the chemotherapy group. In baseline comparison, poor performance status, liver metastases, and larger tumors were most common in Eastern Europe/South America and the least common in Japan. Although the study did not meet the expected goals, the addition of bevacizumab to chemotherapy increased the mPFS and ORR of the first-line treatment of GC, and also showed geographical differences, with the greatest survival benefit in American patients and almost no survival benefit in Asian patients.
Considering the influence of geographical factors in AVAGAST, Chinese researchers designed an AVATAR (92)trial (randomized, double-blind, phase III) similar to AVAGAST for Chinese patients with GC. The patients in the experimental group received bevacizumab combined with capecitabine-cisplatin. There was no difference in mOS between the experimental and placebo groups, and mPFS was also similar. Safety findings were identical to those of a previous US trial of bevacizumab (AVAGAST); bevacizumab plus capecitabine-cisplatin was well tolerated, with 60% of bevacizumab-treated patients and68% of placebo-treated patients reported grade 3-5 adverse events (AEs). It can be seen that the AVATAR trial did not bring survival benefits to Chinese patients with GC.
4 Tyrosine kinase inhibitors currently used to treat gastric cancer angiogenesis
4.1 Apatinib
Apatinib is a small-molecule VEGFR-2 tyrosine kinase inhibitor independently developed in China. It is the first small-molecule targeted drug proven to improve the survival of GC (93)(See Table 2 for more details). Apatinib was approved by the China Food and Drug Administration (CF-DA) in 2014 for the treatment of third-line and above-advanced GC (124), making it a new option for patients with GC who failed in second-line treatment (125). Meanwhile, studies have shown that the overall response rate of apatinib for advanced GC is 42% (126).
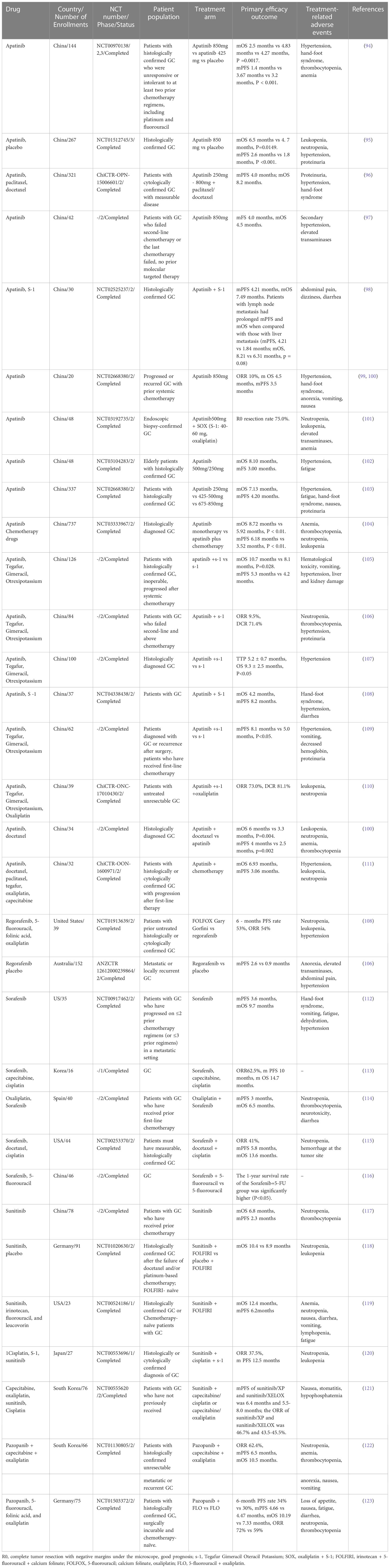
Table 2 Clinical trials of anti-angiogenic targeted tyrosine kinase inhibitors in the treatment of gastric cancer.
Apatinib is mainly used for advanced GC patients who have failed chemotherapy (124). The earliest study of apatinib was a phase II trial for patients with metastatic GC. A total of 144 patients with GC failed in second-line or more chemotherapy was enrolled, of which the apatinib components were 850mg/qd and 425mg/bid. The results showed that both mPFS and mOS were improved, and there were significant statistical differences between apatinib and placebo (94). A randomized, double-blind phase III clinical study of apatinib (95)has shown that for patients with GC for whom two or more prior chemotherapy regimens failed, mOS and mPFS were significantly improved in apatinib group compared with placebo. These suggested that apatinib treatment significantly improved the OS and PFS of patients with GC resistant to two or more prior chemotherapy regimens and increased the survival time of patients. These two trials had consistent conclusions regarding adverse reactions. The most common grade 3 to 4 non-hematological adverse events were hand-foot syndrome, proteinuria, and hypertension. Most patients could tolerate and safety was acceptable. Studies by Shen (96)and Ruan (97) also showed that apatinib had good efficacy and safety in patients with GC irresponsive to two or more prior chemotherapy regimens.
Subsequent studies compared the efficacy of different doses of apatinib on the survival of patients with advanced GC, and the results showed that compared with higher daily doses (675-850mg) of apatinib, lower daily doses (250-500 mg) of apatinib can achieve comparable outcomes in mOS and mPFS while maintaining more benign safety profile (99, 103, 104) in patients with GC. However, the efficacy and safety of apatinib in elderly patients with GC remain unclear, so an open-label, single-arm, phase II study was conducted involving GC patients aged ≥60 years (48 patients). Results showed that apatinib was effective and relatively well-tolerated in elderly patients with unresectable GC who had received at least one line of chemotherapy (102), and a lower initial daily dose (250mg–500mg) may be an appropriate choice for elderly patients in clinical practice (127).
Chemotherapy alone has limited benefit in patients with GC who have failed first-line therapy. Therefore, exploring which chemotherapy regimens can effectively prolong their survival and improve the quality of life by combining apatinib has become a current research focus for advanced GC patients who have failed chemotherapy. In the clinical trials of treatment of GC with apatinib combined with docetaxel, the mPFS and mOS of the apatinib group and the combination group were 2.5 and 4 months, 3.3 and 6 months, respectively, and grade 3/4 adverse reactions such as neutrophils, cytopenia, anemia, thrombocytopenia, and hypertension were milder in the combination group than in the apatinib group (128). Patients with advanced GC benefited more from apatinib plus docetaxel compared with apatinib monotherapy (129). Apatinib plus docetaxel was proved clinically beneficial in previous studies, but the feasibility of combining apatinib with other chemotherapeutic agents was unclear.
Subsequent studies have found that apatinib combined with chemotherapy has also achieved a good curative effect in the second-line treatment of AGC. Apatinib combined with chemotherapy as the second-line treatment of advanced GC has good clinical efficacy and acceptable side effects, and may provide a new second-line treatment option for patients with advanced GC (130). Apatinib and s-1 (tegafur + gimeracil + oteracil potassium) have been approved by the National Medical Products Administration (NMPA) of China for the treatment of GC, and patients can afford these treatments (131). Meanwhile, manageable adverse events reduced the side-effect costs of symptomatic and supportive care (129). Apatinib combined with s-1 therapy was superior to s-1 alone in the second-line treatment of GC. The combination can significantly improve the quality of life of patients, reduce the level of serum tumor markers, prolong the patient’s mOS (105), and mPFS, and improve ORR and disease control rate (DCR) (106). In another study, apatinib also improved the levels of T helper 1 (Th1) and T helper 2 (Th2)-like cytokines (107). Apatinib in combination with S-1 has shown promising efficacy and manageable toxicity as a second-line treatment for patients with GC, especially for elderly patients with poor performance status (108). Combination therapy with apatinib, especially with paclitaxel, may confer a better survival benefit in the first-line treatment (132). However, some studies have also suggested that while increasing the curative effect, combined drug use reduced the quality of life of patients and increased the risk of adverse reactions (109).
Apatinib has also demonstrated certain therapeutic effects targeting metastatic gastric cancer in clinical trials. Apatinib combined with S-1 as a first-line treatment for GC was not superior to other chemotherapy regimens. Toxicities were consistent with known profiles when given as monotherapy (131). Notably, this study compared metastatic sites in GC. Compared with patients with liver metastases, patients with lymph node metastases gained better curative effects as they tended to have prolonged mPFS and mOS. This may support the design of future clinical trials to better define patient populations (98). The study also reported that the most common grade 3 to 4 AEs for apatinib monotherapy were hypertension, hand-foot syndrome, anorexia, vomiting, and nausea. Apatinib combined with SOX (S-1+oxaliplatin) as a neoadjuvant therapy for advanced or metastatic GC also has demonstrated significant efficacy and safety and the common adverse reactions include leukopenia, neutropenia and hypertension. Further randomized clinical trials at a larger scale are needed to confirm these findings (101, 110). Apatinib showed promising efficacy and acceptable safety in GC patients with advanced liver metastases. Anti-angiogenic therapy may be a good strategy for the treatment of GC with liver metastases, a rare subtype of GC (100).
4.2 Fruquintinib
Fruquintinib is an orally available, highly selective small-molecule antagonist of VEGFR1, VEGFR2, and VEGFR3 (111). In September 2018, fruquintinib received its first global approval in China for the treatment of metastatic colorectal cancer in patients who have failed at least two prior systemic antineoplastic treatments (133). Currently, there are ongoing phase 2 and phase 3 studies (NCT02415023, NCT03223376) of fruquintinib combined with paclitaxel in the treatment of GC, and phase 2 trials of fruquintinib combined with SOX (NCT05122091) as neoadjuvant therapy for GC are also underway (111). The phase III clinical development of fruquintinib monotherapy is mainly for patients with advanced non-small cell lung cancer (NSCL) and GC (134)(Table 2 for more details).
4.3 Sorafenib
Sorafenib has been shown to have inhibitory effects against platelet-derived growth factor receptor (PDGFR), VEGFR2, VEGFR-3, PDGFR-β and other receptors (135). It has dual anti-tumor effects (136). On the one hand, it can block the formation of tumor angiogenesis by inhibiting VEGFR and PDGFR to indirectly inhibit the growth of tumor cells (137). On the other hand, it can directly inhibit tumor growth by blocking the RAF/MEK/ERK signaling pathway (138).
