- 1Key Laboratory of Digestive Diseases of Gansu Province, Lanzhou University Second Hospital, Lanzhou, China
- 2The Second Clinical Medical College, Lanzhou University, Lanzhou, China
- 3Department of Gastroenterology, Lanzhou University Second Hospital, Lanzhou, China
- 4Department of Pathology, The 940th Hospital of the Joint Logistic Support of the People’s Liberation Army, Lanzhou, China
- 5Department of Pathology, Lanzhou University Second Hospital, Lanzhou, China
- 6Department of general surgery, Lanzhou University Second Hospital, Lanzhou, China
Background: Colon cancer (CC) is a common tumor, but its pathogenesis is still not well understood. Competitive endogenous RNA (ceRNA) theory, ferroptosis and tumor immune infiltration may be the mechanisms of the development of cancer. The purpose of the study is to seek genes connected with both immunity and ferroptosis, and provide important molecular basis for early noninvasive diagnosis and immunotherapy of CC.
Methods: We extracted messenger RNA (mRNA), microRNA (miRNA), and long noncoding RNA (lncRNA) data of CC from The Cancer Genome Atlas database (TCGA), identified the differentially expressed mRNA (DEmRNA), miRNA (DEmiRNA) and lncRNA (DElncRNA), then constructed a ceRNA network. Venn overlap analysis was used to identify genes associated with immunity and ferroptosis in ceRNA network. The expression and prognosis of target genes were analyzed via Gene Expression Profiling Interactive Analysis (GEPIA) and PrognoScan database, and we analysed the related functions and signaling pathways of target genes by enrichment analysis. The correlation between target genes and tumor immune infiltrating was explored by CIBERSORT and spearman correlation analysis. Finally, the expression of target genes was detected via quantitative reverse transcription-PCR (qRT-PCR) in CC and normal colon tissues.
Results: Results showed that there were 4 DElncRNA, 4 DEmiRNA and 126 DEmRNA in ceRNA network. NADPH oxidase 4 protein (NOX4) was a DEmRNA associated with immunity and ferroptosis in ceRNA network. NOX4 was highly expressed in CC and connected with unfavourable prognosis. NOX4 was obviously enriched in pathways connected with carcinogenesis and significantly correlated with six kinds of immune cells. Immune checkpoints and NOX4 spearman correlation analysis showed that the expression of NOX4 was positively related to programmed cell death protein 1 (PD-1)-PDCD1, programmed cell death-Ligand 1 (PD-L1)-CD274 and cytotoxic T-lymphocyte-associated protein 4 (CTLA4).
Conclusions: To conclude, our study suggests that NOX4 is associated with both ferroptosis and tumor immunity, and might be a biomarker associated with the carcinogenesis, prognosis of CC and a potential target of CC immunotherapy.
Introduction
CC is the third most common cancer worldwide. From 2014 to 2019, the incidence of CC was 36.5 percent and the death rate was 13.4 percent in the United States (1). In recent years, due to the lack of clear early symptoms and concern about the pain of colonoscopy, most CC patients are in advanced stage at the time of diagnosis and have developed distant metastases with poor prognosis (2–4). Therefore, non-invasive early diagnosis and treatment of CC are urgently needed to improve the early recognition rate and prolong the lifetime of CC patients. Now, the standard treatment for advanced CC combines neoadjuvant chemoradiotherapy, molecular-targeted therapy and immunotherapy (5–10). In recent years, the development of cancer immunotherapy is very rapid. The therapy aims to activate the immune system to attack cancer cells through natural mechanisms and improve anti-tumor immune response with fewer off-target effects (11). For example, the remarkable development of immune checkpoint inhibitors has shown surprising clinical efficacy. To be specific, the therapy uses antibodies that block the CTLA-4 and PD-1 pathways to treat cancer patients (12). Similarly, Chen et al. show that PD-L1 expression is upregulated in many human tumors, and the antibodies block the PD-L1/PD-1 interaction, resulting in tumor regression in mice (13). However, the majority of CC patients are not eligible for the treatment, suggesting that cancer immunotherapy still needs more research to elucidate the molecular mechanisms and to identify useful biomarkers (14).
It is well known noncoding RNA, including lncRNA and miRNA, are among the major components of the human transcriptome (15, 16). According to the ceRNA theory, lncRNA and mRNA competitively bind to miRNA, due to the strong affinity between lncRNA and miRNA, the binding of miRNA and mRNA is inhibited, resulting in the weakened inhibitory effect of miRNA on mRNA and the increase of mRNA expression closely related to tumorigenesis (17, 18). The bioinformatic analyses about ceRNA related with CC or other cancers have been studied by many researchers in recent years (19–24).
Ferroptosis is an iron-dependent process of modulating cell death with excessive oxidation of phospholipids (25). In recent years, many studies have reported a correlation between ferroptosis and cancer. For example, Wang et al. report that the ferroptosis-related gene circRNA_101093 is essential for protecting lung adenocarcinoma cells from ferroptosis injury (26). Zhang et al. show that adenylate cyclase10 (ADCY10) is an ferroptosis-related gene and promotes the formation of lung adenocarcinoma. Therefore, lung adenocarcinoma patients with high ADCY10 expression may benefit from ferroptosis therapy. The authors conclude that further studies are needed to identify other genes associated with prognosis and ferroptosis of cancer patients (25). On the other hand, studies have shown that reactive oxygen species (ROS) is produced accompanied by ferroptosis, which is closely related to carcinogenesis by inducing DNA double-strands break and oncogene activation (27–31). Study has shown that ferroptotic cancer cells may secret the immune modulators such as Prostaglandin E2 to disturb the effect of immunotherapy, which raises the correlation between ferroptosis and tumor immunity (32, 33). However, the relationship between ferroptosis and immune infiltration in CC has been less studied.
The purpose of the study is to seek genes connected with both immunity and ferroptosis and provide important molecular basis for early noninvasive diagnosis and immunotherapy of CC. In our study, we constructed a ceRNA network and identified NOX4 as target genes associated with immunity and ferroptosis. We used GEPIA, PrognoScan database and qRT-PCR to analyze the NOX4 expression and prognosis, we analysed the related functions and signaling pathways of NOX4 by enrichment analysis. The correlation between NOX4 and tumor immune infiltrating was explored by CIBERSORT and spearman correlation analysis. The flow chart of the whole article was shown in Supplementary 1.
Methods
Data download
We downloaded the clinical and RNA sequencing data of 371 CC patients from TCGA database (https://portal.gdc.cancer.gov/) using the Data Transfer Tool (34), including 387 CC tissues and 38 normal colon tissues.
Identification of differentially expressed RNA as well as construction of the ceRNA network
“Limma” package of R language (35, 36) was used to obtain DElncRNA, DEmiRNA and DEmRNA with P < 0.05 and absolute value of log2 fold change (|logFC|) > 2.0, “ggplot2” package (37) was used for visualization of volcanos. We used RNAInter database (www.rnainter.org) to identify potential relationships between lncRNA–miRNA and miRNA–mRNA (38), “Venn Diagram” package (39) was used to draw the interaction between DEmiRNA–mRNA an DEmRNA. Finally, we used the Cytoscape to construct a ceRNA network (40).
Acquisition of target genes associated with immunity and ferroptosis in ceRNA network
We used the FerrDb database (http://www.zhounan.org/ferrdb/current/) to acquire the ferroptosis-related genes (41), and the ImmPort database (https://www.immport.org/shared/home) to acquire the immune-related genes (42). The overlapping target genes were identified by Venn overlap analysis.
Expression profile analysis of target genes
We applied TIMER database (https://cistrome.shinyapps.io/timer/) (43) to analyze the expression of target genes in pan-cancer, GEPIA database (http://gepia.cancer-pku.cn/) to analyze the target genes’ expression in CC (44).
Survival analysis
GEPIA database (44) and PrognoScan database (http://dna00.bio.kyutech.ac.jp/PrognoScan/) (45) were applied to analysis the prognosis of target genes. Median expression of target genes was used as cut off value.
Functional enrichment analysis
The Gene Ontology (GO) as well as Kyoto Encyclopedia of Genes and Genomes (KEGG) enrichment analysis were performed by the “clusterProfiler” package (46).
Gene set enrichment analysis
We used GSEA to explore functions of target genes (47). Firstly, the expression data of mRNA gene sets were obtained by R language. We divided CC patients into low expression group and high expression group according to the median of target genes expression. Then, we used GSEA _4.2.3 software to analysis.
Immune−related analysis of target genes
Twenty-two types of immune cells in different tissue samples were evaluated by CIBERSORT algorithm (48). Spearman analysis was used to evaluate the correlations among the expression of target genes selected in ceRNA network, immune cells, and immune checkpoints CTLA4, PD-1 and PD-L1. We performed visualization of correlations using “Ggplot2” package and “pheatmap” package (49).
Clinical tissues collection
We collected normal colon and CC specimens from 19 CC patients, during colectomy between April 25, 2021 and June 6, 2021, in the Lanzhou University Second Hospital.
qRT-PCR
We used RNA lysis solution to extract total RNA (Accurate Biotechnology, China). Evo M-MLV RT Kit II was used to synthesize the first-strand cDNA of mRNA (Accurate Biotechnology, China). CFX 96 real-time PCR system was used to amplify the cDNA. Primer sequences were as follows, β-actin, forward: TGGAACGCTTCACGAATTTGCG, revers: CTAAGTCATAGTCCGCCTAGAAGCA; NOX4, forward: CAGATGTTGGGGCTAGGATTG, revers: GAGTGTTCGGCACATGGGTA.
