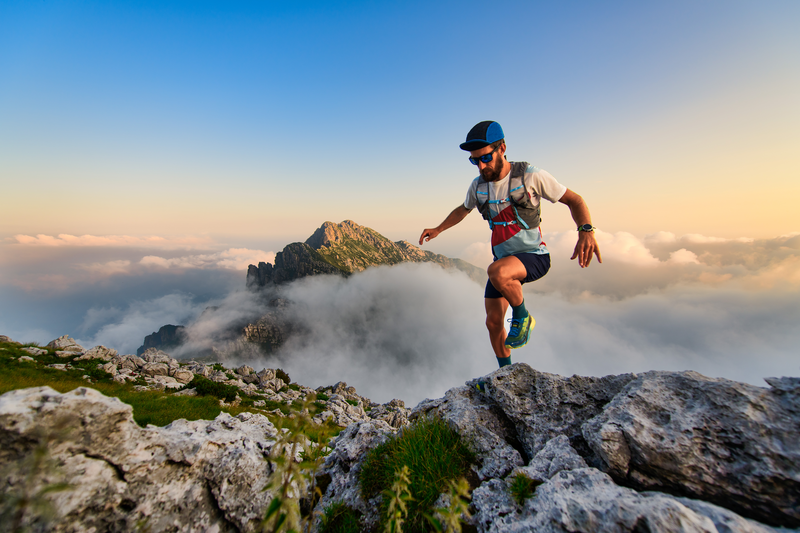
94% of researchers rate our articles as excellent or good
Learn more about the work of our research integrity team to safeguard the quality of each article we publish.
Find out more
REVIEW article
Front. Oncol. , 10 May 2023
Sec. Cancer Molecular Targets and Therapeutics
Volume 13 - 2023 | https://doi.org/10.3389/fonc.2023.1121130
Cancer is a disease with ecological and evolutionary unity, which seriously affects the survival and quality of human beings. Currently, many reports have suggested Gas6 plays an important role in cancer. Binding of gas6 to TAM receptors is associated with the carcinogenetic mechanisms of multiple malignancies, such as in breast cancer, chronic lymphocytic leukemia, non-small cell lung cancer, melanoma, prostate cancer, etc., and shortened overall survival. It is accepted that the Gas6/TAM pathway can promote the malignant transformation of various types of cancer cells. Gas6 has the highest affinity for Axl, an important member of the TAM receptor family. Knockdown of the TAM receptors Axl significantly affects cell cycle progression in tumor cells. Interestingly, Gas6 also has an essential function in the tumor microenvironment. The Gas6/AXL pathway regulates angiogenesis, immune-related molecular markers and the secretion of certain cytokines in the tumor microenvironment, and also modulates the functions of a variety of immune cells. In addition, evidence suggests that the Gas6/AXL pathway is involved in tumor therapy resistance. Recently, multiple studies have begun to explore in depth the importance of the Gas6/AXL pathway as a potential tumor therapeutic target as well as its broad promise in immunotherapy; therefore, a timely review of the characteristics of the Gas6/AXL pathway and its value in tumor treatment strategies is warranted. This comprehensive review assessed the roles of Gas6 and AXL receptors and their associated pathways in carcinogenesis and cancer progression, summarized the impact of Gas6/AXL on the tumor microenvironment, and highlighted the recent research progress on the relationship between Gas6/AXL and cancer drug resistance.
Cancer is a disease with ecological and evolutionary unity, which seriously affects the survival and quality of human beings (1). Cancer cells are described as invasive species and its metastasis as a multidirectional ecological dispersal. The foundational ecological principles include intraspecific relationship (e.g communication) and interspecific relationship (e.g competition, predation, parasitism and mutualism) are interpreted to understand cancer progression. In this review, we will mainly introduce the role of the Gas/AXL signaling in cancer cells, discuss its interaction with the tumor immune microenvironment, and its relationship with tumor progression.
Currently, many reports have suggested TAM receptors and ligands play an important role in cancer. TAM receptor family members include Tyro3, Axl, MerTK (collectively known as TAM) (2). The TAM ligand family includes human growth inhibitor specific 6 (GAS6), PROS1, LGALS3, Tulp-1 etc (3). Typical of these ligands include GAS6 and PROS1. GAS6 activates all members of the TAM receptor family, including AXL, while PROS1 activates only Tyro3 and MerTK (4). GAS6 gene expresses the Gas6 protein, a vitamin K-binding protein, originally reported to be upregulated in fibroblasts induced by growth inhibition under serum starvation conditions (5, 6), and can activate AXL in a concentration-dependent manner (7), showing the highest affinity for the Axl among all the TAM receptor family (8, 9). Gas6 binds to AXL, to regulate cell survival (10–13), promote tumor cell proliferation and migration, induce epithelial mesenchymal transition (EMT), inhibit tumor cell apoptosis, and participate in tumor stem cell maintenance (14–18) by activating multiple downstream pathways including JAK/STAT3 (19), PI3K/AKT/mTOR (20), Grb2/RAS/MEK/ERK1/2 (21) and FAK/Src/NF kappa B (22). In addition, Gas6/AXL shapes the suppressive tumor immune microenvironment by modulating angiogenesis in the tumor microenvironment (23), regulates immune-related molecular markers and controls the secretion of certain cytokines (24), regulates the functions of multiple immune cells (25), and interacts with tumor cells, host immune cells, and abnormal physiological factors (26). In adult normal cells, such as normal brain tissue, hippocampus, heart and liver, AXL expression is relatively low (27, 28); however, in certain malignant cells such as breast cancer, chronic lymphocytic leukemia, non-small cell lung cancer, melanoma, and prostate cancer cells, AXL is abnormally overexpressed (29–40), promotes tumor progression and reduces overall survival. Therefore, AXL may constitute an important prognostic biomarker and a potential therapeutic target. With the current review, we discussed the roles of Gas6 and AXL receptors in carcinogenesis and tumor progression; then we summarized the effects of the Gas6/AXL axis on the TME; finally, we focused on recent progress on the relationship between Gas6/AXL and cancer therapeutic resistance, to provide novel directions for future experimental design and tumor treatment strategies.
The TAM ligand family includes human growth inhibitor specific 6 (GAS6), PROS1, LGALS3, Tulp-1 etc (3). Typical of these ligands include GAS6 and PROS1. GAS6 activates all members of the TAM receptor family, including AXL, while PROS1 activates only Tyro3 and MerTK (4). GAS6 is a vitamin K-dependent protein abundantly expressed in fibroblasts 3T3 cells induced by growth inhibition under serum starvation conditions (6). Sequencing of the Gas6 protein by Manfioletti and colleagues revealed Gas6 is a secreted protein containing 678 amino acids with a molecular weight of 75 kDa (5, 6). It consists of an N-terminal Gla structural domain with a disulfide bond-maintained loop behind it, and four epidermal growth factor (EGF)-like structural domains next to the loop (41, 42) and a sex hormone-binding globulin (SHBG)-like structural domain at the C-terminal.
TAM is the receptor of Gas6. TAM binds to Gas ligands and exerts multiple effects in diverse cells (2). TAM receptors mainly regulate cell survival, mediate removal of apoptotic cells through phagocytes via non-inflammatory reactions, and affect natural killer cell differentiation and platelet aggregation etc. (29, 43–45). The TAM receptor family belongs to receptor tyrosine kinases (RTKs), consisting of the three receptors Axl, MerTK and Tyro3. Gas6 can activate AXL in a concentration-dependent manner. Furthermore, Gas6 shows the highest affinity for the Axl receptor in the TAM family, and it was reported that AXL as one of the receptors has 3-10 times higher affinity for Gas6 compared with the other two members (8).
AXL, firstly identified in 1991, is a 140 kDa protein. In adult normal cells, such as normal brain tissue, hippocampus, heart and liver, AXL expression is relatively low (27, 28), but AXL levels are abnormally high in many human cancers, including non-small cell lung cancer (NSCLC), esophageal cancer, glioblastoma, breast cancer and chronic lymphocytic leukemia (29–40), which is associated with reduced overall survival and enhanced disease progression. Some cancer models further revealed that AXL expression is related to tumor cell motility, metastasis, and invasion. Thus, AXL has great potential as a promising prognostic biomarker and therapeutic target.
TAM has a single hydrophobic transmembrane structural domain, comprising extracellular structural domains similar to intercellular adhesion molecules (ICAM) and vascular cell adhesion molecules (VCAM) (46), which contain the sequence of fibronectin and immunoglobulin (47), thus promoting cell aggregation through homophilic or heterophilic binding (48). In addition, TAM contains a tyrosine kinase structural domain, indicating this family of receptors have both the characteristics of an adhesion molecule and the activity of a tyrosine kinase. The Ig-like structural domain of the TAM receptor interacts with the laminin G-like domains of its ligand, thereby activating an intracellular signaling cascade that regulates many genes transcriptionally (46).
Upregulation of Gas6/AXL is associated with carcinogenesis in multiple malignancies and shortens overall survival, and may be involved in tumor cell proliferation, migration, apoptosis, and maintenance of tumor stem cells through multiple signaling pathways (Figure 1). Signaling pathways downstream Gas6/AXL signaling, including PI3K/AKT/mTOR, NF-κB, JAK/STAT3 and RAS/RAF/MEK/ERK, play critical roles in tumor cell cycle regulation, malignancy and drug resistance (14–17).
