- 1Department of Anesthesiology, National Cancer Center/National Clinical Research Center for Cancer/Cancer Hospital, Chinese Academy of Medical Sciences and Peking Union Medical College, Beijing, China
- 2Department of Anesthesiology, National Cancer Center/National Clinical Research Center for Cancer/Cancer Hospital & Shenzhen Hospital, Chinese Academy of Medical Sciences and Peking Union Medical College, Shenzhen, China
- 3Department of Anesthesiology, National Cancer Center/National Clinical Research Center for Cancer/Hebei Cancer Hospital, Chinese Academy of Medical Sciences, Langfang, China
Clinical and experimental evidence suggested that anesthesia choice can influence cancer progression and patients’ outcomes by modulating tumor microenvironment and tumorigenic pathways. Curative resection is the mainstay of therapy for hepatocellular carcinoma (HCC), which is an intractable disease due to high recurrence and poor prognosis. However, different anesthetics may play different roles in alleviating surgery-induced stress response and inflammatory cytokines release that are considered to be closely associated with proliferation, invasion and metastasis of tumor cells. Propofol, sevoflurane, non-steroidal anti-inflammatory drugs and local anesthetics have shown to exert anti-tumor effect on HCC mainly through regulating microRNAs or signaling pathways, while other inhalational agents, dexmedetomidine and opioids have the potential to promote tumor growth. In terms of anesthetic methods and analgesia strategies, propofol based total intravenous anesthesia and thoracic epidural analgesia could be preferred for HCC patients undergoing open liver resection rather than inhalational anesthesia. Local anesthesia techniques have great potential to attenuate perioperative stress response, hence they may contribute to more favorable outcomes. This review summarized the relations between different anesthesia choices and HCC patients’ long-term outcomes as well as their underlying mechanisms. Due to the complexity of molecules interactions and signaling pathways, further studies are warranted to confirm these results so as to optimize anesthesia strategy for HCC patients.
Introduction
Anesthetic methods and agents can alter immune function and postoperative cancer recurrence (1, 2). There is a large body of literature suggesting the choice of anesthesia could exert either pro or anti-tumor effect on cancer patients (3). Growing evidence from animal and tumor cell line studies have suggested that volatile agents are more likely to enhance cancer progression through suppression of immune cell function and modulation of cancer cell signaling pathways (4–6). In contrast, propofol appears to suppress tumor growth and reduce the risk of migration via regulation of non-coding RNAs (e.g., microRNAs), signaling pathways and restoration of tumor microenvironment (7–9). Meanwhile, local anesthetics induce cancer cell apoptosis and reduce the methylation of tumor suppressor genes to hamper tumor cell proliferation (10, 11).
Hepatocellular carcinoma (HCC) is a lethal disease with poor prognosis and high recurrence rate (12). Although some noble therapies have constantly emerged in recent years, open liver resection has still been the safe choice for patients with risk factors, large tumors or with tumors located in the posterior segments of the liver (13). The postoperative analgesia approach for open hepatectomy seems to be less effective if the incision needs to be extended to the upper-level dermatomes of the abdomen. Insufficient analgesia can cause pulmonary dysfunction, especially with large incisions close to the diaphragm (14). In addition, postoperative coagulopathy is frequently seen following liver resection, hence the time to place and remove the epidural catheter should be within discretion of the anesthesia providers (15). Analgesic strategies for patients undergoing hepatectomy include epidural analgesia, peripheral nerve block (e.g., thoracic paravertebral block, quadratus lumborum block, transversus abdominis plane block, erector spinae plane block etc.) and local wound infiltration. Clinical evidence suggested the combination of epidural and general anesthesia could better attenuate immunosuppression related to stress response than general anesthesia and improve cancer patients’ outcomes (16).
This article reviewed the long-term effects (Table 1) and the relevant mechanisms (Table 2) of commonly used volatile, intravenous, local anesthetic agents and techniques as well as other anesthetic adjuncts (e.g. NSAIDS etc.) on HCC patients.
Volatile anesthetic agents
Inhalational anesthetics like isoflurane, desflurane, halothane have already been proved to reduce the number of natural killer (NK) cells or NK cell tumor cytotoxicity (4, 59), which are closely involved in cancer immunosurveillance and immunoediting (60). Isoflurane and sevoflurane also suppressed the release of interleukin-1β (IL-1β) and tumour necrosis factor α (TNF-α) stimulated by tumor cells in peripheral blood mononuclear cells (PBMCs), thus promoting tumorigenesis (61). A retrospective study indicated the inhalational anesthetics were associated with higher recurrence rate compared with propofol in HCC patients receiving laparoscopic hepatic resection (17).
Sevoflurane
The influence of sevoflurane on cancer is controversial since it has both anti-tumor and pro-tumor effects (62, 63). MicroRNA (miR)-29a, a potential prognostic biomarker, was down-regulated in HCC patients according to the previous studies (64). The exposure of sevoflurane could restore the level of miR-29a and inhibit tumor progression by lowering the expression of long non-coding RNA KCNQ1 opposite strand/antisense transcript 1 (lncRNA KCNQ1OT1) (26), which led to the reduction of DNA methyltransferase 3 alpha (Dnmt3a) (25) and chromebox protein homolog 3 (CBX3) (26) in HCC cell lines. In vitro studies also suggested that sevoflurane-stimulated tumor cells exhibited higher level of phosphatase and tensin homologue (PTEN) and inactivation of phosphatidylinositol 3 kinase (PI3K)/protein kinase B (Akt) signaling pathway (25, 27), a crucial signaling in tumorigenesis (65). Also, in both cell lines and animal models experiments, researchers observed a correlation of increased level of miR-148-3p and decreased Rho-associated protein kinase 1 (ROCK1) after sevoflurane treatment (28), which might account for its antitumor property.
In order to further explore the long term cancer-related outcomes sevoflurane could exert, researchers compared the overall mortality and recurrence-free survival rates of HCC patients in a retrospective cohort, and concluded that there was no difference between propofol based total intravenous anesthesia (TIVA) and sevoflurane anesthesia (18). A meta-analysis study also adequately evidenced that the use of sevoflurane might not exert any unfavorable effect on cancer patients’ overall survival (66). However, recently a study on HCC patients with portal vein tumor thrombus (PVTT) showed that sevoflurane based inhalation anesthesia was related to worse clinical outcomes comparing TIVA (21). Unlike other clincal trials, patients in this cohort had larger tumor burden (87% >10cm), and the distinction between TIVA and sevoflurane anesthesia was amplified by the severity of the disease according to its subgroup analysis.
Isoflurane
In Hep-3B cell line, short-term isoflurane exposure (<12h) might facilitate cancer cell invasion by enhancing the formation of small ubiquitin-like modifier 2 and 3 (SUMO2/3) conjugates, a kind of stress indicators, while the inhibitor of SUMO2/3 could reverse the response (29). But in primary cultured cells from patients undergoing hepatectomy and general anesthesia, evidence indicated that isoflurane could reduce HCC aggressiveness via down-regulating PI3K/AKT signaling pathway mediated nuclear factor kappaB (NF-κB) activity (30). These conflicts could be attributed to the different cell lines and distinctive time of isoflurane exposure (12h versus 24-72h).
Desflurane
Studies showed that desflurane did not affect NK cell counts and was in favor of preserving the ratio of CD4+/CD8+ T cells in breast cancer surgery (67, 68). However, most related studies considered desflurane to be associated with worse outcomes (3, 69). As for HCC patients, clinical evidence showed that the use of desflurane might lead to lower overall survival and higher recurrence rates compared with propofol (19).
To draw a conclusion from the available laboratory and clinical studies, among volatile anesthetic agents, sevoflurane showed anti-tumor effects in most cases, desflurane was more likely to exhibit pro-tumor effects, while isoflurane presented a paradoxical effect on different tumor cell lines. Other inhalational anesthetic agents were seldom studied regarding their long-term effects on HCC patients.
Intravenous anesthetic agents
Propofol
There is increasing evidence suggesting that propofol can inhibit proliferation, metastasis, and induce apoptosis of malignant tumors, thereby influencing the prognosis of cancer patients (7, 31). As of today, the underlying mechanism of its anti-tumor effect has not been fully understood, but it may relate to the regulation of non-coding RNAs (e.g., miRNAs, lnc RNAs), alteration of signaling pathways, as well as restoration of microenvironment.
