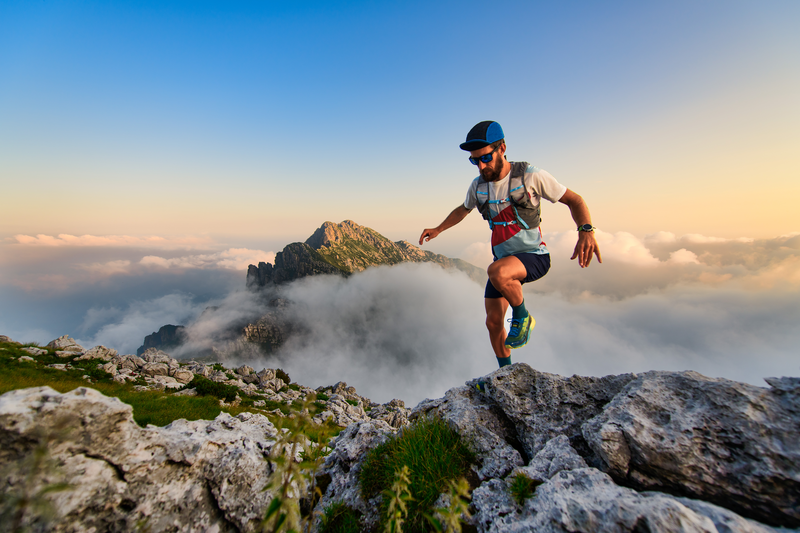
95% of researchers rate our articles as excellent or good
Learn more about the work of our research integrity team to safeguard the quality of each article we publish.
Find out more
REVIEW article
Front. Oncol. , 25 January 2023
Sec. Molecular and Cellular Oncology
Volume 13 - 2023 | https://doi.org/10.3389/fonc.2023.1123464
This article is part of the Research Topic New Therapeutics for Soft Tissue Sarcomas View all 10 articles
Synovial sarcoma is a soft tissue sarcoma accounting for approximately 1,000 cases per year in the United States. Currently, standard treatment of advanced and metastatic synovial sarcoma is anthracycline-based chemotherapy. While advanced synovial sarcoma is more responsive to chemotherapy compared to other soft tissue sarcomas, survival rates are poor, with a median survival time of less than 18 months. Enhanced understanding of tumor antigen expression and molecular mechanisms behind synovial sarcoma provide potential targets for treatment. Adoptive Cell Transfer using engineered T-cell receptors is in clinical trials for treatment of synovial sarcoma, specifically targeting New York esophageal squamous cell carcinoma-1 (NY-ESO-1), preferentially expressed antigen in melanoma (PRAME), and melanoma antigen-A4 (MAGE-A4). In this review, we explore the opportunities and challenges of these treatments. We also describe artificial adjuvant vector cells (aAVCs) and BRD9 inhibitors, two additional potential targets for treatment of advanced synovial sarcoma. This review demonstrates the progress that has been made in treatment of synovial sarcoma and highlights the future study and qualification needed to implement these technologies as standard of care.
Synovial sarcoma (SYN) is a soft tissue sarcoma accounting for 5-14% of all soft tissue sarcomas (1, 2). The incidence of SYN in the United States is approximately 1.42 per million for adults and 0.81 per million for children and adolescents, accounting for roughly 1,000 cases per year (3). SYN presents at an average age of 35-40 years and there is equal distribution of cases between females and males (3–6). SYN most often arises in deep tissues of the extremities but can also present as head and neck, trunk, and lung lesions (3, 7). Epidemiologic studies have found that most patients are diagnosed with local disease while 10-13% of patients initially present with metastatic disease (3, 7).
The diagnosis and staging of SYN involve pathologic and radiographic review. SYN is defined by the presence of translocation of t(X:18) (p11.2;q11.2) using FISH or RT-PCR and is found in more than 95% of tumors (8). This translocation leads to the fusion of genes SYT on Chromosome 18 and SSX on Chromosome X, which causes production of SS18-SSX1, SS18-SSX2, or SS18-SSX4 (9–11). These oncogenic fusion proteins impact cellular transcription and metabolism, leading to sarcomagenesis.
For localized cases of SYN, initial therapy is most commonly surgical resection with or without radiation therapy. Neoadjuvant or adjuvant chemotherapy is considered in select cases (12, 13). SYN has high metastatic potential with a historic five year metastasis-free survival rate of 50-60% (14). For locally advanced or metastatic disease, first line therapy usually incorporates anthracycline-based chemotherapy with or without ifosfamide (13, 15, 16). SYNs are relatively chemosensitive tumors compared to other soft tissue sarcomas. In primary soft tissue sarcomas, early localized and metastatic recurrence have been found to occur at a median of 38.3 and 41.3 months, respectively (17). In contrast, SYN has been found to have local recurrence at a mean of 43 month and metastatic recurrence at 68 months (18). A review of 15 clinical trials of first-line chemotherapy for SYN has shown a 27.8% response rate compared to 18.8% in other soft tissue sarcomas (19). When comparing SYN to other soft tissue sarcomas, progression free survival (PFS) was 6.3 months versus 3.7 months and overall survival (OS) was 15.0 months versus 11.7 months, respectively (19). Despite this response, however, for those with metastatic disease one year survival remains 59.5% and the median overall survival is 17.0 months (95% CI 14.5-19.5) (6).
Currently, after anthracycline-based chemotherapy, the only other systemic therapy for treatment of advanced or metastatic SYN approved by the FDA is pazopanib. This approval was granted after pazopanib was shown to improve PFS compared to placebo in a population of patients with varying non-adipocytic metastatic soft tissue sarcomas which included 30 patients with SYN (20).
Improved understanding of cellular and molecular processes behind the development of SYN and advancements in knowledge of SYN’s antigen expression will allow for potential targets for treatment of advanced SYN. In this review, we explore emerging therapies in the treatment of advanced and metastatic SYN.
