- 1Department of Obstetrics and Gynecology, Renmin Hospital of Wuhan University, Wuhan, China
- 2Department of Obstetrics and Gynecology, First Affiliated Hospital of Gannan Medical University, Ganzhou, China
Cancer is one of the most harmful diseases, while pregnancy is a common condition of females. Placenta is the most important organ for fetal growth, which has not been fully understand. It’s well known that placenta and solid tumor have some similar biological behaviors. What’s more, decidua, the microenvironment of placenta, and metabolism all undergo adaptive shift for healthy pregnancy. Interestingly, decidua and the tumor microenvironment (TME); metabolism changes during pregnancy and cancer cachexia all have underlying links. However, whether the close link between pregnancy and cancer can bring some new ideas to treat cancer is still unclear. So, in this review we note that pregnancy may offer clues to treat cancer related to three categories: from cell perspective, through the shared development process of the placenta and cancer; from microenvironment perspective, though the shared features of the decidua and TME; and from metabolism perspective, through shared metabolites changes during pregnancy and cancer cachexia. Firstly, comparing gene mutations of both placenta and cancer, which is the underlying mechanism of many similar biological behaviors, helps us understand the origin of cancer and find the key factors to restore tumorigenesis. Secondly, exploring how decidua affect placenta development and similarities of decidua and TME is helpful to reshape TME, then to inhibit cancer. Thirdly, we also illustrate the possibility that the altered metabolites during pregnancy may reverse cancer cachexia. So, some key molecules changed in circulation of pregnancy may help relieve cachexia and make survival with cancer realized.
Introduction
Cancer, similar to many other diseases, can be divided into stages of primary lesions and metabolism changes throughout the whole body. The earlier process of tumors is tumorigenesis, which leads to the development of local cancer lesions; the later stages of almost 50-80% of cancer cases involve cachexia, which means wasting conditions with skeleton loss, adipose tissue loss, muscle atrophy and appetite loss (1). Cachexia is most mortal metabolism shift of cancer, responsible for 20% of tumor-related deaths (1), so we mainly discuss cachexia when came to cancer metabolism shift. Though pregnancy is a physiological state, it can also be thought of a two-stage process: the first stage involving synergistic development of the placenta and decidua, and the later-stage involving an adaptive metabolism shift.
Some similarities between the placenta and tumors have been reported (2–4) and are thus only briefly discussed in our review. As early as 2004, the development of the placenta was suggested to be akin to ‘pseudo-tumorigenesis’ (5). Both the placenta and tumors bear a high level of genetic mutations (6, 7) and feature epithelial-mesenchymal transition (EMT), invasion and cell–cell fusion (8); both can form their own blood vessels through vascular mimicry (VM) and angiogenesis to meet their massive energy consumption demands (9, 10). Even with so many similarities, the placenta is under precise regulation and can be rapidly separated from the decidua during labor, while the development of cancer cells is chaotic, uncontrolled and harmful.
The second category of similarities is between the decidua and TME. The decidua is the environment regulating trophoblast growth and differentiation, and the TME provides a similar environment for cancer cells. Trophoblasts and cancer cells both rely on the environment to provide nutrients, and they can crosstalk with and sculp their environment (11). The decidua and TME both contain complex mixtures of cells (fibroblasts and immune cells), extracellular matrix (EMC), and cytokines produced by these cells and feature unique stressors, such as hypoxia (12, 13).
Discrepancies between the placenta and decidua are the root of placental diseases, which are caused by abnormal implantation depth. Shadow implantation and insufficient decidualization can be observed in recurrent spontaneous abortion (RSA) and preeclampsia (PE) (14), while thinner decidua and over invasion placenta can be seen in abnormally invasive placenta (AIP). Given the features shared between the placenta and cancer and between the tumor microenvironment (TME) and decidua, we suggest that cancers and placental diseases can be categorized into similar disease stages. Tumors exist on a spectrum, which includes a variety of placental diseases. This opinion is better for future research to explore tumorigenesis. What’s more, the regulation role of decidua needs more attention, since decidua can both promote placenta development and restrict over invasion.
The third category of similarities is related to the shared reprogramming of metabolism that occurs during pregnancy and cancer cachexia, which involves many of the same molecules, signaling pathways. Embryos are similar to tumors in that they both have massive energy requirement, but the results for each are different. These patterns, provides a new idea for tumor treatment: can the metabolites changed that occur during pregnancy have antitumor effects?
Researches on cancer can fall into the following categories: solid tumor, TME, and cancer cachexia. Similarly, pregnancy research can fall into the following categories: placenta, decidua, and metabolism reprogramming during pregnancy. These three categories are shared between pregnancy and cancer, and research investigating these similarities may increase our understanding of placental diseases and cancer. So, there are three key factors in pregnancy that can be exploited for cancer treatment.
Cell
Trophoblasts and cancer cells can undergo epithelial-mesenchymal transition (EMT) and invasion, cell–cell fusion, and immune escape. In cancer, EMT is an essential step for invasion and metastasis and has been researched for many years (15). In placental development, cytotrophoblasts (CTBs) can undergo pseudo-EMT and convert into extravillous trophoblasts (EVTs) (16–18). EVTs are invasive and remodel the uterine spiral artery. CTBs can also form syncytiotrophoblasts (STs) by cell–cell fusion, which occurs in cancer (19, 20) to respond to gene damage after chemotherapy or radiation (21). Cell–cell fusion in the placenta may help STBs defend against oxidative stress-induced damage caused by blood flow from the uterine spiral artery. Half of the genes of trophoblasts come from the father and can be seen as foreign, similar to cancer cells. Both express special surface antigens to escape attack by immune cells (22).
However, why these cells share so many similarities is still unknown. Exploring the specific factors driving these similarities is important. Recent researches on gene mutations in the placenta has have revealed the underlying mechanisms of tumor-like behaviors. In 2017, Alexander Meissner’s team analyzed changes in global remethylation profiles from mouse preimplantation embryos to early epiblasts and extraembryonic ectoderm. The researchers found that placental development shares some signaling pathways with cancer (23). Different samples of the same placenta exhibited genetically distinct clonal expansion (24). More interestingly, genetic alterations seen in placenta can be observed in childhood cancers, especially neuroblastoma and rhabdomyosarcoma (25). In 2021, Professor Tim HH Coorens published an article in Nature pointing out that extensive mutagenesis in placental tissues and mosaicism are typical features of placental development (6, 24). The placenta can tolerate gene mutation without causing maternal harm, while cancer cells also tolerate chromosomal aberrations but are harmful. An understanding of the mechanism underlying how trophoblasts tolerate chromosomal aberrations will provide new ways to kill cancer. The cells forming the placenta and embryo was separated within the first several weeks of human development (26), and mutations in the placenta are not present in the fetus (27); as such, the placenta has been suggested as a so-called dumping ground for cells with genetic defects located in the decidua, which can then be removed during delivery. These concepts may enlighten the understanding of cancer—which could be seen as a state in which such defective cells are present throughout the body instead of limited to one area. They cannot be put in a garbage like placenta or decidua. Whether we can reshape the microenvironment of cancer and sequester these defective cells will be reviewed in the following section.
Microenvironment
Similar functions of the decidua and TME
The formation of the maternal-fetal interface depends on coordinated maternal and neonatal development, that is, the coordinated development of the decidua and placenta. From the 15th to 19th day of the menstrual cycle, called the secretory phase, endometrial stromal cells proliferate and differentiate into large, round, cytoplasmic, multinucleated decidual cells, which participate in decidualization (28); this process increases the ease of placental implantation.
The microenvironment, decidua and TME share many similar functions. Their important roles in placental and cancer development have drawn much attention (29). The decidua and TME share multiple regulatory processes, including modulation of epigenetics and regulation of EMT, invasion, immune tolerance, and nutritional supply.
Epigenetic regulation
A study showed that cell column trophoblasts (CCTs) of patients with preeclampsia have transcriptomic differences compared with those of normal pregnant women, but when the two groups of CCTs were separated from the original decidual environment and cultured in vitro, the transcriptomic differences between the two groups gradually disappeared (30). It has been demonstrated that the decidua, as the microenvironment for embryo implantation, significantly affects the epigenetics of trophoblasts (31, 32). The earliest interactions between the blastocyst and decidua initiate gene expression reprogramming of the trophectoderm. Mouse blastocysts cocultured with the human endometrial cell line Ishikawa have altered expression of Cdx2 and Gata3 (33), which are critical for cell differentiation. Although no study has dynamically assessed the regulation of the trophoblast transcriptome by the decidua during the entire gestational period, it is certain that the decidua can significantly regulate the transcriptome of trophoblasts.
Epigenetic alterations are also present in tumors in addition to genetic mutations (34, 35). Cancer can reshape the TME, and the TME can affect the cancer cell epigenetics in turn. Pancreatic cancer TME factors promote cancer stemness via the SPP1-CD44 axis (36). In gastric cancer, TME infiltration is closely associated with cancer cell m-6-A regulator-mediated methylation (37).
Translational control of mRNAs allows tumor cells to dynamically adapt the TME in response to anticancer therapies (38). An understanding of the decidua may enable modification of the mRNA translation of tumors to force them to shift from malignant to benign.
Immune tolerance
The status of EVTs, the outermost structure of the embryo that interfaces with the maternal decidua, is important for successful pregnancy. During the differentiation from CTBs to EVTs, HLA-G molecule expression gradually increases. HLA-G belongs to the human leukocyte antigen family, is specifically expressed at the maternal-fetal interface, and mediates the immune tolerance of the placenta. HLA-G is only expressed by EVTs and interacts with the decidual natural killer (dNK) cell inhibitory receptor killer immunoglobin-like receptor (KIR) KIR2DL4 (39) and LILRB (40) to protect trophoblast cells from NK-mediated cytolysis. dNK cells can express KIRs that can interact with HLA-C expressed by trophoblast cells to maintain immune balance at the maternal-fetal interface.
