- 1Department of General Surgery, Qilu Hospital, Shandong University, Jinan, China
- 2Department of General Surgery, Shanxian Central Hospital, Heze, China
- 3Department of General Surgery, Qilu Hospital (Qingdao), Shandong University, Jinan, China
Cholangiocarcinoma (CCA) is a highly malignant tumor of the hepatobiliary system that has failed to respond to many traditional therapies to a certain extent, including surgery, chemotherapy and radiotherapy. In recent years, the new therapeutic schemes based on immunology have fundamentally changed the systemic treatment of various malignant tumors to a certain extent. In view of the immunogenicity of CCA, during the occurrence and development of CCA, some immunosuppressive substances are released from cells and immunosuppressive microenvironment is formed to promote the escape immune response of its own cells, thus enhancing the malignancy of the tumor and reducing the sensitivity of the tumor to drugs. Some immunotherapy regimens for cholangiocarcinoma have produced good clinical effects. Immunotherapy has more precise characteristics and less adverse reactions compared with traditional treatment approaches. However, due to the unique immune characteristics of CCA, some patients with CCA may not benefit in the long term or not benefit at all after current immunotherapy. At present, the immunotherapy of CCA that have been clinically studied mainly include molecular therapy and cell therapy. In this article, we generalized and summarized the current status of immunotherapy strategies including molecular therapy and cell therapy in CCA in clinical studies, and we outlined our understanding of how to enhance the clinical application of these immunotherapy strategies.
1 Introduction
Cholangiocarcinoma (CCA) is a highly malignant tumor of the digestive system with a poor prognosis that poses a significant therapeutic challenge (1). Based on their anatomical distribution, they are classified as intrahepatic (iCCA), perihilar (pCCA), or distal (dCCA) (2). Treatment outcomes are extremely poor due to early local invasion, susceptibility to multiple metastases, poor effectiveness of most treatment options, and frequent drug resistance (3, 4). Moreover, due to the lack of early specific molecular markers to detect cholangiocarcinoma, most cholangiocarcinoma is already at an advanced stage when detected (5, 6). Despite the efforts of many studies, clinical transformation remains stagnant, making the treatment of cholangiocarcinoma more difficult. Surgical resection is the only potentially curative treatment option for CCA. However, only approximately 15% of patients with iCCA have a removable stage at initial diagnosis, and the postoperative recurrence rate is more than 60% (7, 8). Systemic chemotherapy is one of the recognized first-line treatments for patients with metastatic and advanced cholangiocarcinoma, but the prognosis after this treatment remains poor, with 5-year survival rates below 40% (9, 10). Radiotherapy as adjuvant or palliative treatment has a limited impact on the survival of patients with cholangiocarcinoma (11–13). Currently, only a minority of CCA patients have the opportunity to undergo radical resection. Nonetheless, median survival beyond 30 months is challenging even with radical resection (14). Especially for patients with advanced CCA, there are few effective treatments that can improve clinical outcomes. Therefore, there is an urgent need to develop more effective treatment options to improve the clinical prognosis of CCA patients, especially those with advanced CCA.
Over the past decade, tumor immunotherapy has emerged as an emerging treatment for various cancers, including melanoma, urothelial tumors, non-small cell lung cancer, and liver cancer (15). Tumor immunotherapy refers to using immunological principles and methods to activate immune cells in vivo and enhance the body’s anti-tumor immunity, so as to specifically remove minimal residual tumor lesions, slow down tumor growth, and alleviate immune tolerance (16). In other words, the goal of cancer immunotherapy is to break the mechanism of cancer immune escape, thereby reawakening immune cells and destroying tumor cells. Due to its minimal side effects and excellent therapeutic effect, it is gradually becoming the future development direction of tumor treatment, and is known as the fourth most important cancer treatment method after surgery, radiotherapy and chemotherapy (17–19). In a word, from the perspective that CCA is an immunogenic cancer, immunotherapy will provide a promising treatment strategy to improve the survival benefit of patients with CCA.
2 Background on immunotherapy for cholangiocarcinoma
Cholangiocarcinoma is an immunogenic tumor (16). It is characterized by fewer tumor parenchyma cells and more tumor interstitial cells compared with other solid tumors (20). It has a microenvironment controlled by inflammation and rich immune cells, mainly composed of T and B lymphoid cells, macrophages, neutrophils, natural killer cells, and other subsets of immune cells (21). On the one hand, in the innate immune system of cholangiocarcinoma, macrophages, as the first line of defense, have strong heterogeneity and play a crucial role in CCA (22, 23). Macrophage infiltration is closely related to angiogenesis and increased regulatory T cell (Treg) infiltration (24). Dendritic cells (DCs) belong to antigen presenting cells (APCs), which are the sentinels of the human immune system. They can keenly capture the small difference between tumor cells and normal cells, and transmit this difference to the armed police T-lymphocytes in the human immune system, which is crucial in activating the adaptive immune response (25). On the other hand, in adaptive immune response of cholangiocarcinoma, tumor infiltrating lymphocytes (TIL) are a highly heterogeneous population, including CD8 + T cells, CD4 +T cells, B cells and Treg (26, 27). They play an important role in CCA immune surveillance and elimination of tumor cells. CD8 + T cells is one of the main effector cells of tumor immune adoptive therapy. They bind to target cells through the receptor TCR on their membranes, releasing various lysosomes, thus promoting the dissolution and death of target cells (28).
The immune microenvironment in CCA could be characterized by excessive secretion of cytokines and chronic persistent inflammatory infiltration, which could induce the proliferation of tumor bile duct cells (29, 30). The high-density infiltration of tumor associated macrophages may play a role in supporting the metastasis and degradation of extracellular matrix (31). This helps CCA cells escape from immune system (32). Therefore, this immunological characteristic of cholangiocarcinoma creates an unfavorable element for the host’s adaptive immune response to CCA. Our better understanding of the immune microenvironment of cholangiocarcinoma is helpful to improve the prognosis of CCA patients through immunotherapy.
3 Molecular therapy
The main principle of molecular therapy is to inject specific molecular agents into the patient’s body to regulate the body’s specific immune response. The main agent used is immune checkpoint inhibitors (ICIs). The related content of ICIs is an attractive issue at the territory of cancer treatment, which has made vast progress in the treatment of cancers originated from different organizations and organs (33). ICIs mainly include (34): (1) Programmed cell death protein 1 (PD-1) inhibitors. It is represented by pembrolizumab and nivolumab. (2) PD-L1 inhibitors. It is represented by durvalumab, avelumab and atezolizumab. (3) Cytotoxic T lymphocyte-associated antigen 4 (CTLA-4) inhibitors. It is represented by tremelimumab and ipilimumab. CCA cells can use these inhibitors to limit or evade antitumor immune responses, including by affecting the synthesis of immune checkpoint associated proteins (35). At present, numerous clinical studies of ICIs related to iCCA, pCCA and dCCA are performing. Several results of clinical trials and cases have shown that patients with certain genotypes of CCA may benefit from treatment with ICIs.
3.1 PD-1 and PD-L1 inhibitors
PD-1 is a membrane-spanning protein located on the surface of T lymphocytes that eliminates antitumor immune reactions and facilitates cancer immune evade from cytotoxic T cells in the course of carcinogenesis, while PD-L1 is a PD-1 ligand molecule expressed by tumor cells. PD-1 is related to immune responses and the immune microenvironment of cholangiocarcinoma (36). In Tumor Microenvironment (TME), PD-1 and PD-L1 molecules combine to induce T lymphocyte failure, leading to tumor immunosuppression. Hence, blocking the PD-1/PD-L1 signal axis by inhibiting the combination of them can gradually improve the adaptive immune system, reverse the phenomenon of immune suppression, and restore the response of cancer cells to T cells. Using monoclonal antibodies to cut off the PD-1/PD-L1 pathway has shown a good effect with long-lasting responses and prolonged survival in patients with some cancer types including CCA. What’s more, these reactions usually last for many years or never disappear and do not cause severe toxicity for vast majority of patients (15). Based on this, we believe that it is a potential and promising therapeutic strategy for CCA.