Propofol can modulate miRNAs expressions and exert inhibitory effect on many carcinoma-crucial pathways linked to proliferation, migration, and apoptosis (7). An in vitro study showed that propofol could up-regulate miR-199a to inhibit the adhesion of HCC via downregulating metaloproteinases-9 (MMP-9) expression, an enzyme that could degrade extracellular matrix (ECM) proteins and mediate cancer metastasis (31). Also, activation of caspase-8 and caspase-9 mediated by increased level of miR-199a might be the underlying mechanism accounting for propofol-induced HCC cell apoptosis (32). Similarly, propofol appeared to up-regulate miR-134 expression, inhibit B cell lymphoma-2 (BCL-2) expression level, and elevate cleaved caspase-3 level, thereby inducing apoptosis as well (33). Propofol also increased the expression of miR-219-5p, a tumor suppressor candidate, which directly inhibited the glypican-3 mediated activation of Wnt/β-catenin pathway, and subsequently regulating the progression, metastasis and epithelial-mesenchymal transition (EMT) of HCC (38). LncRNAs are mRNA-like transcripts ranging from 200 nucleotides to 100 kilobases. It has been proved that some lncRNAs can modulate the expression of miRNAs so that they act as an axis and can be influenced by propofol at any level. For example, propofol could inhibit HCC progression by increasing the level of miR-4458 via modulating HOMEOBOX A11 antisense RNA (HOXA11-AS)/miR-4458 (39) axis as well as reducing HCC growth and metastasis through lncRNA H19/miR-520a-3p/LIM domain kinase 1 (LIMK1) axis (40). Apart from that, miR-556-3p played an essential role in propofol regulated non-coding RNA activated by DNA damage (NORAD)/miR-556-3p/migration and invasion enhancer 1 (MIEN1) axis, which involved in suppressing HCC cell proliferation and EMT progression (34). In addition, propofol increased the expression of miR-105, a potential JAK2/STAT3 signaling inhibitor, serving as a key mediator in HCC apoptosis process (35).
Evidence shows that some signaling pathways may play critical roles in liver cancer progression, and researchers have explored how propofol regulates tumor behaviors through these signaling pathways. Extracellular signal-regulated kinase 1/2 (ERK1/2) are protein-serine/threonine kinases that participate in the Ras-Raf-MEK-ERK signal transduction cascade, which are activated in one third of human cancers (70). A pathological analysis of HCC samplings showed that high p-ERK1/2 levels might be associated with elevated HCC recurrence and worse overall survival (71). In Fei G et al’s study, the levels of p-ERK1/2 were decreased in HCC cell lines treated with propofol, leading to less aggressive growth and invasion behaviors (8). Down-regulation of p-ERK1/2 might be caused by neuroepithelial cell transforming gene 1 (NET1), a new member of the tetraspanins group and a therapeutic potential target for cancer (72). High mobility group A2 (HMGA2) is an architectural transcriptional regulator that could independently predict the prognosis of HCC patients (73). And in Ou W et al’s study, propofol decreased the level of HMGA2, and subsequently attenuated the activity of Wnt/β-catenin pathway, thereby inducing apoptosis and inhibiting HCC proliferation as well as invasion (36). In fact, signaling pathways like nuclear factor E2-related factor-2 (Nrf2), NF-κB are well-illustrated in the relations between propofol and other kinds of cancers. However, as of yet there is little literature about the direct link between these pathways and the effect of propofol on HCC biological behaviors till now.
The tumor microenvironment (TME) is a mixture of tumoral cells within the ECM, surrounded by a complex mix of stromal cells and the proteins they secrete. The biological processes involved in TME include angiogenesis, inflammation, fibrosis and other processes like hypoxia, oxidative stress and autophagy (Figure 1) (74). Propofol can affect TME in different ways. Vascular endothelial growth factor (VEGF), the most important factor in angiogenesis, could be inhibited by propofol in HCC patients via down-regulating NET1 (8). An experiment examined oxidative stress caused by H2O2, and found that in contrast with pentobarbital, propofol exerted a protective effect on hepatocytes under oxidative stress (75), it is also the case for what had discovered before when comparing propofol to etomidate (76). There was a meta-analysis found that propofol might also have a beneficial impact on HCC patients after liver ischemia reperfusion injury (LIRI), demonstrated by a lower level of malondialdehyde (MDA), alanine transaminase (ALT), aspartate transaminase (AST) and a higher level of superoxide dismutase (SOD) (77). Propofol could also lead to down-regulation of MMPs and increase of E-cadherin by impairing Twist1 expression, and inhibit HCC proliferation, migration, and invasion as a result (37).
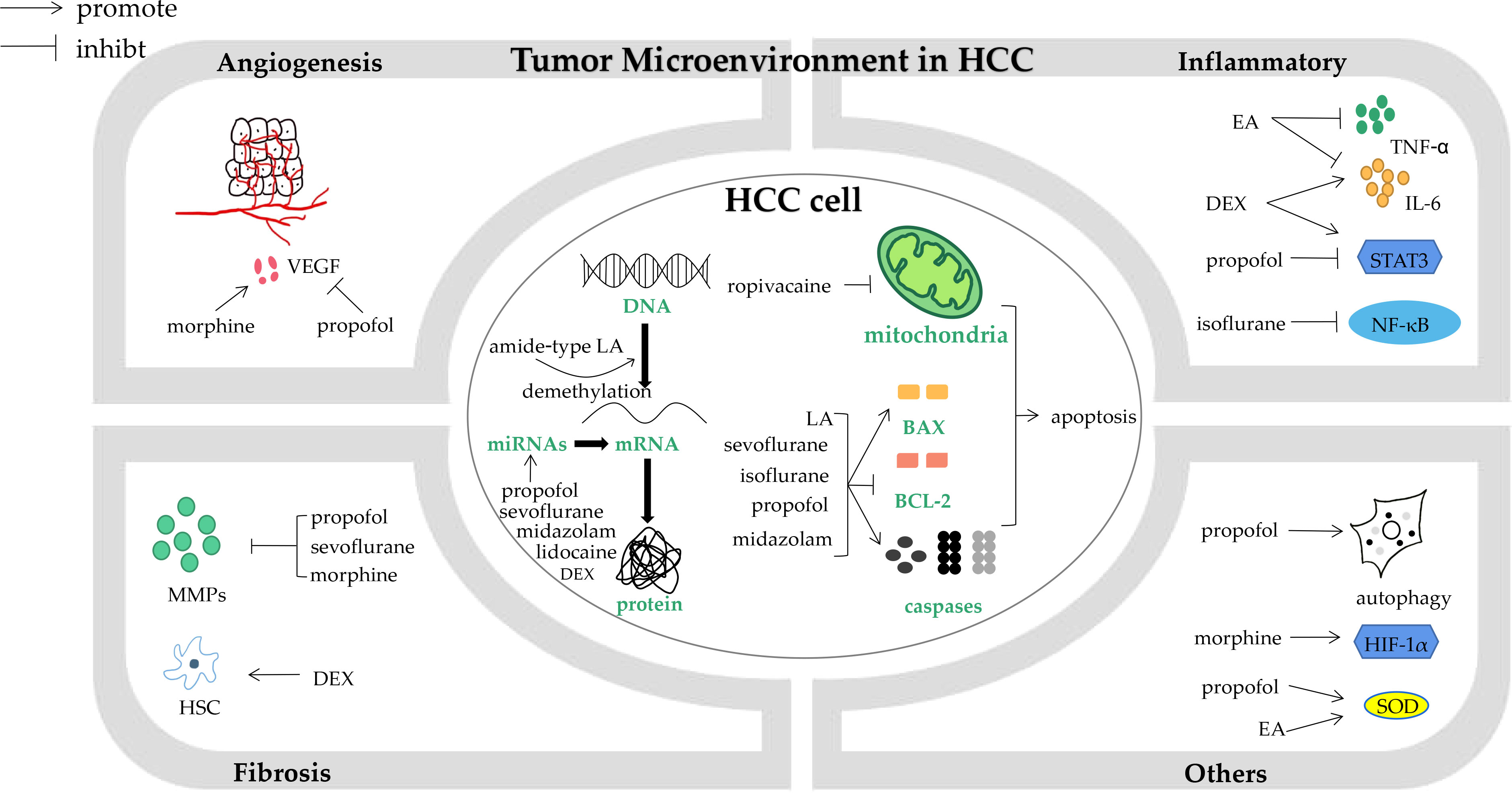
Figure 1 The impact of anesthesia agents and techniques on biological processes in hepatocellular carcinoma cells and tumor microenvironment. HCC, hepatocellular carcinoma; VEGF, vascular endothelial growth factor; MMPs, metaloproteinases; HSC, hepatic stellate cells; DEX, dexmedetomidine; LA, local anesthetics; miRNAs, microRNAs; BCL-2, B cell lymphoma-2; EA, epidural analgesia; TNF-α, tumour necrosis factor α; IL-6, interleukin-6; NF-κB, nuclear factor κB; HIF-1α, hypoxia-inducible factor-1α; SOD, superoxide dismutase.