SYN has been found to express cancer testis antigens (CTAs) (21). CTAs are antigens with predominant expression in the testis and are not normally found in somatic tissue (22). CTAs are a potential target for treatment of malignancies as they elicit humoral and cellular immune responses (23). SYN has been found to have high expression of CTAs (24). Due to their high expressivity, selectivity, and immunologic response, CTAs have been identified as potential targets for treatment of SYN.
Adopted Cell Transfer (or Therapy) (ACT) uses tumor antigen specific T-cells obtained from resected tumor specimens which are expanded in vitro and then infused for treatment of cancers (25, 26). One challenge of this treatment is that not all resected tumors allow for the expansion of autologous tumor infiltrating T-cells (27). This obstacle, along with variable quantities of T-cells within tumors, has prompted study of genetically engineered T-cell receptors to target cancer specific antigens. These T-cells are obtained through the harvesting of patient autologous T-cells which are then genetically modified to express a T-cell receptor for a cancer antigen. This technology is currently in development for the treatment of SYN targeting CTAs (Figure 1).
New York esophageal squamous cell carcinoma-1 (NY-ESO-1) is a CTA that was first described from serological analysis of recombinant cDNA expression libraries (SEREX) of esophageal squamous cell carcinoma (28). NY-ESO-1 is expressed in approximately 80% of SYNs. The immunogenicity of NY-ESO-1 has led to its consideration as a target for treatment of SYN (23, 29).
In 2011, Robbins et al. conduced the first clinical trial of autologous T-cells genetically engineered to have a specific T-cell receptor for NY-ESO-1 for patients with metastatic melanoma and SYN (Table 1) (38). The trial utilized a retroviral vector to create CD4+ and CD8+ autologous T-cells with a T-cell receptor that recognized the SLLMWITQC peptide of NY-ESO-1 for HLA-A*0201, named IG4- α95:LY ([NCI] 08-C-0121) (30). These T-cells were expanded in vitro and then transferred to patients after nonmyeloablative chemotherapy along with IL-2. This trial demonstrated objective clinical response in four out of six patients with SYN. The study was then expanded for 12 additional patients with SYN (31). Results demonstrated complete response for one patient and partial response observed in 10 of the 18 total patients with SYN. The three-year survival rate was 38% and the five-year survival rate was 14%.
All participants had neutropenia and thrombocytopenia during lymphodepleting chemotherapy, and one patient died from E. coli bacteremia three days after transfer of T-cells during a period of neutropenia. In this study, no correlation was measured demonstrating relationship between percentage of anti-NY-ESO-1 CD4+ or CD8+ T-cells at one month post transfer and disease response.
With evidence of activity for genetically engineered T-cells targeting NY-ESO-1, additional studies of genetically engineered T-cell receptors against NY-ESO-1 have been conducted. A Phase I, open-label trial of NY-ESO-1c259 T-cells (letetresgene autoleucel [lete-cel]; GSK3377794) included 45 patients with recurrent or metastatic SYN (NCT01343043) (29). This study resulted in one complete response (34 weeks) and 14 partial responses (29, 32). In this trial, four cohorts were established with varying NY-ESO-1 expression and lymphodepleting chemotherapeutic regimens. Cohort 1 included patients with high NY-ESO-1 expression and a high lymphodepletion regimen (fludarabine and cyclophosphamide). Cohort 2 included patients with low NY-ESO-1 expression with a high lymphodepletion regimen. Cohort 3 included patients with high NY-ESO-1 expression with a differing high lymphodepletion regimen (cyclophosphamide only). Cohort 4 included patients with high NY-ESO-1 expression and a low lymphodepletion regimen (dose reduced cyclophosphamide and fludarabine).
Cohorts 1-3 have complete data available as of January 2020. In Cohort 1, six of 12 patients had at least a partial response, one patient had a complete response, and the median overall survival (OS) was 24.3 months (29). In Cohort 2, four patients of 13 had partial response and the median OS was 9.9 months. In Cohort 3, one patient of 5 had a partial response with an OS of 19.9 months. In Cohort 1 all six responders had presence of anti-NY-ESO-1 T-cells at 6 months post cells transfer (39). More than 40% of patients in all cohorts had Grade 3 or higher hematologic Adverse Events (AEs) and 44% of patients had cytokine release syndrome, of which four were Grade 3 or higher (29). This is similar to toxicity seen for chimeric antigen receptor (CAR) T-cell therapy, where 69% of patients had Grade 3 or higher neutropenia and 92% of patients had cytokine release syndrome, of which 6% were Grade 3 or higher (40). A Phase II master protocol is currently in recruitment to test NY-ESO-1 T-cells for patients with metastatic SYN or myxoid/round cell liposarcoma who have progressed after standard treatment (NCT03967223) (Table 2) (41).
Next generation NY-ESO-1 T-cell products may provide additional benefits, but qualification is needed. Currently, a Phase II master protocol of three different next generation NY-ESO-1 T-cell products is in recruitment for treatment of solid tumors with NY-ESO-1 expression (NCT04526509) (42).
CD8 is a cell surface glycoprotein that acts as a co-receptor with T-cell receptors and assists in T-cell binding to MHC1 (47, 48). Previous in vitro study has found that engineered T-cells targeting a cancer testis antigen that co-expressed CD8α led to greater CD4+ T-cell activity (49). One arm of the master protocol will use anti-NY-ESO-1 T-cells which co-express the CD8α chain to determine efficacy of this technology (GSK3901961) (42).
An additional technology of interest combines anti-NY-ESO-1 T-cells with a dominant negative transforming growth factor- β (TGF-β) type II receptor (GSK3845097) (42). TGF-β is a regulator of immune homeostasis and has been found to inhibit tumor cellular immunity (50, 51). T-cells genetically engineered to target prostate cancer combined with a dominant negative TGF-β receptor have been found to cause tumor regression and enhanced survival in a murine model (51). This technology may improve the tumor microenvironment by limiting the impact of immune down-regulators, specifically TGF- β, in treatment of SYN.
