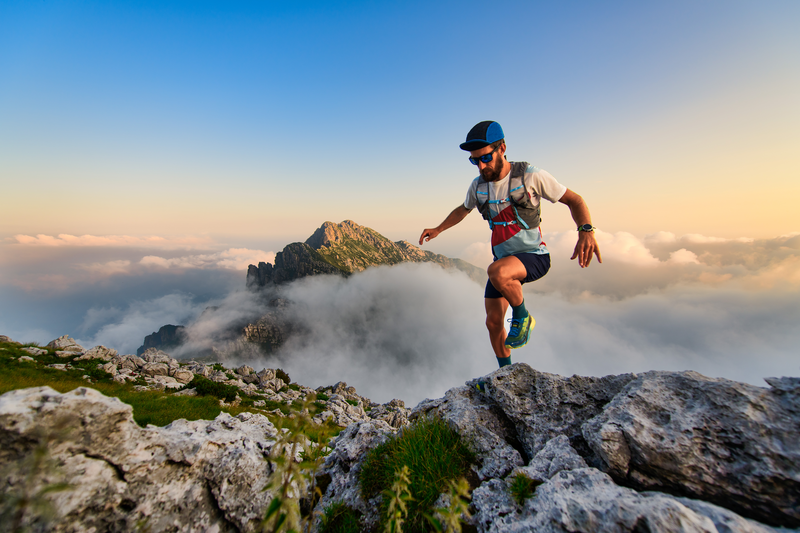
94% of researchers rate our articles as excellent or good
Learn more about the work of our research integrity team to safeguard the quality of each article we publish.
Find out more
ORIGINAL RESEARCH article
Front. Oncol. , 14 February 2023
Sec. Hematologic Malignancies
Volume 13 - 2023 | https://doi.org/10.3389/fonc.2023.1117815
This article is part of the Research Topic Covid-19 Therapies in Patients with Hematologic Malignancies View all 8 articles
Patients affected by myelofibrosis (MF) or polycythemia vera (PV) and treated with ruxolitinib are at high risk for severe coronavirus disease 2019. Now a vaccine against the virus SARS-CoV-2, which is responsible for this disease, is available. However, sensitivity to vaccines is usually lower in these patients. Moreover, fragile patients were not included in large trials investigating the efficacy of vaccines. Thus, little is known about the efficacy of this approach in this group of patients. In this prospective single-center study, we evaluated 43 patients (30 MF patients and 13 with PV) receiving ruxolitinib as a treatment for their myeloproliferative disease. We measured anti-spike and anti-nucleocapsid IgG against SARS-CoV2 15-30 days after the second and the third BNT162b2 mRNA vaccine booster dose. Patients receiving ruxolitinib showed an impaired antibody response to complete vaccination (2 doses), as 32.5% of patients did not develop any response. After the third booster dose with Comirnaty, results slightly improved, as 80% of these patients produced antibodies above the threshold positivity. However, the quantity of produced antibodies was well below that reached than those reported for healthy individuals. PV patients elicited a better response than patients affected by MF. Thus, different strategies should be considered for this high-risk group of patients.
In February 2020, the World Health Organization (WHO) declared the pandemic for COVID-19 infection caused by the novel coronavirus SARS-CoV-2. The clinical course of the disease is very heterogeneous, spanning from asymptomatic infection to acute respiratory distress syndrome (ARDS) and eventually death (1). Compared to healthy people, patients with comorbidities are considered at higher risk of more aggressive disease and developing severe complications, and myeloproliferative disorders are no exception (2, 3).
On December 2020, results of the BNT162b2 mRNA Covid-19 vaccine clinical trial results were published (4), demonstrating that fully vaccinated people gained a 95% protection against Covid-19, usually reaching a titer >1000 AU/ml (5–7). However, the trial was not conducted on specific fragile patient populations, and data for these subgroups were unavailable. Recently, it has been shown that protection gained by vaccination could be lower in specific immunocompromised patients due to the ongoing treatments and/or the disease itself (8–10). Hemato-oncological patients were among those with blunted vaccination efficacy (5, 11–16).
This is mostly true for lymphoproliferative disorders (5, 11–15, 17), while, in patients with myeloproliferative disorders, a response to vaccination with BNT162b2 like that obtained in healthy individuals has been reported (18–20). In myeloproliferative disorders, a lower Ab response has been reported in MF than in PV or ET (20, 21). Furthermore, ruxolitinib, a JAK 1/2 inhibitor, is widely used in the treatment of MF (22–25) and of hydroxyurea intolerant\resistant PV patients (26, 27). This molecule exerts strong immunosuppressive activity (28) and could be, at least in part, responsible for the inferior efficacy of vaccination. Indeed, in a small number of myeloproliferative patients treated with ruxolitinib, a blunted response to the first (19, 29) and second dose of vaccine (21, 30, 31) was reported. As little data were available in myeloproliferative patients treated with ruxolitinib who had completed the vaccination cycle (2 doses) and a third booster dose, in this study, we investigated whether these patients could reach a protective antibody level against the SARS-CoV-2 virus, as in Italy these patients were granted a fast-track vaccination with BNT162b2 (23).
