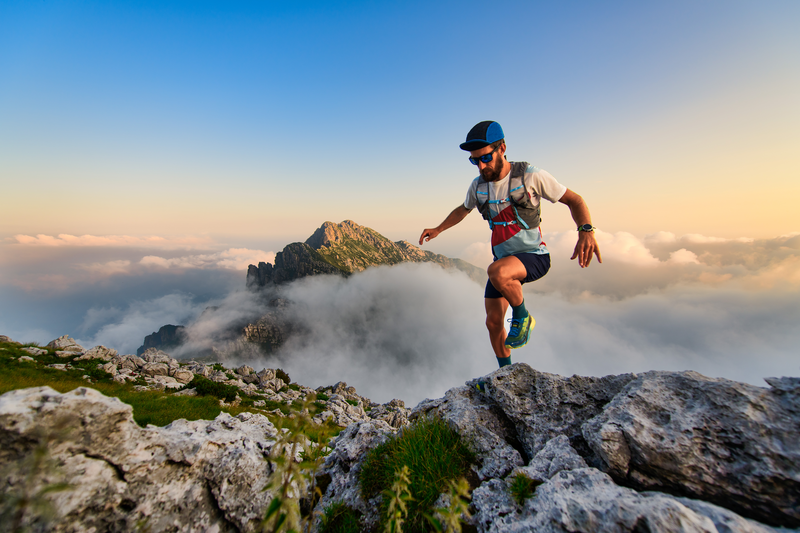
94% of researchers rate our articles as excellent or good
Learn more about the work of our research integrity team to safeguard the quality of each article we publish.
Find out more
REVIEW article
Front. Oncol. , 27 January 2023
Sec. Cancer Epidemiology and Prevention
Volume 13 - 2023 | https://doi.org/10.3389/fonc.2023.1123776
This article is part of the Research Topic Prognostic Research and Precision Oncology in Upper Tract Urothelial Carcinoma-Volume II View all 5 articles
Urothelial carcinoma (UC), which includes urinary bladder urothelial carcinoma (UBUC) and upper tract urothelial carcinoma (UTUC), is one of the most common malignancies worldwide. Accordingly, a comprehensive understanding of the underlying mechanism governing UC development is compulsory. Aberrant CCAAT/enhancer-binding protein delta (CEBPD), a transcription factor, displays an oncogene or tumor suppressor depending on tumor type and microenvironments. However, CEBPD has been reported to possess a clear oncogenic function in UC through multiple regulation pathways. Genomic amplification of CEBPD triggered by MYC-driven genome instability is frequently examined in UC that drives CEBPD overexpression. Upregulated CEBPD transcriptionally suppresses FBXW7 to stabilize MYC protein and further induces hexokinase II (HK2)-related aerobic glycolysis that fuels cell growth. Apart from the MYC-dependent pathway, CEBPD also downregulates the level of hsa-miR-429 to enhance HK2-associated glycolysis and induce angiogenesis driven by vascular endothelial growth factor A (VEGFA). Additionally, aggressive UC is attributed to the tumor metastasis regulated by CEBPD-induced matrix metalloproteinase-2 (MMP2) overexpression. Furthermore, elevated CEBPD induced by cisplatin (CDDP) is identified to have dual functions, namely, CDDP-induced chemotherapy resistance or drive CDDP-induced antitumorigenesis. Given that the role of CEBPD in UC is getting clear but pending a more systemic reappraisal, this review aimed to comprehensively discuss the underlying mechanism of CEBPD in UC tumorigenesis.
Urothelial carcinoma (UC), also referred to as transitional cell carcinoma, is one of the most common malignancies worldwide. The majority of UC, approximately 90%–95%, resides in the bladder organ called urinary bladder urothelial carcinoma (UBUC). In 2020, UBUC was ranked as the 10th most frequently diagnosed cancer with 573,000 new cases and 213,000 new deaths around the world estimated by the World Health Organization (WHO) (1). Men significantly have four times higher risk compared with women to develop UBUC (2). Another relatively rare type of UC called upper tract urothelial carcinoma (UTUC) that accounts for 5%–10% of UC is located in the renal pelvis and ureter (3). The annual incidence of UTUC ranges from 1.2 to 4.7 cases per 100,000 inhabitants and also frequently occurs in men globally (4). In contrast, UTUC has an unusually high incidence that accounts for 40% of UC in Taiwan (5). The incidence rate of UTUC was 4.21 cases per 100,000 women, which was higher than those of men with 3.61 cases per 100,000 men based on the Health Promotion Administration of Taiwan in 2016 (6). According to the depth of invasion, UBUC can be divided into non-muscle-invasive bladder cancer [NMIBC; stages carcinoma in situ (CIS), Ta, T1) or muscle-invasive bladder cancer (MIBC; stages T2, T3, T4) (7). Approximately 70% of newly diagnosed UBUC are NMIBC with roughly 70% as Ta, 20% as T1, and 10% as Tis (8). In the grading system, Ta is divided into papillary urothelial neoplasms of low malignant potential (PUNLMP), low-grade noninvasive papillary urothelial carcinoma, and high-grade noninvasive papillary urothelial carcinoma. By definition, CIS and the greater part of T1 belong to high-grade (9). In general, NMIBC is considered to have low metastasis and a favorable prognosis with 90% 5-year overall survival rates. However, a high recurrence rate of up to 50% after transurethral resection of the bladder tumor (TURBT) and the propensity of CIS progression to an invasive stage largely challenge the treatment of NMIBC (10, 11). In contrast, MIBC is characterized as rapid metastatic progression and subsequently high mortality with 60% and 6% 5-year survival rate at stages T2 and T4, respectively (12, 13).
