- 1Ella Lemelbaum Institute for Immuno-Oncology, Sheba Medical Center, Ramat Gan, Israel
- 2Department of Immunology, The Weizmann Institute of Science, Rehovot, Israel
- 3Department of Immunology and Regenerative Biology, The Weizmann Institute of Science, Rehovot, Israel
- 4Department of Clinical Microbiology and Immunology, Sackler School of Medicine, Tel Aviv University, Tel Aviv, Israel
- 5Davidoff Center, Rabin Medical Center, Petah Tikva, Israel
- 6Advanced Technology Unit, Felsenstein Medical Research Center, Petah Tikva, Israel
A major challenge in developing an effective adoptive cancer immunotherapy is the ex-vivo generation of tumor-reactive cells in sufficient numbers and with enhanced cytotoxic potential. It was recently demonstrated that culturing of activated murine CD8+ T-cells on a “Synthetic Immune Niche” (SIN), consisting of immobilized CCL21 and ICAM-1, enhances T-cell expansion, increases their cytotoxicity against cultured cancer cells and suppresses tumor growth in vivo. In the study reported here, we have tested the effect of the CCL21+ICAM1 SIN, on the expansion and cytotoxic phenotype of Tumor Infiltrating Lymphocytes (TIL) from melanoma patients, following activation with immobilized anti-CD3/CD28 stimulation, or commercial activation beads. The majority of TIL tested, displayed higher expansion when cultured on the coated SIN compared to cells incubated on uncoated substrate and a lower frequency of TIM-3+CD8+ cells after stimulation with anti-CD3/CD28 beads. Comparable enhancement of TIL proliferation was obtained by the CCL21+ICAM1 SIN, in a clinical setting that included a 14-day rapid expansion procedure (REP). Co-incubation of post-REP TIL with matching target cancerous cells demonstrated increased IFNγ secretion beyond baseline in most of the TIL cultures, as well as a significant increase in granzyme B levels following activation on SIN. The SIN did not significantly alter the relative frequency of CD8/CD4 populations, as well as the expression of CD28, CD25, several exhaustion markers and the differentiation status of the expanded cells. These results demonstrate the potential capacity of the CCL21+ICAM1 SIN to reinforce TIL-based immunotherapy for cancer patients.
1 Introduction
Adoptive cancer immunotherapy, employing ex vivo expanded genetically modified or natural autologous T-cells, is a highly promising approach used for the treatment of cancer patients (1–5). Adoptive therapy approaches in cancer patients are mainly based on the administration of either Chimeric Antigen Receptor (CAR)-expressing T-cells (6, 7) or Tumor Infiltrating Lymphocytes (TIL) (8–10).
CAR T-cells are polyclonal T-cells, isolated from the patient’s peripheral blood, that are genetically engineered to express a CAR with the specificity of a monoclonal antibody, directed against a known tumor-associated antigen (TAA), along with a co-stimulatory signaling capacity (11, 12).
Conversely, TIL-based therapy uses the natural infiltration of T-cells into tumors, as indication for their tumor antigen recognition capacity, and their immunotherapeutic potential. TIL, that originally display insufficient proliferative and cytotoxic functionality under the suppressive conditions within the tumor, are isolated from the resected tumor tissue, and cultured ex-vivo under supportive restimulating conditions. Briefly, the ex vivo production process of TIL consists of two major stages (13–15): During the first stage, referred to as pre-Rapid Expansion Procedure (pre-REP), TIL cultures are being established, by the resection of a tumor biopsy, outgrowth of the tumor resident lymphocytes and their expansion, induced by interleukin-2 (IL-2) added to the culture medium. In the next stage - REP, established TIL cultures are massively expanded to treatment levels by addition of soluble anti-CD3 antibody, IL-2, and irradiated feeder cells. By the end of the REP, TIL cultures are administered back to the lympho-depleted cancer patient.
While there are unique advantages to TIL therapy over other adoptive cellular therapies, including its broad TCR diversity, relative safety and effective tumor homing, there are still major challenges to consider for its wide application, including varying clinical response rates in treating different patients and different types of tumors, variations in the REP expansion yields, unpredicted expansion of T-cell clones with different therapeutic capacities (16) and the risk that prolonged TIL expansion may result in impaired functionality, such as T-cell exhaustion or anergy (17–19).
Towards reinforcing adoptive cancer immunotherapy, a ‘Synthetic Immune Niche’ (SIN) strategy was recently developed by Adutler-Lieber et.al., based on the stimulation of T-cells by surfaces, coated with the chemokine C-C motif Ligand 21 (CCL21) and the Intercellular Adhesion Molecule 1 (ICAM-1) (20, 21).
CCL21, secreted by lymphatic stroma and endothelial cells (22) induces several processes critical to immune responses, including co-localization and recruitment of T-cells and dendritic cells (DCs) (23, 24); facilitating cell migration (25, 26); priming T-cells for synapse formation (27); and co-stimulation of naïve T-cell expansion and Th1 polarization (28–30). ICAM1 plays a key role in the formation of immune synapses and promotion of T cell activation, through binding to its integrin ligand, lymphocyte function-associated 1 (LFA1) (31, 32).
These factors are expected to act synergistically, as CCL21 increases LFA1 responsiveness to ICAM1, and mediates the arrest of motile lymphocytes on ICAM1 expressing DCs and endothelial cells, and their clustering with other T-cells (22, 33). Indeed, the developed SIN, consisting of a combination of immobilized CCL21 and ICAM-1, was shown to increase the expansion of activated murine CD4+ (2) and CD8+ (1) T-cells. Furthermore, culturing of OVA-specific CD8+ T-cells on this SIN elevates the cellular levels of granzyme B expressed by these cells and increases their efficiency in killing ovalbumin-expressing cultured cancer cells, as well as their tumor suppressive activity, in vivo (1).
In the present study we apply, for the first time, the CCL21+ICAM1 based SIN technology, on human TIL, derived from melanoma patients. We show here that SIN-treatment of anti CD3/CD28 activated cells display higher expansion rates than cells incubated on uncoated substrate. Comparable enhancement of TIL proliferation was also obtained when the SIN treatment was included in the rapid expansion process. Furthermore, testing of selected, SIN-treated, post-REP TIL cultures demonstrated increased IFNγ secretion and granzyme B expression levels, suggesting that the CCL21+ICAM1 SIN can reinforce the TIL-based immunotherapeutic approach.
2 Material and methods
2.1 Generation and expansion of TIL cultures
TIL samples were obtained from patients with metastatic melanoma enrolled to an open-label phase II ACT trial at the Sheba Medical Center (NCT00287131).
Tumor tissues were subjected to multi-stage processing that included mechanical fragmentation, tissue remnant culture (TRC), and enzymatic digestion (Digest) as previously described (15–17). The tumor was sliced with a scalpel into small pieces, about 1–3 mm3 in size. Enzymatic digestion of the pieces, with 100mg/ml collagenase (Sigma-Aldrich, Israel) and 1mg/ml dornase alfa (Pulmozyme, Genentech, South San Francisco, CA), was performed within 2 to 4 h after surgery for 2 h at 37°C or overnight at room temperature to obtain a single-cell suspension. Small tumor fragments (1–2 mm3 in size) or 1 × 10 E6 live nucleated cells, obtained by Digest or TRC, were plated per well in 24-well plates in 2 ml complete medium (CM) containing 10% human AB serum (Valley Biomedical, Winchester, VA), 2mM L-glutamine (Biological Industries, Israel), Pen/Strep (Biological Industries, Israel) and 3,000 IU/ml IL-2 (Chiron Novartis, New Jersey, USA) in RPMI 1640 medium (Gibco, Thermo Fisher Scientific, Waltham, MA). During the first week, non-adherent TIL cultures were transferred to a new 24-well plate and cultured separately from the adherent melanoma cells. Cells were split or fresh medium was added every 2 to 3 days, to maintain a cell concentration in the range of 0.5–2.0 × 106 cells/ml. TIL cultures were established within 2 to 4 weeks.