All study participants were administered the two-dose regimen BNT162b2 mRNA vaccine (Corminaty, Pfizer-BioNTech), 30 mcg per dose, by intramuscular injection in the deltoid muscle three weeks apart, as indicated by the Italian national guidelines.
After obtaining informed consent, whole blood sera from the peripheral blood of 43 patients were treated with ruxolitinib. 15 patients were affected by primary MF (PMF), 15 by secondary MF (10 post-PV, PPV-MF, and 5 post-ET, PET-MF) and 13 by PV.
Prognostic risk at first vaccination was calculated with Dynamic International Prognostic Scoring System (DIPSS) (32) for PMF patients: 2 were low, 5 intermediate-1 (Int-1), 7 intermediate-2 (Int-2) and 1 high risk. At the time of booster dose administration, there were no changes in the DIPSS score. Thirteen out of 15 harbored the JAK2 V617F driver mutation, the remaining 2 the CALR mutation.
For secondary MF patients, MYSEC-PM prognostic score (33) was used: 1 patient was low, 5 Int-1, 5 Int-2 and 4 high risk at first vaccination; at booster vaccination, only 2 patients progressed, one from Low to Int-1 and the other from Int-2 to High risk. All PPV-MF harbored the JAK2 V617F mutation together with 3 out of 5 PET-MF, while the remaining 2 had the CALR mutation.
Thirteen patients had a diagnosis of PV, 12 harboring the JAK2 V617F mutation and the remaining one the JAK2 Exon 12. All had received hydroxyurea (HU) treatment before switching to ruxolitinib; for 7 patients this treatment change was due to intolerance, while 6 were resistant to HU according to European Leukemia Network (ELN) consensus criteria (34).
At the time of the first vaccination, the median age was 69 years (range 46-86); for MF patients, the median was 72 years (range 46-86 years), while for PV patients was 64 years (range 50-78 years). The median spleen size was 3 centimeters below the costal margin (range 0-20 cm); in MF patients, the median was 4,6 cm (range 0-20), while in PV patients, it was 0 cm (range 0-2 cm).
After completing the standard vaccination cycle, sera were obtained when they were considered fully vaccinated, at least 14 days (median 36 days, range 14-53) since having received the second dose.
Thirty-nine out of 43 patients received the booster dose, as 2 patients died before, and 2 refused vaccination for personal reasons. The third dose was given at least 32 days and not later than 243 days (median 153 days) after the second dose. Samples were obtained just preceding (the same or the day before) and following the booster administration (median 26 days, range 11-49 days).
Sera were immediately frozen at -20°C until analysis. All demographic data are reported in Table 1 and 2.
Ruxolitinib administration was started 10-3370 days (median 1236 days) before the first vaccine dose; for MF patients, 10-3288 days before (median 1414 days) while for PV patients 53-3370 days before (median 817 days).
The median ruxolitinib dose at the beginning of the vaccine cycle was 14.7 mg BID (min, max: 2.5, 25 mg); for MF patients, 16,1 mg BID (range 2.5-25 mg), for PV 11,5 mg BID (range 5-15 mg).
There were no significant differences between primary and secondary MF patients, both for the length of exposure and dosages of ruxolitinib.
The SARS-CoV2 virus produces 4 structural proteins, namely envelope, membrane, nucleocapsid and spike, the latter the more immunogenic. Thus, the BNT162b2 mRNA vaccine was designed to induce a strong anti-spike response, resulting in the generation of neutralizing antibodies in >95% of subjects who received 2 doses (35).
The vaccine immunogenicity was evaluated by measuring the serum IgG neutralizing Ab levels against the RBD portion of the spike protein (anti-S), using the IgG II Quant kit (Abbott, Chicago, IL, USA), a chemiluminescent microparticle immunoassay (CIMSA) according to the manufacturer’s instructions on an Architect i2000SR/i4000SR platform. This assay has an optimized sensitivity of 88-98% and a specificity of 100% (36, 37). A value above 7 binding antibody units (BAU), the standardized value according to World Health Organization, was considered as positive.
Vaccine efficacy, in terms of protective immunity, is correlated to the presence of neutralizing antibodies (38–40). In this study, the level of IgG against spike receptor binding domain was used as surrogate markers of neutralizing antibodies because their levels are linearly correlated (41, 42) and as it was deeply shown in animal models (43, 44).
IgG against SARS-CoV-2 nucleocapsid proteins (anti-N) were measured to rule out a prior or ongoing SARS-CoV2 virus infection before vaccination (7). In fact, vaccinated subjects should be anti-S positive and anti-N negative, while patients exposed to the natural virus are anti-S and anti-N positive at the same time (45).
Two patients, both affected by PMF, resulted in IgG against SARS-CoV-2 nucleocapsid proteins (anti-N), proving a prior exposure to the virus at the time of the first vaccination, while the remaining 41 were negative. Interestingly, these two subjects showed a high antibody response after vaccination (1333.9 and 6901.5 BAU/ml).
