- 1Surgical Oncology Unit, Department of Surgery, Reina Sofia University Hospital, Cordoba, Spain
- 2GE09 Research in peritoneal and retroperitoneal oncological surgery, Maimonides Biomedical Research Institute of Cordoba (IMIBIC), Reina Sofia University Hospital, University of Cordoba, Cordoba, Spain
- 3Pathology Unit, Reina Sofia University Hospital, Cordoba, Spain
Introduction: Pseudomyxoma peritonei (PMP) is a rare malignant disease characterized by a massive multifocal accumulation of mucin within the peritoneal cavity. The current treatment option is based on complete cytoreductive surgery combined with hyperthermic intraperitoneal chemotherapy. However, the recurrence is frequent with subsequent progression and death. To date, most of the studies published in PMP are related to histological and genomic analyses. Thus, the need for further studies unveiling the underlying PMP molecular mechanisms is urgent. In this regard, hypoxia and oxidative stress have been extensively related to tumoral pathologies, although their contribution to PMP has not been elucidated.
Methods: In this manuscript, we have evaluated, for the first time, the intratumoral real-time oxygen microtension (pO2mt) in the tumor (soft and hard mucin) and surrounding healthy tissue from five PMP patients during surgery. In addition, we measured hypoxia (Hypoxia Inducible Factor-1a; HIF-1α) and oxidative stress (catalase; CAT) markers in soft and hard mucin from the same five PMP patient samples and in five control samples.
Results: The results showed low intratumoral oxygen levels, which were associated with increased HIF-1α protein levels, suggesting the presence of a hypoxic environment in these tumors. We also found a significant reduction in CAT activity levels in soft and hard mucin compared with healthy tissue samples.
Discussion: In conclusion, our study provides the first evidence of low intratumoral oxygen levels in PMP patients associated with hypoxia and oxidative stress markers. However, further investigation is required to understand the potential role of oxidative stress in PMP in order to find new therapeutic strategies.
1 Introduction
Pseudomyxoma peritonei (PMP), also known as “Jelly belly”, is a rare condition characterized by the progressive and abundant accumulation of mucinous ascites and peritoneal implants (1–3). The most prevalent cause of this disease is a perforated epithelial tumor of the appendix (4). In the early stages of the disease, the absence of symptoms promotes the accumulation of large volumes of mucin inside the abdomen, eventually leading to severe abdominal enlargement, pain, and malnutrition (5, 6). Intestinal obstruction occurs over time as a consequence of compression on visceral organs, triggering an inflammatory and fibrotic mesothelium response that can be fatal in patients with untreated or recurrent PMP (4–6).
The Peritoneal Surface Oncology Group International (PSOGI) classification, which has been validated on several occasions (7, 8), divides PMP into four groups based on histological characteristics: i) acellular mucin (AM), ii) low-grade mucinous carcinoma (LG-PMP), iii) high-grade mucinous carcinoma (HG-PMP), and iv) PMP with the presence of signet ring cells (SRC) (4). However, histopathology does not completely predict tumor behavior (9). On the other hand, mucin from PMP is classified into soft, semi-hard, and hard mucin samples based on physical and chemical properties as well as visual appearance (10).
Under normal cellular conditions, mitochondrial respiration and NADPH oxidases generate reactive oxygen species (ROS), which are scavenged by cellular non-enzymatic and enzymatic antioxidant mechanisms such as superoxide dismutase [cytosolic (SOD1) and mitochondrial (SOD2)] and catalase (CAT), among others (11, 12). The imbalance between ROS production and their elimination causes oxidative stress, which has been associated with pathogenetic mechanisms in various diseases, including cancer (12, 13). In this sense, ROS overproduction can lead to cellular transformation by activating a wide range of signaling factors such as extracellular signal-regulated kinase 1/2 (ERK1/2), receptor tyrosine kinases (RTK), vascular endothelial growth factor (VEGF) and hypoxia inducible factor-1α (HIF-1α) to promote cellular proliferation, invasion, metastasis, and angiogenesis (14, 15). In addition to ROS, tumoral hypoxia is also responsible for HIF-1α upregulation, which in turn regulates the expression of proangiogenic, antioxidant, and goblet cell-associated factors, as well as mucin genes, allowing cancer cells to survive, proliferate, and undergo epithelial to mesenchymal transition (EMT) (16–18). However, the potential role of hypoxia and/or oxidative stress in PMP remains unknown.
This study aims to demonstrate, both in vitro and in vivo, that PMP is a tumor that grows in the absence of oxygen. Thus, we used intraoperative micro-oximeters, which had previously been used in other surgical disciplines (19–22) but had never been used for this purpose, to make intratumoral measurements of the real-time oxygen levels during the surgical procedure in PMP patients. Furthermore, we correlated the intraoperative pressure of oxygen values with measured cellular hypoxia and oxidative stress markers in tissue samples from these patients.
2 Material and methods
2.1 Patients and samples
This is a prospective analytic study performed in patients with PMP who underwent cytoreductive surgery combined with hyperthermic intraperitoneal chemotherapy (CRS + HIPEC) in our unit. The present study was included in the PI19/01603 study entitled “Molecular characterization of Pseudomyxoma peritonei and development of new therapeutic targets and biomarkers in a PMP xenograft human model˝, funded by Carlos III Research Institute in 2019. The Cordoba Research Ethics Committee reviewed and generated a favorable dictamen for this study on May 28th, 2019. All the patients were informed and signed the informed consent form.
