- 1Division of Neuroimaging Research and Barrow Neuroimaging Innovation Center, Barrow Neuroimaging Institute, Phoenix, AZ, United States
- 2Department of Radiology, Division of Neuroradiology, Mayo Clinic Arizona, Phoenix, AZ, United States
- 3Neuroradiology, Southwest Neuroimaging at Barrow Neurological Institute, Phoenix, AZ, United States
- 4Early Clinical Development, Genentech, San Francisco, CA, United States
- 5Cancer System Imaging, The University of Texas MD Anderson Cancer Center, Houston, TX, United States
Background: Relative cerebral blood volume (rCBV) obtained from dynamic susceptibility contrast (DSC) MRI is widely used to distinguish high grade glioma recurrence from post treatment radiation effects (PTRE). Application of rCBV thresholds yield maps to distinguish between regional tumor burden and PTRE, a biomarker termed the fractional tumor burden (FTB). FTB is generally measured using conventional double-dose, single-echo DSC-MRI protocols; recently, a single-dose, dual-echo DSC-MRI protocol was clinically validated by direct comparison to the conventional double-dose, single-echo protocol. As the single-dose, dual-echo acquisition enables reduction in the contrast agent dose and provides greater pulse sequence parameter flexibility, there is a compelling need to establish dual-echo DSC-MRI based FTB mapping. In this study, we determine the optimum standardized rCBV threshold for the single-dose, dual-echo protocol to generate FTB maps that best match those derived from the reference standard, double-dose, single-echo protocol.
Methods: The study consisted of 23 high grade glioma patients undergoing perfusion scans to confirm suspected tumor recurrence. We sequentially acquired single dose, dual-echo and double dose, single-echo DSC-MRI data. For both protocols, we generated leakage-corrected standardized rCBV maps. Standardized rCBV (sRCBV) thresholds of 1.0 and 1.75 were used to compute single-echo FTB maps as the reference for delineating PTRE (sRCBV < 1.0), tumor with moderate angiogenesis (1.0 < sRCBV < 1.75), and tumor with high angiogenesis (sRCBV > 1.75) regions. To assess the sRCBV agreement between acquisition protocols, the concordance correlation coefficient (CCC) was computed between the mean tumor sRCBV values across the patients. A receiver operating characteristics (ROC) analysis was performed to determine the optimum dual-echo sRCBV threshold. The sensitivity, specificity, and accuracy were compared between the obtained optimized threshold (1.64) and the standard reference threshold (1.75) for the dual-echo sRCBV threshold.
Results: The mean tumor sRCBV values across the patients showed a strong correlation (CCC = 0.96) between the two protocols. The ROC analysis showed maximum accuracy at thresholds of 1.0 (delineate PTRE from tumor) and 1.64 (differentiate aggressive tumors). The reference threshold (1.75) and the obtained optimized threshold (1.64) yielded similar accuracy, with slight differences in sensitivity and specificity which were not statistically significant (1.75 threshold: Sensitivity = 81.94%; Specificity: 87.23%; Accuracy: 84.58% and 1.64 threshold: Sensitivity = 84.48%; Specificity: 84.97%; Accuracy: 84.73%).
Conclusions: The optimal sRCBV threshold for single-dose, dual-echo protocol was found to be 1.0 and 1.64 for distinguishing tumor recurrence from PTRE; however, minimal differences were observed when using the standard threshold (1.75) as the upper threshold, suggesting that the standard threshold could be used for both protocols. While the prior study validated the agreement of the mean sRCBV values between the protocols, this study confirmed that their voxel-wise agreement is suitable for reliable FTB mapping. Dual-echo DSC-MRI acquisitions enable robust single-dose sRCBV and FTB mapping, provide pulse sequence parameter flexibility and should improve reproducibility by mitigating variations in preload dose and incubation time.
Introduction
Glioblastoma (GBM) is the most aggressive and common primary malignant brain tumor in humans. Treatment includes surgical resection followed by radiation treatment and chemotherapy. Within the first 3-6 months of radiation treatment, patients may exhibit MRI findings that are consistent with tumor recurrence and/or post treatment radiation effects (PTRE) (1). Thus, an important challenge in patient management is distinguishing tumor progression from PTRE (2–4). Conventional post-contrast T1-weighted images are not capable of distinguishing tumor recurrence from PTRE because treatment-induced changes and tumor recurrence similarly present as new contrast-enhancement, most often adjacent to the surgical resection cavity and within the radiotherapy treatment field. Although tumor and PTRE share similar radiological features, they represent vastly different responses to the radiation treatment response (4). More specifically, presence of PTRE shows spontaneous stabilization and thus indicates positive response to the treatment, whereas tumor recurrence indicates treatment failure. Hence, an early differentiation between PTRE and tumor progression would improve treatment planning (4–6). Advanced imaging techniques like perfusion, diffusion, and PET imaging have proven useful for delineating tumor from PTRE after therapy (7, 8).
Dynamic susceptibility contrast (DSC)-MRI based perfusion imaging has been widely validated with image-localized histopathology and is recommended for routine use in GBM patients (9–14). The derived relative cerebral blood volume (rCBV) maps provide non-invasive interrogation of the tumor vasculature that arises from aberrant angiogenic pathways. In particular, high grade and progressive tumor regions present with elevated rCBV values, whereas rCBV is markedly lower in regions of PTRE (10, 12, 15). Several studies, across multiple sites, have leveraged localized image-guided histopathology to validate that rCBV measurements reliably differentiate regional tumor recurrence from PTRE via correlation with histologic tumor burden (10, 16, 17). These studies have found that when DSC-MRI data is acquired and analyzed using uniform methodology, common rCBV thresholds can be employed to identify, and regionally map, PTRE and recurrence. The application of these thresholds enables computation of images called fractional tumor burden (FTB) maps (15, 18, 19). Previous studies have used rCBV threshold of 1.0 to differentiate tumor from PTRE (18). To identify more aggressive tumors (eg: predictive of outcomes), FTB maps are often computed using two rCBV thresholds (1.0, to distinguish tumor from PTRE & 1.75, to identify aggressive tumors). Thus, the derived FTB maps enable efficient visualization of 3 FTB classes: FTBlow (rCBV < 1.0) representive of PTRE, FTBmid (1.0 < rCBV < 1.75) highlighting tumor with moderate angiogenesis and FTBhigh (rCBV > 1.75) demarcating aggressive tumor with high angiogenesis, each represented with a unique color (20, 21). Standardization of rCBV, transforms rCBV maps to a standard intensity scale that eliminates the variability associated with user dependent ROIs used for normalization (22). Recent studies have recommended standardization to be an important step toward workflow optimization and consensus methodology (23, 24). In this study, standardized rCBV (sRCBV) values and two thresholds were used to compute FTB maps.