Figure 1 Molecular structure diagram of Gas6 and AXL and the multiple signaling pathways involved in the regulation of tumor cell proliferation, migration and apoptosis, as well as the maintenance of tumor stem cells by Gas6/AXL.
Post-binding activation of AXL and GAS6 is correlated with enhanced proliferation and survival in multiple tumors, including prostate, colorectal, gastric and renal cancers, and osteosarcoma (10–13). The main pathways controlling tumor cell proliferation induction include the STAT3 (19), PI3K/AKT (20), Grb2/RAS/MEK/ERK1/2 (21) and FAK/Src/NF kappa B pathways (22). Gas6 promotes the proliferation of AXL-expressing prostate cancer cells by enhancing Akt phosphorylation (49). Gas6 induces ERK signaling by interacting with AXL and promotes melanoma cell proliferation (50). Gas6/AXL signaling activates Src, local adhesion kinase (FAK) and NFκB to promote proliferation in nerve sheath tumor cells (22). In experimental studies of NSCLC and thyroid cancer, AXL silencing inhibited xenograft growth in nude mice (51, 52). In addition, it was shown cancer cells promote tumor growth by stimulating infiltrating leukocytes to express the mitogenic protein Gas6 (53).
AXL is considered a driver of tumor metastasis. AXL activity highly contributes to the acquisition of migratory potential in cells (54). Tumor metastasis is tightly correlated with EMT. The intercellular adhesion of noncancerous epithelial cells contributes to maintaining tissue integrity; whereas mesenchymal cells migrate and invade (55). AXL activation drives EMT, suppresses the expression of epithelial biomarkers (e.g. E-calmodulin) and promotes the expression of mesenchymal biomarkers (e.g. N-calmodulin, Snail, Vimentin, Slug, α-catenin protein and α-SMA) (18) (36, 56–58),. Li and collaborators observed that Gas6 interaction with AXL induced tumor cell migration mostly by upregulating Slug in prostate and skin cancer cells (59). Yang and colleagues demonstrated AXL affects cell adhesion by phosphorylating myoglutinin on tyrosine in active myoglutinin filaments. This may indicate that AXL is involved in tumor cell migration (60). Similar findings have been reported in liposarcoma, and pancreatic, lung, breast and thyroid cancers (61–63). This further demonstrates an important role for the AXL pathway in tumor cell migration and invasion.
Several reports have shown AXL’s association with suppressed apoptosis. The Gas6/AXL pathway represses apoptosis through PI3K/Akt pathway activation as well as via BAD (BCL2-associated cell death agonist) phosphorylation and ERK1/2 (64) activation. For example, Li et al, have shown that Axl is expressed in the cardiomyocytes in patients with sepsis, exogenous recombinant Gas6 can inhibit the activation of Axl/PI3K/Akt/NF-κB signaling pathway caused by bacterial infection, thereby inhibiting tumor necrosis factor (TNF)-a release and apoptosis, ameliorating sepsis-induced myocardial dysfunction (65). And it was found in tumor cells that the Axl-Gas6 receptor-ligand interaction can inhibit cell apoptosis and promote tumor progression by activating the AKT pathway and activating the NF-κB pathway (66, 67). In the TME, Gas6 can also inhibit apoptotic events in cultured VSMCs by phosphorylating AXL. Gas6 and AXL amounts increase upon vascular injury, playing a major role in neointima formation by inhibiting apoptosis (68). Under serum starvation conditions, acute myeloid leukemia (AML) Nomo-1 and Kasumi-1 cells with Gas6 and AXL silenced with two distinct shRNAs showed a two- to three-fold increase in apoptosis (69).
Cancer stem cells can self-renew, differentiate, and become tumorigenic, which has a dramatic impact on tumor resistance, recurrence and metastasis (70). AXL correlates with many stem cell markers, including Isl1, Cdc2a, Bglap1, CD44 and ALDH1 (18). Gas6/AXL signaling stabilizes β-catenin through a p-AKT-dependent pathway thereby regulating the self-renewal capacity of leukemic stem cells (71). Gas6 can also enhance PI3K/AKT signaling through an AXL-dependent autocrine manner, thereby promoting factor-1 alpha (HIF-1α)-driven secretion of multiple growth factor-mediated maintenance of mesenchymal stem cells function (72).
The abovementioned findings described the cell-autonomous role of Gas6/AXL in malignant cells. The present section mainly summarizes the cell-dependent role of Gas6/AXL in the TME in malignant cells, e.g., the roles of immune and vascular smooth muscle cells (VSMC) on tumor development (Figure 2). The TME consists of tumor cells, tumor-supporting cells such as fibroblasts and vascular endothelial cells, secreted factors, and even impaired physiological conditions (73). In general, AXL is expressed on tumor cells, but recent reports detected AXL on bone marrow-derived cells (BMDC), dendritic cells (DC), giant phagocytosis cells, mononuclear cells, natural killers (NKs) and platelets (74). Myeloid cells may express AXL to apoptotic phagocytotic cells and debris. Additionally, tumor cells upregulate AXL and Gas6 expression in presence of monocyte myeloid-derived suppressor cells (M-MDSCs) and polymorphonuclear myeloid-derived suppressor cells (PMN-MDSCs) (26). Furthermore, hypoxia increases the expression of hypoxia-inducible factors-1 and -2 to upregulate genes conferring an aggressive tumor phenotype (75). Mounting evidence suggests hypoxic upregulation is tightly correlated with AXL expression and stability (76). Besides hypoxia, multiple cytokines upregulate AXL (77). Therefore, interactions among the tumor, host immune cells, and abnormal physiological factors in the TME may upregulate AXL and Gas6, thus promoting a pro-tumor microenvironment. Therefore, AXL might be a key mediator in the tumor malignant microenvironment.
Figure 2 The Gas6/AXL pathway promotes the formation of an immunosuppressive microenvironment through multiple pathways: 1) regulating immune-related biomolecules, i.e., inhibiting the expression of MHC-I molecules and enhancing PD- L1 expression on tumor cells, promoting the secretion of immunosuppressive cytokines (e.g., IL-4, CCL3-5 and G-CSF) and inhibiting the secretion of chemokines that recruit Th1, CD8+ T cells and NK cells (CXCL9, CXCL10 and CXCL11); 2)enhancing the development and activation of NK cells; promoting the expression of Tie2 and VEGFR-2 on vascular endothelial cells, thereby inducing angiogenesis and reducing vascular permeability; enhancing the differentiation of DC cells to the immunosuppressive phenotype; 3) regulating immune cell functions, i.e., promoting M2-type polarization of macrophages, increasing the immunosuppressive activity of Treg cells and promoting T-cell repulsion.
AXL is widely synthesized by endothelial cells and could promote angiogenesis by regulating the production of VEGF and PDGF, thus participating in the mediation of normal and tumor vascular systems. For instance, suppression of AXL reduces the expression of Tie2 and VEGFR-2 (78), leading to impaired vascular endotheliogenesis and affecting vascular permeability. Additionally, AXL suppression in xenograft-bearing mice decreases the endothelial cell marker CD31 in tumors (79) and reduces cancer cell-triggered angiogenesis. Downregulation of AXL or Gas6 can impair the formation and function of the vascular endothelium (80).
Substantial evidence shows GAS6/AXL signaling is important in the promotion of an immunosuppressive TME. The GAS6/AXL axis regulates many tumor immune-related biomolecules, e.g., major histocompatibility complex I (MHC-I) and programmed death ligand-1 (PD-L1) in tumor cells (24), and the levels of secreted anti-inflammatory cytokines such as IL-4, CCL3-5 and granulocyte colony-stimulating factor (G-CSF) (81). In addition, GAS6/AXL signaling also regulates the development and infiltration of several immune cells such as macrophages, DCs, NKs and regulatory T cells (Tregs), thus making it possible for tumors to evade immune surveillance.
MHC-I molecules are found on all nucleated cells. Professional antigen presenting cells (APCs) lyse tumor cells by presenting MHC-I antigen epitopes to CD8+ T cells, inducing them to recognize tumor cells and secreting perforin and granzyme. Evidence indicates AXL is negatively associated with MHC-I molecules. Rothlin et al. found that TAM deficient mice have elevated in vivo amounts of MHC-I in myeloid cells, confirming for the first time an association between MHC-I and Axl (82). Jeon and co-workers demonstrated the AXL suppressor Q702 decreased the expression of TAM signature genes and upregulated MHC-I signature genes in tumor samples, and also reported upregulated CD8 T cell and NK cell signature genes in a time-dependent manner (83). Aguilera and collaborators suggested that in treatment unresponsive tumors, AXL was high expressed with antigen presentation suppressed through MHC-I, mediating immunological microenvironment reprogram and knockout of Axl in tumor cell lines increased surface MHC-I amounts in NF-κB independent pathway (84). Of more concern is the elevated AXL expression and the reduced expression of MHC class I molecules in the melanoma immunotherapy-resistant phenotype (85). In summary, there seems to be an extremely subtle relationship between AXL and MHC-I, which may be one of the mechanisms involved in immune evasion. But the exact regulation mechanism of how does AXL impact on MHC-I expression are still unclear, which deserves further exploration in subsequent studies.
