- 1Department of Urology, Xinhua Hospital, School of Medicine, Shanghai Jiaotong University, Shanghai, China
- 2Department of Urology, Third Affiliated Hospital of the Second Military Medical University, Shanghai, China
Ample evidence indicates that the development and progression of renal cell carcinoma (RCC) are complex pathological processes involving interactions between tumor cells, immune cells and stromal components. Tumor infiltrated immune cells determine whether tumor advancement is promoted or inhibited. Among them, infiltrated B lymphocytes are present in all stages of RCC, playing a major role in determining tumor formation and advancement, as an essential part in the tumor microenvironment (TME). Although the advent of targeted and immune therapies has remarkably improved the survival of patients with advanced RCC, few cases can achieve complete response due to drug resistance. In this review article, we intend to summary the recent studies that outline the interaction networks of B cells with other cells, discuss the role of B cells in RCC development and progression, and assess their impact on RCC immunotherapy.
Introduction
Renal cell carcinoma (RCC) is one of the most prevalent malignant tumors in the human urinary system, accounting for 2-3% of all tumors worldwide (1). Although considerable progress has been made in targeted therapies in improving the 5-year survival rate, the overall clinical outcomes remain unsatisfied due to postoperative recurrence, metastasis or chemotherapy resistance (2, 3). Recently, analysis of the tumor microenvironment (TME) has emerged as a potential approach of RCC treatment (4). Researchers have concentrated on tertiary lymphoid structures (TLS) and lymphoid aggregates in non-lymphoid tissues, where B cells as the principal components in surrounding T cell zones interact with other cells. TLS appear in various stages of maturity in different tumor types, culminating in germinal center (GC) formation (5). Tumor infiltrated B cells are mostly associated with TLS compared with other immune cells. Although B cells can also be recruited to the tumor bed directly rather via TLS, the density of B cells is relatively low under these circumstances. B cells and tumor-associated TLS can be found in the TME of most cancer types and are correlated with tumor immunotherapies (5).
The TME provides a complex tumor ecosystem composed of cancer cells and multiple non-cancerous cells, playing decisive roles in tumor initiation, progression and dissemination (6, 7). Cancer cells interact with stromal cells and immune cells, working together rather separately to form the principal structure of the TME. As one of the characteristics of cancer, prolonged inflammation initiates tumorigenesis or supports tumor progression, during which the entire immune landscape is altered drastically (8). To progress in the body, tumors have derived many mechanisms to escape immune surveillance by producing neoantigens to interfere with the immune system. Therapies targeting the TME have been studied and implemented from bench to bedside. It is described that the therapy of PD-1 and PD-L1 blockade has made therapeutic improvements about metastatic tumors in some reviews, but the objective response rates still remain unsatisfactory. Thus, it is worthwhile to investigate the multiple associations in TME to further explore B lymphocyte-targeted therapies of RCC.
In this review, we address questions regarding the interaction networks of B cells and other cell types by focusing on the association of infiltrated B cells with tumorigenesis, progression and response to immunotherapy of RCC. These cells are collaboratively engaged in aspects of the tumor process and immune TME. We will elucidate each part of B cell interaction that affects immune response in RCC from four specific sections, and review the advances of B cells and TLS with tumor immunotherapies (Figure 1).
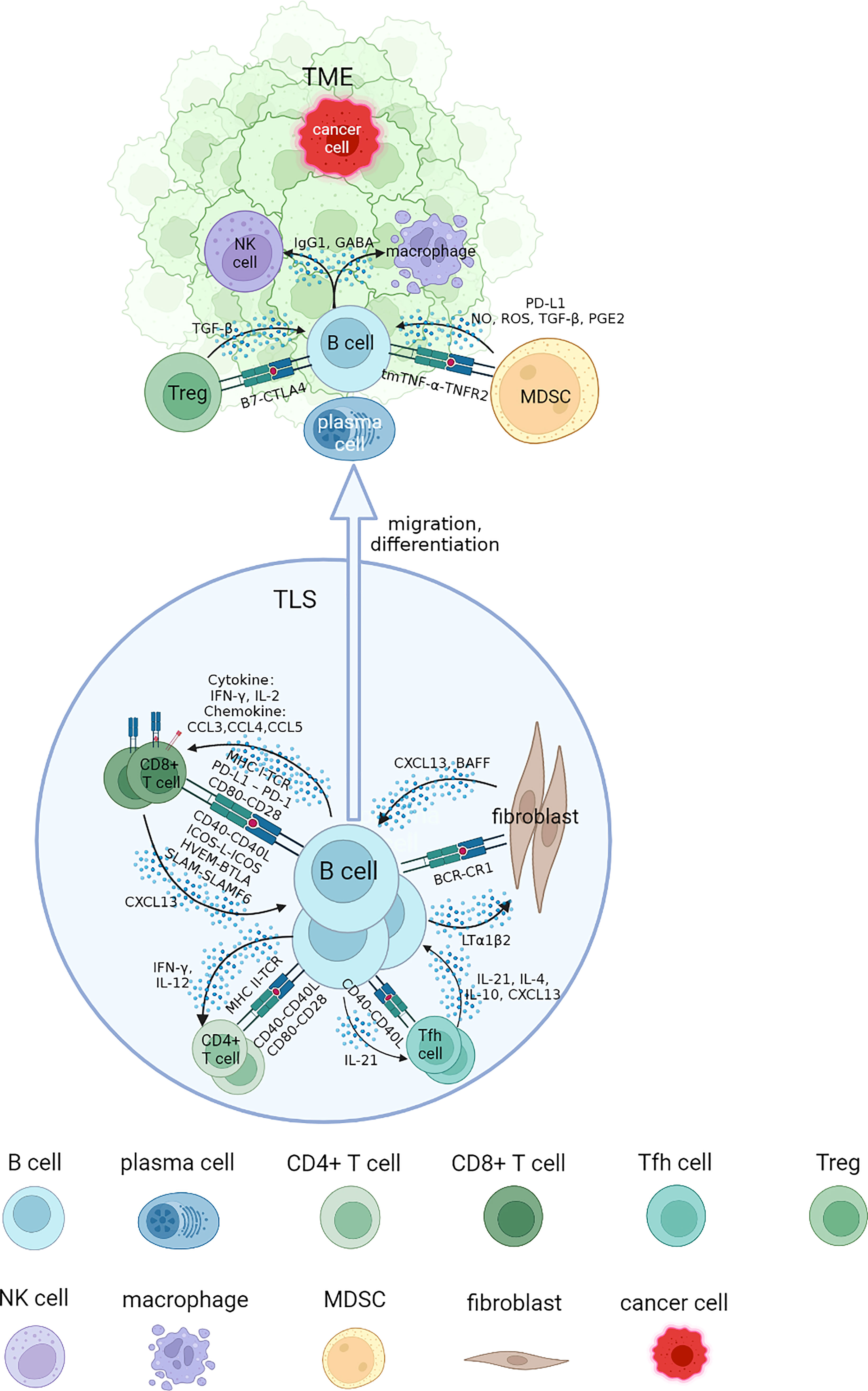
Figure 1 Interaction networks between B cells and other cells in tertiary lymphoid structure (TLS) and tumor microenvironment (TME). B cells cooperate with other cells to perform an immunomodulatory role on tumorigenesis and progression. B cells could be attracted to TLS by CXCL13, where they mature and interact with different types of T cells through specific co-stimulatory and co-inhibitory signal pairs such as CD40-CD40L, CD80-CD28. The class switch recombination and differentiation of B cells are promoted by cytokines including IL-4 and IL-10, and B cells receive IL-21 to develop into plasma cells and memory B cells, migrating to tumor bed. They cooperate with NK cells and macrophages to implement ADCC and ADCP process through antibodies including IgG1 in TME. Some inhibit regulatory effects of MDSCs and Tregs on B cells in TME could promote tumor progression. NK, natural killer; MDSC, myeloid-derived suppressor cell; NO, nitric oxide; ROS, reactive oxygen species; PGE2, prostaglandin E2.
The interaction of B cells with T cells
B lymphocytes first differentiate from hematopoietic stem cells in the bone marrow, experiencing continuous development into immature B cells, and migration to second lymphoid tissues, where they mature (9). The process in the bone marrow involves immunoglobulin light chain gene rearrangement, and VDJ gene segment recombination. Upon stimulation by the antigen, B cells experience antibody class switching and somatic hypermutation in the GC, which has proved to be the mechanism for affinity maturation of antibodies (10, 11). The initiation of GC reaction involves several distinct cell types via a coordinated cascade, guiding antigen-engaged B cells into GC reaction. These processes along with GC formation are well assisted by other immune cells, especially T cells. Additionally, B cells are one of the critical components of the humoral immune system, regulated by numerous control mechanisms at both cellular and molecular levels. The induction of antibody response also requires the collaboration of T and B cells.