In vivo studies provide a holistic perspective of the effect propofol has on HCC. Studies had observed that propofol treatment significantly decreased the tumor volume and slowed proliferation in nude mice model (38), and even in a propofol dose-dependent manner (78). Besides, by constructing Over H19‐Propofol‐Huh7‐exo mouse model, researchers found the high level exsomal lncRNA H19 promote the growth and metastasis of HCC tumors, hence verifying propofol’s anti-tumor effect conversely (40). Also, in tumor-bearing mice models, researchers found that propofol activated tumor-associated macrophages (TAMs) were able to secrete microvesicles (MVs), which delivered extrinsic miR-142-3p to HCC cells, thereby down-regulating ras-related C3 botulinum toxin substrate 1(RAC1) expression and inhibiting HCC invasion (41). In terms of tumor microenvironment, a mice model experiment proved that propofol could decrease levels of MMP-2 and VEGF in a dose-dependent manner (42), which reduced the chance of cancer metastasis. Studies in xenograft mice tumor models also showed autophagy, a catalytic process related to cancer initiation and progression, could be induced by propofol via activating adenosine monophosphate-activated protein kinase (AMPK) pathway (9).
Clinical evidence is also in correspondence with the above results. A retrospective study, which compared the recurrence of early- and intermediate-stage HCC patients after hepatic resection, revealed that the use of propofol as the main anesthetic agent might be superior to inhalational anesthetics in terms of recurrence rate (17). Similarly, Lai and his colleague also found the overall mortality and distant metastasis rate were higher in desflurane group than in propofol based TIVA group (19). Meanwhile, a nationwide retrospective cohort in Japan suggested the overall survival and recurrence-free survival rates were not significantly different between volatile anesthesia and propofol based TIVA groups of patients with digestive tract cancers including HCC (20), which was consistent with that of a Chinese cohort (18). Through combining existing clinical evidence, Chang et al. conducted a meta-analysis and concluded better overall survival and recurrence-free survival for patients with hepatobiliary cancer under propofol-based TIVA (66).
Dexmedetomidine
Dexmedetomidine (DEX), a centrally acting alpha-2 agonist, plays an essential role in perioperative period as it can provide safe sedation, reduce opioids consumption and stabilize hemodynamic status. Clinical evidence indicated that DEX’s central effect reduced the release of norepinephrine and thereby inhibiting inflammatory response. A meta-analysis showed the administration of DEX during perioperative period lowered the levels of catecholamine, cortisol, glucose, IL-6, TNF-α, and C reactive protein (CRP), whereas increased the expression of NK cells, B cells, CD4+T cells, and the ratios of CD4+/CD8+ and Th1/Th2 (79). This inflammatory attenuation may show great anti-tumor effect in liver cancer patients since the inflammatory process provides a pro-tumorigenesis microenvironment. An in vitro study in HCC indicated that DEX could suppress HCC cell proliferation and induce apoptosis by down-regulating miR-130a and inhibiting early growth response 1 (EGR1) expression (43). However, in a fibrosis mouse model experiment, it proved that DEX might not induce the pathogenesis of HCC cell itself. Instead, it promoted HCC progression by activating hepatic stellate cells via ARD2A-induced IL-6 secretion and STAT3 activation (44).
Etomidate
Etomidate is commonly used for elder patients and those with unstable hemodynamic status. Comparing with volatile anesthetics, etomidate shows less inhibition to NK cell-mediated cytotoxicity (59). The treatment of etomidate significantly mitigated lipopolysaccharide (LPS)-induced TNF-α and IL-6 levels and activation of NF-kB signaling pathway in macrophages (80). As for its effect on tumor, studies conducted in lung cancer illustrated that etomidate could curb cancer cell proliferation and invasion through different mechanisms (81, 82).
Ketamine
Ketamine is long believed to affect the immunoregulatory activities of immune cells. It inhibits the maturation of bone marrow-derived dendritic cells (BMDCs) and hampers the ability of dendritic cells (DCs) to prime a Th1-biased immune response (83). NK cell function is largely related to tumor recurrence and mortality (84). In a mouse model experiment using breast cancer cell line, ketamine reduced the number of NK cells and improved metastasis drastically compared with other anesthetics (4). However, Beilin B et al.’s results showed a small dose of ketamine before induction might prevent immune function from inflammatory cascade by inhibiting the release of IL-6 and TNF-α in early postoperative period (85). In recent years, ketamine has been proved not to change natural killer cell cytotoxicity in cancer patients and may even inhibit cancer cell growth (86–88).
Perioperative adjuvant medications
Opioids
Opioids, such as morphine, are widely used to alleviate pain perioperatively, which is generally thought to be immunosuppressive. Opioids exert restraining effect on innate immunity by suppressing NK cell cytotoxicity, inhibiting macrophage and neutrophil phagocytosis, and decreasing cytokine production (89). However, opioids also play an imperative role in relieving postoperative pain, which is a potential risk factor of tumor metastasis and morality (90).
Morphine, a generally studied opiate, has been shown to have both anti- and pro-metastasis potential in HCC. In an in vitro study, HCC cell lines pre-exposed to morphine did not increase the proliferation, instead they showed a less progressive ability of sphere formation and were prone to apoptosis via down-regulating MMP-9 and uPA, which are both ECM-degrading enzymes (45). In a nude mouse model, morphine also reduced lung colonization (45). Another study showed morphine could down-regulate DEAD-box helicase 49 (DDX49) expression and mitogen-activated protein kinases (MAPK) signaling to exert anti-tumor effect (46). On the contrary, morphine was also proved to promote angiogenesis by activating PI3K/Akt/HIF-1α pathway and increasing secretion of VEGFA in HCC (47). Sufentanil is also commonly used during perioperative period. A randomized animal study indicated that sufentanil-based postoperative analgesia could increase Th17 cells and FoxP3+ regulatory T (Treg) cells, but no difference in 5-year mortality was observed (91). A retrospective study pertaining to the choice of postoperative analgesia gave us information that the use of epidural analgesia with morphine might have risk of increasing cancer recurrence and death in contrast to intravenous analgesia with fentanyl (22). The heterogeneous nature of these studies can be attributed to different cell lines and morphine concentrations. Therefore, more studies with same standards are needed to clarify whether opioids could exert anti-tumor effect on HCC or not.
Non-steroidal anti-inflammatory drugs
Non-steroidal anti-inflammatory drugs (NSAIDs) inhibit cyclooxygenase (COX) enzymes, which can catalyze the synthesis of prostaglandins (PGs) in inflammatory processes. Clinical data analysis showed that the COX-2 expression in HCC tumor sample was closely associated with TNM stage, tumor size, lymphovascular invasion and distant metastasis, and 5-year survival rate (48). In vitro experiments suggested celecoxib treatment could up-regulate E-cadherin and inhibit COX-2-PGE2-PGE2 receptor 2 (EP2) -p-Akt/p-ERK pathway, and subsequently inhibit cell proliferation, induce apoptosis, and constrain migration and invasion (48). Recently, a study focused on the mechanisms other than COX-2 found that celecoxib’s anti-tumor effect on HCC might also involve partner of NOB1 (PNO1) and AKT/rapamycin (mTOR) signaling pathway (49). In an animal model, perioperative administration of COX-2 inhibitor could attenuate the inflammatory stress caused by surgery and reduce the risk of tumor metastasis (50). Parecoxib sodium combined with fentanyl for PCIA provided adequate analgesia, preserved immune function, and postponed HCC recurrence more favorably than fentanyl alone (23). Apart from its use for anesthesia, it has been proved by a meta-analysis that long-term NASIDs use could prevent the development of HCC (92), and reduce the recurrence rate of patients at risk after curative resection (93).