In fact, the response of patients with different molecular types of tumors to immunotherapy is inconsistent. At present, it is believed that PD-L1, MMR, MSI, and high TMB positive of some tumor patients may have a good response to immunotherapy. This is because a high level of mutation‐associated neoantigens can be distinguished by immune cells (37). At the same time, the study found that, with the continuous increase of TMB, the progression free survival (PFS) and objective response rate of CCA patients treated with ICIs may increase (38). There are some case reports have showed that patients with irresectable or recurrent metastatic biliary tumor have achieved partial remission (PR) or complete remission (CR) through ICIs treatment. Therefore, these successful individualized treatment schemes provided important guidelines for the management of patients with last-stage cholangiocarcinoma. High expression of immune checkpoints in CCA patients is related to poor prognosis, especially with short relapse free survival and overall survival. Due to the immune escape mechanism, the high expression of PD-L1 may be related to the rapid progress and poor prognosis of the tumor. Meta-analysis of eleven researches about cholangiocarcinoma and PD-1 found that the expression of PD-L1 in cholangiocarcinoma cancer cells went together with TNM staging. Overexpression of PD-L1 predicted poor overall survival rate (OS), and overexpression of PD-L1 also predicted short disease-free survival (DFS) period (39). In addition, similar conclusions have been found in other studies that high lymphatic metastasis, TNM stage and poor prognosis are closely related to PD-L1 positive (40, 41). Another study demonstrated by section staining that a high count of CD8+T cells in tumor parenchyma and stroma resulted in worse OS and DFS, and a high expression of PD-L1 resulted in worse overall survival (OS). Immunosuppression of PD-L1 may be a potential treatment for CCA patients who are unable to undergo surgery (42).
One study showed that PD-L1 is mainly expressed by inflammatory cells in tumors with dense lymphocyte infiltration. Their results suggested that CCA patients who have dense intratumoral lymphocytic infiltration may be a suitable treatment character for PD-L1/PD-1 inhibitors (43). Else, a study showed tumors could create their own tumor microenvironment to enhance PD-L1 expression, and then relying on this pathway to achieve immune escape in CCA with dense lymphocytic infiltrates (44). Driven by these studies, single-inhibitor drugs have gradually entered clinical use, and two of the most representative drugs in CCA are: pembrolizumab and Nivolumab (Opdivo). In 2017, the FDA recommended the utilization of pembrolizumab to treat cancers including cholangiocarcinoma (45). National Comprehensive Cancer Network (NCCN) guidelines recommended the use of pembrolizumab as one of the treatment options for tumors (including biliary malignancies) with defective microsatellite instability (MSI) or mismatch repair (MMR) characteristics. A study of defective mismatch repair cancers treated with pembrolizumab in cholangiocarcinoma patients has showed an excellent objective response rate (ORR=40.9%) (46). Nivolumab is an immune checkpoint inhibitor that essentially exploits its PD-1 -binding properties to IgG4 immunoglobulin. In a small sample size study of cholangiocarcinoma tumor samples, it was found that nivolumab expression could be detected at different levels in tumor microenvironment, both in tumor cells and immune cells (35). And it has been demonstrated that PD-1 expression increased in CCA patients with CD4+ and CD8+ tumor‐infiltrating lymphocytes (TILs), and the level of effector cytokines in TILs was positively correlated with nivolumab (47). One phase II trial showed that TILs could play a significant role in adjusting the interaction between ICI and tumor microenvironment (45). The research also showed that this inhibitor with radiotherapy or chemotherapy in CCA treatment have been examined and shown a good therapeutic prospect (45). These data showed that nivolumab might provide a novel therapeutic choice for cholangiocarcinoma patients.
Certainly, with more and more studies, we have found that ICIs combination therapy is better than ICIs monotherapy. Clinical trials of anti-PD-1/PD-L1 medicine combined with other therapies for CCA patients are currently in full progress at the moment. The study found that nivolumab is superior to single drug immunotherapy when used in combination with CTLA-4 inhibitor ipilimumab (41). In addition, in the model of CCA subcutaneous tumor, the combined use of verteporfin and anti-PD-1antibodies down regulated the tumor burden compared with the use of two medicines alone (48). Another study showed that anti-PD-1 immunotherapy combined with radiotherapy is an up-and-coming one. In this study, Liu et al. presented a 68-years-old iCCA patient in advanced stage who is not suitable for chemotherapy, after six cycles of ICIs therapy, the primary tumor narrowed but new lung and lymph gland metastasis were found, which indicated a mixed response. Radiotherapy was then started, concomitant with continuing ICIs immunotherapy. The combination treatment ultimately led to complete remission of primary tumors and all metastatic tumors, and almost no treatment related adverse events occurred (49). Besides, Zhang et al. reported that the first patient with last-stage intrahepatic cholangiocarcinoma with PD-L1 positive and high tumor mutation load was successfully eradicated after using tyrosine kinase inhibitors (TKIs) combined with ICIs immunotherapy to shrink the tumor. In this case, a thirty-eight years old young woman was diagnosed with stage IV iCCA. The test report of this patient indicated that the tumor mutation load was high and the expression of PD-L1 was positive. After receiving seven cycles of PD-1 inhibitor combined with TKIs therapies, the patient underwent radical operation, and it is noteworthy that her postoperative pathological type is well (50). A case report showed a patient with metastatic iCCA who was not sensitive to first-line chemotherapy therefore was included in the phase I study of sintilimab therapy. In addition, the tumor mutation load in this patient was low and with microsatellite instability. The patient was completely relieved after three courses of treatment. Therefore, the author believed that PD-1 inhibitor combination therapy may be a feasible treatment for patients with advanced cholangiocarcinoma who are not sensitive to chemotherapy (51). Zhao reported four cases of intractable advanced CCA patients who managed to control the tumor with anti-PD-1 antibodies together with SBRT (52). A study conducted in patients with unresectable iCCA showed that the efficacy and safety of HAIC (n=58) or TACE (n=39) combined with ICIs immunotherapy or TKIs were more positive than that of HAIC and TACE alone (53). Li et al. found that PD-1 inhibitor combined with S-1 and nab paclitaxel can be used to achieve the transformation treatment of advanced refractory CCA. This combination therapy is safe and effective, and can significantly prolong the survival of patients (54). Previous evidence suggested that PD-1 inhibitors are suitable for patients who have high TMB, high microsatellite instability (MSI-H), deficient mismatch repair (dMMR), and PD-L1 positive expression. But a new study came to a different conclusion. It found that SBRT combined with PD-1 inhibitor can effectively treat patients who have low TMB, MSI, pMMR and PD-L1 expression negative in advanced or recurrent CCA. This finding is of great significance, because it may expand the indications of combined therapy, so that those patients who were not previously suitable for immunotherapy can benefit from immunotherapy (34). Integrating these findings, we believe that PD-1 and PD-L1 inhibitors have a significant role in the treatment of cholangiocarcinoma, and the combination therapy may be one of the important options for patients with advanced CCA.
Of course, the PD-1/PD-L1 treatment of cholangiocarcinoma is extremely complex, and there are also some other studies on this aspect. Because PD-L1 in CCA is predominantly derived from tumor-associated macrophages (TAMs), PD-1 blockade can be enhanced by targeting tumor-associated macrophages and granulocyte-myeloid-derived suppressor cells (55). One study has found that PD-L1 is related to p-ERK, and the down-regulation of KRAS can down-regulate the expression of PD-L1 through this pathway in CCA (56). Based on this, relevant therapeutic strategies can be developed to influence the responsiveness of CCA to ICIs. Else, the study found that stimulation of some antigen-presenting cells (dendritic cells and macrophages) with CD40 agonists can enhance the ICIs effect in CCA (57). In addition, a very important regulator, CMTM4, was identified in CCA patients, which can positively regulate PD-L1 and stabilize PD-L1 by affecting the post-translational biological manifestations (58).