We determined the real-time oxygen microtension (pO2mt) in tumoral and surrounding healthy tissues in five PMP (four from appendiceal origin and one from caecum-appendiceal stump origin) patients during the CRS + HIPEC (Table 1). Furthermore, hypoxia and oxidative stress markers in soft and hard mucin from the same 5 patient samples and in 5 different control samples were evaluated (3 appendix samples from a prophylactic appendectomy due to another medical condition and 2 normal colon samples from PMP patients). Until processing, all samples were stored at -80°C. Moreover, all samples were histologically studied by experienced pathologists to confirm the diagnosis.
OxyLite™ monitors (Oxford Optronix Ltd. United Kingdom) and specific probes were used to assess the pO2mt of tumor tissue (soft and hard mucin) following the manufacturer´s instructions. This oxygen monitors use a fluorescence-based technique to provide an absolute measurement of dissolved oxygen in mmHg or kPa, giving a direct readout of the balance between oxygen supply and oxygen consumption. The oxygen sensors are based on fluorescence quenching and fiber-optic technology. To excite a platinum-based fluorophore linked to the sensor tip, short pulses of LED light are transmitted along the fiber optic sensor. The instrument detects the resulting emission of fluorescent light, which is quenched by the presence of oxygen molecules. The instrument measures fluorescence lifetime, which is inversely proportional to dissolved oxygen levels and can be used to calculate absolute oxygen values in mmHg or kPa. The oxygen microsensors are all made of optical fibers with an outer diameter of 230μm. The sensors used have a 350μm tip diameter and a minimally invasive ‘bare-fiber’ format (with integrated temperature sensor). Temperatures variations have a minimum effect on fluorescence-based oxygen detection. To achieve the highest level of precision, we used sensors with integrated temperature detectors and monitors with constant temperature input (provided by the thermocouple).
Before performing any intratumor oxygen measurements and after the anesthetic induction, each patient´s haemoglobin oxygen saturation (SaO2), body temperature, and ventilator-determined inspired fraction of oxygen (FiO2) were checked. Moreover, arterial blood gasometry was extracted to obtain the oxygen pressure (mmHg) values in the blood at the moment of micro-oxygenation measurement. To acquire the pO2mt for each fraction, micro-oxygenation probes were placed into i) soft free mucin lakes in each of the abdominal quadrants, ii) hard mucin identified by regions, and iii) healthy tissue, for instance, colon or abdominal wall. The probe was placed gently inserted, trying to cover the tip of the fiber sensor with surrounding tissue. We waited at least 15 seconds for a consistent value to be seen before deciding on it as the pO2mt of that area. The procedure method is supported by video media material (Supplemental videos 1-5).
2.2 Sample processing for molecular determinations
Soft mucin samples (~2mL) were homogenized in 4mL of lysis buffer [50mM Tris HCl pH 7.5, 1mM thylenediaminetetraacetic acid (EDTA) and 1mM phenylmethylsulfonyl fluoride (PMSF)] and control and hard mucin samples (~2g) were homogenized in 8mL of lysis buffer, using Potter homogenizers kept on ice in all cases. After homogenization, samples were vacuum filtered with Whatman filter paper before proceeding to a centrifugation phase (16000g, 15min, 4°C). Finally, supernatants were collected, aliquoted and stored at -20°C until assays were performed.
2.3 Catalase enzymatic assay
A 20mM KH2PO4 buffer (pH 7) and a 1M solution of H2O2 in KH2PO4 buffer were used to determine catalase activity (23). A water bath set at 30°C with circulating water, coupled to a spectrophotometer set at 240nm, was also required. For each sample, 970μL of KH2PO4 buffer, 20μL of 1M H2O2 solution and 10μL of supernatant obtained after sample processing, were added to a cuvette and mixed by inversion. The protocol established for this assay was to measure the absorbance (in triplicate) every 5s for 60s, yielding a unique value of ΔAbs/min per sample. The obtained values were used to calculate total enzymatic activity. Then, those results were extrapolated to get the specific catalase activity for each sample taking into account the protein quantification.
2.4 Western blot
The protein concentration of the samples was determined using the Bradford assay (Bio-Rad) (24). Then, 25μg of total protein was subjected to SDS-PAGE on 12% polyacrylamide gels, electro-transferred on polyvinylidene difluoride membranes (Millipore) using the Trans-Blot Turbo system (Bio-Rad), and incubated overnight at 4°C with the corresponding primary antibody [anti-GAPDH (1/100000; Abcam) and anti-HIF-1α (1/500; Cell Signaling)]. After primary antibody incubation, membranes were incubated with horseradish peroxidase-conjugated secondary antibody [anti-rabbit (1/5000; Abcam)]. For protein detection, chemiluminescence ECL Western Blotting Substrate (Thermo Scientific) was used. Protein levels were normalized using TPN (Total Protein Normalization) method, which calculates the intensity value of total protein from each sample. Densitometric analysis of protein bands was conducted using ImageJ.
2.5 Statistics
Statistical analyses were performed using Prism software v.7.0 (GraphPad Software, La Jolla, CA, USA). Data were assessed for normality using Kolmogorov-Smirnov test, then evaluated using appropriate parametric (unpaired t test or one-way ANOVA followed by post hoc Tukey test) or nonparametric (Mann-Whitney test) tests. P-values less than 0.05 were considered significant. Asterisks (* p< 0.05, ** p< 0.01, *** p< 0.001) indicate statistically significant differences.
3 Results
3.1 Intraoperative oxygen pressure in PMP
The pO2mt was measured intratumorally in soft free mucin, and hard mucin from all the patients included in the study, as well as in blood and surrounding healthy tissues. Interestingly, the pO2mt was clearly reduced in soft and hard mucin tissue compared with blood and healthy tissue in all patients. In patient 4, it was not possible to measure the pO2mt in soft mucin since the tumor was mainly constituted by hard mucin (Figure 1A). Additionally, the statistical analysis of all patients showed significant differences between soft and hard mucin compared with blood and healthy tissue (Figure 1B). Moreover, the O2 levels were maintained under normal parameters in all the patients as shown by FiO2 and SaO2 parameters (Table 1).
