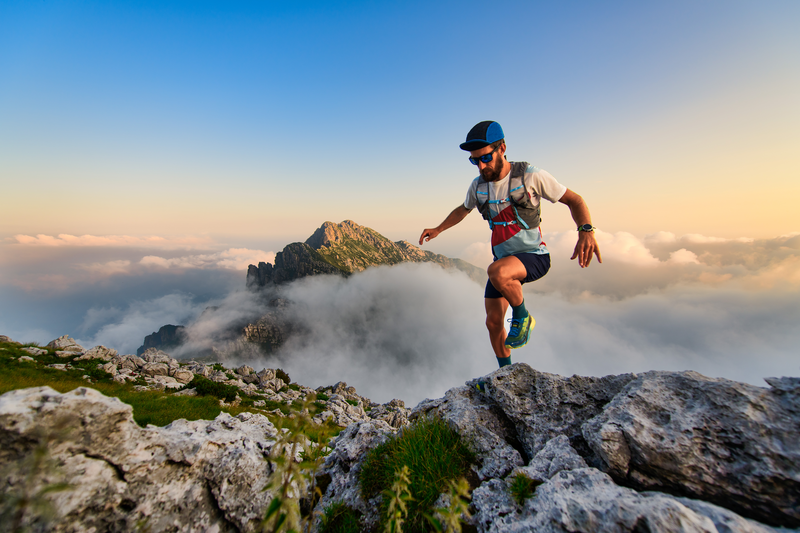
95% of researchers rate our articles as excellent or good
Learn more about the work of our research integrity team to safeguard the quality of each article we publish.
Find out more
REVIEW article
Front. Oncol. , 05 September 2022
Sec. Pediatric Oncology
Volume 12 - 2022 | https://doi.org/10.3389/fonc.2022.982608
This article is part of the Research Topic Developmental Therapeutics in Pediatric Neuro-oncology View all 7 articles
CNS germ cell tumors (GCTs) preferentially occur in pediatric and adolescent patients. GCTs are located predominantly in the neurohypophysis and the pineal gland. Histopathologically, GCTs are broadly classified into germinomas and non-germinomatous GCTs (NGGCTs). In general, germinoma responds well to chemotherapy and radiation therapy, with a 10-year overall survival (OS) rate of approximately 90%. In contrast, NGGCTs have a less favorable prognosis, with a five-year OS of approximately 70%. Germinomas are typically treated with platinum-based chemotherapy and whole-ventricular radiation therapy, while mature teratomas can be surgically cured. Other NGGCTs require intensive chemotherapy with radiation therapy, including whole brain or craniospinal irradiation, depending on the dissemination status and protocols. Long-term treatment-related sequelae, including secondary neoplasms and cerebrovascular events, have been well recognized. These late effects have a tremendous impact in later life, especially since patients are mostly affected in childhood or young adults. Intending to minimize the treatment burden on patients, the identification of biomarkers for treatment stratification and evaluation of treatment response is of critical importance. Recently, tumor cell content in germinomas has been shown to be closely related to prognosis, suggesting that cases with low tumor cell content may be safely treated with a less intensive regimen. Among the copy number alterations, the 12p gain is the most prominent and has been shown to be a negative prognostic factor in NGGCTs. MicroRNA clusters (mir-371-373) were also revealed to be a hallmark of GCTs, demonstrating the potential for the application of liquid biopsy in the diagnosis and detection of recurrence. Recurrent mutations have been detected in the MAPK or PI3K pathways, most typically in KIT and MTOR and low genome-wide methylation has been demonstrated in germinoma; this most likely reflects the cell-of-origin primordial germ cells for this tumor type. These alterations can also be leveraged for liquid biopsies of cell-free DNA and may potentially be targeted for treatment in the future. Advancements in basic research will be translated into clinical practice and can directly impact patient management. Additional understanding of the biology and pathogenesis of GCTs will lead to the development of better-stratified clinical trials, ultimately resulting in improved treatment outcomes and a reduction in long-term treatment-related adverse effects.
Central nervous system (CNS) germ cell tumors (GCTs) mostly occur in adolescents and young adults (AYA), with a median age of onset of 12–16 years (1–3). These tumors have a male predominance, with an estimated male-to-female ratio of 8:2; however, this depends on the site of occurrence. The pineal region is the most frequent location for GCTs, followed by the neurohypophysis, lateral ventricles, basal ganglia, cerebral and cerebellar hemispheres, and brainstem. CNS GCTs are more common in East Asia than in Western countries, and the site of occurrence differs geographically; tumor locations at the basal ganglia are more common in East Asia (4). Histopathologically, GCTs are mainly categorized into germinomas and non-germinomatous GCTs (NGGCTs); NGGCTs can be categorized as teratomas, yolk sac tumors, choriocarcinomas, and embryonal carcinomas (5). Teratomas include mature, immature, and teratoma with somatic-type malignancy. GCTs often present as a mixture of these components. Treatment is based on a combination of surgery, chemotherapy, and radiation therapy. GCTs are known to be sensitive to chemotherapy and radiation therapy (except for mature teratomas). The initial role of surgery in the treatment course is to obtain specimens for diagnosis, especially in cases presenting with negative tumor markers (i.e., human chorionic gonadotropin [HCG] and alpha-fetoprotein [AFP]). A biopsy is often omitted when these markers are elevated, along with imaging and clinical presentations compatible with GCTs. However, remnant tissue after chemotherapy and/or radiation therapy requires resection to decrease the chance of future enlargement and for diagnosis.