Statistical analysis
P < 0.05 was statistically significant. In SPSS23.0 software, we used T-test, Mann-Whitney U test or Wilcoxon rank sum test to count the results.
Results
Acquisition of differentially expressed RNA
We identified 1732 DEmRNA (684 upregulated and 1,048 downregulated), 507 DElncRNA (193 upregulated and 314 downregulated), 45 DEmiRNA (5 upregulated and 40 downregulated) between CC and colon normal specimens. The maps of volcanic distribution were shown in Figure 1.
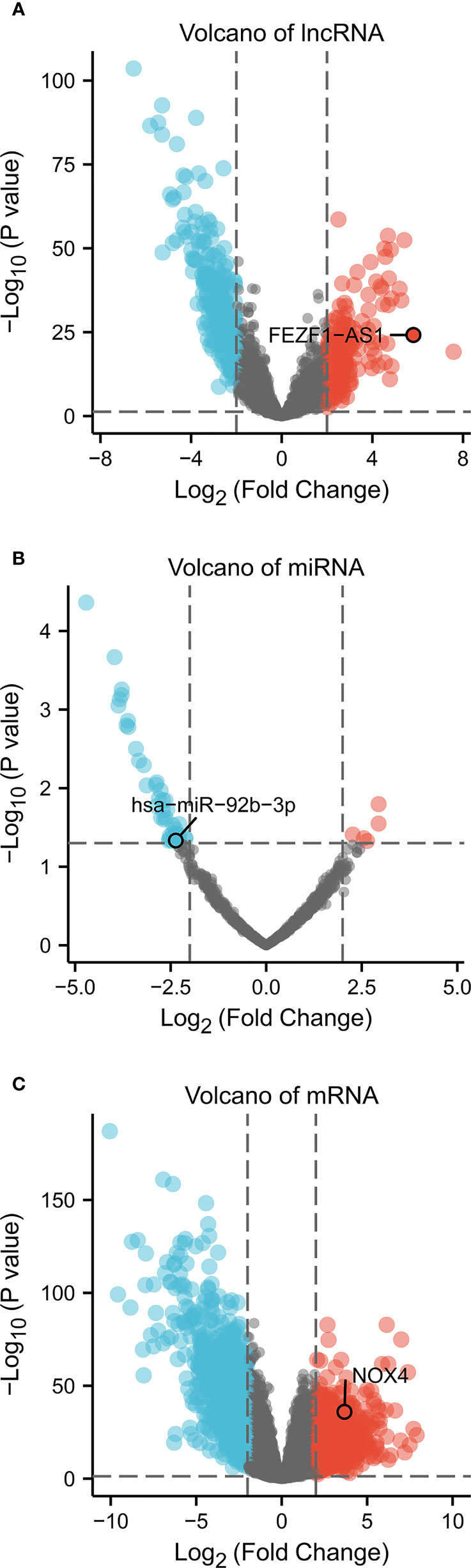
Figure 1 Volcano of differentially expressed RNA in CC (absolute of logFC > 2, P Value < 0.05). Volcano plots of (A) 507 DElncRNA, (B) 45 DEmiRNA, and (C) 1,732 DEmRNA. Red indicates upregulated RNA, and blue represents downregulated RNA. The x-axis depicts log2 Fold Change, the y-axis depicts -log10 (P Value).
Prediction of the target DElncRNA/DEmRNA of miRNA as well as construction of the ceRNA network
We predicted 1,686 lncRNA–miRNA interactions and 19,185 mRNA–miRNA interactions by using RNAInter database and input the 8 DEmiRNA into the RNAInter database to identify the target DElncRNA/DEmRNA. There were 9170 mRNA predicted as the target genes of 8 DEmiRNA, the intersection of these 9,170 mRNA and 1732 DEmRNA resulted in 774 DEmRNA (259 upregulated and 515 downregulated) (Figure 2A). There were 19 DElncRNA predicted as the target genes of 8 DEmiRNA. Thus, we obtained the interaction between DElncRNA–DEmiRNA and DEmiRNA–DEmRNA. To identify highly expressed oncogenes (logFC of lncRNA and mRNA >2, and logFC of miRNA< -2), we identified 4 DElncRNA, 4 DEmiRNA, and 126 DEmRNA to construct the ceRNA network (Figure 2B).
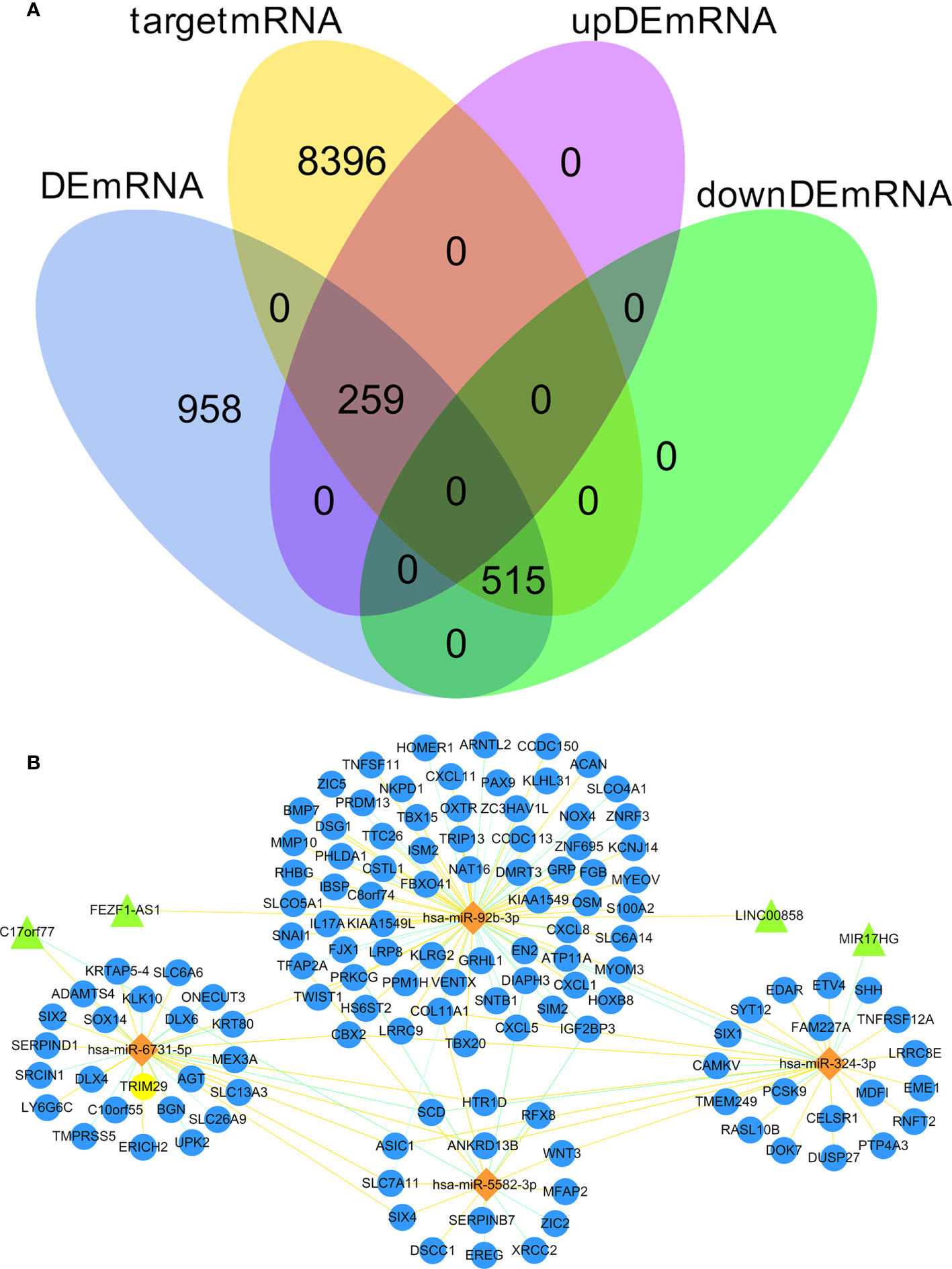
Figure 2 Venn diagram of gene interactions and ceRNA network. (A) In the 774 differentially expressed target genes, 259 were up-regulated and 515 down-regulated. The yellow circle represents 9170 target mRNA, the blue circle represents 1732 DEmRNA, the pink circle represents 259 up-regulated differentially expressed target genes, and the green circle represents 515 down-regulated differentially expressed target genes. (B) The ceRNA network in CC. Triangles denote lncRNA, diamonds denote miRNA, and circles denote mRNA.
Acquisition of genes association with ferroptosis and immune in ceRNA network
Genes associated with immune and ferroptosis were downloaded from ImmPort and FerrDb databases, as shown in Supplementary 2. We used Venn overlap analysis to obtain the overlapping target genes among immune-related genes, ferroptosis-related genes, and 126 DEmRNA of ceRNA network. The results showed that NOX4 was overlapping target gene (Figure 3).