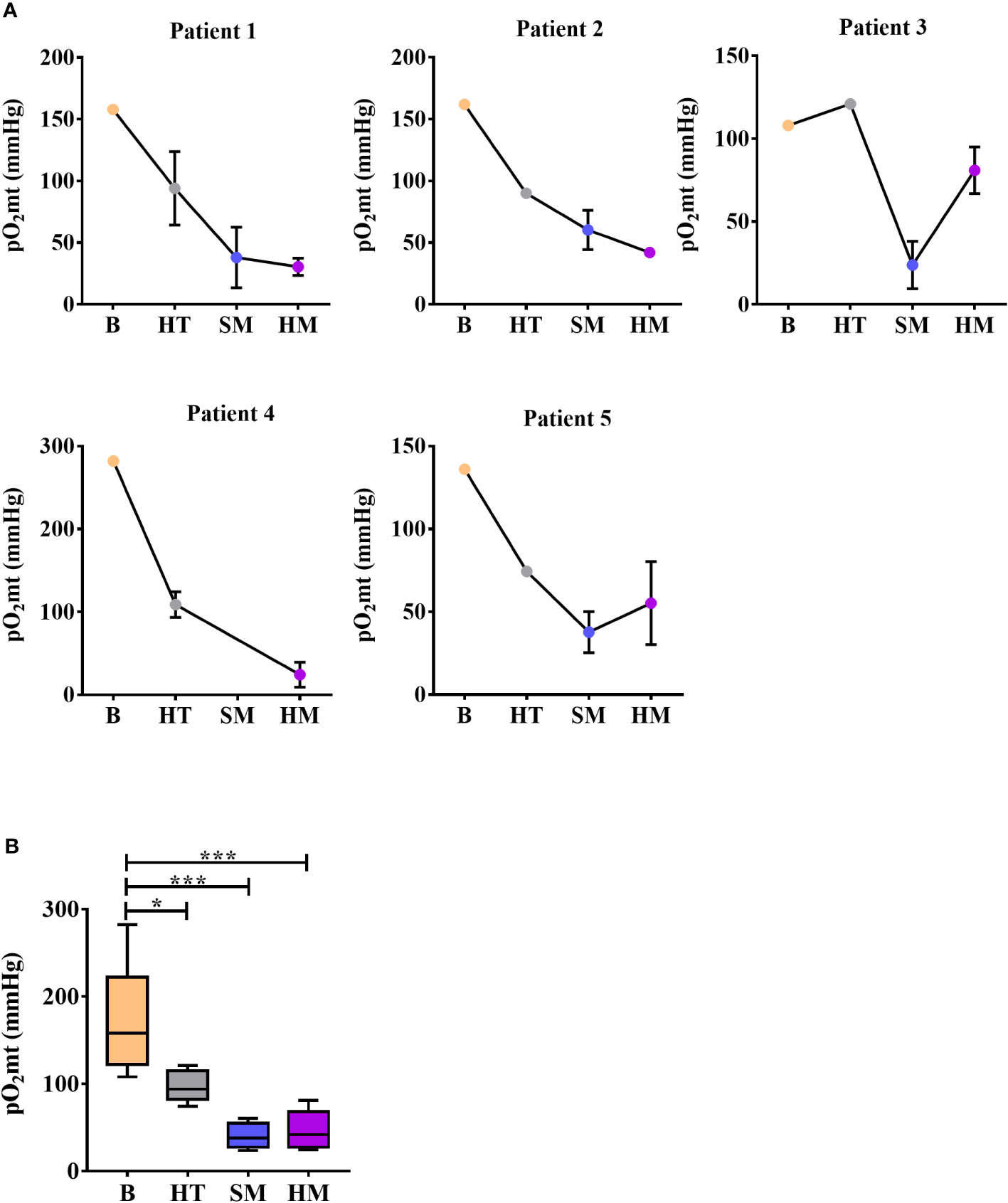
Figure 1 Measurement of intraoperative real-time oxygen microtension (pO2mt) in blood and tissues during surgery. (A) Individual measurements of pO2mt (mmHg) in blood (B), surrounding healthy tissue (HT), soft mucin (SM) and hard mucin (HM) in each PMP patient (n=5). Measurements are represented as the mean ± S.E.M of all values measured per zone in each patient. (B) pO2mt (mmHg) levels per zone taking into account all patients included in the study (n=5). One-way ANOVA analysis was carried out with multiple comparisons. *p < 0.05, ***p < 0.001.
3.2 Determination of hypoxia and oxidative stress markers
Next, we measured hypoxia and oxidative stress markers in order to know if PMP develops not only due to the absence of oxygen but also due to an increase in ROS. In this sense, we found a clear increase in HIF-1α protein expression levels in soft and hard mucin compared with healthy tissue samples (Figure 2A), which supports the reduction of pO2mt observed in the patients. Additionally, we evaluated the catalase enzymatic activity as an oxidative stress marker in our patient´s samples. In this case, and in contrast with the HIF-1α results, we observed a significant reduction of catalase activity in soft and hard mucin compared with healthy tissue samples (Figure 2B).
