- 1Department of Immunology and Cell Biology, Université de Sherbrooke, Sherbrooke, QC, Canada
- 2Department of Surgery, Université de Sherbrooke, Sherbrooke, QC, Canada
- 3Department of Microbiology and Immunology, Dalhousie University, Halifax, NS, Canada
- 4Department of Medical Laboratory Technology, Faculty of Applied Medical Sciences, University of Tabuk, Tabuk, Saudi Arabia
- 5Immunology Research Program, King Abdullah International Medical Research Center, Riyadh, Saudi Arabia
- 6Research Center of the Centre hospitalier universitaire de Sherbrooke (CHUS), Sherbrooke, QC, Canada
Pancreatic ductal adenocarcinoma (PDAC) is a high fatality cancer with one of the worst prognoses in solid tumors. Most patients present with late stage, metastatic disease and are not eligible for potentially curative surgery. Despite complete resection, the majority of surgical patients will recur within the first two years following surgery. Postoperative immunosuppression has been described in different digestive cancers. While the underlying mechanism is not fully understood, there is compelling evidence to link surgery with disease progression and cancer metastasis in the postoperative period. However, the idea of surgery-induced immunosuppression as a facilitator of recurrence and metastatic spread has not been explored in the context of pancreatic cancer. By surveying the existing literature on surgical stress in mostly digestive cancers, we propose a novel practice-changing paradigm: alleviate surgery-induced immunosuppression and improve oncological outcome in PDAC surgical patients by administering oncolytic virotherapy in the perioperative period.
1 Background
Pancreatic ductal adenocarcinoma (PDAC) is a leading cause of cancer-related morbidity and mortality in the western world. A recent study by Rahib et al. demonstrated the rising global incidence of PDAC that is expected to surpass colorectal cancer to become the second leading cause of cancer-related deaths by 2030 (1). Whereas survival in various solid tumors has constantly improved in the last decade, only mild advances have been achieved in PDAC. A 5-year overall survival of only 11% (2), insufficient/limited treatment options and rising incidence, warrant the development of new therapeutic strategies against PDAC.
Metastatic disease is a main contributor to poor outcomes in PDAC (3, 4). By the time patients are diagnosed with pancreatic cancer, most cases are at a locally advanced or metastatic stage (5). This is in part attributed to the lack of symptoms and appropriate screening tools resulting in 80% of patients presenting with metastatic disease at initial diagnosis (5). For these patients, the 5-year overall survival drops drastically to less than 1% as there are no curative treatments and survival is measured in months (6). Currently, surgery remains the only curative treatment for PDAC. However, less than 10 to 20% of patients newly diagnosed with PDAC are eligible for surgery (7). Patients who can receive surgical care have the best prognosis, with a 5-year overall survival of 20% following surgery (5). Despite the curative intent of surgical resection, over 60% of patients will recur within the first two years after surgery (8, 9). This further supports the pressing need to develop new therapies and understand the mechanisms behind recurrence and metastatic spread of pancreatic cancer.
The paradoxical phenomenon of recurrence and metastatic spread following surgical resection has been documented in many solid cancers (10–12). While the underlying mechanism is not fully understood, there is compelling evidence to think surgery could play a role in residual disease progression, specifically in PDAC. It has been described that surgery creates a state of physical trauma and physiological stress that triggers cellular immune dysfunction (13, 14). Our group and others have shown that the postoperative period represents a unique time frame of immunosuppression that can be hijacked by a tumor for its survival advantage in different cancer types (15–18). However, the idea of surgery-induced immunosuppression as a facilitator of recurrence and metastatic spread has not been explored in the context of pancreatic cancer.
2 Standard of care for PDAC
Currently, surgery offers the only realistic chance to cure pancreatic cancer. However, less than 10 to 20% of patients newly diagnosed with PDAC are resectable (7, 19). Patients with tumors located in the head or the uncinate process of the pancreas are offered a pancreatoduodenectomy (PD-Whipple procedure), whereas tumors of the body or tail of pancreas are removed by performing a distal pancreatectomy and splenectomy (5). These procedures are amongst the most complex and aggressive cancer surgeries. Despite tremendous progress in patient care, patients present with long recovery time and high complication rates. Post-operative complications range from wound infection to catastrophic pancreatic fistula and even death (4, 5). Yet, 5-year overall survival after surgical resection alone remains poor at 10% (20, 21). Therefore, 6 months of adjuvant chemotherapy is combined with resectable PDAC because it improves long term survival after surgery (22). Patients with high functional status are offered a postoperative chemotherapy regimen composed of FOLFIRINOX, a highly effective, but cytotoxic chemotherapy. Patients with low functional status, on the other hand, are assigned gemcitabine and capecitabine based chemotherapy, a less effective, but more tolerable agent (22). However, despite adjuvant therapy, recurrence rates remain high, with 69 to 75% of patients experiencing relapse within 2 years following their surgery (4).
Patients with metastatic disease (locally advanced-20-40% and metastatic disease-40-60%) have a far grimmer prognosis. Survival at 5 years is well below 5% (19). The treatment offered is palliative chemotherapy (23). In fact, gemcitabine has been the first line of treatment of metastatic disease for the last 14 years since research has shown its therapeutic advantages in extending progression-free survival (PFS). Regrettably, even with gemcitabine, the overall survival (OS) of patients with metastatic illness is between five and six months (19, 23). The goal of therapy is to control disease progression and symptoms with quality of life as endpoint.
In the last decade, immunotherapy, and more specifically immune checkpoint inhibitors have emerged as a promising therapeutic avenue for PDAC. This modality relies on infiltration of the tumor by the immune system to induce a meaningful antitumor effect (24). However, PDAC has been shown to mostly resist immunotherapy (25–28). This can be attributed to the immunosuppressive tumor immune microenvironment (TIME) and scarce infiltration by effector T cells (29, 30). A small sub-population of PDAC patients respond to anti-PD1 checkpoint inhibitors. These patients have tested positive for specific gene changes, such as a high level of microsatellite instability-high (MSI-H) or changes in the mismatch repair (MMR) genes, which has led to the inclusion of anti-PD1 as second line treatment in recurrent and metastatic disease (23, 31, 32). However, less than 1% of PDAC patients express this genetic signature to benefit from the use of anti-PD1 (3).
3 Surgery induced immunosuppression in solid cancers
Immune suppression following surgical resection is a now well-established concept. This phenomenon can be observed across all types of solid tumors (Figure 1). For example, following surgery for colorectal cancer, interferon production was inhibited and NK cell function profoundly impaired (33). In addition, surgery reduced the overall amount of CD8+T cells, increased the number of myeloid derived suppressor cells (MDSC) in the peritoneal cavity, which was associated with worst overall survival for patients (34). Similarly, postoperative immunosuppression has also been demonstrated in lung (35–38), renal (39, 40), gastric, breast (41, 42), and prostate cancer (43). Systematically, the depth of immune suppression was directly correlated to the extent of surgical resection across these cancer types.
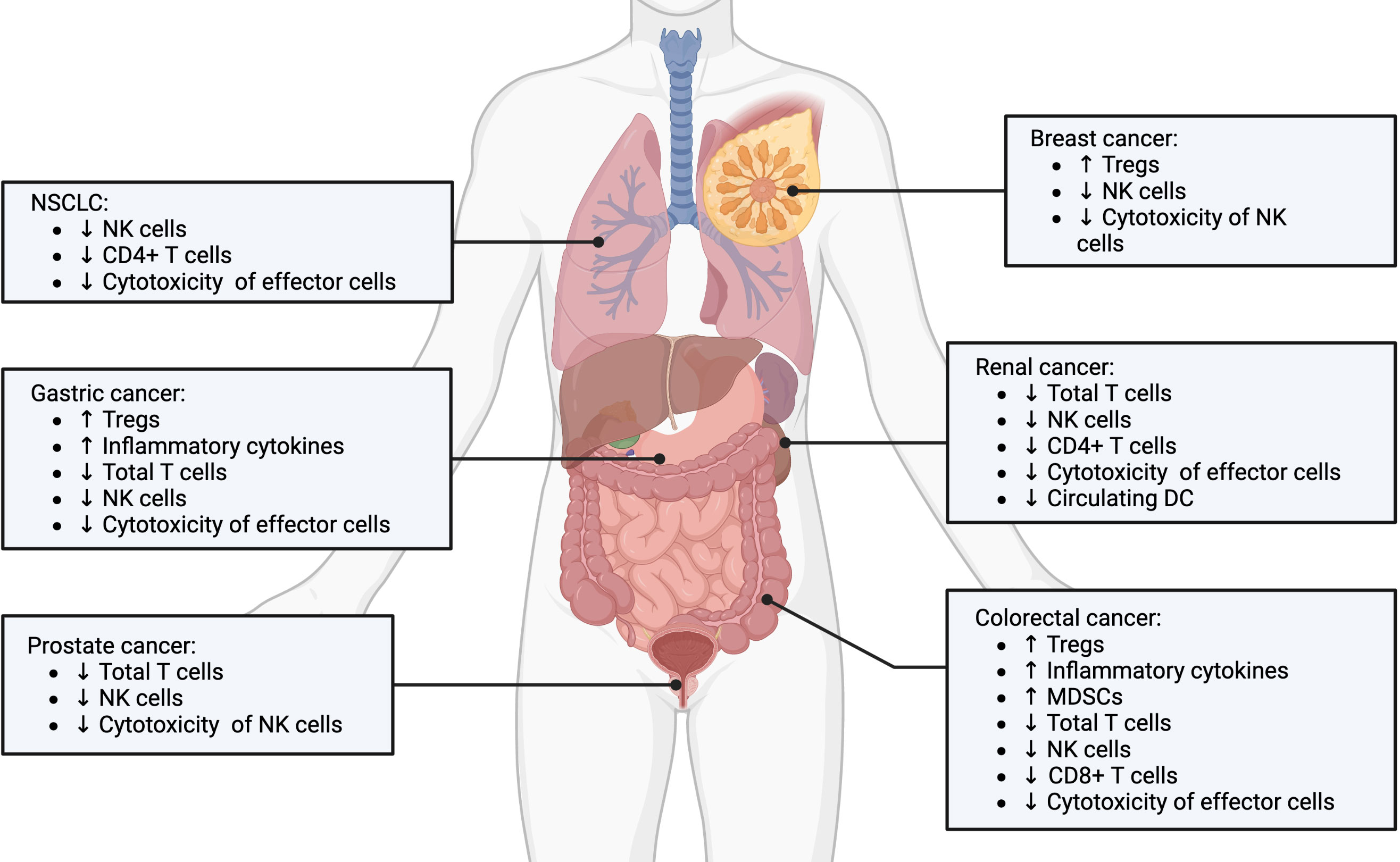
Figure 1 Postoperative immunosuppression in various solid cancer types. Postoperative immunosuppression is a stress and inflammation induced response that is observed across many types of solid tumors, including non-small cell lung, gastric, prostate, breast, renal and colorectal cancers. The underlying cellular mediators involve expansion of pro-tumorogenic regulatory immune cells and dysregulation of tumor-targeted effector immune cells. Created with BioRender.com.
