- 1Cancer Research Center, Shahid Beheshti University of Medical Sciences, Tehran, Iran
- 2Future Foods Lab, Virginia Seafood Agricultural Research and Extension Center, Virginia Tech, Hampton, VA, United States
- 3Department of Medical Biotechnology, School of Advanced Technologies in Medicine, Shahid Beheshti University of Medical Sciences, Tehran, Iran
- 4Department of Tissue Engineering and Applied Cell Sciences, School of Advanced Technologies in Medicine, Shahid Beheshti University of Medical Sciences, Tehran, Iran
- 5Department of Biology, Central Tehran Branch, Islamic Azad University, Tehran, Iran
Intraoperative radiotherapy (IORT) has become a growing therapy for early-stage breast cancer (BC). Some studies claim that wound fluid (seroma), a common consequence of surgical excision in the tumor cavity, can reflect the effects of IORT on cancer inhibition. However, further research by our team and other researchers, such as analysis of seroma composition, affected cell lines, and primary tissues in two-dimensional (2D) and three-dimensional (3D) culture systems, clarified that seroma could not address the questions about IORT effectiveness in the surgical site. In this review, we mention the factors involved in tumor recurrence, direct or indirect effects of IORT on BC, and all the studies associated with BC seroma to attain more information about the impact of IORT-induced seroma to make a better decision to remove or remain after surgery and IORT. Finally, we suggest that seroma studies cannot decipher the mechanisms underlying the effectiveness of IORT in BC patients. The question of whether IORT-seroma has a beneficial effect can only be answered in a trial with a clinical endpoint, which is not even ongoing.
1 Introduction
Breast cancer is the fifth most important reason for cancer death worldwide (1). Global statistics show that in 2020 female breast cancer caused 11.7% and 6.9% of new cases and deaths from all cancer types, respectively (2). Surgical intervention is the primary option for BC patient management. Based on prolonged research, the standard procedure is either an excision plus radiotherapy or a total mastectomy to achieve clear margins. It has been demonstrated that these two strategies are consistently equivalent in relapse-free and overall survival (3). In the early stages of BC, radiotherapy has been approved as a critical part of breast-conserving therapy (4). Following lumpectomy, radiation therapy is associated with fewer BC recurrences (distant or locoregional) and mortality (5). Hypofractionation and dose escalation were used as a standard of care. External beam radiotherapy (EBRT), which is typically administered in daily fractional doses during 5-6 weeks (45–50 Gy fractionated in 1.8–2.0 Gy per day), six weeks after surgery (6), while IORT is a high single dose of irradiation _either electrons (12Gy as boost dose/21Gy as radical dose) or X-rays (21Gy) _ given to the negative tumor margin during the surgery immediately after removing the tumor. Because electrons penetrate deeper than low-energy X-rays, breast tissue must be mobilized, and shields put into the posterior lumpectomy cavity to protect tissues inside the thorax. For measured depth, 20–21 Gy doses are regularly delivered at low-electron energy. Intraoperative electron radiation therapy (IOERT) is a common term for this method (7). Recently, the survival outcomes and local control of electron intraoperative radiotherapy (ELIOT) (using 50 kv IORT) and TARGIT (using 21 Gy IOERT) were released as two randomized clinical trials. They compared IORT and whole-breast EBRT (8, 9). TARGIT: means intraoperative radiotherapy with photon made by ZEISS COMPANY from Germany which is named “Intrabeam”. The dose of partial breast irradiation is 20 GY as Low KV-X Ray, which, based on biological and clinicopathological criteria is called: BOOST or RADICAL dose. ELLIOT: in completing different IORT procedures, here we are using Electron by two different doses: BOOST= 12 GY irradiation by an electron at the flap prepared during surgery that should be completed after surgery by EBRT. RADICAL: 21 GY irradiation by the electron during surgery as the radical dose which does not need EBRT anymore; it takes time less than 2 min. Moreover, the TARGIT-A trial showed risk-adapted targeted intraoperative radiotherapy (TARGIT-IORT) during lumpectomy for BC as impressive as whole-breast EBRT. TARGIT-IORT aims to achieve an accurately-positioned and accelerated form of tumor-bed irradiation, focusing on the target tissues alone, sparing normal tissues and organs such as lung, skin, heart, and chest wall structures from unnecessary and potentially harmful radiation treatment (10). Through ELIOT technique, the mobile linear accelerator delivers a single dose of radiation with electrons to the involved quadrant of the breast during surgery, reducing the radiotherapy course from six weeks to one single session during surgery (11). Both trials announced low local recurrence rates for IORT with tolerable toxicity and remarkable outcomes of overall survival (8, 9). In addition to these trials, emerging studies clarify the benefits and mechanisms underlying the local and systemic IORT in BC patients. Recently, wound fluid (seroma) has attracted the particular interest of researchers. It is typically formed in remained space after surgical excision. It leads to an inflammatory response in wound healing and seroma fluid accumulation in the subcutaneous area. During two recent decades, many studies have been done to clarify the impacts of seroma derived from IORT-treated tumor bed on the decrease of cancer recurrence. Studies by Belletti et al. and Herskind et al. have notified that seroma obtained from patients treated with IORT caused a reduction in proliferation and invasion of BC cell lines in vitro compared to seroma from non-treated patients.
Moreover, IORT treatment reported significant results in invasion (3-D Matrigel) and migration assays. No significant effects were observed on the proliferative capacity of seroma in 2D cell culture using BC cell lines (12, 13). Belletti et al. discovered an anti-cancer effect of TARGIT through changes in cytokines and growth factors in the resection cavity (12). Despite the promising results from these studies that introduced IORT-seroma as a tumor-inhibiting factor, there are many growing kinds of research on the analysis of seroma composition and effects of seroma on cell lines of BC and primary tissues that show contrary outcomes. Some of these studies performed using 3D ex vivo models have recently been performed by our research team. In this review, we will mention critical factors involved in BC recurrence, focusing on the direct and indirect effects of IORT on BC. Then we will discuss all the findings in this field of study to elucidate the benefit of removing or preserving IORT-seroma.
1.1 Factors involved in breast cancer recurrence
Ninety percent of all local relapses happen within proximity of the removed tumor site (14), and it may be due to remaining cancer cells in peritumoral tissue, which is developed by positive resection margins or perilymphatic and perivascular invasion (15). Studies showed that one of the important factors involved in BC recurrences could be the molecular subtype of the removed tumor. On the other hand, the heterogeneity of a single tumor results in drug resistance and recurrence. Moreover, the role of the microenvironment of the tumor bed and immune system in the development of recurrences would be significant. Figure 1 schematically presents the factors that influence the recurrence of the disease.
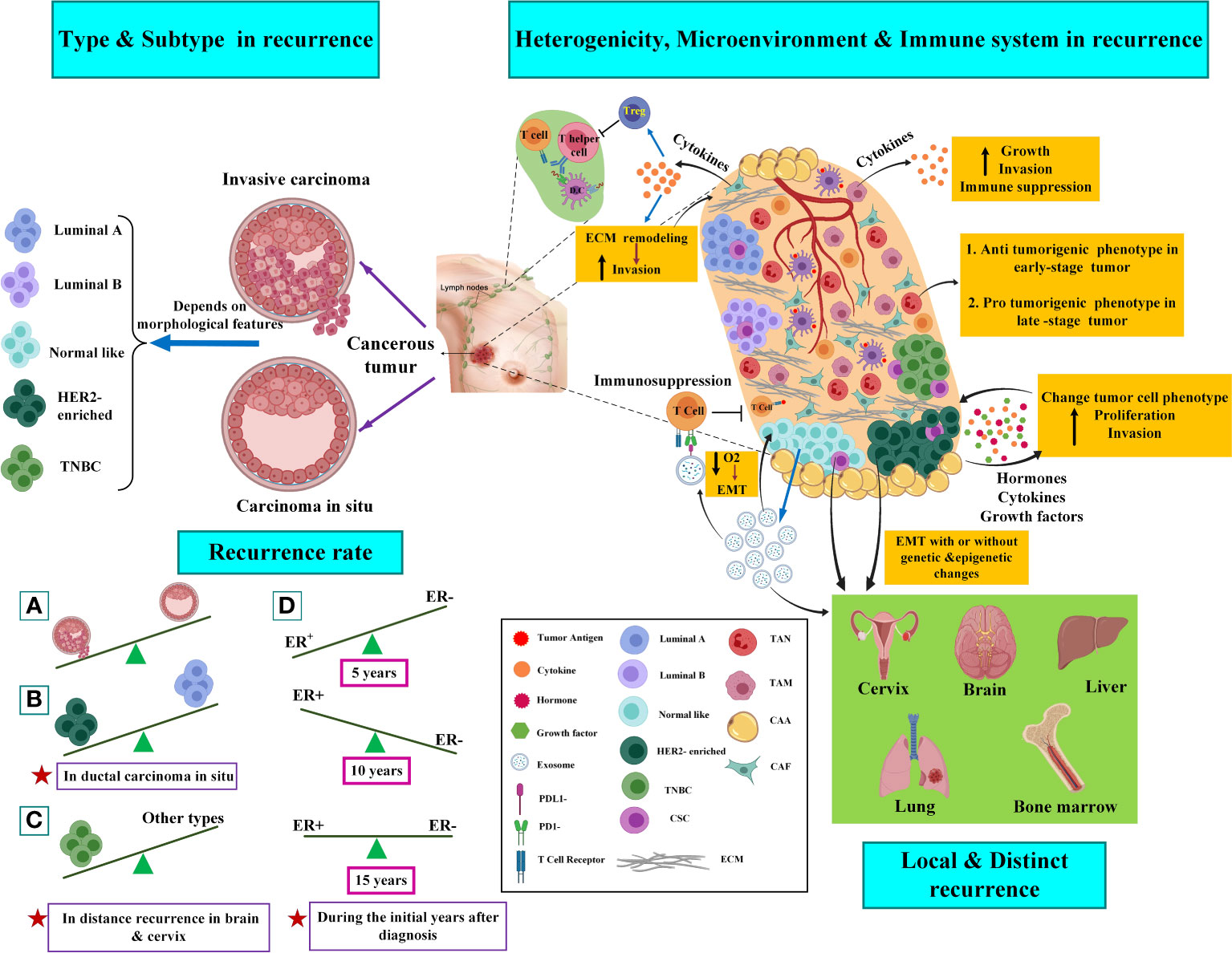
Figure 1 Factors involved in breast cancer recurrence. The left side of the picture shows the histopathologic types and molecular subtypes of the tumors in the recurrence of the disease. The right side of the picture shows the role of tumor heterogeneity, microenvironment, and immune system in breast cancer recurrence. Several clonal tumors containing CSCs are seen in tumor bulk and CSCs can affect the heterogenic migration of various clones in a single tumor. CSC also promote the EMT process and cause metastasis of BC tumor cell to the cervix, brain, liver, lung, and bone marrow. Cancer cells can recruit the immune system to progress tumor or even metastasis. Specific immune cells, including macrophages, lymphocytes, NK cells, dendritic cells (DCs), and neutrophils, are abundant and actively involved in the progression or suppression of cancer dissemination at the site of metastasis. Indeed, cancer cells can indirectly modulate and suppress the immune response (30, 32). CAFs promote tumor progression by initiating extracellular matrix remodeling through cytokine secretion. CAFs could suppress or avoid the immune response by promoting the recruitment of regulatory T cells (Treg), which is mediated by inflammation, or stopping the proliferation of T helper cells and killer T cells (28, 29). Work as tumor-modifying cells that may induce a change in cancer cell phenotype. CAAs produce hormones, growth factors, and cytokines. TAMs are the predominant immune cell types with immunosuppressive M2 polarized phenotypes that secrete tumor cytokines. Exosomes are essential in impairing both the adaptive and innate immune systems. It was shown that exosomal PDL1 derived from BC promotes and protects tumor growth by attaching to the PD-1 receptor of the CD8 T cells; thus, their adaptive killing activities are inhibited. Moreover, T-cells inhibit exosome secretion significantly through their anti-tumor immunity. In addition, uncontrolled cell proliferation induced by exosomes leads to inadequate nutrient and oxygen flow that derives the tumor microenvironment from becoming hypoxic. This process further triggers Epithelial-to-Mesenchymal Transition (EMT) and also promotes a more invasive phenotype. Further explanations are available in the text. TAN, tumor-associated neutrophil; TAM, tumor-associated macrophage; CAA, cancer-associated adipocyte; CAF, cancer-associated fibroblast; ECM, extracellular matrix; EMT, epithelial to mesenchymal transition; TNBC, triple-negative breast cancer; CSC, cancer stem cell. The figure was created using Biorender (https://biorender.com).
1.1.1 Molecular subtype
Different patterns of cancer recurrence have been suggested between various BC subtypes. According to Figure 1 (left side), it seems that estrogen receptor (ER)-negative breast cancers are susceptible to higher recurrence during the first five years than ER-positive breast cancers following diagnosis. For the next ten years, the recurrence risk will chronically enhance in ER-positive breast cancers, and fifteen years after diagnosis, the risk seems to be equivalent for both subtypes. It has been demonstrated that in ductal carcinoma in situ, the human epidermal growth factor receptor 2 (HER2)-positive/progesterone (PR)-negative/ER-negative cancers showed a higher recurrence risk than HER2-negative/PR-positive/ER-positive cancers. Triple-negative breast cancer (TNBCs), which are typified by the absence of PR/ER/HER2, are commonly related to a higher risk of recurrence compared to receptor-positive tumors, particularly with a higher rate of recurrences in distant tissues (in the brain and visceral metastases) (16).