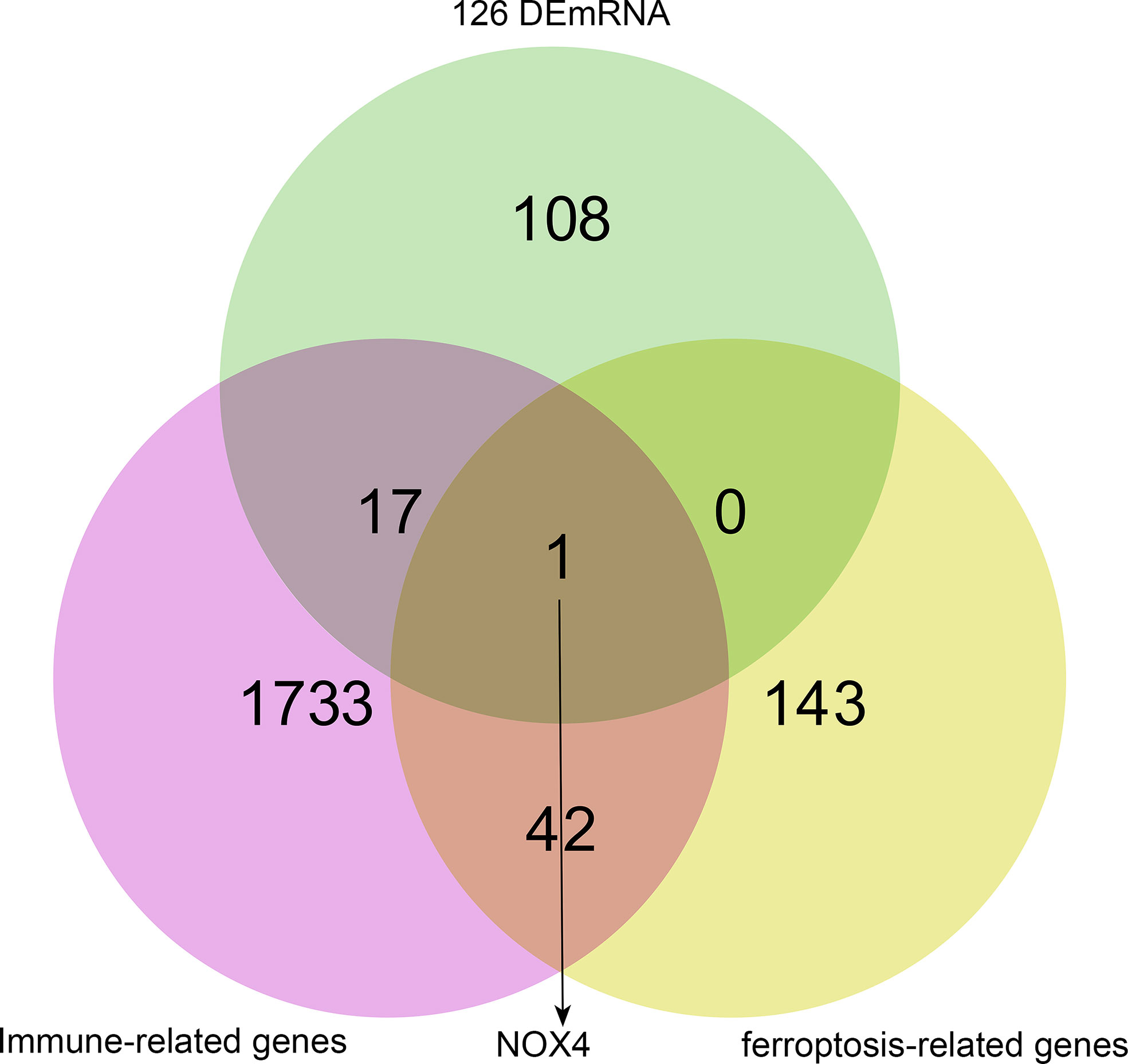
Figure 3 NOX4 was both ferroptosis-related gene and immune-related gene in ceRNA network. The pink circle represents immune-related genes, the yellow circle represents ferroptosis-related genes, the green circle represents 126 DEmRNA.
NOX4 was highly expressed in CC and was connected with bad prognosis
The expression of NOX4 in pan-cancer from TIMER database was shown in Figure 4A. We visualized the expression of NOX4 from TCGA database and GEPIA database, as shown in Figures 4B and C. Then, we collected 19 paired fresh CC and normal colon tissues for qRT-PCR analysis (Figure 4D). The results showed that compared with normal colon tissues, NOX4 was higher expressed in CC. Survival analysis showed that CC patients with high NOX4 expression had poor disease-free survival and overall survival, as shown in Figures 4E–G.
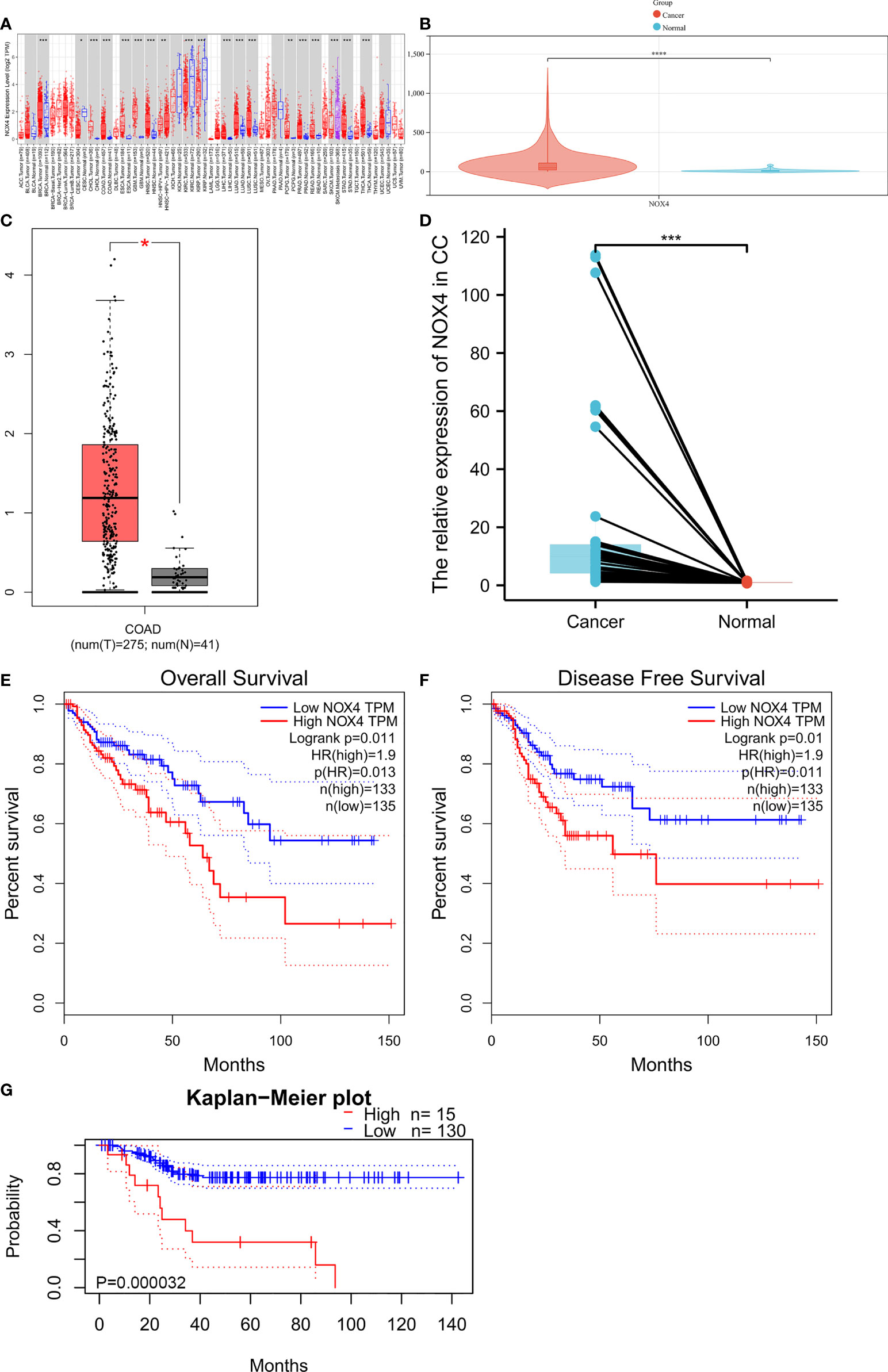
Figure 4 NOX4 was upregulated in CC and associated with poor prognosis. (A) The expression of NOX4 in pan-cancer from TIMER database. (B) The expression of NOX4 in CC from TCGA database. (C) The expression of NOX4 in CC from GEPIA database. (D) The expression of NOX4 in 19 fresh normal colon and CC tissues (n=19). (E) Overall survival curve for NOX4 in GEPIA database. (F) Disease free survival curve for NOX4 in GEPIA database. (G) Overall survival curve for NOX4 in PrognoScan database. *P < 0.05, **P < 0.01, ***P < 0.001, ****P < 0.0001.
Functional enrichment of NOX4 and its co-expressed mRNA
GO includes three components: molecular functions (MF), cellular components (CC), and biological processes (BP). KEGG analysis indicated NOX4 and its co-expressed mRNA were enriched in a total of 12 signaling pathways, the results were shown in Figures 5A, B. Co-expressed mRNA were shown in Supplementary 3.