The physical act of surgical excision instigates a stress response that provokes a surge in cortisol and catecholamines by the sympathetic nervous system (SNS), leading to the activation of physiological mechanisms such as the inflammatory response and angiogenesis that aids in tissue and wound healing. However, angiogenesis and inflammation have also been linked to the survival of residual tumor cells, metastasis, and recurrence of cancer (44, 45). Surgery related risk factors, including duration of surgery, extent of tissue damage, blood loss, hypothermia, and the administration of blood products have all been shown to contribute towards postoperative immunosuppression (14, 33, 45–49). These mediators of stress in turn raise levels of immunosuppressive molecules, such as IL-4, IL-10, TGF-β, and VEGF; and pro-inflammatory cytokines, such as IL-6 and IL-8 (50). These cytokine alterations have been found systemically and in longitudinal tumor resections following surgery (50). Previous and current studies connect these altered cytokine levels with suppression of the effector functions of tumor-targeted NK and effector T cells in the postoperative period (15, 16, 51–54). The activated SNS also promotes the growth of Th2 cells and T regulatory cells (Treg), consistent with Treg’s known functions in inflammation control and tissue healing (17, 45, 50, 55). Surgery induced immunosuppression is further reinforced through the expansion of MDSCs (56–58). These myeloid regulatory cells have been shown to promote tumor growth in the postoperative period by regulating the development of premetastatic niches, stimulating angiogenesis, and promoting tumor cell invasion (16, 45, 59). Notably, cancer prognosis and recurrence in patients with solid cancers are correlated with postoperative MDSC expansion and suppressive function (45). Postoperative cellular immunosuppression has been demonstrated to last several weeks following surgery, with overall dysfunction in NK and T cell function (16, 60) and decreased total lymphocyte counts (61). Studies have described a return to normal levels of cellular immunity weeks to a month after surgery (61, 62). However, recent clinical data suggest that cellular immunity may be compromised for up to 6 months following surgery (63).
Of note, neutrophils have been demonstrated to serve a critical role in tissue repair following surgical injury. This is in addition to their well-documented functions in defending against infection, and both promoting and guarding tumor growth and spread (64). In different cancer models, tumor-associated neutrophils (TANs) are linked with disease progression and poor overall survival (65–67). In the context of cancer surgery, neutrophils have been described to release neutrophil extracellular traps (NETs) that attract cancer cells. Surgical procedures induce the production of NETs, which can encourage the development of metastases by shielding against anti-tumorogenic factors and tumor-targeted immune cells (67, 68)
Taken together, these studies suggest the complex interplay between different immune cell populations, soluble mediators and cancer cells that favor the creation of a local and systemic immunosuppressive tumor microenvironment (TME) (Figure 2). This in turn tilts the balance in favor of promoting the growth of residual tumor cells, which is a critical factor in the capacity of tumors to evade both innate and adaptive immunity.
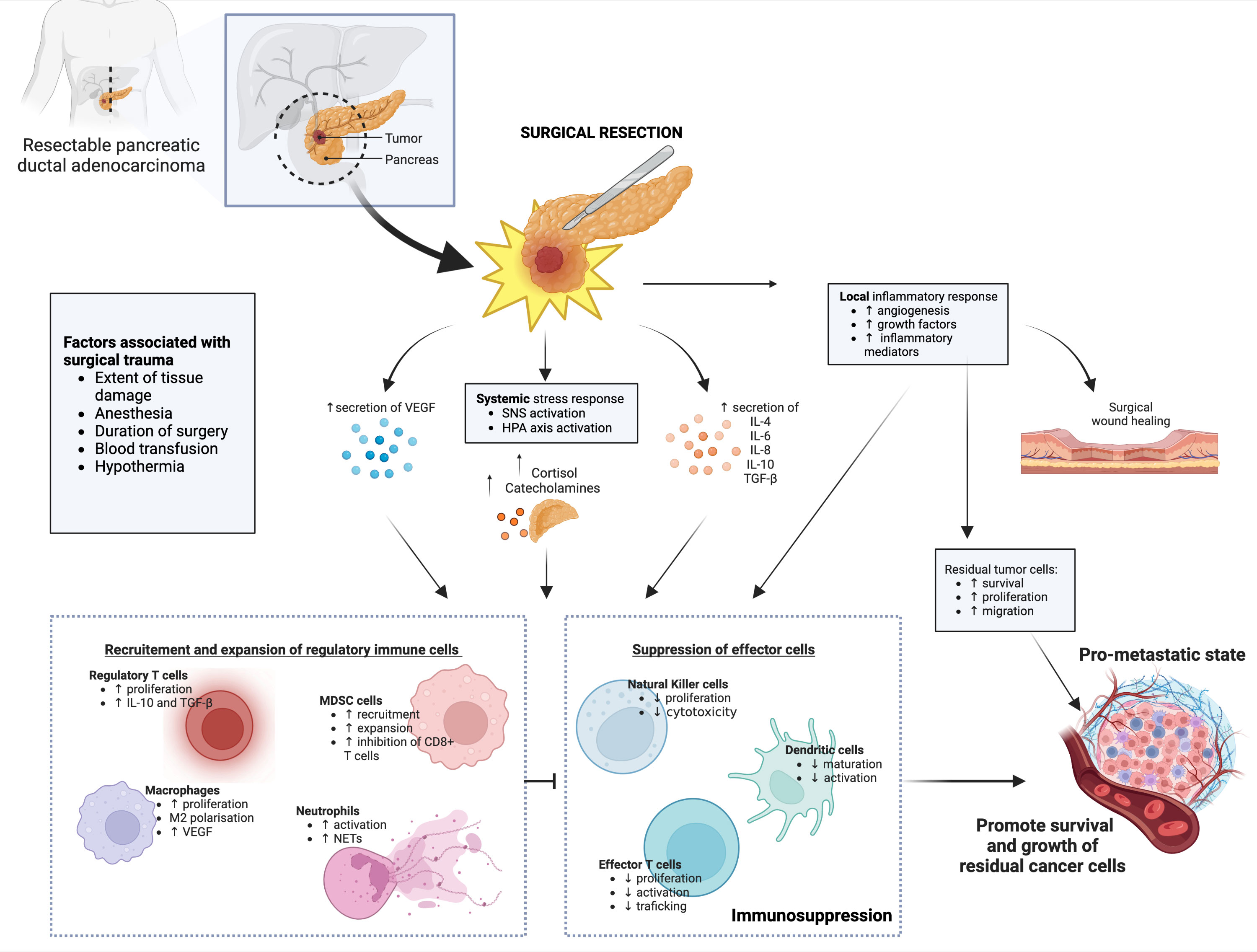
Figure 2 Schematic representation of the mechanisms of postoperative immunosuppression. Surgical resection of pancreatic cancers initiates local and systemic inflammatory and stress responses, which leads to dysregulated cytokine, growth factors and stress hormone secretion levels. While necessary for tissue and cellular repair following surgical excision, these inflammatory responses result in expansion and recruitment of regulatory immune cells, which in turn impair tumor-targeted effector immune cells. When combined with other negative factors associated with surgical trauma, such as duration of anesthesia, blood loss and hypothermia - a pro-metastatic state establishes in the patient, which promotes growth and migration and residual cancer cells. Abbreviations: SNS, Sympathetic nervous system; HPA, hypothalamic-pituitary-adrenal; VEGF, vascular endothelial growth factor; TGF-β, Transforming growth factor beta; MDSC, myeloid derived suppressor cells; NET, neutrophil extracellular traps; IL, interleukins. Created with BioRender.com.
4 Surgery induced immunosuppression in pancreatic cancer
Although we have previously demonstrated that there is a clear correlation between surgery and immune suppression in different solid tumors, there is a dearth of literature in the context of surgical resection for PDAC. Romano et al. was one of the first to show that compared to other gastrointestinal resectable tumors, PDAC surgical patients exhibited higher immune suppression in the postoperative period. There was a statistically significant reduction in total lymphocyte counts (TLC) at days 14 and 50 postoperative, comparing pancreatic to gastric/colorectal patients (69). Many other reports corroborate this finding of altered patient immune status following pancreatic resection leading to poorer survival (8, 70, 71). D’Engremont et al. demonstrated post-operative lymphopenia was an independent risk factor of mortality and lower survival in localized pancreatic cancer in a risk prediction model of 390 resectable PDAC patients. Four years after surgery, only 4.6% of patients with post-operative low TLC (under 1000/mm3) were alive compared to 22.8% of patients with a post-operative high TLC (above 1000/mm3) (70). The aggressive nature of PDAC was used to explain these outcomes without consideration of potential underlying immunosuppressive mechanisms leading to poorer oncologic outcomes.
More recently, Kim et al. confirmed that PDAC patients who successfully underwent curative pancreatectomy, exhibited changes in their immune status following surgery. In this study, most patients had normal TLC levels preoperatively. However, following surgery, TLC levels fell dramatically and neutrophil to lymphocyte ratios (NLR) spiked for 3 to 4 days after. These counts generally increased after the initial postoperative period but could remain altered for as long as 6 months. They showed that low TLC and high NLR in the post-operative period was an important predictor of poor outcome, while both led to worst overall and disease-free survival at 1, 2 and 3 years. When stratifying for NLR, a high value at 1 and/or 6 months postoperatively was a significant risk factor for PDAC recurrence (61). In a separate study by Pointer et al., a high NLR was predictive of patient survival and associated with poor prognosis after resection in early-stage PDAC (62). Postoperative neutrophil recruitment and NET formation, through the activation of STAT3 and NFκB pathways have been associated with recurrence and metastatic progression. This data could provide some mechanistic underpinnings to explain the above clinical observations (11, 72).
