- 1Faculty of Odontology, Autonomous University of Chihuahua, Chihuahua, Mexico
- 2Faculty of Biological and Chemical Sciences, Autonomous University of Sinaloa, Culiacán, Mexico
- 3Autonomous University of Mexico City, Mexico City, Mexico
- 4Faculty of Biology, Autonomous University of Sinaloa, Culiacán, Mexico
- 5Faculty of Odontology , Autonomous University of Sinaloa, Culiacán, Mexico
Head and neck squamous cell carcinoma (HNSCC) originates in the squamous cell lining the mucosal surfaces of the head and neck region, including the oral cavity, nasopharynx, tonsils, oropharynx, larynx, and hypopharynx. The heterogeneity, anatomical, and functional characteristics of the patient make the HNSCC a complex and difficult-to-treat disease, leading to a poor survival rate and a decreased quality of life due to the loss of important physiologic functions and aggressive surgical injury. Alteration of driver-oncogenic and tumor-suppressing lncRNAs has recently been recently in HNSCC to obtain possible biomarkers for diagnostic, prognostic, and therapeutic approaches. This review provides current knowledge about the implication of lncRNAs in drug resistance mechanisms in HNSCC. Chemotherapy resistance is a major therapeutic challenge in HNSCC in which lncRNAs are implicated. Lately, it has been shown that lncRNAs involved in autophagy induced by chemotherapy and epithelial–mesenchymal transition (EMT) can act as mechanisms of resistance to anticancer drugs. Conversely, lncRNAs involved in mesenchymal–epithelial transition (MET) are related to chemosensitivity and inhibition of invasiveness of drug-resistant cells. In this regard, long non-coding RNAs (lncRNAs) play a pivotal role in both processes and are important for cancer detection, progression, diagnosis, therapy response, and prognostic values. As the involvement of more lncRNAs is elucidated in chemoresistance mechanisms, an improvement in diagnostic and prognostic tools could promote an advance in targeted and specific therapies in precision oncology.
Introduction
Cancer is a group of multifactorial diseases with an estimated 9.9 million deaths globally in 2020 (1). HNSCC is the sixth most common cancer in the world, accounting for more than 850,000 cases and 400,000 deaths every year (1). HNSCC originates in the squamous cell lining the mucosal surfaces of the head and neck region, involving the oral cavity, nasopharynx, tonsils, oropharynx, larynx, and hypopharynx (2, 3). The main risk factors related to them are smoking, alcohol consumption, betel nuts, smokeless tobacco, and viral infections, including Epstein–Barr and human papillomavirus (4). Nowadays, the treatment for advanced HNSCC includes chemotherapeutic agents, radiotherapy, and surgical resection, leading to mutilation of essential tissues that affect functions such as breathing, feeding, and speaking, thus decreasing the quality of life of patients (5). The heterogeneous nature of HNSCC leads to a poor 5-year overall survival rate due to its local invasion, chemoresistance, metastasis, and late diagnosis (2, 6).
Chemotherapy has been widely used in recent decades for cancer treatment. The combination of platinum-based 5-fluorouracil (5-FU) and DNA synthesis inhibitor cisplatin (CDDP) is still the main regimen for HNSCC (5). However, combinations like paclitaxel (PTX), carboplatin (CDBCA), and cetuximab have been proposed, with unpredictable results (7, 8). Recently, immune checkpoint blockade (ICB) treatment is gaining importance as an immunologic approach for cancer control. HNSCC has a high tumor mutational burden and a relatively high expression of programmed cell death-1-ligand 1 (PD-L1), making it eligible for ICB (9, 10). Nevertheless, drug resistance (DR) is still a key factor for HNSCC progression and poor prognosis (11). The detailed mechanisms of DR are not fully understood, but recent studies suggest that autophagy (12–14), epithelial–mesenchymal transition (EMT) (13, 15, 16), and cancer cell stemness (17–19) play a pivotal role in this major problem. Other mechanisms implied in DR are inactivation of the drug, multi-drug resistance, apoptosis suppression, alterations in the drug metabolism, epigenetic changes, changes in the drug targets, enhanced DNA-repair, and target gene amplification (20). Besides, there are also biological determinants of drug resistance such as tumor heterogeneity, physical barriers, immune system and tumor microenvironment, undruggable cancer drivers, and selective therapeutic pressure that induces changes in the tumor and its ecosystem, modifying the response of the cells to different drugs (21).
Recent studies indicate that non-coding RNAs (ncRNAs) comprise 98% of the total transcribed RNAs in the human genome, and although at first they were classified as “junk” transcriptional products, nowadays they play crucial roles in many biological processes modulating gene expression (22, 23). ncRNAs dysregulation contributes to an increasing number of human diseases, including cancer (2). Long non-coding RNAs (lncRNAs) are a class of functional RNA composed of at least 200 nucleotides (24). LncRNAs have a high transcriptional rate as they are involved in gene regulation at the transcriptional level in the nucleus and posttranscriptional level in the cytoplasm (25, 26). Moreover, lncRNAs are implicated in various cancer progression mechanisms, including proliferation, differentiation, autophagy, EMT, invasion, and metastasis (27–29). Increasing evidence suggests that lncRNAs are implicated in DR in different types of cancer, including HNSCC (30–33). In this regard, this review provides current knowledge about lncRNAs and their implication in DR through known processes in HNSCC, emphasizing in autophagy, EMT, and cancer cell stemness mechanisms. A systematic search was performed in PubMed, Web of Science, Google Scholar, Cochrane Library, and Embase from 2017 to May 2022 for articles matching the following criteria: (long non-coding RNA (lncRNA) and (head and neck squamous cell carcinoma (HNSCC), or oral cancer, or oral squamous cell carcinoma (OSCC), or buccal cancer, or lip cancer or tongue cancer (TSCC) or pharyngeal carcinoma, or nasopharyngeal carcinoma (NPC) or laryngeal squamous cell carcinoma (LSCC)), and (chemoresistance or drug resistance or cisplatin resistance or CDDP resistance), and (autophagy or epithelial-mesenchymal transition or EMT or stemness or cancer stem cells (CSCs)). The titles and abstracts were screened and acquired relevant full-text manuscripts were for further analysis.
Long non-coding RNAS
Biogenesis, classification, and function
Approximately 93% of the human genome can be transcribed into RNAs, but only 2% of these transcripts are translated into proteins; the remaining 98% are ncRNAs (24). LncRNAs, also referred to as competing endogenous RNAs (ceRNAs) (34), include different kinds of RNA polymerase II (Pol II)-transcribed molecules, mostly 5’-capped, polyadenylated, and spliced (35), and studies suggest there could be more than 10,000 lncRNA transcripts in humans (36, 37). LncRNAs are engaged in multiple functions, including the modulation of crucial functions of other ncRNAs such as micro-RNAs (miRNAs), small nucleolar RNAs (snoRNAs), etc. (38).
Advances in RNA sequencing and other techniques have allowed the discovery of an increasing number of lncRNA classes based on diverse parameters such as transcript length, mRNA resemblance, biogenesis, and unique regulatory mechanisms, among others (39, 40). According to Schmitz et al., one of the most used categorizations is related to the position of the lncRNAs in the genome relative to protein-coding genes (Figure 1) (35). The lncRNA can be divergent (pancRNA) when the lncRNA and neighboring protein-coding gene are transcribed in opposite strands (41), convergent when the lncRNA and protein-coding gene neighbor are transcribed to the same point (42), intergenic when a lncRNA sequence belongs to two genes as a distinct unit (43), overlapping when a protein-coding gene is included in the intron of the same lncRNA in sense or antisense orientation (44), enhancer RNAs expressed as uni- or bidirectional transcripts (45), intronic when the sequence of the lncRNA belongs to the intron of a protein-coding gene (46). Some lncRNAs are generated by back splicing from introns of mRNAs or other lncRNAs and are thus circular (circRNAs) (47).
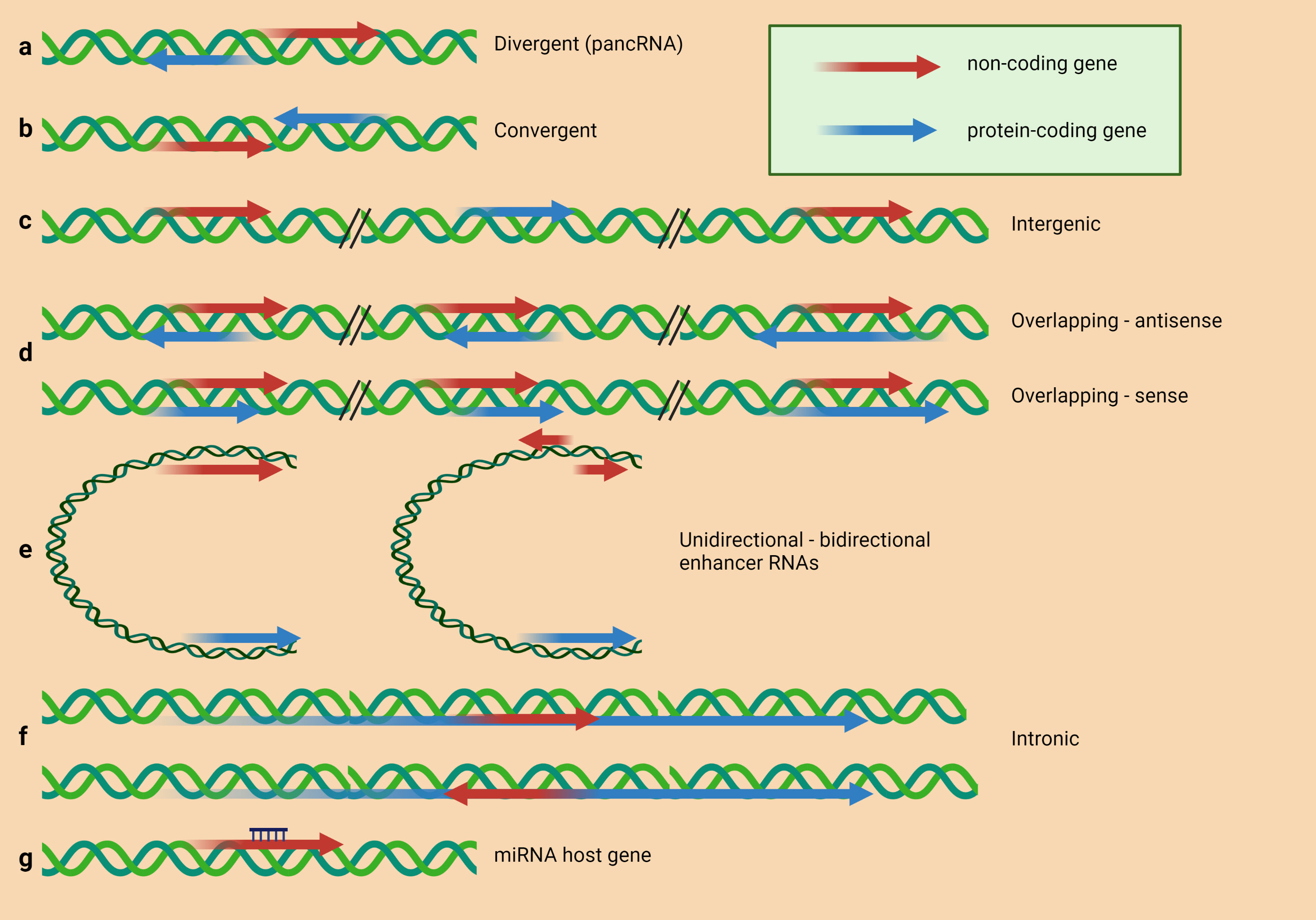
Figure 1 LncRNAs classification based on their structural origin. According to Schmitz et al. (35), lncRNAs can be classified in (A) divergently transcribed lncRNA originating from the same promoter region as the adjacent protein-coding gene, but from the opposite strand; (B) genes encoded on opposite strands, facing each other and convergently transcribed; (C) intergenic lncRNA (lincRNA) located distant from other genes; (D) lncRNAs overlapping with other genes on the same or opposite strand; (E) enhancer RNAs expressed as uni- or bidirectional transcripts; (F) lncRNA transcribed from an intron of another gene; (G) lncRNA hosting miRNA.
An increasing number of lncRNAs have been associated with both important biological functions and pathological conditions such as diabetes, neurodegenerative diseases, rheumatoid arthritis, cardiovascular diseases, and cancer (48–50). Dahariya et al. suggest that activation and inhibition of gene expression are promoted by lncRNAs through diverse molecular mechanisms comprising of four basic mechanisms: signal, decoy, guide, and scaffold. In this regard, recent evidence suggests that signaling mediators like kinases, receptors, and transcription factors are strongly associated with lncRNAs via numerous signaling pathways, such as PI3K/AKT/mechanistic target of rapamycin (mTOR), Wnt, and the MAPK signaling pathways (51–53). On the other hand, decoy lncRNAs can diminish the availability of regulatory factors by presenting binding sites (54). For instance, Zhang et al. (55) demonstrated that LINC00160 functions as a decoy of miRNA-132 targeting PIK3R3 to mediate DR in hepatocellular carcinoma, whereas lncRNA GAS5 can also act as a molecular sponge that blocks their downstream functions by targeting RNA or proteins (56). Besides, lncRNAs can interact with ribonucleoproteins (RNPs) in the genome to guide their precise localization, such as HOTAIR, which directs PRC2 to the HOXD locus, leading to silencing genes involved in metastasis suppression (54, 57). Also, CASC9 acts as a guide for EZH2 and CREB-binding protein (CBP) to the promoter regions of target genes (58). In the case of scaffold lncRNAs, they can act as the central platform for assembling complexes, for example, by binding to RNP K and EZH2 to induce the formation of a complex to repress SOX2 (51, 54, 59). Overall, the diverse functions of lncRNAs depend on their subcellular location (60). Genome sequencing has shown that a large proportion of lncRNAs are localized in the nucleus or associated with chromatin, whereas the remaining fraction is localized in the cytoplasm (61).
Many studies have demonstrated the crosstalk of lncRNAs with many epigenetic factors to regulate gene expression and modulate nuclear structure by facilitating the architecture of nuclear speckles, paraspeckles, and interchromatin granules (36, 62). Some lncRNAs play a role as regulators to initiate, elongate or terminate actions of transcription factors (38). Other types of lncRNAs act as decoys by binding to transcription factors or proteins and deviating from protein factors in their action on target DNA (37). They also act as sponges or molecular sinks for miRNAs, mediating changes in gene expression by acting on transcription factors, cell receptors, growth factors, and splicing regulators (63). The main functions of lncRNAs are depicted in Figure 2.