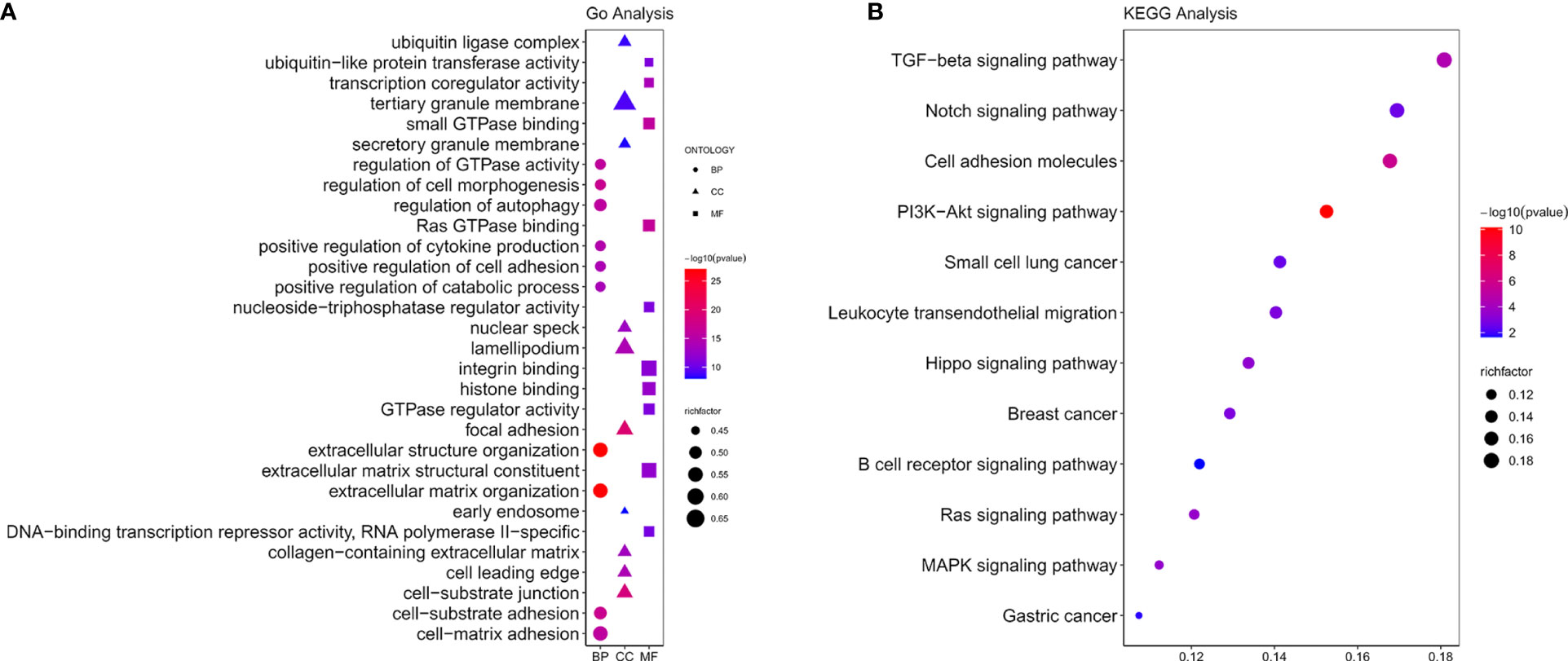
Figure 5 Functional enrichment analysis. (A) GO functions of NOX4 and its co-expressed mRNA. (B) The KEGG pathway analysis of NOX4 and its co-expressed mRNA.
NOX4 related signaling pathways were analyzed by GSEA
In CC patients with high NOX4 expression, GSEA analysis indicated the upregulated hallmark gene sets were mainly enriched to pathways connected with tumorigenesis and immune response, mainly including IL6 JAK STAT3 signaling, interferon gamma response, TNF-α signaling via NF-κB, angiogenesis, KRAS signaling up, and interferon alpha response (Figure 6). However, in CC patients with low NOX4 expression, the significantly downregulated hallmark gene sets were enriched to MYC Targets V1, G2M Checkpoint, MYC Targets V2, DNA Repair, and E2F Targets (Figure 7).
Correlation between NOX4 expression and tumor immunity
We evaluated the tumor infiltrating immune cell composition in tissues by CIBERSORT algorithm, as shown in Figures 8A, B. The expression of NOX4 was significantly correlated with different types of immune cells, such as CD4+ T cells (p =1.57e-18, r = 0.40), B cells (p =0.023, r = 0.11), CD8+ T cells (p = 3.41e-18, r = 0.39), Neutrophil cells (p = 3.85e-36, r = 0.54), Macrophage cells (p = 4.93e-40, r = 0.57), and Myeloid dendritic cells (p = 1.13e-40, r = 0.57) (Figures 9A–F). Spearman correlation analysis indicated NOX4 expression was positively correlated with PD-1 (PDCD1) expression (p = 7.01e-10, r = 0.28), PD-L1 (CD274) expression (p = 3.06 e-21, r = 0.42), and CTLA4 (p = 3.2e-19, r = 0.40) (Figures 9G-I).
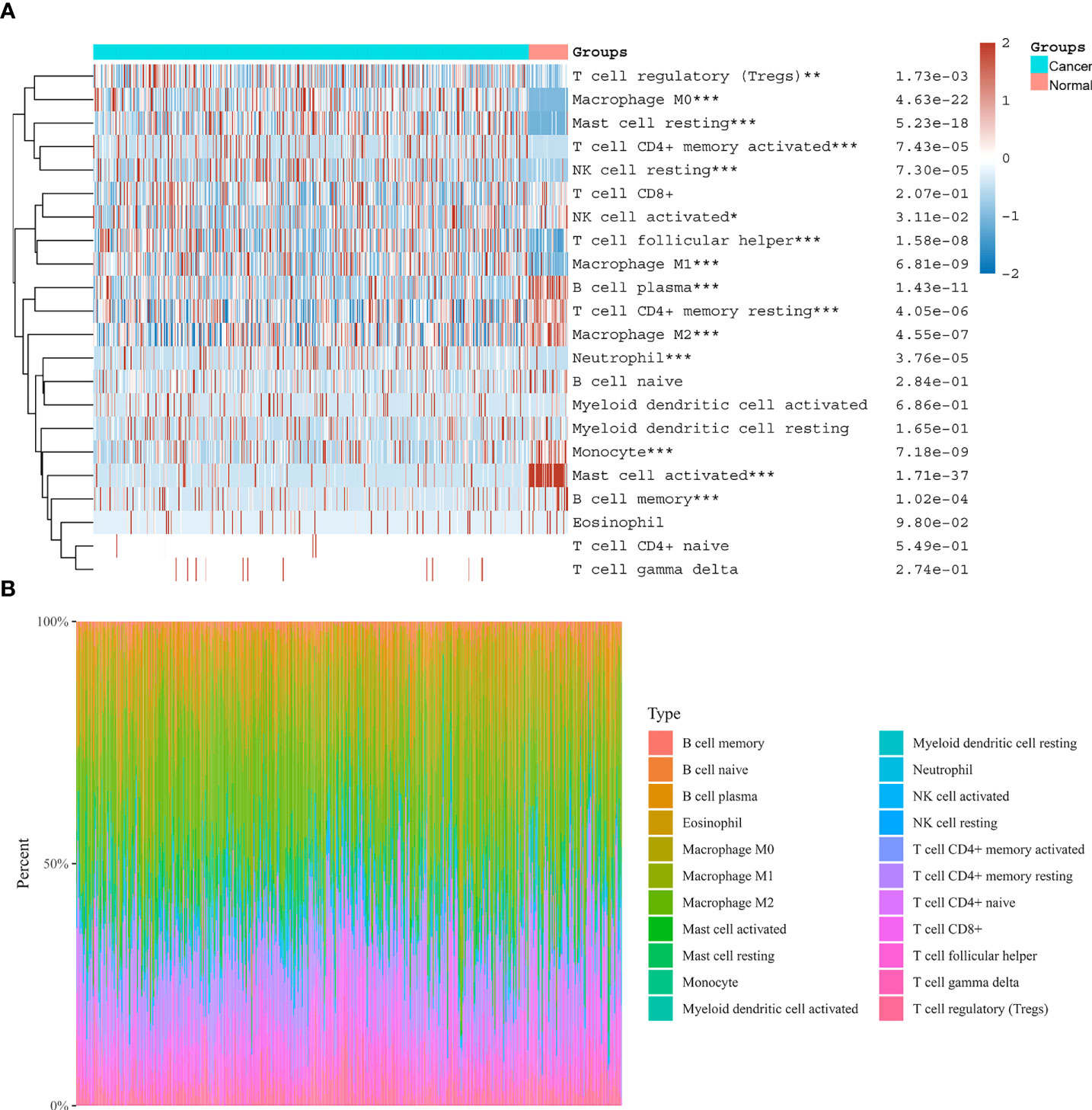
Figure 8 Distribution of immune cells in CC and normal colon tissues. (A) Heatmap of immune cell scores. Abscissa represents different groups, ordinate represents immune cell types. Cancer represents CC tissues, Normal represents normal colon tissues. (B) The proportion of tumor infiltrating immune cells in each CC sample by the CIBERSORT algorithm. Different colors represent different immune cell types. The horizontal axis represents samples, and the vertical axis represents the percentage of each immune cell in each sample. *P < 0.05, **P < 0.01, ***P < 0.001.