The function that T cells provide assistance for B cells has been recognized for decades, resulting in the demonstration of thymus-derived helper cells (12–14). This two-lymphocyte lineage model indicates issues of synergistic function and their cooperation. As the close partner, their history has been reviewed in detail (15). For various types of cancers, the associated T and B networks have already generated interests in immune therapy in many recent studies, and research highlighted the emerging roles of B cells in tumor immunity and the focus on T cell response. These findings could guide a protocol and provide potential therapeutic strategies for RCC patients.
B cells with Tfh cells
T follicular helper (Tfh) cells are the crucial partner of infiltrated B cells, whose crosstalk within TLS in the TME has been verified to affect tumor immunotherapy (16). There exists a bidirectional interaction in the Tfh-B combination. B cells are essential for Tfh cell formation by mediating PI3K-dependent migration of CD4+T cells into follicles (17). In a murine model of lung adenocarcinoma, B cells were found to promote tumor-specific CD4+Tfh cell differentiation, which then produced IL-21 to enhance CD8+T cell responses to drive anti-tumor immunity (18). In turn, mature Tfh cells provide binding CD40 ligand (CD40L or CD154) and IL-21 for GC B cell differentiation into memory B cells and plasma cells (19–21). IL-21 also plays a pivotal role in Tfh cell formation, B cell growth and class switch recombination (CSR), and maintains the expression of Bcl-6 in GC B repertoires (20, 22, 23). These processes are modulated by other Tfh cell cytokines, including IL-4 and IL-10, which favor IgG and IgA production, respectively (17). In addition to offering help for T-dependent B cells, some specialized CXCL13-producing Tfh cells could guide CXCR5+ lymphocyte migration, promote local memory B cell differentiation, and behave as a potential surrogate marker for GC reaction and TLS formation (24–26). Studies suggested that anti-PD-1 therapy in cancer could strengthen B cell capacity by increasing circulating Tfh cells (27). An investigation of the clinical data of clear cell RCC (ccRCC) showed that immune-related prognostic gene signatures were differentially expressed in distinct lymphocyte clusters (28). In a study of metastatic ccRCC patients under ICB therapy, unswitched memory B cells correlated positively with Tfh cells, TLS and CD20+ B cells, associated with higher response rate and better overall survival (29). The related genes and the interaction mechanisms of B cells and Tfh cells may prove to be a biomarker for assessing prognosis of RCC and screening precise patients for specific immunotherapies.
B cells with CD8+T cells
Effects of B cells on CD8+T cells
Some evidence proves that B cells can exert a direct or indirect effect on CD8+T cells. B cells present tumor-specific antigens that they have captured by B-cell receptors (BCRs) to CD8+T cells (30), release cytokines, and participate in the formation of co-stimulatory and co-inhibitory receptors (31). Some cytokines such as IL-2 and IFN-γ secreted by B cells can bind to the receptors on CD8+T cells (31). The co-stimulatory and co-inhibitory signal pairs include CD80-CD28, CD40-CD40L, ICOS-L-ICOS; PD-L1-PD-1, HVEM-BTLA, SLAM-SLAMF6 (32). In addition, B cells can indirectly support CD8+T cells by interacting with Tfh cells through CD40-CD40L (32). In vitro, B cells were found differentiating into plasmablast-like cells and expressing T cell recruitment chemokines like CCL3, CCL4 and CCL5. Plasmablast-like cells increased the activation of PD-1+T cells via anti-PD-1 blockade, and their frequency could predict response and survival to immune checkpoint inhibitor (ICB) (33). In a metastatic RCC pre-surgical trial, B cell signatures were found enriched in tumors of responders of ICB treatment, which was also confirmed in another ICB-treated cohort of melanoma patients (34). Accordingly, we speculate that B cell subpopulations within the TLS could modulate T cell antitumor response and serve as a possible ICB response predictor of RCC.
Effects of CD8+T cells on B cells
Current reports suggest that T cells may appear first in the tumor sites and then promote the recruitment of B cells. CD8+T cells activated by TGF-β will upregulate CD103 and release CXCL13, a potent B cell chemoattractant that binds to CXCR5 receptor of B cells, and then mediate B cell recruitment and TLS formation (35). Researchers observed similar phenomenon that infiltrated B cells were prone to colocalize with CD8+T cells (36), and the significant correlations between them imply their cooperation in a tumor-killing effect of several malignancies (31). A study on ccRCC demonstrated that the abundance of intratumoral CD8+T cells secreting CXCL13 was associated with increased TLS and immunoevasive TME, functioning as a potential immunotherapeutic marker for ccRCC treatment (37). Another study illustrated the prognostic value of CCL4, CCL5, CCL8, CCL19 and CXCL13 expression in ccRCC. Besides, DNA deletion of TLS gene signatures could greatly indicate poor outcome in ccRCC patients compared with wild-type gene signature (38). All these results provide insights into how B cells cooperate with CD8+T cells and their roles in ICB treatment, which may assist the development of RCC therapeutic targets.
Roles of Tregs on B cells and plasma cells
Direct regulations
Regulatory T cells (Tregs) dampen B cell proliferation and plasma cell formation (39). Hyung et al. reported that Tregs could directly interact with B cells to suppress immunoglobin (Ig) response, production and CSR without the help of Tfh cells, and downregulate relative gene expressions of naïve B cells via contact-dependent mechanisms (40, 41). Interestingly, it was elucidated that Tregs reduced all other Ig production but induced B cells to produce IgG4 in a cell contact-, TGF-β- and IL-10-dependent manner (42, 43). Studies have demonstrated that Tregs are related to negative outcomes in RCC patients (44), and the expansion of Tregs could limit the function of IL-2 in RCC treatment (45).
Indirect regulations
Tregs downregulate the function of T helper (Th) cells through various mechanisms, resulting in adverse effects on B cells (46). Tregs could also suppress in vitro B cell Ig production by inhibiting Th function via TGF-β (47). In addition, Tregs migrate to follicles in the GC and regulate GC B cell responses (48). A population of follicular regulatory T (Tfr) cells is also described, which share phenotypes of both Tregs and Tfh recruited during GC reaction, yet are distinct from both (49, 50). Tfr cells could balance the Tfh-mediated B cell responses through CTLA-4 expression (17), and it has been proved that a lack of Tfr expressing CXCR5 and Bcl-6 could lead to greater GC reaction, associated with GC B cells, affinity maturation of antibodies and plasma cells differentiation (51). Consequently, the regulation of B cells in the GC reaction is well counterbalanced by Tfh and Tfr cells. A recently published study indicated another population of Foxp3+T cells which existed in the end-stage of GC, displaying an immediate phenotype of Tfh and Tfr cells (52). Collectively, these suggest the indirect effects of Tregs on B and plasma cells, mainly through Tfh and Tfr cells in the GC.
B cells cooperate with NK and macrophages
ADCC and ADCP
The antitumor activity of NK cells depends on their antibody-dependent cell-mediated cytotoxicity (ADCC) when they encounter tumor-specific IgG1 antibodies secreted by plasma cells (30, 53). Unfortunately, some tumor-derived IgG may impede the ADCC process by binding with specific antigens lacking complement activation. To against high PD-L1-expressing tumors, NK cells combining with anti-PD-L1 antibodies helps promote ADCC activity (54). However, NK cells are generally scarce and become anergic in the TME (55). Tumor-associated macrophages (TAM) participate in the ADCC or phagocytosis process via antibody-dependent cell-mediated phagocytosis (ADCP). Intriguingly, Su et al. reported an unexpected finding that ADCP macrophages may inhibit ADCC and T cell-mediated cytotoxicity by upregulating immune checkpoint such as PD-L1 and indoleamine 2,3-dioxygenase to cause immunosuppression (56). Therefore, a combination of therapeutic antibodies and ICB potentially provide synergistic effects in RCC treatment.
B cells with macrophages
Fc gamma receptor (FcRγ), a receptor binding to Fc region of an antibody, could modulate protumor bioactivities of macrophages. In the absence of B cells or FcRγ, macrophages were found reprogramming towards the M1-type inflammatory state (57). Specifically, B cells could foster tumor development through FcRγ by activating pro-angiogenesis and tissue remodeling of myeloid cells, especially macrophages and mast cells (57). Besides, the reprogramming of macrophages also regulates CD8+T cell recruitment. Researchers discovered that by using B cell-depleting αCD20 monoclonal antibody as an anticancer monotherapy in mice, the chemokine expression of macrophages altered, thus improving CD8+lymphocyte infiltration and chemotherapy response in squamous cell carcinoma (58). A study of pancreatic ductal adenocarcinoma showed that Bregs could induce polarization of M2 macrophages and aggregation of Tregs, thus resulting in T cell suppression (59). Likewise, in ccRCC cohorts, researchers observed that compared with early stage tumors, pro-inflammatory macrophages were reduced, while suppressive M2-like macrophages were elevated in advanced disease (60). In conclusion, immunosuppressive B cells and plasma cells tend to facilitate TAM conversion to protumoral M2 phenotype, while effector B cells could promote TAM conversion to tumoricidal M1 phenotype (30). In the study of ccRCC, researchers found that TAM-derived chemokine CCL5 displayed a correlation with increased B cells and CD8+T cells, and decreased CD4+T cells. Elevated CCL5+TAMs infiltration exhibited higher tumor-infiltrated lymphocytes, but reduced TLS, correlated with a distant prognosis of ccRCC patients (61).