Treatment is mainly stratified according to the histological group, whether the tumor is a germinoma or NGGCT. The exceptions to this are the abovementioned mature teratomas, which only require surgical resection. Germinomas are treated less intensively than NGGCTs because the former tumor type is more sensitive to available therapies. In Europe and North America, cases with typical presentations in terms of imaging findings and clinical backgrounds undergo tumor marker examination. Tumor markers (HCG and AFP) are examined in blood serum and cerebrospinal fluid (CSF) from lumbar puncture or ventricular drainage when it is safe to obtain the sample for the latter. If tumor markers are elevated, patients are diagnosed with NGGCTs; if not, patients require a biopsy for histopathological diagnosis (6–10). In Japan, treatment stratification is unique in that it is based on three prognostic subgroups (i.e., germinoma, intermediate prognosis, and poor prognosis subgroups) that are determined according to histopathological confirmation (Table 1) (11, 12).
One of the ongoing issues in treatment and patient care is that treatment stratification is currently mostly limited to two or three categories and predictive biomarkers for guiding personalized treatment are presently scarce. As previously noted, CNS GCTs predominantly occur in the pediatric, adolescent, and young adult (AYA) population. The profound effects of chemotherapy and radiation therapy on pediatric and young adult patients cannot be overlooked. Secondary malignancies, including gliomas and melanomas, cavernous angiomas, meningiomas, vascular constrictions, and brain atrophy, are only a few of the concerns (13–18). Clinical trials are mainly concerned with the reduction of treatment burden while obtaining similar treatment outcomes; risk stratification has not yet been established.
However, recent clinical and molecular investigations present new key findings that may have prognostic or predictive value. Here, we present candidate biomarkers that have been discovered in research conducted on a global scale.
There is a scientific and medical consensus that the most appropriate treatment for germinomas occurring at typical sites, including the neurohypophysis, pineal gland, and lateral ventricles, is whole ventricular irradiation (WVI, with or without a local irradiation boost) and platinum-based chemotherapy (19). Upfront surgery is reserved for obtaining tumor biopsies for histopathological diagnosis. Historically, clinical trials conducted worldwide have proven that local irradiation is insufficient and that relapses occur (these relapses are mostly restricted to the ventricular system, with rare occurrences in the spinal canal) (7, 20, 21). The SIOP CNS GCT-96 trial demonstrated a 5-year progression-free survival (PFS) of 97 ± 2% in patients treated with craniospinal irradiation (CSI) and a PFS of 88 ± 4% in patients treated with local irradiation (7). COG ACNS1123 (stratum 2) showed a three-year PFS of 94% in patients treated with WVI at 18 Gy plus local irradiation at 12 Gy (Table 2) (22).
An issue of concern is that the treatment strategy is currently homogeneous, without any risk stratification, except for whether one is addressing localized or disseminated disease. Now that the treatment protocol has matured (although without stratification), researchers should endeavor to find clues for identifying cases where the treatment burden can be reduced. Moreover, although the treatment outcome for this tumor type is good, with a 5-year PFS of approximately 90%, the remaining 10% of patients experience treatment failure. Researchers should aim to identify the features that characterize those failure cases at the initial presentation.
Some germinoma cases present with elevated HCG levels in the serum and/or cerebrospinal fluid (CSF), and there are still controversies over the prognostic significance of elevated HCG in germinoma. The so-called “HCG-producing germinoma” is sometimes reflected by the presence of syncytiotrophoblastic giant cells (STGCs) in histopathological specimens, which indicates strong immuno-positivity for HCG. In a previous study of 74 histologically confirmed germinoma cases, 33 (45%) showed a higher than normal cut-off value for serum HCG, and those cases did not present worse relapse-free survival (RFS) than those with normal HCG values (23). In contrast, a study carrying out a highly sensitive measurement of HCG in CSF demonstrated that >85% of germinoma cases showed elevated CSF levels and that those with highly elevated HCG levels in the CSF (9/35 cases) showed worse RFS than those with lower HCG levels (24). Of note, regarding messenger RNA (mRNA) expression levels, almost all germinomas are known to express HCG mRNA (25). Collectively, although germinomas inherently produce HCG at baseline, highly elevated HCG in the CSF (with an undetermined cut-off line) seems to be associated with an unfavorable prognosis; these tumors should be carefully monitored for recurrence or dissemination on follow-up.
