- 1Tumor Stroma Interactions – Department of Cancer Research, Luxembourg Institute of HealthLuxembourg, Luxembourg
- 2Chronic Lymphocytic Leukemia Laboratory, Department of Hematology, Rigshospitalet, Copenhagen, Denmark
- 3PERSIMUNE, Department of Infectious Diseases, Rigshospitalet, Copenhagen, Denmark
- 4Instituto de Medicina Experimental (IMEX)-CONICET, Academia Nacional de Medicina, Buenos Aires, Argentina
- 5Department of Clinical Medicine, University of Copenhagen, Copenhagen, Denmark
Hairy cell leukemia (HCL) is an incurable, rare lymphoproliferative hematological malignancy of mature B cAlthough first line therapy with purine analogues leads to positive results, almost half of HCL patients relapse after 5-10 years, and standard treatment may not be an option due to intolerance or refractoriness. Proliferation and survival of HCL cells is regulated by surrounding accessory cells and soluble signals present in the tumor microenvironment, which actively contributes to disease progression. In vitro studies show that different therapeutic approaches tested in HCL impact the tumor microenvironment, and that this milieu offers a protection affecting treatment efficacy. Herein we explore the effects of the tumor microenvironment to different approved and experimental therapeutic options for HCL. Dissecting the complex interactions between leukemia cells and their milieu will be essential to develop new targeted therapies for HCL patients.
1 Introduction
1.1 Hairy cell leukemia
Representing approximately 2% of all leukemia cases worldwide, hairy cell leukemia (HCL) is an incurable lymphoproliferative B cell malignancy with an incidence rate of 0.3/100 000 in men and 0.1/100 000 in women (1, 2). The median age of HCL patients at diagnosis is close to 54 years (3, 4). The disease is characterized by the presence of abnormal B cells with hairy projections, which progressively accumulate in bone marrow (BM), spleen (causing splenomegaly) and other organs (e.g. liver) leading to a reduction of circulating erythrocytes, white blood cells, and platelets (known as pancytopenia) (5, 6). In contrast to chronic lymphocytic leukemia (CLL), HCL cells (HC) rarely infiltrate lymph nodes (3, 7). Patients affected by HCL can experience fatigue, increased risk of infections and bleeding due to anemia, leukopenia, and thrombocytopenia, respectively. Furthermore, HC infiltration in BM and other organs can lead to increased probability of fractures and impaired organ functions (3).
At diagnosis, HC are present at low frequency in peripheral blood (PB) and are characterized by the expression of typical B cell markers (like CD19, CD20, or CD22), as well as CD25, CD11c, CD103 and CD123, and by the mutation of the B-Raf proto-oncogene (BRAF, BRAFV600E) (8, 9). The latter, in particular, has been identified as a key mutation for the classic HCL subgroup (HCLc), while it is undetected in the variant form of HCL (HCLv) and in patients with IGHV4-34+. HCLv represents nearly 10% of all HCL cases, and up to 20% of patients belong to the IGHV4-34 molecular variant subgroup (10). BRAFV600E mutation has been identified within the hematopoietic stem cell compartment, suggesting an early transformation stage leading to HCLc (11). HCLv (12) and IGHV4-34 (13) groups display a distinct molecular pathogenesis (14). Beyond BRAFV600E mutation, HC express the anti-apoptotic B-cell lymphoma 2 (BCL-2) protein, a well-studied inhibitor of cell death that sustains cell survival, tumor growth and cancer disease progression (15–17). Standard treatment of HCL with cladribine (CDA) or pentostatin (2’-deoxycoformycin, DCF), alone or in combination with anti-CD20 (rituximab) immunotherapy, leads to remission in the vast majority of patients with certain subgroups of HCL patients can have a life expectation close to healthy individuals (18). However, no plateau on progression-free survival (PFS) curves has been achieved, thus most patients eventually relapse (19). Furthermore, the combined immune deficiencies due to HCL itself and to the treatments lead to high risk of infections during the first months after initiating therapy with CDA or DCF (20). This opens up to novel therapy strategies (21–24), clinical trials (detailed in Table 1), and basic research studies (25).
1.2 Characteristics of the tumor microenvironment in HCL
Over the last decades, there has been an evident broadening in the research interests and in the design of treatment strategies from studying exclusively tumor cells, to also consider components of the surrounding microenvironment. This includes deep characterization of different cellular subsets and soluble components, as well as understanding the complex communicational network within the tumor microenvironment (TME) (26, 27). CLL represents one clear case of such shift to this new wider understanding and design in therapies (28).
In HCL, the BM represents a key anatomical site for the disease, where malignant cells proliferate and survive thanks to physical protection and constitutive signals provided from the different microenvironment cells. Sinusoidal endothelial cells, mesenchymal stromal cells (BMSCs) and osteoclasts, express high amounts of CXCL12. This allows hematopoietic stem cells (HSCs) to migrate from the endosteal to the vascular niche replenishing the pool of mature circulating blood cells (29). Given their high expression of CXCR4, HC are strongly attracted to the BM, as well as to the splenic and hepatic niches, where they physically interact with sinusoid cells expressing vascular cell adhesion molecule 1 (VCAM-1) (30). The absence of HC in lymph nodes is due to the lack of expression of the chemokine receptors CXCR5 and CCR7 (31). HC release tumor necrosis factor alpha (TNF-α), which stimulates VCAM-1 expression on surrounding endothelial cells, increasing tumor cell migration in situ (32). Beyond stimulating malignant B cell migration, BMSCs sustain HC survival and proliferation by interacting with the integrin α4β1 (very late antigen-4, VLA-4), expressed on malignant cells, triggering mitogen activated protein (MAP) kinases and the nuclear factor kappa-light-chain-enhancer of activated B cells (NF-κB) downstream pathway (33). To further sustain HC interaction with the extracellular matrix and sinusoidal endothelial cells in the microenvironment, tumor cells express CD44 that binds to hyaluronic acid, present in both BM and hepatic niches, and the integrin αVβ3 binding the platelet/endothelial cell adhesion molecule 1 (PECAM-1) (34). Interactions between laminin and the basement membrane causes endothelial cell replacement by HC in the microenvironment (35). This represent a unique HCL vascular feature, taking place mainly in spleen (splenic pseudosinuses) and liver (hepatic hemangiomatous lesions) (36).
The T cell compartment is also altered in HCL (37). Thus, the expansion of T cells characterized by redundant T-cell receptor β variable region and high reactivity towards HC-surface CD40 results in a skewed T repertoire (38, 39). Given that CD40 downstream signals (MAPK and NF-κB pathways) are essential for HC proliferation (40), the expanded CD40L+ T cells in HCL are thought to have a tumor supportive function rather than being involved in disease suppression (37).
Furthermore, engagement of the B cell receptor (BCR) represents an important event during HCL pathogenesis. The vast majority of HCL patients show HC characterized by mutated immunoglobulin variable region genes (M-IGHV) (41, 42). The minor fraction of HCL cases with unmutated IGHV (UM-IGHV) display higher response to BCR stimulation compared with M-IGHV (43). Within the HCL microenvironment, BCR signaling could be potentially triggered by classical ligand interaction (e.g. auto-antigen) or through a ligand-independent fashion (tonic signaling). In both cases, BCR downstream signaling activates key kinases (SYK, BTK and PI3Kδ), leading to HC proliferation and survival. Moreover, BCR engagement also triggers the release of the chemokines CCL3 and CCL4, used to coordinate monocytes and T cell recruitment to the microenvironment (44–46).