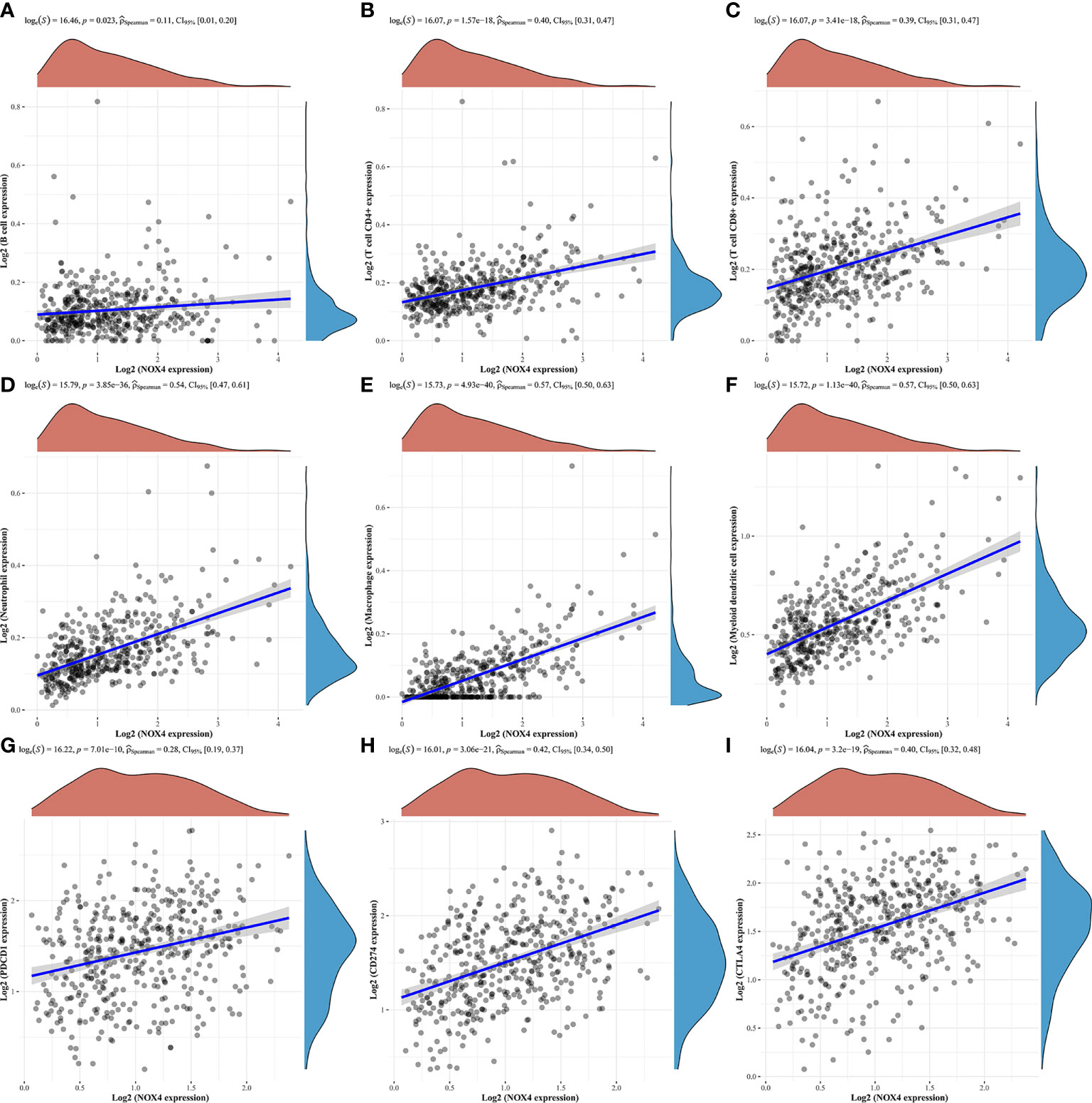
Figure 9 Correlation of NOX4 expression with tumor infiltrating immune cells and immune checkpoints in the tumor microenvironment. NOX4 was significantly correlated with (A) B cells, (B) CD4+ T cells, (C) CD8+ T cells, (D) Neutrophil cells, (E) Macrophage cells, (F) Myeloid dendritic cells, (G) PD-1 (PDCD1), (H) PD-L1 (CD274), and (I) CTLA4 in the TCGA database. P < 0.05.
Discussion
The early diagnosis rate of CC is low, many patients develop drug resistance, and the mortality rate of CC patients has not decreased (2). Immunotherapy is regarded as an innovative treatment for cancer patients. However, due to lack of effective predictive biomarkers, limited clinical efficacy, and treatment-related adverse events, the broader clinical application of immunotherapy is limited (50). Therefore, it is necessary to further search for immune-related genes with diagnostic and therapeutic value for CC.
We acquired clinic information and transcriptome data of CC patients from the TCGA database, obtained the DElncRNA, DEmiRNA and DEmRNA, then we constructed the ceRNA network. There were 126 DEmRNA in ceRNA network, and finally we have found NOX4, a DEmRNA, was related to both ferroptosis and immunity. NOX4 is a member of NADPH oxidase, and can be induced when cells are in a state of ischemia and hypoxia (51–53). Studies have shown that ROS participates in many key cellular processes, such as proliferation, DNA damage response and angiogenesis, which are obviously connected with the occurrence of tumors (54, 55). NOX4 is a key enzyme in ROS production, which makes it to be more and more attractive to researchers (56). It has been indicated that NOX4 promotes cells migration in CC (57), breast cancer (58–60) as well as pancreatic cancer (61). NOX4 also can regulate the cell cycle to promote the carcinogenesis of melanoma and urothelium (62, 63). Xiao et al. (14) has confirmed that NOX4, as an ferroptosis-related gene, is an effective biomarker for the occurrence of gastric cancer. According to the TIMER database, TCGA database and GEPIA database, NOX4 expression was higher in CC than in colon normal samples, moreover, we confirmed the high expression of NOX4 in CC by collecting fresh clinical surgical specimens. Results from the PrognoScan database and GEPIA database showed that NOX4 was related to disease free survival and overall survival of CC patients, suggesting that NOX4 could be a biomarker to assess the prognosis of CC patients.
Based on GO and KEGG pathway enrichment analysis, we found that NOX4 and its co-expressed mRNA exhibited enrichments for signaling pathways such as PI3K/AKT, TGF-β, MAPK signaling and many more, which were connected with the tumor immune evasion as well as the occurrence of tumors. On the one hand, Fridlender et al. confirm that TGF-β signal transduction in tumor microenvironment promotes the aggregation of N2-like tumor-associated neutrophils, further increasing the tumor immune evasion (64). Regulatory T cells (Tregs) are immune-regulatory subsets of T cells. In the tumor microenvironment, abnormally activated TGF-β signaling makes T cells into Tregs, inhibiting natural killer (NK) cells from killing tumor cells and promoting immunosuppressive effect (65, 66). Conversely, NK cells are activated by inhibition of TGF-β signal transduction (67). On the other hand, Zonneville and Itatani et al. demonstrate that TGF-β signaling in the microenvironment of a tumor plays a key role in angiogenesis (68, 69). Study has shown that over activated TGF-β pathway induces MAPK signaling and PI3K/AKT signaling (70). As we all known, PI3K/AKT is one of the signaling pathways in connection with tumorigenesis. Its overactivation leads to abnormal cell cycle progression, inhibits apoptosis, and inducts angiogenesis (71, 72). Bishnupuri et al. (73) report PI3K/AKT signaling pathway is connected with the occurrence of CC. The above results also reflected the relationship between NOX4 and tumor development, and tumor immunosuppression.
In the CC patients with high NOX4 expression, GSEA analysis enriched to IFN-γ response, angiogenesis, IL6 JAK STAT3 signaling, KRAS signaling up, IFN-α response, and TNFα signaling via NF-κB, which were related to tumorigenesis and immune response. Our correlation analysis showed that NOX4 was significantly related to the expressions of CTLA4, PD-L1 (CD274), and PD-1 (PDCD1), acting as immune checkpoints. Elise et al. (74) suggests that IFN-γ increases the expression of PD-L1 in cancer cells, indirectly over-activating the PD-1/PD-L1 signaling pathway. PD-1, which belongs to the CD28 family, is mostly expressed on activated T cells and inhibits host immunity by binding to PD-L1 (75–79). Studies report that cancer cells are protected from the toxic effects of CD8+ T cells by activation of PD-1/PD-L1 signaling pathway, leading to apoptosis and depletion of T cells (75, 80). CTLA-4 is also a T cell inhibitory receptor that binds to CD80 and CD86 expressed by myeloid dendritic cells and inhibits early activation of T cells (81). Based on the above studies, it may be possible to combine PD-1/PD-L1 with NOX4 as immunotherapy targets for CC. Pro-inflammatory mediators such as IL-22, IL-6, IL-2, and IL-17 play a key part in maintaining tumor microenvironment, promoting immunosuppression, angiogenesis, and coordinating the interactions between immune cells (81). It shows IL-6 induces myelogenesis through the STAT3 signaling pathway, inhibits mature bone marrow cells from differentiating, and stimulates the formation of Myeloid-derived suppressor cells (MDSCs) (82). In addition, IL-6 activates MDSCs through the STAT3-NF-κB-IDO pathway in invasive breast cancer (83). MDSCs are immunosuppressive cells that can directly promote the formation of tumor stem cells and protect proliferating tumor cells from apoptosis (84, 85). In the CC patients with low NOX4 expression, GSEA analysis enriched to MYC Targets V1, MYC Targets V2, oxidative phosphorylation, DNA Repair and so on. It has been shown oxidative phosphorylation promotes tumor growth in vivo (86). As a oncogene, MYC can regulates tumor DNA repair, growth, metabolism and apoptosis (87). These findings indicated that NOX4 had potential value in the tumorigenesis and immunosuppression of CC.