DSC-MRI data is most often acquired using single gradient echo acquisitions, but contrast agent leakage effects, due to the disruption of the blood brain barrier, can reduce rCBV accuracy. To mitigate these effects, a preload of contrast agent and/or post-processing leakage correction algorithms can be used (25). Recently, two alternative acquisition strategies have been proposed to mitigate the need for multiple contrast agent doses. First, a low-flip angle, single-dose protocol was shown to provide reliable rCBV maps (26). However, the fidelity and reproducibility of the low-flip angle approach requires uniform and field strength dependent pulse sequence parameters. Alternatively, replacing the single-echo with a dual-echo pulse sequence eliminates T1 leakage effects and the need for preload dosing (25, 27–29). Residual T2* leakage effects can be removed using post-processing leakage correction algorithms. The validity of single-dose, dual-echo DSC-MRI protocols, and the derived rCBV maps, were recently validated by direct comparison to the standard double-dose, single-echo protocol (30). Favorably, the dual-echo approach decouples rCBV accuracy from pulse sequence parameters, enabling greater pulse sequence parameter flexibility and improved reproducibility (30). While these studies demonstrated high accuracy for single-dose rCBV, there is a compelling need to further establish dual-echo DSC-MRI based FTB mapping to differentiate recurrent tumor and treatment effects.
In this study, we determine optimum rCBV thresholds for the single-dose, dual-echo DSC-MRI approach to generate FTB maps that distinguish tumor and PTRE and best match with the reference standard, double-dose, single-echo FTB map.
Material and methods
Patients
This retrospective study was approved by Dignity Health Institutional Review Board (IRB). Data acquisition was performed as part of a clinical standard of care scan, spanning October 2018 to November 2020. Inclusion criteria were presence of contrast enhancing lesions on the DSC-MRI imaging, surgical resection or biopsy of the mass, patient age >18 years, availability of perfusion datasets for both preload and main injection and high-grade glioblastoma. Exclusion criteria included different pulse sequence parameters (n = 9), poor injection (n = 1), susceptibility artifacts (n = 4), partial volume effects (n = 1), missing dynamic data points (n = 16) and low-grade gliomas (n = 14). After screening a total of 68 patients, 23 patients were included in this study. All patients received treatment within 6 weeks after surgery or biopsy. The time between diagnosis, following surgical resection or biopsy, and the date of the perfusion scan ranged from 4 - 32 months.
MRI imaging protocol
All imaging studies were performed on a 3T MRI (Ingenia, Philips Healthcare, Best, Netherlands). The standard pre-contrast and post-contrast 3D anatomical T1-weighted images were obtained using a gradient echo sequence with the following acquisition parameters: TE/TR: 4.4/7.9 ms, acquisition matrix: 512 x 512, voxel size: 1.0 x 1.0 mm2, slice thickness: 1.0 mm, 170 sagittal slices, flip-angle: 8°. Two consecutive DSC-MRI perfusion datasets were acquired for all patients using two sequential full bolus doses of gadolinium-based contrast agent (gadobutrol, Gadavist) injections with spatial resolution of 1.75 x 1.75 mm2 (acquisition matrix: 128 x 128), slice thickness of 5 mm (20 axial slices), and pixel bandwidth of approximately 2 kHz. Bolus injections were administered after 30 seconds of baseline acquisition at a rate of 3 ml/s using a power injector. A dual-echo DSC protocol (TE1/TE2 = 7.4/33.6 ms; TR = 581.9 ms; FA = 75°) was performed for the first bolus injection for the evaluation of the single-bolus, dual-echo protocol. This injection serves as the preload for the standard double-dose, single-echo protocol. After a delay of 6 minutes, a second dose of contrast bolus was injected for the acquisition of the standard single-echo DSC protocol (TE/TR = 30/1400 ms; FA = 60°).
Data analysis
For each patient, standardized relative cerebral blood volume (sRCBV) maps were generated using the commercially available, FDA-approved, clinical software plug-in, IB Neuro X2™ (Imaging Biometrics, Version 21.12, Elm Grove, Wisconsin). Since high-grade tumor disrupts the blood brain barrier and yields discrepancies in rCBV values, the Boxerman-Schmainda-Weisskoff (BSW) leakage correction was performed on both datasets to minimize T1 and T2* leakage effects (31). The sRCBV maps generated were co-registered to the respective T1-weighted post-contrast images using IB Delta Suite™ (Imaging Biometrics, Version 21.05). For semi-automated analysis, enhancing tumor region-of-interests (ROIs) were generated using IB RadTech™ (Imaging Biometrics, Version 21.05) based on ΔT1 images (post-contrast T1w image – pre-contrast T1w image).
Statistical analysis
The sRCBV agreement between the two acquisition protocols on the mean tumor ROI was assessed by computing the concordance correlation coefficient (CCC) across all the patients. To identify optimal sRCBV thresholds that distinguish tumor and PTRE, a receiver operating characteristic (ROC) analysis was performed on the sRCBV values at the voxel-wise level across all patients. The optimal thresholds corresponding to the maximum value of accuracy (defined as the average of sensitivity and specificity) were identified from the ROC curve. The sensitivity, specificity, and accuracy were compared between the obtained optimum threshold and the histologically validated reference threshold (20, 21).
Results
A total of 23 subjects that satisfied the inclusion/exclusion criteria were identified for the analysis in this study. There were 11 males and 12 females included in the study with an average age of 54.9 years (SD = 12.25; Range = 30 - 79).