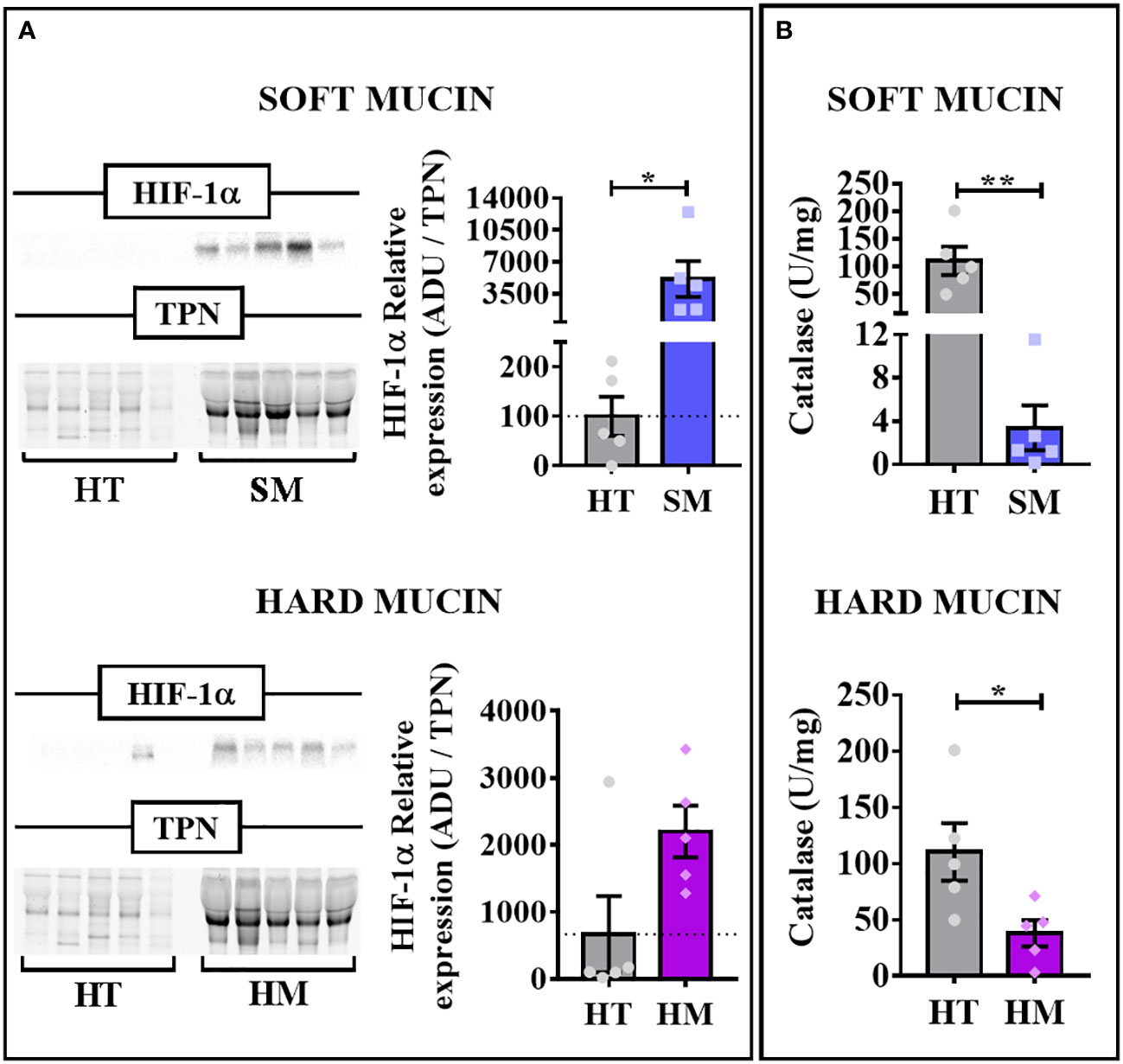
Figure 2 Cellular hypoxia and oxidative stress markers in PMP samples. (A) HIF-1α relative protein expression levels in soft (SM; n=5) and hard mucin (HM; n=5) compared with healthy tissues (HT; n=5; non-tumoral appendix and colon tissues) evaluated by Western Blot. The arbitrary densitometric unit (ADU) for the protein was normalized by the Total Protein Normalization (TPN) value. (B) Catalase activity (U/mg) in soft and hard mucin (n=5) compared to healthy control tissues (n=5; non-tumoral appendix and colon tissues) evaluated by enzymatic assay. Unpaired t test or Mann-Whitney test was used based on Kolmogorov-Smirnov normality test. *p < 0.05, **p < 0.01.
4 Discussion
The scarce research in PMP is mainly focused on the clinical management and classification of the disease, and the small number of articles exploring the PMP pathophysiology are based on histological data and expression analyses of genes already described in other types of cancer (25–27). In this context, it has been postulated that mucinous tumors, like PMP, might use the mucin barrier to configure a favorable local environment for tumor growth (28). In this study, we have demonstrated for the first time that the tumor tissue in PMP grows in hypoxic conditions increasing hypoxia markers such as HIF-1α. This finding is consistent with the fact that most solid malignant diseases show low oxygen levels (hypoxia) in the tumor environment (29–31), increasing the resistance to chemotherapy and radiotherapy.
Cellular adaptation to hypoxia is triggered by overexpression of HIF-1α via modulation of different signaling pathways to increase blood vessels formation, aggressiveness, metastasis, and resistance to treatments in numerous cancer types, such as breast, colorectal and pancreas cancer (17, 29, 31–35). Here, we measured HIF-1α protein levels to confirm the low levels of oxygen detected in vivo in PMP patients. We found a significant increase in HIF-1α protein levels in soft and hard mucin samples compared with healthy tissue samples. In line with these findings, Dilly et al. have shown an increase in HIF-1α protein levels in PMP tissues compared with normal colon samples, as well as an increase in HIF-1α levels in LS174T colon cancer cell line and PMP tissue explants following exposure to hypoxic conditions (18).
