- 1Department of Nuclear Medicine and Radiobiology, Faculty of Medicine and Health Sciences, Université de Sherbrooke, Sherbrooke, QC, Canada
- 2Sherbrooke Molecular Imaging Center (CIMS), Centre de recherche du CHUS, Sherbrooke, QC, Canada
- 3Department of Pathology, Faculty of Medicine and Health Sciences, Université de Sherbrooke, Sherbrooke, QC, Canada
- 4Department of Surgery, Division of urology, Faculty of Medicine and Health Sciences, Université de Sherbrooke, Sherbrooke, QC, Canada
Introduction: We have previously shown that copper-64 (64Cu)-DOTHA2-PSMA can be used for positron emission tomography (PET) imaging of prostate cancer. Owing to the long-lasting, high tumoral uptake of 64Cu-DOTHA2-PSMA, the objective of the current study was to evaluate the therapeutic potential of 64Cu-DOTHA2-PSMA in vivo.
Methods: LNCaP tumor-bearing NOD-Rag1nullIL2rgnull (NRG) mice were treated with an intraveinous single-dose of 64Cu-DOTHA2-PSMA at maximal tolerated injected activity, natCu-DOTHA2-PSMA at equimolar amount (control) or lutetium-177 (177Lu)-PSMA-617 at 120 MBq to assess their impact on survival. Weight, well-being and tumor size were followed until mice reached 62 days post-injection or ethical limits. Toxicity was assessed through weight, red blood cells (RBCs) counts, pathology and dosimetry calculations.
Results: Survival was longer with 64Cu-DOTHA2-PSMA than with natCu-DOTHA2-PSMA (p < 0.001). Likewise, survival was also longer when compared to 177Lu-PSMA-617, although it did not reach statistical significance (p = 0.09). RBCs counts remained within normal range for the 64Cu-DOTHA2-PSMA group. 64Cu-DOTHA2-PSMA treated mice showed non-pathological fibrosis and no other signs of radiation injury. Human extrapolation of dosimetry yielded an effective dose of 3.14 × 10-2 mSv/MBq, with highest organs doses to gastrointestinal tract and liver.
Discussion: Collectively, our data showed that 64Cu-DOTHA2-PSMA-directed radioligand therapy was effective for the treatment of LNCaP tumor-bearing NRG mice with acceptable toxicity and dosimetry. The main potential challenge is the hepatic and gastrointestinal irradiation.
1. Introduction
Prostate cancer is the second most common solid organ cancer in men worldwide and one of the most frequent causes of death by cancer in North America (1–3). There is still a lasting need for treatment options that will lead to better survival and lower side effects in men with metastastic disease (4–6). One promising avenue is radioligand therapy (4–6), which is the intravenous administration of a radioligand that binds to local and distant cancer metastases to irradiate them, usually with beta- (β-) or alpha particles (7, 8). Affected organs and toxicity are dependent on the radioligand distribution (7, 8).
The prostate specific membrane antigen (PSMA) is an interesting target for radioligand therapy, given its important overexpression in most prostate cancer in comparison to normal tissue (8–10). Furthermore, PSMA small-size radioligands are internalized when bound, bringing them closer to the nucleus and thus, making DNA within reach of short-range decay particles like alpha particles and Auger electrons (7–9). Several radioligands have been proposed for PSMA radioligand therapy over the last decade, with small molecules showing the advantage of fast blood clearance over antibodies (9, 11). However, the modular nature of antibodies allowed the generation of smaller fragments, such as scFvs that can overcome some problems observed with antitbodies. Indeed, it has been shown that scFvs can offer appropriate tumor-to-background ratios for imaging and treating PSMA-expressing cancer (12).
The radioligand therapy current clinical arsenal is promising, even if there is still room for improvement. The small molecule PSMA-617 labeled with lutetium-177 (177Lu) (a low linear energy transfer (LET) β- emitter) has been reported to decrease tumoral burden of metastatic, castration resistant prostate cancer with limited systemic side effects (13–15). It was approved in March 2022 for its treatment by the U.S. Food and Drug Administration (16) Its use is limited by 177Lu’s reactor production and there is also a significant proportion of resistance and relapse reported (5, 6, 11). The use of short range (50 to 100 µm), high LET, such as alpha particles emitter actinium-225 (225Ac) coupled to PSMA-617, was suggested for patients non-admissible or with refractory disease to radioligand therapy with a β- emitter (5). Albeit studied in smaller cohorts, 225Ac-PSMA-617 proved efficacious (5, 17). Its use is limited given its association with long-lasting or severe xerostomia and limited current global availability of 225Ac (5, 17). Furthermore, actual therapeutic radioligands do not permit positron emission tomography (PET) imaging for patient selection, therapy planning, and follow-up. Hence, those radioligands are usually paired with similar diagnostic compounds such as gallium-68 (68Ga)-PSMA-617 (9, 10). However, the use of a different radiometal and sometimes different PSMA radioligands for theranostic applications may alter biodistribution and pharmacokinetics (18, 19).
In this project, we hypothesized that in addition to PET imaging, copper-64 (64Cu) could be of interest for radioligand therapy (20–22). 64Cu cyclotron production is easier than the production of 67Cu (20). The production route and mechanism of action (high LET Auger electron) of 64Cu feature a favourable supplement to therapeutic radionuclides such as 177Lu and 225Ac. Testing well-known (177Lu) and promising (64Cu) therapeutic radionuclides in a preclinical setting will allow us to select the most appropriate radioligand for optimal PSMA radioligand therapy. 64Cu has high LET, short range (~126 nm) Auger electron emissions (average energies (Eave) of 6.5 keV [22.7%] and 840 eV [57.4%]) and β− emissions (39% [maximal energy (Emax): 0.58 MeV, Eave: 0.19 MeV]) (20–22). These features offer therapeutic potential and possibly lower toxicity on non-target cells than exclusive or mainly β− emitters (7, 22). 64Cu radioligand therapy was proven effective in preclinical context against various cancer types (23–30). For theranostic applications, 64Cu’s β+ enables PET imaging. (17.4% β+ [Emax: 0.65 MeV, Eave: 0.28 MeV]). In this regard, we developed the radioligand 64Cu-DOTHA2-PSMA (Figure 1) to overcome 64Cu complexation challenges. Indeed, DOTHA2 offers fast complexation kinetics and high in vivo stability (31). It achieved high tumoral uptake up to 24h post-injection (p.i.) on preclinical PET imaging (31). 64Cu-DOTHA2-PSMA shown to be mainly cleared through the hepatobiliary pathway, which could be explained by its lipophilicity (log D = −0.96 ± 0.61) and its binding to plasma proteins; this was accompanied by a low kidney uptake and a fast-urinary clearance pattern (31). PSMA-617 radioligands have mainly urinary clearance with higher kidney uptake, especially at early time points (9).
The main goal of this study is to assess 64Cu-DOTHA2-PSMA’s therapeutic potential by determining its influence on survival and tumor size, as well as its general toxicity profile on organs of interest for PSMA radioligand therapy in comparison to control and to clinically used 177Lu-PSMA-617.
2. Materials and methods
2.1. Radioligand production
64Cu-DOTHA2-PSMA was synthesized, radiolabeled and characterized as previously described with a 116 ± 30 MBq/nmol molar activity (31).
177Lu-PSMA-617 preparation. The synthesis of the PSMA scaffold was achieved as previously described by Benešová et al. (32). Purity of the peptide was verified by HPLC and greater than 95% (Supplementary Figures 1 and 2). Both nuclear magnetic resonance spectroscopy and mass spectroscopy data were consistent with those reported in the literature (32). No-carrier added 177LuCl3 in 0.04 M HCl was obtained from Isotopia Molecular Imaging Ltd (Israël) and ITG Isotope Technologies Garching GmbH (Germany). Labeling of DOTA-PSMA was performed in 0.1 M ammonium acetate buffer pH 5.5 at 90°C for 15 min. Quality control of the drug was performed by Radio-TLC with sodium citrate buffer, pH 5.5, as eluent and always revealed labeling yields greater than 99% with an effective molar activity of 50 MBq/nmol. The radioligand solution was used without further purification steps.
2.2. Animal model
All experiments utilized NOD-Rag1null IL2rgnull (NRG) mice (The Jackson Laboratory, 4-8 weeks old, and 18-26 g at time of tumor implantation) (33). For survival assays, human prostate adenocarcinoma LNCaP cells (ATCC) xenografts were implanted 4 ± 1 weeks before the experiment by injecting 200 µL of a 1:1 matrigel (Fisher Scientific)/phoshate-buffered saline (Wisent) mixture containing 8 × 106 LNCaP cells subcutaneously on mice shoulders. LNCaP PSMA expression was previously verified (31). Protocols were approved by the Animal Ethics Committee of the Université de Sherbrooke according to the Canadian Council on Animal Care guidelines.