The sites of germinoma occurrence are predominantly at the midline of the brain, including the pineal gland, neurohypophysis, and lateral ventricles (and combinations thereof, i.e., typical sites). However, germinomas occasionally occur in the basal ganglia, thalamus, cortex/subcortex of the cerebrum, cerebellum, and spinal cord (i.e., atypical sites). Previous studies have revealed that cases with tumors occurring at atypical sites, such as those described above, show a poor prognosis compared with those occurring at typical sites (1, 26)., This may be due to the non-standard treatment regimens associated with atypical tumors. More specifically, typical site germinoma can be treated with WVI (with or without local irradiation). However, cases at atypical sites have to be managed differently and no appropriate radiation coverage has been elucidated based on clinical trials. Many clinical trials exclude germinomas at atypical sites, partly because these cases rarely occur, especially in Western countries (4).
We note that most of these cases should be treated with whole-brain or craniospinal irradiation. In a retrospective study, cases with basal ganglia germinoma treated with whole-brain or craniospinal irradiation showed better RFS than those treated with focal irradiation or WVI (26). Another potential reason for prognostic differences across tumor types includes biological differences between these tumors; for example, a prior study demonstrated that germinomas occurring at atypical sites had more frequent mutations in the PI3K pathway than those occurring at other sites (1). Atypical-site germinomas may also be more aggressive in nature. Conducting a large-scale study to determine the best management regimen is challenging because of the low frequency of this tumor outside of Asian countries. Future clinical trials should focus on the best radiation coverage and dosing strategies and investigate whether an adjustment in chemotherapy intensity could reduce patients’ radiation treatment burden.
Germinoma has well-known histopathological characteristics, with a germinoma cell and immune cell mixture that is dubbed a “two-cell pattern” (27). Most dominant immune cells are lymphocytes (T-cell and B-cell, approximately 60-70% on average) and natural killer cells, but immune cell repertoire in germinoma also includes myeloid-derived cells such as macrophages accounting for approximately 20-30% on average (28). The ratio between germinoma cells and immune cells is known to vary substantially depending on case characteristics, with <10% to 90% of tumor cells (out of all nucleated cells in tissue specimens) observed on hematoxylin-eosin (H-E) staining (29). As germinoma cells are obviously larger than immune cells and their cell morphologies differ, it would not be very challenging to calculate the ratio of tumor cells to immune cells in daily clinical practice. One of the novel aspects of evaluating and stratifying germinoma treatment strategies is that cases with many immune cells have shown better prognoses than those with a high tumor content in previous research (28). Moreover, this finding remained statistically significant when incorporating information on tumor location, as tumor location is another prognosis-related factor, as described previously (29). Currently, it is not standard practice to only treat germinoma with chemotherapy without radiation therapy, based on the results of previous clinical trials in which frequent relapses were observed in patients with germinoma treated with chemotherapy only (30). Conversely, a study evaluating chemotherapy-only regimens in 13 germinoma cases found that approximately 20–30% of these patients did well; that is, they did not experience recurrence within 5 years (30). Thus, a certain proportion of germinoma cases may be cured with chemotherapy alone. Germinoma presenting with abundant immune cells may be a good candidate for reduced treatment intensity (29).
Biological markers should be comprehensively evaluated in future clinical trials aiming to reduce the radiation dose administered to germinoma cases with abundant immune cells, whether regarding tumor content in H-E-stained specimens or other markers. A recent study revealed that germinoma cases could be subdivided into two subgroups when integrating transcriptome and methylome data (31); this stratification was reflected by differentiating the tumor cell-immune cell ratio. Additional studies are warranted to comprehensively and reliably identify the germinoma subgroup, which can be treated less intensively, and to identify relevant biomarkers.
In contrast to treatment for germinoma, the treatment for NGGCT has not yet been standardized worldwide. First, classifications differ in Europe, North America, and Japan. In Europe/North America, NGGCT is defined according to a case presentation in which tumor markers are elevated or according to histopathological confirmation of NGGCTs when tumor markers are normal (10). In Japan, NGGCT is further subdivided into two classes: an intermediate prognosis group and a poor prognosis group (3, 11)., The intermediate prognosis group includes immature teratomas, teratomas with somatic-type malignancy, and mixed tumors (mainly composed of germinomas and teratomas). The poor prognosis group mainly includes patients with yolk sac tumors, choriocarcinomas, and embryonal carcinomas. These two groups were treated differently in terms of radiation coverage and the selected chemotherapy regimen in previous research (3). Moreover, we note that tumor marker cut-off values differed between SIOP (HCG >50 IU/L, AFP 25 ng/mL) and COG (HCG >100 IU/L, AFP 10 ng/mL) clinical trials, which led to differences in the patients that were registered as NGGCT cases in these trials. These differences in categorization make it difficult to compare treatment outcomes across studies conducted in the three abovementioned regions.
Localized NGGCTs were treated with WVI and local irradiation in COG ACNS 1123 (8), and are presently treated with WVI and spinal irradiation in ACNS 2021 (an ongoing study in North America). In Europe, NGGCTs have been treated with local irradiation in SIOP CNS GCT-96 and CNS GCTII (6, 7). In Japan, NGGCTs are treated with WVI and local irradiation in the intermediate prognosis group, whereas NGGCTs are treated using CSI and local irradiation in the poor prognosis group; these treatments are selected as a matter of protocol.3 Cases with multiple lesions, except for bifocal (neurohypophysis and pineal gland) lesions, are universally treated with CSI (Table 3).
