- 1Department of Oncology, Renmin Hospital of Wuhan University, Wuhan, Hubei, China
- 2Department of Hepatobiliary Surgery, Renmin Hospital of Wuhan University, Wuhan, China
Nasopharyngeal carcinoma (NPC) is a malignant tumor originating from the epithelial cells of the nasopharynx with a unique geographic distribution, and is particularly prevalent in East and Southeast Asia. Due to its anatomical location, the surgery is difficult to access and the high sensitivity of nasopharyngeal cancer to radiotherapy (RT) makes it the main treatment modality. Radical radiotherapy is the first-line treatment for early-stage nasopharyngeal carcinoma and the cornerstone of multidisciplinary treatment for patients with locally advanced nasopharyngeal carcinoma. Nevertheless, radiotherapy interruption is inevitable as a consequence of unavoidable factors such as public holidays, machine malfunction, patient compliance, and adverse response to treatment, which in turn leads to a reduction in bioactivity and causes sublethal loss of tumor cells to repair. Unirradiated tumor cells are more likely to repopulate at or near their original fastest growth rate during this interval. If no measures are taken after the radiotherapy interruption, such as increasing the dose of radiotherapy and systemic therapy, the tumor is most likely to go uncontrolled and then progress. This review describes the effects of radiotherapy interruption on nasopharyngeal carcinoma, the mechanism of the effect, and explores the measures that can be taken in response to such interruption.
1 Introduction
Nasopharyngeal carcinoma (NPC), a malignant tumor, originates from the epithelial cells of the nasopharynx, and is characterized by a unique geographical location with particular prevalence in East and Southeast Asia (1). The incidence can be as high as 25 to 50 cases per 100,000 in southern China (2). According to the International Agency for Research on Cancer (IARC), approximately 129,000 people were diagnosed with nasopharyngeal cancer in 2018, which only accounts for 0.7% of total cancers diagnosed (3). Gender differences exist in the incidence of nasopharyngeal cancer, with a higher incidence in males than in females, and the ratio was approximately 2.5:1 in China in 2015 (4).
The World Health Organization (WHO) classifies nasopharyngeal carcinoma into three histological subtypes, namely keratinizing squamous cell carcinoma, nonkeratinizing (differentiated or undifferentiated) carcinoma, and basal-like carcinoma. Undifferentiated carcinomas are the most common in high prevalence areas, accounting for about 95% or more (1, 5, 6). Nasopharyngeal carcinoma may be associated with Chinese salt cured fish, passive smoking, oral health and oral microbiota as well as with infection of Epstein-Barr virus (EBV) of infection (7).
Compared with computed tomography (CT), magnetic resonance imaging (MRI) can better identify early-stage nasopharyngeal carcinoma, with greater sensitivity and discrimination of infiltration of adjacent soft tissue, skull base and cranial nerve infiltration, and involvement of retropharyngeal lymph nodes. With its advantages of high soft tissue resolution, multiparametric imaging and non-ionizing radiation, MRI has replaced CT as the first choice for diagnosis, staging, efficacy assessment and follow-up of nasopharyngeal carcinoma (8). Surgery is difficult to operate owing to its specific anatomical location. In contrast, nasopharyngeal carcinoma is highly sensitive to radiotherapy (RT), making it the primary treatment modality. Radical radiotherapy is the first-line treatment for early-stage nasopharyngeal carcinoma and the cornerstone of multi-disciplinary treatment for patients with locally advanced nasopharyngeal carcinoma (9, 10).
2 Development of radiotherapy
Conventional two-dimensional radiotherapy (2DCRT) was the main radiotherapy technique until the 1990s. 2DCRT is principally based on contraction field radiation techniques, where the target field is gradually reduced or modified to deliver the desired dose (11). However, conventional radiotherapy of the head and neck is associated with severe acute and late toxicity due to the limitations of its degree of consistency. Mucositis is the most common acute side effect caused by radiation to the oral mucosa, accompanied by severe pain, dysphagia and malnutrition. Other acute and late effects include xerostomia and taste disturbances, hearing loss, persistent xerostomia, radiological osteonecrosis of the mandible, and dysphagia (12–14). Although there is no significant impact on survival outcomes, quality of life can be severely diminished.
Over the past decade, intensity-modulated radiotherapy (IMRT) has replaced 2DCRT, which uses a dynamic multileaf collimator to adjust the shape and intensity of individual beams to achieve optimal dose distribution in the tumor region. A more conformal dose distribution allows IMRT to minimize dose delivery to organs at risk (OAR), including the brainstem, spinal cord, and optic cross (15–17). The application of daily image guidance (image-guided radiation therapy) also reduces the dose in the planned target volume (PTV), which further reduces normal tissue exposure (18). Compared to conventional two-dimensional (2D) or three-dimensional (3D) radiotherapy, IMRT provides high doses of radiation for nasopharyngeal cancer while protecting adjacent vital structures and reducing treatment toxicity (19–23). Moreover, due to dosimetric advantages (24), IMRT is also superior to 2DCRT in terms of preservation of parotid gland, improvement of quality of life (17, 21) and reduction of temporal lobe neuropathy (TLN) rate (20, 25) in patients with nasopharyngeal carcinoma. Patients with nasopharyngeal carcinoma treated with IMRT can achieve local control and overall survival rates of up to 90% and 80%, respectively (26, 27), which are better than those of 2DCRT (19, 22, 23, 28). Table 1 summarized the clinical data on IMRT versus 2D-CRT (29).
3 Interruption of radiotherapy
In order to achieve better prognosis, an uninterrupted routine radiotherapy schedule is an essential necessity for precise radiotherapy of nasopharyngeal carcinoma (36). Disruptions in radiotherapy, however, are inevitable for several unavoidable factors, such as public holidays (37) (the largest share, about 39-46%), machine failures, patient compliance, and adverse effects of treatment (38). Similarly, because of the pandemic of Corona Virus Disease 2019, confirmed positive patients had longer treatment interruptions, which led to fewer patients completing radiotherapy, thus increasing local disease progression (39).