2 Therapeutic options in HCL and the impact of the tumor microenvironment
2.1 Non-targeted agents for HCL: Cytokine alpha-interferon and purine analogs
Before the significant improvement in cancer therapy that occurred with the advent of purine analogs, HCL was mainly treated either through splenectomy, chemotherapy with chlorambucil, rubidazone or methotrexate (among other drugs with more limited efficacy, reviewed in (46) and (47)), or with immune response modifiers such as interferons (47, 48). In 1984, Quesada et al. (49) suggested the use of the cytokine alpha-interferon (IFN-α) by intramuscular route and in 2002, Baker and colleagues deepened into its mechanisms of action (50). They showed that IFN-α exerts its cell death effect on HC by triggering autocrine production of TNF-α and mediating a suppression of inhibitor of apoptosis protein-1 (IAP-1) expression (Figure 1A). Importantly, engagement of the receptors for fibronectin (FN) or vitronectin (VN) in HC prevented this IFN-α-induced downregulation of IAPs, reducing its cytotoxicity. The high abundance of FN and VN in the extracellular matrix of HCL patient’s spleen and BM (51), together with the constitutive expression of integrins at the surface of HC binding these ligands (52), evidence a microenvironment-mediated protection towards IFN-α treatment.
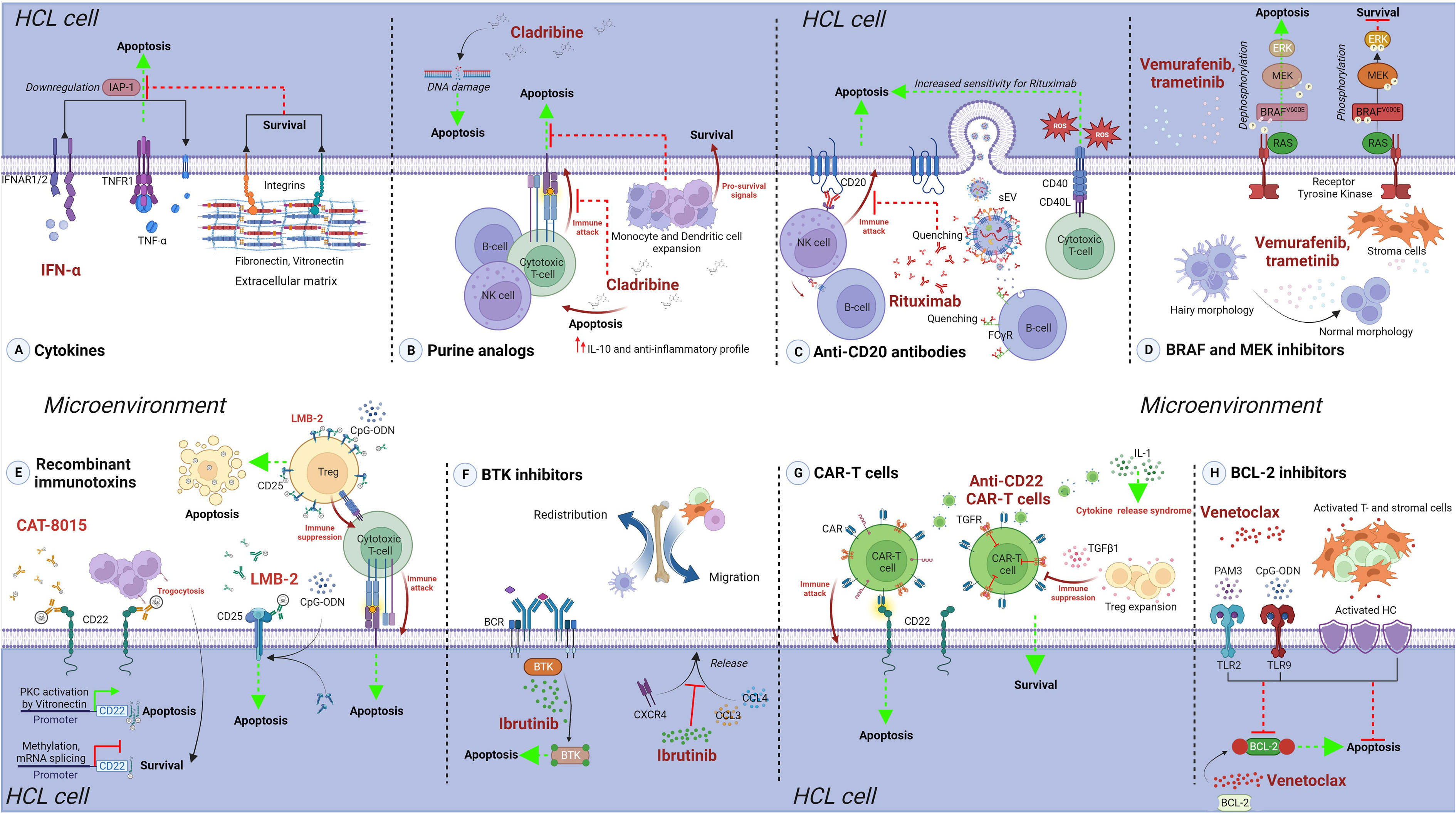
Figure 1 The protective role of the tumor microenvironment against treatments in HCL. Approved and experimental therapeutic options for HCL are presented, and details of microenvironment-mediated protection are provided. (A) Cytokines. IFN-α treatment efficacy is reduced due to engagement of HC to FN or VN receptors, present in the extracellular matrix of the spleen and bone marrow, inhibiting the IFN-α-mediated downregulation of IAPs, ultimately leading to reduced cell death. (B) Purine analogs. Cladribine off-targets effects could reduce the immune response against HC, mediated by cytotoxic T- and NK cells, and increase the levels of IL-10, promoting an anti-inflammatory profile. Indirect expansion of monocytes and dendritic cells can further favor HC survival. (C) Anti-CD20 antibodies. Monoclonal antibody Rituximab efficacy could be highly reduced in HCL due to the secretion of leukemia- and normal B cell-derived CD20+ sEV, as well as the higher expression of CD20 on normal B cells. On the other hand, CD40-CD40L interaction leads to increased sensitivity towards Rituximab in CLL and this could also be the case in HCL. (D) BRAF and MECK inhibitors. The pro-apoptotic effect of vemurafenib and trametinib is reduced by the presence of stromal cells in the microenvironment, which impair the dephosphorylation changes induced by these drugs. (E) Recombinant immunotoxins. The effect of these molecules could be affected by microenvironment modulation of the surface targets, e.g. CD22 availability during CAT-8015 treatment; or increased, e.g. CD25 upregulation during LMB-2 treatment in presence of CpG-ODN. Apoptosis of off-target cells, as regulatory T cells in the case of LMB-2, could indirectly influence the efficacy of the treatment. (F) BTK inhibitors. Ibrutinib treatment is highly efficient in affecting HC, but reduces the secretion of CCL3 and CCL4, and impairs CXCR4 signaling. This could possibly lead to redistribution of HC and other supporting cells in the microenvironment, possibly influencing other treatment regiments. (G) CAR-T cells. Anti-CD22 CAR-T therapy can be impaired by TGF-β1, directly affecting engineered T cells, as well as inducing Treg expansion. High levels of IL-1 in HCL microenvironment enhance the risk of CRS. (H) BCL-2 inhibitors. Venetoclax treatment efficacy is strongly reduced against HC stimulated with TLR2 and TLR9 ligands, as well as by the presence of activated T and stromal cells. Created with BioRender.com.
The introduction of purine analogs implied a major change in the disease outcome of HCL. Indeed, first line treatment with CDA is still the initial option in the majority of cases to date (1), more than 30 years after its initial use. Alternatively, DCF was widely used with excellent results as well, but preference towards CDA became more frequent probably due to a shorter administration scheme (53). The in vitro effect of CDA on PB mononuclear cells of healthy donors shows a reduced proliferative capacity of T and B cells, but not NK cells (54). CDA also impairs the activation and increases the apoptosis of T, B and NK cells in a dose-dependent manner, negatively affects dendritic cells (55), and modulates the cytokine response towards an anti-inflammatory profile (56). Thus, despite being highly effective against HC, CDA also causes severe harm to HCL microenvironment cells such as CD56+ NK cells, CD8+ and CD4+ T cell subsets, and induces profound changes in the composition of soluble factors including an increase in interleukin (IL)-10 production, overall reducing immune surveillance and function (Figure 1B). This is clinically reflected by the high risk of infections in the first weeks and months after treatment of HCL. Interestingly, a recent report making use of BM trephine samples from HCL patients before and after CDA therapy showed a reduction in tumor infiltrating NK and T cells, while proportions of monocytes and dendritic cells increase (57). Since monocytes and macrophages have the capacity to induce HC proliferation by direct interaction in vitro (58), these over represented myeloid cells could play a key role in sustaining the survival of remnant leukemic cells after CDA therapy.