Table 3 Representative clinical trials for non-germinomatous germ cell tumors (NGGCTs) across countries.
The SIOP GCTII study identified patients <6 years old as a high-risk subgroup among patients with NGGCT (6). This coincides with the biological categorization of GCTs into seven classes of whole-body GCTs, where cases <6 years old are categorized as type I (in contrast to many CNS cases that fall into the type II category) (32). Most type I cases comprise yolk sac tumors, teratomas, and their combination. These cases are clinically aggressive and require intensive treatment with high-dose chemotherapy and autologous stem cell rescue.
The SIOP GCTII study identified an AFP level of >1,000 ng/mL as a poor prognostic factor (6) The fraction of these cases was small (19/149, 12.8%); however, this subgroup showed a worse prognosis, with a higher relapse rate. This strongly suggests that patients with yolk sac tumors or yolk sac tumor components have a relatively poor prognosis among the overall population of those with NGGCTs. This finding needs to be validated in a larger cohort. We note that Japanese clinical trials have determined that patients with HCG levels of >2,000 IU/L or AFP levels of >2,000 ng/mL are eligible for upfront chemotherapy and radiation therapy without histopathological confirmation.
Residual masses are often found after chemotherapy +/- irradiation. The presence of a malignant component at histopathological confirmation following chemotherapy helps in stratifying the subsequent treatment regimen. More specifically, the existence of a residual mass has not by itself been shown to have prognostic significance. However, residual masses, with or without second-look surgery, have been associated with a higher relapse rate (6). Interestingly, most residual masses detected after chemotherapy and radiation therapy comprise teratomas and/or necrotic tissue (17, 33). In COG ACNS2021, if viable tumor cells are confirmed in resected specimens at second-look surgery following radiation therapy, high-dose chemotherapy with peripheral stem cell rescue is administered.
GCTs are chromosomally unstable tumors with abundant gains and losses of chromosomal arms (34). Among these, 12p gain is one of the most frequent copy number alterations found in approximately 40% of cases. In contrast, 12p gain is more common in testicular GCTs and is found in up to 90% of these tumors (35). A study on 12p gains in CNS GCTs found that this gain was considerably more common in patients with NGGCTs as compared with patients with germinomas and was especially frequent in patients with NGGCTs accompanied by malignant components (including yolk sac tumors, choriocarcinomas, and embryonal carcinomas). Patients with NGGCTs presenting with a 12p gain have been shown to have a shorter PFS and OS than those without the 12p gain. Moreover, this previous study showed that 12p copy number status was shared among different histological components (i.e., across germinoma and non-germinoma components) in the same tissue specimen when examined by microdissection and fluorescence in situ hybridization (FISH) analysis (36). We note that KRAS is an oncogene in this genomic region that is known to exhibit a pathognomonic mutation in GCTs (37); 12p gain was found to be mutually exclusive with the most frequent mutation in CNS GCTs, KIT mutation (36). 12p gain may serve as a biological marker for poor prognosis, which can be investigated on copy number analysis based on a methylation array or on FISH analysis.
A recent study in patients with testicular GCTs found that 3p25.3 gain was a poor prognostic factor among patients with non-seminomatous GCTs (38). This gain was present in 6.8% of cases, and those that harbored this gain showed shorter PFS and OS than those without. This gain has also been observed in a few seminoma cases and has been associated with resistance to platinum-based chemotherapy. This study did not evaluate specific genes in this genomic region that may potentially be involved in these biologically and clinically significant findings.
The frequency of this copy number alteration in CNS GCT populations has not yet been investigated. A study on the biological and clinical significance of the 3p25.3 gain among CNS counterparts is needed.
According to a study conducting exome sequencing and targeted sequencing in 124 CNS GCTs, mutations in the MAPK pathway (more precisely, the KIT/RAS pathway) and the PI3K pathway were found in 48% and 13% of the evaluated cases, respectively (37). In total, 55% of the analyzed cases exhibited at least one of these mutations. Mutations were found more frequently in germinoma cases (71%) than in NGGCT cases (34%). The KIT mutation was the most predominant. Moreover, regardless of the mutation type, all germinoma cases were immunopositive for the KIT protein, which suggests that high expression of KIT is a marker for germinoma; this corroborates the fact that migrating primordial germ cells (PGCs), which are hypothesized to be the cell-of-origin for this cancer type, depend on the KIT-KIT ligand for proliferation and survival (39). KIT is a receptor tyrosine kinase (RTK) recognized by antibodies to CD117 (c-Kit). KIT inhibitors are novel therapeutic agents that require testing in international clinical trials. Non-specific oral KIT inhibitors exist in the clinic, but of those RTK inhibitors in general use, dasatinib but not imatinib reliably crosses the blood-brain barrier (40). Inhibitors of mTOR, MEK, and ERK may also potentially play a role in the pathogenesis of GCTs.
