- 1Graduate Department, Bengbu Medical College, Bengbu, China
- 2Cancer Center, Department of Radiation Oncology, Zhejiang Provincial People’s Hospital, Affiliated People’s Hospital, Hangzhou Medical College, Hangzhou, China
- 3Graduate Department, Jinzhou Medical University, Jinzhou, China
- 4Cancer Center, Department of Medical Oncology, Zhejiang Provincial People’s Hospital, Affiliated People’s Hospital, Hangzhou Medical College, Hangzhou, China
The long non-coding RNA (lncRNA) PVT1 was first found to activate variant translocations in the plasmacytoma of mice. Human lncPVT1 is located on chromosome 8q24.21, at the same locus as the well-known MYC oncogene. LncPVT1 has been found to promote the progression of various malignancies. Chemoresistance and radioresistance seriously affect tumor treatment efficacy and are associated with the dysregulation of physiological processes in cancer cells, including apoptosis, autophagy, stemness (for cancer stem cells, CSC), hypoxia, epithelial–mesenchymal transition (EMT), and DNA damage repair. Previous studies have also implicated lncPVT1 in the regulation of these physiological mechanisms. In recent years, lncPVT1 was found to modulate chemoresistance and radioresistance in some cancers. In this review, we discuss the mechanisms of lncPVT1-mediated regulation of cellular chemoresistance and radioresistance. Due to its high expression in malignant tumors and sensitization effect in chemotherapy and radiotherapy, lncPVT1 is expected to become an effective antitumor target and chemotherapy and radiotherapy sensitizer, which requires further study.
Introduction
Long non-coding RNAs (lncRNAs), a class of functional RNA molecules larger than 200 nucleotides that cannot be translated into proteins, play an important regulatory role in epigenetics (1). LncPVT1 is an important member of the lncRNA family and was first found to activate variant translocations in the plasmacytoma of mice (2). Human lncPVT1 is located on chromosome 8q24.21, at the same locus as the well-known oncogene MYC (3) (Figure 1). It not only interacts with MYC to promote cancer progression but also performs different oncogenic functions independent of MYC (4). LncPVT1 has been reported to be overexpressed in many types of malignant tumors, promoting cancer progression (5–9) (Figure 2). Moreover, lncPVT1 can regulate proliferation, invasion, autophagy, apoptosis, epithelial–mesenchymal transition (EMT), hypoxia, stemness (for cancer stem cells, CSC), exosomes, and other important physiological mechanisms (10–14).
Chemotherapy is an important cancer treatment regimen that kills cancer cells primarily through the systemic or local use of chemosynthetic drugs and has a significant clinical benefit for patients (15). Chemoresistance leads to cancer recurrence and metastasis, hindering patient survival; hence, it remains the main obstacle in cancer treatment. Therefore, we need to understand the detailed regulatory mechanisms underlying chemoresistance to improve tumor cell sensitivity to chemotherapy (16). The currently reported molecular mechanisms associated with chemoresistance include the action of oncogenes, tumor suppressor genes, mitochondrial changes, DNA repair, autophagy, EMT, CSC, and exosomes (17). These pathways often intersect to increase the tolerance of tumors to cytotoxic drugs; therefore, the inhibition of these pathways will significantly improve the sensitivity of tumors to chemotherapy.
Radiotherapy is also an important form of cancer treatment. It can be used alone or in combination with other forms of treatment, either curative or palliative, for all stages of cancer. More than 50% of patients with cancer receive radiotherapy (18). Unfortunately, radioresistance reduces the efficacy of radiotherapy and seriously affects the quality of life of patients with cancer (19). Radiation therapy kills cancer cells directly or indirectly by causing DNA damage (20). However, ionizing radiation can also activate multiple prosurvival signaling pathways to promote DNA damage checkpoint activation, DNA repair, autophagy, apoptosis inhibition, and CSC. These signaling pathways conjointly protect cancer cells from radiation injury and promote radioresistance (21). Current literature indicates that the tumor microenvironment is closely related to radioresistance. Under hypoxic conditions, it has been shown that the radiosensitivity of tumor cells decreased significantly (22, 23).
LncPVT1 has gradually become a research hotspot regarding the regulation of chemoresistance and radioresistance, as studies have found that the regulatory mechanism of lncPVT1 in tumors is also associated with these processes. In recent years, lncPVT1 has been reported to regulate the chemoresistance of tumor cells through various pathways (Table 1). LncPVT1 silencing has also been shown to enhance radiosensitivity in nasopharyngeal carcinoma and lung cancer (Table 2). In this review, we summarize the various mechanisms through which lncPVT1 regulates chemosensitivity and radiosensitivity. We further emphasized that lncPVT1 is expected to be a new sensitizer of chemotherapy and radiotherapy and that it can be used to develop better clinical therapeutic strategies for patients with cancer.
PVT1 and Chemoresistance
Pancreatic cancer
Pancreatic cancer is a highly malignant tumor with an extremely poor prognosis. The 1- and 5-year survival rates of this disease are only 24% and 9%, respectively (55). Gemcitabine is a first-line chemotherapeutic agent for advanced pancreatic cancer, and resistance to gemcitabine and other chemotherapeutic drugs is an important factor in the poor prognosis of this malignancy (56).