3.2 CTLA4 inhibitors
Another important aspect of ICIs treatment is CTLA4 inhibitor. CTLA-4 molecules can be highly expressed on the surface of activated T cells such as Tregs, and interact with its ligands to produce signals that affect the activation and proliferation of T cells (59–62). The CTLA-4 mono-cloning antibody ipilimumab is the first immunosuppressant approved for marketing by the US FDA. One study has found that ipilimumab could cooperate with Nivolumab to significantly improve the clinical outcome of patients with intrahepatic CCA (63). Furthermore, some research teams have conducted two clinical trials to investigate the efficacy of CTLA-4 monoclonal antibody tremelimumab and PD-1 monoclonal antibody durvalumab combined with first-line chemotherapy or TACE to cure advanced unresectable biliary system malignant tumors (41). Of course, these studies are ongoing, and we expect the results will bring good news for the treatment of cholangiocarcinoma.
Researchers have done a lot of work in clinical research related to cholangiocarcinoma immune checkpoint, and we summarized it (Table 1). Certainly, it is generally believed that PD1/PD-L1 monoclonal antibody has stronger anti-tumor effect than CTLA-4 monoclonal antibody. Compared with CTLA-4, PD-1/PD-L1 inhibitors produce less toxic and side effects on human body and exhibit better overall efficacy. The phase III head-to-head trial has found that the effectiveness of PD-1 pathway blocking (pembrolizumab, 33%-34%) is better than that of CTLA-4 blocking (ipilimumab, 12%). At the same time, the survival rate (1 year) of PD-1 treatment was higher than that of CTLA-4 (71, 72). Therefore, we believed that PD1/PD-L1 will become an important branch of tumor treatment in the following years.
4 Cell therapy
Cell therapy of cholangiocarcinoma is mainly to activate the specific or non-specific immune function of the body through cell transplantation or cell vaccine, enhance the body’s immunity, so as to improve the survival of patients with CCA.
4.1 Treatment with cell transplantation
Main methods of cell transplantation, such as adoptive immune response cell therapy (ACT) is to isolate cells with good immune activity from cancer patients, perform proliferation and functional verification in vitro, and then transplant them into patients, so as to increase the quantity of immune cells against tumor. At present, there are three main methods for this scheme: TIL therapy, chimeric antigen receptor T cell technology (CAR-T) and T cell receptor chimeric T cells (TCR-T) (73). TIL cell therapy refers to the treatment of isolating tumor infiltrating lymphocytes from tumor tissue, which is also a therapy of reinfusion to patients after in vitro culture and expansion. The effector cells of TIL therapy are the population with a high proportion of tumor specific T cells and rich diversity, so it has the advantages of multiple targets, strong tumor tendency and invasion, and small side effects (74). TCR-T therapy is a therapeutic method using antigen specific TCR transduced T cells, while CAR-T therapy is a method using CAR gene to apply T cells (75). TCR-T and CAR-T have received extensive attention and research because they can target and distinguish specific cancer cells by expressing unique receptors. And the birth of the two therapies can remove the restriction on whether more high-quality antigen reactive T cells can be obtained from tumor tissues of CCA patients (76).
The success of ACT in treating CCA has been reported in some case studies, but its exact efficacy still needs to be further proved by large sample trials (77, 78). Presently, the reliable evidence for treating CCA patients with ACT is confined to some case reports and occasional series of cases enrolled in a single arm phase II clinical study (79). In the year of 2006, A case report first proposed the clinical effectiveness of a CCA patient who received adoptive cell therapy. In this study, an iCCA patient with lymph node metastasis acquired curative excision and was transplanted with subsidiary CD3-activated T cells added cancer peptide- or lysate-pulsed dendritic cells. After the treatment, the patient has survived for more than 3.5 years (77). In 2014, Rosenberg’s team from the National Institute of Health (NIH) covered an example of a metastatic cholangiocarcinoma. The patient received TIL infusion which was co-cultured with antigen-presenting cells (APCs) transplanted with non-reproductive cell non-synonymous mutations found in the cancer, leading to cancer extinction for more than half a year (78). Some studies on the prognostic significance of TIL treatment for CCA patients show that patients with large number and high density of CD8+T cells show better OS or DFS (80). The study found that the large number of CD8+T cells at the tumor margin was related to OS prolongation. Similarly, higher CD4+T cell density at tumor margin is associated with better OS or DFS, and the distribution of CD8+T cells within and around the tumor also affects the prognosis of CCA patients (74, 78).
Another phase I clinical trial (NCT01869166) showed that CAR-T cell therapy was safe in people with advanced, unresectable biliary cancer. Among 17 evaluable patients, 1 patient achieved complete remission and 10 patients were in stable condition (81). One case reported a CCA patient with mediastinal lymph node metastasis in stage IV, who received Vγ9Vδ2 T cell immunotherapy, the immune function was improved and TNM stage decreased (82). Besides, one phase II clinical trial reported that a CCA patient who participated in the adoptive cell therapy experiment and transfused mutation-specific CD4+ T cells after chemotherapy failure, and the tumor achieved partial remission 7 months later (78). In addition, some clinical studies on ACT are in progress, such as clinical trial of security and effectiveness of the CAR-T to treat intrahepatic cholangiocarcinoma (NCT03633773). The fourth generation CAR-T cells have been confirmed in the tissue model in vitro by targeting CD133 (83). Since more than 50% of cholangiocarcinoma express CD133, the fourth-generation CAR-T cell therapy targeting this target is of great significance for patients with CCA (83).
There are also some early cell therapies, such as lymphokine activated killer cell (LAK) therapy and cytokine induced killer cell (CIK) therapy. LAK treatment works by stimulating the immunocompetent cells of peripheral blood lymphocytes with interleukin-2 (IL-2). That is a mixture of lymphocytes, including T lymphocytes and NK cells. In vitro, LAK cells have antigen-independent killing effect on tumor cells. They could kill tumor cells not only by recognizing the surface structure of target cells, but also by secreting cytokines. The mechanism of this target killing is very similar to that of NK cells. This therapeutic effect has been well demonstrated in a variety of malignancies (84). Compared with LAK cells, CIK cells have many advantages: faster proliferation rate, wider tumor killing spectrum, higher tumor killing activity, sensitive to multi drug-resistant cancer cells, less toxic to normal bone marrow hematopoietic precursor cells, can resist the apoptosis of effector cells caused by cancer cells (84). Due to CIK cells originate from the venous blood of sick patients or fitness people, it is relatively easy to culture and expand. At present, CIK cells are widely used in adjuvant treatment of tumors (85). According to this, a great number of clinical experiments were carried out to treat lots of tumors, such as kidney cancer Hodgkin’s lymphoma, leukemia and liver cancer. However, there are few studies on the clinical application of CIK cells in CCA. In one study, by using CCA transplantation of SCID mouse models researchers have found that human CIK cells, composed of CD3+T cells and CD3+/CD56+T cells, can reduce the cell growth (86). In addition, for CCA cells, CIK cells which expressing inducible co-stimulator had the cytotoxic effect (87).
4.2 Treatment with vaccines
In addition to cell transplantation, there are also tumor vaccines in the cell therapy of cholangiocarcinoma. Tumor vaccine is extracted from allogeneic or autologous cancer cells, and its components include tumor associated antigen (TAA) or tumor specific antigen (TSA). Its role is to stimulate acquired immune response to attack tumor cells, eliminate the immunosuppression condition result from tumor products, enhance the vaccine potential of TAA, and increase the autoimmunity to attack tumors. It can generally be grouped in four broad categories (tumor cell vaccine, gene vaccine, polypeptide vaccine and dendritic cell (DC) vaccine) by the production source of cancer vaccine.