At the completion of the two-dose standard vaccination cycle, the average level of serum IgG neutralizing antibody levels against the RBD portion of the spike protein (anti-S) was 369.5 BAU/ml (range 0-6901 BAUI/ml).
Fourteen out of 43 patients had anti-S antibodies below the threshold positivity; 13/30 MF patients were negative, while only 1/13 PV patients did not mount an antibody response.; 27/43 patients showed less than 60 BAU/ml and 28/44 below 100 BAU/ml.
Antibody (Ab) levels before the third booster dose were low (median 12.3, range 0-54.2 BAU/ml). After it, the median value of 272.3 BAU/ml (range 0-1399 BAU/ml was reached. In 8/40 patients (7/27 MF and 1/12 PV), Ab did not raise above the positivity threshold; in 19/39, Ab were below 60 BAU/ml, in 24/39, below 100 BAU/ml. Antibodies development after vaccine doses are reported in Tables 1, 2, and in Figure 1.
Figure 1 Levels of antibodies against SARS-CoV-2, expressed in BAU/mL, in the context of polycythemia vera, primary and secondary myelofibrosis, during the different time points.
Vaccination against SARS-CoV2 is considered the most important preventive strategy to counteract severe COVID-19, but its efficacy in hematological malignancy patients seems to be less effective while, on the contrary, these subjects have a high incidence of morbidity and mortality from SARS-CoV-2 infection (2, 17, 46). The blunted response to vaccination has been mainly reported for lymphoproliferative disorders (5, 7, 11, 13–15), while similar responses to those reported in healthy subjects were seen in patients with myeloproliferative disorders (18–20). However, in these patients, a lower response to vaccination could be determined by the type of disease, as it has been reported that MF patients produce fewer antibodies than other bcr\abl negative myeloproliferative disorders (20). The ongoing treatment might also be critical. In particular, ruxolitinib, a widely used JAKi (22–27), is known to downregulate immune response with effects on B, T, dendritic, and NK cells (28) and could be the primary cause of the inferior efficacy of vaccination. Confirming this hypothesis, in patients undergoing ruxolitinib treatment, recently, an impaired response to the first (29) and second dose of vaccine (21, 47) has been demonstrated. Antibodies elicited by vaccination are of key importance to protect subjects from the disease or, in other words, might be able to neutralize the virus (38).
Our study confirmed that ruxolitinib-treated MPN patients who have received 2 standard doses of BNT162b2 show a markedly impaired Ab production. A third booster dose was reported to be able to improve response to vaccination in MF patients (47). This has been shown to be true in our series too. However, although the third booster dose was able to reduce the number of patients who remained fully negative (20% vs. 32.6%), the median Ab value reached was not significantly better, and levels were far from those obtained with the same vaccine dose and schedule in normal subjects. Interestingly, in our and other series (20), MF patients showed a worse response than PV patients, and the explanation could reside in the greater severity of the disease itself (21). Then, it should be taken into consideration that in our series, MF patients showed older age at the time of vaccination, bigger spleen size, longer exposure to ruxolitinib, and at higher median doses, compared to PV patients; each of these factors could play a role in the reduced response to vaccination. Although our results demonstrate a lower humoral response in patients who were assuming ruxolitinib at the same time of Comirnaty administration, caution is needed in concluding that these subjects are not protected against the virus. First, there are not universally validated and accepted antibody cutoffs that correlate with protection against severe COVID-19 disease. A critical point is, in fact, represented by the difference between the antibody positivity, useful to determine whether a subject has been infected by the virus, and the antibody levels that are able to induce a clinically relevant inhibition, that is considered above 50% inhibition (48).
However, in more than 60% of patients, the maximum levels reached are below 100 BAU/ml either after the second and the third vaccine injection. Thus, these patients will have antibodies below the clinically relevant inhibition titer in a short time, considering the known waning in antibody levels over time (42, 49, 50), as it is well known for other coronaviruses. In fact, the decline in total antibodies able to bind the spike protein reflects the decline in neutralizing antibody (41, 42). On the other hand, the disappearance of antibodies reflects the decline in short-lived plasmablasts, while the demonstrated presence of long-lived memory plasma cells could support a rapid response in case of a rechallenge (42). It has been calculated that antibody titers should exponentially fall 250 days after vaccination (40), as it was demonstrated in vivo for antibodies after natural infection (51).
In the healthy population, the Ab decline can be counteracted by a strategy based on the administration of a third booster dose. Disappointingly, in our cohort of patients, less than 40% of subjects have anti-spike antibodies above the 100 BAU/ml threshold even after the booster injection and, in any case, well below the levels reached in healthy subjects. Nonetheless, low levels of neutralizing antibodies could persist over time and represent the first line of defense against viral infection (42). Furthermore, surrogate markers of sterilizing immunity have always to be interpreted, taking into consideration the actual scenario with new variants of the virus that keep on emerging (7). And that might need higher Ab levels to be fought.