1.1.2 Heterogeneity
Histopathologic, genetic, epigenetic, and single-cell sequencing studies, as well as the application of CTC-based assays in breast tumors, indicate that a single primary tumor can affect different regions and also, it may be able to phenotypic and genotypic change over time (17, 18). Tumor heterogeneity can be observed between cells within an individual patient’s tumor (intra-tumoral) or between cells of the same subgroup of tumors in different patients (inter-tumoral). At the genetic level, heterogeneity is connected to the copy number variation (CNV), down-regulation, and overexpression of a gene (due to missense, nonsense, or frameshift mutations) (19). Based on two concepts, including the cancer stem cell (CSC) hypothesis and the clonal evolution/selection model, primary single cells can undergo multiple molecular alterations and develop infinitive proliferative potential. The clonal evolution/selection model implies natural selection and explains how clones with higher epigenetic and genetic complexity can comply more under pressures than clones with low complexity (18). Unlike this model, the concept of CSC mentions self-renewal, capacity for clonal tumor initiation, and the potential for the clonal long-term repopulation (20). Interaction between CSCs and their niche (CSC surrounding microenvironment in a tumor) promotes invasion and metastasis of the tumor due to the production of factors, and the density of CSCs can affect the heterogenic migration of various clones in a single tumor (21, 22).
1.1.3 Tumor microenvironment and immune system
Researchers are increasingly supporting evidence that acellular and cellular components in the tumor microenvironment (TME) play a principal role in tumor growth and response to treatment. Cancer-associated fibroblasts (CAFs) are the main components in cancer stroma and TME. They promote tumor progression by initiating extracellular matrix remodeling through the secretion of cytokines (23). Adipocytes are other cell types that form TME. The most abundant component covering the cells in breast tumors is adipose tissue. Cancer-associated adipocytes (CAAs) work as tumor-modifying cells that may induce a change in cancer cell phenotype (24). Adipocytes produce hormones, growth factors, and cytokines. However, their role in the expansion of BC has not been fully discovered yet (25). Numerous types of immune cells, including macrophages, various phenotypes of T cells, B lymphocytes, natural killer (NK) cells, mast cells, and neutrophils, are found in breast tumors as part of normal tumor anatomy (26). Tumor-associated macrophages (TAMs) are the predominant immune cell types with immunosuppressive M2 polarized phenotypes that secrete tumor cytokines (IL-4, IL-10, and IL-13). These cytokines promote tumor growth by stimulating immune cell differentiation into mature macrophages (27). The interaction of tumor cells and the matured macrophages cause the secretion of various factors, such as vascular endothelial growth factor (VEGF) and colony-stimulating factor–1 (CSF1), via tumor cells, promoting tumor growth and invasion (28).
TAMs, through expressing heme oxygenase-1 (HO-1), an enzyme that inhibits immune system, suppress the endothelial cells’ response to tumor necrosis factor-a (TNFα), an immunogenic cytokine, and then maintain the immunosuppressive tumor microenvironment (29). Cancer progression and anti-cancer response also depend on interactions between cancer cells and the immune system. The interaction can be categorized into four groups: First, the process of immunosurveillance; second, the anti-cancer immune response; third, immunosuppression; and fourth, the cancer assistance program. Through the immunosurveillance mechanism, the healthy immune system continually checks tissues for the manifestation of cancer, particularly the existence of tumor antigens, including abnormally expressed, mutated, or oncoviral proteins. Following prosperous detection of tumor cells, the anti-cancer immune responses proceed and are identified by killer cells and T helper cells and then lyse the cancer cells. Furthermore, cancer cells can indirectly modulate and suppress the immune response. The c activation of b-catenin mediated by cancer cells in DCs is a specific example of this process.
DCs are responsible for presenting killer T cells adhered to the particular tumor antigens with the ability to direct the anti-tumor immune response. DCs cause a repressed cross-priming of CD8+ T cells adhered to tumor antigens following high levels of activated b-catenin; therefore, the entire process of anti-tumor immune response mediated by CD8+ T cell is dampened (30). Then, the immune cells can have a dual function in the tumor microenvironment; while specific features of tumor stroma can trigger immune cells to develop tumor suppression, other signals and features of the tumor can promote immune system-mediated tumor invasion (31–34).
Among acellular components of TME, exosomes possess a crucial role in shaping the microenvironment of the local tumor through paracrine crosstalk between stromal cells and tumor and in organizing future sites of metastasis. Exosomes are small extracellular vesicles with an average size of 100 nm with an endosomal origin that deliver various types of molecular and genetic information (e.g., lipids, proteins, and nucleic acids) to neighbor and distant cells. In pioneering studies, David C. Lyden et al. demonstrated that exosomes have a critical role in pre-metastatic niche formation in distant organs. Furthermore, the studies highlighted the role of exosomal integrins in directing organotropic metastasis. These findings bring further insight into cancer development’s complexity while demonstrating the existing gaps in our knowledge (35).
1.2 Responses of tumor and tumor bed to IORT
IORT is used in a high single dose that targets the wound cavity with a higher recurrence risk while spars the surrounding tissue and providing acceptable cosmetic and toxicological results (36–39). The IORT with direct effects removes survived tumor cells in the margin and non-irradiated neighbor cells through the bystander effect. The tumor microenvironment also receives significant modifications (39). Discovered direct and indirect biological responses of probably remained tumor cells as well as tumor bed cells to IORT are represented here and schematically presented in Figure 2.
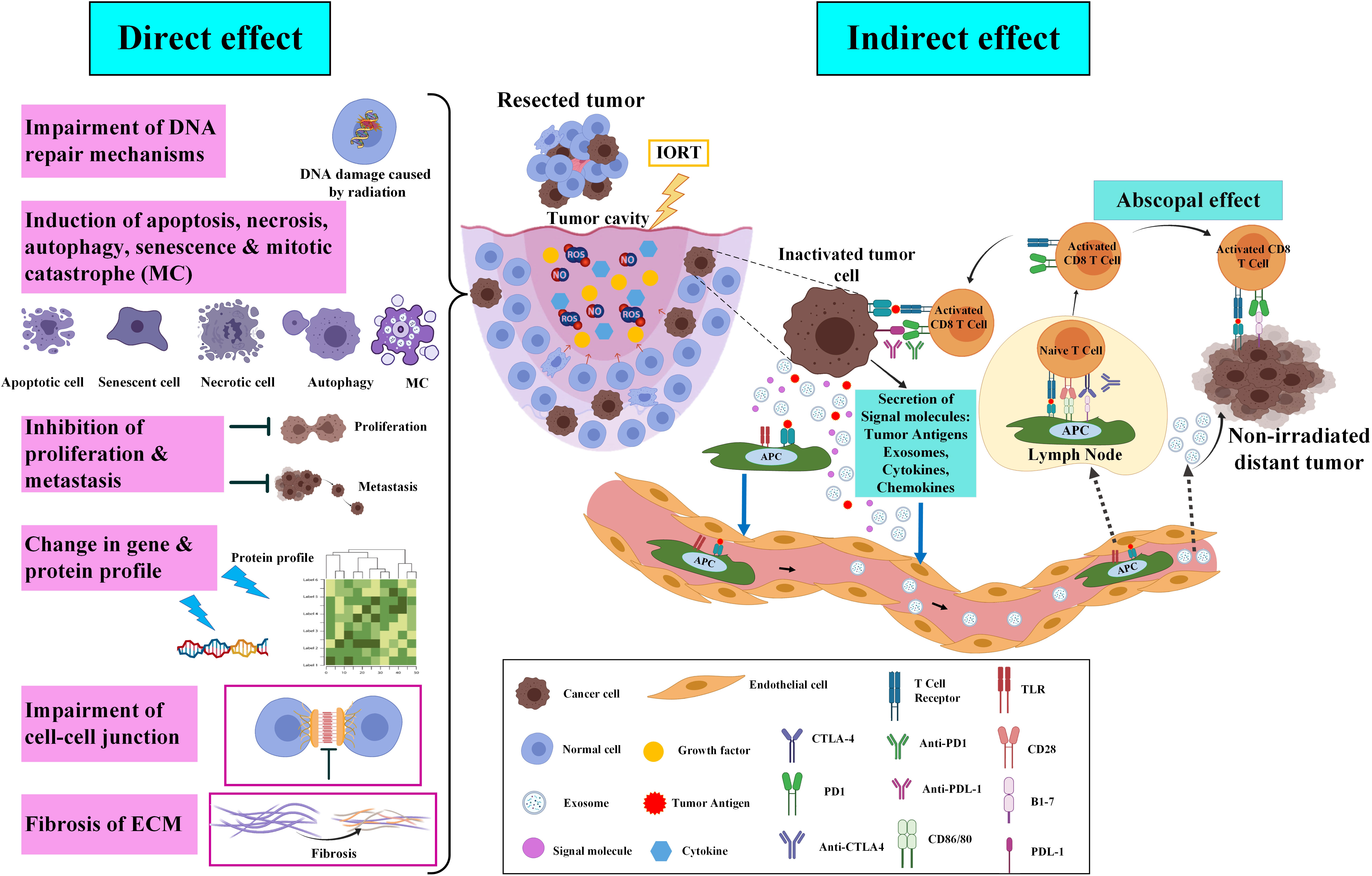
Figure 2 Direct and indirect effects of IORT on cancer inhibition and recurrence. After the breast-conserving surgery, molecular and probably remained cancer cells in the tumor cavity will be affected by IORT. Briefly, irradiation on the tumor cavity impairs DNA repair mechanisms, induces cell death, inhibits proliferation and metastasis, alters gene and protein profiles, impairs the cell-cell junction, and induces ECM fibrosis. In indirect effects of IORT, inactivated remained tumor cells release exosomes and tumor antigens which move and deliver to lymphatic vessels through the circulating system and APCs, respectively. IORT-induced tumor antigens and exosomes effects non-irradiated tumor cells in the body via a mechanism named “abscopal effect” (33). The figure was created using Biorender (https://biorender.com).
1.2.1 DNA repair mechanisms
Mainly, due to less time to repair their damages, IR induces more efficient cell death in proliferative cells than quiescent cells. Oxygen concentration is decreased in tumor tissue, and it was demonstrated that poor-oxygenated cells are less sensitive to IR radiation compared to those well-oxygenated cells. Cancer cells are more susceptible to unrepaired damage due to faster proliferation than normal cells; they often have several mutations that cause continual stimulation of the repair process. It can lead to their survival from damage; however, it may lead to the death of surrounding normal cells (40–42).
1.2.2 Metastasis, proliferation, and metabolism
After BC surgery, IORT targets tumor bed cells through modifying their growth conditions, such as preventing mammary mesenchymal stromal cells (MSC) from outgrowth after IORT (43). Segatto et al. supported this concept by finding the expression of miR-223 in the cells of the tumor bed that undergo IORT. This expression causes the reduction of epidermal growth factor (EGF) expression that finally abrogates cell growth and tumor recurrence (44). The main signaling pathway mediated by growth factors responsible for cancer cell survival and proliferation is the PI3K/AKT/mTOR (45), in which we recently observed downregulation of proteins that are part of the PI3K-Akt signaling pathway led to disrupted proliferation following radiation (46, 47). The tumor margin of cancer after 24h following IORT showed downregulation of central carbon metabolism. Cancer cells alter their metabolism to promote unrestricted cellular proliferation and respond to energetic and biosynthetic demands (48). Warburg first discovered that cancer cells have more demands for glucose; then, the glycolysis process is increased in them (49). After irradiation, processes associated with Glycolysis/Gluconeogenesis were suppressed, and several carbon metabolisms, including citrate cycle and fatty acid and amino acid degradation, were activated. After IORT, changes in the metabolisms could aid the environment in infiltrating immune cells and restrict the proliferation of remaining tumor cells (50).