UBUC and UTUC share some of the same carcinogenic factors such as cigarette smoking and industrially hazardous chemicals (14). Smokers have 2.5–7 times more risk to develop UBUC, the same as UTUC, compared with nonsmokers (15). However, other particular carcinogens such as aristolochic acid (AA) are responsible for an unusually high prevalence of UTUC in Taiwan and some rural regions of southeastern Europe on account of Chinese herb remedy and soil contamination with Aristolochia clematitis, respectively (16, 17). Additionally, substantial pieces of evidence verified genetic predisposition to the development of UC. Genome-wide association study (GWAS) manifests that several suspicious SNPs of SLC14A1, a urea transporter UT-B mapped to chromosome 18q12.3, is strongly related to UBUC (18). Overall, in UTUC and UBUC, the landscape of aberrant genes is similar but a difference exists in the prevalent mutation. High-grade UTUC has a higher tendency for genomic alteration in fibroblast growth factor receptor 3 (FGFR3), Hras proto-oncogene, GTPase (HRAS), and cyclin-dependent kinase inhibitor 2B (CDKN2B), whereas high-grade UBUC is more frequently altered in tumor protein p53 (TP53), AT-rich interaction domain 1A (ARID1A), RB transcriptional corepressor 1 (RB1), and erb-b2 receptor tyrosine kinase 2 (ERBB2) (19, 20). Furthermore, two divergent carcinogenic pathways (i.e., the papillary and the non-papillary pathways) are used to explain the evolution of UBUC. The papillary pathway is driven by point mutations in FGFR3, RAS, and PIK3CA (phosphatidylinositol-4,5-bisphosphate 3-kinase catalytic subunit alpha) to develop hyperplasia, low-grade Ta, and high-grade Ta/T1 through constitutive activation of RTK/RAS/RAF/mitogen-activated protein kinase (MAPK) and phosphoinositide 3-kinase (PI3K)/Akt/mammalian target of rapamycin (mTOR) pathways related to cell proliferation (21, 22). Instead, the nonpapillary pathway is defined by the loss-of-function mutation in genes associated with DNA replication/repair machinery such as p53 and RB that gives rise to the progression of dysplasia, Tis to MIBC (23). Moreover, loss of heterozygosity (LOH) in chromosome 9 harboring various tumor suppressor genes such as CDKN2A (9p21), TSC1 (9q34), and PTCH1 (9q22) exists in high frequency in both hyperplasia and dysplasia, suggesting that copy number alteration of chromosome 9 could be the necessary early event toward the initiation of UBUC (24).
Frequent genetic copy number aberration is an important cancer hallmark strongly associated with carcinogenesis and aggressiveness (25). Aside from chromosome 9, recurrent chromosomal abnormalities in UC were also identified among distinct populations. Using tiling-resolution 32K BAC arrays to conduct genome-wide DNA copy number profiling pointed that copy number gains (CNGs) at chromosomes 1q, 3p, 3q, 5p, 6p, 8q, 18p, 20p, and 20q and copy number loss (CNL) at chromosomes 2q, 5q, 8p, 9p, 9q, 10q, 11p, 13q, 17p, and 22q are identified in the 146 UC specimens from Skåne University Hospital, Sweden (26). Using 40 UBUC samples from Taiwan that were submitted to array comparative genomic hybridization (aCGH) showed that CNG appears at chromosomes 1q, 3q, 5p, 7p, 10p, 11q, 17q, 18p, 19q, 20p, 20q, and 22q, while CNL exists at chromosomes 2q, 3p, 4q, 5q, 6q, 7q, 8p, 9q, 10q, 11p, 13q, 17p, and 18q. Among these genetic aberrations, the frequent chromosomal instability mainly resides in -5q, -9p, and +8q, corresponding to the study by Lindgren et al. (26). Additionally, CNG of chromosome 8q behaved as the most predominant amplification core and tended to result in poor clinical outcomes with developed disease-specific death [dead of disease (DOD)] and distal metastasis (DM) in UC patients than non-event cases (27). Mounting studies have proven that the amplification of MYC mapped to 8q24.21 is the major measure to prompt MYC overexpression and is regularly examined in aggressive cancers including UC with metastasis and unfavorable survival outcome (25, 28). Moreover, CEBPD overexpression driven by amplified CEBPD mapped to 8q11.21 in UC was pointed to have a remarkable predisposition toward the worst prognosis outcomes concerning disease-specific survival (DSS) and metastasis-free survival (MeFS) (27).