T-cell quality impacts success in ACT. Previous study of ex-vivo ACTs has found that stem-like surface markers on T-cells are more likely to lead to response and stem-like T-cells are more capable of in vivo expansion (52). This knowledge has led to the development of technology that improves the stem-like quality of engineered T-cell receptors ex vivo through epigenetic reprogramming (53). The third arm of the master protocol will assess anti-NY-ESO-1 T-cells after a proprietary epigenetic reprogramming process to enhance the stem-like quality of the T-cells (GSK4427296). Combining engineered T-cells with additional genetic modifications may enhance efficacy of ACT targeting NY-ESO-1. Beyond NY-ESO-1 targeted therapies, other ACTs against cancer testis antigens have been developed for the treatment of SYN.
Preferentially expressed antigen in melanoma (PRAME) is a cancer testis antigen that is expressed in 95% of metastatic melanoma (54). It is also expressed homogenously in SYN at high levels (55). PRAME functions through inhibition of apoptosis and signal transduction of the retinoic acid receptor, causing tumorigenesis (56). Based on its expression and impact on sarcomagenesis, it is an additional target for directed engineered T-cell therapy.
The IMA203 trial utilized T- cell receptor engineered T-cells against PRAME in HLA-A*02:01 (NCT03686124) (57). This Phase I trial of 12 evaluable patients resulted in six patients with stable disease and six patients with partial response, three of whom had SYN (33). The most common adverse events were cytopenias, neurotoxicity, and cytokine release syndrome. One patient had a dose limiting toxicity. Another, currently recruiting, trial for treatment of advanced solid tumors with PRAME and HLA-A*02:01 expression will test IMC-F106C, a T-cell receptor against PRAME, both in combination with checkpoint inhibitors and as a single agent (NCT04262466) (44). Results of this trial are expected in 2024.
Melanoma-associated antigen (MAGE) proteins are clustered on the X chromosome. Expression of MAGE protein is generally restricted to reproductive tissues. This protein functions by inhibition of p53 and thereby limits tumor suppression (58, 59). MAGE-A4 is a cancer testis antigen that is expressed in many tumor types including lung cancer (19-35%), breast cancer (13%), ovarian cancer (47%), colon cancer (22%), esophageal cancer (60%), and soft tissue sarcomas, including 50-80% of SYN (24, 60–62).
Afamitresgene autoleucel are autologous T-cells which are isolated from patients, transduced with a lentiviral vector containing the MAGE-A4c1032 T-cell receptor, and expanded prior to infusion. Recently, results of a Phase I dose-escalation and expansion trial of Afamitresgene autoleucel was conducted in patients who were HLA-A*02 positive with advanced cancers that expressed MAGE-A4 (NCT03132922). In this study, patients received lymphodepletion regimen of cyclophosphamide and fludarabine prior to Afamitresgene autoleucel infusion (34, 35).
In the Cohort 3/expansion group (28 patients), 7 of 28 patients had a partial response, 11 of 28 had stable disease, while 10 of 28 either had progressive disease or were not evaluable (34). Results of this study showed no dose limiting toxicities and the most common Grade 3 or higher AE (>30%) were hematologic, including lymphopenia, leukopenia, neutropenia, anemia, and thrombocytopenia. Two patients had trial related deaths due to aplastic anemia and cerebral vascular accident. Notably, all responses to therapy occurred in patients with SYN, perhaps emphasizing the validity of targeting MAGE-A4 in this histology.
A Phase II, single arm, open-label clinical trial of Afamitresgene autoleucel in patients with advanced SYN or myxoid/round cell liposarcoma (MRCLS) called SPEARHEAD-1 is currently underway (NCT04044768) (36). Preliminary results from SPEARHEAD-1 were presented at the 2022 American Society of Clinical Oncology Annual Meeting (37). Patients received Afamitresgene autoleucel and were evaluable for response (Phase I, n = 18; Phase II, n = 51) with all patients expressing the HLA-A*02 allele. The pooled investigator-assessed overall response rate was 36.2% which occurred across MAGE-A4 H-scores of 134-400. The median duration of response was 52 weeks (8.29 – 75.14). Response rate was higher in patients with fewer lines of previous therapy, smaller target lesions, higher MAGE-A4 scores, those without bridging therapy, women, patients over 40, and patients from North America. The SPEARHEAD-1 trial is currently recruiting for Cohort 2 which will specifically evaluate patients with SYN (36).
There has been success in treating SYN through targeting NY-ESO-1, PRAME, and MAGE-A4 using ACT, with more trial results forthcoming. While this is laudable, there are challenges to the treatment of SYN using these technologies. One barrier is the restriction of many of these therapies to patients with HLA-A*02. Studies have found that HLA-A*02 is more common in Caucasian populations compared to African-American and Asian populations (63). Other barriers for ACT include the multi-week time needed for the production of genetically engineered T-cells, the pre-treatment lymphodepletion regimen which often requires hospitalization, and the high cost of therapy (64, 65). While many of these issues may be overcome through improvement in manufacturing techniques and health systems changes, some may be incontrovertible.
One technology in development for the treatment of SYN that does not require HLA matching is artificial adjuvant vector cells (aAVCs). aAVCs are loaded with an exogenous glycolipid ligand, α-galactosylceramide (α-GalCer), which is presented on a CD1d molecule and activates invariant natural killer T (iNKT) cells (Figure 2) (66). aAVCs also express a specific tumor-associated antigen. The α-GalCer synthetic ligand activating iNKT allows iNKT and natural killer (NK) cells to kill aAVCs, leading to the release of the tumor-associated antigen. Endogenous dendritic cells then serve as antigen presenting cells which allow for creation of CD4+ and CD8+ anti-tumor antigen T-cells. Previously, a Phase II trial of patients with non-small cell lung cancer infused with α-GalCer-pulsed Antigen Presenting Cells (APCs) showed efficacy (67). aAVCs that express NY-ESO-1 have been shown in a murine model to elicit NY-ESO-1 specific CD8+ T-cells as well as have an anti-tumor effect (68).
Figure 2 Mechanism of action of Artificial Adjuvant Vector Cells targeting NY-ESO-1 for treatment of SYN.