Both trophoblast and tumor cells are able to escape attack by the immune system. Tumor cells have a variety of harmful gene mutations, and their immune escape mechanisms are complicated. HLA-G can also bind to the NK inhibitory receptor KIR2DL4 in breast cancer (41). Tumor cells can not only avoid being attacked by escaping immune system notice but also secrete TGF-β or other immunosuppressive factors to inhibit the actions of infiltrating cytotoxic T lymphocytes (CTLs) and NK cells (42) and recruit inflammatory cells with aggressive immunosuppressive effects, including regulatory T cells (Tregs) (43) and myeloid-derived suppressor cells (MDSCs). Both inhibit the action of cytotoxic lymphocytes (44).
Nutrient supply
Before vascular formation, trophoblasts and tumor cells exist in their microenvironment and gain nutrients directly from the surrounding tissue (45, 46). After vascular formation, both trophoblasts and tumor cells induce VM. VM is a newly found process by which tumor cells generate additional blood supply that is different from traditional angiogenesis (47); with VM, the tumor blood supply does not depend on invasion of the vascular endothelium, and the tumor itself forms channels (48). VM indicates the formation of tumor cell-lined vasculature, which is different from endothelium-lined vasculature (10). Tumor cell-lined vasculature mainly has four features: expression of endothelium biomarkers, such as VE-cad (48); formation of an extracellular matrix (ECM)- rich network; presence of erythrocytes and plasma; ability to undertake material exchange (10). VM partly explains the poor efficacy of and resistance to angiogenesis inhibitors in the treatment of tumors (49–51). Trophoblasts also have similar physiological characteristics to tumor cells. The trophoblasts that remodel the uterine spiral artery gradually express the VM marker VE-cad. Therefore, the vessels that form in the placenta are also thought to be formed by a kind of VM (9, 52, 53) instead of traditional endothelium-lined vessels (Figure 1).
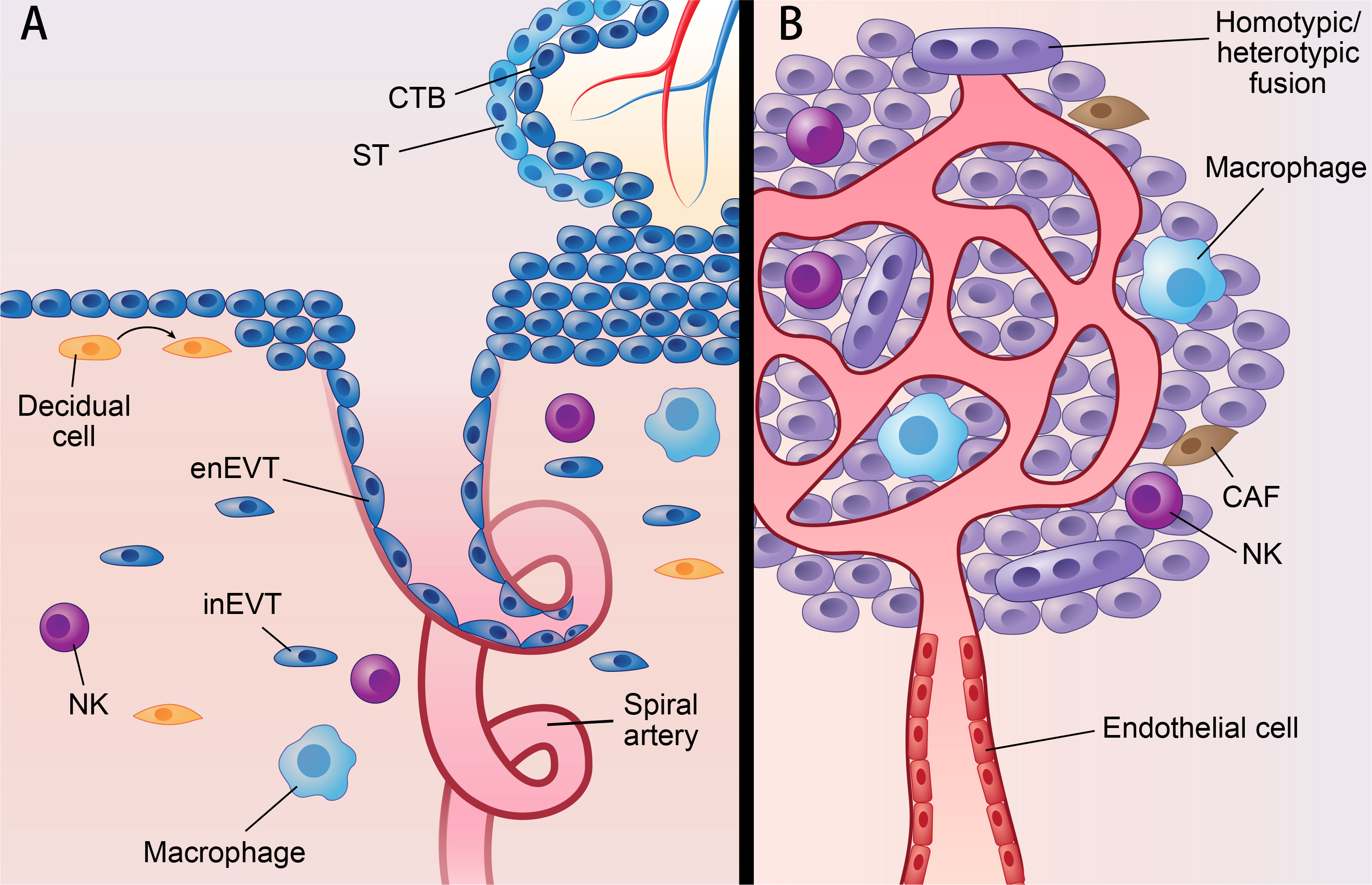
Figure 1 Parallels between placenta and cancer; decidua and TME. Parallels between placenta and cancer; decidua and TME: (A) represents villous of placenta and decidua. (B) represents solid cancer and TME. Figure 1 shows similarities of placenta and cancer; decidua and TME. In placenta, ST originates from CTB by cell-cell fusion, while cancer can fuse with other cells by homotypic/heterotypic fusion. CTB develops into EVT via partial EMT which also occurs in cancer. Decidua and TME also share many components, such as NK cell; macrophage; fibroblast (decidual cell in decidua; CAF in TME). What’s more, spiral artery remodeling in decidua shared features with VM, since both of them mean channels without vascular endothelial cell. CTB, cytotrophoblast; ST, syncytiotrophoblast; EVT, extravillous trophoblast; enEVT, endovascular EVT; inEVT, interstitial EVT; CAF, cancer-associated fibroblasts; NK, natural killer cell; VM, vascular mimicry.
Pericytes surround blood vessels. They have finger-like protrusions that surrounding the vascular endothelium, providing support for blood vessels and controlling vasculature stability and permeability (54). Tumor blood vessels are mainly composed of vascular endothelial cells and pericytes, and pericytes play a key role in angiogenesis and vessel maturation and have been noted as potential targets for tumor therapy (55–58). In the placenta, pericytes preferentially cover endothelial cell–cell junctions. They tend to be most common in regions furthest away from the trophoblast and less common in regions adjacent to the trophoblast, since most transfer occurs here (59). Ang/Tie is an important pathway for signal communication between pericytes and endothelial cells (58), and the vascular endothelium -secreted growth factor PDGFb recruits PDGFRb-expressing pericytes (60). PDGF and its receptors play an important role in tumor pericyte recruitment and communication. Inhibitors of PDGF signaling can disrupt vascular integrity and function by affecting pericytes.
Similar components of the decidua and TME
The decidua contains decidual stromal cells (DSCs) and other immune cells, 70% of which are dNK cells, as well as many kinds of noncellular components, such as cytokines, ECM components and metabolites (Figure 1A). Moreover, the decidua also contains unique features, such as a concentration gradient of oxygen (61) and specific levels of cytokines. It is well known that, similar to the decidua, the TME also contains cells and noncell components. The cells include cancer-associated fibroblasts (CAFs) and immune cells; the noncell components include ECM, cell-secreted products and metabolites (Figure 1B). The TME can also contains unique environmental stressors, such as hypoxia.
Fibroblast and fibroblast-secreted cytokines
DSCs and CAFs play important roles in decidualization and TME modulation (62). Both of these cells and their target cells can reshape the other. A balance between these cell types is necessary for placental development, decidualization, tumorigenesis and TME modulation. The differentiation of DSCs and CAFs involves cell transformation. DSCs experience mesenchymal-epithelial transition (MET) (63), and CAFs experience macrophage-myofibroblast transition (MMT) (60). MET of DSCs occurs spontaneously in humans and is regulated by sex hormones, while the transformation of CAFs requires triggering by cancer cells. Whether we can prevent CAF formation and inhibit cancer remains to be determined.
The cytokines they secrete perform fundamental roles in cell-to-cell interactions. The C-X-C motif chemokine ligand (CXCL) family and C-X-C chemokine receptor (CXCR) family are important in this crosstalk. CXCLs are secreted by trophoblast cells and tumor cells, as well as cells in the decidua and TME (64–66). CXCLs/CXCRs play important roles in the interactions at the maternal-fetal interface (67) and cancer-TME interface. In the decidua, CXCL12 interacts with CXCR4 to promote invasion and suppress apoptosis of trophoblasts (68), while CXCL14, another member of the CXCL family, can block these effects. Aberrant CXCL/CXCR expression has also been observed in placental diseases. CXCL12 and CXCR4/CXCR7 are upregulated in AIP, and their expression can be detected in circulation (69). Snail promotes ovarian cancer progression by recruiting MDSCs via CXCR2 ligand upregulation (70). CCL2, expressed by DSCs, macrophages and EVTs, can help recruit T cells to the maternal-fetal interface (71, 72) and recruit MDSCs to the TME (73), which can be observed in lung cancer and pancreatic ductal adenocarcinoma (74–77). Many signaling pathways are shared between these processes, such as the Wnt (78–83), Notch (84–88), and Hippo signaling pathways (81, 84, 89–91).
Noncellular components
ECM stiffening and degradation drive cancers (92), while decidualization is associated with extensive ECM remodeling (93). ECM remodeling heavily relies on matrix metalloproteinases (MMPs). MMPs can be secreted by trophoblast, decidual, and cancer cells and cell in the TME (94), playing a pivotal role in collagen and fibronectin degeneration (95, 96). Recent studies have indicated that growth factors are also involved in interactions with ECM proteins and proteolytic enzymes, for example, the IGF–IGFR–IGFBP axis (97). ECM remodeling is important for cell invasion and immune cell recruitment.