In addition, it is not known the extent to which humoral response contributes to vaccine efficacy (8). The role of other arms of the immune response, namely cellular immunity elicited by CD4+ and CD8+ T cells, known to be raised by vaccination, must be taken into consideration, and this point is not addressed by our study. In fact, the first subset of T cells is considered a pivot in integrating immune responses, while the latter plays a role in killing cells infected by viruses (52). Both memory and cytotoxic T cells against viruses were shown to last more than 15 years, thus giving the immunized subjects sustained protection over time (53). Indeed, the BNT162b2 vaccine can elicit both humoral and cellular immune responses (54). However, a specific and robust T cell response is more likely to be seen in those patients that elicited a broad functional humoral immune response (50, 55). Thus, antibody levels might be used as a surrogate marker of a good immune response, not limited to B-cells, and can be predictive of protection given by vaccination as the true defensive strength is difficult to assess (38, 39).
It must be considered that the number of participants in our study is relatively low, and no randomized control group has been included. Besides, the limited size of the series does not allow to explore the factors associated with the complete lack of response to the booster dose in around 20% of the patients. Thus, the final word might be given by real-world pharmacovigilance data on the vulnerable population (8). Myeloproliferative patients treated with ruxolitinib should be encouraged to undergo specific vaccination protocols, including prioritization of these patients for a third booster dose that might raise antibody titers (56–59). Furthermore, treatment initiation should be delayed until at least two doses of vaccine have been administered, when clinically possible. For those subjects in which the therapy could not be delayed, newer JAKi, with less immunosuppressive activity, could be considered (28), while for those who are already under ruxolitinib treatment and mount a blunted response, a treatment with Cilgavimab plus Tixagevimab Monoclonal Antibody Cocktail for COVID-19 Prophylaxis could be proposed (23, 60, 61). Anyhow, doctors should be informed that this high-risk group may not be fully protected by vaccination and that risk mitigation, such as social distancing and hygiene measures, should be always implemented by the patients and their caregivers.
The original contributions presented in the study are included in the article/supplementary material. Further inquiries can be directed to the corresponding author/s.
The study was conducted in accordance with the Declaration of Helsinki and approved by Ethical Committee CT1/AOU Policlinico "G. Rodolico"/San Marco, Catania, Italy. Informed consent was obtained from all subjects involved in the study.
GAP, DT, and CG designed the research, analyzed the data, and wrote the manuscript. DC, EL, ALo, ALa and AD collected and analyzed the data. CV, AR, AD, and FD analyzed the data and revised the manuscript. All authors contributed to the article and approved the submitted version.
GAP received honoraria from Abbvie, AOP, AstraZeneca, BMS Celgene, GSK, Incyte, Janssen, and Novartis. CV received honoraria from Jazz Pharmaceuticals and Advisory Board for BMS Celgene. AD received honoraria from BMS Celgene, EusaPharma. AR received honoraria from Amgen, Janssen, Celgene, and Takeda. FD received honoraria from Amgen, Janssen, Celgene, and Takeda.
The remaining authors declare that the research was conducted in the absence of any commercial or financial relationships that could be construed as a potential conflict of interest.
All claims expressed in this article are solely those of the authors and do not necessarily represent those of their affiliated organizations, or those of the publisher, the editors and the reviewers. Any product that may be evaluated in this article, or claim that may be made by its manufacturer, is not guaranteed or endorsed by the publisher.
1. Longhitano L, Tibullo D, Giallongo C, Lazzarino G, Tartaglia N, Galimberti S, et al. Proteasome inhibitors as a possible therapy for SARS-CoV-2. Int J Mol Sci (2020) 21(10):3622-32. doi: 10.3390/ijms21103622