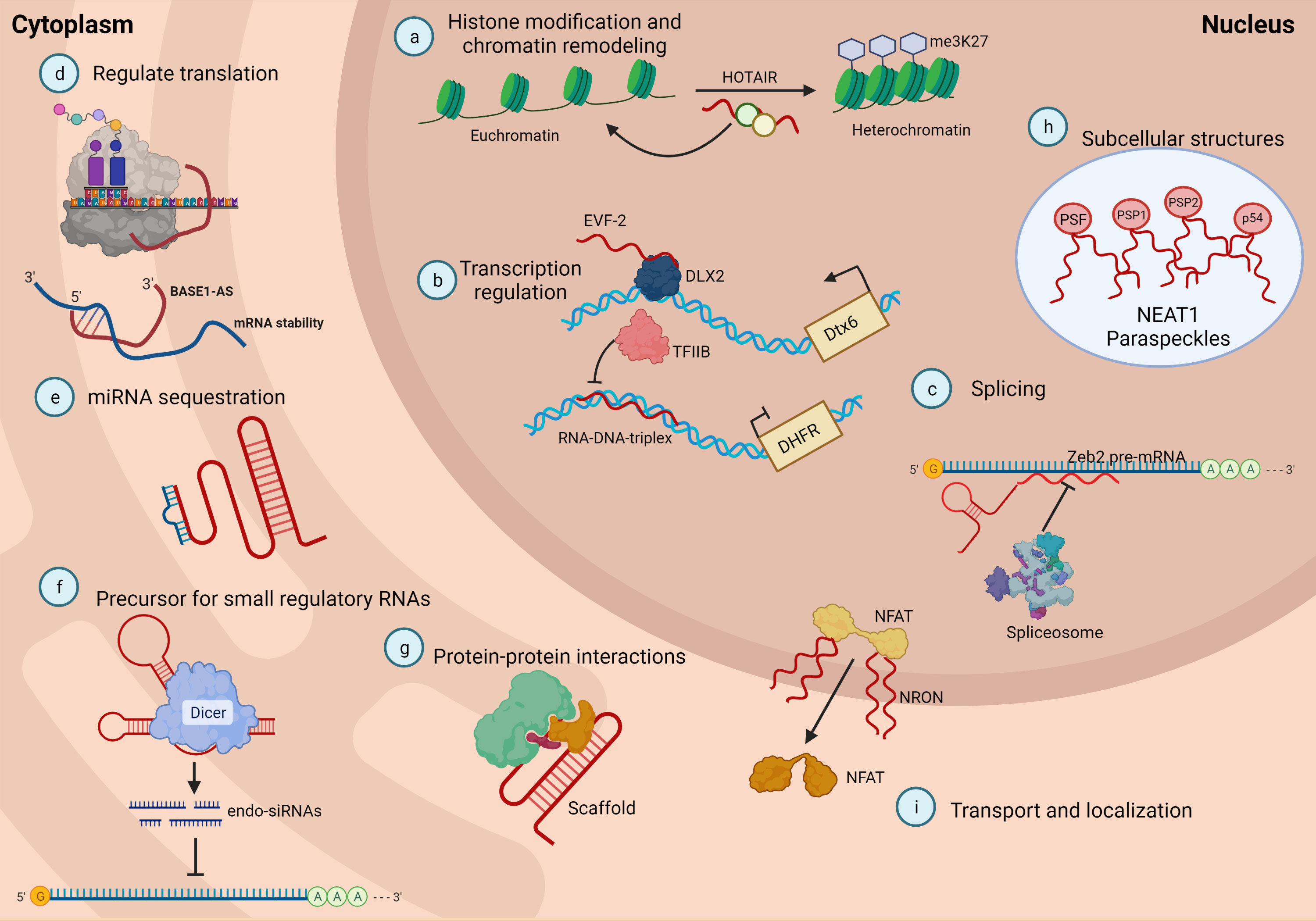
Figure 2 LncRNAs can be classified based on their functions. (A) lncRNA can guide chromatin complexes controlling between transcriptionally active euchromatin and silent heterochromatin; (B) the recruitment of polymerase II and transcription factors can be inhibited or facilitated by lncRNAs; (C) lncRNAs contribute to transcriptome complexity by regulating alternative splicing of pre-mRNAs; (D) lncRNAs affect the stability and translation of mRNA by base pairing with mRNA molecules; (E) they influence in the expression of miRNAs by binding to them and preventing their function; (F) lncRNAs can act as siRNAs and target other RNAs, which subsequently could result in target degradation; (G) lncRNAs can join multiple protein factors as flexible scaffolds to interact or cooperate on protein-protein interactions; (H), (I) the scaffold function is also important for protein activity and localization as well as subcellular structures. Adapted from Meng et al. (64).
Role of lncRNAs in cancer
Recently, an increasing number of studies of high RNA-sequencing have provided resources for the identification of many lncRNAs that are dysregulated in solid tumors (65–67). LncRNAs are often found as regulators in tumorigenesis, progression, and metastasis of cancer by modulating signaling cascades at the epigenetic, transcriptional, posttranscriptional, translational, or posttranslational levels (65). Cancer-controlling lncRNAs are categorized as proto-oncogenic or tumor suppressors based on their function, being the tumorigenic lncRNAs expressed in tumors as cancer drivers that activate the cell cycle, promote proliferation, and/or exert anti-apoptosis effects (65, 68). Moreover, cancer-progressing lncRNAs have been related to EMT, cell migration, and cell invasion (65, 68). Approximately 100 lncRNAs have been identified recently as regulators of the development and progression of multiple cancer types, including prostate (69–72), breast (73–75), lung (76–79), colorectal (80–82), liver (83–85), and leukemia (86–88), among others.
On the other hand, many lncRNAs have been documented as tumor suppressors and they are generally downregulated in tumor biopsies compared with their normal counterparts (65). When these tumor-suppressing lncRNAs are downregulated or suppressed, they can lead to increased proliferation and tumor growth (65). Although many tumor-suppressing lncRNAs are under investigation, the most documented are the growth arrest-specific transcript 5 (GAS5) (89), the maternally expressed gene 3 (MEG3) (90), and the NF-kB interacting lncRNA (NKILA) (91).
LncRNAs in HNSCC
HNSCC comprises a group of cancers that originate in the squamous-cell layer of the mucosa lining in the head and neck region. The heterogeneity, anatomical, and functional features make the HNSCC a complex and difficult-to-treat disease, leading patients to a poor survival rate and a decreased life quality due to the loss of important physiologic functions and aggressive surgical mutilation (2, 24, 25). Alteration of driver-oncogenic and tumor-suppressing lncRNAs has been recently studied in HNSCC to obtain possible biomarkers for diagnostic, prognostic, and therapeutic approaches (2, 24, 25). Table 1 summarizes oncogenic and tumor suppressor lncRNAs commonly found in HNSCC.
Several lncRNAs have been assessed for their involvement at different stages in HNSCC. The metastasis-associated lung adenocarcinoma transcript 1 (MALAT1) is localized in the nuclear speckle periphery and it is widely studied in cancer development and progression (136). This lncRNA has been strongly associated with squamous cell carcinomas (SCC), including oral SCC (100) and laryngeal SCC (132), demonstrating poorer 5-year survival when highly expressed in tumor tissues.
A novel identified homeobox A11 antisense lncRNA (HOXA11-AS) has also been categorized as a facilitator in the process of malignant tumor proliferation and metastasis (137). Wang et al. demonstrated the proliferation of OSCC cells when HOXA11-AS was upregulated, whereas its downregulation increased apoptosis and caspase 3 activity in CDDP-resistant OSCC cells (98). Moreover, HOXA11-AS knockdown inhibited viability, migration, and invasion in LSCC and enhanced cisplatin sensitivity, thus promoting cell apoptosis in NPC tumor tissues (116, 117, 134).
In a tongue SCC study, the upregulation of the lncRNA KCNQ1 opposite strand/antisense transcript 1 (KCNQ1OT1) demonstrated a strong correlation with the survival rate, proliferation, migration, invasion, and EMT of tongue cancer cells (28). Another study by Zhang et al. found that KCNQ1OT1 facilitated tumor growth and chemoresistance by acting as a modulating ceRNA of miR-211-5p (103). Also, in NPC cell lines, the knockdown of KCNQ1OT1 promoted chemosensitivity and decreased cell proliferation, migration, and invasion by interfering with the miR-454/USP47 axis (118).
Some lncRNAs act as tumor suppressors when upregulated. In this regard, MEG3 downregulation is associated with poor survival of most cancer patients (138) since its upregulation enables the expression of tumor suppressor genes p53 and Rb, induces inhibition of angiogenesis-related factors, and can sponge miRNAs (138, 139). In HNSCC, Lin et al. demonstrated that MEG3 expression was downregulated in NPC cells, inhibiting autophagy and apoptosis ability by acting as a ceRNA to miR-21 (113). Another previously identified tumor-suppressing lncRNA related to HNSCC is the nuclear paraspeckle assembly transcript 1 (NEAT1), strongly associated with suppressing cisplatin resistance by modulating several signaling pathways like the Ras-MAPK and the miR-129/Bcl-2 axis in NPC cells (122, 126). Other lncRNAs identified as tumor suppressors in HNSCC are LINC00460 (33), GAS5 (130), MRVI1-AS1 (128), and MPRL (107). Further identification of these transcripts remains to be elucidated.
Several efforts have been made to identify valuable prognostic lncRNA signatures in different head and neck cancers. Jian et al. associated eight different lncRNAs with OSCC/OPSCC (oropharyngeal squamous cell carcinoma) prognosis, indicating a significantly lower overall survival in the high-risk group (13). Moreover, 493 HNSCC patients were screened for 363 prognostic-related lncRNAs, finding 17 lncRNAs related to the progression and prognosis of HNSCC. These differentially expressed genes (DEGs) between high- and low-risk groups are mainly enriched in immune-related pathways and regulated by a prognostic-lncRNA-directed ceRNA network (12). In a study by Li et al., 501 HNSCC cases were obtained from the National Cancer Institute GDC Data Portal and analyzed by gene set enrichment analysis (GSEA) and gene ontology (GO) functional annotation, proving that the autophagy-related lncRNA signature (LINC00958, PSMA3−AS1 UBAC2−AS1, AC008115.3, AL139 9158.2, AC136475.2, AL160006.1, AL3 57033.4, AC007991.2, AC104083.1, A L139287.1, and AL450992.2) could be considered to predict the prognosis of patients with HNSCC (140).
LncRNAs in drug resistance mechanisms
Three of the most important mechanisms of DR in HNSCC, autophagy, EMT, and stemness, are regulated by multiple lncRNAs (Figure 3). Chemotherapy remains a very common treatment option for cancer patients, although it has been established that DR is responsible for around 90% of deaths in cancer patients receiving chemotherapeutics or targeted drugs (141). After the drug is administered, the therapeutic agents pass through a phase of active intracellular metabolism along with the degradation by the liver and other metabolic organs and tissues (142). Furthermore, the dysregulation of enzymes and other proteins responsible for cellular metabolism offers additional challenges that reduce the effectiveness of anti-tumor drugs (143).
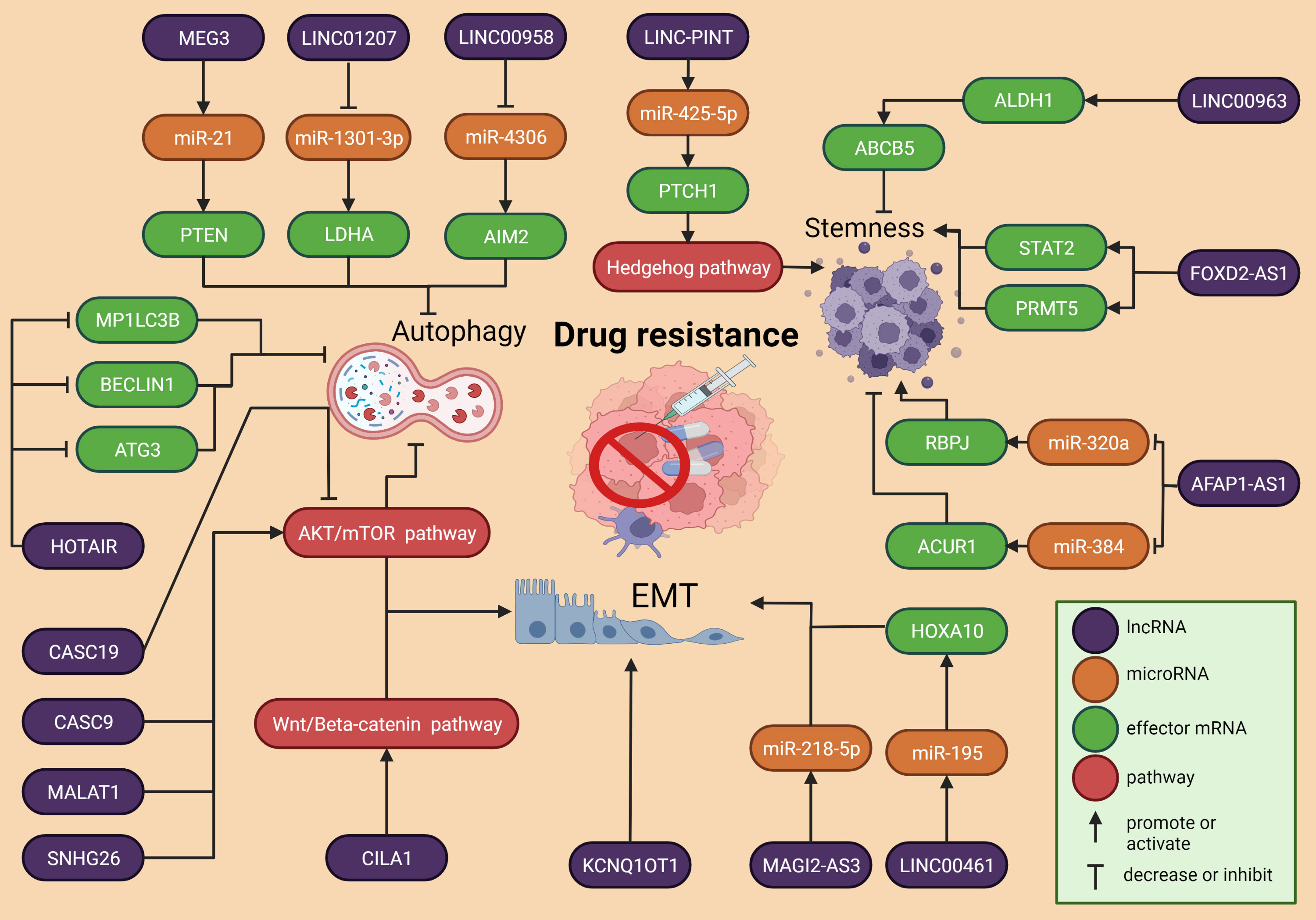
Figure 3 Overview of the molecular mechanisms of lncRNA in HSCC drug resistance. Three of the most important cellular processes involved with drug resistance are autophagy, EMT and stemness. All of them are ruled by regulatory axes that comprise the interaction between lncRNAs, microRNAs and expression of genes.
Three main phases of drug metabolism and disposition have been observed: phases I and II concerning drug metabolism and phase III concerning drug disposition (144). In phase I, enzymes are mostly cytochrome P450 (CYPs) and are involved in the oxidation, reduction, and/or hydrolysis processes that activate or inactivate the agent (145). During phase II, metabolic reactions are carried out by transferases whose primary mission is to deactivate pharmacologically active drugs, facilitating their elimination by making them more soluble in water (146). Finally, phase III consists of drug transporters active in the absorption, distribution, and elimination of drugs (147). Increased expression, transcription activation of involved genes, and activity of efflux drug transporters represent a major mechanism for developing chemoresistance, mostly under the control of epigenetic processes like DNA methylation, histone acetylation, and ncRNA interaction (Figure 4) (142, 144, 148, 149). The involvement of lncRNAs in drug metabolism and efflux phases has been investigated to elucidate the DR mechanisms of cancer (141).