The expression level of lncPVT1 is significantly increased in pancreatic cancer. Moreover, lncPVT1 has been reported to promote the proliferation and migration of pancreatic cancer cells (13). Previous reports have suggested that decreased lncPVT1 levels can increase sensitivity of pancreatic cancer cells to gemcitabine chemotherapy (57, 58). Studies have shown that the activation of the Wnt/β-catenin pathway can induce chemoresistance in cancer cells (59). Autophagy is an important mechanism for tumor cell survival and has been proven to improve the tolerance of tumor cells to radiotherapy and chemotherapy (60). LncPVT1 competitively binds to microRNA-619-5p (miR-619-5p) to regulate the expression of Pygo2, mediating the Wnt/β-catenin pathway to increase the chemoresistance of pancreatic cancer cells to gemcitabine. At the same time, the autophagy-related protein ATG14 is also regulated by lncPVT1/miR-619-5p to increase autophagy, thereby promoting gemcitabine resistance in pancreatic cancer (24).
O6-Methylguanine-DNA methyltransferase (MGMT) is a DNA repair enzyme regulated by Wnt/β-catenin that protects cells from physicochemical damage by repairing damaged DNA (61). In addition, the Hedgehog (Hh) signaling pathway has been shown to be closely associated with tumor resistance and regulates autophagy (62, 63). Its terminal transcription factor Gli is responsible for transmitting signals into the nucleus and promoting transcriptional activation to upregulate the expression level of downstream target genes, MGMT (64, 65). Based on these studies, Yu Shi et al. found that lncPVT1 acts as a competing endogenous RNA (ceRNA) of miR-409 in pancreatic cancer cells. At the same time, miR-409 directly targets The Sonic Hedgehog (SHH) and regulates apoptosis and autophagy through the SHH/GLI/MGMT pathway, thus mediating the resistance of pancreatic cancer to gemcitabine (25). Furthermore, the hypoxia-inducible factor 1-alpha (HIF-1α)/vacuole membrane protein 1 (VMP1) axis mediates therapeutic resistance in colon cancer cells (66). Researchers recently found that lncPVT1 competitively binds to miR-143 and that decreased lncPVT1 levels and upregulated miR-143 levels increase the chemosensitivity of pancreatic cancer cells to gemcitabine through the HIF-1α/VMP1 axis (26).
In addition, lncPVT1 regulates the sensitivity of pancreatic cancer cells to gemcitabine by inhibiting enhancer of zeste homolog-2 (EZH2) at polycomb repressive complex 2 (PRC2), where EZH2 upregulates the expression of lncPVT1. LncPVT1, as an enhancer of MYC, promotes the expression of c-Myc, thus stimulating the activity of CSCs. The dysregulation of CSC in pancreatic cancer can induce chemoresistance (67). Another study has concluded that curcumin downregulates cancer stemness by inhibiting the PRC2/lncPVT1/c-MYC pathway, increasing the sensitivity of pancreatic cancer cells to gemcitabine (27). Sun et al. found that histone acetyltransferase 1 (HAT1) promotes the binding of bromodomain-containing 4 (BRD4) to the lncPVT1 promoter to enhance lncPVT1 expression. Simultaneously, HAT1 competitively binds to the N-terminus of EZH2 with the ubiquitin protein ligase E3 component n-recognin 4 (UBR4), preventing the ubiquitination of EZH2. Thus, HAT1 promotes gemcitabine resistance in pancreatic cancer cells (28). Based on this mechanism, tripolyphosphate (TPP)-siHAT1 nanoparticles have been developed to inhibit HAT1 expression and overcome gemcitabine resistance in pancreatic cancer cells. However, the efficacy and safety of TPP-siHAT1 nanoparticles require further validation.
You et al. have also discussed the mechanism of gemcitabine resistance in pancreatic cancer cells. They found that Drosha ribonuclease III (Drosha) and DGCR8 promote the lncPVT1-mediated processing of miR-1207-5p and miR-1207-3p. The generated products miR-1207-5p and miR-1207-3p inhibit sarcoma gene (SRC) and ras homolog family member A (RhoA), respectively, to increase the sensitivity of pancreatic cancer cells to gemcitabine chemotherapy (29). These studies highlight lncPVT1 as a promising target for improving the sensitivity of pancreatic cancer cells to gemcitabine.
Gastric cancer
Gastric cancer remains the most common malignancy of the digestive system, ranking fifth in incidence and third in causing cancer-related deaths worldwide (68). Although radical resection is the standard treatment for early gastric cancer, patients are often diagnosed with gastric cancer at the advanced stage, making chemotherapy the main treatment regimen after diagnosis. Cisplatin, 5-FU, and other chemotherapeutic drugs are common first-line treatments for gastric cancer (69). However, the emergence of multi-drug resistance (MDR) reduces the sensitivity of chemotherapy and makes the survival benefit of patients with advanced gastric cancer worse (70).