1.2.3 Cell death mechanisms
Growing evidence shows that triggering the cell death mechanism as a therapeutic effect of radiotherapy would be a complicated process. Notably, nowadays, it is detected that suppression of the proliferative capacity of tumor cells behind irradiation happens through various mechanisms, including apoptosis, necrosis, senescence, autophagy, and mitotic catastrophe (MC) (51, 52). In high LET (linear energy transfer) radiation, direct ionization of cell macromolecules such as DNA, RNA, proteins, and lipids induce cell damage. Radiation with low LET leads to indirect damage to these macromolecules because of reactive oxygen species (ROS) production, particularly hydroxide and superoxide radicals produced from the radiolysis process of reactive nitric oxide species (RNOS) and intracellular H2O. These sources of ROSs trigger several intracellular signaling pathways and oxidate macromolecules, which lead to inflammation and stress responses (53–57). IR stimulates pro- and anti-proliferative signaling pathways that unbalance cell decisions about survival/apoptosis (58), which are regulated by several factors and genes involved in DNA repair, inflammation, cell cycle progression, and cell survival/or death (58–61). Altogether, various kinds of tumor cells, including immortalized keratinocytes, lung, colon, and prostate cancer, experience apoptosis after IR radiation (from 1 to 20 Gy). Many non-immortalized cells require higher irradiation doses (>20 Gy) to show apoptosis (51, 62, 63). Moreover, several data support the notion that IR treatment may stimulate p53-independent apoptosis by the mechanism of the membrane stress pathway, in vitro and in vivo, by sphingomyelin transmembrane signaling through the production of ceramide second messenger (57, 64). Radiation-triggered necrosis, unlike apoptosis, is mainly linked with intensified inflammation in the surrounding normal tissue (65). Necrosis is a type of cell death that has commonly been highlighted as a consequence of high IR doses (32 to 50 Gy) (57, 66). Senescence is a recognized procedure throughout aging and tissues under IR-treated or several stress stimuli such as chemotherapeutic agents, oxidative stress, and prolongation of signaling through some cytokines. The senescent cells can consequently initiate the pathology process (67–70). The primary cell response of lower doses of IR is senescence, while higher doses of IR induce necrosis or apoptosis in the same cells. In a study, X-ray irradiation of endothelial cells of the pulmonary artery showed that an intensifying dose of IR radiation induces a range of cell responses from senescence in lower doses and autophagy/apoptosis and necrosis at higher doses (62). Much data have been proposed that senescence in cancer cells-treated with IR could cause a decrease in self-renewal capacity (71, 72). Despite the potential tumor suppression role of senescent cancer cells, they could secrete a particular profile of the Senescence-Associated Secretory Phenotype (SASP), which contains a various range of cytokines, proteases, and growth factors. SASP changes the tissue microenvironment and triggers tumorigenesis and angiogenesis, as well as the EMT process and invasion. Although, SASP has anti-tumor function through tumor cell clearance via the immune system (73–75). Autophagy is a primary catabolic mechanism of cell degradation through lysosomal action that lyses dysfunctional or unnecessary cell components (76). Autophagy is a procedure to maintain metabolic homeostasis in tumor cells undergoing nutrient depletion and chronic hypoxia (41). This pathway can stimulate survival or cell death in IR-treated cells; the mechanisms depend on the gene expression controlling apoptosis, which is also cell and tissue-specific (77, 78). Mitotic catastrophe (MC) is associated with different biochemical and morphological changes. A cell death process occurs after or during aberrant mitosis and incomplete DNA synthesis following radiation (79). Checkpoint deficiencies in tumor cells cause defective DNA replication and malfunctioned repair mechanisms in the aberrant segregation of chromosomes that may lead to MC. Therefore, the control loss of checkpoints in IR-treated cancer cells may generate aneuploid progeny and cell death due to MC (57).
1.2.4 Cell-cell and cell-(extracellular matrix)ECM interaction
Crosstalk between PI3K/AKT/mTOR signaling pathway mediated by growth factors and cell adhesion to the ECM activates several vital biological processes, such as regulation of gene expression as well as proliferation, differentiation, survival, and motility of cells (80, 81). We also found a number of downregulated proteins through analysis of the KEGG pathways 24h after indirect irradiation of the tumor margins of breast-conserving surgery (BCS) plus IORT. The proteins are involved in the processes associated with focal adhesion, ECM-receptor interaction, and Rap1 signaling pathway (46, 82).
1.2.5 Gene and protein expression profile
Despite many significant research interests in the scientific community about the clinical application of IORT on different types of cancers, a limited number of papers were concerned about induced gene expression following treatment with IORT. A recent study on BC cell lines (both tumorigenic and non-tumorigenic cell lines) exposed with doses of 10 and 23 Gy identified differences among various types of cell lines and treatment after using microarray for gene expression profiling (83). According to our previous omics investigations on the tissue of negative tumor margins, radical and boost doses of IOERT change different molecular pathways (82). They could stimulate the activity of some signaling pathways, such as nuclear factor kappa B (NF-κB), TNF, forkhead Box O1 (FoxO), and hypoxia-inducible factors1 (HIF-1). We also detected that apoptosis, B cell receptor, Toll-like receptor, and metabolic pathways were upregulated, known to have systemic and local effects. The proteome profile was obtained from the isobaric tag for relative and absolute quantitation (ITRAQ) technique of tumor margin samples of patients under treatment with IOERT, 21Gy (sample collected before and 24 h of after treatment with IOERT). According to our results, the tumor margin samples collected before and after IORT showed alterations in the expression of many genes and enhanced pathways linked to cell growth, survival, programmed cell death, and cell cycle arrest. In addition, downregulated proteins that were part of the phosphatidylinositol 3-kinase (PI3K)-AKT signaling pathway showed disruption of proliferation after IORT (82). Besides, inhibition of this pathway, directly and indirectly, could influence the radiation results (82).
1.2.6 Abscopal effect
Local IR treatment of tumors often results in systemic responses at distant sites. This phenomenon is termed the “abscopal effect,” which induces and enhances the innate and adaptive immune responses against tumors (84). The abscopal effect is an antitumor consequence of radiation that can be seen in metastatic conditions away from irradiated tissue (85). In radiation therapy, diverse mechanisms are associated with the abscopal effect. Generally, the mechanisms include increasing the lymphocyte infiltration into the tumor’s microenvironment, improving detection and tumor cell death by enhancing the tumor’s antigens expression and antigen presentation machinery, increasing tumor sensitivity, and activating ascending modulatory pathways. The radiation-induced abscopal effect depends on the immune system through various strategies. In some cases, radiation therapy can activate the host immune system, especially in immunogenic cancers such as melanoma, hepatocellular carcinoma, and renal cell carcinoma. The synergistic effect between the host immune response and the abscopal effect induced by radiation therapy stimulates antitumor effects against micro-metastases beyond the irradiated area. A combination of immunotherapy and radiation therapy is used in some other cases, mainly involving less immunological cancers such as BC. Thus, immunogenic reagents, including immune checkpoint blockers and targeted immunomodulators, are combined with radiation therapy in this type of cancer. This combination promotes the host immune response against tumor cells and stimulates the abscopal effect after radiation therapy (86). However, in a rare case, Azami and colleagues reported that local radiation monotherapy in advanced BC, with extensive lymph node, lung, and bone metastases, effectively induced an abscopal effect in non-irradiated metastatic regions. A few months after radiation therapy, they observed that metastatic lesions regressed in the irradiated breast tumors and all non-irradiated areas (87). Generally, in the first antitumor strategy, high-dose radiation therapy with a direct effect on tumor cells kills these cells. It releases the remnants of dead tumor cells that contain potentially immunogenic molecules. These factors stimulate the immune system and lead to immunological cell death through T-regulatory cells, DCs, and suppressive cells (88). The combination of radiation therapy and granulocyte-macrophage colony-stimulating factor (GM-CSF) in some solid metastatic cancers can induce an abscopal effect and increase the overall survival of patients. Formenti and colleagues showed that in metastatic BC, the combination of radiation therapy with a systemic transforming growth factor-β (TGF-β) blocking antibody called Fresolimumab induces a dose-dependent systemic immune response and improves overall survival (89).
1.2.7 Effect of drainage on clinical outcome
Several studies have explored the safety of seroma drainage according to multiple clinical endpoints (Table 1). Quality of life has been reported in various kinds of results in different studies. Better quality of life was seen in long-term drainage (90), reduction of hospital stays, and early drain removal in some studies provided a better quality of life by decreasing post-operative complications or in some studies reported no adverse effects, so early drain removal was preferred by patients (98, 99). To the best of our knowledge, in IORT, no results were reported regarding drainage tube removal time, length of hospitalization, and post-operative complications. However, IORT had promising results, both in terms of saving healthy tissue and local control (74% to 100% at 5 years) and 96.2% disease-free survival (101, 103). The local relapse prompted a series of clinical trials and studies to investigate whether localized IORT could be as efficient at preventing recurrence at local site as standard postoperative radiotherapy of the whole breast while also being more patient-friendly in terms of decreasing the treatment duration. As we mentioned earlier, IORT decreases the possible risk of tumor cell repopulation during the wound healing process through direct radiation therapy of diseased tissue within the tumor bed during the surgical procedure (9).
1.3 Studies associated with effects of IORT-seroma on breast cancer progression
Key information related to all the studies about breast surgery IORT- and non-IORT-seroma and their effects on BC (according to our knowledge) is available in Table 2. See also Figure 3.
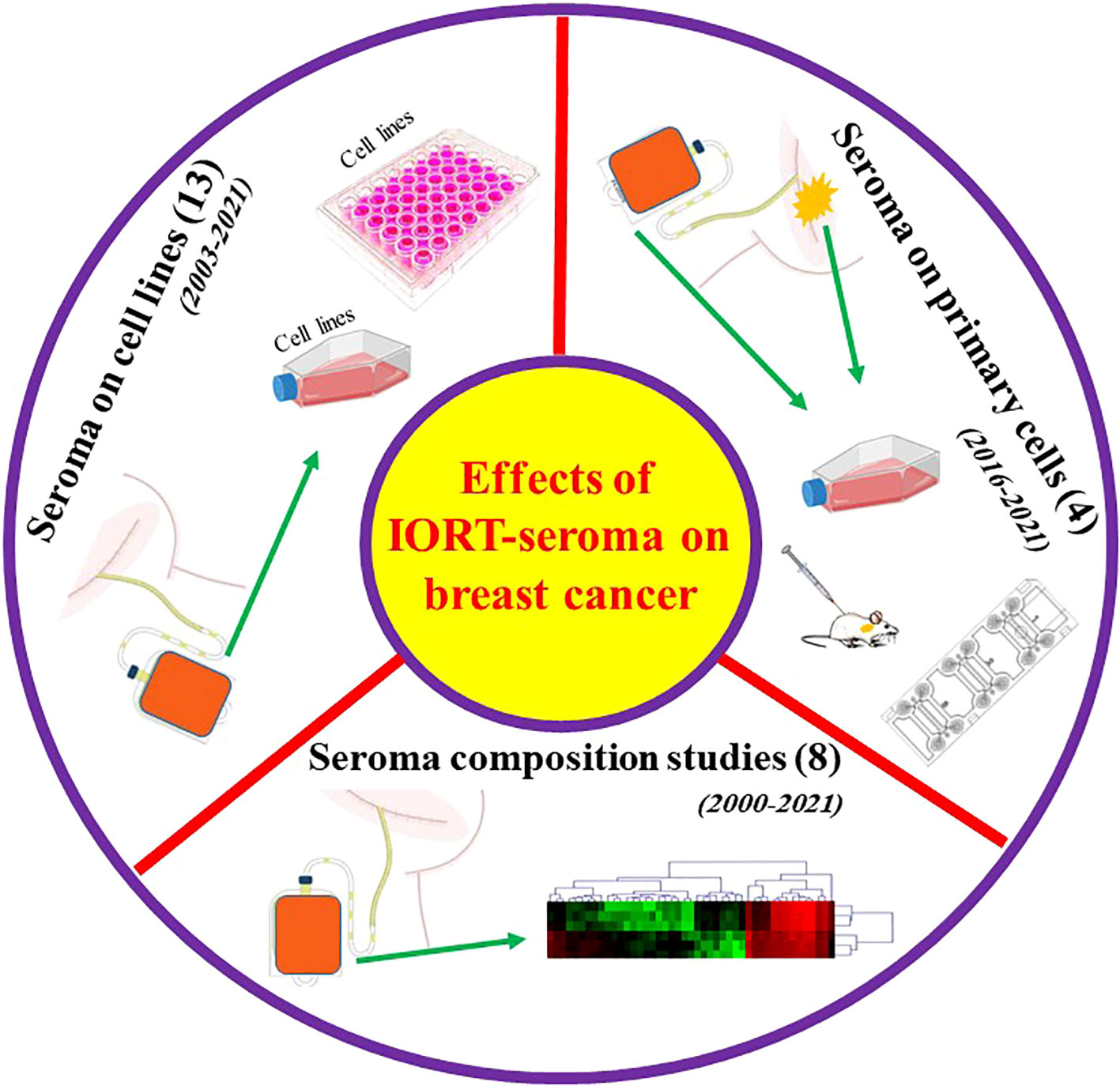
Figure 3 Graphical abstract for performed studies in breast surgery IORT- and non-IORT-seroma and their effects on breast cancer.