CEBPD, a versatile transcription factor, belongs to the C/EBP family that comprises three parts: an N-terminal transactivating region, a basic DNA binding region, and a C-terminal leucine-zipper domain (29, 30). Typically, the CEBPD level is relatively low in many normal physiological statements while it is rapidly elevated by outside stimulations such as immune activation and inflammatory response (31). CEBPD presents as a regulator in terms of cell differentiation, proliferation, motility, growth arrest, and cell death (32). Over the past few years, accumulating research has identified the dual functions of CEBPD as a tumor suppressor in pancreatic ductal adenocarcinoma (PDAC), hepatocellular carcinoma, and breast cancer but an oncogene in glioblastoma and UC, relying on the tumor microenvironment and cell types (27, 33–36). Accordingly, this review intended to comprehensively summarize the regulatory mechanism on initial CEBPD overexpression followed by UC progression and cisplatin (CDDP)-resistant impact.
Previous studies elucidated that epigenetic events such as CpG island hypermethylation get involved in the silence of CEBPD and are associated with metastasis of breast cancer (37). Interestingly, the methylation level at the CEBPD promoter region is usually low and has no difference among the normal epithelium and low and high CEBPD-expressing UC tissues and UC cell lines (HT1197, TCCSUP, BFTC905, BTFC909, J82, RT4), indicating that the effect of CEBPD level is irrelevant to aberrant DNA methylation in UC. Furthermore, the high expression of CEBPD observed in the UC is attributed to the transcriptional activity of CEBPD amplification conferred by the low frequent promoter methylation (27). Intriguingly, UBUC specimens with a specifically gained region on chromosome 8q represented that MYC CNG is usually concurrent with CEBPD CNG and a positive correlation significantly exists between MYC and CEBPD gene dosage/transcripts. Noticeably, only MYC overexpression provoked CEBPD CNG, but CEBPD overexpression failed to cause MYC CNG in UC cells, indicating that a substantial MYC level occurred prior to CEBPD genome instability (38). Aberrant MYC is well known to set off genomic instability followed by cancer initiation (39). An abundant MYC level triggered by MYC amplification has been identified to amplify oncogene ERBB2 (erb-b2 receptor tyrosine kinase 2) mapped to chromosome 17q12 and DHFR (dihydrofolate reductase) mapped to chromosome 5q14.1 to strengthen cell proliferation and high metastatic risk (40, 41). Using next-generation sequencing (NGS)-based loss of heterozygosity (LOH) assay indicated that the MYC overexpression significantly induces genomic LOH in distinct UC cell lines. The NGS-based Hi-C experiment, a method to investigate such long-range interactions between two different loci and important for transcriptional modulation of genes, pointed out that there is no obvious chromosomal contact between MYC and CEBPD loci with 80 Mb apart from each other. The abovementioned results fortified that CEBPD overexpression driven by amplified CEBPD is the consequence of amplified MYC-induced genome instability (38).
A high glucose demand and preferential reprogramming toward aerobic glycolysis are the major hallmarks of cancer progression (42). MYC is documented to potentiate aerobic glycolysis through the reinforcement of transcription activity on all glycolytic-related genes (43). The study by Chan et al. revealed that a novel positive feedback loop between CEBPD- and MYC-centric multilayered exists in UC to strengthen aerobic glycolysis: amplification of MYC-initiated chromosomal CEBPD instability to increase the CEBPD level. The overexpression of CEBPD protein further stabilized the MYC protein from proteasome-mediated degradation through transcription inhibition of FBXW7 (F-box and WD repeat domain containing 7), a tumor suppressor serving as an E3 ubiquitin ligase of SCF (SKP1-CUL1-F-box protein) (44) to promote the level of solute carrier family 2 member 1 (SLC2A1; a central rate-limiting factor to regulate glucose transport in many cancers) and hexokinase II (HK2; a key mediator of aerobic glycolysis responsible for the aggressive phenotype) (45, 46). Subsequently, an increase in glucose uptake, lactate production, extracellular acidification rate (ECAR), and mitochondrial fragmentation/fission and a decrease in oxygen consumption rate (OCR), mitochondrial fusion type that were observed in CEBPD-overexpressing UC cell lines firmly proved the CEBPD-enforced metabolic conversion from mitochondrial oxidative phosphorylation to aerobic glycolysis.