Many studies have suggested that lncPVT1 can be used as a biomarker for the diagnosis and prognosis of gastric cancer (71). In addition, lncPVT1 can promote the growth and invasion of gastric cancer and induce angiogenesis (11, 72). The MDR-associated proteins include MDR1, mTOR, HIF-1α, and MRP. A decrease in lncPVT1 expression was found to increase the apoptosis rate of cisplatin-treated gastric cancer cell lines. Reverse transcription-quantitative polymerase chain reaction (RT-qPCR) and Western blotting (WB) showed that MDR1, mTOR, HIF-1α, and multi-drug resistance-associated protein 1 (MRP1) expression were all increased when lncPVT1 expression increased. The expression of these MDR-related genes promotes the expression of P-glycoprotein (P-gp). P-gp transports chemotherapeutic drugs out of the cell and helps the cell develop resistance to these drugs (73). Hence, lncPVT1 could be an effective target for reversing MDR in gastric cancer (30). Kanglaite (KLT) is a Chinese herbal formulation with antitumor effects (74), which is often combined with chemotherapy drugs to reduce the side effects of chemotherapy and increase chemosensitivity (75). Researchers found that KLT inhibited the expression of lncPVT1, reducing the expression of MDR1 and MRP1 in cisplatin-resistant gastric cancer cell lines (31).
Recently, the mechanism by which lncPVT1 regulates chemoresistance in gastric cancer has been further elaborated. Wu et al. found that lncPVT1 competes with miR-3619-5p to regulate the downstream target transducin beta-like 1 X-linked receptor 1 (TBL1XR1), regulating the sensitivity of gastric cancer cells to cisplatin (32). Moreover, 5-FU is also one of the main chemotherapeutic drugs for gastric cancer (76), so chemoresistance to 5-FU in gastric cancer deserves more attention. Du et al. found that lncPVT1 silencing could inhibit the expression of the anti-apoptotic protein Bcl-2, promoting apoptosis and inducing 5-FU resistance in gastric cancer cells (33). Therefore, lncPVT1 might play an important role in regulating cisplatin resistance in gastric cancer cells.
Ovarian cancer
Ovarian cancer is the deadliest gynecological cancer and is a serious threat to women’s health worldwide. Approximately 70% of patients with this disease are already at an advanced stage upon diagnosis. Platinum-based chemotherapy and cytoreductive operations are standard treatments for ovarian cancer (77). Similarly, the clinical emergence of chemoresistance also poses great challenges for the survival of patients with this disease. Therefore, determining the target of chemoresistance in ovarian cancer is urgently needed.
LncPVT1 plays an oncogene role in ovarian cancer and promotes the proliferation and invasion of ovarian cancer cell (78, 79). Researchers found that lncPVT1 expression levels were significantly elevated in cisplatin-resistant ovarian cancer tissues (80). Liu et al. silenced lncPVT1 expression in cisplatin-resistant ovarian cancer cell lines and measured their cell viability and apoptosis rate after treatment with cisplatin. The results showed that the cell viability was significantly decreased and the apoptosis rate of tumor cells was significantly higher in downregulation of the lncPVT1 group than that of the control group after treatment with cisplatin. They also overexpressed lncPVT1 in cisplatin-sensitive ovarian cancer cell lines, which were then treated with cisplatin. Compared to the control group, cell viability in these lncPVT1-overexpressing cell lines was significantly increased, and the apoptosis rate was significantly reduced. RT-qPCR and WB results showed that the mRNA levels and protein expression of apoptosis-related genes TGF-β1, p-Smad4, and caspase-3 were significantly increased with a decrease in lncPVT1 expression level. Hence, lncPVT1 overexpression induces cisplatin resistance in ovarian cancer cells by inhibiting the apoptotic pathway (34).
Researchers further investigated how lncPVT1 regulates cisplatin resistance in ovarian cancer. Forkhead box M1 (FOXM1) has been reported to be involved in a variety of malignant behaviors of ovarian cancer cells, including growth, proliferation, invasion, and metastasis (72, 81). Yi et al. found that lncPVT1 acts as a molecular sponge for miR370 to inhibit miR370 and promote FOXM1 expression. Furthermore, lncPVT1 directly binds to FOXM1 and increases its protein expression level. Moreover, lncPVT1 promoted cisplatin resistance in ovarian cancer by increasing FOXM1 expression (35). Chen et al. found that lncPVT1 silencing can inhibit the growth and proliferation and promote apoptosis in cisplatin-resistant ovarian cancer cells by downregulating the JAK2/STAT3/PD-1 signaling pathway (36). The combination of lncPVT1-targeted therapy and PD-L1 immunotherapy is expected to improve the efficacy in patients with cisplatin-resistant ovarian cancer (82).