PD-L1 is produced by a variety of tumor cells, and its interaction with its receptor induces pathways for blocking T cell activation (86) to evade the host immune response (87). In cancer immunotherapy, TAM receptors play a key role in regulating immune checkpoint signaling associated with the PD-1 axis (88). In 2014, researchers demonstrated that activation of PtdSer-TAM-PD-L1-PI3k/Akt signaling in breast cancer promotes immune escape and chemotherapy resistance in tumors. A recent analysis showed increased expression of PD-L1 in HPV-negative head-and-neck squamous cell carcinoma (HNSCC) cells through AXL and PI3K signaling, which correlated with resistance to radiotherapy, causing local treatment failure and enhancing mortality in HNSCC (89). In lung adenocarcinoma PC9 and H1975 cells, pharmacological Axl inhibition with the selective Axl inhibitors bemcentinib and BGB324 markedly reduced PD-L1 and PD-L2 expression. In addition, in a preclinical model of breast cancer, combination of AXL suppression and anti-PD-1 resulted in both primary and metastatic tumor shrinkage, increased CD8T cell infiltration, and an increased rate of NK cell activation (90), which could not be achieved after treatment with either drug alone. Thus, AXL receptor kinase may affect the tumor immune microenvironment by regulating PD-L1 expression (91).
Gas6/AXL signaling promotes immunosuppression and generates a pro-tumor microenvironment by altering and regulating the secretion of cytokines associated with immune cell function and movement (92). G-CSF promotes granulocyte-myeloid-derived suppressor cell (G-MDSC) accumulation in the tumor immune microenvironment (93). Axl knockout results in reduced secretion of G-CSF (84). The selective Axl inhibitor bemcentinib reduces G-CSF secretion in pancreatic cancer animal models (94). Further data also showed pharmacological inhibition of Axl downregulates the pro-tumorigenic inflammatory factor IL-4 in tumors (95). Axl inhibition attenuates the secretion of chemokines (CCL 2-4 and CCL 5) involved in the recruitment of immunosuppressive cells, including M2 macrophages and MDSCs, but promotes the secretion of chemokines (CXCL9-11) enhancing the recruitment of immune-effector cells such as CD8+ T cells and NKs (96).
As Gas6/AXL signaling triggers an immunosuppressive tumor microenvironment, the functions of diverse immune cells and the overall makeup of the tumor immune microenvironment are modified in this process.
Tumor cells develop specific mechanisms, including efferocytosis, for removing apoptotic cells to regulate the immune response. AXL expression on specialized phagocytes (macrophages and dendritic cells) in various tumor settings is important for homeostasis under physiological conditions. The main mechanisms involve macrophage polarization and efferocytosis of apoptotic cells (43, 97).
TAM receptor-mediated efferocytosis has tumor-promoting functions such as immunosuppression, tumor metastasis, and resistance to therapeutics (25). Gas6/AXL removes apoptotic residues by activating efferocytosis in macrophages and DCs, an effect impaired in AXL-deficient mice (98). Activation of Axl and Mertk receptor kinases is essential for PtdSer-dependent efferocytosis of apoptotic cells. It was shown Axl- and Mertk-induced efferocytosis of apoptotic cells inhibits innate immune responses orchestrated by macrophages and DCs (99), thereby generating a TME that favors the formation of tumor development and metastasis (100). Chiu and colleagues found that in oral squamous cell carcinoma, AXL signaling controls the polarization of tumor-associated macrophages toward the M2 phenotype with elevated expression of M2 markers and genes (101). After TAM receptor-mediated efferocytosis and phagocytosis, tumor-associated macrophages tend to polarize toward the immunosuppressive phenotype (M2 macrophages) in response to various cytokines and suppress antitumor immunity (102). The underpinning mechanism might involve Axl/PI3K/Akt/NF-κB signaling, in which the TAM receptor binds directly to PI3K, leading to PI3K phosphorylation of Akt. This results in macrophage polarization toward the M2 phenotype while reducing the amounts of M1 macrophages (103).
Additionally, efferocytosis in turn upregulates TAM receptor in tumor APCs, making them polarize to an immunosuppressive phenotype (102). DCs have moderate AXL expression prior to encountering pathogens. However, after pathogen encounter, AXL is significantly overexpressed via strong induction of the JAK/STAT1 pathway (82), thus shifting the pro-inflammatory state to an immunosuppressive state (104). The shift in the APC phenotype leads to diminished T-cell antigen presentation, reduced T-cell activation, and impaired antigen-dependent antitumor immunity, generating a more aggressive and tolerant TME (8).
The Gas6/AXL pathway plays a key role in the regulation of NK cell activity (105). It regulates the normal developmental process of NK cells and the function of killing infected cells (106) by controlling the expression of receptors necessary for NK cell activation (105). Several studies have shown that NK cell dysfunction is associated with tumor progression in multiple aspects, including immune evasion and tumor metastasis. Under hypoxic conditions, inhibition of NK cell function promotes the formation of pre-metastatic niches (107). Recombinant Gas6 and anti-AXL antibodies upregulate NK cell-specific receptors and NK cell-related genes (108), thereby promoting NK cell receptor activation. The cytotoxic function of NK cells was impaired in AXL-inactivated mice. Significantly less NK cells were produced by human CD34+ hematopoietic stem cells after blocking Gas6’s interaction with AXL by AXL-Fc or warfarin (106). In addition, interleukin 15 (IL-15), in case of AXL depletion, fails to induce multiple pathways necessary for NK cell development (2, 109). Thus, AXL is highly correlated with NK cell activation and function.
The physical contact of effector T cells with tumor cells is the basis for the efficacy of immunotherapy. Certain stromal cells in the TME present a state of an immune desert within the tumor by excluding T cells close to malignant cells (110). The main mechanisms include insufficient activation of DCs leading to blunted antigen presentation and the lack of tumor antigens in the TME to initiate the T-cell response (110).
The receptor tyrosine kinase (RTK) AXL may be a potential mediator of T-cell rejection, increasing tumor cell invasion and metastasis and suppressing the immune response by enhancing T-cell rejection (111). The mechanism appears to involve a role for AXL in the inhibition of antigen presentation and production of myeloid-supporting inflammatory molecules, which leads to an inadequate adaptive immunity and T-cell rejection (84). AXL inhibitors have immune activating and antitumor effects. In a previous study, CD4+ and CD8+ T cell amounts were significantly increased in tumor-bearing mice administered the AXL inhibitor R428 (96), corroborating Holtzhausen et al. (26). In transgenic mouse models, AXL gene deletion increases T-cell infiltration in the tumor microenvironment by up to 20 times, while making tumor cells 50 times more sensitive to radiotherapy and immune checkpoint therapy (112). A recent mouse study demonstrated that AXL inhibitors impact the immune status and tumor growth in lung cancer. Application of AXL inhibitors to treat mice resulted in delayed tumor growth, elevated rate of effector memory helper T cells, enhanced infiltration of central memory cytotoxic T cells, increased amounts of CD86+ macrophages, and elevated proportion of CD80 high-expression macrophages in the tumor model (113).
Regulatory T cells (Tregs) regulate immune evasion, considered the primary mechanism of evasion from immune surveillance (114). Tregs inhibit multiple physiological and pathological immune reactions, which are essential for maintaining self-tolerance and immune homeostasis (115). Gas6 enhances the inhibitory effect of Tregs mainly through the AXL receptor (23). The proliferative activity of T cells is obtained mainly via IL-2, a powerful growth factor. After GAS6 addition to a co-culture system comprising CD4+CD25-T cells and CD4+CD25+ Tregs, T-cell proliferation was reduced as well as IL-2 expression. After Axl knockout, Foxp3 expression in Tregs was decreased and IL-2 expression was increased. Therefore, Gas6 can inhibit CD4+ T cells by depleting IL-2 or inhibiting IL-2 production (116). These findings corroborated findings by Zhao and colleagues in mice (116).
Tumor cell resistance is an important issue in cancer therapy, often leading to failed treatment or recurrent disease. Besides its roles in survival, proliferation and migration, AXL expression is a possible mechanism underlying resistance to immunotherapy, chemotherapy and molecularly targeted therapies. AXL may lead to innate or acquired resistance to chemotherapy, immunotherapy, molecularly targeted therapies and even radiotherapy (117, 118).
Hong et al. found that chemotherapeutic agents such as etoposide (VP-16) and cisplatin induce AXL upregulation in resistant acute leukemia, as a potential mechanism of chemoresistance (38). Wang and collaborators demonstrated AXL’s involvement in breast cancer resistance to adriamycin. AXL inhibitors combined with adriamycin markedly decrease the tumor load as well as invasion and metastasis in adriamycin-resistant breast cancer (119). AXL was also reported in pancreatic ductal adenocarcinoma to promote resistance to chemotherapy (120). AXL mRNA amounts were significantly elevated in cisplatin-resistant ovarian cancer cells compared with cisplatin-sensitive cells (121).