2.3. Maximal tolerated injected activity
To estimate maximal tolerated injected activity (IA) for survival assays, 6 NRG non-tumor-bearing mice were divided into 3 groups to test 70 MBq, 120 MBq and 150 MBq of 64Cu-DOTHA2-PSMA in 0.3 mL of saline (bolus injection by tail vein, constant molar activity). Starting IA was based on reported activities with 64Cu therapy in relatively similar conditions and scaled to mouse weight if needed (23–28). Mouse weight and general well-being were monitored daily 19 to 21 days. Maximal tolerated IA was chosen based on absence of general toxicity.
2.4. Survival experiments
When tumor reached a maximal diameter of 5 to 8 mm, LNCaP tumor-bearing mice (n = 30) were divided into 3 treatment groups with comparable tumor size distribution. NRG Mice were injected in the tail vein with 0.3 mL of saline containing previously determined maximal tolerated IA of 64Cu-DOTHA2-PSMA, equimolar amount of nonradioactive natCu-DOTHA2-PSMA as a control or the maximum tolerated IA of 177Lu-PSMA-617 based on literature [120 MBq (34, 35)]. Survival end points were the ethical limit points: sustained weight loss > 20%, tumor diameter > 1 cm, behavior alterations suggestive of pain, tumor ulceration to the skin, non-manageable toxicity signs such as diarrhea or vomiting. Mouse weight, tumor size measured by caliper, and well-being were followed every 1 to 4 days for a maximum of 62 days. Tumor volume was calculated as an ellipsoid volume = (length × width × height)/2, estimating height = width (36). To determine the effect of radiotherapy on control of tumor growth, we suggest two calculated values: time-to-regrowth (TTR) for the interval to regrow after reaching the nadir and time-to-initial volume (TTIV) for the interval to regrow over the initial volume (calculations’ details in Supplementary material).
2.5. Toxicity assessment
General toxicity was assessed by the follow-up of the mouse weight. Mice food was mixed with water for treated mice. To obtain a general evaluation of blood toxicity, red blood cells (RBCs) were counted in survival experiment mice every 3 days from 2 to 18 days p.i. based on literature results (half the group per time point to spread out individual collections to every 6 days, leading in practice to 3 to 7 samples per time point) (sampling details in Supplementary material) (25, 26, 28). Results were compared to values measured in non-treated mice (n = 13).
To evaluate sub-acute toxicity, kidneys, liver and salivary glands of survival experiment mice were collected and stained with hematoxylin and eosin (H&E), Masson’s trichrome and light periodic acid-Schiff for the kidneys (preparation details in Supplementary material). Digitized slides were blindly analyzed by two persons (i.e. a certified pathologist and a medical student), to identify potential signs of radiation injury as suggested by Fajardo (37) and detailed in Supplementary Table 1. Changes could be parenchymal (e.g. necrosis, apoptosis, atrophy), stromal (e.g. fibrosis), vascular (e.g. edema, ischemia, hemorrhage, endothelial wall atypia and foam cells plaques) or inflammatory. Fibrosis was graded and averaged between results of both analysts if discordant. Proportion of live (at time of fixation) tumor cells over total tumor tissue was assessed using a grid. Results were compared between groups and to the results from two non-treated tumor-bearing mice ineligible to survival assays (oversize tumor).
2.6. Dosimetry
Kinetic values were obtained based on previously published healthy organ biodistribution and tumor PET data (31). Dosimetry was calculated for 25 g mouse model, human model, and sphere model for tumor in OLINDA/EXM 2.2.3 (Hermes Medical Solution). Detailed methodology is provided as supplementary material.
2.7. Statistical analysis
Results were reported as mean ± standard deviation unless mentioned otherwise. Tests used were two-tailed Student T test (Holm-Sidak correction for multiple comparisons, Welch test when applicable for non-consistent standard deviations) and two-tail correlation test with calculation of Pearson r coefficients. Survival results were analyzed by Kaplan-Meier curves stratified by treatment groups and comparison was made using Mantel-Cox log-rank test. For all tests, adjusted p < 0.05 was the threshold for significance. Excel and GraphPad Prism 8 were used for calculations.
3. Results
3.1. Maximal tolerated injected activity
In practice, IA tested were: 68.3 MBq (67.8 – 68.8 MBq, n = 2), 117 MBq (115 – 119 MBq, n = 2), and 156 MBq (154 – 159 MBq, n = 2) (Figure 2 and Supplementary Table 2). Mice in the first two groups mainly gained weight from time of injection and showed normal behavior. One mouse in the last group lost up to 7% of its initial weight at first then stagnated around 100% of initial weight without external intervention. Mice from the last group showed signs of fear or stress for a total of 5 and 8 days out of 21 days follow-up but were active and vigorous. In consideration of these data and for practical reasons, we did not escalate to a further IA and determined 150 MBq as maximal tolerated IA to be injected in survival experiments.
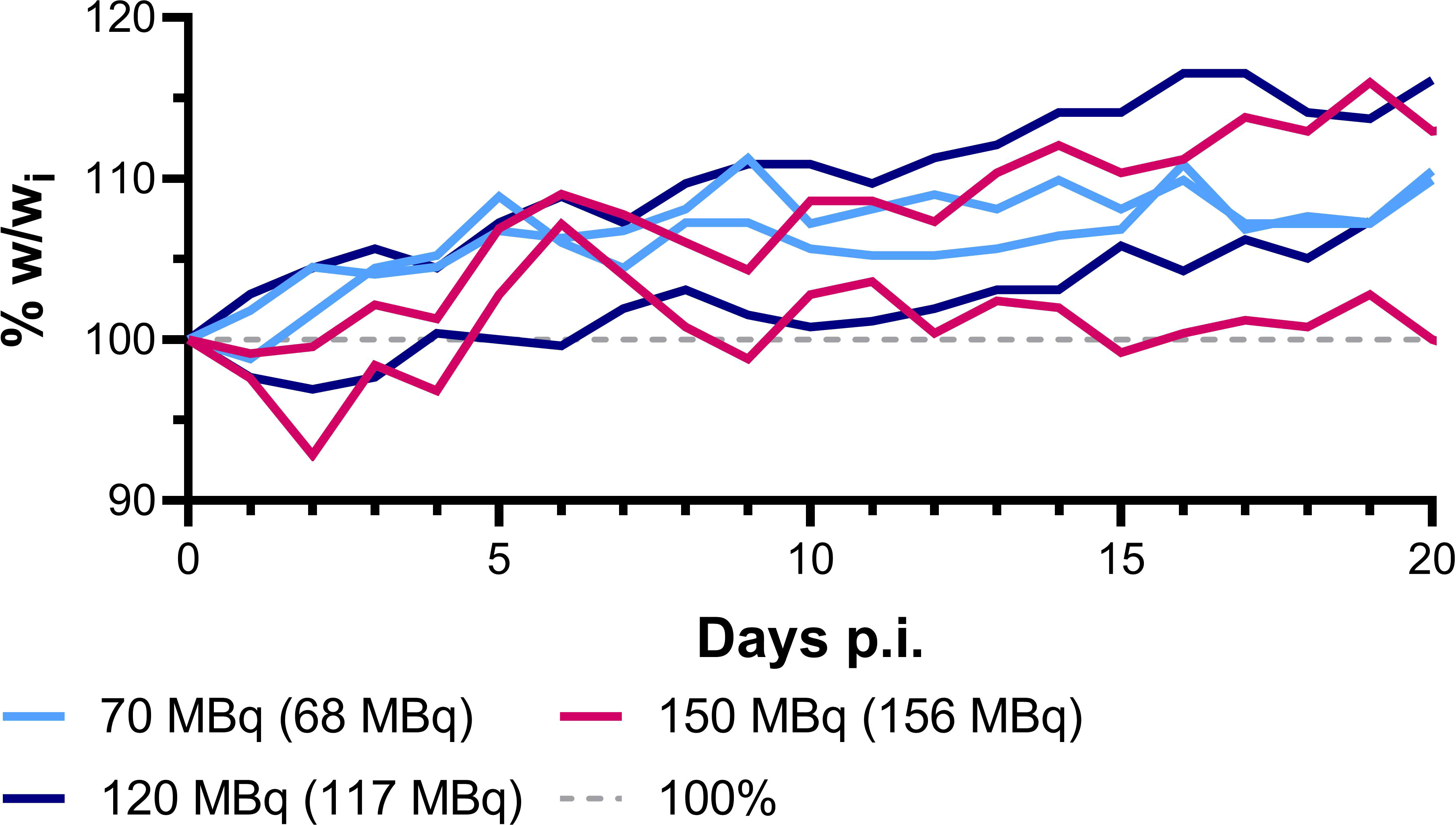
Figure 2 64Cu-DOTHA2-PSMA maximal tolerated IA experiment mice follow-up, weight (w) follow-up. (wi: initial weight).