1.3.1 Composition of breast surgery-induced seroma; benign, malignant, IORT-treated
Seroma is an inflammatory exudate most commonly found during the first step of wound healing (104). It has been illuminated that seroma inoculation near the tumor site in mice with syngeneic BC xenografts led to enhanced tumor growth (105). Seroma derived from surgical sites may show brief information about the cell activity in terms of the release of growth factors, chemokines, and cytokines that are vital in repair and healing (106, 107). The differential expression of pro-oncogenic growth factors and cytokines is secreted from malignant to benign lesions in the post-surgical seroma in breast tumors. Valeta-Magara and colleagues detected that seroma from the surgical cavity of BC patients expresses a higher level of fundamental tumor-promoting cytokines. In contrast, benign surgical lesions in non-cancer patients express a lower level of principal tumor-inhibiting factors. They assessed 80 different cytokines, growth factors, and chemokines in 59 post-surgical seroma (24 patients with benign and 35 with malignant lesions). Although the results showed that 28 cytokines were overexpressed in both groups of seroma. Malignant-derived seroma showed higher expression of 9 biologically important factors. In particular, Leptin, tissue inhibitor of metalloproteinases 2 (TIMP-2), growth-regulated protein (GRO), and epithelial neutrophil-activating peptide 78/chemokine (C-X-C motif) ligand 5 (ENA-78/CXCL5) were highly overexpressed in malignant seroma. At the same time,insulin-like factor binding protein-1(IGFBP-1), IL-3, IL-16, fibroblast growth factors-9 (FGF-9), and IFN-γ showed down-regulation in malignant compared to the benign seroma. The post-surgical cavity of a breast tumor contains pro-inflammatory factors, regardless of being malignant or benign; however, in malignant tumors, a higher amount of additional pro-oncogenic cytokines, chemokines, and growth factors and a reduction in tumor-inhibiting factors are detected. These results showed the preconditioning effect of normal surrounding tissue on the tumor and provided a pro-oncogenic environment that remains after the removal of the tumor by surgery (108). In a recent study, Agresti and collaborators detected 34 cytokines, growth factors, and chemokines in seroma of 27 BC patients that promote the initiation and development of cancer. The results clarified that the molecular characteristics of the removed tumor influence the final composition of the secreted seroma. Specifically, MIP-1a, MIP-1b, IP-10, IL-6, G-CSF, monocyte chemoattractant protein1- monocyte chemotactic and activating factor (MCP1-MCAF), and osteopontin were expressed higher in more aggressive tumors. Furthermore, differential expression of several small molecules was detected in the seroma of BC patients with mastectomy or quadrantectomy. In mastectomized patients, IL-1ra, IL-1b, IFN-γ, IL-6, G-CSF, osteopontin, IP-10, and MIP-1b were significantly higher than in quadrantectomized patients (109). The quantitative molecular diagnosis of cytokeratin-19 (CK19) and carcinoembryonic antigen (CEA) that target cancer cells in axillary seroma showed that they are a predictor of locoregional recurrence in mastectomized BC patients (110). In pioneering research, Belletti et al. compared non-IORT-seroma with IORT-seroma and revealed that TARGIT might possess an anti-tumor effect and surpass cancer cell kill via radiation therapy through altering the cytokines and growth factors existing in the resection cavity. They evaluated the proteomic content of seromas and detected that in seroma derived from TARGIT-treated patients compared to non-treated ones, 10 proteins enhanced while 20 proteins decreased (12). Kulcenty et al. conducted a quantitative investigation of the composition of seroma in patients with BC subtypes of luminal A and luminal B and between two groups of non-treated and treated with IORT. The comparison showed that TNF-beta, macrophage migration inhibitory factor (MIF), IL-7, IL-8, and IL-13 were significantly reduced in IORT-seroma; However, these findings were obtained without a differential diagnosis of molecular subtypes in the seroma groups. Moreover, enhanced concentrations of G-CSF, cutaneous T-cell-attracting chemokine (CTACK), IL-1 beta, hepatocyte growth factor (HGF), and TNF-alpha were characterized in IORT-seroma. They found that several cytokines were overexpressed in the luminal A subtype in the IORT-treated group, which may have anti-tumor characteristics (111). In a recent study, Wuhrer et al. analyzed seromas collected 24h after breast-conserving surgery (from 42 patients) with and without IORT treatment and observed dramatic changes in populations of immune cells and levels of cytokine (112). None of the investigated subpopulations, such as Treg, T cells, and myeloid cells, showed alteration in their activation states or their counts in cellular fraction analysis of the seroma and blood samples of the patients 24 h after IR treatment compared to control. Moreover, both groups did not alter the leucocyte fraction’s apoptosis rate. Thus, IORT did not affect the processes in cellular immunity during the first 24h after surgery in the local environment. In this study, levels of cytokines in seroma were significantly changed in the IORT-treated group; results showed that cytokines including GRO-α,oncostatin-M, and IL-1β are reduced while Leptin is enhanced with IORT treatment. All of these cytokines are linked to inflammation and tumor growth. Figure 4 summarizes the studies related to seroma composition regarding protein changes.
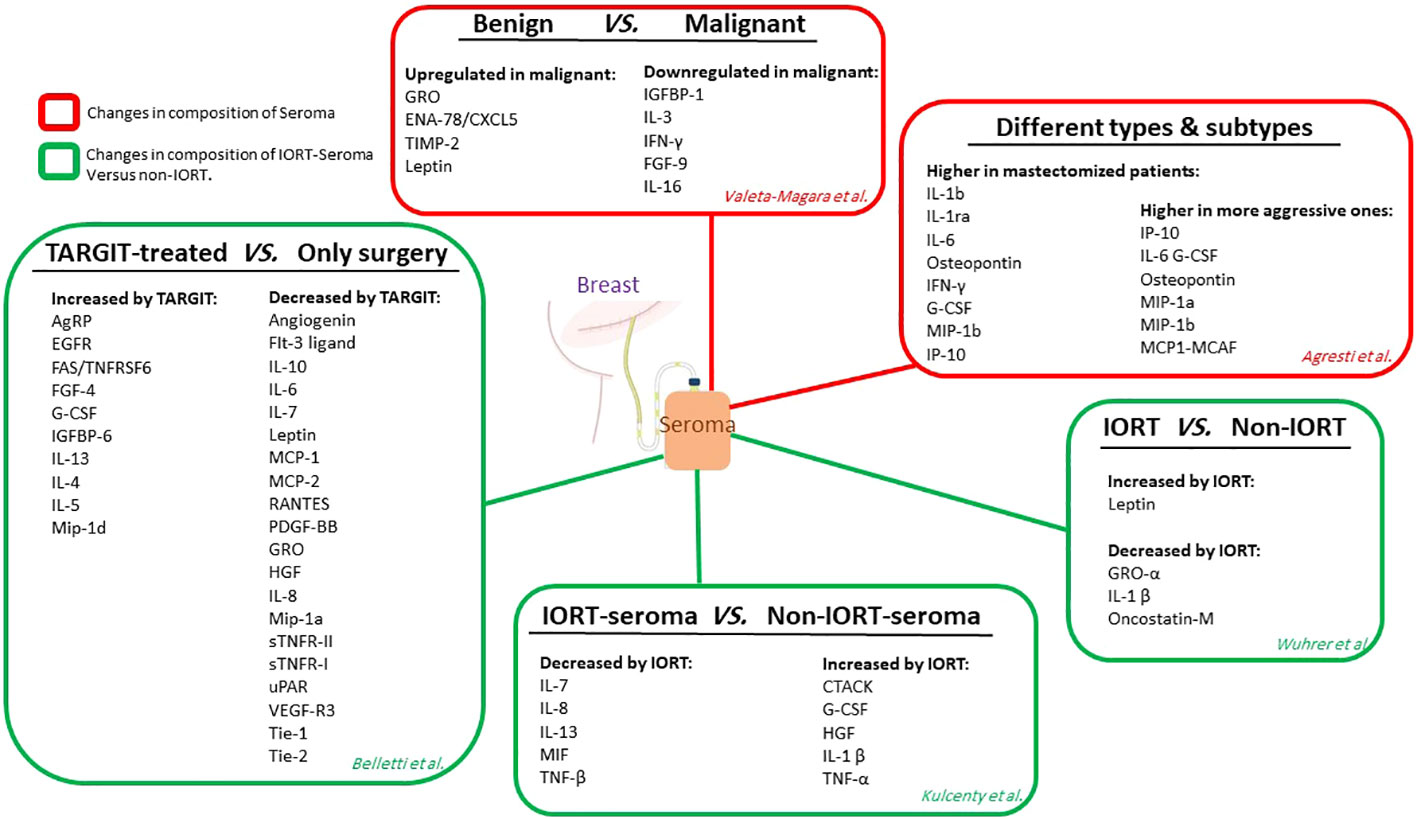
Figure 4 The studies related to seroma composition. Red boxes show the level of protein expression in seroma without considering radiotherapy. Green boxes show protein expression levels in an IORT-affected seroma compared to non-IORT-affected seroma.
1.3.2 Effects of seroma on breast cancer cell lines; IORT vs non-IORT
Tagliabue and collaborators first described the proliferative effects of seroma on cultures of BC cells, who tested 24h post-surgical seroma and serum from 13 BC patients on SKBR-3, MDA-MB-453, MDA-MB361, MDA-MB231, MDA-MB-435, MCF- 7 cell lines. The results clarified that all of the cell lines were stimulated to proliferate in response to the drainage fluids, although the HER2-positive cell lines showed more proliferation levels than the HER2-negative ones. Their findings showed that seroma and post-surgical serum samples comprised growth factors capable of inducing the proliferation of HER-2-positive breast cancers. Although surgical wounds provided favorable conditions for the proliferation of tumor cells, carcinomas with overexpression of HER-2 revealed a higher rate of stimulating growth. It suggests several factors secreted during repair and healing are particularly active in inducing the HER-2-positive cells (113). In their several studies, Belletti and colleagues highlighted that collected seroma from BC patients within 24 hours after surgery plays a principal role in the proliferation, survival, and motility of BC cells (12, 114, 115). Segatto et al. found that the post-surgical collected seroma highly stimulates mammosphere formation in BC cells. The researchers used EGF (as a standard stimulative agent) and seroma on cell lines of MDA-MB-231, BT-474, MDA-MB-468, and MCF-7 to test mammosphere formation. The seroma-stimulated cell lines showed a higher mammosphere forming efficacy (MFE) than those induced with EGF. Seroma highly activates signal transducer and activator of transcription 3 (STAT3) in BC cell lines. The STAT3 affects the proliferative phenotype of BC cells, and its signaling is essential for the self-renewal ability of the seroma-induced cells (116). Another study on the effects of seroma on BC cell lines by Ramolu et al. showed the capability of three types of seroma to induce the proliferation of BC cell lines. They collected seroma from 30 patients who had tumor surgery (10 patients) or underwent induction chemotherapy after tumor surgery (10 patients) or breast reconstruction (10 patients). The seromas were used to grow MCF-7 and HCC1937 cell lines. The results showed that all three groups of seromas induced the proliferation of the cells.
Interestingly, the proliferation index from culturing HCC1937 cells was significantly higher than MCF-7 cells, suggesting more sensitivity of triple-negative cell lines to stimulation by seroma (117). In studies by Belletti et al. and, Herskind et al., seroma obtained from patients treated with IORT led to more reduced invasion and proliferation of BC cell lines in vitro compared to those induced by seroma from non-IORT patients. However, in the short-term 2D cell culture of BC cell lines with molecular types of ER/PR, -Her2/neu, and ER/PR−, Her2/neu+, IORT had no significant effect on the proliferative capacity of seroma; although, it showed the significant effects on invasion assay on 3-D Matrigel and migration test (12, 13). To confirm the results of previous studies, Veldwijk and collaborators evaluated the clonogenic and long-term proliferation effects of IORT-seroma and non-IORT-seroma on the MCF-7 cell line. Their results showed that the difference between these groups was insignificant and that the cells required 3% FBS in addition to seroma for short-term and clonogenic proliferation (118). Recently, Agresti et al. treated MDA-MB-231, MCF-7, SKBR-3, HCC1937, BT-549, and T-47D with post-surgery seroma (collected 24h after surgery) from 27 BC patients. Measurement of cell growth in 2D culture over 4 days showed that seroma stimulated robust cell proliferation and migration in all cell lines (109).
Zaleska et al. treated 8 BC cell lines with seroma collected from conservative−breast surgery (WF) and compared data to that of seroma from IOERT treatment RT-WF (≤10 Gy) for 4 days to indicate the effect of seromas on the phenotype of cancer stem cells. Then, the differentiation cluster of CD44+/CD24-/low phenotype and activity of aldehyde dehydrogenase 1 (ALDH1) were characterized. Each of the two types of fluids impacted the CD44+/CD24-/low phenotype. They showed different consequences between cell lines, even in histologically similar subtypes. RT−WF led to the decreased CD44+/CD24-/low population in basal−like MDA−MB−468 and BT−549, while the two fluids inhibited these populations in the luminal type MCF7 cell line. The HER2 −overexpressing subtypes protected a minimal population of CD44+/CD24-/low, but the two postoperative fluids stimulated the growth of SK−BR−3. Compared to RT−WF, WF showed a more substantial effect on ALDH1 activity. Depending on the histological subtype of the cell lines, a different stimulatory effect was observed. The most robust stimulation was in the control group for the luminal subtypes with low dehydrogenase activity (119).