Widespread overexpression of AXL is also found in tumors following resistance to various targeted therapies (122, 123), resulting in cell tolerance or under-response to molecular targeted therapies such as EGFR, VEGFR, ALK, ERK, and PI3Kα inhibitors (79, 122). Inhibition of AXL, either by silencing or pharmacological intervention, effectively circumvents the resistance of targeted drug-resistant cell lines to certain targeted drugs. AXL overexpression and Kit downregulation were detected in imatinib-resistant gastrointestinal mesenchymal tumors, hence the term ‘‘tyrosine kinase switch’’ was coined for AXL (118). The same findings were reported in NSCLC models with resistance to erlotinib. Taniguchi et al. further showed that EGFR mutant NSCLC administered ostatinib had increased AXL expression, the extent of which was inversely correlated with the effect of ostatinib. The combination of AXL inhibitors increased sensitivity to ostatinib treatment compared with ostatinib monotherapy, both in primary and resistant cases, thereby reducing tumor size and slowing tumor growth (124). In addition, current evidence suggests that AXL overexpression modulates acquired resistance to cetuximab in NSCLC and HNSCC models (122).
As described in Section 3 of this paper, the Gas6/AXL pathway regulates the immune microenvironment by modulating important components of the immune microenvironment, including the tumor’s vascular system; critical biomarkers such as MHC-I molecules and PD-L1; important cytokines such as IL-4, CCL3-5 and G-CSF; and key immune cells such as phagocytes, DCs, NK cells, effector T cells and Tregs. In addition, it induces the formation of an immunosuppressive microenvironment, suppresses the host’s antitumor immunity, and mediates tumor immune escape. Therefore, the relationship between Gas6/AXL signaling and resistance to immunotherapy deserves further attention. By comparing the transcriptomes of PD-1-responding and non-responding tumors, Hugo et al. found that AXL is upregulated in non-responding tumors (125) and may be one of the key genes mediating resistance to immunotherapy. The relationship between AXL and resistance to immunotherapy was further investigated by Aguilera and colleagues, who found that in Py8119 cells, a mouse breast cancer cell line expressing Axl, radiotherapy combined with immune checkpoint inhibitors induced a limited initial immune response, exhibiting an immunotherapy-resistant state. Py8119 cells with Axl knockdown (by the CRISPR technology) transplanted into naive C57Bl/6 mice showed sensitivity to immunotherapy, delayed tumor growth, increased expression of MHC-I molecules and enhanced infiltration of mature DCs, CD4+ T cells, and CD8+ T cells in the tumor tissue (84). Mechanistically, AXL-mediated immune resistance involves a complex molecular network of multiple pathways and targets. Targeting AXL to sensitize to immunotherapy is associated with multiple biological events, including MAPK inhibition, NF-κB activation, and ICAM1 and ULBP1 upregulation (126). Further studies should focus on validating these findings and exploring how AXL drives immune resistance.
The above evidence provides a theoretical basis for the development of AXL-related drugs in combination with conventional therapeutic modalities based on synthetic lethality in the context of tumor resistance to therapy. Given the role of AXL in cancer growth and metastasis as well as its relatively low expression in normal tissues compared with tumor tissues, AXL represents a highly potential therapeutic target in cancer therapy. Currently, a series of therapeutic drugs targeting AXL have been developed, including small molecule inhibitors (117, 127), monoclonal antibodies (mAbs), antibody-drug conjugates (ADCs) (128), soluble receptors (129), and chimeric antigen receptors (CARs) T Cells (130). Some of drugs show obvious anti-tumor activity (Tables 1, 2). In addition, the potential of AXL inhibitors in combination with other anti-tumor therapies (especially checkpoint inhibition) has also received increasing attention. For example, multiple AXL drugs including bemcentinib, ONO-7475 (131), sitravantinib (127), mecbotamab vedotin (128), and batiraxcept (129) in combination with ICI for patients refractory to first-line immune drugs are currently in phase I-III trails (Tables 1, 2). However, there are still many problems to be solved in the current research on AXL-related drugs, which need to be further clarified according to the research results.
Roles for Gas6/AXL signaling in cancer development and progression, and shaping of the tumor microenvironment are gradually being revealed. Recently, substantial resources have been deployed to develop broad therapies targeting Gas6/AXL in cancer (132). Currently, cancer drug development strategies targeting AXL and other family members of its receptor include small-molecule inhibitors, monoclonal antibodies, and soluble receptors. Most of these studies focused on the exploration of small molecule inhibitors, while some reports focused on the regulation of its upstream effector Gas6. Among these drugs, BGB324 currently shows promising results in early studies of acute myeloid leukemia, based on its high specificity (133, 134). In addition, as mentioned above, a growing number of studies have started to examine the impact of AXL on conventional therapy, e.g., AXL inhibitors in combination with chemotherapy, targeted therapies, and especially immunotherapy, and these advances have increased our understanding of tumor biology, tumor progression, and the tumor immune microenvironment, showing promising prospect. However, the current analysis is still in the nascent stage and many questions remain unaddressed. For example, the mechanisms involved in the regulation of AXL in immunotherapy have not been fully elucidated. Future research should focus on preclinical determination of the optimal combination of cytotoxic and immunomodulatory therapies, initiation of innovative trials to assess the most promising combinations, and evaluation of the efficacy and toxicity of these therapies. The combination of anti-AXL therapies with chemotherapy and/or immunotherapy may represent an excellent opportunity.
Guarantor of integrity of the entire study: XP, QZ and LL. Study conception and design: XP, QZ and LL. Literature search: XZ, DP, RW, JZ, YL, YW, NZ. Clinical studies: XZ, DP, RW, JZ, YL, YW, NZ. Experimental studies/data analysis: XZ, DP, RW, JZ, YL, YW, NZ. Statistical analysis: XZ, DP, RW, JZ, YL, YW, NZ. Manuscript preparation: All authors. Manuscript editing: All authors. All authors contributed to the article and approved the submitted version.
This work was supported by the Regional Innovation Cooperation Project of Sichuan Science and Technology Program (2021YFQ0029) and the 1 · 3 · 5 project for disciplines of excellence –Clinical Research Fund, West China Hospital, Sichuan University, and Program of Education Department of Hubei (ZZD20006) and Program of Hubei Minzu University (4198003).
The authors declare that the research was conducted in the absence of any commercial or financial relationships that could be construed as a potential conflict of interest.
All claims expressed in this article are solely those of the authors and do not necessarily represent those of their affiliated organizations, or those of the publisher, the editors and the reviewers. Any product that may be evaluated in this article, or claim that may be made by its manufacturer, is not guaranteed or endorsed by the publisher.
1. Luo W. Nasopharyngeal carcinoma ecology theory: cancer as multidimensional spatiotemporal “Unity of ecology and evolution” pathological ecosystem. Theranostics (2023) 13(5):1607–31. doi: 10.7150/thno.82690