Benzodiazepines
Several meta-analysis articles suggested that the use of benzodiazepines might not be associated with cancer mortality (94, 95). But preclinical experiment in HCC cells showed that midazolam increased the level of miR-124-3p and consequently repressed the expression of PIM-1, a survival kinase, leading to cell cycle arrest and apoptosis (51). After treated with midazolam, HCC cell viability, migration were inhibited and apoptosis rated was enhanced in accordance with the increasing level of miR-217 (52).
Regional anesthesia
Since the regional nerve block techniques gain popularity in these years, the perioperative administration of local anesthetics (LA) has been widely employed, especially for cancer patients. Numerous studies and experiments have suggested that LA could improve postoperative rehabilitation and reduce cancer recurrence (96, 97).
Local anesthetics
The commonly used LA are amide-type, such as lidocaine, bupivacaine, and ropivacaine, which can impede the release of inflammatory mediators and down-regulate the activation of immune cells, thereby suppressing immune and inflammatory response (98). And the analgesic characteristic of LA presented an anti-tumor effect by inducing the consumption of opioids through effectively alleviating the pain of surgery and cancer (99). In addition to these indirect action, LA can exert inhibitory effect on tumor progression directly. It has been well accepted that LA can cause apoptosis in cancer cells. In HCC cell lines, Xing W et al.’s study had seen significantly increased apoptosis rate in cells treated with lidocaine. They also proved this might be the result of activation of ERK1/2 and p38, and then the activation increased cleaved caspase-3 and Bax levels and decreased BCL-2 level (10). The similar pro-apoptotic effect has been observed with bupivacaine as well (55). Another study validated ropivacaine’s effect on caspase-3, and proposed that ropivacaine could damage the structure of mitochondria and interfere its function, then lead to cell death (56). Furthermore, LA can also inhibit HCC cell proliferation, migration, invasion by regulation of signaling pathways. PI3K/AKT/mTOR signaling pathway is usually activated in HCC patients and closely related to the anti-tumor effect of LA (100). Lidocaine down-regulated PI3K/Akt pathway via the reduction of ubiquitin specific peptidase 14 (USP14) level, leading to lower-level proliferation and invasion capacity (54). Similarly, ropivacaine and bupivacaine both could suppress PI3K/Akt pathway and reduce tumor cell proliferation and metastasis (55, 57). Other pathways like MAPK can also be down-regulated with LA treatment and involved in HCC progression (55).
Transcriptional silencing of tumor suppressor genes through methylation of their promoters could lead to tumorigenesis (101). Tumor suppressive gene ras association domain family 1A (RASSF1A) has been shown to be silenced by promoter methylation in HCC according to previous findings (102). Dongtai Chen and his colleagues evaluated methylation levels of RASSF1A in HCC cell lines treated by amide-type LA and discovered a decreased level of methylation and increased expression of RASSF1A both in mRNA and protein levels, followed by reduced cell proliferation (11). This demethylating effect of LA on hepatoma cells was firstly discovered in 2007 in a study concerning procaine, an esters-type agent (58). In addition, the increased level of cytoplasmic polyadenylation element binding protein 3 (CPEB3) induced by lidocaine might also be a part of LA’s anti-tumor mechanisms, which could be regulated by circ_ITCH/miR-421/CPEB3 axis (53).
Local anesthesia techniques
Thoracic epidural block (TEA) is a historically classic pain-relief method, which was strongly recommended by American Pain Society (103). TEA combined with general anesthesia (GA) could provide elder HCC patients with better cognitive status, less release of inflammatory factors, such as IL-6, IL-1, TNF-α, and lower stress response than GA alone (104, 105). Application of TEA was associated with less activation of hypothalamic-pituitary-adrenal (HPA) axis, better preservation of the Th1/Th2 balance and maintenance of CD3+, CD4+, and CD4+/CD8+ T cells, indicating an immune protective effect on HCC patients under surgery (106–108).
Clinical trials have been conducted to clarify whether TEA combined with GA could exert anti-tumor effect on HCC patients during surgery. A retrospective cohort recruiting 772 patients undergoing selective curative resection surgery suggested that GA combining with TEA showed higher 3-year overall survival and disease-free survival rates, rendering GA solely as the independent predictors of poor survival and high risk of recurrence, respectively (16). But another single-center study using electronic medical records found that TEA did not have a definite relation with the recurrence-free survival and overall survival of HCC patients (24). These two retrospective cohorts were based on patients with different baseline characteristics. Patients in the latter were with higher AFP level, larger tumor size, and longer anesthesia time, and the follow-up time was longer than the former. Therefore, with the intrinsic retrospective limitations, it is a call for future randomized controlled trial to be conducted.
Other regional anesthesia techniques like transverse abdominal plane block (TAPB), thoracic paravertebral blocks (TPVBs), quadratus lumborum block (QLB), erector spinae plane block (ESPB) and local wound infiltration have been reported to be used as analgesic methods for HCC patients undergoing open liver resection, but there is devoid of evidence concerning the relationship between these techniques and long-term prognosis. An analysis of ACS NSQIP database regarding the one-month mortality and overall morbidity rates of patients under hepatectomy revealed that the use of regional abdominal wall nerve block (mostly TAPB) was associated with better short-term morbidity like shorter duration of hospital stay compared with TEA, while there was no significant difference pertaining to overall morality rates (109).
Conclusions
New techniques, drugs and regimens are constantly explored and tentatively conducted in clinical practices, especially for cancer patients. The perioperative factors linked with recurrence are the circulating tumor cells and minimal residual disease. Therefore, it is pivotal for us anesthesiologists to maintain the homeostasis and preserve patients’ immunity in this period. Despite there is no conclusive evidence to provide a clear protocol, there are some beneficial actions we can take. Firstly, propofol-based TIVA should be routinely used if possible for HCC patients undergoing open hepatectomy, if not, sevoflurane-based inhalational anesthesia ought to be the alternative. Next, local anesthesia agents and techniques are recommended to implement, especially for patients contraindicated to epidural anesthesia. Because local anesthesia shows great superiority in postoperative analgesia and attenuation of inflammatory response. Hence, the ability to skillful use the ultrasound should be added to the anesthesia education system. Furthermore, in accordance with enhanced recovery after surgery (ERAS) program, the effective multimodal analgesic methods including opioids and NSAIDS are indispensable. Undoubtedly, a clear anesthesia protocol for HCC patients far from well established, more prospective RCTs and multicenter studies on cancer recurrence are warranted for more scientific and convinced results.
Author contributions
RZ and XX wrote the manuscript. LS and GZ revised and corrected the manuscript. All authors read and approved the final manuscript.
Funding
This work was funded by Sanming Project of Medicine in Shenzhen(No.SZSM201812069)
Acknowledgments
We thank our colleagues at the Department of Anesthesiology, National Cancer Center/National Clinical Research Center for Cancer/Cancer Hospital, Chinese Academy of Medical Sciences and Peking Union Medical College, Beijing, 100021, China.
Conflict of interest
The authors declare that the research was conducted in the absence of any commercial or financial relationships that could be construed as a potential conflict of interest.
Publisher’s note
All claims expressed in this article are solely those of the authors and do not necessarily represent those of their affiliated organizations, or those of the publisher, the editors and the reviewers. Any product that may be evaluated in this article, or claim that may be made by its manufacturer, is not guaranteed or endorsed by the publisher.