Although interactions of lymphocytes are regulated with various cell-bound proteins, small metabolites, as essential intermediates in biochemical processes, could reflect neighboring cells when released into extracellular milieu (62, 63). Metabolite and neurotransmitter GABA, synthesized and secreted by activated B cells and plasma cells, could promote monocyte differentiation into macrophages, polarizing towards an anti-inflammatory phenotype. They function as protumor cells through releasing interleukin (IL)-10 and limiting CD8+T cell function (64). Furthermore, in RCC microenvironment where B cells and IgA+ plasma cells were highly infiltrated, GABA was almost exclusively detected. It may suggest that GABA could regulate T cells and monocytes in the TME of RCC (64). Further studies are needed to unravel the occasions of intracellular metabolites mediate interactions between cells to inhibit tumor growth or enhance B cell immunity to cancer.
The interaction between fibroblasts, dendritic cells and B cells
Fibroblast reticular cells (FRCs) are considered immunologically specialized myofibroblasts originating from mesenchymal stem cells (65). Inside the encapsulated sponge-like network of FRCs in lymph node congregated B cells, T cells, plasma cells, dendritic cells (DCs) and macrophages. FRCs in B cell zone provide B cell growth factor—B cell-activating factor (BAFF) and transcribe B cell chemoattractant CXCL13 to maintain and attract B cells in support of B cell survival (66). During infection, FRCs rapidly upregulate the CXCL13 expression via crosstalk with B cells, and control the expansion of B cell follicle boundaries upon inflammation (67).
Of note, DCs are specialized non-hematopoietic stromal cells residing in lymphoid follicles and GC. They participate in optimal selection of B cells that secret antibodies (68). In addition, B cells populate in the network of follicular dendritic cells (FDCs). Mature TLS comprise a GC with CD23+FDCs, which could present antigens to B cell selection with high-affinity BCRs, and promote B cell activation and differentiation (69). FDCs present naïve antigens to GC B cells via complement receptors 1 (CR1) (70). In cohorts of prospective and retrospective lung cancers, a high density of mature DC is strongly linked with a substantial infiltration of T cells with effector-memory characteristics, T-cell activation gene expression and T-helper 1 phenotype (71). These results indicate that mature DCs may generate some specific immune contexture that influences infiltrated B cells and TLS in TME.
Researchers have demonstrated that fibroblasts could directly support TLS formation and development (72). In the settings of inflammation and persistent antigen presentation, TLS-associated fibroblasts differentiate from locally activated mesenchyme (73). Likewise, stromal cell priming and lymphocytes accumulation have close relationships and the former might occur before lymphocyte migration. This kind of cancer-associated fibroblasts (CAFs) play a pivotal role as lymphoid tissue organizer cells (LTo) and coordinate with multiple cell types that synergistically act as lymphoid tissue inducer cells (LTi) cells, such as Intratumoral CD8+T cells and B cells that drive TLS development. CAFs work as surrogate LTo and participate in TLS formation and orchestration. On the other hand, the reticular network of CAF is mediated by CD8+T cells, and its accumulation relies on the recruitment of B cells expressing lymphotoxin, namely intratumoral LTα1β2+B cells. Imaging analysis has confirmed an elevated density of B cells coexisting with a reticular network of LTo-like CAF (74). Analysis of TCGA data of RCC showed that CAF infiltration was higher in RCC, especially in RCC with advanced tumor pathological grades and stages, than that in normal tissues (75). In addition, studies of RCC have revealed that TLS are sites of in situ B cell maturation into plasma cells. The plasma cells formed in the TLS are embedded in the dense network of fibroblasts, and disseminate into the tumor beds along fibroblastic tracks (76).
MDSC-mediated regulation of B cell response
Myeloid-derived suppressor cells (MDSCs) were first termed in 2007, representing a series of immunosuppressive macrophages, DCs and granulocyte precursor cells produced in response to tumor-derived cytokines (77). Since then, MDSCs have been considered a great obstacle for cancer immunotherapies because they have close relationships with other immune cells. Studies have identified that normal B cells could be transformed into a subtype of immune regulatory B cells (Bregs) inhibiting T cell response in the presence of MDSCs. Besides, some immune checkpoint molecules including PD-1 and PD-L1 might be changed and remolded predominantly. A novel MDSC-Induced B cell subset (PD-1-PD-L1+CD19+) has been demonstrated to inhibit T cell responses (78). Specifically, activation of the PI3K/AKT/NF-kB axis enhances the PD-1-PD-L1+ Breg protumor function (79). Another study on glioblastoma discovered that MDSCs delivered functional membrane-bound PD-L1 via microvesicles to Bregs, conferring the effector phenotype and function (80). Cell experiments have elucidated that 1) MDSCs suppress B cell proliferation by releasing suppressive mediators; 2) MDSCs induce decreased expression of B cell surface markers including IgM, HLA-DR, CD80 and CD86; 3) MDSCs induce specific B cell subset phenotypic alterations including antibody-secreting cells death; and 4) MDSCs elicit specific gene transcriptional changes which are associated with apoptosis, class-switch regulation and B cell differentiation and effector function (81).
In addition, some indirect regulatory effects of MDSCs on B cells have also been elaborated. MDSCs increase the number of FoxP3+ Treg cells, and facilitate the development of Tregs (82, 83). Tregs, along with Bregs, have similar suppressive characteristics and close relationships with B cells. FoxP3+Treg cells inhibit antibody production, activation and differentiation of B cells. Besides, it is reported that MDSCs modulate B cells via different pathways, including TNF, STAT3 and TGF-signaling, and MDSC-derived nitric oxide (NO), reactive oxygen species (ROS), TGF-β, and prostaglandin E2 (PGE2) play roles in suppressing B cells (84). RCC is regarded as an immunogenic tumor, which elicits the influx of immune-inhibitory cells such as Tregs and MDSCs into the TME, resulting in immune dysfunction (85). Many possible mechanisms have been proposed to explain how MDSCs target immune suppression (86), and it has resulted in clinical response in some patients with RCC (85, 87). The regulation of B cells by MDSCs may be a prospective target for immunotherapy in RCC.
Indications of TLS and immunotherapy
TLS have been identified in various types of tumors at every stage of disease, but their presence is in high heterogeneity between different cancer types and patients (88, 89). It is thought that TLS actively modulate antitumor immune activities rather than simply being a surrogate marker of rapid immune response (34). Mature TLS show indications of GC development. In colorectal cancer, TLS are associated with favorable outcomes and harbor prognostic information of disease recurrence (90). In lung squamous cell carcinoma, TLS are the independent prognostic marker and their development can be affected by chemotherapy and steroids (91). Interestingly, between different types of genitourinary cancer, one study showed that TLS displayed a distinct status in terms of the clinical outcome and immunogenomic profile (92). Researchers showed that the TLS detected in RCC cohort were less mature, contributing to poor outcomes, while in bladder cancer cohort, TLS were more mature with GC structures and associated with better outcomes (92). Another study certificated that in three gradual levels of immune infiltration of ccRCC clusters, higher abundance of T cells and TLS, suggesting an immune-enriched TME, was related to poor clinical outcome (93). These results suggest the heterogeneity of TLS and indicate that comparison of the TLS characteristics may help show differences in the immune TME and prognostic effects in RCC.