2. Hafizi S, Dahlback B. Signalling and functional diversity within the axl subfamily of receptor tyrosine kinases. Cytokine Growth Factor Rev (2006) 17(4):295–304. doi: 10.1016/j.cytogfr.2006.04.004
3. Herrera-Rivero M, Santarelli F, Brosseron F, Kummer MP, Heneka MT. Dysregulation of Tlr5 and Tam ligands in the alzheimer's brain as contributors to disease progression. Mol Neurobiol (2019) 56(9):6539–50. doi: 10.1007/s12035-019-1540-3
4. Burstyn-Cohen T, Hochberg A. Tam Signaling in the nervous system. Brain Plast (2021) 7(1):33–46. doi: 10.3233/BPL-210125
5. Schneider C, King RM, Philipson L. Genes specifically expressed at growth arrest of mammalian cells. Cell (1988) 54(6):787–93. doi: 10.1016/s0092-8674(88)91065-3
6. Manfioletti G, Brancolini C, Avanzi G, Schneider C. The protein encoded by a growth arrest-specific gene (Gas6) is a new member of the vitamin K-dependent proteins related A negative coregulator in the blood coagulation cascade. Mol Cell Biol (1993) 13(8):4976–85. doi: 10.1128/mcb.13.8.4976-4985.1993
7. Shafit-Zagardo B, Gruber RC, DuBois JC. The role of Tam family receptors and ligands in the nervous system: from development to pathobiology. Pharmacol Ther (2018) 188:97–117. doi: 10.1016/j.pharmthera.2018.03.002
8. Graham DK, DeRyckere D, Davies KD, Earp HS. The Tam family: phosphatidylserine sensing receptor tyrosine kinases gone awry in cancer. Nat Rev Cancer (2014) 14(12):769–85. doi: 10.1038/nrc3847
9. Wu X, Liu X, Koul S, Lee CY, Zhang Z, Halmos B. Axl kinase as a novel target for cancer therapy. Oncotarget (2014) 5(20):9546–63. doi: 10.18632/oncotarget.2542
10. Paccez JD, Vasques GJ, Correa RG, Vasconcellos JF, Duncan K, Gu X, et al. The receptor tyrosine kinase axl is an essential regulator of prostate cancer proliferation and tumor growth and represents a new therapeutic target. Oncogene (2013) 32(6):689–98. doi: 10.1038/onc.2012.89
11. Shiozawa Y, Pedersen EA, Patel LR, Ziegler AM, Havens AM, Jung Y, et al. Gas6/Axl axis regulates prostate cancer invasion, proliferation, and survival in the bone marrow niche. Neoplasia (2010) 12(2):116–27. doi: 10.1593/neo.91384
12. Sawabu T, Seno H, Kawashima T, Fukuda A, Uenoyama Y, Kawada M, et al. Growth arrest-specific gene 6 and axl signaling enhances gastric cancer cell survival Via akt pathway. Mol Carcinog (2007) 46(2):155–64. doi: 10.1002/mc.20211
13. Woo SM, Min KJ, Seo SU, Kim S, Kubatka P, Park JW, et al. Axl inhibitor R428 enhances trail-mediated apoptosis through downregulation of c-flip and survivin expression in renal carcinoma. Int J Mol Sci (2019) 20(13):3253. doi: 10.3390/ijms20133253
14. Dent P. Crosstalk between erk, akt, and cell survival. Cancer Biol Ther (2014) 15(3):245–6. doi: 10.4161/cbt.27541
15. Huang H, Liu H, Yan R, Hu M. Pi3k/Akt and Erk/Mapk signaling promote different aspects of neuron survival and axonal regrowth following rat facial nerve axotomy. Neurochem Res (2017) 42(12):3515–24. doi: 10.1007/s11064-017-2399-1
16. Sen T, Tong P, Diao L, Li L, Fan Y, Hoff J, et al. Targeting axl and mtor pathway overcomes primary and acquired resistance to Wee1 inhibition in small-cell lung cancer. Clin Cancer Res (2017) 23(20):6239–53. doi: 10.1158/1078-0432.CCR-17-1284
17. Kariolis MS, Miao YR, Diep A, Nash SE, Olcina MM, Jiang D, et al. Inhibition of the Gas6/Axl pathway augments the efficacy of chemotherapies. J Clin Invest (2017) 127(1):183–98. doi: 10.1172/JCI85610
18. Asiedu MK, Beauchamp-Perez FD, Ingle JN, Behrens MD, Radisky DC, Knutson KL. Axl induces epithelial-to-Mesenchymal transition and regulates the function of breast cancer stem cells. Oncogene (2014) 33(10):1316–24. doi: 10.1038/onc.2013.57
19. Yanagita M, Arai H, Nakano T, Ohashi K, Mizuno K, Fukatsu A, et al. Gas6 induces mesangial cell proliferation Via latent transcription factor Stat3. J Biol Chem (2001) 276(45):42364–9. doi: 10.1074/jbc.M107488200
20. Mao S, Wu Y, Wang R, Guo Y, Bi D, Ma W, et al. Overexpression of Gas6 promotes cell proliferation and invasion in bladder cancer by activation of the Pi3k/Akt pathway. Onco Targets Ther (2020) 13:4813–24. doi: 10.2147/OTT.S237174
21. Wu G, Ma Z, Hu W, Wang D, Gong B, Fan C, et al. Molecular insights of Gas6/Tam in cancer development and therapy. Cell Death Dis (2017) 8(3):e2700. doi: 10.1038/cddis.2017.113
22. Ammoun S, Provenzano L, Zhou L, Barczyk M, Evans K, Hilton DA, et al. Axl/Gas6/Nfkappab signalling in schwannoma pathological proliferation, adhesion and survival. Oncogene (2014) 33(3):336–46. doi: 10.1038/onc.2012.587
23. Rothlin CV, Lemke G. Tam Receptor signaling and autoimmune disease. Curr Opin Immunol (2010) 22(6):740–6. doi: 10.1016/j.coi.2010.10.001
24. Tanaka M, Siemann DW. Gas6/Axl signaling pathway in the tumor immune microenvironment. Cancers (Basel) (2020) 12(7):1850. doi: 10.3390/cancers12071850
25. Roy S, Bag AK, Dutta S, Polavaram NS, Islam R, Schellenburg S, et al. Macrophage-derived neuropilin-2 exhibits novel tumor-promoting functions. Cancer Res (2018) 78(19):5600–17. doi: 10.1158/0008-5472.CAN-18-0562
26. Holtzhausen A, Harris W, Ubil E, Hunter DM, Zhao J, Zhang Y, et al. Tam Family receptor kinase inhibition reverses mdsc-mediated suppression and augments anti-Pd-1 therapy in melanoma. Cancer Immunol Res (2019) 7(10):1672–86. doi: 10.1158/2326-6066.CIR-19-0008
27. Vajkoczy P, Knyazev P, Kunkel A, Capelle HH, Behrndt S, von Tengg-Kobligk H, et al. Dominant-negative inhibition of the axl receptor tyrosine kinase suppresses brain tumor cell growth and invasion and prolongs survival. Proc Natl Acad Sci U.S.A. (2006) 103(15):5799–804. doi: 10.1073/pnas.0510923103
28. Funakoshi H, Yonemasu T, Nakano T, Matumoto K, Nakamura T. Identification of Gas6, a putative ligand for sky and axl receptor tyrosine kinases, as a novel neurotrophic factor for hippocampal neurons. J Neurosci Res (2002) 68(2):150–60. doi: 10.1002/jnr.10211
29. Fujimori T, Grabiec AM, Kaur M, Bell TJ, Fujino N, Cook PC, et al. The axl receptor tyrosine kinase is a discriminator of macrophage function in the inflamed lung. Mucosal Immunol (2015) 8(5):1021–30. doi: 10.1038/mi.2014.129
30. Gay CM, Balaji K, Byers LA. Giving axl the axe: targeting axl in human malignancy. Br J Cancer (2017) 116(4):415–23. doi: 10.1038/bjc.2016.428
31. Balaji K, Vijayaraghavan S, Diao L, Tong P, Fan Y, Carey JP, et al. Axl inhibition suppresses the DNA damage response and sensitizes cells to parp inhibition in multiple cancers. Mol Cancer Res (2017) 15(1):45–58. doi: 10.1158/1541-7786.MCR-16-0157
32. Maacha S, Hong J, von Lersner A, Zijlstra A, Belkhiri A. Axl mediates esophageal adenocarcinoma cell invasion through regulation of extracellular acidification and lysosome trafficking. Neoplasia (2018) 20(10):1008–22. doi: 10.1016/j.neo.2018.08.005
33. Corno C, Gatti L, Lanzi C, Zaffaroni N, Colombo D, Perego P. Role of the receptor tyrosine kinase axl and its targeting in cancer cells. Curr Med Chem (2016) 23(15):1496–512. doi: 10.2174/0929867323666160405112954
34. Debruyne DN, Bhatnagar N, Sharma B, Luther W, Moore NF, Cheung NK, et al. Alk inhibitor resistance in Alk(F1174l)-driven neuroblastoma is associated with axl activation and induction of emt. Oncogene (2016) 35(28):3681–91. doi: 10.1038/onc.2015.434
35. Elkabets M, Pazarentzos E, Juric D, Sheng Q, Pelossof RA, Brook S, et al. Axl mediates resistance to Pi3kalpha inhibition by activating the Egfr/Pkc/Mtor axis in head and neck and esophageal squamous cell carcinomas. Cancer Cell (2015) 27(4):533–46. doi: 10.1016/j.ccell.2015.03.010
36. Gjerdrum C, Tiron C, Hoiby T, Stefansson I, Haugen H, Sandal T, et al. Axl is an essential epithelial-to-Mesenchymal transition-induced regulator of breast cancer metastasis and patient survival. Proc Natl Acad Sci U.S.A. (2010) 107(3):1124–9. doi: 10.1073/pnas.0909333107