Hypoxia is an important characteristic of the decidua and TME. In the first few weeks of placental development, CTBs form trophoblast plugs that block the uterine spiral artery, disturbing blood flow into the intervillous space and resulting in a limited oxygenation environment around trophoblast cells (98). A moderately hypoxic environment is believed to promote CTB exit from the cell cycle, induction of EMT, and acquisition of invasive ability.
Hypoxia is also very important for tumors (99, 100). Hypoxia can induce many tumor processes, such as invasion, vasculogenesis, angiogenesis and VM, to generate a nutrient supply system (101). Hypoxia can increase the expression of the hypoxia-inducible factors (Hifs), which is associated with a poor prognosis (102–104). Hifs are a key link between hypoxia and different cell behaviors and are important factors promoting trophoblast invasion.
Importantly, these factors also influence each other (105). Hypoxia promotes high CXCR expression, which in turn promotes invasion (106). Moreover, hypoxia may contribute to VM (51, 107). The ECM provides an environment that allows T-cell invasion into tissues or directly inhibits T-cell proliferation to support and suppress adaptive immune responses, respectively (108).
Cancer and placental diseases
The decidua can regulate and support the growth and differentiation of trophoblast cells. Discrepancies between the placenta and decidua can cause placental diseases, such as RSA and PE (14).
RSA, defined as two or more consecutive pregnancy losses before 20 weeks of gestation, occurs in 2-5% of women during their reproductive years (109, 110). An impaired decidua can lead to RSA (111, 112). PE is another common obstetric complication that occurs in 2%-8% of pregnant women (113). Patients with two miscarriages are more likely to have preeclampsia (114). PE occurs when in the second half of pregnancy, the systolic blood pressure (SBP) changes to >=140 mmHg and/or the diastolic blood pressure (DBP) changes to >=90 mmHg in patients whose previous blood pressure was normal (115). During placental development, trophoblast cells invade the uterine spiral artery and replace vascular endothelial cells, expanding the diameter of the uterine spiral artery and reducing vascular resistance to meet the nutrient needs of the fetus (116). The preclinical stages of RSA and PE both involve defective trophoblast invasion and insufficient decidualization (31, 117–119). Failure of implantation causes fetal loss; insufficient uterine spiral artery remodeling can cause trophoblast ischemia, and the injured placenta releases inflammatory factors and/or exosomes, causing hypertension (120) (Figures 2A, B). Although it is regrettable that impaired decidualization and insufficient invasion of trophoblast cells lead to poor pregnancy outcomes, the defects in gene expression of the injured decidua and placenta revealed by transcriptional profiling may reveal factors that can be targeted to treat cancer. Whether the factors in the decidua of RSA and PE can have antitumor effects is an interesting point to research in the future.
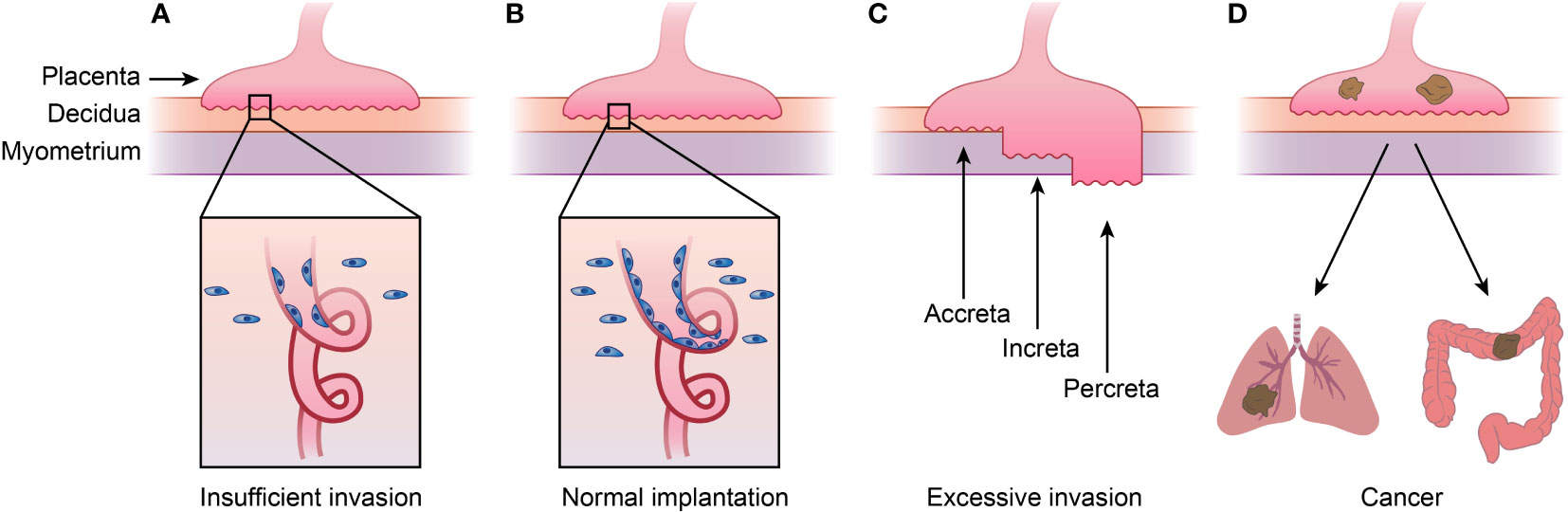
Figure 2 Placenta diseases and Choriocarcinoma. (A)shows placenta diseases caused by insufficient invasion of trophoblast and spiral artery remodeling, which is caused by both unhealthy trophoblast and decidua. (B) represents normal implantation, and (C) shows AIP caused by excessive invasion of trophoblast and thinner decidua. AIP can be categorized into placenta accreta, placenta increta and placenta percreta depending the depth of implantation. (D) shows choriocarcinoma, meaning cancer happened of trophoblast which tend to metastasis to lung or other organs. This figure shows that placenta diseases and choriocarcinoma or even other kind cancers are in a spectrum. AIP, abnormally invasive placenta.
In another placental disease, AIP, the decidua thins and the placenta invades the deeper uterine wall (121), which are processes similar to those seen in malignant tumors (122). AIP is now recognized as a series of diseases. According to the depth of implantation, AIP is divided into three types: placenta accreta (PA; attachment to myometrium without intervening decidua), placenta increta (PI; invasion into the myometrium), and placenta percreta (PP; invasion through the uterine wall). AIP affects 1 in 1000 pregnant women (123) (Figures 2B, C). The occurrence of AIP is closely related to the absence of decidua caused by cesarean delivery (124–127). Uterine scarring after c-section can lead to endometrial defects and failure of normal decidualization in the incision area, which leads to an abnormal depth of implantation (128).
As mentioned above, the decidua not only promotes or inhibits the invasion of trophoblasts but also ensures that process occur within a suitable depth. The decidua can not only provide the proper environment for trophoblasts to invade but also inhibit villi from invading beyond 2/3 of the uterine wall. During delivery, the placenta is rapidly released from the decidua, acting as a container that temporarily holds and provides energy for the embryo while restricting overgrowth. However, there is no equivalent structure to the decidua in tumors. The diseases above are in a spectrum of diseases. The absence of the decidua causes placental overgrowth, and decidual dysplasia restricts the development of the placenta. These ideas imply that the placental microenvironment in PE or RSA may inhibit tumor growth (Figure 2).
Metabolites
Placenta and cancer both feature metabolism reprogramming throughout the body. During pregnancy, in addition to changes in the female reproductive system, many systems change to meet the increased body weight and energy requirements of the fetus (129). The basal metabolic rate of the mother increases significantly, and increased blood volume, hyperglycemia, and a positive nitrogen balance also occur. Similarly, tumors not only affect the primary and metastatic tumor sites but also result in changes in the whole body. Cachexia mainly manifests as abnormal metabolism, especially the loss of skeletal muscle (130, 131). Removing tumors or effectively treating them can partially ameliorate cancer cachexia (132–134), suggesting that cancer cachexia is caused by tumor cell-released factors. 50%-80% patients with advanced tumors will suffer cachexia (1), which can also be seen in a variety of chronic diseases, such as chronic heart failure. Importantly, this depleted state cannot be reversed by the additional intake of more nutrients. Therefore, there is a shared mechanism underlying cancer and other disorders. From the perspective of energy metabolism, tumors and other wasting-related metabolic diseases exist on the same disease spectrum. Cachexia involves many of the same adaptive processes, so metabolome changes during pregnancy may offer insights for reversing cachexia in cancer and even other metabolic disorders.
Insulin resistance
Insulin, secreted by pancreatic β cells, is one of the most important hormones of metabolism, and its main function is regulating glucose metabolism. Insulin can increase the utilization of glucose and promote the synthesis of glycogen, adipose tissue and protein. Insulin resistance (IR) refers to a decrease in the efficiency of insulin to promote glucose uptake and utilization due to various reasons, including decreased insulin receptor phosphorylation when combined with insulin, decreased expression of insulin receptor substrate 1 (IRS-1), and decreased phosphoinositide 3-kinase (PI3K) response to insulin (135). IR is most common in diabetes but can also be observed in both pregnancy (136) and cancer cachexia (135, 137). IR tends to occur in the third trimester to maintain maternal euglycemia. During normal pregnancy, a nearly 50% reduction in insulin-mediated glucose disposal and increased insulin independence occurs with increasing gestational age (138). Changes in growth hormone (GH), human chorionic somatomammotropin (hCS) and several other blood sugar-regulating hormones lead to euglycemia during pregnancy. However, restoration of the balance between the decrease in hormones and increase in blood sugar may lead to chronic hyperglycemia or hypoglycemia (139). Even though the exact etiology of IR in pregnancy and cancer cachexia is not fully understood, some interesting similarities exist.