B cells and immunotherapy
Recent studies have contributed to an appreciation for B cells influencing immunotherapy outcome (30). Researchers have already reached consensus on the surface phenotype markers of various B subtypes except Bregs (94), and single-cell RNA sequencing (scRNA-seq) technique provides a broader perspective on cell markers and characteristics. scRNA-seq and cell-cell communication analysis in several recent studies have demonstrated that interactions of infiltrated B cells could influence tumor clinical outcomes (Table 1). The heterogeneity across B-cell subpopulations has been studied by single-cell techniques. Some single cell methods have helped dissect tumor heterogeneity and study the anti-tumor drug responses. However, the specific B-cell gene signatures between different cancer types still need more investigations. When initiating an antitumor response, tumor-infiltrated B cells (TIL-Bs) are first required to be recognized by tumor-specific neoantigens via BCRs. Studies have suggested that complementarity determining region-3 (CDR3), highly changeable regions in the BCR, have the potential to be prognostic biomarkers of different malignancies (104–106). Furthermore, the diversity of intratumoral BCR repertoires could reflect clonal expansion in response to tumor-associated antigens. Compared to scRNA-seq, repertoire studies may better characterize TIL-Bs including the investigation of B-cell phenotypes and BCR diversity within the RCC microenvironment (107). Some distinct RCC-associated gene mutations displayed by genomic techniques also have correlations with BCR repertories. Researchers found that among RCC patients with mutations in KDM5C, PBRM1, VHL and PTEN, BCR repertoire diversity was decreased (108). Intriguingly, PBRM1 mutation is pertinent with immune checkpoint inhibiters (ICI) response of RCC (109, 110). Some gene segments may be enriched in TIL-Bs with particular gene expression phenotypes. Besides, some BCR pathway molecules are upregulated and BCR-related kinases play a role in the TME of various tumors, suggesting an anticancer activity of targeting BCR-immune complex and BCR-related kinases (106, 111). Overall, this may provide a new insight of exploring B cell subpopulations most affected by molecular features of tumor and contribute to new targets of immunotherapy with the combination of scRNA-seq and BCR repertories.
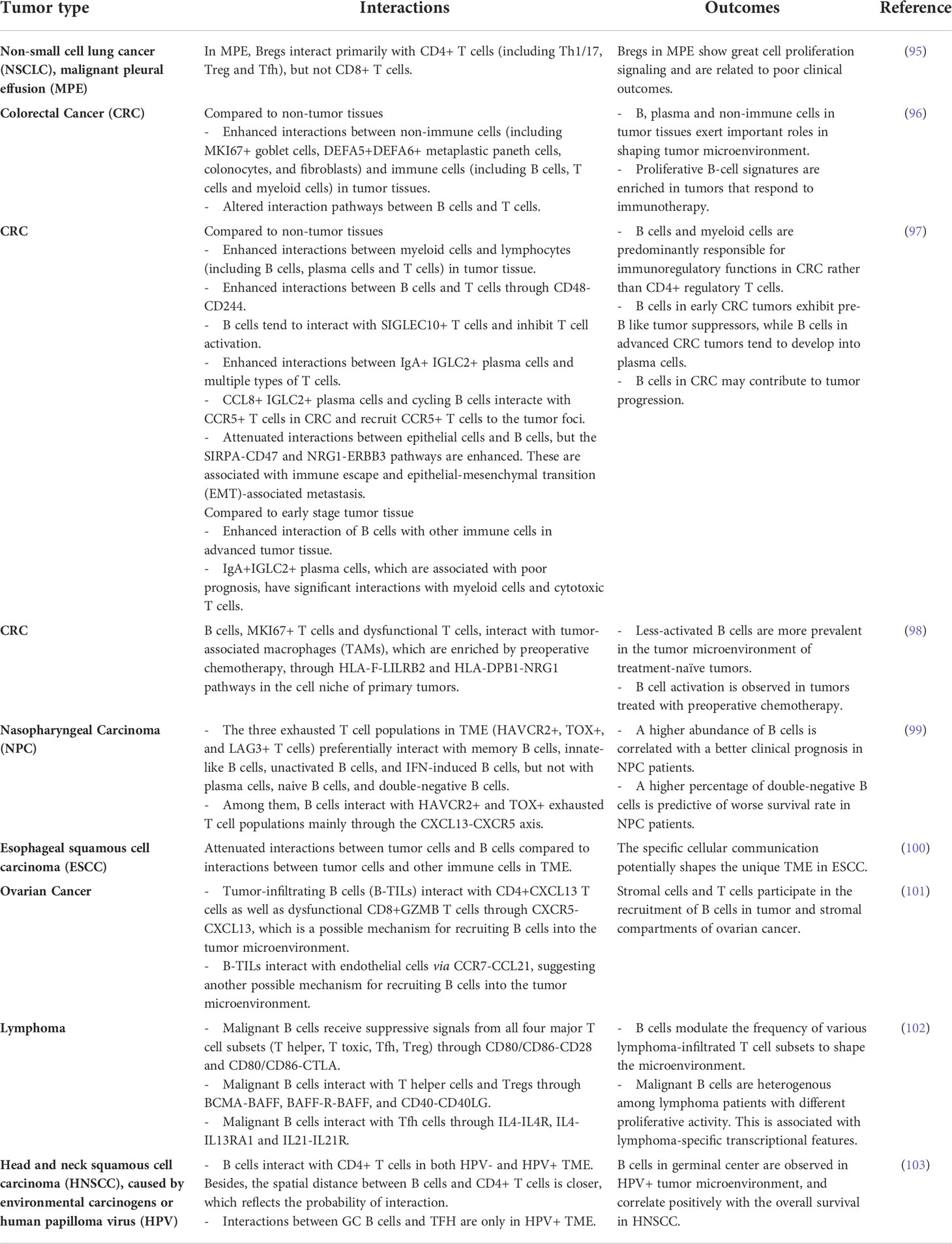
Table 1 Current research about the interactions of B cells influencing tumor clinical outcomes, using scRNA-seq or cell-cell communication analysis.
Antibodies produced by B cells have associations with effective antitumor immune response. Researchers demonstrated a high level of plasmablasts in the blood of metastatic RCC patients who had not exhibited tumor progression for over a year, and reactive antibodies from B cell response are commonly detected, which exhibit a great level of somatic hypermutation (112). A study indicated that TIL-Bs had unique antibody repertoire characteristics, including elevated clonal polarization and high somatic hypermutation rates in treated-tumor-bearing mice (113). The signs of B cell activation and clonal expansion were similarly discovered in other human malignancies (111, 114). These results suggest a possible persistent B cell response targeting tumor antigens. The identification of antibody repertoire signatures could perform as markers to identify tumor-reactive B cells, and provide a new paradigm for discovering antitumor antibodies with RCC diagnosis and immunotherapy.
Can B cells and TLS be predictive and prognostic factors in RCC?
We have reviewed the crosstalk between B cells and other cells in the TME, highly expecting that indications of B cells and TLS with immunotherapy could be the predictive and prognostic factor in RCC. Some current studies and clinical trials have confirmed the value for different B subsets in RCC. A clinical study on the plasma sample of RCC patients demonstrated that the blood concentration of unswitched memory B cells was correlated with the response condition to immune checkpoint blockade and survival rate in metastatic ccRCC patients (29). Similarly, researchers found that a subset of B cells with a memory phenotype was associated with positive outcomes in RCC patients treated with immune checkpoint inhibitors, and could predict response to checkpoint immunotherapy (115). Meylan et al. (76) used spatial transcriptomics to investigate B cell immunity within intratumoral TLS in RCC, and proved that TLS sustained B cell maturation and antibody generation, with response to immunotherapy possibly via direct antitumor effects (76). It has been proved that RCC exhibits distinct immune phenotypes and proteogenomic characteristics (116). Growing evidence indicates the diversity and heterogeneity of TME and tumor cells affect immunotherapy (117). Although no large comparative study has been reported to explore the specific effects and mechanisms of B cells on RCC patients in clinical trials, the clinically related outcomes of B cells and TLS with RCC occurred in previous studies. Therefore, further investigations are needed to confirm the predictive and prognostic value of B cells and TLS in RCC.
Conclusion
Increasing evidence has demonstrated the important role of B cells in tumor immunotherapy, and some innovative techniques including scRNA-seq and BCR repertoires have provided intensive insights into B cells and TME. In this review, we focus on the interaction networks of B cells with other cells in RCC microenvironment. Some subtypes of T cells including CD8+T cells and Tfh cells contribute to the recruitment, maturation and differentiation of B cells. Besides, B cells also act as a provider of antigen presenting cells and release cytokines to help T cells perform their duties in tumor sites. On the contrary, subsets of Tregs and MDSCs suppress the performance of B cells. In addition, NK cells and macrophages perform ADCC and ADCP function through using antibodies, and fibroblasts perform an essential role in the maturation of B cells in TLS. We speculate that the study of the interaction networks of immune cells can provide valuable information for RCC treatment, and how to improve the response rate to immunotherapy of RCC is an important issue that needs to be considered seriously.
Author contributions
All authors had full access to all the data in the study and take responsibility for the integrity of the literature. All authors were involved in critical revision of the manuscript for important intellectual content. All authors contributed to the article and approved the submitted version.