In a retrospective study, 65 advanced CCA patients received DC vaccine treatment at the same time, 15% of them were relatively stable after 6 months of treatment and had good tolerance to the vaccine (88). A recent study also found that DC vaccine combined with chemotherapy can improve the prognosis of CCA patients more than DC vaccine alone (89). One study has found some distinct antigens, such as FCGR1A, TRRAP and CD247, which can be used to manufacture anti-cholangiocarcinoma mRNA vaccine (90). Other studies have found that two antigens that CCA is more sensitive to are mucin protein 1 (MUC1) and wilm’s tumor protein 1 (WT1) (91, 92). A phase I study result of MUC1 vaccine in the patients with biliary cancer also showed that the vaccine was safe (93). A clinical research using WT1 peptide vaccine combined with gemcitabine in the treatment of biliary cancer included 8 patients with advanced CCA, and found that the tumor control rate of biliary cancer was close to 50% (94). In some case reports and prior clinical experiments, vaccines originated from peptide and DC vaccines have shown positive clinical results (95). In a phase 1 study, nine patients with unresectable BTC were treated with vaccines from four polypeptides, including LY6K, DEPDC1, IMP3, and TTK protein kinase, and the 4 peptides were shown to be extremely effective. What’s more, DEPDC1 and LY6K are very promising candidates for causing an obvious cytotoxic lymphocyte (CTL) reaction, and thereby improving the PFS and OS (96). In one research, thirty-six iCCA patients were injected with autologous tumor lysate added DC vaccine, and activated T cells were transferred at the same time. The OS and median PFS of patients receiving adjuvant treatment with tumor vaccine were 31.9 months and 18.3 months respectively, which were significantly improved compared with 17.4 months and 7.7 months of patients receiving surgery alone (97). DC vaccine would be an acceptable and excellent treatment to prevent postoperative recurrence and improve survival rate of CCA patients.
However, according to the current research conclusion, for most tumors, including cholangiocarcinoma, the single therapy with cancer vaccine usually cannot achieve good clinical effect. Because cancer has multiple immune escape mechanisms, the efficacy of single cancer vaccine therapy is relatively low, and any cancer vaccine alone cannot achieve the expected efficacy. In addition, it is often difficult to evaluate the clinical efficacy of cancer vaccines in patients with cholangiocarcinoma, because the immune status of these patients is often seriously affected by their exposure to other treatments such as surgery, radiotherapy and chemotherapy. The difficulty in evaluating the efficacy further hinders the development of new cancer vaccine drugs (76). The impact of tumor vaccine on CCA patients needs further research and verification. But it is believed that tumor vaccine can be considered as adjuvant therapy for advanced CCA.
5 Summary
Immune checkpoint monoclonal antibody plays the role anti-carcinoma by strengthening the now available immune system, but to some extent, it cannot promote immunocytes to target tumors. Cancer vaccine eliminates tumor cells by stimulating unique immune response, but the curative effect is not excellent. However, ACT can directly kill tumors or stimulate the immune response of the body to kill tumor cells, but this research direction is still in the initial stage. The specificity and targeting of the therapy are the research focus at present and the advanced direction in the future. As a matter of fact, we deeply think that researches are needed to assess the clinical application of cell therapy for the treatment of CCA. There is still a long way to go to treat cholangiocarcinoma with this method.
The treatment of cholangiocarcinoma is complex and difficult, immunotherapy provides us with a new vision in this sphere. In this article, we summarized four immunotherapeutic strategies related to cholangiocarcinoma (Table 2, Figure 1). We hope that the existing and ongoing studies can be used as a reference for the immunotherapy of cholangiocarcinoma to further enhance the efficacy of immunotherapy and mitigate adverse effects during treatment. However, according to the conclusions of various studies we have obtained, the immunotherapy methods for CCA have their own advantages and disadvantages, and not one scheme can solve a large class of problems. In addition, we still need to note that compared with other tumors, CCA is highly heterogeneous, and the application of immunotherapy in patients with CCA varies significantly from individual to individual. Moreover, there is currently a lack of very specific targets for immunotherapy of CCA, which leads to the slow progress compared with other diseases. Of course, researchers have also made many attempts in these areas, especially immunotherapy combined with targeted therapy and chemotherapy, or local treatment of CCA, which may open up new options and further expand the treatment scope of CCA. We believe that, in the future, with a deeper understanding of the immunotherapy model for cholangiocarcinoma, these immunotherapy methods and immune combination therapies will provide new ideas for the treatment and management of cholangiocarcinoma.
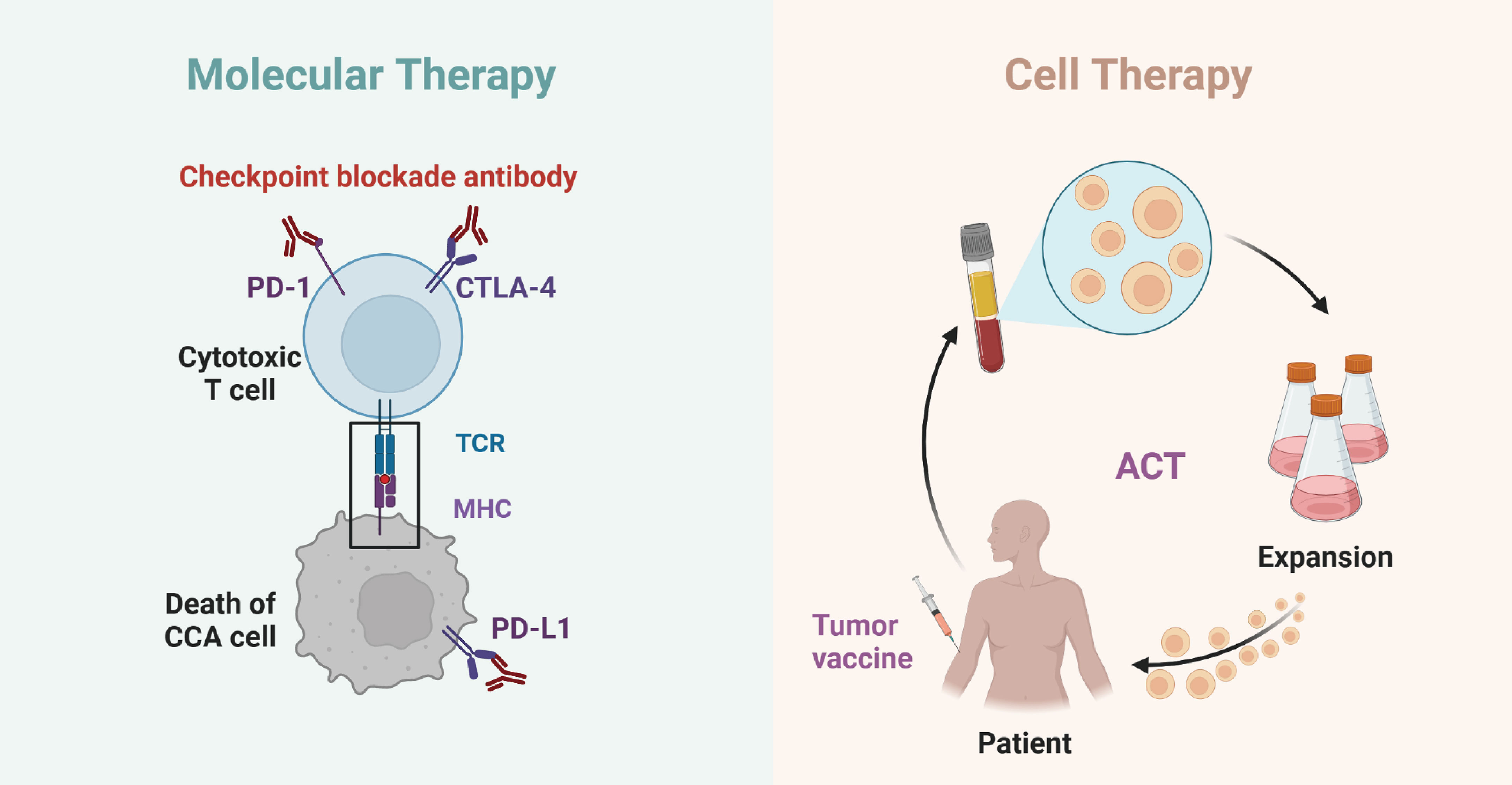
Figure 1 Schematics of molecular and cell therapy in cholangiocarcinoma. The interaction of PD-1/PD-L1 and CTLA4 with cholangiocarcinoma immunotherapy (left); The interaction of tumor vaccine and ACT with cholangiocarcinoma immunotherapy (right).