Our group previously demonstrated that surgical trauma induces profound NK cell dysfunction leading to poorer outcomes in preclinical models. We hypothesized that poor prognosis was mainly driven by NK cells which we know to play a crucial role in cancer cell clearance and immunosurveillance in humans (15, 57, 73). In 2015, Iannone et al. demonstrated that pancreatic cancer surgery decreased NK cell numbers and impaired their function, particularly their ability to release IFN-γ (74). Through a comprehensive examination of peripheral lymphocyte subsets in pancreatic cancer patients before and at various intervals after duodenopancreatectomy, they were able to demonstrate an alteration in NK cell numbers and function in patients at post-operative days 7 and 30. The same authors also performed an observational analysis performed on the small subset of patients who survived at 2-years. Their findings suggest the importance of NK cell frequency. In fact, survivors (8/12 patients) exhibited a median NK cell frequency distribution at post-operative day 30 that was significantly higher than that of deceased patients; this holds true even when advanced cancer patients were considered. At two months after surgery, NK cell numbers and function normalized for all patients. The authors suggest that the dysregulation of NK cell–modulating cytokines, such as IL-2, IL-12, and IL-18, an CD4/CD25 regulatory T-cell subsets in pancreatic cancer patients could set up an altered microenvironment, in which NK cells adopted aberrant proliferation, differentiation, and functional behavior, which correlated with patient survival.
While we await more mechanistic studies, clinical evidence provides support for the altered antitumor host defense after pancreatic resection and its effect on cancer outcome. Many groups refer to the unique period following surgery as a “perioperative window of opportunity” that if acted on could potentially alter the course of disease prognosis (45, 47, 49, 75). However, in a cancer where surgery is the only curative option, it is paramount to have a better understanding of the PDAC TIME, in order to turn this postoperative immunosuppression into a therapeutic window of opportunity.
5 The tumor microenvironment in PDAC
The TIME is classified according to the degree of immune T cell infiltration. Immunologically “hot” tumors display high rates of effector T cell infiltration. Classically “hot” cancers include melanoma, bladder, kidney, head and neck, and non-small cell lung cancer. On the other hand, “cold” tumors are nonimmunogenic as they exhibit low rates of effector T cell infiltration. Ovarian, prostate, brain and pancreatic cancers are typically cold tumors (24). The TIME has been shown to be a critical determinant of immunotherapy resistance. PDAC is one of the most stroma dense tumors, with up to 90% of its tumor volume made up of extracellular matrix (ECM) (76). This highly dense and fibrous stroma contributes to increased intra-tumoral pressure. The scar tissue-like desmoplasic stroma creates a physical barrier around the tumor cells, while the hypoxic gradients recruit fibroblasts and leukocytes, which together impedes both the entry and efficacy of existing therapeutics (77, 78). In response to factors secreted by the tumor and the hypoxic microenvironment, cancer-associated fibroblasts (CAF) secrete high quantities of collagen and extra-cellular matrix, contributing further to the desmoplasia. Moreover, their cross talk with multiple components of the immune system helps to maintain a stubborn state of immune suppression (79, 80).
As for immune infiltrates, their presence in PDAC is complex and heterogeneous. Myeloid cells are the most prominent population (>50%) and are associated with worse prognosis in patients with surgically resected PDAC. MDSCs are present early in the carcinogenesis of PDAC and play a central role in the immunosuppressive environment (81–83). Likewise, tumor associated macrophages (TAM) are in relative abundance in PDAC. Macrophages are polarized to their immunosuppressive M2 phenotype and are thought to increase in number with invasiveness of the tumor and are associated with worse clinical outcomes (55). In terms of dendritic cells (DCs), they are present in low numbers and are mostly immature, rendering them dysfunctional (84). NK cells, which are essential for the recognition and elimination of cancer cells, are not frequent and are mostly dysfunctional (81). Similarly, PDAC tumors present few tumor infiltrating lymphocytes (TILs) and most of them are Treg cells, a subset of T-cells known for their immunosuppressive activity. As for effector CD8+ cytotoxic T-cells and CD4+ helper T-cells, they are found in lower overall proportion in PDAC. Regarding their spatial contexture, tumor infiltrating CD3+ T-cells are located far from cancer cells and are trapped within the dense stroma. Furthermore, these CD3+ T cells are most often found in a state of exhaustion and less than 1% demonstrate tumor reactivity (30). It is well established that the presence of TILs and DCs is associated with better prognosis for patients. Overall, this paints a heavily immune suppressed TIME, which favors tumor escape and therapy resistance (28, 55).
6 Immune evasive mechanisms in PDAC
The immune evasion mechanisms employed by PDAC allows the cancer to proliferate and spread months to years before the first clinical symptoms are detected (85). This can be partially attributed to pancreatic tumor cell-intrinsic pathways, driven by mutated KRAS. Oncogenic mutated KRAS is found in up to 90% of patients and is thought to be the driving mutation in PDAC oncogenesis (4). Pro-tumorogenic cytokines and chemokines, such as IL-6, IL-8, IL-10, TGF-β, M-CSF, VEGF and CXCL12, are released by PDAC cells and are tightly controlled by oncogenic KRAS dependent mechanisms. The abundant release of these soluble mediators tips the immunological balance from effective immune surveillance to immune escape. The proliferation, migration, and angiogenesis of tumor cells are all influenced by these immunological alterations (86). The secretion of this cocktail of modulators recruits and activates immunosuppressive cells, such as MDSCs, TAMs, Treg cells, CAFs, Th2 cells, and neutrophils into the TIME (87). The recruitment of these immunosuppressive cell subsets coupled to the heavy release of pro-tumorogenic cytokines listed above prevent the anti-tumor functions of NK and CD4+/CD8+ T cells and prevent the maturation and survival of DCs (82, 86). Since DCs are potent antigen-presenting cells (APCs) that are crucial for activating an effective anti-tumor T cell response, lower DC levels in blood and tumor tissue of PDAC patients have been correlated with diminished survival (56). PDAC also possesses a low mutation burden, another feature which allows it to evade the immune system (88). The resulting absence/rarity of neoantigens, which act as immune targeting molecules necessary to induce a productive T cell response, also contribute to poor infiltration by T cells (89).
In parallel, PDAC cells downregulate their antigen presentation machinery. This is achieved by the downregulation of major histocompatibility complex (MHC) class I molecules, which allows cancer cells to escape CD8+ T cell recognition (90). Furthermore, there is an upregulation of inhibitory immune checkpoint ligands, including PD-L1, which promotes T-cell dysregulation and loss of effector function (91).
Through the activation of CAFs, PDAC is also able to physically restrict anti-tumor immune cells from entering the TME, in addition to immunological evasion (92, 93). Through collagen deposition and ECM reorganizations, these CAFs encourage fibrosis, which causes a desmoplastic reaction. This creates a physical barrier that prevents normal vascularization, hinders the infiltration of anti-tumor immune cells, and contributes to therapeutic resistance (79, 92, 94). The dense fibrous stroma of PDAC further contributes to immune evasion by creating a hypoxic and hostile environment for effector T cells.
VEGF overexpression is a frequent finding in biopsies of human pancreatic tumors, demonstrating the importance of hypoxia in PDAC pathophysiology (95, 96). Hypoxia is thought to influence tumor development dynamics and confers immunotherapeutic resistance. Hypoxia-induced production of VEGF, along with other cytokines (such as IL-10, IL-6, and G-CSF) inhibit DC maturation, hence decreasing overall numbers of DCs and rendering dysfunction in CD3+ T cells. In addition, matrix metalloprotease type-9 (MMP-9) generated by tumor-associated neutrophils (TANs) and macrophages in the TIME potentiate the effects of VEGF. Indeed, the overexpression of MMP-9 seen in PDAC plays a critical and complex role in immune suppression, tumor progression, invasion, and metastasis (94, 97). During carcinogenesis, MMP-9 increases endothelial cell migration and activates the angiogenic switch in tumors through the release of VEGF from the matrix, suggesting the pro-angiogenic role of MMP-9 in cancer tissues (98). In contrast, the direct proteolytic action of MMP-9 leads to cancer spread, most likely via the control of VEGF and angiostatin production and in association with angiogenesis (99, 100). This suggests that the increased vascular permeability leading to early dissemination of PDAC is partly induced by the over-expression of MMP-9 in the TME (98, 101).
Hypoxia also plays a role in immune evasion through the activation of pancreatic stellate cells (PSC). PSC are myofibroblast-like cells that regulate the synthesis of extracellular matrix (ECM). Although the synthesis, deposition, and remodeling of fibrous connective tissue is protective at steady state, over-activated PSCs hinder normal pancreatic function. Recently, PSCs have emerged as a focal point in pancreatic cancer research in their potential regulation of PDAC carcinogenesis. There are emerging data describing their contributing towards the depletion and malfunction of T cells as well as chemoresistance (21). Activated PSCs govern T-cell migration and block anti-tumor CD8+ T-cells from approaching cancer cell clusters, thus reducing cancer cell access (102, 103). Activated PSCs also accelerate the polarization of M1 macrophages towards a pro-tumoral M2 phenotype (104).
7 Treating postoperative immunosuppression in PDAC with oncolytic virotherapy
Very little research has been conducted on overcoming surgery induced immunosuppression in PDAC using immunomodulators aside from a few studies of perioperative cytokine and chemotherapy delivery. In 2008, a clinical trial was conducted to determine the effect of presurgical IL-2 immunotherapy on immune status and survival in PDAC patients undergoing pancreatic resection. Thirty consecutive patients undergoing a Whipple (pancreatoduodenectomy) procedure were randomly assigned preoperative IL-2 immunotherapy plus surgery or surgery alone. In the immediate post-operative period, patients treated with IL-2 displayed no post-operative lymphopenia as they had higher total lymphocyte counts compared to the control group. After 36 months of follow-up, the free-from-progression period (FFPP) and OS were increased in patients treated with IL-2 (105). This underpowered study provided evidence that immune modulation in the perioperative period could lead to improved oncological outcomes. However, the low half-life and potential toxicity of systemic IL-2 therapy limited its clinical progress.