Genome-wide methylation array analysis has shown that germinomas are characterized by strikingly low levels of methylation across the genome (41, 42). Hypomethylation may render GCTs unstable on a chromosome and genome level, making these tumors prone to progression. Notably, hypomethylation is observed in retrotransposons, such as LINE-1 and Alu; hypomethylation can activate retrotransposons in the genome, thereby leading to genomic structural abnormalities.
Transcriptome analysis by RNA sequencing has demonstrated that germinoma is characterized by a high expression of PGC- and meiosis-related marker genes, and that NGGCT is characterized by a high expression of genes related to tissue/organ development, Wnt/beta-catenin pathway signaling, and epithelial-mesenchymal transition (31) (43). These features were corroborated by protein expression findings in immunohistochemistry, where pluripotency markers (including KIT, Oct3/4, NANOG, TFAP2c) and germ cell markers (including MAGEA4, NY-ESO-1, TSPY) were highly positive in germinomas (44).
Moreover, comparative studies evaluating CNS and testicular GCTs showed that germinomas and seminomas, as well as NGGCTs and non-seminomatous GCTs (NSGCTs), were grouped together on methylation analysis. While there were differences in expression between germinomas and seminomas, NGGCTs and NSGCTs were clustered on expression analysis (31). This suggests that the cell-of-origin for GCTs is shared across GCTs of different origins and that these tumor types might share similar pathogenesis. We note that studies on new biomarkers and novel therapeutic agents may be possible using shared platforms across different organs.
These omics analyses have clarified many biological aspects of CNS GCTs. Although the development of targeted therapies and the identification of clinically useful biomarkers based on these findings is still under-investigated, this field has considerable potential.
MicroRNAs (miRNAs) derived from miR-371a-373p, and miR-302/367 clusters have been implicated in the pathogenesis of GCTs. These miRNAs are consistently expressed in germinomas, and TP53 is suspected to be functionally inactivated by miRNAs arising from the miR-371a-373p cluster (45). These biomarkers were reported to outperform standard tumor markers in terms of sensitivity and specificity for detecting GCTs of the testis in a prospective study (46). For CNS GCTs, their roles would include early detection of GCTs (before the appearance on imaging) and early detection of tumor recurrence during and after treatment (47). Prospective studies conducted in a large cohort, including various histological subtypes, are warranted to elucidate the function of these miRNAs in CNS GCTs. Moreover, because of their suspected involvement in the TP53-MDM2 signaling pathway, a targeted treatment approach may be applicable in reference to these biomolecules.
The past decade has seen reports of a few key clinical trials originating from Europe and North America. Based on these findings, new clinical trials that are necessary to further elucidate the appropriate treatment intensities for both germinomas and NGGCTs have been started. Alongside this, basic research (including several omics studies) has been ongoing; this research has clarified important biological aspects of GCTs (Table 4). However, risk stratification is still underdeveloped as compared with that for other pediatric brain tumors. Additional efforts are needed to investigate potential risk factors, and clinical trials examining how to incorporate these factors into clinical practice in order to adjust treatment intensity are necessary. Because GCT is primarily a pediatric and adolescent disease, timely studies informing the reduction of long-term treatment-related sequelae and the resulting clinical implementation of these evidence-based findings are of critical importance.
HT designed and wrote the manuscript. KI wrote and checked the manuscript. All authors contributed to the article and approved the submitted version.
This study was partially sponsored by the Japanese Foundation for Multidisciplinary Treatment of Cancer (JFMC2021; no grant number) and Grant-in-Aid for Young Scientists, KAKENHI No. 22K16650 from the Japan Society for the Promotion of Science (JSPS).
The authors declare that the research was conducted in the absence of any commercial or financial relationships that could be construed as a potential conflict of interest.
All claims expressed in this article are solely those of the authors and do not necessarily represent those of their affiliated organizations, or those of the publisher, the editors and the reviewers. Any product that may be evaluated in this article, or claim that may be made by its manufacturer, is not guaranteed or endorsed by the publisher.