2.2 Surface coating and functionalization with CCL21 and ICAM-1
Substrate functionalization was performed by overnight incubation with 5 µg/ml CCL21 and 50 µg/ml ICAM1 (R&D Systems, Minneapolis, MN, USA), in PBS.
2.3 Stimulation of TIL with anti CD3/CD28 antibodies
TIL cultures were stimulated for 7 days with anti-biotin MACSiBead Particles loaded with biotinylated CD3/CD28 antibodies (Miltenyi Biotec, Bergisch Gladbach, Germany). The preparation of the conjugated beads was carried out according to the manufacturer’s recommendation (activation/expansion kit, Miltenyi Biotec). Antibody-loaded anti-biotin MACSiBead™ were added to the TIL at a cell/bead ratio of 1:1. For plate-bound expansion, 24-well plates were coated with 0.3 ml PBS per well containing 2 μg/ml anti-CD3 (clone OKT-3; Miltenyi Biotech), 2 μg/ml anti-CD28 (clone: 15E8, Miltenyi Biotech) with or without addition of 5 µg/ml CCL21+ 50 µg/ml ICAM1 and incubated overnight at 4°C. The following day, plates were washed with PBS, 0.01 x106 TIL in 2 ml CM medium with 3,000 IU/ml IL-2 were added per well for stimulation.
2.4 Rapid expansion procedure (REP) with CCL21 and ICAM-1 surface coating
The REP was initiated by stimulating 0.01-0.03 x106 TIL with 30 ng/ml anti-CD3 antibodies (MACS GMP CD3 pure, clone OKT-3; Miltenyi Biotech, Germany), 3,000 IU/ml IL-2, and irradiated peripheral blood mononuclear cells from three non-related donors as feeder cells (50 Gy, TIL to feeder cells ratio = 1:100) in 50% CM, 50% AIM-V medium (Invitrogen, Thermo Fisher Scientific, Waltham, MA). REP was performed in 24-well plates. TIL seeding at days 0, 7, 11 started from range of 2-8 wells. CCL21+ICAM1 coating was prepared one day prior to the initiation or cell splitting (days -1, 6 and 10). TILs were cultured for 14 days and split on day 7 and 11 to maintain a cell concentration of 0.3-2.0x106/well. Fold expansion was calculated by dividing the total number of cells at each time point by the number of cells at day 0.
2.5 Antibodies and flow cytometry
The following antibodies were used, in this study, for flow cytometry: Pacific blue-CD3 (clone SK7, BioLegend, San Diego, CA, United States), PECy7 or FITC-CD8 (clone HIT8a, BioLegend), FITC-PD-1 (clone EH12.2H7, BioLegend), FITC-TIM-3 (clone F38-2E2, BioLegend), FITC-LAG-3 (clone 11C3C65, BioLegend) APC-CD25 (clone BC96, BioLegend), APC-CD28 (clone CD28.2, BioLegend), APC-vio770-CD45RA (clone HI100, BioLegend) and PerCP-CCR7 (clone G043H7, BioLegend). TIL cultures were washed and re-suspended in cell-staining buffer (BioLegend). Cells were incubated for 30 min with the antibodies on ice, washed in buffer and measured using MACSQuant flow cytometer (Miltenyi Biotech).
For localization and quantification of granzyme B, cells were fixed (BLG420801, Biolegend), permeabilized (BLG421002, Biolegend), and stained with granzyme B-specific antibodies (BLG515406, BioLegend). For carboxy fluorescein succinimidyl ester (CFSE) cell proliferation assays, TIL cultures were stained before seeding at day 11 of the REP with 5 μM CFSE (Thermo Fisher Scientific) for 20 min at 37°C, according to the manufacturer’s instructions. Four days later cells were taken for flow cytometry analysis and their CFSE fluorescence intensity was determined. Samples were analyzed using FlowJo software (FlowJo LLC, Ashland, OR).
2.6 IFNγ ELISA
1x105 TIL were co-cultured with autologous melanoma autologous melanoma cells in 96-well plates overnight at 1:1 effector-to-target ratio. Supernatant was collected and IFNγ levels were determined by ELISA (BioLegend) according to the manufacturer’s protocol.
2.7 Cell-mediated cytotoxicity assay
1x105 TIL were co-cultured with autologous melanoma cells in 96-well plates overnight at a 1:1 effector-to-target ratio. Levels of lactate dehydrogenase (LDH) in the medium were determined by CytoTox 96 Non-Radioactive Cytotoxicity Assay (Promega, Madison, WI, Cat. no. G1780), carried out according to the manufacturer’s instructions. The luminescence of the samples was measured at 490 nm in a plate-reading luminometer (GloMax microplate luminescence reader, Promega Company, USA). Experiments were performed in triplicates.
2.8 Statistics
Significance of variation between groups was evaluated using a two-tailed Student’s t-test. Test for differences between proportions was performed using two-sided Fisher’s exact test with p ≤ 0.05 values considered as significant. (*p <.05, **p <.01, ***p <.001, ****p < .0001).
3 Results
3.1 Impact of CCL21+ICAM1 coated surface on TIL proliferation, following CD3/CD28 stimulation
To evaluate the effect of a CCL21+ICAM1 coated surface on the proliferative capacity of TIL cultures, the cells were seeded in 24-well plates, coated with CCL21+ICAM1 (“coated-TIL”) or a non-coated plates (“uncoated-TIL”) and activated by CD3/CD28 beads stimulation. Six TIL cultures were tested. TIL cultures were derived from five patients with metastatic melanoma (Pt. 014, 124, 132, 145 and 151) and two, independently established TIL cultures from the same patient (Pt. 014/F3 and 014/F4). Seven days following the initial stimulation, five out of the six TIL cultures showed higher fold expansion values following exposure to CCL21+ICAM1 (Figure 1A). In these five cultures, the expansion of coated-TIL was, on average, 2.5 ± 1.2-fold higher (range 1.6-4.6, p=.026) after 7 days, achieving an 8.4 ± 11.3-fold expansion for coated-TIL compared with 4.5 ± 7.3-fold for TIL cultured on uncoated surfaces (Figure 1B, normalized for uncoated TIL).