Moreover, HIF-1α has been reported to be modulated by hypoxia-independent factors, including ROS (29, 36). In this sense, we evaluated the CAT enzymatic activity as an oxidative stress marker in order to know if the hypoxic environment found in PMP is also linked to ROS. Thus, we have described a significant reduction in CAT enzymatic activity in soft and hard mucin compared with healthy tissue samples. Interestingly, CAT has been described as downregulated in several tumoral pathologies (37–41) while, at the same time, being elevated in other cancer subtypes (38, 42, 43). This discrepancy is due to its complex regulatory process. In this regard, CAT has been reported to be regulated at different levels: transcriptional (high number of transcription factors that induce or repress the transcriptional activity of CAT promoters), post-transcriptional (mRNA stability), and post-translational (phosphorylation and ubiquitination). Furthermore, epigenetic or genetic changes may have a role in regulating CAT activity (44).
Due to the harsh environment developed in mucinous tumors, which is characterized by hypoxic conditions, low pH, and no vascularization (45), conventional chemotherapy (e.g., bleomycin or doxorubicin, both oxygen-dependent drugs) and radiotherapy are ineffective in most cases (46–48). As a result, several research groups have concentrated on seeking suitable therapeutic options for oncologic patients suffering from this type of tumor by different means. Thus, Dilly et al. showed a reduction in MUC2 expression levels using a HIF-1α siRNA or specific HIF-1α inhibitors (BAY 87-2243 and YC-1) in LS174T cell line. Interestingly, chronic BAY 87-2243 treatment also reduced mucinous tumor growth in a PMP xenograft mouse model (18). Indeed, modulation of the HIF pathway at different levels has been proposed as a promising cancer therapy (49). Thus, transcription and translation have been demonstrated to be suppressed by different compounds, including aminoflavone, anthracyclines, steroids, or topoisomerase inhibitors. Remarkably, digoxigenin inhibited HIF-1α translation, increasing pancreatic cancer cells’ sensitivity to gemcitabine (49). Moreover, several histone deacetylase (HDAC) inhibitors, such as Panobinostat, MPT0G157, and Vorinostat, have been reported to reduce HIF-1α stability and induce its degradation in different tumoral pathologies (49). In line with this, Kim et al. reported the use of trichostatin A, a HDAC inhibitor, to downregulate HIF-1 and hypoxia-induced angiogenesis in an in vivo Lewis lung carcinoma model (50). Additionally, Kishimoto et al. proposed using hypoxia-activated prodrugs such as Evofosfamide in combination with radiotherapy and antiproliferative treatments to improve the clinical outcome of cancer patients (48).
On the other hand, harnessing ROS-induced oxidative stress via targeted inhibition of the cancer antioxidant defense machinery has been proposed as a potential anticancer strategy (51). In this sense, ATN-224 (choline tetrathiomolybdate) is an orally bioavailable inhibitor that inhibits SOD activity via copper chelation. This inhibitor has been used in phase I and II clinical trials and has been related to reduced angiogenesis and tumor proliferation (52, 53). In the same line, 4,5-dichloro-2-(3-tolyl)pyridazin3(2H)-one (LSC-1) has been related to the reduction of lung adenocarcinoma cell growth through SOD inhibition (54, 55). Furthermore, the combination of Capecitabine (a prodrug that is converted to 5-FU by thymidine phosphorylase) and Celecoxib (a Cox-2 inhibitor) reduced the number of mice with pancreatic adenocarcinoma, which was associated with a recovery of SOD and CAT activity (56).
In conclusion, this work provides the first evidence of low intratumoral oxygen levels in PMP patients during surgery, as well as an increase in HIF-1α protein levels together with low catalase activity, suggesting the presence of a hypoxic environment in these tumors. Further research is required to deeply understand the mechanisms underlying hypoxia and the potential role of oxidative stress in this pathology in order to identify new targets and strategies to treat this devastating disease.
Data availability statement
The original contributions presented in the study are included in the article/Supplementary Material. Further inquiries can be directed to the corresponding authors.
Ethics statement
The studies involving human participants including the use of OxyLiteTM systems were reviewed and approved by Cordoba Research Ethics Committee (protocol code PI19/01603). Written informed consent to participate in this study was provided by all the patients/participants.
Author contributions
Conception and design: AA-S, MV-B, AR-R; Development of methodology: AA-S, MV-B, AR-R, FV-M, FB, MG, CM, JA; Acquisition of samples and data: FV-M, BR-A, SR-P, AC-A, JS-H, LR-O; Analysis and interpretation of data: AA-S, MV-B, AR-R, FV-M, FB, CM, JA. All authors contributed to the article and approved the submitted version.
Funding
This work was supported by National Institute of Health Carlos III (ISCIII), Ministry of Science and Innovation. Reference: PI19/01603.
Conflict of interest
The authors declare that the research was conducted in the absence of any commercial or financial relationships that could be construed as a potential conflict of interest.
Publisher’s note
All claims expressed in this article are solely those of the authors and do not necessarily represent those of their affiliated organizations, or those of the publisher, the editors and the reviewers. Any product that may be evaluated in this article, or claim that may be made by its manufacturer, is not guaranteed or endorsed by the publisher.