3.2. Survival assays
Survival curves are displayed in Figure 3A and survival experiments details including mice and tumors individual characteristics are available in Supplementary Tables 3–6. Survival assays mice injected with 146 ± 14 MBq of 64Cu-DOTHA2-PSMA group (n = 12) survived significantly longer than controls injected with the same amount of natCu-DOTHA2-PSMA (1.23 nmol, n = 10) (p < 0.001, hazard ratio: 0.208 (95%CI: 0.0646 – 0.670)). Their survival was not significantly different from mice injected with 123 ± 13 MBq of 177Lu-PSMA-617 (n = 7) (p = 0.09, hazard ratio 0.490 (95%CI: 0.165 – 1.45)). Median survival was 35.5 days with 64Cu- DOTHA2-PSMA (interquartile range (IQR): 26.5 days), 5 days with natCu-DOTHA2-PSMA (IQR: 1 day) and 30 days with 177Lu-PSMA-617 (IQR: 4 days). Average tumor volumes at injection were 108 ± 55 mm3, 93.3 ± 56.1 mm3, and 107 ± 35 mm3 for 64Cu-DOTHA2-PSMA, natCu-DOTHA2-PSMA and 177Lu-PSMA-617 groups, respectively (no significant difference between groups (p > 0.91)). One 64Cu-DOTHA2-PSMA treated mouse and one 177Lu-PSMA-617 treated mouse were retrospectively rejected from survival experiments after injection, as they should not have been included in the protocol because of an oversized tumor at the beginning of treatment. They were, however, included in toxicity results because this rejection criteria did not affect healthy organs or general well-being.
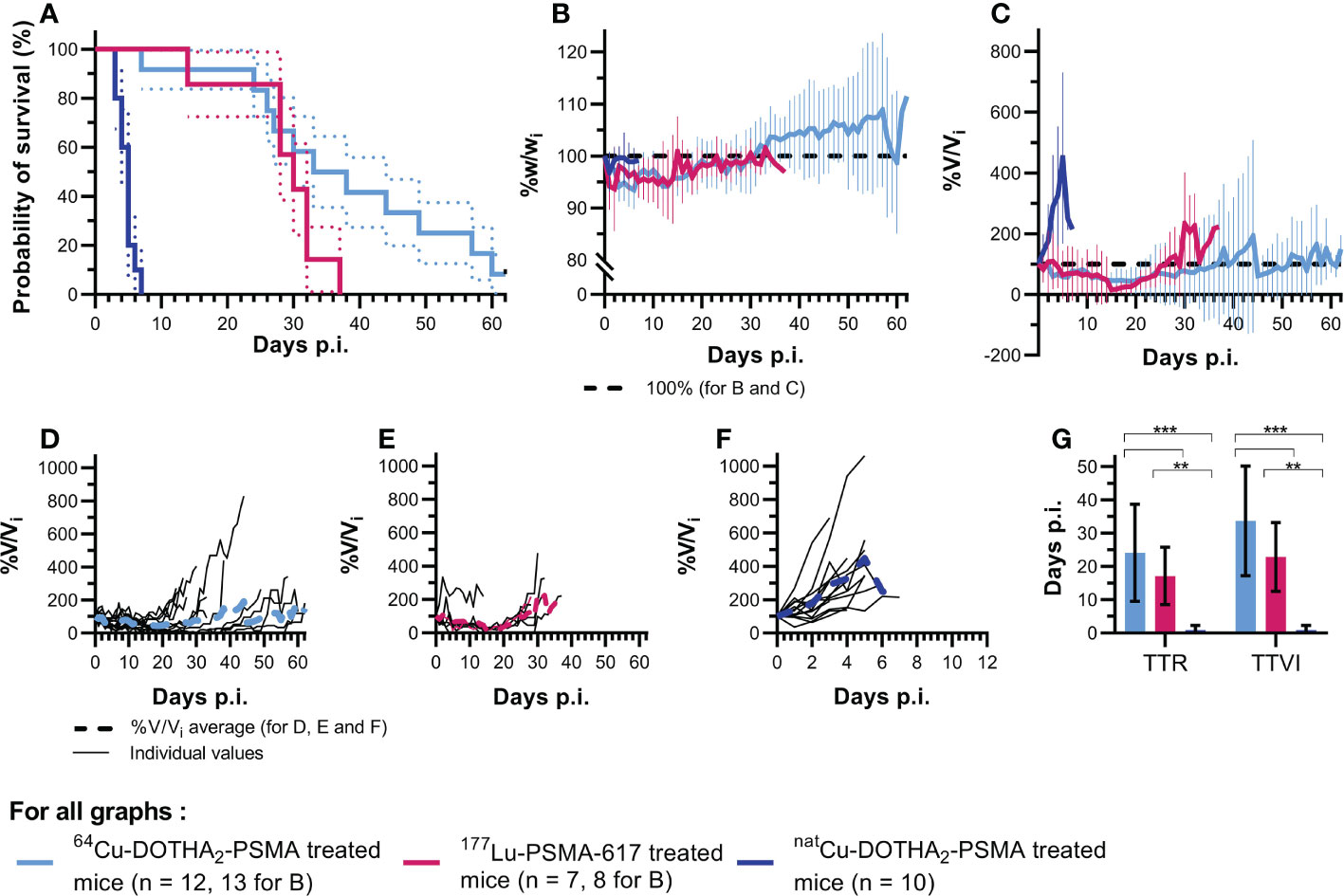
Figure 3 Survival assays results (A) Kaplan-Meier survival curves with significantly longer survival with 64Cu-DOTHA2-PSMA than with natCu-DOTHA2-PSMA (p < 0.001, hazard ratio: 0.208 (95%CI: 0.0646 – 0.670) and no significant difference between 64Cu-DOTHA2-PSMA and 177Lu-PSMA-617 (p = 0.09, hazard ratio: 0.490 (95%CI: 0.165 – 1.45)), (B) Average weight evolution per group, (C) Average tumor size per group, (D-F) Individual tumor size for 64Cu-DOTHA2-PSMA, 177Lu-PSMA-617 and natCu-DOTHA2-PSMA treated mice, respectively, (G) Time-to-regrowth (TTR) per group, (H) Time to initial volume (TTIV) per group. (V: tumoral volume, Vi: initial tumoral volume. **p < 0.01, ***p < 0.001. Errors bars are standard errors for (A) and standard deviations for B, C and G).
The cause of euthanasia was reaching tumor size limit for all but one mouse in 177Lu-PSMA-617 group (maximal weight loss, day 14) and two mice in the 64Cu-DOTHA2-PSMA group (pain behavior, day 60 and reaching follow-up limit, day 62). Two 64Cu-DOTHA2-PSMA and two natCu-DOTHA2-PSMA mice had a second tumor of similar size at time of therapy that did not reach 10 mm tumor diameter limit, but mainly showed similar evolution patterns.
Individual and group average tumor volume progression is displayed in Figures 3C–F. In comparison to the control group, TTR and TTIV (Figure 3G) were significantly higher in groups treated with 64Cu-DOTHA2-PSMA (24.1 ± 14.6 days (p < 0.001) and 33.7 ± 16.5 days (p < 0.001), respectively) or with 177Lu-PSMA-617 (17.1 ± 8.6 days (p = 0.003) and 22.9 ± 10.4 days (p = 0.003), respectively). TTR and TTIV values for the control group were both of 0.857 ± 1.406 days. No statistically significant difference was observed between 64Cu-DOTHA2-PSMA and 177Lu-PSMA-617 (TTT: p = 0.26; TTIV: p = 0.24).
Within individual treatment groups 177Lu-PSMA-617 and 64Cu-DOTHA2-PSMA, there is no correlation between IA (absolute and per gram) and survival (R2 < 0.3). 64Cu-DOTHA2-PSMA treated tumors with bigger initial volume had shorter TTR, TTIV and survival, but correlation was low (R2 < 0.5). For 177Lu-PSMA-617 treated tumors, there were no correlation between initial tumor volume and survival or TTR, but there was a moderate positive correlation between initial tumor volume and TTIV (R2 = 0.611, p = 0.04). This correlation was mainly dependent on the mouse with the smallest initial tumor (49.0 mm3) being euthanized early for maximal weight loss and not for reaching the maximal tumor size. When testing without this mouse, there was no correlation (R2 = 0.179).