ASP0739 is an aAVC product targeting NY-ESO-1 being developed for treatment of SYN. Currently, a Phase I trial is in recruitment to test ASP0739 in patients with solid tumors including SYN, myxoid/round cell liposarcoma, ovarian carcinoma, non-small cell lung cancer, and esophageal squamous cell carcinoma (NCT04939701) (43). Phase II of the trial will use ASP0739 in combination with pembrolizumab, an antibody against PD-1 on lymphocytes that prevents de-activation of T-cells by tumors. While the results of these studies are yet to come, these trials will hopefully provide an additional therapeutic opportunity for treatment of SYN without the need for HLA matching.
BRD9 small molecule inhibitors are currently in development for the treatment of SYN (Figure 3). Mammalian SWI/SNF (mSWI/SNF or BAF) complexes are chromatin remodelers that allow for alterations in gene expression and DNA transcription. SS18-SSX fusion oncoprotein has been found to hijack the BAF complex, displacing wild-type SS18, resulting in changes in transcription and thus the development of SYN (69). These findings have led to the recognition of BAF complexes and specific subunits as potential targets for treatment of SYN (70).
BRD9 is a non-BET bromodomain protein and subunit of BAF complexes that has been recognized as a potential target for cancer treatment. In 2017, the first BRD9 chemical degrader was created that bridges the BRD9 bromodomain and E3 ubiquitin ligase complexes in vitro (71). Since then, numerous BRD9 inhibitors and have been developed (72–75). Degradation of BRD9 inhibits SYN tumor progression in a murine model (76). Therefore, BRD9 inhibition and/or degradation is a potential target for treatment of SYN.
CFT8634 is an oral heterobifunctional degrader that bridges BRD9 with E3 ligase, causing ubiquitination and proteasomal degradation of BRD9 (77). FHD-609 is an intravenous BRD9 degrader that bridges BRD9 with cereblon (CRBN) E3 ubiquitin ligase substrate that leads to proteasomal degradation (78). These therapies are currently undergoing Phase I trials for patients with advanced SYN (45, 46). The results of these trials are anticipated as potential therapies for treatment of SYN.
While standard of care treatment of advanced and metastatic SYN remains anthracycline based chemotherapy, there are numerous technologies in development for the treatment of advanced and metastatic SYN. These technologies stem from improved understanding of the tumor antigen expression and molecular mechanisms behind SYN. Engineered T-cell receptor therapies targeting CTAs has shown success in early-stage trials. Optimization of these engineered TCR treatments is currently being studied, with efforts to enhance T-cell antigen binding, alter the tumor microenvironment, and improve the quality of T-cells used for treatment. Alternative therapies without the need for HLA matching that are currently in recruitment for Phase I trials include aAVCs and BRD9 inhibitors.
Reviewing the new targeted and cellular therapies shows the tremendous progress that has been made over the preceding decades. Nonetheless, further study and qualification are required to ensure that we are doing the best for our patients. We anticipate that with the accelerated pace of discovery and application of new agents, treatment for patients with SYN will make remarkable strides in the upcoming years.
MA conceptualized the manuscript. JRF and JWF wrote the original draft. JRF, JWF, BS, and MA were responsible for writing and editing subsequent drafts and providing final approval for the manuscript. All authors contributed to the article and approved the submitted version.
Figures created in Biorender.com.
MA serves on the advisory board for Aadi Biosciences, Bayer, Deciphera and Regeneron. BS serves on the advisory board for Aadi Biosciences and provides consulting services for Caris Life Sciences.
The remaining authors declare that the research was conducted in the absence of any commercial or financial relationships that could be constructed as a potential conflict of interest.
All claims expressed in this article are solely those of the authors and do not necessarily represent those of their affiliated organizations, or those of the publisher, the editors and the reviewers. Any product that may be evaluated in this article, or claim that may be made by its manufacturer, is not guaranteed or endorsed by the publisher.
1. Mastrangelo G, Coindre JM, Ducimetière F, Dei Tos AP, Fadda E, Blay J-Y, et al. Incidence of soft tissue sarcoma and beyond: A population-based prospective study in 3 European regions. Cancer (2012) 118(21):5339–48. doi: 10.1002/cncr.27555
2. Toro JR, Travis LB, Wu HJ, Zhu K, Fletcher CDM, Devesa SS. Incidence patterns of soft tissue sarcomas, regardless of primary site, in the surveillance, epidemiology and end results program, 1978-2001: An analysis of 26,758 cases. Int J Cancer (2006) 119(12):2922–30. doi: 10.1002/ijc.22239
3. Sultan I, Rodriguez-Galindo C, Saab R, Yasir S, Casanova M, Ferrari A. Comparing children and adults with synovial sarcoma in the surveillance, epidemiology, and end results program, 1983 to 2005: An analysis of 1268 patients. Cancer (2009) 115(15):3537–47. doi: 10.1002/cncr.24424
4. Vlenterie M, Ho VKY, Kaal SEJ, Vlenterie R, Haas R, van der Graaf WTA. Age as an independent prognostic factor for survival of localised synovial sarcoma patients. Br J Cancer (2015) 113:1602–6. doi: 10.1038/bjc.2015.375
5. Palmerini E, Staals EL, Alberghini M, Zanella L, Ferrari C, Benassi MS, et al. Synovial sarcoma: Retrospective analysis of 250 patients treated at a single institution. Cancer (2009) 115(13):2988–98. doi: 10.1002/cncr.24370
6. Aytekin MN, Öztürk R, Amer K, Yapar A. Epidemiology, incidence, and survival of synovial sarcoma subtypes: SEER database analysis. J Orthop Surg (Hong Kong) (2020) 28(2):2988–98. doi: 10.1177/2309499020936009
7. Smolle MA, Parry M, Jeys L, Abudu S, Grimer R. Synovial sarcoma: Do children do better? Eur J Surg Oncol (2018) 45(2):254–60. doi: 10.1016/j.ejso.2018.07.006
8. Sun B, Sun Y, Wang J, Zhao X, Zhang S, Liu Y, et al. The diagnostic value of SYT-SSX detected by reverse transcriptase–polymerase chain reaction (RT-PCR) and fluorescence in situ hybridization (FISH) for synovial sarcoma: A review and prospective study of 255 cases. Cancer Sci (2008) 99:1355–61. doi: 10.1111/j.1349-7006.2008.00830.x
9. Dos Santos NR, de Bruijn DRH, van Kessel AG. Molecular mechanisms underlying human synovial sarcoma development. Gene Chromosome Canc (2000) 30(1):1–14. doi: 10.1002/1098-2264(2000)9999:9999<::AID-GCC1056>3.0.CO;2-G
10. Saito T, Nagai M, Ladanyi M. SYT-SSX1 and SYT-SSX2 interfere with repression of e-cadherin by snail and slug: A potential mechanism for aberrant mesenchymal to epithelial transition in human synovial sarcoma. Cancer Res (2006) 66(14):6919–27. doi: 10.1158/0008-5472.CAN-05-3697
11. Ladanyi M, Antonescu CR, Leung DH, Woodruff JM, Kawai A, Healey JH, et al. Impact of SYT-SSX fusion type on the clinical behavior of synovial sarcoma: A multi-institutional retrospective study of 243 patients. Cancer Res (2002) 62(1):135–40.
