- 1King Abdullah International Medical Research Center (KAIMRC), King Saud Bin Abdulaziz University for Health Sciences, Ministry of National Guard - Health Affairs, Jeddah, Saudi Arabia
- 2Oncology Department, Princess Nourah Cancer Center, King Saud bin Abdulaziz University for Health Sciences, Ministry of National Guard - Health Affairs, Jeddah, Saudi Arabia
- 3Division of Molecular Medicine, Leeds Institute of Medical Research (LIMR), St James’s University Hospital, University of Leeds, Leeds, United Kingdom
The MCPH1 gene, also known as BRCT-repeat inhibitor of hTERT expression (BRIT1), has three BRCA1 carboxyl-terminal domains which is an important regulator of DNA repair, cell cycle checkpoints and chromosome condensation. MCPH1/BRIT1 is also known as a tumour suppressor in different types of human cancer. The expression level of the MCPH1/BRIT1 gene is decreased at the DNA, RNA or protein level in a number of types of cancers including breast cancer, lung cancer, cervical cancer, prostate cancer and ovarian cancer compared to normal tissue. This review also showed that deregulation of MCPH1/BRIT1 is significantly associated with reduced overall survival in 57% (12/21) and relapsed free survival in 33% (7/21) of cancer types especially in oesophageal squamous cell carcinoma and renal clear cell carcinoma. A common finding of this study is that the loss of MCPH1/BRIT1 gene expression plays a key role in promoting genome instability and mutations supporting its function as a tumour suppressor gene.
Introduction
Overview
MCPH1 was identified as the first gene associated with primary microcephaly (MCPH). MCPH is an autosomal recessive genetic disorder that produces a smaller-than-normal head circumference at birth (1). MCPH1 has been referred to as BRIT1 (BRCT-repeat inhibitor of hTERT expression) due to its identification in a screen as a negative regulator of telomerase function (2). Mutations in MCPH1/BRIT1 were also found to cause premature chromosome condensation syndrome (PCC) in which cells demonstrate deregulation chromosome condensation (3).
MCPH1/BRIT1 structure
MCPH1/BRIT1 is located on chromosome 8p23; it contains 14 exons encoding an 835 amino acid (aa) protein called Microcephalin (Figure 1) (4). The MCPH1/BRIT1 protein comprises three BRCT domains with repeated 85–95aa long domains separated by variable linker regions no longer than 24aa (1). The first BRCT domain is located in the N-terminal of the MCPH1/BRIT1 protein between 2–83aa and the other two BRCT 2/3 domains lie between 320–399aa and 432-502aa, respectively in the C-terminal of the protein (5, 6). These domains play an important role in MCPH1/BRIT1 functions and protein-protein interactions (7) (Figure 1). Multiple sequence alignment of MCPH1/BRIT1 across H.sapiens, P.troglodytes, C.lupus, B.taurus, M.musculus, R.norvegicus, and G.gallus using ClustalX, revealed that the MCPH1/BRIT1 sequence is conserved (Table 1).
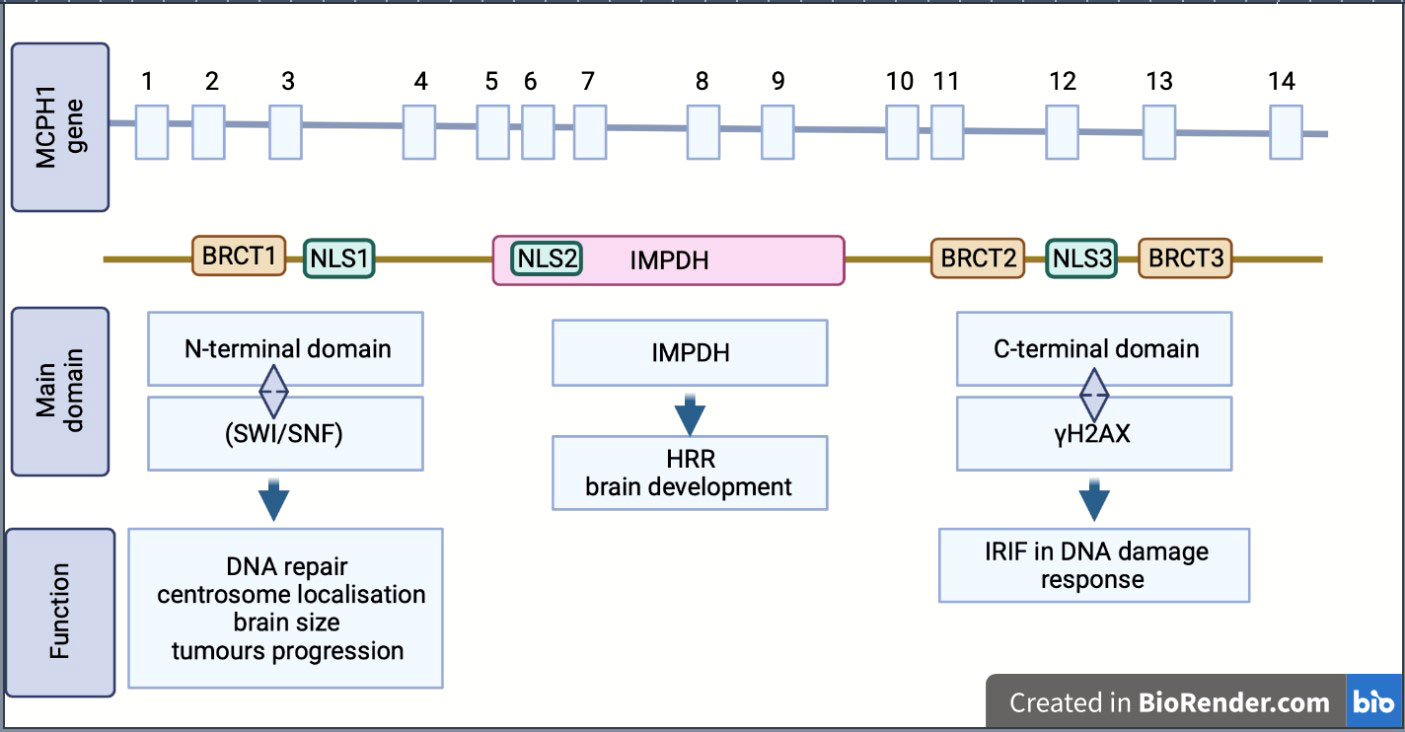
Figure 1 Schematic showing the structure, isoform and biological function of MCPH1/BRIT1. MCPH1/BRIT 1 gene consist of 14 exon and express Microcephalin protein which comprises of three BRCT domains, separated by variable linker regions. BRCT domains play an important role in MCPH1/BRIT1 functions and protein-protein interactions. Inosine 5′-monophosphate dehydrogenase (IMPDH), Homologous recombination repair (HRR), Ionizing radiation induced foci (IRIF), nuclear localization signal or sequence (NLS), SWItch/Sucrose Non-Fermentable (SWI/SNF).
Moreover, the association of these domains with protein function was reported in many studies; the N-terminal BRCT domain interacts with the chromatin remodelling complex (SWI/SNF) in DNA repair and associates with centrosome localisation (5, 7). This region is important in disease discovery due to its association with many mutations that arise in MCPH1/BRIT1; furthermore, many studies have suggested there is a link between N-terminal domains and determining brain size, gonad development and tumour progression (8, 9). The other domains, the tandem C-terminal BRCT domains, are essential for forming oligomer and ionizing radiation-induced foci (IRIF) by interacting with γH2AX in DNA damage response (5, 6). The central region of MCPH1/BRIT1, called the central microcephalin protein domain (IMPDH), is predicted to have a role in the homologous recombination repair (HRR) and brain development (6, 10).
MCPH1/BRIT1 isoforms
The MCPH1/BRIT1 protein exists in multiple isoforms (variants), including the full length-MCPH1/BRIT1 that contains the three BRCT domains, the transcript MCPH1/BRIT1 Δe9–14, which is lacking the last six exons, the transcript MCPH1/BRIT1 Δe1–3, which lacks the first three exons and causes the drop of the N-terminal BRCT domain and finally the transcript MCPH1/BRIT1 Δe8 which is missing exon 8 resulting in the loss of the canonical nuclear localisation signal (NLS) motif (1, 2, 11) (Figure 1).
MCPH1/BRIT1 expression
High expression of this MCPH1/BRIT1 protein was observed in the human foetal brain, testis, liver, pancreas and kidneys, while lower expression was reported in the heart, lungs, thymus and spleen (1). Many studies have reported that the expression level of the MCPH1/BRIT1 gene is decreased in different types of cancers including lung cancer, cervical cancer, breast cancer, prostate cancer and ovarian cancer when compared with normal tissues.
MCPH1/BRIT1 function
MCPH1/BRIT1 performs a wide range of biological functions which supports its role as a tumour suppressor gene, these are outlined below and summarized in (Figure 2).