Colorectal cancer
Colorectal cancer (CRC) is the third most common cancer worldwide. Cisplatin and 5-FU are the first-line chemotherapy agents for colorectal cancer (83). However, MDR is the main cause of treatment failure in patients with this disease. Researchers have found that lncPVT1 acts as a molecular sponge targeting miR-16-5p, thereby promoting proliferation, invasion, and migration of CRC cells by regulating VEGFA/VEGFR1/AKT signaling pathway (84). Moreover, lncPVT1/VEGFA axis promotes colon cancer metastasis and stemness by downregulation of miR-152-3p (10). LncPVT1 has also been found to promote proliferation, invasion, and migration of CRC cells by regulating the miR-106b-5p/FJX1 axis (85). Furthermore, lncPVT1 knockdown increased the apoptosis rate of cisplatin-resistant colorectal cancer cells, whereas the overexpression of lncPVT1 significantly enhanced the resistance of colorectal cancer cells to cisplatin. Moreover, silencing lncPVT1 increased the expression levels of pro-apoptotic proteins Bax and cleaved caspase-3 in cisplatin-resistant colorectal cancer cells while at the same time decreasing the expression levels of MDR1, MRP1, and the anti-apoptotic protein Bcl-2. We concluded that lncPVT1 regulates the sensitivity of colorectal cancer cells to cisplatin through the apoptotic pathway (37).
In addition, increased lncPVT1 expression promoted colorectal cancer cell tolerance to 5-FU. RT-qPCR and WB showed that lncPVT1 upregulated the expression levels of MRP1, mTOR, P-gp, and Bcl-2 (38). Therefore, lncPVT1 is an effective target for treating chemoresistance in colorectal cancer.
Bladder cancer
Bladder cancer is a fatal malignancy of the urinary system that mainly affects men over 65 years of age. Surgical treatment, radiotherapy, chemotherapy, immunotherapy, and bladder perfusion therapy are used for patients with different stages of bladder cancer (86). As an oncogene of bladder cancer cells, lncPVT1 acts as a ceRNA targeting miR-194-5p to regulate the expression level of BCLAF1, thereby promoting the proliferation, migration, and anti-apoptosis of bladder cancer cells (87). A previous study showed that lncPVT1 promotes growth, migration, and invasion of bladder cancer by miR-31/CDK1 (88).
Researchers found a significant increase in lncPVT1 expression in bladder cancer cells resistant to doxorubicin (DOX) and cisplatin (DDP). Moreover, lncPVT1 silencing not only attenuated the growth, proliferation, and other malignant behaviors of drug-resistant bladder cancer cell lines but also increased their cell apoptosis rate and sensitivity to DOX and DDP. WB results showed that the expression levels of the drug-resistant related proteins MDR1 and MRP1 decreased with a decrease in lncPVT1 expression. Further studies on the regulatory mechanism of lncPVT1 revealed that lncPVT1 regulates the expression levels of drug-resistant related proteins MDR1 and MRP1 through positive regulation of the Wnt/β-catenin pathway, thus affecting the sensitivity of bladder cancer cells to DOX and DDP (39). In another study, Jiang et al. found that increased lncPVT1 expression promoted mouse double minute 2 (MDM2) and MDM2-mediated aurora kinase B (AURKB) expression. Subsequently, p53 ubiquitination is enhanced, increasing resistance to doxorubicin (ADM) in bladder cancer cells (40).
Lung cancer
Lung cancer is the leading cause of cancer-related deaths worldwide, and smoking remains a major risk factor for this disease. Platinum-based chemotherapy is one of the main treatments for inoperable lung cancer (89); however, chemoresistance limits the application of platinum-based drugs in patients with lung cancer patients, increasing their mortality (90). Therefore, there is a need to study the mechanism of cisplatin tolerance in lung cancer and identify an effective target to solve this clinical problem.
LncPVT1 is highly expressed in non-small cell lung cancer (NSCLC) and is associated with poor prognosis (91). Wang et al. found that lncPVT1 facilitates the proliferation, migration, and invasion of NSCLC cells by indirectly mediating FGFR1 via targeting miR-551b (92). In another study, lncPVT1 activates the Wnt/β-catenin signaling pathway by miR-361-3p/SOX9 axis, thereby promoting the proliferation, migration, invasion, and anti-apoptosis of NSCLC cells (93). A previous study showed that lncPVT1 promotes angiogenesis by regulating the miR-29c/VEGF signaling axis in NSCLC (94). Beclin-1 is a biomarker for autophagy that has been reported to be involved in the malignant biological behavior of lung cancer cells (95). Chen et al. found that lncPVT1 sponges miR216b to inhibit Beclin-1 expression and induce cisplatin tolerance in NSCLC cells by regulating apoptosis and autophagy (41). Further studies have found that HIF-1α and other related pathways are activated to induce lncPVT1 expression under hypoxic conditions. The induction of lncPVT1 expression increases the expression level of ATG5 through competitive binding with miR140-3p and reduces the sensitivity of lung cancer cells to cisplatin by influencing the autophagy pathway (42). These studies indicate that lncPVT1 is expected to become a new target to solve the problem of chemoresistance in lung cancer and improve the efficacy of treatment for this disease.
Breast Cancer
Breast cancer has replaced lung cancer as the most common malignant tumor worldwide. Triple-negative breast cancer (TNBC) is a subtype of breast cancer with negative estrogen receptor (ER), progesterone receptor (PR), and human epidermal growth factor receptor 2 (HER-2) expression. It is characterized by its invasiveness, aggressive metastasis, and recurrence (96). Chemotherapy is the standard treatment for TNBC; unfortunately, chemoresistance is a major obstacle in treating patients with breast cancer.