The sRCBV correlation on the mean tumor ROI between the single-dose, dual-echo protocol and the standard double-dose, single-echo protocol is depicted in Figure 1. Consistent with the prior study (26), there is strong agreement with a CCC value of 0.96 between the dual-echo and single-echo protocols.
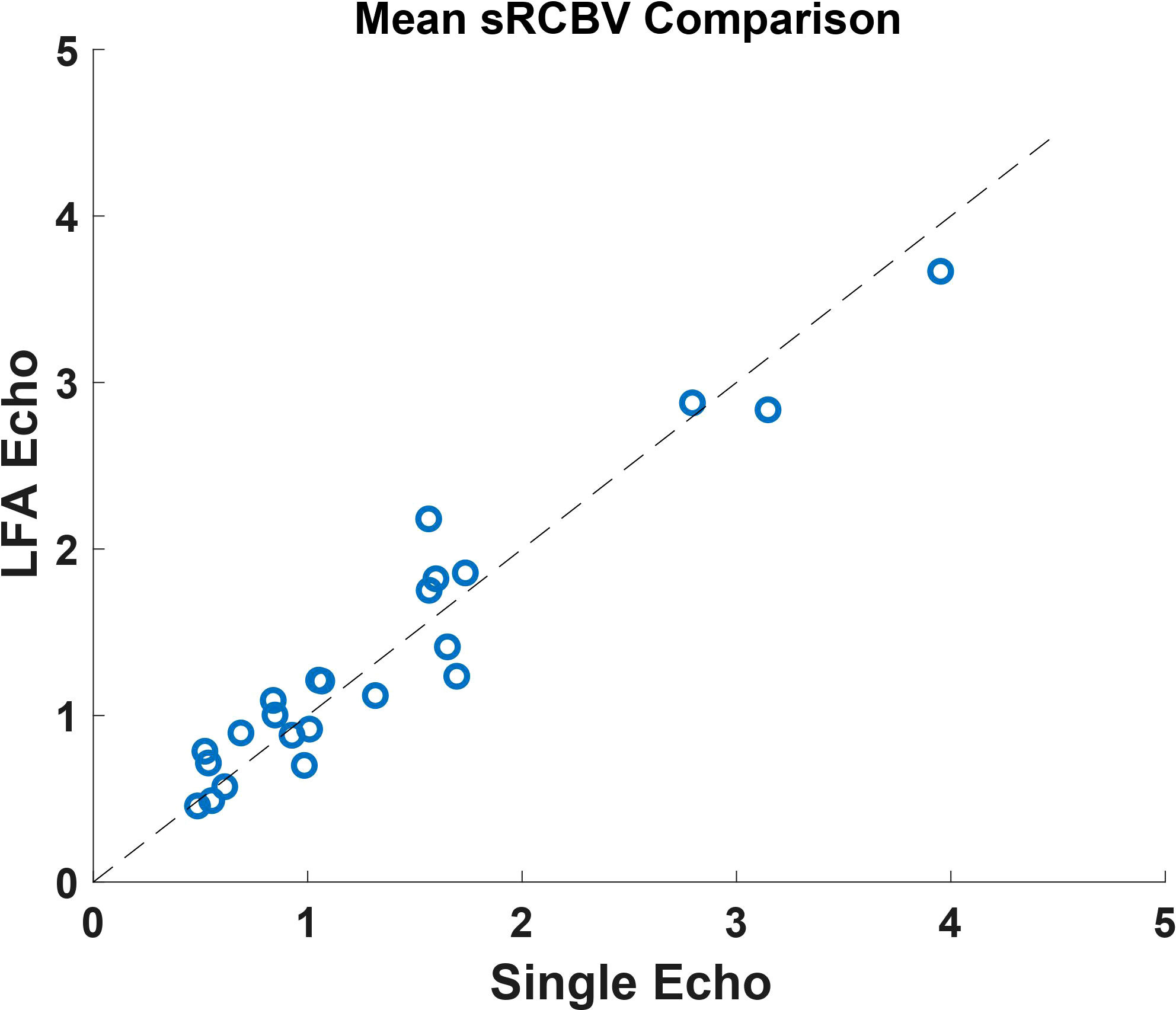
Figure 1 A comparison of the mean tumor ROI between the single-dose, dual-echo and the double-dose, single-echo based sRCBV values across all the patients (n=23) included in the study. This shows a strong agreement across the two protocols with a concordance correlation coefficient value of 0.96.
Using the sRCBV values, the receiver operating characteristic (ROC) curve identified the sensitivity and specificity across different thresholds as shown in Figure 2. The area under the ROC (AUROC) curve for sRCBV < 1.0 and sRCBV > 1.64 was 0.89 and 0.91, respectively. A lower threshold of 1.0 (delineating PTRE and tumor voxels) and an upper threshold of 1.64 (delineating tumor with high and moderate angiogenesis) provided the maximum accuracy of 82.32% and 84.73%, respectively. The sensitivity and specificity corresponding to the maximum accuracy is 87.77% and 76.88% for 1.0 threshold and 84.48% and 84.97% for 1.64 threshold, respectively.