Funding
This work was sponsored by the National Natural Science Foundation of China (No. 81974391, 82072806, 82173265); the Clinical Research Plan of SHDC (SHDC2020CR4025); Shanghai “Rising Stars of Medical Talent” Youth Development Program: Youth Medical Talents - Specialist Program (X. Pan); Shanghai Municipal Commission of Health and Family Planning (20204Y0042); the Hospital Funded Clinical Research of Xinhua Hospital Affiliated to Shanghai Jiaotong University School of Medicine (21XHDB06).
Conflict of interest
The authors declare that the research was conducted in the absence of any commercial or financial relationships that could be construed as a potential conflict of interest.
Publisher’s note
All claims expressed in this article are solely those of the authors and do not necessarily represent those of their affiliated organizations, or those of the publisher, the editors and the reviewers. Any product that may be evaluated in this article, or claim that may be made by its manufacturer, is not guaranteed or endorsed by the publisher.
References
1. Conejo-Garcia JR, Biswas S, Chaurio R. Humoral immune responses: Unsung heroes of the war on cancer. Semin Immunol (2020) 49:101419. doi: 10.1016/j.smim.2020.101419
2. Kamli H, Li L, Gobe GC. Limitations to the therapeutic potential of tyrosine kinase inhibitors and alternative therapies for kidney cancer. Ochsner J (2019) 19:138–51. doi: 10.31486/toj.18.0015
3. Linehan WM. Genetic basis of kidney cancer: role of genomics for the development of disease-based therapeutics. Genome Res (2012) 22:2089–100. doi: 10.1101/gr.131110.111
4. Vuong L, Kotecha RR, Voss MH, Hakimi AA. Tumor microenvironment dynamics in clear-cell renal cell carcinoma. Cancer Discov (2019) 9:1349–57. doi: 10.1158/2159-8290.CD-19-0499
5. Calderaro J, Petitprez F, Becht E, Laurent A, Hirsch TZ, Rousseau B, et al. Intra-tumoral tertiary lymphoid structures are associated with a low risk of early recurrence of hepatocellular carcinoma. J Hepatol (2019) 70:58–65. doi: 10.1016/j.jhep.2018.09.003
6. Pitt JM, Marabelle A, Eggermont A, Soria J-C, Kroemer G, Zitvogel L. Targeting the tumor microenvironment: removing obstruction to anticancer immune responses and immunotherapy. Ann Oncol Off J Eur Soc Med Oncol (2016) 27:1482–92. doi: 10.1093/annonc/mdw168
7. Chen S, Zhu G, Yang Y, Wang F, Xiao Y-T, Zhang N, et al. Single-cell analysis reveals transcriptomic remodellings in distinct cell types that contribute to human prostate cancer progression. Nat Cell Biol (2021) 23:87–98. doi: 10.1038/s41556-020-00613-6
8. Hiam-Galvez KJ, Allen BM, Spitzer MH. Systemic immunity in cancer. Nat Rev Cancer (2021) 21:345–59. doi: 10.1038/s41568-021-00347-z
9. Melchers F. Checkpoints that control b cell development. J Clin Invest (2015) 125:2203–10. doi: 10.1172/JCI78083
10. Jacob J, Kelsoe G, Rajewsky K, Weiss U. Intraclonal generation of antibody mutants in germinal centres. Nature (1991) 354:389–92. doi: 10.1038/354389a0
12. Miller JF, Mitchell GF. Cell to cell interaction in the immune response. i. hemolysin-forming cells in neonatally thymectomized mice reconstituted with thymus or thoracic duct lymphocytes. J Exp Med (1968) 128:801–20. doi: 10.1084/jem.128.4.801
13. Mitchell GF, Miller JF. Cell to cell interaction in the immune response. II. the source of hemolysin-forming cells in irradiated mice given bone marrow and thymus or thoracic duct lymphocytes. J Exp Med (1968) 128:821–37. doi: 10.1084/jem.128.4.821
14. Nossal GJ, Cunningham A, Mitchell GF, Miller JF. Cell to cell interaction in the immune response. 3. chromosomal marker analysis of single antibody-forming cells in reconstituted, irradiated, or thymectomized mice. J Exp Med (1968) 128:839–53. doi: 10.1084/jem.128.4.839
15. Crotty S. A brief history of T cell help to b cells. Nat Rev Immunol (2015) 15:185–9. doi: 10.1038/nri3803
16. Garaud S, Dieu-Nosjean MC, Willard-Gallo K. T follicular helper and B cell crosstalk in tertiary lymphoid structures and cancer immunotherapy. Nat Commun (2022) 13(1):2259. doi: 10.1038/s41467-022-29753-z
17. Tangye SG, Brink R, Goodnow CC, Phan TG. SnapShot: Interactions between b cells and T cells. Cell (2015) 162:926–926.e1. doi: 10.1016/j.cell.2015.07.055
18. Cui C, Wang J, Fagerberg E, Chen P-M, Connolly KA, Damo M, et al. Neoantigen-driven b cell and CD4 T follicular helper cell collaboration promotes anti-tumor CD8 T cell responses. Cell (2021) 184:6101–6118.e13. doi: 10.1016/j.cell.2021.11.007
19. Cyster JG, Allen CDC. B cell responses: Cell interaction dynamics and decisions. Cell (2019) 177:524–40. doi: 10.1016/j.cell.2019.03.016
20. Elgueta R, Benson MJ, de Vries VC, Wasiuk A, Guo Y, Noelle RJ. Molecular mechanism and function of CD40/CD40L engagement in the immune system. Immunol Rev (2009) 229:152–72. doi: 10.1111/j.1600-065X.2009.00782.x
21. Kurosaki T, Kometani K, Ise W. Memory b cells. Nat Rev Immunol (2015) 15:149–59. doi: 10.1038/nri3802
22. Linterman MA, Beaton L, Yu D, Ramiscal RR, Srivastava M, Hogan JJ, et al. IL-21 acts directly on b cells to regulate bcl-6 expression and germinal center responses. J Exp Med (2010) 207:353–63. doi: 10.1084/jem.20091738
23. Zotos D, Coquet JM, Zhang Y, Light A, D’Costa K, Kallies A, et al. IL-21 regulates germinal center b cell differentiation and proliferation through a b cell-intrinsic mechanism. J Exp Med (2010) 207:365–78. doi: 10.1084/jem.20091777
24. Gu-Trantien C, Migliori E, Buisseret L, de Wind A, Brohée S, Garaud S, et al. CXCL13-producing TFH cells link immune suppression and adaptive memory in human breast cancer. JCI Insight (2017) 2:91487. doi: 10.1172/jci.insight.91487
25. Hollern DP, Xu N, Thennavan A, Glodowski C, Garcia-Recio S, Mott KR, et al. B cells and T follicular helper cells mediate response to checkpoint inhibitors in high mutation burden mouse models of breast cancer. Cell (2019) 179:1191–1206.e21. doi: 10.1016/j.cell.2019.10.028
26. Thornhill JP, Fidler S, Klenerman P, Frater J, Phetsouphanh C. The role of CD4+ T follicular helper cells in HIV infection: From the germinal center to the periphery. Front Immunol (2017) 8:46. doi: 10.3389/fimmu.2017.00046
27. Sánchez-Alonso S, Setti-Jerez G, Arroyo M, Hernández T, Martos MI, Sánchez-Torres JM, et al. A new role for circulating T follicular helper cells in humoral response to anti-PD-1 therapy. J Immunother Cancer (2020) 8:e001187. doi: 10.1136/jitc-2020-001187
28. Wang Q, Tang H, Luo X, Chen J, Zhang X, Li X, et al. Immune-associated gene signatures serve as a promising biomarker of immunotherapeutic prognosis for renal clear cell carcinoma. Front Immunol (2022) 13:890150. doi: 10.3389/fimmu.2022.890150
29. Carril-Ajuria L, Desnoyer A, Meylan M, Dalban C, Naigeon M, Cassard L, et al. Baseline circulating unswitched memory b cells and b-cell related soluble factors are associated with overall survival in patients with clear cell renal cell carcinoma treated with nivolumab within the NIVOREN GETUG-AFU 26 study. J Immunother Cancer (2022) 10:e004885. doi: 10.1136/jitc-2022-004885
30. Sharonov GV, Serebrovskaya EO, Yuzhakova DV, Britanova OV, Chudakov DM. B cells, plasma cells and antibody repertoires in the tumour microenvironment. Nat Rev Immunol (2020) 20:294–307. doi: 10.1038/s41577-019-0257-x