3.3. Toxicity assays in survival experiment mice.
General toxicity was assessed through weight follow-up. Only one mouse from the 177Lu-PSMA-617 group maintained a weight loss until reaching the ethical limit (20% weight loss). As depicted in Figure 3B, mice from all groups tended to lose weight at the beginning of study, but later maintained their weight or gained back at least their initial weight.
Normal range obtained from non-treated mice was 1.19 ± 0.21 × 1010 RBCs/mL (95% confidence interval (CI): 1.07 × 1010 to 1.30 × 1010 RBCs/mL, n = 13), Supplementary Figure 3 and Table 7. Samples from 64Cu-DOTHA2-PSMA treated mice showed no significant difference from measured normal range, with values at days 8 and 17 lower than normal (all p > 0.35). Samples from 177Lu-PSMA-617 treated mice showed a significantly lower count on day 8 with 9.13 ± 0.49 × 109 RBCs/mL (p < 0.001) and values staying low until day 14. Control natCu-DOTHA2-PSMA treated mice RBCs counts could only be obtained on day 2 (n = 4) given the low median survival and did not significantly differ from normal range (p = 0.98).
Histopathological analysis of healthy organs showed little to no signs of radiotoxicity, as displayed in Figure 4 for comparison between control, non-treated mice and 64Cu-DOTHA2-PSMA treated mice (detailed numerical values for all groups in Supplementary Tables 8, 9). In kidneys (Figures 4A–D), liver (Figures 4E–G), salivary glands (Figures 4H–J), fibrosis increase in thickness and length was mainly found in the perivascular area, including the portal area for the liver and in addition to surrounding excretory ducts for the salivary glands (fibrosis score: Figure 4N). There were at most rare signs of interstitial fibrosis in the kidneys. In the liver, rare septal fibrosis was formed without important extensions between portal spaces and central veins to form nodules. Fibrosis was increased with 64Cu-DOTHA2-PSMA in comparison to the non-treated control in the kidneys, liver and salivary gland (p < 0.001, p = 0.006 and p < 0.001, respectively). For 177Lu-PSMA-617 group, fibrosis was significantly increased in comparison to non-treated controls in the liver (p = 0.009). For natCu-DOTHA2-PSMA, fibrosis was increased in comparison to non-treated mice in the kidneys, liver and salivary glands (p < 0.001, p = 0.009 and p < 0.001, respectively). There were no significant differences between 64Cu-DOTHA2-PSMA, 177Lu-PSMA-617 and natCu-DOTHA2-PSMA groups in all organs. In all healthy organs, there was neither necrosis, hemorrhage, cells or structure atrophy, edema (present surrounding the gland in one sample of natCu-DOTHA2-PSMA), endothelial wall atypia or foam cells plaques, nor glomerular changes for the kidney. Limited, rare and locally circumscribed inflammation was noted in liver only, similar in all groups.
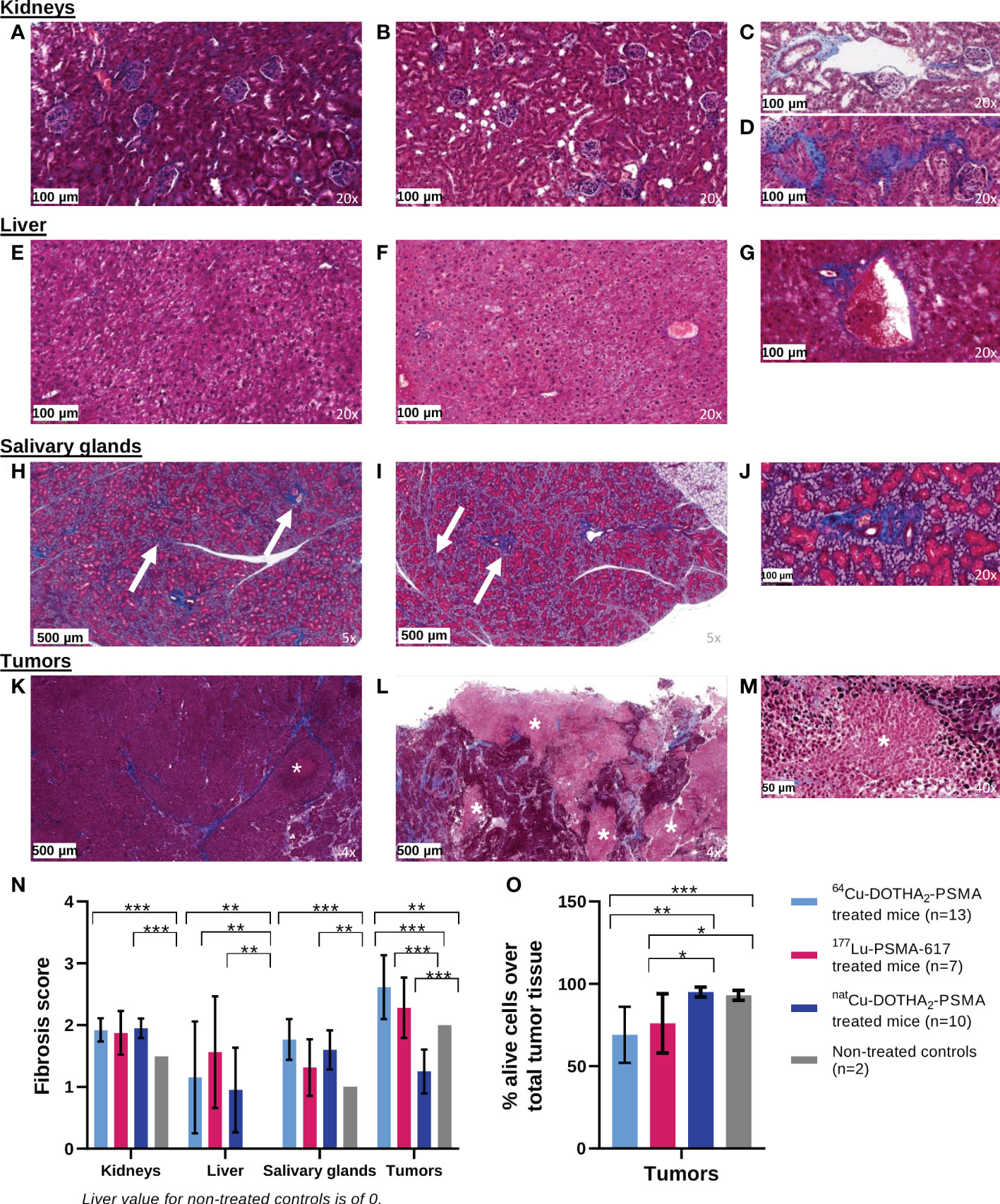
Figure 4 Pathology analysis results from controls, non-treated mice (left colum) in comparison to 64Cu-DOTHA2-PSMA treated mice (middle and right columns): Normal kidney histology was mainly found in controls (A) and treated mice (B), with frequent non-pathological perivascular fibrosis (C) and rare, locally limited interstitial fibrosis (D). Liver analysis also showed normal, non-injured tissue in controls (E) and treated mice (F) with some periportal fibrosis (G). All salivary glands displayed non-pathological, expected fibrosis surrounding vessels and excretory duct and in septa in controls (H) and treated mice (I, J). For LNCaP tumors, controls mainly showed alive tumor bulk, with fibrosis and infrequent necrosis (K). In opposite, the irradiated tumors showed a lower proportion of alive cells and more necrosis (L, M). Fibrosis scores by organs are presented in N and the proportion of alive tumor cells over total tumor tissue is presented in O. Stained by Masson’s trichome. Fibrosis: blue staining, arrow when needed. Necrosis in the tumor: lighter pink, asterix. In graphs, only significatively different relations are shown. *p < 0.05. **p< 0.01. ***p < 0.001.
LNCaP tumors (Figures 4K–M) showed necrosis, apoptosis, fibrosis and bleeding, in higher proportion in tumors treated with radioactivity. Hence, the ratios of alive tumor cells to total tumor tissue in 64Cu-DOTHA2-PSMA and 177Lu-PSMA-617 groups were lower than with natCu-DOTHA2-PSMA group (p < 0.001 and p = 0.04, respectively) and with non-treated control group (p = 0.002 and p = 0.04, respectively) (Figure 4O). Tumoral fibrosis was significantly increased in 64Cu-DOTHA2-PSMA and 177Lu-PSMA-617 groups in comparison to natCu-DOTHA2-PSMA (p < 0.001 for both) and to non-treated mice for 64Cu-DOTHA2-PSMA (p = 0.005) (Figure 4N).
