- 1Department of Gastric Surgery, The Cancer Hospital of the University of Chinese Academy of Sciences (Zhejiang Cancer Hospital), Institutes of Basic Medicine and Cancer (IBMC), Chinese Academy of Sciences, Hangzhou, China
- 2Zhejiang Provincial Research Center for Upper Gastrointestinal Tract Cancer, Zhejiang Cancer Hospital, Hangzhou, China
- 3Zhejiang Key Lab of Prevention, Diagnosis and Therapy of Upper Gastrointestinal Cancer, Zhejiang Cancer Hospital, Hangzhou, China
Cancers of the gastrointestinal (GI) tract greatly contribute to the global cancer burden and cancer-related death. Claudin-18.2(CLDN18.2), a transmembrane protein, is a major component of tight junctions and plays an important role in the maintenance of barrier function. Its characteristic widespread expression in tumour tissues and its exposed extracellular loops make it an ideal target for researchers to develop targeted strategies and immunotherapies for cancers of the GI tract. In the present review, we focus on the expression pattern of CLDN18.2 and its clinical significance in GI cancer. We also discuss the tumour-promoting and/or tumour-inhibiting functions of CLDN18.2, the mechanisms regulating its expression, and the current progress regarding the development of drugs targeting CLDN18.2 in clinical research.
1 Introduction
Cancers of the gastrointestinal (GI) tract accounted for an estimated 4.8 million new cancer cases and 3.4 million cancer-related deaths worldwide in 2018; these numbers correspond to over one-quarter (26%) of global cancer cases and over one-third (35%) of all cancer-related deaths (1). Thus, cancers of the GI tract remain important contributors to the global cancer burden. Improving the efficacy of tumour treatment is the key to improving the survival and prognosis of patients with GI tumours.
In tumour treatment, surgery and chemotherapy are the common treatment approaches (2). For patients with early cancer, surgical resection remains the primary treatment option; for patients with advanced metastasis or patients who cannot undergo surgical resection, chemotherapy is the most indispensable treatment (3). However, chemotherapy resistance and tumour progression and recurrence in cancer patients have become the most common causes of treatment failure (4). In the past decade, remarkable advances made by studies of tumour biology, tumour immunotherapy and targeted therapy have revolutionised the treatment of a fair number of cancers (5). Small molecule targeted drugs have shown certain advantages in tumour treatment.
Recently, increasing attention has been given to a potential target, Claudin18.2 (CLDN18.2). Its restricted expression in normal tissues and specific expression in tumour tissues makes it an ideal target for researchers to develop targeted therapy and immunotherapies for solid tumours (6). CLDN18.2 was initially identified as a gastric tumour-specific target, and many clinical trials of monoclonal antibodies targeting CLDN18.2 have been conducted for the treatment of patients with gastric and gastroesophageal cancer. However, several other novel targeting methods, including chimeric antigen receptor T (CAR-T) therapy, bispecific antibodies and antibody–drug conjugates (ADCs), have rapidly emerged in recent years, and a variety of corresponding clinical trials that have broadened their treatment adaptation to populations, especially those with GI tract cancer, have been conducted. This review will discuss the expression pattern of CLDN18.2, its roles in tumours and its relevant molecular mechanisms, as well as the current progress in the development of drugs targeting CLDN18.2 in clinical research.
2 Basic information of CLDN18
Claudins, a family of at least 27 transmembrane proteins, are important components and functional structures of tight cell junctions. Claudin-18 is a major component of tight junctions located on the cell membrane surface; it plays an important role in the maintenance of cell polarity and barrier function and promotes acid resistance (7–10). For example, in the stomach, claudin-18 normally forms a paracellular barrier against H(+), promotes acid resistance, and causes paracellular H(+) leakage, persistent upregulation of proinflammatory cytokines and atrophic gastritis in mice (11). The human CLDN18 gene locus on chromosome 3q22 has a molecular weight of approximately 35 kb and contains 6 exons and 5 introns. The first exon of CLDN18 can be alternatively spliced, forming two different splice mutants (CLDN18.1 and CLDN18.2) that have highly homologous amino acid sequences (12). The transmembrane protein CLDN18 consists of two extracellular loops (ECLs), four transmembrane domains and a cytoplasmic domain. Both the C-terminus and the N-terminus of CLDN 18 are located in the cytoplasm (Figure 1) (6). Two CLDN18 protein isoforms are expressed in a tissue-specific manner—CLDN18.1 and CLDN18.2 are specifically expressed in normal stomach and lung tissues, respectively (13). CLDN18 is also expressed in cancer tissues and has altered functions that are linked to tumour formation, proliferation, invasion and migration (6, 14–18).
CLDN18.1 is highly expressed in lung alveolar epithelium and is involved in regulating solute and ion permeability, as well as cytoskeletal rearrangement (9). It has been found that CLDN18.1 knockout increased solute permeability and alveolar fluid clearance, and enhanced epithelial sodium channel and Na-K-ATPase activity (9). However, CLDN18.1 expression was significantly decreased in lung cancer and may play a role in inhibiting tumour progression (19, 20). Luo et al. found that when restored in lung adenocarcinoma (LUAD) cells that have lost expression, CLDN18.1 markedly attenuates tumour growth in vivo as well as cell proliferation, migration, invasion and colony formation in vitro (20). Zhou et al. also elucidated that knockout of Cldn18.1 results in increased lung size, progenitor cell proliferation, and tumourgenesis in mice (17).
CLDN18.2 is also involved in regulating ion permeability including H(+) and other cations (11). CLDN18.2 deficiency leads to neutrophils recruiment and inflammation development (11). As the focus of our review, the expression pattern and role of CLDN18.2 in tumours will be elaborated in detail later in the article.
ARHGAP26 is a GTPase-activating protein (GAP) that promotes conversion of RHO GTPases to the GDP state and has been indicated in enhancing cellular motility (21). CLDN18-ARHGAP26 fusion gene is one of the most frequent somatic genomic rearrangements in gastric cancer, especially in the genomically stable subtype (22, 23). Cell survival and migration studies performed by Ushiku et al. showed that CLDN18-ARHGAP fusions complemented cancer cell survival and induced cell migration under RHOA knockdown condition. RHOA mutations exist specifically in diffuse type gastric cancers (DGC) and are considered one of the DGC driver genes. Thus, CLDN18-ARHGAP fusion is possible a factor for RHOA mutation to maintain cancer cell survival and promoted cell migration (24).
3 The expression pattern and clinical significance of CLDN18.2 in cancers of the GI tract
For a long time, CLDN18.2 has been widely studied in patients with various solid tumours, especially in patients with cancers of the GI tract. Data for the expression pattern and clinical significance of CLDN18.2 in cancers of the GI tract are summarised in Table 1.
Up to present, for CLDN18.2 detection modalities, the vast majority of clinical studies use tissue samples to detect its protein expression by immunohistochemistry, including all studies in Table 1. Besides, Sanada et al. also tested CLDN18.2 mRNA expression by reverse transcription (RT)-polymerase chain reaction (PCR) (41). In addition, one study innovatively designed a CLDN18.2 molecular beacon (MB) with high resolution and selectivity to distinguish CLDN18.2 RNA expression in circulating tumour cells (CTCs). They compared immunohistochemistry to MB data in 10 GC patients and verified that the concordance in the expression of CLDN18.2 between CTCs and tissue biopsy is 100% (negative: 3 vs 3; positive: 7 vs 7) (42). Thus, CTCs detection in peripheral blood, a non-invasive and method, may be a promising approach for evaluating CLDN18.2 expression and subsequently contribute to timely treatment for patients diagnosed with CLDN18.2 highly expressed GC. Further investigations are needed for the other courses of tumours like prognosis and the tests for more patients.
The CLDN18.2 protein is a highly selective gastric lineage marker expressed in short-lived differentiated cells but not the stem cell zone of stomach mucosa (43). In addition to its orthotopic expression, frequent ectopic activation of CLDN18.2 was detected in pancreatic, oesophageal, biliary tract, ovarian, and lung tumours, whereas downregulation was found in gastric cancer (GC) and sarcoma (gastric stromal tumour) tissues compared to their corresponding normal tissues (38, 43–45). It is worth noting that CLDN18.2 expression is decreased but still stable during gastric malignant transformation. Moreover, CLDN18.2 activation in metastatic lesions (lymph node and liver metastases) is comparable to that in primary lesions (26, 30, 31). These findings are of great significance, as they indicate that not only those with locally advanced disease but also those with metastatic cancer may be candidate patient populations for clinical trial targeting CLDN18.2.
CLDN18 expression is associated with histological subtype in GC. In GCs grouped by the Lauren subtype, CLDN18.2 expression was significantly higher in diffuse-type GCs (26, 30). In terms of the mucin phenotype, samples with the intestinal phenotype predominantly showed downregulation of CLDN18.2, suggesting that the loss of CLDN18 is involved in the pathogenesis of tumours of the intestinal phenotype and indicating that its expression can be used as a marker for the gastric phenotype (28, 41, 46).
In the relationship between CLDN18.2 expression and clinicopathological factors, Jun et al. showed a significant inverse correlation between claudin-18 expression and perineural invasion (27). In addition, a study of Japanese patients with gastric adenocarcinoma revealed that moderate-to-strong CLDN18.2 expression positively correlated with a higher grade of dedifferentiation (59.0% vs. 37.7% for G3 and G1/2 tumours, respectively; P = 0.005) (26). However, Soini et al. identified an inconsistent result with the previous study that positive CLDN18 expression is associated with a higher differentiation stage (35). In the pancreas, a correlation analysis revealed that lymph node-positive tumours had significantly higher CLDN18.2 expression indicating by the positive cell fractions and membrane staining intensities (33).
Claudin-18 has also been reported to be correlated with survival in GC patients. Jun et al. suggested the loss of claudin-18 as an independent indicator of a poor prognosis in patients with GC, as patients with claudin-18 expression had longer overall survival (OS) than those without claudin-18 expression (27). Sanada et al. also indicated that downregulation of claudin-18 was correlated with poor survival in patients with advanced GCs (41). However, in pancreatic cancer (PC), although there was an association between CLDN18.2 expression and lymph node metastasis (LNM) and dedifferentiation stage, as mentioned above, no correlation between CLDN18.2 expression and patient survival was found (33–36). In endocervical adenocarcinoma, gastric immunophenotypes (CLDN18.2) were identified as an independent predictor of poorer progression-free survival (PFS) (47).