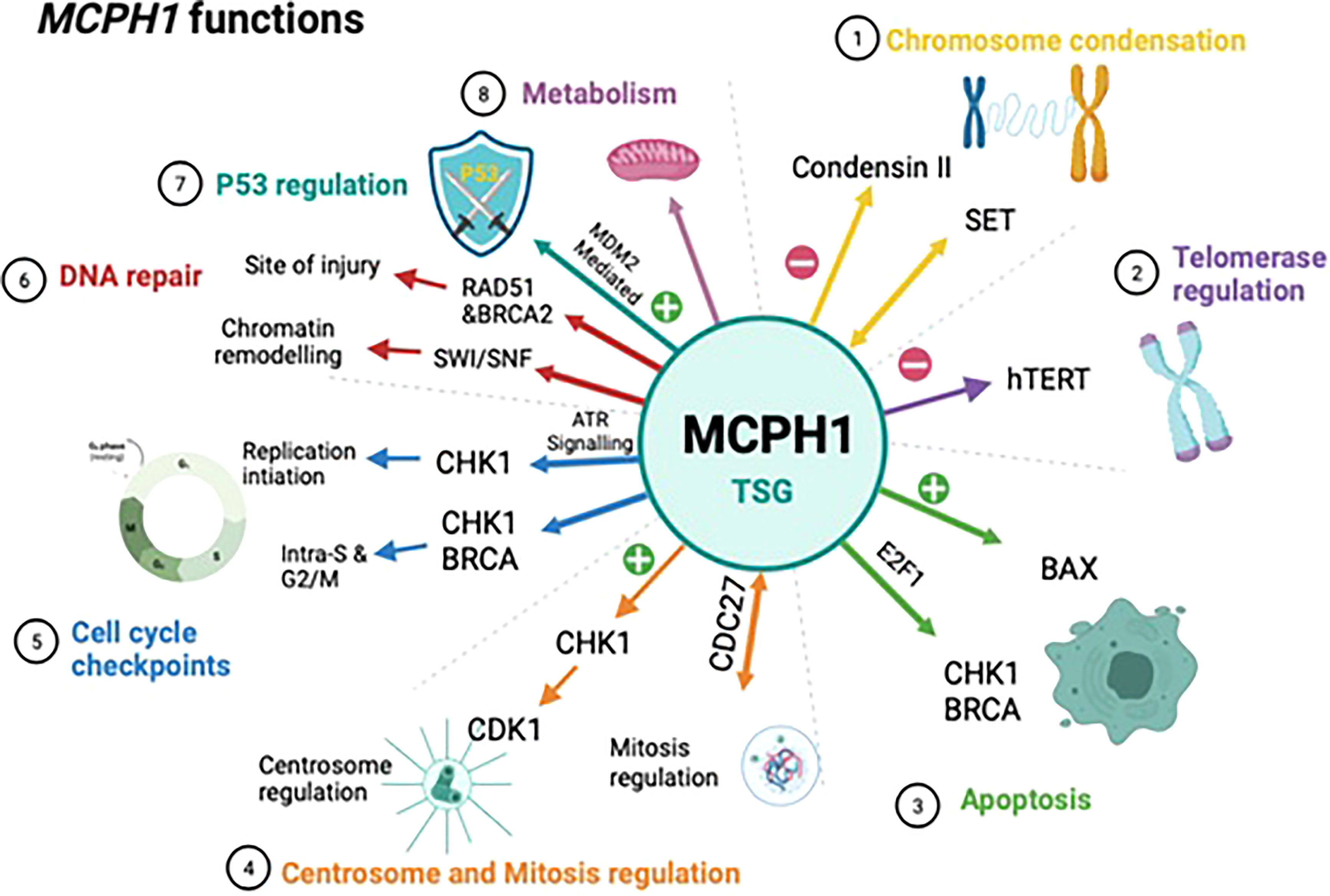
Figure 2 Schematic showing the biological functions of MCPH1/BRIT1. This schematic summarises the biological functions of MCPH1/BRIT1 which supports to its role as a tumour suppressor. 1) MCPH1/BRIT1 affects chromosomal condensation by inhibiting condensin II when it interacts with both non-SMC condensin II complex subunit D3 (NCAPD3) and non-SMC condensin II Complex Subunit G2 (NCAPG2) causing an abnormality in spindle structure and chromosome misalignment. 2) The telomere binding protein (TRF2) interacts with the MCPH1/BRIT1 protein forming the MCPH1/BRIT1–TRF2 complex which increases DNA damage factors localisation, resulting in repair of defective telomeres and increasing telomere replication. 3) MCPH1/BRIT1 promotes cell apoptosis and cell cycle arrest in the S and G2/M phases and overexpression of MCPH1/BRIT1 inhibits uncontrolled cell proliferation. 4) MCPH1/BRIT1 deficient cells demonstrate early mitotic entry which results in spindle organization abnormalities and chromosomal misalignment. 5) MCPH1/BRIT1 regulates the intra-S and G2/M cell cycle checkpoints. MCPH1/BRIT1 is required for adaptive response to avoid topoisomerase II inhibition-mediated G2 arrest and proper chromosomal alignment during prometaphase. 6) Mutations in MCPH1/BRIT1 could lead to defects in DNA repair and cancer development, since MCPH1/BRIT1 is required to recruit and maintain the BRCA2-Rad51 complex at the site of DNA injury and regulate homologous recombination repair of DNA DSBs in a BRCA2-dependent manner. 7) High MCPH1/BRIT1 expression stabilizes p53 function by inhibiting MDM2 and may impact chemotherapy response. 8) MCPH1/BRIT1 is crucial for controlling mitochondrial activity and participating in bioenergetic pathways including oxidative phosphorylation pathways.
Chromosome condensation
Neitzel et al. (2002) characterizes novel autosomal recessive condition with premature chromosomal condensation (PCC) in the early G2 phase in two microcephaly-affected consanguineous siblings with mental retardation. They noted a high frequency of prophase-like cells (>10%) in their lymphocytes, fibroblasts and lymphoblast cells. Neitzel postulated that this PCC is caused by early mitosis as a result of changes in these two siblings’ cell-cycle regulatory genes (12). Years later MCPH1/BRIT1 was discovered to be a chromosomal condensation regulator, inhibiting condensin II by interacting with both NCAPD3 and NCAPG2 subunits (11, 13). Previous research demonstrated that the MCPH1/BRIT1 mutation caused transcriptional alterations that significantly increased aberrant chromosomal condensation. When MCPH1/BRIT1 is not expressed, early entrance into mitosis was reported, which can result in abnormalities in the spindle’s structure and chromosome misalignment linked to premature chromosome condensation (PCC) (14–17).
Telomerase regulation
Telomeres are repeating nucleotide sequences present at the ends of chromosomes that assist to protect the end of the chromosome from DNA damage (18, 19). Somatic cell telomeres shorten with each cell cycle (20). In cancer cells the activation of the telomerase enzyme inhibits DNA shortening in by adding repeated nucleotide sequences to the ends, which helps keep the cells alive (21). It has been reported that MCPH1/BRIT1 influences telomerase activity (2). Cicconi et al. revealed that the telomere binding protein TRF2 interacts with the MCPH1/BRIT1 protein. MCPH1–TRF2 complex increases localisation of DNA damage factors and directs repair of defective telomeres. MCPH1/BRIT1 also participates in the increasing telomere replication fork development and the initiation blocked telomere replication forks (19).
Telomerase enzyme consists of subunits, one of which is an RNA component (hTR) and the other a telomerase reverse transcriptase (hTERT) (22). MCPH1/BRIT1 was initially identified as a result of a genetic screen for transcriptional repressors of the human telomerase catalytic subunit (hTERT). Since hTERT and MCPH1 are negatively connected, MCPH1 is often referred to as BRIT1, which stands for (BRCT-repeats inhibitor of hTERT expression) (2). It might be as a result of direct interaction with the hTERT promotor, which would reduce hTERT expression and consequently lower telomerase activation (23).
Alternative splicing of the hTERT gene has been shown to impact the activity of the telomerase enzyme (hTERT) and contribute to a range of different diseases (24). Thyroid cancer, gastrointestinal carcinoma, ovarian cancer, and myelodysplastic disorders have all been linked to splice variants (25–28). According to a prior study, MCPH1/BRIT1 acts as a negative regulator of the functional variant α+/β+ hTERT in ovarian cancer, while, the α-/β+ hTERT and α-/β- hTERT variants have a positive relationship with MCPH1/BRIT1 supporting its function as a potent inhibitor of telomerase activity (27).
Apoptosis
MCPH1/BRIT1 control apoptosis and mitosis entry by promoting cell apoptosis and arresting the cell cycle in the S and G2/M phases. Further investigation revealed that the CDC25C-cyclinB/CDC2, p53/p21, cyclinA2/CDK2, and CDC2 pathways were engaged in the S phase arrest induced by MCPH1/BRIT1 overexpression (29). Moreover, the overexpression of MCPH1/BRIT1 inhibits uncontrolled cell proliferation in combination with a significant increase in the levels of Bax and active caspase-3 and a decline in the level of Bcl-2 (30). Mai et al. reported that the overexpression of MCPH1/BRIT1 activated mitochondrial apoptosis through regulating several apoptosis-related proteins such as p53, cytochrome c and PARP-1 (29).
Centrosome and mitosis regulation
Centrosomes in human cells are responsible for arranging microtubules, which enable a number of cellular functions such as cell polarization and the formation of the mitotic spindle to ensure proper chromosomal segregation during mitosis. Centrosome amplification or increase in centrosomes number, is frequent in cancer and is associated with more aggressive clinical characteristics. It was postulated that MCPH1/BRIT deficiency is one of the key reasons for centrosome amplification and increase in centriole number (16, 31). In presence of MCPH1/BRIT1, the N-terminal BRCT domain of MCPH1/BRIT1 is required for centrosomal localization in irradiated cells (6, 16). In MCPH1/BRIT1 deficient cells, early mitotic entry is seen, which results in spindle organization abnormalities and/or chromosomal misalignment (16).