2. Passamonti F, Cattaneo C, Arcaini L, Bruna R, Cavo M, Merli F, et al. Clinical characteristics and risk factors associated with COVID-19 severity in patients with haematological malignancies in Italy: a retrospective, multicentre, cohort study. Lancet Haematol (2020) 7(10):e737–e45. doi: 10.1016/S2352-3026(20)30251-9
3. Barbui T, Iurlo A, Masciulli A, Carobbio A, Ghirardi A, Rossi G, et al. Long-term follow-up of recovered MPN patients with COVID-19. Blood Cancer J (2021) 11(6):115. doi: 10.1038/s41408-021-00509-0
4. Polack FP, Thomas SJ, Kitchin N, Absalon J, Gurtman A, Lockhart S, et al. Safety and efficacy of the BNT162b2 mRNA covid-19 vaccine. N Engl J Med (2020) 383(27):2603–15. doi: 10.1056/NEJMoa2034577
5. Herishanu Y, Avivi I, Aharon A, Shefer G, Levi S, Bronstein Y, et al. Efficacy of the BNT162b2 mRNA COVID-19 vaccine in patients with chronic lymphocytic leukemia. Blood (2021) 137(23):3165–73. doi: 10.1182/blood.2021011568
6. Addeo A, Shah PK, Bordry N, Hudson RD, Albracht B, Di Marco M, et al. Immunogenicity of SARS-CoV-2 messenger RNA vaccines in patients with cancer. Cancer Cell (2021) 39(8):1091–8.e2. doi: 10.1016/j.ccell.2021.06.009
7. Bradley BT, Bryan A, Fink SL, Goecker EA, Roychoudhury P, Huang ML, et al. Anti-SARS-CoV-2 antibody levels measured by the AdviseDx SARS-CoV-2 assay are concordant with previously available serologic assays but are not fully predictive of sterilizing immunity. J Clin Microbiol (2021) 59(9):e0098921. doi: 10.1128/JCM.00989-21
8. Mahil SK, Bechman K, Raharja A, Domingo-Vila C, Baudry D, Brown MA, et al. The effect of methotrexate and targeted immunosuppression on humoral and cellular immune responses to the COVID-19 vaccine BNT162b2: a cohort study. Lancet Rheumatol (2021) 3(9):e627–e37. doi: 10.1016/S2665-9913(21)00212-5
9. Monin L, Laing AG, Munoz-Ruiz M, McKenzie DR, Del Molino Del Barrio I, Alaguthurai T, et al. Safety and immunogenicity of one versus two doses of the COVID-19 vaccine BNT162b2 for patients with cancer: interim analysis of a prospective observational study. Lancet Oncol (2021) 22(6):765–78. doi: 10.1016/S1470-2045(21)00213-8
10. Furer V, Eviatar T, Zisman D, Peleg H, Paran D, Levartovsky D, et al. Immunogenicity and safety of the BNT162b2 mRNA COVID-19 vaccine in adult patients with autoimmune inflammatory rheumatic diseases and in the general population: a multicentre study. Ann Rheum Dis (2021) 80(10):1330–8. doi: 10.1136/annrheumdis-2021-220647
11. Kastritis E, Terpos E, Sklirou A, Theodorakakou F, Fotiou D, Papanagnou ED, et al. Antibody response after initial vaccination for SARS-CoV-2 in patients with amyloidosis. Hemasphere (2021) 5(8):e614. doi: 10.1097/HS9.0000000000000614
12. Gavriatopoulou M, Terpos E, Kastritis E, Briasoulis A, Gumeni S, Ntanasis-Stathopoulos I, et al. Low neutralizing antibody responses in WM, CLL and NHL patients after the first dose of the BNT162b2 and AZD1222 vaccine. Clin Exp Med (2022) 22(2):319–23. doi: 10.1007/s10238-021-00746-4
13. Terpos E, Gavriatopoulou M, Ntanasis-Stathopoulos I, Briasoulis A, Gumeni S, Malandrakis P, et al. The neutralizing antibody response post COVID-19 vaccination in patients with myeloma is highly dependent on the type of anti-myeloma treatment. Blood Cancer J (2021) 11(8):138. doi: 10.1038/s41408-021-00530-3
14. Roeker LE, Knorr DA, Thompson MC, Nivar M, Lebowitz S, Peters N, et al. COVID-19 vaccine efficacy in patients with chronic lymphocytic leukemia. Leukemia (2021) 35(9):2703–5. doi: 10.1038/s41375-021-01270-w
15. Avivi I, Balaban R, Shragai T, Sheffer G, Morales M, Aharon A, et al. Humoral response rate and predictors of response to BNT162b2 mRNA COVID19 vaccine in patients with multiple myeloma. Br J Haematol (2021) 195(2):186–93. doi: 10.1111/bjh.17608
16. Thakkar A, Gonzalez-Lugo JD, Goradia N, Gali R, Shapiro LC, Pradhan K, et al. Seroconversion rates following COVID-19 vaccination among patients with cancer. Cancer Cell (2021) 39(8):1081–90.e2. doi: 10.1016/j.ccell.2021.06.002
17. Mato AR, Roeker LE, Lamanna N, Allan JN, Leslie L, Pagel JM, et al. Outcomes of COVID-19 in patients with CLL: a multicenter international experience. Blood (2020) 136(10):1134–43. doi: 10.1182/blood.2020006965
18. Harrington P, Doores KJ, Radia D, O'Reilly A, Lam HPJ, Seow J, et al. Single dose of BNT162b2 mRNA vaccine against severe acute respiratory syndrome coronavirus-2 (SARS-CoV-2) induces neutralising antibody and polyfunctional T-cell responses in patients with chronic myeloid leukaemia. Br J Haematol (2021) 194(6):999–1006. doi: 10.1111/bjh.17568