The results of the phase II clinical study (NCT00917462) showed (112) that single-agent sorafenib can improve OS and PFS in patients with advanced gastroesophageal junction adenocarcinoma. Treatment-related adverse reactions include hand-foot syndrome, rash, dehydration and fatigue, and mutations of P53 and other related gene identified by tumor exome sequencing. This may bring new opportunities for sorafenib in the treatment of gastroesophageal junction adenocarcinoma (139). Sorafenib can also be used in combination with various chemotherapy drugs, including paclitaxel, cisplatin, and 5- fluorouracil. Subsequent studies have showed that the triple combination of sorafenib, docetaxel, and cisplatin had clinical activity. There are few works of literature on the maximum tolerated dose of sorafenib combined with chemotherapy drugs. A phase I trial once mentioned sorafenib (400mg/bid), capecitabine (800mg/m2/bid) and cisplatin (60mg/m2) were recommended as a first-line treatment in GC (113). In addition, a phase II trial demonstrated the efficacy of sorafenib in combination with docetaxel and cisplatin for the treatment of advanced GC, with an mOS of 13.6 months (114) and the most common grade 3/4 adverse reactions being neutropenia. In a phase I study of sorafenib in combination with S-1 and cisplatin for the treatment of advanced GC, pharmacokinetic analysis showed no significant difference in the sorafenib exposure between the sorafenib group and combination group, with adverse reactions including anorexia, rash, neutropenia, thrombocytopenia, and nausea (115). Sorafenib in combination with 5-FU can effectively decrease serum VEGF and HIF-1α levels and improve 1-year survival rate (116). In a trial of sorafenib in combination with oxaliplatin as a second-line treatment for advanced GC, the mPFS was 3 months and the mOS was 6.5 months. However, subgroup analyses of this trial showed that the progression-free time of first-line treatment determined the different prognosis of patients, and the grade 3/4 adverse reactions were neutropenia and thrombocytopenia (140).
From the above, it can be seen that sorafenib alone or in combination with different chemotherapy drugs can be used for the treatment of advanced GC, but results of high-quality trials are needed to support the viewpoint. Therefore, more in-depth research on the use of sorafenib in the treatment of advanced gastric cancer should be carried out. Meanwhile, dose change of sorafenib was in correlation with the occurrence of adverse events (141), so the combination of sorafenib with chemotherapy drugs should be further explored in large-scale cohort studies. (Table 2 for more details).
4.4 Sunitinib
Sunitinib is also a multi-target tyrosine kinase inhibitor against VEGF and PDGFR-β (142). Sunitinib monotherapy (117)was tolerated in GC, but tumor responses were limited. Although sunitinib monotherapy only has insufficient clinical value as a second-line treatment for GC, its role in combination with chemotherapy deserves further study (143). Later studies found that sunitinib combined with FOLFIRI tended to improve the overall survival of GC (118, 119), but the primary endpoint was not reached. Therefore, the clinical efficacy of sunitinib in patients with GC who failed first-line treatment is not satisfactory. Phase I dose trial suggested that sunitinib plus cisplatin 80 mg/m2 and 5-FU 4,000 mg/m2 were combinable with controllable adverse events (144), and the maximum tolerated dose of sunitinib (MTD) was determined to be 25 mg/day. A Japanese clinical study (120) showed that in a phase I trial of sunitinib combined with s-1 and cisplatin in patients with GC, the MTD of sunitinib combined with cisplatin/S-1 was 25 mg/day. The regimen showed a manageable safety profile and preliminary antitumor activity. Among Korean patients, sunitinib combined with XELOX (oxaliplatin + capecitabine) in patients with advanced GC (121) had an mPFS of 5.5-8.0 months and an ORR of 43.5-45.5%. This suggests that sunitinib has shown good safety in Asian countries such as Japan and South Korea, with relatively consistent tolerated doses (Table 2 for more details).
4.5 Pazopanib
Pazopanib is an orally available and selective tyrosine kinase inhibitor against targets such as VEGFR-1/-2/-3 and PDGFR to inhibit angiogenesis, which has been approved for advanced kidney cancer and soft tissue sarcoma treatment (145, 146). Kim et al. designed a single-arm, open-label phase II study (122) to determine the efficacy and toxicity of pazopanib plus XELOX in GC treatment. The published results of the study indicated that the combination showed moderate activity and an acceptable toxicity profile in patients with GC. The main adverse reactions of grade 3 or above were neutropenia, anemia, thrombocytopenia, and loss of appetite. Subsequent case reports suggested that pazopanib alone can produce sustained efficacy in recurrent and metastatic gastroesophageal adenocarcinoma (147). An open-label randomized phase II trial (123) (2:1) investigated the efficacy of pazopanib plus FLO (5-fluorouracil + oxaliplatin) versus FLO monotherapy as first-line therapy in patients with GC. The results indicated that adding pazopanib to chemotherapy showed signs of efficacy, but no significant improvement. The combination was well tolerated but had high toxicity, and the main adverse events included loss of appetite, nausea, and fatigue (Table 2 for more details).
5 Response biomarkers of anti-angiogenic drug therapy
Anti-angiogenic drugs mainly act on vascular epidermal growth factor, and have shown curative effects in most clinical trials to prolong the survival time of some patients with GC (148). Many clinical studies showed the potential efficacy benefits of anti-angiogenic drugs and their combination therapy, but there are still challenges (149). Determining which patients can get the most benefit from this treatment is the top challenge and it requires specific biomarkers for screening. The following summary expands on response biomarkers (Details in Table 3).
At present, anti-angiogenic drugs have obtained positive results in the treatment of GC, but the discovered response biomarkers have not been verified as predictive or prognostic. Biomarkers in tumor tissue or the circulation of cancer patients may serve as response biomarkers (155). As mentioned earlier, in GC, ramucirumab alone or its combination with paclitaxel as second-line therapy has survival benefits. Although VEGF-D is a potential biomarker for ramucirumab in colorectal and hepatocellular carcinoma, earlier studies did not identify it as a useful biomarker for patients with GC (156). Later studies (150) evaluated the possibility of VEGF-A, VEGF-D and soluble vascular endothelial cell growth factor receptor-2 (sVEGFR-2) serving as the response biomarkers of resistance or efficacy in ramucirumab and paclitaxel combination. The results showed an association between higher baseline levels of VEGF-A and shorter OS, and there was an association between elevated sVEGFR-2 after one week and prolonged PFS and OS. This was also the first report supporting sVEGFR-2 as a positive marker after treating metastatic GC with the combination of paclitaxel and ramucirumab. Ramucirumab binds to VEGFR2 on vascular endothelial cells to inhibit VEGF ligand binding and receptor signaling and limit VEGF-induced angiogenesis and endothelial cell migration, thus slowing tumor growth (157). VEGFR-2 signaling was an important therapeutic target in GC (35). GC with VEGFR-2 overexpression have a poor prognosis, indicating VEGFR-2 may be a negative prognostic marker (158). However, the REGARD trial analysis found that the prognostic trend between high VEGFR-2 endothelial expression and shortened progression-free survival was not significant. Further studies are needed to investigate the predictive potential of high VEGFR2 expression in patients with GC treated with ramucirumab (159). In addition to predictable biomarkers in the VEGF family, patients in a ramucirumab plus FOLFIRI arm who underwent genomic analysis were all microsatellite stable and programmed death ligand 1 (PD -L1) may be a potential positive prognostic marker (69).
The addition of bevacizumab to chemotherapy improved progression-free survival and tumor response rates of patients with GC, but overall survival was not affected. To test the hypothesis that angiogenic markers might have predictive value for the efficacy of bevacizumab in GC, AVAGAST included a prospective, mandatory biomarker program (151). Plasma was available from 712 patients (92%) and tumor samples were available from 727 patients (94%). Baseline plasma VEGF-A levels and neuropilin-1 expression were identified as potential predictors of bevacizumab efficacy (160).
Currently, the analysis of biomarkers of apatinib in the treatment of GC after chemotherapy is mainly based on serum VEGFR-2 (95), serum carbohydrate antigen 19-9, carcinoembryonic antigen (CEA), tumor supplied group factor (TSGF), tumor necrosis factor- α (TNF-α) and inflammatory factors (105, 107). It has also been suggested (98) that TP53 was the most commonly mutated gene, with CDH1 and APC genes being the second most common. Early anti-angiogenesis-related adverse events, such as hypertension, proteinuria, and hand-foot syndrome (120.126), were listed as feasible biomarkers of efficacy.
Preliminary biomarker analysis of the INTEGRATE trial showed a similar benefit of regorafenib in patients with VEGF-A levels above and below the median (106). The ERBB2 gene was also predicted to be a viable efficacy biomarker, as this gene amplification benefited from regorafenib plus FOLFOX treatment.
Sorafenib is used for the treatment of gastrointestinal stromal tumors and metastatic renal cell carcinoma in patients who do not respond to or cannot tolerate standard therapies (161). Sorafenib can selectively target certain proteins to regulate tumor cell growth and metabolism (162). Tumor cells can proliferate under hypoxic conditions and this is closely related to the activation of hypoxia-inducible factor-1α (HIF-1α) and VEGF. HIF-1α can enhance cell metabolism under hypoxic conditions and contribute to the activation of VEGF to induce tumor angiogenesis. HIF-1α expression may be a predictor of poor prognosis in GC, especially in Asia (152). Chemotherapy combined with sorafenib can effectively reduce serum HIF-1α and VEGF levels in patients with GC to improve their 1-year survival rate and prognosis (116). Diffuse expression of HIF-1α in gastric tumors may lead to resistance to adjuvant chemotherapy with 5-FU (163). Local amplification of associated genes ARID1A, PIK3CA, and P53, as well as HMGA2 and MET, also benefited from sorafenib treatment (112).
Tumor VEGF-C expression (compared with no expression) was associated with significantly shorter median PFS and above-median OS in a subgroup of sunitinib monotherapy trials of patients with GC, but tumor control rates did not differ (153). Serum VEGF-A, VEGFR2 and VEGF-D have also been shown to be sensitive to this therapy (118).
FGFR2 gene expression by immunohistochemistry may be a useful biomarker for predicting patients with metastatic or recurrent advanced GC to receive pazopanib combined with CapeOx (154).
6 Challenges and solutions of anti-angiogenic targeted therapy for GC
Anti-angiogenic drugs target various aspects of tumor angiogenesis to block the formation of blood vessels to cut off the nutrient and supply to tumor cells, resulting in a hypoxic microenvironment (164). This therapy has shown limited efficacy, with survival benefits ranging from weeks to several months (165), which may be related to the fact that tumors can activate alternative pathways of angiogenesis, increase invasiveness and metastasis, or develop resistance to anti-angiogenic therapy by immune system inhibition (166). Tumor vessels display tortuosity, disorganization, leakiness, slow blood flow, and hypoxia (167), thus early use of anti-angiogenic drugs can improve the “chaotic” state and normalize gastric tumor vessels (168). However, the early balance of pro-angiogenic and anti-angiogenic factors is temporary (169). Although there has been some progress in anti-angiogenic therapy for GC, the survival benefits of this treatment still face many challenges.