Supplementary material
The Supplementary Material for this article can be found online at: https://www.frontiersin.org/articles/10.3389/fonc.2023.1076500/full#supplementary-material
References
1. Choudry HA, O’Malley ME, Guo ZS, Zeh HJ, Bartlett DL. Mucin as a therapeutic target in pseudomyxoma peritonei. J Surg Oncol (2012) 106(7):911–7. doi: 10.1002/jso.23146
2. Mall AS, Chirwa N, Govender D, Lotz Z, Tyler M, Rodrigues J, et al. MUC2, MUC5AC and MUC5B in the mucus of a patient with pseudomyxoma peritonei: biochemical and immunohistochemical study. Pathol Int (2007) 57(8):537–47. doi: 10.1111/j.1440-1827.2007.02137.x
3. Amini A, Masoumi-Moghaddam S, Ehteda A, Morris DL. Secreted mucins in pseudomyxoma peritonei: pathophysiological significance and potential therapeutic prospects. Orphanet J Rare Dis (2014) 9(1):71–1. doi: 10.1186/1750-1172-9-71
4. Carr NJ, Cecil TD, Mohamed F, Sobin LH, Sugarbaker PH, González-Moreno S, et al. A consensus for classification and pathologic reporting of pseudomyxoma peritonei and associated appendiceal neoplasia: The results of the peritoneal surface oncology group international (PSOGI) modified Delphi process. In Am J Surg Pathol. (2016) 40(1), 14–26. doi: 10.1097/PAS.0000000000000535
5. Dayal S, Taflampas P, Riss S, Chandrakumaran K, Cecil TD, Mohamed F, et al. Complete cytoreduction for pseudomyxoma peritonei is optimal but maximal tumor debulking may be beneficial in patients in whom complete tumor removal cannot be achieved. Dis Colon Rectum (2013) 56(12):1366–72. doi: 10.1097/DCR.0b013e3182a62b0d
6. Govaerts K, Lurvink RJ, Hingh IHJTD, der Speeten KV, Villeneuve L, Kusamura S, et al. Appendiceal tumours and pseudomyxoma peritonei: Literature review with PSOGI/EURACAN clinical practice guidelines for diagnosis and treatment. Eur J Surg Oncol (2020) 47(1), 11–35. doi: 10.1016/j.ejso.2020.02.012
7. Baratti D, Kusamura S, Milione M, Bruno F, Guaglio M, Deraco M. Validation of the recent PSOGI pathological classification of pseudomyxoma peritonei in a single-center series of 265 patients treated by cytoreductive surgery and hyperthermic intraperitoneal chemotherapy. Ann Surg Oncol (2017) 25(2):1–10. doi: 10.1245/s10434-017-6252-1
8. Rufián-Andujar B, Valenzuela-Molina F, Rufián-Peña S, Casado-Adam Á, Sánchez-Hidalgo JM, Rodríguez-Ortiz L, et al. From the ronnett to the PSOGI classification system for pseudomyxoma peritonei: A validation study. Ann Surg Oncol (2021) 33(Suppl 1):1–9. doi: 10.1245/s10434-020-09560-w
9. Ye S, Zheng S. Comprehensive understanding and evolutional therapeutic schemes for pseudomyxoma peritonei. Am J Clin Oncol (2022) 45(5):223–31. doi: 10.1097/COC.0000000000000911
10. Pillai K, Akhter J, Mekkawy A, Chua TC, Morris DL. Physical and chemical characteristics of mucin secreted by pseudomyxoma peritonei (PMP). Int J Med Sci (2017) 14(1):18–28. doi: 10.7150/ijms.16422
11. Li B, Huang Y, Ming H, Nice EC, Xuan R, Huang C. Redox control of the dormant cancer cell life cycle. Cells (2021) 10(10):2707. doi: 10.3390/cells10102707
12. Moloney JN, Cotter TG. ROS signalling in the biology of cancer. Semin Cell Dev Biol (2018) 80:50–64. doi: 10.1016/j.semcdb.2017.05.023
13. Ďuračková Z. Some current insights into oxidative stress. Physiol Res (2010) 59(4):459–69. doi: 10.33549/physiolres.931844
14. Assi M. The differential role of reactive oxygen species in early and late stages of cancer. Am J Physiol-Regul Integr Comp Physiol (2017) 313(6):R646–53. doi: 10.1152/ajpregu.00247.2017
15. Sajadimajd S, Khazaei M. Oxidative stress and cancer: The role of Nrf2. Curr Cancer Drug Tar. (2018) 18(6):538–57. doi: 10.2174/1568009617666171002144228
16. Hayes JD, Dinkova-Kostova AT, Tew KD. Oxidative stress in cancer. Cancer Cell (2020) 38(2):167–97. doi: 10.1016/j.ccell.2020.06.001
17. Chen PS, Chiu WT, Hsu PL, Lin SC, Peng IC, Wang CY, et al. Pathophysiological implications of hypoxia in human diseases. J BioMed Sci (2020) 27(1):63. doi: 10.1186/s12929-020-00658-7
18. Dilly AK, Lee YJ, Zeh HJ, Guo ZS, Bartlett DL, Choudry HA. Targeting hypoxia-mediated mucin 2 production as a therapeutic strategy for mucinous tumors. Transl Res (2016) 169:19–30.e1. doi: 10.1016/j.trsl.2015.10.006
19. Holekamp NM, Shui YB, Beebe D. Lower intraocular oxygen tension in diabetic patients: Possible contribution to decreased incidence of nuclear sclerotic cataract. Am J Ophthalmol (2006) 141(6):1027–32. doi: 10.1016/j.ajo.2006.01.016
20. Ciria R, Navarro E, Sánchez-Frías M, Gallardo A, Medina J, Ayllón M, et al. Preliminary results from the use of intraoperative real-time biliary oxygen monitoring in liver transplantation. Clin Transplant. (2018) 32(12):e13433. doi: 10.1111/ctr.13433