We used CIBERSORT algorithm to evaluate the infiltrating immune cell composition in tissues. The results showed that a large proportion of immunosuppressive cells infiltrated in CC tissues. Tumor cells with high expression of ferroptosis related genes recruit and reprogram numerous immunosuppressive cells by secreting cytokines, chemokines, ROS and other proinflammatory mediators. Studies have shown that tumor cells, immunosuppressive cells and tumor stromal cells form an acidic tumor microenvironment, which makes tumor cells more conducive to escape immune surveillance of the host and promotes growth, invasion and metastasis of tumor cells (32, 81, 88). In tumor microenvironment, tumor immunosuppression includes inhibition of activity of CD8+T cells and NK, abnormal function of Myeloid dendritic cells, abnormal transformation from Th1 to Th2, as well as enhanced activity of immunosuppressive cells, including Tregs and MDSCs (81). Our correlation analysis indicated NOX4 was positively connected with the expressions of Neutrophile, CD4+T cells, Macrophage, CD8+T, Myeloid dendritic cells, and B cells. Oshima’s results show cancer cells expressing membrane-Type1-matrix metalloproteinase (MMP) are surrounded by macrophages, leading to the activation of MMP2, which increases the migration and invasion of cancer cells (89). Researches have demonstrated that a tumor’s microenvironment can control tumor occurrence and development through macrophages and myeloid dendritic cells (90, 91). Our results indicated that in the microenvironment of CC tumors, NOX4, a ferroptosis-related gene, was associated closely with immune infiltration and immunosuppression.
Conclusions
To conclude, our study suggests that NOX4 is associated with both ferroptosis and tumor immunity, and may be a biomarker associated with the prognosis and tumorigenesis of CC and a potential target for immunotherapy of CC in the future.
Data availability statement
Publicly available datasets were analyzed in this study. This data can be found here: TCGA database (https://portal.gdc.cancer.gov/).
Ethics statement
The studies involving human participants were reviewed and approved by Lanzhou University Second Hospital medical ethics committee (approval number: 2021A-468). The patients/participants provided their written informed consent to participate in this study.
Author contributions
XY, PW, and ZRW: software learning, data analysis, and results visualization. XY, ZPW, and JG: experimental verification. XY and XS: manuscript writing. DZ and YY: conceptualization and design, and administration and funding acquisition. All authors contributed to the article and approved the submitted version.
Funding
This study was supported by Cuiying Scientific and Technological Innovation Program of Lanzhou University Second Hospital (NO. CY2021-QN-A05); Lanzhou Science and technology project (NO. 2022-ZD-104); Cuiying Scientific and Technological Key Cultivation Program of Lanzhou University Second Hospital (CY2018-ZD01). Industrial support and guidance project for institutions of higher learning in Gansu province (2019C-21).
Acknowledgments
Thanks to TCGA database and RNAInter database for data acquisition, Cuiying Biomedical Research Center for their support of our laboratory equipment and laboratory technology.
Conflict of interest
The authors declare that the research was conducted in the absence of any commercial or financial relationships that could be construed as a potential conflict of interest.
Publisher’s note
All claims expressed in this article are solely those of the authors and do not necessarily represent those of their affiliated organizations, or those of the publisher, the editors and the reviewers. Any product that may be evaluated in this article, or claim that may be made by its manufacturer, is not guaranteed or endorsed by the publisher.
Supplementary material
The Supplementary Material for this article can be found online at: https://www.frontiersin.org/articles/10.3389/fonc.2022.968043/full#supplementary-material
References
1. Siegel RL, Miller KD, Fuchs HE, Jemal A. Cancer statistics, 2022. CA: Cancer J For Clin (2022) 72(1):7–33. doi: 10.3322/caac.21708
2. Wu H, Qin W, Lu S, Wang X, Zhang J, Sun T, et al. Long noncoding rna Zfas1 promoting small nucleolar rna-mediated 2’-O-Methylation Via Nop58 recruitment in colorectal cancer. Mol Cancer (2020) 19(1):95. doi: 10.1186/s12943-020-01201-w
3. Zhang X, Zhang H, Shen B, Sun X-F. Chromogranin-a expression as a novel biomarker for early diagnosis of colon cancer patients. Int J Mol Sci (2019) 20(12):2919. doi: 10.3390/ijms20122919
4. Ludwig JA, Weinstein JN. Biomarkers in cancer staging, prognosis and treatment selection. Nat Rev Cancer (2005) 5(11):845–56. doi: 10.1038/nrc1739
5. Wang H, Wang L, Xie Z, Zhou S, Li Y, Zhou Y, et al. Nitric oxide (No) and no synthases (Nos)-based targeted therapy for colon cancer. Cancers (Basel) (2020) 12(7):1881. doi: 10.3390/cancers12071881
6. Georgieva M, Gospodinova Z, Keremidarska-Markova M, Kamenska T, Gencheva G, Krasteva N. Pegylated nanographene oxide in combination with near-infrared laser irradiation as a smart nanocarrier in colon cancer targeted therapy. Pharmaceutics (2021) 13(3):424. doi: 10.3390/pharmaceutics13030424
7. Du W, Yang X, He S, Wang J, Guo Y, Kou B, et al. Novel hyaluronic acid oligosaccharide-loaded and Cd44v6-targeting oxaliplatin nanoparticles for the treatment of colorectal cancer. Drug Delivery (2021) 28(1):920–9. doi: 10.1080/10717544.2021.1914777
8. Lopes N, McIntyre C, Martin S, Raverdeau M, Sumaria N, Kohlgruber AC, et al. Distinct metabolic programs established in the thymus control effector functions of Γδ T cell subsets in tumor microenvironments. Nat Immunol (2021) 22(2):179–92. doi: 10.1038/s41590-020-00848-3
9. Wu X, Yang H, Chen X, Gao J, Duan Y, Wei D, et al. Nano-herb medicine and pdt induced synergistic immunotherapy for colon cancer treatment. Biomaterials (2021) 269:120654. doi: 10.1016/j.biomaterials.2021.120654
10. Ding D, Zhong H, Liang R, Lan T, Zhu X, Huang S, et al. Multifunctional nanodrug mediates synergistic photodynamic therapy and mdscs-targeting immunotherapy of colon cancer. Adv Sci (Weinh) (2021) 8(14):e2100712. doi: 10.1002/advs.202100712
11. Riley RS, June CH, Langer R, Mitchell MJ. Delivery technologies for cancer immunotherapy. Nat Rev Drug Discovery (2019) 18(3):175–96. doi: 10.1038/s41573-018-0006-z
12. Yang Y. Cancer immunotherapy: Harnessing the immune system to battle cancer. J Clin Invest (2015) 125(9):3335–7. doi: 10.1172/JCI83871
13. Dong H, Strome SE, Salomao DR, Tamura H, Hirano F, Flies DB, et al. Tumor-associated B7-H1 promotes T-cell apoptosis: A potential mechanism of immune evasion. Nat Med (2002) 8(8):793–800. doi: 10.1038/nm730
14. Xiao R, Wang S, Guo J, Liu S, Ding A, Wang G, et al. Ferroptosis-related gene Nox4, Chac1 and Hif1a are valid biomarkers for stomach adenocarcinoma. J Cell Mol Med (2022) 26(4):1183–93. doi: 10.1111/jcmm.17171
15. Weng M, Wu D, Yang C, Peng H, Wang G, Wang T, et al. Noncoding rnas in the development, diagnosis, and prognosis of colorectal cancer. Transl Res (2017) 181:108–20. doi: 10.1016/j.trsl.2016.10.001
16. Ezkurdia I, Juan D, Rodriguez JM, Frankish A, Diekhans M, Harrow J, et al. Multiple evidence strands suggest that there may be as few as 19,000 human protein-coding genes. Hum Mol Genet (2014) 23(22):5866–78. doi: 10.1093/hmg/ddu309
17. Tan X, Banerjee P, Liu X, Yu J, Gibbons DL, Wu P, et al. The epithelial-to-Mesenchymal transition activator Zeb1 initiates a prometastatic competing endogenous rna network. J Clin Invest (2018) 128(4):1267–82. doi: 10.1172/JCI97225
18. Chang L, Yuan Y, Li C, Guo T, Qi H, Xiao Y, et al. Upregulation of Snhg6 regulates Zeb1 expression by competitively binding mir-101-3p and interacting with Upf1 in hepatocellular carcinoma. Cancer Lett (2016) 383(2):183–94. doi: 10.1016/j.canlet.2016.09.034
19. Zhuang M, Zhao S, Jiang Z, Wang S, Sun P, Quan J, et al. Malat1 sponges mir-106b-5p to promote the invasion and metastasis of colorectal cancer Via Slain2 enhanced microtubules mobility. EBioMedicine (2019) 41:286–98. doi: 10.1016/j.ebiom.2018.12.049