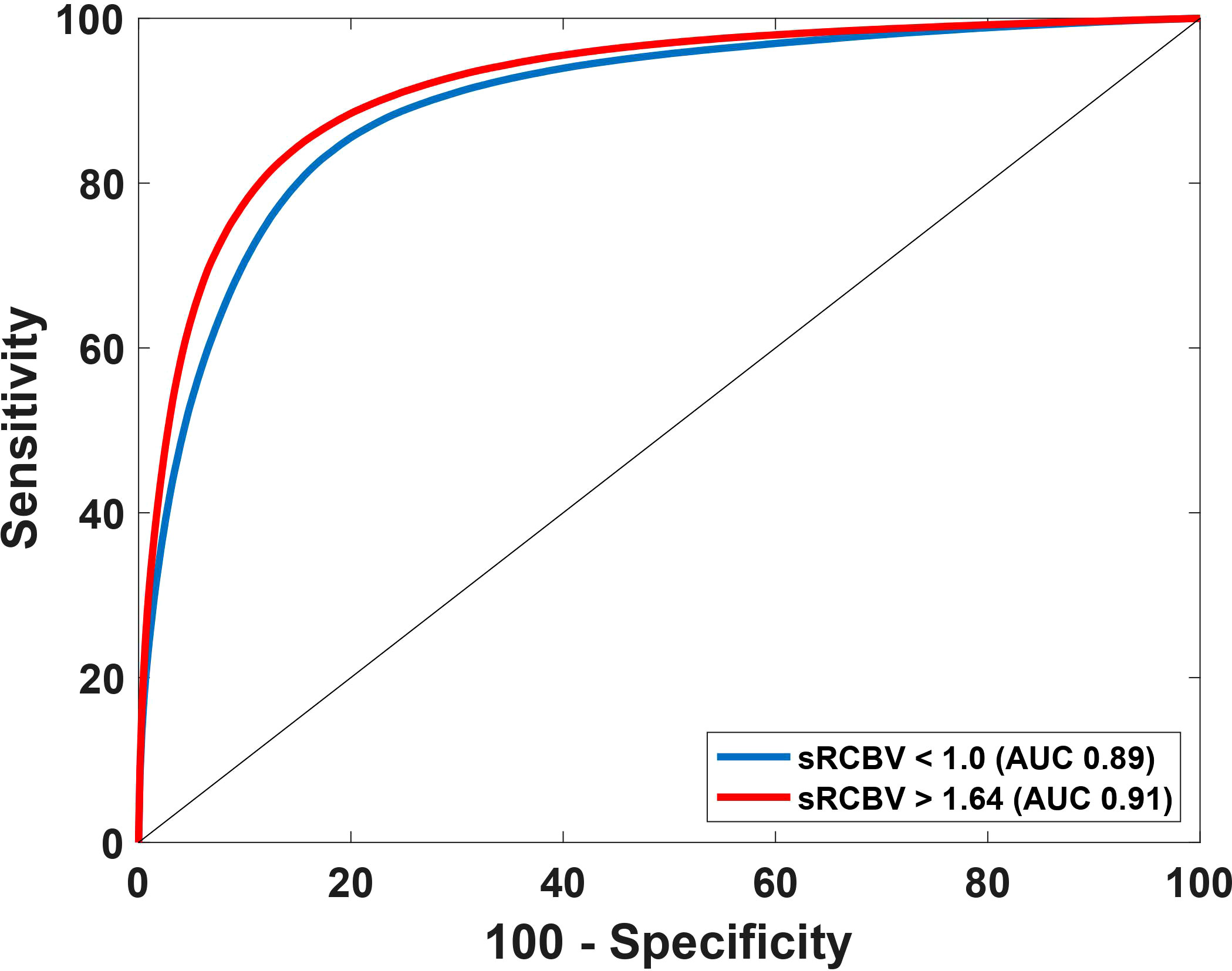
Figure 2 Receiver Operating Characteristics (ROC) curve using sRCBV values to identify the dual-echo thresholds. The AUROC for thresholds 1.0 and 1.64 are 0.89 and 0.91 respectively.
According to prior studies (18), the histologically validated standard rCBV threshold to differentiate PTRE and tumor is 1.0, while 1.75 is often chosen as a marker of aggressive tumor (20, 21). By comparing the sensitivity, specificity, and accuracy between the reference threshold of 1.75 and the obtained optimized threshold of 1.64, we noted that although the sensitivity and specificity varies between the thresholds as shown in Table 1, the value of accuracy remains the same, which is depicted in Figure 3.

Table 1 Comparison of sensitivity, specificity and accuracy between the sRCBV threshold generated from ROC analysis (1.64) and the reference standard threshold (1.75) across 23 subjects.
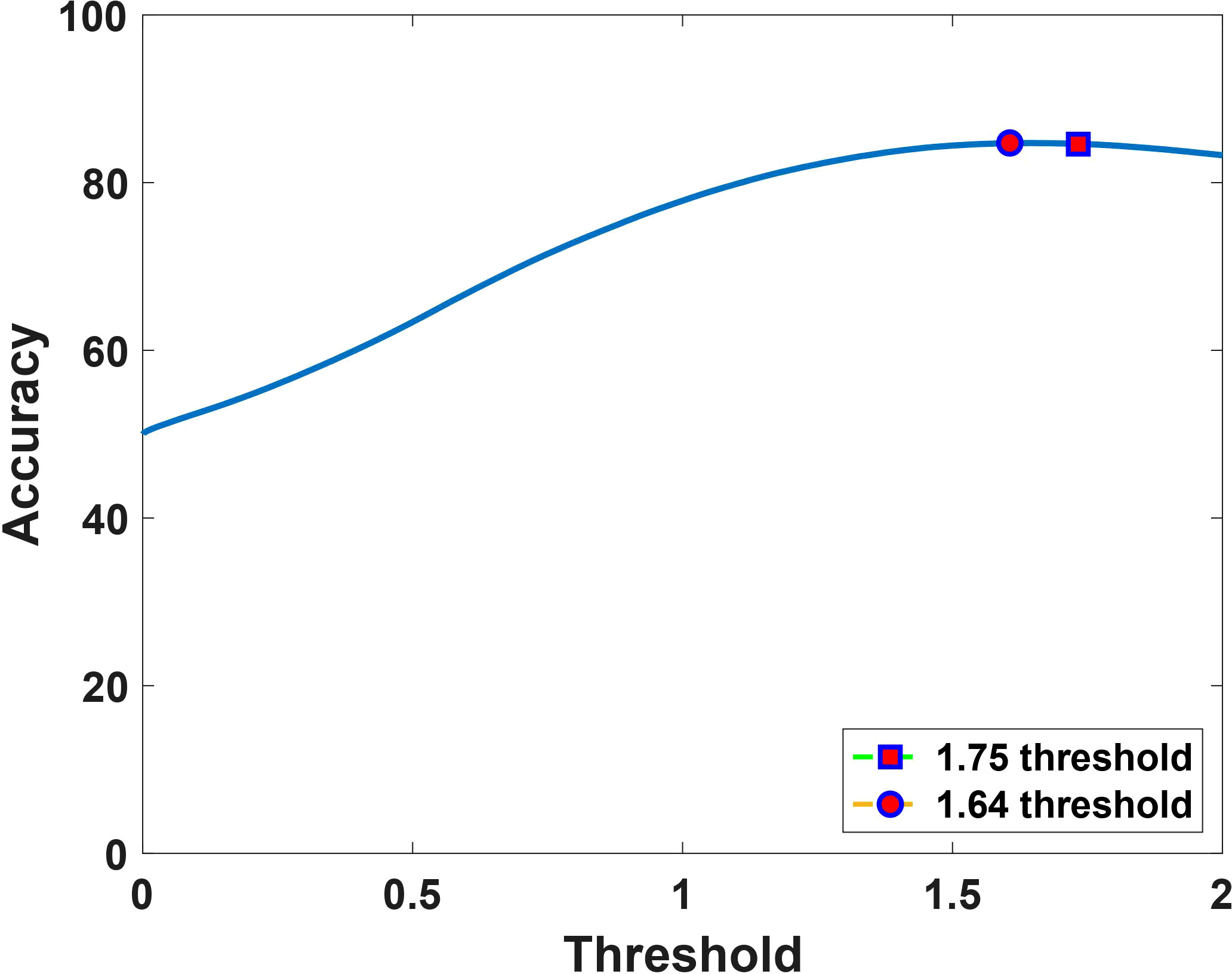
Figure 3 Accuracy as a function of the dual-echo derived sRCBV threshold, showing that the computed optimal upper threshold, 1.64 (marked in circle) has consistent accuracy (84%) with the reference value, 1.75 (marked in square).