The development of IR in pregnancy is caused by imbalance of multiple hormones, including insulin-stimulating hormones, lactogenic hormones, prolactin and placental lactogens, which can promote the proliferation and secretion of pancreatic β cells, and hormones that induce IR, such as human placental prolactin lactogen (hPL), placental growth hormone (GH-2) (140) and insulin-like growth factor-1 (IGF-1) (141). In cancer cachexia, inflammation rather than tumor location, tumor attrition status, and tumor stage may play a key role in promoting IR (142). Chronic exposure to inflammatory cytokines, tumor necrosis factor α (TNF-α), IL-6, and IGFBPs can induce IR (143). The placenta and tumors, although employing different mechanisms, are sources of IR. After delivery of the placenta, maternal metabolism gradually returns to normal and similarly, if a tumor is treated or surgically removed, IR will gradually disappear (144, 145). However, the potential shared mechanisms between the placenta and tumors related to IR remain to be elucidated in further detail (146). IR is a mechanism related to cachexia (147). In tumors, IR occurs via suppression of the anabolic PI3K–AKT pathway and activation of the ubiquitin-mediated proteasome pathway (148), which in turn leads to muscle breakdown.
Similarly altered circulating molecules
Exosomes are a newly identified method of cell-to-cell communication (149). One study innovatively identified some miRNAs carried by exosomes in maternal circulation that can be targeted to treat or prevent cancer. The study showed that exomiR-302d-3p, exomiR-223-3p and exomiR-451a, which are commonly associated with cancer metastasis and immune evasion, were highly expressed in pregnant women (150). Circulating cell-free DNA (cfDNA) contains short fragments of double-stranded DNA and is present in blood and other body fluids (151). Similar to miRNAs, cfDNA can also be packaged in exosomes (152) and can be released into the blood to maintain homeostasis and protect DNA molecules against nucleases and the immune system (153), and cfDNA can also reflect epigenetic changes in the body. There are similarities between cfDNA in cancer (154) and pregnancy (152, 155, 156). For example, hypermethylated RASSF1 can be found in the circulation in breast cancer, lung cancer and PE.
So far, studies have mainly focused on miRNA or cfDNA in exosomes. However, the impact of the placenta and tumor cells on the whole body reaches far beyond exosomes and miRNAs. Therefore, attention should also be paid to other kinds of molecules.
Metabolism during pregnancy is under precise regulation (157). Insufficient or excessive weight gain during pregnancy can lead to miscarriage, preterm gestational diabetes, and postpartum complications and can even affect offspring health (158). A study of the metabolomes of pregnant women at different gestational weeks demonstrated that metabolic reprogramming during pregnancy is related to many factors other than weight gain. The researchers found that 4,995 metabolic features (of 9,651 total), 460 annotated compounds (of 687 total), and 34 human metabolic pathways (of 48 total) were altered (157). This is the only study that reveals the characterization of the human pregnancy metabolome weekly and providing a high-resolution and detailed landscape. Another research used a serum and urine metabolomics approach to reveal the metabolic profile of cancer cachexia (159). More researches need to be done to reveal detailed landscape of pregnancy and cachexia. Another review suggested that metabolic abnormalities are shared by cancer and a variety of other chronic diseases that share underlying pathological processes. Cancer can share glucose-related, pyruvate-related and metabolism characteristics with diabetes, cardiovascular diseases, and neurological diseases (160). Metabolic abnormalities, including cachexia and hyperglycemia, can disrupt homeostasis, alter the body status, and in turn affect the development of tumors. Exploring the shared mechanisms between pregnancy and cancer cachexia by metabolomics may aid the development of new treatments.
One important molecule that is changed during pregnancy is steroid hormones, including the pregnancy-related hormones progesterone, estrogen, prolactin, and GH (161). It was once thought that these hormones mainly play roles in pregnancy maintenance, but it was later found that they were closely related to the occurrence and development of tumors, even several years after pregnancy, especially for tumors that develop in pregnancy-related organs, such as breast cancer (162), ovarian cancer and endometrial cancer (163). Carrying a pregnancy over 34 w can reduce the risk of breast cancer (162), which has been confirmed in a number of clinical studies.
Conclusions
In this review, we attempted to summarize up the similarities between pregnancy and cancer in three levels. Embryogenesis and cancer metastasis share many cellular similarities (164). Trophoblast and cancer cells share gene mutations, EMT and invasion processes, cell–cell fusion features and other biological behaviors. The placenta can be thought of as a physiological tumor and is also considered a repository for damaged cells during embryonic development (6). More researches should be performed to explore the link between placenta and cancer. Regarding placenta as “pseudo-tumor” may be helpful to explore why accumulation of gene mutation in cancer becomes uncontrolled. Furthermore, the decidua and TME can both regulate epigenetics, migration, invasion, and immune tolerance and provide a hypoxic environment (12, 98). Uniquely, the placenta is removed from the decidua during childbirth or miscarriage. The decidua serves as a container that limits the overgrowth of the placenta while promoting its healthy development. Whether decidua, especially decidua of PE and RSA has antitumor effects is an interesting point to explore in future. Since decidua has powerful effect of regulating placenta development, if the differences or similarities of decidua and TME can be revealed in detail, it’s possible to reshape TME and find more ways to treat cancer can be found.
The last level of similarities between pregnancy and cancer is metabolites. Changes in metabolites in the circulation of cancer cachexia patients and pregnant women involve many of the same molecules, such as hormones and exosomes (150), but lead to totally different ending. IR is a feature shared by both patients with cachexia and pregnant individual (146, 147). If some metabolites of pregnancy have anti-cachexia effect, the future research can be done to identify some key molecules which can relieve cachexia and make survival with tumor realized. We hope our review can bring some new ideas to find cancer treatment targets.
Author contributions
HP, DL, and YG wrote the draft. YY, TL, YL, and TC drew the figures. CF designed the study and helped draft the manuscript. All authors contributed to the article and approved the submitted version.
Conflict of interest
The authors declare that the research was conducted in the absence of any commercial or financial relationships that could be construed as a potential conflict of interest.
Publisher’s note
All claims expressed in this article are solely those of the authors and do not necessarily represent those of their affiliated organizations, or those of the publisher, the editors and the reviewers. Any product that may be evaluated in this article, or claim that may be made by its manufacturer, is not guaranteed or endorsed by the publisher.
References
1. Argilés JM, Busquets S, Stemmler B, López-Soriano FJ. Cancer cachexia: understanding the molecular basis. Nat Rev Cancer (2014) 14:754–62. doi: 10.1038/nrc3829
2. Costanzo V, Bardelli A, Siena S, Abrignani S. Exploring the links between cancer and placenta development. Open Biol (2018) 8:180081. doi: 10.1098/rsob.180081
3. Lala PK, Nandi P, Hadi A, Halari C. A crossroad between placental and tumor biology: What have we learnt? Placenta (2021) 116:12–30. doi: 10.1016/j.placenta.2021.03.003
4. Ferretti C, Bruni L, Dangles-Marie V, Pecking AP, Bellet D. Molecular circuits shared by placental and cancer cells, and their implications in the proliferative, invasive and migratory capacities of trophoblasts. Hum Reprod Update (2007) 13:121–41. doi: 10.1093/humupd/dml048
5. Soundararajan R, Rao AJ. Trophoblast ‘pseudo-tumorigenesis’: significance and contributory factors. Reprod Biol Endocrinol: RB&E (2004) 2:15. doi: 10.1186/1477-7827-2-15
6. Burki TK. Placenta can provide new insights on the genetics of cancer. Lancet Oncol (2021) 22:591. doi: 10.1016/S1470-2045(21)00208-4