20. Liang H, Yu T, Han Y, Jiang H, Wang C, You T, et al. Lncrna ptar promotes emt and invasion-metastasis in serous ovarian cancer by competitively binding mir-101-3p to regulate Zeb1 expression. Mol Cancer (2018) 17(1):119. doi: 10.1186/s12943-018-0870-5
21. Li C, Wan L, Liu Z, Xu G, Wang S, Su Z, et al. Long non-coding rna xist promotes tgf-B-Induced epithelial-mesenchymal transition by regulating mir-367/141-Zeb2 axis in non-Small-Cell lung cancer. Cancer Lett (2018) 418:185–95. doi: 10.1016/j.canlet.2018.01.036
22. Yao J, Yang Z, Yang J, Wang Z-G, Zhang Z-Y. Long non-coding rna Fezf1-As1 promotes the proliferation and metastasis of hepatocellular carcinoma Via targeting mir-107/Wnt/B-Catenin axis. Aging (Albany NY) (2021) 13:13726–38. doi: 10.18632/aging.202960
23. Zhang Z, Sun L, Zhang Y, Lu G, Li Y, Wei Z. Long non-coding rna Fezf1-As1 promotes breast cancer stemness and tumorigenesis Via targeting mir-30a/Nanog axis. J Cell Physiol (2018) 233(11):8630–8. doi: 10.1002/jcp.26611
24. Dasgupta P, Kulkarni P, Majid S, Hashimoto Y, Shiina M, Shahryari V, et al. Lncrna Cdkn2b-As1/Mir-141/Cyclin d network regulates tumor progression and metastasis of renal cell carcinoma. Cell Death Dis (2020) 11(8):660. doi: 10.1038/s41419-020-02877-0
25. Zhang X, Yu K, Ma L, Qian Z, Tian X, Miao Y, et al. Endogenous glutamate determines ferroptosis sensitivity Via Adcy10-dependent yap suppression in lung adenocarcinoma. Theranostics (2021) 11(12):5650–74. doi: 10.7150/thno.55482
26. Zhang X, Xu Y, Ma L, Yu K, Niu Y, Xu X, et al. Essential roles of exosome and Circrna_101093 on ferroptosis desensitization in lung adenocarcinoma. Cancer Commun (Lond) (2022) 42(4):287–313. doi: 10.1002/cac2.12275
27. Dolma S, Lessnick SL, Hahn WC, Stockwell BR. Identification of genotype-selective antitumor agents using synthetic lethal chemical screening in engineered human tumor cells. Cancer Cell (2003) 3(3):285–96. doi: 10.1016/S1535-6108(03)00050-3
28. Yang WS, Stockwell BR. Synthetic lethal screening identifies compounds activating iron-dependent, nonapoptotic cell death in oncogenic-Ras-Harboring cancer cells. Chem Biol (2008) 15(3):234–45. doi: 10.1016/j.chembiol.2008.02.010
29. Dixon SJ, Lemberg KM, Lamprecht MR, Skouta R, Zaitsev EM, Gleason CE, et al. Ferroptosis: An iron-dependent form of nonapoptotic cell death. Cell (2012) 149(5):1060–72. doi: 10.1016/j.cell.2012.03.042
30. Käsmann L, Eze C, Taugner J, Roengvoraphoj O, Dantes M, Schmidt-Hegemann N-S, et al. Chemoradioimmunotherapy of inoperable stage iii non-small cell lung cancer: Immunological rationale and current clinical trials establishing a novel multimodal strategy. Radiat Oncol (2020) 15(1):167. doi: 10.1186/s13014-020-01595-3
31. Torti SV, Manz DH, Paul BT, Blanchette-Farra N, Torti FM. Iron and cancer. Annu Rev Nutr (2018) 38:97–125. doi: 10.1146/annurev-nutr-082117-051732
32. Friedmann Angeli JP, Krysko DV, Conrad M. Ferroptosis at the crossroads of cancer-acquired drug resistance and immune evasion. Nat Rev Cancer (2019) 19(7):405–14. doi: 10.1038/s41568-019-0149-1
33. Zhu D, Wu S, Li Y, Zhang Y, Chen J, Ma J, et al. Ferroptosis-related gene Slc1a5 is a novel prognostic biomarker and correlates with immune infiltrates in stomach adenocarcinoma. Cancer Cell Int (2022) 22(1):124. doi: 10.1186/s12935-022-02544-8
34. Weinstein JN, Collisson EA, Mills GB, Shaw KRM, Ozenberger BA, Ellrott K, et al. The cancer genome atlas pan-cancer analysis project. Nat Genet (2013) 45(10):1113–20. doi: 10.1038/ng.2764
35. Tippmann S. Programming tools: Adventures with r. Nature (2015) 517(7532):109–10. doi: 10.1038/517109a
36. Ritchie ME, Phipson B, Wu D, Hu Y, Law CW, Shi W, et al. Limma powers differential expression analyses for rna-sequencing and microarray studies. Nucleic Acids Res (2015) 43(7):e47. doi: 10.1093/nar/gkv007
37. Postma M, Goedhart J. Plotsofdata-a web app for visualizing data together with their summaries. PloS Biol (2019) 17(3):e3000202. doi: 10.1371/journal.pbio.3000202
38. Lin Y, Liu T, Cui T, Wang Z, Zhang Y, Tan P, et al. Rnainter in 2020: Rna interactome repository with increased coverage and annotation. Nucleic Acids Res (2020) 48(D1):D189–D97:Epub 2020/01/08. doi: 10.1093/nar/gkz804
39. Jia A, Xu L, Wang Y. Venn Diagrams in bioinformatics. Briefings In Bioinf (2021) 22(5):bbab108. doi: 10.1093/bib/bbab108
40. Shannon P, Markiel A, Ozier O, Baliga NS, Wang JT, Ramage D, et al. Cytoscape: A software environment for integrated models of biomolecular interaction networks. Genome Res (2003) 13(11):2498–504. doi: 10.1101/gr.1239303
41. Zhou N, Bao J. Ferrdb: A manually curated resource for regulators and markers of ferroptosis and ferroptosis-disease associations. Database (Oxford) (2020) 2020:baaa021. doi: 10.1093/database/baaa021
42. Dai Y, Qiang W, Lin K, Gui Y, Lan X, Wang D. An immune-related gene signature for predicting survival and immunotherapy efficacy in hepatocellular carcinoma. Cancer Immunol Immunother (2021) 70(4):967–79. doi: 10.1007/s00262-020-02743-0
43. Li T, Fan J, Wang B, Traugh N, Chen Q, Liu JS, et al. Timer: A web server for comprehensive analysis of tumor-infiltrating immune cells. Cancer Res (2017) 77(21):e108–e10. doi: 10.1158/0008-5472.CAN-17-0307
44. Tang Z, Li C, Kang B, Gao G, Li C, Zhang Z. Gepia: A web server for cancer and normal gene expression profiling and interactive analyses. Nucleic Acids Res (2017) 45(W1):W98–102. doi: 10.1093/nar/gkx247
45. Pan JH, Zhou H, Cooper L, Huang JL, Zhu SB, Zhao XX, et al. Layn is a prognostic biomarker and correlated with immune infiltrates in gastric and colon cancers. Front Immunol (2019) 10:6. doi: 10.3389/fimmu.2019.00006
46. Yu G, Wang L-G, Han Y, He Q-Y. Clusterprofiler: An r package for comparing biological themes among gene clusters. OMICS (2012) 16(5):284–7. doi: 10.1089/omi.2011.0118
47. Subramanian A, Tamayo P, Mootha VK, Mukherjee S, Ebert BL, Gillette MA, et al. Gene set enrichment analysis: A knowledge-based approach for interpreting genome-wide expression profiles. Proc Natl Acad Sci U.S.A. (2005) 102(43):15545–50. doi: 10.1073/pnas.0506580102
48. Chong W, Shang L, Liu J, Fang Z, Du F, Wu H, et al. Ma regulator-based methylation modification patterns characterized by distinct tumor microenvironment immune profiles in colon cancer. Theranostics (2021) 11(5):2201–17. doi: 10.7150/thno.52717
49. Fang Y, Huang S, Han L, Wang S, Xiong B. Comprehensive analysis of peritoneal metastasis sequencing data to identify Linc00924 as a prognostic biomarker in gastric cancer. Cancer Manag Res (2021) 13:5599–611. doi: 10.2147/CMAR.S318704
50. Camidge DR, Doebele RC, Kerr KM. Comparing and contrasting predictive biomarkers for immunotherapy and targeted therapy of nsclc. Nat Rev Clin Oncol (2019) 16(6):341–55. doi: 10.1038/s41571-019-0173-9
51. Cheng G, Cao Z, Xu X, van Meir EG, Lambeth JD. Homologs of Gp91phox: Cloning and tissue expression of Nox3, Nox4, and Nox5. Gene (2001) 269(1-2):131–40. doi: 10.1016/S0378-1119(01)00449-8
52. Vermot A, Petit-Härtlein I, Smith SME, Fieschi F. Nadph oxidases (Nox): An overview from discovery, molecular mechanisms to physiology and pathology. Antioxidants (Basel) (2021) 10(6):890. doi: 10.3390/antiox10060890
53. Casas AI, Geuss E, Kleikers PWM, Mencl S, Herrmann AM, Buendia I, et al. Nox4-dependent neuronal autotoxicity and bbb breakdown explain the superior sensitivity of the brain to ischemic damage. Proc Natl Acad Sci U.S.A. (2017) 114(46):12315–20. doi: 10.1073/pnas.1705034114
54. Bonner MY, Arbiser JL. Targeting nadph oxidases for the treatment of cancer and inflammation. Cell Mol Life Sci (2012) 69(14):2435–42. doi: 10.1007/s00018-012-1017-2
55. Weyemi U, Dupuy C. The emerging role of ros-generating nadph oxidase Nox4 in DNA-damage responses. Mutat Res (2012) 751(2):77–81. doi: 10.1016/j.mrrev.2012.04.002
56. Martyn KD, Frederick LM, von Loehneysen K, Dinauer MC, Knaus UG. Functional analysis of Nox4 reveals unique characteristics compared to other nadph oxidases. Cell Signal (2006) 18(1):69–82. doi: 10.1016/j.cellsig.2005.03.023
57. Bauer KM, Watts TN, Buechler S, Hummon AB. Proteomic and functional investigation of the colon cancer relapse-associated genes Nox4 and Itga3. J Proteome Res (2014) 13(11):4910–8. doi: 10.1021/pr500557n