The length of delayed treatment is a key indicator of the severity of treatment interruption (36, 40). It has been shown that 5-year survival is reduced by 10-20% in patients with squamous head and neck cancer who are treated for a total duration of up to 10 days beyond the original schedule, and even a one-day interruption results in 1.4% reduction in local control (41). The timing of radiotherapy interruption is of course important (42). Skladowsky et al. (43) reported that patients with supraglottic laryngeal carcinoma who interrupted radiotherapy on day 19 had lower local tumor control than those without a treatment gap. Generally speaking, nasopharyngeal cancer is extremely sensitive to radiotherapy. Interruption of radiotherapy or prolongation of treatment can have an adverse effect on the prognosis of patients (44, 45).
3.1 Split-course radiotherapy
Split-course radiotherapy is a form of radiotherapy fractionation. In contrast to conventional radiotherapy, the single dose is greater, the total radiotherapy dose is lower, and radiotherapy sessions is less frequent. Split-course radiotherapy is usually given at high doses of 3-5 Gy per day or even higher (46). Split-course radiotherapy is usually divided into two courses, typically 1-2.5 weeks, with an interval of 4-6 weeks between treatments, that can increase the total treatment time. Effectiveness and tolerability are assessed by the physician during this interval. Recovery of normal tissue also occurs during this interval, which reduces the incidence of acute grade ≥3 toxicity to 41-53% (47).
Some studies have shown that the poorer efficacy of split-course radiotherapy in comparison to continuous radiotherapy (48–50) may be related to the interruption of treatment during the interval and the accelerated repopulation of malignant cells, which leads to reduced efficacy (47, 51).
3.2 Length of interruption time
The study by Kong et al. found median interruption time of 3 days was detrimental for prognosis (3-year OS: 94.4% vs 64.2%, P=0.046) (52). In the study by Xu et al, patients with nasopharyngeal cancer were analyzed for the effects of interruptions >2 days vs ≤ 2 days, >3 days vs ≤ 3 days, and 4 days vs ≤ 4 days on LFRS, PFS, and OS, respectively. The results demonstrated that the interruption time threshold of 4 days had significant influence on PFS (1-year PFS:92.9% vs 91.2%; 3-year PFS:72.1% vs 81.9%; P=0.010), and OS (1-year OS:97.6% vs 97.4%; 3-year OS:80.8% vs 87.9%; P=0.002) (53). In the prognostic study of IMRT combined with or without chemotherapy in patients with nasopharyngeal cancer by Shyh-An Yeh et al (54), we could find that radiotherapy interruption (≥5 days) was a poor prognostic factor for overall survival (OS) (5-year OS: 83.4% vs 67.8%, P=0.007). Another study by Yao et al. also found interruption of radiotherapy for more than 5 days in nasopharyngeal cancer patients with stage T3-T4 was an unfavorable factor impacting prognosis (5-year LRFS: 97% vs 83%, P < 0.001; multivariate analysis: HR = 9.64, 95% CI= 4.10-22.65). Besides, patients receiving a schedule dose of 70 Gy in 33 fractions (2.12 Gy/F) were significantly (P = 0.013) more likely to have a longer radiotherapy interruption (> 5 days) than patients who received a dose of 68 Gy in 30 F (2.27 Gy/F) (36). While another study showed that interruption of more than 7 days was detrimental for prognosis (training cohort: 5-year OS: 82.4% vs 86.5%, P = 0.001; validation cohort: 5-year OS, 85.2% vs 86.7%, P = 0.013). Time of interruption was also confirmed as an independent prognostic factor by further multifactorial analysis (training cohort: HR= 1.49, 95% CI=1.14-1.95, P = 0.003; validation cohort: HR=1.37, 95% CI=1.07-1.65, P=0.031) (55).
3.3 Stages in which radiotherapy interruptions occur
Kwong et al. briefly explored the time point at which radiotherapy interruptions occurred throughout the course of treatment and found that interruptions occurring at or near the beginning of treatment did not significantly affect prognosis. Besides, they also found that the rate of loco-regional failure increased by 3.3% for each day of treatment interruption (56). In the study of Yang et al., patients were categorized into prior and subsequent interruptions based on whether they were halfway through their radiotherapy schedule, and were subsequently grouped again according to the duration of the interruption. The results showed that prior interruptions longer than 1 day (5-year OS: 89.6% vs. 85.7%, p<0.001; 5-year DFS: 81.4% vs. 76.4%, p<0.001) and subsequent interruptions longer than 4 days (88.4% vs. 82.3%, p<0.001; 79.2% vs. 75.1%, p=0.006) were significantly detrimental to DFS and OS. In the further multifactorial analysis, interruptions longer than 3 or 4 days afterwards were both poor prognostic factors (57). Certainly, it has been reported that the prolongation of the treatment time has no effect on the prognosis of the patients (57).
The time of treatment initiation is also critical, with the exception of factors such as prolonged radiotherapy and interruption of radiotherapy that can negatively affect patient outcomes. One study showed that for each additional week of time between diagnosis and formal initiation of treatment for head and neck cancer patients, their local control rate decreased by 1%. And, after waiting 28 days (the median waiting time), 62% of patients had a 46% increase in tumor volume and 20% had metastases to lymph nodes (58, 59). Evan M et al. also comprehensively analyzed the effect of delayed treatment on the prognosis of patients with head and neck cancer and concluded that the delay in the time from diagnosis to treatment and the prolonged postoperative wait for adjuvant radiotherapy could adversely affect the prognosis of patients (60). A short postoperative interval to adjuvant radiotherapy was found to be beneficial in improving patient survival, and this interval was usually considered optimal to be controlled at 6 weeks or less (61–63). Table 2 summarized real-world data on the impact of radiotherapy interruptions.
4 Possible mechanisms
Tumor tissue regenerates at a faster rate than normal tissue, and the onset of rapid cell regeneration in tumor tissue during the treatment interval results in a lower radiobiologic dose to the planned target volume (PTV) (64). Radiotherapy interruption for nearly a full workweek and reduction in radiation service utilization may compromise the therapeutic benefit for patients because of the reduction in biological activity, which can lead to sublethal loss of repair (65).