7. Lorincz MC, Schübeler D. Evidence for converging DNA methylation pathways in placenta and cancer. Dev Cell (2017) 43:257–8. doi: 10.1016/j.devcel.2017.10.009
8. Huang Q, Chen H, Li J, Oliver M, Ma X, Byck D, et al. Epigenetic and non-epigenetic regulation of syncytin-1 expression in human placenta and cancer tissues. Cell Signal (2014) 26:648–56. doi: 10.1016/j.cellsig.2013.11.002
9. Rai A, Cross JC. Development of the hemochorial maternal vascular spaces in the placenta through endothelial and vasculogenic mimicry. Dev Biol (2014) 387:131–41. doi: 10.1016/j.ydbio.2014.01.015
10. Hendrix MJ, Seftor EA, Hess AR, Seftor RE. Vasculogenic mimicry and tumour-cell plasticity: Lessons from melanoma. Nat Rev Cancer (2003) 3:411–21. doi: 10.1038/nrc1092
11. Zhu K, Lv Z, Xiong J, Zheng H, Zhang S, Jin H, et al. MET inhibitor, capmatinib overcomes osimertinib resistance via suppression of MET/Akt/snail signaling in non-small cell lung cancer and decreased generation of cancer-associated fibroblasts. Aging (2021) 13:6890–903. doi: 10.18632/aging.202547
12. Sharma S, Godbole G, Modi D. Decidual control of trophoblast invasion. Am J Reprod Immunol (New York NY: 1989) (2016) 75:341–50. doi: 10.1111/aji.12466
13. Hinshaw DC, Shevde LA. The tumor microenvironment innately modulates cancer progression. Cancer Res (2019) 79:4557–66. doi: 10.1158/0008-5472.CAN-18-3962
14. Burton GJ, Jauniaux E. Placental oxidative stress: From miscarriage to preeclampsia. J Soc Gynecol Invest (2004) 11:342–52. doi: 10.1016/j.jsgi.2004.03.003
15. Bakir B, Chiarella AM, Pitarresi JR, Rustgi AK. EMT, MET, plasticity, and tumor metastasis. Trends Cell Biol (2020) 30:764–76. doi: 10.1016/j.tcb.2020.07.003
16. Illsley NP, DaSilva-Arnold SC, Zamudio S, Alvarez M, Al-Khan A. Trophoblast invasion: Lessons from abnormally invasive placenta (placenta accreta). Placenta (2020) 102:61–6. doi: 10.1016/j.placenta.2020.01.004
17. Pirinen E, Soini Y. A survey of zeb1, twist and claudin 1 and 4 expression during placental development and disease. APMIS: Acta Pathol Microbiol Immunol Scand (2014) 122:530–8. doi: 10.1111/apm.12187
18. Aiello NM, Maddipati R, Norgard RJ, Balli D, Li J, Yuan S, et al. EMT subtype influences epithelial plasticity and mode of cell migration. Dev Cell (2018) 45:681–95.e4. doi: 10.1016/j.devcel.2018.05.027
19. Gauck D, Keil S, Niggemann B, Zänker KS, Dittmar T. Hybrid clone cells derived from human breast epithelial cells and human breast cancer cells exhibit properties of cancer stem/initiating cells. BMC Cancer (2017) 17:515. doi: 10.1186/s12885-017-3509-9
20. Xu MH, Gao X, Luo D, Zhou XD, Xiong W, Liu GX. EMT and acquisition of stem cell-like properties are involved in spontaneous formation of tumorigenic hybrids between lung cancer and bone marrow-derived mesenchymal stem cells. PloS One (2014) 9:e87893. doi: 10.1371/journal.pone.0087893
21. Orr-Weaver TL. When bigger is better: the role of polyploidy in organogenesis. Trends Genetics: TIG (2015) 31:307–15. doi: 10.1016/j.tig.2015.03.011
22. Soeters PB, Grimble RF. The conditional role of inflammation in pregnancy and cancer. Clin Nutr (Edinburgh Scotland) (2013) 32:460–5. doi: 10.1016/j.clnu.2012.07.010
23. Smith ZD, Shi J, Gu H, Donaghey J, Clement K, Cacchiarelli D, et al. Epigenetic restriction of extraembryonic lineages mirrors the somatic transition to cancer. Nature (2017) 549:543–7. doi: 10.1038/nature23891
24. Coorens THH, Oliver TRW, Sanghvi R, Sovio U, Cook E, Vento-Tormo R, et al. Inherent mosaicism and extensive mutation of human placentas. Nature (2021) 592:80–5. doi: 10.1038/s41586-021-03345-1
25. Gröbner SN, Worst BC, Weischenfeldt J, Buchhalter I, Kleinheinz K, Rudneva VA, et al. The landscape of genomic alterations across childhood cancers. Nature (2018) 555:321–7. doi: 10.1038/nature25480
26. Io S, Kondoh E, Chigusa Y, Kawasaki K, Mandai M, Yamada AS. New era of trophoblast research: Integrating morphological and molecular approaches. Hum Reprod Update (2020) 26:611–33. doi: 10.1093/humupd/dmaa020
27. Kalousek DK, Dill FJ. Chromosomal mosaicism confined to the placenta in human conceptions. Sci (New York NY) (1983) 221:665–7. doi: 10.1126/science.6867735
28. Gellersen B, Brosens JJ. Cyclic decidualization of the human endometrium in reproductive health and failure. Endocr Rev (2014) 35:851–905. doi: 10.1210/er.2014-1045
29. Maman S, Witz IP. A history of exploring cancer in context. Nat Rev Cancer (2018) 18:359–76. doi: 10.1038/s41568-018-0006-7
30. Zhou Y, Gormley MJ, Hunkapiller NM, Kapidzic M, Stolyarov Y, Feng V, et al. Reversal of gene dysregulation in cultured cytotrophoblasts reveals possible causes of preeclampsia. J Clin Invest (2013) 123:2862–72. doi: 10.1172/JCI66966
31. Garrido-Gomez T, Dominguez F, Quiñonero A, Diaz-Gimeno P, Kapidzic M, Gormley M, et al. Defective decidualization during and after severe preeclampsia reveals a possible maternal contribution to the etiology. Proc Natl Acad Sci USA (2017) 114:E8468–e77. doi: 10.1073/pnas.1706546114
32. Lockwood CJ, Huang SJ, Krikun G, Caze R, Rahman M, Buchwalder LF, et al. Decidual hemostasis, inflammation, and angiogenesis in pre-eclampsia. Semin Thromb Hemost (2011) 37:158–64. doi: 10.1055/s-0030-1270344
33. Ruane PT, Berneau SC, Koeck R, Watts J, Kimber SJ, Brison DR, et al. Apposition to endometrial epithelial cells activates mouse blastocysts for implantation. Mol Hum Reproduct (2017) 23:617–27. doi: 10.1093/molehr/gax043
34. Sager R. Expression genetics in cancer: shifting the focus from DNA to RNA. Proc Natl Acad Sci USA (1997) 94:952–5. doi: 10.1073/pnas.94.3.952
35. Kaur S, Lotsari-Salomaa JE, Seppänen-Kaijansinkko R, Peltomäki P. MicroRNA methylation in colorectal cancer. Adv Exp Med Biol (2016) 937:109–22. doi: 10.1007/978-3-319-42059-2_6
36. Nallasamy P, Nimmakayala RK, Karmakar S, Leon F, Seshacharyulu P, Lakshmanan I, et al. Pancreatic tumor microenvironment factor promotes cancer stemness via SPP1-CD44 axis. Gastroenterology (2021) 161:1998–2013.e7. doi: 10.1053/j.gastro.2021.08.023
37. Zhang B, Wu Q, Li B, Wang D, Wang L, Zhou YL. m(6)A regulator-mediated methylation modification patterns and tumor microenvironment infiltration characterization in gastric cancer. Mol Cancer (2020) 19:53. doi: 10.1186/s12943-020-01170-0
38. Fabbri L, Chakraborty A, Robert C, Vagner S. The plasticity of mRNA translation during cancer progression and therapy resistance. Nat Rev Cancer (2021) 21:558–77. doi: 10.1038/s41568-021-00380-y
39. Tan CY, Chong YS, Loganath A, Chan YH, Ravichandran J, Lee CG, et al. Possible gene-gene interaction of KIR2DL4 with its cognate ligand HLA-G in modulating risk for preeclampsia. Reprod Sci (Thousand Oaks Calif) (2009) 16:1135–43. doi: 10.1177/1933719109342280
40. Kuroki K, Matsubara H, Kanda R, Miyashita N, Shiroishi M, Fukunaga Y, et al. Structural and functional basis for LILRB immune checkpoint receptor recognition of HLA-G isoforms. J Immunol (Baltimore Md: 1950) (2019) 203:3386–94. doi: 10.4049/jimmunol.1900562
41. Zheng G, Guo Z, Li W, Xi W, Zuo B, Zhang R, et al. Interaction between HLA-G and NK cell receptor KIR2DL4 orchestrates HER2-positive breast cancer resistance to trastuzumab. Signal Transduct Target Ther (2021) 6:236. doi: 10.1038/s41392-021-00629-w
42. Kondo Y, Suzuki S, Takahara T, Ono S, Goto M, Miyabe S, et al. Improving function of cytotoxic T-lymphocytes by transforming growth factor-β inhibitor in oral squamous cell carcinoma. Cancer Sci (2021) 112:4037–49. doi: 10.1111/cas.15081
43. Zhang Q, Fu L, Liang Y, Guo Z, Wang L, Ma C, et al. Exosomes originating from MSCs stimulated with TGF-β and IFN-γ promote treg differentiation. J Cell Physiol (2018) 233:6832–40. doi: 10.1002/jcp.26436
44. Yang M, Li J, Gu P, Fan X. The application of nanoparticles in cancer immunotherapy: Targeting tumor microenvironment. Bioactive Mater (2021) 6:1973–87. doi: 10.1016/j.bioactmat.2020.12.010
45. Hempstock J, Cindrova-Davies T, Jauniaux E, Burton GJ. Endometrial glands as a source of nutrients, growth factors and cytokines during the first trimester of human pregnancy: a morphological and immunohistochemical study. Reprod Biol Endocrinol: RB&E (2004) 2:58. doi: 10.1186/1477-7827-2-58
46. Jeschke U, Kunert-Keil C, Mylonas I, Hammer A, Schiessl B, Lomba I, et al. Expression of glycodelin a in decidual tissue of preeclamptic, HELLP and intrauterine growth-restricted pregnancies. Virchows Archiv: An Int J Pathol (2005) 446:360–8. doi: 10.1007/s00428-004-1201-3
47. Maniotis AJ, Folberg R, Hess A, Seftor EA, Gardner LM, Pe’er J, et al. Vascular channel formation by human melanoma cells in vivo and in vitro: vasculogenic mimicry. Am J Pathol (1999) 155:739–52. doi: 10.1016/S0002-9440(10)65173-5
48. Delgado-Bellido D, Serrano-Saenz S, Fernández-Cortés M, Oliver FJ. Vasculogenic mimicry signaling revisited: Focus on non-vascular VE-cadherin. Mol Cancer (2017) 16:65. doi: 10.1186/s12943-017-0631-x