Moreover, CLDN18-ARHGAP26/6 fusions have been identified in GCs (48). Tanaka et al. analysed 254 cases of GC (172 diffuse-type and 82 intestinal-type) using RT-PCR and situ hybridisation (FISH) and identified 26 fusion-positive cases (26/254, 10.24%), 22 of which were diffuse GCs (22/172, 12.79%), indicating that the CLDN18-ARHGAP26/6 fusion was specific to diffuse-type GCs (49). However, Hashimoto et al. found that intestinal-type adenocarcinoma with anastomosing glands, a genetically distinct group of intestinal-type adenocarcinoma, also frequently had CLDN18-ARHGAP fusion (50). Shu et al. found that the frequency of CLDN18-ARHGAP fusion is 18.25% in signet-ring cell carcinoma, and the CLDN18-ARHGAP26/6 fusion is associated with signet-ring cell content, age at diagnosis, female/male ratio, and TNM stage. The survival rate of patients with CLDN18-ARHGAP26/6 fusion is worse, and patients with this fusion obtain no benefit from oxaliplatin/fluoropyrimidine-based chemotherapy (51). Further studies have identified significant drug resistance acquired in CLDN18-ARHGAP26-introduced cell lines (51). Matsusaka et al. found that the combination of CDH17 and CLDN18 demonstrates homogeneous and robust expression in more than 90% of GC cases, and their coupling is helpful to detect and localise GC metastasis in vivo, which provides a possibility to overcome the challenge of intratumoural heterogeneity (52). Nakayama et al. found that the CLDN18‐ARHGAP fusion rate was 15.1% in 146 patients with GC under the age of 40. Among them, 18 cases (12.3%) were CLDN18-ARHGAP26 fusions, and 2 cases (1.4%) were CLDN18-ARHGAP6 fusions. Two new fusion types, CLDN18‐ARHGAP10 and CLDN18‐ARHGAP42, were identified. Moreover, patients with fusion were identified to more likely to have large tumours, LNM, and advanced stage and to have exhibited significantly lower OS by Kaplan‐Meier analysis. These observations indicate that the CLDN18‐ARHGAP fusion is enriched in younger age‐onset GCs, and its presence could contribute to their aggressive characteristics (53). Yao et al. demonstrated that the CLDN18-ARHGAP26 fusion mediates epithelial disintegration and contributes to GC by loss of CLDN18 and gain of ARHGAP26 functions (54). The CLDN18-ARHGAP fusion is one of the molecular characteristics of diffuse GC and is also an independent prognostic risk factor for GC. In addition, it is related to multiple clinicopathological characteristics, including age, sex, chemotherapy resistance, LNM and tumour stage. However, further exploration is required to elucidate the mechanism of the CLDN18-ARHGAP fusion gene and identify potential targeted therapeutic strategies.
4 Regulation of CLDN18 expression and its functions in tumours
It is universally acknowledged that a comprehensive and systematic understanding of target molecules’ functions in tumours is rather essential, partly because it can help to correctly apply therapeutic strategies to treat tumour patients. Studies for molecular regulatory mechanism include upstream mechanism study aiming to explore the pathways and molecules that regulate target molecules, and downstream mechanism study aiming to explore the pathways and molecules that are regulated by target molecules. The focus is on the latter in most cases. However, understanding the upstream mechanism is also very important. A comprehensive understanding of upstream regulatory factors can better clarify the source of target molecules and the causes of their changes, providing more insights for antitumour therapies that target molecular markers. In this section, we review the factors that regulate CLDN18 expression, as well as the roles of CLDN18 in cancers of the GI tract.
4.1 Regulation of CLDN18 expression
Previous studies have shown that carbonic anhydrase 9 (CAIX), phorbol 12-myristate 13-acetate (PMA), c-jun, epidermal growth factor (EGF) and RAS can upregulate the expression of CLDN18 (15, 55–57) and that interleukin-1β (IL-1β), hyperoxia, STE20/SPS1-related proline/alanine-rich kinase (SPAK) can inhibit CLDN18 expression (57, 58). The possible mechanisms are summarised as follows:
4.1.1 Methylation of CpG islands
Methylation of CpGs in promoter regions was suggested as a mechanism for the regulation of CLDN18 expression. The methylation status of CpG is correlated with CLDN18 gene expression (Figure 2). According to an analysis of data from the LUAD patient cohort of TCGA, CLDN18.1 mRNA expression is inversely correlated with the methylation of its promoter CpG island (20). It has been validated that in vitro methylation of the CLDN18.1 promoter can strongly inhibit the transcription of a linked luciferase reporter, suggesting that methylation of the CpG island may suppress the binding of transcription factors to the CLDN18.1 promoter and attenuate CLDN18.1 expression (20). The Claudin-18a1 promoter contains two binding sites of T/EBP/NKX2.1, which is a homeodomain-containing transcription factor (59). T/EBP/NKX2.1 binds to the claudin-18a1 promoter and promotes the transactivation of claudin-18a1 expression, and mutation of each motif attenuates claudin-18a1 promoter activity by approximately one-half, while mutation of both sites nearly abolishes the transcriptional activity of the gene (60). In addition, Sahin et al. indicated that methylation of CpG islands could completely prevent the transcription factor CREB from binding the promoter regions of CLDN18.2, which is required to activate CLDN18.2 transcription (43).
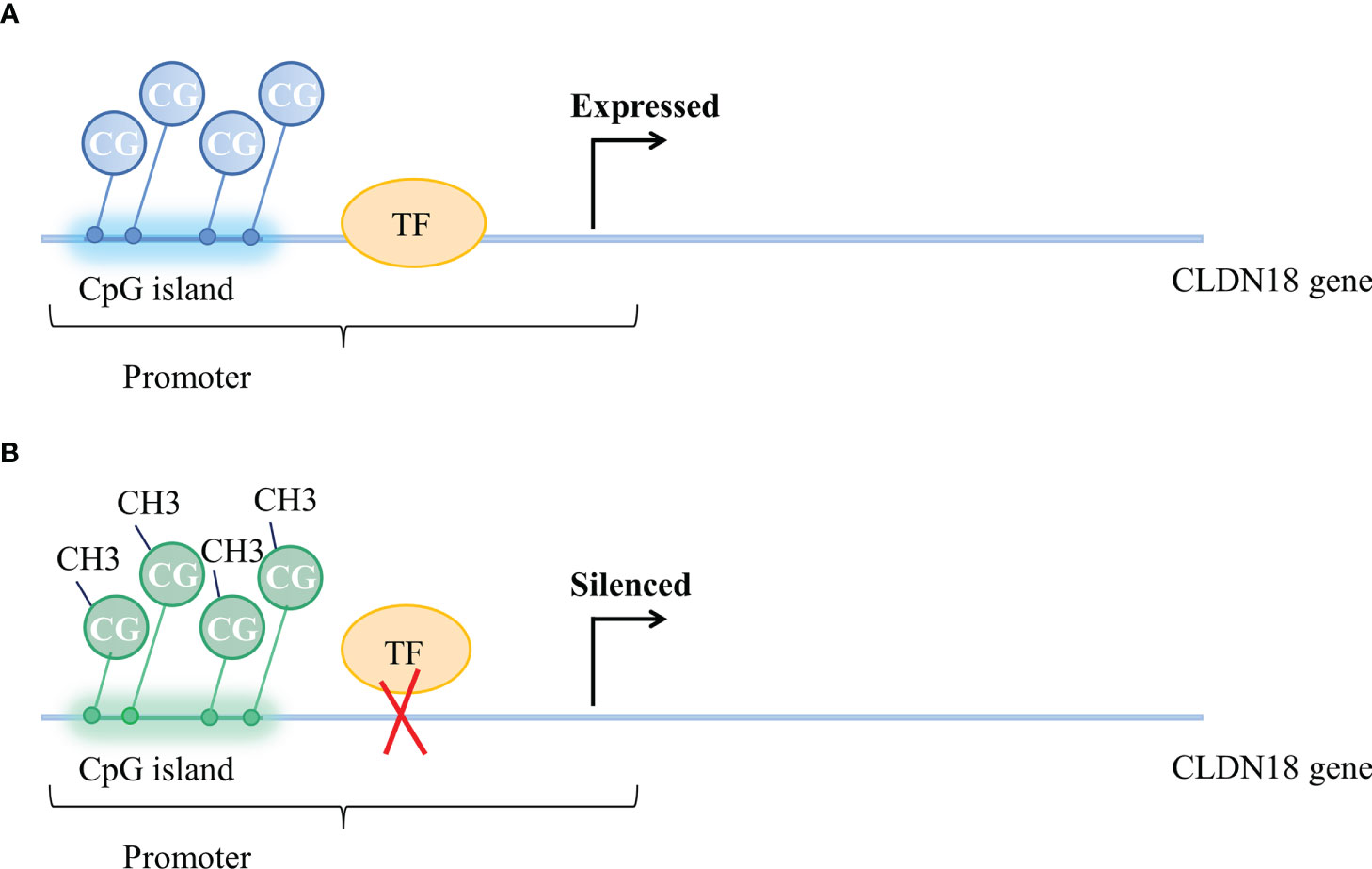
Figure 2 Methylation status of CpG islands and CLDN18 gene expression. (A) Unmethylated CpG island and expressed CLDN18.2 gene. (B) Methylation of CpG islands blocks the binding of transcription factors to the CLDN18.2 promoter, resulting in CLDN18.2 gene silencing.
4.1.2 PKC pathway and ERK/MAPK pathway
Protein kinase C (PKC) is an effector in the G protein-coupled receptor system and can promote the phosphorylation of a variety of proteins involved in many physiological, biochemical and pathological processes of cells, including the induction of cell activation and proliferation, differentiation, motility, and survival. In addition, PKC affects transcription factors in the nucleus and thus plays an important role in the regulation of gene expression (61).
Studies have found that the PKC pathway and extracellular signal-related kinase (ERK)/Mitogen-activated protein kinase (MAPK) pathway are involved in regulating the expression of CLDN18.2. The PKC pathway and ERK/MAPK pathway regulate the expression of CLDN18.2 by regulating an intracellular transcriptional activator (Figure 3). PMA is the most commonly used phorbol ester; it binds to and activates PKC, causing a wide range of effects in cells and tissues. Studies have demonstrated that when treated with PMA, the mRNA and protein expression of CLDN18.2 in pancreatic and GC cell lines is upregulated. However, a PKC inhibitor completely repressed the upregulation of CLDN18.2 induced by PMA, indicating that the PKC pathway is involved in regulating the expression of CLDN18.2 in carcinogenesis (34, 55, 62). The SPAK-p38 MAPK signalling pathway is also involved in hyperoxia-induced barrier dysfunction of the alveolar epithelium by suppressing the expression of claudin-18 (57).