2.2 Immunotherapy with anti-CD20 antibodies
HCL cases that relapse before 2 years after initial therapy, as well as HCLv patients, are treated with CDA plus rituximab (1). As first line therapy, this combination has so far showed promising results, which further improve when administered in a sequential scheme (59). Rituximab is an anti-CD20 monoclonal antibody successfully used in CLL and different B lymphomas (60) that exerts its cell death effect in normal and neoplastic B cells by initiating the complement cascade and, mainly, through antibody dependent cellular cytotoxicity (ADCC) mediated by NK and monocyte/macrophages (61). Another anti-CD20 tested in HCL is obinutuzumab (62, 63), a second generation monoclonal antibody currently undergoing two different clinical trials (NCT04578600 and NCT03410875, Table 1). Obinutuzumab was used in combination with chlorambucil for treatment-naive CLL patients, showing superiority as compared to chlorambucil plus rituximab (64), and was also tested in vitro, where an improved ADCC towards CLL cells was detected when it was compared with rituximab (65). Another second generation anti-CD20 antibody used for refractory or intolerant CLL cases is ofatumumab, which binds a CD20 epitope different from the CD20 binding site of rituximab and obinutuzumab, that partially overlap (66). To the best of our knowledge, ofatumumab has not been tested in HCL yet.
In CLL, microenvironment-mediated stimulation of leukemia cells through CD40 leads to an increase in their sensitivity to rituximab (67). On the other side, CD20+ small extracellular vesicles (sEV) released in the tumor microenvironment by both CLL and normal B cell have the capacity to quench this antibody and decrease its availability for neoplastic cells (68). These or other possible effects linking anti-CD20 antibodies to the TME in HCL have not been studied so far. It is reported, however, that expression of CD20 is higher in normal B cells than in HC (69). Therefore, it is expected that normal B cells will be negatively affected by anti-CD20 based therapies, and that the CD20+/hi sEV secreted by these cells will actively reduce their availability (Figure 1C). Additional effects that could be mediated by other leukocytes binding anti-CD20 antibodies through the Fc gamma Receptors (FcγR), involving mechanisms such as phagocytosis and cytokine release, remain to be elucidated in HCL.
2.3 Additional therapeutic options in HCL: BRAF and MEK inhibitors, immunotoxins, and BTK inhibitors
HCL patients from the classic group carry the BRAFV600E mutation, while it is virtually absent in other B-cell leukemias and lymphomas (9, 70). Inhibiting BRAF-related signaling pathways represents a very interesting therapeutic approach that has been tested in the last decade in HCL, showing promising results (71). Pettirossi and colleagues showed that vemurafenib and trametinib, BRAF and MEK inhibitors respectively, cause strong MEK/ERK dephosphorylation and silence the transcriptional output of the activated BRAF-MEK-ERK pathway leading to the loss of the hairy morphology and to apoptosis (29). Treatment of relapsed or refractory HCL with vemurafenib as monotherapy leads to high overall response rates and 1-year PFS above 70% (72); while the combination of vemurafenib with trametinib has reported 89% overall response rates with 2-year PFS of 94% (73). Interestingly, stromal cells can partially protect HC from the cell death effect induced by BRAF inhibition (Figure 1D). Indeed, dephosphorylation of the BRAF-MEK-ERK pathway by the BRAF inhibitors vemurafenib and dabrafenib is reduced when HC are co-cultured with the stroma cell line HS-5, decreasing the drug’s pro-apoptotic effect (29). Thus, cells present in the HCL tumor microenvironment have the capacity to thwart dephosphorylation changes induced by certain drugs used in therapy, having a concrete impact on leukemic cells survival.
Recombinant immunotoxins are engineered chimeric proteins formed by a monoclonal antibody fragment fused to toxin, such as Pseudomonas exotoxin A (PE) (74). Once bound to its target by the antibody part, immunotoxins are internalized inducing cell death by arrest of protein synthesis (75). In HCL, the first immunotoxin tested, LMB-2, is directed against CD25 and showed a marked cytotoxicity against HC in vitro (76). In patients, LMB-2 showed positive results and achieved, in some cases, complete remission (77, 78). Importantly, regulatory T cells (Tregs), expressing CD25, have a key role in tumor immunosuppression in different lymphoid malignancies such as CLL, and represent a target for novel therapeutic approaches (79). LMB-2 eliminates human PB-derived Tregs in vitro (80), and selectively reduces circulating and tumor infiltrating Tregs in melanoma patients (81). Little is known about Tregs in HCL and there is no information about the effect of LMB-2 towards this cell subset in HCL. Still, these works open the question if LMB-2 could have also an impact on the Tregs present in the HCL-TME, which may indirectly contribute to the efficacy of treatment (Figure 1E). On the other hand, stimulation of CLL cells with phosphorothioate CpG-oligodeoxynucleotide (CpG-ODN) increases their sensitivity to LMB-2 in vitro due to upregulation of CD25, an effect also seen to a lesser extent in normal B cells of healthy donors (82). It has not yet been tested in HCL whether TLR9 engagement of HC, or of normal B cells, affects LMB-2 treatment.
Another approach using immunotoxins in HCL is the case of CAT-8015, or Moxetumomab Pasudotox (Moxe), a fusion of the toxin PE to CD22 that showed improved efficacy compared to previous CD22-targeting immunotoxins (83). Clinical benefit was observed in relapsed/refractory HCL patients treated with Moxe in different studies (84, 85). The US Food and Drug Administration (FDA), approved Moxe under the name of Lumoxiti in 2017 for HCL patients after 2 or more prior systemic therapies with at least one being a purine analog. In acute lymphoblastic leukemia (ALL) and in CLL, Moxe showed a limited response rate, probably due to a lower CD22 expression (86–88). Protein kinase C (PKC) activation leads to upregulation of CD22 in CLL (89). In HCL, PKC is constitutively activated, in part due to the interaction of cell adhesion molecules of HC to VN (90), abundantly present in the extracellular matrix of the spleen (Figure 1E). This may explain the higher sensitivity to Moxe in HCL, and could imply a different response in key anatomical sites within this disease. Resistance to Moxe in ALL was also linked to alternative splicing of CD22 mRNA and to genome methylation (91, 92), while CD22 antigen downregulation in leukemic cells by monocyte trogocytosis via FcγR was described in the context of other anti-CD22 targeted therapies (93). Whether these mechanisms are also ongoing in HCL, remains to be experimentally tested.
The rationale of targeting the BCR signaling represents one of the most successful novel introductions for B-cell neoplasia therapy in the last decade. One example is the Bruton Tyrosine Kinase (BTK) inhibitor ibrutinib, approved for CLL and mantle cell lymphoma (94). In HCL, Sivina and colleagues showed that stimulation of the BCR signaling triggers BTK, ERK and AKT phosphorylation, and that ibrutinib decreases these effects, reducing HC survival (95). Interestingly, ibrutinib also impairs the secretion of CCL3 and CCL4, as well as CXCR4 signaling. These data suggest a possible impact of ibrutinib on the HCL microenvironment interaction at least on three levels: 1) by affecting BCR signaling induced by microenvironment (auto-)antigens; 2) by impairing tumor-supporting cell migration mediated by CCL3 and CCL4; and 3) by redistribution of leukemic cells as consequence of a thwarted CXCR4 cascade (Figure 1F). For the moment, ibrutinib monotherapy has been used in single cases of multiple relapse HCLv and in a multicenter trial (NCT01841723), showing clinical benefits (96, 97). Beyond ibrutinib, a second generation BTK inhibitor, acalabrutinib, is currently in one clinical trial for different hematological malignancies including HCL (NCT02362035). The final data of this trial is estimated to be available in two years.