LncPVT1 promotes breast cancer cell proliferation, migration, invasion, and anti-apoptosis via regulating miR-543/TRPS1 axis (97). In TNBC cells cultured with mature adipogenic medium (MAM), lncPVT1 facilitates EMT, cell proliferation, and cell migration by regulating p21 expression (98). A recent study showed that lncPVT1 promotes cell migration and invasion by regulating miR-148a-3p/ROCK1 axis in breast cancer (99). The Keap1/Nrf2/ARE pathway is an important antioxidant stress signaling pathway in the body, which has been observed to regulate drug resistance in tumor cells (100, 101). Using bioinformatics analysis, Luo et al. concluded that lncPVT1 could interact with Keap1. They then conducted experiments to verify that lncPVT1 blocked the Keap1-mediated degradation of Nrf2 through competitive binding with Keap1. In turn, this binding increased the expression of Nrf2 and increased the expression of downstream drug-resistance-related molecules. Moreover, lncPVT1 increased adriamycin resistance in TNBC through this mechanism (43).
Osteosarcoma
Osteosarcoma (OS) is a malignant tumor that usually occurs in children and adolescents. Patients with this disease often develop lung metastases. Surgical treatment, chemotherapy, and radiotherapy are the main therapeutic methods for OS (102, 103). In recent years, there have been breakthroughs in the application of immunotherapy in patients with OS (104). Chemotherapy treatments for OS often involve multi-drug combination therapy, usually including adriamycin, cisplatin, bleomycin, cyclophosphamide, and gemcitabine, among other drugs. Chemoresistance often leads to recurrence and metastasis in patients with osteosarcoma.
LncPVT1 plays a carcinogenic role in OS, promoting OS cell glucose metabolism, growth, proliferation, and invasion through regulating miR-497/HK2 axis (105). In a previous study, exosomes secreted by bone marrow mesenchymal stem cells (BMSCs) transfer lncPVT1 into osteosarcoma cells; the upregulation of lncPVT1 can promote ERG expression by inhibiting ERG ubiquitination and sponging miR-183-5p. LncPVT1 promotes the growth and metastasis of OS by regulating ERG expression (106). Moreover, lncPVT1 plays a potential role in regulating CSCs in OS (107). LncPVT1 is a ceRNA of miR-152. It activates the c-MET/PI3K/AKT pathway to enhance the resistance of OS cells to gemcitabine (44). Moreover, c-MET is a hepatocyte growth factor (HGF) receptor that has been reported to promote the progression of various cancers (108). The inhibition of c-MET has been shown to enhance the sensitivity of OS cells to cisplatin by inhibiting the PI3K/AKT pathway (109).
Glioma
Glioma is the most common primary malignant brain tumor in adults. The treatment for this disease includes a combination of surgery, radiotherapy, and chemotherapy. Due to the blood–brain barrier and tumor heterogeneity, gliomas have strong drug resistance, leading to a high degree of malignancy and poor prognosis (110); hence, understanding the mechanisms of drug resistance in glioma is expected to improve patient prognosis.
LncPVT1 is highly expressed in gliomas, indicating poor prognosis. In addition, lncPVT1 can promote angiogenesis by regulating miR-1207-3p/hepatocyte nuclear factor 1β (HNF1B)/EMT axis in glioma (111). In another study, lncPVT1 influence bone morphogenetic protein (BMP) signaling pathway by regulating miR-128-3p/GREM1 axis, thereby promoting glioma cell proliferation, invasion, migration, and anti-apoptosis (112). A recent study showed that tumor suppressor gene p53 inhibits glioma cell proliferation, migration, and invasion while inducing apoptosis by blocking lncPVT1/TGF-β/Smad signaling pathway (113). Researchers knocked down lncPVT1 expression levels in SHG-44 glioma cells, added different concentrations of paclitaxel, and then measured their apoptosis rate. The results showed that the apoptosis rate of glioma cells was significantly higher in the lncPVT1-silenced group compared to the control group. These results suggest that decreased lncPVT1 expression increases the sensitivity of glioma cells to paclitaxel (45). Further research found that lncPVT1 acts as ceRNA to target miR-365 and positively regulate the expression level of E74-like ETS transcription factor 4 (ELF4). ELF4 has been reported to be highly expressed in glioma and can upregulate SOX2 expression to promote stemness (114). In conclusion, lncPVT1 facilitates stemness and TMZ resistance through regulating miR-365/ELF4/SOX2 signal axis in glioma (46).
Cervical Cancer
Cervical cancer is the fourth most common cancer in women worldwide. Human papillomavirus (HPV) infection has been confirmed to be closely related to the occurrence and development of cervical cancer. HPV16, 18, 31, and 45 are the main causes of cervical cancer (115). Chemotherapy is the main treatment for advanced and recurrent cervical cancer, and platinum-based chemotherapy, such as cisplatin combined with paclitaxel, is often used. However, the emergence of chemoresistance significantly reduces the efficacy of this regimen in patients with cervical cancer.