Figure 4 visually summarizes two separate cases showing the post-contrast T1-weighted images with the enhancing tumor and the corresponding FTB maps for the single-dose, dual-echo and the double-dose, single-echo protocols using the thresholds 1.0 and 1.75. The PTRE voxels are represented in blue, where the sRCBV value is less than 1.0 and is considered as FTBlow. The sRCBV voxels between 1.0 and 1.75 are FTBmid in yellow, considered as tumor with moderate angiogenesis. The FTBhigh constitute the tumor voxels with high angiogenesis in red with sRCBV values greater than 1.75. Visually, the FTB maps across the two protocols are in strong agreement, each identifying the same regions of tumor and PTRE.
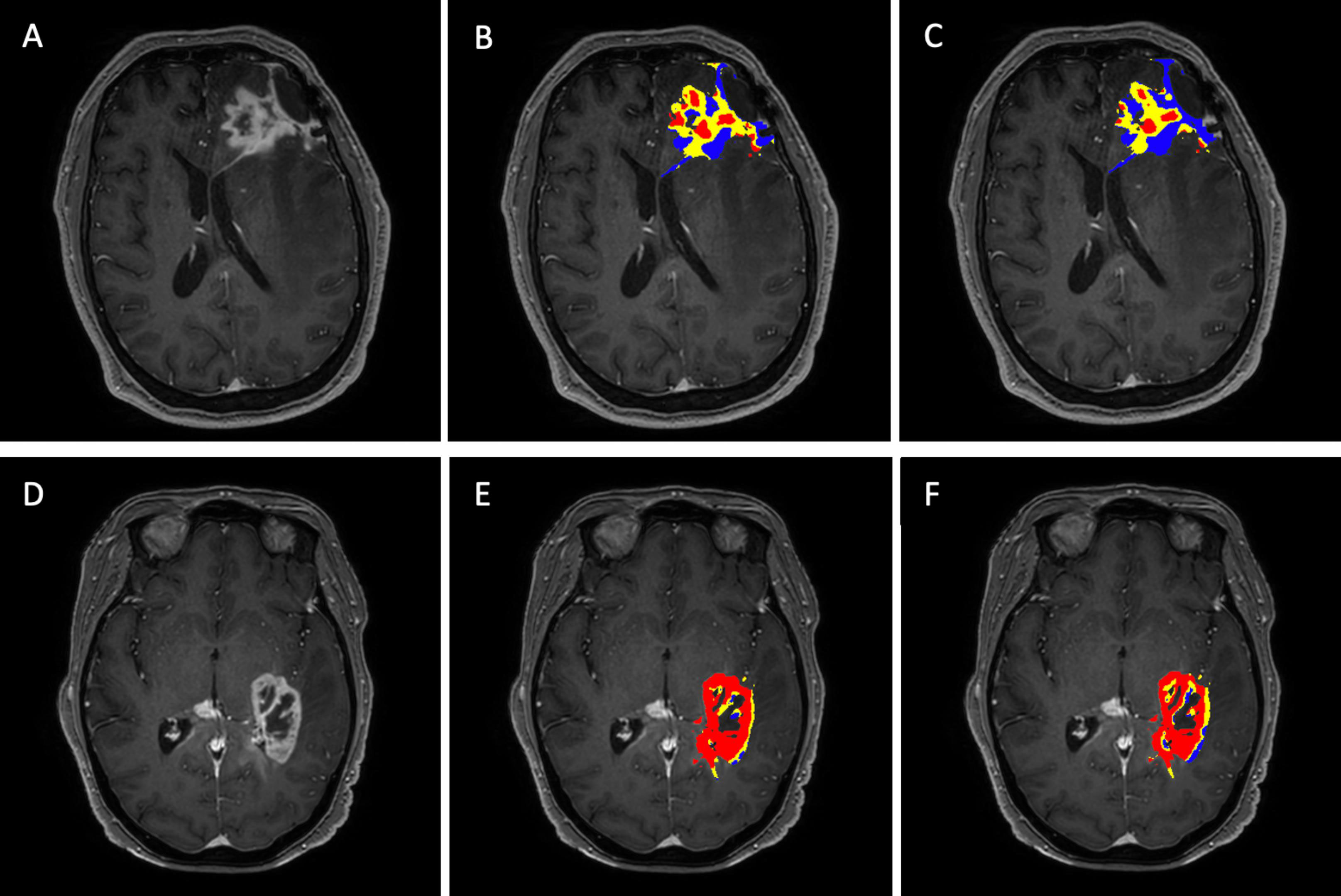
Figure 4 FTB maps generated for the single-dose, dual-echo and double-dose, single-echo protocol for two separate patients with the anatomical post-contrast T1 – weighted images in the far-left column (A, D). Patient (A–C) is a 58-year-old male with grade IV glioblastoma presenting 11 months after the stereotactic radiosurgery. Patient (D–F) is a 65-year-old female with grade IV glioblastoma presenting 4 months after biopsy. The middle column corresponds to the FTB maps (B, E) generated for the single-dose, dual-echo protocol. The far-right column corresponds to the FTB maps (C, F) generated from the standard reference double-dose, single-echo protocol. The standard thresholds of 1 and 1.75 were used for both protocols. Blue represents areas of PTRE, yellow and red represents tumor with moderate and high angiogenesis, respectively.