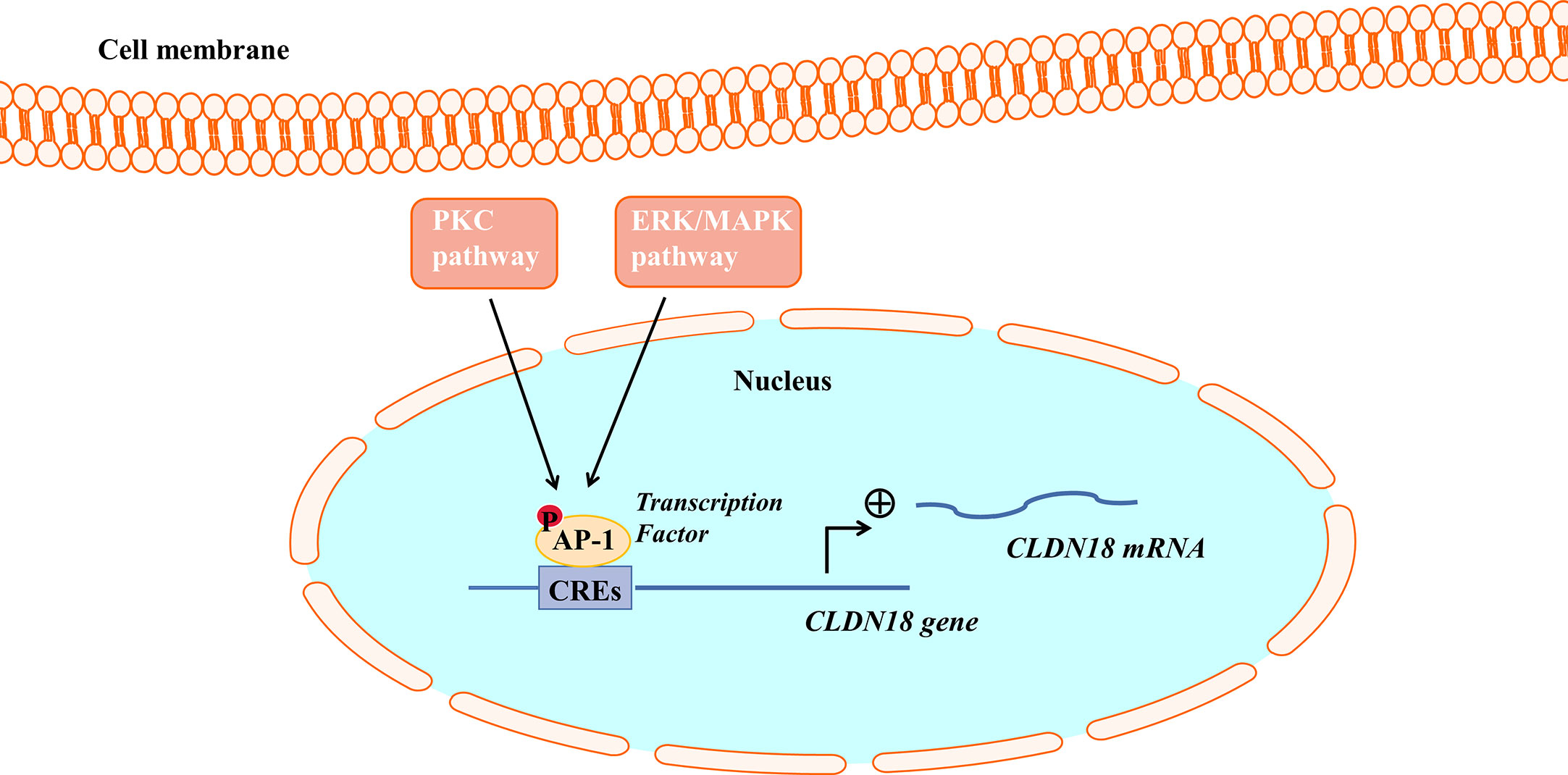
Figure 3 The PKC pathway and ERK/MAPK pathway regulate CLDN18.2 expression. AP-1 could bind to cis-regulatory elements (CREs) of the CLDN18.2 promoter, increasing the transcriptional activity of CLDN18.2. The PKC and ERK-MAPK pathways enhance the transcription of CLDN18.2 mRNA by stimulating the phosphorylation and activation of AP-1.
Activator protein (AP)-1 is an intracellular transcriptional activator and is required for cell-specific gene expression. AP-1 sites were shown to bind to cis-regulatory elements of the CLDN18a2 promoter, increasing the transcriptional activity of CLDN18.2 (55). It was further found that PMA induced the PKC and ERK-MAPK pathways and stimulated the phosphorylation and activation of AP-1, thereby enhancing the transcription of CLDN18.2 mRNA (55).
4.1.3 HER2/HER3 signalling pathway
The HER family consists of four membrane-bound tyrosine kinase receptors with similar structures: HER1 (EGFR), HER2, HER3, and HER4 (63). HER2 has no specific ligand, so only when it partners with another HER family member can it be activated. HER3 is the receptor for the ligand neuregulin-1 (NRG-1), but HER3 lacks intrinsic signalling properties (64). Upon NRG-1 binding, HER3 heterodimerises with HER2, resulting in activation of the downstream signalling pathway and involvement in normal cell growth and development as well as in the pathogenesis of epithelial malignancies (Figure 4) (65).
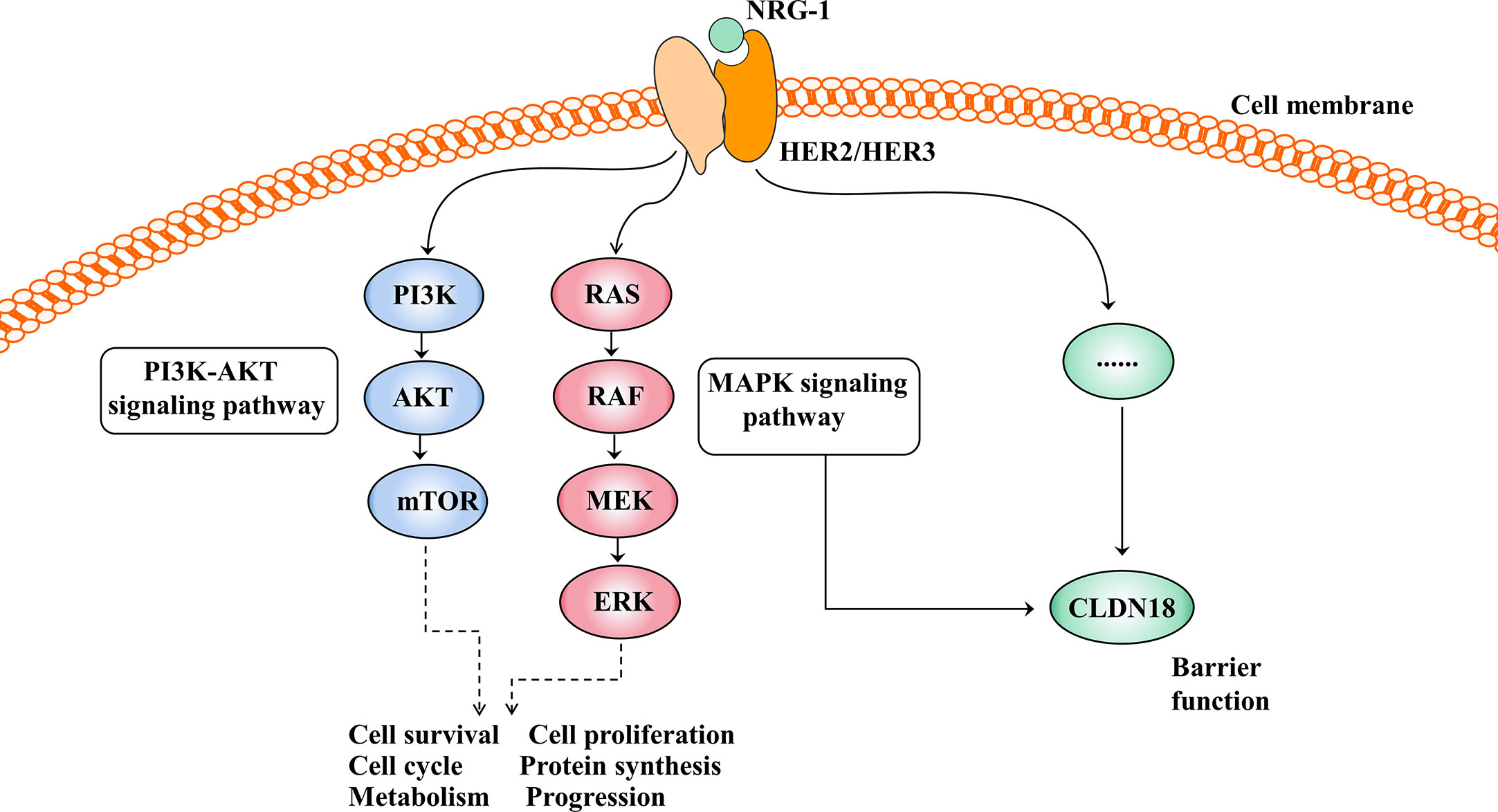
Figure 4 Activation of the HER2/HER3 signalling pathway and its effect after activation. The PI3K-AKT and MAPK signalling pathways are the two main signal transduction pathways downstream of HER2/3 heterodimerisation activation and are involved in cell growth, cell proliferation, the cell cycle, protein synthesis and so on. In addition, the HER2/HER3 signalling pathway regulates barrier function by regulating the expression of CLDN18, but the mechanism is unclear.
The HER2/HER3 signalling pathway is also involved in barrier function by regulating the expression of claudin18 (Figure 4). Finigan et al. revealed that the NRG-1-HER2/3 signalling pathway participates in IL-1β-mediated lung barrier dysfunction (66). In another study to identify potential corresponding pathways by which IL-1β promotes the development of acute respiratory distress syndrome (ARDS), Ma et al. showed that the HER2 blocker lapatinib blocked the effect of IL-1β on the downregulated expression and cell membrane localisation of claudin18, suggesting that IL-1β suppresses the protein expression and cell membrane localisation of claudin18 by activating the HER2/HER3-associated signalling pathway (58).
4.1.4 MicroRNA
MicroRNAs (miRNAs) are a large family of small endogenous regulatory RNAs that regulate posttranscriptional regulators of gene expression (67, 68). MiRNAs target both messenger RNA (mRNA) degradation and suppression of protein translation based on sequence complementarity between the miRNA and its targeted mRNA (69). MiRNAs regulate the expression of CLDN18 by binding to CLDN18 mRNA (Figure 5). Western blot analysis and luciferase reporter assays showed that miR-1303 could markedly attenuate the expression of claudin-18 by binding to the putative binding sites in the CLDN18 mRNA 3’-UTR (70). Wan et al. reported that miR-767-3p targets CLDN18, thus inhibiting tumour cell proliferation, migration, and invasion, providing a promising therapeutic target for LUAD (71).