There was no correlation between IA or IA/g with fibrosis score per groups for all organs (R2: 0 – 0.38). In healthy organs, there was no correlation between survival (and therefore age) and fibrosis score (R2: 0.001 – 0.16). Concordance between the fibrosis score results of both analysts were of 85%, 82%, 67% and 67% for the kidneys, liver, salivary glands and tumors, respectively. Differences, when present, were of one point or less.
3.4. Dosimetry
Mouse dosimetry results are presented in Table 1 with kinetics values used for calculations presented in Supplementary Table 10. Tumor dose was 2.67 × 102 mGy/MBq (95% CI: 1.94 × 102 – 3.4 × 102 mGy/MBq), using average tumor weight of 0.1 g. In mouse, highest organ dose was estimated to the liver, leading to a tumor to liver ratio of 0.730 (0.464 – 1.09). Second highest ratio was to the stomach wall (estimated from stomach kinetics), at 1.84 (1.18 – 2.70). Tumor-to-kidney ratio was 2.23 (1.42 – 3.30).
Human extrapolation of dosimetry presented in Table 2 (kinetic values in Supplementary Table 11) yielded effective dose of 3.14 × 10-2 mSv/MBq (95% CI: 2.67 × 10-2 – 3.61 × 10-2 mSv/MBq).
4. Discussion
The goal of this preclinical study was to evaluate 64Cu-DOTHA2-PSMA therapeutic potential based on its ability to increase survival and control tumor size with low toxicity profile and dosimetry. To our knowledge, this is the first study to evaluate 64Cu for PSMA radioligand therapy.
4.1. Maximal tolerated injected activity
64Cu-DOTHA2-PSMA maximal tolerated IA was estimated to be 150 MBq in NRG mice. This value is slightly higher than that reported for 177Lu-PSMA-617 in immunodeficient mice (34, 35) suggesting that the 64Cu-radioligand is well tolerated in immunodeficient mice.
4.2. Survival experiments
64Cu-DOTHA2-PSMA showed therapeutic efficacy. It showed survival and growth delay (TTR and TTIV values) superior to control and similar to 177Lu-PSMA-617, the lead radioligand for prostate cancer radioligand therapy. Other non-PSMA 64Cu radioligands in literature showed improvement in survival and tumor size control, but comparison is limited given their application with another target (23–30). For 177Lu-PSMA-617 with a slightly higher volume endpoint (1000 mm3), Fendler et al. found similar survival results to ours using 120 MBq (34) and Kuo et al. found higher median survival (58 days) even with the use of low IA (18.5 MBq) (38). Banerjee et al. found a 130 days median survival in a different prostate cancer model (PSMA+ PC3 PIP cells) with a higher tumoral volume endpoint (1800 mm3) (35).
4.3. Toxicity assessment
On the whole, toxicity results pointed to limited toxicity for 64Cu-DOTHA2-PSMA, similar to 177Lu-PSMA-617. Mice weight was maintained above limit endpoints for all but one 177Lu-PSMA-617 treated mouse, suggesting a low toxicity for both 64Cu-DOTHA2-PSMA and 177Lu-PSMA-617. Thought the only significant drop in red blood cells was observed in 177Lu-PSMA-617 mice samples at 8 days p.i., period of days 8 to 17 could be an interval of potential myelotoxicity for both radioactive compounds. Regarding kidneys, liver and salivary glands, only non-significant fibrosis in normal location was found with no other sign of radiation injuries nor pathological processes (e.g. nodule formation in liver, significant interstitial fibrosis in kidneys) (37, 39, 40). The difference between the control group injected with natCu-DOTHA2-PSMA and the non-treated controls suggests an interaction of the ligand with the PSMA enzyme influencing its function (41). However, this is not likely to have important toxic implications at injected activities similar to the present study given that all fibrosis noted was non-pathological fibrosis. The fibrosis scale was obtained by blind comparison of samples, but on the whole the difference between scores were low and fibrosis was present in low quantity.
For LNCaP tumors, pathological analysis at varying time after implantation could have a confounder effect on the results. Non-treated LNCaP tumors can demonstrate necrosis and fibrosis without treatment (42). This phenomenon was witnessed in both control groups, but in a significantly smaller proportion than the ones noticed in the groups injected with radioactivity. In clinical pathology practice, important proportion of dead tumor cells is considered more likely to be induced at least in part by treatment. Furthermore, in mice treated with radioligands, necrosis was found over all tumor bulk and not only in the middle of a highly alive section. For healthy organs, few weeks difference in age between controls and treated mice is less likely to explain the difference between survival groups and non-treated controls since there is no correlation between time of death and fibrosis score.
For comparison regarding toxicity, preclinical studies of other 64Cu radioligand therapy compounds noted no to only transient weight loss and no to only mild signs in pathological examination (23–27, 29). Transient drop in RBCs was noted in a study 7 days p.i. at its highest IA (28) while no significant drop in RBCs was noted with others (23–26). For preclinical studies of 177Lu-PSMA-617, no weight loss was reported at a lower IA (38) and no change in RBCs was noted at 4 and 8 weeks p.i (34, 35).. With 177Lu-PSMA-617, Banerjee et al. noted mild changes in salivary glands, minimal changes in kidneys and significant changes in lacrimal glands and testes 8 weeks p.i (35).
4.4. Dosimetry
Dosimetry calculations yielded an effective dose within normal limits and showed that the main organs at risk for toxicity are the liver and gastrointestinal tract. High dose in liver for human and low tumor-to-liver ratio in mouse were expected from previous distribution results (31). Interestingly, kidneys and salivary glands dose estimations in humans were low for 64Cu-DOTHA2-PSMA, with favorable tumor-to-kidney ratio in mouse. In a theranostic perspective, human dosimetry estimation of 64Cu-DOTHA2-PSMA’s effective dose is within safe levels for imaging with diagnostic IA. For instance, if one was to inject 200 MBq as for 68Ga-PSMA-617 human scan, effective dose to the patient would be 6.28 mSv. This is similar to 18F-fluorodesoxyglucose PET for tumor imaging (~6.7 mSv), 68Ga-PSMA-11 and 68Ga-PSMA-617 (43–45). For comparison regarding therapy, human studies with 177Lu-PSMA-617 showed that organs receiving among the highest calculated doses are the kidneys (0.39 to 0.88 mGy/MBq) and salivary glands (0.44 to 1.17 mGy/MBq), respectively 7 to 16 times and 23 to 62 times higher values than the 64Cu-DOTHA2-PSMA human model absorbed doses estimations (46–48). Lacrymal gland dose estimation is unknown for 64Cu-DOTHA2-PSMA, since it is unavailable in the OLINDA/EXM human model used. It was, however, the highest dose in VISION phase III trial dosimetry sub-study (2.1 ± 0.5 mGy/MBq) (48). Liver, spleen and effective doses are similar for both radioligands, even with the important liver uptake for 64Cu-DOTHA2-PSMA (46, 47). Red marrow doses were higher for 177Lu-PSMA-617 than estimated for 64Cu-DOTHA2-PSMA, but this could be in part due to bone metastasis in patients for 177Lu-PSMA-617 (46–48).
Irradiation tolerance is considered to be higher for radioligand therapy than for external beam radiotherapy (47, 49). However, external beam radiotherapy dose limits are sometimes still used to guide radioligand therapy safety. Considering limits or standards of 23 Gy for the kidneys, 30 Gy for the liver, 20 − 25 Gy to the salivary glands, 2 Gy to the red marrow, 40 Gy to the intestines and 12 Gy for whole-lung irradiation (46, 47, 50–53), the injection of 44 GBq of 64Cu-DOTHA2-PSMA over several cycles such as for 177Lu-PSMA-617 (13) would not reach organ doses limits. Up to a total of 187 GBq could be injected before reaching the medullary and liver limit doses (calculations based on organ absorbed doses). We do not suggest using this high injected activity and it is simply underlined here to put 64Cu-DOTHA2-PSMA dosimetry profile in perspective. Clinical studies should be conducted to determine 64Cu-DOTHA2-PSMA biodistribution and dosimetry in humans to choose an appropriate injected activity.