2.4 Recent therapeutic options tested in HCL: CAR-T cells and BCL-2 inhibitors
A phase I study of anti-CD22 Chimeric Antigen Receptor-T (CAR-T) cells in patients with relapsed/refractory HCLc and in HCLv is currently being developed (NCT04815356), along with other CAR-T cell approaches (see Table 1). The T cell compartment in HCL has been associated to different dysfunctions and linked to a non-responsive state (98). Successful CAR-T therapies highly depend on the extent of immunosuppression within the tumor milieu (99, 100). In HCL, TGF-β1 is present at high levels in BM and PB (101), and this cytokine activates signals that severely hinder T-cell based therapies (102–104). On the other hand, IL-1 actively contributes to the cytokine release syndrome (CRS), which represents one of the main cytotoxic side-effects associated with CAR-T cell therapy (105). Serum levels of IL-1 increase during HCL progression and are elevated as compared to healthy donors and other leukemia and lymphoma patients (106), representing a risky “steady state” scenario that may favor the initiation of CRS (Figure 1G). Whether the CAR-T cell approaches will overcome these pitfalls and show patient benefit in HCL is currently an open question of the highest interest.
Venetoclax is a small drug that specifically binds the BH3-binding groove of BCL-2, competing with additional anti-apoptotic members. It is the first BCL-2 antagonist approved for cancer therapy, successfully used in CLL and in acute myeloid leukemia (AML) (107–109). In a recent study, we showed that venetoclax is able to induce cell death in primary HCL samples (25). Importantly, stimulation of T cells through CD3 engagement and co-cultures with HS-5 stromal cells activated primary HC and decreased the pro-apoptotic effect of venetoclax, clearly showing a protective effect of the tumor microenvironment towards BCL-2 inhibition. In addition, stimulation of TLR2 and TLR9, using PAM3 and CpG respectively, also partially rescued the cell death induced by venetoclax (Figure 1H). It is currently not known through which mechanisms the activation of HCL cells leads to protection towards BCL-2 inhibition. Venetoclax has been tested, in combination with ibrutinib, in one patient with biclonal IGHV4-34+ HCL and CLL, showing promising results (21), but is not currently under any clinical trial for HCL. To our knowledge, no other BH3 mimetics available for hematological malignancies (110) has been tested in HCL until the present.
3 Conclusions and perspectives
The TME exerts a protective effect towards some of the most relevant treatment options in HCL, both approved and ongoing experimental molecules, as summarized in Figure 1. This opens the path to consider combined therapies to simultaneously attack different TME components and HC.
As examples, interactions between HC and stromal cells could be tackled by different strategies (111), including TGF-β (112) or PKC-β inhibition (113); the latter being already tested in leukemia models using BM stromal cells and showing promising results. Since HC express high levels of CXCR4, interrupting its interaction with CXCL12 by blocking antibody (114), or a drug-mediated inhibition of this axis (115), represent interesting strategies to inhibit the homing of HC to BM. These approaches could be applied in combination with standard treatments directly inducing HC apoptosis. On the other hand, ibrutinib enhances CAR-T cell activity in CLL and in an in vivo model of resistant acute lymphocytic leukemia (116), and these therapies are included in different ongoing HCL clinical trials separately (Table 1), thus a combined regimen of these two treatments could be considered. Hitherto, the combination of CD20 targeting with cladribine, the targeting of the BRAF-MEK-ERK pathway, CD22 targeting by immunotoxins or CAR-T and BTK inhibition has been developed the furthest towards clinical targeting of the microenvironment in HCL.
In summary, given the vast potential of targetable pathways in the TME landscape of HCL, a new array of therapeutic possibilities remains to be tested in HCL. Due to the rarity of HCL, in addition to testing in clinical trials, any clinical use of such drugs outside trials should also be reported to speed up development of clinical options for patients with HCL. This perspective positions the interactions between HC and their milieu as a key aspect to target in order to increase therapeutic benefit for HCL patients.
Author contributions
EG and PM wrote the manuscript and created the figure. MG, CN, EM and JP finalized the writing. PM supervised the team. All authors contributed to the article and approved the submitted version.
Funding
This work was supported by grants from the Luxembourg National Research Fund (FNR) and Fondation Cancer to EG, EM and JP (PRIDE15/10675146/CANBIO, C20/BM/14582635, and C20/BM/14592342), from FNRS-Télévie and the European commission to PM (7.8506.19, H2020-MSCA-IF-2020: 101029602), from CONICET, Argentina to MG and from the Danish Cancer Society and the EU funded ERA PERMED program to CN.
Conflict of interest
CN received research funding and/or consultancy fees outside this work from Abbvie, AstraZeneca, Octapharma, Janssen, CSL Behring, Beigene, Genmab, Eli Lilly and Takeda.
The remaining authors declare that the research was conducted in the absence of any commercial or financial relationships that could be construed as a potential conflict of interest.
Publisher’s note
All claims expressed in this article are solely those of the authors and do not necessarily represent those of their affiliated organizations, or those of the publisher, the editors and the reviewers. Any product that may be evaluated in this article, or claim that may be made by its manufacturer, is not guaranteed or endorsed by the publisher.
Glossary
References
1. Troussard X, Maitre E, Cornet E. Hairy cell leukemia 2022: Update on diagnosis, risk-stratification, and treatment. Am J Hematol (2022) 97(2):226–36. doi: 10.1002/ajh.26390
2. Teras LR, DeSantis CE, Cerhan JR, Morton LM, Jemal A, Flowers CR. US Lymphoid malignancy statistics by world health organization subtypes. CA Cancer J Clin (2016) 66(6):443–59. doi: 10.3322/caac.21357
3. Campo E, Swerdlow SH, Harris NL, Pileri S, Stein H, Jaffe ES. The 2008 WHO classification of lymphoid neoplasms and beyond: evolving concepts and practical applications. Blood (2011) 117(19):5019–32. doi: 10.1182/blood-2011-01-293050
4. Troussard X, Grever MR. The revised guidelines for the diagnosis and management of hairy cell leukaemia and the hairy cell leukaemia variant. Br J Haematol (2021) 193(1):11–4. doi: 10.1111/bjh.17201
5. Bouroncle BA, Wiseman BK, Doan CA. Leukemic reticuloendotheliosis. Blood (1958) 13(7):609–30. doi: 10.1182/blood.V13.7.609.609
6. Schrek R, Donnelly WJ. “Hairy” cells in blood in lymphoreticular neoplastic disease and “flagellated” cells of normal lymph nodes. Blood (1966) 27(2):199–211. doi: 10.1182/blood.V27.2.199.199
7. Ponzoni M, Doglioni C, Caligaris-Cappio F. Chronic lymphocytic leukemia: the pathologist’s view of lymph node microenvironment. Semin Diagn Pathol (2011) 28(2):161–6. doi: 10.1053/j.semdp.2011.02.014
8. Matutes E, Morilla R, Owusu-Ankomah K, Houliham A, Meeus P, Catovsky D. The immunophenotype of hairy cell leukemia (HCL). proposal for a scoring system to distinguish HCL from b-cell disorders with hairy or villous lymphocytes. Leuk Lymphoma (1994) 14(Suppl 1):57–61.