(1) Anti-angiogenic therapy can lead to hypoxia-induced apoptosis of tumor cells while cloning hypoxia-resistant tumor cells, which can lead to drug resistance (170). HIF-1α induces epithelial-mesenchymal transition (EMT), which further enhances the ability of tumor cells to tolerate hypoxia, locally invade, infiltrate blood vessels, and survive in peripheral blood vessels (171). Experimental studies on gastric cancer cell lines CUM-2MD3 and OCUM-12 have found that under hypoxic conditions, GC cells form EMT through autocrine stimulation of TGFβ factors (172). The EMT cell transcriptome is characterized by the expression of proteins with multiple functions, such as growth factors and corresponding protein factor receptors (TGFβ, HGF, HGFR), accessory transcription factors (Wnt, Notch, NFkB), integrin receptors, proteoglycan joint receptors CD44, and glucose-6-phosphate isomerase (GPI) (173). Among these factors, Notch and Wnt are closely related to the HIF-1α signaling pathway (174).
(2) The hypoxic state induces and recruits bone marrow cells to assist in tumor-induced neovascularization (175). Under hypoxic conditions, HIF-1α and its targets, Stromal cell-derived factor-1 (SDF-1) and VEGF will increase to attract a heterogeneous group of bone marrow-derived cells composed of vascular progenitor cells and pro-angiogenic monocytes (176). Endothelial and pericyte progenitor cells are bound as part of the new blood vessels to directly build new blood vessels (177). Pro-angiogenic monocytes provide energy to the tumor by producing pro-angiogenic cytokines, growth factors, and proteases (178). All of these contribute to the formation of new blood vessels.
(3) When tumors become hypoxic, compensatory pathways can be activated to circumvent anti-angiogenic therapy by switching to different pro-angiogenic factors that lead to neovascularization and upregulation of tumor invasiveness, ultimately resulting in tumor recurrence (179). Studies have shown (180) that continuous use of anti-angiogenic drugs can enhance hypoxia and induce upregulation of other factors associated with angiogenesis, such as PIGF, fibroblast growth factors (FGFs), and inflammatory chemokines. This can also recruit Tie2-expressing monocytes (TEMs) and tumor-associated macrophages (TAMs) to promote angiogenesis and disrupt the temporary balance achieved by anti-angiogenic therapy, leading to chaotic and disordered growth of tumor vasculature (181).
(4) TKI can increase vascular permeability and lead to the hematogenous metastasis of cancer cells when it disrupts tumor vascular stability (182). The migration of tumor cells towards blood vessels is influenced by tumor-associated macrophages (TAMs), which can stimulate tumor angiogenesis, making tumor vessels more chaotic. Meanwhile, TAMs secrete EGF and stimulate the EGFR to strengthen the invasive ability of tumors (183). The increase of the expression of matrix metalloproteinases (MMPs), urokinase-type plasminogen activator receptor (uPAR), and tissue proteases will improve the vascular permeability, making tumor cells more prone to intravasation (184).
(5) Vasculogenic mimicry (VM) is the formation of vessel-like channels supplying blood to tumor tissue, which is achieved through the interaction between tumor cells and the extracellular matrix (185). It is also the result of high expression of HIF-1α in tumor cells.
(6) VEGF and its signaling pathway inhibit the maturation of dendritic cells and induce the development of regulatory T cells in the tumor microenvironment. VEGF also promotes the expression of PD-1 in tumor cells, which leads to T cell exhaustion and ultimately destroys the anti-tumor immune response, resulting in immune cell inhibition (186).
There are available solutions to the above issues. Firstly, we have to find the treatment regimens of combination therapy with anti-angiogenic drugs. The combination of anti-angiogenic drugs and chemotherapy drug docetaxel can effectively inhibit the synthesis of mitochondrial DNA in hypoxic tumor stem cells, thereby improving the efficacy of anti-angiogenic drugs (187). There are also studies on the combination of anti-angiogenic drugs with other targeted drugs, such as the combination of bevacizumab and trastuzumab to improve mPFS and mOS in advanced GC patients (188). The combination of anti-angiogenic therapy and immunotherapy has also achieved certain effectiveness, such as the combination of bevacizumab and PD-1 inhibitor (189). Nanoparticles have also shown the ability to target endothelial cells, effectively delivering anti-angiogenic drugs to the tumor site and enhancing the therapeutic effect by reducing systemic toxicity (190).This therapy is still at an early stage of development and shows great potential in inhibiting tumor angiogenesis (191).
7 Discussion
Anti-angiogenic targeted therapy aims to block the formation of new blood vessels that nourish tumors. Anti-angiogenic therapy may have some advantages in GC treatment because patients with this type of cancer have high levels of VEGF, a key factor that stimulates angiogenesis (192).
This review summarizes current clinical trials and response biomarkers of anti-angiogenic targeted therapy for GC with complete data published in open-access journals. We first summarize the efficacy of the reported clinical trials of anti-angiogenic targeted therapy drugs in patients with GC. The clinical trials of ramucirumab were large-scale, involving multiple continents and countries, and it has been proved that single drug or ramucirumab paclitaxel combination in the treatment of GC can prolong the PFS and OS of patients, thus becoming the standard second-line therapy for GC. Bevacizumab was first approved for the treatment of metastatic colorectal cancer. Compared with ramucirumab (158), its single-drug effect was not obvious and clinical trials were not sufficient. Later studies found that bevacizumab combined with conventional chemotherapy can improve the curative effect and prolong OS in patients with GC. Apatinib tyrosine kinase inhibitors entered clinical trials to test GC at the beginning, and it showed good efficacy in patients with GC after they failed chemotherapy. Subsequent studies confirmed that low-dose apatinib was more effective and safer for patients with GC who have received prior extensive treatment. Apatinib is orally available and that is conducive to clinical promotion (159). Sorafenib has proven clinical activity in clinical trials, but sunitinib, pazopanib and fruquintinib have been less consistent. Most of the response biomarkers of anti-angiogenic targeted therapy have not been verified.
We also found the characteristics of clinical trials of anti-angiogenic targeted therapy. There were only a few single drug interventions in the clinical trials summarized in this article as most of the trials were drugs combined with chemotherapy. Besides, most studies were single-arm trials, and the research endpoints were mainly ORR, mPFS, mOS, and side effects. However, there were also problems. First, there were many types of anti-angiogenic targeted drugs, while clinical trials of single drugs were limited, and trials with positive results were even fewer. Secondly, there were many combined treatment options for anti-angiogenic targeted drugs and some were still in the initial stage. Third, the efficacy of these drugs was different in different patient groups, but the subgroup analysis for this issue was not comprehensive. Fourth, only a few drugs in the review have undergone clinical trials of the optimal dose selection. Fifth, the discovery of response biomarkers in this paper was not deep enough, and the main prediction was limited to response markers. Many biomarkers have been reported but they have not been widely used in clinical practice, thus the overall predictive efficiency and level of evidence were low. It can be seen that anti-angiogenic therapy cannot cure gastric cancer nor is it effective for all patients. It may only benefit a subset of patients with certain molecular features or biomarkers that predict response to the therapy (193). Over time, however, anti-angiogenic therapy may lead to resistance or relapse as tumors adapt to the lack of blood supply (194).
Future clinical research on anti-angiogenic targeted drugs can focus on the following directions: First, when selecting anti-angiogenic targeted drugs, researchers should pay attention to the stage and type of GC of the patient, and continue to explore the best time for applying the drugs for specific GC. Secondly, pay attention to the design of the intervention arm and the establishment of research endpoints in designing clinical trials. Thirdly, pay attention to the detailed subgroup analysis (race, age, different centers, etc.). Fourthly, pay attention to the selection of the optimal dose, index setting and dose tolerance for curative effect evaluation. Fifth, explore the efficacy of anti-angiogenic targeted drugs combined with different chemotherapeutic drugs or different types of targeted drugs for better curative effects and less toxic and side effects. Sixth, the verification of biomarkers still needs a large number of prospective studies (efficacy, toxicity, and drug resistance).
Author contributions
DX, YL designed the study together, equal contribution, Listed as co-first author. GF, PY as co-corresponding author, PW, JL, LM, JH, HZ, XY, LL and YZ, were all involved in the revision of the manuscript. GF and PY made final critical revisions. All authors contributed to the article and approved the submitted version.
Funding
Macau SAR (SKL-QRCM (MUST)-2020-2022) (Grant approval No. 0011/2021/A); Guangxi Health Commission Key Laboratory of Applied Fundamental Research of Zhuang Medicine (Guangxi University of Chinese Medicine) (Gui Wei Ke Jiao Fa (2020) No.17); Training Program under “139” Plan for Developing High-level Medical Talents in Guangxi (Gui Wei Ke Jiao Fa (2020) No.15), the development program of high-level talent team under Qihuang project of Guangxi university of Chinese medicine (Grant No.2021003).
Acknowledgments
We thank PY and GF for their helpful suggestions.
Conflict of interest
The authors declare that the research was conducted in the absence of any commercial or financial relationships that could be construed as a potential conflict of interest.
Publisher’s note
All claims expressed in this article are solely those of the authors and do not necessarily represent those of their affiliated organizations, or those of the publisher, the editors and the reviewers. Any product that may be evaluated in this article, or claim that may be made by its manufacturer, is not guaranteed or endorsed by the publisher.