Compared to other immunotherapeutic modalities, oncolytic viruses (OV) attack cancer cells in a multimodal way. OVs are a class of naturally occurring or bioengineered live therapeutics that selectively infect, replicate within, and lyse cancer cells, resulting in immunogenic cell death (ICD) of the tumor cells (106). The activation of cell stress and danger pathways along with the release of otherwise hidden tumor associated antigens (TAA), recruits and activates tumor-targeted immune effector cells. The tumor is prevented from eluding the immune system and results in a rapid, specific, and long-lasting anti-tumor immune response against TAA, both at the primary tumor site and at distant metastatic sites (106, 107) (Figure 3). While the use of OV for PDAC treatment has been explored in numerous preclinical and clinical settings (108–114), the timing of OV administration in the surgical context nor its potential to combat postoperative immunosuppression have been investigated in the setting of PDAC. Increasing preclinical and clinical evidence supports the benefits of neoadjuvant systemic therapy for all patients with resectable PDAC (20, 115). Kubo et al. examined the association between neoadjuvant therapy and pathological outcomes in patients with PDAC following resection. They found that patients who received neoadjuvant therapy had lower rates of micro-vascular invasion (MVI) (43%) compared to patients who had surgery without neoadjuvant treatment (62%). Patients with MVI had an odds ratio of 2.38 to experience liver recurrence and MVI was found to be an important prognostic factor (116). These findings highlight the importance of the neoadjuvant treatment period and reinforces the importance of research to rationalize timing and combination treatments to optimize outcome for resectable PDAC patients. When it comes to OV dosing and administration strategy, Warner et al. summarize it into 3 categories: (1) Hit hard and early – the administration of a highly concentrated dose once and early in the treatment regimen; (2) kill softly - frequent small doses until tumor regression; and (3) prime-boost approach – high dose of a first virus to prime an immune response, followed days to weeks later by a second high dose of a different virus to boost the anti-tumoral immune response and maximize oncolysis without the rapid antigen-based viral clearance targeted at the prime virus (117). For now, there is no clear evidence to which strategy is best when it comes to PDAC. Given that surgery is the only chance for cure in PDAC patients, we postulate that perioperative oncolytic virotherapy administered to PDAC patients immediately before or after surgery may prime anti-tumor immune responses and prevent or reduce postoperative immunosuppression. Cell-mediated immunity may be more effectively activated immediately prior to the immunosuppressive effects of surgery (49, 118). Our group previously showed in a mouse model of surgical stress that neoadjuvant administration of oncolytic vaccinia virus can reverse NK cell dysfunction and reduce postoperative colorectal lung metastases (15, 53, 57). In a phase 1b window-of-opportunity trial, 9 patients bearing colorectal liver metastases or metastatic melanoma scheduled for surgery, went on to receive neoadjuvant oncolytic vaccinia virus (JX-594) 10 to 22 days prior to surgery (119). Neoadjuvant OV increased both NK and T cell functional anti-tumor immune responses in all patients. Moreover, activated T cell response remained for up to 3 months following viral infusion in all patients. Notably, these functional immune findings translated to increased survival. At the 3-year follow-up, 5 of 9 patients were still alive and 3 patients remained cancer-free. While these results were not obtained from PDAC patients, it is not difficult to envisage the potential of OV in the perioperative period following pancreatic resection, which often bears liver metastases and may have overlapping pathways of tissue repair and immunosuppression. On a clinical note, preventing immune suppression following surgery, could also have the added benefit of decreasing post-operative infections rates and this could lead to further improvements in survival for already fragile PDAC surgical patients.
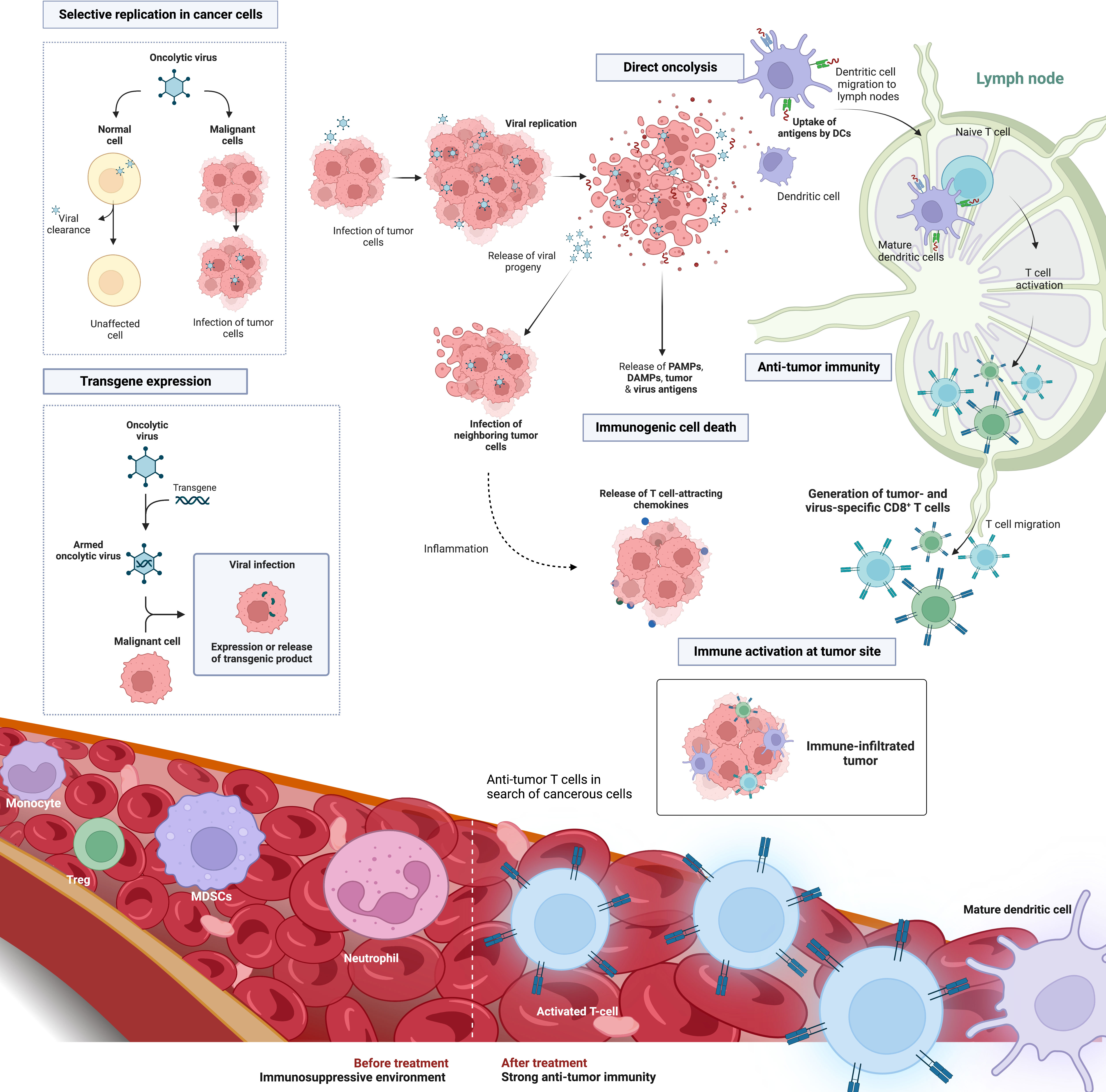
Figure 3 Oncolytic virus platform in the treatment of solid cancers. OVs are a versatile tumor targeting therapeutic platform involving direct tumor cell oncolysis, immune activation and transgene expression to enhance tumor lysis. Selective oncolysis enables viral replication as well as infection and lysis of both neighboring and distant tumor cells. Viral replication results in the release of both viral and tumor-associated antigens. Depending on the viral backbone, oncolysis is characterized by an immunogenic form of cell death and the release of immunomodulatory molecules such as DAMPs and PAMPs. This causes the recruitment and maturation of dendritic cells that may cross-present TAAs to CD8+T cells, hence producing TAA-specific T-cell populations. These biomarkers of ICD recruit and activate tumor-targeted immune T cells that infiltrate the TME. OVs can also be engineered to harbor select transgenes that can further enhance tumor eradiation. Examples include immune stimulating cytokines, tumor-targeted miRNAs, and reporter genes to track viral infection. Abbreviations: OV, oncolytic virus; ICD, immunogenic cell death; DAMPs, danger associated molecular patterns; PAMPs, pathogen associated molecular patterns; TAA, Tumor associated antigen; TME, tumor microenvironment; miRNA, microRNAs. Created with BioRender.com.
8 Clinical considerations for perioperative virotherapy in PDAC patients
Currently, oncolytic virotherapy is experiencing a resurgence in research and clinical interest. However, it is a relatively young field of research. There is little human data and few clinical trials of its use in the perioperative period, let alone in PDAC. For future research, certain critical elements need to be prudently considered when planning clinical trials and future treatments.
Firstly, the timing of administration needs to be carefully evaluated. Matzner et al. make the compelling argument that the focus needs to be on the immediate perioperative period to start treatment (75). According to the National Cancer Institute, this perioperative period loosely refers to the weeks prior to surgery, followed by admission, hospitalization, preparations for surgery, anesthesia, and surgical procedures, as well as following convalescence and functional recovery. In clinical trials, interventions limited to the immediate perioperative period have been shown to improve disease-free survival and overall survival (12, 51, 120, 121). Furthermore, preclinical studies have demonstrated repeatedly the anti-metastatic effect of combining surgery with immunomodulatory approaches (51, 122, 123). Therefore, the scheduling of neoadjuvant virotherapy could be limited to this small treatment window before resection. Virotherapy could also be administered in the days following surgery to stay within the perioperative window. However, the immediate days following resection is a critical period for wound healing and tissue repair, especially in complex pancreas surgery (124). Any intervention that disrupts this complex organ and the physiological processes that it regulates, could result in catastrophic surgical complications such as post-operative pancreatic fistula or pancreatitis (7). The existing evidence appears to suggest that the immediate perioperative period is disproportionately critical in predicting long-term cancer outcomes. Therefore, any clinical success in this period yields the potential for long lasting effects on patients’ survival.
Secondly, we need to consider the best route of administration. As with any treatment, the administration method must be thoroughly investigated. This is critical when establishing an OV treatment for a disease as aggressive as PDAC. Most clinical trials of OVs in other solid tumors have disproportionately selected intratumoral administration to increase viral biodistribution to cancer cells and to prevent neutralization by systemic humoral immune responses. While the rationale is undeniable, it is important to understand the disease we are treating. The primary drawback of intratumoral delivery is the difficulty of reaching deep visceral tumors such as pancreatic cancer, particularly when repeated treatment is required (125). Therefore, when designing a novel OV trial for PDAC, this component needs to be factored in the decision-making process. Although endoscopic trans-gastric access permits intratumoral injection, repeated delivery may expose patients to an elevated risk of complications. In addition, endoscopic access may be difficult in the early post-operative period. Most patients who have Whipple surgery will have extensive restoration of their proximal digestive tract (3, 126). Therefore, endoscopic operations during the early perioperative period may significantly compromise the structural integrity of a newly created anastomosis and increase the chance of a catastrophic anastomotic leak. All things considered, intravenous administration of OV could be the more pragmatic and safer method for treating PDAC. There have been reports of successful intravenous administration of OV, but the therapeutic benefit has yet to be validated in larger, randomized clinical trials.