At the beginning of radiotherapy, numerous tumor cells will be far from the capillaries and will therefore be in various states of oxygen deprivation. They will either be in a quiescent state or multiply at a much slower rate than when they were initially created. In addition, cell loss factor (CLF) is usually high while treatment is starting, especially in larger tumors. Tumors become smaller as radiotherapy proceeds, vascular distribution begins to improve, and CLF decreases. As a result, any cells that have not been killed by radiation begin to become better oxidized and begin to grow (repopulate) at or near their fastest rate (66). This involves the well-known 4R principles of radiotherapy, namely regeneration, repair, reoxygenation and redistribution. The kinetics of tumor regeneration are graphically summarized in Figure 1, where the characteristic “dog-leg” shape shows that tumor repopulation remains close to zero after the start of treatment, meaning that the dose required to maintain TCP is essentially constant (horizontal line). After a delay of several weeks, the remaining cells begin to repopulate rapidly, and the additional dose required to kill new cells and maintain TCP increases linearly with time. Therefore, the uncompensated interruptions that lead to the extension of treatment to this period are particularly problematic (67). Unless additional doses are added, eradication of newly generated cells becomes unlikely and tumor progression is thus possible.
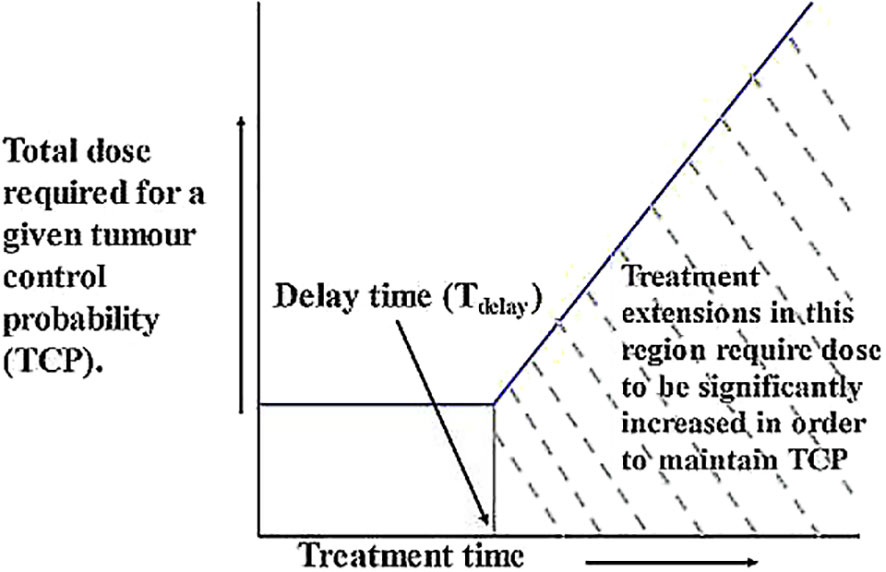
Figure 1 Graphical representation of the relationship between TCP and treatment duration. After the start of treatment, tumor repopulation remains close to zero, meaning that the dose required to maintain TCP remains essentially constant (horizontal line). After a delay of several weeks, the remaining cells begin to repopulate rapidly, and the additional dose required to kill new cells and maintain TCP increases linearly with time (67).
The time to tumor multiplication (Tpot) is an important issue in cancer treatment. A study by Delahaut et al. revealed a mean absolute tumor progression rate of 0.23 ± 0.2 cm3/day in 19 patients with squamous cell carcinoma of the head and neck (68). In more aggressive tumors, Tpot values may be surprisingly low, usually much less than 7 days, and re-proliferation rates are much faster (69–71). The delay of starting radiotherapy may lead to residual tumor proliferation after surgery. Besides, any tumor cells which were still existing at the end of the treatment are likely to grow at the fastest rate. If treatment is prolonged at this point, the increased time will allow for the generation of more cells.
Unless additional doses are added, eradication of newly generated cells becomes unlikely and tumor progression is thus possible. The kinetics of tumor regeneration are graphically summarized in Figure 1, where the characteristic “dog-leg” shape shows that tumor repopulation remains close to zero after the start of treatment, meaning that the dose required to maintain TCP is essentially constant (horizontal line). After a delay of several weeks, the remaining cells begin to repopulate rapidly, and the additional dose required to kill new cells and maintain TCP increases linearly with time. Therefore, the uncompensated interruptions that lead to the extension of treatment to this period are particularly problematic (67).
5 Measures that can be taken
5.1 Preventive measures
Nutritional assessment prior to treatment is also quite important. Some studies have shown that patients with pre-treatment malnutrition were significantly more likely to interrupt treatment than patients with normal nutrition (72). Oral prophylactic nutritional supplements can improve patient tolerance to concurrent radiotherapy (73). Oral care issues should not be underestimated either (74). Oral mucositis is a common toxic side effect during concurrent radiotherapy for nasopharyngeal cancers.
Adequate protein intake minimizes the severity of oral mucositis in patients with head and neck cancer undergoing radiotherapy (75). Early nutritional intervention, including oral feeding, nasogastric tube, gastrostomy, etc., can significantly improve weight loss and interrupt or delay of radiotherapy (76). Parenteral supplementation with glutamine (bipeptide) can also significantly reduce the rate of treatment interruption and the incidence of serious adverse reactions (77). The standard treatment of malnutrition should follow the five-step treatment principle of the European Society for Clinical Nutrition and Metabolism (ESPEN). ONS is the preferred, but not the only, form of enteral nutrition recognized by guidelines and expert consensus. It is also quite essential to establish good relationship with patients and pay attention to their psychological problems. According to Chen et al, being depressed before treatment was significantly associated with radiotherapy interruption and low survival in head and neck cancer patients (78). Pre-treatment should also focus on the mental health and mental status of patients. Patients with depressive symptoms are also more likely to have disrupted treatment, so it is important to focus on screening for depression and timely intervention during treatment (79).