37. Holland SJ, Pan A, Franci C, Hu Y, Chang B, Li W, et al. R428, a selective small molecule inhibitor of axl kinase, blocks tumor spread and prolongs survival in models of metastatic breast cancer. Cancer Res (2010) 70(4):1544–54. doi: 10.1158/0008-5472.CAN-09-2997
38. Hong CC, Lay JD, Huang JS, Cheng AL, Tang JL, Lin MT, et al. Receptor tyrosine kinase axl is induced by chemotherapy drugs and overexpression of axl confers drug resistance in acute myeloid leukemia. Cancer Lett (2008) 268(2):314–24. doi: 10.1016/j.canlet.2008.04.017
39. Hsieh MS, Yang PW, Wong LF, Lee JM. The axl receptor tyrosine kinase is associated with adverse prognosis and distant metastasis in esophageal squamous cell carcinoma. Oncotarget (2016) 7(24):36956–70. doi: 10.18632/oncotarget.9231
40. Kanlikilicer P, Ozpolat B, Aslan B, Bayraktar R, Gurbuz N, Rodriguez-Aguayo C, et al. Therapeutic targeting of axl receptor tyrosine kinase inhibits tumor growth and intraperitoneal metastasis in ovarian cancer models. Mol Ther Nucleic Acids (2017) 9:251–62. doi: 10.1016/j.omtn.2017.06.023
41. Huang M, Rigby AC, Morelli X, Grant MA, Huang G, Furie B, et al. Structural basis of membrane binding by gla domains of vitamin K-dependent proteins. Nat Struct Biol (2003) 10(9):751–6. doi: 10.1038/nsb971
42. Sasaki T, Knyazev PG, Clout NJ, Cheburkin Y, Gohring W, Ullrich A, et al. Structural basis for Gas6-axl signalling. EMBO J (2006) 25(1):80–7. doi: 10.1038/sj.emboj.7600912
43. Linger RM, Keating AK, Earp HS, Graham DK. Tam Receptor tyrosine kinases: biologic functions, signaling, and potential therapeutic targeting in human cancer. Adv Cancer Res (2008) 100:35–83. doi: 10.1016/S0065-230X(08)00002-X
44. Scaltriti M, Elkabets M, Baselga J. Molecular pathways: axl, a membrane receptor mediator of resistance to therapy. Clin Cancer Res (2016) 22(6):1313–7. doi: 10.1158/1078-0432.CCR-15-1458
45. Meertens L, Labeau A, Dejarnac O, Cipriani S, Sinigaglia L, Bonnet-Madin L, et al. Axl mediates zika virus entry in human glial cells and modulates innate immune responses. Cell Rep (2017) 18(2):324–33. doi: 10.1016/j.celrep.2016.12.045
46. Heiring C, Dahlback B, Muller YA. Ligand recognition and homophilic interactions in Tyro3: structural insights into the Axl/Tyro3 receptor tyrosine kinase family. J Biol Chem (2004) 279(8):6952–8. doi: 10.1074/jbc.M311750200
47. Bellosta P, Costa M, Lin DA, Basilico C. The receptor tyrosine kinase ark mediates cell aggregation by homophilic binding. Mol Cell Biol (1995) 15(2):614–25. doi: 10.1128/MCB.15.2.614
48. McCloskey P, Fridell YW, Attar E, Villa J, Jin Y, Varnum B, et al. Gas6 mediates adhesion of cells expressing the receptor tyrosine kinase axl. J Biol Chem (1997) 272(37):23285–91. doi: 10.1074/jbc.272.37.23285
49. Sainaghi PP, Castello L, Bergamasco L, Galletti M, Bellosta P, Avanzi GC. Gas6 induces proliferation in prostate carcinoma cell lines expressing the axl receptor. J Cell Physiol (2005) 204(1):36–44. doi: 10.1002/jcp.20265
50. Wen L, Zheng Y, Wen X, Zhang Y, Zeng W. Increased expression of long noncoding rna Gas6-As2 promotes proliferation and inhibits apoptosis of melanoma cells Via upregulating Gas6 expression. IUBMB Life (2019) 71(10):1503–14. doi: 10.1002/iub.2071
51. Angelillo-Scherrer A, de Frutos P, Aparicio C, Melis E, Savi P, Lupu F, et al. Deficiency or inhibition of Gas6 causes platelet dysfunction and protects mice against thrombosis. Nat Med (2001) 7(2):215–21. doi: 10.1038/84667
52. Wimmel A, Glitz D, Kraus A, Roeder J, Schuermann M. Axl receptor tyrosine kinase expression in human lung cancer cell lines correlates with cellular adhesion. Eur J Cancer (2001) 37(17):2264–74. doi: 10.1016/s0959-8049(01)00271-4
53. Loges S, Schmidt T, Tjwa M, van Geyte K, Lievens D, Lutgens E, et al. Malignant cells fuel tumor growth by educating infiltrating leukocytes to produce the mitogen Gas6. Blood (2010) 115(11):2264–73. doi: 10.1182/blood-2009-06-228684
54. Lay JD, Hong CC, Huang JS, Yang YY, Pao CY, Liu CH, et al. Sulfasalazine suppresses drug resistance and invasiveness of lung adenocarcinoma cells expressing axl. Cancer Res (2007) 67(8):3878–87. doi: 10.1158/0008-5472.CAN-06-3191
55. Nieto MA. Epithelial-mesenchymal transitions in development and disease: old views and new perspectives. Int J Dev Biol (2009) 53(8-10):1541–7. doi: 10.1387/ijdb.072410mn
56. Thiery JP, Acloque H, Huang RY, Nieto MA. Epithelial-mesenchymal transitions in development and disease. Cell (2009) 139(5):871–90. doi: 10.1016/j.cell.2009.11.007
57. Cichon MA, Szentpetery Z, Caley MP, Papadakis ES, Mackenzie IC, Brennan CH, et al. The receptor tyrosine kinase axl regulates cell-cell adhesion and stemness in cutaneous squamous cell carcinoma. Oncogene (2014) 33(32):4185–92. doi: 10.1038/onc.2013.388
58. Goyette MA, Duhamel S, Aubert L, Pelletier A, Savage P, Thibault MP, et al. The receptor tyrosine kinase axl is required at multiple steps of the metastatic cascade during Her2-positive breast cancer progression. Cell Rep (2018) 23(5):1476–90. doi: 10.1016/j.celrep.2018.04.019
59. Lee Y, Lee M, Kim S. Gas6 induces cancer cell migration and epithelial-mesenchymal transition through upregulation of mapk and slug. Biochem Biophys Res Commun (2013) 434(1):8–14. doi: 10.1016/j.bbrc.2013.03.082
60. Yang B, Lieu ZZ, Wolfenson H, Hameed FM, Bershadsky AD, Sheetz MP. Mechanosensing controlled directly by tyrosine kinases. Nano Lett (2016) 16(9):5951–61. doi: 10.1021/acs.nanolett.6b02995
61. May CD, Garnett J, Ma X, Landers SM, Ingram DR, Demicco EG, et al. Axl is a potential therapeutic target in dedifferentiated and pleomorphic liposarcomas. BMC Cancer (2015) 15:901. doi: 10.1186/s12885-015-1916-3
62. Li Y, Ye X, Tan C, Hongo JA, Zha J, Liu J, et al. Axl as a potential therapeutic target in cancer: role of axl in tumor growth, metastasis and angiogenesis. Oncogene (2009) 28(39):3442–55. doi: 10.1038/onc.2009.212
63. Koorstra JB, Karikari CA, Feldmann G, Bisht S, Rojas PL, Offerhaus GJ, et al. The axl receptor tyrosine kinase confers an adverse prognostic influence in pancreatic cancer and represents a new therapeutic target. Cancer Biol Ther (2009) 8(7):618–26. doi: 10.4161/cbt.8.7.7923
64. Melaragno MG, Wuthrich DA, Poppa V, Gill D, Lindner V, Berk BC, et al. Increased expression of axl tyrosine kinase after vascular injury and regulation by G protein-coupled receptor agonists in rats. Circ Res (1998) 83(7):697–704. doi: 10.1161/01.res.83.7.697
65. Li M, Ye J, Zhao G, Hong G, Hu X, Cao K, et al. Gas6 attenuates Lipopolysaccharide−Induced Tnf−α expression and apoptosis in H9c2 cells through Nf−κb and mapk inhibition Via the Axl/Pi3k/Akt pathway. Int J Mol Med (2019) 44(3):982–94. doi: 10.3892/ijmm.2019.4275
66. Kong L, Wu Z, Zhao Y, Lu X, Shi H, Liu S, et al. Qigesan reduces the motility of esophageal cancer cells Via inhibiting Gas6/Axl and nf-κb expression. Biosci Rep (2019) 39(6):BSR20190850. doi: 10.1042/bsr20190850
67. Lee WP, Wen Y, Varnum B, Hung MC. Akt is required for axl-Gas6 signaling to protect cells from E1a-mediated apoptosis. Oncogene (2002) 21(3):329–36. doi: 10.1038/sj.onc.1205066
68. Korshunov VA, Mohan AM, Georger MA, Berk BC. Axl, a receptor tyrosine kinase, mediates flow-induced vascular remodeling. Circ Res (2006) 98(11):1446–52. doi: 10.1161/01.RES.0000223322.16149.9a
69. Lee-Sherick AB, Eisenman KM, Sather S, McGranahan A, Armistead PM, McGary CS, et al. Aberrant mer receptor tyrosine kinase expression contributes to leukemogenesis in acute myeloid leukemia. Oncogene (2013) 32(46):5359–68. doi: 10.1038/onc.2013.40
70. Prieto-Vila M, Takahashi RU, Usuba W, Kohama I, Ochiya T. Drug resistance driven by cancer stem cells and their niche. Int J Mol Sci (2017) 18(12):2574. doi: 10.3390/ijms18122574
71. Jin Y, Nie D, Li J, Du X, Lu Y, Li Y, et al. Gas6/Axl signaling regulates self-renewal of chronic myelogenous leukemia stem cells by stabilizing beta-catenin. Clin Cancer Res (2017) 23(11):2842–55. doi: 10.1158/1078-0432.CCR-16-1298
