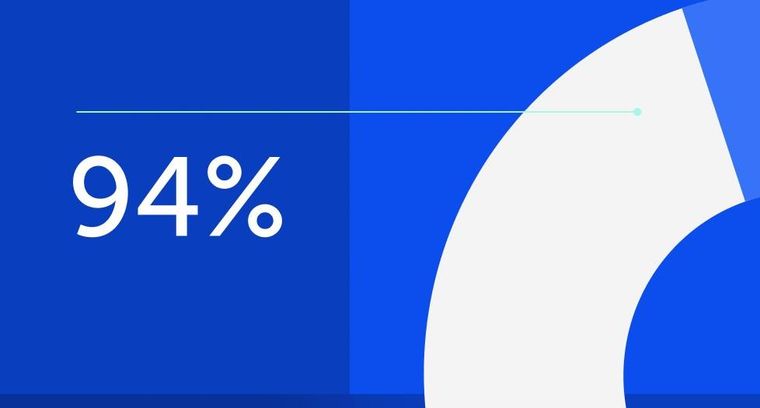
94% of researchers rate our articles as excellent or good
Learn more about the work of our research integrity team to safeguard the quality of each article we publish.
Find out more
ORIGINAL RESEARCH article
Front. Oncol., 16 June 2023
Sec. Skin Cancer
Volume 13 - 2023 | https://doi.org/10.3389/fonc.2023.1075823
This article is part of the Research TopicWomen in Skin Cancer Vol II: 2022View all 10 articles
Background: Pre-clinical studies have shown that metformin reduces intratumoral hypoxia, improves T-cell function, and increases sensitivity to PD-1 blockade, and metformin exposure has been associated with improved clinical outcomes in various types of cancer. However, the impact of this drug in diabetic melanoma patients has not yet been fully elucidated.
Methods: We reviewed 4,790 diabetic patients with stage I-IV cutaneous melanoma treated at the UPMC-Hillman Cancer Center and Memorial Sloan Kettering Cancer Center between 1996-2020. The primary endpoints included recurrence rates, progression free survival (PFS), and overall survival (OS) with and without metformin exposure. Tabulated variables included BRAF mutational status, immunotherapy (IMT) by type, and incidence of brain metastases.
Results: The five-year incidence of recurrence in stage I/II patients was significantly reduced with metformin exposure (32.3% vs 47.7%, p=0.012). The five-year recurrence rate for stage III patients was also significantly reduced (58.3% vs 77.3%, p=0.013) in the metformin cohort. OS was numerically increased in nearly all stages exposed to metformin, though this did not reach statistical significance. The incidence of brain metastases was significantly lower in the metformin cohort (8.9% vs 14.6%, p=0.039).
Conclusion: This is the first study to demonstrate significantly improved clinical outcomes in diabetic melanoma patients exposed to metformin. Overall, these results provide further rationale for ongoing clinical trials studying the potential augmentation of checkpoint blockade with metformin in advanced melanoma.
Melanoma is the fifth most common cancer diagnosed in the United States, with an incidence that continues to rise (1). Most patients have localized disease with excellent survival outcomes; however, five-year survival rates dramatically decrease for patients with locoregionally advanced or metastatic disease (1). The current era of immunotherapy (IMT) has revolutionized the treatment of advanced melanoma in both the adjuvant and metastatic setting (2–5), though validated predictive biomarkers are lacking to date (6). Metformin, a commonly utilized type II diabetes drug, has been shown to metabolically reprogram the tumor microenvironment (TME) (7), and in pre-clinical models, to augment the effectiveness of anti-programmed cell death protein 1 (PD1) IMT (8). The purpose of this study is to examine the impact of metformin on the clinical outcomes of diabetic patients with melanoma.
Several retrospective studies of various cancer types have demonstrated improved clinical outcomes in patients taking metformin (9). An analysis of over 300 diabetic patients with endometrial cancer revealed improved progression free survival (PFS) (HR 0.59, p=0.01) and overall survival (OS) (HR 0.43, p=0.005) in patients taking metformin versus those not exposed to the drug (10). A study of 302 diabetic patients with pancreatic cancer also demonstrated improved OS when comparing patients with and without metformin exposure (HR 0.68, p=0.003) (11). A 2018 retrospective study of 55 patients with unresectable stage IIIC and stage IV melanoma patients treated with IMT showed improved overall response rate (ORR) and PFS in patients exposed to metformin (12), though these results were not statistically significant. Furthermore, this study only included patients with advanced stage disease, and compared diabetic patients treated with metformin with non-diabetic patients who were thus not exposed to metformin. We therefore sought to analyze a large cohort of diabetic melanoma patients across all stages of disease to assess the association between metformin exposure and recurrence rates, PFS, OS, and incidence of brain metastases, among other variables.