19. Harrington P, de Lavallade H, Doores KJ, O'Reilly A, Seow J, Graham C, et al. Single dose of BNT162b2 mRNA vaccine against SARS-CoV-2 induces high frequency of neutralising antibody and polyfunctional T-cell responses in patients with myeloproliferative neoplasms. Leukemia (2021) 35(12):3573–7. doi: 10.1038/s41375-021-01300-7
20. Pimpinelli F, Marchesi F, Piaggio G, Giannarelli D, Papa E, Falcucci P, et al. Fifth-week immunogenicity and safety of anti-SARS-CoV-2 BNT162b2 vaccine in patients with multiple myeloma and myeloproliferative malignancies on active treatment: preliminary data from a single institution. J Hematol Oncol (2021) 14(1):81. doi: 10.1186/s13045-021-01090-6
21. Auteri G, Bartoletti D, Di Pietro C, Sutto E, Mazzoni C, Romagnoli AD, et al. Longer-term response to SARS-CoV-2 vaccine in MPN patients: Role of ruxolitinib and disease severity. Leuk Res (2022) 116:106819. doi: 10.1016/j.leukres.2022.106819
22. Al-Ali HK, Griesshammer M, Foltz L, Palumbo GA, Martino B, Palandri F, et al. Primary analysis of JUMP, a phase 3b, expanded-access study evaluating the safety and efficacy of ruxolitinib in patients with myelofibrosis, including those with low platelet counts. Br J Haematol (2020) 189(5):888–903. doi: 10.1111/bjh.16462
23. Breccia M, Piciocchi A, Messina M, Soddu S, De Stefano V, Bellini M, et al. Covid-19 in Philadelphia-negative myeloproliferative neoplasms: a GIMEMA survey on incidence, clinical management and vaccine. Leukemia (2022) 36(10):2548–50. doi: 10.1038/s41375-022-01675-1
24. Palandri F, Tiribelli M, Breccia M, Bartoletti D, Elli EM, Benevolo G, et al. Ruxolitinib rechallenge in resistant or intolerant patients with myelofibrosis: Frequency, therapeutic effects, and impact on outcome. Cancer (2021) 127(15):2657–65. doi: 10.1002/cncr.33541
25. Palandri F, Tiribelli M, Benevolo G, Tieghi A, Cavazzini F, Breccia M, et al. Efficacy and safety of ruxolitinib in intermediate-1 IPSS risk myelofibrosis patients: Results from an independent study. Hematol Oncol (2018) 36(1):285–90. doi: 10.1002/hon.2429
26. Altomare I, Parasuraman S, Paranagama D, Kish J, Lord K, Yu J, et al. Real-world dosing patterns of ruxolitinib in patients with polycythemia Vera who are resistant to or intolerant of hydroxyurea. Clin Lymphoma Myeloma Leuk (2021) 21(11):e915–e21. doi: 10.1016/j.clml.2021.06.023
27. Raman I, Pasricha SR, Prince HM, Yannakou CK. Management of hydroxyurea resistant or intolerant polycythemia vera. Leuk Lymphoma (2021) 62(10):2310–9. doi: 10.1080/10428194.2021.1901092
28. Elli EM, Barate C, Mendicino F, Palandri F, Palumbo GA. Mechanisms underlying the anti-inflammatory and immunosuppressive activity of ruxolitinib. Front Oncol (2019) 9:1186. doi: 10.3389/fonc.2019.01186
29. Guglielmelli P, Mazzoni A, Maggi L, Kiros ST, Zammarchi L, Pilerci S, et al. Impaired response to first SARS-CoV-2 dose vaccination in myeloproliferative neoplasm patients receiving ruxolitinib. Am J Hematol (2021) 96(11):E408–E10. doi: 10.1002/ajh.26305
30. Kozak KE, Ouyang L, Derkach A, Sherman A, McCall SJ, Famulare C, et al. Serum antibody response in patients with philadelphia-chromosome positive or negative myeloproliferative neoplasms following vaccination with SARS-CoV-2 spike protein messenger RNA (mRNA) vaccines. Leukemia (2021) 35(12):3578–80. doi: 10.1038/s41375-021-01457-1
31. Caocci G, Mulas O, Mantovani D, Costa A, Galizia A, Barabino L, et al. Ruxolitinib does not impair humoral immune response to COVID-19 vaccination with BNT162b2 mRNA COVID-19 vaccine in patients with myelofibrosis. Ann Hematol (2022) 101(4):929–31. doi: 10.1007/s00277-021-04613-w
32. Passamonti F, Cervantes F, Vannucchi AM, Morra E, Rumi E, Pereira A, et al. A dynamic prognostic model to predict survival in primary myelofibrosis: a study by the IWG-MRT (International working group for myeloproliferative neoplasms research and treatment). Blood (2010) 115(9):1703–8. doi: 10.1182/blood-2009-09-245837
33. Passamonti F, Giorgino T, Mora B, Guglielmelli P, Rumi E, Maffioli M, et al. A clinical-molecular prognostic model to predict survival in patients with post polycythemia vera and post essential thrombocythemia myelofibrosis. Leukemia (2017) 31(12):2726–31. doi: 10.1038/leu.2017.169