In a recent study, Kulcenty and colleagues published reports about the effects of IORT-seroma on BC cells. To evaluate the marker expression related to extrinsic and intrinsic apoptosis pathways, they incubated MCF-7 cell lines with IORT-seroma and non-IORT-seroma from BC patients for 4 days. Their result indicated the activation of the extrinsic apoptosis pathway by IORT-seroma (120). To clarify bystander effects of IORT-seroma on BC cells, they incubated MDA-MB-468 and MCF-7 cell lines with non-IORT-seroma, IORT-seroma from 16 patients, and conditioned media (CM) from irradiated cells. They measured the level of apoptosis induction, the rate of breaks in double-strand DNA, and the alterations in DNA repair-associated gene expression. They found that despite the induction by non-IORT-seroma, the induction by IORT-seroma and non-IORT-seroma+CM stimulated the double-strand breaks and enhanced the expression of DNA repair-associated genes (121). They incubated MDA-MB-468 and MCF-7 cell lines with non-IORT-seroma and IORT-seroma to determine the underlying mechanisms leading to the reduced tumorigenic potential of IORT-seroma and confirm its effect on the activation of bystander effects in cell lines. The phenotype modification of CSCs in the EMT process was investigated to determine the inductive migration effect of seroma on BC cells. Their results showed that seroma triggers the phenotype of CSC and EMT process in BC cell lines; however, its impact was partly questioned when incubated with IORT-seroma. In addition, the radiation-stimulated bystander effect’s role in changing WF properties to persuade the EMT process and CSC phenotype formation was confirmed (122). To compare the biological effects of seroma and IORT-seroma on non-irradiated neighbors of the cancer cells (bystander effects), the MDA-MB-468 cell line was treated with non-IORT-seroma, IORT-seroma, and CM derived from irradiated cells. Then, the microarray analysis was carried out. The analysis showed that IORT-seroma and non-IORT-seroma+RIBE groups have a similar effect on the same biological processes, such as enhancing cell-cycle regulation, oxidative phosphorylation, and DNA repair. The non-IORT-seroma group has its effect through over-activation of the involved pathways on the inflammatory response, INF-α and INF-γ response, and the signaling pathway of IL6 JAK/STAT3. These results showed that MDA-MB-468 cells induced by IORT-seroma and cells stimulated with non-IORT-seroma plus RIBE share common biological processes (123). A recent study on IORT-seroma and non-IORT-seroma on MDA-MB-231 and mesenchymal stromal cells clarified that seroma from IORT-treated patients affected the MSC behavior and modified the secretome of these cells. After 34h, IORT-seroma inhibits the proliferation of the MSCs with a similar method and kinetics related to the MSC’s doubling time (30–40 h). Overall, these studies provide the results that IORT alters the factor composition of seroma, which decreases the proliferation of the MSCs, the capacity of wound healing, and the activity of chemotactic migration.
Moreover, analysis of MSCs-CM cultured in 0.5% IORT- and control-seroma and collected after 72h showed significantly decreased RANTES, GRO-α, and VEGF in the IORT-seroma group (112). To confirm the tumor inhibitory effects of IORT-seroma compared with non-IORT-seroma, we evaluated migration, proliferation, viability, and invasion in three BC cell lines. The viability and proliferation results clarified that MDA-MB-231 cells benefit more than SKBR-3 and MCF-7 from the anticancer effects of IORT-seroma. The findings of the clonal survival assay in MCF-7 cells showed that the number of colonies was reduced in IORT-seroma-treated cells compared with the other groups. IORT-seroma-treated and non-IORT-seroma-treated cells showed no significant change in expression levels of proteins associated with cell cycle arrest (P16, P21) and the expression level of Caspase 3. Furthermore, our results confirmed the previous findings about tumor progressive effects of seroma on these three BC cell lines (124). Figure 5 presents the studies related to the effects of seroma on BC cell lines.
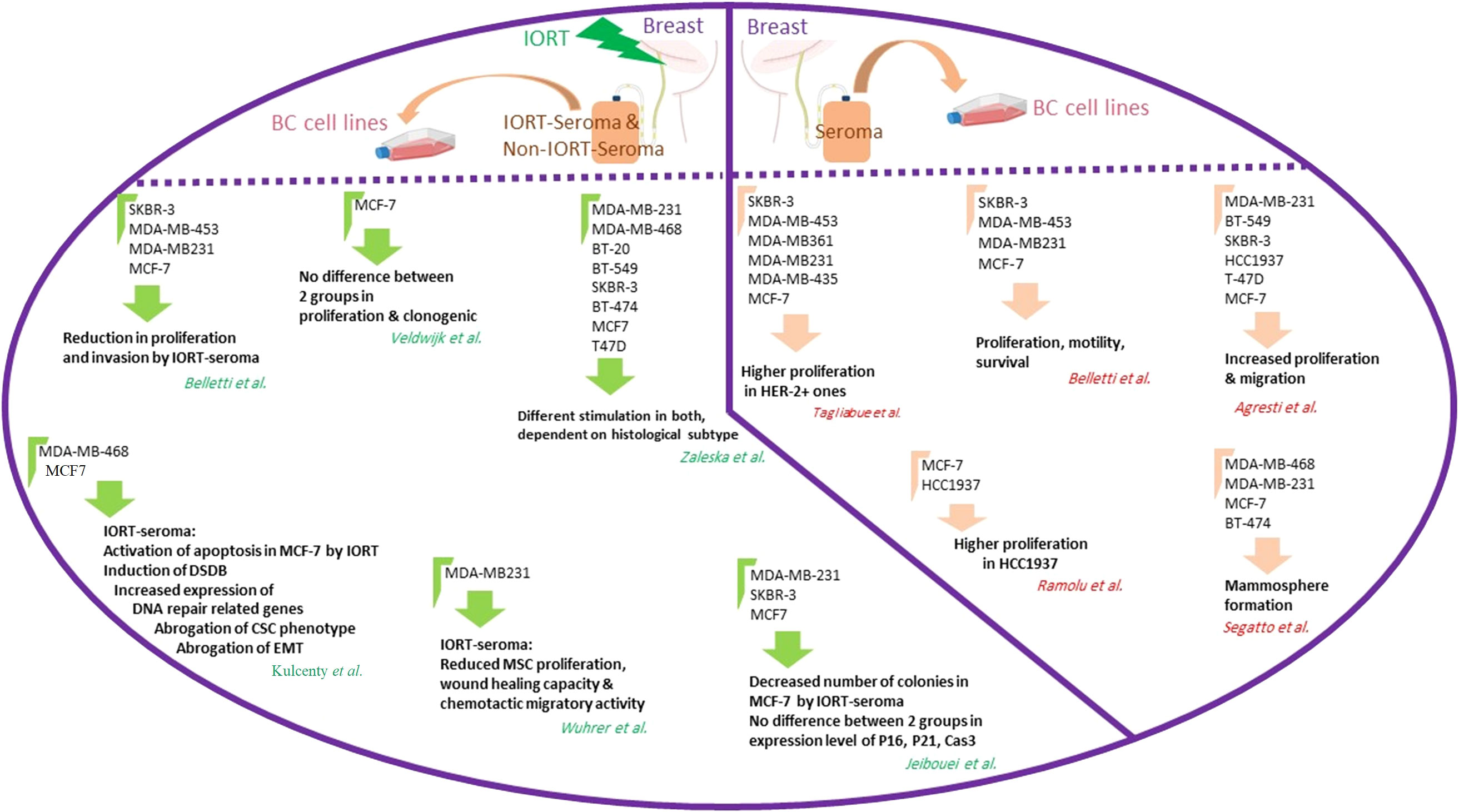
Figure 5 The studies related to the effects of seroma on BC cell lines. The right part of the circle shows the results from the effects of breast cancer seroma on breast cancer cell lines. The left part of the circle shows the results of the effects of IORT-treated and non-IORT-treated breast cancer seroma on breast cancer cell lines.
1.3.3 Effects of seroma on breast cancer primary cells; IORT vs non-IORT
Most data indicate post-surgery seroma strongly induces proliferative and aggressive phenotypes in BC cell lines. To achieve more reliable results, these findings in vivo outcomes are required. Zhang et al. cultured primary cells from BC cells with or without seroma and then treated the cells with different anticancer drugs. Generally, a remarkable enhancement in survival rates was observed in the seroma-treated cells compared to the non-treated cells among different subgroups of the various anticancer drugs. The BC cells treated with seroma collected from premenopausal patients displayed a significantly higher rate of survival compared to those of the control group in all anticancer drugs. Finally, seroma-treated primary BC cells reported higher resistance to chemotherapy drugs (125). To mimic the tumor’s in vivo microenvironment and re-evaluate previous in vitro effects of seroma on breast tumor cells, we designed a 3D model using human-derived specimens. Spheroids from 23 breast tumors were cultured in the collagen matrix in microfluidic devices. Spheroids derived from each patient were treated for six days with the 24h seroma collected from the patients. Final data from fluorescent live/dead staining on day 6 showed that in 22 samples, the percentage of live cells was significantly higher in seroma-treated samples compared to cells treated with Roswell Park Memorial Institute (RPMI) (as a control for each sample) (124).
Interestingly, one sample displayed the opposite result. We concluded that, however, most BC patients take advantage of removing seroma, the effects of seroma on tumor progression may not show a similar effect in all patients, and it can depend on many unknown factors (126). In another study, we assessed the radiobiological impact of IORT-seroma on human-derived specimens in a 3D model mentioned above. No significant difference in the percentage of live cells was observed between IORT-seroma-treated specimens with non-IORT-seroma-treated specimens after six days of treatment. The caspase 3 and E-cadherin expression levels in these specimens showed that despite similar caspase 3 in both groups, IORT-seroma-treated spheroids showed a higher level of E-cadherin compared to non-IORT-seroma-treated spheroids. It is worth noting that in both IORT-seroma and non-IORT-seroma groups, the expression levels of both E-cadherin and Caspase 3 were significantly higher in seroma-treated spheroids compared to RPMI-treated spheroids (as control). This study suggested IORT-seroma as a fluid containing inhibitory factors for tumor migration in the microfluidic system (124). Also, we showed increased proliferative and migrative characteristics of spheroids from four BC patients under IORT-seroma treatment using time-lapse imaging (127). Figure 6 presents studies on seroma’s effects on primary BC cells.
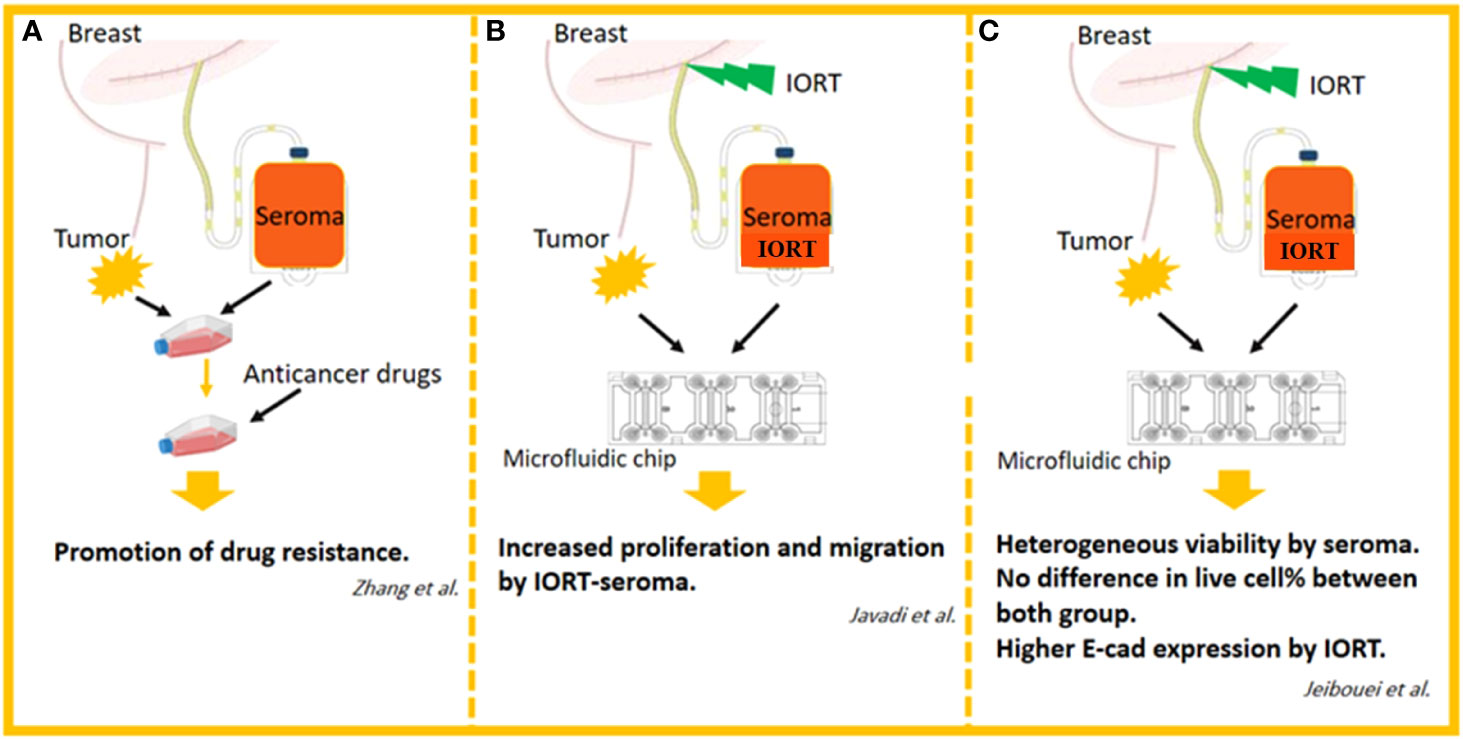
Figure 6 The studies related to the effects of seroma on primary BC cells. (A) Effects of drugs on seroma-treated human-derived breast cancer cells in 2D cell culture system. (B) Effects of IORT-treated and non-IORT-treated seroma on human-derived breast tumor spheroids in 3D microfluidic chips (visual evaluation of proliferation and migration). (C) Effects of IORT-treated and non-IORT-treated seroma on human-derived breast tumor spheroids in 3D microfluidic chips (visual and molecular evaluation of proliferation, apoptosis, migration, and heterogeneity).