Cell cycle checkpoints
Cellular checkpoints regulate mitotic entrance by delaying the initiation of mitosis until the abnormality is completely repaired. MCPH1/BRIT1 activity is not necessary for maintenance of the decatenation checkpoint (32), however it has been recognized as a crucial cell cycle regulator (16). MCPH1/BRIT1 is a regulator of the intra-S and G2/M checkpoints. MCPH1/BRIT1 is necessary for the checkpoint kinase1 (Chk1) localization (17). In MCPH1/BRIT1 deficient cells, active Cdk1 is required for the premature onset of chromosomal condensation (33). MCPH1/BRIT1 is required for the adaptive response that avoids topoisomerase II inhibition-mediated G2 arrest (32). Furthermore, MCPH1/BRIT1 interacts with the anaphase-promoting complex via Cdc27, connecting transcription to cell cycle progression (16). MCPH1/BRIT1 is also necessary for proper chromosomal alignment during prometaphase (33).
DNA repair
MCPH1/BRIT1 plays a role in DNA repair at the site of injury. DNA double strand breaks (DSBs) are harmful to cells and could lead to mutations and cancer progression if not repaired. The BRCA2-Rad51 complex repairs these DSBs and it has been shown that MCPH1/BRIT1 is required to recruit and maintain the BRCA2-Rad51 complex at the site of injury whilst DNA repair occurs (34). Furthermore, MCPH1/BRIT1 can regulate homologous recombination repair of DNA DSBs in a BRCA2-dependent manner. Therefore, reduction or mutation in MCPH1/BRIT1 could progress to defects in DNA repair and subsequently to cancer (34).
Chromatin remodelling is an area of DNA repair that involves MCPH1/BRIT1. DNA repair can only occur if DNA damage repair (DDR) proteins have access to detect and repair the damaged DNA, this cannot happen when the chromatin is condensed. ATP-dependent chromatin remodelling is used by cells to loosen chromatin and give access to DDR proteins (35). MCPH1/BRIT1 is a regulator of the ATP-dependent chromatin remodelling complex SWI/SNF. When DNA is damaged the interaction of MCPH1/BRIT1 with SWI/SNF is increased by the ATM/ATR-dependent phosphorylation of the BAF170 subunit. This increased interaction allows SWI/SNF to be recruited at the site of DNA damage and promotes chromatin relaxation whilst the recruitment of DDR proteins takes place (8). Loss of MCPH1/BRIT1 causes a decrease in chromatin relaxation through the reduced MCPH1-SWI/SNF interaction which could lead to cancer development (8).
P53 regulation
In p53(-/-) cells, Mcph1/Brit1 deficiency resulted in significantly increased chromosomal and chromatid breakage, aberrant centrosome proliferation and aneuploidy (36). MCPH1/BRIT1 has been shown to increase p53 protein stability in breast cancer cell lines by inhibiting MDM2-mediated p53 ubiquitination (37). Hence, MCPH1/BRIT1 deficiency induces genomic instability and enhances cancer risk. The substantial pre-chemotherapy connection between MCPH1/BRIT1 and p53 verifies the concept that high MCPH1/BRIT1 expression stabilizes p53 function and may impact chemotherapy response (38).
Metabolism
MCPH1/BRIT1 colocalize with GRP75 which interacts with the voltage-dependent anion channel 1 (VDAC1) in the mitochondria. VDAC1 protein is required for metabolite exchange between mitochondria and the intracellular environment, as well as calcium flow from the endoplasmic reticulum into mitochondria for tricarboxylic acid cycle activation (39–41). Hence, MCPH1/BRIT1 is crucial for controlling mitochondrial activity and participating in bioenergetic pathways including oxidative phosphorylation pathways (41).
Method
Inclusion and exclusion criteria for studies
We conducted a systematic scoping review to search and identify eligible studies in October 2022 by searching databases included MEDLINE, COCHRANE, EMBASE, Science Direct, and PUBMED. We used the “MCPH1/BRIT1 or Microcephalin” in combination with at least one of the following words: “cancer”, “tumour”, “tumor” and malignancy”. We included articles meeting the following criteria: the abstract was available, evaluating the potential role of MCPH1/BRIT1 in different types of cancers and articles published between years 2000 to 2022. We excluded non-English articles, and articles published before 2000.
Data abstraction and reporting of results
The search for selected published articles was made by three authors (MA, SMB and RA). The summary of included studies is shown in Table 2. We performed an independent review of article titles and abstracts extracting data according to the predefined eligibility criteria. To assess the Quality of the selected studies we used the Newcastle–Ottawa scale (NOS) which scores a study with a total of 9 points according to the assessment criteria such as criteria for selection, comparability and outcome (case–control studies) or exposure (cohort studies) (70). A good quality study has 3 or 4 points in selection criteria, 1 or 2 points in comparability criteria, and 2 or 3 points in outcome/exposure criteria.
Results
Perspective on the involvement of MCPH1/BRIT1 in cancer progression
In this review we are interested in MCPH1/BRIT1 expression levels and its association with tumorigenesis in various forms of cancer, whether at the DNA, RNA or protein level. Interrogation of The Cancer Genome Atlas (TCGA; cBioPortal Cancer Genomics database http://cancergenome.nih.gov/) has identified alterations in MCPH1/BRIT1 in many malignant tumours such as breast cancer (~5%), prostate cancer (5.7%), bladder urothelial carcinoma (8%), lung cancer (7%), ovarian cancer (7%), liver cancer (7%) and colorectal adenocarcinoma (7.4%) (Figure 3). Deep deletions and mutations were the most common inactivation methods in most cancer types with amplifications in a minority of cancers, while structural variants were quite rare. This was confirmed in a study conducted on various types of tumours, which identified MCPH1/BRIT1 gene deletions in 5–15% of cancers (31).
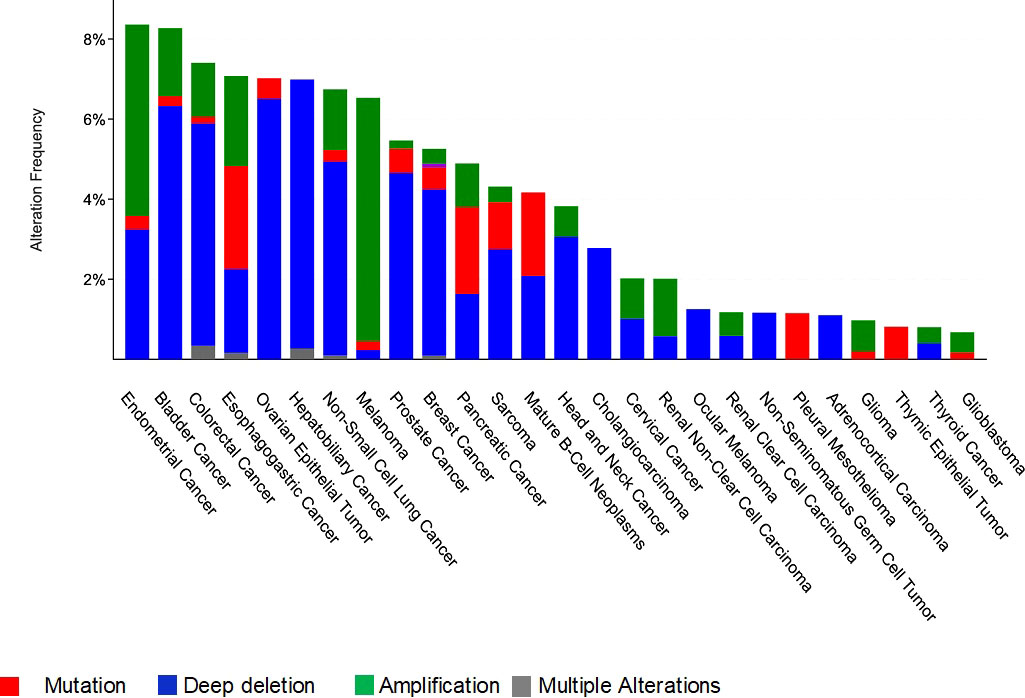
Figure 3 Frequency and type of genetic alterations at the MCPH1/BRIT1 locus in different cancer types.
To determine the clinical relevance of MCPH1/BRIT1 expression, pan-cancer analysis of RNA-SEQ data was performed using KM Plotter (71). MCPH1/BRIT1 expression was associated with overall survival (OS) in 57% (12/21) and relapse-free survival (RFS) in 33% (7/21) of cancer types (Figure 4). Low MCPH1/BRIT1 expression in cervical squamous cell carcinoma, oesophageal squamous cell carcinoma, head-neck squamous cell carcinoma, renal clear cell carcinoma, rectal adenocarcinoma, stomach adenocarcinoma, thymoma and uterine corpus endometroid cancer predicted reduced OS. Although high MCPH1/BRIT1 expression in a few cancers, oesophageal adenocarcinoma, renal papillary cell carcinoma, HCC, and thyroid carcinoma predicted reduced OS. Similarly, low MCPH1/BRIT1 expression in oesophageal squamous cell carcinoma, renal clear cell carcinoma and testicular germ cell tumours predicted reduced RFS while high MCPH1/BRIT1 expressions in oesophageal adenocarcinoma, renal papillary cell carcinoma, pancreatic ductal adenocarcinoma and stomach adenocarcinoma predicted reduced RFS (71).