5.2 Compensation for radiotherapy interruption
Treatment interruptions are inevitable, especially in the context of a new coronary pneumonia pandemic. For how to compensate for interrupted doses, Hendry et al. made the following recommendations: a. Use weekend time for radiotherapy; b. Increase the number of daily radiotherapy sessions, such as splitting twice a day; c. Increase the dose of a single radiotherapy session without extending the total treatment time; and d. Extend the total treatment time (80). However, regimen b increases the probability of normal tissue complications due to incomplete repair of normal tissue between divisions, resulting in increased sublethal damage to normal tissue (64). Regimens c and d either result in reduced local control rates or excessive late adverse effects (80).
The risk of radiotherapy interruption should be taken into account when the treatment regimen is developed and a set of compensatory measures, such as an increased compensatory dose (65), i.e., equivalent dose of 2 Gy per fraction (EQD2) (81), should be developed based on the physical condition of the patient, the severity of the disease. EQD2VH can be used as a decision tool when making a decision on the most appropriate compensation package for patients. It provides radiobiological dose-volume histograms that explain inhomogeneous dose distributions, as well as direct visual and quantitative comparisons between the plan being studied and the expected plan. Key dose-volume histogram statistics are provided for each plan to help monitor dose and compare with dose limits (64).
Dose compensation usually takes into account the histologically relevant factors κ (Gy/d) (41) and trigger time TK (67) for accelerated cell repopulation of 28 days. Monte Carlo simulations, which quantifies the biological effects of radiotherapy interruptions as well as assessing statistical uncertainty, are available to provide time factor κ (Gy/d) algorithm, assess the daily rate of BED decline, and calculate the residual fractionated dose to guide the subsequent treatment (41, 80, 82). It has been demonstrated that prolonged total treatment time is associated with decreased local control rates in head and neck cancers (83). The same can be inferred for other tumors, particularly in cases with high tumor growth rates (41, 80, 82).
Systemic therapy is also an appropriate option (84). Reducing the negative impact of radiotherapy interruption relies not only on a flexible response from radiation oncologists, but also on appropriate comprehensive care and dedicated multidisciplinary collaboration (85).
6 Conclusion
Radiotherapy interruption can have varying degrees of impact on patient outcomes, and the possibility of such interruptions should be minimized in actual clinical practice. However, due to the existence of some irresistible factors, sometimes radiotherapy interruption cannot be avoided. When radiotherapy is interrupted, remedial measures should be taken as much as possible. For example, increase the number or dose of radiotherapy, or combine other treatment modalities to reduce the adverse effects caused by radiotherapy interruption.
Author contributions
FZ drafted the article. DY revised it critically. XL did final approval of the version to be submitted. All authors contributed to the article and approved the submitted version.
Funding
This research was supported by grants No.Y-HS202101- 0079 from Cisco hausen Cancer Research Foundation.
Conflict of interest
The authors declare that the research was conducted in the absence of any commercial or financial relationships that could be construed as a potential conflict of interest.
Publisher’s note
All claims expressed in this article are solely those of the authors and do not necessarily represent those of their affiliated organizations, or those of the publisher, the editors and the reviewers. Any product that may be evaluated in this article, or claim that may be made by its manufacturer, is not guaranteed or endorsed by the publisher.
References
1. Chen YP, Chan ATC, Le QT, Blanchard P, Sun Y, Ma J. Nasopharyngeal carcinoma. Lancet (2019) 394:64–80. doi: 10.1016/S0140-6736(19)30956-0
3. Bray F, Ferlay J, Soerjomataram I, Siegel RL, Torre LA, Jemal A. Global cancer statistics 2018: GLOBOCAN estimates of incidence and mortality worldwide for 36 cancers in 185 countries. CA Cancer J Clin (2018) 68:394–424. doi: 10.3322/caac.21492
4. Chen W, Zheng R, Baade PD, Zhang S, Zeng H, Bray F, et al. Cancer statistics in China, 2015. CA Cancer J Clin (2016) 66:115–32. doi: 10.3322/caac.21338
5. Chua MLK, Wee JTS, Hui EP, Chan ATC. Nasopharyngeal carcinoma. Lancet (2016) 387:1012–24. doi: 10.1016/S0140-6736(15)00055-0
6. Wang HY, Chang YL, To KF, Hwang JS, Mai HQ, Feng YF, et al. A new prognostic histopathologic classification of nasopharyngeal carcinoma. Chin J Cancer (2016) 35:41. doi: 10.1186/s40880-016-0103-5
7. Chang ET, Ye W, Zeng YX, Adami HO. The evolving epidemiology of nasopharyngeal carcinoma. Cancer Epidemiol Biomarkers Prev (2021) 30:1035–47. doi: 10.1158/1055-9965.EPI-20-1702