72. Shan S, Liu Z, Guo T, Wang M, Tian S, Zhang Y, et al. Growth arrest-specific gene 6 transfer promotes mesenchymal stem cell survival and cardiac repair under hypoxia and ischemia Via enhanced autocrine signaling and paracrine action. Arch Biochem Biophys (2018) 660:108–20. doi: 10.1016/j.abb.2018.10.016
73. Quail DF, Joyce JA. Microenvironmental regulation of tumor progression and metastasis. Nat Med (2013) 19(11):1423–37. doi: 10.1038/nm.3394
74. Deng T, Zhang Y, Chen Q, Yan K, Han D. Toll-like receptor-mediated inhibition of Gas6 and pros expression facilitates inflammatory cytokine production in mouse macrophages. Immunology (2012) 135(1):40–50. doi: 10.1111/j.1365-2567.2011.03511.x
75. Semenza GL. Targeting hif-1 for cancer therapy. Nat Rev Cancer (2003) 3(10):721–32. doi: 10.1038/nrc1187
76. Mishra A, Wang J, Shiozawa Y, McGee S, Kim J, Jung Y, et al. Hypoxia stabilizes Gas6/Axl signaling in metastatic prostate cancer. Mol Cancer Res (2012) 10(6):703–12. doi: 10.1158/1541-7786.MCR-11-0569
77. Wilson C, Ye X, Pham T, Lin E, Chan S, McNamara E, et al. Axl inhibition sensitizes mesenchymal cancer cells to antimitotic drugs. Cancer Res (2014) 74(20):5878–90. doi: 10.1158/0008-5472.CAN-14-1009
78. Ruan GX, Kazlauskas A. Axl is essential for vegf-a-Dependent activation of Pi3k/Akt. EMBO J (2012) 31(7):1692–703. doi: 10.1038/emboj.2012.21
79. Tanaka M, Siemann DW. Axl signaling is an important mediator of tumor angiogenesis. Oncotarget (2019) 10(30):2887–98. doi: 10.18632/oncotarget.26882
80. Pinato DJ, Chowdhury S, Stebbing J. Taming resistance to multi-targeted kinase inhibitors through axl and met inhibition. Oncogene (2016) 35(21):2684–6. doi: 10.1038/onc.2015.374
81. Nagarsheth N, Wicha MS, Zou W. Chemokines in the cancer microenvironment and their relevance in cancer immunotherapy. Nat Rev Immunol (2017) 17(9):559–72. doi: 10.1038/nri.2017.49
82. Rothlin CV, Ghosh S, Zuniga EI, Oldstone MB, Lemke G. Tam Receptors are pleiotropic inhibitors of the innate immune response. Cell (2007) 131(6):1124–36. doi: 10.1016/j.cell.2007.10.034
83. Jeon Y, Kang H, Yang Y, Park D, Choi B, Kim J, et al. A novel selective Axl/Mer/Csf1r kinase inhibitor as a cancer immunotherapeutic agent targeting both immune and tumor cells in the tumor microenvironment. Cancers (Basel) (2022) 14(19):4821. doi: 10.3390/cancers14194821
84. Aguilera TA, Rafat M, Castellini L, Shehade H, Kariolis MS, Hui AB, et al. Reprogramming the immunological microenvironment through radiation and targeting axl. Nat Commun (2016) 7:13898. doi: 10.1038/ncomms13898
85. Lee JH, Shklovskaya E, Lim SY, Carlino MS, Menzies AM, Stewart A, et al. Transcriptional downregulation of mhc class I and melanoma de- differentiation in resistance to pd-1 inhibition. Nat Commun (2020) 11(1):1897. doi: 10.1038/s41467-020-15726-7
86. Keir ME, Liang SC, Guleria I, Latchman YE, Qipo A, Albacker LA, et al. Tissue expression of pd-L1 mediates peripheral T cell tolerance. J Exp Med (2006) 203(4):883–95. doi: 10.1084/jem.20051776
87. Iwai Y, Ishida M, Tanaka Y, Okazaki T, Honjo T, Minato N. Involvement of pd-L1 on tumor cells in the escape from host immune system and tumor immunotherapy by pd-L1 blockade. Proc Natl Acad Sci U.S.A. (2002) 99(19):12293–7. doi: 10.1073/pnas.192461099
88. Sadahiro H, Kang KD, Gibson JT, Minata M, Yu H, Shi J, et al. Activation of the receptor tyrosine kinase axl regulates the immune microenvironment in glioblastoma. Cancer Res (2018) 78(11):3002–13. doi: 10.1158/0008-5472.CAN-17-2433
89. Skinner HD, Giri U, Yang LP, Kumar M, Liu Y, Story MD, et al. Integrative analysis identifies a novel axl-Pi3 kinase-Pd-L1 signaling axis associated with radiation resistance in head and neck cancer. Clin Cancer Res (2017) 23(11):2713–22. doi: 10.1158/1078-0432.CCR-16-2586
90. Goyette MA, Elkholi IE, Apcher C, Kuasne H, Rothlin CV, Muller WJ, et al. Targeting axl favors an antitumorigenic microenvironment that enhances immunotherapy responses by decreasing hif-1alpha levels. Proc Natl Acad Sci U.S.A. (2021) 118(29):e2023868118. doi: 10.1073/pnas.2023868118
91. Tsukita Y, Fujino N, Miyauchi E, Saito R, Fujishima F, Itakura K, et al. Axl kinase drives immune checkpoint and chemokine signalling pathways in lung adenocarcinomas. Mol Cancer (2019) 18(1):24. doi: 10.1186/s12943-019-0953-y
92. Waight JD, Hu Q, Miller A, Liu S, Abrams SI. Tumor-derived G-csf facilitates neoplastic growth through a granulocytic myeloid-derived suppressor cell-dependent mechanism. PloS One (2011) 6(11):e27690. doi: 10.1371/journal.pone.0027690
93. Dranoff G. Cytokines in cancer pathogenesis and cancer therapy. Nat Rev Cancer (2004) 4(1):11–22. doi: 10.1038/nrc1252
94. Ludwig KF, Du W, Sorrelle NB, Wnuk-Lipinska K, Topalovski M, Toombs JE, et al. Small-molecule inhibition of axl targets tumor immune suppression and enhances chemotherapy in pancreatic cancer. Cancer Res (2018) 78(1):246–55. doi: 10.1158/0008-5472.CAN-17-1973
95. Li Z, Chen L, Qin Z. Paradoxical roles of il-4 in tumor immunity. Cell Mol Immunol (2009) 6(6):415–22. doi: 10.1038/cmi.2009.53
96. Guo Z, Li Y, Zhang D, Ma J. Axl inhibition induces the antitumor immune response which can be further potentiated by pd-1 blockade in the mouse cancer models. Oncotarget (2017) 8(52):89761–74. doi: 10.18632/oncotarget.21125
97. Nguyen KQ, Tsou WI, Calarese DA, Kimani SG, Singh S, Hsieh S, et al. Overexpression of mertk receptor tyrosine kinase in epithelial cancer cells drives efferocytosis in a gain-of-Function capacity. J Biol Chem (2014) 289(37):25737–49. doi: 10.1074/jbc.M114.570838
98. Cohen PL, Caricchio R, Abraham V, Camenisch TD, Jennette JC, Roubey RA, et al. Delayed apoptotic cell clearance and lupus-like autoimmunity in mice lacking the c-mer membrane tyrosine kinase. J Exp Med (2002) 196(1):135–40. doi: 10.1084/jem.20012094
99. Bosurgi L, Cao YG, Cabeza-Cabrerizo M, Tucci A, Hughes LD, Kong Y, et al. Macrophage function in tissue repair and remodeling requires il-4 or il-13 with apoptotic cells. Science (2017) 356(6342):1072–6. doi: 10.1126/science.aai8132
100. Cook RS, Jacobsen KM, Wofford AM, DeRyckere D, Stanford J, Prieto AL, et al. Mertk inhibition in tumor leukocytes decreases tumor growth and metastasis. J Clin Invest (2013) 123(8):3231–42. doi: 10.1172/JCI67655
101. Chiu KC, Lee CH, Liu SY, Chou YT, Huang RY, Huang SM, et al. Polarization of tumor-associated macrophages and Gas6/Axl signaling in oral squamous cell carcinoma. Oral Oncol (2015) 51(7):683–9. doi: 10.1016/j.oraloncology.2015.04.004
102. Werfel TA, Cook RS. Efferocytosis in the tumor microenvironment. Semin Immunopathol (2018) 40(6):545–54. doi: 10.1007/s00281-018-0698-5
103. Vergadi E, Ieronymaki E, Lyroni K, Vaporidi K, Tsatsanis C. Akt signaling pathway in macrophage activation and M1/M2 polarization. J Immunol (2017) 198(3):1006–14. doi: 10.4049/jimmunol.1601515
104. Sun B, Qi N, Shang T, Wu H, Deng T, Han D. Sertoli cell-initiated testicular innate immune response through toll-like receptor-3 activation is negatively regulated by Tyro3, axl, and mer receptors. Endocrinology (2010) 151(6):2886–97. doi: 10.1210/en.2009-1498
105. Caraux A, Lu Q, Fernandez N, Riou S, Di Santo JP, Raulet DH, et al. Natural killer cell differentiation driven by Tyro3 receptor tyrosine kinases. Nat Immunol (2006) 7(7):747–54. doi: 10.1038/ni1353
106. Park IK, Giovenzana C, Hughes TL, Yu J, Trotta R, Caligiuri MA. The Axl/Gas6 pathway is required for optimal cytokine signaling during human natural killer cell development. Blood (2009) 113(11):2470–7. doi: 10.1182/blood-2008-05-157073
107. Sceneay J, Chow MT, Chen A, Halse HM, Wong CS, Andrews DM, et al. Primary tumor hypoxia recruits Cd11b+/Ly6cmed/Ly6g+ immune suppressor cells and compromises nk cell cytotoxicity in the premetastatic niche. Cancer Res (2012) 72(16):3906–11. doi: 10.1158/0008-5472.CAN-11-3873