Discussion
The use of DSC-MRI, with the double contrast agent dose and single echo pulse sequence, has been validated for distinguishing tumor recurrence from treatment related effects like pseudoprogression or radiation necrosis (25, 27, 32). The accuracy of the computed CBV maps derives from the reduction of T1 leakage effects, from the contrast agent preload, and T2* leakage effects using the BSW leakage correction algorithm (33). Similarly, dual-echo pulse sequences enable reliable CBV mapping with a single contrast agent dose through elimination of T1 leakage effects and mitigation of T2* effects using the BSW correction (30, 34). This reduces the contrast agent dose, provides significant flexibility in pulse sequence parameters, and improves reproducibility by mitigating the variation in the preload dose and incubation time (30). In a study using a patient-based and validated DSC-MRI digital reference object (DRO), multi-echo acquisitions have shown more robust results than single-echo protocols as it essentially decouples both TR and FA from rCBV accuracy. This leads to a wide range of pulse sequence parameters to yield robust rCBV maps (34). Further, as previously shown dual-echo acquisitions enable simultaneous acquisitions of DSC- and DCE-MRI data, enabling quantification of tumor permeability via the contrast agent transfer constant known as Ktrans (35, 36). In this study, we aimed to further expand the clinical utility of dual-echo DSC-MRI by determination of a dual-echo derived voxel-wise CBV threshold for FTB mapping by comparison to that computed using the double-dose, single-echo standard.
To facilitate the clinical translation of the derived threshold, we employed FDA- approved clinically available software that has the capability of deriving CBV maps from single and dual-echo DSC-MRI data. In the analysis, we also leveraged standardization of rCBV maps, as this eliminates the variability associated with user-dependent ROIs for normalization (22). Prior studies have demonstrated that standardized rCBV values increase CBV reproducibility across patients and sites (22–24). Recent studies have also shown that standardization of rCBV, derived from single-echo data, achieves similar accuracy when compared with the normalized rCBV in differentiating recurrent tumor from PTRE (23).
Our study further corroborates the strong agreement between the mean sRCBV tumor values across the single-dose, dual-echo and double-dose, single-echo protocols (30). Agreement at the voxel-wise level is necessary for reliable FTB mapping. For the reference single-echo protocol, the histologically validated sRCBV threshold to distinguish tumor and PTRE is 1.0, and other studies employ an upper threshold of 1.75 as a marker of aggressive tumor (18, 20, 21, 23). ROC analysis performed on the dual-echo sRCBV identified 1.0 and 1.64 as the optimum thresholds yielding maximum accuracy. When the accuracy of the upper threshold of 1.64 was compared with the reference standard 1.75 for the dual-echo protocol, the accuracy remained effectively the same.
Therefore, with the standard threshold, the single-dose, dual-echo approach yields FTB map that strongly agree with the reference standard, single-echo FTB map, providing a compelling option to reduce contrast agent dose and improve standardization via dual-echo acquisitions in a clinical setting.
In conclusion, this study demonstrates that the single-dose, dual-echo protocol can be reliably used to distinguish tumor and PTRE. Validation in a larger sample size and across MRI vendors and sites would further strengthen the clinical utility of dual-echo DSC-MRI. Eventhough the well-validated double-dose, single-echo protocol generated FTB maps was used as the ground truth reference, one of the limitations of the study was the lack of histopathology correlation. An important finding of this study is the similarity between the single-dose, dual-echo FTB maps and the standard double dose, single-echo FTB maps using the standard sRCBV thresholds (1 and 1.75). This indicates that existing clinical software for FTB mapping can be reliably applied to dual-echo DSC-MRI data to diagnose and quantify recurrent high-grade tumor from treatment effects. Given that dual-echo acquisitions eliminate the need for a preload injection, decouples rCBV accuracy from pulse sequence parameters, and is more reliable across a range of brain tumor types, this study provides further motivation to continue its refinement and use in clinical studies and trials.
Data availability statement
The raw data supporting the conclusions of this article will be made available by the authors, without undue reservation.
Author contributions
CQ acquired the funding for the study. CQ, AS, LH and LB designed the studies. CQ, AS, LA and JK guided interpretation of results. AS, LA and JK were responsible for data acquisition. AA and RC were responsible for data analysis and AA for manuscript preparation. All authors contributed to the article and approved the submitted version.
Funding
This work was funded by NIH R01 CA158079, NIH R01 CA213158.
Acknowledgments
We would like to acknowledge Belinda Gutierrez for helping to acquire the data.
Conflict of interest
LB is employed by Genentech Inc.
The remaining authors declare that the research was conducted in the absence of any commercial or financial relationships that could be construed as a potential conflict of interest.
Publisher’s note
All claims expressed in this article are solely those of the authors and do not necessarily represent those of their affiliated organizations, or those of the publisher, the editors and the reviewers. Any product that may be evaluated in this article, or claim that may be made by its manufacturer, is not guaranteed or endorsed by the publisher.