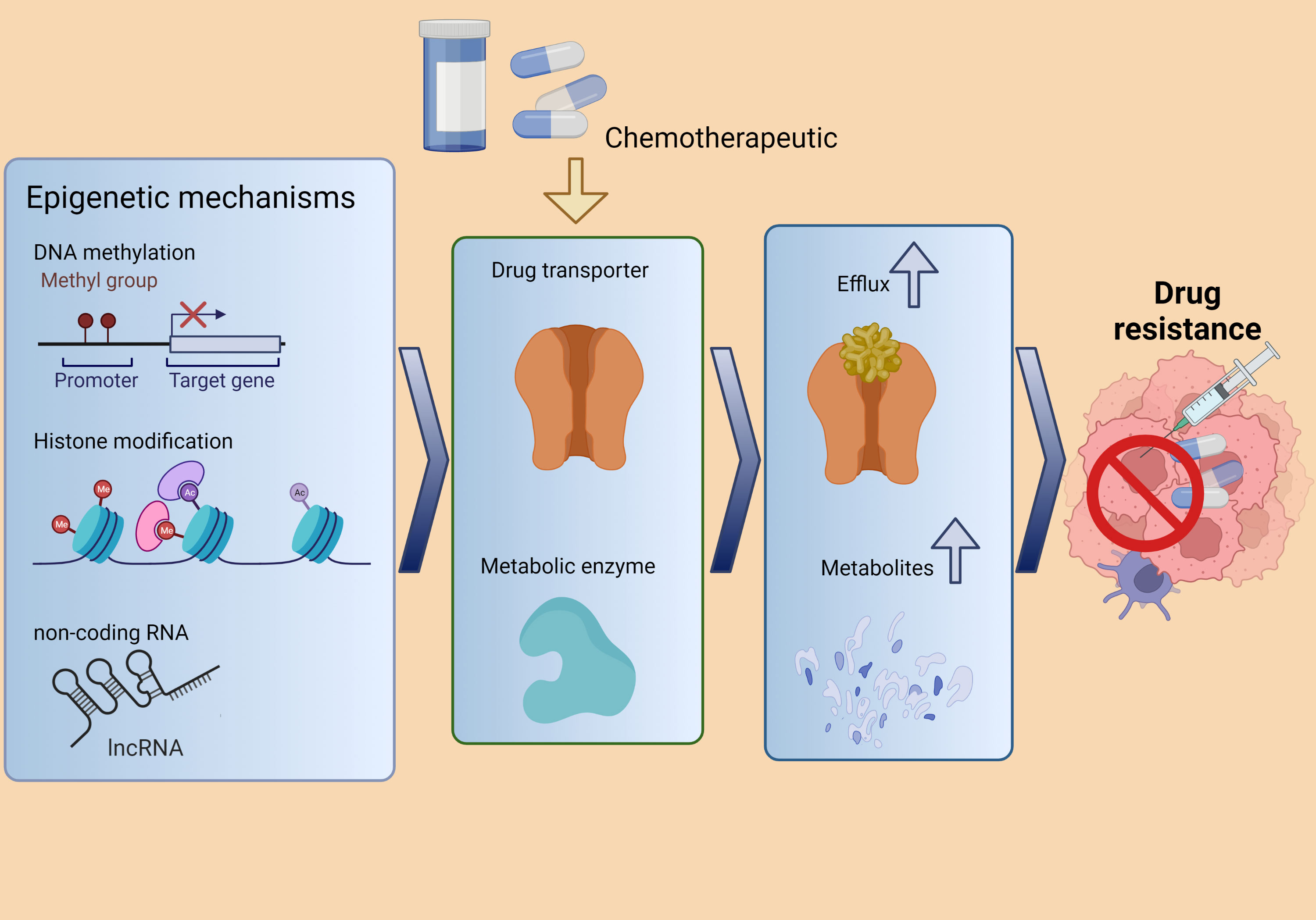
Figure 4 The epigenetic processes involved in cancer chemoresistance includes DNA methylation, histone acetylation, and lncRNA interaction. These processes regulate drug transporters and metabolic enzymes, promoting drug resistance. Adapted from Zhou et al. (148).
Some studies have demonstrated the involvement of lncRNAs in the metabolism and disposition of anti-cancer drugs, influencing directly the development of DR (142, 148). In HNSCC, DR has become an increasingly concerning challenge for the scientific community and clinicians (16, 31, 135). Along with many others, the long intergenic non-coding RNA 00958 (LINC00958) has been studied as apoptosis- and autophagy-related lncRNA in HNSCC as part of prognostic signature, establishing a worse overall survival rate of patients when the signature is present (140, 150). Moreover, Jian et al. concluded that LINC00958 downregulates miR-4306 levels to activate the pyroptosis pathway mediated by AIM2 and promotes cancer cell survival in OSCC (96). Another study suggests that LINC00958 interplay with c-Myc as a feedback loop facilitating HNSCC development and resistance to chemo- and radiotherapy, and its upregulation is associated with poor tumor differentiation, advanced tumor stage, and shorter overall survival of patients (31) Another lncRNA related to DR is the plasmacytoma variant translocation 1 (PVT1), identified as upregulated in cisplatin-resistant cancer cell lines and tissue samples (108) and as a promoter of decreased sensitivity to cetuximab (135). Table 2 summarizes the most important dysregulated lncRNAs that influence DR on HNSCC.
The homeobox A11 antisense lncRNA (HOXA11-AS) has also been related to chemoresistance. In this regard, OSCC tumor tissues and cell lines were analyzed, concluding that the upregulation of HOXA11-AS promoted proliferation in CDDP-sensitive cells and inhibited CDDP-induced cytotoxicity by intervention in the miR214-3p/PIM1 axis (98). Other studies demonstrated that the knockdown of HOXA11-AS enhances CDDP resistance via the miR-98/PBX3 axis (116) and can inhibit the c-Met/AKT/mTOR pathway by specifically upregulating miR-454-3p, thus promoting cell apoptosis and enhancing the sensitivity of cisplatin-resistant NPC cells to cisplatin (117). Conversely, Shen et al. analyzed LSCC tissues and cell lines, showing that HOXA11-AS1 knockdown inhibits the viability, migration, and invasion but promotes the apoptosis of cells (134).
Another lncRNA actively involved in DR in HNSCC is the KCNQ1 overlapping transcript 1 (KCNQ1OT1). It has been established that its upregulation facilitates tumor growth and chemoresistance in tongue SCC by sponging miR-124-3p (28) and by acting as a ceRNA to modulate the expression of miR-211-5p (103). Moreover, Yuan et al. showed that KCNQ1OT1 knockdown promotes chemosensitivity in DDP-resistant NPC cells by significantly decreasing cell proliferation, migration, and invasion via the miR-454/USP47 axis (118). NEAT1 (nuclear paraspeckle assembly transcript 1) has also been associated with HNSCC chemoresistance, especially in NPC where its depletion repressed the cisplatin resistance of NPC cells and phenocopied the effect of miR-129 overexpression, which also enhanced apoptosis by the histone deacetylase inhibitor SAHA (122, 126).
The metastasis-associated lung adenocarcinoma transcript 1 (MALAT1) has been identified as a prognostic factor in patients with lung cancer (151), and its overexpression is related to poor clinical outcome, chemoresistance, and progression in many cancer types, including kidney (152, 153), pancreatic (154), prostate (155, 156), esophageal (157, 158), breast (159, 160), gastric (161), ovarian (162), and colorectal (163, 164). In both oral and laryngeal SCC, the over-expression of MALAT1 contributes to the enhanced chemoresistance and metastatic power of several cell lines (100, 132).
In the case of H19, it has been demonstrated that it is upregulated in LSCC, exerting an inhibiting effect at the autophagy level and DR by downregulating HMGB1 by targeting miR-107 (131). A similar effect has been observed in many other cancer types, with anti-apoptotic, pro-proliferative, and pro-migratory functions, along with the induction of EMT, activation of oncogenic signaling pathways, and changes in the tumor microenvironment, contributing to anti-cancer DR (165). Another well-studied lncRNA that promotes the proliferation, migration, and chemoresistance of several cancer types is the myocardial infarction-associated transcript (MIAT) (166–169). According to Zhu et al., an elevated MIAT level upregulates HMB1 expression, contributing to cisplatin resistance and poor clinical outcomes (121).
An increasing number of other lncRNAs have been proposed as promoters of DR in HNSCC. For instance, the urothelial cancer-associated lncRNA 1 (UCA1) plays an important role in the tumorigenesis, progression, and diagnosis of many cancers, mainly bladder cancer (170, 171). In oral cancer tissues and cell lines, UCA1 accelerates proliferation, increases CDDP chemoresistance and restrains apoptosis partly by modulating SF1 by sponging miR-184 (97). In another study, the results showed that CAF-secreted midkine enhanced OSCC resistance to cisplatin by inducing ANRIL expression and increasing the anti-apoptotic protein Bcl-2 expression (101). Chen et al. studied 146 paraffin-embedded OSCC specimens along with OSCC cell lines CAL-27 and SCC4 and found that the cytoskeleton regulator RNA (CYTOR) acts as a ceRNA to inhibit miR-1252-5p and miR-3148, upregulating the lipoma-preferred partner (LPP) protein and therefore proving essential for FOXD1-induced EMT and chemoresistance (15). Another forkhead box (FOX) reported as important for laryngeal SCC DR is FOXD2-AS1, which acts as a scaffold for STAT3 and PRMT5, promoting STAT3 transcriptional activity, maintaining cancer stemness, and promoting chemotherapeutic resistance (18).
LncRNAs in autophagy
The intracellular degradation systems encompass two major protein pathways that are directly involved in the maintenance of metabolic homeostasis; one of them is the ubiquitin–proteasome pathway, responsible for degrading short-lived and damaged proteins; and the other is the lysosome-autophagy system, whose target is long-lived macromolecular complexes and organelles (172). Autophagy is a highly conserved and successive cellular process in which damaged organelles, intracellular microbes, and pathogenic long-lived proteins are degraded, recycling amino acids, nucleotides, and fatty acids to maintain cellular homeostasis (173–175). Thus, autophagy is closely related to the occurrence of a wide variety of human diseases (176).
Autophagy-related genes (ATGs) are responsible for autophagy occurring under micro-environmental stress such as hypoxia, heat, nutrient deficiency, and accumulation of reactive oxygen species (175, 176). The main successive autophagy stages are the initiation of phagophore assembly, autophagosomal formation, and lysosomal fusion (177, 178). Two highly conserved serine–threonine kinases, the mammalian target of rapamycin (mTOR) and the mammalian homologs of yeast ATG1-Unc-51-like kinases 1 (ULK1), regulate cell growth and survival and are the central modulators of autophagy, responding to intra- and extra-cellular changes by forming autophagosomes (179, 180). mTOR is activated under favorable conditions, inhibiting autophagy and protein degradation, whereas it is inactivated in hostile environments related to poor nutritional conditions (172).
Recent studies have demonstrated an important role for autophagy in tumorigenesis and the progression of cancer (181). A dual function has been proposed since autophagy can stabilize the genome and prevent the formation of tumor cells, while once the tumor cells have been formed, autophagy plays a pivotal role in tumor initiation, progression, and resistance to chemotherapy (172, 177). LncRNAs are involved in autophagy, modulating the expression of ATG genes by acting as ceRNAs for miRNAs (36, 180). Recently, several autophagy-related lncRNAs have been proposed as biomarkers and signatures for diagnostic and prognostic purposes for many cancer types, including breast (182, 183), bladder (184, 185), pancreatic (186), colorectal (187, 188), and lung (189, 190). In most cases, the overall survival of patients in high-risk groups is significantly lower based on the presence of each proposed signature.
Many studies have established prognostic signatures in HNSCC. For instance, in a recent study, 910 autophagy-related (AR) lncRNAs from mRNA sequences and clinical data of HNSCC patients and controls from The Cancer Genome Atlas (TCGA) were analyzed. The principal component analysis distinguished two categories based on the nine lncRNA prognostic signatures, resulting in a significantly worse overall survival rate in the high-risk group (150). Another study consulted the TCGA database to obtain 155 HNSCC samples (mainly laryngeal, nasopharyngeal, tonsil, and lip cancer) and the RNA profile indicated that ATG12, BECN1, and MAP1LC3B have prognostic value, and their related pathways may be involved in regulating HNSCC prognosis (191). Guo et al. included 17 prognostic-related autophagy- and ferroptosis-related lncRNAs as the main components of a ceRNA network that regulates differentially expressed genes mainly enriched in immune-related pathways (12). Similarly, gene set enrichment analysis (GSEA) and gene ontology (GO) functional annotation proved that autophagy-related pathways are mainly enriched when 13 autophagy-related lncRNAs are present in HNSCC patients (140). Regarding oral and oropharyngeal SCC, the signature-based on nine autophagy-related lncRNAs acted as an independent prognostic indicator, showing a significantly lower overall survival in high-risk groups (13). The autophagy-related (AR) signatures of lncRNAs proposed as biomarkers in HNSCC are summarized in Table 3.
Quantitative reverse transcription PCR (RT-qPCR) was performed to analyze the cancer susceptibility candidate 9 (CASC9) expression in OSCC tissues and cell lines, demonstrating that CASC9 promotes progression by enhancing cell proliferation and suppressing autophagy-mediated cell apoptosis via the AKT/mTOR pathway (92). Another study by Chen et al. showed that when the gastric cancer-associated transcript 1 (GACAT1) was inhibited in OSCC samples, it promoted apoptosis and autophagy, mainly related to the targeting of miRNA-149 (93). Long intergenic non-coding RNA 01207 (LINC01207) and LINC00958 were also upregulated in OSCC tissues and cells. LINC01207 upregulates LDHA expression to promote cell proliferation and migration and inhibits apoptosis and autophagy by acting as a ceRNA that sponges miR-1301-3p (94), whereas LINC00958 downregulates miR-4306 levels to activate a pyroptosis pathway mediated by AIM2 and promotes cancer cell survival (96).
A broadly studied oncogenic trans-acting lncRNA is the HOX transcript antisense RNA (HOTAIR), which is found overexpressed in a wide variety of cancers and is mainly associated with metastasis and poor prognosis (192). In OSCC cells, HOTAIR silencing inhibited autophagy with the downregulated expression of MAP1LC3B, Beclin1, ATG3, and ATG7; proliferation, migration, and invasion of OSCC cells were suppressed, along with an enhanced apoptosis rate and an improvement in sensitivity to cisplatin (95).
As previously addressed, MEG3 is considered a tumor-suppressor lncRNA. Lin et al. concluded that MEG3 promotes autophagy and apoptosis of NPC cells by enhancing PTEN expression by binding to miR-21 (113). Another important tumor-suppressor lncRNA involved in autophagy activation is the growth arrest-specific 5 RNA (GAS5). In LSCC, GAS5 inhibited the viability of AMC-HN-8 cells and induced apoptosis, acting as a tumor suppressor by regulating the miR-26a-5o/ULK2 axis (130). Conversely, CASC19 suppressed cellular autophagy by inhibiting the AMPK/mTOR pathway, contributing to the radioresistance of NPC by regulating autophagy (114).
LncRNAs in EMT
Epithelial-to-mesenchymal transition (EMT) is described as a process where epithelial cells are transformed into mesenchymal stem cells and plays an important role in both development and tumorigenesis (177, 193). Moreover, EMT has been broadly related to tumor proliferation, metastasis, and DR (194, 195). However, this transition is reversible since tumor cells will go through the opposite process of mesenchymal-to-epithelial transition (MET) once they have reached a suitable place where they can metastasize, re-expressing epithelial characteristics (196). A wide variety of signaling pathways can be involved in EMT, including the transforming growth factor-beta (TGF-beta) pathway (196, 197), the Wnt/beta-catenin pathway (198, 199), the Notch signaling pathway (200), the Hedgehog pathway (201), and the signal transducer and activator of transcription 3 (STAT3) pathway (202), among others. These signaling molecules can subsequently activate different EMT transcription factors like Snail, basic helix-loop-helix (TWIST), and zing-finger E-box-binding homeobox (ZEB) to repress epithelial markers and activate the EMT program (6, 177, 196).
Recently, several lncRNAs have been linked to EMT since they play fundamental roles in the above-mentioned signaling cascades, epigenetics, and transcription factors (203–205). The lncRNA MALAT1 induces EMT and CDDP resistance in OSCC cells via the activation of the PI3K/AKT/mTOR signaling pathway in cell lines CAL-27 and SCC-9 (100). LncRNA KCNQ1OT1 has also been related to EMT in tongue cancer tissues and cells, promoting survival rate proliferation, migration, and invasion (28). Similarly, quantitative PCR performed in pituitary adenoma samples found the same lncRNA to be upregulated, and its knockdown inhibited cell stemness, angiogenesis, and EMT (206).