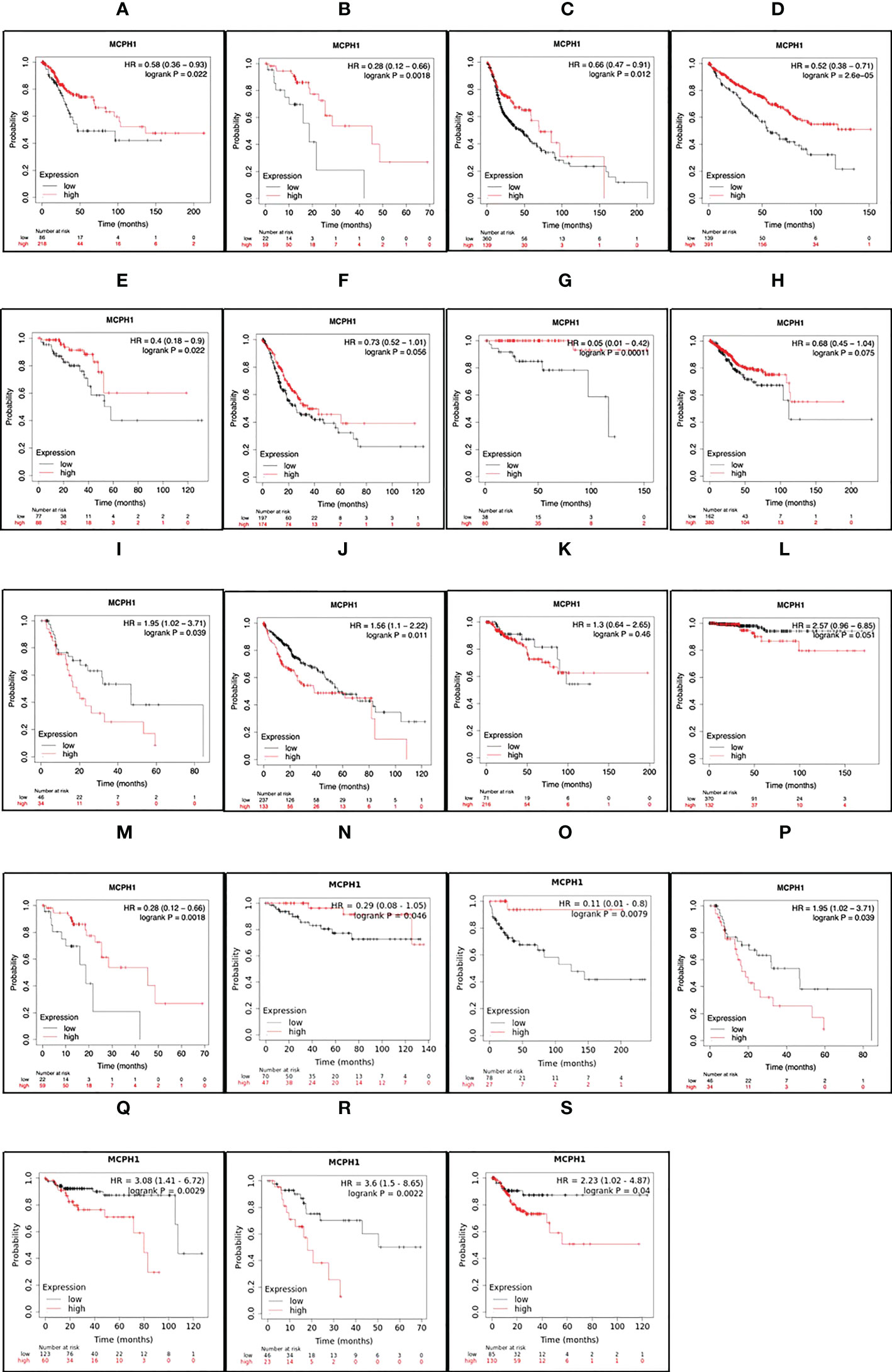
Figure 4 MCPH1/BRIT1 expression correlated with overall survival (OS) and relapse free survival (RFS). Low MCPH1/BRIT1 expression in cervical squamous cell carcinoma (A), oesophageal squamous cell carcinoma (B), head-neck squamous cell carcinoma (C), renal clear cell carcinoma (D), rectal adenocarcinoma (E), stomach adenocarcinoma (F), thymoma (G) and uterine corpus endometroid cancer (H) predicted reduced OS. High MCPH1/BRIT1 expression in oesophageal adenocarcinoma (I), renal papillary cell carcinoma (J), HCC (K), and thyroid carcinoma (L) predicted reduced OS. Low MCPH1/BRIT1 expression in oesophageal squamous cell carcinoma (M), renal clear cell carcinoma (N) and testicular germ cell tumours (O) predicted reduced RFS while high MCPH1/BRIT1 expressions in oesophageal adenocarcinoma (P), renal papillary cell carcinoma (Q), pancreatic ductal adenocarcinoma (R) and stomach adenocarcinoma (S) predicted reduced RFS.
Discussion
MCPH1/BRIT1 in breast cancer
Previously we have identified reduced MCPH1/BRIT1 expression in 29% (93/319) of breast cancer cases associated with high tumour grade and triple negative phenotype. Importantly MCPH1/BRIT1 was an independent predictor of overall survival (42). Furthermore, previous studies found MCPH1/BRIT1 to be associated in all subtypes (48). Another study identified low MCPH1/BRIT1 nuclear expression in 52.4% (43/82) of breast cancers which was associated with the presence of allele T in the MCPH1/BRIT1 polymorphisms (rs2912010 and rs1057090). This study also reported that increased MCPH1/BRIT1 cytoplasmic levels were associated with tumour grade (P =0.010) (43). Decreased MCPH1/BRIT1 protein and RNA expression levels, and/or deletions and inactivation due to methylation were reported in 96% (121/126) of breast cancer cases. Additionally, patients with MCPH1/BRIT1 inactivation by methylation showed a correlation with negative ER status. Moreover, the change in both MCPH1/BRIT1 and ATM together was significantly associated with an increased in tumour grade (44).
MCPH1/BRIT1 has also been identified as a novel hereditary breast cancer gene. In a study conducted to assess the expression of DNA damage proteins, 40% (50/125) of sporadic cases and 65.3% (47/72) of familial breast cancer cases demonstrated reduced MCPH1/BRIT1 expression which was also associated with higher tumour grade (45). The function of MCPH1/BRIT1 as a breast cancer susceptibility gene was supported by another study in which a recurrent heterozygous MCPH1/BRIT1 mutation c.904_916del was identified in 3.4% (5/145) familial and 1.4% (16/1150) sporadic breast cancer cases (46). Blood samples from MCPH1/BRIT1 mutation carriers also demonstrated high levels of chromosomal rearrangements suggesting MCPH1/BRIT1 haploinsufficiency causes increased genomic instability and cancer susceptibility (46). CRISPR gene editing was subsequently used to generate the cancer predisposing allele p.Arg304Valfs-Ter3 in the normal breast cell line MCF10A which removed the two C-terminal BRCT domains. This study showed that the MCPH1/BRIT1 mutation led to transcriptional changes causing a significant increase in migration and invasion as well as abnormal chromosomal condensation (47).
MCPH1/BRIT1 has been shown to control the protein stability of p53 in breast cancer cell lines by blocking murine double minute 2-mediated (MDM2) p53 ubiquitination (56). In MCPH1/BRIT1 depleted cells p53 expression levels were reduced which was found to promote cell proliferation and survival in normal (MCF10A) breast cells. Depletion of MCPH1/BRIT1 in normal MCF10A cells caused transformation into oncogenic cells. Furthermore, the overexpression of MCPH1/BRIT1 in the MCF7 breast cancer cell line suppressed proliferation both in vivo and in vitro (56). Interestingly, breast cancer patients who are carriers of the MCPH1/BRIT1 p.Arg304ValfsTer3 mutation also demonstrated p53 mutations in breast tumours (47).
MCPH1/BRIT1 in colorectal cancer
Lack of heterozygosity (LOH) on chromosome 8p23.1, the MCPH1/BRIT1 locus has frequently been identified in advanced stage colorectal carcinoma patients, indicating the presence of a tumour suppressor gene. Approximately one-third of colorectal cancer cause microsatellite instability (MSI) because of the failure in mismatch repair (49). Frequently, tumour suppressor genes are targets for mutations at mononucleotide repeats within the genes in high MSI cancers (49). The MCPH1/BRIT1 gene contains a single nucleotide repeat (A9), which is a mutation target in cancers with MSI that inhibits the TSG activities contributing to tumorigenesis (49). Methylation of the MLH1 promoter is not an explanation for many colorectal cancers with MSI (50). Lynch syndrome is caused by germline mutations in other mismatch repair (MMR) genes (50). These Lynch-like syndrome patients, on the other hand, have somatic mutations in MMR genes (50). Xicola et al. discovered rare, likely pathogenic germline variants in repair genes that maintain genome integrity in Lynch-like syndrome, including a stop mutation in MCPH1/BRIT1 (50). Furthermore, interrogation of the Cancer Genome Atlas (TCGA; cBioPortal Cancer Genomics database http://cancergenome.nih.gov/) identified MCPH1/BRIT1 mutations in 7.4% of colorectal adenocarcinoma (Figure 3).
In cancer, centrosome amplification is common and associated with severe clinical features and poor patient outcomes (31). Interrogation of TCGA genomic and transcriptomic data identified 367 genes that encode proteins with centrosome localisation (31). Cellulo analysis of these candidates (633 colorectal cancer patients), demonstrated that the deletion of MCPH1/BRIT1 resulted in the most significant increase in centriole number (31). MCPH1/BRIT1 deletion, mutation and amplification have all been linked to colorectal cancer. MCPH1/BRIT1 deletion was discovered to be strongly linked to p53 abnormality (31). Patients with MCPH1/BRIT1 deletions have a poor outcome. The Kaplan–Meier curve shows that disease-free survival (DFS) is shorter in colorectal cancer patients with MCPH1/BRIT1 deletion than in patients with wild-type MCPH1/BRIT1 (P =0.025) (31). In addition, Zhang Du et al. (2020) discovered a link between Angpt2 (rs12674822) single nucleotide polymorphisms (SNPs) and colorectal cancer patients’ progression-free survival (PFS) (52). This SNP (rs12674822) is located in the Angpt2 and MCPH1/BRIT1 introns (52). In another study, they performed whole-exome sequencing on a cohort of 30 adults, 30 adolescent and young adult and 2 pediatric colon cancers (51). A statistically significant difference in mutational frequency between adolescent, young adult and adult samples was observed in 43 genes, including MCPH1/BRIT1 (51). Many of these mutations are damaging because they are nonsynonymous, missense, stop-gain, or frameshift mutations (51).