Author contributions
L-MZ and A-DS sort out the references. L-MZ, A-DS and Y-CT wrote the manuscript. YY, Z-LL, X-QH and LZ-S read the article and offered some modification comments. Y-CT and Z-LZ devoted to supervising the work and improved the original manuscript. All authors contributed to the article and approved the submitted version.
Funding
Shandong Province Key R&D Program (Major Scientific Innovation Projects,2021CXGC011105), Key Research and Development Program of Shandong Province (Grant No. 2019GSF108254), Clinical Research Foundation of Shandong University (Grant No. 2020SDUCRCA018), Shandong Province Natural Science Foundation (Grant No. ZR2021QH079), the National Natural Science Foundation of China (Grant No. 82172791).
Conflict of interest
The authors declare that the research was conducted in the absence of any commercial or financial relationships that could be construed as a potential conflict of interest.
Publisher’s note
All claims expressed in this article are solely those of the authors and do not necessarily represent those of their affiliated organizations, or those of the publisher, the editors and the reviewers. Any product that may be evaluated in this article, or claim that may be made by its manufacturer, is not guaranteed or endorsed by the publisher.
References
1. Rizvi S, Khan SA, Hallemeier CL, Kelley RK, Gores GJ. Cholangiocarcinoma - evolving concepts and therapeutic strategies. Nat Rev Clin Oncol (2018) 15(2):95–111. doi: 10.1038/nrclinonc.2017.157
2. Liu J, Ren G, Li K, Liu Z, Wang Y, Chen T, et al. The Smad4-Myo18a-Pp1a complex regulates β-catenin phosphorylation and pemigatinib resistance by inhibiting Pak1 in cholangiocarcinoma. Cell Death differentiation (2022) 29(4):818–31. doi: 10.1038/s41418-021-00897-7
3. Banales JM, Cardinale V, Carpino G, Marzioni M, Andersen JB, Invernizzi P, et al. Expert consensus document: Cholangiocarcinoma: Current knowledge and future perspectives consensus statement from the European network for the study of cholangiocarcinoma (Ens-cca). Nat Rev Gastroenterol Hepatol (2016) 13(5):261–80. doi: 10.1038/nrgastro.2016.51
4. Valle JW, Kelley RK, Nervi B, Oh DY, Zhu AX. Biliary tract cancer. Lancet (London England) (2021) 397(10272):428–44. doi: 10.1016/s0140-6736(21)00153-7
5. Li Z, Liu J, Chen T, Sun R, Liu Z, Qiu B, et al. Hmga1-Trip13 axis promotes stemness and epithelial mesenchymal transition of perihilar cholangiocarcinoma in a positive feedback loop dependent on c-myc. J Exp Clin Cancer Res CR (2021) 40(1):86. doi: 10.1186/s13046-021-01890-1
6. Xu YF, Liu ZL, Pan C, Yang XQ, Ning SL, Liu HD, et al. Hmgb1 correlates with angiogenesis and poor prognosis of perihilar cholangiocarcinoma Via elevating Vegfr2 of vessel endothelium. Oncogene (2019) 38(6):868–80. doi: 10.1038/s41388-018-0485-8
7. Bridgewater J, Galle PR, Khan SA, Llovet JM, Park JW, Patel T, et al. Guidelines for the diagnosis and management of intrahepatic cholangiocarcinoma. J Hepatol (2014) 60(6):1268–89. doi: 10.1016/j.jhep.2014.01.021
8. Endo I, Gonen M, Yopp AC, Dalal KM, Zhou Q, Klimstra D, et al. Intrahepatic cholangiocarcinoma: Rising frequency, improved survival, and determinants of outcome after resection. Ann Surg (2008) 248(1):84–96. doi: 10.1097/SLA.0b013e318176c4d3
9. Kelley RK, Bridgewater J, Gores GJ, Zhu AX. Systemic therapies for intrahepatic cholangiocarcinoma. J Hepatol (2020) 72(2):353–63. doi: 10.1016/j.jhep.2019.10.009
10. Wang ML, Ke ZY, Yin S, Liu CH, Huang Q. The effect of adjuvant chemotherapy in resectable cholangiocarcinoma: A meta-analysis and systematic review. Hepatobil pancreatic Dis Int HBPD Int (2019) 18(2):110–6. doi: 10.1016/j.hbpd.2018.11.001
11. Zeng ZC, Tang ZY, Fan J, Zhou J, Qin LX, Ye SL, et al. Consideration of the role of radiotherapy for unresectable intrahepatic cholangiocarcinoma: A retrospective analysis of 75 patients. Cancer J (Sudbury Mass) (2006) 12(2):113–22.
12. Nantajit D, Trirussapanich P, Rojwatkarnjana S, Soonklang K, Pattaranutraporn P, Laebua K, et al. Clinical analysis of cholangiocarcinoma patients receiving adjuvant radiotherapy. Mol Clin Oncol (2016) 5(6):797–802. doi: 10.3892/mco.2016.1072
13. Leng KM, Liu YP, Wang ZD, Zhong XY, Liao GQ, Kang PC, et al. Results of adjuvant radiation therapy for locoregional perihilar cholangiocarcinoma after curative intent resection. OncoTargets Ther (2017) 10:2257–66. doi: 10.2147/ott.s131873
14. Sirica AE, Gores GJ, Groopman JD, Selaru FM, Strazzabosco M, Wei Wang X, et al. Intrahepatic cholangiocarcinoma: Continuing challenges and translational advances. Hepatol (Baltimore Md) (2019) 69(4):1803–15. doi: 10.1002/hep.30289
15. Yuan M, Zhu Z, Mao W, Wang H, Qian H, Wu J, et al. Anlotinib combined with anti-Pd-1 antibodies therapy in patients with advanced refractory solid tumors: A single-center, observational, prospective study. Front Oncol (2021) 11:683502. doi: 10.3389/fonc.2021.683502
16. Menon S, Shin S, Dy G. Advances in cancer immunotherapy in solid tumors. Cancers (2016) 8(12):106. doi: 10.3390/cancers8120106
17. Waldman AD, Fritz JM, Lenardo MJ. A guide to cancer immunotherapy: From T cell basic science to clinical practice. Nat Rev Immunol (2020) 20(11):651–68. doi: 10.1038/s41577-020-0306-5
18. Hegde PS, Chen DS. Top 10 challenges in cancer immunotherapy. Immunity (2020) 52(1):17–35. doi: 10.1016/j.immuni.2019.12.011
19. Esfahani K, Roudaia L, Buhlaiga N, Del Rincon SV, Papneja N, Miller WH Jr. A review of cancer immunotherapy: From the past, to the present, to the future. Curr Oncol (Toronto Ont) (2020) 27(Suppl 2):S87–s97. doi: 10.3747/co.27.5223
20. Sirica AE, Gores GJ. Desmoplastic stroma and cholangiocarcinoma: Clinical implications and therapeutic targeting. Hepatol (Baltimore Md) (2014) 59(6):2397–402. doi: 10.1002/hep.26762
21. Rimassa L, Personeni N, Aghemo A, Lleo A. The immune milieu of cholangiocarcinoma: From molecular pathogenesis to precision medicine. J Autoimmun (2019) 100:17–26. doi: 10.1016/j.jaut.2019.03.007
22. Krenkel O, Tacke F. Liver macrophages in tissue homeostasis and disease. Nat Rev Immunol (2017) 17(5):306–21. doi: 10.1038/nri.2017.11
23. Tacke F, Zimmermann HW. Macrophage heterogeneity in liver injury and fibrosis. J Hepatol (2014) 60(5):1090–6. doi: 10.1016/j.jhep.2013.12.025
24. Hasita H, Komohara Y, Okabe H, Masuda T, Ohnishi K, Lei XF, et al. Significance of alternatively activated macrophages in patients with intrahepatic cholangiocarcinoma. Cancer Sci (2010) 101(8):1913–9. doi: 10.1111/j.1349-7006.2010.01614.x
25. Jonuleit H, Schmitt E, Schuler G, Knop J, Enk AH. Induction of interleukin 10-producing, nonproliferating Cd4(+) T cells with regulatory properties by repetitive stimulation with allogeneic immature human dendritic cells. J Exp Med (2000) 192(9):1213–22. doi: 10.1084/jem.192.9.1213
26. Naito Y, Saito K, Shiiba K, Ohuchi A, Saigenji K, Nagura H, et al. Cd8+ T cells infiltrated within cancer cell nests as a prognostic factor in human colorectal cancer. Cancer Res (1998) 58(16):3491–4.