21. Sharifian M, Hosseini SA, Rasekhi A, Kazemi K. The use of micro pulse oximetery as a new detector of tissue perfusion in solid organ transplantation. Saudi J Kidney Dis Transplant. (2012) 23(4):715. doi: 10.4103/1319-2442.98144
22. Jonas R, Schaal T, Krimmel M, Gülicher D, Reinert S, Hoffmann J. Monitoring in microvascular tissue transfer by measurement of oxygen partial pressure: Four years experience with 125 microsurgical transplants. J Cranio Maxill Surg (2013) 41(4):303–9. doi: 10.1016/j.jcms.2012.10.008
23. Hadwan MH, Abed HN. Data supporting the spectrophotometric method for the estimation of catalase activity. Data Brief. (2015) 6:194–9. doi: 10.1016/j.dib.2015.12.012
24. Campion EM, Loughran ST, Walls D. Protein quantitation and analysis of purity. Methods Mol Biol Clifton N J (2016) 1485:225–55. doi: 10.1007/978-1-4939-6412-3_12
25. Lund-Andersen C, Torgunrud A, Fleten KG, Flatmark K. Omics analyses in peritoneal metastasis–utility in the management of peritoneal metastases from colorectal cancer and pseudomyxoma peritonei: a narrative review. J Gastrointest Oncol (2020) 12(S1):S191–203. doi: 10.21037/jgo-20-136
26. Alakus H, Babicky ML, Ghosh P, Yost S, Jepsen K, Dai Y, et al. Genome-wide mutational landscape of mucinous carcinomatosis peritonei of appendiceal origin. Genome Med (2014) 6(5):43–3. doi: 10.1186/gm559
27. Gleeson EM, Feldman R, Mapow BL, Mackovick LT, Ward KM, Morano WF, et al. Appendix-derived pseudomyxoma peritonei (PMP). Am J Clin Oncol (2018) 41(8):777–83. doi: 10.1097/COC.0000000000000376
28. Hollingsworth MA, Swanson BJ. Mucins in cancer: protection and control of the cell surface. Nat Rev Cancer (2004) 4(1):45–60. doi: 10.1038/nrc1251
29. Muz B, de la Puente P, Azab F, Azab AK. The role of hypoxia in cancer progression, angiogenesis, metastasis, and resistance to therapy. Adv Exp Med Biol (2015) 3:83–92. doi: 10.2147/HP.S93413
30. Wilson WR, Hay MP. Targeting hypoxia in cancer therapy. Nat Rev Cancer. (2011) 11(6):393–410. doi: 10.1038/nrc3064
31. Paredes F, Williams HC, Martin AS. Metabolic adaptation in hypoxia and cancer. Cancer Lett (2021) 502:133–42. doi: 10.1016/j.canlet.2020.12.020
32. Kim YI, Yi EJ, Kim YD, Lee AR, Chung J, Ha HC, et al. Local stabilization of hypoxia-inducible factor-1α controls intestinal inflammation via enhanced gut barrier function and immune regulation. Front Immunol (2021) 11:609689. doi: 10.3389/fimmu.2020.609689
33. Ebright RY, Zachariah MA, Micalizzi DS, Wittner BS, Niederhoffer KL, Nieman LT, et al. HIF1A signaling selectively supports proliferation of breast cancer in the brain. Nat Commun (2020) 11(1):6311. doi: 10.1038/s41467-020-20144-w
34. Xu K, Zhan Y, Yuan Z, Qiu Y, Wang H, Fan G, et al. Hypoxia induces drug resistance in colorectal cancer through the HIF-1α/miR-338-5p/IL-6 feedback loop. Mol Ther (2019) 27(10):1810–24. doi: 10.1016/j.ymthe.2019.05.017
35. Tiwari A, Tashiro K, Dixit A, Soni A, Vogel K, Hall B, et al. Loss of HIF1A from pancreatic cancer cells increases expression of PPP1R1B and degradation of p53 to promote invasion and metastasis. Gastroenterology (2020) 159(5):1882–1897.e5. doi: 10.1053/j.gastro.2020.07.046
36. Movafagh S, Crook S, Vo K. Regulation of hypoxia-inducible factor-1a by reactive oxygen Species : New developments in an old debate: Regulation of hypoxia-inducible factor-1a. J Cell Biochem (2015) 116(5):696–703. doi: 10.1002/jcb.25074
37. Baker AM, Oberley LW, Cohen MB. Expression of antioxidant enzymes in human prostatic adenocarcinoma. Prostate (1997) 32(4):229–33. doi: 10.1002/(SICI)1097-0045(19970901)32:4<229::AID-PROS1>3.0.CO;2-E
38. Chung-man Ho J, Zheng S, Comhair SA, Farver C, Erzurum SC. Differential expression of manganese superoxide dismutase and catalase in lung cancer. Cancer Res (2001) 61(23):8578–85.
39. Cullen JJ, Mitros FA, Oberley LW. Expression of antioxidant enzymes in diseases of the human pancreas: Another link between chronic pancreatitis and pancreatic cancer. Pancreas (2003) 26(1):23–7. doi: 10.1097/00006676-200301000-00005
40. Lauer C, Völkl A, Riedl S, Fahimi HD, Beier K. Impairment of peroxisomal biogenesis in human colon carcinoma. Carcinogenesis (1999) 20(6):985–9. doi: 10.1093/carcin/20.6.985
41. Marklund SL, Westman NG, Lundgren E, Roos G. Copper- and zinc-containing superoxide dismutase, manganese-containing superoxide dismutase, catalase, and glutathione peroxidase in normal and neoplastic human cell lines and normal human tissues. Cancer Res (1982) 42(5):1955–61.