MCPH1/BRIT1 in ovarian cancer
Previously we have reported reduced MCPH1/BRIT1 staining in 19% (7/36) of primary ovarian cancer cells cultured from ascites samples (53). This reduced expression was associated with increased tumour grade and poor prognosis. Building on this study we next examined MCPH1/BRIT1 expression in normal ovarian, endometrium and fallopian tube tissue. Strong nuclear MCPH1/BRIT1 staining was identified in ovarian epithelial cells by (90%) compared to endometrium and fallopian tube samples which are (70–80%) (27). Similarly, we identified reduced MCPH1/BRIT1 expression in 33% (84/252) of epithelial ovarian cancer tumour samples. Again, low MCPH1/BRIT1 expression was statistically associated with high grade tumours and advanced stage tumours (27). MCPH1/BRIT1 expression levels were not associated with different epithelial ovarian cancer subtypes. Consequently, the expression of MCPH1/BRIT1 could be a useful biomarker in epithelial ovarian cancer (27). This work complements studies which have identified decreased MCPH1/BRIT1 DNA copy number and mRNA levels in 40% (35/87) and 63% (19/30) of ovarian cancer respectively (54). In a further ovarian cancer study, the expression levels of the hTERT regulators were investigated as potential biomarkers to treat epithelial ovarian cancer. MCPH1/BRIT1 and the functional form of hTERT were associated negatively which in turn identified MCPH1/BRIT1 as a negative telomerase regulator in primary epithelial ovarian cancer samples (55).
MCPH1/BRIT1 in lung cancer
Three lung cancer studies related to MCPH1/BRIT1 were identified, all of which were conducted in Chongqing, China (30, 56, 57). These studies have shown that the defect in the expression of MCPH1/BRIT1 may participate in the development of lung cancer. The initial study used immunohistochemistry to evaluate the expression of MCPH1/BRIT1 protein in two groups, 188 patients with lung cancer and 20 patients with normal lung tissues. In all normal lung samples positive MCPH1/BRIT1 staining was found. In the lung cancer samples significant lower numbers of MCPH1/BRIT1-positive cells were identified compared with normal tissues. The expression of MCPH1/BRIT1 varied among different histological subtypes, patients with lung adenocarcinoma expressed higher MCPH1/BRIT1 than patients with squamous cell lung carcinoma (56). A small follow up study confirmed significantly reduced MCPH1/BRIT1 mRNA expression in lung cancer samples compared to adjacent normal tissue. Functional studies were also performed in A549 lung cancer cells, which demonstrated over-expression of MCPH1/BRIT1 caused reduced proliferation due to cell-cycle arrest at S and G2/M and increased apoptosis (30).
A further study revealed that MCPH1/BRIT1 over-expression inhibits the migration and invasion capacities of A549 lung cancer cells by suppressing Snail and Slug proteins by blocking Mdm2-mediated p53 ubiquitination (57). Additionally, MCPH1/BRIT1 is an inclusion eligibility criterion in 2 clinical trials for squamous cell lung carcinoma, 3 clinical trials for non-small cell lung carcinoma, and 5 clinical trials for small cell lung carcinoma (72). All of these studies point to the possibility that MCPH1/BRIT1 functions as a tumour suppressor in the lungs.
MCPH1/BRIT1 role in other different cancer types
We searched PubMed and found 16 studies identified the potential role of MCPH1/BRIT1 in different cancer types such as, lymphoma, chronic myeloid leukemia, cervical cancer, endometrial cancer, hepatocellular carcinoma, renal carcinoma, brain cancer, oral squamous cell carcinoma, melanoma and prostate cancer.
MCPH1/BRIT1 in lymphoma and chronic myeloid leukemia
A study by Liang et al. used knockout mouse models, Mcph1 deficient and Mcph1/p53 deficient mice (61). They showed that Mcph1- and Mcph1-/p53- mice developed lymphomas earlier than in Mcph1+/p53¯ mice. By using metaphase spread assay and spectral karyotyping analysis they concluded that Mcph1 depletion regulates genomic instability and induces carcinogenesis. Giallongo et al. investigated genomic instability and the ability of chronic myeloid leukemia cells to arrest mitotic division following exposure to the genotoxic drug hydroxyurea (62). In agreement with the key role of MCPH1/BRIT1 in the regulation of cell cycle progression at the G2/M checkpoint, they found that chronic myeloid leukemia cells have a low level of MCPH1/BRIT1 and a defective G2/M arrest, confirming the genomic instability of these cells. In addition, A study showed that downregulation of MCPH1/BRIT1 accompanied by loss of its promoter methylation resulted in upregulation of ANGP2 which is a crucial factor in tumour angiopoiesis in chronic lymphocytic leukemia (63).
MCPH1/BRIT1 in cervical endometrial cancer
An investigation of MCPH1/BRIT1 in cervical cancer demonstrated decreased expression of MCPH1/BRIT1 in 61.3% (19/31) of cases at the mRNA level and 69.8% (44/63) at the protein level in cervical tumour tissues compared to non-tumour tissues. The downregulation of MCPH1/BRIT1 correlated with increased tumour grade (29). They also demonstrated that overexpression of MCPH1/BRIT1 induced S phase arrest and mitochondrial apoptosis (29). Moreover, a study by Bilbao et al. investigated whether endometrial cancer is caused by DSB repair with MSI (64). Mononucleotide microsatellite tracts of 14 genes of the DSB repair system including MCPH1/BRIT1 were analyzed in a series of 41 endometrial cancers with MSI. Mutations in MCPH1/BRIT1 were detected in 12% (5/41) of endometrial cancer indicating that MCPH1/BRIT1 could be a target gene of MSI (64).
MCPH1/BRIT1 in gastric carcinoma
Similarly, investigation of MCPH1/BRIT1 frameshift mutations in gastric cancer found that 11% (4/34) of cases with high MSI contained MCPH1/BRIT1 frameshift mutations compared to (0/45) with low MSI (49). Another study characterising long non-coding RNAs (lncRNA) in gastric cancer identified over-expression of 6 key lncRNA including MCPH1/BRIT1 antisense RNA 1 (CTD-2541M15) which were associated with invasion and metastasis (58).
MCPH1/BRIT1 in hepatocellular carcinoma
Scientists identified that MCPH1/BRIT1 deficiency is a potential factor in hepatocellular carcinoma (HCC) development. HCC samples were analyzed for MCPH1/BRIT1 alterations at DNA, RNA, and protein levels (65). Deletion and/or downregulation of MCPH1/BRIT1 was found in ~30% of HCC samples. Notably, the lack in survival ratio and increase in the recurrence of HCC were caused by MCPH1/BRCA1 deficiency (65). The K659fsX10 mutation in MCPH1/BRIT1 represented in HCC blocked DNA repair function. Moreover, MCPH1/BRIT1 depleted-HCC cells were HR defective and more sensitive to PARPi olaparib alone or plus PI3K inhibitor BEZ235. Ectopic MCPH1/BRIT1 significantly decreased the cytotoxicity of olaparib alone or with BEZ235. In addition, in MCPH1/BRIT1 depleted cells, BEZ235 induced poly (ADP-ribose) release, DSB ratio and single-strand breaks (65). MCPH1/BRIT1 deficiency is therefore a regulator for HCC growth and using olaparib and/or BEZ235 as a treatment is highly sensitive in MCPH1/BRIT1 deficient HCC. PI3K blocking is considered a major cause for DNA damage and MCPH1/BRIT1 depleted-cells become more dependent on PARP activity, thus, the synthetic lethal ability of PARP and PI3K inhibitors is enhanced by the deficiency of MCPH1/BRIT1 in HCC (65).
MCPH1/BRIT1 in renal cancer
A study evaluated the expression of MCPH1/BRIT1 in 188 renal cancer and 20 normal renal tissues by immunohistochemistry (66). They found that all normal renal samples displayed positive MCPH1/BRIT1 staining while renal carcinoma tissues showed lower levels of MCPH1/BRIT1 staining compared to normal tissues. Functional analysis in renal cancer cell lines demonstrated that over-expression of MCPH1/BRIT1 reduced proliferation, migration and invasion while increasing apoptosis supporting MCPH1/BRIT1’s role as a tumour suppressor gene in renal cancer. The down regulation of MCPH1/BRIT1 expression was also shown to be correlated with miRNA-27a and linked to miRNA-27 seed regions identified in MCPH1/BRIT1 3’UTR (66).
MCPH1/BRIT1 in brain cancer
MCPH1/BRIT1 promoter methylation was identified in 96.6% (28/30) of brain tumour samples (67). In a follow up study MCPH1/BRIT1 promoter methylation was determined in 14 paired circulating cell-free DNA (cfDNA) from serum samples and genomic DNA from tumour tissue also 18 isolated serum samples with different grades of brain tumours. MCPH1/BRIT1 promoter methylation was identified in 78% of tissue samples and 54% cfDNA samples with agreement between samples in 57%. MCPH1/BRIT1 promoter methylation was also significantly associated with high tumour grade suggesting it could be an epimarker in the detection of brain tumours (67). In contrast, it was found that the expression of MCPH1/BRIT1 did not alter during glioma development (59).