Aside from employing MYC-dependent transcriptional regulation, CEBPD also utilizes an alternative pathway to coactivate aerobic glycolysis through transcriptional inhibition of hsa-miR-429 to boost HK2 expression (38). hsa-miR-429 belongs to the hsa-miR-200 family as a tumor suppressor in various cancers (47). The study by Chan et al. also disclosed the oncogenic characteristic of CEBPD on angiogenesis promotion through directly repressing the transcription of hsa-miR-429 to elevate the transcript of vascular endothelial growth factor A (VEGFA; a pivotal factor of angiogenesis and systemic metastasis) in UC cells (48, 49). Moreover, the upregulated expression of an angiogenesis-related gene called MMP2 (matrix metalloproteinase-2) driven by CEBPD amplification has been identified and is remarkably associated with UC cell invasiveness (27).
Hyperactivated MAPK and PI3K/AKT/mTOR axis are pivotal signalings for cancer cell proliferation and survival (Makker et al., 2012). Hence, CEBPD likely raised cell viability and proliferation through the upregulated phosphorylation levels of MAPK3/1, PI3K, AKT1, mTOR, RPS6, and EIF4EBP1 in UC cell lines (38). The mTOR is one of the important glucose-sensing centers to adapt to the change in environmental glucose level. mTOR is activated and further hyperphosphorylates its downstream proteins to promote cell proliferation and metabolism regulation under glucose-sufficient conditions. Oppositely, glucose scarcity hampers mTOR activity and its anabolic processes to keep energy for cell survival (Leprivier and Rotblat, 2020). Of note, the influence of CEBPD on extreme glucose addiction to satisfy the substantial energy demand for abnormal anabolism responsive to hyperactivated mTOR pathway contrarily devastates glucose-deprived UC cells (38).
Clinical significance indicated that a high level of CEBPD has a positive correlation with the expression of MYC, HK2, VEGFA, and MMP2 and a negative association with hsa-miR-429 in UC patients. These genetic patterns also reflect the inferior survival outcome. Furthermore, abundant CEBPD synergized the mismanagement of glucose metabolism to augment the deteriorated effect on cancer aggressiveness and survival rate in UC patients with diabetes mellitus (DM; a disease with imbalanced glucose homeostasis accompanied by excessive glucose level in peripheral tissue) and SCID/beige mouse model with high-fat diet-induced DM. Summarily, CEBPD prompts the cancerization of the urothelium through the multi-manipulations on glycolytic metabolism shift and angiogenesis with CEBPD/MYC/HK2 axis, CEBPD/hsa-miR-429/HK2 axis, CEBPD/hsa-miR-429/VEGFA axis, and CEBPD/MMP2 axis (27, 38, 49).
At present, CDDP-based neoadjuvant chemotherapy (NAC) accompanied by radical cystectomy is regarded as a frontline treatment for patients with aggressive UC (50).
CDDP is a type of platinum-based antineoplastic medication. CDDP-DNA adduct formation for DNA damage and upregulation of reactive oxygen species (ROS) to lead to apoptosis are the foremost anticancer effects of CDDP (51, 52). Nevertheless, patients with cancer including UC usually have an excellent response to CDDP initially while suffering from relapse later on account of the CDDP resistance to decline clinical effectiveness (53, 54). The level of CEBPD has been reported to be upregulated after CDDP treatment. However, the side effect of CDDP-induced CEBPD on UC treatment is still ambiguous to be defined as dual functions to result in CDDP-induced chemotherapy resistance or drive CDDP-induced antitumorigenesis. To date, studies by Hour et al. (55) and Wang et al. (56) implicated that CEBPD possesses an oncogenic characteristic to promote CDDP-based chemotherapy resistance. The previous one showed that CDDP-induced CEBPD transcriptionally upregulates the level of Cu/Zn-superoxide dismutase (SOD1) to lower the level of ROS and apoptosis caused by CDDP treatment in UC. The later research indicated that the ATP-binding cassette (ABC) transporters (ABCB1, ABCC2) that function in multidrug resistance (MDR) in malignancy (57) are transcriptionally increased by EGFR/STAT3-driven CEBPD after CDDP treatment and has a high correlation with CDDP-related resistance in UC (55, 56). In contrast, the study by Lin et al. (58) pointed out that CEBPD-triggered hsa-miR-193b-3p directly targets cyclin D1 (CCND1) and ETS proto-oncogene 1 (ETS1) to cause cell cycle G1 arrest, invasive inhibition after CDDP treatment, suggesting that CDDP-induced CEBPD strengthens its tumor suppression.
Aside from the importance of CEBPD expression in cancer cells to tumor progression, the impact of non-cancer cells with CEBPD on the regulation of malignant aggressiveness has been paid close attention to recently. The tumor is a complex composed of cancer cells and tumor stroma including non-cellular [e.g., extracellular matrix (ECM)] and cellular components [e.g., activated cancer-associated fibroblasts (CAFs), mesenchymal stromal cells (MSCs), pericytes]. Tumor stroma is an essential part to support tumor growth and metastasis and even confers therapeutic resistance (59, 60). The study elucidated that substantial CEBPD is observed in the stromal compartments of PDAC and presents high relevance to the pancreatic cancer extravasation as well as metastasis but not tumor growth (61). In addition, treating breast cancer with CDDP promoted the level of CEBPD in CAFs as well as tumor-associated macrophages (TAMs) and led to chemoresistance through pentraxin 3 (PTX3)-induced invasion, metastasis, and stemness (62). Moreover, CDDP-induced CEBPD enforced the differentiation of fibroblasts toward myofibroblasts in the lung cancer microenvironment and activated the stromal cell-derived factor 4 (SDF4)/C-X-C motif chemokine receptor 4 (CXCR4) to trigger angiogenesis and distal metastasis (63). However, the contribution of systematic CEBPD-expressing tumor stroma and immune microenvironment to UC progression and its clinical relevance remain largely unknown; hence, more explorations are still necessary.