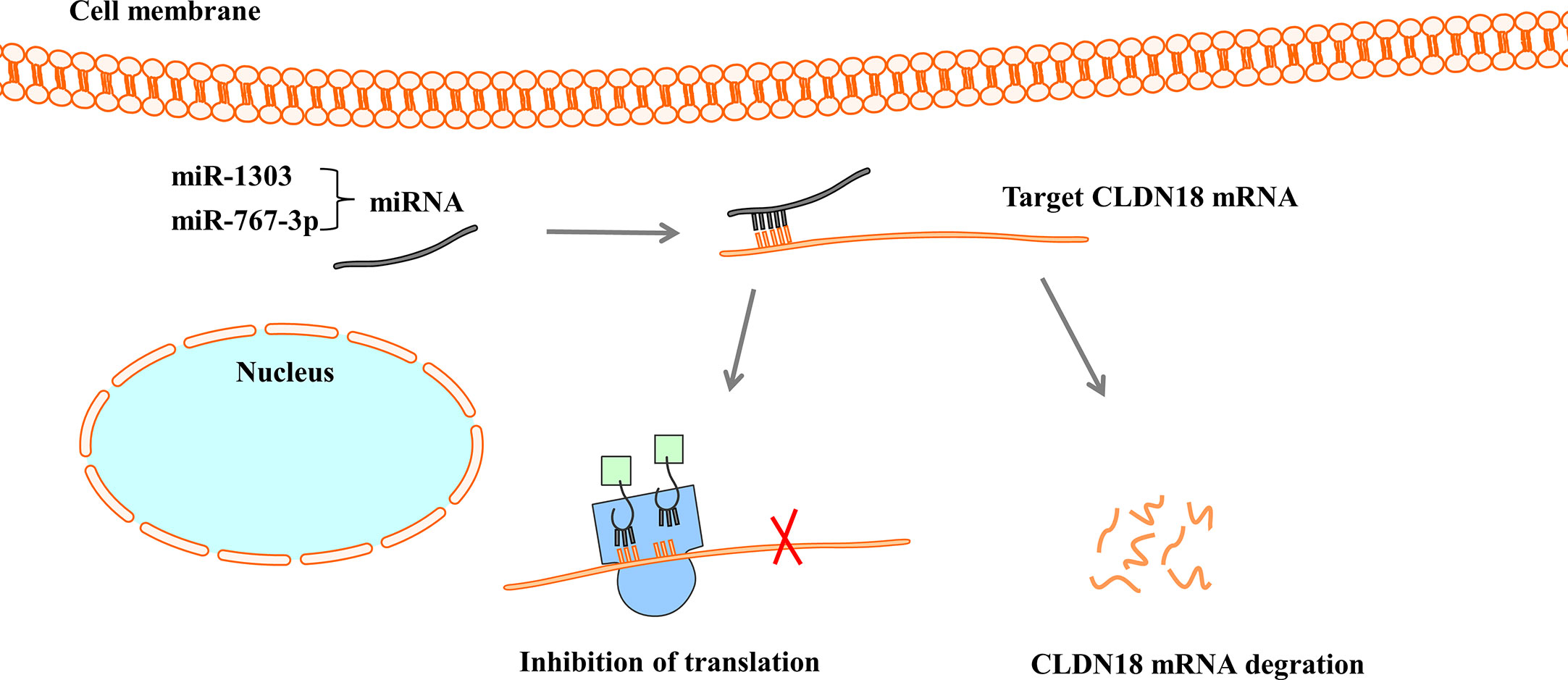
Figure 5 MiRNAs regulate the expression of CLDN18. MiRNAs bind with 3’-UTR of CLDN18 mRNA, resulting in the degradation of mRNA or the inhibition of translation.
The findings to date indicate that CLDN18 is regulated at the transcriptional level through the methylation of CpG islands, the PKC pathway, the ERK/MAPK pathway, and the HER signalling pathway and is regulated at the posttranscriptional level by miRNAs, providing new approaches for CLDN18-based cancer therapy.
4.2 Dichotomous roles of CLDN18 in cancers
CLDN18 has dichotomous roles in cancers: it can act as a tumour promoter or suppressor depending on the tumour type. CLDN18 plays a tumour suppressor role in gastric and lung cancers but functions as a tumour promoter in other cancers of the GI tract, including oesophageal, pancreatic, colorectal and bile duct cancers, which is consistent with the ectopic expression pattern described above. Although many studies have explored the different roles of CLDN18 in tumours, only a few articles have clarified its mechanisms.
4.2.1 The tumour suppressor role of CLDN18
Claudin-18 expression is significantly decreased in GC tissue compared to surrounding gastric normal mucosa or intestinal metaplasia. In early GC lesions removed via endoscopic mucosal resection or endoscopic submucosal resection, Oshima et al. evaluated the expression patterns of claudin-18 and Ki-67, a marker of cell proliferation, and then identified an inverse correlation between them at the invasive front. Subsequent experiments in GC cell lines further confirmed that knockdown of Claudin-18 could significantly promote cell proliferation and invasion, indicating a tumour suppressor role for CLDN18 (14). Hagen et al. showed that H. pylori infection in mice attenuates the expression of CLDN18 early in GC development, which is similar to the results in human patients. Mechanistically, the loss of CLDN18 promotes the rapid development of cancer cells in the stomach of mice, possibly because CLDN18 is a top regulator and effector of the network that regulates the cytokine, stemness, Wnt and Notch signalling pathways (16). In the lung, Zhou et al. identified that CLDN18 could reversely regulate the nuclear localisation and activity of the downstream transcriptional coactivator Yes-associated protein (YAP) and thus promotes the proliferation of AT2 cells in normal lung as well as that of tumour cells found in lung adenocarcinomas thought to originate from AT2 cells (17, 72). Given that expression of the lung-specific isoform (CLDN18.1) is markedly decreased in LUAD and that aged Cldn18 -/- mice have an increased propensity to develop LUAD, CLDN18.1 was assumed to have a tumour suppressor role in LUAD, and this was validated by Luo et al. They demonstrated that CLDN18.1 expression is inversely correlated with promoter methylation and with LUAD patient mortality. In addition, the restoration of CLDN18.1 expression in LUAD cells that have lost expression markedly attenuated malignant properties, including xenograft tumour growth in vivo as well as cell proliferation, migration, invasion and anchorage-independent colony formation in vitro. Further mechanistic studies have shown that CLDN18.1 attenuates malignancy by inhibiting insulin-like growth factor-1 receptor (IGF-1R)/AKT and the YAP/TAZ/AKT axes (20). Studies also found that claudin-18.1 could suppress the proliferation and motility of lung epithelial cells by inhibiting the phosphatidylinositol-3 kinase (PI3K)/pyruvate dehydrogenase kinase-1 (PDK1)/Akt signalling pathway (19, 73). In addition, downregulating the CLDN18-dependent ZO-2 expression in CLDN18/A549 cells enhanced the expression and activity of matrix metalloproteinase 2 (MMP2), resulting in the promotion of cell migration (18).
4.2.2 The tumour promoter role of CLDN18
The cancer-promoting effects of CLDN18 are mostly manifested in cancers with ectopic activation of this factor. Three distinct epithelial lesions—pancreatic intraepithelial neoplasias (PanINs), intraductal papillary mucinous neoplasms (IPMNs), and mucinous cystic neoplasms (MCNs)—are recognised as precursors of PDAC (34). CLDN18 is a marker for the early carcinogenetic process in PDAC because all three types of precursor lesions (PanIN, IPMN, and MCN) exhibit frequent immunoreactivity for CLDN18. In the oesophagus, hybrid stomach-intestinal chromatin states, including those involving CLDN18, underlie human Barrett’s metaplasia (74). The change from a CLDN18-deficient tight junction in healthy squamous epithelium from subjects without oesophageal disease to a Cldn-18-rich tight junction in specialised columnar epithelium of Barrett’s oesophagus contributes to preventing the squamous epithelium from being attacked and destroyed by luminal acid, thereby promoting tumourigenesis (15, 75). For bile duct carcinoma, it was found that the knockdown of claudin-18 expression or an antibody specific to claudin-18 can significantly attenuate the proliferation, invasion and in vivo tumourigenesis of bile duct adenocarcinoma cells. Researchers further revealed that the EGFR pathway and active RAS can induce claudin-18 expression by activating ERK1/2. Enhanced claudin-18 expression subsequently activates ERK1/2. Thus, they form a positive feedback loop, playing a role in the promotion of malignancy in the bile duct (15). These above research findings preliminarily suggested its tumour promoter role in cancers with ectopic activation of CLDN18. Nevertheless, more comprehensive, in-depth studies are required as the mechanism evidence exploration yet is shallow.
5 Research progress on drugs targeting CLDN18.2
Researchers have successively developed more specific or effective antibodies to detect CLDN18.2 expression (76, 77) or target it as a therapeutic candidate for tumours (78–82). In the field of therapies targeting Claudin18.2, there are many drugs in the clinical development stage, including monoclonal antibodies, CAR-T cells, bispecific antibodies and ADCs. Among them, several clinical studies of monoclonal antibody therapy and CAR-T therapy have disclosed clinical trial data to date. The latter two emerging drug types, ADCs and bispecific antibodies, have been approved for clinical testing in recent years, and studies are still ongoing. In contrast to previous clinical trials that only assessed the safety and efficacy of CLDN18.2-targeted therapies in populations with advanced gastric and oesophagogastric junction cancers, recently, approved clinical trials have also expanded to include patients with other advanced solid tumours, mostly tumours of the digestive system, including PC, colorectal cancer, intrahepatic cholangiocarcinoma, and extrahepatic bile duct cancer. The CLDN18.2 clinical trials with disclosed results are shown in Table 2; ongoing clinical trials of CLDN18.2 are shown in Table 3.
5.1 Monoclonal antibody therapies
In a basic study investigating the antitumour activity and mechanism of IMAB362, Tureci identified that zolbetuximab bound with high specificity and affinity to human PC cells expressing CLDN18.2 and induced antibody-dependent cellular cytotoxicity (ADCC) and complement-dependent cytotoxicity (CDC), the amplitude of which directly correlated with CLDN18.2 levels, resulting in the lysis of human PC cells. In vivo experiments also showed that zolbetuximab slowed tumour growth, improved survival, and attenuated metastasis development (90). Mechanisms of CLDN18.2-targeted monoclonal antibody intervention are showed in Figure 6.
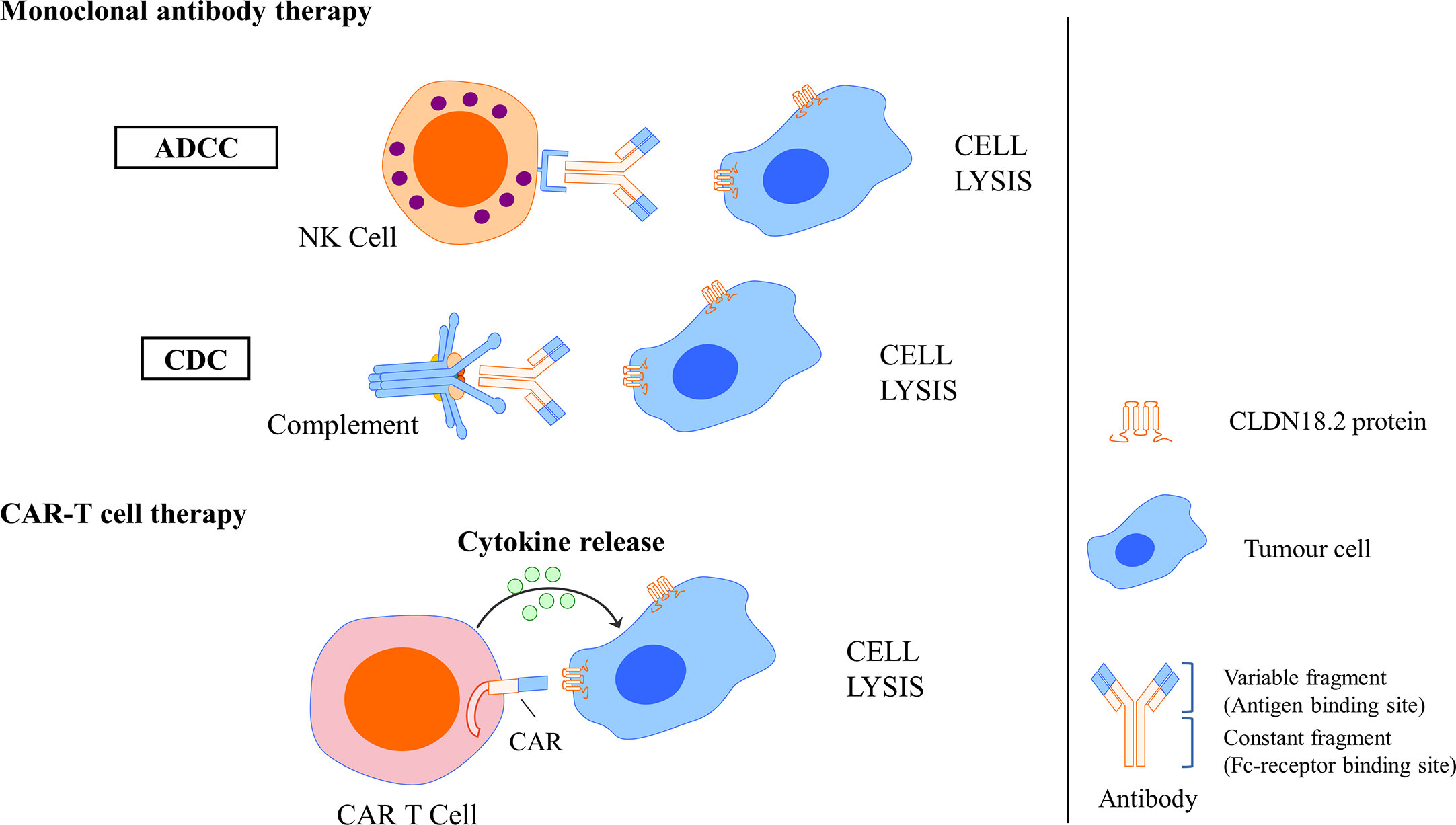
Figure 6 Mechanisms of CLDN18.2-targeted monoclonal antibody intervention and CAR T cell interaction. Monoclonal antibody could specifically bind to CLDN18.2 expressed tumour cells and induce ADCC and CDC, resulting in the cell lysis. CLDN18.2 CAR T cell could also specifically recognise CLDN18.2 expressed tumour cells and induce cytokine release, resulting in the cell lysis.