References
1. Joshi SS, Badgwell BD. Current treatment and recent progress in gastric cancer. CA Cancer J Clin (2021) 71(3):264–79. doi: 10.3322/caac.21657
2. Thrift AP, El-Serag HB. Burden of gastric cancer. Clin Gastroenterol Hepatol (2020) 18(3):534–42. doi: 10.1016/j.cgh.2019.07.045
3. Park JY, Herrero R. Recent progress in gastric cancer prevention. Best Pract Res Clin Gastroenterol (2021) 50-51:101733. doi: 10.1016/j.bpg.2021.101733
4. Sexton RE, Al Hallak MN, Diab M, Azmi AS. Gastric cancer: a comprehensive review of current and future treatment strategies. Cancer Metastasis Rev (2020) 39(4):1179–203. doi: 10.1007/s10555-020-09925-3
5. Liu D, Wang N, Sun Y, Guo T, Zhu X, Guo J. Expression of VEGF with tumor incidence, metastasis and prognosis in human gastric carcinoma. Cancer biomark (2018) 22(4):693–700. doi: 10.3233/CBM-171163
6. Machlowska J, Baj J, Sitarz M, Maciejewski R, Sitarz R. Gastric cancer: epidemiology, risk factors, classification, genomic characteristics and treatment strategies. Int J Mol Sci (2020) 21(11):4012. doi: 10.3390/ijms21114012
7. Tan Z. Recent advances in the surgical treatment of advanced gastric cancer: a review. Med Sci Monit (2019) 25:3537–41. doi: 10.12659/MSM.916475
8. Petryszyn P, Chapelle N, Matysiak-Budnik T. Gastric cancer: where are we heading? Dig Dis (2020) 38(4):280–5. doi: 10.1159/000506509
9. Gullo I, Grillo F, Mastracci L, Vanoli A, Carneiro F, Saragoni L, et al. Precancerous lesions of the stomach, gastric cancer and hereditary gastric cancer syndromes. Pathologica (2020) 112(3):166–85. doi: 10.32074/1591-951X-166
10. Wagner AD, Syn NL, Moehler M, Grothe W, Yong WP, Tai BC, et al. Chemotherapy for advanced gastric cancer. Cochrane Database Syst Rev (2017) 8(8):CD004064. doi: 10.1002/14651858.CD004064.pub4
11. Zhao WX, Liu ZF, Li XL, Li Z. Correlations of serum homocysteine, VEGF and gastrin 17 with gastric cancer and precancerous lesions. Eur Rev Med Pharmacol Sci (2019) 23(10):4192–8. doi: 10.26355/eurrev_201905_17922
12. Partyka R, Gonciarz M, Jałowiecki P, Kokocińska D, Byrczek T. VEGF and metalloproteinase 2 (MMP 2) expression in gastric cancer tissue. Med Sci Monit (2012) 18(4):BR130–4. doi: 10.12659/msm.882614
13. Apte RS, Chen DS, Ferrara N. VEGF in signaling and disease: beyond discovery and development. Cell (2019) 176(6):1248–64. doi: 10.1016/j.cell.2019.01.021
14. Liu Y, Li C, Lu Y, Liu C, Yang W. Tumor microenvironment-mediated immune tolerance in development and treatment of gastric cancer. Front Immunol (2022) O13:1016817. doi: 10.3389/fimmu.2022.1016817
15. Carmeliet P. VEGF as a key mediator of angiogenesis in cancer. Oncology (2005) 3:4–10. doi: 10.1159/000088478
16. Hicklin DJ, Ellis LM. Role of the vascular endothelial growth factor pathway in tumor growth and angiogenesis. J Clin Oncol (2005) 23(5):1011–27. doi: 10.1200/JCO.2005.06.081
17. Alessandrini L, Manchi M, De Re V, Dolcetti R, Canzonieri V. Proposed molecular and miRNA classification of gastric cancer. Int J Mol Sci (2018) 19(6):1683. doi: 10.3390/ijms19061683
18. Ouyang J, Song F, Li H, Yang R, Huang H. miR-126 targeting GOLPH3 inhibits the epithelial-mesenchymal transition of gastric cancer BGC-823 cells and reduces cell invasion. Eur J Histochem (2020) 64(4):3168. doi: 10.4081/ejh.2020.3168
19. Li Z, Liu ZM, Xu BH. A meta-analysis of the effect of microRNA-34a on the progression and prognosis of gastric cancer. Eur Rev Med Pharmacol Sci (2018) 22(23):8281–7. doi: 10.26355/eurrev_201812_16525
20. Hu K, Olsen BR. Osteoblast-derived VEGF regulates osteoblast differentiation and bone formation during bone repair. J Clin Invest (2016) 126(2):509–26. doi: 10.1172/JCI82585
21. Oya Y, Hayakawa Y, Koike K. Tumor microenvironment in gastric cancers. Cancer Sci (2020) 111(8):2696–707. doi: 10.1111/cas.14521
22. Kakeji Y, Maehara Y, Sumiyoshi Y, Oda S, Emi Y. Angiogenesis as a target for gastric cancer. Surgery (2002) 131(1 Suppl):S48–54. doi: 10.1067/msy.2002.119304
23. Itatani Y, Kawada K, Yamamoto T, Sakai Y. Resistance to anti-angiogenic therapy in cancer-alterations to anti-VEGF pathway. Int J Mol Sci (2018) 19(4):1232. doi: 10.3390/ijms19041232
24. Nussenbaum F, Herman IM. Tumor angiogenesis: insights and innovations. J Oncol (2010) 2010:132641. doi: 10.1155/2010/132641
25. Liu G, Chen T, Ding Z, Wang Y, Wei Y, Wei X. Inhibition of FGF-FGFR and VEGF-VEGFR signalling in cancer treatment. Cell Prolif (2021) 54(4):e13009. doi: 10.1111/cpr.13009
26. Aprile G, Ongaro E, Del Re M, Lutrino SE, Bonotto M, Ferrari L, et al. Angiogenic inhibitors in gastric cancers and gastroesophageal junction carcinomas: a critical insight. Crit Rev Oncol Hematol (2015) 95(2):165–78. doi: 10.1016/j.critrevonc.2015.02.009
27. Venerito M, Link A, Rokkas T, Malfertheiner P. Gastric cancer - clinical and epidemiological aspects. Helicobacter (2016) 21 Suppl 1:39–44. doi: 10.1111/hel.12339
28. Bilgiç CI, Tez M. Serum VEGF levels in gastric cancer patients: correlation with clinicopathological parameters. Turk J Med Sci (2015) 45(1):112–7.
29. Matsumoto K, Ema M. Roles of VEGF-a signalling in development, regeneration, and tumours. J Biochem (2014) 156(1):1–10. doi: 10.1093/jb/mvu031
30. Peach CJ, Mignone VW, Arruda MA, Alcobia DC, Hill SJ, Kilpatrick LE, et al. Molecular pharmacology of VEGF-a isoforms: binding and signalling at VEGFR2. Int J Mol Sci (2018) 19(4):1264. doi: 10.3390/ijms19041264
31. Woolard J, Bevan HS, Harper SJ, Bates DO. Molecular diversity of VEGF-a as a regulator of its biological activity. Microcirculation (2009) 16(7):572–92. doi: 10.1080/10739680902997333
32. Li H, Cai E, Cheng H, Ye X, Ma R, Zhu H, et al. FGA controls VEGFA secretion to promote angiogenesis by activating the VEGFR2-FAK signalling pathway. Front Endocrinol (Lausanne) (2022) 13:791860. doi: 10.3389/fendo.2022.791860
33. Gray RT, O'Donnell ME, Maxwell P, McGuigan JA, Spence GM. Long-term follow-up of immunocytochemical analysis of vascular endothelial growth factor (VEGF), and its two receptors, VEGF-R1 (Flt-1) and VEGF-R2 (Flk-1/KDR), in oesophagogastric cancer. Int J Biol Markers (2013) 28(1):63–70. doi: 10.5301/JBM.2012.10433
34. Ling M, Quan L, Lai X, Lang L, Li F, Yang X, et al. VEGFB promotes myoblasts proliferation and differentiation through VEGFR1-PI3K/Akt signaling pathway. Int J Mol Sci (2021) 22(24):13352. doi: 10.3390/ijms222413352
35. Katoh Y, Katoh M. Comparative integromics on VEGF family members. Int J Oncol (2006) 28(6):1585–9.
36. Li XF, Zhang TG, Zhang YX. Correlation among VEGFR3 gene promoter methylation, protein overexpression, and clinical pathology in early gastric cancer. Transl Cancer Res (2020) 9(5):3499–506. doi: 10.21037/tcr.2020.03.74
37. Ribatti D, Pezzella F. Overview on the different patterns of tumor vascularization. Cells (2021) 10(3):639. doi: 10.3390/cells10030639
38. Zara S, Pokorski M, Cataldi A, Porzionato A, De Caro R, Antosiewicz J, et al. Development and aging are oxygen-dependent and correlate with VEGF and NOS along life span. Adv Exp Med Biol (2013) 756:223–8. doi: 10.1007/978-94-007-4549-0_28
39. Heinolainen K, Karaman S, D'Amico G, Tammela T, Sormunen R, Eklund L, et al. VEGFR3 modulates vascular permeability by controlling VEGF/VEGFR2 signaling. Circ Res (2017) 120(9):1414–25. doi: 10.1161/CIRCRESAHA.116.310477
40. Sahni A, Sahni SK, Simpson-Haidaris PJ, Francis CW. Fibrinogen binding potentiates FGF-2 but not VEGF induced expression of u-PA, u-PAR, and PAI-1 in endothelial cells. J Thromb Haemost (2004) 2(9):1629–36. doi: 10.1111/j.1538-7836.2004.00845.x
41. Shiose S, Hata Y, Noda Y, Sassa Y, Takeda A, Yoshikawa H, et al. Fibrinogen stimulates in vitro angiogenesis by choroidal endothelial cells via autocrine VEGF. Graefes Arch Clin Exp Ophthalmol (2004) 242(9):777–83. doi: 10.1007/s00417-004-0910-2
42. Ito TK, Ishii G, Saito S, Yano K, Hoshino A, Suzuki T, et al. Degradation of soluble VEGF receptor-1 by MMP-7 allows VEGF access to endothelial cells. Blood (2009) 113(10):2363–9. doi: 10.1182/blood-2008-08-172742
43. Wang M, Crisostomo PR, Herring C, Meldrum KK, Meldrum DR. Human progenitor cells from bone marrow or adipose tissue produce VEGF, HGF, and IGF-I in response to TNF by a p38 MAPK-dependent mechanism. Am J Physiol Regul Integr Comp Physiol (2006) 291(4):R880–4. doi: 10.1152/ajpregu.00280
44. Pin AL, Houle F, Guillonneau M, Paquet ER, Simard MJ, Huot J. miR-20a represses endothelial cell migration by targeting MKK3 and inhibiting p38 MAP kinase activation in response to VEGF. Angiogenesis (2012) 15(4):593–608. doi: 10.1007/s10456-012-9283-z
45. Ahmed A, Dunk C, Ahmad S, Khaliq A. Regulation of placental vascular endothelial growth factor (VEGF) and placenta growth factor (PIGF) and soluble flt-1 by oxygen–a review. Placenta (2000) 21 Suppl:A:S16–24. doi: 10.1053/plac.1999.0524
46. Shibuya M. Vascular endothelial growth factor and its receptor system: physiological functions in angiogenesis and pathological roles in various diseases. J Biochem (2013) 153(1):13–9. doi: 10.1093/jb/mvs136
47. Claesson-Welsh L, Welsh M. VEGFA and tumour angiogenesis. J Intern Med (2013) 273(2):114–27. doi: 10.1111/joim.12019
48. Sawada J, Li F, Komatsu M. R-ras inhibits VEGF-induced p38MAPK activation and HSP27 phosphorylation in endothelial cells. J Vasc Res (2015) 52(5):347–59. doi: 10.1159/000444526
49. Su JL, Yen CJ, Chen PS, Chuang SE, Hong CC, Kuo IH, et al. The role of the VEGF-C/VEGFR-3 axis in cancer progression. Br J Cancer (2007) 96(4):541–5. doi: 10.1038/sj.bjc.6603487
50. Matboli M, El-Nakeep S, Hossam N, Habieb A, Azazy AE, Ebrahim AE, et al. Exploring the role of molecular biomarkers as a potential weapon against gastric cancer: a review of the literature. World J Gastroenterol (2016) 22(26):5896–908. doi: 10.3748/wjg.v22.i26.5896
51. Zhou Y, Li G, Wu J, Zhang Z, Wu Z, Fan P, et al. Clinicopathological significance of e-cadherin, VEGF, and MMPs in gastric cancer. Tumour Biol (2010) 31(6):549–58. doi: 10.1007/s13277-010-0068-y
52. Zheng H, Takahashi H, Murai Y, Cui Z, Nomoto K, Niwa H, et al. Expressions of MMP-2, MMP-9 and VEGF are closely linked to growth, invasion, metastasis and angiogenesis of gastric carcinoma. Anticancer Res (2006) 26(5A):3579–83.