A novel lncRNA named chemotherapy-induced lncRNA 1 (CILA1) was upregulated in cisplatin-resistant tongue SCC cells, displaying EMT features, promoting invasiveness and chemo-resistance, mainly activating the Wnt/beta-catenin pathway (110). Another lncRNA related to EMT is the recently discovered small nucleolar RNA host gene 26 (SNHG26), first described by Tong et al. as part of a prognosis signature along with the other 13 lncRNAs in bladder cancer (207). Similarly, a four-lncRNA signature that included SNHG26 was associated with immune infiltration and prognosis in colon cancer; the signature-divided colon cancer patients of TCGA into high- and low-risk groups with significantly different outcomes (208). In TSCC, SNHG26 expression was positively correlated with proliferation, EMT, migration, invasion, and cisplatin resistance by activating the AKT/mTOR signaling pathway (104).
Another lncRNA recently linked to EMT in cancer is the long intergenic non-protein coding RNA 461 (LINC00461), highly expressed in non-small cell lung cancer and directly involved in cell proliferation, migration, and EMT by targeting the miR-4478/E2F1 axis (209). Similarly, Wu et al. showed that the upregulation of LINC00461, LINC00402, and SFTA1P had suppressive effects on the homologous pleckstrin-homology (PH)-domain leucine-rich-repeat protein phosphatases (PHLPP2), reported previously as a tumor suppressor in colon cancer (210). In HNSCC, LINC00461 was highly expressed in 52 tissues analyzed, and it was found that LINC00461 downregulates the expression of miR-195 to subsequently upregulates the expression of HOXA10, promoting EMT and enhancing chemoresistance in HSNCC (16).
The membrane-associated guanylate kinase 2 antisense RNA 3 (MAGI2-AS3) was recently identified in NPC, driving cell proliferation, migration, and EMT through the miR-218-5p/GDPD5/SEC61A1 axis, conferring cisplatin resistance in cell lines (124). The same lncRNA expression was detected by quantitative real-time PCR in pancreatic cancer cells, and its upregulation promoted EMT through the regulation of miR-490-5p (211). Moreover, MAGI2-AS1 was identified to be EMT-related and highly co-expressed with ZEB1/2 in both gastric tissues and normal stomach tissue (212). Conversely, MAGI2.AS3 overexpression inhibited bladder cancer progression by regulating MAGI2/PTEN/EMT in 80 bladder cancer tissues (213).
Although several studies have encompassed the involvement of lncRNAs as regulators of EMT and, consequently, DR in HNSCC, more studies must complement the information available at present time.
LncRNAs in stemness
The stemness of cancer cells is an important cellular feature that grants tumor heterogeneity, enhanced growth capacity, DR, and augmented metastatic ability through the CSC properties of self-renewal, quiescent state, high cell turnover, increased expression of drug transporters, and other resistance genes (177, 214, 215). Evidence suggests that CSCs retain properties that make them highly resistant to currently available chemotherapy drugs since CSCs remain in an inactive state of the cell cycle and most of these treatments attack cells with a high proliferative rate (216, 217). Additional properties like rapid DNA repair (218), tumor microenvironment (219, 220), and extracellular matrix contribute to maintaining cancer stemness and chemoresistance (221, 222).
As previously addressed, even though lncRNAs have no protein-coding capacity, they are emerging as master regulators of gene transcription and act as proto-oncogenes or tumor suppressors (223, 224). LncRNAs like LINC00617 (225), lncSOX2OT (226), and HOTAIR (227, 228) play an important role in CSC regulation of various types of cancer through several mechanisms and signaling pathways. Furthermore, in colorectal cancer, the lncTCF7 interacts with subunits of the SWI/SNF chromatin remodeling complex, regulating the transcription of the TCF7 gene and activating the Wnt cascade, involved in stem cell self-renewal (229). H19 is another lncRNA overexpressed in many cancers and confer stem-like properties in correlation with stem cell markers like SOX2, OCT4, NOTCH1, c-Myc, and ABCG2 (230–232).
Little research has been done concerning the role of lncRNAs in DR associated with cancer cell stemness. Lee et al. studied OSCC tumor tissues and cell lines and concluded that the downregulation of the long intergenic non-coding RNA 963 (LINC00963) inhibited CSC hallmarks, such as migration, invasion, and colony formation capacity. Moreover, the suppression of LINC00963 reduced the activity of ALDH1, the percentage of self-renewal, chemoresistance, and the expression of multidrug-resistance transporter ABCB5 (17). Another long intergenic non-coding RNA recently involved in chemoresistance driven by cancer cell stemness is the p53-induced non-coding transcript (LINC-PINT). Interestingly, the tumor suppressor PTCSC3 was studied along with LINC-PINT in gastric cancer tissues, inhibiting tumor growth and stemness when both were over-expressed (233). The same lncRNA was observed in 24 LSCC samples and cells, targeting miR-425-5p and subsequently targeting PTCH1, affecting the Hedgehog pathway and its downregulation was associated with increased cancer stemness and chemoresistance to cisplatin (19).
LncRNA FOXD2-AS1 has been related to different forms of malignancy and CSCs, mainly involving gliomas (234). In laryngeal SCC chemotherapy-resistant patients, FOXD2-AS1 showed increased expression and acted as a scaffold for STAT2 and PRMT5, both essential to maintain cancer stemness and promote chemotherapy resistance (18). Oncogenic actin filament-associated protein 1-antisense RNA 1 (AFAP1-AS1) is a recently discovered lncRNA related to cancer stemness (235). The first documented association between AFAP1-AS1 high expression, stemness, and DR was found in LSCC specimens, increasing RBPJ expression by negatively regulating miR-320a; subsequently, RBPJ overexpression rescued stemness and chemoresistance inhibited by AFAP1-AS1 silencing (120). Another study suggested that AFAP1-AS1 functions as an endogenous RNA by competitively binding to miR-384 to regulate ACVR1, thus conferring inhibitory effects on pancreatic cell stemness and tumorigenicity (236).
Clinical relevance of lncRNAs in drug resistance mechanisms in HNSCC
The clinical importance of establishing a correlation between the increasing number of newly discovered lncRNAs and the various mechanisms of DR lies in the implications that they have on the prognosis, molecular staging, and treatment possibilities of many tumors. In this regard, CASC9 was strongly associated with tumor size, clinical stage, regional lymph node metastasis, and overall survival time in OSCC patients (92). Similarly, the upregulation of PVT1 was strongly correlated with the worse overall survival of 83 OSCC patients (108). Lin et al. proposed CILA1 as a biomarker in TSCC, correlating its expression levels with cisplatin resistance and advanced disease stage (110). Moreover, SNHG26 expression was also associated with the occurrence, progression, and poor prognosis of TSCC (104).
It is important to remember that DR can be categorized as intrinsic and acquired resistance, the first being defined as the lack of tumor regression following treatment (which is the result of mechanisms that existed before therapy). Meanwhile, acquired resistance denotes the elimination of an observed response after an initial clinical benefit following treatment (237). Because stemness of cancer cells is an intrinsic mechanism of DR, its clinical relevance stands out given that lncRNAs such as LINC00963, LINC-PINT, FOXD2-AS1, and AFAP1-AS1 have been found overexpressed, conferring the stemness state to cells. Nevertheless, to date, there is no evidence of a relationship between these specific lncRNAs and a particular drug.
As prognostic signatures, several studies have linked lncRNAs with the prognosis of HNSCC, particularly autophagy- and ferroptosis-related lncRNAs (12, 13, 140, 150, 191). In all cases, the signatures proposed exhibited prognostic value concerning HNSCC (Table 3), and their related pathways may be involved in regulating HNSCC prognosis.
Future directions for lncRNA research
The role of lncRNAs in many diseases has become a widely investigated field, especially in cancer research. Given the increasing evidence of the involvement of lncRNAs in several drug resistance mechanisms, research should be directed toward new horizons to elucidate the molecular pathways by which lncRNAs interact to drive the resistance of certain cell lines (51). This understanding would help in the improvement of the diagnosis and treatment strategies of HNSCC (3, 238). A potential line of research could involve the upstream regulatory mechanisms of lncRNAs since previous evidence suggests regulation by histone status, DNA methylation patterns (107), transcription factors (239), and post-transcriptional regulation (240, 241).
With the development of high-throughput sequencing technology, the library of lncRNAs has notably increased. However, most of the mechanisms of influence on DR through phenomena such as EMT (15), autophagy (131), and stemness (18) have not been fully understood, and some even remain unexplored. A better comprehension of the regulatory networks between lncRNAs, genetic, and epigenetic alterations could give rise to therapeutic strategies that promote improvements in dealing with DR mechanisms (107). Also, the elucidation of all regulatory networks could lead to the formulation of clinical trials targeting specific lncRNAs.
To achieve the clinical application of lncRNAs, molecular techniques such as microarrays, RNA-seq, and qRT–PCR (242) have been used to quantify their expression, but still numerous limitations that need to be overcome. For instance, technical procedures such as ensuring stability, sample preparation, lncRNA extraction, and detection must be standardized. Besides, the sensitivity and specificity of lncRNAs must be ensured. Thus, until all the technical difficulties have been overcome, the detection of circulating lncRNAs would be applied in regular clinical practice (243).
Conclusion
In recent years, the pivotal role of lncRNAs in DR has begun to gain importance in the mechanisms that harbor and promote chemoresistance in HNSCC. As the involvement of more lncRNAs is elucidated, an improvement in diagnostic and prognostic tools could promote an advance in targeted and specific therapies in precision oncology.
Author contributions
JP-F, MB, and RR-P conceived and designed the content of this review. JP-F, MB, CV-M, US-B, DM-C, AA-R, BP-A, AL-P, CL-C, JL-G, JG-P, ME-M, JC-Q, and AB-G wrote the paper. All authors contributed to the final version of the paper and approved the submitted version.
Conflict of interest
The authors declare that the research was conducted in the absence of any commercial or financial relationships that could be construed as a potential conflict of interest.
Publisher’s note
All claims expressed in this article are solely those of the authors and do not necessarily represent those of their affiliated organizations, or those of the publisher, the editors and the reviewers. Any product that may be evaluated in this article, or claim that may be made by its manufacturer, is not guaranteed or endorsed by the publisher.
References
1. Sung H, Ferlay J, Siegel RL, Laversanne M, Soerjomataram I, Jemal A, et al. Global cancer statistics 2020: GLOBOCAN estimates of incidence and mortality worldwide for 36 cancers in 185 countries. CA Cancer J Clin (2021) 71(3):209–49. doi: 10.3322/caac.21660
2. Akbari Dilmaghani N, Khoshsirat S, Shanaki-Bavarsad M, Pourbagheri-Sigaroodi A, Bashash D. The contributory role of long non-coding RNAs (lncRNAs) in head and neck cancers: Possible biomarkers and therapeutic targets? Eur J Pharmacol (2021) 900:174053. doi: 10.1016/j.ejphar.2021.174053
3. Sharma A, Kansara S, Mahajan M, Yadav B, Garg M, Pandey AK. Long non-coding RNAs orchestrate various molecular and cellular processes by modulating epithelial-mesenchymal transition in head and neck squamous cell carcinoma. Biochim Biophys Acta Mol Basis Dis (2021) 1867(11):166240. doi: 10.1016/j.bbadis.2021.166240
4. Leemans CR, Snijders PJF, Brakenhoff RH. The molecular landscape of head and neck cancer. Nat Rev Cancer (2018) 18(5):269–82. doi: 10.1038/nrc.2018.11
5. Kitamura N, Sento S, Yoshizawa Y, Sasabe E, Kudo Y, Yamamoto T. Current trends and future prospects of molecular targeted therapy in head and neck squamous cell carcinoma. Int J Mol Sci (2020) 22(1):240. doi: 10.3390/ijms22010240
6. Meng X, Lou QY, Yang WY, Wang YR, Chen R, Wang L, et al. The role of non-coding RNAs in drug resistance of oral squamous cell carcinoma and therapeutic potential. Cancer Commun (Lond). (2021) 41(10):981–1006. doi: 10.1002/cac2.12194
7. Enokida T, Ogawa T, Homma A, Okami K, Minami S, Nakanome A, et al. A multicenter phase II trial of paclitaxel, carboplatin, and cetuximab followed by chemoradiotherapy in patients with unresectable locally advanced squamous cell carcinoma of the head and neck. Cancer Med (2020) 9(5):1671–82. doi: 10.1002/cam4.2852
8. Weiss J, Gilbert J, Deal AM, Weissler M, Hilliard C, Chera B, et al. Induction chemotherapy with carboplatin, nab-paclitaxel and cetuximab for at least N2b nodal status or surgically unresectable squamous cell carcinoma of the head and neck. Oral Oncol (2018) 84:46–51. doi: 10.1016/j.oraloncology.2018.06.028