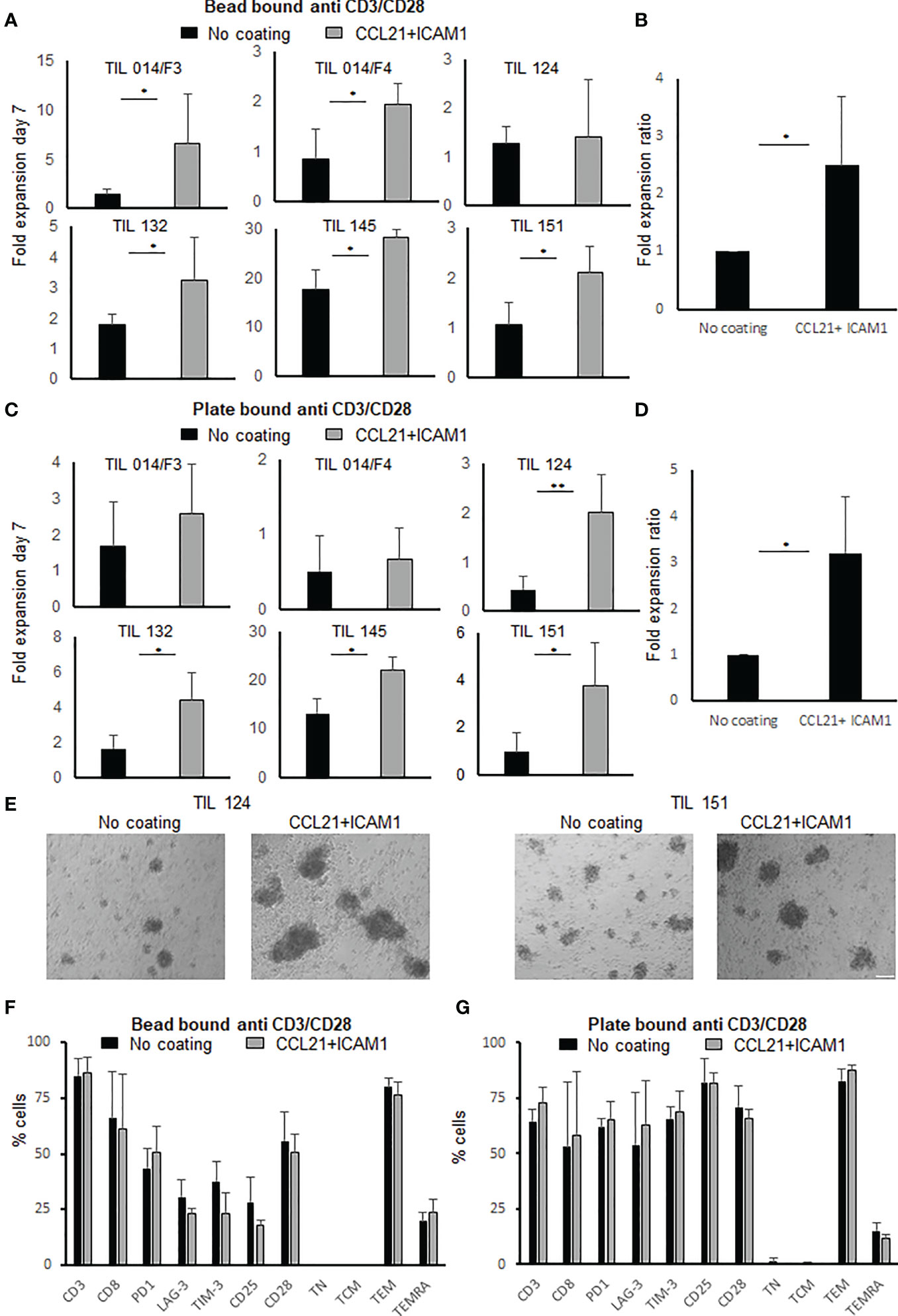
Figure 1 Fold expansion and phenotype analysis following anti-CD3/CD28 stimulation of TIL with or without exposure to SIN, consisting of CCL21+ICAM1 coated surface. (A) Fold expansion of TIL cultures (n=6) stimulated by anti-CD3/CD28 activation beads. Graph bars show comparison between TIL cultured on uncoated vs. CCL21+ICAM1 coated surfaces (B) Average fold expansion ratio between coated to uncoated TIL cultures (No coating=1), stimulated with activation beads (n=5). (C) Fold expansion of TIL cultures (n=6) stimulated by plate-bound anti-CD3/CD28 antibodies for 7 days. Graph bars show comparison between TIL cultured on uncoated vs. CCL21+ICAM1 coated surfaces. (D) Average fold expansion ratio between coated to uncoated TIL cultures (No coating=1), stimulated with plate-bound CD3/CD28 antibodies (n=4). (E) Morphology following 7-day plate-bound CD3/CD28 activation (scale bar = 100 µm). (F, G) Percentage of CD3, CD8, Distribution of surface markers: co-inhibitory: PD1, LAG-3, TIM-3, activation marker: CD25, co-stimulatory CD28 and TIL differentiation status: TN (naïve), CD3+CD45RA+CCR7+; TCM (central memory), CD3+CD45RA−CCR7+; TEM (effector memory), CD3+CD45RA−CCR7−; TEMRA (effector), CD3+CD45RA+CCR7− (n=4). (*p < .05, **p < .01).
In addition, when stimulating TIL cultures with plate-bound anti-CD3 and anti-CD28 antibodies (instead of activation beads), four out of six TIL cultures exposed to CCL21+ICAM1 demonstrated higher fold expansion values (uncoated-TIL, 4.0 ± 6.1-fold; coated-TIL, 8.1 ± 9.4-fold, range 1.69-4.49) (Figure 1C). In these four cultures expansion of coated-TIL was on average 3.2 ± 1.2-fold higher proliferation (p = .012) over TIL cultured on uncoated dishes (Figure 1D, normalized for uncoated TIL). Moreover, microscopy-based monitoring demonstrated that SIN-stimulated TIL cultures tended to form larger cell aggregates than those incubated on non-coated plates (Figure 1E). By the end of the expansion, 77 ± 13% of the cells were CD3+ T cells. Independent of the anti-CD3/CD28 stimulation method (beads or immobilized), CCL21+ICAM1 exposure had no apparent impact on the CD4/CD8 subpopulation distribution, expression of the co-inhibitory molecules PD-1, LAG-3 and TIM-3, the co-stimulatory molecule CD28, the activation marker CD25 or the differentiation status of TIL (p values ≥.05) (Figures 1F, G). Moreover, there was no significant difference in the expression of sub-population markers within the CD4 or CD8 populations (p- values ≥.05) except for the inhibitory molecule TIM-3 and the activation marker CD25, which were significantly lower within the CD8+ T cells population following seven days of CD3/CD28 beads stimulation (TIM-3+CD8+, uncoated-TIL 31.2 ± 8.4%, coated-TIL 15.1 ± 7.4%, p=.029; CD25+CD8+ uncoated-TIL 12.1 ± 4.2%, coated-TIL 5.4 ± 2.8%, p=.036) (Supplementary Tables 1, 2). The gating strategy and representative flow cytometric plots are shown in Suppl. Figure 1.
3.2 CCL21+ICAM1 coated surfaces increased TIL proliferation during the rapid expansion procedure (REP)
In the clinical setting, TIL cultures are expanded rapidly to large cell numbers by adding 40-50 Gy irradiated PBMC feeder cells, soluble anti-CD3 antibody and IL-2 to the cell culture. On day 14 of the ex vivo expansion, TIL cultures are harvested and prepared for the i.v. infusion. To test whether CCL21+ICAM1 coated surfaces improve the manufacturing process, small-scale REPs were initiated, closely resembling the clinical setting, using GMP-compliant reagents. Six TIL cultures obtained from five melanoma patients (Pt. 014, 052, 219, 031 and 174) were expanded according to the REP protocol for 14 days. As shown in Figure 2A, expansion was significantly increased on CCL21+ICAM1 coated surfaces by 2.3 ± 0.7-fold (p=.002) in 5 out of the 6 TIL cultures tested.