49. Shin SU, Cho HM, Das R, Gil-Henn H, Ramakrishnan S, Al Bayati A, et al. Inhibition of vasculogenic mimicry and angiogenesis by an anti-EGFR IgG1-human endostatin-P125A fusion protein reduces triple negative breast cancer metastases. Cells (2021) 10:2904. doi: 10.3390/cells10112904
50. Qu B, Sheng G, Guo L, Yu F, Chen G, Lu Q, et al. MIG7 is involved in vasculogenic mimicry formation rendering invasion and metastasis in hepatocellular carcinoma. Oncol Rep (2018) 39:679–86. doi: 10.3892/or.2017.6138
51. Wei X, Chen Y, Jiang X, Peng M, Liu Y, Mo Y, et al. Mechanisms of vasculogenic mimicry in hypoxic tumor microenvironments. Mol Cancer (2021) 20:7. doi: 10.1186/s12943-020-01288-1
52. Zhou Y, Fisher SJ, Janatpour M, Genbacev O, Dejana E, Wheelock M, et al. Human cytotrophoblasts adopt a vascular phenotype as they differentiate. A strategy for successful endovascular invasion? J Clin Invest (1997) 99:2139–51. doi: 10.1172/JCI119387
53. Zhou Y, Damsky CH, Fisher SJ. Preeclampsia is associated with failure of human cytotrophoblasts to mimic a vascular adhesion phenotype. one cause of defective endovascular invasion in this syndrome? J Clin Invest (1997) 99:2152–64. doi: 10.1172/JCI119388
54. Barreto RSN, Romagnolli P, Cereta AD, Coimbra-Campos LMC, Birbrair A, Miglino MA. Pericytes in the placenta: Role in placental development and homeostasis. Adv Exp Med Biol (2019) 1122:125–51. doi: 10.1007/978-3-030-11093-2_8
55. Zhang XN, Yang KD, Chen C, He ZC, Wang QH, Feng H, et al. Pericytes augment glioblastoma cell resistance to temozolomide through CCL5-CCR5 paracrine signaling. Cell Res (2021) 31:1072–87. doi: 10.1038/s41422-021-00528-3
56. Figueiredo AM, Villacampa P, Diéguez-Hurtado R, José Lozano J, Kobialka P, Cortazar AR, et al. Phosphoinositide 3-Kinase-Regulated pericyte maturation governs vascular remodeling. Circulation (2020) 142:688–704. doi: 10.1161/CIRCULATIONAHA.119.042354
57. Er EE, Valiente M, Ganesh K, Zou Y, Agrawal S, Hu J, et al. Pericyte-like spreading by disseminated cancer cells activates YAP and MRTF for metastatic colonization. Nat Cell Biol (2018) 20:966–78. doi: 10.1038/s41556-018-0138-8
58. Teichert M, Milde L, Holm A, Stanicek L, Gengenbacher N, Savant S, et al. Pericyte-expressed Tie2 controls angiogenesis and vessel maturation. Nat Commun (2017) 8:16106. doi: 10.1038/ncomms16106
59. Harris SE, Matthews KS, Palaiologou E, Tashev SA, Lofthouse EM, Pearson-Farr J, et al. Pericytes on placental capillaries in terminal villi preferentially cover endothelial junctions in regions furthest away from the trophoblast. Placenta (2021) 104:1–7. doi: 10.1016/j.placenta.2020.10.032
60. Tang PC, Chung JY, Xue VW, Xiao J, Meng XM, Huang XR, et al. Smad3 promotes cancer-associated fibroblasts generation via macrophage-myofibroblast transition. Adv Sci (Weinheim Baden Wurttemberg Germany) (2022) 9:e2101235. doi: 10.1002/advs.202101235
61. Colson A, Sonveaux P, Debiève F, Sferruzzi-Perri AN. Adaptations of the human placenta to hypoxia: Opportunities for interventions in fetal growth restriction. Hum Reprod Update (2021) 27:531–69. doi: 10.1093/humupd/dmaa053
62. Sahai E, Astsaturov I, Cukierman E, DeNardo DG, Egeblad M, Evans RM, et al. A framework for advancing our understanding of cancer-associated fibroblasts. Nat Rev Cancer (2020) 20:174–86. doi: 10.1038/s41568-019-0238-1
63. Owusu-Akyaw A, Krishnamoorthy K, Goldsmith LT, Morelli SS. The role of mesenchymal-epithelial transition in endometrial function. Hum Reprod Update (2019) 25:114–33. doi: 10.1093/humupd/dmy035
64. Mei J, Yan Y, Li SY, Zhou WJ, Zhang Q, Li MQ, et al. CXCL16/CXCR6 interaction promotes endometrial decidualization via the PI3K/AKT pathway. Reprod (Cambridge England) (2019) 157:273–82. doi: 10.1530/REP-18-0417
65. Ramhorst R, Grasso E, Paparini D, Hauk V, Gallino L, Calo G, et al. Decoding the chemokine network that links leukocytes with decidual cells and the trophoblast during early implantation. Cell Adhesion Migration (2016) 10:197–207. doi: 10.1080/19336918.2015.1135285
66. Fan DX, Zhou WJ, Jin LP, Li MQ, Xu XH, Xu CJ. Trophoblast-derived CXCL16 decreased granzyme b production of decidual γδ T cells and promoted bcl-xL expression of trophoblasts. Reprod Sci (Thousand Oaks Calif) (2019) 26:532–42. doi: 10.1177/1933719118777638
67. Shi JW, Yang HL, Fan DX, Yang SL, Qiu XM, Wang Y, et al. The role of CXC chemokine ligand 16 in physiological and pathological pregnancies. Am J Reprod Immunol (New York NY: 1989) (2020) 83:e13223. doi: 10.1111/aji.13223
68. Zheng J, Wang H, Zhou W. Modulatory effects of trophoblast-secreted CXCL12 on the migration and invasion of human first-trimester decidual epithelial cells are mediated by CXCR4 rather than CXCR7. Reprod Biol Endocrinol: RB&E (2018) 16:17. doi: 10.1186/s12958-018-0333-2
69. Long Y, Jiang Y, Zeng J, Dang Y, Chen Y, Lin J, et al. The expression and biological function of chemokine CXCL12 and receptor CXCR4/CXCR7 in placenta accreta spectrum disorders. J Cell Mol Med (2020) 24:3167–82. doi: 10.1111/jcmm.14990
70. Taki M, Abiko K, Baba T, Hamanishi J, Yamaguchi K, Murakami R, et al. Snail promotes ovarian cancer progression by recruiting myeloid-derived suppressor cells via CXCR2 ligand upregulation. Nat Commun (2018) 9:1685. doi: 10.1038/s41467-018-03966-7
71. Svensson-Arvelund J, Mehta RB, Lindau R, Mirrasekhian E, Rodriguez-Martinez H, Berg G, et al. The human fetal placenta promotes tolerance against the semiallogeneic fetus by inducing regulatory T cells and homeostatic M2 macrophages. J Immunol (Baltimore Md: 1950) (2015) 194:1534–44. doi: 10.4049/jimmunol.1401536
72. Choudhury RH, Dunk CE, Lye SJ, Aplin JD, Harris LK, Jones RL. Extravillous trophoblast and endothelial cell crosstalk mediates leukocyte infiltration to the early remodeling decidual spiral arteriole wall. J Immunol (Baltimore Md: 1950) (2017) 198:4115–28. doi: 10.4049/jimmunol.1601175
73. Chun E, Lavoie S, Michaud M, Gallini CA, Kim J, Soucy G, et al. CCL2 promotes colorectal carcinogenesis by enhancing polymorphonuclear myeloid-derived suppressor cell population and function. Cell Rep (2015) 12:244–57. doi: 10.1016/j.celrep.2015.06.024
74. Yang X, Lin Y, Shi Y, Li B, Liu W, Yin W, et al. FAP promotes immunosuppression by cancer-associated fibroblasts in the tumor microenvironment via STAT3-CCL2 signaling. Cancer Res (2016) 76:4124–35. doi: 10.1158/0008-5472.CAN-15-2973
75. Gu H, Deng W, Zheng Z, Wu K, Sun F. CCL2 produced by pancreatic ductal adenocarcinoma is essential for the accumulation and activation of monocytic myeloid-derived suppressor cells. Immunity Inflammation Disease (2021) 9:1686–95. doi: 10.1002/iid3.523
76. Wang Y, Zhang X, Yang L, Xue J, Hu G. Blockade of CCL2 enhances immunotherapeutic effect of anti-PD1 in lung cancer. J Bone Oncol (2018) 11:27–32. doi: 10.1016/j.jbo.2018.01.002
77. Li BH, Garstka MA, Li ZF. Chemokines and their receptors promoting the recruitment of myeloid-derived suppressor cells into the tumor. Mol Immunol (2020) 117:201–15. doi: 10.1016/j.molimm.2019.11.014
78. Yang Y, Ye YC, Chen Y, Zhao JL, Gao CC, Han H, et al. Crosstalk between hepatic tumor cells and macrophages via wnt/β-catenin signaling promotes M2-like macrophage polarization and reinforces tumor malignant behaviors. Cell Death Disease (2018) 9:793. doi: 10.1038/s41419-018-0818-0
79. Zhang X, Shi M, Chen T, Zhang B. Characterization of the immune cell infiltration landscape in head and neck squamous cell carcinoma to aid immunotherapy. Mol Ther Nucleic Acids (2020) 22:298–309. doi: 10.1016/j.omtn.2020.08.030
80. Takeuchi Y, Tanegashima T, Sato E, Irie T, Sai A, Itahashi K, et al. Highly immunogenic cancer cells require activation of the WNT pathway for immunological escape. Sci Immunol (2021) 6:eabc6424. doi: 10.1126/sciimmunol.abc6424
81. Deng L, Meng T, Chen L, Wei W, Wang P. The role of ubiquitination in tumorigenesis and targeted drug discovery. Signal Transduct Target Ther (2020) 5:11. doi: 10.1038/s41392-020-0107-0
82. Zhang L, Sang M, Li Y, Li Y, Yuan E, Yang L, et al. WNT3 hypomethylation counteracts low activity of the wnt signaling pathway in the placenta of preeclampsia. Cell Mol Life Sci: CMLS. (2021) 78:6995–7008. doi: 10.1007/s00018-021-03941-4
83. Wang X, Zhang Z, Zeng X, Wang J, Zhang L, Song W, et al. Wnt/β-catenin signaling pathway in severe preeclampsia. J Mol Histol (2018) 49:317–27. doi: 10.1007/s10735-018-9770-7
84. Meurette O, Mehlen P. Notch signaling in the tumor microenvironment. Cancer Cell (2018) 34:536–48. doi: 10.1016/j.ccell.2018.07.009