27. Yasunaga M, Tabira Y, Nakano K, Iida S, Ichimaru N, Nagamoto N, et al. Accelerated growth signals and low tumor-infiltrating lymphocyte levels predict poor outcome in T4 esophageal squamous cell carcinoma. Ann Thorac Surg (2000) 70(5):1634–40. doi: 10.1016/s0003-4975(00)01915-9
28. Reina-Campos M, Scharping NE, Goldrath AW. Cd8(+) T cell metabolism in infection and cancer. Nat Rev Immunol (2021) 21(11):718–38. doi: 10.1038/s41577-021-00537-8
29. Isomoto H, Mott JL, Kobayashi S, Werneburg NW, Bronk SF, Haan S, et al. Sustained il-6/Stat-3 signaling in cholangiocarcinoma cells due to socs-3 epigenetic silencing. Gastroenterology (2007) 132(1):384–96. doi: 10.1053/j.gastro.2006.10.037
30. Park J, Tadlock L, Gores GJ, Patel T. Inhibition of interleukin 6-mediated mitogen-activated protein kinase activation attenuates growth of a cholangiocarcinoma cell line. Hepatol (Baltimore Md) (1999) 30(5):1128–33. doi: 10.1002/hep.510300522
31. Xiang X, Wang J, Lu D, Xu X. Targeting tumor-associated macrophages to synergize tumor immunotherapy. Signal transduction targeted Ther (2021) 6(1):75. doi: 10.1038/s41392-021-00484-9
32. Pang L, Han S, Jiao Y, Jiang S, He X, Li P. Bu fei decoction attenuates the tumor associated macrophage stimulated proliferation, migration, invasion and immunosuppression of non-small cell lung cancer, partially Via il-10 and pd-L1 regulation. Int J Oncol (2017) 51(1):25–38. doi: 10.3892/ijo.2017.4014
33. Pellino A, Loupakis F, Cadamuro M, Dadduzio V, Fassan M, Guido M, et al. Precision medicine in cholangiocarcinoma. Trans Gastroenterol Hepatol (2018) 3:40. doi: 10.21037/tgh.2018.07.02
34. Liu X, Yao J, Song L, Zhang S, Huang T, Li Y. Local and abscopal responses in advanced intrahepatic cholangiocarcinoma with low tmb, mss, pmmr and negative pd-L1 expression following combined therapy of sbrt with pd-1 blockade. J immunother Cancer (2019) 7(1):204. doi: 10.1186/s40425-019-0692-z
35. Chen WX, Li GX, Hu ZN, Zhu P, Zhang BX, Ding ZY. Significant response to anti-Pd-1 based immunotherapy plus lenvatinib for recurrent intrahepatic cholangiocarcinoma with bone metastasis: A case report and literature review. Medicine (2019) 98(45):e17832. doi: 10.1097/md.0000000000017832
36. Kwok G, Yau TC, Chiu JW, Tse E, Kwong YL. Pembrolizumab (Keytruda). Hum Vaccines immunotherapeutics (2016) 12(11):2777–89. doi: 10.1080/21645515.2016.1199310
37. Valle JW. Advances in the treatment of metastatic or unresectable biliary tract cancer. Ann Oncol (2010) 21 Suppl 7:vii345–8. doi: 10.1093/annonc/mdq420
38. Rizvi H, Sanchez-Vega F, La K, Chatila W, Jonsson P, Halpenny D, et al. Molecular determinants of response to anti-programmed cell death (Pd)-1 and anti-programmed death-ligand 1 (Pd-L1) blockade in patients with non-Small-Cell lung cancer profiled with targeted next-generation sequencing. J Clin Oncol Off J Am Soc Clin Oncol (2018) 36(7):633–41. doi: 10.1200/jco.2017.75.3384
39. Xu G, Sun L, Li Y, Xie F, Zhou X, Yang H, et al. The clinicopathological and prognostic value of pd-L1 expression in cholangiocarcinoma: A meta-analysis. Front Oncol (2019) 9:897. doi: 10.3389/fonc.2019.00897
40. Ma K, Wei X, Dong D, Wu Y, Geng Q, Li E. Pd-L1 and pd-1 expression correlate with prognosis in extrahepatic cholangiocarcinoma. Oncol Lett (2017) 14(1):250–6. doi: 10.3892/ol.2017.6105
41. Zeng FL, Chen JF. Application of immune checkpoint inhibitors in the treatment of cholangiocarcinoma. Technol Cancer Res Treat (2021) 20:15330338211039952. doi: 10.1177/15330338211039952
42. Deng M, Li SH, Fu X, Yan XP, Chen J, Qiu YD, et al. Relationship between pd-L1 expression, Cd8+ T-cell infiltration and prognosis in intrahepatic cholangiocarcinoma patients. Cancer Cell Int (2021) 21(1):371. doi: 10.1186/s12935-021-02081-w
43. Fontugne J, Augustin J, Pujals A, Compagnon P, Rousseau B, Luciani A, et al. Pd-L1 expression in perihilar and intrahepatic cholangiocarcinoma. Oncotarget (2017) 8(15):24644–51. doi: 10.18632/oncotarget.15602
44. Le DT, Uram JN, Wang H, Bartlett BR, Kemberling H, Eyring AD, et al. Pd-1 blockade in tumors with mismatch-repair deficiency. New Engl J Med (2015) 372(26):2509–20. doi: 10.1056/NEJMoa1500596
45. Le DT, Durham JN, Smith KN, Wang H, Bartlett BR, Aulakh LK, et al. Mismatch repair deficiency predicts response of solid tumors to pd-1 blockade. Sci (New York NY) (2017) 357(6349):409–13. doi: 10.1126/science.aan6733
46. Marabelle A, Le DT, Ascierto PA, Di Giacomo AM, De Jesus-Acosta A, Delord JP, et al. Efficacy of pembrolizumab in patients with noncolorectal high microsatellite Instability/Mismatch repair-deficient cancer: Results from the phase ii keynote-158 study. J Clin Oncol (2020) 38(1):1–10. doi: 10.1200/jco.19.02105
47. Zhou G, Sprengers D, Mancham S, Erkens R, Boor PPC, van Beek AA, et al. Reduction of immunosuppressive tumor microenvironment in cholangiocarcinoma by ex vivo targeting immune checkpoint molecules. J Hepatol (2019) 71(4):753–62. doi: 10.1016/j.jhep.2019.05.026
48. Fu J, McGrath NA, Lee J, Wang X, Brar G, Xie C. Verteporfin synergizes the efficacy of anti-Pd-1 in cholangiocarcinoma. Hepatobil pancreatic Dis Int HBPD Int (2022) 21(5):485–92. doi: 10.1016/j.hbpd.2022.03.006
49. Liu ZL, Liu X, Peng H, Peng ZW, Long JT, Tang D, et al. Anti-Pd-1 immunotherapy and radiotherapy for stage iv intrahepatic cholangiocarcinoma: A case report. Front Med (2020) 7:368. doi: 10.3389/fmed.2020.00368
50. Zhang Z, Zhang W, Wang H, Hu B, Wang Z, Lu S. Successful treatment of advanced intrahepatic cholangiocarcinoma with a high tumor mutational burden and pd-L1 expression by pd-1 blockade combined with tyrosine kinase inhibitors: A case report. Front Immunol (2021) 12:744571. doi: 10.3389/fimmu.2021.744571
51. Zhang J, Wu L, Liu J, Lin M. A metastatic intrahepatic cholangiocarcinoma treated with programmed cell death 1 inhibitor: A case report and literature review. Immunotherapy (2020) 12(8):555–61. doi: 10.2217/imt-2019-0100
52. Zhao Q, Chen Y, Du S, Yang X, Chen Y, Ji Y, et al. Integration of radiotherapy with anti-Pd-1 antibody for the treatment of intrahepatic or hilar cholangiocarcinoma: Reflection from four cases. Cancer Biol Ther (2021) 22(3):175–83. doi: 10.1080/15384047.2020.1834792