8. Tang LL, Chen YP, Chen CB, Chen MY, Chen NY, Chen XZ, et al. The Chinese society of clinical oncology (CSCO) clinical guidelines for the diagnosis and treatment of nasopharyngeal carcinoma. Cancer Commun (Lond) (2021) 41:1195–227. doi: 10.1002/cac2.12218
9. Kwong DL, Nicholls J, Wei WI, Chua DT, Sham JS, Yuen PW, et al. The time course of histologic remission after treatment of patients with nasopharyngeal carcinoma. Cancer (1999) 85:1446–53. doi: 10.1002/(SICI)1097-0142(19990401)85:7<1446::AID-CNCR4>3.0.CO;2-3
10. Pfister DG, Spencer S, Adelstein D, Adkins D, Anzai Y, Brizel DM, et al. Head and neck cancers, version 2.2020, NCCN clinical practice guidelines in oncology. J Natl Compr Canc Netw (2020) 18:873–98. doi: 10.6004/jnccn.2020.0031
11. Gluckman JL, Portugal LG. Photodynamic therapy for cancer of the head and neck. Cancer Treat Res (1995) 74:159–71. doi: 10.1007/978-1-4615-2023-8_9
12. Trotti A, Bellm LA, Epstein JB, Frame D, Fuchs HJ, Gwede CK, et al. Mucositis incidence, severity and associated outcomes in patients with head and neck cancer receiving radiotherapy with or without chemotherapy: a systematic literature review. Radiother Oncol (2003) 66:253–62. doi: 10.1016/S0167-8140(02)00404-8
13. Tsai CJ, Hofstede TM, Sturgis EM, Garden AS, Lindberg ME, Wei Q, et al. Osteoradionecrosis and radiation dose to the mandible in patients with oropharyngeal cancer. Int J Radiat Oncol Biol Phys (2013) 85:415–20. doi: 10.1016/j.ijrobp.2012.05.032
14. Eisbruch A, Lyden T, Bradford CR, Dawson LA, Haxer MJ, Miller AE, et al. Objective assessment of swallowing dysfunction and aspiration after radiation concurrent with chemotherapy for head-and-neck cancer. Int J Radiat Oncol Biol Phys (2002) 53:23–8. doi: 10.1016/S0360-3016(02)02712-8
15. Lee N, Xia P, Quivey JM, Sultanem K, Poon I, Akazawa C, et al. Intensity-modulated radiotherapy in the treatment of nasopharyngeal carcinoma: an update of the UCSF experience. Int J Radiat Oncol Biol Phys (2002) 53:12–22. doi: 10.1016/S0360-3016(02)02724-4
16. Chau RM, Teo PM, Kam MK, Leung SF, Cheung KY, Chan AT. Dosimetric comparison between 2-dimensional radiation therapy and intensity modulated radiation therapy in treatment of advanced T-stage nasopharyngeal carcinoma: to treat less or more in the planning organ-at-risk volume of the brainstem and spinal cord. Med Dosim (2007) 32:263–70. doi: 10.1016/j.meddos.2007.02.006
17. Chen YY, Zhao C, Wang J, Ma HL, Lai SZ, Liu Y, et al. Intensity-modulated radiation therapy reduces radiation-induced trismus in patients with nasopharyngeal carcinoma: a prospective study with >5 years of follow-up. Cancer (2011) 117:2910–6. doi: 10.1002/cncr.25773
18. Chen AM, Farwell DG, Luu Q, Donald PJ, Perks J, Purdy JA. Evaluation of the planning target volume in the treatment of head and neck cancer with intensity-modulated radiotherapy: what is the appropriate expansion margin in the setting of daily image guidance? Int J Radiat Oncol Biol Phys (2011) 81:943–9. doi: 10.1016/j.ijrobp.2010.07.017
19. Peng G, Wang T, Yang KY, Zhang S, Zhang T, Li Q, et al. A prospective, randomized study comparing outcomes and toxicities of intensity-modulated radiotherapy vs. conventional two-dimensional radiotherapy for the treatment of nasopharyngeal carcinoma. Radiother Oncol (2012) 104:286–93. doi: 10.1016/j.radonc.2012.08.013
20. Kam MK, Leung SF, Zee B, Chau RM, Suen JJ, Mo F, et al. Prospective randomized study of intensity-modulated radiotherapy on salivary gland function in early-stage nasopharyngeal carcinoma patients. J Clin Oncol (2007) 25:4873–9. doi: 10.1200/JCO.2007.11.5501
21. Pow EH, Kwong DL, McMillan AS, Wong MC, Sham JS, Leung LH, et al. Xerostomia and quality of life after intensity-modulated radiotherapy vs. conventional radiotherapy for early-stage nasopharyngeal carcinoma: initial report on a randomized controlled clinical trial. Int J Radiat Oncol Biol Phys (2006) 66:981–91. doi: 10.1016/j.ijrobp.2006.06.013
22. Du T, Xiao J, Qiu Z, Wu K. The effectiveness of intensity-modulated radiation therapy versus 2D-RT for the treatment of nasopharyngeal carcinoma: A systematic review and meta-analysis. PloS One (2019) 14:e0219611. doi: 10.1371/journal.pone.0219611
23. Luo MS, Huang GJ, Liu HB. Oncologic outcomes of IMRT versus CRT for nasopharyngeal carcinoma: A meta-analysis. Med (Baltimore) (2019) 98:e15951. doi: 10.1097/MD.0000000000015951
24. Kam MK, Chau RM, Suen J, Choi PH, Teo PM. Intensity-modulated radiotherapy in nasopharyngeal carcinoma: dosimetric advantage over conventional plans and feasibility of dose escalation. Int J Radiat Oncol Biol Phys (2003) 56:145–57. doi: 10.1016/S0360-3016(03)00075-0
25. Zhou GQ, Yu XL, Chen M, Guo R, Lei Y, Sun Y, et al. Radiation-induced temporal lobe injury for nasopharyngeal carcinoma: a comparison of intensity-modulated radiotherapy and conventional two-dimensional radiotherapy. PloS One (2013) 8:e67488. doi: 10.1371/journal.pone.0067488
26. Lin S, Lu JJ, Han L, Chen Q, Pan J. Sequential chemotherapy and intensity-modulated radiation therapy in the management of locoregionally advanced nasopharyngeal carcinoma: experience of 370 consecutive cases. BMC Cancer (2010) 10:39. doi: 10.1186/1471-2407-10-39