9. Tiacci E, Trifonov V, Schiavoni G, Holmes A, Kern W, Martelli MP, et al. BRAF mutations in hairy-cell leukemia. N Engl J Med (2011) 364(24):2305–15. doi: 10.1056/NEJMoa1014209
10. Xi L, Arons E, Navarro W, Calvo KR, Stetler-Stevenson M, Raffeld M, et al. Both variant and IGHV4-34-expressing hairy cell leukemia lack the BRAF V600E mutation. Blood (2012) 119(14):3330–2. doi: 10.1182/blood-2011-09-379339
11. Chung SS, Kim E, Park JH, Chung YR, Lito P, Teruya-Feldstein J, et al. Hematopoietic stem cell origin of BRAFV600E mutations in hairy cell leukemia. Sci Transl Med (2014) 6(238):238ra71. doi: 10.1126/scitranslmed.3008004
12. Cawley JC, Burns GF, Hayhoe FG. A chronic lymphoproliferative disorder with distinctive features: a distinct variant of hairy-cell leukaemia. Leuk Res (1980) 4(6):547–59. doi: 10.1016/0145-2126(80)90066-1
13. Arons E, Suntum T, Stetler-Stevenson M, Kreitman RJ. VH4-34+ hairy cell leukemia, a new variant with poor prognosis despite standard therapy. Blood (2009) 114(21):4687–95. doi: 10.1182/blood-2009-01-201731
14. Waterfall JJ, Arons E, Walker RL, Pineda M, Roth L, Killian JK, et al. High prevalence of MAP2K1 mutations in variant and IGHV4-34-expressing hairy-cell leukemias. Nat Genet (2014) 46(1):8–10. doi: 10.1038/ng.2828
15. Zaja F, Di Loreto C, Amoroso V, Salmaso F, Russo D, Silvestri F, et al. BCL-2 immunohistochemical evaluation in b-cell chronic lymphocytic leukemia and hairy cell leukemia before treatment with fludarabine and 2-chloro-deoxy-adenosine. Leuk Lymphoma (1998) 28(5-6):567–72. doi: 10.3109/10428199809058365
16. Tessoulin B, Papin A, Gomez-Bougie P, Bellanger C, Amiot M, Pellat-Deceunynck C, et al. BCL2-family dysregulation in b-cell malignancies: From gene expression regulation to a targeted therapy biomarker. Front Oncol (2018) 8:645. doi: 10.3389/fonc.2018.00645
17. Strasser A, Vaux DL. Cell death in the origin and treatment of cancer. Mol Cell (2020) 78(6):1045–54. doi: 10.1016/j.molcel.2020.05.014
18. Bohn JP, Neururer S, Pirklbauer M, Pircher A, Wolf D. Hairy cell leukemia patients have a normal life expectancy-a 35-year single-center experience and comparison with the general population. Cancers (Basel) (2022) 14(5):1242. doi: 10.3390/cancers14051242
19. Maevis V, Mey U, Schmidt-Wolf G, Schmidt-Wolf IG. Hairy cell leukemia: short review, today’s recommendations and outlook. Blood Cancer J (2014) 4:e184. doi: 10.1038/bcj.2014.3
20. Epperla N, Pavilack M, Olufade T, Bashyal R, Li J, Kabadi SM, et al. Adverse event rates and economic burden associated with purine nucleoside analogs in patients with hairy cell leukemia: A US population-retrospective claims analysis. Orphanet J Rare Dis (2020) 15(1):47. doi: 10.1186/s13023-020-1325-9
21. Jain P, Kanagal-Shamanna R, Konoplev S, Zuo Z, Estrov Z. Biclonal IGHV-4-34 hairy cell leukemia variant and CLL - successful treatment with ibrutinib and venetoclax. Am J Hematol (2018) 93(12):1568–9. doi: 10.1002/ajh.25264
22. Kreitman RJ. Hairy cell leukemia: present and future directions. Leuk Lymphoma (2019) 60(12):2869–79. doi: 10.1080/10428194.2019.1608536
23. Sarvaria A, Saven A. Novel therapeutics in hairy cell leukemia. Expert Rev Hematol (2019) 12(11):983–7. doi: 10.1080/17474086.2019.1652589
24. Maitre E, Paillassa J, Troussard X. Novel targeted treatments in hairy cell leukemia and other hairy cell-like disorders. Front Oncol (2022) 12:1068981. doi: 10.3389/fonc.2022.1068981
25. Vereertbrugghen A, Colado A, Gargiulo E, Bezares RF, Fernandez Grecco H, Cordini G, et al. In vitro sensitivity to venetoclax and microenvironment protection in hairy cell leukemia. Front Oncol (2021) 11:598319. doi: 10.3389/fonc.2021.598319
26. Fowler NH, Cheah CY, Gascoyne RD, Gribben J, Neelapu SS, Ghia P, et al. Role of the tumor microenvironment in mature b-cell lymphoid malignancies. Haematologica (2016) 101(5):531–40. doi: 10.3324/haematol.2015.139493
27. Burger JA, Ghia P, Rosenwald A, Caligaris-Cappio F. The microenvironment in mature b-cell malignancies: A target for new treatment strategies. Blood (2009) 114(16):3367–75. doi: 10.1182/blood-2009-06-225326
28. Svanberg R, Janum S, Patten PEM, Ramsay AG, Niemann CU. Targeting the tumor microenvironment in chronic lymphocytic leukemia. Haematologica (2021) 106(9):2312–24. doi: 10.3324/haematol.2020.268037
29. Pettirossi V, Santi A, Imperi E, Russo G, Pucciarini A, Bigerna B, et al. BRAF inhibitors reverse the unique molecular signature and phenotype of hairy cell leukemia and exert potent antileukemic activity. Blood (2015) 125(8):1207–16. doi: 10.1182/blood-2014-10-603100
30. Vincent AM, Burthem J, Brew R, Cawley JC. Endothelial interactions of hairy cells: the importance of alpha 4 beta 1 in the unusual tissue distribution of the disorder. Blood (1996) 88(10):3945–52. doi: 10.1182/blood.V88.10.3945.bloodjournal88103945
31. Wong S, Fulcher D. Chemokine receptor expression in b-cell lymphoproliferative disorders. Leuk Lymphoma (2004) 45(12):2491–6. doi: 10.1080/10428190410001723449
32. Lindemann A, Ludwig WD, Oster W, Mertelsmann R, Herrmann F. High-level secretion of tumor necrosis factor-alpha contributes to hematopoietic failure in hairy cell leukemia. Blood (1989) 73(4):880–4. doi: 10.1182/blood.V73.4.880.880
33. Sivina M, Kreitman RJ, Peled A, Ravandi F, Burger JA. Adhesion of hairy cells leukemia (HCL) cells to stromal cells can be inhibited by blocking VLA-4 integrins and CXCR4 chemokine receptors. Blood (2011) 118(21):1760–. doi: 10.1182/blood.V118.21.1760.1760
34. Aziz KA, Till KJ, Zuzel M, Cawley JC. Involvement of CD44-hyaluronan interaction in malignant cell homing and fibronectin synthesis in hairy cell leukemia. Blood (2000) 96(9):3161–7. doi: 10.1182/blood.V96.9.3161
35. Karttunen T, Apaja-Sarkkinen M, Alavaikko M, Autio-Harmainen H. Altered basement membrane structure of the spleen in hairy cell leukaemia. demonstration of laminin in hairy cells. Pathol Res Pract (1987) 182(2):233–9. doi: 10.1016/S0344-0338(87)80110-3
36. Nanba K, Soban EJ, Bowling MC, Berard CW. Splenic pseudosinuses and hepatic angiomatous lesions. distinctive features of hairy cell leukemia. Am J Clin Pathol (1977) 67(5):415–26. doi: 10.1093/ajcp/67.5.415