Mouse dosimetry was obtained mainly to calculate ratios between tumor and healthy organs irradiation. In 2018, Kuo et al. reported mouse dosimetry for 177Lu-PSMA-617 with a methodology highly similar to ours using OLINDA/EXM 2.0 while we used the latest version 2.2.3 (38). Their results per organs for 177Lu-PSMA-617 were in average ~500 times lower than ours for 64Cu-DOTHA2-PSMA. We recalculated 177Lu-PSMA-617 dosimetry with OLINDA/EXM 2.2.3 based on their kinetics values and obtained results closer to 64Cu-DOTHA2-PSMA, notably 5.43 × 102 mGy/MBq, 3.08 × 102 mGy/MBq, and 5.85 mGy/MBq for tumor, kidneys and liver, respectively. Other organs doses ranged from 2.54 mGy/MBq to 14.2 mGy/MBq, except for the bladder dose calculated with a different voiding model than our methodology. The difference between 177Lu-PSMA-617’s results reported with OLINDA/EXM 2.0 and those obtained with the version 2.2.3 is notable. With 2.2.3, the tumor-to-kidney ratio is 1.76, slightly lower than for 64Cu-DOTHA2-PSMA, and the tumor-to-liver ratio is 92.8, importantly higher than for 64Cu-DOTHA2-PSMA.
Taking into consideration mouse and human models dosimetry results and analysis, the liver rather than the kidneys or the salivary glands would be at greater risk with 64Cu-DOTHA2-PSMA when compared to 177Lu-PSMA-617, though the liver dose is similar for both compounds. This dosimetry pattern could be a challenge for clinical use. On the other hand, it could also be of interest to complete the clinical arsenal with a radioligand with different toxicity profile to offer an endotherapeutic option to more patients (e.g. with renal insufficiency or important prior bladder irradiation) or for patients experiencing side effects (e.g. xerostomia). Their irradiation should also be considered as radioligand therapy is being increasingly studied at earlier stages of the disease (e.g. adjuvant to prostatectomy) (13). Patient living longer could experience different side effects over the long term or eventual use adjuvant to external beam radiotherapy could modify the risk profile, with increasing risk to pelvic organs in the irradiation field.
It is important to note that dosimetry estimates to organs and tumor do not take account of dose rates and repetitions of irradiation, which can both influence the therapeutic outcome and toxicity (23, 24, 28, 29, 54).
4.5. Limitations and variability
Some limitations and sources of variability should be underlined for the present study. Variation in group size can be explained by the few days variability in LNCaP xenograft growth parallel to radioisotope production and importation logistics. Variation in IA or tumor size at time of the experiment, which is expected for preclinical studies, did not significantly impact survival assays and toxicity results as verified by correlation tests. Fibrosis scores concordance rates between both analysts’ results were high to intermediate, with only one-point difference when present. Concordance in future studies results could be increased by making the fibrosis scale more precise thanks to the experience acquired in this project.
In hindsight, the implantation of one tumor per shoulder in the first treated mice was a suboptimal method. Although this is a common practice in LNCaP implantation to increase the probability of experiment success [estimated 50% success rate in xenografting (42, 55)], it also yielded more complicated survival analyses. For instance, if two tumors were present at the time of therapy, both did not necessarily reach the size limit on the very same day, even if response to treatment were mainly similar for both tumors. Consequently, four mice had to be euthanized when a first tumor reached the ethical limit, and therefore, growth follow-up for the second tumor was stopped and the time to size endpoint was not obtained. In an attempt to mitigate this issue, we selected TTIV and TTR methods for growth calculation, which also had the advantage to include in the analysis mice that reached limits other than tumor size. One tumor was implanted per mouse for subsequent radioligand therapy rounds.
The animal model has other limitations. The important immunosuppression required for tumor implantation in mice may lead to an underestimation of signs of toxicity through decreased inflammation (33). Furthermore, 64Cu-DOTHA2-PSMA binding can vary between mouse and human PSMA, which limits the interpretation of toxicity results in healthy PSMA-rich tissues. Although conduction of toxicity evaluation in survival assays mice is not ideal, it does allow for the assessment of a broad profile.
4.6. Perspectives
Future studies could evaluate the immunoreactivity of 64Cu-DOTHA2-PSMA as well as apoptosis and DNA double-strand breaks on tumor tissue after treatment. The PSMA expression and Ki67 marker expression could also be measured to confirm the level of PSMA positivity of surviving tumor cells and to have information on the proliferative capacity of the tumor cells respectively. Different injected activities and multidoses for radioligand therapy could be evaluated. Multidose of 64Cu-DOTHA2-PSMA could have an impact on tumor size control and toxicity, based on dose fractionation principles and similarly to previously published preclinical results with 64Cu and 177Lu and to 177Lu-PSMA-617 clinical protocols (14, 23–25, 27–30). Copper-67 (67Cu) has a similar β- emission profile to 177Lu with a 2.6 days half-life and can be used in theranostic pair with copper-64 (64Cu) for PET imaging (20). 64Cu/67Cu-SAR-bisPSMA is currently under clinical trial for prostate cancer radioligand therapy (SECuRE trial, NCT04868604). Further research is also needed for a more complete toxicity profile, using healthy and immunocompetent mice followed over months with a wider panel of blood cells and specific time points of euthanasia for pathologic evaluation as groups. Other experiences could be added such as blood and urine markers of kidney and liver functions e.g. AST, ALT, blood urea nitrogen, creatinine and glomerular filtration rate. Finally, another interesting future research could evaluate the link between pathological findings, radioligand distribution in tissue, emission range and microdosimetry. For instance, the presence of radioligand in blood circulation could irradiate vasculature and be linked to perivascular fibrosis.
In conclusion, 64Cu-DOTHA2-PSMA showed efficiency for radionuclide therapy in comparison to control and similarly to the most clinically studied PSMA radioligand, 177Lu-PSMA-617. Insights on toxicity suggest a safety profile similar to the one of 177Lu-PSMA-617, but further confirmation studies are required. Dosimetry estimation yielded a low dose to the kidneys and salivary glands. Higher doses estimations were obtained for the liver and gastrointestinal tract, a perceived challenge to clinical use. Nevertheless, 64Cu-DOTHA2-PSMA offers the possibility to complement the current clinical arsenal with a theranostic radioligand that has different mechanism of action (both β- particle and high LET Auger electron), clearance and dosimetry profile than previous radioligands. It could act as another alternative to 177Lu-PSMA-617, similarly to 225Ac-PSMA-617, to allow personalization of radioligand therapy based on patients’ comorbidities, prior treatments or experienced side effects.
Data availability statement
The original contributions presented in the study are included in the article/Supplementary Material. Further inquiries can be directed to the corresponding author.
Ethics statement
The animal study was reviewed and approved by Animal Ethics Committee of the Université de Sherbrooke according to the Canadian Council on Animal Care guidelines.
Author contributions
M-CM: conceptualization, data acquisition, analysis, writing original draft, editing. OB-B and VD-P: conceptualization, data acquisition, review. SA-M: development, conceptualization, synthesis and chemical data acquisition, review. SG: pathology conceptualization and data analysis, review. PR: writing and review, supervision. ER: conceptualization, dosimetry data treatment and analysis, writing, review, supervision. BG: conceptualization, writing, review, editing, project management, supervision. All authors contributed to the article and approved the submitted version.
Funding
This research received financial support from the Oncopole (funded by Merck Canada Inc., Fonds de recherche du Québec – Santé (FRQS), and the Cancer Research Society). MC Milot received scholarships from the Canadian Institute of Health Research, FRQS, and the University of Sherbrooke (UdS). B Guérin is the holder of the Jeanne and J.-Louis Lévesque Chair in Radiobiology at the Université de Sherbrooke. The funders were not involved in the study design, collection, analysis, interpretation of data, the writing of this article or the decision to submit it for publication. All authors declare no other competing interests.
Acknowledgments
The authors thank the cyclotron team from the Sherbrooke Molecular Imaging Centre (CIMS)/Centre de recherche du CHUS and the partners that offered additional support (UdS, CRCHUS, les Fondations du CHUS et du CHUQc-UL, the Nuclear Medicine Clinical Research Unit at the CHUQc-UL, CIMS, the Ministère de Santé et Services sociaux du Québec, the Comité stratégique des patients-partenaires du CRCHUS/Initiative patients-partenaires de l’UdS, the Association des médecins spécialistes en médecine nucléaire du Québec, the Association des urologues du Québec).
Conflict of interest
The authors declare that the research was conducted in the absence of any commercial or financial relationships that could be construed as a potential conflict of interest.