A variety of mechanisms have been proposed to explain the improved clinical outcomes in cancer patients with metformin exposure. Many of these hypotheses center around metabolic alterations of the TME, which is understood to have important implications for tumor infiltrating lymphocytes (TIL), and thus, clinical outcomes with IMT (13). Metformin has been shown to activate a variety of both AMP-activated protein kinase (AMPK)-dependent and independent cellular signaling pathways that may alter the metabolic milieu of the TME and corresponding T-cell function (14). Metformin alters pro-inflammatory cytokine signaling in the TME, thereby rescuing exhausted CD8+ TILs and promoting anti-tumor effects (7, 15). Additionally, metformin was found to inhibit PD-L1 signaling via endoplasmic reticulum associated degradation; an effectual checkpoint blockade further enhancing TIL function (16). Metformin-mediated metabolic shifts have also been shown to inhibit regulatory T cells (Treg) in the TME and may also enhance immunity via alteration in the microbiome (17, 18). Furthermore, the association between metformin, a known complex I inhibitor, and hypoxia reversal in the TME is of significant interest, as it is now understood that the impact of hypoxia on immune function is largely detrimental. Hypoxia can induce an immunosuppressive state via enhanced Treg function and inhibitory T-cell receptor signaling (19). Hypoxic signaling can also lead to TIL dysfunction and phenotypic exhaustion, with important clinical implications for immune-based therapy (20, 21).
Notably, the related and previously studied biguanide, phenformin, has been shown to be a more potent mitochondrial inhibitor as compared to metformin (22). While both biguanides have a similar mechanism of action related to AMPK activation and oxidative phosphorylation (ox-phos) inhibition, phenformin has been shown to reduce tumorigenesis to a greater degree in some pre-clinical murine models (23). Additional pre-clinical work suggests that phenformin mediated AMPK activation can directly inhibit the mitogen-activated protein kinase (MAPK) pathway and provide a synergistic effect in combination with BRAF/MEK inhibitors in melanoma tumors with BRAF V600E/K mutations (24). Given these findings, an ongoing phase I clinical trial (NCT03026517) aims to assess the safety and efficacy of phenformin plus BRAF and MEK inhibitors in patients with BRAF mutant advanced melanoma. Apart from this trial, phenformin has been largely withdrawn from clinical use based on prior reports linking phenformin with higher rates of lactic acidosis and thus was not included in this retrospective study.
We and others have reported that the metabolic landscape of the TME is innately immunosuppressive (25, 26). Deregulated metabolism of tumor cells results in both a lack of nutrients such as glucose and oxygen, and buildup of toxic byproducts such as lactic acid. We previously demonstrated that melanoma patient tumor cells can be metabolically profiled directly from biopsies, and that deregulation of metabolism in tumor cells reveals insight into the status of the antitumor immune response to checkpoint blockade (27). Specifically, we showed that oxidative tumor cell metabolism is linked to resistance to anti-PD1 IMT; TIL isolated from melanoma tumors with high oxidative metabolism are more exhausted and less functional. High oxidative metabolism in tumor cells and the consequent generation of tumor hypoxia is associated with resistance to anti-PD1 and worse clinical outcomes, including decreased PFS, duration of response (DOR), and OS (27). We therefore developed a murine melanoma model to evaluate the impact of complex I inhibition on intra-tumoral hypoxia and T-cell function (27). Tumors from mice with complex I knock-down showed reduced ox-phos, improved T cell function, and decreased T cell exhaustion. Furthermore, only the complex I knock-down models showed response to anti-PD1, whereas GLUT1 knock down did not impact responses to IMT, suggesting that oxidative metabolism is implicated in resistance to anti-PD1 based IMT (27). Given these findings, we sought to evaluate actual clinical outcomes via this large retrospective analysis of diabetic melanoma patients with and without metformin exposure.