For clinical trials, to date, 5 studies that measured the efficacy and safety of the monoclonal antibody IMAB362 have disclosed data. Among them, two are phase I studies, which suggested that IMAB362 has good tolerability and safety (83, 85), and three are phase II studies indicating that IMAB362 alone and IMAB362 combined with chemotherapy are safe strategies and have antitumour activity in patients with CLDN18.2-positive advanced gastric adenocarcinoma (GA), gastroesophageal junction cancer (GEJC), and oesophageal adenocarcinoma (EAC) (84, 86, 87). In addition, compared with chemotherapy alone, the combination therapy group maintained good quality of life and low symptom burden for a longer period (91). However, there is a great difference in survival benefits among these 3 phase II studies. The overall response rate (ORR) and clinical benefit rate (CBR) of the clinical trials (NCT01197885) of IMAB362 alone were 9% and 23%, respectively (84); in the study (NCT01630083) of treatment with IMAB362 plus epirubicin, oxaliplatin and capecitabine (EOX) chemotherapy, the ORR was 29%, and the median progression-free survival (mPFS) and median overall survival (mOS) were 7.5 and 13.0 months, respectively (86); the study (NCT03505320) investigating IMAB362 + mFOLFOX6 chemotherapy showed that the ORR was 63.2% and the mPFS was 13.7 months (87). The combination of the monoclonal antibody and chemotherapy had better survival benefits than monoclonal antibody alone. The differences among the 3 studies may be affected by other factors, including the combined chemotherapy regimen, drug dose, population inclusion criteria and so on.
Apart from the antitumour activity in GC and GEJC as mentioned above, IMAB362 is also suggested as an antibody for pancreatic cancers expressing CLDN18.2. A phase 2, open-label, randomised study (NCT03816163) of a large group of 369 patients with claudin 18.2-positive, metastatic pancreatic adenocarcinoma, began in 2019 and is still ongoing (92). The purpose of this study is to assess the efficacy and safety of IMAB362 in combination with nab-paclitaxel and gemcitabine (Nab-P + GEM) as first-line treatment. The primary outcomes are dose limiting toxicity (DLT), OS and adverse events (AEs). The results of this large-scale clinical trial are worthy of public expectation. In any case, it will be instructive for selection of patients applying CLDN18.2-targed therapies.
5.2 CAR-T cell therapy
Cancer immunotherapy with CAR-T cells is a breakthrough therapy of the twenty-first century for the management of different malignancies, including lymphomas and leukaemias (93– 95). Despite the progress made in treating haematological malignancies, challenges still remain for the use of CAR-T-cell therapy to treat solid tumours due to immune effector cell-related neurotoxicity syndrome (ICANS), treatment-related death, and severe cytokine-release syndrome (CRS). Encouragingly, the efficacy and safety of CAR-T-cell treatments for solid tumours have been improved mainly through optimisation of T-cell characteristics and reversal of the immunosuppressive microenvironment (96, 97).
A basic study successfully developed humanised CLDN18.2-specific single-chain variable fragments (scFvs) specifically recognising CLDN18.2 but not CLDN18.1. Then, CLDN18.2-specific CAR T cells that comprise the CD28 costimulatory domain were generated using scFv as targeting components. In GC, an in vitro assay showed that these CLDN18.2-specific CAR T cells could efficiently produce cytokine and lyse CLDN18.2-positive cells but not CLDN18.2-negative cells, indicating that they had intrinsic target-dependent cytotoxic activity. Potent suppression of tumour growth was also observed in in vivo tumour models after CAR T-cell treatment, whether in a cancer cell line xenograft mouse model or in patient-derived xenograft (PDX) models. Researchers have also demonstrated that these CAR T cells persist well in vivo and infiltrate efficiently into tumour tissues. In addition, in an assessment of safety, CLDN18.2 CAR T cells did not induce apparent damage to normal gastric tissues or other organs. These results demonstrated that CLDN18.2-specific CAR T cells could be a promising treatment strategy for GC (78). Mechanisms of CLDN18.2-targeted CAR-T cell interaction are showed in Figure 6.
Two clinical phase I trials of CAR-T therapy have disclosed results. One study (NCT03159819) evaluated CAR-CLDN18.2 T cells in 12 subjects with CLDN18.2-positive metastatic gastric or pancreatic adenocarcinoma. The total ORR was 33.3%, and the median PFS was 130 days (88). Another study (NCT03874897) enrolled 37 patients with cancer of the digestive system, including 28 with GC, 5 with PDAC and 4 with other cancer types. The ORRs for all patients and patients with GC were 48.6% and 57.1%, respectively. The disease control rates (DCRs) for all patients and patients with GC were 73.0% and 75.0%, respectively (89). In addition, the overall ORR, median PFS and OS values for 18 patients with GC who failed at least 2 prior lines of therapy were 61.1%, 5.4 months and 9.5 months, respectively, indicating that CT041 also displayed a significantly improved efficacy compared to historical data (98). In general, CAR-CLDN18.2 T-cell therapy showed an acceptable safety profile and promising antitumour activities in patients with advanced CLDN18.2+ cancers of the digestive system, taking the lead in the global solid tumour CAR-T field.
In contrast to the previous studies that mostly enrolled postoperative tumour patients, clinical studies covering patients who are not ideal candidates for surgery are emerging, mainly for advanced solid tumours. This is conducive to the settlement of some weaknesses regarding the lack of data on the damage to target organs and other adverse reactions caused by CAR-T cells. We are looking forward to the exploration of the efficacy and adverse reactions, especially the occurrence of cytokine storms and their prevention and control measures in the context of CAR-CLDN18.2 T therapy after multiline treatment.
5.3 ADCs and bispecific antibodies
Monoclonal antibody-based targeted therapy against specific molecules has greatly improved treatment approaches for patients. However, the long-term efficacy of therapeutic antibodies is limited by resistance mechanisms (99). Bispecific antibodies (bsAbs) and ADCs are the most prominent products of engineering and modification approaches being devised and applied to the conventional immunoglobulin molecular format and can overcome the limitations of such antibodies and enhance their efficacy (100). They are being developed as innovative targeted antibody therapies that eliminate cancer by redirecting immune cells or delivering a potent cytotoxic payload to tumours (101, 102).
Zhu et al. produced bispecific compounds by combining CLDN18.2- and CD3-targeting arms through hinge mutations and produced ADCs by conjugating anti-CLDN18.2 antibody to the cleavable auristatin. They showed the anti-CLDN18.2 ADC and anti-CLDN18.2-CD3 bsAb can effectively inhibit the growth of GC (KATO-III/hCLDN18.2) and PC (BxPC3/hCLDN18.2) cells in vitro and the PDX model with high expression of CLDN18.2. In conclusion, this study offers preclinical proof-of-concept that targeting CLDN18.2 with an ADC or bispecific modality could be an effective therapy for the treatment of gastric and pancreatic cancer (79). One study conducted by Liang et al. designed anti-CLDN18.2-CD28 bsAbs by connecting two scFvs against CLDN18.2 and CD28. In vivo mouse tumour models, compared with anti-CLDN18.2, anti-CLDN18.2-anti-CD28 showed a greater increase in the percentage of CD8+ T cells and higher expression of CD69 on CD8+ T cells in tumour tissues as well as a greater reduction in tumour burden. Treatment with anti-CLDN18.2-anti-CD28 also produced more interferon-γ (IFN-γ) and tumour necrosis factor-α (TNF-α) in splenocytes and reduced the levels of immunosuppressive cells, including tumour-associated macrophages and myeloid-derived suppressor cells, in tumour tissues. Thus, it is suggested that anti-CLDN18.2-anti-CD28 could play a tumour-killing role by improving antitumour immune response and attenuating the suppressive tumour microenvironment (80). Obvious side effects or signs of toxicity after anti-CLDN18.2 ADC and anti-CLDN18.2-CD3/CD28 bispecific treatment, even in CLDN18.2-expressing gastric tissues, were not observed in the above two studies. These findings collectively suggest that therapies targeting CLDN18.2 with ADCs and bispecific antibodies were both effective and safe in mouse tumour models.
Clinical trials of ADCs and bispecific antibodies are still ongoing and have not disclosed results. AMG-910 is a CD3/CLDN18.2 bsAb and the first CLDN18.2 bsAb that entered clinical trials. A phase I study of AMG-910 (NCT04260191) is ongoing, with the aim of evaluating its safety and tolerability in patients with claudin 18.2-positive GA and GEJA and determining the maximum tolerated dose (MTD) and/or recommended phase 2 dose (RP2D). Q-1802 is a bsAb targeting CLDN18.2 and the immune checkpoint PD-L1. The ongoing phase I study of Q-1802 (NCT04856150) consists of two compartments: the dose-exploration stage and the dose-extension stage. The aim is to establish safety and tolerance of Q-1802 in patients with advanced solid tumours and to assess the pharmacokinetic characteristics and efficacy. Subsequently, several studies aiming to evaluate the safety and efficacy of other ADCs or bsAbs, including CMG-901 SYSA1801, LM-302 and RC118, for the treatment of GC, GEJC and PC, are also still in phase I.