References
1. Sanghera P, Perry J, Sahgal A, Symons S, Aviv R, Morrison M, et al. Pseudoprogression following chemoradiotherapy for glioblastoma multiforme. Can J Neurol Sci (2010) 37(1):36–42. doi: 10.1017/S0317167100009628
2. Brandsma D, van den Bent MJ. Pseudoprogression and pseudoresponse in the treatment of gliomas. Curr Opin Neurol (2009) 22(6):633–8. doi: 10.1097/WCO.0b013e328332363e
3. Ellingson BM, Chung C, Pope WB, Boxerman JL, Kaufmann TJ. Pseudoprogression, radionecrosis, inflammation or true tumor progression? challenges associated with glioblastoma response assessment in an evolving therapeutic landscape. J Neurooncol (2017) 134(3):495–504. doi: 10.1007/s11060-017-2375-2
4. Tran DT, Jensen R. Treatment-related brain tumor imaging changes: So-called “Pseudoprogression” vs. tumor progression: Review and future research opportunities. Surg Neurol Int (2013) 4(4):129.
5. Geer CP, Simonds J, Anvery A, Chen MY, Burdette JH, Zapadka ME, et al. Does MR perfusion imaging impact management decisions for patients with brain tumors? a prospective study. AJNR Am J Neuroradiol (2012) 33(3):556–62. doi: 10.3174/ajnr.A2811
6. Law M, Young RJ, Babb JS, Peccerelli N, Chheang S, Gruber ML, et al. Gliomas: Predicting time to progression or survival with cerebral blood volume measurements at dynamic susceptibility-weighted contrast-enhanced perfusion MR imaging. Radiology (2008) 247(2):490–8. doi: 10.1148/radiol.2472070898
7. Qin D, Yang G, Jing H, Tan Y, Zhao B, Zhang H. Tumor progression and treatment-related changes: Radiological diagnosis challenges for the evaluation of post treated glioma. Cancers (2022) 14(15):3771. doi: 10.3390/cancers14153771
8. Soni N, Ora M, Mohindra N, Menda Y, Bathla G. Diagnostic performance of PET and perfusion-weighted imaging in differentiating tumor recurrence or progression from radiation necrosis in posttreatment gliomas: A review of literature. AJNR Am J Neuroradiol (2020) 41(9):1550-1557. doi: 10.3174/ajnr.A6685
9. Shiroishi MS, Boxerman JL, Pope WB. Physiologic MRI for assessment of response to therapy and prognosis in glioblastoma. Neuro Oncol (2016) 18(4):467–78. doi: 10.1093/neuonc/nov179
10. Wang L, Wei L, Wang J, Li N, Gao Y, Ma H, et al. Evaluation of perfusion MRI value for tumor progression assessment after glioma radiotherapy: A systematic review and meta-analysis. Medicine (2020) 99(52):e23766. doi: 10.1097/MD.0000000000023766
11. Prah MA, Al-Gizawiy MM, Mueller WM, Cochran EJ, Hoffmann RG, Connelly JM, et al. Spatial discrimination of glioblastoma and treatment effect with histologically-validated perfusion and diffusion magnetic resonance imaging metrics. J Neurooncol (2018) 136(1):13–21. doi: 10.1007/s11060-017-2617-3
12. Fatterpekar GM, Galheigo D, Narayana A, Johnson G, Knopp E. Treatment-related change versus tumor recurrence in high-grade gliomas: A diagnostic conundrum–use of dynamic susceptibility contrast-enhanced (DSC) perfusion MRI. Am J Roentgenol (2012) 198(1):19–26. doi: 10.2214/AJR.11.7417
13. Prager AJ, Martinez N, Beal K, Omuro A, Zhang Z, Young RJ. Diffusion and perfusion MRI to differentiate treatment-related changes including pseudoprogression from recurrent tumors in high-grade gliomas with histopathologic evidence. AJNR Am J Neuroradiol (2015) 36(5):877–85. doi: 10.3174/ajnr.A4218
14. Kim YH, Oh SW, Lim YJ, Park CK, Lee SH, Kang KW, et al. Differentiating radiation necrosis from tumor recurrence in high-grade gliomas: Assessing the efficacy of 18F-FDG PET, 11C-methionine PET and perfusion MRI. Clin Neurol Neurosurg (2010) 112(9):758–65. doi: 10.1016/j.clineuro.2010.06.005
15. Gasparetto EL, Pawlak MA, Patel SH, Huse J, Woo JH, Krejza J, et al. Posttreatment recurrence of malignant brain neoplasm: Accuracy of relative cerebral blood volume fraction in discriminating low from high malignant histologic volume fraction. Radiology (2009) 250(3):887–96. doi: 10.1148/radiol.2502071444
16. Patel P, Baradaran H, Delgado D, Askin G, Christos P, John Tsiouris A, et al. MR perfusion-weighted imaging in the evaluation of high-grade gliomas after treatment: A systematic review and meta-analysis. Neuro-Oncology (2017) 19(1):118–27. doi: 10.1093/neuonc/now148
17. Hu LS, Baxter LC, Smith KA, Feuerstein BG, Karis JP, Eschbacher JM, et al. Relative cerebral blood volume values to differentiate high-grade glioma recurrence from posttreatment radiation effect: Direct correlation between image-guided tissue histopathology and localized dynamic susceptibility-weighted contrast-enhanced perfusion MR imaging measurements. AJNR Am J Neuroradiol (2009) 30(3):552–8. doi: 10.3174/ajnr.A1377
18. Hu LS, Eschbacher JM, Heiserman JE, Dueck AC, Shapiro WR, Liu S, et al. Reevaluating the imaging definition of tumor progression: perfusion MRI quantifies recurrent glioblastoma tumor fraction, pseudoprogression, and radiation necrosis to predict survival. Neuro-Oncology (2012) 14(7):919–30. doi: 10.1093/neuonc/nos112
19. Connelly JM, Prah MA, Santos-Pinheiro F, Mueller W, Cochran E, Schmainda KM. Magnetic resonance imaging mapping of brain tumor burden: Clinical implications for neurosurgical management: Case report. Neurosurg Open (2022) 2(4):okab029. doi: 10.1093/neuopn/okab029
20. Iv M, Liu X, Lavezo J, Gentles AJ, Ghanem R, Lummus S, et al. Perfusion MRI-based fractional tumor burden differentiates between tumor and treatment effect in recurrent glioblastomas and informs clinical decision-making. AJNR Am J Neuroradiol (2019) 40(10):1649-57. doi: 10.3174/ajnr.A6211