References
1. Ackerman RS, Luddy KA, Icard BE, Pineiro FJ, Gatenby RA, Muncey AR. The effects of anesthetics and perioperative medications on immune function: A narrative review. Anesth ANALG (2021) 133(3):676–89. doi: 10.1213/ANE.0000000000005607
2. Snyder GL, Greenberg S. Effect of anaesthetic technique and other perioperative factors on cancer recurrence. Br J Anaesth (2010) 105(2):106–15. doi: 10.1093/bja/aeq164
3. Wu ZF, Lee MS, Wong CS, Lu CH, Huang YS, Lin KT, et al. Propofol-based total intravenous anesthesia is associated with better survival than desflurane anesthesia in colon cancer surgery. ANESTHESIOLOGY (2018) 129(5):932–41. doi: 10.1097/ALN.0000000000002357
4. Melamed R, Bar-Yosef S, Shakhar G, Shakhar K, Ben-Eliyahu S. Suppression of natural killer cell activity and promotion of tumor metastasis by ketamine, thiopental, and halothane, but not by propofol: mediating mechanisms and prophylactic measures. Anesth ANALG (2003) 97(5):1331–9. doi: 10.1213/01.ANE.0000082995.44040.07
5. Benzonana LL, Perry NJ, Watts HR, Yang B, Perry IA, Coombes C, et al. Isoflurane, a commonly used volatile anesthetic, enhances renal cancer growth and malignant potential via the hypoxia-inducible factor cellular signaling pathway in vitro. ANESTHESIOLOGY (2013) 119(3):593–605. doi: 10.1097/ALN.0b013e31829e47fd
6. Huang H, Benzonana LL, Zhao H, Watts HR, Perry NJ, Bevan C, et al. Prostate cancer cell malignancy via modulation of HIF-1alpha pathway with isoflurane and propofol alone and in combination. Br J Cancer (2014) 111(7):1338–49. doi: 10.1038/bjc.2014.426
7. Buschmann D, Brandes F, Lindemann A, Maerte M, Ganschow P, Chouker A, et al. Propofol and sevoflurane differentially impact MicroRNAs in circulating extracellular vesicles during colorectal cancer resection: A pilot study. ANESTHESIOLOGY (2020) 132(1):107–20. doi: 10.1097/ALN.0000000000002986
8. Fei G, Cao M, Ge C, Cui L. Propofol suppresses hepatocellular carcinoma by inhibiting NET1 through downregulating ERK/VEGF signaling pathway. Sci Rep (2020) 10(1):11208. doi: 10.1038/s41598-020-67693-0
9. Wang Y, Xu B, Zhou J, Wu X. Propofol activates AMPK to inhibit the growth of HepG2 cells in vitro and hepatocarcinogenesis in xenograft mouse tumor models by inducing autophagy. J Gastrointest Oncol (2020) 11(6):1322–32. doi: 10.21037/jgo-20-472
10. Xing W, Chen DT, Pan JH, Chen YH, Yan Y, Li Q, et al. Lidocaine induces apoptosis and suppresses tumor growth in human hepatocellular carcinoma cells In vitro and in a xenograft model In vivo. ANESTHESIOLOGY (2017) 126(5):868–81. doi: 10.1097/ALN.0000000000001528
11. Chen D, Yan Y, Xie J, Pan J, Chen Y, Li Q, et al. Amide-type local anesthetics may suppress tumor cell proliferation and sensitize human hepatocellular carcinoma cells to cisplatin via upregulation of RASSF1A expression and demethylation. J Cancer (2020) 11(24):7312–9. doi: 10.7150/jca.46630
12. Ghouri YA, Mian I, Rowe JH. Review of hepatocellular carcinoma: Epidemiology, etiology, and carcinogenesis. J Carcinog (2017) 16:1. doi: 10.4103/jcar.JCar_9_16
13. Shin H, Cho JY, Han HS, Yoon YS, Lee HW, Lee JS, et al. Risk factors and long-term implications of unplanned conversion during laparoscopic liver resection for hepatocellular carcinoma located in anterolateral liver segments. J Minim Invasive Surg (2021) 24(4):191–9. doi: 10.7602/jmis.2021.24.4.191
14. Ganapathi S, Roberts G, Mogford S, Bahlmann B, Ateleanu B, Kumar N. Epidural analgesia provides effective pain relief in patients undergoing open liver surgery. Br J Pain (2015) 9(2):78–85. doi: 10.1177/2049463714525140
15. Singh SA, Vivekananthan P, Sharma A, Sharma S, Bharathy KG. Retrospective analysis of post-operative coagulopathy after major hepatic resection at a tertiary care centre in northern India. Indian J Anaesth (2017) 61(7):575–80. doi: 10.4103/ija.IJA_734_16
16. Zhang H, Guo K, Sun X, Liu Y, Zhong Z, Zhou W, et al. Impact of anesthesia methods on perioperative systemic inflammation and long-term outcomes in patients undergoing surgery for hepatocellular carcinoma: a propensity score-matched analysis. Ann Transl Med (2021) 9(1):49. doi: 10.21037/atm-20-3704
17. Koo BW, Lim DJ, Oh AY, Na HS. Retrospective comparison between the effects of propofol and inhalation anesthetics on postoperative recurrence of early- and intermediate-stage hepatocellular carcinoma. Med Princ Pract (2020) 29(5):422–8. doi: 10.1159/000506637
18. Yan T, Zhao JJ, Bi XY, Cai JQ, Zheng H. [Effect of different anesthetic techniques on the prognosis of hepatocellular carcinoma after hepatectomy]. Zhonghua Yi Xue Za Zhi (2017) 97(22):1719–23. doi: 10.3760/cma.j.issn.0376-2491.2017.22.009
19. Lai HC, Lee MS, Lin C, Lin KT, Huang YH, Wong CS, et al. Propofol-based total intravenous anaesthesia is associated with better survival than desflurane anaesthesia in hepatectomy for hepatocellular carcinoma: a retrospective cohort study. Br J Anaesth (2019) 123(2):151–60. doi: 10.1016/j.bja.2019.04.057
20. Makito K, Matsui H, Fushimi K, Yasunaga H. Volatile versus total intravenous anesthesia for cancer prognosis in patients having digestive cancer surgery. ANESTHESIOLOGY (2020) 133(4):764–73. doi: 10.1097/ALN.0000000000003440
21. Meng XY, Zhang XP, Sun Z, Wang HQ, Yu WF. Distant survival for patients undergoing surgery using volatile versus IV anesthesia for hepatocellular carcinoma with portal vein tumor thrombus: a retrospective study. BMC ANESTHESIOL (2020) 20(1):233. doi: 10.1186/s12871-020-01111-w
22. Cao L, Chang Y, Lin W, Zhou J, Tan H, Yuan Y, et al. Long-term survival after resection of hepatocelluar carcinoma: a potential risk associated with the choice of postoperative analgesia. Anesth ANALG (2014) 118(6):1309–16. doi: 10.1213/ANE.0000000000000207
23. Wang RD, Zhu JY, Zhu Y, Ge YS, Xu GL, Jia WD. Perioperative analgesia with parecoxib sodium improves postoperative pain and immune function in patients undergoing hepatectomy for hepatocellular carcinoma. J Eval Clin Pract (2020) 26(3):992–1000. doi: 10.1111/jep.13256
24. Chang WK, Lee MY, Tai YH, Kuo YM, Tsou MY, Chang KY. Does epidural analgesia improve the cancer outcome in hepatocellular carcinoma after resection surgery? a retrospective analysis. J Chin Med Assoc (2019) 82(4):295–299. doi: 10.1097/JCMA.0000000000000054
25. Song G, Tian L, Cheng Y, Liu J, Wang K, Li S, et al. Antitumor activity of sevoflurane in HCC cell line is mediated by miR-29a-induced suppression of Dnmt3a. J Cell Biochem (2019) 120(10):18152–61. doi: 10.1002/jcb.29121
26. Zhou W, Li H, Shang S, Liu F. lncRNA KCNQ1OT1 reverses the effect of sevoflurane on hepatocellular carcinoma progression via regulating the miR-29a-3p/CBX3 axis. Braz J Med Biol Res (2021) 54(7):e10213. doi: 10.1590/1414-431x2020e10213