37. Sabbe LJ, Meijer CJ, Jansen J. T Lymphocyte function in hairy cell leukaemia. Clin Exp Immunol (1980) 42(2):336–44.
38. van de Corput L, Kluin-Nelemans HC, Kester MG, Willemze R, Falkenburg JH. Hairy cell leukemia-specific recognition by multiple autologous HLA-DQ or DP-restricted T-cell clones. Blood (1999) 93(1):251–9. doi: 10.1182/blood.V93.1.251
39. Kluin-Nelemans JC, Kester MG, Melenhorst JJ, Landegent JE, van de Corput L, Willemze R, et al. Persistent clonal excess and skewed T-cell repertoire in T cells from patients with hairy cell leukemia. Blood (1996) 87(9):3795–802. doi: 10.1182/blood.V87.9.3795.bloodjournal8793795
40. Kluin-Nelemans HC, Beverstock GC, Mollevanger P, Wessels HW, Hoogendoorn E, Willemze R, et al. Proliferation and cytogenetic analysis of hairy cell leukemia upon stimulation via the CD40 antigen. Blood (1994) 84(9):3134–41. doi: 10.1182/blood.V84.9.3134.3134
41. Arons E, Roth L, Sapolsky J, Suntum T, Stetler-Stevenson M, Kreitman RJ. Evidence of canonical somatic hypermutation in hairy cell leukemia. Blood (2011) 117(18):4844–51. doi: 10.1182/blood-2010-11-316737
42. Forconi F, Sahota SS, Raspadori D, Ippoliti M, Babbage G, Lauria F, et al. Hairy cell leukemia: at the crossroad of somatic mutation and isotype switch. Blood (2004) 104(10):3312–7. doi: 10.1182/blood-2004-03-0950
43. Forconi F. Hairy cell leukaemia: biological and clinical overview from immunogenetic insights. Hematol Oncol (2011) 29(2):55–66. doi: 10.1002/hon.975
44. Burger JA, Quiroga MP, Hartmann E, Burkle A, Wierda WG, Keating MJ, et al. High-level expression of the T-cell chemokines CCL3 and CCL4 by chronic lymphocytic leukemia b cells in nurselike cell cocultures and after BCR stimulation. Blood (2009) 113(13):3050–8. doi: 10.1182/blood-2008-07-170415
45. Krzysiek R, Lefevre EA, Zou W, Foussat A, Bernard J, Portier A, et al. Antigen receptor engagement selectively induces macrophage inflammatory protein-1 alpha (MIP-1 alpha) and MIP-1 beta chemokine production in human b cells. J Immunol (1999) 162(8):4455–63. doi: 10.4049/jimmunol.162.8.4455
46. Bystry RS, Aluvihare V, Welch KA, Kallikourdis M, Betz AG. B cells and professional APCs recruit regulatory T cells via CCL4. Nat Immunol (2001) 2(12):1126–32. doi: 10.1038/ni735
47. Damasio EE, Pagnucco G, Federico M, Annino L, Chisesi T, Lamparelli T, et al. Hairy cell leukemia. a therapeutical update. Haematologica (1989) 74(2):205–18.
48. Andritsos LA, Grever MR. Historical overview of hairy cell leukemia. Best Pract Res Clin Haematol (2015) 28(4):166–74. doi: 10.1016/j.beha.2015.10.018
49. Quesada JR, Reuben J, Manning JT, Hersh EM, Gutterman JU. Alpha interferon for induction of remission in hairy-cell leukemia. N Engl J Med (1984) 310(1):15–8. doi: 10.1056/NEJM198401053100104
50. Baker PK, Pettitt AR, Slupsky JR, Chen HJ, Glenn MA, Zuzel M, et al. Response of hairy cells to IFN-alpha involves induction of apoptosis through autocrine TNF-alpha and protection by adhesion. Blood (2002) 100(2):647–53. doi: 10.1182/blood.V100.2.647
51. Burthem J, Cawley JC. The bone marrow fibrosis of hairy-cell leukemia is caused by the synthesis and assembly of a fibronectin matrix by the hairy cells. Blood (1994) 83(2):497–504. doi: 10.1182/blood.V83.2.497.497
52. Burthem J, Baker PK, Hunt JA, Cawley JC. Hairy cell interactions with extracellular matrix: expression of specific integrin receptors and their role in the cell’s response to specific adhesive proteins. Blood (1994) 84(3):873–82. doi: 10.1182/blood.V84.3.873.873
53. Kreitman RJ, Arons E. Diagnosis and treatment of hairy cell leukemia as the COVID-19 pandemic continues. Blood Rev (2022) 51:100888. doi: 10.1016/j.blre.2021.100888
54. Fissolo N, Calvo-Barreiro L, Eixarch H, Boschert U, Espejo C, Montalban X, et al. Immunomodulatory effects associated with cladribine treatment. Cells (2021) 10(12):3488. doi: 10.3390/cells10123488
55. Kraus SH, Luessi F, Trinschek B, Lerch S, Hubo M, Poisa-Beiro L, et al. Cladribine exerts an immunomodulatory effect on human and murine dendritic cells. Int Immunopharmacol (2014) 18(2):347–57. doi: 10.1016/j.intimp.2013.11.027
56. Korsen M, Bragado Alonso S, Peix L, Broker BM, Dressel A. Cladribine exposure results in a sustained modulation of the cytokine response in human peripheral blood mononuclear cells. PloS One (2015) 10(6):e0129182. doi: 10.1371/journal.pone.0129182
57. Koldej RM, Prabahran A, Tan CW, Ng AP, Davis MJ, Ritchie DS. Dissection of the bone marrow microenvironment in hairy cell leukaemia identifies prognostic tumour and immune related biomarkers. Sci Rep (2021) 11(1):19056. doi: 10.1038/s41598-021-98536-1
58. Griffiths SD, Cawley JC. Monocytes/Macrophages stimulate hairy-cell proliferation. Leuk Lymphoma (1991) 4(5-6):325–33. doi: 10.3109/10428199109068082
59. Chihara D, Kantarjian H, O’Brien S, Jorgensen J, Pierce S, Faderl S, et al. Long-term durable remission by cladribine followed by rituximab in patients with hairy cell leukaemia: update of a phase II trial. Br J Haematol (2016) 174(5):760–6. doi: 10.1111/bjh.14129
60. Salles G, Barrett M, Foa R, Maurer J, O’Brien S, Valente N, et al. Rituximab in b-cell hematologic malignancies: A review of 20 years of clinical experience. Adv Ther (2017) 34(10):2232–73. doi: 10.1007/s12325-017-0612-x
61. Pierpont TM, Limper CB, Richards KL. Past, present, and future of rituximab-the world’s first oncology monoclonal antibody therapy. Front Oncol (2018) 8:163. doi: 10.3389/fonc.2018.00163
62. Al-Sarayfi D, Meeuwes FO, Munnink TO, Plattel W, Rosati S, Matutes E, et al. Successful treatment of hairy cell leukemia variant with obinutuzumab. Ann Hematol (2022) 101(3):703–4. doi: 10.1007/s00277-021-04559-z
63. Bohn JP, Willenbacher E, Steurer M. Obinutuzumab in multidrug-resistant hairy cell leukemia. Ann Hematol (2016) 95(2):351–2. doi: 10.1007/s00277-015-2520-y
64. Goede V, Fischer K, Busch R, Engelke A, Eichhorst B, Wendtner CM, et al. Obinutuzumab plus chlorambucil in patients with CLL and coexisting conditions. N Engl J Med (2014) 370(12):1101–10. doi: 10.1056/NEJMoa1313984
65. Bologna L, Gotti E, Manganini M, Rambaldi A, Intermesoli T, Introna M, et al. Glycoengineered, anti-CD20 monoclonal antibody GA101 in b-chronic lymphocytic leukemia whole blood assays in comparison with rituximab and alemtuzumab. J Immunol (2011) 186(6):3762–9. doi: 10.4049/jimmunol.1000303