Writing—original draft: T-CC. Writing—review and editing: C-FL, Y-LS. Funding acquisition: C-FL. Study supervision: C-FL, Y-LS. All authors contributed to the article and approved the submitted version.
This work was sponsored by Taiwan National Ministry of Science and Technology (111-2628-B-384 -001 to CF Li) and National Health Research Institutes (CA-110-PP-11 to CF Li).
The authors declare that the research was conducted in the absence of any commercial or financial relationships that could be construed as a potential conflict of interest.
All claims expressed in this article are solely those of the authors and do not necessarily represent those of their affiliated organizations, or those of the publisher, the editors and the reviewers. Any product that may be evaluated in this article, or claim that may be made by its manufacturer, is not guaranteed or endorsed by the publisher.
1. Sung H, Ferlay J, Siegel RL, Laversanne M, Soerjomataram I, Jemal A, et al. Global cancer statistics 2020: GLOBOCAN estimates of incidence and mortality worldwide for 36 cancers in 185 countries. CA: A Cancer J Clin (2021) 71:209–49. doi: 10.3322/caac.21660
2. Pasin E, Josephson DY, Mitra AP, Cote RJ, Stein JP. Superficial bladder cancer: an update on etiology, molecular development, classification, and natural history. Rev Urol (2008) 10:31–43.
3. Clark PE, Agarwal N, Biagioli MC, Eisenberger MA, Greenberg RE, Herr HW, et al. Bladder cancer. J Natl Compr Cancer Network J Natl Compr Canc Netw (2013) 11:446–75. doi: 10.6004/jnccn.2013.0059
4. Lin M-Y, Niu S-W, Li W-M, Lee H-L, Chen L-T, Wu W-J, et al. Incidence and survival variations of upper tract urothelial cancer in taiwa–2010). Int J Urol (2022) 29:121–7. doi: 10.1111/iju.14731
5. Wu Y-T, Luo HL, Wang HJ, Chen YT, Cheng YT, Chiang PH. Gender effect on the oncologic outcomes of upper urinary tract urothelial carcinoma in Taiwan. Int Urol Nephrol (2020) 52:1043–8. doi: 10.1007/s11255-020-02396-z
6. Cancer registry annual report. Taiwan: Health promotion administration, ministry of health and welfare (2018) Taiwan. https://www.hpa.gov.tw/EngPages/List.aspx?nodeid=1070
7. Fong MHY, Feng M, Mcconkey DJ, Choi W. Update on bladder cancer molecular subtypes. Transl Androl Urol (2020) 9:2881–9. doi: 10.21037/tau-2019-mibc-12
8. Van Rhijn BWG, Burger M, Lotan Y, Solsona E, Stief CG, Sylvester RJ, et al. Recurrence and progression of disease in non–Muscle-Invasive bladder cancer: From epidemiology to treatment strategy. Eur Urol (2009) 56:430–42. doi: 10.1016/j.eururo.2009.06.028
9. Hurst CD, Knowles MA. Mutational landscape of non-muscle-invasive bladder cancer. Urologic Oncology: Semin Original Investigations (2022) 40:295–303. doi: 10.1016/j.urolonc.2018.10.015
10. Sylvester RJ, van der Meijden A, Witjes JA, Jakse G, Nonomura N, Cheng C, et al. High-grade Ta urothelial carcinoma and carcinoma in situ of the bladder. Urology (2005) 66:90–107. doi: 10.1016/j.urology.2005.06.135
11. Tokuyama N, Saito A, Muraoka R, Matsubara S, Hashimoto T, Satake N, et al. Prediction of non-muscle invasive bladder cancer recurrence using machine learning of quantitative nuclear features. Modern Pathol (2022) 35:533–8. doi: 10.1038/s41379-021-00955-y
12. Van Kessel K. Personalized bladder cancer management. Ph.D. thesis. Rotterdam, Netherlands: Erasmus University Rotterdam (2018).