53. Zhang N, Yu BR, Wang YX, Zhao YM, Zhou JM, Wang M, et al. Clinical outcomes of hepatic arterial infusion chemotherapy combined with tyrosine kinase inhibitors and anti-Pd-1 immunotherapy for unresectable intrahepatic cholangiocarcinoma. J digestive Dis (2022) 23(8-9):535–45. doi: 10.1111/1751-2980.13127
54. Li X, Jiang Z, Wu Y, Gong W, Liao X, Li X. Case report: Conversion therapy for advanced intrahepatic cholangiocarcinoma using pd-1 inhibitor plus s-1 and nab-paclitaxel. Front Oncol (2022) 12:935817. doi: 10.3389/fonc.2022.935817
55. Loeuillard E, Yang J, Buckarma E, Wang J, Liu Y, Conboy C, et al. Targeting tumor-associated macrophages and granulocytic myeloid-derived suppressor cells augments pd-1 blockade in cholangiocarcinoma. J Clin Invest (2020) 130(10):5380–96. doi: 10.1172/jci137110
56. Gao Z, Chen JF, Li XG, Shi YH, Tang Z, Liu WR, et al. Kras acting through erk signaling stabilizes pd-L1 Via inhibiting autophagy pathway in intrahepatic cholangiocarcinoma. Cancer Cell Int (2022) 22(1):128. doi: 10.1186/s12935-022-02550-w
57. Diggs LP, Ruf B, Ma C, Heinrich B, Cui L, Zhang Q, et al. Cd40-mediated immune cell activation enhances response to anti-Pd-1 in murine intrahepatic cholangiocarcinoma. J Hepatol (2021) 74(5):1145–54. doi: 10.1016/j.jhep.2020.11.037
58. Chui NN, Cheu JW, Yuen VW, Chiu DK, Goh CC, Lee D, et al. Inhibition of Cmtm4 sensitizes cholangiocarcinoma and hepatocellular carcinoma to T cell-mediated antitumor immunity through pd-L1. Hepatol Commun (2022) 6(1):178–93. doi: 10.1002/hep4.1682
59. Brunner MC, Chambers CA, Chan FK, Hanke J, Winoto A, Allison JP. Ctla-4-Mediated inhibition of early events of T cell proliferation. J Immunol (Baltimore Md 1950) (1999) 162(10):5813–20. doi: 10.4049/jimmunol.162.10.5813
60. Gavin M, Rudensky A. Control of immune homeostasis by naturally arising regulatory Cd4+ T cells. Curr Opin Immunol (2003) 15(6):690–6. doi: 10.1016/j.coi.2003.09.011
61. Chambers CA, Allison JP. Costimulatory regulation of T cell function. Curr Opin Cell Biol (1999) 11(2):203–10. doi: 10.1016/s0955-0674(99)80027-1
62. Alegre ML, Frauwirth KA, Thompson CB. T-Cell regulation by Cd28 and ctla-4. Nat Rev Immunol (2001) 1(3):220–8. doi: 10.1038/35105024
63. Klein O, Kee D, Nagrial A, Markman B, Underhill C, Michael M, et al. Evaluation of combination nivolumab and ipilimumab immunotherapy in patients with advanced biliary tract cancers: Subgroup analysis of a phase 2 nonrandomized clinical trial. JAMA Oncol (2020) 6(9):1405–9. doi: 10.1001/jamaoncol.2020.2814
64. Zhou G, Boor PPC, Bruno MJ, Sprengers D, Kwekkeboom J. Immune suppressive checkpoint interactions in the tumour microenvironment of primary liver cancers. Br J Cancer (2022) 126(1):10–23. doi: 10.1038/s41416-021-01453-3
65. Kim RD, Chung V, Alese OB, El-Rayes BF, Li D, Al-Toubah TE, et al. A phase 2 multi-institutional study of nivolumab for patients with advanced refractory biliary tract cancer. JAMA Oncol (2020) 6(6):888–94. doi: 10.1001/jamaoncol.2020.0930
66. Oh DY, Lee KH, Lee DW, Yoon J, Kim TY, Bang JH, et al. Gemcitabine and cisplatin plus durvalumab with or without tremelimumab in chemotherapy-naive patients with advanced biliary tract cancer: An open-label, single-centre, phase 2 study. Lancet Gastroenterol Hepatol (2022) 7(6):522–32. doi: 10.1016/s2468-1253(22)00043-7
67. Piha-Paul SA, Oh DY, Ueno M, Malka D, Chung HC, Nagrial A, et al. Efficacy and safety of pembrolizumab for the treatment of advanced biliary cancer: Results from the keynote-158 and keynote-028 studies. Int J Cancer (2020) 147(8):2190–8. doi: 10.1002/ijc.33013
68. Doki Y, Ueno M, Hsu CH, Oh DY, Park K, Yamamoto N, et al. Tolerability and efficacy of durvalumab, either as monotherapy or in combination with tremelimumab, in patients from Asia with advanced biliary tract, esophageal, or head-and-Neck cancer. Cancer Med (2022) 11(13):2550–60. doi: 10.1002/cam4.4593
69. Yarchoan M, Cope L, Ruggieri AN, Anders RA, Noonan AM, Goff LW, et al. Multicenter randomized phase ii trial of atezolizumab with or without cobimetinib in biliary tract cancers. J Clin Invest (2021) 131(24):e152670. doi: 10.1172/jci152670
70. Xie C, Duffy AG, Mabry-Hrones D, Wood B, Levy E, Krishnasamy V, et al. Tremelimumab in combination with microwave ablation in patients with refractory biliary tract cancer. Hepatol (Baltimore Md) (2019) 69(5):2048–60. doi: 10.1002/hep.30482
71. Buchbinder EI, Desai A. Ctla-4 and pd-1 pathways: Similarities, differences, and implications of their inhibition. Am J Clin Oncol (2016) 39(1):98–106. doi: 10.1097/coc.0000000000000239
72. Farolfi A, Ridolfi L, Guidoboni M, Nicoletti SV, Piciucchi S, Valmorri L, et al. Ipilimumab in advanced melanoma: Reports of long-lasting responses. Melanoma Res (2012) 22(3):263–70. doi: 10.1097/CMR.0b013e328353e65c
73. Wang S, Chen S, Zhong Q, Liu Y. Immunotherapy for the treatment of advanced nasopharyngeal carcinoma: A promising new era. J Cancer Res Clin Oncol (2022). doi: 10.1007/s00432-022-04214-8
74. Liu D, Heij LR, Czigany Z, Dahl E, Lang SA, Ulmer TF, et al. The role of tumor-infiltrating lymphocytes in cholangiocarcinoma. J Exp Clin Cancer Res CR (2022) 41(1):127. doi: 10.1186/s13046-022-02340-2
75. Eshhar Z, Waks T, Gross G, Schindler DG. Specific activation and targeting of cytotoxic lymphocytes through chimeric single chains consisting of antibody-binding domains and the gamma or zeta subunits of the immunoglobulin and T-cell receptors. Proc Natl Acad Sci United States America (1993) 90(2):720–4. doi: 10.1073/pnas.90.2.720
76. Vind J, Sørensen MA, Rasmussen MD, Pedersen S. Synthesis of proteins in escherichia coli is limited by the concentration of free ribosomes. expression from reporter genes does not always reflect functional mrna levels. J Mol Biol (1993) 231(3):678–88. doi: 10.1006/jmbi.1993.1319
77. Higuchi R, Yamamoto M, Hatori T, Shimizu K, Imai K, Takasaki K. Intrahepatic cholangiocarcinoma with lymph node metastasis successfully treated by immunotherapy with Cd3-activated T cells and dendritic cells after surgery: Report of a case. Surg Today (2006) 36(6):559–62. doi: 10.1007/s00595-006-3201-1
78. Tran E, Turcotte S, Gros A, Robbins PF, Lu YC, Dudley ME, et al. Cancer immunotherapy based on mutation-specific Cd4+ T cells in a patient with epithelial cancer. Sci (New York NY) (2014) 344(6184):641–5. doi: 10.1126/science.1251102