A retrospective cohort study was conducted at the UPMC-Hillman Cancer Center (HCC) and Memorial Sloan Kettering Cancer Center (MSKCC). With IRB approval from each institution, 4,790 charts were reviewed from patients seen between 1996-2020. Relevant charts were identified based on coding for the diagnoses of cutaneous melanoma and type 2 diabetes (T2DM). After initial review, 668 patients were found to have T2DM, with stage identified at initial diagnosis (Figure 1). This final subset was further categorized into metformin versus no metformin exposure and the key variables were tabulated for each patient, including: stage at initial diagnosis (AJCC 7 criteria), age at diagnosis, sex, BMI, performance status (Eastern Cooperative Oncology Group, ECOG), ulceration, BRAF mutational status, metformin exposure (yes/no), metformin dose, recurrence status, time to recurrence, use of IMT and indication (adjuvant/systemic), type of IMT (high dose interferon alpha-2b (HDI), high-dose IL-2, anti-PD1, anti-CTLA4, anti-PD1 plus anti-CTLA4), presence of brain metastases, date of last follow up and vital status. Histologic subtype was also collected but consistent data was not available in a large portion of our early patient records. Nondiabetic patients were not included in order to reduce confounding variables between metformin exposed and non-exposed patients.
Figure 1 Five-year recurrence rates stratified by stage and metformin exposure. *Rates of disease progression.
The primary objectives of this study included recurrence rates with and without metformin in patients with stage I-III melanoma, and PFS and OS in stage I-IV patients (with and without metformin). Secondary outcomes included recurrence and survival outcomes stratified by BMI, IMT treatment, and presence of brain metastases with or without metformin exposure. Recurrence rates were defined as the proportion of patients with documented recurrence (stage I-III) or disease progression (PD) (stage IV). PFS was defined as time in months from the diagnosis of melanoma to PD or death or being censored at the last follow-up. OS was defined as time in months from the diagnosis of melanoma to death or being censored at the last follow-up.
Comparison of recurrence and survival rates was performed using the Cox proportional hazards model. Normal continuous variables were tested using the t-test. Non-normal continuous variables were tested using the Wilcoxon rank-sum test. Categorical variables were tested using the Chi-squared test, with Fisher’s exact test being used for small counts. Crude recurrence rates between metformin groups were compared using the Fisher’s exact test. All analyses were performed using R version 4.1.1.
Patient demographics (n=668) are listed in Table 1. In total, 422 patients were treated with metformin and 246 patients were not. The mean age of the entire cohort was 64.2 years, and 68.2% were male. At initial diagnosis, 210 patients had stage I, 183 stage II, 195 stage III, and 80 patients had stage IV disease (AJCC 7). BRAFV600E/K mutation was found in 33.6% of the entire cohort, and this was not significantly different between the two groups. Most patients had an ECOG performance status of 0 or 1, and there was a higher proportion of ECOG 0 patients in the metformin cohort (55.8% vs 37.9%, p=0.001). Ulceration was present in 34.3% (n=169) of patients at diagnosis; this was not significantly different between the two groups. LDH levels at diagnosis were similar between the cohorts.
Adjuvant therapy was reported in 36.7% (n=112) of eligible patients; this was similar between the two groups, with HDI being most common. 52.4% (n=350) of patients were exposed to IMT, with the most common being HDI (n=108), anti-PD1 therapy (n=100), and anti-CTLA4 (n=44); this was similar between the metformin and no metformin groups.
The median metformin dose was 1000 mg daily (range 250-2000 mg; dose reported in 269 of 422 patients). Of 436 patients with BMI data, 39.7% were obese (BMI >30) with 60.3% non-obese (BMI ≤30). Other variables including years since T2DM diagnosis, mean Hgb A1C, utilization of other hypoglycemic agents, and duration of metformin therapy were not consistently available.
For the overall cohort (n=668), the five-year incidence of recurrence was significantly lower in patients exposed to metformin (43.8% vs 58.2, p=0.002). In a pooled cohort of patients with stage I or II melanoma (n=393), we again note that the five-year incidence of recurrence was significantly lower in patients exposed to metformin (32.3% vs 47.7%, p=0.012); this was still significant after adjusting for age, gender, and BMI (Figure 1 and Table 2). Evaluating individual stage cohorts for stage I and II, we find a consistent numerical increase in 5-year recurrence rates for patients not exposed to metformin, though this did not reach statistical significance (Table 2).
Similarly, the five-year recurrence rate in all stage III patients (n=195) was lower with metformin compared to no metformin exposure (58.3% vs 77.3%, p=0.013) (Table 2). In patients treated with adjuvant therapy (stage IIB-IIIC, n=112), the recurrence rate was lower 59.3% vs 67.6% with metformin exposure, though this did not reach statistical significance (p=0.42). The overall incidence of brain metastases (MBM) was significantly lower in the metformin cohort (8.9% vs 14.6%, p=0.039) (Table 1), though significant changes in survival were not seen in the MBM subgroup. A low BMI (<30) was marginally protective against recurrence in the metformin group (45.9% vs 64.0%, p=0.068) (Table 2). Regardless of metformin exposure, BRAF mutation was not associated with any difference in recurrence. Additionally, higher BMI was associated with a nonsignificant reduction in recurrence regardless of metformin status (36.5% vs 44.7%, p=0.089).