MCPH1/BRIT1 in oral squamous cell carcinoma
In other cancer types such as oral squamous cell carcinoma (OSCC), LOH was performed in a panel of 81 matched normal oral tissues and OSCC samples and observed that 19.72% (14/71) informative samples showed LOH at 8p23 (60). MCPH1/BRIT1 was downregulated at the transcript and protein levels in 51.22% (21/41) and 76% (19/25) of OSCC samples respectively. MCPH1/BRIT1 over-expression in oral squamous KB cells reduced proliferation, cell invasion and anchorage-independent growth and tumour growth in nude mice (60). This study also demonstrated that miR-27a targets MCPH1/BRIT1 and negatively regulates its levels (60). In a recent study, the 5-year survival of patients with early-stage (I/II) OSCC was predicted by combining clinicopathologic features with a gene methylation signature. The most significant methylation difference between patients who lived for over five years and those who died was found in MCPH1/BRIT1. Therefore, indicating MCPH1/BRIT1 gene methylation predicts poor prognosis in early-stage (I/II) OSCC (68).
MCPH1/BRIT1 in melanoma
The involvement of MCPH1/BRIT1 dysfunction was suggested in the development and progression of cutaneous melanoma. Immunohistochemistry was performed identifying abnormal MCPH1/BRIT1 expression in 10% (1/10) of melanocytic nevi and in 86.3% (44/51) of primary cutaneous melanomas (69).
MCPH1/BRIT1 in prostate cancer
A number of pan-cancer studies have reported an association between MCPH1/BRIT1 and prostate cancer. The MCPH1/BRIT1 human chromosomal locus 8p23.1 was previously described as deleted in prostate cancer and associated with a poor prognosis. A small prostate study showed a reduction in MCPH1/BRIT1 protein levels in cancer samples compared to normal tissues (54). Bioinformatics analysis performed on TCGA datasets to identify centrosome genes associated with centrosome amplification and cancer development found that prostate adenocarcinoma cases with MCPH1/BRIT1 gene deletion demonstrated a worse OS and DFS (31). Importantly MCPH1/BRIT1 gene dysregulation has been identified in 12-16% of prostate cancer cases suggesting MCPH1/BRIT1 warrants further study in this cancer type (31).
Despite significant advances in cancer treatment, novel therapeutic strategies are required
Personalized medicine became a reality after the human genome project was finished in 2001. Understanding the genetic code would pave the way for the development of anti-cancer medications that directly target dysregulated pathways. As an illustration, personalized medicine has dramatically reduced mortality for several cancer types. Nowadays, clinical practice utilizes commercially available genetic testing to direct treatment decisions (73, 74). In general, developments in cancer treatment have resulted in better patient outcomes; given the clinical relevance of MCPH1/BRIT1 expression we anticipate that MCPH1/BRIT1 defective patients would benefit from targeted therapies.
Immunotherapy has become an important therapeutic option for many malignancies particularly advanced stage cancers. A number of features have been identified which predict if the use of immune checkpoint inhibitors (ICIs) will be successful. For example, tumours with homologous recombination defects, with high expression of PD-1 and PD-L1, represent better susceptibility to ICIs (75). Also using PARPi synergy with the ICIs in tumours with homologous recombination damage reported increases in the mutational load in tumour cells and increased expression of PD-L1 by ATM-ATR-Checkpoint kinase 1 pathway (76, 77). In a small renal cell carcinoma study, loss of function mutation in one component of the SWI/SNF (PBRM1) complex, demonstrated a better response to anti-PD-L1 therapy (78). Also, high-throughput CRISPR screening identified an association between SWI/SNF complex genes and better response to ICIs (79). These previous studies suggest a rationale to use ICIs with MCPH1/BRIT1-deficient cancers.
Another promising approach to investigate would be MCPH1/BRIT1 synthetic lethality. MCPH1/BRIT1 deficiency facilitates the synthetic lethality of PARP and PI3K inhibitors in HCC (65). This provides a new biological foundation for dramatically expanding PARPi’s therapeutic potential in various types of cancer with depletion in MCPH1/BRIT1. It is critical to discover new compounds and genes that cause synthetic lethality in MCPH1-deficient cells. The identification of these targets and molecules, as well as research of the cellular pathways in which they are engaged, may aid in cancer treatment discovery.
Conclusions
In conclusion, the common findings of the role of MCPH1/BRIT1 in tumorigenesis in various types of cancer, is that the loss of MCPH1/BRIT1 gene plays a key role in promoting genome instability and mutations, which is one of cancer’s hallmarks. Consequently, future studies are warrants to examine MCPH1/BRIT1 as a tumour suppressor.
Author contributions
MA designed the review, directed and took the lead in writing the manuscript. All authors contributed to the article and approved the submitted version. RA devised the review and proof outline. SB provided critical feedback and helped shape the manuscript.
Acknowledgments
We gratefully acknowledge the assistance of King Abdullah International Medical Center (KAIMRC), King Saud bin Abdulaziz University for Health Sciences (KSAU-HS), and the University of Leeds. We would also like to thank the Saudi Ministry of Education for their financial support.
Conflict of interest
The authors declare that the research was conducted in the absence of any commercial or financial relationships that could be construed as a potential conflict of interest.
Publisher’s note
All claims expressed in this article are solely those of the authors and do not necessarily represent those of their affiliated organizations, or those of the publisher, the editors and the reviewers. Any product that may be evaluated in this article, or claim that may be made by its manufacturer, is not guaranteed or endorsed by the publisher.
References
1. Jackson AP, Eastwood H, Bell SM, Adu J, Toomes C, Carr IM, et al. Identification of microcephalin, a protein implicated in determining the size of the human brain. Am J Hum Genet (2002) 71(1):136–42. doi: 10.1086/341283
2. Lin S-Y, Elledge SJ. Multiple tumor suppressor pathways negatively regulate telomerase. Cell (2003) 113(7):881–9. doi: 10.1016/S0092-8674(03)00430-6
3. Trimborn M, Bell SM, Felix C, Rashid Y, Jafri H, Griffiths PD, et al. Mutations in microcephalin cause aberrant regulation of chromosome condensation. Am J Hum Genet (2004) 75(2):261–6. doi: 10.1086/422855
4. Glover JNM, Williams RS, Lee MS. Interactions between BRCT repeats and phosphoproteins: tangled up in two. Trends Biochem Sci (2004) 29(11):579–85. doi: 10.1016/j.tibs.2004.09.010
5. Gavvovidis I, Rost I, Trimborn M, Kaiser FJ, Purps J, Wiek C, et al. A novel MCPH1 isoform complements the defective chromosome condensation of human MCPH1-deficient cells. PloS One (2012) 7(8):e40387. doi: 10.1371/journal.pone.0040387
6. Jeffers LJ, Coull BJ, Stack SJ, Morrison CG. Distinct BRCT domains in Mcph1/Brit1 mediate ionizing radiation-induced focus formation and centrosomal localization. Oncogene (2008) 27(1):139–44. doi: 10.1038/sj.onc.1210595
7. Kristofova M, Ori A, Wang Z-Q. Multifaceted microcephaly-related gene MCPH1. Cells (2022) 11(2):275. doi: 10.3390/cells11020275
8. Peng G, Yim E-K, Dai H, Jackson AP, van der BI, Pan M-R, et al. BRIT1/MCPH1 links chromatin remodelling to DNA damage response. Nat Cell Biol (2009) 11(7):865–72. doi: 10.1038/ncb1895
9. Kristofova M, Wang Z-Q. MCPH1, beyond its role deciding the brain size. Aging (2021) 13:23437–9. doi: 10.18632/aging.203658
10. Liu X, Schneble-Löhnert N, Kristofova M, Qing X, Labisch J, Hofmann S, et al. The n-terminal BRCT domain determines MCPH1 function in brain development and fertility. Cell Death Dis (2021) 12(2):143. doi: 10.1038/s41419-021-03406-3
11. Yamashita D, Shintomi K, Ono T, Gavvovidis I, Schindler D, Neitzel H, et al. MCPH1 regulates chromosome condensation and shaping as a composite modulator of condensin II. J Cell Biol (2011) 194(6):841–54. doi: 10.1083/jcb.201106141