79. Tariq NU, Vogel A, McNamara MG, Valle JW. Biliary tract cancer: Implicated immune-mediated pathways and their associated potential targets. Oncol Res Treat (2018) 41(5):298–304. doi: 10.1159/000488997
80. Asahi Y, Hatanaka KC, Hatanaka Y, Kamiyama T, Orimo T, Shimada S, et al. Prognostic impact of Cd8+ T cell distribution and its association with the hla class I expression in intrahepatic cholangiocarcinoma. Surg Today (2020) 50(8):931–40. doi: 10.1007/s00595-020-01967-y
81. Guo Y, Feng K, Liu Y, Wu Z, Dai H, Yang Q, et al. Phase I study of chimeric antigen receptor-modified T cells in patients with egfr-positive advanced biliary tract cancers. Clin Cancer Res (2018) 24(6):1277–86. doi: 10.1158/1078-0432.ccr-17-0432
82. Alnaggar M, Xu Y, Li J, He J, Chen J, Li M, et al. Allogenic V ³9v ´2 T cell as new potential immunotherapy drug for solid tumor: A case study for cholangiocarcinoma. J immunother Cancer (2019) 7(1):36. doi: 10.1186/s40425-019-0501-8
83. Sangsuwannukul T, Supimon K, Sujjitjoon J, Phanthaphol N, Chieochansin T, Poungvarin N, et al. Anti-tumour effect of the fourth-generation chimeric antigen receptor T cells targeting Cd133 against cholangiocarcinoma cells. Int Immunopharmacol (2020) 89(Pt B):107069. doi: 10.1016/j.intimp.2020.107069
84. Piatakova A, Polakova I, Smahelova J, Johari SD, Nunvar J, Smahel M. Distinct responsiveness of tumor-associated macrophages to immunotherapy of tumors with different mechanisms of major histocompatibility complex class I downregulation. Cancers (2021) 13(12):3057. doi: 10.3390/cancers13123057
85. Yoshida H, Katayose Y, Unno M, Suzuki M, Kodama H, Takemura S, et al. A novel adenovirus expressing human 4-1bb ligand enhances antitumor immunity. Cancer immunol immunother CII (2003) 52(2):97–106. doi: 10.1007/s00262-002-0334-y
86. Wongkajornsilp A, Somchitprasert T, Butraporn R, Wamanuttajinda V, Kasetsinsombat K, Huabprasert S, et al. Human cytokine-induced killer cells specifically infiltrated and retarded the growth of the inoculated human cholangiocarcinoma cells in scid mice. Cancer Invest (2009) 27(2):140–8. doi: 10.1080/07357900802189832
87. He M, Wang Y, Shi WJ, Wang SJ, Sha HF, Feng JX, et al. Immunomodulation of inducible Co-stimulator (Icos) in human cytokine-induced killer cells against cholangiocarcinoma through Icos/Icos ligand interaction. J digestive Dis (2011) 12(5):393–400. doi: 10.1111/j.1751-2980.2011.00527.x
88. Kobayashi M, Sakabe T, Abe H, Tanii M, Takahashi H, Chiba A, et al. Dendritic cell-based immunotherapy targeting synthesized peptides for advanced biliary tract cancer. J gastrointestinal Surg (2013) 17(9):1609–17. doi: 10.1007/s11605-013-2286-2
89. Jiraviriyakul A, Songjang W, Kaewthet P, Tanawatkitichai P, Bayan P, Pongcharoen S. Honokiol-enhanced cytotoxic T lymphocyte activity against cholangiocarcinoma cells mediated by dendritic cells pulsed with damage-associated molecular patterns. World J Gastroenterol (2019) 25(29):3941–55. doi: 10.3748/wjg.v25.i29.3941
90. Huang X, Tang T, Zhang G, Liang T. Identification of tumor antigens and immune subtypes of cholangiocarcinoma for mrna vaccine development. Mol Cancer (2021) 20(1):50. doi: 10.1186/s12943-021-01342-6
91. Marks EI, Yee NS. Immunotherapeutic approaches in biliary tract carcinoma: Current status and emerging strategies. World J gastrointestinal Oncol (2015) 7(11):338–46. doi: 10.4251/wjgo.v7.i11.338
92. Takahashi R, Yoshitomi M, Yutani S, Shirahama T, Noguchi M, Yamada A, et al. Current status of immunotherapy for the treatment of biliary tract cancer. Hum Vaccines immunotherapeutics (2013) 9(5):1069–72. doi: 10.4161/hv.23844
93. Yamamoto K, Ueno T, Kawaoka T, Hazama S, Fukui M, Suehiro Y, et al. Muc1 peptide vaccination in patients with advanced pancreas or biliary tract cancer. Anticancer Res (2005) 25(5):3575–9.
94. Kaida M, Morita-Hoshi Y, Soeda A, Wakeda T, Yamaki Y, Kojima Y, et al. Phase 1 trial of wilms tumor 1 (Wt1) peptide vaccine and gemcitabine combination therapy in patients with advanced pancreatic or biliary tract cancer. J immunother (Hagerstown Md 1997) (2011) 34(1):92–9. doi: 10.1097/CJI.0b013e3181fb65b9
95. Han S, Lee SY, Wang WW, Tan YB, Sim RHZ, Cheong R, et al. A perspective on cell therapy and cancer vaccine in biliary tract cancers (Btcs). Cancers (2020) 12(11):3404. doi: 10.3390/cancers12113404
96. Aruga A, Takeshita N, Kotera Y, Okuyama R, Matsushita N, Ohta T, et al. Long-term vaccination with multiple peptides derived from cancer-testis antigens can maintain a specific T-cell response and achieve disease stability in advanced biliary tract cancer. Clin Cancer Res (2013) 19(8):2224–31. doi: 10.1158/1078-0432.ccr-12-3592
97. Shimizu K, Kotera Y, Aruga A, Takeshita N, Takasaki K, Yamamoto M. Clinical utilization of postoperative dendritic cell vaccine plus activated T-cell transfer in patients with intrahepatic cholangiocarcinoma. J hepato-biliary-pancreatic Sci (2012) 19(2):171–8. doi: 10.1007/s00534-011-0437-y
Glossary
Keywords: immunotherapy, PD-1/PD-L1 inhibitor, CTLA4 inhibitor, molecular therapy, cell transplantation, vaccines, cell therapy, cholangiocarcinoma
Citation: Zhao L-m, Shi A-d, Yang Y, Liu Z-l, Hu X-Q, Shu L-Z, Tang Y-c and Zhang Z-l (2023) Advances in molecular and cell therapy for immunotherapy of cholangiocarcinoma. Front. Oncol. 13:1140103. doi: 10.3389/fonc.2023.1140103
Received: 13 January 2023; Accepted: 17 March 2023;
Published: 29 March 2023.
Edited by:
Qun Zhang, Nanjing Medical University, ChinaReviewed by:
Peng Huang, Xiangya Hospital, Central South University, ChinaXiu-Ping Zhang, People’s Liberation Army General Hospital, China
Chen Shuxian, Third Affiliated Hospital of Sun Yat-sen University, China
Copyright © 2023 Zhao, Shi, Yang, Liu, Hu, Shu, Tang and Zhang. This is an open-access article distributed under the terms of the Creative Commons Attribution License (CC BY). The use, distribution or reproduction in other forums is permitted, provided the original author(s) and the copyright owner(s) are credited and that the original publication in this journal is cited, in accordance with accepted academic practice. No use, distribution or reproduction is permitted which does not comply with these terms.
*Correspondence: Yong-chang Tang, tangyongchang@sdu.edu.cn; Zong-li Zhang, zzlzzl1900@163.com
†These authors have contributed equally to this work