31. Shi J-Y, Gao Q, Wang Z-C, Zhou J, Wang X-Y, Min Z-H, et al. Margin-infiltrating CD20(+) b cells display an atypical memory phenotype and correlate with favorable prognosis in hepatocellular carcinoma. Clin Cancer Res Off J Am Assoc Cancer Res (2013) 19:5994–6005. doi: 10.1158/1078-0432.CCR-12-3497
32. Trüb M, Zippelius A. Tertiary lymphoid structures as a predictive biomarker of response to cancer immunotherapies. Front Immunol (2021) 12:674565. doi: 10.3389/fimmu.2021.674565
33. Griss J, Bauer W, Wagner C, Simon M, Chen M, Grabmeier-Pfistershammer K, et al. B cells sustain inflammation and predict response to immune checkpoint blockade in human melanoma. Nat Commun (2019) 10:4186. doi: 10.1038/s41467-019-12160-2
34. Helmink BA, Reddy SM, Gao J, Zhang S, Basar R, Thakur R, et al. B cells and tertiary lymphoid structures promote immunotherapy response. Nature (2020) 577:549–55. doi: 10.1038/s41586-019-1922-8
35. Workel HH, Lubbers JM, Arnold R, Prins TM, van der Vlies P, de Lange K, et al. A transcriptionally distinct CXCL13+CD103+CD8+ T-cell population is associated with b-cell recruitment and neoantigen load in human cancer. Cancer Immunol Res (2019) 7:784–96. doi: 10.1158/2326-6066.CIR-18-0517
36. Nielsen JS, Sahota RA, Milne K, Kost SE, Nesslinger NJ, Watson PH, et al. CD20+ tumor-infiltrating lymphocytes have an atypical CD27- memory phenotype and together with CD8+ T cells promote favorable prognosis in ovarian cancer. Clin Cancer Res Off J Am Assoc Cancer Res (2012) 18:3281–92. doi: 10.1158/1078-0432.CCR-12-0234
37. Dai S, Zeng H, Liu Z, Jin K, Jiang W, Wang Z, et al. Intratumoral CXCL13+CD8+T cell infiltration determines poor clinical outcomes and immunoevasive contexture in patients with clear cell renal cell carcinoma. J Immunother Cancer (2021) 9:e001823. doi: 10.1136/jitc-2020-001823
38. Xu W, Ma C, Liu W, Anwaier A, Tian X, Shi G, et al. Prognostic value, DNA variation and immunologic features of a tertiary lymphoid structure-related chemokine signature in clear cell renal cell carcinoma. Cancer Immunol Immunother CII (2022) 71:1923–35. doi: 10.1007/s00262-021-03123-y
39. Adjobimey T, Satoguina J, Oldenburg J, Hoerauf A, Layland LE. Co-Activation through TLR4 and TLR9 but not TLR2 skews treg-mediated modulation of igs and induces IL-17 secretion in treg: B cell co-cultures. Innate Immun (2014) 20:12–23. doi: 10.1177/1753425913479414
40. Lim HW, Hillsamer P, Banham AH, Kim CH. Cutting edge: direct suppression of b cells by CD4+ CD25+ regulatory T cells. J Immunol Baltim Md 1950 (2005) 175:4180–3. doi: 10.4049/jimmunol.175.7.4180
41. Weingartner E, Golding A. Direct control of b cells by tregs: An opportunity for long-term modulation of the humoral response. Cell Immunol (2017) 318:8–16. doi: 10.1016/j.cellimm.2017.05.007
42. Satoguina JS, Adjobimey T, Arndts K, Hoch J, Oldenburg J, Layland LE, et al. Tr1 and naturally occurring regulatory T cells induce IgG4 in b cells through GITR/GITR-l interaction, IL-10 and TGF-beta. Eur J Immunol (2008) 38:3101–13. doi: 10.1002/eji.200838193
43. Akdis CA, Akdis M. Mechanisms and treatment of allergic disease in the big picture of regulatory T cells. J Allergy Clin Immunol (2009) 123:735–46. doi: 10.1016/j.jaci.2009.02.030
44. Şenbabaoğlu Y, Gejman RS, Winer AG, Liu M, Van Allen EM, de Velasco G, et al. Tumor immune microenvironment characterization in clear cell renal cell carcinoma identifies prognostic and immunotherapeutically relevant messenger RNA signatures. Genome Biol (2016) 17:231. doi: 10.1186/s13059-016-1092-z
45. Sharma M, Khong H, Fa’ak F, Bentebibel S-E, Janssen LME, Chesson BC, et al. Bempegaldesleukin selectively depletes intratumoral tregs and potentiates T cell-mediated cancer therapy. Nat Commun (2020) 11:661. doi: 10.1038/s41467-020-14471-1
46. Wing JB, Sakaguchi S. Foxp3+ t(reg) cells in humoral immunity. Int Immunol (2014) 26:61–9. doi: 10.1093/intimm/dxt060
47. Eddahri F, Oldenhove G, Denanglaire S, Urbain J, Leo O, Andris F. CD4+ CD25+ regulatory T cells control the magnitude of T-dependent humoral immune responses to exogenous antigens. Eur J Immunol (2006) 36:855–63. doi: 10.1002/eji.200535500
48. Lim HW, Hillsamer P, Kim CH. Regulatory T cells can migrate to follicles upon T cell activation and suppress GC-Th cells and GC-Th cell-driven b cell responses. J Clin Invest (2004) 114:1640–9. doi: 10.1172/JCI22325
49. Linterman MA, Pierson W, Lee SK, Kallies A, Kawamoto S, Rayner TF, et al. Foxp3+ follicular regulatory T cells control the germinal center response. Nat Med (2011) 17:975–82. doi: 10.1038/nm.2425
50. Wollenberg I, Agua-Doce A, Hernández A, Almeida C, Oliveira VG, Faro J, et al. Regulation of the germinal center reaction by Foxp3+ follicular regulatory T cells. J Immunol Baltim Md 1950 (2011) 187:4553–60. doi: 10.4049/jimmunol.1101328
51. Chung Y, Tanaka S, Chu F, Nurieva RI, Martinez GJ, Rawal S, et al. Follicular regulatory T cells expressing Foxp3 and bcl-6 suppress germinal center reactions. Nat Med (2011) 17:983–8. doi: 10.1038/nm.2426
52. Jacobsen JT, Hu W R, Castro TB, Solem S, Galante A, Lin Z, et al. Expression of Foxp3 by T follicular helper cells in end-stage germinal centers. Science (2021) 373:eabe5146. doi: 10.1126/science.abe5146
53. Park J-E, Kim S-E, Keam B, Park H-R, Kim S, Kim M, et al. Anti-tumor effects of NK cells and anti-PD-L1 antibody with antibody-dependent cellular cytotoxicity in PD-L1-positive cancer cell lines. J Immunother Cancer (2020) 8:e000873. doi: 10.1136/jitc-2020-000873
54. Kdimati S, Mullins CS, Linnebacher M. Cancer-Cell-Derived IgG and its potential role in tumor development. Int J Mol Sci (2021) 22:11597. doi: 10.3390/ijms222111597
55. Platonova S, Cherfils-Vicini J, Damotte D, Crozet L, Vieillard V, Validire P, et al. Profound coordinated alterations of intratumoral NK cell phenotype and function in lung carcinoma. Cancer Res (2011) 71:5412–22. doi: 10.1158/0008-5472.CAN-10-4179
56. Su S, Zhao J, Xing Y, Zhang X, Liu J, Ouyang Q, et al. Immune checkpoint inhibition overcomes ADCP-induced immunosuppression by macrophages. Cell (2018) 175:442–457.e23. doi: 10.1016/j.cell.2018.09.007
57. Andreu P, Johansson M, Affara NI, Pucci F, Tan T, Junankar S, et al. FcRgamma activation regulates inflammation-associated squamous carcinogenesis. Cancer Cell (2010) 17:121–34. doi: 10.1016/j.ccr.2009.12.019
58. Affara NI, Ruffell B, Medler TR, Gunderson AJ, Johansson M, Bornstein S, et al. B cells regulate macrophage phenotype and response to chemotherapy in squamous carcinomas. Cancer Cell (2014) 25:809–21. doi: 10.1016/j.ccr.2014.04.026
59. Gunderson AJ, Kaneda MM, Tsujikawa T, Nguyen AV, Affara NI, Ruffell B, et al. Bruton tyrosine kinase-dependent immune cell cross-talk drives pancreas cancer. Cancer Discov (2016) 6:270–85. doi: 10.1158/2159-8290.CD-15-0827
60. Braun DA, Street K, Burke KP, Cookmeyer DL, Denize T, Pedersen CB, et al. Progressive immune dysfunction with advancing disease stage in renal cell carcinoma. Cancer Cell (2021) 39:632–648.e8. doi: 10.1016/j.ccell.2021.02.013