12. Canter RJ, Qin L-X, Maki RG, Brennan MF, Ladanyi M, Singer S. A synovial sarcoma-specific preoperative nomogram supports a survival benefit to ifosfamide-based chemotherapy and improves risk stratification for patients. Clin Cancer Res (2008) 14(24):8191–7. doi: 10.1158/1078-0432.CCR-08-0843
13. von Mehren M, Kane JM, Agulnik M, Bui MM, Carr-Ascher J, Choy E, et al. Soft tissue sarcoma, version 2.2022, NCCN clinical practice guidelines in oncology. J Natl Compr Canc Netw (2022) 20:815–33. doi: 10.6004/jnccn.2022.0035
14. Ferrari A, Gronchi A, Casanova M, Meazza C, Gandola L, Collini P, et al. Synovial sarcoma: A retrospective analysis of 271 patients of all ages treated at a single institution. Cancer (2004) 101(3):627–34. doi: 10.1002/cncr.20386
15. Eilber FC, Brennan MF, Eilber FR, Eckardt JJ, Grobmyer SR, Riedel E, et al. Chemotherapy is associated with improved survival in adult patients with primary extremity synovial sarcoma. Ann Surg (2007) 246(1):105–13. doi: 10.1097/01.sla.0000262787.88639.2b
16. Sleijfer S, Ouali M, van Glabbeke M, Krarup-Hansen A, Rodenhuis S, Le Cesne A, et al. Prognostic and predictive factors for outcome to first-line ifosfamide-containing chemotherapy for adult patients with advanced soft tissue sarcomas: an exploratory, retrospective analysis on large series from the European organization for research and treatment of cancer-soft tissue and bone sarcoma group (EORTC-STBSG). Eur J Cancer (2009) 46(1):72–83. doi: 10.1016/j.ejca.2009.09.022
17. Stojadinovic A, Leung DHY, Allen P, Lewis JJ, Jaques DP, Brennan MF. Primary adult soft tissue sarcoma: Time-dependent influence of prognostic variables. J Clin Oncol (2002) 20(21):4344–52. doi: 10.1200/JCO.2002.07.154
18. Krieg AH, Hefti F, Speth BM, Jundt G, Guillou L, Exner UG, et al. Synovial sarcomas usually metastasize after >5 years: a multicenter retrospective analysis with minimum follow-up of 10 years for survivors. Ann Oncol (2011) 22(2):458–67. doi: 10.1093/annonc/mdq394
19. Vlenterie M, Litière S, Rizzo E, Marréaud S, Judson I, Gelderblom H, et al. Outcome of chemotherapy in advanced synovial sarcoma patients: Review of 15 clinical trials from the European organisation for research and treatment of cancer soft tissue and bone sarcoma group; setting a new landmark for studies in this entity. Eur J Cancer (2016) 58:62–72. doi: 10.1016/j.ejca.2016.02.002
20. van der Graaf WTA, Blay JY, Chawla SP, Kim DW, Bui-Nguyen B, Casali PG, et al. Pazopanib for metastatic soft-tissue sarcoma (PALETTE): A randomised, double-blind, placebo-controlled phase 3 trial. Lancet (2012) 379(9829):1879–86. doi: 10.1016/S0140-6736(12)60651-5
21. Jungbluth AA, Antonescu CR, Busam KJ, Iversen K, Kolb D, Coplan K, et al. Monophasic and biphasic synovial sarcomas abundantly express cancer/testis antigen ny-eso-1 but not mage-a1 or ct7. Int J Cancer (2001) 95(2):252–6. doi: 10.1002/ijc.1451
22. Chen YT, Güre AO, Tsang S, Stockert E, Jäger E, Knuth A, et al. Identification of multiple cancer/testis antigens by allogeneic antibody screening of a melanoma cell line library. Proc Natl Acad Sci USA (1998) 95(12):6919–23. doi: 10.1073/pnas.95.12.6919
23. Jäger E, Nagata Y, Gnjatic S, Wada H, Stockert E, Karbach J, et al. Monitoring CD8 T cell responses to NY-ESO-1: Correlation of humoral and cellular immune responses. Proc Natl Acad Sci USA (2000) 97:4760–5. doi: 10.1073/pnas.97.9.4760
24. Iura K, Maekawa A, Kohashi K, Ishii T, Bekki H, Otsuka H, et al. Cancer-testis antigen expression in synovial sarcoma: NY-ESO-1, PRAME, MAGEA4, and MAGEA1. Hum Pathol (2017) 61:130–9. doi: 10.1016/j.humpath.2016.12.006
25. Dudley ME, Yang JC, Sherry R, Hughes MS, Royal R, Kammula U, et al. Adoptive cell therapy for patients with metastatic melanoma: Evaluation of intensive myeloablative chemoradiation preparative regimens. J Clin Oncol (2008) 26(32):5233–9. doi: 10.1200/JCO.2008.16.5449
26. Dudley ME, Wunderlich JR, Robbins PF, Yang JC, Hwu P, Schwartzentruber DJ, et al. Cancer regression and autoimmunity in patients after clonal repopulation with antitumor lymphocytes. Science (2002) 298(5594):850–4. doi: 10.1126/science.1076514
27. Tran K, Zhou J, Durflinger KH, Langhan MM, Shelton TE, Wunderlich JR, et al. Minimally cultured tumor-infiltrating lymphocytes display optimal characteristics for adoptive cell therapy. J Immunother (2008) 31(8):742–51. doi: 10.1097/CJI.0b013e31818403d5
28. Chen YT, Scanlan MJ, Sahin U, Tureci O, Gure A, Tsang S. A testicular antigen aberrantly expressed in human cancers detected by autologous antibody screening. Proc Natl Acad Sci USA (1997) 94(5):1914–8. doi: 10.1073/pnas.94.5.1914
29. D’Angelo S, Demetri G, Tine BV, Druta M, Glod J, Chow W, et al. 298 final analysis of the phase 1 trial of NY-ESO-1–specific T-cell receptor (TCR) T-cell therapy (letetresgene autoleucel; GSK3377794) in patients with advanced synovial sarcoma (SS). J Immunother Cancer (2020) 8:A182. doi: 10.1136/jitc-2020-SITC2020.0298
30. US National Library of Medicine. Chemotherapy followed by ESO-1 lymphocytes and adesleukin to treat metastatic cancer (2019). Available at: https://clinicaltrials.gov/ct2/show/NCT00670748 (Accessed December 6, 2022).