34. Barosi G, Birgegard G, Finazzi G, Griesshammer M, Harrison C, Hasselbalch H, et al. A unified definition of clinical resistance and intolerance to hydroxycarbamide in polycythaemia vera and primary myelofibrosis: results of a European LeukemiaNet (ELN) consensus process. Br J Haematol (2010) 148(6):961–3. doi: 10.1111/j.1365-2141.2009.08019.x
35. Morales-Nunez JJ, Munoz-Valle JF, Meza-Lopez C, Wang LF, Machado Sulbaran AC, Torres-Hernandez PC, et al. Neutralizing antibodies titers and side effects in response to BNT162b2 vaccine in healthcare workers with and without prior SARS-CoV-2 infection. Vaccines (Basel) (2021) 9(7):742-54 . doi: 10.3390/vaccines9070742
36. Ismail A, Shurrab FM, Al-Jighefee HT, Al-Sadeq DW, Qotba H, Al-Shaar IA, et al. Can commercial automated immunoassays be utilized to predict neutralizing antibodies after SARS-CoV-2 infection? a comparative study between three different assays. Front Biosci (Landmark Ed) (2021) 26(7):198–206. doi: 10.52586/4934
37. Poore B, Nerenz RD, Brodis D, Brown CI, Cervinski MA, Hubbard JA. A comparison of SARS-CoV-2 nucleocapsid and spike antibody detection using three commercially available automated immunoassays. Clin Biochem (2021) 95:77–80. doi: 10.1016/j.clinbiochem.2021.05.011
38. Plotkin SA. Vaccines: correlates of vaccine-induced immunity. Clin Infect Dis (2008) 47(3):401–9. doi: 10.1086/589862
39. Amanna IJ, Messaoudi I, Slifka MK. Protective immunity following vaccination: how is it defined? Hum Vaccin (2008) 4(4):316–9. doi: 10.4161/hv.4.4.5751
40. Khoury DS, Cromer D, Reynaldi A, Schlub TE, Wheatley AK, Juno JA, et al. Neutralizing antibody levels are highly predictive of immune protection from symptomatic SARS-CoV-2 infection. Nat Med (2021) 27(7):1205–11. doi: 10.1038/s41591-021-01377-8
41. Crawford KHD, Dingens AS, Eguia R, Wolf CR, Wilcox N, Logue JK, et al. Dynamics of neutralizing antibody titers in the months after severe acute respiratory syndrome coronavirus 2 infection. J Infect Dis (2021) 223(2):197–205. doi: 10.1093/infdis/jiaa618
42. Cohen KW, Linderman SL, Moodie Z, Czartoski J, Lai L, Mantus G, et al. Longitudinal analysis shows durable and broad immune memory after SARS-CoV-2 infection with persisting antibody responses and memory b and T cells. Cell Rep Med (2021) 2(7):100354. doi: 10.1016/j.xcrm.2021.100354
43. Rogers TF, Zhao F, Huang D, Beutler N, Burns A, He WT, et al. Isolation of potent SARS-CoV-2 neutralizing antibodies and protection from disease in a small animal model. Science (2020) 369(6506):956–63. doi: 10.1126/science.abc7520
44. Hassan AO, Case JB, Winkler ES, Thackray LB, Kafai NM, Bailey AL, et al. A SARS-CoV-2 infection model in mice demonstrates protection by neutralizing antibodies. Cell (2020) 182(3):744–53.e4. doi: 10.1016/j.cell.2020.06.011
45. Cuykx M, Mortele O, Jansens H, Schouwers S, Meskal A, Hoffbauer I, et al. Serological response in health care workers after a single dose of SARS-CoV-2 vaccine using six automated SARS-CoV-2 antibody assays. Diagn Microbiol Infect Dis (2021) 101(2):115486. doi: 10.1016/j.diagmicrobio.2021.115486
46. Passamonti F, Romano A, Salvini M, Merli F, Porta MGD, Bruna R, et al. COVID-19 elicits an impaired antibody response against SARS-CoV-2 in patients with haematological malignancies. Br J Haematol (2021) 195(3):371–7. doi: 10.1111/bjh.17704
47. Fiorino F, Ciabattini A, Sicuranza A, Pastore G, Santoni A, Simoncelli M, et al. The third dose of mRNA SARS-CoV-2 vaccines enhances the spike-specific antibody and memory b cell response in myelofibrosis patients. Front Immunol (2022) 13:1017863. doi: 10.3389/fimmu.2022.1017863
48. Walsh EE, Frenck RW Jr., Falsey AR, Kitchin N, Absalon J, Gurtman A, et al. Safety and immunogenicity of two RNA-based covid-19 vaccine candidates. N Engl J Med (2020) 383(25):2439–50. doi: 10.1056/NEJMoa2027906
49. Ibarrondo FJ, Hofmann C, Fulcher JA, Goodman-Meza D, Mu W, Hausner MA, et al. Primary, recall, and decay kinetics of SARS-CoV-2 vaccine antibody responses. ACS Nano (2021) 15(7):11180–91. doi: 10.1021/acsnano.1c03972
50. Chen J, Liu X, Zhang X, Lin Y, Liu D, Xun J, et al. Decline in neutralising antibody responses, but sustained T-cell immunity, in COVID-19 patients at 7 months post-infection. Clin Transl Immunol (2021) 10(7):e1319. doi: 10.1002/cti2.1319