Finally, many other factors related to the OV under investigation should be taken into consideration. This includes choosing the appropriate viral backbone, arming, or not arming the virus with a payload, the type of payload to use, using OV as a monotherapy or in combination with existing standard-of-care chemotherapy or immunotherapy, etc. (125, 127). These are some virus-related factors to take into account for future PDAC trials, but a deeper discussion of these considerations goes beyond the scope of this review. One thing is certain, OV is an extremely versatile platform with great potential to both directly target pancreatic cancer and reverse the immunosuppressive effect of surgical stress on the pathophysiology of PDAC. Concerns related to the potential negative effects of virotherapy must be carefully weighed against the possible advantages of multi-pronged immunomodulation. As previously suggested, the immunostimulating capacity of OV might result in both improved survival outcomes and reduction in post-operative infectious complications. A delicate balance must be struck between immune activation and post-operative adverse events. However, the lack of perioperative clinical trials in surgical cancer patients do not allow us to directly assess these critical issues, leaving clinically relevant questions unanswered and underpinning mechanisms unknown.
9 Conclusions and perspectives
Due the improvements observed in the past decades for chemotherapeutic regimens (mFolfirinox, Gemcitabine-nab-Paclitaxel), better perioperative care and centralization of pancreatic surgery to high volume centers, neoadjuvant/perioperative immunotherapy for the treatment of PDAC has lost momentum (120). While mortality and morbidity following surgery have decreased (4, 128–130), survival, however, remains stagnant (2, 131). Emerging successes following immunotherapy treatments in other aggressive solid cancers provide the rationale to pursue immunotherapy and novel viro-immunotherapies in high-fatality PDAC. Given the cold immune phenotype of PDAC, we reason that oncolytic virotherapy, administered within the perioperative window of opportunity should be exploited to maximize survival benefits for patients. Oncolytic virotherapy, an emerging, multi-mechanistic therapy should be considered as viable therapeutics for the treatment of PDAC or as an essential component of multimodal therapy, which takes into consideration timing of treatment and benefits/drawbacks of frontline therapies. This will only be feasible with further research and clinical studies to understand the therapeutic potential of the perioperative period.
Author contributions
SM and LHT conceived the main ideas for this paper, wrote and revised the manuscript. LD wrote and revised the manuscript. NA, ML, JEB, AAA and YC revised the manuscript. YC and LHT provided supervision for the activities of the manuscript. All authors contributed to the article and approved the submitted version.
Funding
This study was supported by the Université de Sherbrooke CRMUS Chair for Translational Immunotherapy Research and the Deanship of Scientific Research at the University of Tabuk through project no. (0305-1440-S).
Conflict of interest
The authors declare that the research was conducted in the absence of any commercial or financial relationships that could be construed as a potential conflict of interest.
Publisher’s note
All claims expressed in this article are solely those of the authors and do not necessarily represent those of their affiliated organizations, or those of the publisher, the editors and the reviewers. Any product that may be evaluated in this article, or claim that may be made by its manufacturer, is not guaranteed or endorsed by the publisher.
References
1. Rahib L, Wehner MR, Matrisian LM, Nead KT. Estimated projection of US cancer incidence and death to 2040. JAMA Netw Open (2021) 4(4):e214708–e214708. doi: 10.1001/jamanetworkopen.2021.4708
2. Siegel RL, Miller KD, Fuchs HE, Jemal A. Cancer statistics, 2022. CA Cancer J Clin (2022) 72(1):7–33. doi: 10.3322/caac.21708
3. Robatel S, Schenk M. Current limitations and novel perspectives in pancreatic cancer treatment. Cancers (Basel) (2022) 14(4). doi: 10.3390/cancers14040985
4. Park W, Chawla A, O’Reilly EM. Pancreatic cancer: A review. JAMA (2021) 326(9):851–62. doi: 10.1001/jama.2021.13027
5. Wright FC, Escallon JM, Cukier M, Tsang ME, Hameed U. Surgical oncology manual. 3rd edition Toronto, Canada, Springer (2020)
6. Niesen W, Hank T, Büchler M, Strobel O. Local radicality and survival outcome of pancreatic cancer surgery. Ann Gastroenterol Surg (2019) 3(5):464–75. doi: 10.1002/ags3.12273
7. Zeni LB, Russi FR, Faleiro Fialho A, Fonseca ALP, Sombrio LS, Rocha IC. Morbidity and mortality of pancreatic tumors undergoing surgical treatment. Arq Bras Cir Dig (2014) 27(4):275. doi: 10.1590/S0102-67202014000400011
8. Sohn TA, Yeo CJ, Cameron JL, Koniaris L, Kaushal S, Abrams RA, et al. Resected adenocarcinoma of the pancreas-616 patients: Results, outcomes, and prognostic indicators. J Gastrointest Surg (2000) 4(6):567–79. doi: 10.1016/S1091-255X(00)80105-5
9. Kalisvaart M, Broadhurst D, Marcon F, Pande R, Schlegel A, Sutcliffe R, et al. Recurrence patterns of pancreatic cancer after pancreatoduodenectomy: Systematic review and a single-centre retrospective study. HPB. (2020) 22(9):1240–9. doi: 10.1016/j.hpb.2020.01.005
10. Tohme S, Simmons RL, Tsung A. Surgery for cancer: A trigger for metastases HHS public access. Cancer Res (2017) 77(7):1548–52. doi: 10.1158/0008-5472.CAN-16-1536
11. Alieva M, van Rheenen J, Broekman MLD. Potential impact of invasive surgical procedures on primary tumor growth and metastasis. Clin Exp Metastasis (2018) 35(4):319–31. doi: 10.1007/s10585-018-9896-8
12. Neeman E, Ben-Eliyahu S. The perioperative period and promotion of cancer metastasis: New outlooks on mediating mechanisms and immune involvement. Brain Behav Immun (2013) 30(Suppl):S32. doi: 10.1016/j.bbi.2012.03.006
13. Menges P, Kessler W, Kloecker C, Feuerherd M, Gaubert S, Diedrich S, et al. Surgical trauma and postoperative immune dysfunction. Eur Surg Res (2012) 48(4):180–6. doi: 10.1159/000338196
14. Hogan BV, Peter MB, Shenoy HG, Horgan K, Hughes TA. Surgery induced immunosuppression. Surgeon (2011) 9(1):38–43. doi: 10.1016/j.surge.2010.07.011
15. Seth R, Tai LH, Falls T, de Souza CT, Bell JC, Carrier M, et al. Surgical stress promotes the development of cancer metastases by a coagulation-dependent mechanism involving natural killer cells in a murine model. Ann Surg (2013) 258(1):158–68. doi: 10.1097/SLA.0b013e31826fcbdb
16. Ananth AA, Tai LH, Lansdell C, Alkayyal AA, Baxter KE, Angka L, et al. Surgical stress abrogates pre-existing protective T cell mediated anti-tumor immunity leading to postoperative cancer recurrence. PloS One (2016) 11(5). doi: 10.1371/journal.pone.0155947
17. Chen Z, Zhang P, Xu Y, Yan J, Liu Z, Lau WB, et al. Surgical stress and cancer progression: The twisted tango. Mol Cancer (2019) 18:1. doi: 10.1186/s12943-019-1058-3
18. Meguro S, Haga N, Imai H, Yoshida Y, Takinami-Honda R, Matsuoka K, et al. Association between surgical stress and biochemical recurrence after robotic radical prostatectomy. JSLS (2021) 25(1):1475–88. doi: 10.4293/JSLS.2020.00078
19. Smithy JW, O’ EM, Md R, O’reilly EM. Pancreas cancer: Therapeutic trials in metastatic disease. J Surg Oncol (2021) 123(6):1475–88. doi: 10.1002/jso.26359
20. Versteijne E, van Dam JL, Suker M, Janssen QP, Groothuis K, Akkermans-Vogelaar JM, et al. Neoadjuvant chemoradiotherapy versus upfront surgery for resectable and borderline resectable pancreatic cancer: Long-term results of the Dutch randomized PREOPANC trial. J Clin Oncol (2022) 40(11):1220–30. doi: 10.1200/JCO.21.02233
21. Wang S, Li Y, Xing C, Ding C, Zhang H, Chen L, et al. Tumor microenvironment in chemoresistance, metastasis and immunotherapy of pancreatic cancer. Am J Cancer Res (2020) 10(7):1937.