31. Robbins PF, Kassim SH, Tran TLN, Crystal JS, Morgan RA, Feldman SA, et al. A pilot trial using lymphocytes genetically engineered with an NY-ESO-1-reactive T-cell receptor: long-term follow-up and correlates with response. Clin Cancer Res (2015) 21(5):1019–27. doi: 10.1158/1078-0432.CCR-14-2708
32. Mackall C, Tap WD, Glod J, Druta M, Chow WA, Araujo DM, et al. Open label, non-randomized, multi-cohort pilot study of genetically engineered NY-ESO-1 specific NY-ESO-1c259t in HLA-A2+ patients with synovial sarcoma (NCT01343043). J Clin Oncol (2017) 35(15):3000–0. doi: 10.1200/JCO.2017.35.15_suppl.3000
33. Wermke M, Tsimberidou AM, Mohamed A, Mayer-Mokler A, Satelli A, Reinhardt C, et al. Safety and anti-tumor activity of TCR-engineered autologous, PRAME-directed T cells across multiple advanced solid cancers at low doses – clinical update on the ACTengine® IMA203 trial. J Immunother Cancer (2021) 9:A1009. doi: 10.1136/jitc-2021-SITC2021.959
34. Hong DS, Van Tine BA, Olszanski AJ, Johnson ML, Liebner DA, Trivedi T, et al. Phase I dose escalation and expansion trial to assess the safety and efficacy of ADP-A2M4 SPEAR T cells in advanced solid tumors. J Clin Oncol (2020) 38(15):102–2. doi: 10.1200/JCO.2020.38.15_suppl.102
35. US National Library of Medicine. MAGE-A4c1o32T for multi-tumor (2022). Available at: https://clinicaltrials.gov/ct2/show/NCT03132922 (Accessed December 6, 2022).
36. US National Library of Medicine. Spearhead 1 study in subjects with advanced synovial sarcoma or Mxyoid/Round cell liposarcoma (2022). Available at: https://clinicaltrials.gov/ct2/show/NCT04044768 (Accessed December 6, 2022).
37. D’Angelo SP, Attia S, Blay JY, Strauss SJ, Valverde Morales CM, Abdul Razak AR, et al. Identification of response stratification factors from pooled efficacy analyses of afamitresgene autoleucel (“Afami-cel” [Formerly ADP-A2M4]) in metastatic synovial sarcoma and myxoid/round cell liposarcoma phase 1 and phase 2 trials. J Clin Onc (2022) 40(16):11562–2. doi: 10.1200/JCO.2022.40.16_suppl.11562
38. Robbins PF, Morgan RA, Feldman SA, Yang JC, Sherry RM, Dudley ME, et al. Tumor regression in patients with metastatic synovial cell sarcoma and melanoma using genetically engineered lymphocytes reactive with NY-ESO-1. J Clin Oncol (2011) 29(7):917–24. doi: 10.1200/JCO.2010.32.2537
39. D’Angelo SP, Melchiori L, Merchant MS, Bernstein D, Glod J, Kaplan R, et al. Antitumor activity associated with prolonged persistence of adoptively transferred NY-ESO-1 c259T cells in synovial sarcoma. Cancer Discovery (2018) 8(8):944–57. doi: 10.1158/2159-8290.CD-17-1417
40. Locke FL, Miklos DB, Jacobson CA, Perales MA, Kersten MJ, Oluwole OO, et al. Axicabtagene ciloleucel as second-line therapy for Large b-cell lymphoma. N Engl J Med (2022) 386:640–54. doi: 10.1056/NEJMoa2116133
41. Araujo DM, Ladle BH, He K, Powers B, McGillivray A, Mitrica I, et al. ZENYTH-ESO: Master protocol to assess the safety and recommended phase II dose of next generation NY-ESO-1-specific TCR T-cells in HLA-A*02 patients with synovial sarcoma and myxoid/round cell liposarcoma [Substudy 3, GSK4427296]. J Clin Oncol (2022) 40(16). doi: 10.1200/JCO.2022.40.16_suppl.TPS2681
42. US National Library of Medicine. Master protocol to assess safety and dose of first time in human next generation engineered T cells in NY-ESO-1 and/or LAGE-1a positive advanced solid tumor (2022). Available at: https://clinicaltrials.gov/ct2/show/NCT04526509 (Accessed December 6, 2022).
43. US National Library of Medicine. Study of ASP0739 aline and with pembrolizumab in advanced solid tumors with NY-ESO-1 expression participants (2022). Available at: https://clinicaltrials.gov/ct2/show/NCT04939701 (Accessed December 6, 2022).