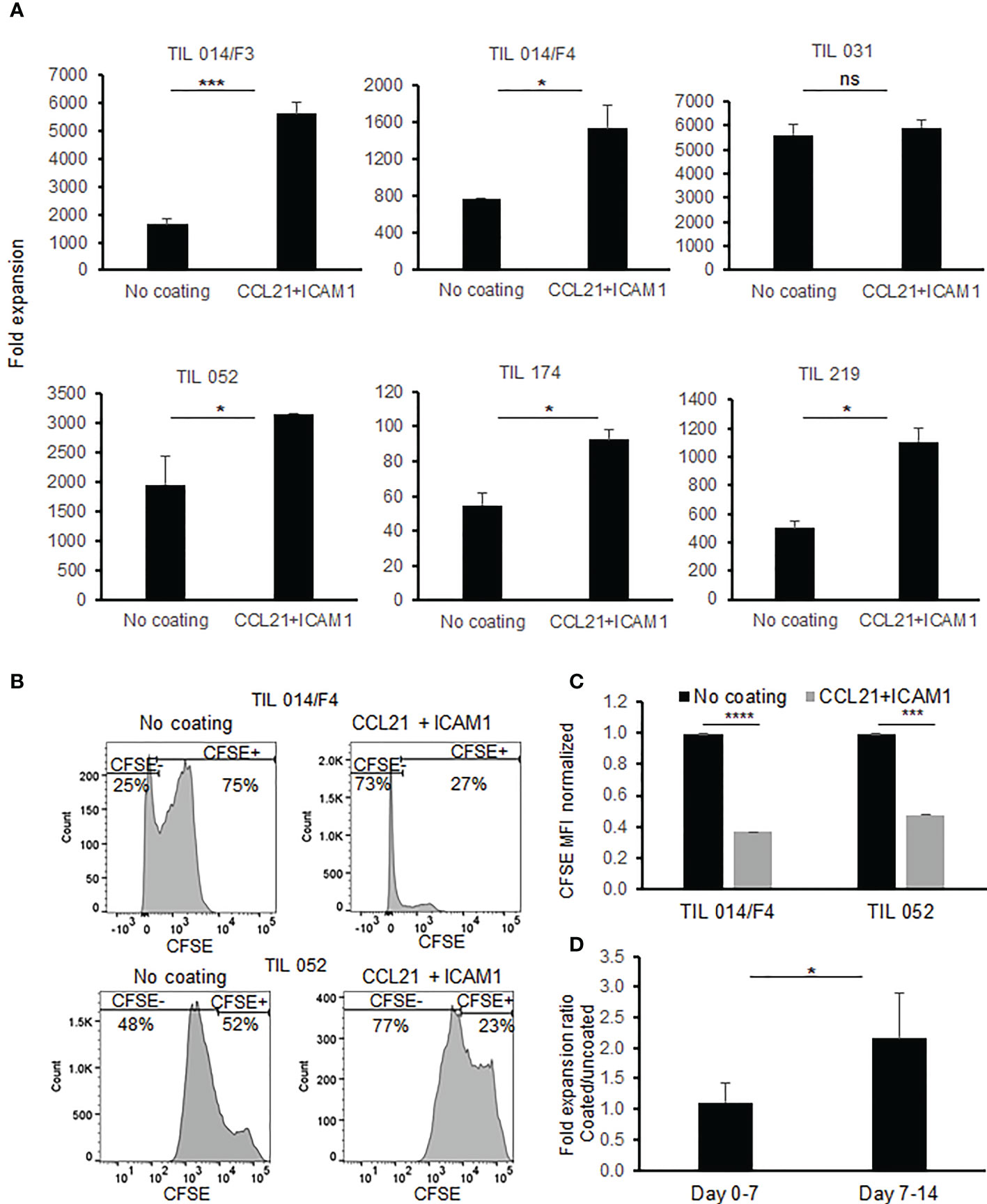
Figure 2 Comparison of TIL during REP with/without exposure to SIN, consisting of CCL21+ICAM1 coated surface. (A) Fold expansion on day 14 (n=6). All data are representatives of two experiments. The graphs show the mean ± SD values. (B) Histograms of CFSE staining (C) Bar graph shows mean fluorescence intensity of CFSE. TIL cultured on CCL21+ICAM1 coated surfaces normalized to the uncoated TIL (no coating= 1). (D) The average frequencies of fold expansions at day 0-7 and day 7-14, with or without incubation on CCL21+ICAM1 coated surface (n = 5). (*p < .05, ***p < .001, ****p < .0001, ns, not significant).
To confirm that CCL21+ICAM1 coating contributes to the enhanced expansion by increasing the proliferation rate, TIL cultures were stained with carboxyfluorescein succinimidyl ester (CFSE) dye on day 11 of the REP and analyzed on day 14. As shown in Figures 2B, C, a decrease in CFSE fluorescence intensity was observed in the SIN-stimulated TIL cultures indicating that the increased expansion is indeed attributable to elevated cell proliferation.
It was further shown that the major contribution of the CCL21+ICAM1 coated surface to the increased proliferation occurred during the second week of the REP (fold increase day 0-7 = 1.11 ± 0.32; fold increase day 7-14 = 2.15 ± 0.74; p = .020) (Figure 2D). The impact between day 7 to 11 and day 11 to 14 day was similar (fold increase day 7-11 = 1.61 ± 0.59; fold increase day 11-14: 1.39 ± 0.37; p=.511).
3.3 Increased expansion of TIL on CCL21+ICAM1 coated surface had no major impact on TIL phenotype
Analysis of pre-REP TIL cultures, derived from five patients, showed that 94.2 ± 7.3% of the pre-REP cells were CD3+ T cells.
To investigate the impact of CCL21+ICAM1 coated surfaces on the TIL phenotype, post-REP TIL cultures (n=6) were analyzed for CD4 and CD8 expression. CCL21+ICAM1 coating had no impact on the average frequency of CD4+ and CD8+ TIL (% CD4, coated-TIL = 45.1 ± 34.3%, uncoated-TIL 43.0% ± 33.0%, p = .917; %CD8 coated-TIL = 54.9 ± 34.8%, uncoated TIL = 57.0 ± 33.0%, p = .917) (Figure 3A).