27. Wong FC, Ng AW, Lee VH, Lui CM, Yuen KK, Sze WK, et al. Whole-field simultaneous integrated-boost intensity-modulated radiotherapy for patients with nasopharyngeal carcinoma. Int J Radiat Oncol Biol Phys (2010) 76:138–45. doi: 10.1016/j.ijrobp.2009.01.084
28. Co J, Mejia MB, Dizon JM. Evidence on effectiveness of intensity-modulated radiotherapy versus 2-dimensional radiotherapy in the treatment of nasopharyngeal carcinoma: Meta-analysis and a systematic review of the literature. Head Neck (2016) 38(Suppl 1):E2130–42. doi: 10.1002/hed.23977
29. Moon SH, Cho KH, Lee CG, Keum KC, Kim YS, Wu HG, et al. IMRT vs. 2D-radiotherapy or 3D-conformal radiotherapy of nasopharyngeal carcinoma: Survival outcome in a Korean multi-institutional retrospective study (KROG 11-06). Strahlenther Onkol (2016) 192:377–85. doi: 10.1007/s00066-016-0959-y
30. Lai SZ, Li WF, Chen L, Luo W, Chen YY, Liu LZ, et al. How does intensity-modulated radiotherapy versus conventional two-dimensional radiotherapy influence the treatment results in nasopharyngeal carcinoma patients? Int J Radiat Oncol Biol Phys (2011) 80:661–8. doi: 10.1016/j.ijrobp.2010.03.024
31. Qiu WZ, Peng XS, Xia HQ, Huang PY, Guo X, Cao KJ. A retrospective study comparing the outcomes and toxicities of intensity-modulated radiotherapy versus two-dimensional conventional radiotherapy for the treatment of children and adolescent nasopharyngeal carcinoma. J Cancer Res Clin Oncol (2017) 143:1563–72. doi: 10.1007/s00432-017-2401-y
32. Tang LL, Chen L, Mao YP, Li WF, Sun Y, Liu LZ, et al. Comparison of the treatment outcomes of intensity-modulated radiotherapy and two-dimensional conventional radiotherapy in nasopharyngeal carcinoma patients with parapharyngeal space extension. Radiother Oncol (2015) 116:167–73. doi: 10.1016/j.radonc.2015.07.038
33. Zhang MX, Li J, Shen GP, Zou X, Xu JJ, Jiang R, et al. Intensity-modulated radiotherapy prolongs the survival of patients with nasopharyngeal carcinoma compared with conventional two-dimensional radiotherapy: A 10-year experience with a large cohort and long follow-up. Eur J Cancer (Oxford England: 1990) (2015) 51:2587–95. doi: 10.1016/j.ejca.2015.08.006
34. Zhong H, Chen G, Lin D, Chen G. Comparison of side effects of intensity modulated radiotherapy and conventional radiotherapy in 69 cases with nasopharyngeal carcinoma. Lin Chung Er Bi Yan Hou Tou Jing Wai Ke Za Zhi (2013) 27:462–4.
35. Lee AW, Ng WT, Chan LL, Hung WM, Chan CC, Sze HC, et al. Evolution of treatment for nasopharyngeal cancer–success and setback in the intensity-modulated radiotherapy era. Radiother Oncol (2014) 110:377–84. doi: 10.1016/j.radonc.2014.02.003
36. Yao JJ, Jin YN, Wang SY, Zhang F, Zhou GQ, Zhang WJ, et al. The detrimental effects of radiotherapy interruption on local control after concurrent chemoradiotherapy for advanced T-stage nasopharyngeal carcinoma: an observational, prospective analysis. BMC Cancer (2018) 18:740. doi: 10.1186/s12885-018-4495-2
37. Xu C, Yang KB, Feng RJ, Chen L, Du XJ, Mao YP, et al. Radiotherapy interruption due to holidays adversely affects the survival of patients with nasopharyngeal carcinoma: a joint analysis based on large-scale retrospective data and clinical trials. Radiat Oncol (London England) (2022) 17:36. doi: 10.1186/s13014-022-02006-5
38. James ND, Williams MV, Summers ET, Jones K, Cottier B. Royal college of radiologists clinical audit s. the management of interruptions to radiotherapy in head and neck cancer: an audit of the effectiveness of national guidelines. Clin Oncol (R Coll Radiol) (2008) 20:599–605. doi: 10.1016/j.clon.2008.05.003
39. Barik SK, Singh AK, Mishra M, Amritt A, Sahu DP, Das Majumdar SK, et al. Effect of treatment interruptions and outcomes in cancer patients undergoing radiotherapy during the first wave of COVID-19 pandemic in a tertiary care institute. J Egyptian Natl Cancer Institute (2022) 34:28. doi: 10.1186/s43046-022-00129-0
40. Chen M, Jiang GL, Fu XL, Wang LJ, Qian H, Chen GY, et al. The impact of overall treatment time on outcomes in radiation therapy for non-small cell lung cancer. Lung Cancer (2000) 28:11–9. doi: 10.1016/S0169-5002(99)00113-0
41. Bese NS, Hendry J, Jeremic B. Effects of prolongation of overall treatment time due to unplanned interruptions during radiotherapy of different tumor sites and practical methods for compensation. Int J Radiat Oncol Biol Phys (2007) 68:654–61. doi: 10.1016/j.ijrobp.2007.03.010
42. Xu C, Chen YP, Liu X, Tang LL, Chen L, Mao YP, et al. Socioeconomic factors and survival in patients with non-metastatic head and neck squamous cell carcinoma. Cancer Sci (2017) 108:1253–62. doi: 10.1111/cas.13250
43. Skladowski K, Law MG, Maciejewski B, Steel GG. Planned and unplanned gaps in radiotherapy: the importance of gap position and gap duration. Radiother Oncol (1994) 30:109–20. doi: 10.1016/0167-8140(94)90039-6
44. Wang W, Feng M, Fan Z, Li J, Lang J. Clinical outcomes and prognostic factors of 695 nasopharyngeal carcinoma patients treated with intensity-modulated radiotherapy. BioMed Res Int (2014) 2014:814948. doi: 10.1155/2014/814948
45. Xu L, Pan J, Wu J, Pan C, Zhang Y, Lin S, et al. Factors associated with overall survival in 1706 patients with nasopharyngeal carcinoma: significance of intensive neoadjuvant chemotherapy and radiation break. Radiother Oncol (2010) 96:94–9. doi: 10.1016/j.radonc.2010.04.006