13. Minoli M, Kiener M, Thalmann GN, Kruithof-De Julio M, Seiler R. Evolution of urothelial bladder cancer in the context of molecular classifications. Int J Mol Sci (2020) 21:5670. doi: 10.3390/ijms21165670
14. Miyazaki J, Nishiyama H. Epidemiology of urothelial carcinoma. Int J Urol (2017) 24:730–4. doi: 10.1111/iju.13376
15. Szarvas T, Módos O, Horváth A, Nyirády P. Why are upper tract urothelial carcinoma two different diseases? Transl Androl Urol (2016) 5:636–47. doi: 10.21037/tau.2016.03.23
16. Broe M. Chinese Herbs nephropathy and Balkan endemic nephropathy: Toward a single entity, aristolochic acid nephropathy. Kidney Int (2012) 81:513–5. doi: 10.1038/ki.2011.428
17. Lai HY, Wu LC, Kong PH, Tsai HH, Chen YT, Cheng YT, et al. High level of aristolochic acid detected with a unique genomic landscape predicts early UTUC onset after renal transplantation in Taiwan. Front Oncol (2021) 11:828314. doi: 10.3389/fonc.2021.828314
18. Hou R, Kong X, Yang B, Xie Y, Chen G. SLC14A1: A novel target for human urothelial cancer. Clin Trans Oncol (2017) 19:1438–46. doi: 10.1007/s12094-017-1693-3
19. Sfakianos JP, Cha EK, Iyer G, Scott SN, Zabor EC, Shah RH, et al. Genomic characterization of upper tract urothelial carcinoma. Eur Urol (2015) 68:970–7. doi: 10.1016/j.eururo.2015.07.039
20. Audenet F, Isharwal S, Cha EK, Donoghue MTA, Drill EN, Ostrovnaya I, et al. Clonal relatedness and mutational differences between upper tract and bladder urothelial carcinoma. Clin Cancer Res (2019) 25:967–76. doi: 10.1158/1078-0432.CCR-18-2039
21. Hayashi T, Fujita K, Hayashi Y, Hatano K, Kawashima A, Mcconkey DJ, et al. Mutational landscape and environmental effects in bladder cancer. Int J Mol Sci (2020) 21(17):6072. doi: 10.3390/ijms21176072
22. Tripathi K, Goel A, Singhai A, Garg M. Mutational analysis of ras hotspots in patients with urothelial carcinoma of the bladder. World J Clin Oncol (2020) 11:614–28. doi: 10.5306/wjco.v11.i8.614
23. Inamura K. Bladder cancer: New insights into its molecular pathology. Cancers (Basel) (2018) 10(4):100. doi: 10.3390/cancers10040100
24. Knowles MA, Hurst CD. Molecular biology of bladder cancer: New insights into pathogenesis and clinical diversity. Nat Rev Cancer (2015) 15:25–41. doi: 10.1038/nrc3817
25. Florl AR, Schulz WA. Chromosomal instability in bladder cancer. Arch Toxicol (2008) 82:173–82. doi: 10.1007/s00204-008-0280-3
26. Lindgren D, Sjödahl G, Lauss M, Staaf J, Chebil G, Lövgren K, et al. Integrated genomic and gene expression profiling identifies two major genomic circuits in urothelial carcinoma. PloS One (2012) 7:e38863. doi: 10.1371/journal.pone.0038863
27. Wang YH, Wu WJ, Wang WJ, Huang HY, Li WM, Yeh BW, et al. CEBPD amplification and overexpression in urothelial carcinoma: A driver of tumor metastasis indicating adverse prognosis. Oncotarget (2015) 6:31069–84. doi: 10.18632/oncotarget.5209
28. Kalkat M, De Melo J, Hickman KA, Lourenco C, Redel C, Resetca D, et al. MYC deregulation in primary human cancers. Genes (Basel) (2017) 8(6):151. doi: 10.3390/genes8060151
29. Wedel A, Ziegler-Heitbrock HW. The C/EBP family of transcription factors. Immunobiology (1995) 193:171–85. doi: 10.1016/S0171-2985(11)80541-3
30. Akasaka T, Balasas T, Russell LJ, Sugimoto K-J, Majid A, Walewska R, et al. Five members of the CEBP transcription factor family are targeted by recurrent IGH translocations in b-cell precursor acute lymphoblastic leukemia (BCP-ALL). Blood (2006) 109:3451–61. doi: 10.1182/blood-2006-08-041012
31. Lai H-Y, Hsu L-W, Tsai H-H, Lo Y-C, Yang S-H, Liu P-Y, et al. CCAAT/enhancer-binding protein delta promotes intracellular lipid accumulation in M1 macrophages of vascular lesions. Cardiovasc Res (2017) 113:1376–88. doi: 10.1093/cvr/cvx134
32. Balamurugan K, Sterneck E. The many faces of C/EBPδ and their relevance for inflammation and cancer. Int J Biol Sci (2013) 9:917–33. doi: 10.7150/ijbs.7224
33. Mendoza-Villanueva DY, Kim S, Ali HR, Sharan S, Sarkar TR, Caldas C, et al. Abstract 3464: CEBPD (C/EBPδ) acts as a tumor suppressor in hormone receptor positive breast cancer cells and may serve as biomarker to predict the need for adjuvant therapy. Cancer Res (2013) 73:3464–4. doi: 10.1158/1538-7445.AM2013-3464