85. Jackstadt R, van Hooff SR, Leach JD, Cortes-Lavaud X, Lohuis JO, Ridgway RA, et al. Epithelial NOTCH signaling rewires the tumor microenvironment of colorectal cancer to drive poor-prognosis subtypes and metastasis. Cancer Cell (2019) 36:319–36.e7. doi: 10.1016/j.ccell.2019.08.003
86. Jiang H, Zhou C, Zhang Z, Wang Q, Wei H, Shi W, et al. Jagged1-Notch1-deployed tumor perivascular niche promotes breast cancer stem cell phenotype through Zeb1. Nat Commun (2020) 11:5129. doi: 10.1038/s41467-020-18860-4
87. Marchetto NM, Begum S, Wu T, O’Besso V, Yarborough CC, Valero-Pacheco N, et al. Endothelial Jagged1 antagonizes Dll4/Notch signaling in decidual angiogenesis during early mouse pregnancy. Int J Mol Sci (2020) 21:6477. doi: 10.3390/ijms21186477
88. Levin HI, Sullivan-Pyke CS, Papaioannou VE, Wapner RJ, Kitajewski JK, Shawber CJ, et al. Dynamic maternal and fetal notch activity and expression in placentation. Placenta (2017) 55:5–12. doi: 10.1016/j.placenta.2017.04.014
89. Wu F, Yang J, Liu J, Wang Y, Mu J, Zeng Q, et al. Signaling pathways in cancer-associated fibroblasts and targeted therapy for cancer. Signal Transduct Target Ther (2021) 6:218. doi: 10.1038/s41392-021-00641-0
90. Yu HF, Zheng LW, Yang ZQ, Wang YS, Wang TT, Yue ZP, et al. TAZ as a novel regulator of oxidative damage in decidualization via Nrf2/ARE/Foxo1 pathway. Exp Mol Med (2021) 53:1307–18. doi: 10.1038/s12276-021-00655-2
91. Chen H, Song Y, Yang S, Fu J, Feng X, Huang W. YAP mediates human decidualization of the uterine endometrial stromal cells. Placenta (2017) 53:30–5. doi: 10.1016/j.placenta.2017.03.013
92. Najafi M, Farhood B, Mortezaee K. Extracellular matrix (ECM) stiffness and degradation as cancer drivers. J Cell Biochem (2019) 120:2782–90. doi: 10.1002/jcb.27681
93. Covarrubias AE, Barrence FC, Zorn TM. The absence of the embryo in the pseudopregnant uterus alters the deposition of some ECM molecules during decidualization in mice. Connective Tissue Res (2015) 56:253–63. doi: 10.3109/03008207.2015.1023432
94. Hassona Y, Cirillo N, Heesom K, Parkinson EK, Prime SS. Senescent cancer-associated fibroblasts secrete active MMP-2 that promotes keratinocyte dis-cohesion and invasion. Br J Cancer (2014) 111:1230–7. doi: 10.1038/bjc.2014.438
95. Thomé R, dos Santos HB, Sato Y, Rizzo E, Bazzoli N. Distribution of laminin β2, collagen type IV, fibronectin and MMP-9 in ovaries of the teleost fish. J Mol Histol (2010) 41:215–24. doi: 10.1007/s10735-010-9281-7
96. Zhang Y, Lin Z, Foolen J, Schoen I, Santoro A, Zenobi-Wong M, et al. Disentangling the multifactorial contributions of fibronectin, collagen and cyclic strain on MMP expression and extracellular matrix remodeling by fibroblasts. Matrix Biol: J Int Soc Matrix Biol (2014) 40:62–72. doi: 10.1016/j.matbio.2014.09.001
97. Murekatete B, Shokoohmand A, McGovern J, Mohanty L, Meinert C, Hollier BG, et al. Targeting insulin-like growth factor-I and extracellular matrix interactions in melanoma progression. Sci Rep (2018) 8:583. doi: 10.1038/s41598-017-19073-4
98. Turco MY, Moffett A. Development of the human placenta. Dev (Cambridge England) (2019) 146:dev163428. doi: 10.1242/dev.163428
99. Macklin PS, McAuliffe J, Pugh CW, Yamamoto A. Hypoxia and HIF pathway in cancer and the placenta. Placenta (2017) 56:8–13. doi: 10.1016/j.placenta.2017.03.010
100. de Heer EC, Jalving M, Harris AL. HIFs, angiogenesis, and metabolism: elusive enemies in breast cancer. J Clin Invest (2020) 130:5074–87. doi: 10.1172/JCI137552
101. Jing X, Yang F, Shao C, Wei K, Xie M, Shen H, et al. Role of hypoxia in cancer therapy by regulating the tumor microenvironment. Mol Cancer (2019) 18:157. doi: 10.1186/s12943-019-1089-9
102. Zheng H, Ning Y, Zhan Y, Liu S, Yang Y, Wen Q, et al. Co-Expression of PD-L1 and HIF-1α predicts poor prognosis in patients with non-small cell lung cancer after surgery. J Cancer (2021) 12:2065–72. doi: 10.7150/jca.53119
103. Qian Y, Li Y, Ge Y, Song W, Fan H. Elevated LncRNA TRERNA1 correlated with activation of HIF-1α predicts poor prognosis in hepatocellular carcinoma. Pathol Res Pract (2021) 227:153612. doi: 10.1016/j.prp.2021.153612
104. Leppänen J, Helminen O, Huhta H, Kauppila JH, Isohookana J, Haapasaari KM, et al. Weak HIF-1alpha expression indicates poor prognosis in resectable pancreatic ductal adenocarcinoma. World J Surg Oncol (2018) 16:127. doi: 10.1186/s12957-018-1432-4
105. Mao X, Xu J, Wang W, Liang C, Hua J, Liu J, et al. Crosstalk between cancer-associated fibroblasts and immune cells in the tumor microenvironment: new findings and future perspectives. Mol Cancer (2021) 20:131. doi: 10.1186/s12943-021-01428-1
106. Korbecki J, Kojder K, Kapczuk P, Kupnicka P, Gawrońska-Szklarz B, Gutowska I, et al. The effect of hypoxia on the expression of CXC chemokines and CXC chemokine receptors-a review of literature. Int J Mol Sci (2021) 22:843. doi: 10.3390/ijms22020843
107. Wang M, Zhao X, Zhu D, Liu T, Liang X, Liu F, et al. HIF-1α promoted vasculogenic mimicry formation in hepatocellular carcinoma through LOXL2 up-regulation in hypoxic tumor microenvironment. J Exp Clin Cancer Res: CR (2017) 36:60. doi: 10.1186/s13046-017-0533-1
108. Pickup MW, Mouw JK, Weaver VM. The extracellular matrix modulates the hallmarks of cancer. EMBO Rep (2014) 15:1243–53. doi: 10.15252/embr.201439246
109. Ding J, Zhang Y, Cai X, Zhang Y, Yan S, Wang J, et al. Extracellular vesicles derived from M1 macrophages deliver miR-146a-5p and miR-146b-5p to suppress trophoblast migration and invasion by targeting TRAF6 in recurrent spontaneous abortion. Theranostics (2021) 11:5813–30. doi: 10.7150/thno.58731
110. Windsperger K, Dekan S, Pils S, Golletz C, Kunihs V, Fiala C, et al. Extravillous trophoblast invasion of venous as well as lymphatic vessels is altered in idiopathic, recurrent, spontaneous abortions. Hum Reprod (Oxford England) (2017) 32:1208–17. doi: 10.1093/humrep/dex058
111. Cha J, Sun X, Dey SK. Mechanisms of implantation: strategies for successful pregnancy. Nat Med (2012) 18:1754–67. doi: 10.1038/nm.3012
112. Salker MS, Christian M, Steel JH, Nautiyal J, Lavery S, Trew G, et al. Deregulation of the serum- and glucocorticoid-inducible kinase SGK1 in the endometrium causes reproductive failure. Nat Med (2011) 17:1509–13. doi: 10.1038/nm.2498
113. Abalos E, Cuesta C, Grosso AL, Chou D, Say L. Global and regional estimates of preeclampsia and eclampsia: a systematic review. Eur J Obstet Gynecol Reprod Biol (2013) 170:1–7. doi: 10.1016/j.ejogrb.2013.05.005
114. Trogstad L, Magnus P, Moffett A, Stoltenberg C. The effect of recurrent miscarriage and infertility on the risk of pre-eclampsia. BJOG: an Int J Obstet Gynaecol (2009) 116:108–13. doi: 10.1111/j.1471-0528.2008.01978.x
115. Rana S, Lemoine E, Granger JP, Karumanchi SA. Preeclampsia: Pathophysiology, challenges, and perspectives. Circ Res (2019) 124:1094–112. doi: 10.1161/CIRCRESAHA.118.313276
116. Aplin JD, Myers JE, Timms K, Westwood M. Tracking placental development in health and disease. Nat Rev Endocrinol (2020) 16:479–94. doi: 10.1038/s41574-020-0372-6
117. Garrido-Gómez T, Castillo-Marco N, Cordero T, Simón C. Decidualization resistance in the origin of preeclampsia. Am J Obstet Gynecol (2022) 226(2S):S886–94 doi: 10.1016/j.ajog.2020.09.039
118. Conrad KP, Rabaglino MB, Post Uiterweer ED. Emerging role for dysregulated decidualization in the genesis of preeclampsia. Placenta (2017) 60:119–29. doi: 10.1016/j.placenta.2017.06.005
119. Dimitriadis E, Menkhorst E, Saito S, Kutteh WH, Brosens JJ. Recurrent pregnancy loss. Nat Rev Dis Primers (2020) 6:98. doi: 10.1038/s41572-020-00228-z
120. Wang Y. Wnt/Planar cell polarity signaling: a new paradigm for cancer therapy. Mol Cancer Ther (2009) 8:2103–9. doi: 10.1158/1535-7163.MCT-09-0282
121. Bloomfield V, Rogers S, Leyland N. Placenta accreta spectrum. CMAJ: Can Med Assoc J J l’Association Medicale Canadienne (2020) 192:E980. doi: 10.1503/cmaj.200304
122. Bartels HC, Postle JD, Downey P, Brennan DJ. Placenta accreta spectrum: A review of pathology, molecular biology, and biomarkers. Dis Markers (2018) 2018:1507674. doi: 10.1155/2018/1507674
123. Jauniaux E, Bunce C, Grønbeck L, Langhoff-Roos J. Prevalence and main outcomes of placenta accreta spectrum: a systematic review and meta-analysis. Am J Obstet Gynecol (2019) 221:208–18. doi: 10.1016/j.ajog.2019.01.233
124. Morlando M, Collins S. Placenta accreta spectrum disorders: Challenges, risks, and management strategies. Int J Women’s Health (2020) 12:1033–45. doi: 10.2147/IJWH.S224191
125. Jauniaux E, Collins S, Burton GJ. Placenta accreta spectrum: pathophysiology and evidence-based anatomy for prenatal ultrasound imaging. Am J Obstet Gynecol (2018) 218:75–87. doi: 10.1016/j.ajog.2017.05.067
126. Tuzović L, Djelmis J, Ilijić M. Obstetric risk factors associated with placenta previa development: case-control study. Croatian Med J (2003) 44:728–33.