6 Conclusions and perspectives
CLDN18.2 is restricted to normal gastric tissue and is downregulated in GC tissues but is highly activated in other tumour tissues, including pancreatic, oesophageal, biliary tract, ovarian, and lung tumours. In addition, CLDN18.2 still has high expression during gastric tumour formation and retained expression in metastatic lesions. Therefore, CLDN18.2 is suggested as an ideal target for tumour treatment, especially in cancers of the GI tract. Moreover, CLDN18.2 can act as an oncogene or a tumour suppressor gene in different tumour types, and its expression is modulated by the methylation status of CpG islands, PKC, ERK/MAPK, HER2/HER3 signalling pathways and microRNA.
From the disclosed data, monoclonal antibody and CAR-T therapies have shown good safety in CLDN18.2-positive gastric and pancreatic cancer populations and have achieved encouraging preliminary results in terms of clinical efficacy. In addition, we are very much looking forward to encouraging the results of ongoing clinical trials of long-researched or emerging drugs targeting CLDN18.2 in various tumours, which may provide several new potential methods and benefits for millions of people with CLDN18.2-positive tumours, including GC, PC, hepatocellular carcinoma, and bile duct cancer. Further attention should be given to the definition of populations with high CLDN18.2 expression that may benefit from treatment, the prediction of marker efficacy, the prevention and control measures for adverse reactions, the selection of treatment regimens for multitarget coexpression populations, and the combination of immunotherapy, which are all directions for future exploration. Subsequent clinical trials of CAR-T cells, ADCs, and bsAb drugs will undoubtedly provide more options for patients with high CLDN18.2 expression and poor efficacy with targeted drugs or advanced multiline treatment.
Author contributions
XC and LY conceptualised the manuscript. JC, ZX, CH, SZ, and MZ collected the literature, JC and ZX collected the literature, wrote the manuscript and made the figures. XC and LY edited and made significant revisions to the manuscript. All authors contributed to the article and approved the submitted version.
Funding
This study was supported by National Natural Science Foundation of China (82074245, 81973634), Natural Science Foundation of Zhejiang Province (HDMY22H160008), Medical Science and Technology Project of Zhejiang Province (2022KY114, WKJ-ZJ-2104), Chinese Postdoctoral Science Foundation (2022M713203), Program of Zhejiang Provincial TCM Sci-tech Plan (2022ZQ020), Zhejiang Provincial Research Center for Upper Gastrointestinal Tract Cancer (JBZX-202006).
Conflict of interest
The authors declare that the research was conducted in the absence of any commercial or financial relationships that could be construed as a potential conflict of interest.
Publisher’s note
All claims expressed in this article are solely those of the authors and do not necessarily represent those of their affiliated organizations, or those of the publisher, the editors and the reviewers. Any product that may be evaluated in this article, or claim that may be made by its manufacturer, is not guaranteed or endorsed by the publisher.
References
1. Arnold M, Abnet CC, Neale RE, Vignat J, Giovannucci EL, Mcglynn KA, et al. Global burden of 5 major types of gastrointestinal cancer. Gastroenterology (2020) 159:335–49.e15. doi: 10.1053/j.gastro.2020.02.068
2. Smyth EC, Moehler M. Late-line treatment in metastatic gastric cancer: today and tomorrow. Ther Adv Med Oncol (2019) 11:1758835919867522. doi: 10.1177/1758835919867522
3. Yuan L, Xu ZY, Ruan SM, Mo S, Qin JJ, Cheng XD. Long non-coding RNAs towards precision medicine in gastric cancer: Early diagnosis, treatment, and drug resistance. Mol Cancer (2020) 19:96. doi: 10.1186/s12943-020-01219-0
4. Biagioni A, Skalamera I, Peri S, Schiavone N, Cianchi F, Giommoni E, et al. Update on gastric cancer treatments and gene therapies. Cancer Metastasis Rev (2019) 38:537–48. doi: 10.1007/s10555-019-09803-7
5. Wang DK, Zuo Q, He QY, Li B. Targeted immunotherapies in gastrointestinal cancer: From molecular mechanisms to implications. Front Immunol (2021) 12:2021.705999. doi: 10.3389/fimmu.2021.705999
6. Singh P, Toom S, Huang Y. Anti-claudin 18.2 antibody as new targeted therapy for advanced gastric cancer. J Hematol Oncol (2017) 10:105. doi: 10.1186/s13045-017-0473-4
7. Furuse M, Fujita K, Hiiragi T, Fujimoto K, Tsukita S. Claudin-1 and -2: novel integral membrane proteins localizing at tight junctions with no sequence similarity to occludin. J Cell Biol (1998) 141:1539–50. doi: 10.1083/jcb.141.7.1539
8. Lafemina MJ, Sutherland KM, Bentley T, Gonzales LW, Allen L, Chapin CJ, et al. Claudin-18 deficiency results in alveolar barrier dysfunction and impaired alveologenesis in mice. Am J Respir Cell Mol Biol (2014) 51:550–8. doi: 10.1165/rcmb.2013-0456OC
9. Li G, Flodby P, Luo J, Kage H, Sipos A, Gao D, et al. Knockout mice reveal key roles for claudin 18 in alveolar barrier properties and fluid homeostasis. Am J Respir Cell Mol Biol (2014) 51:210–22. doi: 10.1165/rcmb.2013-0353OC
10. Hashimoto I, Oshima T. Claudins and gastric cancer: An overview. Cancers (Basel) (2022) 14(4):290. doi: 10.3390/cancers14020290
11. Hayashi D, Tamura A, Tanaka H, Yamazaki Y, Watanabe S, Suzuki K, et al. Deficiency of claudin-18 causes paracellular h+ leakage, up-regulation of interleukin-1beta, and atrophic gastritis in mice. Gastroenterology (2012) 142:292–304. doi: 10.1053/j.gastro.2011.10.040
12. Tureci O, Koslowski M, Helftenbein G, Castle J, Rohde C, Dhaene K, et al. Claudin-18 gene structure, regulation, and expression is evolutionary conserved in mammals. Gene (2011) 481:83–92. doi: 10.1016/j.gene.2011.04.007
13. Sato K, Matsumoto I, Suzuki K, Tamura A, Shiraishi A, Kiyonari H, et al. Deficiency of lung-specific claudin-18 leads to aggravated infection with cryptococcus deneoformans through dysregulation of the microenvironment in lungs. Sci Rep (2021) 11:21110. doi: 10.1038/s41598-021-00708-6
14. Oshima T, Shan J, Okugawa T, Chen X, Hori K, Tomita T, et al. Down-regulation of claudin-18 is associated with the proliferative and invasive potential of gastric cancer at the invasive front. PloS One (2013) 8:e74757. doi: 10.1371/journal.pone.0074757
15. Takasawa K, Takasawa A, Osanai M, Aoyama T, Ono Y, Kono T, et al. Claudin-18 coupled with EGFR/ERK signaling contributes to the malignant potentials of bile duct cancer. Cancer Lett (2017) 403:66–73. doi: 10.1016/j.canlet.2017.05.033
16. Hagen SJ, Ang LH, Zheng Y, Karahan SN, Wu J, Wang YE, et al. Loss of tight junction protein claudin 18 promotes progressive neoplasia development in mouse stomach. Gastroenterology (2018) 155:1852–67. doi: 10.1053/j.gastro.2018.08.041
17. Zhou B, Flodby P, Luo J, Castillo DR, Liu Y, Yu FX, et al. Claudin-18-mediated YAP activity regulates lung stem and progenitor cell homeostasis and tumorigenesis. J Clin Invest (2018) 128:970–84. doi: 10.1172/JCI90429
18. Akizuki R, Eguchi H, Endo S, Matsunaga T, Ikari A. ZO-2 suppresses cell migration mediated by a reduction in matrix metalloproteinase 2 in claudin-18-Expressing lung adenocarcinoma A549 cells. Biol Pharm Bull (2019) 42:247–54. doi: 10.1248/bpb.b18-00670
19. Shimobaba S, Taga S, Akizuki R, Hichino A, Endo S, Matsunaga T, et al. Claudin-18 inhibits cell proliferation and motility mediated by inhibition of phosphorylation of PDK1 and akt in human lung adenocarcinoma A549 cells. Biochim Biophys Acta (2016) 1863:1170–8. doi: 10.1016/j.bbamcr.2016.02.015
20. Luo J, Chimge NO, Zhou B, Flodby P, Castaldi A, Firth AL, et al. CLDN18.1 attenuates malignancy and related signaling pathways of lung adenocarcinoma in vivo and in vitro. Int J Cancer (2018) 143:3169–80. doi: 10.1002/ijc.31734
21. Cancer Genome Atlas Research, N. Comprehensive molecular characterization of gastric adenocarcinoma. Nature (2014) 513(7517):202–9. doi: 10.1038/nature13480
22. Zhang WH, Zhang SY, Hou QQ, Qin Y, Chen XZ, Zhou ZG, et al. The significance of the CLDN18-ARHGAP fusion gene in gastric cancer: A systematic review and meta-analysis. Front Oncol (2020) 10:1214. doi: 10.3389/fonc.2020.01214
23. Usui G, Matsusaka K, Mano Y, Urabe M, Funata S, Fukayama M, et al. DNA Methylation and genetic aberrations in gastric cancer. Digestion (2021) 102:25–32. doi: 10.1159/000511243
24. Ushiku T, Ishikawa S, Kakiuchi M, Tanaka A, Katoh H, Aburatani H, et al. RHOA mutation in diffuse-type gastric cancer: a comparative clinicopathology analysis of 87 cases. Gastric Cancer (2016) 19:403–11. doi: 10.1007/s10120-015-0493-0
25. Baek JH, Park DJ, Kim GY, Cheon J, Kang BW, Cha HJ, et al. Clinical implications of Claudin18.2 expression in patients with gastric cancer. Anticancer Res (2019) 39:6973–9. doi: 10.21873/anticanres.13919
26. Rohde C, Yamaguchi R, Mukhina S, Sahin U, Itoh K, Tureci O. Comparison of claudin 18.2 expression in primary tumors and lymph node metastases in Japanese patients with gastric adenocarcinoma. Jpn J Clin Oncol (2019) 49:870–6. doi: 10.1093/jjco/hyz068
27. Jun KH, Kim JH, Jung JH, Choi HJ, Chin HM. Expression of claudin-7 and loss of claudin-18 correlate with poor prognosis in gastric cancer. Int J Surg (2014) 12:156–62. doi: 10.1016/j.ijsu.2013.11.022