34. Liu P, Cao W, Ma B, Li M, Chen K, Sideras K, et al. Action and clinical significance of CCAAT/enhancer-binding protein delta in hepatocellular carcinoma. Carcinogenesis (2018) 40:155–63. doi: 10.1093/carcin/bgy130
35. Hartl L, Duitman J, Aberson HL, Chen K, Dijk F, Roelofs JJTH, et al. CCAAT/Enhancer-binding protein delta (C/EBPδ): A previously unrecognized tumor suppressor that limits the oncogenic potential of pancreatic ductal adenocarcinoma cells. Cancers (2020) 12:2546. doi: 10.3390/cancers12092546
36. Wang S-M, Lin W-C, Lin H-Y, Chen Y-L, Ko C-Y, Wang J-M. CCAAT/Enhancer-binding protein delta mediates glioma stem-like cell enrichment and ATP-binding cassette transporter ABCA1 activation for temozolomide resistance in glioblastoma. Cell Death Discovery (2021) 7:8. doi: 10.1038/s41420-020-00399-4
37. Ko C-Y, Hsu H-C, Shen M-R, Chang W-C, Wang J-M. Epigenetic silencing of CCAAT/Enhancer-binding protein δ activity by YY1/Polycomb Group/DNA methyltransferase complex*. J Biol Chem (2008) 283:30919–32. doi: 10.1074/jbc.M804029200
38. Chan TC, Chen YT, Tan KT, Wu CL, Wu WJ, Li WM, et al. Biological significance of MYC and CEBPD coamplification in urothelial carcinoma: Multilayered genomic, transcriptional and posttranscriptional positive feedback loops enhance oncogenic glycolysis. Clin Transl Med (2021) 11:e674. doi: 10.1002/ctm2.674
39. Kuzyk A, Mai S. C-MYC-induced genomic instability. Cold Spring Harb Perspect Med (2014) 4:a014373. doi: 10.1101/cshperspect.a014373
40. Mai S, Hanley-Hyde J, Fluri M. C-myc overexpression associated DHFR gene amplification in hamster, rat, mouse and human cell lines. Oncogene (1996) 12:277–88.
41. Park K, Kwak K, Kim J, Lim S, Han S. C-myc amplification is associated with HER2 amplification and closely linked with cell proliferation in tissue microarray of nonselected breast cancers. Hum Pathol (2005) 36:634–9. doi: 10.1016/j.humpath.2005.04.016
42. Enzo E, Santinon G, Pocaterra A, Aragona M, Bresolin S, Forcato M, et al. Aerobic glycolysis tunes YAP/TAZ transcriptional activity. EMBO J (2015) 34:1349–70. doi: 10.15252/embj.201490379
43. Dong Y, Tu R, Liu H, Qing G. Regulation of cancer cell metabolism: oncogenic MYC in the driver’s seat. Signal Transduction and Targeted Therapy, (2020) 5(1):124. doi: 10.1038/s41392-020-00235-2
44. Yeh C-H, Bellon M, Nicot C. FBXW7: A critical tumor suppressor of human cancers. Mol Cancer (2018) 17:115. doi: 10.1186/s12943-018-0857-2
45. Mathupala SP, Ko YH, Pedersen PL. Hexokinase II: cancer's double-edged sword acting as both facilitator and gatekeeper of malignancy when bound to mitochondria. Oncogene (2006) 25:4777–86. doi: 10.1038/sj.onc.1209603
46. Wang J, Ye C, Chen C, Xiong H, Xie B, Zhou J, et al. Glucose transporter GLUT1 expression and clinical outcome in solid tumors: a systematic review and meta-analysis. Oncotarget (2017) 8(17):6072. doi: 10.18632/oncotarget.15171
47. Wang Z, Zhu Z, Lin Z, Luo Y, Liang Z, Zhang C, et al. miR-429 suppresses cell proliferation, migration and invasion in nasopharyngeal carcinoma by downregulation of TLN1. Cancer Cell Int (2019) 19:115. doi: 10.1186/s12935-019-0831-0
48. Apte RS, Chen DS, Ferrara N. VEGF in signaling and disease: Beyond discovery and development. Cell (2019) 176:1248–64. doi: 10.1016/j.cell.2019.01.021
49. Chan TC, Hsing CH, Shiue YL, Huang SK, Hsieh KL, Kuo YH, et al. Angiogenesis driven by the CEBPD-hsa-miR-429-VEGFA signaling axis promotes urothelial carcinoma progression. Cells (2022) 11(4):638. doi: 10.3390/cells11040638
50. Wu J, Xie RY, Cao CZ, Shang BQ, Shi HZ, Shou JZ. Disease management of clinical complete responders to neoadjuvant chemotherapy of muscle-invasive bladder cancer: A review of literature. Front Oncol (2022) 12:816444. doi: 10.3389/fonc.2022.816444
51. Choi YM, Kim HK, Shim W, Anwar MA, Kwon JW, Kwon HK, et al. Mechanism of cisplatin-induced cytotoxicity is correlated to impaired metabolism due to mitochondrial ROS generation. PloS One (2015) 10:e0135083. doi: 10.1371/journal.pone.0135083