27. Cao Y, Lv W, Ding W, Li J. Sevoflurane inhibits the proliferation and invasion of hepatocellular carcinoma cells through regulating the PTEN/Akt/GSK3beta/betacatenin signaling pathway by downregulating miR253p. Int J Mol Med (2020) 46(1):97–106. doi: 10.3892/ijmm.2020.4577
28. Sun Y, Liu L, Xing W, Sun H. microRNA-148a-3p enhances the effects of sevoflurane on hepatocellular carcinoma cell progression via ROCK1 repression. Cell Signal (2021) 83:109982. doi: 10.1016/j.cellsig.2021.109982
29. Wang P, Xue N, Zhang C, Shan S, Jiang Z, Wu W, et al. Inhibition of SUMO2/3 antagonizes isoflurane-induced cancer-promoting effect in hepatocellular carcinoma Hep3B cells. Oncol Lett (2021) 21(4):274. doi: 10.3892/ol.2021.12535
30. Hu J, Hu J, Jiao H, Li Q. Anesthetic effects of isoflurane and the molecular mechanism underlying isofluraneinhibited aggressiveness of hepatic carcinoma. Mol Med Rep (2018) 18(1):184–92. doi: 10.3892/mmr.2018.8945
31. Zhang J, Zhang D, Wu GQ, Feng ZY, Zhu SM. Propofol inhibits the adhesion of hepatocellular carcinoma cells by upregulating microRNA-199a and downregulating MMP-9 expression. Hepatob Pancreat Dis Int (2013) 12(3):305–9. doi: 10.1016/S1499-3872(13)60048-X
32. Zhang J, Wu GQ, Zhang Y, Feng ZY, Zhu SM. Propofol induces apoptosis of hepatocellular carcinoma cells by upregulation of microRNA-199a expression. Cell Biol Int (2013) 37(3):227–32. doi: 10.1002/cbin.10034
33. Hu X, Hu X, Wang Q. Propofol induces apoptosis of hepatocellular carcinoma cells by upregulating miR-134 expression. Transl Cancer Res (2021) 10(6):3004–12. doi: 10.21037/tcr-21-830
34. Liu Y, Wang X, Li H, Guan E, Luo K. Propofol ameliorates the proliferation and epithelial-mesenchymal transition of hepatoma carcinoma cells via non-coding RNA activated by DNA damage (NORAD)/microRNA (miR)-556-3p/Migration and invasion enhancer 1 (MIEN1) axis. J Environ Pathol Toxicol Oncol (2021) 40(4):87–97. doi: 10.1615/JEnvironPatholToxicolOncol.2021039471
35. Li J, Liu M, Zeng B, Wang Z. Propofol induces hepatocellular carcinoma cell apoptosis via regulating miR-105/JAK2/STAT3 axis. CYTOKINE (2021) 148:155649. doi: 10.1016/j.cyto.2021.155649
36. Ou W, Lv J, Zou X, Yao Y, Wu J, Yang J, et al. Propofol inhibits hepatocellular carcinoma growth and invasion through the HMGA2-mediated wnt/beta-catenin pathway. Exp Ther Med (2017) 13(5):2501–6. doi: 10.3892/etm.2017.4253
37. Zheng H, Fu Y, Yang T. Propofol inhibits proliferation, migration, and invasion of hepatocellular carcinoma cells by downregulating twist. J Cell Biochem (2019) 120(8):12803–9. doi: 10.1002/jcb.28551
38. Gong T, Ning X, Deng Z, Liu M, Zhou B, Chen X, et al. Propofol-induced miR-219-5p inhibits growth and invasion of hepatocellular carcinoma through suppression of GPC3-mediated wnt/beta-catenin signalling activation. J Cell Biochem (2019) 120(10):16934–45. doi: 10.1002/jcb.28952
39. Song F, Liu J, Feng Y, Jin Y. Propofol−induced HOXA11−AS promotes proliferation, migration and invasion, but inhibits apoptosis in hepatocellular carcinoma cells by targeting miR−4458. Int J Mol Med (2020) 46(3):1135–45. doi: 10.3892/ijmm.2020.4667
40. Wang D, Xing N, Yang T, Liu J, Zhao H, He J, et al. Exosomal lncRNA H19 promotes the progression of hepatocellular carcinoma treated with propofol via miR-520a-3p/LIMK1 axis. Cancer Med (2020) 9(19):7218–30. doi: 10.1002/cam4.3313
41. Zhang J, Shan WF, Jin TT, Wu GQ, Xiong XX, Jin HY, et al. Propofol exerts anti-hepatocellular carcinoma by microvesicle-mediated transfer of miR-142-3p from macrophage to cancer cells. J Transl Med (2014) 12:279. doi: 10.1186/s12967-014-0279-x
42. Liu Y, Zhang N, Cao Q, Cui X, Zhou Q, Yang C. The effects of propofol on the growth behavior of hepatoma xenografts in balb/c mice. BioMed Pharmacother (2017) 90:47–52. doi: 10.1016/j.biopha.2017.03.041
43. Zhou L, Li J, Liu X, Tang Y, Li T, Deng H, et al. Dexmedetomidine promotes apoptosis and suppresses proliferation of hepatocellular carcinoma cells via microRNA-130a/EGR1 axis. Cell Death Discovery (2022) 8(1):31. doi: 10.1038/s41420-021-00805-5
44. Chen P, Luo X, Dai G, Jiang Y, Luo Y, Peng S, et al. Dexmedetomidine promotes the progression of hepatocellular carcinoma through hepatic stellate cell activation. Exp Mol Med (2020) 52(7):1062–74. doi: 10.1038/s12276-020-0461-6
45. Zhang HW, Wang F, Zhou YQ, Xu SP, Yu SY, Zhang ZG. Morphine suppresses liver cancer cell tumor properties In vitro and In vivo. FRONT ONCOL. Frontiers in oncology (2021) 11:666446. doi: 10.3389/fonc.2021.666446
46. Dai H, Feng J, Nan Z, Wei L, Lin F, Jin R, et al. Morphine may act via DDX49 to inhibit hepatocellular carcinoma cell growth. Aging (Albany NY) (2021) 13(9):12766–79. doi: 10.18632/aging.202946
47. Wang Z, Jiang L, Wang J, Chai Z, Xiong W. Morphine promotes angiogenesis by activating PI3K/Akt/HIF-1alpha pathway and upregulating VEGF in hepatocellular carcinoma. J Gastrointest Oncol (2021) 12(4):1761–72. doi: 10.21037/jgo-20-394
48. Tai Y, Zhang LH, Gao JH, Zhao C, Tong H, Ye C, et al. Suppressing growth and invasion of human hepatocellular carcinoma cells by celecoxib through inhibition of cyclooxygenase-2. Cancer MANAG Res (2019) 11:2831–48. doi: 10.2147/CMAR.S183376
49. Dai H, Zhang S, Ma R, Pan L. Celecoxib inhibits hepatocellular carcinoma cell growth and migration by targeting PNO1. Med Sci Monit (2019) 25:7351–60. doi: 10.12659/MSM.919218
50. Benish M, Bartal I, Goldfarb Y, Levi B, Avraham R, Raz A, et al. Perioperative use of beta-blockers and COX-2 inhibitors may improve immune competence and reduce the risk of tumor metastasis. ANN SURG ONCOL. Annals of surgical oncology (2008) 15(7):2042–52. doi: 10.1245/s10434-008-9890-5
51. Qi Y, Yao X, Du X. Midazolam inhibits proliferation and accelerates apoptosis of hepatocellular carcinoma cells by elevating microRNA-124-3p and suppressing PIM-1. IUBMB Life (2020) 72(3):452–64. doi: 10.1002/iub.2171
52. Shen Q, Xia Y, Yang L, Wang B, Peng J. Midazolam suppresses hepatocellular carcinoma cell metastasis and enhances apoptosis by elevating miR-217. Comput Math Methods Med (2022) 2022:2813521. doi: 10.1155/2022/2813521
53. Zhao L, Ma N, Liu G, Mao N, Chen F, Li J. Lidocaine inhibits hepatocellular carcinoma development by modulating circ_ITCH/miR-421/CPEB3 axis. Dig Dis Sci (2021) 66(12):4384–97. doi: 10.1007/s10620-020-06787-1
54. Zhang Y, Jia J, Jin W, Cao J, Fu T, Ma D, et al. Lidocaine inhibits the proliferation and invasion of hepatocellular carcinoma by downregulating USP14 induced PI3K/Akt pathway. Pathol Res Pract (2020) 216(8):152963. doi: 10.1016/j.prp.2020.152963
55. Wang L, Guo W, Guan H, Yan N, Cai X, Zhu L. Local anesthetic bupivacaine inhibits proliferation and metastasis of hepatocellular carcinoma cells via suppressing PI3K/Akt and MAPK signaling. J Biochem Mol Toxicol (2021) 35(10):e22871. doi: 10.1002/jbt.22871