9. Oliva M, Spreafico A, Taberna M, Alemany L, Coburn B, Mesia R, et al. Immune biomarkers of response to immune-checkpoint inhibitors in head and neck squamous cell carcinoma. Ann Oncol (2019) 30(1):57–67. doi: 10.1093/annonc/mdy507
10. Qiao XW, Jiang J, Pang X, Huang MC, Tang YJ, Liang XH, et al. The evolving landscape of PD-1/PD-L1 pathway in head and neck cancer. Front Immunol (2020) 11:1721. doi: 10.3389/fimmu.2020.01721
11. Garcia-Mayea Y, Mir C, Masson F, Paciucci R, ME LL. Insights into new mechanisms and models of cancer stem cell multidrug resistance. Semin Cancer Biol (2020) 60:166–80. doi: 10.1016/j.semcancer.2019.07.022
12. Guo Q, Zhang X, Shen T, Wang X. Identification of autophagy- and ferroptosis-related lncrnas functioned through immune-related pathways in head and neck squamous carcinoma. Life (Basel). (2021) 11(8):835. doi: 10.3390/life11080835
13. Jiang Q, Xue D, Shi F, Qiu J. Prognostic significance of an autophagy-related long non-coding RNA signature in patients with oral and oropharyngeal squamous cell carcinoma. Oncol Lett (2021) 21(1):29. doi: 10.3892/ol.2020.12290
14. Yang C, Shen S, Zheng X, Ye K, Ge H, Sun Y, et al. Long non-coding RNA LINC00337 induces autophagy and chemoresistance to cisplatin in esophageal squamous cell carcinoma cells via upregulation of TPX2 by recruiting E2F4. FASEB J (2020) 34(5):6055–69. doi: 10.1096/fj.201900731RR
15. Chen S, Yang M, Wang C, Ouyang Y, Chen X, Bai J, et al. Forkhead box D1 promotes EMT and chemoresistance by upregulating lncRNA CYTOR in oral squamous cell carcinoma. Cancer Lett (2021) 503:43–53. doi: 10.1016/j.canlet.2020.11.046
16. Guan Y, Guan A, Chen L, Gong A. LINC00461 facilitates HNSCC development and reduces chemosensitivity by impairing miR-195-mediated inhibition of HOXA10. Mol Ther Oncolytics. (2021) 21:74–86. doi: 10.1016/j.omto.2021.01.008
17. Lee SP, Hsieh PL, Fang CY, Chu PM, Liao YW, Yu CH, et al. LINC00963 promotes cancer stemness, metastasis, and drug resistance in head and neck carcinomas via abcb5 regulation. Cancers (Basel) (2020) 12(5):1073. doi: 10.3390/cancers12051073
18. Li R, Chen S, Zhan J, Li X, Liu W, Sheng X, et al. Long noncoding RNA FOXD2-AS1 enhances chemotherapeutic resistance of laryngeal squamous cell carcinoma via STAT3 activation. Cell Death Dis (2020) 11(1):41. doi: 10.1038/s41419-020-2232-7
19. Yuan Z, Xiu C, Liu D, Zhou G, Yang H, Pei R, et al. Long noncoding RNA LINC-PINT regulates laryngeal carcinoma cell stemness and chemoresistance through miR-425-5p/PTCH1/SHH axis. J Cell Physiol (2019) 234(12):23111–22. doi: 10.1002/jcp.28874
20. Mansoori B, Mohammadi A, Davudian S, Shirjang S, Baradaran B. The different mechanisms of cancer drug resistance: A brief review. Adv Pharm Bull (2017) 7(3):339–48. doi: 10.15171/apb.2017.041
21. Vasan N, Baselga J, Hyman DM. A view on drug resistance in cancer. Nature. (2019) 575(7782):299–309. doi: 10.1038/s41586-019-1730-1
22. Anastasiadou E, Jacob LS, Slack FJ. Non-coding RNA networks in cancer. Nat Rev Cancer (2018) 18(1):5–18. doi: 10.1038/nrc.2017.99
23. Saw PE, Xu X, Chen J, Song EW. Non-coding RNAs: the new central dogma of cancer biology. Sci China Life Sci (2021) 64(1):22–50. doi: 10.1007/s11427-020-1700-9
24. Guglas K, Bogaczyńska M, Kolenda T, Ryś M, Teresiak A, Bliźniak R, et al. lncRNA in HNSCC: challenges and potential. Contemp Oncol (Pozn). (2017) 21(4):259–66. doi: 10.5114/wo.2017.72382
25. Ghafouri-Fard S, Mohammad-Rahimi H, Jazaeri M, Taheri M. Expression and function of long non-coding RNAs in head and neck squamous cell carcinoma. Exp Mol Pathol (2020) 112:104353. doi: 10.1016/j.yexmp.2019.104353
26. Yan K, Arfat Y, Li D, Zhao F, Chen Z, Yin C, et al. Structure prediction: New insights into decrypting long noncoding RNAs. Int J Mol Sci (2016) 17(1):132. doi: 10.3390/ijms17010132
27. Fu X, Cui G, Liu S, Zhao S. Linc01014 regulates gefitinib resistance in oesophagus cancer via EGFR-PI3K-AKT-mTOR signalling pathway. J Cell Mol Med (2020) 24(2):1670–5. doi: 10.1111/jcmm.14860
28. Qiao CY, Qiao TY, Jin H, Liu LL, Zheng MD, Wang ZL. LncRNA KCNQ1OT1 contributes to the cisplatin resistance of tongue cancer through the KCNQ1OT1/miR-124-3p/TRIM14 axis. Eur Rev Med Pharmacol Sci (2020) 24(1):200–12. doi: 10.26355/eurrev_202001_19912
29. Zhang H, Wang J, Xun W, Wang J, Song W, Wang X. Long non-coding RNA PTCSC3 inhibits human oral cancer cell proliferation by inducing apoptosis and autophagy. Arch Med Sci (2021) 17(2):492–9. doi: 10.5114/aoms.2020.96409
30. Han W, Niu L, Wang L, Liu J, Li H. Downregulation of long non-coding RNA b-raf proto-oncogene-activated non-coding RNA reverses cisplatin resistance in laryngeal squamous cell carcinoma. Arch Med Sci (2021) 17(5):1164–74. doi: 10.5114/aoms.2019.91352
31. Huang S, Zhan Z, Li L, Guo H, Yao Y, Feng M, et al. LINC00958-MYC positive feedback loop modulates resistance of head and neck squamous cell carcinoma cells to chemo- and radiotherapy in vitro. Onco Targets Ther (2019) 12:5989–6000. doi: 10.2147/OTT.S208318
32. Jiang Y, Guo H, Tong T, Xie F, Qin X, Wang X, et al. lncRNA lnc-POP1-1 upregulated by VN1R5 promotes cisplatin resistance in head and neck squamous cell carcinoma through interaction with MCM5. Mol Ther (2022) 30(1):448–67. doi: 10.1016/j.ymthe.2021.06.006
33. Xue K, Li J, Nan S, Zhao X, Xu C. Downregulation of LINC00460 decreases STC2 and promotes autophagy of head and neck squamous cell carcinoma by up-regulating microRNA-206. Life Sci (2019) 231:116459. doi: 10.1016/j.lfs.2019.05.015
34. Wang Y, Gao L, Zhu B, Zhu H, Luo Y, Wang Q, et al. Integrative analysis of long non-coding RNA acting as ceRNAs involved in chilling injury in tomato fruit. Gene. (2018) 667:25–33. doi: 10.1016/j.gene.2018.05.030
35. Schmitz SU, Grote P, Herrmann BG. Mechanisms of long noncoding RNA function in development and disease. Cell Mol Life Sci (2016) 73(13):2491–509. doi: 10.1007/s00018-016-2174-5
36. Bermúdez M, Aguilar-Medina M, Lizárraga-Verdugo E, Avendaño-Félix M, Silva-Benítez E, López-Camarillo C, et al. LncRNAs as regulators of autophagy and drug resistance in colorectal cancer. Front Oncol (2019) 9:1008. doi: 10.3389/fonc.2019.01008
37. Dahariya S, Paddibhatla I, Kumar S, Raghuwanshi S, Pallepati A, Gutti RK. Long non-coding RNA: Classification, biogenesis and functions in blood cells. Mol Immunol (2019) 112:82–92. doi: 10.1016/j.molimm.2019.04.011
38. Long Y, Wang X, Youmans DT, Cech TR. How do lncRNAs regulate transcription? Sci Adv (2017) 3(9):eaao2110. doi: 10.1126/sciadv.aao2110
39. Statello L, Guo CJ, Chen LL, Huarte M. Gene regulation by long non-coding RNAs and its biological functions. Nat Rev Mol Cell Biol (2021) 22(2):96–118. doi: 10.1038/s41580-020-00315-9
40. Zampetaki A, Albrecht A, Steinhofel K. Long non-coding RNA structure and function: Is there a link? Front Physiol (2018) 9:1201. doi: 10.3389/fphys.2018.01201
41. Kazimierczyk M, Kasprowicz MK, Kasprzyk ME, Wrzesinski J. Human long noncoding RNA interactome: Detection, characterization and function. Int J Mol Sci (2020) 21(3):1027. doi: 10.3390/ijms21031027
42. Li J, Liu C. Coding or noncoding, the converging concepts of RNAs. Front Genet (2019) 10:496. doi: 10.3389/fgene.2019.00496
43. Ransohoff JD, Wei Y, Khavari PA. The functions and unique features of long intergenic non-coding RNA. Nat Rev Mol Cell Biol (2018) 19(3):143–57. doi: 10.1038/nrm.2017.104
44. Derrien T, Johnson R, Bussotti G, Tanzer A, Djebali S, Tilgner H, et al. The GENCODE v7 catalog of human long noncoding RNAs: analysis of their gene structure, evolution, and expression. Genome Res (2012) 22(9):1775–89. doi: 10.1101/gr.132159.111
45. Kopp F, Mendell JT. Functional classification and experimental dissection of long noncoding RNAs. Cell (2018) 172(3):393–407. doi: 10.1016/j.cell.2018.01.011
46. Wang W, Min L, Qiu X, Wu X, Liu C, Ma J, et al. Biological function of long non-coding RNA (LncRNA) xist. Front Cell Dev Biol (2021) 9:645647. doi: 10.3389/fcell.2021.645647
47. Panda AC. Circular RNAs act as miRNA sponges. Adv Exp Med Biol (2018) 1087:67–79. doi: 10.1007/978-981-13-1426-1_6
48. Chi Y, Wang D, Wang J, Yu W, Yang J. Long non-coding RNA in the pathogenesis of cancers. Cells (2019) 8(9):1015. doi: 10.3390/cells8091015
49. Hennessy EJ. LncRNAs and cardiovascular disease. Adv Exp Med Biol (2022) 1363:71–95. doi: 10.1007/978-3-030-92034-0_5
50. Xu X, Cui L, Zhong W, Cai Y. Autophagy-associated lncRNAs: Promising targets for neurological disease diagnosis and therapy. Neural Plast (2020) 2020:8881687. doi: 10.1155/2020/8881687
51. Lin C, Yang L. Long noncoding RNA in cancer: Wiring signaling circuitry. Trends Cell Biol (2018) 28(4):287–301. doi: 10.1016/j.tcb.2017.11.008
52. Peng WX, Koirala P, Mo YY. LncRNA-mediated regulation of cell signaling in cancer. Oncogene. (2017) 36(41):5661–7. doi: 10.1038/onc.2017.184
53. He J, Zhu S, Liang X, Zhang Q, Luo X, Liu C, et al. LncRNA as a multifunctional regulator in cancer multi-drug resistance. Mol Biol Rep (2021) 48(8):1–15. doi: 10.1007/s11033-021-06603-7
54. Jin KT, Yao JY, Fang XL, Di H, Ma YY. Roles of lncRNAs in cancer: Focusing on angiogenesis. Life Sci (2020) 252:117647. doi: 10.1016/j.lfs.2020.117647
55. Zhang W, Liu Y, Fu Y, Han W, Xu H, Wen L, et al. Long non-coding RNA LINC00160 functions as a decoy of microRNA-132 to mediate autophagy and drug resistance in hepatocellular carcinoma via inhibition of PIK3R3. Cancer Lett (2020) 478:22–33. doi: 10.1016/j.canlet.2020.02.014
56. Zhou Y, Chen B. GAS5−mediated regulation of cell signaling (Review). Mol Med Rep (2020) 22(4):3049–56. doi: 10.3892/mmr.2020.11435
57. Wei JW, Huang K, Yang C, Kang CS. Non-coding RNAs as regulators in epigenetics (Review). Oncol Rep (2017) 37(1):3–9. doi: 10.3892/or.2016.5236
58. Luan S, Yang Y, Zhou Y, Zeng X, Xiao X, Liu B, et al. The emerging role of long noncoding RNAs in esophageal carcinoma: from underlying mechanisms to clinical implications. Cell Mol Life Sci (2021) 78(7):3403–22. doi: 10.1007/s00018-020-03751-0
59. Chen X, Xie R, Gu P, Huang M, Han J, Dong W, et al. Long noncoding RNA LBCS inhibits self-renewal and chemoresistance of bladder cancer stem cells through epigenetic silencing of sox2. Clin Cancer Res (2019) 25(4):1389–403. doi: 10.1158/1078-0432.CCR-18-1656
60. Chen LL. Linking long noncoding rna localization and function. Trends Biochem Sci (2016) 41(9):761–72. doi: 10.1016/j.tibs.2016.07.003
61. Bridges MC, Daulagala AC, Kourtidis A. LNCcation: lncRNA localization and function. J Cell Biol (2021) 220(2):e202009045. doi: 10.1083/jcb.202009045
62. Hanly DJ, Esteller M, Berdasco M. Interplay between long non-coding RNAs and epigenetic machinery: emerging targets in cancer? Philos Trans R Soc Lond B Biol Sci (2018) 373(1748):20170074. doi: 10.1098/rstb.2017.0074
63. Paraskevopoulou MD, Hatzigeorgiou AG. Analyzing MiRNA-LncRNA interactions. Methods Mol Biol (2016) 1402:271–86. doi: 10.1007/978-1-4939-3378-5_21
64. Meng X, Wang ZF, Lou QY, Rankine AN, Zheng WX, Zhang ZH, et al. Long non-coding RNAs in head and neck squamous cell carcinoma: Diagnostic biomarkers, targeted therapies, and prognostic roles. Eur J Pharmacol (2021) 902:174114. doi: 10.1016/j.ejphar.2021.174114
65. Park EG, Pyo SJ, Cui Y, Yoon SH, Nam JW. Tumor immune microenvironment lncRNAs. Brief Bioinform (2022) 23(1):bbab504. doi: 10.1093/bib/bbab504
66. Li Y, Jiang T, Zhou W, Li J, Li X, Wang Q, et al. Pan-cancer characterization of immune-related lncRNAs identifies potential oncogenic biomarkers. Nat Commun (2020) 11(1):1000. doi: 10.1038/s41467-020-14802-2
67. Zhang Y, Xu Y, Feng L, Li F, Sun Z, Wu T, et al. Comprehensive characterization of lncRNA-mRNA related ceRNA network across 12 major cancers. Oncotarget. (2016) 7(39):64148–67. doi: 10.18632/oncotarget.11637
68. Lin W, Zhou Q, Wang CQ, Zhu L, Bi C, Zhang S, et al. LncRNAs regulate metabolism in cancer. Int J Biol Sci (2020) 16(7):1194–206. doi: 10.7150/ijbs.40769
69. Gunelli R, Fragalà E, Fiori M. PCA3 in prostate cancer. Methods Mol Biol (2021) 2292:105–13. doi: 10.1007/978-1-0716-1354-2_9
70. Kretschmer A, Tilki D. Biomarkers in prostate cancer - current clinical utility and future perspectives. Crit Rev Oncol Hematol (2017) 120:180–93. doi: 10.1016/j.critrevonc.2017.11.007
71. Liu H, He X, Li T, Qu Y, Xu L, Hou Y, et al. PCGEM1 promotes proliferation, migration and invasion in prostate cancer by sponging miR-506 to upregulate TRIAP1. BMC Urol (2022) 22(1):14. doi: 10.1186/s12894-022-00969-x
72. Shang Z, Yu J, Sun L, Tian J, Zhu S, Zhang B, et al. LncRNA PCAT1 activates AKT and NF-κB signaling in castration-resistant prostate cancer by regulating the PHLPP/FKBP51/IKKα complex. Nucleic Acids Res (2019) 47(8):4211–25. doi: 10.1093/nar/gkz108
73. Alkhathami AG, Hadi A, Alfaifi M, Alshahrani MY, Verma AK, Beg MMA. Serum-based lncRNA ANRIL, TUG1, UCA1, and HIT expressions in breast cancer patients. Dis Markers (2022) 2022:9997212. doi: 10.1155/2022/9997212
74. Rivandi M, Khorrami MS, Fiuji H, Shahidsales S, Hasanzadeh M, Jazayeri MH, et al. The 9p21 locus: A potential therapeutic target and prognostic marker in breast cancer. J Cell Physiol (2018) 233(7):5170–9. doi: 10.1002/jcp.26332
75. Zhao W, Geng D, Li S, Chen Z, Sun M. LncRNA HOTAIR influences cell growth, migration, invasion, and apoptosis via the miR-20a-5p/HMGA2 axis in breast cancer. Cancer Med (2018) 7(3):842–55. doi: 10.1002/cam4.1353
76. Esfandi F, Fallah H, Arsang-Jang S, Taheri M, Ghafouri-Fard S. The expression of CCAT2, UCA1, PANDA and GHET1 long non-coding RNAs in lung cancer. Rep Biochem Mol Biol (2019) 8(1):36–41.