52. Rocha CRR, Silva MM, Quinet A, Cabral-Neto JB, Menck CFM. DNA Repair pathways and cisplatin resistance: An intimate relationship. Clinics (Sao Paulo) (2018) 73:e478s. doi: 10.6061/clinics/2018/e478s
53. Shen DW, Pouliot LM, Hall MD, Gottesman MM. Cisplatin resistance: A cellular self-defense mechanism resulting from multiple epigenetic and genetic changes. Pharmacol Rev (2012) 64:706–21. doi: 10.1124/pr.111.005637
54. Skowron MA, Melnikova M, Van Roermund JGH, Romano A, Albers P, Thomale J, et al. Multifaceted mechanisms of cisplatin resistance in long-term treated urothelial carcinoma cell lines. Int J Mol Sci (2018) 19. doi: 10.3390/ijms19020590
55. Hour TC, Lai YL, Kuan CI, Chou CK, Wang JM, Tu HY, et al. Transcriptional up-regulation of SOD1 by CEBPD: A potential target for cisplatin resistant human urothelial carcinoma cells. Biochem Pharmacol (2010) 80:325–34. doi: 10.1016/j.bcp.2010.04.007
56. Wang WJ, Li CF, Chu YY, Wang YH, Hour TC, Yen CJ, et al. Inhibition of the EGFR/STAT3/CEBPD axis reverses cisplatin cross-resistance with paclitaxel in the urothelial carcinoma of the urinary bladder. Clin Cancer Res (2017) 23:503–13. doi: 10.1158/1078-0432.CCR-15-1169
57. Choi CH. ABC Transporters as multidrug resistance mechanisms and the development of chemosensitizers for their reversal. Cancer Cell Int (2005) 5:30. doi: 10.1186/1475-2867-5-30
58. Lin S-R, Yeh H-C, Wang W-J, Ke H-L, Lin H-H, Hsu W-C, et al. MiR-193b mediates CEBPD-induced cisplatin sensitization through targeting ETS1 and cyclin D1 in human urothelial carcinoma cells. J Cell Biochem (2017) 118:1563–73. doi: 10.1002/jcb.25818
59. Valkenburg KC, De Groot AE, Pienta KJ. Targeting the tumour stroma to improve cancer therapy. Nat Rev Clin Oncol (2018) 15:366–81. doi: 10.1038/s41571-018-0007-1
60. Xu M, Zhang T, Xia R, Wei Y, Wei X. Targeting the tumor stroma for cancer therapy. Mol Cancer (2022) 21:208. doi: 10.1186/s12943-022-01670-1
61. Duitman J, Hartl L, Roelofs J, Bijlsma MF, Spek CA. Non-tumor CCAAT/Enhancer-binding protein delta potentiates tumor cell extravasation and pancreatic cancer metastasis formation. Biomolecules (2021) 11(8):1079. doi: 10.3390/biom11081079
62. Chi JY, Hsiao YW, Li CF, Lo YC, Lin ZY, Hong JY, et al. Targeting chemotherapy-induced PTX3 in tumor stroma to prevent the progression of drug-resistant cancers. Oncotarget (2015) 6:23987–4001. doi: 10.18632/oncotarget.4364
Keywords: CEBPD, urothelial carcinoma, chromosomal instabilities, glycolysis, resistance
Citation: Chan T-C, Shiue Y-L and Li C-F (2023) The biological impacts of CEBPD on urothelial carcinoma development and progression. Front. Oncol. 13:1123776. doi: 10.3389/fonc.2023.1123776
Received: 14 December 2022; Accepted: 05 January 2023;
Published: 27 January 2023.
Edited by:
Hsin-Chih Yeh, Kaohsiung Medical University, TaiwanReviewed by:
I-Wei Chang, Taipei Medical University, TaiwanCopyright © 2023 Chan, Shiue and Li. This is an open-access article distributed under the terms of the Creative Commons Attribution License (CC BY). The use, distribution or reproduction in other forums is permitted, provided the original author(s) and the copyright owner(s) are credited and that the original publication in this journal is cited, in accordance with accepted academic practice. No use, distribution or reproduction is permitted which does not comply with these terms.
*Correspondence: Yow-Ling Shiue, c2hpcmxleUBpbXN0Lm5zeXN1LmVkdS50dw==; Chien-Feng Li, YW5nZWxvLnBAeWFob28uY29tLnR3
Disclaimer: All claims expressed in this article are solely those of the authors and do not necessarily represent those of their affiliated organizations, or those of the publisher, the editors and the reviewers. Any product that may be evaluated in this article or claim that may be made by its manufacturer is not guaranteed or endorsed by the publisher.
Research integrity at Frontiers
Learn more about the work of our research integrity team to safeguard the quality of each article we publish.