56. Wang W, Zhu M, Xu Z, Li W, Dong X, Chen Y, et al. Ropivacaine promotes apoptosis of hepatocellular carcinoma cells through damaging mitochondria and activating caspase-3 activity. Biol Res (2019) 52(1):36. doi: 10.1186/s40659-019-0242-7
57. Zhang R, Lian Y, Xie K, Cai Y, Pan Y, Zhu Y. Ropivacaine suppresses tumor biological characteristics of human hepatocellular carcinoma via inhibiting IGF-1R/PI3K/AKT/mTOR signaling axis. BIOENGINEERED (2021) 12(2):9162–73. doi: 10.1080/21655979.2021.1995103
58. Tada M, Imazeki F, Fukai K, Sakamoto A, Arai M, Mikata R, et al. Procaine inhibits the proliferation and DNA methylation in human hepatoma cells. Hepatol Int (2007) 1(3):355–64. doi: 10.1007/s12072-007-9014-5
59. Tazawa K, Koutsogiannaki S, Chamberlain M, Yuki K. The effect of different anesthetics on tumor cytotoxicity by natural killer cells. Toxicol Lett (2017) 266:23–31. doi: 10.1016/j.toxlet.2016.12.007
60. Dunn GP, Old LJ, Schreiber RD. The immunobiology of cancer immunosurveillance and immunoediting. IMMUNITY (2004) 21(2):137–48. doi: 10.1016/j.immuni.2004.07.017
61. Mitsuhata H, Shimizu R, Yokoyama MM. Suppressive effects of volatile anesthetics on cytokine release in human peripheral blood mononuclear cells. Int J Immunopharmacol (1995) 17(6):529–34. doi: 10.1016/0192-0561(95)00026-X
62. Takeyama E, Miyo M, Matsumoto H, Tatsumi K, Amano E, Hirao M, et al. Long-term survival differences between sevoflurane and propofol use in general anesthesia for gynecologic cancer surgery. J Anesth (2021) 35(4):495–504. doi: 10.1007/s00540-021-02941-9
63. Liang H, Yang CX, Zhang B, Zhao ZL, Zhong JY, Wen XJ. Sevoflurane attenuates platelets activation of patients undergoing lung cancer surgery and suppresses platelets-induced invasion of lung cancer cells. J Clin Anesth (2016) 35:304–12. doi: 10.1016/j.jclinane.2016.08.008
64. Fouda MS, Omran MM, Tarek G, Hady AAWA. Development of a novel panel based on micro-RNAs (21, 29a, 200 and 335) and alpha-fetoprotein as diagnostic biomarkers for hepatocellular carcinoma associated with hepatitis c infection. ARAB J Gastroenterol (2021) 22(1):28–33. doi: 10.1016/j.ajg.2020.08.004
65. Haddadi N, Lin Y, Travis G, Simpson AM, Nassif NT, McGowan EM. PTEN/PTENP1: ‘Regulating the regulator of RTK-dependent PI3K/Akt signalling’, new targets for cancer therapy. Mol Cancer (2018) 17(1):37. doi: 10.1186/s12943-018-0803-3
66. Chang CY, Wu MY, Chien YJ, Su IM, Wang SC, Kao MC. Anesthesia and long-term oncological outcomes: A systematic review and meta-analysis. Anesth ANALG (2021) 132(3):623–34. doi: 10.1213/ANE.0000000000005237
67. Pirbudak CL, Ugur MG, Karadasli H. Comparison of effects of low-flow sevoflurane and desflurane anesthesia on neutrophil and T-cell populations. Curr Ther Res Clin Exp (2012) 73(1-2):41–51. doi: 10.1016/j.curtheres.2012.02.005
68. Woo JH, Baik HJ, Kim CH, Chung RK, Kim DY, Lee GY, et al. Effect of propofol and desflurane on immune cell populations in breast cancer patients: A randomized trial. J KOREAN Med Sci (2015) 30(10):1503–8. doi: 10.3346/jkms.2015.30.10.1503
69. Lai HC, Lee MS, Lin KT, Huang YH, Chen JY, Lin YT, et al. Propofol-based total intravenous anesthesia is associated with better survival than desflurane anesthesia in robot-assisted radical prostatectomy. PloS One (2020) 15(3):e230290. doi: 10.1371/journal.pone.0230290
70. Roskoski RJ. ERK1/2 MAP kinases: structure, function, and regulation. Pharmacol Res (2012) 66(2):105–43. doi: 10.1016/j.phrs.2012.04.005
71. Gao X, Shan W, Liu X, Zhang J, Zheng J, Yao H. JNK1/2 and ERK1/2 provides vital clues about tumor recurrence and survival in hepatocellular carcinoma patients. Future Oncol (2018) 14(24):2471–81. doi: 10.2217/fon-2018-0171
72. Chen C, Ji Q. Net1, a therapeutic potential target for cancer. Hum Pathol (2018) 71:168. doi: 10.1016/j.humpath.2017.07.018
73. Zhao YC, Jiao Y, Li YQ, Fu Z, Yang ZY, He M. Elevated high mobility group A2 expression in liver cancer predicts poor patient survival. Rev ESP ENFERM DIG (2020) 112(1):27–33. doi: 10.17235/reed.2019.6365/2019
74. Hernandez-Gea V, Toffanin S, Friedman SL, Llovet JM. Role of the microenvironment in the pathogenesis and treatment of hepatocellular carcinoma. GASTROENTEROLOGY (2013) 144(3):512–27. doi: 10.1053/j.gastro.2013.01.002
75. Lee JY, Shin JW, Lee EH, Baek SH, Ku SW, Kim JU. Comparison of the effects of propofol and pentobarbital on hydrogen peroxide-stimulated hepatic SNU761 cells. Korean J Anesthesiol (2010) 58(3):277–82. doi: 10.4097/kjae.2010.58.3.277
76. Lee EH, Shin JW, Yoon SK, Son HJ, Lee JY, Ku SW, et al. Effects of propofol and etomidate on hydrogen peroxide-induced oxidative damage in hepatocyte. Korean J Anesthesiol (2009) 57(3):331–6. doi: 10.4097/kjae.2009.57.3.331
77. Feng C, Qian D, Chen C. A meta-analysis and systematic review of propofol on liver ischemia-reperfusion injury protection during hepatocellular carcinoma anesthesia surgery. Ann Palliat Med (2021) 10(6):6726–35. doi: 10.21037/apm-21-1242
78. Song F, Liu J, Feng Y, Jin Y. Propofolinduced HOXA11AS promotes proliferation, migration and invasion, but inhibits apoptosis in hepatocellular carcinoma cells by targeting miR4458. Int J Mol Med (2020) 46(3):1135–45. doi: 10.3892/ijmm.2020.4667
79. Wang K, Wu M, Xu J, Wu C, Zhang B, Wang G, et al. Effects of dexmedetomidine on perioperative stress, inflammation, and immune function: systematic review and meta-analysis. Br J Anaesth (2019) 123(6):777–94. doi: 10.1016/j.bja.2019.07.027
80. Liu M, Zhang Y, Xiong JY, Wang Y, Lv S. Etomidate mitigates lipopolysaccharide-induced CD14 and TREM-1 expression, NF-kappaB activation, and pro-inflammatory cytokine production in rat macrophages. INFLAMMATION (2016) 39(1):327–35. doi: 10.1007/s10753-015-0253-7
81. Li D, Zhang J, Yin L, Jin Z, Chen X, Meng X. Etomidate inhibits cell proliferation and induces apoptosis in A549 non-small cell lung cancer cells via downregulating WWP2. Exp Ther Med (2021) 22(5):1254. doi: 10.3892/etm.2021.10689
82. Chu CN, Wu KC, Chung WS, Zheng LC, Juan TK, Hsiao YT, et al. Etomidate suppresses invasion and migration of human A549 lung adenocarcinoma cells. Anticancer Res (2019) 39(1):215–23. doi: 10.21873/anticanres.13100
83. Ohta N, Ohashi Y, Fujino Y. Ketamine inhibits maturation of bone marrow-derived dendritic cells and priming of the Th1-type immune response. Anesth ANALG (2009) 109(3):793–800. doi: 10.1213/ane.0b013e3181adc384
84. Fujisawa T, Yamaguchi Y. Autologous tumor killing activity as a prognostic factor in primary resected nonsmall cell carcinoma of the lung. CANCER-AM Cancer Soc (1997) 79(3):474–81. doi: 10.1002/(SICI)1097-0142(19970201)79:3<474::AID-CNCR8>3.0.CO;2-I
85. Beilin B, Rusabrov Y, Shapira Y, Roytblat L, Greemberg L, Yardeni IZ, et al. Low-dose ketamine affects immune responses in humans during the early postoperative period. Br J Anaesth (2007) 99(4):522–7. doi: 10.1093/bja/aem218