53. Pang L, Wang J, Fan Y, Xu R, Bai Y, Bai L. Correlations of TNM staging and lymph node metastasis of gastric cancer with MRI features and VEGF expression. Cancer biomark (2018) 23(1):53–9. doi: 10.3233/CBM-181287
54. Viallard C, Larrivée B. Tumor angiogenesis and vascular normalization: alternative therapeutic targets. Angiogenesis (2017) 20(4):409–26. doi: 10.1007/s10456-017-9562-9
55. Wang L, Chang Y, Xu J, Zhang Q. Predictive significance of serum level of vascular endothelial growth factor in gastric cancer patients. BioMed Res Int (2016) 2016:8103019. doi: 10.1155/2016/8103019
56. Ma C, Xie J, Luo C, Yin H, Li R, Wang X, et al. OxLDL promotes lymphangiogenesis and lymphatic metastasis in gastric cancer by upregulating VEGF-C expression and secretion. Int J Oncol (2019) 54(2):572–84. doi: 10.3892/ijo.2018.4648
57. Zhong J, Chen Y, Wang LJ. Emerging molecular basis of hematogenous metastasis in gastric cancer. World J Gastroenterol (2016) 22(8):2434–40. doi: 10.3748/wjg.v22.i8.2434
58. Konno H, Baba M, Tanaka T, Kamiya K, Ota M, Oba K, et al. Overexpression of vascular endothelial growth factor is responsible for the hematogenous recurrence of early-stage gastric carcinoma. Eur Surg Res (2000) 32(3):177–81. doi: 10.1159/000008760
59. Karayiannakis AJ, Syrigos KN, Polychronidis A, Zbar A, Kouraklis G, Simopoulos C, et al. Circulating VEGF levels in the serum of gastric cancer patients: correlation with pathological variables, patient survival, and tumor surgery. Ann Surg (2002) 236(1):37–42. doi: 10.1097/00000658-200207000-00007
60. Zhang Y, Brekken RA. Direct and indirect regulation of the tumor immune microenvironment by VEGF. J Leukoc Biol (2022) 111(6):1269–86. doi: 10.1002/JLB.5RU0222-082R
61. Poole RM, Vaidya A. Ramucirumab: first global approval. Drugs (2014) 74(9):1047–58. doi: 10.1007/s40265-014-0244-2
62. Jain RK. Normalization of tumor vasculature: an emerging concept in antiangiogenic therapy. Science (2005) 307(5706):58–62. doi: 10.1126/science.1104819
63. Greig SL, Keating GM. Ramucirumab: a review in advanced gastric cancer. BioDrugs (2015) 29(5):341–51. doi: 10.1007/s40259-015-0138-1
64. Spratlin JL, Mulder KE, Mackey JR. Ramucirumab (IMC-1121B): a novel attack on angiogenesis. Future Oncol (2010) 6(7):1085–94. doi: 10.2217/fon.10.75
65. Elimova E, Lin Q, Song S, Ajani JA. Current status of ramucirumab in gastroesophageal adenocarcinoma. Future Oncol (2017) 13(18):1585–92. doi: 10.2217/fon-2016-0556
66. Wilke H, Muro K, Van Cutsem E, Oh SC, Bodoky G, Shimada Y, et al. Ramucirumab plus paclitaxel versus placebo plus paclitaxel in patients with previously treated advanced gastric or gastro-oesophageal junction adeno- carcinoma (RAINBOW): a double-blind, randomised phase 3 trial. Lancet Oncol (2014) 15(11):1224–35. doi: 10.1016/S1470-2045(14)70420-6
67. Fuchs CS, Shitara K, Di Bartolomeo M, Lonardi S, Al-Batran SE, Van Cutsem E, et al. Ramucirumab with cisplatin and fluoropyrimidine as first-line therapy in patients with metastatic gastric or junctional adenocarcinoma (RAINFALL): a double-blind, randomised, placebo-controlled, phase 3 trial. Lancet Oncol (2019) 20(3):420–35. doi: 10.1016/S1470-2045(18)30791-5
68. Cascinu S, Bodoky G, Muro K, Van Cutsem E, Oh SC, Folprecht G, et al. Tumor response and symptom palliation from RAINBOW, a phase III trial of ramucirumab plus paclitaxel in previously treated advanced gastric cancer. Oncologist (2021) 26(3):e414–24. doi: 10.1002/onco.13623
69. Klempner SJ, Maron SB, Chase L, Lomnicki S, Wainberg ZA, Catenacci DVT. Initial report of second-line FOLFIRI in combination with ramucirumab in advanced gastroesophageal adenocarcinomas: a multi-institutional retrospec- tive analysis. Oncologist (2019) 24(4):475–82. doi: 10.1634/theoncologist.2018-0602
70. Yoshikawa T, Muro K, Shitara K, Oh DY, Kang YK, Chung HC, et al. Effect of first-line s-1 plus oxaliplatin with or without ramucirumab followed by paclitaxel plus ramucirumab on advanced gastric cancer in East Asia: the phase 2 RAINSTORM randomized clinical trial. JAMA Netw Open (2019) 2(8):e198 243. doi: 10.1001/jamanetworkopen.2019.8243
71. Ohtsu A, Shah MA, Van Cutsem E, Rha SY, Sawaki A, Park SR, et al. Bevacizumab in combination with chemotherapy as first-line therapy in advanced gastric cancer: a randomized, double-blind, placebo-controlled phase III study. J Clin Oncol (2011) 29(30):3968–76. doi: 10.1200/JCO.2011.36.2236
72. Shah MA, Ramanathan RK, Ilson DH, Levnor A, D, Adamo D, O'Reilly E, et al. Multicenter phase II study of irinotecan, cisplatin, and bevacizumab in patients with metastatic gastric or gastroesophageal junction adenocarcinoma. J Clin Oncol (2006) 24:5201–6. doi: 10.1200/JCO.2006.08.0887
73. Fuchs CS, Tomasek J, Yong CJ, Dumitru F, Passalacqua R, Goswami C, et al. Ramucirumab monotherapy for previously treated advanced gastric or gastro-oesophageal junction adenocarcinoma (REGARD): an international, randomised, multicentre, placebo-controlled, phase 3 trial. Lancet (2014) 383(9911):31–9. doi: 10.1016/S0140-6736(13)61719-5
74. Yamaguchi K, Fujitani K, Nagashima F, Omuro Y, Machida N, Nishina T, et al. Ramucirumab for the treatment of metastatic gastric or gastroesophageal junction adenocarcinoma following disease progression on first-line platinum- or fluoropyrimidine-containing combination therapy in Japanese patients: a phase 2, open-label study. Gastric Cancer (2018) 21(6):1041–9. doi: 10.1007/s10120-018-0811-4
75. Sasako M. Ramucirumab: second-line therapy for gastric cancer. Lancet Oncol (2014) 15(11):1182–4. doi: 10.1016/S1470-2045(14)70464-4
76. Shitara K, Muro K, Shimada Y, Hironaka S, Sugimoto N, Komatsu Y, et al. Subgroup analyses of the safety and efficacy of ramucirumab in Japanese and Western patients in RAINBOW: a randomized clinical trial in second-line treatment of gastric cancer. Gastric Cancer (2016) 19(3):927–38. doi: 10.1007/s10120-015-0559-z
77. Muro K, Oh SC, Shimada Y, Lee KW, Yen CJ, Chao Y, et al. Subgroup analysis of East asians in RAINBOW: a phase 3 trial of ramucirumab plus paclitaxel for advanced gastric cancer. J Gastroenterol Hepatol (2016) 31(3):581–9. doi: 10.1111/jgh.13153
78. Davidson M, Chau I. Variations in outcome for advanced gastric cancer between Japanese and Western patients: a subgroup analysis of the RAINBOW trial. Transl Gastroenterol Hepatol (2016) 1:46. doi: 10.21037/tgh.2016.05.06
79. Xu RH, Zhang Y, Pan H, Feng J, Zhang T, Liu T, et al. Efficacy and safety of weekly paclitaxel with or without ramucirumab as second-line therapy for the treatment of advanced gastric or gastroesophageal junction adenocarcinoma (RAINBOW-asia): a randomised, multicentre, double-blind, phase 3 trial. Lancet Gastroenterol Hepatol (2021) 6(12):1015–24. doi: 10.1016/S2468-1253(21)00313-7
80. Yoon HH. Ramucirumab plus paclitaxel for gastric cancer in China. Lancet Gastroenterol Hepatol (2021) 6(12):975–6. doi: 10.1016/S2468-1253(21)00342-3
81. Chellappan DK, Leng KH, Jia LJ, Aziz NABA, Hoong WC, Qian YC, et al. The role of bevacizumab in tumor angiogenesis and in the management of gynecologic cancers: a review. BioMed Pharmacother (2018) 102:1127–44. doi: 10.1016/biopha.2018.03.061
82. Oza AM, Dubois F, Hegg R, Hernandez CA, Finocchiaro G, Ghiringhelli F, et al. A long-term extension study of bevacizumab in patients with solid tumors. Oncologist (2021) 26(12):e2254–64. doi: 10.1002/onco.13971
83. Gay ND, Tao D, Prasad V. Multiplicity and the marginal benefits of bevacizumab in malignant solid tumours. Curr Oncol (2019) 26(6):e791–2. doi: 10.3747/co.26.4845
84. Ferrara N, Hillan KJ, Gerber HP, Novotny W. Discovery and development of bevacizumab, an anti-VEGF antibody for treating cancer. Nat Rev Drug Discovery (2004) 3(5):391–400. doi: 10.1038/nrd1381
85. Papachristos A, Kemos P, Kalofonos H, Sivolapenko G. Correlation between bevacizumab exposure and survival in patients with metastatic colorectal cancer. Oncologist (2020) 25(10):853–8. doi: 10.1634/theoncologist.2019-0835