77. Feng C, Zhao Y, Li Y, Zhang T, Ma Y, Liu Y. LncRNA MALAT1 promotes lung cancer proliferation and gefitinib resistance by acting as a miR-200a sponge. Arch Bronconeumol (Engl Ed). (2019) 55(12):627–33. doi: 10.1016/j.arbres.2019.03.026
78. Wang X, Chen Y, Wang X, Tian H, Wang Y, Jin J, et al. Stem cell factor SOX2 confers ferroptosis resistance in lung cancer via upregulation of SLC7A11. Cancer Res (2021) 81(20):5217–29. doi: 10.1158/0008-5472.CAN-21-0567
79. Wei S, Wang K, Huang X, Zhao Z, Zhao Z. LncRNA MALAT1 contributes to non-small cell lung cancer progression via modulating miR-200a-3p/programmed death-ligand 1 axis. Int J Immunopathol Pharmacol (2019) 33:2058738419859699. doi: 10.1177/2058738419859699
80. Duan Q, Cai L, Zheng K, Cui C, Huang R, Zheng Z, et al. lncRNA KCNQ1OT1 knockdown inhibits colorectal cancer cell proliferation, migration and invasiveness via the PI3K/AKT pathway. Oncol Lett (2020) 20(1):601–10. doi: 10.3892/ol.2020.11619
81. Ren J, Ding L, Zhang D, Shi G, Xu Q, Shen S, et al. Carcinoma-associated fibroblasts promote the stemness and chemoresistance of colorectal cancer by transferring exosomal lncRNA H19. Theranostics. (2018) 8(14):3932–48. doi: 10.7150/thno.25541
82. Wu H, Qin W, Lu S, Wang X, Zhang J, Sun T, et al. Long noncoding RNA ZFAS1 promoting small nucleolar RNA-mediated 2'-o-methylation via NOP58 recruitment in colorectal cancer. Mol Cancer. (2020) 19(1):95. doi: 10.1186/s12943-020-01201-w
83. Ghafouri-Fard S, Esmaeili M, Taheri M, Samsami M. Highly upregulated in liver cancer (HULC): An update on its role in carcinogenesis. J Cell Physiol (2020) 235(12):9071–9. doi: 10.1002/jcp.29765
84. Malakar P, Stein I, Saragovi A, Winkler R, Stern-Ginossar N, Berger M, et al. Long noncoding RNA MALAT1 regulates cancer glucose metabolism by enhancing mTOR-mediated translation of TCF7L2. Cancer Res (2019) 79(10):2480–93. doi: 10.1158/0008-5472.CAN-18-1432
85. Zhang C, Yang X, Qi Q, Gao Y, Wei Q, Han S. lncRNA-HEIH in serum and exosomes as a potential biomarker in the HCV-related hepatocellular carcinoma. Cancer biomark (2018) 21(3):651–9. doi: 10.3233/CBM-170727
86. El-Khazragy N, Abdel Aziz MA, Hesham M, Matbouly S, Mostafa SA, Bakkar A, et al. Upregulation of leukemia-induced non-coding activator RNA (LUNAR1) predicts poor outcome in pediatric T-acute lymphoblastic leukemia. Immunobiology (2021) 226(6):152149. doi: 10.1016/j.imbio.2021.152149
87. Wang C, Li L, Li M, Wang W, Liu Y, Wang S. Silencing long non-coding RNA XIST suppresses drug resistance in acute myeloid leukemia through down-regulation of MYC by elevating microRNA-29a expression. Mol Med (2020) 26(1):114. doi: 10.1186/s10020-020-00229-4
88. Wu DM, Wen X, Han XR, Wang S, Wang YJ, Shen M, et al. Role of circular RNA DLEU2 in human acute myeloid leukemia. Mol Cell Biol (2018) 38(20):e00259–18. doi: 10.1128/MCB.00259-18
89. Yang X, Xie Z, Lei X, Gan R. Long non-coding RNA GAS5 in human cancer. Oncol Lett (2020) 20(3):2587–94. doi: 10.3892/ol.2020.11809
90. Wei GH, Wang X. lncRNA MEG3 inhibit proliferation and metastasis of gastric cancer via p53 signaling pathway. Eur Rev Med Pharmacol Sci (2017) 21(17):3850–6.
91. Tao F, Xu Y, Yang D, Tian B, Jia Y, Hou J, et al. LncRNA NKILA correlates with the malignant status and serves as a tumor-suppressive role in rectal cancer. J Cell Biochem (2018) 119(12):9809–16. doi: 10.1002/jcb.27300
92. Yang Y, Chen D, Liu H, Yang K. Increased expression of lncRNA CASC9 promotes tumor progression by suppressing autophagy-mediated cell apoptosis via the AKT/mTOR pathway in oral squamous cell carcinoma. Cell Death Dis (2019) 10(2):41. doi: 10.1038/s41419-018-1280-8
93. Chen J, Chen X, Fu L, Chen J, Chen Y, Liu F. LncRNA GACAT1 targeting miRNA-149 regulates the molecular mechanism of proliferation, apoptosis and autophagy of oral squamous cell carcinoma cells. Aging (Albany NY) (2021) 13(16):20359–71. doi: 10.18632/aging.203416
94. Lu X, Chen L, Li Y, Huang R, Meng X, Sun F. Long non-coding RNA LINC01207 promotes cell proliferation and migration but suppresses apoptosis and autophagy in oral squamous cell carcinoma by the microRNA-1301-3p/lactate dehydrogenase isoform a axis. Bioengineered (2021) 12(1):7780–93. doi: 10.1080/21655979.2021.1972784
95. Wang X, Liu W, Wang P, Li S. RNA Interference of long noncoding RNA HOTAIR suppresses autophagy and promotes apoptosis and sensitivity to cisplatin in oral squamous cell carcinoma. J Oral Pathol Med (2018) 47(10):930–7. doi: 10.1111/jop.12769
96. Jiang L, Ge W, Cui Y, Wang X. The regulation of long non-coding RNA 00958 (LINC00958) for oral squamous cell carcinoma (OSCC) cells death through absent in melanoma 2 (AIM2) depending on microRNA-4306 and Sirtuin1 (SIRT1) in vitro. Bioengineered (2021) 12(1):5085–98. doi: 10.1080/21655979.2021.1955561
97. Fang Z, Zhao J, Xie W, Sun Q, Wang H, Qiao B. LncRNA UCA1 promotes proliferation and cisplatin resistance of oral squamous cell carcinoma by sunppressing miR-184 expression. Cancer Med (2017) 6(12):2897–908. doi: 10.1002/cam4.1253
98. Wang X, Li H, Shi J. LncRNA HOXA11-AS promotes proliferation and cisplatin resistance of oral squamous cell carcinoma by suppression of miR-214-3p expression. BioMed Res Int (2019) 2019:8645153. doi: 10.1155/2019/8645153
99. Ma SQ, Wang YC, Li Y, Li XY, Yang J, Sheng YM. LncRNA XIST promotes proliferation and cisplatin resistance of oral squamous cell carcinoma by downregulating miR-27b-3p. J Biol Regul Homeost Agents (2020) 34(6):1993–2001. doi: 10.23812/20-222-A
100. Wang R, Lu X, Yu R. lncRNA MALAT1 promotes emt process and cisplatin resistance of oral squamous cell carcinoma via PI3K/AKT/m-TOR signal pathway. Onco Targets Ther (2020) 13:4049–61. doi: 10.2147/OTT.S251518
101. Zhang D, Ding L, Li Y, Ren J, Shi G, Wang Y, et al. Midkine derived from cancer-associated fibroblasts promotes cisplatin-resistance via up-regulation of the expression of lncRNA ANRIL in tumour cells. Sci Rep (2017) 7(1):16231. doi: 10.1038/s41598-017-13431-y
102. Xiao Z, Li J, Jin Q, Liu D. Long non-coding RNA OIP5-AS1 contributes to cisplatin resistance of oral squamous cell carcinoma through the miR-27b-3p/TRIM14 axis. Exp Ther Med (2021) 21(4):408. doi: 10.3892/etm.2021.9839
103. Zhang S, Ma H, Zhang D, Xie S, Wang W, Li Q, et al. LncRNA KCNQ1OT1 regulates proliferation and cisplatin resistance in tongue cancer via miR-211-5p mediated Ezrin/Fak/Src signaling. Cell Death Dis (2018) 9(7):742. doi: 10.1038/s41419-018-0793-5
104. Jiang Q, Wang Z, Qi Q, Li J, Xin Y, Qiu J. lncRNA SNHG26 promoted the growth, metastasis, and cisplatin resistance of tongue squamous cell carcinoma through PGK1/Akt/mTOR signal pathway. Mol Ther Oncolytics. (2022) 24:355–70. doi: 10.1016/j.omto.2021.12.021
105. Li J, Xu X, Zhang D, Lv H, Lei X. LncRNA LHFPL3-AS1 promotes oral squamous cell carcinoma growth and cisplatin resistance through targeting miR-362-5p/CHSY1 pathway. Onco Targets Ther (2021) 14:2293–300. doi: 10.2147/OTT.S298679
106. Qiao X, Liu J, Zhu L, Song R, Zhong M, Guo Y. Long noncoding RNA CEBPA-DT promotes cisplatin chemo-resistance through CEBPA/BCL2 mediated apoptosis in oral squamous cellular cancer. Int J Med Sci (2021) 18(16):3728–37. doi: 10.7150/ijms.64253
107. Tian T, Lv X, Pan G, Lu Y, Chen W, He W, et al. Long noncoding rna mprl promotes mitochondrial fission and cisplatin chemosensitivity via disruption of pre-miRNA processing. Clin Cancer Res (2019) 25(12):3673–88. doi: 10.1158/1078-0432.CCR-18-2739
108. Wang F, Ji X, Wang J, Ma X, Yang Y, Zuo J, et al. LncRNA PVT1 enhances proliferation and cisplatin resistance via regulating mir-194-5p/hif1a axis in oral squamous cell carcinoma. Onco Targets Ther (2020) 13:243–52. doi: 10.2147/OTT.S232405
109. Wang X, Yu H, Yu Z, Wang D. Exosomal lncRNA HEIH promotes cisplatin resistance in tongue squamous cell carcinoma via targeting miR-3619-5p/HDGF axis. Acta Histochem (2020) 122(8):151647. doi: 10.1016/j.acthis.2020.151647
110. Lin Z, Sun L, Xie S, Zhang S, Fan S, Li Q, et al. Chemotherapy-induced long non-coding RNA 1 promotes metastasis and chemo-resistance of TSCC via the wnt/β-catenin signaling pathway. Mol Ther (2018) 26(6):1494–508. doi: 10.1016/j.ymthe.2018.04.002
111. Li S, Shi Z, Fu S, Li Q, Li B, Sang L, et al. Exosomal-mediated transfer of APCDD1L-AS1 induces 5-fluorouracil resistance in oral squamous cell carcinoma via miR-1224-5p/nuclear receptor binding SET domain protein 2 (NSD2) axis. Bioengineered. (2021) 12(1):7188–204. doi: 10.1080/21655979.2021.1979442
112. Zhang K, Zhou H, Yan B, Cao X. TUG1/miR-133b/CXCR4 axis regulates cisplatin resistance in human tongue squamous cell carcinoma. Cancer Cell Int (2020) 20:148. doi: 10.1186/s12935-020-01224-9
113. Lin L, Liu X, Lv B. Long non-coding RNA MEG3 promotes autophagy and apoptosis of nasopharyngeal carcinoma cells via PTEN up-regulation by binding to microRNA-21. J Cell Mol Med (2021) 25(1):61–72. doi: 10.1111/jcmm.15759
114. Liu H, Zheng W, Chen Q, Zhou Y, Pan Y, Zhang J, et al. lncRNA CASC19 contributes to radioresistance of nasopharyngeal carcinoma by promoting autophagy via AMPK-mTOR pathway. Int J Mol Sci (2021) 22(3):1407. doi: 10.3390/ijms22031407
115. Peng J, Zheng H, Liu F, Wu Q, Liu S. The m6A methyltransferase METTL3 affects autophagy and progression of nasopharyngeal carcinoma by regulating the stability of lncRNA ZFAS1. Infect Agent Cancer (2022) 17(1):1. doi: 10.1186/s13027-021-00411-1
116. Li H, Huang J, Yu S, Li H, Zhou Y, Wu Q. HOXA11-AS induces cisplatin resistance by modulating the microRNA-98/PBX3 axis in nasopharyngeal carcinoma. Oncol Lett (2021) 21(6):493. doi: 10.3892/ol.2021.12754
117. Lin FJ, Lin XD, Xu LY, Zhu SQ. Long noncoding RNA HOXA11-AS modulates the resistance of nasopharyngeal carcinoma cells to cisplatin via miR-454-3p/c-Met. Mol Cells (2020) 43(10):856–69. doi: 10.14348/molcells.2020.0133
118. Yuan F, Lou Z, Zhou Z, Yan X. Long non−coding RNA KCNQ1OT1 promotes nasopharyngeal carcinoma cell cisplatin resistance via the miR−454/USP47 axis. Int J Mol Med (2021) 47(4):54. doi: 10.3892/ijmm.2021.4887
119. Zheng ZQ, Li ZX, Guan JL, Liu X, Li JY, Chen Y, et al. Long noncoding RNA TINCR-mediated regulation of acetyl-coa metabolism promotes nasopharyngeal carcinoma progression and chemoresistance. Cancer Res (2020) 80(23):5174–88. doi: 10.1158/0008-5472.CAN-19-3626
120. Yuan Z, Xiu C, Song K, Pei R, Miao S, Mao X, et al. Long non-coding RNA AFAP1-AS1/miR-320a/RBPJ axis regulates laryngeal carcinoma cell stemness and chemoresistance. J Cell Mol Med (2018) 22(9):4253–62. doi: 10.1111/jcmm.13707
121. Zhu X, Liu L, Wang Y, Cong J, Lin Z, Wang Y, et al. lncRNA MIAT/HMGB1 axis is involved in cisplatin resistance via regulating IL6-mediated activation of the JAK2/STAT3 pathway in nasopharyngeal carcinoma. Front Oncol (2021) 11:651693. doi: 10.3389/fonc.2021.651693
122. Liu F, Tai Y, Ma J. LncRNA NEAT1/let-7a-5p axis regulates the cisplatin resistance in nasopharyngeal carcinoma by targeting rsf-1 and modulating the ras-MAPK pathway. Cancer Biol Ther (2018) 19(6):534–42. doi: 10.1080/15384047.2018.1450119
123. Cui Z, Pu T, Zhang Y, Wang J, Zhao Y. Long non-coding RNA LINC00346 contributes to cisplatin resistance in nasopharyngeal carcinoma by repressing miR-342-5p. Open Biol (2020) 10(5):190286. doi: 10.1098/rsob.190286
124. Cao C, Zhou S, Hu J. Long noncoding RNA MAGI2-AS3/miR-218-5p/GDPD5/SEC61A1 axis drives cellular proliferation and migration and confers cisplatin resistance in nasopharyngeal carcinoma. Int Forum Allergy Rhinol (2020) 10(8):1012–23. doi: 10.1002/alr.22562
125. Ren S, Li G, Liu C, Cai T, Su Z, Wei M, et al. Next generation deep sequencing identified a novel lncRNA n375709 associated with paclitaxel resistance in nasopharyngeal carcinoma. Oncol Rep (2016) 36(4):1861–7. doi: 10.3892/or.2016.4981
126. Xue F, Cheng Y, Xu L, Tian C, Jiao H, Wang R, et al. LncRNA NEAT1/miR-129/Bcl-2 signaling axis contributes to HDAC inhibitor tolerance in nasopharyngeal cancer. Aging (Albany NY) (2020) 12(14):14174–88. doi: 10.18632/aging.103427
127. Wang Q, Zhang W, Hao S. LncRNA CCAT1 modulates the sensitivity of paclitaxel in nasopharynx cancers cells via miR-181a/CPEB2 axis. Cell Cycle (2017) 16(8):795–801. doi: 10.1080/15384101.2017.1301334
128. Zhu Y, He D, Bo H, Liu Z, Xiao M, Xiang L, et al. The MRVI1-AS1/ATF3 signaling loop sensitizes nasopharyngeal cancer cells to paclitaxel by regulating the hippo-TAZ pathway. Oncogene. (2019) 38(32):6065–81. doi: 10.1038/s41388-019-0858-7
129. Li H, Huang J, Yu S, Lou Z. Long non-coding RNA DLEU1 up-regulates BIRC6 expression by competitively sponging miR-381-3p to promote cisplatin resistance in nasopharyngeal carcinoma. Onco Targets Ther (2020) 13:2037–45. doi: 10.2147/OTT.S237456