It was observed that serum lncPVT1 levels in cervical cancer patients is generally elevated and correlated with cervical cancer tumor size, lymph node metastasis, and clinical stage. This suggests that lncPVT1 may be a novel serum biomarker for early diagnosis of cervical cancer (116). LncPVT1 acts as a ceRNA or sponge of miR-424 to promote the proliferation, migration, and invasion of cervical cancer cells (117). In addition, lncPVT1 has been found to regulate the sensitivity of cervical cancer cells to paclitaxel. Specifically, lncPVT1 directly and competitively binds to miR-195 and recruits EZH2 to induce histone H3 lysine 27 trimethylation (H3K27me3) in the miR-195 promoter region, thereby inhibiting the expression of miR-195. Downregulation of miR-195 promotes EMT in cervical cancer cells, thereby enhancing their chemoresistance to paclitaxel (47).
PVT1 and Radioresistance
Nasopharyngeal Cancer
Nasopharyngeal carcinoma (NPC) is a malignant tumor originating from the epithelial cells of the nasopharynx. This disease often occurs in southern China, with a significant regional concentration and ethnic susceptibility (118). Although the incidence of NPC is not high, NPC cells easily invade other tissues and metastasize, showing a strong degree of malignancy. NPC is closely related to Epstein–Barr virus infections (119). Radiotherapy has been established as the cornerstone of NPC treatment since 1965. However, in recent years, the development of intensity-modulated radiotherapy (IMRT) and proton radiotherapy (PRT) has brought significant survival benefits to patients with NPC (120). However, 10%–20% of NPC patients develop recurrence after radiotherapy due to radioresistance (121). Therefore, it is important to explore the molecular mechanism underlying radioresistance in NPC and search for new clues for radiotherapy sensitization in this disease (122).
In NPC, lncPVT1 has been found to promote cell proliferation and induce stemness by targeting miR-1207 (123). It was previously reported that the expression level of lncPVT1 was significantly upregulated in a radiation-induced mouse model, wherein RT-qPCR analysis showed that the expression level of lncPVT1 was significantly increased in the irradiated mice (124). Yi He et al. found that lncPVT1 silencing led to a decrease in phosphorylation of ATM/Chk2/p53 signaling pathway in NPC cells, which further led to the inhibition of the ATM-mediated homologous recombination (HR) repair pathway. Hence, more cells became apoptotic due to DNA damage that cannot be repaired in time (125). Meanwhile, decreased lncPVT1 expression levels after radiotherapy activated caspase, which is a core pro-apoptotic caspase, resulting in a cascade reaction that successively activates caspase-9, caspase-7, and PARP. PARP is believed to be the receptor for DNA damage and the cleavage substrate of caspase-7. Apoptosis is induced when activated caspase-7 binds to PARP (126). Moreover, the presence of lncPVT1 and MYC on the same chromosome band forms a positive feedback loop to promote tumor progression synergistically (3). In conclusion, lncPVT1 can promote DNA repair by phosphorylation of ATM/Chk2/p53 signaling pathway in NPC cells. LncPVT1 can also significantly inhibit apoptosis by blocking caspase-9/caspase-7/PARP axis, thereby leading to radioresistance (50).
Hypoxia induces radioresistance in tumor cells. HIF-1α is a major regulator of the hypoxia response and can be stably expressed under hypoxic conditions (127). Kyoto Encyclopedia of Genes and Genomes (KEGG) analysis showed that lncPVT1 was associated with the hypoxic phenotype. Because tumor hypoxia is common in NPC and it is associated with disease progression and resistance to therapy, Hong et al. suggested that targeting tumor hypoxia could be an effective approach for NPC treatment (128). Further studies revealed that lncPVT1 acts as a molecular scaffold for KAT2A and WDR5, coordinating their localization, and enabling KAT2A to acetylate H3K9 effectively. Acetylated H3K9 then recruits TIF1β to bind to chromatin, forming an H3K9ac/TIF1β complex that induces the transcription of NF90 and stabilizes HIF-1α expression (51). LncPVT1 induces radioresistance in NPC by activating the above-mentioned pathways and generating a hypoxic tumor microenvironment.
In addition, Han et al. found that lncPVT1 silencing inhibited cell proliferation, promoted cell apoptosis, and increased radiosensitivity in NPC cells. Moreover, lncPVT1 binds to miR-515-5p and negatively regulates its expression. An increase in lncPVT1 expression can reverse the inhibitory effect of miR-515-5p overexpression on the growth and proliferation of cancer cells and the promotive effect of miR-515-5p on the apoptosis and radiosensitivity of cancer cells. Meanwhile, miR-515-5p overexpression also reversed the inhibition of cyclin D1 expression and the elevation of Bax and cleaved caspase-3 levels. Further studies have shown that PIK3CA promotes growth and proliferation, inhibits apoptosis, and increases the radioresistance of NPC cells, whereas the overexpression of miR-515-5p reversed these effects. Moreover, miR-515-5p inhibition reversed the inhibitory effect of lncPVT1 silencing on the expression levels of PIK3CA and p-AKT. It has previously been reported that the p-AKT pathway is involved in regulating radioresistance in various cancers, and that PIK3CA can activate this pathway (129, 130). In conclusion, increased lncPVT1 expression activates the P-AKT pathway through the miR-515-5p/PIK3CA axis, thereby promoting cell proliferation, inhibiting apoptosis, and inducing radioresistance (52).