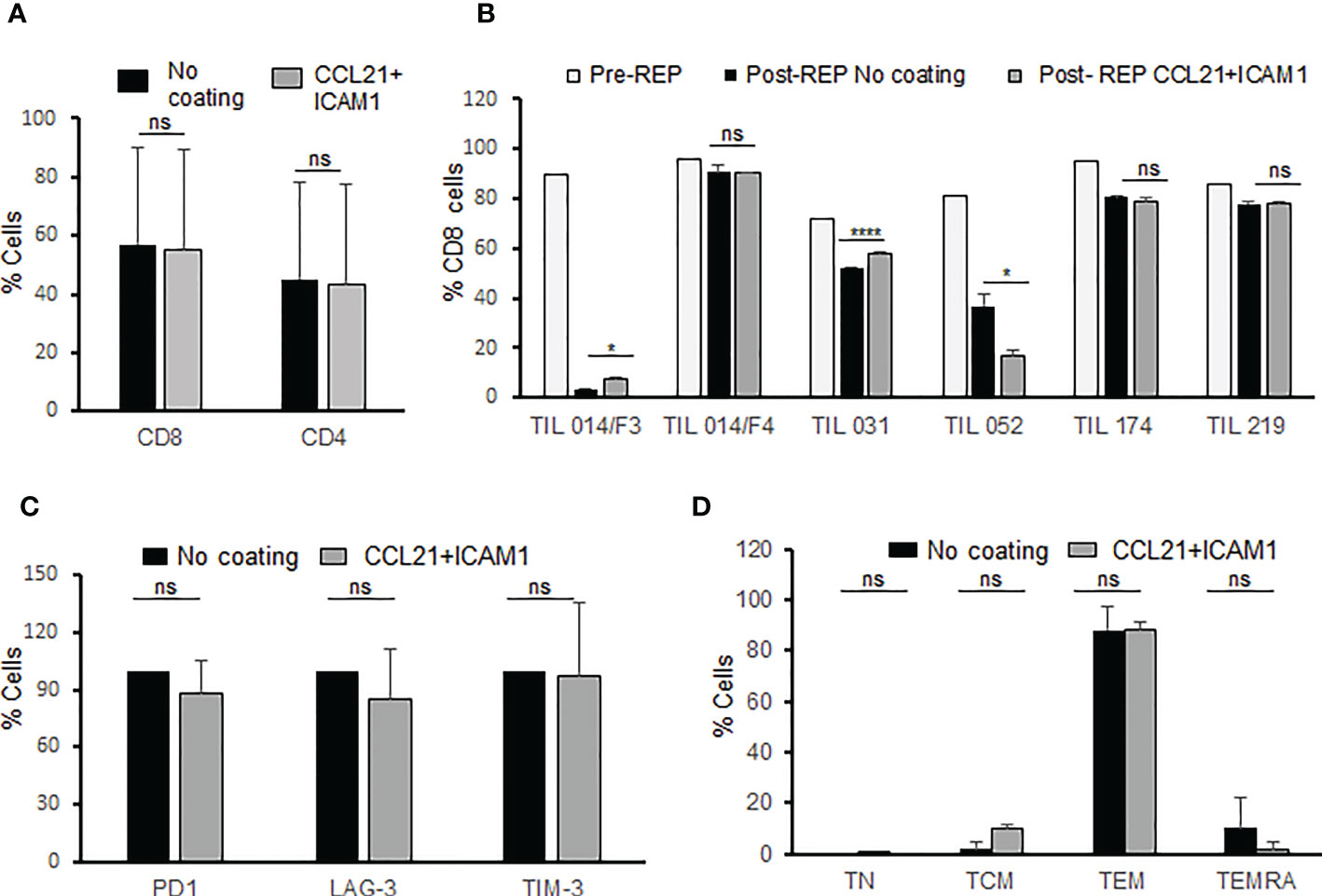
Figure 3 Phenotype analysis of post-REP TIL. (A) Frequency of CD8 and CD4 T cells for CCL21+ICAM1 coated and uncoated post-REP TIL cultures (n=6). (B) Frequency of CD8 T cells pre-REP and CCL21+ICAM1 coated and uncoated post-REP TIL cultures (n=6). (C) TIL cultures were characterized for expression of inhibitory molecules PD-1, LAG-3, TIM-3 (n = 4). The expression values of coated surface TIL were normalized to uncoated surface TIL (100%) (D) Distribution of differentiation subsets. TN (naive), CD3+CD45RA+CCR7+; TCM (central memory), CD3+CD45RA−CCR7+; TEM (effector memory), CD3+CD45RA−CCR7−; TEMRA (effector), CD3+CD45RA+CCR7−; (n=3). (*p < .05, ****p < .0001, ns, not significant).
As shown in Figure 3B (pre-REP versus post-REP), the rapid expansion procedure itself had a major impact on the percentage of CD8+ (and CD4+), however the effect of CCL21+ICAM1 coating was minor (Figure 3B) and on average insignificant (Figure 3A).
Four post-REP TIL cultures were further characterized for the expression of the inhibitory molecules PD-1, LAG-3, and TIM-3 on all CD3 T cells and on the CD4/CD8 T cell subpopulations. As shown in Table 1, expression of the inhibitory molecules was insignificantly different between coated and non-coated post-REP TIL cultures (p-values ≥.210) (Figure 3C).
The differentiation status of three post-REP TIL cultures was defined based on the expression of CD45RA, in combination with CCR7. The majority of post-REP TIL, independently of whether they were cultured on a CCL21+ICAM1 surface or not, were CD45RA-CCR7-, effector memory T cells (TEM) (coated surfaces, TEM = 88.1 ± 3.4%; uncoated, TEM = 86.7 ± 9.6%; p = .830) (Figure 3D).
3.4 CCL21+ICAM1 coating increased the anti-tumor reactivity of TIL
To determine whether the CCL21+ICAM1 coated surfaces enhance anti-tumor reactivity, IFNγ secretion and granzyme B expression were determined after a co-culture of post-REP TIL cultures with or without autologous tumor target cells.
Interestingly, the basal IFNγ level of TIL (without target cells) was significantly lower in cells exposed to coated surfaces compared with uncoated TIL (p = .0.001, Figure 4A). Three of five post-REP TIL cultures demonstrated significantly increased IFNγ secretion beyond baseline following exposure to the CCL21+ICAM1 coated surface (Figure 4B). Absolute IFNγ concentrations are shown in Supplementary Table 3.