2 Conclusion
Suction drainage placement after BCS is popular to prevent seroma formation in BC cases. However, it has some distinct drawbacks, such as an infection caused by the retrograde entry of skin bacteria through the drain, patient discomfort due to drain placement, and a need for daily nursing at home. Moreover, policies of drain removal are broadly different across various BC centers. Several studies have explored the safety of early drain removal according to multiple clinical endpoints. Studies revealed that seroma acts as a stimulative factor in tumor development through its interaction with cytokines, chemokines, and MMPs. According to data indicating beneficial direct and indirect effects of IORT on BC patients, some researchers assumed that IORT-induced seroma might mediate a part of these therapeutic effects of IORT. However, many studies such as analysis of IORT-seroma composition, treatment of BC cell lines and human tumor tissues, and assessment of their behavior under treatment of the collected seroma in 2D and 3D systems revealed that IORT-seroma has the same results as non-IORT-seroma in tumor cavity after the surgery.
The tumor heterogeneity could be a role player in the effectiveness of seroma on tumor behavior. Furthermore, in a 3D microfluidic study, we observed that heterogeneity of tumor and seroma have different effects in different patients. Our proteomic and transcriptomic data from tumor bed analysis also showed that IORT could affect tumor bed and probably remain cancer cells in tumor margins through immune system infiltration. Overall, evidence indicates that studies on seroma or IORT could not discover their mechanisms of tumor inhibition because of the variation in body reactions of patients. It seems that it is related to the immune system and probably unknown or unstudied factors in this area, such as microbiota in the body of patients. Deciphering mechanisms associated with immune system infiltration and abscopal effects consider personalized medicine by using profiling to address the questions about the inhibiting effects of IORT. In conclusion, we cannot provide a rationale for preserving or removing seroma in IORT-treated BC patients. The question of whether IORT-seroma has a beneficial effect can only be answered in a trial with a clinical endpoint, which needs to be investigated.
Author contributions
The concept of the manuscript was conceived by MA and HZ. SJ drafted the manuscript, and the other authors made direct intellectual and substantial contributions to the work, and accepted the manuscript for publication. All authors contributed to the article and approved the submitted version.
Acknowledgments
Authors thank Dr. Ebrahim Mostafavi for his unconditional support. Authors apologize to the scientists whose helps to IORT and personalized medicine in breast cancer could not be acknowledged because of space limitations.
Conflict of interest
The authors declare that the research was conducted in the absence of any commercial or financial relationships that could be construed as a potential conflict of interest.
Publisher’s note
All claims expressed in this article are solely those of the authors and do not necessarily represent those of their affiliated organizations, or those of the publisher, the editors and the reviewers. Any product that may be evaluated in this article, or claim that may be made by its manufacturer, is not guaranteed or endorsed by the publisher.
References
1. Fitzmaurice C, Abate D, Abbasi N. Global burden of disease cancer collaboration. global, regional, and national cancer incidence, mortality, years of life lost, years lived with disability, and disability-adjusted life-years for 29 cancer groups, 1990to 2017: A systemic analysis for the global burden of disease study (vol 5, pg 1749, 2019). JAMA Oncol (2020) 6(3):444–4. doi: 10.1001/jamaoncol.2019.2996
2. Sung H, Ferlay J, Siegel RL, Laversanne M, Soerjomataram I, Jemal A, et al. Global cancer statistics 2020: GLOBOCAN estimates of incidence and mortality worldwide for 36 cancers in 185 countries. CA: Cancer J Clin (2021) 71(3):209–49. doi: 10.3322/caac.21660
3. Fisher B, Anderson S, Bryant J, Margolese RG, Deutsch M, Fisher ER, et al. Twenty-year follow-up of a randomized trial comparing total mastectomy, lumpectomy, and lumpectomy plus irradiation for the treatment of invasive breast cancer. New Engl J Med (2002) 347(16):1233–41. doi: 10.1056/NEJMoa022152
4. Vaidya JS, Bulsara M, Baum M, Wenz F, Massarut S, Pigorsch S, et al. Long term survival and local control outcomes from single dose targeted intraoperative radiotherapy during lumpectomy (TARGIT-IORT) for early breast cancer: TARGIT-a randomised clinical trial. Bmj (2020) 370:m2836. doi: 10.1136/bmj.m2836
5. Waks AG, Winer EP. Breast cancer treatment: a review. Jama (2019) 321(3):288–300. doi: 10.1001/jama.2018.19323
6. Ruano-Ravina A, Cantero-Munoz P, Urién AE. Efficacy and safety of intraoperative radiotherapy in breast cancer: A systematic review. Cancer Lett (2011) 313(1):15–25. doi: 10.1016/j.canlet.2011.08.020
7. Harris EE, Small W Jr. Intraoperative radiotherapy for breast cancer. Front Oncol (2017) 7:317. doi: 10.3389/fonc.2017.00317
8. Veronesi U, Orecchia R, Maisonneuve P, Viale G, Rotmensz N, Sangalli C, et al. Intraoperative radiotherapy versus external radiotherapy for early breast cancer (ELIOT): A randomised controlled equivalence trial. Lancet Oncol (2013) 14(13):1269–77. doi: 10.1016/S1470-2045(13)70497-2
9. Vaidya JS, Wenz F, Bulsara M, Tobias JS, Joseph DJ, Keshtgar M, et al. Risk-adapted targeted intraoperative radiotherapy versus whole-breast radiotherapy for breast cancer: 5-year results for local control and overall survival from the TARGIT-a randomised trial. Lancet (2014) 383(9917):603–13. doi: 10.1016/S0140-6736(13)61950-9
10. Vaidya JS, Bulsara M, Baum M, Wenz F, Massarut S, Pigorsch S, et al. New clinical and biological insights from the international TARGIT-a randomised trial of targeted intraoperative radiotherapy during lumpectomy for breast cancer. Br J Cancer (2021) 125(3):380–9. doi: 10.1038/s41416-021-01440-8
11. Veronesi U, Orecchia R, Luini A, Galimberti V, Gatti G, Intra M, et al. Full-dose intra-operative radiotherapy with electrons (ELIOT) during breast-conserving surgery: experience with 1246 cases. ecancermedicalscience (2008) 242(1):101–6. doi: 10.3332/eCMS.2008.65
12. Belletti B, Vaidya JS, D'Andrea S, Entschladen F, Roncadin M, Lovat F, et al. Targeted intraoperative radiotherapy impairs the stimulation of breast cancer cell proliferation and invasion caused by surgical wounding. Clin Cancer Res (2008) 14(5):1325–32. doi: 10.1158/1078-0432.CCR-07-4453
13. Herskind C, Wenz F. Radiobiological aspects of intraoperative tumour-bed irradiation with low-energy X-rays (LEX-IORT). Transl Cancer Res (2014) 3(1):3–17. doi: 10.1667/rr3292
14. Kurtz JM, Amalric R, Brandone H, Ayme Y, Jacquemier J, Pietra JC, et al. Local recurrence after breast-conserving surgery and radiotherapy. frequency, time course, and prognosis. Cancer (1989) 63(10):1912–7. doi: 10.1002/1097-0142(19890515)63:10<1912
15. Veronesi U, Luini A, Del Vecchio M, Greco M, Galimberti V, Merson M, et al. Radiotherapy after breast-preserving surgery in women with localized cancer of the breast. New Engl J Med (1993) 328(22):1587–91. doi: 10.1056/NEJM199306033282202
16. Ahmad A. Pathways to breast cancer recurrence. Int Sch Res Not (2013) 2013:290568. doi: 10.1155/2013/290568
17. Turashvili G, Brogi E. Tumor heterogeneity in breast cancer. Front Med (2017) 4:227. doi: 10.3389/fmed.2017.00227
18. Martelotto LG, Ng CK, Piscuoglio S, Weigelt B, Reis-Filho JS. Breast cancer intra-tumor heterogeneity. Breast Cancer Res (2014) 16(3):1–11. doi: 10.1186/bcr3658
19. Jeibouei S, Akbari ME, Kalbasi A, Aref AR, Ajoudanian M, Rezvani A, et al. Personalized medicine in breast cancer: pharmacogenomics approaches. Pharmacogenomics person Med (2019) 12:59. doi: 10.2147/PGPM.S167886
20. Clarke MF, Dick JE, Dirks PB, Eaves CJ, Jamieson CH, Jones DL, et al. Cancer stem cells–perspectives on current status and future directions: AACR workshop on cancer stem cells. Cancer Res (2006) 66(19):9339–44. doi: 10.1158/0008-5472.CAN-06-3126
21. Oskarsson T, Batlle E, Massagué J. Metastatic stem cells: sources, niches, and vital pathways. Cell Stem Cell (2014) 14(3):306–21. doi: 10.1016/j.stem.2014.02.002
22. Ye J, Wu D, Wu P, Chen Z, Huang J. The cancer stem cell niche: cross talk between cancer stem cells and their microenvironment. Tumor Biol (2014) 35(5):3945–51. doi: 10.1007/s13277-013-1561-x
23. Shiga K, Hara M, Nagasaki T, Sato T, Takahashi H, Takeyama H. Cancer-associated fibroblasts: their characteristics and their roles in tumor growth. Cancers (2015) 7(4):2443–58. doi: 10.3390/cancers7040902
24. Iyengar P, Combs TP, Shah SJ, Gouon-Evans V, Pollard JW, Albanese C, et al. Adipocyte-secreted factors synergistically promote mammary tumorigenesis through induction of anti-apoptotic transcriptional programs and proto-oncogene stabilization. Oncogene (2003) 22(41):6408–23. doi: 10.1038/sj.onc.1206737
25. Tan J, Buache E, Chenard M-P, Dali-Youcef N, Rio M-C. Adipocyte is a non-trivial, dynamic partner of breast cancer cells. Int J Dev Biol (2011) 55(7-8-9):851–9. doi: 10.1387/ijdb.113365jt
26. DeNardo DG, Andreu P, Coussens LM. Interactions between lymphocytes and myeloid cells regulate pro-versus anti-tumor immunity. Cancer Metastasis Rev (2010) 29(2):309–16. doi: 10.1007/s10555-010-9223-6
27. Mantovani A, Sozzani S, Locati M, Allavena P, Sica A. Macrophage polarization: tumor-associated macrophages as a paradigm for polarized M2 mononuclear phagocytes. Trends Immunol (2002) 23(11):549–55. doi: 10.1016/S1471-4906(02)02302-5
28. Roland CL, Dineen SP, Lynn KD, Sullivan LA, Dellinger MT, Sadegh L, et al. Inhibition of vascular endothelial growth factor reduces angiogenesis and modulates immune cell infiltration of orthotopic breast cancer xenografts. Mol Cancer Ther (2009) 8(7):1761–71. doi: 10.1158/1535-7163.MCT-09-0280
29. Arnold JN, Magiera L, Kraman M, Fearon DT. Tumoral immune suppression by macrophages expressing fibroblast activation protein-α and heme oxygenase-1. Cancer Immunol Res (2014) 2(2):121–6. doi: 10.1158/2326-6066.CIR-13-0150
30. Liang X, Fu C, Cui W, Ober‐Blöbaum JL, Zahner SP, Shrikant PA, et al. β-catenin mediates tumor-induced immunosuppression by inhibiting cross-priming of CD8+ T cells. J leukocyte Biol (2014) 95(1):179–90. doi: 10.1189/jlb.0613330
31. Spiegel A, Brooks MW, Houshyar S, Reinhardt F, Ardolino M, Fessler E, et al. Neutrophils suppress intraluminal NK cell–mediated tumor cell clearance and enhance extravasation of disseminated carcinoma cells. Cancer Discovery (2016) 6(6):630–49. doi: 10.1158/2159-8290.CD-15-1157
32. Mattner J, Wirtz S. Friend or foe? the ambiguous role of innate lymphoid cells in cancer development. Trends Immunol (2017) 38(1):29–38. doi: 10.1016/j.it.2016.10.004
33. Zamarron BF, Chen W. Dual roles of immune cells and their factors in cancer development and progression. Int J Biol Sci (2011) 7(5):651. doi: 10.7150/ijbs.7.651