66. Osterborg A, Jewell RC, Padmanabhan-Iyer S, Kipps TJ, Mayer J, Stilgenbauer S, et al. Ofatumumab monotherapy in fludarabine-refractory chronic lymphocytic leukemia: final results from a pivotal study. Haematologica (2015) 100(8):e311–4. doi: 10.3324/haematol.2014.121459
67. Jak M, van Bochove GG, van Lier RA, Eldering E, van Oers MH. CD40 stimulation sensitizes CLL cells to rituximab-induced cell death. Leukemia (2011) 25(6):968–78. doi: 10.1038/leu.2011.39
68. Paggetti J, Haderk F, Seiffert M, Janji B, Distler U, Ammerlaan W, et al. Exosomes released by chronic lymphocytic leukemia cells induce the transition of stromal cells into cancer-associated fibroblasts. Blood (2015) 126(9):1106–17. doi: 10.1182/blood-2014-12-618025
69. Bellosillo B, Villamor N, Lopez-Guillermo A, Marce S, Esteve J, Campo E, et al. Complement-mediated cell death induced by rituximab in b-cell lymphoproliferative disorders is mediated in vitro by a caspase-independent mechanism involving the generation of reactive oxygen species. Blood (2001) 98(9):2771–7. doi: 10.1182/blood.V98.9.2771
70. Arcaini L, Zibellini S, Boveri E, Riboni R, Rattotti S, Varettoni M, et al. The BRAF V600E mutation in hairy cell leukemia and other mature b-cell neoplasms. Blood (2012) 119(1):188–91. doi: 10.1182/blood-2011-08-368209
71. Falini B, De Carolis L, Tiacci E. How I treat refractory/relapsed hairy cell leukemia with BRAF inhibitors. Blood (2022) 139(15):2294–305. doi: 10.1182/blood.2021013502
72. Handa S, Lee JO, Derkach A, Stone RM, Saven A, Altman JK, et al. Long term outcomes in patients with relapsed or refractory hairy cell leukemia treated with vemurafenib monotherapy. Blood (2022) 140(25):2663–71. doi: 10.1182/blood.2022016183
73. Kreitman RJ, Moreau P, Ravandi F, Hutchings M, Gazzah A, Michallet AS, et al. Dabrafenib plus trametinib in patients with relapsed/refractory BRAF V600E mutation-positive hairy cell leukemia. Blood (2022), blood.2021013658. doi: 10.1182/blood.2021013658
74. Havaei SM, Aucoin MG, Jahanian-Najafabadi A. Pseudomonas exotoxin-based immunotoxins: Over three decades of efforts on targeting cancer cells with the toxin. Front Oncol (2021) 11:781800. doi: 10.3389/fonc.2021.781800
75. Akbari B, Farajnia S, Ahdi Khosroshahi S, Safari F, Yousefi M, Dariushnejad H, et al. Immunotoxins in cancer therapy: Review and update. Int Rev Immunol (2017) 36(4):207–19. doi: 10.1080/08830185.2017.1284211
76. Robbins DH, Margulies I, Stetler-Stevenson M, Kreitman RJ. Hairy cell leukemia, a b-cell neoplasm that is particularly sensitive to the cytotoxic effect of anti-Tac(Fv)-PE38 (LMB-2). Clin Cancer Res (2000) 6(2):693–700.
77. Kreitman RJ, Wilson WH, Robbins D, Margulies I, Stetler-Stevenson M, Waldmann TA, et al. Responses in refractory hairy cell leukemia to a recombinant immunotoxin. Blood (1999) 94(10):3340–8. doi: 10.1182/blood.V94.10.3340.422k19_3340_3348
78. Kreitman RJ, Wilson WH, White JD, Stetler-Stevenson M, Jaffe ES, Giardina S, et al. Phase I trial of recombinant immunotoxin anti-Tac(Fv)-PE38 (LMB-2) in patients with hematologic malignancies. J Clin Oncol (2000) 18(8):1622–36. doi: 10.1200/JCO.2000.18.8.1622
79. Maharaj K, Uriepero A, Sahakian E, Pinilla-Ibarz J. Regulatory T cells (Tregs) in lymphoid malignancies and the impact of novel therapies. Front Immunol (2022) 13:943354. doi: 10.3389/fimmu.2022.943354
80. Attia P, Powell DJ Jr., Maker AV, Kreitman RJ, Pastan I, Rosenberg SA. Selective elimination of human regulatory T lymphocytes in vitro with the recombinant immunotoxin LMB-2. J Immunother (2006) 29(2):208–14. doi: 10.1097/01.cji.0000187959.45803.0c
81. Powell DJ Jr., Felipe-Silva A, Merino MJ, Ahmadzadeh M, Allen T, Levy C, et al. Administration of a CD25-directed immunotoxin, LMB-2, to patients with metastatic melanoma induces a selective partial reduction in regulatory T cells in vivo. J Immunol (2007) 179(7):4919–28. doi: 10.4049/jimmunol.179.7.4919
82. Decker T, Hipp S, Kreitman RJ, Pastan I, Peschel C, Licht T. Sensitization of b-cell chronic lymphocytic leukemia cells to recombinant immunotoxin by immunostimulatory phosphorothioate oligodeoxynucleotides. Blood (2002) 99(4):1320–6. doi: 10.1182/blood.V99.4.1320.h8001320_1320_1326
83. Alderson RF, Kreitman RJ, Chen T, Yeung P, Herbst R, Fox JA, et al. CAT-8015: a second-generation pseudomonas exotoxin a-based immunotherapy targeting CD22-expressing hematologic malignancies. Clin Cancer Res (2009) 15(3):832–9. doi: 10.1158/1078-0432.CCR-08-1456
84. Kreitman RJ, Tallman MS, Robak T, Coutre S, Wilson WH, Stetler-Stevenson M, et al. Minimal residual hairy cell leukemia eradication with moxetumomab pasudotox: phase 1 results and long-term follow-up. Blood (2018) 131(21):2331–4. doi: 10.1182/blood-2017-09-803072
85. Kreitman RJ, Dearden C, Zinzani PL, Delgado J, Robak T, le Coutre PD, et al. Moxetumomab pasudotox in heavily pre-treated patients with relapsed/refractory hairy cell leukemia (HCL): long-term follow-up from the pivotal trial. J Hematol Oncol (2021) 14(1):35. doi: 10.1186/s13045-020-01004-y
86. Kreitman RJ, Pastan I. Antibody fusion proteins: anti-CD22 recombinant immunotoxin moxetumomab pasudotox. Clin Cancer Res (2011) 17(20):6398–405. doi: 10.1158/1078-0432.CCR-11-0487
87. Muller F, Cunningham T, Stookey S, Tai CH, Burkett S, Jailwala P, et al. 5-azacytidine prevents relapse and produces long-term complete remissions in leukemia xenografts treated with moxetumomab pasudotox. Proc Natl Acad Sci U S A (2018) 115(8):E1867–E75. doi: 10.1073/pnas.1714512115
88. Mussai F, Campana D, Bhojwani D, Stetler-Stevenson M, Steinberg SM, Wayne AS, et al. Cytotoxicity of the anti-CD22 immunotoxin HA22 (CAT-8015) against paediatric acute lymphoblastic leukaemia. Br J Haematol (2010) 150(3):352–8. doi: 10.1111/j.1365-2141.2010.08251.x
89. Biberacher V, Decker T, Oelsner M, Wagner M, Bogner C, Schmidt B, et al. The cytotoxicity of anti-CD22 immunotoxin is enhanced by bryostatin 1 in b-cell lymphomas through CD22 upregulation and PKC-betaII depletion. Haematologica (2012) 97(5):771–9. doi: 10.3324/haematol.2011.049155
90. Kamiguti AS, Harris RJ, Slupsky JR, Baker PK, Cawley JC, Zuzel M. Regulation of hairy-cell survival through constitutive activation of mitogen-activated protein kinase pathways. Oncogene (2003) 22(15):2272–84. doi: 10.1038/sj.onc.1206398
91. Zheng S, Gillespie E, Naqvi AS, Hayer KE, Ang Z, Torres-Diz M, et al. Modulation of CD22 protein expression in childhood leukemia by pervasive splicing aberrations: Implications for CD22-directed immunotherapies. Blood Cancer Discovery (2022) 3(2):103–15. doi: 10.1158/2643-3230.BCD-21-0087