Lung cancer
Patients with lung cancer typically receive a combination of treatments, but radiotherapy is the only treatment used for all types and stages of lung cancer. According to evidence-based models, 77% of patients with lung cancer are eligible for radiotherapy (131). With advancements in technology, radiotherapy has effectively improved treatment efficacy in patients with lung cancer while reducing the incidence of adverse reactions (132). However, radioresistance in lung cancer usually leads to recurrence and metastasis, which significantly reduces patient survival. Therefore, there is an urgent need to identify new targets for lung cancer radiotherapy sensitization to improve patient prognosis (133).
It has been reported that reduced lncPVT1 expression significantly inhibits the growth and proliferation of NSCLC cells. The apoptosis rate of NSCLC cells treated with a certain radiation dose was determined. The results showed that the apoptosis rate of the lncPVT1-silenced group was significantly higher than that of the control group, indicating that lncPVT1 silencing enhances the radiosensitivity of NSCLC cells. Luciferase reporter assays showed that lncPVT1 could directly interact with miR-195 and negatively regulate its expression. miR-195 inhibitors reversed the inhibitory effect of lncPVT1 silencing on cell proliferation and promoted apoptosis in vitro. In addition, miR-195 inhibitors also reversed the radiosensitization effect of lncPVT1 silencing in NSCLC cells. In conclusion, lncPVT1 acts as a molecular sponge of miR-195 in NSCLC, and the decreased expression level of lncPVT1 inhibits cell proliferation and promotes cell apoptosis, thus increasing the radiosensitivity of NSCLC cells (53).
Other related studies have shown that coactivator-associated arginine methyltransferase 1 (CARM1) can co-activate transcriptional regulation with PRMT1 and is overexpressed in NSCLC (134). CARM1 has been reported to be involved in regulating radiosensitivity in cervical cancer cells and colorectal cancer cells (135, 136). Wang et al. found that lncPVT1, as a ceRNA of miR-424-5p, promoted the expression of CARM1. The decrease in lncPVT1 levels or the increase in miR-424-5p levels inhibited the growth, proliferation, invasion, metastasis, and other malignant behaviors of NSCLC cells, promoted the apoptosis of NSCLC cells, and upregulated their radiosensitivity. RT-qPCR and WB showed that lncPVT1 silencing or miR-424-5p overexpression regulated the expression levels of MMP-2, MMP-9, Bcl-2, and Bax, which are related to tumor progression and apoptosis. Therefore, lncPVT1, as a ceRNA of miR-424-5p, regulates the radiosensitivity of NSCLC by regulating CARM1 (54).
LncPVT1 and Resistance to other drugs
Head and neck squamous cell carcinoma (HNSCC) is the most common type of head and neck cancer and originates from the mucosal surface of the head and neck. Alcohol, tobacco, and HPV infection are risk factors for the induction of squamous cell carcinoma of the head and neck (137). EGFR is also usually highly expressed in HNSCC and is involved in regulating tumor occurrence and progression. Therefore, EGFR-targeted therapies are becoming increasingly popular for this disease. Cetuximab, a monoclonal antibody targeting EGFR, is often used in combination with radiotherapy to treat locally advanced HNSCC and has achieved good efficacy (138). LncPVT1 promotes laryngeal squamous cell carcinoma cell proliferation, migration, and anti-apoptosis by acting as a molecular sponge to regulate miR-519d-3p (139). In addition, lncPVT1 competitively combined with miR-150-5p to regulate GLUT1, thereby promoting the proliferation, migration, invasion, and anti-apoptosis of oral squamous cell carcinoma (140). LncPVT1 overexpression promoted HNSCC growth, significantly reduced the apoptosis rate of HNSCC cells treated with cetuximab, and reduced the drug sensitivity of HNSCC cells. As a tumor suppressor, miR-124-3p can reverse HNSCC tolerance to cetuximab. Furthermore, lncPVT1 can promote the resistance of HNSCC to cetuximab by inducing the methylation of the miR-124-3p promoter and downregulating miR-124-3p expression (48).
Prostate cancer is one of the most common malignant tumors in men worldwide, seriously affecting their health. Since androgens regulate the occurrence and progression of tumors, androgen-stripping therapy, specifically castration therapy, has become an important part of the prostate cancer treatment regimen. The clinical use of androgen inhibitors has significantly benefited patients with metastatic prostate cancer; however, the use of these drugs in metastatic castration-resistant prostate cancer (mCRPC), which occurs in some patients, remains a challenge (141). LncPVT1 could be used as a prognostic factor in patients with prostate cancer, representing poor survival (142). In prostate cancer, lncPVT1 has been found to promote EMT by regulating miR-186/Twist1 (143). LncPVT1 competitively binds to miR-15b-5p, miR-143-3p, miR-27a-3p, and miR-627-5p, then promotes prostate cancer invasion and metastasis by upregulating NOP2 (144). In another study, lncPVT1 promoted the viability of prostate cancer cells and inhibited their apoptosis by targeting miR-146a (145). Abiraterone is a second-generation androgen inhibitor used clinically for prostate cancer treatment. Abiraterone was used to treat lncPVT1-overexpressing prostate cancer cell lines and untreated pancreatic cancer cell lines in vitro. It was shown that lncPVT1 overexpression retained higher cell viability than the control group, suggesting that lncPVT1 induces castration resistance in prostate cancer cells (49).