130. Wang J, Zhu Y, Ni S, Liu S. LncRNA GAS5 suppressed proliferation and promoted apoptosis in laryngeal squamous cell carcinoma by targeting MiR-26a-5p and modifying ULK2. Cancer Manag Res (2021) 13:871–87. doi: 10.2147/CMAR.S250778
131. Chen L, Xu Z, Zhao J, Zhai X, Li J, Zhang Y, et al. H19/miR-107/HMGB1 axis sensitizes laryngeal squamous cell carcinoma to cisplatin by suppressing autophagy in vitro and in vivo. Cell Biol Int (2021) 45(3):674–85. doi: 10.1002/cbin.11520
132. Jiang Q, Liu S, Hou L, Guan Y, Yang S, Luo Z. The implication of LncRNA MALAT1 in promoting chemo-resistance of laryngeal squamous cell carcinoma cells. J Clin Lab Anal (2020) 34(4):e23116. doi: 10.1002/jcla.23116
133. Song K, Yu P, Zhang C, Yuan Z, Zhang H. The LncRNA FGD5-AS1/miR-497-5p axis regulates septin 2 (SEPT2) to accelerate cancer progression and increase cisplatin-resistance in laryngeal squamous cell carcinoma. Mol Carcinog (2021) 60(7):469–80. doi: 10.1002/mc.23305
134. Shen N, Duan X, Feng Y, Zhang J, Qiao X, Ding W. Long non-coding RNA HOXA11 antisense RNA upregulates spermatogenesis-associated serine-rich 2-like to enhance cisplatin resistance in laryngeal squamous cell carcinoma by suppressing microRNA-518a. Bioengineered. (2022) 13(1):974–84. doi: 10.1080/21655979.2021.2016038
135. Yang S, Yuan ZJ, Zhu YH, Chen X, Wang W. lncRNA PVT1 promotes cetuximab resistance of head and neck squamous cell carcinoma cells by inhibiting miR-124-3p. Head Neck (2021) 43(9):2712–23. doi: 10.1002/hed.26742
136. Goyal B, Yadav SRM, Awasthee N, Gupta S, Kunnumakkara AB, Gupta SC. Diagnostic, prognostic, and therapeutic significance of long non-coding RNA MALAT1 in cancer. Biochim Biophys Acta Rev Cancer. (2021) 1875(2):188502. doi: 10.1016/j.bbcan.2021.188502
137. Xue JY, Huang C, Wang W, Li HB, Sun M, Xie M. HOXA11-AS: a novel regulator in human cancer proliferation and metastasis. Onco Targets Ther (2018) 11:4387–93. doi: 10.2147/OTT.S166961
138. Sanchez Calle A, Kawamura Y, Yamamoto Y, Takeshita F, Ochiya T. Emerging roles of long non-coding RNA in cancer. Cancer Sci (2018) 109(7):2093–100. doi: 10.1111/cas.13642
139. Ghafouri-Fard S, Taheri M. Maternally expressed gene 3 (MEG3): A tumor suppressor long non coding RNA. BioMed Pharmacother (2019) 118:109129. doi: 10.1016/j.biopha.2019.109129
140. Li Q, Wang J, Meng X, Chen W, Feng J, Mao J. Identification of autophagy-related gene and lncRNA signatures in the prognosis of HNSCC. Oral Dis (2021). doi: 10.1111/odi.13889
141. Bukowski K, Kciuk M, Kontek R. Mechanisms of multidrug resistance in cancer chemotherapy. Int J Mol Sci (2020) 21(9):3233. doi: 10.3390/ijms21093233
142. Wang Y, Fang Z, Hong M, Yang D, Xie W. Long-noncoding RNAs (lncRNAs) in drug metabolism and disposition, implications in cancer chemo-resistance. Acta Pharm Sin B (2020) 10(1):105–12. doi: 10.1016/j.apsb.2019.09.011
143. Asano T. Drug resistance in cancer therapy and the role of epigenetics. J Nippon Med Sch (2020) 87(5):244–51. doi: 10.1272/jnms.JNMS.2020_87-508
144. Almazroo OA, Miah MK, Venkataramanan R. Drug metabolism in the liver. Clin Liver Dis (2017) 21(1):1–20. doi: 10.1016/j.cld.2016.08.001
145. Zhao M, Ma J, Li M, Zhang Y, Jiang B, Zhao X, et al. Cytochrome P450 enzymes and drug metabolism in humans. Int J Mol Sci (2021) 22(23):12808. doi: 10.3390/ijms222312808
146. Kaur G, Gupta SK, Singh P, Ali V, Kumar V, Verma M. Drug-metabolizing enzymes: role in drug resistance in cancer. Clin Transl Oncol (2020) 22(10):1667–80. doi: 10.1007/s12094-020-02325-7
147. Liu X. Transporter-mediated drug-drug interactions and their significance. Adv Exp Med Biol (2019) 1141:241–91. doi: 10.1007/978-981-13-7647-4_5
148. Zhou Y, Sun W, Qin Z, Guo S, Kang Y, Zeng S, et al. LncRNA regulation: New frontiers in epigenetic solutions to drug chemoresistance. Biochem Pharmacol (2021) 189:114228. doi: 10.1016/j.bcp.2020.114228
149. Roden DM, McLeod HL, Relling MV, Williams MS, Mensah GA, Peterson JF, et al. Pharmacogenomics. Lancet (2019) 394(10197):521–32. doi: 10.1016/S0140-6736(19)31276-0
150. Shen L, Li N, Zhou Q, Li Z, Shen L. Development and validation of an autophagy-related LncRNA prognostic signature in head and neck squamous cell carcinoma. Front Oncol (2021) 11:743611. doi: 10.3389/fonc.2021.743611
151. Sun Y, Ma L. New insights into long non-coding RNA MALAT1 in cancer and metastasis. Cancers (Basel) (2019) 11(2):216. doi: 10.3390/cancers11020216
152. Xiao H, Tang K, Liu P, Chen K, Hu J, Zeng J, et al. LncRNA MALAT1 functions as a competing endogenous RNA to regulate ZEB2 expression by sponging miR-200s in clear cell kidney carcinoma. Oncotarget (2015) 6(35):38005–15. doi: 10.18632/oncotarget.5357
153. Ye Y, Zhang F, Chen Q, Huang Z, Li M. LncRNA MALAT1 modified progression of clear cell kidney carcinoma (KIRC) by regulation of miR-194-5p/ACVR2B signaling. Mol Carcinog (2019) 58(2):279–92. doi: 10.1002/mc.22926
154. Pang EJ, Yang R, Fu XB, Liu YF. Overexpression of long non-coding RNA MALAT1 is correlated with clinical progression and unfavorable prognosis in pancreatic cancer. Tumour Biol (2015) 36(4):2403–7. doi: 10.1007/s13277-014-2850-8
155. Zhang D, Fang C, Li H, Lu C, Huang J, Pan J, et al. Long ncRNA MALAT1 promotes cell proliferation, migration, and invasion in prostate cancer via sponging miR-145. Transl Androl Urol (2021) 10(6):2307–19. doi: 10.21037/tau-20-1526
156. Hao T, Wang Z, Yang J, Zhang Y, Shang Y, Sun J. MALAT1 knockdown inhibits prostate cancer progression by regulating miR-140/BIRC6 axis. BioMed Pharmacother (2020) 123:109666. doi: 10.1016/j.biopha.2019.109666
157. Li Z, Zhou Y, Tu B, Bu Y, Liu A, Kong J. Long noncoding RNA MALAT1 affects the efficacy of radiotherapy for esophageal squamous cell carcinoma by regulating Cks1 expression. J Oral Pathol Med (2017) 46(8):583–90. doi: 10.1111/jop.12538
158. Zhao X, Chen Q, Cai Y, Chen D, Bei M, Dong H, et al. TRA2A binds with LncRNA MALAT1 to promote esophageal cancer progression by regulating EZH2/β-catenin pathway. J Cancer (2021) 12(16):4883–90. doi: 10.7150/jca.55661
159. Wu Y, Sarkissyan M, Ogah O, Kim J, Vadgama JV. Expression of MALAT1 promotes trastuzumab resistance in HER2 overexpressing breast cancers. Cancers (Basel) (2020) 12(7):1918. doi: 10.3390/cancers12071918
160. Yue X, Wu WY, Dong M, Guo M. LncRNA MALAT1 promotes breast cancer progression and doxorubicin resistance via regulating miR-570-3p. BioMed J (2021) 44(6s2):S296–s304. doi: 10.1016/j.bj.2020.11.002
161. Zhang Y, Chen Z, Li MJ, Guo HY, Jing NC. Long non-coding RNA metastasis-associated lung adenocarcinoma transcript 1 regulates the expression of Gli2 by miR-202 to strengthen gastric cancer progression. BioMed Pharmacother (2017) 85:264–71. doi: 10.1016/j.biopha.2016.11.014
162. Mao TL, Fan MH, Dlamini N, Liu CL. LncRNA MALAT1 facilitates ovarian cancer progression through promoting chemoresistance and invasiveness in the tumor microenvironment. Int J Mol Sci (2021) 22(19):10201. doi: 10.3390/ijms221910201
163. Sun Z, Ou C, Liu J, Chen C, Zhou Q, Yang S, et al. YAP1-induced MALAT1 promotes epithelial-mesenchymal transition and angiogenesis by sponging miR-126-5p in colorectal cancer. Oncogene (2019) 38(14):2627–44. doi: 10.1038/s41388-018-0628-y
164. Xu Y, Zhang X, Hu X, Zhou W, Zhang P, Zhang J, et al. The effects of lncRNA MALAT1 on proliferation, invasion and migration in colorectal cancer through regulating SOX9. Mol Med (2018) 24(1):52. doi: 10.1186/s10020-018-0050-5
165. Ghafouri-Fard S, Shoorei H, Bahroudi Z, Abak A, Taheri M. The role of H19 lncRNA in conferring chemoresistance in cancer cells. BioMed Pharmacother (2021) 138:111447. doi: 10.1016/j.biopha.2021.111447
166. Guo K, Qian K, Shi Y, Sun T, Wang Z. LncRNA-MIAT promotes thyroid cancer progression and function as ceRNA to target EZH2 by sponging miR-150-5p. Cell Death Dis (2021) 12(12):1097. doi: 10.1038/s41419-021-04386-0
167. Lin D, Xu HP, Lin JH, Hu HH, Wang Q, Zhang J. Long non-coding RNA MIAT promotes non-small cell lung cancer progression by sponging miR-1246. Eur Rev Med Pharmacol Sci (2020) 24(17):8626. doi: 10.26355/eurrev_202009_22762
168. Ye T, Feng J, Cui M, Yang J, Wan X, Xie D, et al. LncRNA MIAT services as a noninvasive biomarker for diagnosis and correlated with immune infiltrates in breast cancer. Int J Womens Health (2021) 13:991–1004. doi: 10.2147/IJWH.S312714
169. Zhang L, Ge S, Cao B. Long non-coding RNA MIAT promotes cervical cancer proliferation and migration. J Biochem (2020) 168(2):183–90. doi: 10.1093/jb/mvaa037
170. Ding Z, Ying W, He Y, Chen X, Jiao Y, Wang J, et al. lncRNA-UCA1 in the diagnosis of bladder cancer: A meta-analysis. Med (Baltimore) (2021) 100(11):e24805. doi: 10.1097/MD.0000000000024805
171. Wang Y, Li X, Chen W, Wu W. The common region of lncRNAs UCA1 and UCA1α contributes to the bladder cancer tumorigenesis. Eur J Cancer Prev (2021) 30(5):389–92. doi: 10.1097/CEJ.0000000000000642
172. Li YJ, Lei YH, Yao N, Wang CR, Hu N, Ye WC, et al. Autophagy and multidrug resistance in cancer. Chin J Cancer (2017) 36(1):52. doi: 10.1186/s40880-017-0219-2
173. Ferro F, Servais S, Besson P, Roger S, Dumas JF, Brisson L. Autophagy and mitophagy in cancer metabolic remodelling. Semin Cell Dev Biol (2020) 98:129–38. doi: 10.1016/j.semcdb.2019.05.029
174. Li X, He S, Ma B. Autophagy and autophagy-related proteins in cancer. Mol Cancer (2020) 19(1):12. doi: 10.1186/s12943-020-1138-4
175. Levine B, Kroemer G. Biological functions of autophagy genes: A disease perspective. Cell (2019) 176(1-2):11–42. doi: 10.1016/j.cell.2018.09.048
176. Wang Y, Zhang H. Regulation of autophagy by mtOR signaling pathway. Adv Exp Med Biol (2019) 1206:67–83. doi: 10.1007/978-981-15-0602-4_3
177. Babaei G, Aziz SG, Jaghi NZZ. EMT. Cancer stem cells and autophagy; the three main axes of metastasis. BioMed Pharmacother (2021) 133:110909. doi: 10.1016/j.biopha.2020.110909
178. Saha S, Panigrahi DP, Patil S, Bhutia SK. Autophagy in health and disease: A comprehensive review. BioMed Pharmacother (2018) 104:485–95. doi: 10.1016/j.biopha.2018.05.007
179. Al-Bari MAA, Xu P. Molecular regulation of autophagy machinery by mTOR-dependent and -independent pathways. Ann N Y Acad Sci (2020) 1467(1):3–20. doi: 10.1111/nyas.14305
180. Zhang H, Lu B. The roles of ceRNAs-mediated autophagy in cancer chemoresistance and metastasis. Cancers (Basel) (2020) 12(10):2926. doi: 10.3390/cancers12102926
181. Liu W, Meng Y, Zong C, Zhang S, Wei L. Autophagy and tumorigenesis. Adv Exp Med Biol (2020) 1207:275–99. doi: 10.1007/978-981-15-4272-5_20
182. Li X, Jin F, Li Y. A novel autophagy-related lncRNA prognostic risk model for breast cancer. J Cell Mol Med (2021) 25(1):4–14. doi: 10.1111/jcmm.15980
183. Zhang R, Zhu Q, Yin D, Yang Z, Guo J, Zhang J, et al. Identification and validation of an autophagy-related lncRNA signature for patients with breast cancer. Front Oncol (2020) 10:597569. doi: 10.3389/fonc.2020.597569
184. Gao X, Zhang S, Chen Y, Wen X, Chen M, Wang S, et al. Development of a novel six-long noncoding RNA signature predicting survival of patients with bladder urothelial carcinoma. J Cell Biochem (2019) 120(12):19796–809. doi: 10.1002/jcb.29285
185. Sun Z, Jing C, Xiao C, Li T. An autophagy-related long non-coding RNA prognostic signature accurately predicts survival outcomes in bladder urothelial carcinoma patients. Aging (Albany NY) (2020) 12(15):15624–37. doi: 10.18632/aging.103718
186. Deng Z, Li X, Shi Y, Lu Y, Yao W, Wang J. A novel autophagy-related IncRNAs signature for prognostic prediction and clinical value in patients with pancreatic cancer. Front Cell Dev Biol (2020) 8:606817. doi: 10.3389/fcell.2020.606817
187. Wei J, Ge X, Tang Y, Qian Y, Lu W, Jiang K, et al. An autophagy-related long noncoding rna signature contributes to poor prognosis in colorectal cancer. J Oncol (2020) 2020:4728947. doi: 10.1155/2020/4728947
188. Zhao D, Sun X, Long S, Yao S. An autophagy-related long non-coding RNA signature for patients with colorectal cancer. Physiol Int (2021) 108(2):202–20. doi: 10.1556/2060.2021.00125
189. Wu L, Wen Z, Song Y, Wang L. A novel autophagy-related lncRNA survival model for lung adenocarcinoma. J Cell Mol Med (2021) 25(12):5681–90. doi: 10.1111/jcmm.16582
190. Zhang X, Cao Y, Chen L. Construction of a prognostic signature of autophagy-related lncRNAs in non-small-cell lung cancer. BMC Cancer (2021) 21(1):921. doi: 10.1186/s12885-021-08654-2