92. Wei H, Xiang L, Wayne AS, Chertov O, FitzGerald DJ, Bera TK, et al. Immunotoxin resistance via reversible methylation of the DPH4 promoter is a unique survival strategy. Proc Natl Acad Sci USA (2012) 109(18):6898–903. doi: 10.1073/pnas.1204523109
93. Rossi EA, Goldenberg DM, Michel R, Rossi DL, Wallace DJ, Chang CH. Trogocytosis of multiple b-cell surface markers by CD22 targeting with epratuzumab. Blood (2013) 122(17):3020–9. doi: 10.1182/blood-2012-12-473744
94. Brown JR. Ibrutinib in chronic lymphocytic leukemia and b cell malignancies. Leuk Lymphoma (2014) 55(2):263–9. doi: 10.3109/10428194.2013.803226
95. Sivina M, Kreitman RJ, Arons E, Ravandi F, Burger JA. The bruton tyrosine kinase inhibitor ibrutinib (PCI-32765) blocks hairy cell leukaemia survival, proliferation and b cell receptor signalling: a new therapeutic approach. Br J Haematol (2014) 166(2):177–88. doi: 10.1111/bjh.12867
96. Bohn JP, Wanner D, Steurer M. Ibrutinib for relapsed refractory hairy cell leukemia variant. Leuk Lymphoma (2017) 58(5):1224–6. doi: 10.1080/10428194.2016.1239262
97. Rogers KA, Andritsos LA, Wei L, McLaughlin EM, Ruppert AS, Anghelina M, et al. Phase 2 study of ibrutinib in classic and variant hairy cell leukemia. Blood (2021) 137(25):3473–83. doi: 10.1182/blood.2020009688
98. Van De Corput L, Falkenburg JH, Kluin-Nelemans JC. T-Cell dysfunction in hairy cell leukemia: An updated review. Leuk Lymphoma (1998) 30(1-2):31–9. doi: 10.3109/10428199809050927
99. Frankel T, Lanfranca MP, Zou W. The role of tumor microenvironment in cancer immunotherapy. Adv Exp Med Biol (2017) 1036:51–64. doi: 10.1007/978-3-319-67577-0_4
100. Boulch M, Cazaux M, Loe-Mie Y, Thibaut R, Corre B, Lemaitre F, et al. A cross-talk between CAR T cell subsets and the tumor microenvironment is essential for sustained cytotoxic activity. Sci Immunol (2021) 6(57):eabd4344. doi: 10.1126/sciimmunol.abd4344
101. Shehata M, Schwarzmeier JD, Hilgarth M, Hubmann R, Duechler M, Gisslinger H. TGF-beta1 induces bone marrow reticulin fibrosis in hairy cell leukemia. J Clin Invest (2004) 113(5):676–85. doi: 10.1172/JCI19540
102. Dahmani A, Delisle JS. TGF-beta in T cell biology: Implications for cancer immunotherapy. Cancers (Basel) (2018) 10(6):194. doi: 10.3390/cancers10060194
103. Noh KE, Lee JH, Choi SY, Jung NC, Nam JH, Oh JS, et al. TGF-beta/IL-7 chimeric switch receptor-expressing CAR-T cells inhibit recurrence of CD19-positive b cell lymphoma. Int J Mol Sci (2021) 22(16):8706. doi: 10.3390/ijms22168706
104. Riese MJ, Wang LC, Moon EK, Joshi RP, Ranganathan A, June CH, et al. Enhanced effector responses in activated CD8+ T cells deficient in diacylglycerol kinases. Cancer Res (2013) 73(12):3566–77. doi: 10.1158/0008-5472.CAN-12-3874
105. Giavridis T, van der Stegen SJC, Eyquem J, Hamieh M, Piersigilli A, Sadelain M. CAR T cell-induced cytokine release syndrome is mediated by macrophages and abated by IL-1 blockade. Nat Med (2018) 24(6):731–8. doi: 10.1038/s41591-018-0041-7
106. Cimino G, Annino L, Giona F, Sgadari C, Di Gregorio AO, Cava MC, et al. Serum interleukin-1 beta levels correlate with neoplastic bulk in hairy cell leukemia. Leukemia (1991) 5(7):602–5.
107. Souers AJ, Leverson JD, Boghaert ER, Ackler SL, Catron ND, Chen J, et al. ABT-199, a potent and selective BCL-2 inhibitor, achieves antitumor activity while sparing platelets. Nat Med (2013) 19(2):202–8. doi: 10.1038/nm.3048
108. Eradat H. Venetoclax for the treatment of chronic lymphocytic leukemia. Curr Hematol Malig Rep (2019) 14(5):469–76. doi: 10.1007/s11899-019-00539-3
109. Choi JH, Bogenberger JM, Tibes R. Targeting apoptosis in acute myeloid leukemia: Current status and future directions of BCL-2 inhibition with venetoclax and beyond. Target Oncol (2020) 15(2):147–62. doi: 10.1007/s11523-020-00711-3
110. Klener P, Sovilj D, Renesova N, Andera L. BH3 mimetics in hematologic malignancies. Int J Mol Sci (2021) 22(18):10157. doi: 10.3390/ijms221810157
111. Valkenburg KC, de Groot AE, Pienta KJ. Targeting the tumour stroma to improve cancer therapy. Nat Rev Clin Oncol (2018) 15(6):366–81. doi: 10.1038/s41571-018-0007-1
112. Timmins MA, Ringshausen I. Transforming growth factor-beta orchestrates tumour and bystander cells in b-cell non-Hodgkin lymphoma. Cancers (Basel) (2022) 14(7):1772. doi: 10.3390/cancers14071772
113. Park E, Chen J, Moore A, Mangolini M, Santoro A, Boyd JR, et al. Stromal cell protein kinase c-beta inhibition enhances chemosensitivity in b cell malignancies and overcomes drug resistance. Sci Transl Med (2020) 12(526):eaax9340. doi: 10.1126/scitranslmed.aax9340
114. Kashyap MK, Amaya-Chanaga CI, Kumar D, Simmons B, Huser N, Gu Y, et al. Targeting the CXCR4 pathway using a novel anti-CXCR4 IgG1 antibody (PF-06747143) in chronic lymphocytic leukemia. J Hematol Oncol (2017) 10(1):112. doi: 10.1186/s13045-017-0435-x
115. Zhao R, Liu J, Li Z, Zhang W, Wang F, Zhang B. Recent advances in CXCL12/CXCR4 antagonists and nano-based drug delivery systems for cancer therapy. Pharmaceutics (2022) 14(8):1541. doi: 10.3390/pharmaceutics14081541
Keywords: HCL, leukemia microenvironment, treatment resistance, microenvironment targeting, novel therapies
Citation: Gargiulo E, Giordano M, Niemann CU, Moussay E, Paggetti J and Morande PE (2023) The protective role of the microenvironment in hairy cell leukemia treatment: Facts and perspectives. Front. Oncol. 13:1122699. doi: 10.3389/fonc.2023.1122699
Received: 13 December 2022; Accepted: 22 February 2023;
Published: 08 March 2023.
Edited by:
Ingo Ringshausen, University of Cambridge, United KingdomReviewed by:
Ozren Jaksic, University Hospital Dubrava, CroatiaCopyright © 2023 Gargiulo, Giordano, Niemann, Moussay, Paggetti and Morande. This is an open-access article distributed under the terms of the Creative Commons Attribution License (CC BY). The use, distribution or reproduction in other forums is permitted, provided the original author(s) and the copyright owner(s) are credited and that the original publication in this journal is cited, in accordance with accepted academic practice. No use, distribution or reproduction is permitted which does not comply with these terms.
*Correspondence: Pablo Elías Morande, pabloelias.morande@lih.lu; pabloemorande@gmail.com; Etienne Moussay, etienne.moussay@lih.lu; Jérôme Paggetti, jerome.paggetti@lih.lu
†These authors share senior authorship