Publisher’s note
All claims expressed in this article are solely those of the authors and do not necessarily represent those of their affiliated organizations, or those of the publisher, the editors and the reviewers. Any product that may be evaluated in this article, or claim that may be made by its manufacturer, is not guaranteed or endorsed by the publisher.
Supplementary material
The Supplementary Material for this article can be found online at: https://www.frontiersin.org/articles/10.3389/fonc.2023.1073491/full#supplementary-material
References
1. International Agency for Research on Cancer. Cancer today (2020). Available at: https://gco.iarc.fr/today/home (Accessed January 7, 2021).
2. American Cancer Society. Key statistics for prostate cancer (2020). Available at: https://www.cancer.org/cancer/prostate-cancer/about/key-statistics.html (Accessed January 7, 2021).
3. Société canadienne sur le cancer. Statistiques canadiennes sur le cancer (2022). Available at: www.cancer.ca (Accessed January 7, 2022).
4. Eapen RS, Nzenza TC, Murphy DG, Hofman MS, Cooperberg M, Lawrentschuk N. PSMA PET applications in the prostate cancer journey: from diagnosis to theranostics. World J Urol (2019) 37:1255–61. doi: 10.1007/s00345-018-2524-z
5. Juzeniene A, Stenberg VY, Bruland ØS, Larsen RH. Preclinical and clinical status of PSMA-targeted alpha therapy for metastatic castration-resistant prostate cancer. Cancers (Basel) (2021) 13:1–25. doi: 10.3390/CANCERS13040779
6. Ruigrok EAM, Van Weerden WM, Nonnekens J, De Jong M. The future of PSMA-targeted radionuclide therapy: An overview of recent preclinical research. Pharmaceutics (2019) 11:560. doi: 10.3390/pharmaceutics11110560
7. Kassis AI. Therapeutic radionuclides: Biophysical and radiobiologic principles. Semin Nucl Med (2008) 38:358–66. doi: 10.1053/j.semnuclmed.2008.05.002
8. Zoller F, Eisenhut M, Haberkorn U, Mier W. Endoradiotherapy in cancer treatment - basic concepts and future trends. Eur J Pharmacol (2009) 625:55–62. doi: 10.1016/j.ejphar.2009.05.035
9. Kratochwil C, Afshar-Oromieh A, Kopka K, Haberkorn U, Giesel FL. Current status of prostate-specific membrane antigen targeting in nuclear medicine: clinical translation of chelator containing prostate-specific membrane antigen ligands into diagnostics and therapy for prostate cancer. Semin Nucl Med (2016) 46:405–18. doi: 10.1053/j.semnuclmed.2016.04.004
10. Kopka K, Benešová M, Bařinka C, Haberkorn U, Babich J. Glu-ureido-based inhibitors of prostate-specific membrane antigen: Lessons learned during the development of a novel class of low-molecular-weight theranostic radiotracers. J Nucl Med (2017) 58:17S–26S. doi: 10.2967/jnumed.116.186775
11. Iravani A, Violet J, Azad A, Hofman MS. Lutetium-177 prostate-specific membrane antigen (PSMA) theranostics: practical nuances and intricacies. Prostate Cancer Prostatic Dis (2020) 23:38–52. doi: 10.1038/s41391-019-0174-x
12. Frigerio B, Luison E, Desideri A, Iacovelli F, Camisaschi C, Seregni EC, et al. Validity of anti-PSMA ScFvD2B as a theranostic tool: A narrative- focused review. Biomedicines (2021) 9:1870. doi: 10.3390/dbiomedicines9121870
13. Seitzer KE, Seifert R, Kessel K, Roll W, Schlack K, Boegemann M, et al. Lutetium-177 labelled PSMA targeted therapy in advanced prostate cancer: current status and future perspectives. Cancer (Basel) (2021) 13:3715. doi: 10.3390/cancers13153715
14. Hofman MS, Emmett L, Sandhu S, Iravani A, Joshua AM, Goh JC, et al. [177Lu]Lu-PSMA-617 versus cabazitaxel in patients with metastatic castration-resistant prostate cancer (TheraP): a randomised, open-label, phase 2 trial. Lancet (2021) 397:797–804. doi: 10.1016/s0140-6736(21)00237-3
15. Sartor O, de Bono J, Chi KN, Fizazi K, Herrmann K, Rahbar K, et al. Lutetium-177-PSMA-617 for metastatic castration-resistant prostate cancer. N Engl J Med (2021) 385:1091–103. doi: 10.1056/NEJMOA2107322
16. FDA approves Pluvicto for metastatic castration-resistant prostate cancer. FDA Approves pluvicto for metastatic castration-resistant prostate cancer (2022) (Accessed June 28, 2022).
17. Engle J. The production of Ac-225. Curr Radiopharm (2018) 11:173–9. doi: 10.2174/1874471011666180418141357
18. Wang J, Zang J, Wang H, Liu Q, Li F, Lin Y, et al. Pretherapeutic 68 Ga-PSMA-617 PET may indicate the dosimetry of 177 Lu-PSMA-617 and 177 Lu-EB-PSMA-617 in main organs and tumor lesions. Clin Nucl Med (2019) 44:431–8. doi: 10.1097/RLU.0000000000002575
19. Duan H, Iagaru A, Aparici CM. Radiotheranostics – precision medicine in nuclear medicine and molecular imaging. Nanotheranostics (2022) 6:103–17. doi: 10.7150/NTNO.64141
20. Ahmedova A, Todorov B, Burdzhiev N, Goze C. Copper radiopharmaceuticals for theranostic applications. Eur J Med Chem (2018) 157:1406–25. doi: 10.1016/j.ejmech.2018.08.051
21. Brookhaven National Laboratory. National nuclear data center 3.0, in: Brookhaven natl Lab (2022). Available at: https://www.nndc.bnl.gov/nudat3/ (Accessed February 22, 2022).
22. McMillan DD, Maeda J, Bell JJ, Genet MD, Phoonswadi G, Mann KA, et al. Validation of 64Cu-ATSM damaging DNA via high-LET auger electron emission. J Radiat Res (2015) 56:784–91. doi: 10.1093/JRR
23. Anderson CJ, Jones LA, Bass LA, Sherman ELC, Mccarthy DW, Cutler PD, et al. Radiotherapy, toxicity and dosimetry of copper-64-TETA-octreotide in tumor-bearing rats. J Nucl Med (1998) 39:1944–51.
24. Lewis JS, Lewis MR, Cutler PD, Srinivasan A, Schmidt MA, Schwarz SW, et al. Radiotherapy and dosimetry of 64Cu-TETA-Tyr3-octreotate in a somatostatin receptor-positive, tumor-bearing rat model. Clin Cancer Res (1999) 5:3608–16.