There was no significant difference in PFS between the metformin cohorts (Table 3 and S1). Patients with lower stage disease (I/II) were noted to have an OS hazard ratio of 0.563 (p=0.084) in the metformin group (Table 4 and S2). When stratified by higher BMI, patients exposed to metformin had an OS hazard ratio of 0.598 (p=0.076) (Table 4). All survival data were adjusted for age, sex, and BMI at diagnosis. Other covariates including BRAF mutation, ulceration, performance status, and adjuvant therapy were not included in the Cox regression models due to the relatively large proportion of missing data.
Table 3 Progression free survival hazard ratios (HR) for each stage and subgroup by metformin exposure.
Over the past decade, IMT has led to a groundbreaking improvement in clinical outcomes of patients with advanced melanoma, with 6.5 year OS for ipilimumab plus nivolumab of 49% (95% CI 44-55%) (28). However, a significant subset of patients develop primary or secondary resistance (29). Considerable effort is underway to better understand the mechanisms of resistance to IMT. It is now well understood that immunosuppressive and metabolically hostile TME, including decreased pH, altered amino acid metabolism, mitochondrial dysfunction, and hypoxia have substantial effects on the phenotype and function of TIL (8). This altered metabolic milieu of the TME may help to explain why only half of patients ultimately benefit from checkpoint blockade.
Seahorse cell analysis has been used to quantitatively measure oxidative phosphorylation (OCR) and glycolytic metabolism (ECAR) in melanoma tumor cells, and baseline tumor cell metabolism has implications on the TME, TIL function and clinical outcomes (8). Metformin reduced OCR in B16 bearing mice, and resulted in decreased intratumoral hypoxia. Furthermore, when mice were inoculated with a PD1 resistant melanoma cell line, the combination of metformin with anti-PD1 led to tumor regression in 80% of mice, whereas metformin or anti-PD1 monotherapy showed minimal anti-tumor efficacy, suggesting a synergistic effect on T-cell function in the TME (8). We have also shown that high oxidative metabolism in patient derived melanoma tumor cells is associated with decreased function and increased exhaustion of TIL, with significantly worse clinical outcomes, suggesting that high oxidative metabolism in melanoma tumor cells is associated with resistance to anti-PD1 (27). Furthermore, an experimental ox-phos inhibitor, IACS-010759, has shown improved survival in a pre-clinical murine MBM model (30).
While these pre-clinical models show that metformin has a beneficial impact on TIL function, our present clinical data shows a notable improvement in clinical outcomes for diabetic melanoma patients taking metformin. In the metformin group, we note a significant reduction in 5-year recurrence rates (stages I-III) and rates of PD (stage IV) for patients in the overall cohort, and also for patients with stage I/II and stage III disease. The finding of a numerical increase in 5-year recurrence in patients not on metformin may not have reached statistical significance in the individual stage I and II cohorts due to a smaller sample size. The significant reduction in recurrence rates noted here for the overall cohort, and for stage I/II patients taking metformin is certainly of interest, albeit difficult to attribute to any single metabolic change that might derive from metformin’s multiple downstream signaling effects. Based on pre-clinical data (8, 27), one may hypothesize that patients with early stage melanoma taking metformin have a more favorable TME and more efficient TIL anti-tumor activity at a critically early stage of pathogenesis. A similar study of 242 diabetic gastric cancer patients with or without metformin exposure showed significantly improved survival in only the localized (N0) subgroup, promoting this hypothesis in early stage disease (31). Our study also showed decreased 5-year recurrence in patients with stage III melanoma who took metformin. Overall, these findings in the context of our pre-clinical work suggest a more concerted and targeted metabolic effect secondary to metformin exposure (e.g. reduction in tumor cell oxidative metabolism improved TIL function, and reversal of hypoxia) (8, 25), though definitive correlation would require tumor-derived biomarker assessment. The decrease in recurrence rates for metformin-exposed stage III patients treated with or without IMT was not significantly improved with IMT, potentially due to the relatively small number of patients in this subgroup. Additionally, while there was an overall trend towards improved survival across all stages, patients with metastatic disease at diagnosis (n=80) did not exhibit any difference in survival with metformin exposure, though this was relatively small cohort. Further, patients may be less likely to derive benefit from metformin at more advanced stages of disease, when the drug is less able to significantly impact the TME due to nutrient competition, restricted drug delivery, and multiple acquired mechanisms of resistance.