86. Li T, Yang J, Yang B, Zhao G, Lin H, Liu Q, et al. Ketamine inhibits ovarian cancer cell growth by regulating the lncRNA-PVT1/EZH2/p57 axis. Front Genet (2020) 11:597467. doi: 10.3389/fgene.2020.597467
87. Kubota M, Niwa H, Seya K, Kawaguchi J, Kushikata T, Hirota K. Ketamine does not change natural killer cell cytotoxicity in patients undergoing cancer surgery: Basic experiment and clinical trial. J Oncol (2022) 2022:8946269. doi: 10.1155/2022/8946269
88. Kawaguchi J, Ota D, Niwa H, Sugo Y, Kushikata T, Hirota K. Immunomodulation by ketamine as an adjunct to total intravenous anesthesia in patients undergoing minimally invasive radical prostatectomy: A randomized pilot trial. Mol Clin Oncol (2020) 13(2):203–8. doi: 10.3892/mco.2020.2060
89. Eisenstein TK. The role of opioid receptors in immune system function. Front Immunol (2019) 10:2904. doi: 10.3389/fimmu.2019.02904
90. Page GG, Ben-Eliyahu S, Yirmiya R, Liebeskind JC. Morphine attenuates surgery-induced enhancement of metastatic colonization in rats. PAIN. Pain (1993) 54(1):21–8. doi: 10.1016/0304-3959(93)90095-7
91. Peng Y, Yang J, Guo D, Zheng C, Sun H, Zhang Q, et al. Sufentanil postoperative analgesia reduce the increase of T helper 17 (Th17) cells and FoxP3(+) regulatory T (Treg) cells in rat hepatocellular carcinoma surgical model: A randomised animal study. BMC ANESTHESIOL (2020) 20(1):212. doi: 10.1186/s12871-020-01129-0
92. Pang Q, Jin H, Qu K, Man Z, Wang Y, Yang S, et al. The effects of nonsteroidal anti-inflammatory drugs in the incident and recurrent risk of hepatocellular carcinoma: a meta-analysis. Onco Targets Ther (2017) 10:4645–56. doi: 10.2147/OTT.S143154
93. Tan R, Lockart I, Abdel SC, Danta M. Systematic review with meta-analysis: The effects of non-steroidal anti-inflammatory drugs and anti-platelet therapy on the incidence and recurrence of hepatocellular carcinoma. Aliment Pharmacol Ther (2021) 54(4):356–67. doi: 10.1111/apt.16515
94. O’Donnell SB, Nicholson MK, Boland JW. The association between benzodiazepines and survival in patients with cancer: A systematic review. J Pain Symptom Manage (2019) 57(5):999–1008. doi: 10.1016/j.jpainsymman.2019.01.010
95. O’Donnell SB, Nicholson MK, Boland JW. The association of benzodiazepines and z-drugs with mortality in patients with cancer: a systematic review. BMJ Support Palliat Care (2018) 8(Suppl 1):A9. doi: 10.1136/bmjspcare-2018-ASPabstracts.23
96. Vigneault L, Turgeon AF, Cote D, Lauzier F, Zarychanski R, Moore L, et al. Perioperative intravenous lidocaine infusion for postoperative pain control: A meta-analysis of randomized controlled trials. Can J Anaesth (2011) 58(1):22–37. doi: 10.1007/s12630-010-9407-0
97. Exadaktylos AK, Buggy DJ, Moriarty DC, Mascha E, Sessler DI. Can anesthetic technique for primary breast cancer surgery affect recurrence or metastasis? ANESTHESIOLOGY (2006) 105(4):660–4. doi: 10.1097/00000542-200610000-00008
98. Fischer LG, Bremer M, Coleman EJ, Conrad B, Krumm B, Gross A, et al. Local anesthetics attenuate lysophosphatidic acid-induced priming in human neutrophils. Anesth ANALG (2001) 92(4):1041–7. doi: 10.1097/00000539-200104000-00044
99. Khan JS, Hodgson N, Choi S, Reid S, Paul JE, Hong N, et al. Perioperative pregabalin and intraoperative lidocaine infusion to reduce persistent neuropathic pain after breast cancer surgery: A multicenter, factorial, randomized, controlled pilot trial. J Pain (2019) 20(8):980–93. doi: 10.1016/j.jpain.2019.02.010
100. Zhou Q, Lui VW, Yeo W. Targeting the PI3K/Akt/mTOR pathway in hepatocellular carcinoma. Future Oncol (2011) 7(10):1149–67. doi: 10.2217/fon.11.95
101. Lewandowska J, Bartoszek A. DNA Methylation in cancer development, diagnosis and therapy–multiple opportunities for genotoxic agents to act as methylome disruptors or remediators. MUTAGENESIS (2011) 26(4):475–87. doi: 10.1093/mutage/ger019
102. Zhang C, Li J, Huang T, Duan S, Dai D, Jiang D, et al. Meta-analysis of DNA methylation biomarkers in hepatocellular carcinoma. Oncotarget (2016) 7(49):81255–67. doi: 10.18632/oncotarget.13221
103. Chou R, Gordon DB, de Leon-Casasola OA, Rosenberg JM, Bickler S, Brennan T, et al. Management of postoperative pain: A clinical practice guideline from the American pain society, the American society of regional anesthesia and pain medicine, and the American society of anesthesiologists’ committee on regional anesthesia, executive committee, and administrative council. J Pain (2016) 17(2):131–57. doi: 10.1016/j.jpain.2015.12.008
104. Su Y, Pu Y, Zhao Z, Yang X. Influence of combined epidural anesthesia on cognitive function, inflammation and stress response in elderly liver cancer patients undergoing surgery. Oncol Lett (2020) 19(4):2733–8. doi: 10.3892/ol.2020.11395
105. Meng Z, Gao C, Li X, Shen J, Hong T, He X, et al. Effects of combined epidural anesthesia and general anesthesia on cognitive function and stress responses of elderly patients undergoing liver cancer surgery. J Oncol (2021) 2021:8273722. doi: 10.1155/2021/8273722
106. Zhou D, Gu FM, Gao Q, Li QL, Zhou J, Miao CH. Effects of anesthetic methods on preserving anti-tumor T-helper polarization following hepatectomy. World J Gastroenterol (2012) 18(24):3089–98. doi: 10.3748/wjg.v18.i24.3089
107. Wada H, Seki S, Takahashi T, Kawarabayashi N, Higuchi H, Habu Y, et al. Combined spinal and general anesthesia attenuates liver metastasis by preserving TH1/TH2 cytokine balance. ANESTHESIOLOGY (2007) 106(3):499–506. doi: 10.1097/00000542-200703000-00014
108. Sun HZ, Song YL, Wang XY. Effects of different anesthetic methods on cellular immune and neuroendocrine functions in patients with hepatocellular carcinoma before and after surgery. J Clin Lab Anal (2016) 30(6):1175–82. doi: 10.1002/jcla.22000
Keywords: anesthesia, hepatocellular carcinoma, long-term outcome, propofol, inhalational anesthetics
Citation: Zhao R, Xu X, Sun L and Zhang G (2023) Long-term effect of anesthesia choice on patients with hepatocellular carcinoma undergoing open liver resection. Front. Oncol. 12:960299. doi: 10.3389/fonc.2022.960299
Received: 07 June 2022; Accepted: 28 December 2022;
Published: 13 January 2023.
Edited by:
Goverdhan Puri, Post Graduate Institute of Medical Education and Research (PGIMER), IndiaCopyright © 2023 Zhao, Xu, Sun and Zhang. This is an open-access article distributed under the terms of the Creative Commons Attribution License (CC BY). The use, distribution or reproduction in other forums is permitted, provided the original author(s) and the copyright owner(s) are credited and that the original publication in this journal is cited, in accordance with accepted academic practice. No use, distribution or reproduction is permitted which does not comply with these terms.
*Correspondence: Li Sun, eWt5emx5eXN1bmxpQDEyNi5jb20=; Guohua Zhang, ZDE5NzRAMTYzLmNvbQ==
†These authors have contributed equally to this work and share first authorship