86. de Gramont A, Van Cutsem E. Investigating the potential of bevacizumab in other indications: metastatic renal cell, non-small cell lung, pancreatic and breast cancer. Oncol (2005) (69) Suppl 3:46–56. doi: 10.1159/000088483
87. Mountzios G, Pentheroudakis G, Carmeliet P. Bevacizumab and micrometastases: revisiting the preclinical and clinical rollercoaster. Pharmacol Ther (2014) 141(2):117–24. doi: 10.1016/j.pharmthera.2013.09.003
88. Goldstein DA, Chen Q, Ayer T, Chan KKW, Virik K, Hammerman A, et al. Bevacizumab for metastatic colorectal cancer: a global cost-effectiveness analysis. Oncologist (2017) 22(6):694–9. doi: 10.1634/theoncologist.2016-0455
89. Norguet E, Dahan L, Seitz JF. Targetting esophageal and gastric cancers with monoclonal antibodies. Curr Top Med Chem (2012) 12(15):1678–82. doi: 10.2174/156802612803531360
90. Cunningham D, Stenning SP, Smyth EC, Okines AF, Allum WH, Rowley S, et al. Peri-operative chemotherapy with or without bevacizumab in operable oesophagogastric adenocarcinoma (UK medical research council ST03): primary analysis results of a multicentre, open-label, randomised phase 2-3 trial. Lancet Oncol (2017) 18(3):357–70. doi: 10.1016/S1470-2045(17)30043-8
91. Thiel A, Ristimäki A. Targeted therapy in gastric cancer. APMIS (2015) 123(5):365–72. doi: 10.1111/apm.12359
92. Shen L, Li J, Xu J, Pan H, Dai G, Qin S, et al. Bevacizumab plus capecitabine and cisplatin in Chinese patients with inoperable locally advanced or metastatic gastric or gastroesophageal junction cancer: randomized, double-blind, phase III study (AVATAR study). Gastric Cancer (2015) 18(1):168–76. doi: 10.1007/s10120-014-0351-5
93. Xue JM, Astère M, MX Z, Lin H, Shen J, Zhu YX. Efficacy and safety of apatinib treatment for gastric cancer, hepatocellular carcinoma and non-small cell lung cancer: a meta-analysis. Onco Targets Ther (2018) 11:6119–28. doi: 10.2147/OTT.S172717
94. Li J, Qin S, Xu J, Guo W, Xiong J, Bai Y, et al. Apatinib for chemotherapy-refractory: results from a randomized, placebo-controlled, parallel-arm, phase II trial. J Clin Oncol (2013) 31(26):3219–25. doi: 10.1200/JCO.2013.48.8585
95. Li J, Qin S, Xu J, Xiong J, Wu C, Bai Y, et al. Randomized, double-blind, placebo-controlled phase III trial of apatinib in patients with chemotherapy-refractory advanced or metastatic adenocarcinoma of the stomach or gastroesophageal junction. J Clin Oncol (2016) 34(13):1448–54. doi: 10.1200/JCO.2015.63.5995
96. Shen B, Jiang H, Wang L, Qian J, Shu Y, Chen P, et al. Effectiveness and safety of apatinib in patients with advanced or metastatic adenocarcinoma of stomach or gastroesophageal junction: a prospective observation study. Onco Targets Ther (2020) 13:4457–64. doi: 10.2147/OTT.S232287
97. Ruan H, Dong J, Zhou X, Xiong J, Wang H, Zhong X, et al. Multicenter phase II study of apatinib treatment for metastatic gastric cancer after failure of second-line chemotherapy. Oncotarget (2017) 8(61):104552–9. doi: 10.18632/oncotarge-t.21053
98. Zhou N, Zhang C, Liu D, Liu K, Wang G, Zhu H, et al. Apatinib in combination with s-1 as first-line treatment in patients with advanced metastatic gastric cancer: results from an open, exploratory, single-arm, phase II trial. Oncologist (2021) 26(3):e374–81. doi: 10.1002/onco.13613
99. Wang X, Zhang R, Du N, Yang M, Zang A, Liu L, et al. An open label, multicenter, noninterventional study of apatinib in advanced gastric cancer patients (AHEAD-G202). Ther Adv Med Oncol (2020) 12:1758835920905424. doi: 10.1177/1758835920905424
100. Li N, Bai C, Zhang R, Ma L, Ren X, Zhang J, et al. Efficacy and safety of apatinib for the treatment of AFP-producing gastric cancer. Transl Oncol (2021) 14(2):101 004. doi: 10.1016/j.tranon.2020.101004
101. Lin JX, Xu YC, Lin W, Xue FQ, Ye JX, Zang WD, et al. Effectiveness and safety of apatinib plus chemotherapy as neoadjuvant treatment for locally advanced gastric cancer: a nonrandomized controlled trial. JAMA Netw Open (2021) 4(7):e2116240. doi: 10.1001/jamanetworkopen.2021.16240
102. Ren D, Wang G, Zhang Y, Kan J, Dong Q, Zhao J, et al. Efficacy and safety of apatinib for elderly patients with advanced or metastatic gastric cancer after failure of at least first-line chemotherapy: a multi-center, single-arm, phase II study. Onco Targets Ther (2021) 14:4499–508. doi: 10.2147/OTT.S322710
103. Wang X, Yu J, Yang M, Liu L, Gao J, Ren Y, et al. Safety and effectiveness of apatinib in patients with previously treated metastatic gastric cancer: a sub-analysis from the real-world study of apatinib for gastric cancer treatment (AHEAD-G202). Am J Cancer Res (2020) 10(3):987–96.
104. Du Y, Cao Q, Jiang C, Liang H, Ning Z, Ji C, et al. Effectiveness and safety of low-dose apatinib in advanced gastric cancer: a real-world study. Cancer Med (2020) 9(14):5008–14. doi: 10.1002/cam4.3105
105. Chen H, Xue L, Liu L, Li P. Efficacy of apatinib combined with tegafur gimeracil and oteracil potassium in the second-line treatment of advanced gastric cancer. J BUON (2021) 26(3):917–23.
106. Wu Q, Fu Y, Wen W, Xi T, Zhao G. Efficacy and prognosis analyses of apatinib combined with s-1 in third-line chemotherapy for advanced gastric cancer. J BUON (2020) 25(2):987–94.
107. Gu J, Ni X, Ji J, Wei G, Shi L, Xu C. Efficacy of apatinib plus s-1 therapy in the treatment of advanced gastric cancer patients and the effect on the levels of tumor markers and Th1 and Th2-like cytokines. Evid Based Complement Alternat Med (2022) 2022:8060026. doi: 10.1155/2022/8060026
108. Jing C, Bai Z, Zhang J, Jiang H, Yang X, Yan S, et al. Apatinib plus s-1 for previously treated, advanced gastric or gastro-oesophageal junction adenocarcinoma: a phase 2, single-arm, prospective study. J Gastrointest Oncol (2021) 12(5):2035–44. doi: 10.21037/jgo-21-186
109. Li C, Tang T, Wang W. Combination use of tegafur and apatinib as first-line therapy in treatment of advanced gastric cancer: a single-blinded randomized study. Gastroenterol Res Pract (2020) 2020:3232950. doi: 10.1155/2020/3232950
110. Ye Z, Zeng Y, Wei S, Wang Y, Lin Z, Chen S, et al. Short-term survival and safety of apatinib combined with oxaliplatin and s-1 in the conversion therapy of unresectable gastric cancer. BMC Cancer (2021) 21(1):702. doi: 10.1186/s12885-021-08459-3
111. Shirley M. Fruquintinib: first global approval. Drugs (2018) 78(16):1757–61. doi: 10.1007/s40265-018-0998-z
112. Janjigian YY, Vakiani E, Ku GY, Herrera JM, Tang LH, Bouvier N, et al. Phase II trial of sorafenib in patients with chemotherapy refractory metastatic esophageal and gastroesophageal (GE) junction cancer. PloS One (2015) 10(8):e0134731. doi: 10.1371/journal.pone.0134731
113. Kim C, Lee JL, Choi YH, Kang BW, Ryu MH, Chang HM, et al. Phase I dose-finding study of sorafenib in combination with capecitabine and cisplatin as a first-line treatment in patients with advanced gastric cancer. Invest New Drugs (2012) 30(1):306–15. doi: 10.1007/s10637-010-9531-2
114. Sun W, Powell M, O'Dwyer PJ, Catalano P, Ansari RH, Benson AB 3rd. Phase II study of sorafenib in combination with docetaxel and cisplatin in the treatment of metastatic or advanced gastric and gastroesophageal junction adenocarcinoma: ECOG 5203. J Clin Oncol (2010) 28(18):2947–51. doi: 10.1200/JCO.2009.27.7988
115. Yamada Y, Kiyota N, Fuse N, Kato K, Minami H, Hashizume K, et al. A phase I study of sorafenib in combination with s-1 plus cisplatin in patients with advanced gastric cancer. Gastric Cancer (2014) 17(1):161–72. doi: 10.1007/s10120-013-0247-9
116. Cheng R, Yong H, Xia Y, Xie Q, Gao G, Zhou X. Chemotherapy regimen based on sorafenib combined with 5-FU on HIF-1α and VEGF expression and survival in advanced gastric cancer patients. Oncol Lett (2017) 13(4):2703–7. doi: 10.3892/ol.2017.5769
117. Bang YJ, Kang YK, Kang WK, Boku N, Chung HC, Chen JS, et al. Phase II study of sunitinib as second-line treatment for advanced gastric cancer. Invest New Drugs (2011) 29(6):1449–58. doi: 10.1007/s10637-010-9438-y