Metformin exposure was associated with reduced incidence of brain metastases in this study. Our data aligns with previous work demonstrating improved OS in a MBM murine model after administration of an ox-phos inhibitor (30). In correlation, a recent gene expression analysis of intracranial and extracranial melanoma metastases revealed a significant increase in ox-phos expression along with immunosuppression in melanoma brain metastases (30), suggesting a selective pressure favoring the outgrowth of highly oxidative hypermetabolic clones. Given the biochemical properties permitting metformin to cross the blood-brain barrier, high prevalence of brain metastases in melanoma, and association with increased morbidity and mortality, these studies provide grounds for ongoing translational research in the field of immunometabolism (32, 33).
Notably, our data showing significantly improved recurrence rates with metformin exposure did not correlate with survival outcomes. Given the retrospective nature of this study with some of the patient data originating from over two decades ago, there are significant confounding factors that likely affected differential survival in these patient cohorts. Namely, improvements in SOC treatments leading to improved overall survival may have over time diluted metformin-mediated survival advantages. Regardless, after adjusting for various baseline characteristics, patients with stage I-II melanoma who were exposed to metformin had reduced recurrence rates. Though tissue biomarkers were not available in this retrospective study, our prior data suggests a biological shift towards an anti-tumor phenotype of more functional TILs with critical relevance towards combatting the immunosuppressive nature of the TME (8, 25).
Hahn et al. have shown that obesity is associated with both improved survival and reduced ox-phos in metastatic melanoma (34), and we therefore assessed obesity related outcomes in our patient population. While lower BMI was marginally protective against recurrence in the metformin cohort, survival outcomes suggested the alternative. Patients with an elevated BMI and metformin exposure had a lower OS hazard ratio. Further, a nonsignificant reduction in recurrence was seen in patients with higher BMI, regardless of metformin exposure. Taken together, these data suggest reduced ox-phos, potentially via metabolic changes induced through obesity or metformin, could lead to improved outcomes in patients with melanoma (35).
Our retrospective study has several limitations inherent to its nature. Conclusions drawn are based on data available in the electronic medical record. However, all the patients included in this cohort were seen at regular intervals for routine follow-up, and information on demographics and clinical outcomes such as response rates, treatment utilized, and recurrence was readily available. Furthermore, data on response to therapy was based on investigator assessment, which may increase variability with regards to this outcome. Our sample size for patients with stage III treated with adjuvant immunotherapy precludes our ability to draw clear conclusions in this patient population. Similarly, our cohort size for stage IV was smaller than for patients with earlier stage melanoma, as would be expected. Most covariates were well balanced between the two cohorts. However, a significantly higher proportion of patients with an ECOG 0 or 1 were found in the metformin exposed group, a potential confounding variable. Further, more patients in the metformin cohort had received adjuvant therapy, though this did not reach statistical significance. Despite its limitations, this analysis generates a hypothesis of clinically improved outcomes in diabetic melanoma patients treated with metformin, compared to diabetic melanoma patients not exposed to this drug. This is in line with reports of metformin in other malignancies and is confirmatory of our pre-clinical findings. In addition, we screened nearly 5,000 patients for inclusion in this study, resulting in a considerable cohort size of diabetic melanoma patients treated across two tertiary medical centers.
Overall, these data provide further rationale for prospectively investigating the role of metformin in the treatment of advanced melanoma and/or prevention of recurrence in the adjuvant setting. A prospective translational trial investigating anti-PD1 with or without metformin is underway (NCT03311308) and will assess the role of decreasing tumor cell ox-phos and reversing hypoxia in the TME together with checkpoint inhibition for the treatment of advanced melanoma.
The original contributions presented in the study are included in the article/Supplementary Material. Further inquiries can be directed to the corresponding author.
The studies involving human participants were reviewed and approved by University of Pittsburgh IRB Committee. Written informed consent for participation was not required for this study in accordance with the national legislation and the institutional requirements.
RA: Data collection, analysis, manuscript writing ZH, FD, SZ, JM, AS, MD, CS: Data collection, statistical support DD, JM, GD: Editorial support AW, YN: project design, analysis, editorial oversight. All authors contributed to the article and approved the submitted version.
This project used the UPMC Hillman Cancer Center Bioinformatics Core that is supported in part by award P30CA047904. ABW is supported in part by P30CA08747. This project was supported in part by the Hillman Fellows for Innovative Cancer Research Program funded by the Henry L. Hillman Foundation. This research was supported in part by the University of Pittsburgh UPMC Hillman Cancer Center award P30CA04904.