12. Neitzel H, Neumann LM, Schindler D, Wirges A, Tönnies H, Trimborn M, et al. Premature chromosome condensation in humans associated with microcephaly and mental retardation: A novel autosomal recessive condition. Am J Hum Genet [Internet] (2002) 70(4):1015–22. doi: 10.1086/339518
13. Wood JL, Liang Y, Li K, Chen J. Microcephalin/MCPH1 associates with the condensin II complex to function in homologous recombination repair∗. J Biol Chem (2008) 283(43):29586–92. doi: 10.1074/jbc.M804080200
14. Pulvers JN, Journiac N, Arai Y, Nardelli J. MCPH1: A window into brain development and evolution. Front Cell Neurosci (2015) 9(March):1–14. doi: 10.3389/fncel.2015.00092
15. Gruber R, Zhou Z, Sukchev M, Joerss T, Frappart P-O, Wang Z-Q. MCPH1 regulates the neuroprogenitor division mode by coupling the centrosomal cycle with mitotic entry through the Chk1-Cdc25 pathway. Nat Cell Biol (2011) 13(11):1325–34. doi: 10.1038/ncb2342
16. Liu X, Zhou Z-W, Wang Z-Q. The DNA damage response molecule MCPH1 in brain development and beyond. Acta Biochim Biophys Sin (Shanghai) (2016) 48(7):678–85. doi: 10.1093/abbs/gmw048
17. Lin S-Y, Rai R, Li K, Xu Z-X, Elledge SJ. BRIT1/MCPH1 is a DNA damage responsive protein that regulates the Brca1-Chk1 pathway, implicating checkpoint dysfunction in microcephaly. Proc Natl Acad Sci U S A (2005) 102(42):15105–9. doi: 10.1073/pnas.0507722102
18. Counter CM, Avilion AA, LeFeuvre CE, Stewart NG, Greider CW, Harley CB, et al. Telomere shortening associated with chromosome instability is arrested in immortal cells which express telomerase activity. EMBO J (1992) 11(5):1921–9. doi: 10.1002/j.1460-2075.1992.tb05245.x
19. Cicconi A, Rai R, Xiong X, Broton C, Al-Hiyasat A, Hu C, et al. Microcephalin 1/BRIT1-TRF2 interaction promotes telomere replication and repair, linking telomere dysfunction to primary microcephaly. Nat Commun (2020) 11(1):1–18. doi: 10.1038/s41467-020-19674-0
20. Hemann MT, Strong MA, Hao L-Y, Greider CW. The shortest telomere, not average telomere length, is critical for cell viability and chromosome stability. Cell (2001) 107(1):67–77. doi: 10.1016/S0092-8674(01)00504-9
21. Opitz OG. Telomeres, telomerase and malignant transformation. Curr Mol Med (2005) 5(2):219–26. doi: 10.2174/1566524053586626
22. Wick M, Zubov D, Hagen G. Genomic organization and promoter characterization of the gene encoding the human telomerase reverse transcriptase (hTERT). Gene (1999) 232(1):97–106. doi: 10.1016/S0378-1119(99)00108-0
23. Shi L, Li M, Su B. MCPH1/BRIT1 represses transcription of the human telomerase reverse transcriptase gene. Gene (2012) 495(1):1–9. doi: 10.1016/j.gene.2011.12.053
24. Kilian A, Bowtell DDL, Abud HE, Hime GR, Venter DJ, Keese PK, et al. Isolation of a candidate human telomerase catalytic subunit gene, which reveals complex splicing patterns in different cell types. Hum Mol Genet (1997) 6(12):2011–9. doi: 10.1093/hmg/6.12.2011
25. Wang Y, Kowalski J, Tsai H-L, Marik R, Prasad N, Somervell H, et al. Differentiating alternative splice variant patterns of human telomerase reverse transcriptase in thyroid neoplasms. Thyroid (2008) 18(10):1055–63. doi: 10.1089/thy.2008.0101
26. Xu J-H, Wang Y-C, Geng X, Li Y-Y, Zhang W-M. Changes of the alternative splicing variants of human telomerase reverse transcriptase during gastric carcinogenesis. Pathobiology (2009) 76(1):23–9. doi: 10.1159/000178152
27. Alsiary R, Brownhill SC, Brüning-Richardson A, Hutson R, Griffin N, Morrison EE, et al. Expression analysis of the MCPH1/BRIT1 and BRCA1 tumor suppressor genes and telomerase splice variants in epithelial ovarian cancer. Gene (2018) :672:34–44. doi: 10.1016/j.gene.2018.05.113
28. Dong W, Qian Y, Yang L. Telomerase, hTERT and splice variants in patients with myelodysplastic syndromes. Leuk Res (2014) 38(7):830–5. doi: 10.1016/j.leukres.2014.04.008
29. Mai L, Yi F, Gou X, Zhang J, Wang C, Liu G, et al. The overexpression of MCPH1 inhibits cell growth through regulating cell cycle-related proteins and activating cytochrome c-caspase 3 signaling in cervical cancer. Mol Cell Biochem (2014) 392(1–2):95–107. doi: 10.1007/s11010-014-2022-6
30. Zhou L, Bai Y, Li Y, Liu X, Tan T, Meng S, et al. Overexpression of MCPH1 inhibits uncontrolled cell growth by promoting cell apoptosis and arresting the cell cycle in s and G2/M phase in lung cancer cells. Oncol Lett (2016) 11(1):365–72. doi: 10.3892/ol.2015.3857
31. Denu RA, Burkard ME. Analysis of the “centrosome-ome” identifies MCPH1 deletion as a cause of centrosome amplification in human cancer. Sci Rep (2020) 10(1):11921. doi: 10.1038/s41598-020-68629-4
32. Arroyo M, Kuriyama R, Guerrero I, Keifenheim D, Cañuelo A, Calahorra J, et al. MCPH1 is essential for cellular adaptation to the G(2)-phase decatenation checkpoint. FASEB J Off Publ Fed Am Soc Exp Biol (2019) 33(7):8363–74. doi: 10.1096/fj.201802009RR
33. Arroyo M, Kuriyama R, Trimborn M, Keifenheim D, Cañuelo A, Sánchez A, et al. MCPH1, mutated in primary microcephaly, is required for efficient chromosome alignment during mitosis. Sci Rep (2017) 7(1):1–12. doi: 10.1038/s41598-017-12793-7
34. Wu X, Mondal G, Wang X, Wu J, Yang L, Pankratz VS, et al. Microcephalin regulates BRCA2 and Rad51-associated DNA double-strand break repair. Cancer Res (2009) 69(13):5531–6. doi: 10.1158/0008-5472.CAN-08-4834
35. Morrison AJ, Highland J, Krogan NJ, Arbel-Eden A, Greenblatt JF, Haber JE, et al. INO80 and γ-H2AX interaction links ATP-dependent chromatin remodeling to DNA damage repair. Cell (2004) 119(6):767–75. doi: 10.1016/j.cell.2004.11.037
36. Liang Y, Gao H, Lin S-Y, Goss JA, Du C, Li K. Mcph1/Brit1 deficiency promotes genomic instability and tumor formation in a mouse model. Oncogene (2015) 34(33):4368–78. doi: 10.1038/onc.2014.367
37. Zhang B, Wang E, Dai H, Hu R, Liang Y, Li K, et al. BRIT1 regulates p53 stability and functions as a tumor suppressor in breast cancer. Carcinogenesis (2013) 34(10):2271–80. doi: 10.1093/carcin/bgt190
38. Awaji AA, Shaaban A, Shukla S, Bond J, Morrison E, Cookson V, et al. Evaluation of the role of MCPH1 and p53 expression in response to chemotherapy and subsequent survival in breast cancer. Ann Oncol (2015) 26:iii15. doi: 10.1093/annonc/mdv117.17
39. Szabadkai G, Bianchi K, Várnai P, De Stefani D, Wieckowski MR, Cavagna D, et al. Chaperone-mediated coupling of endoplasmic reticulum and mitochondrial Ca2+ channels. J Cell Biol (2006) 175(6):901–11. doi: 10.1083/jcb.200608073
40. Betz C, Stracka D, Prescianotto-Baschong C, Frieden M, Demaurex N, Hall MN. Feature article: mTOR complex 2-akt signaling at mitochondria-associated endoplasmic reticulum membranes (MAM) regulates mitochondrial physiology. Proc Natl Acad Sci U S A (2013) 110(31):12526–34. doi: 10.1073/pnas.1302455110
41. Journiac N, Gilabert-Juan J, Cipriani S, Benit P, Liu X, Jacquier S, et al. Cell metabolic alterations due to Mcph1 mutation in microcephaly. Cell Rep (2020) 31(2):107506. doi: 10.1016/j.celrep.2020.03.070
42. Richardson J, Shaaban AM, Kamal M, Alisary R, Walker C, Ellis IO, et al. Microcephalin is a new novel prognostic indicator in breast cancer associated with BRCA1 inactivation. Breast Cancer Res Treat (2011) 127(3):639–48. doi: 10.1007/s10549-010-1019-4
43. Jo YH, Kim HO, Lee J, Lee SS, Cho CH, Kang IS, et al. MCPH1 protein expression and polymorphisms are associated with risk of breast cancer. Gene (2013) 517(2):184–90. doi: 10.1016/j.gene.2012.12.088
44. Bhattacharya N, Mukherjee N, Singh RK, Sinha S, Alam N, Roy A, et al. Frequent alterations of MCPH1 and ATM are associated with primary breast carcinoma: clinical and prognostic implications. Ann Surg Oncol (2013) 20 Suppl 3:S424–32. doi: 10.1245/s10434-012-2715-6
45. Partipilo G, Simone G, Scattone A, Scarpi E, Azzariti A, Mangia A. Expression of proteins involved in DNA damage response in familial and sporadic breast cancer patients. Int J cancer (2016) 138(1):110–20. doi: 10.1002/ijc.29699
46. Mantere T, Winqvist R, Kauppila S, Grip M, Jukkola-Vuorinen A, Tervasmäki A, et al. Targeted next-generation sequencing identifies a recurrent mutation in MCPH1 associating with hereditary breast cancer susceptibility. PloS Genet [Internet] (2016) 12(1):e1005816. doi: 10.1371/journal.pgen.1005816
47. Tervasmäki A, Mantere T, Eshraghi L, Laurila N, Tuppurainen H, Ronkainen V-P, et al. Tumor suppressor MCPH1 regulates gene expression profiles related to malignant conversion and chromosomal assembly. Int J cancer (2019) 145(8):2070–81. doi: 10.1002/ijc.32234