22. Conroy T, Hammel P, Hebbar M, Abdelghani M, Wei AC, Raoul JL, et al. FOLFIRINOX or gemcitabine as adjuvant therapy for pancreatic cancer. New Engl J Med (2018) 379(25):2395–406. doi: 10.1056/NEJMoa1809775
23. Strobel O, Büchler MW. Pancreatic cancer: Clinical practice guidelines-what is the evidence? Nat Rev Clin Oncol (2016) 13(10):593. doi: 10.1038/nrclinonc.2016.127
24. Galon J, Bruni D. Approaches to treat immune hot, altered and cold tumours with combination immunotherapies. Nat Rev Drug Discovery (2018) 18:3. doi: 10.1038/s41573-018-0007-y
25. Schizas D, Charalampakis N, Kole C, Economopoulou P, Koustas E, Gkotsis E, et al. Immunotherapy for pancreatic cancer: A 2020 update. Cancer Treat Rev (2020) 86:102016. doi: 10.1016/j.ctrv.2020.102016
26. Carpenter E, Nelson S, Bednar F, Cho C, Nathan H, Sahai V, et al. Immunotherapy for pancreatic ductal adenocarcinoma. J Surg Oncol (2021) 123(3):751–9. doi: 10.1002/jso.26312
27. Miyazawa M, Katsuda M, Kawai M, Hirono S, Okada K, Kitahata Y, et al. Advances in immunotherapy for pancreatic ductal adenocarcinoma. J Hepatobiliary Pancreat Sci (2021) 28(5):419–30. doi: 10.1002/jhbp.944
28. Principe DR, Korc M, Kamath SD, Munshi HG, Rana A. Trials and tribulations of pancreatic cancer immunotherapy. Cancer Lett (2021) 504:1–14. doi: 10.1016/j.canlet.2021.01.031
29. Bazhin AV, Shevchenko I, Umansky V, Werner J, Karakhanova S. Two immune faces of pancreatic adenocarcinoma: possible implication for immunotherapy. Cancer Immunol Immunother (2014) 63(1):59–65. doi: 10.1007/s00262-013-1485-8
30. Beatty GL, Eghbali S, Kim R. Deploying immunotherapy in pancreatic cancer: Defining mechanisms of response and resistance. Am Soc Clin Oncol Educ Book (2017) 37):267–78. doi: 10.1200/EDBK_175232
31. Mahalingam D, Wilkinson GA, Eng KH, Fields P, Raber P, Moseley JL, et al. Pembrolizumab in combination with the oncolytic virus pelareorep and chemotherapy in patients with advanced pancreatic adenocarcinoma: A phase ib study. Clin Cancer Res (2020) 26(1):71–81. doi: 10.1158/1078-0432.CCR-19-2078
32. Ullman NA, Burchard PR, Dunne RF, Linehan DC. Immunologic strategies in pancreatic cancer: Making cold tumors hot. J Clin Oncol (2022) 40(24):2789–805. doi: 10.1200/JCO.21.02616
33. Torrance HDT, Rebecca Longbottom E, Vivian ME, Lalabekyan B, Abbott TEF, Ackland GL, et al. Post-operative immune suppression is mediated via reversible, interleukin-10 dependent pathways in circulating monocytes following major abdominal surgery. PloS One (2018) 13(9). doi: 10.1371/journal.pone.0203795
34. Xu P, He H, Gu Y, Wang Y, Sun Z, Yang L, et al. Surgical trauma contributes to progression of colon cancer by downregulating CXCL4 and recruiting MDSCs. Exp Cell Res (2018) 370(2):692–8. doi: 10.1016/j.yexcr.2018.07.035
35. Leaver HA, Craig SR, Yap PL, Walker WS. Lymphocyte responses following open and minimally invasive thoracic surgery. Eur J Clin Invest (2000) 30(3):230–8. doi: 10.1046/j.1365-2362.2000.00622.x
36. Whitson BA, D’Cunha J, Andrade RS, Kelly RF, Groth SS, Wu B, et al. Thoracoscopic versus thoracotomy approaches to lobectomy: differential impairment of cellular immunity. Ann Thorac Surg (2008) 86(6):1735–44. doi: 10.1016/j.athoracsur.2008.07.001
37. Zhang S, Pan SB, Lyu QH, Wu P, Qin GM, Wang Q, et al. Postoperative regulatory T-cells and natural killer cells in stage I nonsmall cell lung cancer underwent video-assisted thoracoscopic lobectomy or thoracotomy. Chin Med J (Engl) (2015) 128(11):1502–9. doi: 10.4103/0366-6999.157672
38. Ng CSH, Lee TW, Wan S, Wan IYP, Sihoe ADL, Arifi AA, et al. Thoracotomy is associated with significantly more profound suppression in lymphocytes and natural killer cells than video-assisted thoracic surgery following major lung resections for cancer. Journal of Investigative Surgery (2009) 18(2):81–8. doi: 10.1080/08941930590926320
39. Böhm M, Ittenson A, Philipp C, Röhl FW, Ansorge S, Allhoff EP. Complex perioperative immuno-dysfunction in patients with renal cell carcinoma. J Urol (2001) 166(3):831–6. doi: 10.1016/s0022-5347(05)65846-6
40. Klatte T, Ittenson A, Röhl FW, Ecke M, Allhoff EP, Böhm M. Perioperative immunomodulation with interleukin-2 in patients with renal cell carcinoma: Results of a controlled phase II trial. Br J Cancer (2006) 95:9. doi: 10.1038/sj.bjc.6603391
41. Li Y, Zhou L, Sun B, Li X, Duan K, Wu Y, et al. Interleukin-2 administration after modified radical mastectomy in breast cancer therapy increases peripheral regulatory T cells. Int J Clin Exp Med (2015) 8(5):7816.
42. Ma X, Wang M, Yin T, Zhao Y, Wei X. Myeloid-derived suppressor cells promote metastasis in breast cancer after the stress of operative removal of the primary cancer. Front Oncol (2019) 9:9:855. doi: 10.3389/fonc.2019.00855
43. Lu YC, Kuo MC, Hong JH, Jaw FS, Huang CY, Cheng JCH, et al. Lower postoperative natural killer cell activity is associated with positive surgical margins after radical prostatectomy. J Formosan Med Assoc (2020) 119(11):1673–83. doi: 10.1016/j.jfma.2019.12.015
44. Choi H, Hwang W. Perioperative inflammatory response and cancer recurrence in lung cancer surgery: A narrative review. Front Surg (2022) 9. doi: 10.3389/fsurg.2022.888630
45. Tang F, Tie Y, Tu C, Wei X. Surgical trauma-induced immunosuppression in cancer: Recent advances and the potential therapies. Clin Transl Med (2020) 10(1):199. doi: 10.1002/ctm2.24
46. Ramirez MF, Cata JP. Anesthesia techniques and long-term oncological outcomes. Front Oncol (2021) 11:5197. doi: 10.3389/fonc.2021.788918
47. Shakhar G, Ben-Eliyahu S. Potential prophylactic measures against postoperative immunosuppression: Could they reduce recurrence rates in oncological patients? Ann Surg Oncol (2003) 10:8. doi: 10.1245/ASO.2003.02.007
48. Kim R. Effects of surgery and anesthetic choice on immunosuppression and cancer recurrence. J Transl Med (2018) 16(1):1–13. doi: 10.1186/s12967-018-1389-7
49. Bakos O, Lawson C, Rouleau S, Tai LH. Combining surgery and immunotherapy: Turning an immunosuppressive effect into a therapeutic opportunity. J Immunother Cancer (2018) 6(1):1–11. doi: 10.1186/s40425-018-0398-7
50. Predina J, Eruslanov E, Judy B, Kapoor V, Cheng G, Wang LC, et al. Changes in the local tumor microenvironment in recurrent cancers may explain the failure of vaccines after surgery. Proc Natl Acad Sci USA (2013) 110(5):E415–24. doi: 10.1073/pnas.1211850110
51. Ben-Eliyahu S. Tumor excision as a metastatic Russian roulette: Perioperative interventions to improve long-term survival of cancer patients. Trends Canc (2020) 6(11):951–9. doi: 10.1016/j.trecan.2020.06.004
52. Benish M, Ben-Eliyahu S. Surgery as a double-edged sword: A clinically feasible approach to overcome the metastasis-promoting effects of surgery by blunting stress and prostaglandin responses. Cancers (Basel) (2010) 2(4):1929–51. doi: 10.3390/cancers2041929
53. Tai LH, Zhang J, Auer RC. Preventing surgery-induced NK cell dysfunction and cancer metastases with influenza vaccination. Oncoimmunology (2013) 2(11). doi: 10.4161/onci.26618
54. Niavarani SR, Lawson C, Tai LH. Treatment of metastatic disease through natural killer cell modulation by infected cell vaccines. Viruses (2019) 11(5). doi: 10.3390/v11050434
55. di Federico A, Mosca M, Pagani R, Carloni R, Frega G, de Giglio A, et al. Immunotherapy in pancreatic cancer: Why do we keep failing? a focus on tumor immune microenvironment, predictive biomarkers and treatment outcomes. Cancers (Basel) (2022) 14(10). doi: 10.3390/cancers14102429
56. Yamamoto T, Yanagimoto H, Satoi S, Toyokawa H, Yamao J, Kim S, et al. Circulating myeloid dendritic cells as prognostic factors in patients with pancreatic cancer who have undergone surgical resection. J Surg Res (2012) 173(2):299–308. doi: 10.1016/j.jss.2010.09.027
57. Tai LH, Alkayyal AA, Leslie AL, Sahi S, Bennett S, Tanese de Souza C, et al. Phosphodiesterase-5 inhibition reduces postoperative metastatic disease by targeting surgery-induced myeloid derived suppressor cell-dependent inhibition of natural killer cell cytotoxicity. Oncoimmunology (2018) 7(6). doi: 10.1080/2162402X.2018.1431082
58. Bayne LJ, Beatty GL, Jhala N, Clark CE, Rhim AD, Stanger BZ, et al. Tumor-derived granulocyte-macrophage colony-stimulating factor regulates myeloid inflammation and T cell immunity in pancreatic cancer. Cancer Cell (2012) 21:822–35. doi: 10.1016/j.ccr.2012.04.025
59. Wichmann MW, Hüttl TP, Winter H, Spelsberg F, Angele MK, Heiss MM, et al. Immunological effects of laparoscopic vs open colorectal surgery. A prospective Clin study Arch Surg (2005) 140(7):692–7. doi: 10.1001/archsurg.140.7.692
60. Tai LH, de Souza CT, Bélanger S, Ly L, Alkayyal AA, Zhang J, et al. Preventing postoperative metastatic disease by inhibiting surgery-induced dysfunction in natural killer cells. Cancer Res (2013) 73(1):97–107. doi: 10.1158/0008-5472.CAN-12-1993
61. Kim EY, Hong H, Hong H. Changes in total lymphocyte count and neutrophil-to-lymphocyte ratio after curative pancreatectomy in patients with pancreas adenocarcinoma and their prognostic role. J Surg Oncol (2019) 120(7):1102–11. doi: 10.1002/jso.25725
62. Pointer DT, Roife D, Powers BD, Murimwa G, Elessawy S, Thompson ZJ, et al. Neutrophil to lymphocyte ratio, not platelet to lymphocyte or lymphocyte to monocyte ratio, is predictive of patient survival after resection of early-stage pancreatic ductal adenocarcinoma. BMC Cancer (2020) 20(1). doi: 10.1186/s12885-020-07182-9
63. Tohme S, Simmons RL, Tsung A. Surgery for cancer: A trigger for metastases. Cancer Res (2017) 77(7):1548. doi: 10.1158/0008-5472.CAN-16-1536
64. Poto R, Cristinziano L, Modestino L, de Paulis A, Marone G, Loffredo S, et al. Neutrophil extracellular traps, angiogenesis and cancer. Biomedicines (2022) 10(2):431. doi: 10.3390/biomedicines10020431
65. Ilie M, Hofman V, Ortholan C, Bonnetaud C, Coëlle C, Mouroux J, et al. Predictive clinical outcome of the intratumoral CD66b-positive neutrophil-to-CD8-positive T-cell ratio in patients with resectable nonsmall cell lung cancer. Cancer. (2012) 118(6):1726–37. doi: 10.1002/cncr.26456
66. Jensen HK, Donskov F, Marcussen N, Nordsmark M, Lundbeck F, von der Maase H. Presence of intratumoral neutrophils is an independent prognostic factor in localized renal cell carcinoma. J Clin Oncol (2009) 27(28):4709–17. doi: 10.1200/JCO.2008.18.9498
67. Schmidt H, Suciu S, Punt CJA, Gore M, Kruit W, Patel P, et al. Pretreatment levels of peripheral neutrophils and leukocytes as independent predictors of overall survival in patients with American joint committee on cancer stage IV melanoma: results of the EORTC 18951 biochemotherapy trial. J Clin Oncol (2007) 25(12):1562–9. doi: 10.1200/JCO.2006.09.0274
68. Teramukai S, Kitano T, Kishida Y, Kawahara M, Kubota K, Komuta K, et al. Pretreatment neutrophil count as an independent prognostic factor in advanced non-small-cell lung cancer: An analysis of Japan multinational trial organisation LC00-03. Eur J Canc (2009) 45(11):1950–8. doi: 10.1016/j.ejca.2009.01.023
69. Romano F, Uggeri F, Crippa S, di Stefano G, Scotti M, Scaini A, et al. Immunodeficiency in different histotypes of radically operable gastrointestinal cancers. J Exp Clin Cancer Res (2004) 23(2):195–200.