1. Takami H, Fukuoka K, Fukushima S, Nakamura T, Mukasa A, Saito N, et al. Integrated clinical, histopathological, and molecular data analysis of 190 central nervous system germ cell tumors from the iGCT consortium. Neuro-Oncology (2019) 21(12):1565–77. doi: 10.1093/neuonc/noz139
2. Jennings MT, Gelman R, Hochberg F. Intracranial germ-cell tumors: natural history and pathogenesis. J Neurosurg (1985) 63(2):155–67. doi: 10.3171/jns.1985.63.2.0155
3. Matsutani M, Sano K, Takakura K, Fujimaki T, Nakamura O, Funata N, et al. Primary intracranial germ cell tumors: a clinical analysis of 153 histologically verified cases. J Neurosurg (1997) 86(3):446–55. doi: 10.3171/jns.1997.86.3.0446
4. Takami H, Perry A, Graffeo CS, Giannini C, Narita Y, Nakazato Y, et al. Comparison on epidemiology, tumor location, histology, and prognosis of intracranial germ cell tumors between Mayo clinic and Japanese consortium cohorts. J Neurosurg (2020) 1(aop):1–11. doi: 10.3171/2019.11.JNS191576
5. Louis DN, Perry A, Wesseling P, Brat DJ, Cree IA, Figarella-Branger D, et al. The 2021 WHO classification of tumors of the central nervous system: a summary. Neuro-Oncology (2021) 23(8):1231–51. doi: 10.1093/neuonc/noab106
6. Calaminus G, Frappaz D, Kortmann RD, Krefeld B, Saran F, Pietsch T, et al. Outcome of patients with intracranial non-germinomatous germ cell tumors–lessons from the SIOP-CNS-GCT-96 trial. Neuro Oncol (2017) 19(12):1661–72. doi: 10.1093/neuonc/nox122
7. Calaminus G, Kortmann R, Worch J, Nicholson JC, Alapetite C, Garre ML, et al. SIOP CNS GCT 96: final report of outcome of a prospective, multinational nonrandomized trial for children and adults with intracranial germinoma, comparing craniospinal irradiation alone with chemotherapy followed by focal primary site irradiation for patients with localized disease. Neuro Oncol (2013) 15(6):788–96. doi: 10.1093/neuonc/not019
8. Fangusaro J, Wu S, MacDonald S, Murphy E, Shaw D, Bartels U, et al. Phase II trial of response-based radiation therapy for patients with localized CNS nongerminomatous germ cell tumors: a children's oncology group study. J Clin Oncol (2019) 37(34):3283–90. doi: 10.1200/JCO.19.00701
9. Goldman S, Bouffet E, Fisher PG, Allen JC, Robertson PL, Chuba PJ, et al. Phase II trial assessing the ability of neoadjuvant chemotherapy with or without second-look surgery to eliminate measurable disease for nongerminomatous germ cell tumors: A children's oncology group study. J Clin Oncol (2015) 33(22):2464. doi: 10.1200/JCO.2014.59.5132
10. Frappaz D, Dhall G, Murray MJ, Goldman S, Faure Conter C, Allen J, et al. EANO, SNO and euracan consensus review on the current management and future development of intracranial germ cell tumors in adolescents and young adults. Neuro-Oncology (2022) 24(4):516–27. doi: 10.1093/neuonc/noab252
11. Nakamura H, Takami H, Yanagisawa T, Kumabe T, Fujimaki T, Arakawa Y, et al. The Japan society for neuro-oncology guideline on the diagnosis and treatment of central nervous system germ cell tumors. Neuro-Oncology (2022) 24(4):503–15. doi: 10.1093/neuonc/noab242
12. Takami H, Nakamura H, Ichimura K, Nishikawa R. Still divergent but on the way to convergence: clinical practice of CNS germ cell tumors in Europe and north America from the perspectives of the East. Neuro-Oncology Advances. (2022) 4(1):vdac061. doi: 10.1093/noajnl/vdac061
13. Elsamadicy AA, Babu R, Kirkpatrick JP, Adamson DC. Radiation-induced malignant gliomas: a current review. World Neurosurg (2015) 83(4):530–42. doi: 10.1016/j.wneu.2014.12.009
14. Salvati M, Frati A, Russo N, Caroli E, Polli FM, Minniti G, et al. Radiation-induced gliomas: report of 10 cases and review of the literature. Surg Neurol (2003) 60(1):60–7. doi: 10.1016/S0090-3019(03)00137-X
15. Vinchon M, Leblond P, Caron S, Delestret I, Baroncini M, Coche B. Radiation-induced tumors in children irradiated for brain tumor: a longitudinal study. Childs Nerv. Syst (2011) 27(3):445–53. doi: 10.1007/s00381-011-1390-4
16. Pettorini BL, Park YS, Caldarelli M, Massimi L, Tamburrini G, Di Rocco C. Radiation-induced brain tumours after central nervous system irradiation in childhood: a review. Childs Nerv. Syst (2008) 24(7):793–805. doi: 10.1007/s00381-008-0631-7
17. Takami H, Perry A, Graffeo CS, Giannini C, Daniels DJ. Novel diagnostic methods and posttreatment clinical phenotypes among intracranial germ cell tumors. Neurosurgery. (2020) 87(3):563–72. doi: 10.1093/neuros/nyaa108
18. Choudhary A, Pradhan S, Huda MF, Mohanty S, Kumar M. Radiation induced meningioma with a short latent period following high dose cranial irradiation - case report and literature review. J Neurooncol. (2006) 77(1):73–7. doi: 10.1007/s11060-005-9009-9