127. Usta IM, Hobeika EM, Musa AA, Gabriel GE, Nassar AH. Placenta previa-accreta: Risk factors and complications. Am J Obstet Gynecol (2005) 193:1045–9. doi: 10.1016/j.ajog.2005.06.037
128. Garmi G, Goldman S, Shalev E, Salim R. The effects of decidual injury on the invasion potential of trophoblastic cells. Obstet Gynecol (2011) 117:55–9. doi: 10.1097/AOG.0b013e31820094f3
129. King JC, Butte NF, Bronstein MN, Kopp LE, Lindquist SA. Energy metabolism during pregnancy: Influence of maternal energy status. Am J Clin Nutr (1994) 59:439s–45s. doi: 10.1093/ajcn/59.2.439S
130. Biswas AK, Acharyya S. Understanding cachexia in the context of metastatic progression. Nat Rev Cancer (2020) 20:274–84. doi: 10.1038/s41568-020-0251-4
131. Martin L, Birdsell L, Macdonald N, Reiman T, Clandinin MT, McCargar LJ, et al. Cancer cachexia in the age of obesity: Skeletal muscle depletion is a powerful prognostic factor, independent of body mass index. J Clin Oncol (2013) 31:1539–47. doi: 10.1200/JCO.2012.45.2722
132. Ni X, Yang J, Li M. Imaging-guided curative surgical resection of pancreatic cancer in a xenograft mouse model. Cancer Lett (2012) 324:179–85. doi: 10.1016/j.canlet.2012.05.013
133. Gallagher IJ, Stephens NA, MacDonald AJ, Skipworth RJ, Husi H, Greig CA, et al. Suppression of skeletal muscle turnover in cancer cachexia: Evidence from the transcriptome in sequential human muscle biopsies. Clin Cancer Res (2012) 18:2817–27. doi: 10.1158/1078-0432.CCR-11-2133
134. Salazar-Degracia A, Granado-Martínez P, Millán-Sánchez A, Tang J, Pons-Carreto A, Barreiro E. Reduced lung cancer burden by selective immunomodulators elicits improvements in muscle proteolysis and strength in cachectic mice. J Cell Physiol (2019) 234:18041–52. doi: 10.1002/jcp.28437
135. Yaribeygi H, Farrokhi FR, Butler AE, Sahebkar A. Insulin resistance: Review of the underlying molecular mechanisms. J Cell Physiol (2019) 234:8152–61. doi: 10.1002/jcp.27603
136. Liu Y, Kuang A, Talbot O, Bain JR, Muehlbauer MJ, Hayes MG, et al. Metabolomic and genetic associations with insulin resistance in pregnancy. Diabetologia (2020) 63:1783–95. doi: 10.1007/s00125-020-05198-1
137. Zhu S, Surampudi P, Field NT, Chondronikola M. Meal timing and glycemic control during pregnancy-is there a link? Nutrients (2021) 13:3379. doi: 10.3390/nu13103379
138. Catalano PM. Trying to understand gestational diabetes. Diabetic Med: J Br Diabetic Assoc (2014) 31:273–81. doi: 10.1111/dme.12381
139. Phelps RL, Metzger BE, Freinkel N. Carbohydrate metabolism in pregnancy. XVII. diurnal profiles of plasma glucose, insulin, free fatty acids, triglycerides, cholesterol, and individual amino acids in late normal pregnancy. Am J Obstet Gynecol (1981) 140:730–6. doi: 10.1016/0002-9378(81)90731-6
140. Barbour LA, Shao J, Qiao L, Pulawa LK, Jensen DR, Bartke A, et al. Human placental growth hormone causes severe insulin resistance in transgenic mice. Am J Obstet Gynecol (2002) 186:512–7. doi: 10.1067/mob.2002.121256
141. Gluckman PD, Pinal CS. Maternal-placental-fetal interactions in the endocrine regulation of fetal growth: role of somatotrophic axes. Endocrine (2002) 19:81–9. doi: 10.1385/ENDO:19:1:81
142. Yoshikawa T, Noguchi Y, Doi C, Makino T, Nomura K. Insulin resistance in patients with cancer: Relationships with tumor site, tumor stage, body-weight loss, acute-phase response, and energy expenditure. Nutr (Burbank Los Angeles County Calif) (2001) 17:590–3. doi: 10.1016/S0899-9007(01)00561-5
143. Kumar A, Sundaram K, Mu J, Dryden GW, Sriwastva MK, Lei C, et al. High-fat diet-induced upregulation of exosomal phosphatidylcholine contributes to insulin resistance. Nat Commun (2021) 12:213. doi: 10.1038/s41467-020-20500-w
144. Yoshikawa T, Noguchi Y, Matsumoto A. Effects of tumor removal and body weight loss on insulin resistance in patients with cancer. Surgery (1994) 116:62–6.
145. Poulia KA, Sarantis P, Antoniadou D, Koustas E, Papadimitropoulou A, Papavassiliou AG, et al. Pancreatic cancer and cachexia-metabolic mechanisms and novel insights. Nutrients (2020) 12:1543. doi: 10.3390/nu12061543
146. Kampmann U, Knorr S, Fuglsang J, Ovesen P. Determinants of maternal insulin resistance during pregnancy: An updated overview. J Diabetes Res (2019) 2019:5320156. doi: 10.1155/2019/5320156
147. Dev R, Bruera E, Dalal S. Insulin resistance and body composition in cancer patients. Ann Oncol (2018) 29:ii18–26. doi: 10.1093/annonc/mdx815
148. Wang X, Hu Z, Hu J, Du J, Mitch WE. Insulin resistance accelerates muscle protein degradation: Activation of the ubiquitin-proteasome pathway by defects in muscle cell signaling. Endocrinology (2006) 147:4160–8. doi: 10.1210/en.2006-0251
149. Sun Z, Yang S, Zhou Q, Wang G, Song J, Li Z, et al. Emerging role of exosome-derived long non-coding RNAs in tumor microenvironment. Mol Cancer (2018) 17:82. doi: 10.1186/s12943-018-0831-z
150. Pillay P, Moodley K, Vatish M, Moodley J. Exosomal MicroRNAs in pregnancy provides insight into a possible cure for cancer. Int J Mol Sci (2020) 21:5384. doi: 10.3390/ijms21155384
151. Ignatiadis M, Sledge GW, Jeffrey SS. Liquid biopsy enters the clinic - implementation issues and future challenges. Nat Rev Clin Oncol (2021) 18:297–312. doi: 10.1038/s41571-020-00457-x
152. Bianchi DW, Chiu RWK. Sequencing of circulating cell-free DNA during pregnancy. New Engl J Med (2018) 379:464–73. doi: 10.1056/NEJMra1705345
153. Fox-Fisher I, Piyanzin S, Ochana BL, Klochendler A, Magenheim J, Peretz A, et al. Remote immune processes revealed by immune-derived circulating cell-free DNA. eLife (2021) 10:1243–57. doi: 10.1038/s41422-019-0182-3.
154. Li W, Zhang X, Lu X, You L, Song Y, Luo Z, et al. 5-hydroxymethylcytosine signatures in circulating cell-free DNA as diagnostic biomarkers for human cancers. Cell Res (2017) 27:1243–57. doi: 10.1038/cr.2017.121
155. Ngo TTM, Moufarrej MN, Rasmussen MH, Camunas-Soler J, Pan W, Okamoto J, et al. Noninvasive blood tests for fetal development predict gestational age and preterm delivery. Sci (New York NY) (2018) 360:1133–6. doi: 10.1126/science.aar3819
156. Karin-Kujundzic V, Sola IM, Predavec N, Potkonjak A, Somen E, Mioc P, et al. Novel epigenetic biomarkers in pregnancy-related disorders and cancers. Cells (2019) 8:464–73. doi: 10.3390/cells8111459
157. Liang L, Rasmussen MH, Piening B, Shen X, Chen S, Röst H, et al. Metabolic dynamics and prediction of gestational age and time to delivery in pregnant women. Cell (2020) 181:1680–92.e15. doi: 10.1097/OGX.0000000000000864
158. Wowdzia JB, McHugh TL, Thornton J, Sivak A, Mottola MF, Davenport MH. Elite athletes and pregnancy outcomes: A systematic review and meta-analysis. Med Sci Sports Exercise (2021) 53:534–42. doi: 10.1249/MSS.0000000000002510
159. Yang QJ, Zhao JR, Hao J, Li B, Huo Y, Han YL, et al. Serum and urine metabolomics study reveals a distinct diagnostic model for cancer cachexia. J Cachexia Sarcopenia Muscle (2018) 9:71–85. doi: 10.1002/jcsm.12246
160. Le A, Udupa S, Zhang C. The metabolic interplay between cancer and other diseases. Trends Cancer (2019) 5:809–21. doi: 10.1016/j.trecan.2019.10.012
161. Clarke GS, Gatford KL, Young RL, Grattan DR, Ladyman SR, Page AJ. Maternal adaptations to food intake across pregnancy: Central and peripheral mechanisms. Obes (Silver Spring Md) (2021) 29:1813–24. doi: 10.1002/oby.23224
162. Slepicka PF, Cyrill SL, Dos Santos CO. Pregnancy and breast cancer: Pathways to understand risk and prevention. Trends Mol Med (2019) 25:866–81. doi: 10.1016/j.molmed.2019.06.003
163. Merritt MA, Cramer DW. Molecular pathogenesis of endometrial and ovarian cancer. Cancer biomarkers: section A Dis Markers (2010) 9:287–305. doi: 10.3233/CBM-2011-0167
Keywords: Decidua, placenta, TME (tumor microenvironment), cancer, cachexia, placenta diseases
Citation: Pang H, Lei D, Guo Y, Yu Y, Liu T, Liu Y, Chen T and Fan C (2022) Three categories of similarities between the placenta and cancer that can aid cancer treatment: Cells, the microenvironment, and metabolites. Front. Oncol. 12:977618. doi: 10.3389/fonc.2022.977618
Received: 27 June 2022; Accepted: 29 July 2022;
Published: 18 August 2022.
Edited by:
Federica Sotgia, University of Salford, United KingdomReviewed by:
Lais Viana, State University of Campinas, BrazilCopyright © 2022 Pang, Lei, Guo, Yu, Liu, Liu, Chen and Fan. This is an open-access article distributed under the terms of the Creative Commons Attribution License (CC BY). The use, distribution or reproduction in other forums is permitted, provided the original author(s) and the copyright owner(s) are credited and that the original publication in this journal is cited, in accordance with accepted academic practice. No use, distribution or reproduction is permitted which does not comply with these terms.
*Correspondence: Cuifang Fan, fancuifang@whu.edu.cn