70. d’Engremont C, Vernerey D, Pointet AL, Simone G, Fein F, Heyd B, et al. Additive value of pre-operative and one-month post-operative lymphocyte count for death-risk stratification in patients with resectable pancreatic cancer: A multicentric study. BMC Cancer (2016) 16(1):1–12. doi: 10.1186/s12885-016-2860-6
71. Garcea G, Ladwa N, Neal CP, Metcalfe MS, Dennison AR, Berry DP. Preoperative neutrophil-to-lymphocyte ratio (NLR) is associated with reduced disease-free survival following curative resection of pancreatic adenocarcinoma. World J Surg (2011) 35(4):868–72. doi: 10.1007/s00268-011-0984-z
72. Cools-Lartigue J, Spicer J, McDonald B, Gowing S, Chow S, Giannias B, et al. Neutrophil extracellular traps sequester circulating tumor cells and promote metastasis. J Clin Invest (2013) 123:3446–58. doi: 10.1172/JCI67484
73. Niavarani SR, Lawson C, Boudaud M, Simard C, Tai LH. Oncolytic vesicular stomatitis virus–based cellular vaccine improves triple-negative breast cancer outcome by enhancing natural killer and CD8+ T-cell functionality. J Immunother Cancer (2020) 8(1). doi: 10.1136/jitc-2019-000465
74. Iannone F, Porzia A, Peruzzi G, Birarelli P, Milana B, Sacco L, et al. Effect of surgery on pancreatic tumor-dependent lymphocyte asset: modulation of natural killer cell frequency and cytotoxic function. Pancreas (2015) 44(3):386–93. doi: 10.1097/MPA.0000000000000288
75. Matzner P, Sandbank E, Neeman E, Zmora O, Gottumukkala V, Ben-Eliyahu S. Harnessing cancer immunotherapy during the unexploited immediate perioperative period. Nat Rev Clin Oncol (2020) 17:5. doi: 10.1038/s41571-019-0319-9
76. Luo G, Long J, Zhang B, Liu C, Xu J, Ni Q, et al. Stroma and pancreatic ductal adenocarcinoma: An interaction loop. Biochim Biophys Acta (BBA) - Rev Canc (2012) 1826(1):170–8. doi: 10.1016/j.bbcan.2012.04.002
77. Perez VM, Kearney JF, Yeh JJ. The PDAC extracellular matrix: A review of the ECM protein composition, tumor cell interaction, and therapeutic strategies. Front Oncol (2021) 11:4114. doi: 10.3389/fonc.2021.751311
78. Feig C, Gopinathan A, Neesse A, Chan DS, Cook N, Tuveson DA. The pancreas cancer microenvironment. Clin Cancer Res (2012) 18(16):4266–76. doi: 10.1158/1078-0432.CCR-11-3114
79. Vaish U, Jain T, Are AC, Dudeja V. Cancer-associated fibroblasts in pancreatic ductal adenocarcinoma: An update on heterogeneity and therapeutic targeting. Int J Mol Sci (2021) 22(24). doi: 10.3390/ijms222413408
80. Masugi Y. The desmoplastic stroma of pancreatic cancer: Multilayered levels of heterogeneity, clinical significance, and therapeutic opportunities. Cancers (Basel) (2022) 14(13). doi: 10.3390/cancers14133293
81. Kunk PR, Bauer TW, Slingluff CL, Rahma OE. From bench to bedside a comprehensive review of pancreatic cancer immunotherapy. J Immunother Cancer (2016) 4(1):1–12. doi: 10.1186/s40425-016-0119-z
82. Sperb N, Tsesmelis M, Wirth T. Crosstalk between tumor and stromal cells in pancreatic ductal adenocarcinoma. Int J Mol Sci (2020) 21(15):1–23. doi: 10.3390/ijms21155486
83. Wörmann SM, Diakopoulos KN, Lesina M, Algül H. The immune network in pancreatic cancer development and progression. Oncogene. (2014) 33(23):2956–67. doi: 10.1038/onc.2013.257
84. Rucki AA, Zheng L. Pancreatic cancer stroma: Understanding biology leads to new therapeutic strategies. World J Gastroenterology: WJG (2014) 20(9):2237. doi: 10.3748/wjg.v20.i9.2237
85. Walter FM, Mills K, Mendonça SC, Abel GA, Basu B, Carroll N, et al. Symptoms and patient factors associated with diagnostic intervals for pancreatic cancer (SYMPTOM pancreatic study): A prospective cohort study. Lancet Gastroenterol Hepatol (2016) 1(4):298. doi: 10.1016/S2468-1253(16)30079-6
86. Sideras K, Braat H, Kwekkeboom J, van Eijck CH, Peppelenbosch MP, Sleijfer S, et al. Role of the immune system in pancreatic cancer progression and immune modulating treatment strategies. Cancer Treat Rev (2014) 40(4):513–22. doi: 10.1016/j.ctrv.2013.11.005
87. Martinez-Bosch N, Vinaixa J, Navarro P. Immune evasion in pancreatic cancer: From mechanisms to therapy. Cancers (Basel) (2018) 10(1). doi: 10.3390/cancers10010006
88. Tang R, Liu X, Wang W, Hua J, Xu J, Liang C, et al. Role of tumor mutation burden-related signatures in the prognosis and immune microenvironment of pancreatic ductal adenocarcinoma. Cancer Cell Int (2021) 21(1):196. doi: 10.1186/s12935-021-01900-4
89. Das M, Zhou X, Liu Y, Das A, Vincent BG, Li J, et al. Tumor neoantigen heterogeneity impacts bystander immune inhibition of pancreatic cancer growth. Transl Oncol (2020) 13(12). doi: 10.1016/j.tranon.2020.100856
90. Yamamoto K, Venida A, Yano J, Biancur DE, Kakiuchi M, Gupta S, et al. Autophagy promotes immune evasion of pancreatic cancer by degrading MHC-I. Nature (2020) 581(7806):100–5. doi: 10.1038/s41586-020-2229-5
91. Freed-Pastor WA, Lambert LJ, Ely ZA, Pattada NB, Bhutkar A, Eng G, et al. The CD155/TIGIT axis promotes and maintains immune evasion in neoantigen-expressing pancreatic cancer. Cancer Cell (2021) 39(10):1342–1360.e14. doi: 10.1016/j.ccell.2021.07.007
92. Neuzillet C, Tijeras-Raballand A, Ragulan C, Cros J, Patil Y, Martinet M, et al. Inter- and intra-tumoural heterogeneity in cancer-associated fibroblasts of human pancreatic ductal adenocarcinoma. J Pathology (2019) 248(1):51–65. doi: 10.1002/path.5224
93. Domen A, Quatannens D, Zanivan S, Deben C, van Audenaerde J, Smits E, et al. Cancer-associated fibroblasts as a common orchestrator of therapy resistance in lung and pancreatic cancer. Cancers (Basel) (2021) 13(5):1–22. doi: 10.3390/cancers13050987
94. Narayanan S, Vicent S, Ponz-Sarvisé M. PDAC as an immune evasive disease: Can 3D model systems aid to tackle this clinical problem? Front Cell Dev Biol (2021) 9. doi: 10.3389/fcell.2021.787249
95. Herting CJ, Karpovsky I, Lesinski GB. The tumor microenvironment in pancreatic ductal adenocarcinoma: current perspectives and future directions. Cancer Metastasis Rev (2021) 40(3):675–89. doi: 10.1007/s10555-021-09988-w
96. Looi CK, Chung FFL, Leong CO, Wong SF, Rosli R, Mai CW. Therapeutic challenges and current immunomodulatory strategies in targeting the immunosuppressive pancreatic tumor microenvironment. J Exp Clin Cancer Res (2019) 38:1. doi: 10.1186/s13046-019-1153-8
97. Awasthi N, Mikels-Vigdal AJ, Stefanutti E, Schwarz MA, Monahan S, Smith V, et al. Therapeutic efficacy of anti-MMP9 antibody in combination with nab-paclitaxel-based chemotherapy in pre-clinical models of pancreatic cancer. J Cell Mol Med (2019) 23(6):3878. doi: 10.1111/jcmm.14242
98. Bergers G, Brekken R, McMahon G, Vu TH, Itoh T, Tamaki K, et al. Matrix metalloproteinase-9 triggers the angiogenic switch during carcinogenesis. Nat Cell Biol (2000) 2(10):737–44. doi: 10.1038/35036374
99. Gao H, Lan X, Li S, Xue Y. Relationships of MMP-9, e-cadherin, and VEGF expression with clinicopathological features and response to chemosensitivity in gastric cancer. Tumor Biol (2017) 39(5). doi: 10.1177/1010428317698368
100. Quintero-Fabián S, Arreola R, Becerril-Villanueva E, Torres-Romero JC, Arana-Argáez V, Lara-Riegos J, et al. Role of matrix metalloproteinases in angiogenesis and cancer. Front Oncol (2019) 9:1370. doi: 10.3389/fonc.2019.01370
101. Itoh Y. Proteolytic modulation of tumor microenvironment signals during cancer progression. Front Oncol (2022) 12:12:4590. doi: 10.3389/fonc.2022.935231
102. Ene-Obong A, Clear AJ, Watt J, Wang J, Fatah R, Riches JC, et al. Activated pancreatic stellate cells sequester CD8+ T cells to reduce their infiltration of the juxtatumoral compartment of pancreatic ductal adenocarcinoma. Gastroenterology (2013) 145(5):1121–32. doi: 10.1053/j.gastro.2013.07.025
103. Garg B, Giri B, Modi S, Sethi V, Castro I, Umland O, et al. NFκB in pancreatic stellate cells reduces infiltration of tumors by cytotoxic T cells and killing of cancer cells, via up-regulation of CXCL12. Gastroenterology. (2018) 155(3):880–891.e8. doi: 10.1053/j.gastro.2018.05.051