34. Kim J, Bae J-S. Tumor-associated macrophages and neutrophils in tumor microenvironment. Mediators Inflammation (2016) 2016:6058147. doi: 10.1155/2016/6058147
35. Murdocca M, Torino F, Pucci S, Costantini M, Capuano R, Greggi C, et al. Urine LOX-1 and volatilome as promising tools towards the early detection of renal cancer. Cancers (2021) 13(16):4213. doi: 10.3390/cancers13164213
36. Wenz F, Welzel G, Blank E, Hermann B, Steil V, Sütterlin M, et al. Intraoperative radiotherapy as a boost during breast-conserving surgery using low-kilovoltage X-rays: The first 5 years of experience with a novel approach. Int J Radiat Oncol Biol Phys (2010) 77(5):1309–14. doi: 10.1016/j.ijrobp.2009.06.085
37. Vaidya J, Baum M, Tobias J, Massarut S, Wenz F, Hilaris B, et al. Targeted intraoperative radiotherapy (Targit) boost after breast conserving surgery results in a remarkably low recurrence rate in a standard risk population: 5 year results. AACR (2009) 69(24):4104. doi: 10.1158/0008-5472.SABCS-09-4104
38. Kraus-Tiefenbacher U, Bauer L, Scheda A, Fleckenstein K, Keller A, Herskind C, et al. Long-term toxicity of an intraoperative radiotherapy boost using low energy X-rays during breast-conserving surgery. Int J Radiat Oncol Biol Phys (2006) 66(2):377–81. doi: 10.1016/j.ijrobp.2006.05.042
39. Pez M, Keller A, Welzel G, Abo-Madyan Y, Ehmann M, Tuschy B, et al. Long-term outcome after intraoperative radiotherapy as a boost in breast cancer. Strahlentherapie und Onkologie (2020) 196(4):349–55. doi: 10.1007/s00066-019-01525-7
40. Ghardi M, Moreels M, Chatelain B, Chatelain C, Baatout S. Radiation-induced double strand breaks and subsequent apoptotic DNA fragmentation in human peripheral blood mononuclear cells. Int J Mol Med (2012) 29(5):769–80. doi: 10.3892/ijmm.2012.907
41. Minafra L, Bravatà V. Cell and molecular response to IORT treatment. Transl Cancer Res (2014) 3(1):32–47. doi: 10.3978/j.issn.2218-676X.2014.02.03
42. Huber SM, Butz L, Stegen B, Klumpp D, Braun N, Ruth P, et al. Ionizing radiation, ion transports, and radioresistance of cancer cells. Front Physiol (2013) 4:212. doi: 10.3389/fphys.2013.00212
43. Uhlig S, Wuhrer A, Berlit S, Tuschy B, Sütterlin M, Bieback K. Intraoperative radiotherapy for breast cancer treatment efficiently targets the tumor bed preventing breast adipose stromal cell outgrowth. Strahlentherapie und Onkologie (2020) 196(4):398–404. doi: 10.1007/s00066-020-01586-z
44. Fabris L, Berton S, Citron F, D'andrea S, Segatto I, Nicoloso M, et al. Radiotherapy-induced miR-223 prevents relapse of breast cancer by targeting the EGF pathway. Oncogene (2016) 35(37):4914–26. doi: 10.1038/onc.2016.23
45. Miricescu D, Totan A, Stanescu-Spinu I-I, Badoiu SC, Stefani C, Greabu M. PI3K/AKT/mTOR signaling pathway in breast cancer: From molecular landscape to clinical aspects. Int J Mol Sci (2020) 22(1):173. doi: 10.3390/ijms22010173
46. Yu C-C, Hung S-K, Lin H-Y, Chiou W-Y, Lee M-S, Liao H-F, et al. Targeting the PI3K/AKT/mTOR signaling pathway as an effectively radiosensitizing strategy for treating human oral squamous cell carcinoma in vitro and in vivo. Oncotarget (2017) 8(40):68641. doi: 10.18632/oncotarget.19817
47. Shima H, Kutomi G, Sato K, Kuga Y, Wada A, Satomi F, et al. An optimal timing for removing a drain after breast surgery: A systematic review and meta-analysis. J Surg Res (2021) 267:267–73. doi: 10.1016/j.jss.2021.05.031
48. Vander Heiden MG, DeBerardinis RJ. Understanding the intersections between metabolism and cancer biology. Cell (2017) 168(4):657–69. doi: 10.1016/j.cell.2016.12.039
49. Warburg O, Wind F, Negelein E. The metabolism of tumors in the body. J Gen Physiol (1927) 8(6):519. doi: 10.1085/jgp.8.6.519
50. Jiang W, Chan CK, Weissman IL, Kim BY, Hahn SM. Immune priming of the tumor microenvironment by radiation. Trends Cancer (2016) 2(11):638–45. doi: 10.1016/j.trecan.2016.09.007
51. Surova O, Zhivotovsky B. Various modes of cell death induced by DNA damage. Oncogene (2013) 32(33):3789–97. doi: 10.1038/onc.2012.556
52. Jason CY, Formenti SC. Integration of radiation and immunotherapy in breast cancer-treatment implications. Breast (2018) 38:66–74. doi: 10.1016/j.breast.2017.12.005
53. Hall EJ. Radiation biology for pediatric radiologists. Pediatr Radiol (2009) 39(1):57–64. doi: 10.1007/s00247-008-1027-2
54. Zhao W, Diz D, Robbins M. Oxidative damage pathways in relation to normal tissue injury. Br J Radiol (2007) 80(special_issue_1):S23–31. doi: 10.1259/bjr/18237646
55. Lomax M, Folkes L, O'neill P. Biological consequences of radiation-induced DNA damage: relevance to radiotherapy. Clin Oncol (2013) 25(10):578–85. doi: 10.1016/j.clon.2013.06.007
56. Mladenov E, Magin S, Soni A, Iliakis G. DNA Double-strand break repair as determinant of cellular radiosensitivity to killing and target in radiation therapy. Front Oncol (2013) 3:113. doi: 10.3389/fonc.2013.00113
57. Golden E, Pellicciotta I, Demaria S, Barcellos-Hoff MH, Formenti SC. The convergence of radiation and immunogenic cell death signaling pathways. Front Oncol (2012) 2:88. doi: 10.3389/fonc.2012.00088
58. Multhoff G, Radons J. Radiation, inflammation, and immune responses in cancer. Front Oncol (2012) 2:58. doi: 10.3389/fonc.2012.00058
59. Kuribayashi K, Finnberg NK, Jeffers JR, Zambetti GP, El-Deiry WS. The relative contribution of pro-apoptotic p53-target genes in the triggering of apoptosis following DNA damage in vitro and in vivo. Cell Cycle (2011) 10(14):2380–9. doi: 10.4161/cc.10.14.16588
60. Pant V, Xiong S, Jackson JG, Post SM, Abbas HA, Quintás-Cardama A, et al. The p53–Mdm2 feedback loop protects against DNA damage by inhibiting p53 activity but is dispensable for p53 stability, development, and longevity. Genes Dev (2013) 27(17):1857–67. doi: 10.1101/gad.227249.113
61. Chipuk JE, Bouchier-Hayes L, Kuwana T, Newmeyer DD, Green DR. PUMA couples the nuclear and cytoplasmic proapoptotic function of p53. Science (2005) 309(5741):1732–5. doi: 10.1126/science.1114297
62. Panganiban RAM, Mungunsukh O, Day RM. X-Irradiation induces ER stress, apoptosis, and senescence in pulmonary artery endothelial cells. Int J Radiat Biol (2013) 89(8):656–67. doi: 10.3109/09553002.2012.711502
63. Han Y, Wang Y, Xu H-T, Yang L-H, Wei Q, Liu Y, et al. X-Radiation induces non-small-cell lung cancer apoptosis by upregulation of axin expression. Int J Radiat Oncol Biol Phys (2009) 75(2):518–26. doi: 10.1016/j.ijrobp.2009.05.040
64. Kolesnick R, Fuks Z. Radiation and ceramide-induced apoptosis. Oncogene (2003) 22(37):5897–906. doi: 10.1038/sj.onc.1206702
65. Mullins ME, Barest GD, Schaefer PW, Hochberg FH, Gonzalez RG, Lev MH. Radiation necrosis versus glioma recurrence: Conventional MR imaging clues to diagnosis. Am J Neuroradiol (2005) 26(8):1967–72.
66. Jella KK, Garcia A, McClean B, Byrne HJ, Lyng FM. Cell death pathways in directly irradiated cells and cells exposed to medium from irradiated cells. Int J Radiat Biol (2013) 89(3):182–90. doi: 10.3109/09553002.2013.734942
67. Campisi J. Aging, cellular senescence, and cancer. Annu Rev Physiol (2013) 75:685. doi: 10.1146/annurev-physiol-030212-183653
68. Muller M. Cellular senescence: molecular mechanisms, in vivo significance, and redox considerations. Antioxid Redox Signaling (2009) 11(1):59–98. doi: 10.1089/ars.2008.2104
69. Tchkonia T, Zhu Y, Van Deursen J, Campisi J, Kirkland JL, et al. Cellular senescence and the senescent secretory phenotype: therapeutic opportunities. J Clin Invest (2013) 123(3):966–72. doi: 10.1172/JCI64098
70. Tominaga K, Pereira-Smith OM. The role of chromatin reorganization in the process of cellular senescence. Curr Drug Targets (2012) 13(13):1593–602. doi: 10.2174/138945012803529983
71. Muñoz-Espín D, Serrano M. Cellular senescence: from physiology to pathology. Nat Rev Mol Cell Biol (2014) 15(7):482–96. doi: 10.1038/nrm3823
72. Fumagalli M, Rossiello F, Mondello C, d’Adda di Fagagna F. Stable cellular senescence is associated with persistent DDR activation. PloS One (2014) 9(10):e110969. doi: 10.1371/journal.pone.0110969
73. Young AR, Narita M. SASP reflects senescence. EMBO Rep (2009) 10(3):228–30. doi: 10.1038/embor.2009.22
74. Coppé J-P, Desprez P-Y, Krtolica A, Campisi J. The senescence-associated secretory phenotype: the dark side of tumor suppression. Annu Rev Pathol (2010) 5:99. doi: 10.1146/annurev-pathol-121808-102144
75. Davalos AR, Coppe J-P, Campisi J, Desprez P-Y. Senescent cells as a source of inflammatory factors for tumor progression. Cancer Metastasis Rev (2010) 29(2):273–83. doi: 10.1007/s10555-010-9220-9
76. Denton D, Nicolson S, Kumar S. Cell death by autophagy: facts and apparent artefacts. Cell Death Differ (2012) 19(1):87–95. doi: 10.1038/cdd.2011.146
77. Rodriguez-Rocha H, Garcia-Garcia A, Panayiotidis MI, Franco R. DNA Damage and autophagy. Mutat Res/Fundam Mol Mech Mutagen (2011) 711(1-2):158–66. doi: 10.1016/j.mrfmmm.2011.03.007
78. Palumbo S, Comincini S. Autophagy and ionizing radiation in tumors: the “survive or not survive” dilemma. J Cell Physiol (2013) 228(1):1–8. doi: 10.1002/jcp.24118
79. Castedo M, Perfettini J-L, Roumier T, Andreau K, Medema R, Kroemer G. Cell death by mitotic catastrophe: A molecular definition. Oncogene (2004) 23(16):2825–37. doi: 10.1038/sj.onc.1207528
80. Desgrosellier JS, Cheresh DA. Integrins in cancer: biological implications and therapeutic opportunities. Nat Rev Cancer (2010) 10(1):9–22. doi: 10.1038/nrc2748
81. Hamidi H, Ivaska J. Every step of the way: integrins in cancer progression and metastasis. Nat Rev Cancer (2018) 18(9):533–48. doi: 10.1038/s41568-018-0038-z
82. Shahani M, Shakeri J, Akbari ME, Arefnezhad B, Tafti A, Zali H, et al. Transcriptomic and proteomic approaches reveal biological basis of intraoperative radiotherapy-treated tumor bed modification in breast cancer patients: A pilot study. J Proteomics (2020) 212:103596. doi: 10.1016/j.jprot.2019.103596
83. Bravatà V, Minafra L, Cammarata FP, Pisciotta P, Lamia D, Marchese V, et al. Gene expression profiling of breast cancer cell lines treated with proton and electron radiations. Br J Radiol (2018) 91(1089):20170934. doi: 10.1259/bjr.20170934
84. Welsh J, Bevelacqua J, Dobrzyński L, Mortazavi S. Abscopal effect following radiation therapy in cancer patients: A new look from the immunological point of view. J Biomed Phys Eng (2020) 10(4):537. doi: 10.31661/jbpe.v0i0.1066
85. Van de Walle M, Demol J, Staelens L, Rottey S. Abscopal effect in metastatic renal cell carcinoma. Acta Clin Belg (2017) 72(4):245–9. doi: 10.1080/17843286.2016.1201614
86. Hu ZI, McArthur HL, Ho AY. The abscopal effect of radiation therapy: what is it and how can we use it in breast cancer? Curr Breast Cancer Rep (2017) 9(1):45–51. doi: 10.1007/s12609-017-0234-y
87. Azami A, Suzuki N, Azami Y, Seto I, Sato A, Takano Y, et al. Abscopal effect following radiation monotherapy in breast cancer: A case report. Mol Clin Oncol (2018) 9(3):283–6. doi: 10.3892/mco.2018.1677
88. Farias VdA, Tovar I, Del Moral R, O'Valle F, Expósito J, Oliver FJ, et al. Enhancing the bystander and abscopal effects to improve radiotherapy outcomes. Front Oncol (2020) 9:1381. doi: 10.3389/fonc.2019.01381