21. Kuo F, Ng NN, Nagpal S, Pollom EL, Soltys S, Hayden-Gephart M, et al. DSC perfusion MRI–derived fractional tumor burden and relative CBV differentiate tumor progression and radiation necrosis in brain metastases treated with stereotactic radiosurgery. AJNR Am J Neuroradiol (2022) 43(5):689–95. doi: 10.3174/ajnr.A7501
22. Bedekar D, Jensen T, Schmainda KM. Standardization of relative cerebral blood volume (rCBV) image maps for ease of both inter- and intrapatient comparisons. Magn Reson Med (2010) 64(3):907–13. doi: 10.1002/mrm.22445
23. Hoxworth JM, Eschbacher JM, Gonzales AC, Singleton KW, Leon GD, Smith KA, et al. Performance of standardized relative CBV for quantifying regional histologic tumor burden in recurrent high-grade glioma: Comparison against normalized relative CBV using image-localized stereotactic biopsies. AJNR Am J Neuroradiol (2020) 41(3):408–15. doi: 10.3174/ajnr.A6486
24. Prah MA, Stufflebeam SM, Paulson ES, Kalpathy-Cramer J, Gerstner ER, Batchelor TT, et al. Repeatability of standardized and normalized relative CBV in patients with newly diagnosed glioblastoma. AJNR Am J Neuroradiol (2015) 36(9):1654–61. doi: 10.3174/ajnr.A4374
25. Hu LS, Baxter LC, Pinnaduwage DS, Paine TL, Karis JP, Feuerstein BG, et al. Optimized preload leakage-correction methods to improve the diagnostic accuracy of dynamic susceptibility-weighted contrast-enhanced perfusion MR imaging in posttreatment gliomas. AJNR Am J Neuroradiol (2010) 31(1):40–8. doi: 10.3174/ajnr.A1787
26. Schmainda KM, Prah MA, Hu LS, Quarles CC, Semmineh N, Rand SD, et al. Moving toward a consensus DSC-MRI protocol: Validation of a low–flip angle single-dose option as a reference standard for brain tumors. AJNR Am J Neuroradiol (2019) 40(4):626-633. doi: 10.3174/ajnr.A6015
27. Boxerman JL, Quarles CC, Hu LS, Erickson BJ, Gerstner ER, Smits M, et al. Consensus recommendations for a dynamic susceptibility contrast MRI protocol for use in high-grade gliomas. Neuro-Oncology (2020) 22(9):1262–75. doi: 10.1093/neuonc/noaa141
28. Welker K, Boxerman J, Kalnin A, Kaufmann T, Shiroishi M, Wintermark M, et al. ASFNR recommendations for clinical performance of MR dynamic susceptibility contrast perfusion imaging of the brain. AJNR Am J Neuroradiol (2015) 36(6):E41–51. doi: 10.3174/ajnr.A4341
29. Paulson ES, Schmainda KM. Comparison of dynamic susceptibility-weighted contrast-enhanced MR methods: Recommendations for measuring relative cerebral blood volume in brain tumors. Radiology (2008) 249(2):601–13. doi: 10.1148/radiol.2492071659
30. Stokes AM, Bergamino M, Alhilali L, Hu LS, Karis JP, Baxter LC, et al. Evaluation of single bolus, dual-echo dynamic susceptibility contrast MRI protocols in brain tumor patients. J Cereb Blood Flow Metab (2021) 41(12):3378–90. doi: 10.1177/0271678X211039597
31. Boxerman JL, Schmainda KM, Weisskoff RM. Relative cerebral blood volume maps corrected for contrast agent extravasation significantly correlate with glioma tumor grade, whereas uncorrected maps do not. AJNR Am J Neuroradiol (2006) 27(4):859–67.
32. Schmainda KM, Prah MA, Rand SD, Liu Y, Logan B, Muzi M, et al. Multisite concordance of DSC-MRI analysis for brain tumors: Results of a national cancer institute quantitative imaging network collaborative project. AJNR Am J Neuroradiol (2018) 39(6):1008–16. doi: 10.3174/ajnr.A5675
33. Semmineh NB, Bell LC, Stokes AM, Hu LS, Boxerman JL, Quarles CC. Optimization of acquisition and analysis methods for clinical dynamic susceptibility contrast MRI using a population-based digital reference object. AJNR Am J Neuroradiol (2018) 39(11):1981–8. doi: 10.3174/ajnr.A5827
34. Stokes AM, Semmineh NB, Nespodzany A, Bell LC, Quarles CC. Systematic assessment of multi-echo dynamic susceptibility contrast MRI using a digital reference object. Magn Reson Med (2020) 83(1):109–23. doi: 10.1002/mrm.27914
35. Quarles CC. Comparison of dual-echo DSC-MRI- and DCE-MRI-derived contrast agent kinetic parameters. Magn Reson Imaging (2012) 30:944–53. doi: 10.1016/j.mri.2012.03.008
Keywords: DSC MRI, glioma, pseudoprogression, perfusion imaging, fractional tumor burden, CBV
Citation: Anil A, Stokes AM, Chao R, Hu LS, Alhilali L, Karis JP, Bell LC and Quarles CC (2023) Identification of single-dose, dual-echo based CBV threshold for fractional tumor burden mapping in recurrent glioblastoma. Front. Oncol. 13:1046629. doi: 10.3389/fonc.2023.1046629
Received: 16 September 2022; Accepted: 03 January 2023;
Published: 17 January 2023.
Edited by:
Bo Gao, Affiliated Hospital of Guizhou Medical University, ChinaReviewed by:
Simona Gaudino, Agostino Gemelli University Polyclinic (IRCCS), ItalyMichael Iv, Stanford University, United States
Copyright © 2023 Anil, Stokes, Chao, Hu, Alhilali, Karis, Bell and Quarles. This is an open-access article distributed under the terms of the Creative Commons Attribution License (CC BY). The use, distribution or reproduction in other forums is permitted, provided the original author(s) and the copyright owner(s) are credited and that the original publication in this journal is cited, in accordance with accepted academic practice. No use, distribution or reproduction is permitted which does not comply with these terms.
*Correspondence: C. Chad Quarles, ccquarles@mdanderson.org