42. Sander CS, Hamm F, Elsner P, Thiele JJ. Oxidative stress in malignant melanoma and non-melanoma skin cancer. Brit J Dermatol (2003) 148(5):913–22. doi: 10.1046/j.1365-2133.2003.05303.x
43. Rainis T, Maor I, Lanir A, Shnizer S, Lavy A. Enhanced oxidative stress and leucocyte activation in neoplastic tissues of the colon. Digest Dis Sci (2007) 52(2):526–30. doi: 10.1007/s10620-006-9177-2
44. Glorieux C, Calderon PB. Catalase, a remarkable enzyme: targeting the oldest antioxidant enzyme to find a new cancer treatment approach. Biol Chem (2017) 398(10):1095–108. doi: 10.1515/hsz-2017-0131
45. Khramtsov VV, Gillies RJ. Janus-faced tumor microenvironment and redox. Antioxid Redox Sign. (2014) 21(5):723–9. doi: 10.1089/ars.2014.5864
46. Martínez-Sánchez G, Giuliani A. Cellular redox status regulates hypoxia inducible factor-1 activity. role in tumour development. J Exp Clin Cancer Res Cr. (2007) 26(1):39–50.
47. Hyodo F, Davis RM, Hyodo E, Matsumoto S, Krishna MC, Mitchell JB. The relationship between tissue oxygenation and redox status using magnetic resonance imaging. Int J Oncol (2012) 41(6):2103–8. doi: 10.3892/ijo.2012.1638
48. Kishimoto S, Brender JR, Chandramouli GVR, Saida Y, Yamamoto K, Mitchell JB, et al. Hypoxia-activated prodrug evofosfamide treatment in pancreatic ductal adenocarcinoma xenografts alters the tumor redox status to potentiate radiotherapy. Antioxid Redox Sign. (2021) 35(11):904–15. doi: 10.1089/ars.2020.8131
49. Infantino V, Santarsiero A, Convertini P, Todisco S, Iacobazzi V. Cancer cell metabolism in hypoxia: Role of HIF-1 as key regulator and therapeutic target. Int J Mol Sci (2021) 22(11):5703. doi: 10.3390/ijms22115703
50. Kim MS, Kwon HJ, Lee YM, Baek JH, Jang JE, Lee SW, et al. Histone deacetylases induce angiogenesis by negative regulation of tumor suppressor genes. Nat Med (2001) 7(4):437–43. doi: 10.1038/86507
51. Gorrini C, Harris IS, Mak TW. Modulation of oxidative stress as an anticancer strategy. Nat Rev Drug Discovery (2013) 12(12):931–47. doi: 10.1038/nrd4002
52. Lowndes SA, Adams A, Timms A, Fisher N, Smythe J, Watt SM, et al. Phase I study of copper-binding agent ATN-224 in patients with advanced solid tumors. Clin Cancer Res (2008) 14(22):7526–34. doi: 10.1158/1078-0432.CCR-08-0315
53. Lin J, Beer TM, Ryan CJ, Mathew P, Wilding G, Morris M, et al. A randomized, phase II study of ATN-224 in patients with biochemically relapsed, hormone-naive prostate cancer: A DOD/PCF prostate cancer clinical trials consortium trial. J Clin Oncol (2009) 27(15_suppl):5135–5. doi: 10.1200/jco.2009.27.15_suppl.5135
54. Somwar R, Shum D, Djaballah H, Varmus H. Identification and preliminary characterization of novel small molecules that inhibit growth of human lung adenocarcinoma cells. J Biomol Screen. (2009) 14(10):1176–84. doi: 10.1177/1087057109350919
55. Somwar R, Erdjument-Bromage H, Larsson E, Shum D, Lockwood WW, Yang G, et al. Superoxide dismutase 1 (SOD1) is a target for a small molecule identified in a screen for inhibitors of the growth of lung adenocarcinoma cell lines. Proc Natl Acad Sci (2011) 108(39):16375–80. doi: 10.1073/pnas.1113554108
Keywords: Pseudomyxoma peritonei, mucin, oxygen, hypoxia, HIF-1α, catalase, ROS
Citation: Valenzuela-Molina F, Bura FI, Vázquez-Borrego MC, Granados-Rodríguez M, Rufián-Andujar B, Rufián-Peña S, Casado-Adam Á, Sánchez-Hidalgo JM, Rodríguez-Ortiz L, Ortega-Salas R, Martínez-López A, Michán C, Alhama J, Arjona-Sánchez Á and Romero-Ruiz A (2023) Intraoperative oxygen tension and redox homeostasis in Pseudomyxoma peritonei: A short case series. Front. Oncol. 13:1076500. doi: 10.3389/fonc.2023.1076500
Received: 21 October 2022; Accepted: 11 January 2023;
Published: 26 January 2023.
Edited by:
Wim Ceelen, Ghent University, BelgiumReviewed by:
Dario Baratti, Fondazione IRCCS Istituto Nazionale Tumori, ItalyMichela Giulii Capponi, Santo Spirito in Sassia Hospital, Italy
Gianni Lazzarin, Abano Terme Hospital, Italy
Copyright © 2023 Valenzuela-Molina, Bura, Vázquez-Borrego, Granados-Rodríguez, Rufián-Andujar, Rufián-Peña, Casado-Adam, Sánchez-Hidalgo, Rodríguez-Ortiz, Ortega-Salas, Martínez-López, Michán, Alhama, Arjona-Sánchez and Romero-Ruiz. This is an open-access article distributed under the terms of the Creative Commons Attribution License (CC BY). The use, distribution or reproduction in other forums is permitted, provided the original author(s) and the copyright owner(s) are credited and that the original publication in this journal is cited, in accordance with accepted academic practice. No use, distribution or reproduction is permitted which does not comply with these terms.
*Correspondence: Álvaro Arjona Sánchez, YWx2YXJvYXJqb25hQGhvdG1haWwuY29t; Mari C. Vázquez Borrego, bWFydmF6Ym9yQGdtYWlsLmNvbQ==
†These authors have contributed equally to this work and share first authorship