28. Dottermusch M, Kruger S, Behrens HM, Halske C, Rocken C. Expression of the potential therapeutic target claudin-18.2 is frequently decreased in gastric cancer: results from a large Caucasian cohort study. Virchows Arch (2019) 475:563–71. doi: 10.1007/s00428-019-02624-7
29. Arnold A, Daum S, Von Winterfeld M, Berg E, Hummel M, Rau B, et al. Prognostic impact of claudin 18.2 in gastric and esophageal adenocarcinomas. Clin Transl Oncol (2020) 22:2357–63. doi: 10.1007/s12094-020-02380-0
30. Coati I, Lotz G, Fanelli GN, Brignola S, Lanza C, Cappellesso R, et al. Claudin-18 expression in oesophagogastric adenocarcinomas: a tissue microarray study of 523 molecularly profiled cases. Br J Cancer (2019) 121:257–63. doi: 10.1038/s41416-019-0508-4
31. Pellino A, Brignola S, Riello E, Niero M, Murgioni S, Guido M, et al. Association of CLDN18 protein expression with clinicopathological features and prognosis in advanced gastric and gastroesophageal junction adenocarcinomas. J Pers Med (2021) 11:1095. doi: 10.3390/jpm11111095
32. Pereira MA, Ramos M, Dias AR, Cardili L, Ribeiro RRE, De Castria TB, et al. RhoA, claudin 18, and c-MET in gastric cancer: Clinicopathological characteristics and prognostic significance in curative resected patients. Med Sci (Basel) (2021) 10(1):4. doi: 10.3390/medsci10010004
33. Woll S, Schlitter AM, Dhaene K, Roller M, Esposito I, Sahin U, et al. Claudin 18.2 is a target for IMAB362 antibody in pancreatic neoplasms. Int J Cancer (2014) 134:731–9. doi: 10.1002/ijc.28400
34. Tanaka M, Shibahara J, Fukushima N, Shinozaki A, Umeda M, Ishikawa S, et al. Claudin-18 is an early-stage marker of pancreatic carcinogenesis. J Histochem Cytochem (2011) 59:942–52. doi: 10.1369/0022155411420569
35. Soini Y, Takasawa A, Eskelinen M, Juvonen P, Karja V, Hasegawa T, et al. Expression of claudins 7 and 18 in pancreatic ductal adenocarcinoma: association with features of differentiation. J Clin Pathol (2012) 65:431–6. doi: 10.1136/jclinpath-2011-200400
36. Wang X, Zhang CS, Dong XY, Hu Y, Duan BJ, Bai J, et al. Claudin 18.2 is a potential therapeutic target for zolbetuximab in pancreatic ductal adenocarcinoma. World J Gastrointest Oncol (2022) 14:1252–64. doi: 10.4251/wjgo.v14.i7.1252
37. Zhang Z, Liu X, Zhou L, Zhang M, Liang Z. Investigation of clinical application of claudin 18 isoform 2 in pancreatic ductal adenocarcinoma: A retrospective analysis of 302 chinese patients. Histol Histopathol (2022) 37(10):1031–40. doi: 10.14670/HH-18-477
38. Shinozaki A, Shibahara J, Noda N, Tanaka M, Aoki T, Kokudo N, et al. Claudin-18 in biliary neoplasms. its significance in the classification of intrahepatic cholangiocarcinoma. Virchows Arch (2011) 459:73–80. doi: 10.1007/s00428-011-1092-z
39. Iwaya M, Hayashi H, Nakajima T, Matsuda K, Kinugawa Y, Tobe Y, et al. Colitis-associated colorectal adenocarcinomas frequently express claudin 18 isoform 2: implications for claudin 18.2 monoclonal antibody therapy. Histopathology (2021) 79:227–37. doi: 10.1111/his.14358
40. Xu B, Liu F, Liu Q, Shi T, Wang Z, Wu N, et al. Highly expressed Claudin18.2 as a potential therapeutic target in advanced gastric signet-ring cell carcinoma (SRCC). J Gastrointest Oncol (2020) 11:1431–9. doi: 10.21037/jgo-20-344
41. Sanada Y, Oue N, Mitani Y, Yoshida K, Nakayama H, Yasui W. Down-regulation of the claudin-18 gene, identified through serial analysis of gene expression data analysis, in gastric cancer with an intestinal phenotype. J Pathol (2006) 208:633–42. doi: 10.1002/path.1922
42. Fan L, Chong X, Zhao M, Jia F, Wang Z, Zhou Y, et al. Ultrasensitive gastric cancer circulating tumor cellular CLDN18.2 RNA detection based on a molecular beacon. Anal Chem (2021) 93:665–70. doi: 10.1021/acs.analchem.0c04055
43. Sahin U, Koslowski M, Dhaene K, Usener D, Brandenburg G, Seitz G, et al. Claudin-18 splice variant 2 is a pan-cancer target suitable for therapeutic antibody development. Clin Cancer Res (2008) 14:7624–34. doi: 10.1158/1078-0432.CCR-08-1547
44. Espinoza JA, Riquelme I, Sagredo EA, Rosa L, Garcia P, Bizama C, et al. Mucin 5B, carbonic anhydrase 9 and claudin 18 are potential theranostic markers of gallbladder carcinoma. Histopathology (2019) 74:597–607. doi: 10.1111/his.13797
45. Li J, Zhang Y, Hu D, Gong T, Xu R, Gao J. Analysis of the expression and genetic alteration of CLDN18 in gastric cancer. Aging (Albany NY) (2020) 12:14271–84. doi: 10.18632/aging.103457
46. Oue N, Sentani K, Sakamoto N, Yasui W. Clinicopathologic and molecular characteristics of gastric cancer showing gastric and intestinal mucin phenotype. Cancer Sci (2015) 106:951–8. doi: 10.1111/cas.12706
47. Asaka S, Nakajima T, Ida K, Asaka R, Kobayashi C, Ito M, et al. Clinicopathological and prognostic significance of immunophenotypic characterization of endocervical adenocarcinoma using CLDN18, CDH17, and PAX8 in association with HPV status. Virchows Arch (2022) 480:269–80. doi: 10.1007/s00428-021-03207-1
48. Yan HHN, Siu HC, Law S, Ho SL, Yue SSK, Tsui WY, et al. A comprehensive human gastric cancer organoid biobank captures tumor subtype heterogeneity and enables therapeutic screening. Cell Stem Cell (2018) 23:882–897.e11. doi: 10.1016/j.stem.2018.09.016
49. Tanaka A, Ishikawa S, Ushiku T, Yamazawa S, Katoh H, Hayashi A, et al. Frequent CLDN18-ARHGAP fusion in highly metastatic diffuse-type gastric cancer with relatively early onset. Oncotarget (2018) 9:29336–50. doi: 10.18632/oncotarget.25464
50. Hashimoto T, Ogawa R, Tang TY, Yoshida H, Taniguchi H, Katai H, et al. RHOA mutations and CLDN18-ARHGAP fusions in intestinal-type adenocarcinoma with anastomosing glands of the stomach. Mod Pathol (2019) 32:568–75. doi: 10.1038/s41379-018-0181-9
51. Shu Y, Zhang W, Hou Q, Zhao L, Zhang S, Zhou J, et al. Prognostic significance of frequent CLDN18-ARHGAP26/6 fusion in gastric signet-ring cell cancer. Nat Commun (2018) 9:2447. doi: 10.1038/s41467-018-04907-0
52. Matsusaka K, Ushiku T, Urabe M, Fukuyo M, Abe H, Ishikawa S, et al. Coupling CDH17 and CLDN18 markers for comprehensive membrane-targeted detection of human gastric cancer. Oncotarget (2016) 7:64168–81. doi: 10.18632/oncotarget.11638
53. Nakayama I, Shinozaki E, Sakata S, Yamamoto N, Fujisaki J, Muramatsu Y, et al. Enrichment of CLDN18-ARHGAP fusion gene in gastric cancers in young adults. Cancer Sci (2019) 110:1352–63. doi: 10.1111/cas.13967
54. Yao F, Kausalya JP, Sia YY, Teo AS, Lee WH, Ong AG, et al. Recurrent fusion genes in gastric cancer: CLDN18-ARHGAP26 induces loss of epithelial integrity. Cell Rep (2015) 12:272–85. doi: 10.1016/j.celrep.2015.06.020
55. Yano K, Imaeda T, Niimi T. Transcriptional activation of the human claudin-18 gene promoter through two AP-1 motifs in PMA-stimulated MKN45 gastric cancer cells. Am J Physiol Gastrointest Liver Physiol (2008) 294:G336–43. doi: 10.1152/ajpgi.00328.2007
56. Li T, Liu X, Riederer B, Nikolovska K, Singh AK, Makela KA, et al. Genetic ablation of carbonic anhydrase IX disrupts gastric barrier function via claudin-18 downregulation and acid backflux. Acta Physiol (Oxf) (2018) 222:e12923. doi: 10.1111/apha.12923
57. Shen CH, Lin JY, Lu CY, Yang SS, Peng CK, Huang KL. SPAK-p38 MAPK signal pathway modulates claudin-18 and barrier function of alveolar epithelium after hyperoxic exposure. BMC Pulm Med (2021) 21:58. doi: 10.1186/s12890-021-01408-7
58. Ma X, Yu X, Zhou Q. The IL1beta-HER2-CLDN18/CLDN4 axis mediates lung barrier damage in ARDS. Aging (Albany NY) (2020) 12:3249–65. doi: 10.18632/aging.102804
59. Srisodsai A, Kurotani R, Chiba Y, Sheikh F, Young HA, Donnelly RP, et al. Interleukin-10 induces uteroglobin-related protein (UGRP) 1 gene expression in lung epithelial cells through homeodomain transcription factor T/EBP/NKX2.1. J Biol Chem (2004) 279:54358–68. doi: 10.1074/jbc.M405331200
60. Niimi T, Nagashima K, Ward JM, Minoo P, Zimonjic DB, Popescu NC, et al. Claudin-18, a novel downstream target gene for the T/EBP/NKX2.1 homeodomain transcription factor, encodes lung- and stomach-specific isoforms through alternative splicing. Mol Cell Biol (2001) 21:7380–90. doi: 10.1128/MCB.21.21.7380-7390.2001
61. Isakov N. Protein kinase c (PKC) isoforms in cancer, tumor promotion and tumor suppression. Semin Cancer Biol (2018) 48:36–52. doi: 10.1016/j.semcancer.2017.04.012
62. Ito T, Kojima T, Yamaguchi H, Kyuno D, Kimura Y, Imamura M, et al. Transcriptional regulation of claudin-18 via specific protein kinase c signaling pathways and modification of DNA methylation in human pancreatic cancer cells. J Cell Biochem (2011) 112:1761–72. doi: 10.1002/jcb.23095
63. Yarden Y, Sliwkowski MX. Untangling the ErbB signalling network. Nat Rev Mol Cell Biol (2001) 2:127–37. doi: 10.1038/35052073
64. Galdy S, Lamarca A, Mcnamara MG, Hubner RA, Cella CA, Fazio N, et al. HER2/HER3 pathway in biliary tract malignancies; systematic review and meta-analysis: a potential therapeutic target? Cancer Metastasis Rev (2017) 36:141–57. doi: 10.1007/s10555-016-9645-x
65. Miricescu D, Totan A, Stanescu S, Ii B, Stefani C, Greabu M. PI3K/AKT/mTOR signaling pathway in breast cancer: From molecular landscape to clinical aspects. Int J Mol Sci (2020) 22(1):173. doi: 10.3390/ijms22010173
66. Finigan JH, Faress JA, Wilkinson E, Mishra RS, Nethery DE, Wyler D, et al. Neuregulin-1-human epidermal receptor-2 signaling is a central regulator of pulmonary epithelial permeability and acute lung injury. J Biol Chem (2011) 286:10660–70. doi: 10.1074/jbc.M110.208041