DD: Grant/research support: Bristol Meyers Squibb, Checkmate, Merck, GlaskoSmith Kline, Tesaro, Amgen
Honoraria: GlaxoSmith Kline, Tesaro, Instil Bio, Array Biopharma. Advisory board: GlaxoSmith Kline. JK: Grant/research support and/or consultant: BMS, Merck, Novartis, Roche, Genentech, EMD, Serono, Array Biopharma, Prometheus. GD: Grant/research support: Pfizer, Bluebirdbio, TCR2 Therapeutics. Consultant: TTMS, Pieris Pharmaceuticals. AW: Consulting/advisory: Nanobiotix, Iovance, Novartis, Shanghai Jo’Ann Medical Technology, BluePath Solutions, Pfizer, Instil Bio. YGN: Grant/research support: Merck, Bristol Meyers Squibb, Pfizer, Replimune. Consulting/Advisory board: Array Biopharma, InterVenn Bio, Merck, Novartis, Pfizer, BMS and Immunocore. Non-CE Honoraria: Pfizer, Immuncore.
The remaining authors declare that the research was conducted in the absence of any commercial or financial relationships that could be construed as a potential conflict of interest.
All claims expressed in this article are solely those of the authors and do not necessarily represent those of their affiliated organizations, or those of the publisher, the editors and the reviewers. Any product that may be evaluated in this article, or claim that may be made by its manufacturer, is not guaranteed or endorsed by the publisher.
The Supplementary Material for this article can be found online at: https://www.frontiersin.org/articles/10.3389/fonc.2023.1075823/full#supplementary-material
1. Melanoma of the skin {{/amp]]mdash; cancer stat facts. Available at: https://seer.cancer.gov/statfacts/html/melan.html (Accessed October 22, 2021).
2. Larkin J, Chiarion-Sileni V, Gonzalez R, Grob J-J, Rutkowski P, Lao CD, et al. Five-year survival with combined nivolumab and ipilimumab in advanced melanoma. N Engl J Med (2019) 381(16):1535–46. doi: 10.1056/NEJMoa1910836
3. Robert C, Ribas A, Schachter J, Arance A, Grob J-J, Mortier L, et al. Pembrolizumab versus ipilimumab in advanced melanoma (KEYNOTE-006): post-hoc 5-year results from an open-label, multicentre, randomised, controlled, phase 3 study. Lancet Oncol (2019) 20(9):1239–51. doi: 10.1016/S1470-2045(19)30388-2
4. Eggermont AMM, Blank CU, Mandalà M, Long GV, Atkinson VG, Dalle S, et al. Adjuvant pembrolizumab versus placebo in resected stage III melanoma (EORTC 1325-MG/KEYNOTE-054): distant metastasis-free survival results from a double-blind, randomised, controlled, phase 3 trial. Lancet Oncol (2021) 22(5):643–54. doi: 10.1016/S1470-2045(21)00065-6
5. Weber J, Mandala M, Del Vecchio M, Gogas HJ, Arance AM, Cowey CL, et al. Adjuvant nivolumab versus ipilimumab in resected stage III or IV melanoma. N Engl J Med (2017) 377(19):1824–35. doi: 10.1056/NEJMoa1709030
6. Nowicki TS, Hu-Lieskovan S, Ribas A. Mechanisms of resistance to PD-1 and PD-L1 blockade. Cancer J (2018) 24(1):47–53. doi: 10.1097/PPO.0000000000000303
7. Eikawa S, Nishida M, Mizukami S, Yamazaki C, Nakayama E, Udono H. Immune-mediated antitumor effect by type 2 diabetes drug, metformin. Proc Natl Acad Sci USA (2015) 112(6):1809–14. doi: 10.1073/pnas.1417636112
8. Scharping NE, Menk AV, Whetstone RD, Zeng X, Delgoffe GM. Efficacy of PD-1 blockade is potentiated by metformin-induced reduction of tumor hypoxia. Cancer Immunol Res (2017) 5(1):9–16. doi: 10.1158/2326-6066.CIR-16-0103
9. Dulskas A, Patasius A, Linkeviciute-Ulinskiene D, Zabuliene L, Urbonas V, Smailyte G. Metformin increases cancer specific survival in colorectal cancer patients-national cohort study. Cancer Epidemiol (2019) 62:101587. doi: 10.1016/j.canep.2019.101587
10. Ko EM, Walter P, Jackson A, Clark L, Franasiak J, Bolac C, et al. Metformin is associated with improved survival in endometrial cancer. Gynecol Oncol (2014) 132(2):438–42. doi: 10.1016/j.ygyno.2013.11.021