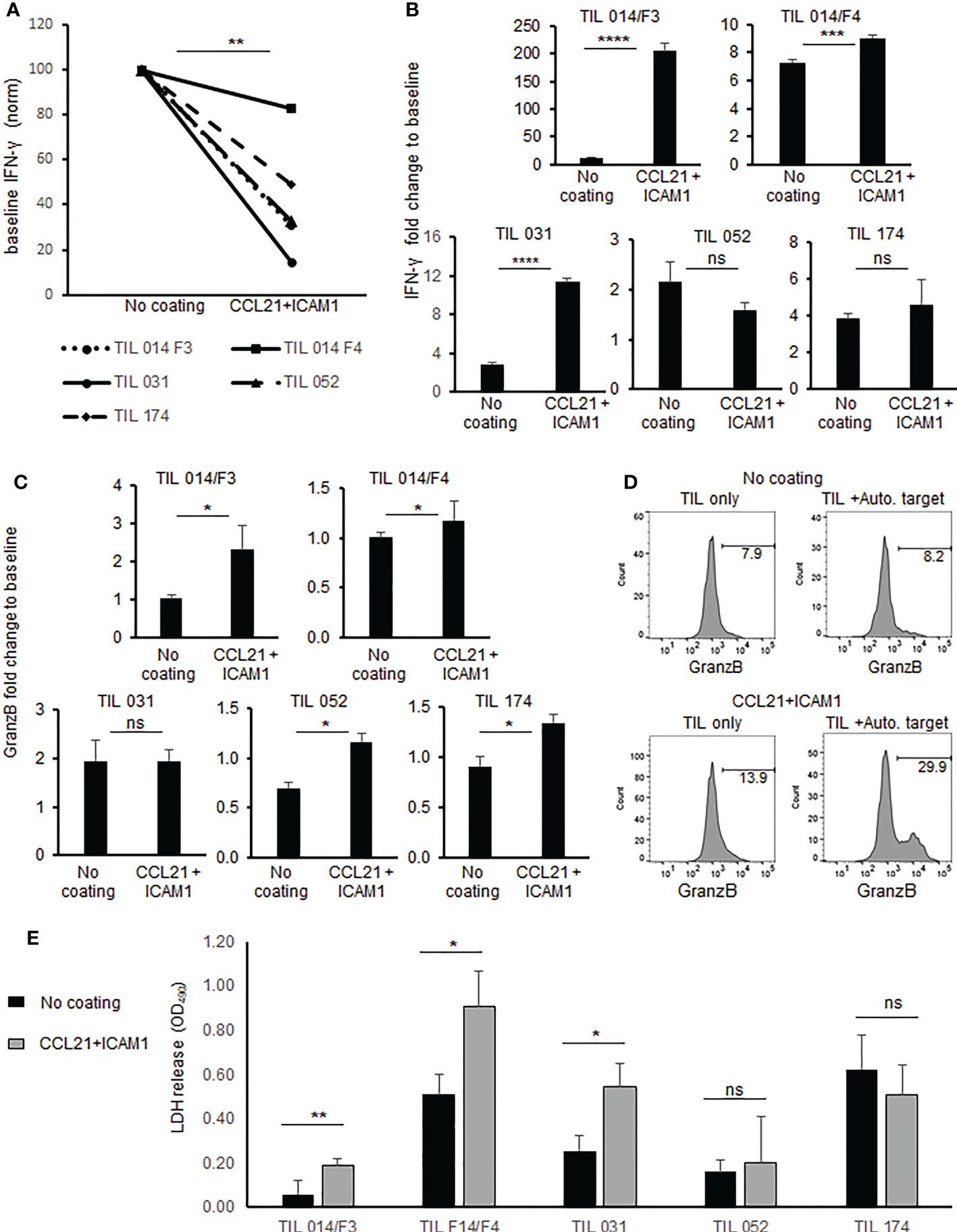
Figure 4 Anti-tumor reactivity and cytotoxicity of TIL cultured on SIN, consisting of CCL21+ICAM1 coated surfaces. (A) Connecting line histogram demonstrated different baseline (TIL only) of IFNγ secretion from CCL21+ICAM1 coated TIL or uncoated surfaces. (B) Fold change of IFNγ following co-cultures of TIL with autologous tumor versus TIL only. In each experiment, IFNγ concentration after co-culture was divided by the “TIL only” (= baseline) concentration and expressed as fold change. The bar plots show the mean fold change ± standard deviation (SD) of three independent experiments. (C) Fold change of MFI for granzyme B in CD8+ cells co-cultured with tumor cells. MFI values after co-culture were divided by the “TIL only” (= baseline) values and are presented as the mean ± standard deviation (SD) obtained in three independent experiments. (D) Representative FACS Histogram plots for granzyme (B, E). Cytotoxicity measured by LDH assay. Experiments were performed in triplicates wells. Results expressed as mean ± standard deviation (SD). (*p < .05, **p < .01, ***p < .001, ****p < .0001, ns, not significant).
Granzyme B expression was significantly increased (p ≤.05) in four out of five post REP TIL cultures which were expanded on coated surfaces (Figure 4C). Representative flow cytometric histograms are shown in Figure 4D. Direct cytotoxicity assay against autologous melanoma cells using LDH assay revealed in three of five post-REP TIL cultures significantly higher killing activity by TIL cultured on CCL21+ICAM1 coated surfaces, compared with uncoated TIL (Figure 4E and Supp. Figure 2).
4 Discussion
The primary motivation underlying the development of an ex vivo “synthetic immune niche”, that might improve the performance of therapeutic T-cells, is based on current experiments with diverse adoptive immunotherapies, whose effectiveness varies greatly between patients and tumor types (34). In our earlier studies we have shown that stimulation of murine CD4+ (1) or CD8+ T-cells (2) by a synthetic immune niche, consisting of immobilized ICAM-1 and CCL21, enhances the proliferation of both cell types, and increases the cytotoxic efficacy of CD8+ cells, both ex vivo and in vivo. These encouraging findings indicated that specific environmental stimulation can alter T-cell proliferation and activity, yet the experimental settings of these studies (35) (murine OT-1 and OT-2 systems), were very different from current clinical settings in humans. The primary goal of the current study was to determine the capacity of human CCL21+ICAM1 SIN to exert similar effects in a clinically relevant system.
The system we chose, namely TIL therapy, has earned great success in treating solid tumors (36), particularly metastatic melanoma (37–41), and does not require a prediction of the actual TAA, or the characteristics of its binding receptor. TIL cultures are naturally heterogeneous, potentially targeting a variety of tumor antigens, a feature that is particularly relevant to melanoma, which displays a high mutational load (42).
Moreover, the indication that the CCL21+ICAM1 SIN enhances expansion of T-cells, render the SIN approach highly suitable for TIL therapy, in which high numbers of cells are a favorable prognostic indicators for successful therapy in different tumor types (43–45) and specifically in foretelling the success of checkpoint therapies (46). The additional feature of this SIN, based on the results obtained using the murine system, namely, potentiation of the cytotoxic machinery by increasing granzyme B levels (1), is also highly relevant to TIL therapy, in which failed therapies are often attributed to exhaustion and anergy (21, 47).
The results presented herein strongly confirm that using the human SIN components CCL21+ICAM during anti-CD3/CD28 antibody stimulation significantly enhances patient-derived TIL expansion. In addition, CCL21+ICAM coated-TIL stimulated with anti-CD3/CD28 beads, demonstrated a significantly lower expression the co-inhibitory molecule TIM-3 and the activation marker CD25 on CD8+ T cells.
Also, when tested in a clinical setting that includes a 14-day rapid expansion procedure in the presence of irradiated feeder cells, anti-CD3 and IL-2, melanoma-derived TIL. Although the fold expansion values largely varied between different TIL cultures (1758 ± 2016-fold), in 5/6 TIL exposure to CCL21+ICAM SIN significantly increased the expansion rate (2900 ± 2425-fold).
Importantly, the increased expansion did not impact the expression of inhibitory molecules such as TIM-3, LAG-3 and PD-1 or the differentiation status of TIL. Moreover, post-REP TIL demonstrated increased IFNγ secretion beyond baseline in three of five cultures and a significant increase in granzyme B levels in four of five tested TIL cultures. Direct killing assay demonstrated enhanced killing of target cells following exposure of TIL to CCL21+ICAM1 surface
Further studies would be required to determine whether overexpression of CCL21+ICAM1 in the irradiated feeder cells could exert positive impact on TIL expansion during REP comparable to that of the substrate-immobilized SIN.
Given the results presented here, we propose that the CCL21+ICAM1 synthetic niche, which proved to enhance expansion in the murine OVA systems, and elevate the cytotoxic capacity of murine CD8 cells, is capable to reinforce human TIL, derived from melanoma patients. These results presented here strongly suggest that incorporation of SIN stimulation into the TIL expansion process might significantly improve the therapeutic performance of these cells.
Data availability statement
The raw data supporting the conclusions of this article will be made available by the authors, without undue reservation.
Author contributions
BG and MB contributed conception and design of the study. SY and SA-L performed experiments and acquired the data. SY wrote the first draft of the manuscript. BG, NF, and MB revised it critically for important intellectual content. All authors contributed to manuscript revision, read and approved the submitted version.
Acknowledgments
This study was supported by grants from the Israel Science Foundation (program: Israel Precision Medicine Partnership (IPMP) and the Volkswagen Foundation to BG and by the generous support of the Lemelbaum family to MB and SY. We would like to thank Dr. Erez Greenstein, The Weizmann Institute for his advice on the analysis of the experimental data.