67. Krol J, Loedige I, Filipowicz W. The widespread regulation of microRNA biogenesis, function and decay. Nat Rev Genet (2010) 11:597–610. doi: 10.1038/nrg2843
68. Lu TX, Rothenberg ME. MicroRNA. J Allergy Clin Immunol (2018) 141:1202–7. doi: 10.1016/j.jaci.2017.08.034
69. Ying SY, Chang DC, Lin SL. The MicroRNA. Methods Mol Biol (2013) 936:1–19. doi: 10.1007/978-1-62703-083-0_1
70. Zhang SJ, Feng JF, Wang L, Guo W, Du YW, Ming L, et al. miR-1303 targets claudin-18 gene to modulate proliferation and invasion of gastric cancer cells. Dig Dis Sci (2014) 59:1754–63. doi: 10.1007/s10620-014-3107-5
71. Wan YL, Dai HJ, Liu W, Ma HT. miR-767-3p inhibits growth and migration of lung adenocarcinoma cells by regulating CLDN18. Oncol Res (2018) 26:637–44. doi: 10.3727/096504017X15112639918174
72. Kotton DN. Claudin-18: unexpected regulator of lung alveolar epithelial cell proliferation. J Clin Invest (2018) 128:903–5. doi: 10.1172/JCI99799
73. Akizuki R, Shimobaba S, Matsunaga T, Endo S, Ikari A. Claudin-5, -7, and -18 suppress proliferation mediated by inhibition of phosphorylation of akt in human lung squamous cell carcinoma. Biochim Biophys Acta Mol Cell Res (2017) 1864:293–302. doi: 10.1016/j.bbamcr.2016.11.018
74. Singh H, Ha K, Hornick JL, Madha S, Cejas P, Jajoo K, et al. Hybrid stomach-intestinal chromatin states underlie human barrett's metaplasia. Gastroenterology (2021) 161:924–39:e11. doi: 10.1053/j.gastro.2021.05.057
75. Jovov B, Itallie V, Shaheen NJ, Carson JL, Gambling TM, Anderson JM, et al. Claudin-18: a dominant tight junction protein in barrett's esophagus and likely contributor to its acid resistance. Am J Physiol Gastrointest Liver Physiol (2007) 293:G1106–13. doi: 10.1152/ajpgi.00158.2007
76. Hu G, Zhu W, Liu Y, Wang Y, Zhang Z, Zhu S, et al. Development and comparison of three (89)Zr-labeled anti-CLDN18.2 antibodies to noninvasively evaluate CLDN18.2 expression in gastric cancer: a preclinical study. Eur J Nucl Med Mol Imaging (2022) 49:2634–44. doi: 10.1007/s00259-022-05739-3
77. Zhao C, Rong Z, Ding J, Wang L, Wang B, Ding L, et al. Targeting claudin 18.2 using a highly specific antibody enables cancer diagnosis and guided surgery. Mol Pharm (2022) 19:3530–41. doi: 10.1021/acs.molpharmaceut.1c00947
78. Jiang H, Shi Z, Wang P, Wang C, Yang L, Du G, et al. Claudin18.2-specific chimeric antigen receptor engineered T cells for the treatment of gastric cancer. J Natl Cancer Inst (2019) 111:409–18. doi: 10.1093/jnci/djy134
79. Zhu G, Foletti D, Liu X, Ding S, Melton Witt J, Hasa-Moreno A, et al. Targeting CLDN18.2 by CD3 bispecific and ADC modalities for the treatments of gastric and pancreatic cancer. Sci Rep (2019) 9:8420. doi: 10.1038/s41598-019-44874-0
80. Liang J, Zhang H, Huang Y, Fan L, Li F, Li M, et al. A CLDN18.2-targeting bispecific T cell Co-stimulatory activator for cancer immunotherapy. Cancer Manag Res (2021) 13:6977–87. doi: 10.2147/CMAR.S330637
81. Xu G, Qian N, Liu Y, Li H, Yang C, Wang J, et al. Preclinical characterization of a fab-like CD3/CLDN18.2 XFab(R) bispecific antibody against solid tumors. Immunobiology (2022) 227:152283. doi: 10.1016/j.imbio.2022.152283
82. Zhong W, Lu Y, Ma Z, He Y, Ding Y, Yao G, et al. Development of a humanized VHH based recombinant antibody targeting claudin 18.2 positive cancers. Front Immunol (2022) 13:2022.885424. doi: 10.3389/fimmu.2022.885424
83. Sahin U, Schuler M, Richly H, Bauer S, Krilova A, Dechow T, et al. A phase I dose-escalation study of IMAB362 (Zolbetuximab) in patients with advanced gastric and gastro-oesophageal junction cancer. Eur J Cancer (2018) 100:17–26. doi: 10.1016/j.ejca.2018.05.007
84. Tureci O, Sahin U, Schulze-Bergkamen H, Zvirbule Z, Lordick F, Koeberle D, et al. A multicentre, phase IIa study of zolbetuximab as a single agent in patients with recurrent or refractory advanced adenocarcinoma of the stomach or lower oesophagus: the MONO study. Ann Oncol (2019) 30:1487–95. doi: 10.1093/annonc/mdz199
85. Sahin U, Al.-Batran. SE, Hozaeel W, Zvirbule Z, Freiberg.-Richter. J, Lordick F, et al. IMAB362 plus zoledronic acid (ZA) and interleukin2 (IL2) in patients (pts) with advanced gastroesophageal cancer (GEC): Clinical activity and safety data from the PILOT phase I trial. J Clin Oncol (2015) 33:15.
86. Sahin U, Tureci O, Manikhas G, Lordick F, Rusyn A, Vynnychenko I, et al. FAST: a randomised phase II study of zolbetuximab (IMAB362) plus EOX versus EOX alone for first-line treatment of advanced CLDN18.2-positive gastric and gastro-oesophageal adenocarcinoma. Ann Oncol (2021) 32:609–19. doi: 10.1016/j.annonc.2021.02.005
87. Klempner SJ, Lee K-W, Metges J-P, Catenacci DVT, Loupakis F, Ilson DH, et al. Phase 2 study of zolbetuximab plus mFOLFOX6 in claudin 18.2- positive locally advanced or metastatic gastric or gastroesophageal junction adenocarcinoma (G/GEJ): ILUSTRO cohort 2. J Clin Oncol (2021) 39:15. doi: 10.1200/JCO.2021.39.15_suppl.e16063
88. Zhan X, Wang B, Li Z, Li J, Wang H, Chen L, et al. Phase I trial of claudin 18.2-specific chimeric antigen receptor T cells for advanced gastric and pancreatic adenocarcinoma. J Clin Oncol (2019) 37:Supplement 15. doi: 10.1200/JCO.2019.37.15_suppl.2509
89. Qi C, Gong J, Li J, Liu D, Qin Y, Ge S, et al. Claudin18.2-specific CAR T cells in gastrointestinal cancers: phase 1 trial interim results. Nat Med (2022) 28:1189–98. doi: 10.1038/s41591-022-01800-8
90. Tureci, Mitnacht-Kraus R, Woll S, Yamada T, Sahin U. Characterization of zolbetuximab in pancreatic cancer models. Oncoimmunology (2019) 8:e1523096. doi: 10.1080/2162402X.2018.1523096
91. Lordick F, Al-Batran SE, Ganguli A, Morlock R, Sahin U, Tureci O. Patient-reported outcomes from the phase II FAST trial of zolbetuximab plus EOX compared to EOX alone as first-line treatment of patients with metastatic CLDN18.2+ gastroesophageal adenocarcinoma. Gastric Cancer (2021) 24:721–30. doi: 10.1007/s10120-020-01153-6
92. Park W, O'reilly EM, Furuse J, Li C-P, Oh D-Y, Garcia-Carbonero R, et al. Zolbetuximab plus gemcitabine and nab-paclitaxel (GN) in first-line treatment of claudin 18.2–positive metastatic pancreatic cancer (mPC): Phase 2, open-label, randomized study. J Clin Oncol (2023) 41:TPS782–2. doi: 10.1200/JCO.2023.41.4_suppl.TPS782
93. Kalos M, Levine BL, Porter DL, Katz S, Grupp SA, Bagg A, et al. T Cells with chimeric antigen receptors have potent antitumor effects and can establish memory in patients with advanced leukemia. Sci Transl Med (2011) 3:95ra73. doi: 10.1126/scitranslmed.3002842
94. Porter DL, Levine BL, Kalos M, Bagg A, June CH. Chimeric antigen receptor-modified T cells in chronic lymphoid leukemia. N Engl J Med (2011) 365:725–33. doi: 10.1056/NEJMoa1103849
95. Nair R, Westin J. CAR T-cells. Adv Exp Med Biol (2020) 1244:215–33. doi: 10.1007/978-3-030-41008-7_10
96. Ma S, Li X, Wang X, Cheng L, Li Z, Zhang C, et al. Current progress in CAR-T cell therapy for solid tumors. Int J Biol Sci (2019) 15:2548–60. doi: 10.7150/ijbs.34213
97. Sterner RC, Sterner RM. CAR-T cell therapy: current limitations and potential strategies. Blood Cancer J (2021) 11(4):69. doi: 10.1038/s41408-021-00459-7
98. Qi C, Qin Y, Liu D, Gong J, Ge S, Zhang M, et al. 1372O CLDN 18.2-targeted CAR-T cell therapy in patients with cancers of the digestive system. Ann Oncol (2021) 32:S1040. doi: 10.1016/j.annonc.2021.08.1481
99. Thakur A, Huang M, Lum LG. Bispecific antibody based therapeutics: Strengths and challenges. Blood Rev (2018) 32:339–47. doi: 10.1016/j.blre.2018.02.004
100. Shim H. Bispecific antibodies and antibody-drug conjugates for cancer therapy: Technological considerations. Biomolecules (2020) 10(3):360. doi: 10.3390/biom10030360
101. Labrijn AF, Janmaat ML, Reichert JM, Parren P. Bispecific antibodies: a mechanistic review of the pipeline. Nat Rev Drug Discov (2019) 18:585–608. doi: 10.1038/s41573-019-0028-1
Keywords: Claudin-18.2, gastrointestinal (GI) tract, monoclonal antibody, antibody–drug conjugate (ADC), chimeric antigen receptor T (CAR-T)
Citation: Chen J, Xu Z, Hu C, Zhang S, Zi M, Yuan L and Cheng X (2023) Targeting CLDN18.2 in cancers of the gastrointestinal tract: New drugs and new indications. Front. Oncol. 13:1132319. doi: 10.3389/fonc.2023.1132319
Received: 30 December 2022; Accepted: 27 February 2023;
Published: 10 March 2023.
Edited by:
Hugo de Jonge, University of Pavia, ItalyReviewed by:
Wungki Park, Memorial Sloan Kettering Cancer Center, United StatesJenny Paredes, Memorial Sloan Kettering Cancer Center, United States
Yasutoshi Kimura, Sapporo Medical University, Japan
Copyright © 2023 Chen, Xu, Hu, Zhang, Zi, Yuan and Cheng. This is an open-access article distributed under the terms of the Creative Commons Attribution License (CC BY). The use, distribution or reproduction in other forums is permitted, provided the original author(s) and the copyright owner(s) are credited and that the original publication in this journal is cited, in accordance with accepted academic practice. No use, distribution or reproduction is permitted which does not comply with these terms.
*Correspondence: Li Yuan, eXVhbmxpMjc2OEB6amNjLm9yZy5jbg==; Xiangdong Cheng, Y2hlbmd4ZEB6amNjLm9yZy5jbg==
†These authors have contributed equally to this work and share first authorship