89. Formenti SC, Lee P, Adams S, Goldberg JD, Li X, Xie MW, et al. Focal irradiation and systemic TGFβ blockade in metastatic breast cancer. Clin Cancer Res (2018) 24(11):2493–504. doi: 10.1158/1078-0432.CCR-17-3322
90. Jafari Nedooshan J, Dashti Rahmatabadi MH, Kargar S, Lotfi MH. Evaluation of complications of short-term and long-term drainage following mastectomy with removal of axillary lymph nodes: A randomized clinical trial. Asian Pac J Cancer Prev (2022) 23(8):2873–8. doi: 10.31557/APJCP.2022.23.8.2873
91. Wen N, Ouyang C, Hu X, Hou L, He L, Liu C, et al. What is the optimal strategy for drain removal after mastectomy and axillary surgery in breast cancer patients? a multicenter, three-arm randomized clinical trial. J Surg Res (2022) 277:148–56. doi: 10.1016/j.jss.2022.02.034
92. Khambri D, Harahap WA. Association of early drain removal with formation of seroma in breast cancer patients after modified radical mastectomy. Biosci Med: J Biomed Trans Res (2022) 6(7):1989–93. doi: 10.37275/bsm.v6i7.544
93. Schmidt G, Zuschlag M, Gerlinger C, Endrikat J, Müller C, Gabriel L, et al. Efficacy and safety of two post-operative drains: Results of a prospectively randomized clinical study in breast cancer patients after breast conserving surgery. Arch Gynecol Obstet (2019) 300(6):1687–92. doi: 10.1007/s00404-019-05360-0
94. Isozaki H, Yamamoto Y, Murakami S, Matsumoto S, Takama T. Impact of the surgical modality for axillary lymph node dissection on postoperative drainage and seroma formation after total mastectomy. Patient Saf Surg (2019) 13:20. doi: 10.1186/s13037-019-0199-z
95. Ten Wolde B, Strobbe FFR, Schlooz-de Vries M, van den Wildenberg FJH, Keemers-Gels M, de Wilt JHW, et al. Omitting postoperative wound drainage after mastectomy with skin-flap quilting. Ann Surg Oncol (2019) 26(9):2773–8. doi: 10.1245/s10434-019-07411-x
96. Vos H, Smeets A, Neven P, Laenen A, Vandezande L, Nevelsteen I. Early drain removal improves quality of life and clinical outcomes in patients with breast cancer - results from a randomised controlled trial. Eur J Oncol Nurs (2018) 36:112–8. doi: 10.1016/j.ejon.2018.08.007
97. Okada N, Narita Y, Takada M, Kato H, Ambo Y, Nakamura F, et al. Early removal of drains and the incidence of seroma after breast surgery. Breast Cancer (2015) 22(1):79–83. doi: 10.1007/s12282-013-0457-3
98. Clegg-Lamptey JN, Dakubo JC, Hodasi WM. Comparison of four-day and ten-day post-mastectomy passive drainage in Accra, Ghana. East Afr Med J (2007) 84(12):561–5. doi: 10.4314/eamj.v84i12.9592
99. Baas-Vrancken Peeters MJ, Kluit AB, Merkus JW, Breslau PJ. Short versus long-term postoperative drainage of the axilla after axillary lymph node dissection. a prospective randomized study. Breast Cancer Res Treat (2005) 93(3):271–5. doi: 10.1007/s10549-005-5348-7
100. Gupta R, Pate K, Varshney S, Goddard J, Royle GT. A comparison of 5-day and 8-day drainage following mastectomy and axillary clearance. Eur J Surg Oncol (2001) 27(1):26–30. doi: 10.1053/ejso.2000.1054
101. Ciabattoni A, Gregucci F, Llange K, Alessandro M, Corazzi F, Ivaldi GB, et al. Intra-operative electron radiation therapy (IOERT) anticipated boost in breast cancer treatment: An Italian multicenter experience. Cancers (2022) 14(2):292. doi: 10.3390/cancers14020292
102. Hu X, He Y, Chen X, Xia T, Cao T, Jia H, et al. Impact of intraoperative radiotherapy on the perioperative period of patients after breast-conserving surgery. Gland Surg (2020) 9(5):1535–42. doi: 10.21037/gs-20-727
103. Patas d’Illiers LB, Lafay F, Faure C, Klingler S, Pleynet E, Racadot S. Up to 5 years outcomes following intraoperative radiotherapy for early breast cancer. Trans Cancer Res (2018) 7(4):859–70. doi: 10.21037/tcr.2018.06.07
104. McCaul J, Aslaam A, Spooner R, Louden I, Cavanagh T, Purushotham A. Aetiology of seroma formation in patients undergoing surgery for breast cancer. Breast (2000) 9(3):144–8. doi: 10.1054/brst.1999.0126
105. Stuelten CH, Barbul A, Busch JI, Sutton E, Katz R, Sato M, et al. Acute wounds accelerate tumorigenesis by a T cell–dependent mechanism. Cancer Res (2008) 68(18):7278–82. doi: 10.1158/0008-5472.CAN-08-1842
106. Yussof SJM, Omar E, Pai DR, Sood S. Cellular events and biomarkers of wound healing. Indian J Plast Surg (2012) 45(02):220–8. doi: 10.4103/0970-0358.101282
107. Werner S, Grose R. Regulation of wound healing by growth factors and cytokines. Physiol Rev (2003) 83(3):835–70. doi: 10.1152/physrev.2003.83.3.835
108. Valeta-Magara A, Hatami R, Axelrod D, Roses DF, Guth A, Formenti S, et al. Pro-oncogenic cytokines and growth factors are differentially expressed in the post-surgical wound fluid from malignant compared to benign breast lesions. SpringerPlus (2015) 4(1):1–11. doi: 10.1186/s40064-015-1260-8
109. Agresti R, Triulzi T, Sasso M, Ghirelli C, Aiello P, Rybinska I, et al. Wound healing fluid reflects the inflammatory nature and aggressiveness of breast tumors. Cells (2019) 8(2):181. doi: 10.3390/cells8020181
110. Zhang Y, Ma QY, Dang CX, Moureau-Zabotto M, Chen WK. Quantitative molecular diagnosis of axillary drainage fluid for prediction of locoregional failure in patients with one to three positive axillary nodes after mastectomy without adjuvant radiotherapy. Int J Radiat Oncol Biol Phys (2006) 64(2):505–11. doi: 10.1016/j.ijrobp.2005.07.984
111. Kulcenty K, Piotrowski I, Wróblewska JP, Wasiewicz J, Suchorska WM. The composition of surgical wound fluids from breast cancer patients is affected by intraoperative radiotherapy treatment and depends on the molecular subtype of breast cancer. Cancers (2019) 12(1):11. doi: 10.3390/cancers12010011
112. Wuhrer A, Uhlig S, Tuschy B, Berlit S, Sperk E, Bieback K, et al. Wound fluid from breast cancer patients undergoing intraoperative radiotherapy exhibits an altered cytokine profile and impairs mesenchymal stromal cell function. Cancers (2021) 13(9):2140. doi: 10.3390/cancers13092140
113. Tagliabue E, Agresti R, Carcangiu ML, Ghirelli C, Morelli D, Campiglio M, et al. Role of HER2 in wound-induced breast carcinoma proliferation. Lancet (2003) 362(9383):527–33. doi: 10.1016/S0140-6736(03)14112-8
114. Segatto I, Berton S, Sonego M, Massarut S, D'Andrea S, Perin T, et al. Inhibition of breast cancer local relapse by targeting p70S6 kinase activity. J Mol Cell Biol (2013) 5(6):428–31. doi: 10.1093/jmcb/mjt027
115. Segatto I, Berton S, Sonego M, Massarut S, Fabris L, Armenia J, et al. p70S6 kinase mediates breast cancer cell survival in response to surgical wound fluid stimulation. Mol Oncol (2014) 8(3):766–80. doi: 10.1016/j.molonc.2014.02.006
116. Segatto I, Berton S, Sonego M, Massarut S, Perin T, Piccoli E, et al. Surgery-induced wound response promotes stem-like and tumor-initiating features of breast cancer cells, via STAT3 signaling. Oncotarget (2014) 5(15):6267. doi: 10.18632/oncotarget.2195
117. Ramolu L, Christ D, Abecassis J, Rodier J-F. Stimulation of breast cancer cell lines by post-surgical drainage fluids. Anticancer Res (2014) 34(7):3489–92.
118. Veldwijk MR, Neumaier C, Gerhardt A, Giordano FA, Sütterlin M, Herskind C, et al. Comparison of the proliferative and clonogenic growth capacity of wound fluid from breast cancer patients treated with and without intraoperative radiotherapy. Transl Cancer Res (2015) 4(2):173–7. doi: 10.3978/j.issn.2218-676X.2015.04.01
119. Zaleska K, Suchorska WM, Przybyła A, Murawa D. Effect of surgical wound fluids after intraoperative electron radiotherapy on the cancer stem cell phenotype in a panel of human breast cancer cell lines. Oncol Lett (2016) 12(5):3707–14. doi: 10.3892/ol.2016.5167
120. Kulcenty KI, Piotrowski I, Zaleska K, Murawa D, Suchorska WM. Wound fluids collected from patients after IORT treatment activates extrinsic apoptotic pathway in MCF7 breast cancer cell line. Ginekol Polska (2018) 89(4):175–82. doi: 10.5603/GP.a2018.0030
121. Piotrowski I, Kulcenty K, Murawa D, Suchorska W. Surgical wound fluids from patients treated with intraoperative radiotherapy induce radiobiological response in breast cancer cells. Med Oncol (2019) 36(2):1–12. doi: 10.1007/s12032-018-1243-z
122. Kulcenty K, Piotrowski I, Zaleska K, Wichtowski M, Wróblewska J, Murawa D, et al. Wound fluids collected postoperatively from patients with breast cancer induce epithelial to mesenchymal transition but intraoperative radiotherapy impairs this effect by activating the radiation-induced bystander effect. Sci Rep (2019) 9(1):1–11. doi: 10.1038/s41598-019-44412-y
123. Kulcenty K, Piotrowski I, Rucinski M, Wroblewska JP, Jopek K, Murawa D, et al. Surgical wound fluids from patients with breast cancer reveal similarities in the biological response induced by intraoperative radiation therapy and the radiation-induced bystander effect–transcriptomic approach. Int J Mol Sci (2020) 21(3):1159. doi: 10.3390/ijms21031159
124. Jeibouei S, Hojat A, Mostafavi E, Aref AR, Kalbasi A, Niazi V, et al. Radiobiological effects of wound fluid on breast cancer cell lines and human-derived tumor spheroids in 2D and microfluidic culture. Sci Rep (2022) 12(1):1–21. doi: 10.1038/s41598-022-11023-z
125. Zhang Y, Yan D, Zhang H, Ou X, Zhao Z, Wang D, et al. Influence of wound fluid on chemotherapy sensitivity in primary breast cancer cells. Oncotarget (2016) 7(40):65034. doi: 10.18632/oncotarget.11345
126. Hojat A, Jeibouei S, Aref AR, Kalbasi A, Moghaddam M, Mohammadi F, et al. Effects of wound fluid on breast cancer-derived spheroids in a 3D culture system: A case series study. Iranian J Pharm Res (2022) 21(1):e123828. doi: 10.5812/ijpr.123828
127. Javadi SM, Abdolahad M, Hashemi S, Khayamian M, Parizi MS, Vanaei S, et al. Effect of post IORT wound fluid secretion (PIWFS) on the behavior of breast cancer cells: Stimulator or inhibitor; report of an experimental study on breast cancer. Arch Iranian Med (2022) 25(2):78. doi: 10.34172/aim.2022.13
Keywords: breast cancer, IORT, seroma, personalized medicine, tumor microenvironment
Citation: Jeibouei S, Shams F, Mohebichamkhorami F, Sanooghi D, Faal B, Akbari ME and Zali H (2022) Biological and clinical review of IORT-induced wound fluid in breast cancer patients. Front. Oncol. 12:980513. doi: 10.3389/fonc.2022.980513
Received: 28 June 2022; Accepted: 19 October 2022;
Published: 21 November 2022.
Edited by:
San-Gang Wu, First Affiliated Hospital of Xiamen University, ChinaReviewed by:
Hans-Christian Kolberg, Marienhospital Bottrop, GermanyWiktoria Maria Suchorska, Greater Poland Cancer Center (GPCC), Poland
Copyright © 2022 Jeibouei, Shams, Mohebichamkhorami, Sanooghi, Faal, Akbari and Zali. This is an open-access article distributed under the terms of the Creative Commons Attribution License (CC BY). The use, distribution or reproduction in other forums is permitted, provided the original author(s) and the copyright owner(s) are credited and that the original publication in this journal is cited, in accordance with accepted academic practice. No use, distribution or reproduction is permitted which does not comply with these terms.
*Correspondence: Mohammad Esmaeil Akbari, profmeakbari@gmail.com; Hakimeh Zali, hakimehzali@gmail.com; h.zali@sbmu.ac.ir