Conflict of interest
The authors declare that the research was conducted in the absence of any commercial or financial relationships that could be construed as a potential conflict of interest.
Publisher’s note
All claims expressed in this article are solely those of the authors and do not necessarily represent those of their affiliated organizations, or those of the publisher, the editors and the reviewers. Any product that may be evaluated in this article, or claim that may be made by its manufacturer, is not guaranteed or endorsed by the publisher.
Supplementary material
The Supplementary Material for this article can be found online at: https://www.frontiersin.org/articles/10.3389/fonc.2023.1116328/full#supplementary-material
References
1. Rohaan MW, Wilgenhof S, Haanen J. Adoptive cellular therapies: the current landscape. Virchows Arch (2019) 474(4):449–61. doi: 10.1007/s00428-018-2484-0
2. Bear AS, Fraietta JA, Narayan VK, O'Hara M, Haas NB, et al. Adoptive cellular therapy for solid tumors. Am Soc Clin Oncol Educ Book (2021) 41:57–65. doi: 10.1200/EDBK_321115
3. Restifo NP, Dudley ME, Rosenberg SA. Adoptive immunotherapy for cancer: harnessing the T cell response. Nat Rev Immunol (2012) 12(4):269–81. doi: 10.1038/nri3191
4. Rosenberg SA, Restifo NP. Adoptive cell transfer as personalized immunotherapy for human cancer. Science (2015) 348(6230):62–8. doi: 10.1126/science.aaa4967
5. Waldman AD, Fritz JM, Lenardo MJ. A guide to cancer immunotherapy: from T cell basic science to clinical practice. Nat Rev Immunol (2020) 20(11):651–68. doi: 10.1038/s41577-020-0306-5
6. Ma S, Li X, Wang X, Cheng L, Li Z, Zhang C, et al. Current progress in CAR-T cell therapy for solid tumors. Int J Biol Sci (2019) 15(12):2548–60. doi: 10.7150/ijbs.34213
7. Wang Z, Wu Z, Liu Y, Han W. New development in CAR-T cell therapy. J Hematol Oncol (2017) 10(1):53. doi: 10.1186/s13045-017-0423-1
8. Lin B, Du L, Li H, Zhu X, Cui L, Li X, et al. Tumor-infiltrating lymphocytes: Warriors fight against tumors powerfully. BioMed Pharmacother (2020) 132:110873. doi: 10.1016/j.biopha.2020.110873
9. Badalamenti G, Fanale D, Incorvaia L, Barraco N, Listi A, Maragliano R, et al. Role of tumor-infiltrating lymphocytes in patients with solid tumors: Can a drop dig a stone? Cell Immunol (2019) 343:103753. doi: 10.1016/j.cellimm.2018.01.013
10. Paijens ST, Vledder A, de Bruyn M, Nijman HW. Tumor-infiltrating lymphocytes in the immunotherapy era. Cell Mol Immunol (2021) 18(4):842–59. doi: 10.1038/s41423-020-00565-9
11. Almasbak H, Aarvak T, Vemuri MC. CAR T cell therapy: A game changer in cancer treatment. J Immunol Res (2016) 2016:5474602. doi: 10.1155/2016/5474602
12. Cartellieri M, Bachmann M, Feldmann A, Bippes C, Stamova S, Wehner R, et al. Chimeric antigen receptor-engineered T cells for immunotherapy of cancer. J BioMed Biotechnol (2010) 2010:956304. doi: 10.1155/2010/956304
13. Besser MJ, Shapira-Frommer R, Itzhaki O, Treves AJ, Zippel DB, Levy D, et al. Adoptive transfer of tumor-infiltrating lymphocytes in patients with metastatic melanoma: Intent-to-treat analysis and efficacy after failure to prior immunotherapies. Clin Cancer Res (2013) 19(17):4792–800. doi: 10.1158/1078-0432.CCR-13-0380
14. Itzhaki O, Hovav E, Ziporen Y, Levy D, Kubi A, Zikich D, et al. Establishment and large-scale expansion of minimally cultured "young" tumor infiltrating lymphocytes for adoptive transfer therapy. J Immunother (2011) 34(2):212–20. doi: 10.1097/CJI.0b013e318209c94c
15. Besser MJ, Shapira-Frommer R, Treves AJ, Zippel D, Itzhaki O, Hershkovitz L, et al. Clinical responses in a phase II study using adoptive transfer of short-term cultured tumor infiltration lymphocytes in metastatic melanoma patients. Clin Cancer Res (2010) 16(9):2646–55. doi: 10.1158/1078-0432.CCR-10-0041
16. Schmidt A, Oberle N, Krammer PH. Molecular mechanisms of treg-mediated T cell suppression. Front Immunol (2012) 3:51. doi: 10.3389/fimmu.2012.00051
18. Schwartz RH. T Cell anergy. Annu Rev Immunol (2003) 21:305–34. doi: 10.1146/annurev.immunol.21.120601.141110
19. Wang S, Sun J, Chen K, Ma P, Lei Q, Xing S, et al. Perspectives of tumor-infiltrating lymphocyte treatment in solid tumors. BMC Med (2021) 19(1):140. doi: 10.1186/s12916-021-02006-4
20. Adutler-Lieber S, Friedman N, Geiger B. Expansion and antitumor cytotoxicity of T-cells are augmented by substrate-bound CCL21 and intercellular adhesion molecule 1. Front Immunol (2018) 9:1303. doi: 10.3389/fimmu.2018.01303
21. Adutler-Lieber S, Zaretsky I, Sabany H, Kartvelishvily E, Golani O, Geiger B, Friedman N, et al. Substrate-bound CCL21 and ICAM1 combined with soluble IL-6 collectively augment the expansion of antigen-specific murine CD4(+) T cells. Blood Adv (2017) 1(15):1016–30. doi: 10.1182/bloodadvances.2016001545
22. Stein JV, Rot A, Luo Y, Narasimhaswamy M, Nakano H, Gunn MD, et al. The CC chemokine thymus-derived chemotactic agent 4 (TCA-4, secondary lymphoid tissue chemokine, 6Ckine, exodus-2) triggers lymphocyte function-associated antigen 1-mediated arrest of rolling T lymphocytes in peripheral lymph node high endothelial venules. J Exp Med (2000) 191(1):61–76. doi: 10.1084/jem.191.1.61
23. Gretz JE, Kaldjian EP, Anderson AO, Shaw S. Sophisticated strategies for information encounter in the lymph node: the reticular network as a conduit of soluble information and a highway for cell traffic. J Immunol (1996) 157(2):495–9. doi: 10.4049/jimmunol.157.2.495
24. Kaldjian EP, Gretz JE, Anderson AO, Shi Y, Shaw S. Spatial and molecular organization of lymph node T cell cortex: a labyrinthine cavity bounded by an epithelium-like monolayer of fibroblastic reticular cells anchored to basement membrane-like extracellular matrix. Int Immunol (2001) 13(10):1243–53. doi: 10.1093/intimm/13.10.1243
25. Gonen-Wadmany M, Oss-Ronen L, Seliktar D. Protein-polymer conjugates for forming photopolymerizable biomimetic hydrogels for tissue engineering. Biomaterials (2007) 28(26):3876–86. doi: 10.1016/j.biomaterials.2007.05.005