191. Guo Y, Yang PT, Wang ZW, Xu K, Kou WH, Luo H. Identification of three autophagy-related long non-coding RNAs as a novel head and neck squamous cell carcinoma prognostic signature. Front Oncol (2020) 10:603864. doi: 10.3389/fonc.2020.603864
192. Qu X, Alsager S, Zhuo Y, Shan B. HOX transcript antisense RNA (HOTAIR) in cancer. Cancer Lett (2019) 454:90–7. doi: 10.1016/j.canlet.2019.04.016
193. Zhang Y, Weinberg RA. Epithelial-to-mesenchymal transition in cancer: complexity and opportunities. Front Med (2018) 12(4):361–73. doi: 10.1007/s11684-018-0656-6
194. Chen T, You Y, Jiang H, Wang ZZ. Epithelial-mesenchymal transition (EMT): A biological process in the development, stem cell differentiation, and tumorigenesis. J Cell Physiol (2017) 232(12):3261–72. doi: 10.1002/jcp.25797
195. Pastushenko I, Blanpain C. EMT transition states during tumor progression and metastasis. Trends Cell Biol (2019) 29(3):212–26. doi: 10.1016/j.tcb.2018.12.001
196. Lin YT, Wu KJ. Epigenetic regulation of epithelial-mesenchymal transition: focusing on hypoxia and TGF-β signaling. J BioMed Sci (2020) 27(1):39. doi: 10.1186/s12929-020-00632-3
197. Hao Y, Baker D, Ten Dijke P. TGF-β-mediated epithelial-mesenchymal transition and cancer metastasis. Int J Mol Sci (2019) 20(11):2767. doi: 10.3390/ijms20112767
198. Tang Q, Chen J, Di Z, Yuan W, Zhou Z, Liu Z, et al. TM4SF1 promotes EMT and cancer stemness via the wnt/β-catenin/SOX2 pathway in colorectal cancer. J Exp Clin Cancer Res (2020) 39(1):232. doi: 10.1186/s13046-020-01690-z
199. Tian H, Zhou T, Chen H, Li C, Jiang Z, Lao L, et al. Bone morphogenetic protein-2 promotes osteosarcoma growth by promoting epithelial-mesenchymal transition (EMT) through the wnt/β-catenin signaling pathway. J Orthop Res (2019) 37(7):1638–48. doi: 10.1002/jor.24244
200. Wang Y, Zhong Y, Hou T, Liao J, Zhang C, Sun C, et al. PM2.5 induces EMT and promotes CSC properties by activating notch pathway in vivo and vitro. Ecotoxicol Environ Saf (2019) 178:159–67. doi: 10.1016/j.ecoenv.2019.03.086
201. Xu H, Dun S, Gao Y, Ming J, Hui L, Qiu X. TMEM107 inhibits EMT and invasion of NSCLC through regulating the hedgehog pathway. Thorac Cancer (2021) 12(1):79–89. doi: 10.1111/1759-7714.13715
202. Gao S, Hu J, Wu X, Liang Z. PMA treated THP-1-derived-IL-6 promotes EMT of SW48 through STAT3/ERK-dependent activation of wnt/β-catenin signaling pathway. BioMed Pharmacother (2018) 108:618–24. doi: 10.1016/j.biopha.2018.09.067
203. Grelet S, Link LA, Howley B, Obellianne C, Palanisamy V, Gangaraju VK, et al. A regulated PNUTS mRNA to lncRNA splice switch mediates EMT and tumour progression. Nat Cell Biol (2017) 19(9):1105–15. doi: 10.1038/ncb3595
204. Wang X, Lai Q, He J, Li Q, Ding J, Lan Z, et al. LncRNA SNHG6 promotes proliferation, invasion and migration in colorectal cancer cells by activating TGF-β/Smad signaling pathway via targeting UPF1 and inducing EMT via regulation of ZEB1. Int J Med Sci (2019) 16(1):51–9. doi: 10.7150/ijms.27359
205. Zhang H, Wang J, Yin Y, Meng Q, Lyu Y. The role of EMT-related lncRNA in the process of triple-negative breast cancer metastasis. Biosci Rep (2021) 41(2):BSR20203121. doi: 10.1042/BSR20203121
206. Li Z, Ren R, Wang L, Wang Z, Zong X, Sun P, et al. lncRNA KCNQ1OT1 promotes emt, angiogenesis, and stemness of pituitary adenoma by upregulation of RAB11A. J Oncol (2022) 2022:4474476. doi: 10.1155/2022/4474476
207. Tong H, Li T, Gao S, Yin H, Cao H, He W. An epithelial-mesenchymal transition-related long noncoding RNA signature correlates with the prognosis and progression in patients with bladder cancer. Biosci Rep (2021) 41(1):BSR20203944. doi: 10.1042/BSR20203944
208. Wang Y, Liu J, Ren F, Chu Y, Cui B. Identification and validation of a four-long non-coding rna signature associated with immune infiltration and prognosis in colon cancer. Front Genet (2021) 12:671128. doi: 10.3389/fgene.2021.671128
209. Meng Q, Liu M, Cheng R. LINC00461/miR-4478/E2F1 feedback loop promotes non-small cell lung cancer cell proliferation and migration. Biosci Rep (2020) 40(2):BSR20191345. doi: 10.1042/BSR20191345
210. Wu HK, Liu C, Li XX, Ji W, Xin CD, Hu ZQ, et al. PHLPP2 is regulated by competing endogenous RNA network in pathogenesis of colon cancer. Aging (Albany NY) (2020) 12(13):12812–40. doi: 10.18632/aging.103246
211. Xu Z, Chen Z, Peng M, Zhang Z, Luo W, Shi R, et al. MicroRNA MiR-490-5p suppresses pancreatic cancer through regulating epithelial-mesenchymal transition via targeting MAGI2 antisense RNA 3. Bioengineered. (2022) 13(2):2673–85. doi: 10.1080/21655979.2021.2024653
212. Li D, Wang J, Zhang M, Hu X, She J, Qiu X, et al. LncRNA MAGI2-AS3 is regulated by brd4 and promotes gastric cancer progression via maintaining zeb1 overexpression by sponging miR-141/200a. Mol Ther Nucleic Acids (2020) 19:109–23. doi: 10.1016/j.omtn.2019.11.003
213. Shen D, Xu J, Cao X, Cao X, Tan H, Deng H. Long noncoding RNA MAGI2-AS3 inhibits bladder cancer progression through MAGI2/PTEN/epithelial-mesenchymal transition (EMT) axis. Cancer biomark (2021) 30(2):155–65. doi: 10.3233/CBM-201421
214. Pan G, Liu Y, Shang L, Zhou F, Yang S. EMT-associated microRNAs and their roles in cancer stemness and drug resistance. Cancer Commun (Lond). (2021) 41(3):199–217. doi: 10.1002/cac2.12138
215. Smith AG, Macleod KF. Autophagy, cancer stem cells and drug resistance. J Pathol (2019) 247(5):708–18. doi: 10.1002/path.5222
216. Huang T, Song X, Xu D, Tiek D, Goenka A, Wu B, et al. Stem cell programs in cancer initiation, progression, and therapy resistance. Theranostics (2020) 10(19):8721–43. doi: 10.7150/thno.41648
217. Safa AR. Resistance to drugs and cell death in cancer stem cells (CSCs). J Transl Sci (2020) 6(3):341. doi: 10.15761/JTS.1000341
218. Liu YP, Zheng CC, Huang YN, He ML, Xu WW, Li B. Molecular mechanisms of chemo- and radiotherapy resistance and the potential implications for cancer treatment. MedComm (2020) (2021) 2(3):315–40. doi: 10.1002/mco2.55
219. Erin N, Grahovac J, Brozovic A, Efferth T. Tumor microenvironment and epithelial mesenchymal transition as targets to overcome tumor multidrug resistance. Drug Resist Updat. (2020) 53:100715. doi: 10.1016/j.drup.2020.100715
220. Xia S, Pan Y, Liang Y, Xu J, Cai X. The microenvironmental and metabolic aspects of sorafenib resistance in hepatocellular carcinoma. EBioMedicine. (2020) 51:102610. doi: 10.1016/j.ebiom.2019.102610
221. Prieto-Vila M, Takahashi RU, Usuba W, Kohama I, Ochiya T. Drug resistance driven by cancer stem cells and their niche. Int J Mol Sci (2017) 18(12):2574. doi: 10.3390/ijms18122574
222. Yang L, Shi P, Zhao G, Xu J, Peng W, Zhang J, et al. Targeting cancer stem cell pathways for cancer therapy. Signal Transduct Target Ther (2020) 5(1):8. doi: 10.1038/s41392-020-0110-5
223. Castro-Oropeza R, Melendez-Zajgla J, Maldonado V, Vazquez-Santillan K. The emerging role of lncRNAs in the regulation of cancer stem cells. Cell Oncol (Dordr) (2018) 41(6):585–603. doi: 10.1007/s13402-018-0406-4
224. Yue J, Wu Y, Qiu L, Zhao R, Jiang M, Zhang H. LncRNAs link cancer stemness to therapy resistance. Am J Cancer Res (2021) 11(4):1051–68.
225. Li H, Zhu L, Xu L, Qin K, Liu C, Yu Y, et al. Long noncoding RNA linc00617 exhibits oncogenic activity in breast cancer. Mol Carcinog (2017) 56(1):3–17. doi: 10.1002/mc.22338
226. Li Z, Jiang P, Li J, Peng M, Zhao X, Zhang X, et al. Tumor-derived exosomal lnc-Sox2ot promotes EMT and stemness by acting as a ceRNA in pancreatic ductal adenocarcinoma. Oncogene. (2018) 37(28):3822–38. doi: 10.1038/s41388-018-0237-9
227. Huang Y, Wang L, Liu D. HOTAIR regulates colorectal cancer stem cell properties and promotes tumorigenicity by sponging miR-211-5p and modulating FLT-1. Cell Cycle (2021) 20(19):1999–2009. doi: 10.1080/15384101.2021.1962636
228. Deng J, Yang M, Jiang R, An N, Wang X, Liu B. Long non-coding RNA HOTAIR regulates the proliferation, self-renewal capacity, tumor formation and migration of the cancer stem-like cell (CSC) subpopulation enriched from breast cancer cells. PLoS One (2017) 12(1):e0170860. doi: 10.1371/journal.pone.0170860
229. Li T, Zhu J, Wang X, Chen G, Sun L, Zuo S, et al. Long non-coding RNA lncTCF7 activates the wnt/β-catenin pathway to promote metastasis and invasion in colorectal cancer. Oncol Lett (2017) 14(6):7384–90. doi: 10.3892/ol.2017.7154
230. Lecerf C, Peperstraete E, Le Bourhis X, Adriaenssens E. Propagation and maintenance of cancer stem cells: a major influence of the long non-coding RNA H19. Cells (2020) 9(12):2613. doi: 10.3390/cells9122613
231. Shima H, Kida K, Adachi S, Yamada A, Sugae S, Narui K, et al. Lnc RNA H19 is associated with poor prognosis in breast cancer patients and promotes cancer stemness. Breast Cancer Res Treat (2018) 170(3):507–16. doi: 10.1007/s10549-018-4793-z
232. Sasaki N, Toyoda M, Yoshimura H, Matsuda Y, Arai T, Takubo K, et al. H19 long non-coding RNA contributes to sphere formation and invasion through regulation of CD24 and integrin expression in pancreatic cancer cells. Oncotarget (2018) 9(78):34719–34. doi: 10.18632/oncotarget.26176
233. Hong L, Wang H, Wang J, Wei S, Zhang F, Han J, et al. LncRNA PTCSC3 inhibits tumor growth and cancer cell stemness in gastric cancer by interacting with lncRNA linc-pint. Cancer Manag Res (2019) 11:10393–9. doi: 10.2147/CMAR.S231369
234. Momtazmanesh S, Rezaei N. Long non-coding RNAs in diagnosis, treatment, prognosis, and progression of glioma: a state-of-the-art review. Front Oncol (2021) 11:712786. doi: 10.3389/fonc.2021.712786
235. Xiong F, Zhu K, Deng S, Huang H, Yang L, Gong Z, et al. AFAP1-AS1: a rising star among oncogenic long non-coding RNAs. Sci China Life Sci (2021) 64(10):1602–11. doi: 10.1007/s11427-020-1874-6
236. Wu XB, Feng X, Chang QM, Zhang CW, Wang ZF, Liu J, et al. Cross-talk among AFAP1-AS1, ACVR1 and microRNA-384 regulates the stemness of pancreatic cancer cells and tumorigenicity in nude mice. J Exp Clin Cancer Res (2019) 38(1):107. doi: 10.1186/s13046-019-1051-0
237. Picon H, Guddati AK. Mechanisms of resistance in head and neck cancer. Am J Cancer Res (2020) 10(9):2742–51.
238. Zhou JC, Zhang JJ, Ma W, Zhang W, Ke ZY, Ma LG. Anti-tumor effect of HOTAIR-miR-613-SNAI2 axis through suppressing EMT and drug resistance in laryngeal squamous cell carcinoma. RSC Adv (2018) 8(52):29879–89. doi: 10.1039/C8RA04514C
239. Liu Y, Liu X, Lin C, Jia X, Zhu H, Song J, et al. Noncoding RNAs regulate alternative splicing in cancer. J Exp Clin Cancer Res (2021) 40(1):11. doi: 10.1186/s13046-020-01798-2
240. Gao N, Li Y, Li J, Gao Z, Yang Z, Li Y, et al. Long non-coding RNAs: the regulatory mechanisms, research strategies, and future directions in cancers. Front Oncol (2020) 10:598817. doi: 10.3389/fonc.2020.598817
241. Corrà F, Agnoletto C, Minotti L, Baldassari F, Volinia S. The network of non-coding rnas in cancer drug resistance. Front Oncol (2018) 8:327. doi: 10.3389/fonc.2018.00327
242. Shi T, Gao G, Cao Y. Long noncoding RNAs as novel biomarkers have a promising future in cancer diagnostics. Dis Markers (2016) 2016:9085195. doi: 10.1155/2016/9085195
Keywords: lncRNA, autophagy, cancer, EMT, stemness, HNSCC (head and neck squamous cell carcinoma), drug resistance, chemoresistance
Citation: Peña-Flores JA, Bermúdez M, Ramos-Payán R, Villegas-Mercado CE, Soto-Barreras U, Muela-Campos D, Álvarez-Ramírez A, Pérez-Aguirre B, Larrinua-Pacheco AD, López-Camarillo C, López-Gutiérrez JA, Garnica-Palazuelos J, Estrada-Macías ME, Cota-Quintero JL and Barraza-Gómez AA (2022) Emerging role of lncRNAs in drug resistance mechanisms in head and neck squamous cell carcinoma. Front. Oncol. 12:965628. doi: 10.3389/fonc.2022.965628
Received: 09 June 2022; Accepted: 01 July 2022;
Published: 01 August 2022.
Edited by:
Aamir Ahmad, University of Alabama at Birmingham, United StatesReviewed by:
Dong Ye, Ningbo Medical Centre Li Huili Hospital, ChinaAbilash Gangula, University of Missouri, United States
Copyright © 2022 Peña-Flores, Bermúdez, Ramos-Payán, Villegas-Mercado, Soto-Barreras, Muela-Campos, Álvarez-Ramírez, Pérez-Aguirre, Larrinua-Pacheco, López-Camarillo, López-Gutiérrez, Garnica-Palazuelos, Estrada-Macías, Cota-Quintero and Barraza-Gómez. This is an open-access article distributed under the terms of the Creative Commons Attribution License (CC BY). The use, distribution or reproduction in other forums is permitted, provided the original author(s) and the copyright owner(s) are credited and that the original publication in this journal is cited, in accordance with accepted academic practice. No use, distribution or reproduction is permitted which does not comply with these terms.
*Correspondence: Mercedes Bermúdez, mbermudez@uach.mx
†These authors share first authorship