48. Cava C, Pisati M, Frasca M, Castiglioni I. Identification of breast cancer subtype-specific biomarkers by integrating copy number alterations and gene expression profiles. Medicina (B Aires) (2021) 57(3):261. doi: 10.3390/medicina57030261
49. Jo YS, Kim SS, Kim MS, Yoo NJ, Lee SH. Candidate tumor suppressor gene MCPH1 is mutated in colorectal and gastric cancers. Int J Colorectal Dis (2017) 32(1):161–2. doi: 10.1007/s00384-016-2675-6
50. Xicola RM, Clark JR, Carroll T, Alvikas J, Marwaha P, Regan MR, et al. Implication of DNA repair genes in lynch-like syndrome. Fam Cancer (2019) 18(3):331–42. doi: 10.1007/s10689-019-00128-6
51. Tricoli JV, Boardman LA, Patidar R, Sindiri S, Jang JS, Walsh WD, et al. A mutational comparison of adult and adolescent and young adult (AYA) colon cancer. Cancer (2018) 124(5):1070–82. doi: 10.1002/cncr.31136
52. Du Z, Tang C-H, Li L-J, Kang L, Zhao J, Jin L, et al. Angiopoietin-2 gene polymorphisms are biomarkers for the development and progression of colorectal cancer in han Chinese. Int J Med Sci (2020) 17(1):97–102. doi: 10.7150/ijms.37675
53. Brüning-Richardson A, Bond J, Alsiary R, Richardson J, Cairns DA, McCormack L, et al. ASPM and microcephalin expression in epithelial ovarian cancer correlates with tumour grade and survival. Br J Cancer (2011) 104(10):1602–10. doi: 10.1038/bjc.2011.117
54. Rai R, Dai H, Multani AS, Li K, Chin K, Gray J, et al. BRIT1 regulates early DNA damage response, chromosomal integrity, and cancer. Cancer Cell (2006) 10(2):145–57. doi: 10.1016/j.ccr.2006.07.002
55. Alsiary R, Brüning-Richardson A, Bond J, Morrison EE, Wilkinson N, Bell SM. Deregulation of microcephalin and ASPM expression are correlated with epithelial ovarian cancer progression. PLoS One (2014) 9(5):e97059. doi: 10.1371/journal.pone.0097059
56. Zhang J, Wu X-B, Fan J-J, Mai L, Cai W, Li D, et al. MCPH1 protein expression in normal and neoplastic lung tissues. Asian Pacific J Cancer Prev APJCP (2013) 14(12):7295–300. doi: 10.7314/APJCP.2013.14.12.7295
57. Wu X, Liu W, Liu X, Ai Q, Yu J. Overexpression of MCPH1 inhibits the migration and invasion of lung cancer cells. Onco Targets Ther (2018) 11:3111–7. doi: 10.2147/OTT.S156102
58. Li C, Liang G, Yao W, Sui J, Shen X, Zhang Y, et al. Identification and functional characterization of long non-coding RNAs in human gastric cancer. Oncol Lett (2018) 15(6):8805–15. doi: 10.3892/ol.2018.8369
59. Hagemann C, Anacker J, Kühnel S, Said H, Patel R, Kämmerer U, et al. Expression analysis of the autosomal recessive primary microcephaly genes MCPH1 (microcephalin) and MCPH5 (ASPM, abnormal spindle-like, microcephaly associated) in human malignant gliomas. Oncol Rep (2008) 20(2):301–8. doi: 10.3892/or_00000007
60. Venkatesh T, Nagashri MN, Swamy SS, Mohiyuddin SMA, Gopinath KS, Kumar A. Primary microcephaly gene MCPH1 shows signatures of tumor suppressors and is regulated by miR-27a in oral squamous cell carcinoma. PloS One (2013) 8(3):e54643. doi: 10.1371/journal.pone.0054643
61. Liang Y. Mcph1/Brit1 deficiency promotes genomic instability and tumor formation in a mouse model yulong. Physiol Behav (2017) 176(33):139–48. doi: 10.1038/onc.2014.367
62. Giallongo C, Tibullo D, La Cava P, Branca A, Parrinello N, Spina P, et al. BRIT1/MCPH1 expression in chronic myeloid leukemia and its regulation of the G2/M checkpoint. Acta Haematol (2011) 126(4):205–10. doi: 10.1159/000329911
63. Kopparapu PK, Miranda C, Fogelstrand L, Mishra K, Andersson PO, Kanduri C, et al. MCPH1 maintains long-term epigenetic silencing of ANGPT2 in chronic lymphocytic leukemia. FEBS J (2015) 282(10):1939–52. doi: 10.1111/febs.13245
64. Bilbao C, Ramírez R, Rodríguez G, Falcón O, León L, Díaz-Chico N, et al. Double strand break repair components are frequent targets of microsatellite instability in endometrial cancer. Eur J Cancer (2010) 46(15):2821–7. doi: 10.1016/j.ejca.2010.06.116
65. Liang Y, Yu L, Zhang D, Zhao X, Gao H, Slagle BL, et al. BRIT1 dysfunction confers synergistic inhibition of hepatocellular carcinoma by targeting poly (ADP-ribose) polymerases and PI3K. Am J Cancer Res (2020) 10(6):1900–18.
66. Wang N, Lu H, Chen W, Gan M, Cao X, Zhang J, et al. Primary microcephaly gene MCPH1 shows a novel molecular biomarker of human renal carcinoma and is regulated by miR-27a. Int J Clin Exp Pathol (2014) 7(8):4895–903. doi: 10.1371/journal.pone.0054643
67. Ghodsi M, Shahmohammadi M, Modarressi MH, Karami F. Investigation of promoter methylation of MCPH1 gene in circulating cell-free DNA of brain tumor patients. Exp Brain Res (2020) 238(9):1903–9. doi: 10.1007/s00221-020-05848-1
68. Viet CT, Yu G, Asam K, Thomas CM, Yoon AJ, Wongworawat YC, et al. The REASON score: an epigenetic and clinicopathologic score to predict risk of poor survival in patients with early stage oral squamous cell carcinoma. biomark Res (2021) 9(1):1–13. doi: 10.1186/s40364-021-00292-x
69. Russo D, Travaglino A, Varricchio S, Merolla F, Ilardi G, Raffone A, et al. BRIT-1 expression and its relationship with PARP-1 and CAF-1/p60 in cutaneous melanoma. J Eur Acad Dermatol Venereol (2021) 35(4):e254–7. doi: 10.1111/jdv.16977
70. Norris JM, Simpson BS, Ball R, Freeman A, Kirkham A, Parry MA, et al. A modified Newcastle-Ottawa scale for assessment of study quality in genetic urological research. Eur Urol (2021) 79(3):325–6. doi: 10.1016/j.eururo.2020.12.017
71. Nagy Á, Munkácsy G, Győrffy B. Pancancer survival analysis of cancer hallmark genes. Sci Rep (2021) 11(1):1–10. doi: 10.1038/s41598-021-84787-5
72. Disease search - my cancer genome. Available at: https://www.mycancergenome.org/content/disease/.
73. Van’t Veer LJ, Dai H, Van De Vijver MJ, He YD, Hart AAM, Mao M, et al. Gene expression profiling predicts clinical outcome of breast cancer. Nature (2002) 415(6871):530–6. doi: 10.1038/415530a
74. Fan C, Oh DS, Wessels L, Weigelt B, Nuyten DSA, Nobel AB, et al. Concordance among gene-expression–based predictors for breast cancer. N Engl J Med (2006) 355(6):560–9. doi: 10.1056/NEJMoa052933
75. Strickland KC, Howitt BE, Shukla SA, Rodig S, Ritterhouse LL, Liu JF, et al. Association and prognostic significance of BRCA1/2-mutation status with neoantigen load, number of tumor-infiltrating lymphocytes and expression of PD-1/PD-L1 in high grade serous ovarian cancer. Oncotarget (2016) 7(12):13587. doi: 10.18632/oncotarget.7277
76. Jiao S, Xia W, Yamaguchi H, Wei Y, Chen M-K, Hsu J-M, et al. PARP inhibitor upregulates PD-L1 expression and enhances cancer-associated ImmunosuppressionPARPi upregulates PD-L1 expression. Clin Cancer Res (2017) 23(14):3711–20. doi: 10.1158/1078-0432.CCR-16-3215
77. Sato H, Niimi A, Yasuhara T, Permata TBM, Hagiwara Y, Isono M, et al. DNA Double-strand break repair pathway regulates PD-L1 expression in cancer cells. Nat Commun (2017) 8(1):1–11. doi: 10.1038/s41467-017-01883-9
78. Bond J, Roberts E, Springell K, Lizarraga S, Scott S, Higgins J, et al. A centrosomal mechanism involving CDK5RAP2 and CENPJ controls brain size. Nat Genet (2005) 37(4):353–5. doi: 10.1038/ng1539
Keywords: MCPH1, microcephalin, BRIT1, cancer, breast cancer
Citation: Alsolami M, Aboalola D, Malibari D, Alghamdi T, Alshekhi W, Jad H, Rumbold-Hall R, Altowairqi AS, Bell SM and Alsiary RA (2023) The emerging role of MCPH1/BRIT1 in carcinogenesis. Front. Oncol. 13:1047588. doi: 10.3389/fonc.2023.1047588
Received: 18 September 2022; Accepted: 16 January 2023;
Published: 31 January 2023.
Edited by:
Liusheng Peng, Third Military Medical University, ChinaReviewed by:
Haiyang Zhang, Tianjin Medical University, ChinaManoj Kumar Kashyap, Amity University Gurgaon, India
Yukun Tan, University of Texas MD Anderson Cancer Center, United States
Copyright © 2023 Alsolami, Aboalola, Malibari, Alghamdi, Alshekhi, Jad, Rumbold-Hall, Altowairqi, Bell and Alsiary. This is an open-access article distributed under the terms of the Creative Commons Attribution License (CC BY). The use, distribution or reproduction in other forums is permitted, provided the original author(s) and the copyright owner(s) are credited and that the original publication in this journal is cited, in accordance with accepted academic practice. No use, distribution or reproduction is permitted which does not comply with these terms.
*Correspondence: Rawiah Abdullah Alsiary, alsiaryra@ngha.med.sa