104. Pandol SJ, Edderkaoui M. What are the macrophages and stellate cells doing in pancreatic adenocarcinoma? Front Physiol (2015) 6:125. doi: 10.3389/fphys.2015.00125
105. Romano F, Cesana G, Berselli M, Piacentini MG, Caprotti R, Bovo G, et al. Biological, histological, and clinical impact of preoperative IL-2 administration in radically operable gastric cancer patients. J Surg Oncol (2004) 88(4):240–7. doi: 10.1002/jso.20155
106. Achard C, Surendran A, Wedge ME, Ungerechts G, Bell J, Ilkow CS. Lighting a fire in the tumor microenvironment using oncolytic immunotherapy. EBioMedicine (2018) 31:17. doi: 10.1016/j.ebiom.2018.04.020
107. Wang L, Chard Dunmall LS, Cheng Z, Wang Y. Remodeling the tumor microenvironment by oncolytic viruses: Beyond oncolysis of tumor cells for cancer treatment. J Immunother Cancer (2022) 10(5). doi: 10.1136/jitc-2021-004167
108. Hajda J, Leuchs B, Angelova AL, Frehtman V, Rommelaere J, Mertens M, et al. Phase 2 trial of oncolytic h-1 parvovirus therapy shows safety and signs of immune system activation in patients with metastatic pancreatic ductal adenocarcinoma. Clin Cancer Res (2021) 27(20):5546–56. doi: 10.1158/1078-0432.CCR-21-1020
109. Noonan AM, Farren MR, Geyer SM, Huang Y, Tahiri S, Ahn D, et al. Randomized phase 2 trial of the oncolytic virus pelareorep (Reolysin) in upfront treatment of metastatic pancreatic adenocarcinoma. Mol Ther (2016) 24(6):1150–8. doi: 10.1038/mt.2016.66
110. Rodríguez-García A, Giménez-Alejandre M, Rojas JJ, Moreno R, Bazan-Peregrino M, Cascalló M, et al. Safety and efficacy of VCN-01, an oncolytic adenovirus combining fiber HSG-binding domain replacement with RGD and hyaluronidase expression. Clin Cancer Res (2015) 21(6):1406–18. doi: 10.1158/1078-0432.CCR-14-2213
111. Watanabe K, Luo Y, Da T, Guedan S, Ruella M, Scholler J, et al. Pancreatic cancer therapy with combined mesothelin-redirected chimeric antigen receptor T cells and cytokine-armed oncolytic adenoviruses. JCI Insight. (2018) 3(7). doi: 10.1172/jci.insight.99573
112. Quixabeira DCA, Zafar S, Santos JM, Cervera-Carrascon V, Havunen R, Kudling T v., et al. Oncolytic adenovirus coding for a variant interleukin 2 (vIL-2) cytokine re-programs the tumor microenvironment and confers enhanced tumor control. Front Immunol (2021) 12. doi: 10.3389/fimmu.2021.674400
113. Hirooka Y, Kasuya H, Ishikawa T, Kawashima H, Ohno E, Villalobos IB, et al. A phase I clinical trial of EUS-guided intratumoral injection of the oncolytic virus, HF10 for unresectable locally advanced pancreatic cancer. BMC Cancer (2018) 18(1). doi: 10.1186/s12885-018-4453-z
114. Holbrook MC, Goad DW, Grdzelishvili VZ. Expanding the spectrum of pancreatic cancers responsive to vesicular stomatitis virus-based oncolytic virotherapy: Challenges and solutions. Cancers (Basel) (2021) 13(5):1–33. doi: 10.3390/cancers13051171
115. Oba A, Ho F, Bao QR, Al-Musawi MH, Schulick RD, del Chiaro M. Neoadjuvant treatment in pancreatic cancer. Front Oncol (2020) 10:245. doi: 10.3389/fonc.2020.00245
116. Kubo H, Ohgi K, Sugiura T, Ashida R, Yamada M, Otsuka S, et al. The association between neoadjuvant therapy and pathological outcomes in pancreatic cancer patients after resection: Prognostic significance of microscopic venous invasion. Ann Surg Oncol (2022) 29(8):4992–5002. doi: 10.1245/s10434-022-11628-8
117. Warner SG, O’Leary MP, Fong Y. Therapeutic oncolytic viruses: Clinical advances and future directions. Curr Opin Oncol (2017) 29(5):359–65. doi: 10.1097/CCO.0000000000000388
118. Thomas RJ, Bartee E. The use of oncolytic virotherapy in the neoadjuvant setting. J Immunother Cancer (2022) 10(4):e004462. doi: 10.1136/jitc-2021-004462
119. Samson A, West EJ, Carmichael J, Scott KJ, Turnbull S, Kuszlewicz B, et al. Neoadjuvant intravenous oncolytic vaccinia virus therapy promotes anticancer immunity in patients. Cancer Immunol Res (2022) 10(6):745–56. doi: 10.1158/2326-6066.CIR-21-0171
120. Caprotti R, Brivio F, Fumagalli L, Nobili C, Degrate L, Lissoni P, et al. Free-from-progression period and overall short preoperative immunotherapy with IL-2 increases the survival of pancreatic cancer patients treated with macroscopically radical surgery. Anticancer Res (2008) 28(3B):1951–4.
121. Neeman E, Zmora O, Ben-Eliyahu S. A new approach to reducing post-surgical cancer recurrence: Perioperative targeting of catecholamines and prostaglandins. Clin Cancer Res (2012) 18(18):4895.
122. Goldfarb Y, Sorski L, Benish M, Levi B, Melamed R, Ben-Eliyahu S. Improving postoperative immune status and resistance to cancer metastasis: A combined perioperative approach of immunostimulation and prevention of excessive surgical stress responses. Ann Surg (2011) 253(4):798–810. doi: 10.1097/SLA.0b013e318211d7b5
123. Badwe R, Hawaldar R, Parmar V, Nadkarni M, Shet T, Desai S, et al. Single-injection depot progesterone before surgery and survival in women with operable breast cancer: a randomized controlled trial. J Clin Oncol (2011) 29(21):2845–51. doi: 10.1200/JCO.2010.33.0738
124. MacCarthy-Morrogh L, Martin P. The hallmarks of cancer are also the hallmarks of wound healing. Sci Signal (2020) 13(648). doi: 10.1126/scisignal.aay8690
125. Bommareddy PK, Shettigar M, Kaufman HL. Integrating oncolytic viruses in combination cancer immunotherapy. Nat Rev Immunol (2018) 18:8. doi: 10.1038/s41577-018-0014-6
126. Khorana AA, Mangu PB, Berlin J, Engebretson A, Hong TS, Maitra A, et al. Potentially curable pancreatic cancer: American society of clinical oncology clinical practice guideline. J Clin Oncol (2016) 34(21):2541–56. doi: 10.1200/JCO.2016.67.5553
127. Harrington K, Freeman DJ, Kelly B, Harper J, Soria JC. Optimizing oncolytic virotherapy in cancer treatment. Nat Rev Drug Discovery (2019) 18:9. doi: 10.1038/s41573-019-0029-0
128. Gouma DJ, van Geenen RCI, van Gulik TM, de Haan RJ, de Wit LT, Busch ORC, et al. Rates of complications and death after pancreaticoduodenectomy: Risk factors and the impact of hospital volume. Ann Surg (2000) 232(6):786. doi: 10.1097/00000658-200012000-00007
129. Macedo FIB, Jayanthi P, Mowzoon M, Yakoub D, Dudeja V, Merchant N. The impact of surgeon volume on outcomes after pancreaticoduodenectomy: A meta-analysis. J Gastrointestinal Surg (2017) 21(10):1723–31. doi: 10.1007/s11605-017-3498-7
130. Sheetz KH, Nuliyalu U, Nathan H, Sonnenday CJ. Association of surgeon case numbers of pancreaticoduodenectomies vs related procedures with patient outcomes to inform volume-based credentialing. JAMA Netw Open (2020) 3(4). doi: 10.1001/jamanetworkopen.2020.3850
Keywords: pancreatic ductal adenocarcinoma (PADC), surgery, perioperative period, neoadjuvant, oncolytic virus, tumor microenvironment, immunosuppression, pancreatic cancer
Citation: Mansouri S, Daniel L, Amhis N, Leveille M, Boudreau JE, Alkayyal AA, Collin Y and Tai L-H (2023) Perioperative oncolytic virotherapy to counteract surgery-induced immunosuppression and improve outcomes in pancreatic ductal adenocarcinoma. Front. Oncol. 13:1071751. doi: 10.3389/fonc.2023.1071751
Received: 17 October 2022; Accepted: 30 January 2023;
Published: 16 February 2023.
Edited by:
Zipeng Lu, Nanjing Medical University, ChinaReviewed by:
Timothy Donahue, University of California, Los Angeles, United StatesAbhilasha Purohit, National Institutes of Health (NIH), United States
Copyright © 2023 Mansouri, Daniel, Amhis, Leveille, Boudreau, Alkayyal, Collin and Tai. This is an open-access article distributed under the terms of the Creative Commons Attribution License (CC BY). The use, distribution or reproduction in other forums is permitted, provided the original author(s) and the copyright owner(s) are credited and that the original publication in this journal is cited, in accordance with accepted academic practice. No use, distribution or reproduction is permitted which does not comply with these terms.
*Correspondence: Lee-Hwa Tai, bGVlLWh3YS50YWlAdXNoZXJicm9va2UuY2E=