26. Dikovsky D, Bianco-Peled H, Seliktar D. The effect of structural alterations of PEG-fibrinogen hydrogel scaffolds on 3-d cellular morphology and cellular migration. Biomaterials (2006) 27(8):1496–506. doi: 10.1016/j.biomaterials.2005.09.038
27. Friedman RS, Jacobelli J, Krummel MF. Surface-bound chemokines capture and prime T cells for synapse formation. Nat Immunol (2006) 7(10):1101–8. doi: 10.1038/ni1384
28. Flanagan K, Moroziewicz D, Kwak H, Horig H, Kaufman HL. The lymphoid chemokine CCL21 costimulates naive T cell expansion and Th1 polarization of non-regulatory CD4+ T cells. Cell Immunol (2004) 231(1-2):75–84. doi: 10.1016/j.cellimm.2004.12.006
29. Gollmer K, Asperti-Boursin F, Tanaka Y, Okkenhaug K, Vanhaesebroeck B, Peterson JR, et al. CCL21 mediates CD4+ T-cell costimulation via a DOCK2/Rac-dependent pathway. Blood (2009) 114(3):580–8. doi: 10.1182/blood-2009-01-200923
30. Molon B, Gri G, Bettella M, Gomez-Mouton C, Lanzavecchia A, Martinez AC, et al. T Cell costimulation by chemokine receptors. Nat Immunol (2005) 6(5):465–71. doi: 10.1038/ni1191
31. Mitchison NA. An exact comparison between the efficiency of two- and three-cell-type clusters in mediating helper activity. Eur J Immunol (1990) 20(3):699–702. doi: 10.1002/eji.1830200335
32. Ingulli E, Mondino A, Khoruts A, Jenkins MK. In vivo detection of dendritic cell antigen presentation to CD4(+) T cells. J Exp Med (1997) 185(12):2133–41. doi: 10.1084/jem.185.12.2133
33. Woolf E, Grigorova I, Sagiv A, Grabovsky V, Feigelson SW, Shulman Z, et al. Lymph node chemokines promote sustained T lymphocyte motility without triggering stable integrin adhesiveness in the absence of shear forces. Nat Immunol (2007) 8(10):1076–85. doi: 10.1038/ni1499
34. Morotti M, Albukhari A, Alsaadi A, Artibani M, Brenton JD, Curbishley SM, et al. Promises and challenges of adoptive T-cell therapies for solid tumours. Br J Cancer (2021) 124(11):1759–76. doi: 10.1038/s41416-021-01353-6
35. Miyagawa F, Gutermuth J, Zhang H, Katz SI. The use of mouse models to better understand mechanisms of autoimmunity and tolerance. J Autoimmun (2010) 35(3):192–8. doi: 10.1016/j.jaut.2010.06.007
36. Kverneland AH, Chamberlain CA, Borch TH, Nielsen M, Mork SK, Kjeldsen JW, et al. Adoptive cell therapy with tumor-infiltrating lymphocytes supported by checkpoint inhibition across multiple solid cancer types. J Immunother Cancer (2021) 9(10). doi: 10.1136/jitc-2021-003499
37. Rosenberg SA, Packard BS, Aebersold PM, Solomon D, Topalian SL, Toy ST, et al. Use of tumor-infiltrating lymphocytes and interleukin-2 in the immunotherapy of patients with metastatic melanoma. a preliminary report. N Engl J Med (1988) 319(25):1676–80. doi: 10.1056/NEJM198812223192527
38. Maibach F, Sadozai H, Seyed Jafari SM, Hunger RE, Schenk M, et al. Tumor-infiltrating lymphocytes and their prognostic value in cutaneous melanoma. Front Immunol (2020) 11:2105. doi: 10.3389/fimmu.2020.02105
39. Rohaan MW, van den Berg JH, Kvistborg P, Haanen J. Adoptive transfer of tumor-infiltrating lymphocytes in melanoma: a viable treatment option. J Immunother Cancer (2018) 6(1):102. doi: 10.1186/s40425-018-0391-1
40. Lee S, Margolin K. Tumor-infiltrating lymphocytes in melanoma. Curr Oncol Rep (2012) 14(5):468–74. doi: 10.1007/s11912-012-0257-5
41. Dundr P, Nemejcova K, Bartu M, Ticha I, Jaksa R. [Evaluation of inflammatory cells (tumor infiltrating lymphocytes - TIL) in malignant melanoma]. Cesk Patol. (2018) 54(1):27–31.
42. Chalmers ZR, Connelly CF, Fabrizio D, Gay L, Ali SM, Ennis R. Analysis of 100,000 human cancer genomes reveals the landscape of tumor mutational burden. Genome Med (2017) 9(1):34. doi: 10.1186/s13073-017-0424-2
43. Pages F, Galon J, Dieu-Nosjean MC, Tartour E, Sautes-Fridman C, Fridman WH, et al. Immune infiltration in human tumors: a prognostic factor that should not be ignored. Oncogene (2010) 29(8):1093–102. doi: 10.1038/onc.2009.416
44. Schatton T, Scolyer RA, Thompson JF, Mihm MC. Tumor-infiltrating lymphocytes and their significance in melanoma prognosis. Methods Mol Biol (2014) 1102:287–324. doi: 10.1007/978-1-62703-727-3_16
45. Badalamenti G, Fanale D, Incorvaia L, Barraco N, Listi A, Maragliano R, et al. Role of tumor-infiltrating lymphocytes in patients with solid tumors: Can a drop dig a stone? Cell Immunol (2018) 343: p. 103753. doi: 10.1016/j.cellimm.2018.01.013
46. Zito Marino F, Ascierto PA, Rossi G, Staibano S, Montella M, Russo D, et al. Are tumor-infiltrating lymphocytes protagonists or background actors in patient selection for cancer immunotherapy? Expert Opin Biol Ther (2017) 17(6):735–46. doi: 10.1080/14712598.2017.1309387
Keywords: T-cells, tumor infiltrating lymphocytes (TIL), cancer immunotherapy, synthetic immune niche (SIN), adoptive cell therapy
Citation: Yunger S, Geiger B, Friedman N, Besser MJ and Adutler-Lieber S (2023) Modulating the proliferative and cytotoxic properties of patient-derived TIL by a synthetic immune niche of immobilized CCL21 and ICAM1. Front. Oncol. 13:1116328. doi: 10.3389/fonc.2023.1116328
Received: 05 December 2022; Accepted: 01 February 2023;
Published: 03 March 2023.
Edited by:
Yi Zhang, First Affiliated Hospital of Zhengzhou University, ChinaReviewed by:
Else Marit Inderberg, Oslo University Hospital, NorwayJaya Lakshmi Thangaraj, University of California, San Diego, United States
Copyright © 2023 Yunger, Geiger, Friedman, Besser and Adutler-Lieber. This is an open-access article distributed under the terms of the Creative Commons Attribution License (CC BY). The use, distribution or reproduction in other forums is permitted, provided the original author(s) and the copyright owner(s) are credited and that the original publication in this journal is cited, in accordance with accepted academic practice. No use, distribution or reproduction is permitted which does not comply with these terms.
*Correspondence: Benjamin Geiger, YmVubnkuZ2VpZ2VyQHdlaXptYW5uLmFjLmls; Michal J. Besser, bWJlc3NlckB0YXVleC50YXUuYWMuaWw=
†Deceased
‡These authors have contributed equally to this work