11. Sadeghi N, Abbruzzese JL, Yeung S-CJ, Hassan M, Li D. Metformin use is associated with better survival of diabetic patients with pancreatic cancer. Clin Cancer Res (2012) 18(10):2905–12. doi: 10.1158/1078-0432.CCR-11-2994
12. Afzal MZ, Mercado RR, Shirai K. Efficacy of metformin in combination with immune checkpoint inhibitors (anti-PD-1/anti-CTLA-4) in metastatic malignant melanoma. J Immunother Cancer (2018) 6(1):64. doi: 10.1186/s40425-018-0375-1
13. Allison KE, Coomber BL, Bridle BW. Metabolic reprogramming in the tumour microenvironment: a hallmark shared by cancer cells and T lymphocytes. Immunology (2017) 152(2):175–84. doi: 10.1111/imm.12777
14. Verdura S, Cuyàs E, Martin-Castillo B, Menendez JA. Metformin as an archetype immuno-metabolic adjuvant for cancer immunotherapy. Oncoimmunology (2019) 8(10):e1633235. doi: 10.1080/2162402X.2019.1633235
15. Hirsch HA, Iliopoulos D, Struhl K. Metformin inhibits the inflammatory response associated with cellular transformation and cancer stem cell growth. Proc Natl Acad Sci USA (2013) 110(3):972–7. doi: 10.1073/pnas.1221055110
16. Cha J-H, Yang W-H, Xia W, Wei Y, Chan L-C, Lim S-O, et al. Metformin promotes antitumor immunity via endoplasmic-Reticulum-Associated degradation of PD-L1. Mol Cell (2018) 71(4):606–620.e7. doi: 10.1016/j.molcel.2018.07.030
17. Kunisada Y, Eikawa S, Tomonobu N, Domae S, Uehara T, Hori S, et al. Attenuation of CD4+CD25+ regulatory T cells in the tumor microenvironment by metformin, a type 2 diabetes drug. EBioMedicine (2017) 25:154–64. doi: 10.1016/j.ebiom.2017.10.009
18. Pollak M. The effects of metformin on gut microbiota and the immune system as research frontiers. Diabetologia (2017) 60(9):1662–7. doi: 10.1007/s00125-017-4352-x
19. Shi LZ, Wang R, Huang G, Vogel P, Neale G, Green DR, et al. HIF1alpha-dependent glycolytic pathway orchestrates a metabolic checkpoint for the differentiation of TH17 and treg cells. J Exp Med (2011) 208(7):1367–76. doi: 10.1084/jem.20110278
20. Phan AT, Goldrath AW. Hypoxia-inducible factors regulate T cell metabolism and function. Mol Immunol (2015) 68(2 Pt C):527–35. doi: 10.1016/j.molimm.2015.08.004
21. Rivadeneira DB, Delgoffe GM. Antitumor T-cell reconditioning: improving metabolic fitness for optimal cancer immunotherapy. Clin Cancer Res (2018) 24(11):2473–81. doi: 10.1158/1078-0432.CCR-17-0894
22. Janzer A, German NJ, Gonzalez-Herrera KN, Asara JM, Haigis MC, Struhl K. Metformin and phenformin deplete tricarboxylic acid cycle and glycolytic intermediates during cell transformation and NTPs in cancer stem cells. Proc Natl Acad Sci USA (2014) 111(29):10574–9. doi: 10.1073/pnas.1409844111
23. Appleyard MVCL, Murray KE, Coates PJ, Wullschleger S, Bray SE, Kernohan NM, et al. Phenformin as prophylaxis and therapy in breast cancer xenografts. Br J Cancer (2012) 106(6):1117–22. doi: 10.1038/bjc.2012.56
24. Yuan P, Ito K, Perez-Lorenzo R, Guzzo CD, Lee JH, Shen C-H, et al. Phenformin enhances the therapeutic benefit of BRAF(V600E) inhibition in melanoma. Proc Natl Acad Sci USA (2013) 110(45):18226–31. doi: 10.1073/pnas.1317577110
25. Scharping NE, Menk AV, Moreci RS, Whetstone RD, Dadey RE, Watkins SC, et al. The tumor microenvironment represses T cell mitochondrial biogenesis to drive intratumoral T cell metabolic insufficiency and dysfunction. Immunity (2016) 45(2):374–88. doi: 10.1016/j.immuni.2016.07.009
26. Jiang Y, Li Y, Zhu B. T-Cell exhaustion in the tumor microenvironment. Cell Death Dis (2015) 6:e1792. doi: 10.1038/cddis.2015.162
27. Najjar YG, Menk AV, Sander C, Rao U, Karunamurthy A, Bhatia R, et al. Tumor cell oxidative metabolism as a barrier to PD-1 blockade immunotherapy in melanoma. JCI Insight (2019) 4(5):e124989. doi: 10.1172/jci.insight.124989