51. Chia WN, Zhu F, Ong SWX, Young BE, Fong SW, Le Bert N, et al. Dynamics of SARS-CoV-2 neutralising antibody responses and duration of immunity: a longitudinal study. Lancet Microbe (2021) 2(6):e240–e9. doi: 10.1016/S2666-5247(21)00025-2
52. Carrillo J, Izquierdo-Useros N, Avila-Nieto C, Pradenas E, Clotet B, Blanco J. Humoral immune responses and neutralizing antibodies against SARS-CoV-2; implications in pathogenesis and protective immunity. Biochem Biophys Res Commun (2021) 538:187–91. doi: 10.1016/j.bbrc.2020.10.108
53. Hellerstein M. What are the roles of antibodies versus a durable, high quality T-cell response in protective immunity against SARS-CoV-2? Vaccine X (2020) 6:100076. doi: 10.1016/j.jvacx.2020.100076
54. Sahin U, Muik A, Vogler I, Derhovanessian E, Kranz LM, Vormehr M, et al. BNT162b2 vaccine induces neutralizing antibodies and poly-specific T cells in humans. Nature (2021) 595(7868):572–7. doi: 10.1038/s41586-021-03653-6
55. Bartsch YC, Fischinger S, Siddiqui SM, Chen Z, Yu J, Gebre M, et al. Discrete SARS-CoV-2 antibody titers track with functional humoral stability. Nat Commun (2021) 12(1):1018. doi: 10.1038/s41467-021-21336-8
56. Hill JA, Ujjani CS, Greninger AL, Shadman M, Gopal AK. Immunogenicity of a heterologous COVID-19 vaccine after failed vaccination in a lymphoma patient. Cancer Cell (2021) 39(8):1037–8. doi: 10.1016/j.ccell.2021.06.015
57. Del Bello A, Abravanel F, Marion O, Couat C, Esposito L, Lavayssiere L, et al. Efficiency of a boost with a third dose of anti-SARS-CoV-2 messenger RNA-based vaccines in solid organ transplant recipients. Am J Transplant (2022) 22(1):322–3. doi: 10.1111/ajt.16775
58. Kamar N, Abravanel F, Marion O, Couat C, Izopet J, Del Bello A. Three doses of an mRNA covid-19 vaccine in solid-organ transplant recipients. N Engl J Med (2021) 385(7):661–2. doi: 10.1056/NEJMc2108861
59. Werbel WA, Boyarsky BJ, Ou MT, Massie AB, Tobian AAR, Garonzik-Wang JM, et al. Safety and immunogenicity of a third dose of SARS-CoV-2 vaccine in solid organ transplant recipients: A case series. Ann Intern Med (2021) 174(9):1330–2. doi: 10.7326/L21-0282
60. Al-Obaidi MM, Gungor AB, Kurtin SE, Mathias AE, Tanriover B, Zangeneh TT. The prevention of COVID-19 in high-risk patients using tixagevimab-cilgavimab (Evusheld): Real-world experience at a Large academic center. Am J Med (2023) 136(1):96–9. doi: 10.1016/j.amjmed.2022.08.019
Keywords: Ruxolitinb, Myelofìbrosis, mRNA vaccine, BNT162.b2, immune response, Polycythemia Vera, COVID-19, SARS-CoV-2
Citation: Palumbo GA, Cambria D, La Spina E, Duminuco A, Laneri A, Longo A, Vetro C, Giallongo S, Romano A, Di Raimondo F, Tibullo D and Giallongo C (2023) Ruxolitinib treatment in myelofibrosis and polycythemia vera causes suboptimal humoral immune response following standard and booster vaccination with BNT162b2 mRNA COVID-19 vaccine. Front. Oncol. 13:1117815. doi: 10.3389/fonc.2023.1117815
Received: 06 December 2022; Accepted: 31 January 2023;
Published: 14 February 2023.
Edited by:
Adrián Mosquera Orgueira, University Hospital of Santiago de Compostela, SpainReviewed by:
Claudio Cerchione, Scientific Institute of Romagna for the Study and Treatment of Tumors (IRCCS), ItalyCopyright © 2023 Palumbo, Cambria, La Spina, Duminuco, Laneri, Longo, Vetro, Giallongo, Romano, Di Raimondo, Tibullo and Giallongo. This is an open-access article distributed under the terms of the Creative Commons Attribution License (CC BY). The use, distribution or reproduction in other forums is permitted, provided the original author(s) and the copyright owner(s) are credited and that the original publication in this journal is cited, in accordance with accepted academic practice. No use, distribution or reproduction is permitted which does not comply with these terms.
*Correspondence: Giuseppe A. Palumbo, cGFsdW1iby5nYUBnbWFpbC5jb20=; Daniele Tibullo, ZC50aWJ1bGxvQHVuaWN0Lml0
Disclaimer: All claims expressed in this article are solely those of the authors and do not necessarily represent those of their affiliated organizations, or those of the publisher, the editors and the reviewers. Any product that may be evaluated in this article or claim that may be made by its manufacturer is not guaranteed or endorsed by the publisher.
Research integrity at Frontiers
Learn more about the work of our research integrity team to safeguard the quality of each article we publish.