44. US National Library of Medicine. Safety and efficacy of IMC-F106C as a single agent and in combination with checkpoint inhibitors (2022). Available at: https://clinicaltrials.gov/ct2/show/study/NCT04262466 (Accessed December 6, 2022).
45. US National Library of Medicine. A study to assess the safety and tolerability of CFT8634 in locally advanced or metastatic SMARCB1-perturbed cancers, including synovial sarcoma and SMARCB1-null tumors (2022). Available at: https://clinicaltrials.gov/ct2/show/NCT05355753 (Accessed December 5, 2022).
46. US National Library of Medicine. FHD-609 in subjects with advanced synovial sarcoma or advanced SMARCB1-loss tumors (2022). Available at: https://clinicaltrials.gov/ct2/show/NCT04965753 (Accessed December 5, 2022).
47. Sun J, Kavathas PB. Comparison of the roles of CD8 alpha alpha and CD8 alpha beta in interaction with MHC class I. J Immunol (1997) 159(12):6077–82. doi: 10.4049/jimmunol.159.12.6077
48. Wooldridge L, van den Berg HA, Glick M, Gostick E, Laugel B, Hutchinson SL, et al. Interaction between the CD8 coreceptor and major histocompatibility complex class I stabilizes T cell receptor-antigen complexes at the cell surface. J Biol Chem (2005) 280(30):27491–501. doi: 10.1074/jbc.M500555200
49. Bajwa G, Gunesch J, Azoulay-Alfaguter I, Mata M, Mohamed A, Kalra M, et al. Improved anti-tumor activity of next-generation TCR-engineered T cells through CD8 co-expression. J Immunother Cancer (2021) 9:A1–A1054. doi: 10.1136/jitc-2021-SITC2021.163
50. Bollard CM, Rössig C, Calonge MJ, Huls MH, Wagner HJ, Massague J, et al. Adapting a transforming growth factor β–related tumor protection strategy to enhance antitumor immunity. Blood (2002) 99(9):3179–87. doi: 10.1182/blood.V99.9.3179
51. Bendle GM, Linnemann C, Bies L, Song JY, Schumacher TNM. Blockade of TGF-β signaling greatly enhances the efficacy of TCR gene therapy of cancer. J Immunol (2013) 191(6):3232–9. doi: 10.4049/jimmunol.1301270
52. Krishna S, Lowery FJ, Copeland AR, Bahadiroglu E, Mukherjee R, Jia L, et al. Stem-like CD8 T cells mediate response of adoptive cell immunotherapy against human cancer. Science (2020) 370:1328–34. doi: 10.1126/science.abb9847
53. Patel Y, Harris B, Bedard M, Kritikou J, Casas MG, Harms C, et al. The epi-RTM technology produces a polyclonal TIL product (LYL845) with a greater expansion success rate across hot and cold tumors, improved product phenotype, and maintenance of TCR diversity. J Immunother Cancer (2022) 10:A389. doi: 10.1136/jitc-2022-SITC2022.0370
54. Griffioen M, Kessler JH, Borghi M, van Soest RA, van der Minne CE, Nouta J, et al. Detection and functional analysis of CD8+ T cells specific for PRAME: A target for T-cell therapy. Clin Cancer Res (2006) 12(10):3130–6. doi: 10.1158/1078-0432.CCR-05-2578
55. Roszik J, Wang WL, Livingston JA, Roland CL, Ravi V, Yee C, et al. Overexpressed PRAME is a potential immunotherapy target in sarcoma subtypes. Clin Sarcoma Res (2017) 7(11):11. doi: 10.1186/s13569-017-0077-3
56. Wadelin F, Fulton J, McEwan PA, Spriggs KA, Emsley J, Heery DM. Leucine-rich repeat protein PRAME: Expression, potential functions and clinical implications for leukaemia. Mol Cancer (2010) 9:226. doi: 10.1186/1476-4598-9-226
57. US National Library of Medicine. ACTengine® IMA203/IMA203CD8 as monotherapy or in combination with nivolumab in recurrent and/or refractory solid tumors (ACTengine) (2022). Available at: https://clinicaltrials.gov/ct2/show/record/NCT03686124 (Accessed December 6, 2022).
58. Marcar L, Ihrig B, Hourihan J, Bray SE, Quinlan PR, Jordan LB, et al. MAGE-a Cancer/Testis antigens inhibit MDM2 ubiquitylation function and promote increased levels of MDM4. PloS One (2015) 10:e0127713. doi: 10.1371/journal.pone.0127713
59. Marcar L, MacLaine NJ, Hupp TR, Meek DW. Mage-a Cancer/Testis antigens inhibit p53 function by blocking its interaction with chromatin. Cancer Res (2010) 70:10362–70. doi: 10.1158/0008-5472.CAN-10-1341
60. Weon JL, Potts PR. The MAGE protein family and cancer. Curr Opin Cell Biol (2015) 37:1–8. doi: 10.1016/j.ceb.2015.08.002
61. Saito T, Wada H, Yamasaki M, Miyata H, Nishikawa H, Sato E, et al. High expression of MAGE-A4 and MHC class I antigens in tumor cells and induction of MAGE-A4 immune responses are prognostic markers of CHP-MAGE-A4 cancer vaccine. Vaccine (2014) 32(45):5901–7. doi: 10.1016/j.vaccine.2014.09.002
62. Kakimoto T, Matsumine A, Kageyama S, Asanuma K, Matsubara T, Nakamura T, et al. Immunohistochemical expression and clinicopathological assessment of the cancer testis antigens NY-ESO-1 and MAGE-A4 in high-grade soft-tissue sarcoma. Oncol Lett (2019) 17:3937–43. doi: 10.3892/ol.2019.10044
63. Cao K, Hollenbach J, Shi X, Shi W, Chopek M, Fernández-Viña MA. Analysis of the frequencies of HLA-a, b, and c alleles and haplotypes in the five major ethnic groups of the united states reveals high levels of diversity in these loci and contrasting distribution patterns in these populations. Hum Immunol (2001) 62:1009–30. doi: 10.1016/S0198-8859(01)00298-1