58. Tobar N, Guerrero J, Smith PC, Martínez J. Nox4-dependent ros production by stromal mammary cells modulates epithelial mcf-7 cell migration. Br J Cancer (2010) 103(7):1040–7. doi: 10.1038/sj.bjc.6605847
59. Zhang B, Liu Z, Hu X. Inhibiting cancer metastasis Via targeting napdh oxidase 4. Biochem Pharmacol (2013) 86(2):253–66. doi: 10.1016/j.bcp.2013.05.011
60. Boudreau HE, Casterline BW, Rada B, Korzeniowska A, Leto TL. Nox4 involvement in tgf-beta and Smad3-driven induction of the epithelial-to-Mesenchymal transition and migration of breast epithelial cells. Free Radic Biol Med (2012) 53(7):1489–99. doi: 10.1016/j.freeradbiomed.2012.06.016
61. Hiraga R, Kato M, Miyagawa S, Kamata T. Nox4-derived ros signaling contributes to tgf-B-Induced epithelial-mesenchymal transition in pancreatic cancer cells. Anticancer Res (2013) 33(10):4431–8. doi: 10.0000/PMID24123012
62. Shimada K, Fujii T, Anai S, Fujimoto K, Konishi N. Ros generation Via Nox4 and its utility in the cytological diagnosis of urothelial carcinoma of the urinary bladder. BMC Urol (2011) 11:22. doi: 10.1186/1471-2490-11-22
63. Yamaura M, Mitsushita J, Furuta S, Kiniwa Y, Ashida A, Goto Y, et al. Nadph oxidase 4 contributes to transformation phenotype of melanoma cells by regulating G2-m cell cycle progression. Cancer Res (2009) 69(6):2647–54. doi: 10.1158/0008-5472.CAN-08-3745
64. Fridlender ZG, Sun J, Kim S, Kapoor V, Cheng G, Ling L, et al. Polarization of tumor-associated neutrophil phenotype by tgf-beta: “N1” versus “N2” tan. Cancer Cell (2009) 16(3):183–94. doi: 10.1016/j.ccr.2009.06.017
65. Shitara K, Nishikawa H. Regulatory T cells: A potential target in cancer immunotherapy. Ann N Y Acad Sci (2018) 1417(1):104–15. doi: 10.1111/nyas.13625
66. Yamada N, Kuranaga Y, Kumazaki M, Shinohara H, Taniguchi K, Akao Y. Colorectal cancer cell-derived extracellular vesicles induce phenotypic alteration of T cells into tumor-growth supporting cells with transforming growth factor-B1-Mediated suppression. Oncotarget (2016) 7(19):27033–43. doi: 10.18632/oncotarget.7041
67. Otegbeye F, Ojo E, Moreton S, Mackowski N, Lee DA, de Lima M, et al. Inhibiting tgf-beta signaling preserves the function of highly activated, in vitro expanded natural killer cells in aml and colon cancer models. PloS One (2018) 13(1):e0191358. doi: 10.1371/journal.pone.0191358
68. Zonneville J, Safina A, Truskinovsky AM, Arteaga CL, Bakin AV. Tgf-B signaling promotes tumor vasculature by enhancing the pericyte-endothelium association. BMC Cancer (2018) 18(1):670. doi: 10.1186/s12885-018-4587-z
69. Itatani Y, Kawada K, Yamamoto T, Sakai Y. Resistance to anti-angiogenic therapy in cancer-alterations to anti-vegf pathway. Int J Mol Sci (2018) 19(4):1232. doi: 10.3390/ijms19041232
70. Hao Y, Baker D, Ten Dijke P. Tgf-B-Mediated epithelial-mesenchymal transition and cancer metastasis. Int J Mol Sci (2019) 20(11):2767. doi: 10.3390/ijms20112767
71. Fruman DA, Chiu H, Hopkins BD, Bagrodia S, Cantley LC, Abraham RT. The Pi3k pathway in human disease. Cell (2017) 170(4):605–35. doi: 10.1016/j.cell.2017.07.029
72. Yang Q, Jiang W, Hou P. Emerging role of Pi3k/Akt in tumor-related epigenetic regulation. Semin Cancer Biol (2019) 59:112–24. doi: 10.1016/j.semcancer.2019.04.001
73. Bishnupuri KS, Alvarado DM, Khouri AN, Shabsovich M, Chen B, Dieckgraefe BK, et al. Ido1 and kynurenine pathway metabolites activate Pi3k-akt signaling in the neoplastic colon epithelium to promote cancer cell proliferation and inhibit apoptosis. Cancer Res (2019) 79(6):1138–50. doi: 10.1158/0008-5472.CAN-18-0668
74. Alspach E, Lussier DM, Schreiber RD. Interferon Γ and its important roles in promoting and inhibiting spontaneous and therapeutic cancer immunity. Cold Spring Harb Perspect Biol (2019) 11(3):a028480. doi: 10.1101/cshperspect.a028480
75. Gong J, Chehrazi-Raffle A, Reddi S, Salgia R. Development of pd-1 and pd-L1 inhibitors as a form of cancer immunotherapy: A comprehensive review of registration trials and future considerations. J Immunother Cancer (2018) 6(1):8. doi: 10.1186/s40425-018-0316-z
76. Mahoney KM, Freeman GJ, McDermott DF. The next immune-checkpoint inhibitors: Pd-1/Pd-L1 blockade in melanoma. Clin Ther (2015) 37(4):764–82. doi: 10.1016/j.clinthera.2015.02.018
77. Francisco LM, Sage PT, Sharpe AH. The pd-1 pathway in tolerance and autoimmunity. Immunol Rev (2010) 236:219–42. doi: 10.1111/j.1600-065X.2010.00923.x
78. Ostrand-Rosenberg S, Horn LA, Haile ST. The programmed death-1 immune-suppressive pathway: Barrier to antitumor immunity. J Immunol (2014) 193(8):3835–41. doi: 10.4049/jimmunol.1401572
79. Fife BT, Pauken KE. The role of the pd-1 pathway in autoimmunity and peripheral tolerance. Ann N Y Acad Sci (2011) 1217:45–59. doi: 10.1111/j.1749-6632.2010.05919.x
80. Rozali EN, Hato SV, Robinson BW, Lake RA, Lesterhuis WJ. Programmed death ligand 2 in cancer-induced immune suppression. Clin Dev Immunol (2012) 2012:656340. doi: 10.1155/2012/656340
81. Wang D, DuBois RN. Immunosuppression associated with chronic inflammation in the tumor microenvironment. Carcinogenesis (2015) 36(10):1085–93. doi: 10.1093/carcin/bgv123
82. Gabrilovich DI, Nagaraj S. Myeloid-derived suppressor cells as regulators of the immune system. Nat Rev Immunol (2009) 9(3):162–74. doi: 10.1038/nri2506
83. Yu J, Wang Y, Yan F, Zhang P, Li H, Zhao H, et al. Noncanonical nf-Kb activation mediates Stat3-stimulated ido upregulation in myeloid-derived suppressor cells in breast cancer. J Immunol (2014) 193(5):2574–86. doi: 10.4049/jimmunol.1400833
84. Cui TX, Kryczek I, Zhao L, Zhao E, Kuick R, Roh MH, et al. Myeloid-derived suppressor cells enhance stemness of cancer cells by inducing Microrna101 and suppressing the corepressor Ctbp2. Immunity (2013) 39(3):611–21. doi: 10.1016/j.immuni.2013.08.025
85. Di Mitri D, Toso A, Chen JJ, Sarti M, Pinton S, Jost TR, et al. Tumour-infiltrating gr-1+ myeloid cells antagonize senescence in cancer. Nature (2014) 515(7525):134–7. doi: 10.1038/nature13638
86. Sica V, Bravo-San Pedro JM, Stoll G, Kroemer G. Oxidative phosphorylation as a potential therapeutic target for cancer therapy. Int J Cancer (2020) 146(1):10–7. doi: 10.1002/ijc.32616
87. Duffy MJ, O’Grady S, Tang M, Crown J. Myc as a target for cancer treatment. Cancer Treat Rev (2021) 94:102154. doi: 10.1016/j.ctrv.2021.102154
88. Itatani Y, Kawada K, Sakai Y. Transforming growth factor-B signaling pathway in colorectal cancer and its tumor microenvironment. Int J Mol Sci (2019) 20(23):5822. doi: 10.3390/ijms20235822
89. Oshima H, Nakayama M, Han T-S, Naoi K, Ju X, Maeda Y, et al. Suppressing tgfβ signaling in regenerating epithelia in an inflammatory microenvironment is sufficient to cause invasive intestinal cancer. Cancer Res (2015) 75(4):766–76. doi: 10.1158/0008-5472.CAN-14-2036
90. Engblom C, Pfirschke C, Pittet MJ. The role of myeloid cells in cancer therapies. Nat Rev Cancer (2016) 16(7):447–62. doi: 10.1038/nrc.2016.54
Keywords: colon cancer (CC), ceRNA, NOX4, ferroptosis, immune infiltration, prognosis
Citation: Yang X, Yu Y, Wang Z, Wu P, Su X, Wu Z, Gan J and Zhang D (2022) NOX4 has the potential to be a biomarker associated with colon cancer ferroptosis and immune infiltration based on bioinformatics analysis. Front. Oncol. 12:968043. doi: 10.3389/fonc.2022.968043
Received: 13 June 2022; Accepted: 07 September 2022;
Published: 28 September 2022.
Edited by:
Jian Song, University Hospital Münster, GermanyReviewed by:
Xiao Zhang, Shanghai Jiao Tong University, ChinaYousef Nami, Agricultural Biotechnology Research Institute of Iran, Iran
Yalda Rahbar Saadat, Tabriz University of Medical Sciences, Iran
Copyright © 2022 Yang, Yu, Wang, Wu, Su, Wu, Gan and Zhang. This is an open-access article distributed under the terms of the Creative Commons Attribution License (CC BY). The use, distribution or reproduction in other forums is permitted, provided the original author(s) and the copyright owner(s) are credited and that the original publication in this journal is cited, in accordance with accepted academic practice. No use, distribution or reproduction is permitted which does not comply with these terms.
*Correspondence: Dekui Zhang, zhangdk8616@126.com