108. Kim EM, Lee EH, Lee HY, Choi HR, Ji KY, Kim SM, et al. Axl signaling induces development of natural killer cells in vitro and in vivo. Protoplasma (2017) 254(2):1091–101. doi: 10.1007/s00709-016-1016-5
109. Waldmann TA, Tagaya Y. The multifaceted regulation of interleukin-15 expression and the role of this cytokine in nk cell differentiation and host response to intracellular pathogens. Annu Rev Immunol (1999) 17:19–49. doi: 10.1146/annurev.immunol.17.1.19
110. Joyce JA, Fearon DT. T Cell exclusion, immune privilege, and the tumor microenvironment. Science (2015) 348(6230):74–80. doi: 10.1126/science.aaa6204
111. Aguilera TA, Giaccia AJ. Molecular pathways: oncologic pathways and their role in T-cell exclusion and immune evasion-a new role for the axl receptor tyrosine kinase. Clin Cancer Res (2017) 23(12):2928–33. doi: 10.1158/1078-0432.CCR-17-0189
112. Paolino M, Penninger JM. The role of Tam family receptors in immune cell function: implications for cancer therapy. Cancers (Basel) (2016) 8(10):97. doi: 10.3390/cancers8100097
113. Lee W, Kim DK, Synn CB, Lee HK, Park S, Jung DS, et al. Incorporation of ski-G-801, a novel axl inhibitor, with anti-Pd-1 plus chemotherapy improves anti-tumor activity and survival by enhancing T cell immunity. Front Oncol (2022) 12:821391. doi: 10.3389/fonc.2022.821391
114. Jacobs JF, Nierkens S, Figdor CG, de Vries IJ, Adema GJ. Regulatory T cells in melanoma: the final hurdle towards effective immunotherapy? Lancet Oncol (2012) 13(1):e32–42. doi: 10.1016/S1470-2045(11)70155-3
115. Sakaguchi S, Yamaguchi T, Nomura T, Ono M. Regulatory T cells and immune tolerance. Cell (2008) 133(5):775–87. doi: 10.1016/j.cell.2008.05.009
116. Zhao GJ, Zheng JY, Bian JL, Chen LW, Dong N, Yu Y, et al. Growth arrest-specific 6 enhances the suppressive function of Cd4(+)Cd25(+) regulatory T cells mainly through axl receptor. Mediators Inflammation (2017) 2017:6848430. doi: 10.1155/2017/6848430
117. Brand TM, Iida M, Stein AP, Corrigan KL, Braverman CM, Coan JP, et al. Axl is a logical molecular target in head and neck squamous cell carcinoma. Clin Cancer Res (2015) 21(11):2601–12. doi: 10.1158/1078-0432.CCR-14-2648
118. Mahadevan D, Cooke L, Riley C, Swart R, Simons B, Della Croce K, et al. A novel tyrosine kinase switch is a mechanism of imatinib resistance in gastrointestinal stromal tumors. Oncogene (2007) 26(27):3909–19. doi: 10.1038/sj.onc.1210173
119. Wang C, Jin H, Wang N, Fan S, Wang Y, Zhang Y, et al. Gas6/Axl axis contributes to chemoresistance and metastasis in breast cancer through Akt/Gsk-3beta/Beta-Catenin signaling. Theranostics (2016) 6(8):1205–19. doi: 10.7150/thno.15083
120. D'Errico G, Alonso-Nocelo M, Vallespinos M, Hermann PC, Alcala S, Garcia CP, et al. Tumor-associated macrophage-secreted 14-3-3zeta signals Via axl to promote pancreatic cancer chemoresistance. Oncogene (2019) 38(27):5469–85. doi: 10.1038/s41388-019-0803-9
121. Macleod K, Mullen P, Sewell J, Rabiasz G, Lawrie S, Miller E, et al. Altered erbb receptor signaling and gene expression in cisplatin-resistant ovarian cancer. Cancer Res (2005) 65(15):6789–800. doi: 10.1158/0008-5472.CAN-04-2684
122. Brand TM, Iida M, Stein AP, Corrigan KL, Braverman CM, Luthar N, et al. Axl mediates resistance to cetuximab therapy. Cancer Res (2014) 74(18):5152–64. doi: 10.1158/0008-5472.CAN-14-0294
123. Zhang Z, Lee JC, Lin L, Olivas V, Au V, LaFramboise T, et al. Activation of the axl kinase causes resistance to egfr-targeted therapy in lung cancer. Nat Genet (2012) 44(8):852–60. doi: 10.1038/ng.2330
124. Taniguchi H, Yamada T, Wang R, Tanimura K, Adachi Y, Nishiyama A, et al. Axl confers intrinsic resistance to osimertinib and advances the emergence of tolerant cells. Nat Commun (2019) 10(1):259. doi: 10.1038/s41467-018-08074-0
125. Hugo W, Zaretsky JM, Sun L, Song C, Moreno BH, Hu-Lieskovan S, et al. Genomic and transcriptomic features of response to anti-Pd-1 therapy in metastatic melanoma. Cell (2016) 165(1):35–44. doi: 10.1016/j.cell.2016.02.065
126. Terry S, Abdou A, Engelsen AST, Buart S, Dessen P, Corgnac S, et al. Axl targeting overcomes human lung cancer cell resistance to nk- and ctl-mediated cytotoxicity. Cancer Immunol Res (2019) 7(11):1789–802. doi: 10.1158/2326-6066.CIR-18-0903
127. Msaouel P, Goswami S, Thall PF, Wang X, Yuan Y, Jonasch E, et al. A phase 1-2 trial of sitravatinib and nivolumab in clear cell renal cell carcinoma following progression on antiangiogenic therapy. Sci Transl Med (2022) 14(641):eabm6420. doi: 10.1126/scitranslmed.abm6420
128. Sharp LL, Chang C, Frey G, Wang J, Liu H, Xing C, et al. Abstract 827: anti-tumor efficacy of Ba3011, a novel conditionally active biologic (Cab) anti-Axl-Adc. Cancer Res (2018) 78(13_Supplement):827–. doi: 10.1158/1538-7445.AM2018-827
129. Fuh KC, Bookman MA, Liu JF, Coleman RL, Herzog TJ, Thaker PH, et al. Phase 1b study of avb-500 in combination with paclitaxel or pegylated liposomal doxorubicin platinum-resistant recurrent ovarian cancer. Gynecol Oncol (2021) 163(2):254–61. doi: 10.1016/j.ygyno.2021.08.020
130. Wei J, Sun H, Zhang A, Wu X, Li Y, Liu J, et al. A novel axl chimeric antigen receptor endows T cells with anti-tumor effects against triple negative breast cancers. Cell Immunol (2018) 331:49–58. doi: 10.1016/j.cellimm.2018.05.004
131. Okura N, Nishioka N, Yamada T, Taniguchi H, Tanimura K, Katayama Y, et al. Ono-7475, a novel axl inhibitor, suppresses the adaptive resistance to initial egfr-tki treatment in egfr-mutated non-small cell lung cancer. Clin Cancer Res (2020) 26(9):2244–56. doi: 10.1158/1078-0432.Ccr-19-2321
132. Cummings CT, Deryckere D, Earp HS, Graham DK. Molecular pathways: mertk signaling in cancer. Clin Cancer Res (2013) 19(19):5275–80. doi: 10.1158/1078-0432.CCR-12-1451
133. Kariolis MS, Miao YR, Jones DS 2nd, Kapur S, Mathews II, Giaccia AJ, et al. An engineered axl 'Decoy receptor' effectively silences the Gas6-axl signaling axis. Nat Chem Biol (2014) 10(11):977–83. doi: 10.1038/nchembio.1636
Keywords: Gas6/TAM, AXL, cancer development, TME, drug resistance
Citation: Zhai X, Pu D, Wang R, Zhang J, Lin Y, Wang Y, Zhai N, Peng X, Zhou Q and Li L (2023) Gas6/AXL pathway: immunological landscape and therapeutic potential. Front. Oncol. 13:1121130. doi: 10.3389/fonc.2023.1121130
Received: 11 December 2022; Accepted: 10 April 2023;
Published: 10 May 2023.
Edited by:
Swapna Chaudhuri, Chittaranjan National Cancer Institute (CNCI), IndiaReviewed by:
Douglas K. Graham, Emory University, United StatesCopyright © 2023 Zhai, Pu, Wang, Zhang, Lin, Wang, Zhai, Peng, Zhou and Li. This is an open-access article distributed under the terms of the Creative Commons Attribution License (CC BY). The use, distribution or reproduction in other forums is permitted, provided the original author(s) and the copyright owner(s) are credited and that the original publication in this journal is cited, in accordance with accepted academic practice. No use, distribution or reproduction is permitted which does not comply with these terms.
*Correspondence: Xuan Peng, NTkzMjM0QHFxLmNvbQ==; Qinghua Zhou, cHJvZl9xaF96aG91QDEyNi5jb20=; Lu Li, d2luZGZsb3dlcjE5OTFAdmlwLjE2My5jb20=
†These authors have contributed equally to this work and share first authorship
Disclaimer: All claims expressed in this article are solely those of the authors and do not necessarily represent those of their affiliated organizations, or those of the publisher, the editors and the reviewers. Any product that may be evaluated in this article or claim that may be made by its manufacturer is not guaranteed or endorsed by the publisher.
Research integrity at Frontiers
Learn more about the work of our research integrity team to safeguard the quality of each article we publish.