46. Hunter D, Mauldon E, Anderson N. Cost-containment in hypofractionated radiation therapy: a literature review. J Med Radiat Sci (2018) 65:148–57. doi: 10.1002/jmrs.273
47. Chen AM, Vaughan A, Narayan S, Vijayakumar S. Palliative radiation therapy for head and neck cancer: toward an optimal fractionation scheme. Head Neck (2008) 30:1586–91. doi: 10.1002/hed.20894
48. Luo RX, Tang QX, Huang YW, Liao YP, Mou XD, Hu ZX. Comparison of continuous and split-course radiotherapy for nasopharyngeal carcinoma. Int J Radiat Oncol Biol Phys (1989) 16:307–10. doi: 10.1016/0360-3016(89)90319-2
49. Luo RX, Tang QX, Guo KP, Huang YW, Hu ZX. Comparison of continuous and split-course radiotherapy for nasopharyngeal carcinoma–an analysis of 1446 cases with squamous cell carcinoma grade 3. Int J Radiat Oncol Biol Phys (1994) 30:1107–9. doi: 10.1016/0360-3016(94)90316-6
50. Levendag PC, Nowak PJ, van der Sangen MJ, Jansen PP, Eijkenboom WM, Planting AS, et al. Local tumor control in radiation therapy of cancers in the head and neck. Am J Clin Oncol (1996) 19:469–77. doi: 10.1097/00000421-199610000-00009
51. Agarwal JP, Nemade B, Murthy V, Ghosh-Laskar S, Budrukkar A, Gupta T, et al. Hypofractionated, palliative radiotherapy for advanced head and neck cancer. Radiother Oncol (2008) 89:51–6. doi: 10.1016/j.radonc.2008.06.007
52. Kong M, Lim YJ, Kim Y. Concurrent chemoradiotherapy for loco-regionally advanced nasopharyngeal carcinoma: Treatment outcomes and prognostic factors. Asian Pac J Cancer Prev (2018) 19:1591–9. doi: 10.22034/APJCP.2018.19.6.1591
53. Xu GZ, Li L, Zhu XD. Effect of interrupted time during intensity modulated radiation therapy on survival outcomes in patients with nasopharyngeal cancer. Oncotarget (2017) 8:37817–25. doi: 10.18632/oncotarget.13713
54. Yeh SA, Hwang TZ, Wang CC, Yang CC, Lien CF, Wang CC, et al. Outcomes of patients with nasopharyngeal carcinoma treated with intensity-modulated radiotherapy. J Radiat Res (2021) 62:438–47. doi: 10.1093/jrr/rrab008
55. Yao JJ, Zhang F, Gao TS, Zhang WJ, Lawrence WR, Zhu BT, et al. Survival impact of radiotherapy interruption in nasopharyngeal carcinoma in the intensity-modulated radiotherapy era: A big-data intelligence platform-based analysis. Radiother Oncol (2019) 132:178–87. doi: 10.1016/j.radonc.2018.10.018
56. Kwong DL, Sham JS, Chua DT, Choy DT, Au GK, Wu PM. The effect of interruptions and prolonged treatment time in radiotherapy for nasopharyngeal carcinoma. Int J Radiat Oncol Biol Phys (1997) 39:703–10. doi: 10.1016/S0360-3016(97)00339-8
57. Yang XL, Zhou GQ, Lin L, Zhang LL, Chen FP, Lv JW, et al. Prognostic value of radiation interruption in different periods for nasopharyngeal carcinoma patients in the intensity-modulated radiation therapy era. Cancer Med (2021) 10:143–55. doi: 10.1002/cam4.3580
58. Wyatt RM, Beddoe AH, Dale RG. The effects of delays in radiotherapy treatment on tumour control. Phys Med Biol (2003) 48:139–55. doi: 10.1088/0031-9155/48/2/301
59. Jensen AR, Nellemann HM, Overgaard J. Tumor progression in waiting time for radiotherapy in head and neck cancer. Radiother Oncol (2007) 84:5–10. doi: 10.1016/j.radonc.2007.04.001
60. Graboyes EM, Kompelli AR, Neskey DM, Brennan E, Nguyen S, Sterba KR, et al. Association of treatment delays with survival for patients with head and neck cancer: A systematic review. JAMA Otolaryngol Head Neck Surg (2019) 145:166–77. doi: 10.1001/jamaoto.2018.2716
61. Shaikh T, Handorf EA, Murphy CT, Mehra R, Ridge JA, Galloway TJ. The impact of radiation treatment time on survival in patients with head and neck cancer. Int J Radiat Oncol Biol Phys (2016) 96:967–75. doi: 10.1016/j.ijrobp.2016.08.046
62. Harris JP, Chen MM, Orosco RK, Sirjani D, Divi V, Hara W. Association of survival with shorter time to radiation therapy after surgery for US patients with head and neck cancer. JAMA Otolaryngol Head Neck Surg (2018) 144:349–59. doi: 10.1001/jamaoto.2017.3406
63. Huang J, Barbera L, Brouwers M, Browman G, Mackillop WJ. Does delay in starting treatment affect the outcomes of radiotherapy? a systematic review. J Clin Oncol (2003) 21:555–63. doi: 10.1200/JCO.2003.04.171
64. O’Shea K, Coleman L, Fahy L, Kleefeld C, Foley MJ, Moore M. Compensation for radiotherapy treatment interruptions due to a cyberattack: An isoeffective DVH-based dose compensation decision tool. J Appl Clin Med Phys (2022) 23:e13716. doi: 10.1002/acm2.13716
65. Kawahara D, Nakano H, Saito A, Ozawa S, Nagata Y. Dose compensation based on biological effectiveness due to interruption time for photon radiation therapy. Br J Radiol (2020) 93:20200125. doi: 10.1259/bjr.20200125
66. Dale RG, Jones B. Radiotherapy treatment interruptions during the covid-19 pandemic: The UK experience and implications for radiobiology training. Radiat Phys Chem (Oxford England: 1993) (2022) 200:110214. doi: 10.1016/j.radphyschem.2022.110214
67. Withers HR, Taylor JM, Maciejewski B. The hazard of accelerated tumor clonogen repopulation during radiotherapy. Acta Oncol (Stockholm Sweden) (1988) 27:131–46. doi: 10.3109/02841868809090333
68. Delahaut G, Temam S, Ambroise J, Tao Y, Janot F, van der Vorst S. Prognostic impact of tumor growth velocity in head and neck squamous cell carcinoma treated by radiotherapy: A pilot study. Head Neck (2019) 41:3041–8. doi: 10.1002/hed.25789