118. Moehler M, Gepfner-Tuma I, Maderer A, Thuss-Patience PC, Ruessel J, Hegewisch-Becker S, et al. Sunitinib added to FOLFIRI versus FOLFIRI in patients with chemorefractory advanced adenocarcinoma of the stomach or lower esophagus: a randomized, placebo-controlled phase II AIO trial with serum biomarker program. BMC Cancer (2016) 16(1):699. doi: 10.1186/s12885-016-2736-9
119. Mukherjee S, Fountzilas C, Boland PM, Gosain R, Attwood K, Tan W, et al. Phase I study of Irinotecan/5-Fluorouracil/Leucovorin (FOLFIRI) with sunitinib for advanced gastric or gastroesophageal junction adenocarcinoma. Target Oncol (2020) 15(1):85–92. doi: 10.1007/s11523-019-00692-y
120. Boku N, Muro K, Machida N, Hashigaki S, Kimura N, Suzuki M, et al. Phase I study of sunitinib plus s-1 and cisplatin in Japanese patients with advanced or metastatic gastric cancer. Invest New Drugs (2014) 32(2):261–70. doi: 10.1007/s10637-013-9948-5
121. Lee KW, Park SR, Oh DY, Park YI, Khosravan R, Lin X, et al. Phase I study of sunitinib plus capecitabine/cisplatin or capecitabine/oxaliplatin in advanced gastric cancer. Invest New Drugs (2013) 31(6):1547–58. doi: 10.1007/s10637-013-0032-y
122. Kim ST, Lee J, Lee SJ, Park SH, Jung SH, Park YS, et al. Prospective phase II trial of pazopanib plus CapeOX (capecitabine and oxaliplatin) in previously untreated patients with advanced gastric cancer. Oncotarget (2016) 7(17):24088–96. doi: 10.18632/oncotarget.8175
123. Högner A, Al-Batran SE, Siveke JT, Lorenz M, Bartels P, Breithaupt K, et al. Pazopanib with 5-FU and oxaliplatin as first line therapy in advanced gastric cancer: a randomized phase-II study-the PaFLO trial. a study of the arbeitsgemeinschaft internistische onkologie AIO-STO-0510. Int J Cancer (2022) 150(6):1007–17. doi: 10.1002/ijc.33864
124. Scott LJ. Apatinib: a review in advanced gastric cancer and other advanced cancers. Drugs (2018) 78(7):747–58. doi: 10.1007/s40265-018-0903-9
125. Tian Z, Niu X, Yao W. Efficacy and response biomarkers of apatinib in the treatment of malignancies in China: a review. Front Oncol (2021) 11:749083. doi: 10.3389/fonc.2021.749083
126. Mizukami T, Piao Y. Role of nutritional care and general guidance for patients with advanced or metastatic gastric cancer. Future Oncol (2021) 17(23):3101–9. doi: 10.2217/fon-2021-0186
127. Wang X, Zhong D, Zhang J, Du N, Ren Y, Gao J, et al. Safety and effectiveness of apatinib in elderly patients with metastatic gastric cancer: a sub-analysis from the large-scale, prospective observational study of apatinib for gastric cancer treatment in a real-world clinical setting (AHEAD-G202). J Gastrointest Oncol (2022) 13(4):1679–89. doi: 10.21037/jgo-22-727
128. Lin H, Han D, Fu G, Liu C, Wang L, Han S, et al. Concurrent apatinib and docetaxel vs apatinib monotherapy as third- or subsequent-line therapy for advanced gastric adenocarcinoma: a retrospective study. Onco Targets Ther (2019) 12:1681–9. doi: 10.2147/OTT.S193801
129. Cheng H, Sun A, Guo Q, Zhang Y. Efficacy and safety of apatinib combined with chemotherapy for the treatment of advanced gastric cancer in the Chinese population: a systematic review and meta-analysis. Drug Des Devel Ther (2018) 12:2173–83. doi: 10.2147/DDDT.S170678
130. Zhang Y, Xu J, Wang Q, Ling G, Mao Y, Cai M, et al. Efficacy and safety of second-line therapy with apatinib combined with chemotherapy as second-line therapy in advanced gastric cancer: a single-arm, open-label, prospective, multicenter study. Ann Transl Med (2022) 10(11):641. doi: 10.21037/atm-22-2752
131. Geng R, Song L, Li J, Zhao L. The safety of apatinib for the treatment of gastric cancer. Expert Opin Drug Saf (2018) 17(11):1145–50. doi: 10.1080/14740338.2018.1535592
132. Peng W, Zhang F, Wang Z, Li D, He Y, Ning Z, et al. Large Scale, multicenter, prospective study of apatinib in advanced gastric cancer: a real-world study from China. Cancer Manag Res (2020) 12:6977–85. doi: 10.2147/CMAR.S249153
133. Li J, Qin S, Xu RH, Shen L, Xu J, Bai Y, et al. Effect of fruquintinib vs placebo on overall survival in patients with previously treated metastatic colorectal cancer: the FRESCO randomized clinical trial. JAMA (2018) 319(24):2486–96. doi: 10.1001/jama.2018.7855
134. Sun Q, Zhou J, Zhang Z, Guo M, Liang J, Zhou F, et al. Discovery of fruquintinib, a potent and highly selective small molecule inhibitor of VEGFR 1, 2, 3 tyrosine kinases for cancer therapy. Cancer Biol Ther (2014) 15(12):1635–45. doi: 10.4161/15384047.2014.964087
135. Anastassiadis T, Deacon SW, Devarajan K, Ma H, Peterson JR. Comprehensive assay of kinase catalytic activity reveals features of kinase inhibitor selectivity. Nat Biotechnol (2011) 29(11):1039–45. doi: 10.1038/nbt.2017
136. Jayson GC, Kerbel R, Ellis LM, Harris AL. Antiangiogenic therapy in oncology: current status and future directions. Lancet (2016) 388(10043):518–29. doi: 10.1016/S0140-6736(15)01088-0
137. Hartmann JT, Haap M, Kopp HG, Lipp HP. Tyrosine kinase inhibitors - a review on pharmacology, metabolism and side effects. Curr Drug Metab (2009) 10(5):470–81. doi: 10.2174/138920009788897975
138. Liu L, Cao Y, Chen C, Zhang X, McNabola A, Wilkie D, et al. Sorafenib blocks the RAF/MEK/ERK pathway, inhibits tumor angiogenesis, and induces tumor cell apoptosis in hepatocellular carcinoma model PLC/PRF/5. Cancer Res (2006) 66(24):11851–8. doi: 10.1158/0008-5472.CAN-06-1377
139. Kim HM, Kim SA, Park SB, Cho JH, Song SY. Sorafenib inhibits 5-fluorouracil-resistant gastric cancer cell growth. Scand J Gastroenterol (2017) 52(5):577–84. doi: 10.1080/00365521.2017.1278786
140. Martin-Richard M, Gallego R, Pericay C, Garcia Foncillas J, Queralt B, Casado E, et al. Multicenter phase II study of oxaliplatin and sorafenib in advanced gastric adenocarcinoma after failure of cisplatin and fluoropyrimidine treatment. A GEMCAD study Invest New Drugs (2013) 31(6):1573–9. doi: 10.1007/s10637-013-0020-2
141. Tao C, Lin H, Chen S. The regulation of ERK and p-ERK expression by cisplatin and sorafenib in gastric cancer cells. Gene (2014) 552(1):106–15. doi: 10.1016/j.gene.2014.09.019
142. Cidon EU, Ellis SG, Inam Y, Adeleke S, Zarif S, Geldart T. Molecular targeted agents for gastric cancer: a step forward towards personalized therapy. Cancers (Basel) (2013) 5(1):64–91. doi: 10.3390/cancers5010064
143. Zhang Y, Wang Q. Sunitinib reverse multidrug resistance in gastric cancer cells by modulating Stat3 and inhibiting p-gp function. Cell Biochem Biophys (2013) 67(2):575–81. doi: 10.1007/s12013-013-9544-5
144. Gómez-Martín C, Salazar R, Montagut C, Gil-Martín M, Núñez JA, Puig M, et al. Dose-finding study of sunitinib combined with cisplatin and 5-fluorouracil in patients with advanced gastric cancer. Invest New Drugs (2013) 31(2):390–8. doi: 10.1007/s10637-012-9830-x
145. Frampton JE. Pazopanib: a review in advanced renal cell carcinoma. Target Oncol (2017) 12(4):543–54. doi: 10.1007/s11523-017-0511-8
146. Schöffski P. Pazopanib in the treatment of soft tissue sarcoma. Expert Rev Anticancer Ther (2012) 12(6):711–23. doi: 10.1586/era.12.41
147. Gupta VG, Rangaraju RR, Saha R, Bajpai P. Pazopanib monotherapy is active in relapsed and refractory metastatic gastroesophageal adenocarcinoma and can produce durable response. J Gastrointest Cancer (2019) 50(4):943–6. doi: 10.1007/s12029-018-0130-3
148. Tyczyńska M, Kędzierawski P, Karakuła K, Januszewski J, Kozak K, Sitarz M, et al. Treatment strategies of gastric cancer-molecular targets for anti-angiogenic therapy: a state-of-the-art review. J Gastrointest Cancer (2021) 52(2):476–88. doi: 10.1007/s12029-021-00629-7
149. Chan DL, Sjoquist KM, Goldstein D, Price TJ, Martin AJ, Bang YJ, et al. The effect of anti-angiogenic agents on overall survival in metastatic oesophago-gastric cancer: a systematic review and meta-analysis. PloS One (2017) 12(2):e0172307. doi: 10.1371/journal.pone.0172307
150. Fornaro L, Musettini G, Orlandi P, Pecora I, Vivaldi C, Banchi M, et al. Early increase of plasma soluble VEGFR-2 is associated with clinical benefit from second-line treatment of paclitaxel and ramucirumab in advanced gastric cancer. Am J Cancer Res (2022) 12(7):3347–56.