61. Xu W, Wu Y, Liu W, Anwaier A, Tian X, Su J, et al. Tumor-associated macrophage-derived chemokine CCL5 facilitates the progression and immunosuppressive tumor microenvironment of clear cell renal cell carcinoma. Int J Biol Sci (2022) 18:4884–900. doi: 10.7150/ijbs.74647
62. Loftus RM, Finlay DK. Immunometabolism: Cellular metabolism turns immune regulator. J Biol Chem (2016) 291:1–10. doi: 10.1074/jbc.R115.693903
63. Geltink RIK, Kyle RL, Pearce EL. Unraveling the complex interplay between T cell metabolism and function. Annu Rev Immunol (2018) 36:461–88. doi: 10.1146/annurev-immunol-042617-053019
64. Zhang B, Vogelzang A, Miyajima M, Sugiura Y, Wu Y, Chamoto K, et al. B cell-derived GABA elicits IL-10+ macrophages to limit anti-tumour immunity. Nature (2021) 599:471–6. doi: 10.1038/s41586-021-04082-1
65. Malhotra D, Fletcher AL, Astarita J, Lukacs-Kornek V, Tayalia P, Gonzalez SF, et al. Transcriptional profiling of stroma from inflamed and resting lymph nodes defines immunological hallmarks. Nat Immunol (2012) 13:499–510. doi: 10.1038/ni.2262
66. Fletcher AL, Acton SE, Knoblich K. Lymph node fibroblastic reticular cells in health and disease. Nat Rev Immunol (2015) 15:350–61. doi: 10.1038/nri3846
67. Mionnet C, Mondor I, Jorquera A, Loosveld M, Maurizio J, Arcangeli M-L, et al. Identification of a new stromal cell type involved in the regulation of inflamed b cell follicles. PloS Biol (2013) 11:e1001672. doi: 10.1371/journal.pbio.1001672
68. De Silva NS, Klein U. Dynamics of b cells in germinal centres. Nat Rev Immunol (2015) 15:137–48. doi: 10.1038/nri3804
69. Gu-Trantien C, Loi S, Garaud S, Equeter C, Libin M, de Wind A, et al. CD4+ follicular helper T cell infiltration predicts breast cancer survival. J Clin Invest (2013) 123:2873–92. doi: 10.1172/JCI67428
70. Kranich J, Krautler NJ. How follicular dendritic cells shape the b-cell antigenome. Front Immunol (2016) 7:225. doi: 10.3389/fimmu.2016.00225
71. Goc J, Germain C, Vo-Bourgais TKD, Lupo A, Klein C, Knockaert S, et al. Dendritic cells in tumor-associated tertiary lymphoid structures signal a Th1 cytotoxic immune contexture and license the positive prognostic value of infiltrating CD8+ T cells. Cancer Res (2014) 74:705–15. doi: 10.1158/0008-5472.CAN-13-1342
72. Nayar S, Campos J, Smith CG, Iannizzotto V, Gardner DH, Mourcin F, et al. Immunofibroblasts are pivotal drivers of tertiary lymphoid structure formation and local pathology. Proc Natl Acad Sci U.S.A. (2019) 116:13490–7. doi: 10.1073/pnas.1905301116
73. Barone F, Gardner DH, Nayar S, Steinthal N, Buckley CD, Luther SA. Stromal fibroblasts in tertiary lymphoid structures: A novel target in chronic inflammation. Front Immunol (2016) 7:477. doi: 10.3389/fimmu.2016.00477
74. Rodriguez AB, Peske JD, Woods AN, Leick KM, Mauldin IS, Meneveau MO, et al. Immune mechanisms orchestrate tertiary lymphoid structures in tumors via cancer-associated fibroblasts. Cell Rep (2021) 36:109422. doi: 10.1016/j.celrep.2021.109422
75. Liu B, Chen X, Zhan Y, Wu B, Pan S. Identification of a gene signature for renal cell carcinoma-associated fibroblasts mediating cancer progression and affecting prognosis. Front Cell Dev Biol (2020) 8:604627. doi: 10.3389/fcell.2020.604627
76. Meylan M, Petitprez F, Becht E, Bougoüin A, Pupier G, Calvez A, et al. Tertiary lymphoid structures generate and propagate anti-tumor antibody-producing plasma cells in renal cell cancer. Immunity (2022) 55:527–541.e5. doi: 10.1016/j.immuni.2022.02.001
77. Gabrilovich DI, Bronte V, Chen S-H, Colombo MP, Ochoa A, Ostrand-Rosenberg S, et al. The terminology issue for myeloid-derived suppressor cells. Cancer Res (2007) 67:425. doi: 10.1158/0008-5472.CAN-06-3037
78. Shen M, Wang J, Yu W, Zhang C, Liu M, Wang K, et al. A novel MDSC-induced PD-1-PD-L1+ b-cell subset in breast tumor microenvironment possesses immuno-suppressive properties. Oncoimmunology (2018) 7:e1413520. doi: 10.1080/2162402X.2017.1413520
79. Liu M, Wei F, Wang J, Yu W, Shen M, Liu T, et al. Myeloid-derived suppressor cells regulate the immunosuppressive functions of PD-1-PD-L1+ bregs through PD-L1/PI3K/AKT/NF-κB axis in breast cancer. Cell Death Dis (2021) 12:465. doi: 10.1038/s41419-021-03745-1
80. Lee-Chang C, Rashidi A, Miska J, Zhang P, Pituch KC, Hou D, et al. Myeloid-derived suppressive cells promote b cell-mediated immunosuppression via transfer of PD-L1 in glioblastoma. Cancer Immunol Res (2019) 7:1928–43. doi: 10.1158/2326-6066.CIR-19-0240
81. Jaufmann J, Lelis FJN, Teschner AC, Fromm K, Rieber N, Hartl D, et al. Human monocytic myeloid-derived suppressor cells impair b-cell phenotype and function in vitro. Eur J Immunol (2020) 50:33–47. doi: 10.1002/eji.201948240
82. Luan Y, Mosheir E, Menon MC, Wilson D, Woytovich C, Ochando J, et al. Monocytic myeloid-derived suppressor cells accumulate in renal transplant patients and mediate CD4(+) Foxp3(+) treg expansion. Am J Transplant Off J Am Soc Transplant Am Soc Transpl Surg (2013) 13:3123–31. doi: 10.1111/ajt.12461
83. Huang B, Pan P-Y, Li Q, Sato AI, Levy DE, Bromberg J, et al. Gr-1+CD115+ immature myeloid suppressor cells mediate the development of tumor-induced T regulatory cells and T-cell anergy in tumor-bearing host. Cancer Res (2006) 66:1123–31. doi: 10.1158/0008-5472.CAN-05-1299
84. Özkan B, Lim H, Park S-G. Immunomodulatory function of myeloid-derived suppressor cells during b cell-mediated immune responses. Int J Mol Sci (2018) 19:E1468. doi: 10.3390/ijms19051468
85. Díaz-Montero CM, Rini BI, Finke JH. The immunology of renal cell carcinoma. Nat Rev Nephrol (2020) 16:721–35. doi: 10.1038/s41581-020-0316-3
86. Lelis FJN, Jaufmann J, Singh A, Fromm K, Teschner AC, Pöschel S, et al. Myeloid-derived suppressor cells modulate b-cell responses. Immunol Lett (2017) 188:108–15. doi: 10.1016/j.imlet.2017.07.003
87. Aggen DH, Ager CR, Obradovic AZ, Chowdhury N, Ghasemzadeh A, Mao W, et al. Blocking IL1 beta promotes tumor regression and remodeling of the myeloid compartment in a renal cell carcinoma model: Multidimensional analyses. Clin Cancer Res Off J Am Assoc Cancer Res (2021) 27:608–21. doi: 10.1158/1078-0432.CCR-20-1610
88. Colbeck EJ, Ager A, Gallimore A, Jones GW. Tertiary lymphoid structures in cancer: Drivers of antitumor immunity, immunosuppression, or bystander sentinels in disease? Front Immunol (2017) 8:1830. doi: 10.3389/fimmu.2017.01830
89. Sautès-Fridman C, Petitprez F, Calderaro J, Fridman WH. Tertiary lymphoid structures in the era of cancer immunotherapy. Nat Rev Cancer (2019) 19:307–25. doi: 10.1038/s41568-019-0144-6
90. Posch F, Silina K, Leibl S, Mündlein A, Moch H, Siebenhüner A, et al. Maturation of tertiary lymphoid structures and recurrence of stage II and III colorectal cancer. Oncoimmunology (2018) 7:e1378844. doi: 10.1080/2162402X.2017.1378844
91. Siliņa K, Soltermann A, Attar FM, Casanova R, Uckeley ZM, Thut H, et al. Germinal centers determine the prognostic relevance of tertiary lymphoid structures and are impaired by corticosteroids in lung squamous cell carcinoma. Cancer Res (2018) 78:1308–20. doi: 10.1158/0008-5472.CAN-17-1987
92. Masuda T, Tanaka N, Takamatsu K, Hakozaki K, Takahashi R, Anno T, et al. Unique characteristics of tertiary lymphoid structures in kidney clear cell carcinoma: prognostic outcome and comparison with bladder cancer. J Immunother Cancer (2022) 10:e003883. doi: 10.1136/jitc-2021-003883