69. Begg AC, Hofland I, Moonen L, Bartelink H, Schraub S, Bontemps P, et al. The predictive value of cell kinetic measurements in a European trial of accelerated fractionation in advanced head and neck tumors: an interim report. Int J Radiat Oncol Biol Phys (1990) 19:1449–53. doi: 10.1016/0360-3016(90)90357-P
70. Haustermans KM, Hofland I, Van Poppel H, Oyen R, Van de Voorde W, Begg AC, et al. Cell kinetic measurements in prostate cancer. Int J Radiat Oncol Biol Phys (1997) 37:1067–70. doi: 10.1016/S0360-3016(96)00579-2
71. Rew DA, Wilson GD. Cell production rates in human tissues and tumours and their significance. Part II: Clin data. Eur J Surg Oncol (2000) 26:405–17. doi: 10.1053/ejso.1999.0907
72. Hung CY, Hsueh SW, Lu CH, Chang PH, Chen PT, Yeh KY, et al. A prospective nutritional assessment using mini nutritional assessment-short form among patients with head and neck cancer receiving concurrent chemoradiotherapy. Support Care Cancer (2021) 29:1509–18. doi: 10.1007/s00520-020-05634-3
73. Huang S, Piao Y, Cao C, Chen J, Sheng W, Shu Z, et al. A prospective randomized controlled trial on the value of prophylactic oral nutritional supplementation in locally advanced nasopharyngeal carcinoma patients receiving chemo-radiotherapy. Oral Oncol (2020) 111:105025. doi: 10.1016/j.oraloncology.2020.105025
74. Demian NM, Shum JW, Kessel IL, Eid A. Oral surgery in patients undergoing chemoradiation therapy. Oral Maxillofac Surg Clin North Am (2014) 26:193–207. doi: 10.1016/j.coms.2014.01.006
75. Ferreira IB, Lima E, Canto PPL, Gontijo CA, Maia YCP, Pena GDG. Oral nutritional supplementation affects the dietary intake and body weight of head and neck cancer patients during (Chemo) radiotherapy. Nutrients (2020) 12:2516. doi: 10.3390/nu12092516
76. Zheng Z, Zhao X, Zhao Q, Zhang Y, Liu S, Liu Z, et al. The effects of early nutritional intervention on oral mucositis and nutritional status of patients with head and neck cancer treated with radiotherapy. Front Oncol (2020) 10:595632. doi: 10.3389/fonc.2020.595632
77. Wang CC, Hwang TZ, Yang CC, Lien CF, Wang CC, Shih YC, et al. Impact of parenteral glutamine supplement on oncologic outcomes in patients with nasopharyngeal cancer treated with concurrent chemoradiotherapy. Nutrients (2022) 14:997. doi: 10.3390/nu14050997
78. Chen AM, Hsu S, Felix C, Garst J, Yoshizaki T. Effect of psychosocial distress on outcome for head and neck cancer patients undergoing radiation. Laryngoscope (2018) 128:641–5. doi: 10.1002/lary.26751
79. Zimmaro LA, Sephton SE, Siwik CJ, Phillips KM, Rebholz WN, Kraemer HC, et al. Depressive symptoms predict head and neck cancer survival: Examining plausible behavioral and biological pathways. Cancer (2018) 124:1053–60. doi: 10.1002/cncr.31109
80. Hendry JH, Bentzen SM, Dale RG, Fowler JF, Wheldon TE, Jones B, et al. A modelled comparison of the effects of using different ways to compensate for missed treatment days in radiotherapy. Clin Oncol (R Coll Radiol) (1996) 8:297–307. doi: 10.1016/S0936-6555(05)80715-0
81. Pechačová Z, Lohynská R. Clinical application of time factor principles in radiotherapy in compensation of radiation series interruptions. Klinicka onkologie: casopis Ceske Slovenske onkologicke spolecnosti (2021) 34:455–62. doi: 10.48095/ccko2021455
82. Dale RG, Hendry JH, Jones B, Robertson AG, Deehan C, Sinclair JA. Practical methods for compensating for missed treatment days in radiotherapy, with particular reference to head and neck schedules. Clin Oncol (R Coll Radiol) (2002) 14:382–93. doi: 10.1053/clon.2002.0111
83. Gonzalez Ferreira JA, Jaen Olasolo J, Azinovic I, Jeremic B. Effect of radiotherapy delay in overall treatment time on local control and survival in head and neck cancer: Review of the literature. Rep Pract Oncol Radiother (2015) 20:328–39. doi: 10.1016/j.rpor.2015.05.010
84. Ying X, Bi J, Ding Y, Wei X, Wei W, Xin F, et al. Management and outcomes of patients with radiotherapy interruption during the COVID-19 pandemic. Front Oncol (2021) 11:754838. doi: 10.3389/fonc.2021.754838
Keywords: nasopharyngeal carcinoma, radiotherapy, interruption, mechanism, NPC
Citation: Zhao F, Yang D and Li X (2023) Effect of radiotherapy interruption on nasopharyngeal cancer. Front. Oncol. 13:1114652. doi: 10.3389/fonc.2023.1114652
Received: 02 December 2022; Accepted: 27 March 2023;
Published: 06 April 2023.
Edited by:
Sunil Dutt Sharma, Bhabha Atomic Research Centre (BARC), IndiaReviewed by:
QingFeng Liu, Tianjin First Central Hospital, ChinaChunyan Chen, Sun Yat-sen University Cancer Center (SYSUCC), China
Copyright © 2023 Zhao, Yang and Li. This is an open-access article distributed under the terms of the Creative Commons Attribution License (CC BY). The use, distribution or reproduction in other forums is permitted, provided the original author(s) and the copyright owner(s) are credited and that the original publication in this journal is cited, in accordance with accepted academic practice. No use, distribution or reproduction is permitted which does not comply with these terms.
*Correspondence: Xiangpan Li, rm001227@whu.edu.cn