64. Mahalingam P, Julve M, Huang P, Furness AJS, Pollack SM, Jones RL. Immunotherapy of sarcomas with modified T cells. Curr Opin Oncol (2022) 34:362–70. doi: 10.1097/CCO.0000000000000843
65. Perica K, Varela JC, Oelke M, Schneck J. Adoptive T cell immunotherapy for cancer. Rambam Maimonides Med J (2015) 6:e0004. doi: 10.5041/RMMJ.10179
66. Fujii S, Shimizu K. Immunotherapy with artificial adjuvant vector cells. OncoImmunology (2013) 2:e23432. doi: 10.4161/onci.23432
67. Toyoda T, Kamata T, Tanaka K, Ihara F, Takami M, Suzuki H, et al. Phase II study of α-galactosylceramide-pulsed antigen-presenting cells in patients with advanced or recurrent non-small cell lung cancer. J Immunother Cancer (2020) 8:e000316. doi: 10.1136/jitc-2019-000316
68. Fujii S, Yamasaki S, Hanada K, Ueda S, Kawamura M, Shimizu K. Cancer immunotherapy using artificial adjuvant vector cells to deliver NY-ESO-1 antigen to dendritic cells in situ. Cancer Sci (2022) 113(3):864–74. doi: 10.1111/cas.15259
69. McBride MJ, Pulice JL, Beird HC, Ingram DR, D’Avino AR, Shern JF, et al. The SS18-SSX fusion oncoprotein hijacks BAF complex targeting and function to drive synovial sarcoma. Cancer Cell (2018) 33(6):1128–1141.e7. doi: 10.1016/j.ccell.2018.05.002
70. Michel BC, D’Avino AR, Cassel SH, Mashtalir N, McKenzie ZM, McBride MJ, et al. A non-canonical SWI/SNF complex is a synthetic lethal target in cancers driven by BAF complex perturbation. Nat Cell Biol (2018) 20:1410–20. doi: 10.1038/s41556-018-0221-1
71. Remillard D, Buckley DL, Paulk J, Brien GL, Sonnett M, Seo HS, et al. Degradation of the BAF complex factor BRD9 by heterobifunctional ligands. Angew Chem Int Ed Engl (2017) 56:5738–43. doi: 10.1002/anie.201611281
72. Theodoulou NH, Bamborough P, Bannister AJ, Becher I, Bit RA, Che KH, et al. Discovery of I-BRD9, a selective cell active chemical probe for bromodomain containing protein 9 inhibition. J Med Chem (2016) 59(4):1425–39. doi: 10.1021/acs.jmedchem.5b00256
73. Hay AD, Rogers CM, Fedorov O, Tallant C, Martin S, Monteiro OP, et al. Design and synthesis of potent and selective inhibitors of BRD7 and BRD9 bromodomains. MedChemComm (2015) 6:1381–6. doi: 10.1039/C5MD00152H
74. Clark PGK, Vieira LCC, Tallant C, Fedorov O, Singleton DC, Rogers CM, et al. Discovery and synthesis of the first selective BRD7/9 bromodomain inhibitor. Angewandte Chemie (2015) 127:6315–9. doi: 10.1002/ange.201501394
75. Martin LJ, Koegl M, Bader G, Cockcroft X-L, Fedorov O, Fiegen D, et al. Structure-based design of an in vivo active selective BRD9 inhibitor. J Med Chem (2016) 59(10):4462–75. doi: 10.1021/acs.jmedchem.5b01865
76. Brien GL, Remillard D, Shi J, Hemming ML, Chabon J, Wynne K, et al. Targeted degradation of BRD9 reverses oncogenic gene expression in synovial sarcoma. eLife (2018) 7:1–26. doi: 10.7554/eLife.41305
77. Jackson KL, Agafonov RV, Carlson MW, Chaturvedi P, Cocozziello D, Cole K, et al. Abstract ND09: The discovery and characterization of CFT8634: A potent and selective degrader of BRD9 for the treatment of SMARCB1-perturbed cancers. Cancer Res (2022) 82. doi: 10.1158/1538-7445.AM2022-ND09
78. Collins M, Wan J, Garbitt-Amaral V, Antonakos B, Wu HJ, Xu C, et al. (2022). Preclinical validation of target engagement assay and investigation of mechanistic impacts of FHD-609, a clinical-stage BD9 degrader being developed for the treatment of synovial sarcoma, in: Connective Tissue Oncology Society Annual Meeting, Vancouver, Canada, 2022 Nov 17.
Keywords: synovial sarcoma, soft tissue sarcoma, therapeutics, clinical trial, adoptive cell transfer
Citation: Fuchs JR, Schulte BC, Fuchs JW and Agulnik M (2023) Emerging targeted and cellular therapies in the treatment of advanced and metastatic synovial sarcoma. Front. Oncol. 13:1123464. doi: 10.3389/fonc.2023.1123464
Received: 14 December 2022; Accepted: 09 January 2023;
Published: 25 January 2023.
Edited by:
Brian Van Tine, Washington University in St. Louis, United StatesReviewed by:
Zhen Wang, Beijing Cancer Hospital, ChinaCopyright © 2023 Fuchs, Schulte, Fuchs and Agulnik. This is an open-access article distributed under the terms of the Creative Commons Attribution License (CC BY). The use, distribution or reproduction in other forums is permitted, provided the original author(s) and the copyright owner(s) are credited and that the original publication in this journal is cited, in accordance with accepted academic practice. No use, distribution or reproduction is permitted which does not comply with these terms.
*Correspondence: Mark Agulnik, bWFndWxuaWtAY29oLm9yZw==
Disclaimer: All claims expressed in this article are solely those of the authors and do not necessarily represent those of their affiliated organizations, or those of the publisher, the editors and the reviewers. Any product that may be evaluated in this article or claim that may be made by its manufacturer is not guaranteed or endorsed by the publisher.
Research integrity at Frontiers
Learn more about the work of our research integrity team to safeguard the quality of each article we publish.