19. Murray MJ, Bartels U, Nishikawa R, Fangusaro J, Matsutani M, Nicholson JC. Consensus on the management of intracranial germ-cell tumours. Lancet Oncol (2015) 16(9):e470–7. doi: 10.1016/S1470-2045(15)00244-2
20. Jensen AW, Laack NNI, Buckner JC, Schomberg PJ, Wetmore CJ, Brown PD. Long-term follow-up of dose-adapted and reduced-field radiotherapy with or without chemotherapy for central nervous system germinoma. Int J Radiat Oncol Biol Physics. (2010) 77(5):1449–56. doi: 10.1016/j.ijrobp.2009.06.077
21. Eom KY, Kim IH, Park CI, Kim HJ, Kim JH, Kim K, et al. Upfront chemotherapy and involved-field radiotherapy results in more relapses than extended radiotherapy for intracranial germinomas: modification in radiotherapy volume might be needed. Int J Radiat. Oncol Biol Phys (2008) 71(3):667–71. doi: 10.1016/j.ijrobp.2008.01.061
22. Bartels U, Onar-Thomas A, Patel SK, Shaw D, Fangusaro J, Dhall G, et al. Phase II trial of response-based radiation therapy for patients with localized germinoma: a children’s oncology group study. Neuro-Oncology (2022) 24(6):974–83. doi: 10.1093/neuonc/noab270
23. Ogino H, Shibamoto Y, Takanaka T, Suzuki K, Ishihara S, Yamada T, et al. CNS germinoma with elevated serum human chorionic gonadotropin level: clinical characteristics and treatment outcome. Int J Radiat. Oncol Biol Phys (2005) 62(3):803–8. doi: 10.1016/j.ijrobp.2004.10.026
24. Fukuoka K, Yanagisawa T, Suzuki T, Shirahata M, Adachi Mishima J-i K, et al. Human chorionic gonadotropin detection in cerebrospinal fluid of patients with a germinoma and its prognostic significance: assessment by using a highly sensitive enzyme immunoassay. J Neurosurgery: Pediatrics. (2016) 1–5. doi: 10.3171/2016.4.PEDS1658
25. Takami H, Fukushima S, Fukuoka K, Suzuki T, Yanagisawa T, Matsushita Y, et al. Human chorionic gonadotropin is expressed virtually in all intracranial germ cell tumors. J Neurooncol. (2015) 124(1):23–32. doi: 10.1007/s11060-015-1809-y
26. Koh K-N, Wong RX, Lee D-E, Han JW, Byun HK, Yoon HI, et al. Outcomes of intracranial germinoma–a retrospective multinational Asian study on effect of clinical presentation and differential treatment strategies. Neuro-Oncology (2022) 24(8):1389–99. doi: 10.14791/btrt.2022.10.F-1321
27. Nitta T, Hishii M, Sato K, Okumura K. Immunohistochemical characterization of ‘small, lymphoid-like cell populations’ within germinomas: immunologic and molecular approaches to diagnosis. Cancer Lett (1995) 90(2):183–9. doi: 10.1016/0304-3835(95)99394-9
28. Takami H, Fukushima S, Aoki K, Satomi K, Narumi K, Hama N, et al. Intratumoural immune cell landscape in germinoma reveals multipotent lineages and exhibits prognostic significance. Neuropathol. Appl Neurobiol (2020) 46(2):111–24. doi: 10.1111/nan.12570
29. Takami H, Satomi K, Fukuoka K, Fukushima S, Matsushita Y, Yamasaki K, et al. Low tumor cell content predicts favorable prognosis in germinoma patients. Neuro-Oncology Advances. (2021) 3(1):vdab110. doi: 10.1093/noajnl/vdab110
30. da Silva NS, Cappellano AM, Diez B, Cavalheiro S, Gardner S, Wisoff J, et al. Primary chemotherapy for intracranial germ cell tumors: results of the third international CNS germ cell tumor study. Pediatr Blood cancer. (2010) 54(3):377–83. doi: 10.1002/pbc.22381
31. Takami H, Elzawahry A, Mamatjan Y, Fukushima S, Fukuoka K, Suzuki T, et al. Transcriptome and methylome analysis of CNS germ cell tumor finds its cell-of-origin in embryogenesis and reveals shared similarities with testicular counterparts. Neuro-Oncology (2022) 24(8):1246–58. doi: 10.1093/neuonc/noac021
32. Oosterhuis JW, Looijenga LH. Human germ cell tumours from a developmental perspective. Nat Rev Cancer. (2019) 19(9):522–37. doi: 10.1038/s41568-019-0178-9
33. Weiner HL, Finlay JL. Surgery in the management of primary intracranial germ cell tumors. Childs Nerv. Syst (1999) 15(11-12):770–3. doi: 10.1007/s003810050469
34. Schneider DT, Zahn S, Sievers S, Alemazkour K, Reifenberger G, Wiestler OD, et al. Molecular genetic analysis of central nervous system germ cell tumors with comparative genomic hybridization. Mod Pathol (2006) 19(6):864. doi: 10.1038/modpathol.3800607
35. Mostert M, Van de Pol M, Weghuis DO, Suijkerbuijk R, van Kessel AG, van Echten J, et al. Comparative genomic hybridization of germ cell tumors of the adult testis: confirmation of karyotypic findings and identification of a 12p-amplicon. Cancer Genet Cytogenet. (1996) 89(2):146–52. doi: 10.1016/0165-4608(96)00043-X