25. Lewis JS, Laforest R, Buettner TL, Song SK, Fujibayashi Y, Connett JM, et al. Copper-64-diacetyl-bis(N4-methylthiosemicarbazone): An agent for radiotherapy. Proc Natl Acad Sci U.S.A. (2001) 98:1206–11. doi: 10.1073/pnas.98.3.1206
26. Jin Z-H, Furukawa T, Elissa Degardin M, Sugyo A, Tsuji AB, Yamasaki T, et al. Small molecule therapeutics a V b 3 integrin-targeted radionuclide therapy with 64 Cu-cyclam-RAFT-c(-RGDfK-) 4. Mol Cancer Ther (2016) 15:2076–85. doi: 10.1158/1535-7163.MCT-16-0040
27. Qin C, Liu H, Chen K, Hu X, Ma X, Lan X, et al. Theranostics of malignant melanoma with 64CuCl2. J Nucl Med (2014) 55:812–7. doi: 10.2967/jnumed.113.133850
28. Yoshii Y, Matsumoto H, Yoshimoto M, Zhang MR, Oe Y, Kurihara H, et al. Multiple administrations of 64Cu-ATSM as a novel therapeutic option for glioblastoma: a translational study using mice with xenografts. Transl Oncol (2018) 11:24–30. doi: 10.1016/j.tranon.2017.10.006
29. Ferrari C, Asabella AN, Villano C, Giacobbi B, Coccetti D, Panichelli P, et al. Copper-64 dichloride as theranostic agent for glioblastoma multiforme: A preclinical study. BioMed Res Int (2015) 2015. doi: 10.1155/2015/129764
30. Yoshii Y, Yoshimoto M, Matsumoto H, Tashima H, Iwao Y, Takuwa H, et al. Integrated treatment using intraperitoneal radioimmunotherapy and positron emission tomography-guided surgery with 64Culabeled cetuximab to treat early- and late-phase peritoneal dissemination in human gastrointestinal cancer xenografts. Oncotarget (2018) 9:28935–50. doi: 10.18632/oncotarget.25649
31. Milot M-C, Bélissant Benesty O, Dumulon-Perreault V, Ait-Mohand S, Richard PO, Rousseau É, et al. 64Cu-DOTHA2-PSMA, a novel PSMA PET radiotracer for prostate cancer with a long imaging time window. Pharmaceuticals (2022) 15:996. doi: 10.3390/PH15080996
32. Benešová M, Bauder-Wüst U, Schäfer M, Klika K, Mier W, Haberkorn U, et al. Linker modification strategies to control the prostate-specific membrane antigen (PSMA)-targeting and pharmacokinetic properties of DOTA-conjugated PSMA inhibitors. J Med Chem (2016) 59:1761–75. doi: 10.1021/ACS.JMEDCHEM.5B01210
33. Pearson T, Shultz LD, Miller D, King M, Laning J, Fodor W, et al. Non-obese diabetic-recombination activating gene-1 (NOD-Rag1 null ) interleukin (IL)-2 receptor common gamma chain (IL2rg null ) null mice: a radioresistant model for human lymphohaematopoietic engraftment. Clin Exp Immunol (2008) 154:270–84. doi: 10.1111/j.1365-2249.2008.03753.x
34. Fendler WP, Stuparu AD, Evans-Axelsson S, Lückerath K, Wei L, Kim W, et al. Establishing 177Lu-PSMA-617 radioligand therapy in a syngeneic model of murine prostate cancer. J Nucl Med (2017) 58:1786–92. doi: 10.2967/jnumed.117.193359
35. Banerjee SR, Kumar V, Lisok A, Chen J, Minn I, Brummet M, et al. 177Lu-labeled low-molecular-weight agents for PSMA-targeted radiopharmaceutical therapy. Eur J Nucl Med Mol Imaging (2019) 46:2545–57. doi: 10.1007/s00259-019-04434-0
36. Tomayko MM, Reynolds CP. Determination of subcutaneous tumor size in athymic (nude) mice. Cancer Chemother Pharmacol (1989) 24:148–54. doi: 10.1007/BF00300234
37. Fajardo LF. The pathology of ionizing radiation as defined by morphologic patterns. Acta Oncol (2005) 44:13–22. doi: 10.1080/02841860510007440
38. Kuo HT, Merkens H, Zhang Z, Uribe CF, Lau J, Zhang C, et al. Enhancing treatment efficacy of 177Lu-PSMA-617 with the conjugation of an albumin-binding motif: Preclinical dosimetry and endoradiotherapy studies. Mol Pharm (2018) 15:5183–91. doi: 10.1021/acs.molpharmaceut.8b00720
40. MacSween RNM. Pathology of the liver. 4th edition. London; New York: Churchill Livingstone (2002). 982 p. doi: 10.1002/dc.10338
41. Bouchelouche K, Capala J. Prostate specific membrane antigen-a target for imaging and therapy with radionuclides. Discovery Med (2010) 9:55–61.
42. Warnier M, Roudbaraki M, Derouiche S, Delcourt P, Bokhobza A, Prevarskaya N, et al. CACNA2D2 promotes tumorigenesis by stimulating cell proliferation and angiogenesis. Oncogene (2015) 34:5383–94. doi: 10.1038/ONC.2014.467
43. Directorate General for Energy. EUROPEAN COMMISSION RADIATION PROTECTION n° 180 medical radiation exposure of the European population part 1/2 (2014). Available at: http://europa.eu (Accessed August 5, 2021).
44. Afshar-Oromieh A, Hetzheim H, Kratochwil C, Benesova M, Eder M, Neels OC, et al. The theranostic PSMA ligand PSMA-617 in the diagnosis of prostate cancer by PET/CT: Biodistribution in humans, radiation dosimetry, and first evaluation of tumor lesions. J Nucl Med (2015) 56:1697–705. doi: 10.2967/jnumed.115.161299
45. Fendler WP, Eiber M, Beheshti M, Bomanji J, Ceci F, Cho S, et al. 68Ga-PSMA PET/CT: Joint EANM and SNMMI procedure guideline for prostate cancer imaging: version 1.0. Eur J Nucl Med Mol Imaging (2017) 44:1014–24. doi: 10.1007/s00259-017-3670-z
46. Kabasakal L, AbuQbeitah M, Aygün A, Yeyin N, Ocak M, Demirci E, et al. Pre-therapeutic dosimetry of normal organs and tissues of (177)Lu-PSMA-617 prostate-specific membrane antigen (PSMA) inhibitor in patients with castration-resistant prostate cancer. Eur J Nucl Med Mol Imaging (2015) 42:1976–83. doi: 10.1007/S00259-015-3125-3
47. Violet J, Jackson P, Ferdinandus J, Sandhu S, Akhurst T, Iravani A, et al. Dosimetry of 177Lu-PSMA-617 in metastatic castration-resistant prostate cancer: Correlations between pretherapeutic imaging and whole-body tumor dosimetry with treatment outcomes. J Nucl Med (2019) 60:517–23. doi: 10.2967/jnumed.118.219352
48. Herrmann K, Rahbar K, Eiber M, Krause BJ, Lassmann M, Jentzen W, et al. Dosimetry of 177Lu-PSMA-617 for the treatment of metastatic castration-resistant prostate cancer: results from the VISION trial sub-study. J Clin Oncol (2022) 40:97–7. doi: 10.1200/JCO.2022.40.6_SUPPL.097
49. Pandit-Taskar N, Iravani A, Lee D, Jacene H, Pryma D, Hope T, et al. Dosimetry in clinical radiopharmaceutical therapy of cancer: practicality versus perfection in current practice. J Nucl Med (2021) 62:60S–72S. doi: 10.2967/JNUMED.121.262977
50. Emami D. Tolerance of normal tissue to therapeutic radiation. Rep Radiother Oncol (2013) 1:35–48. doi: 10.1016/0360-3016(91)90171-y
51. Jadon R, Higgins E, Hanna L, Evans M, Coles B, Staffurth J. A systematic review of dose-volume predictors and constraints for late bowel toxicity following pelvic radiotherapy. Radiat Oncol (2019) 14:57. doi: 10.1186/s13014-019-1262-8
52. Stanic S, Mayadev JS. Tolerance of the small bowel to therapeutic irradiation: a focus on late toxicity in patients receiving para-aortic nodal irradiation for gynecologic malignancies. Int J Gynecol Cancer (2013) 23:592–7. doi: 10.1097/IGC.0B013E318286AA68
53. Sampath S, Schultheiss TE, Wong J. Dose response and factors related to interstitial pneumonitis after bone marrow transplant. Int J Radiat Oncol Biol Phys (2005) 63:876–84. doi: 10.1016/J.IJROBP.2005.02.032
54. Terashima S, Hosokawa Y, Tsuruga E, Mariya Y, Nakamura T. Impact of time interval and dose rate on cell survival following low-dose fractionated exposures. J Radiat Res (2017) 58:782–90. doi: 10.1093/jrr/rrx025
Keywords: prostate cancer, radioligand therapy, copper-64, DOTHA2-PSMA, theranostic
Citation: Milot M-C, Bélissant-Benesty O, Dumulon-Perreault V, Ait-Mohand S, Geha S, Richard PO, Rousseau É and Guérin B (2023) Theranostic 64Cu-DOTHA2-PSMA allows low toxicity radioligand therapy in mice prostate cancer model. Front. Oncol. 13:1073491. doi: 10.3389/fonc.2023.1073491
Received: 18 October 2022; Accepted: 05 January 2023;
Published: 18 January 2023.
Edited by:
Vikas Prasad, Radiology Mallinckrodt Institute of Radiology Division of Nuclear Medicine Washington University, United StatesReviewed by:
Benjamin John Blyth, Peter MacCallum Cancer Centre, AustraliaBenyi Li, University of Kansas Medical Center, United States
Giulio Fracasso, University of Verona, Italy
Copyright © 2023 Milot, Bélissant-Benesty, Dumulon-Perreault, Ait-Mohand, Geha, Richard, Rousseau and Guérin. This is an open-access article distributed under the terms of the Creative Commons Attribution License (CC BY). The use, distribution or reproduction in other forums is permitted, provided the original author(s) and the copyright owner(s) are credited and that the original publication in this journal is cited, in accordance with accepted academic practice. No use, distribution or reproduction is permitted which does not comply with these terms.
*Correspondence: Brigitte Guérin, QnJpZ2l0dGUuZ3VlcmluMkB1c2hlcmJyb29rZS5jYQ==