93. Xu W, Anwaier A, Ma C, Liu W, Tian X, Su J, et al. Prognostic immunophenotyping clusters of clear cell renal cell carcinoma defined by the unique tumor immune microenvironment. Front Cell Dev Biol (2021) 9:785410. doi: 10.3389/fcell.2021.785410
94. Fridman WH, Meylan M, Petitprez F, Sun C-M, Italiano A, Sautès-Fridman C. B cells and tertiary lymphoid structures as determinants of tumour immune contexture and clinical outcome. Nat Rev Clin Oncol (2022) 19(7):441–57. doi: 10.1038/s41571-022-00619-z
95. Huang Z-Y, Shao M-M, Zhang J-C, Yi F-S, Du J, Zhou Q, et al. Single-cell analysis of diverse immune phenotypes in malignant pleural effusion. Nat Commun (2021) 12:6690. doi: 10.1038/s41467-021-27026-9
96. Mei Y, Xiao W, Hu H, Lu G, Chen L, Sun Z, et al. Single-cell analyses reveal suppressive tumor microenvironment of human colorectal cancer. Clin Transl Med (2021) 11:e422. doi: 10.1002/ctm2.422
97. Wang W, Zhong Y, Zhuang Z, Xie J, Lu Y, Huang C, et al. Multiregion single-cell sequencing reveals the transcriptional landscape of the immune microenvironment of colorectal cancer. Clin Transl Med (2021) 11:e253. doi: 10.1002/ctm2.253
98. Che L-H, Liu J-W, Huo J-P, Luo R, Xu R-M, He C, et al. A single-cell atlas of liver metastases of colorectal cancer reveals reprogramming of the tumor microenvironment in response to preoperative chemotherapy. Cell Discovery (2021) 7:80. doi: 10.1038/s41421-021-00312-y
99. Gong L, Kwong DL-W, Dai W, Wu P, Li S, Yan Q, et al. Comprehensive single-cell sequencing reveals the stromal dynamics and tumor-specific characteristics in the microenvironment of nasopharyngeal carcinoma. Nat Commun (2021) 12:1540. doi: 10.1038/s41467-021-21795-z
100. Chen Z, Zhao M, Liang J, Hu Z, Huang Y, Li M, et al. Dissecting the single-cell transcriptome network underlying esophagus non-malignant tissues and esophageal squamous cell carcinoma. EBioMedicine (2021) 69:103459. doi: 10.1016/j.ebiom.2021.103459
101. Hornburg M, Desbois M, Lu S, Guan Y, Lo AA, Kaufman S, et al. Single-cell dissection of cellular components and interactions shaping the tumor immune phenotypes in ovarian cancer. Cancer Cell (2021) 39:928–944.e6. doi: 10.1016/j.ccell.2021.04.004
102. Roider T, Seufert J, Uvarovskii A, Frauhammer F, Bordas M, Abedpour N, et al. Dissecting intratumour heterogeneity of nodal b-cell lymphomas at the transcriptional, genetic and drug-response levels. Nat Cell Biol (2020) 22:896–906. doi: 10.1038/s41556-020-0532-x
103. Cillo AR, Kürten CHL, Tabib T, Qi Z, Onkar S, Wang T, et al. Immune landscape of viral- and carcinogen-driven head and neck cancer. Immunity (2020) 52:183–99.e9. doi: 10.1016/j.immuni.2019.11.014
104. Callahan BM, Yavorski JM, Tu YN, Tong WL, Kinskey JC, Clark KR, et al. T-Cell receptor-β V and J usage, in combination with particular HLA class I and class II alleles, correlates with cancer survival patterns. Cancer Immunol Immunother CII (2018) 67:885–92. doi: 10.1007/s00262-018-2139-7
105. Chobrutskiy BI, Zaman S, Diviney A, Mihyu MM, Blanck G. T-Cell receptor-α CDR3 domain chemical features correlate with survival rates in bladder cancer. J Cancer Res Clin Oncol (2019) 145:615–23. doi: 10.1007/s00432-018-2815-1
106. Burger JA, Wiestner A. Targeting b cell receptor signalling in cancer: preclinical and clinical advances. Nat Rev Cancer (2018) 18:148–67. doi: 10.1038/nrc.2017.121
107. Krishna C, Hakimi AA. Rules of engagement: The lymphocyte receptor ecosystem in renal cell carcinoma. Cancer Res (2022) 82:764–5. doi: 10.1158/0008-5472.CAN-22-0146
108. Ferrall-Fairbanks MC, Chakiryan NH, Chobrutskiy BI, Kim Y, Teer JK, Berglund A, et al. Quantification of T- and b-cell immune receptor distribution diversity characterizes immune cell infiltration and lymphocyte heterogeneity in clear cell renal cell carcinoma. Cancer Res (2022) 82:929–42. doi: 10.1158/0008-5472.CAN-21-1747
109. Motzer RJ, Escudier B, McDermott DF, George S, Hammers HJ, Srinivas S, et al. Nivolumab versus everolimus in advanced renal-cell carcinoma. N Engl J Med (2015) 373:1803–13. doi: 10.1056/NEJMoa1510665
110. Hakimi AA, Attalla K, DiNatale RG, Ostrovnaya I, Flynn J, Blum KA, et al. A pan-cancer analysis of PBAF complex mutations and their association with immunotherapy response. Nat Commun (2020) 11:4168. doi: 10.1038/s41467-020-17965-0
111. Harris RJ, Cheung A, Ng JCF, Laddach R, Chenoweth AM, Crescioli S, et al. Tumor-infiltrating b lymphocyte profiling identifies IgG-biased, clonally expanded prognostic phenotypes in triple-negative breast cancer. Cancer Res (2021) 81:4290–304. doi: 10.1158/0008-5472.CAN-20-3773
112. DeFalco J, Harbell M, Manning-Bog A, Baia G, Scholz A, Millare B, et al. Non-progressing cancer patients have persistent b cell responses expressing shared antibody paratopes that target public tumor antigens. Clin Immunol Orlando Fla (2018) 187:37–45. doi: 10.1016/j.clim.2017.10.002
113. Aizik L, Dror Y, Taussig D, Barzel A, Carmi Y, Wine Y. Antibody repertoire analysis of tumor-infiltrating b cells reveals distinct signatures and distributions across tissues. Front Immunol (2021) 12:705381. doi: 10.3389/fimmu.2021.705381
114. Zirakzadeh AA, Marits P, Sherif A, Winqvist O. Multiplex b cell characterization in blood, lymph nodes, and tumors from patients with malignancies. J Immunol Baltim Md 1950 (2013) 190:5847–55. doi: 10.4049/jimmunol.1203279
115. Wu Z, Zhou J, Xiao Y, Ming J, Zhou J, Dong F, et al. CD20+CD22+ADAM28+ b cells in tertiary lymphoid structures promote immunotherapy response. Front Immunol (2022) 13:865596. doi: 10.3389/fimmu.2022.865596
116. Qu Y, Feng J, Wu X, Bai L, Xu W, Zhu L, et al. A proteogenomic analysis of clear cell renal cell carcinoma in a Chinese population. Nat Commun (2022) 13:2052. doi: 10.1038/s41467-022-29577-x
Keywords: renal cell carcinoma, tumor microenvironment, B cells, immune cells, interaction network, immunotherapy
Citation: Wang Y-q, Chen W-j, Li W-y, Pan X-w and Cui X− (2022) Impact of interaction networks of B cells with other cells on tumorigenesis, progression and response to immunotherapy of renal cell carcinoma: A review. Front. Oncol. 12:995519. doi: 10.3389/fonc.2022.995519
Received: 16 July 2022; Accepted: 31 October 2022;
Published: 17 November 2022.
Edited by:
Walter J. Storkus, University of Pittsburgh, United StatesReviewed by:
Manoj Chelvanambi, University of Texas MD Anderson Cancer Center, United StatesLilit Karapetyan, Moffitt Cancer Center, United States
Copyright © 2022 Wang, Chen, Li, Pan and Cui. This is an open-access article distributed under the terms of the Creative Commons Attribution License (CC BY). The use, distribution or reproduction in other forums is permitted, provided the original author(s) and the copyright owner(s) are credited and that the original publication in this journal is cited, in accordance with accepted academic practice. No use, distribution or reproduction is permitted which does not comply with these terms.
*Correspondence: Xiu-wu Pan, cGFueGl1d3VAMTI2LmNvbQ==; Xin−gang Cui, Y3VpeGluZ2FuZ0B4aW5odWFtZWQuY29tLmNu
†These authors have contributed equally to this work and share first authorship