151. Van Cutsem E, de Haas S, Kang YK, Ohtsu A, Tebbutt NC, Ming Xu J, et al. Bevacizumab in combination with chemotherapy as first-line therapy in advanced gastric cancer: a biomarker evaluation from the AVAGAST randomized phase III trial. J Clin Oncol (2012) 30(17):2119–27. doi: 10.1200/JCO.2011.39.9824
152. Zhu CL, Huang Q, Liu CH, Lin XS, Xie F. Prognostic value of HIF-1α expression in patients with gastric cancer. Mol Biol Rep (2013) 40(11):6055–62. doi: 10.1007/s11033-013-2715-z
153. Moehler M, Mueller A, Hartmann JT, Ebert MP, Al-Batran SE, Reimer P, et al. An open-label, multicentre biomarker-oriented AIO phase II trial of sunitinib for patients with chemo-refractory advanced gastric cancer. Eur J Cancer (2011) 47(10):1511–20. doi: 10.1016/j.ejca.2011.04.006
154. Kim ST, Ahn S, Lee J, Lee SJ, Park SH, Park YS, et al. Value of FGFR2 expression for advanced gastric cancer patients receiving pazopanib plus CapeOX (capecitabine and oxaliplatin). J Cancer Res Clin Oncol (2016) 142(6):1231–7. doi: 10.1007/s00432-016-2143-2
155. Shan F, Miao R, Xue K, Li Z, Li Z, Bu Z, et al. Controlling angiogenesis in gastric cancer: a systematic review of anti-angiogenic trials. Cancer Lett (2016) 380(2):598–607. doi: 10.1016/j.canlet.2015.12.023
156. Tabernero J, Ohtsu A, Muro K, Van Cutsem E, Oh SC, Bodoky G, et al. Exposure-response analyses of ramucirumab from two randomized, phase III trials of second-line treatment for advanced gastric or gastroesophageal junction cancer. Mol Cancer Ther (2017) 16(10):2215–22. doi: 10.1158/1535-7163.MCT-16-0895
157. Spratlin JL, Cohen RB, Eadens M, Gore L, Camidge DR, Diab S, et al. Phase I pharmacologic and biologic study of ramucirumab (IMC-1121B), a fully human immunoglobulin G1 monoclonal antibody targeting the vascular endothelial growth factor receptor-2. J Clin Oncol (2010) 28(5):780–7. doi: 10.1200/JCO.2009.23.7537
158. Liu W, Dong Z, Hu R, Wang C. Association of vascular endothelial growth factor (VEGF) gene polymorphisms with gastric cancer and its development, prognosis, and survival. Technol Cancer Res Treat (2018) 17:1533034617753810. doi: 10.1177/1533034617753810
159. Fuchs CS, Tabernero J, Tomášek J, Chau I, Melichar B, Safran H, et al. Biomarker analyses in REGARD gastric/GEJ carcinoma patients treated with VEGFR2-targeted antibody ramucirumab. Br J Cancer (2016) 115(8):974–82. doi: 10.1038/bjc.2016.293
160. Teleanu RI, Chircov C, Grumezescu AM, Teleanu DM. Tumor angiogenesis and anti-angiogenic strategies for cancer treatment. J Clin Med (2019) 9(1):84. doi: 10.3390/jcm9010084
161. Lyu N, Wang X, Li JB, Lai JF, Chen QF, Li SL, et al. Arterial chemotherapy of oxaliplatin plus fluorouracil versus sorafenib in advanced hepatocellular carcinoma: a biomolecular exploratory, randomized, phase III trial (FOHAIC-1). J Clin Oncol (2022) 40(5):468–80. doi: 10.1200/JCO.21.01963
162. Blumenschein GR Jr, Saintigny P, Liu S, Kim ES, Tsao AS, Herbst RS, et al. Comprehensive biomarker analysis and final efficacy results of sorafenib in the BATTLE trial. Clin Cancer Res (2013) 19(24):6967–75. doi: 10.1158/1078-0432.CCR-12-1818
163. Nakamura J, Kitajima Y, Kai K, Mitsuno M, Ide T, Hashiguchi K, et al. Hypoxia-inducible factor-1alpha expression predicts the response to 5-fluorouracil-based adjuvant chemotherapy in advanced gastric cancer. Oncol Rep (2009) 22(4):693–9. doi: 10.3892/or_00000489
164. Shahneh FZ, Baradaran B, Zamani F, Aghebati-Maleki L. Tumor angiogenesis and anti-angiogenic therapies. Hum Antibodies (2013) 22(1-2):15–9. doi: 10.3233/HAB-130267
165. Olejarz W, Kubiak-Tomaszewska G, Chrzanowska A, Lorenc T. Exosomes in angiogenesis and anti-angiogenic therapy in cancers. Int J Mol Sci (2020) 21(16):5840. doi: 10.3390/ijms21165840
166. Lopes-Coelho F, Martins F, Pereira SA, Serpa J. Anti-angiogenic therapy: current challenges and future perspectives. Int J Mol Sci (2021) 22(7):3765. doi: 10.3390/ijms22073765
167. Goel S, Duda DG, Xu L, Munn LL, Boucher Y, Fukumura D, et al. Normalization of the vasculature for treatment of cancer and other diseases. Physiol Rev (2011) 91(3):1071–121. doi: 10.1152/physrev.00038.2010
168. De Palma M, Biziato D, Petrova TV. Microenvironmental regulation of tumour angiogenesis. Nat Rev Cancer (2017) 17(8):457–74. doi: 10.1038/nrc.2017.51
169. Kuczynski EA, Vermeulen PB, Pezzella F, Kerbel RS, Reynolds AR. Vessel co-option in cancer. Nat Rev Clin Oncol (2019) 16(8):469–93. doi: 10.1038/s41571-019-0181-9
170. Han YL, Chen L, Qin R, Wang GQ, Lin XH, Dai GH. Lysyl oxidase and hypoxia-inducible factor 1α: biomarkers of gastric cancer. World J Gastroenterol (2019) 25(15):1828–39. doi: 10.3748/wjg.v25.i15.1828
171. Suarez-Carmona M, Lesage J, Cataldo D, Gilles C. EMT and inflammation: inseparable actors of cancer progression. Mol Oncol (2017) 11(7):805–23. doi: 10.1002/1878-0261.12095
172. Matsuoka J, Yashiro M, Doi Y, Fuyuhiro Y, Kato Y, Shinto O, et al. Hypoxia stimulates the EMT of gastric cancer cells through autocrine TGFβ signaling. PloS One (2013) 8(5):e62310. doi: 10.1371/journal.pone.0062310
173. Majmundar AJ, Wong WJ, Simon MC. Hypoxia-inducible factors and the response to hypoxic stress. Mol Cell (2010) 40(2):294–309. doi: 10.1016/j.molcel.2010.09.022
174. Pennacchietti S, Michieli P, Galluzzo M, Mazzone M, Giordano S, Comoglio PM. Hypoxia promotes invasive growth by transcriptional activation of the met protooncogene. Cancer Cell (2003) 3(4):347–61. doi: 10.1016/s1535-6108(03)00085-0
175. Kim JH, Park SS, Park SH, Kim SJ, Mok YJ, Kim CS, et al. Clinical significance of immunohistochemically-identified lymphatic and/or blood vessel tumor invasion in gastric cancer. J Surg Res (2010) 162(2):177–83. doi: 10.1016/j.jss.2009.07.015
176. Du R, Lu KV, Petritsch C, Liu P, Ganss R, Passegué E, et al. HIF1alpha induces the recruitment of bone marrow-derived vascular modulatory cells to regulate tumor angiogenesis and invasion. Cancer Cell (2008) 13(3):206–20. doi: 10.1016/j.ccr.2008.01.034
177. Seandel M, Butler J, Lyden D, Rafii S. A catalytic role for proangiogenic marrow-derived cells in tumor neovascularization. Cancer Cell (2008) 13(3):181–3. doi: 10.1016/j.ccr.2008.02.016
178. Mizukami Y, Sasajima J, Ashida T, Kohgo Y. Abnormal tumor vasculatures and bone marrow-derived pro-angiogenic cells in cancer. Int J Hematol (2012) 95(2):125–30. doi: 10.1007/s12185-012-1017-x
179. Casanovas O, Hicklin DJ, Bergers G, Hanahan D. Drug resistance by evasion of antiangiogenic targeting of VEGF signaling in late-stage pancreatic islet tumors. Cancer Cell (2005) 8(4):299–309. doi: 10.1016/j.ccr.2005.09.005
180. Ebos JM, Lee CR, Cruz-Munoz W, Bjarnason GA, Christensen JG, Kerbel RS. Accelerated metastasis after short-term treatment with a potent inhibitor of tumor angiogenesis. Cancer Cell (2009) 15(3):232–9. doi: 10.1016/j.ccr.2009.01.021
181. De Falco S. Antiangiogenesis therapy: an update after the first decade. Korean J Intern Med (2014) 29(1):1–11. doi: 10.3904/kjim.2014.29.1.1
182. Maeda H, Wu J, Sawa T, Matsumura Y, Hori K. Tumor vascular permeability and the EPR effect in macromolecular therapeutics: a review. J Control Release (2000) 65(1-2):271–84. doi: 10.1016/s0168-3659(99)00248-5
183. Ostuni R, Kratochvill F, Murray PJ, Natoli G. Macrophages and cancer: from mechanisms to therapeutic implications. Trends Immunol (2015) 36(4):229–39. doi: 10.1016/j.it.2015.02.004
184. John A, Tuszynski G. The role of matrix metalloproteinases in tumor angiogenesis and tumor metastasis. Pathol Oncol Res (2001) 7(1):14–23. doi: 10.1007/BF03032599
185. Wei X, Chen Y, Jiang X, Peng M, Liu Y, Mo Y, et al. Mechanisms of vasculogenic mimicry in hypoxic tumor microenvironments. Mol Cancer (2021) 20(1):7. doi: 10.1186/s12943-020-01288-1
186. Colli LM, Machiela MJ, Zhang H, Myers TA, Jessop L, Delattre O, et al. Landscape of combination immunotherapy and targeted therapy to improve cancer management. Cancer Res (2017) 77(13):3666–71. doi: 10.1158/0008-5472.CAN-16-3338
187. Syukri A, Budu, Hatta M, Amir M, Rohman MS, Mappangara I, et al. Doxorubicin induced immune abnormalities and inflammatory responses via HMGB1, HIF1-α and VEGF pathway in progressive of cardiovascular damage. Ann Med Surg (Lond) (2022) 76:103501. doi: 10.1016/j.amsu.2022.103501
188. Meulendijks D, Beerepoot LV, Boot H, de Groot JW, Los M, Boers JE, et al. Trastuzumab and bevacizumab combined with docetaxel, oxaliplatin and capecitabine as first-line treatment of advanced HER2-positive gastric cancer: a multicenter phase II study. Invest New Drugs (2016) 34(1):119–28. doi: 10.1007/s10637-015-0309-4
189. Zeng H, Xu Q, Wang J, Xu X, Luo J, Zhang L, et al. The effect of anti-PD-1/PD-L1 antibodies combined with VEGF receptor tyrosine kinase inhibitors versus bevacizumab in unresectable hepatocellular carcinoma. Front Immunol (2023) 14:1073133. doi: 10.3389/fimmu.2023.1073133
190. Bhattarai P, Hameed S, Dai Z. Recent advances in anti-angiogenic nanomedicines for cancer therapy. Nanoscale (2018) 10(12):5393–423. doi: 10.1039/c7nr09612g
191. Saeed BA, Lim V, Yusof NA, Khor KZ, Rahman HS, Abdul Samad N. Antiangiogenic properties of nanoparticles: a systematic review. Int J Nanomedicine (2019) 14:5135–46. doi: 10.2147/IJN.S199974
192. Chen Z, Li Y, Tan B, Zhao Q, Fan L, Li F, et al. Progress and current status of molecule-targeted therapy and drug resistance in gastric cancer. Drugs Today (Barc) (2020) 56(7):469–82. doi: 10.1358/dot.2020.56.7.3112071
193. Ramjiawan RR, Griffioen AW, Duda DG. Anti-angiogenesis for cancer revisited: is there a role for combinations with immunotherapy? Angiogenesis (2017) 20(2):185–204. doi: 10.1007/s10456-017-9552-y
Keywords: gastric cancer, anti-angiogenic, tyrosine kinase inhibitor, response biomarker, clinical progress
Citation: Xu D, Luo Y, Wang P, Li J, Ma L, Huang J, Zhang H, Yang X, Li L, Zheng Y, Fang G and Yan P (2023) Clinical progress of anti-angiogenic targeted therapy and combination therapy for gastric cancer. Front. Oncol. 13:1148131. doi: 10.3389/fonc.2023.1148131
Received: 19 January 2023; Accepted: 12 May 2023;
Published: 12 June 2023.
Edited by:
Zequn Li, The Affiliated Hospital of Qingdao University, ChinaReviewed by:
Xiaobo Du, Mianyang Central Hospital, ChinaSutapa Mukherjee, Chittaranjan National Cancer Institute (CNCI), India
Copyright © 2023 Xu, Luo, Wang, Li, Ma, Huang, Zhang, Yang, Li, Zheng, Fang and Yan. This is an open-access article distributed under the terms of the Creative Commons Attribution License (CC BY). The use, distribution or reproduction in other forums is permitted, provided the original author(s) and the copyright owner(s) are credited and that the original publication in this journal is cited, in accordance with accepted academic practice. No use, distribution or reproduction is permitted which does not comply with these terms.
*Correspondence: Gang Fang, Zmdsenl6bm5AZ3h0Y211LmVkdS5jbg==; Peiyu Yan, cHl5YW5AbXVzdC5lZHUubW8=
†These authors have contributed equally to this work