28. Wolchok JD, Chiarion-Sileni V, Gonzalez R, Grob J-J, Rutkowski P, Lao CD, et al. CheckMate 067: 6.5-year outcomes in patients (pts) with advanced melanoma. JCO (2021) 39(15_suppl):9506–6. doi: 10.1200/JCO.2021.39.15_suppl.9506
29. Kluger HM, Tawbi HA, Ascierto ML, Bowden M, Callahan MK, Cha E, et al. Defining tumor resistance to PD-1 pathway blockade: recommendations from the first meeting of the SITC immunotherapy resistance taskforce. J Immunother Cancer (2020) 8(1):e000398. doi: 10.1136/jitc-2019-000398
30. Fischer GM, Jalali A, Kircher DA, Lee W-C, McQuade JL, Haydu LE, et al. Molecular profiling reveals unique immune and metabolic features of melanoma brain metastases. Cancer Discovery (2019) 9(5):628–45. doi: 10.1158/2159-8290.CD-18-1489
31. Seo HS, Jung YJ, Kim JH, Lee HH, Park CH. The effect of metformin on prognosis in patients with locally advanced gastric cancer associated with type 2 diabetes mellitus. Am J Clin Oncol (2019) 42(12):909–17. doi: 10.1097/COC.0000000000000627
32. Davies MA, Liu P, McIntyre S, Kim KB, Papadopoulos N, Hwu W-J, et al. Prognostic factors for survival in melanoma patients with brain metastases. Cancer (2011) 117(8):1687–96. doi: 10.1002/cncr.25634
33. Łabuzek K, Suchy D, Gabryel B, Bielecka A, Liber S, Okopień B. Quantification of metformin by the HPLC method in brain regions, cerebrospinal fluid and plasma of rats treated with lipopolysaccharide. Pharmacol Rep (2010) 62(5):956–65. doi: 10.1016/S1734-1140(10)70357-1
34. Hahn AW, Xu M, Li J, Haydu LE, Khan MAW, Gide TN, et al. Abstract LB208: obesity is associated with lower?tumor oxidative phosphorylation (OXPhos) in metastatic melanoma (MM). In:?Molecular and cellular Biology/Genetics. Philadelphia (PA): American Association for Cancer Research (2021). p. LB208–8. doi: 10.1158/1538-7445.AM2021-LB208
Keywords: tumor microenvironment (TME), melanoma, checkpoint blockade, oxidative phosphorylation, tumor infiltrating lymphocyte, progression-free survival, metformin
Citation: Augustin RC, Huang Z, Ding F, Zhai S, McArdle J, Santisi A, Davis M, Sander C, Davar D, Kirkwood JM, Delgoffe GM, Warner AB and Najjar YG (2023) Metformin is associated with improved clinical outcomes in patients with melanoma: a retrospective, multi-institutional study. Front. Oncol. 13:1075823. doi: 10.3389/fonc.2023.1075823
Received: 21 October 2022; Accepted: 27 March 2023;
Published: 16 June 2023.
Edited by:
Selma Ugurel, Essen University Hospital, GermanyReviewed by:
Elizabeth Gaughan, University of Virginia, United StatesCopyright © 2023 Augustin, Huang, Ding, Zhai, McArdle, Santisi, Davis, Sander, Davar, Kirkwood, Delgoffe, Warner and Najjar. This is an open-access article distributed under the terms of the Creative Commons Attribution License (CC BY). The use, distribution or reproduction in other forums is permitted, provided the original author(s) and the copyright owner(s) are credited and that the original publication in this journal is cited, in accordance with accepted academic practice. No use, distribution or reproduction is permitted which does not comply with these terms.
*Correspondence: Yana G. Najjar, bmFqamFyeWdAdXBtYy5lZHU=
†These authors have contributed equally to this work
Disclaimer: All claims expressed in this article are solely those of the authors and do not necessarily represent those of their affiliated organizations, or those of the publisher, the editors and the reviewers. Any product that may be evaluated in this article or claim that may be made by its manufacturer is not guaranteed or endorsed by the publisher.
Research integrity at Frontiers
Learn more about the work of our research integrity team to safeguard the quality of each article we publish.