36. Satomi K, Takami H, Fukushima S, Yamashita S, Matsushita Y, Nakazato Y, et al. 12p gain is predominantly observed in non-germinomatous germ cell tumors and identifies an unfavorable subgroup of central nervous system germ cell tumors. Neuro-Oncology (2022) 24(5):834–46. doi: 10.1093/neuonc/noab246
37. Ichimura K, Fukushima S, Totoki Y, Matsushita Y, Otsuka A, Tomiyama A, et al. Recurrent neomorphic mutations of MTOR in central nervous system and testicular germ cell tumors may be targeted for therapy. Acta Neuropathol. (2016) 131(6):889–901. doi: 10.1007/s00401-016-1557-x
38. Timmerman DM, Eleveld TF, Sriram S, Dorssers LC, Gillis AJ, Schmidtova S, et al. Chromosome 3p25. 3 gain is associated with cisplatin resistance and is an independent predictor of poor outcome in Male malignant germ cell tumors. J Clin Oncol (2022). doi: 10.1200/JCO.21.02809
39. Runyan C, Schaible K, Molyneaux K, Wang Z, Levin L, Wylie C. Steel factor controls midline cell death of primordial germ cells and is essential for their normal proliferation and migration. Development (2006) 133(24):4861–9. doi: 10.1242/dev.02688
40. Guntner AS, Peyrl A, Mayr L, Englinger B, Berger W, Slavc I, et al. Cerebrospinal fluid penetration of targeted therapeutics in pediatric brain tumor patients. Acta neuropathologica Commun (2020) 8(1):1–13. doi: 10.1186/s40478-020-00953-2
41. Schulte SL, Waha A, Steiger B, Denkhaus D, Dorner E, Calaminus G, et al. CNS germinomas are characterized by global demethylation, chromosomal instability and mutational activation of the kit-, Ras/Raf/Erk- and akt-pathways. Oncotarget. (2016) 7(34):55026–42. doi: 10.18632/oncotarget.10392
42. Fukushima S, Yamashita S, Kobayashi H, Takami H, Fukuoka K, Nakamura T, et al. Genome-wide methylation profiles in primary intracranial germ cell tumors indicate a primordial germ cell origin for germinomas. Acta Neuropathol. (2017) 133(3):445–62. doi: 10.1007/s00401-017-1673-2
43. Wang HW, Wu YH, Hsieh JY, Liang ML, Chao ME, Liu DJ, et al. Pediatric primary central nervous system germ cell tumors of different prognosis groups show characteristic miRNome traits and chromosome copy number variations. BMC Genomics (2010) 11:132. doi: 10.1186/1471-2164-11-132
44. Hoei-Hansen CE, Sehested A, Juhler M, Lau YF, Skakkebaek NE, Laursen H, et al. New evidence for the origin of intracranial germ cell tumours from primordial germ cells: expression of pluripotency and cell differentiation markers. J Pathol (2006) 209(1):25–33. doi: 10.1002/path.1948
45. Voorhoeve PM, Le Sage C, Schrier M, Gillis AJ, Stoop H, Nagel R, et al. A genetic screen implicates miRNA-372 and miRNA-373 as oncogenes in testicular germ cell tumors. Cell. (2006) 124(6):1169–81. doi: 10.1016/j.cell.2006.02.037
46. Dieckmann K-P, Radtke A, Geczi L, Matthies C, Anheuser P, Eckardt U, et al. Serum levels of microRNA-371a-3p (M371 test) as a new biomarker of testicular germ cell tumors: results of a prospective multicentric study. J Clin Oncol (2019) 37(16):1412. doi: 10.1200/JCO.18.01480
Keywords: germ cell tumor, germinoma, non-germinomatous, biomarker, clinical trial
Citation: Takami H and Ichimura K (2022) Biomarkers for risk-based treatment modifications for CNS germ cell tumors: Updates on biological underpinnings, clinical trials, and future directions. Front. Oncol. 12:982608. doi: 10.3389/fonc.2022.982608
Received: 30 June 2022; Accepted: 15 August 2022;
Published: 05 September 2022.
Edited by:
Atsushi Makimoto, Tokyo Metropolitan Children’s Medical Center, JapanReviewed by:
Kyogo Suzuki, Tokyo Metropolitan Children’s Medical Center, JapanCopyright © 2022 Takami and Ichimura. This is an open-access article distributed under the terms of the Creative Commons Attribution License (CC BY). The use, distribution or reproduction in other forums is permitted, provided the original author(s) and the copyright owner(s) are credited and that the original publication in this journal is cited, in accordance with accepted academic practice. No use, distribution or reproduction is permitted which does not comply with these terms.
*Correspondence: Hirokazu Takami, dGFrYW1pLXRreUB1bWluLmFjLmpw
Disclaimer: All claims expressed in this article are solely those of the authors and do not necessarily represent those of their affiliated organizations, or those of the publisher, the editors and the reviewers. Any product that may be evaluated in this article or claim that may be made by its manufacturer is not guaranteed or endorsed by the publisher.
Research integrity at Frontiers
Learn more about the work of our research integrity team to safeguard the quality of each article we publish.