Conclusion
Cancer treatment should be diversified to improve the survival of patients with various malignancies. As two powerful tools of cancer treatment, chemotherapy and radiotherapy have been shown to improve the survival of patients with cancer, which is of great significance (20, 146). However, the consequent chemoresistance and radioresistance have become the main obstacles to maintain the efficacies of these treatments. Hence, there is an urgent need to find new targets to overcome this barrier. The physiological mechanisms involved in the regulatory activities of lncPVT1 have many similarities with those involved in chemoresistance and radioresistance, including DNA damage repair, apoptosis, autophagy, EMT, stem cells, and hypoxia (Figure 3). This has led to extensive research on the relationship between lncPVT1, chemoresistance, and radioresistance (147, 148).
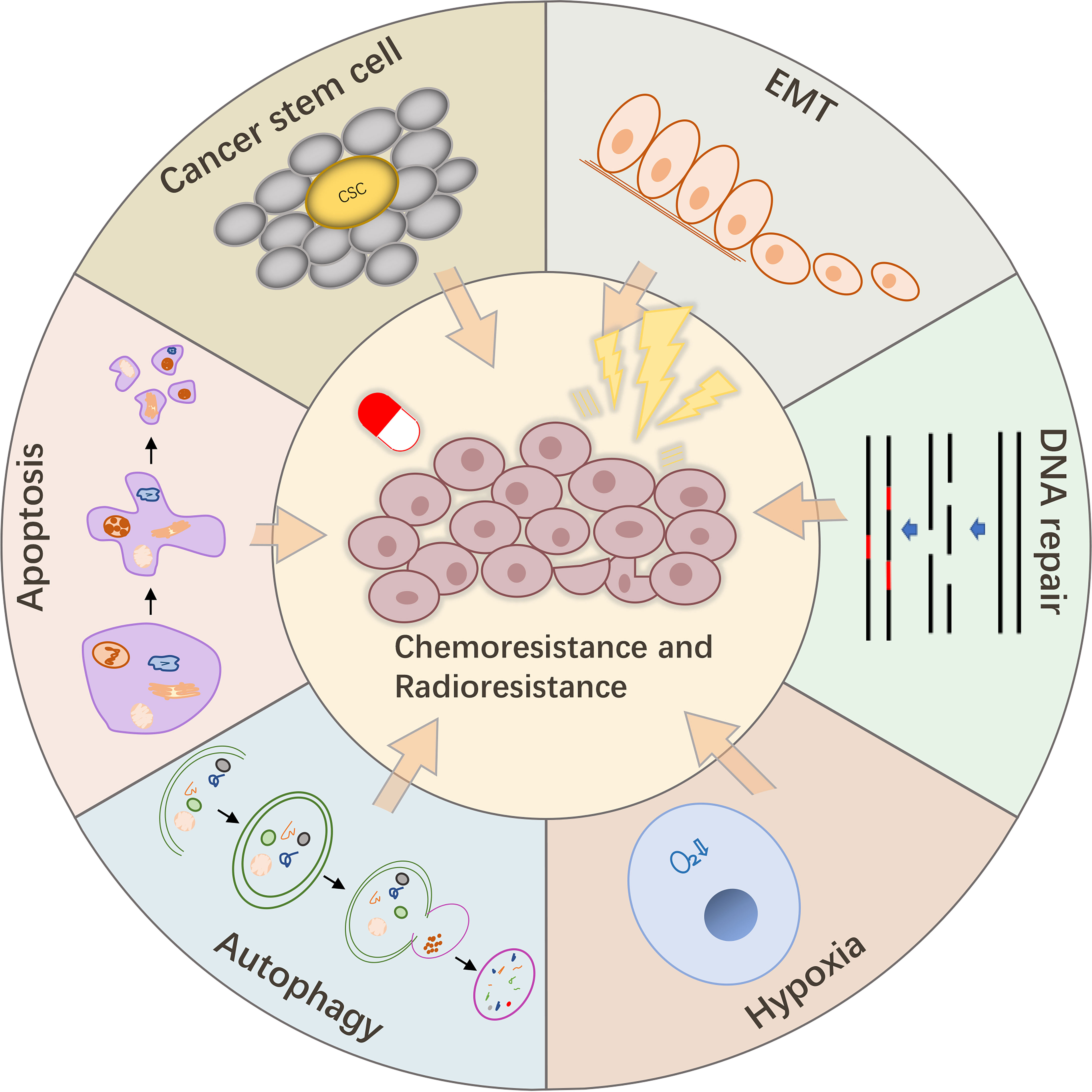
Figure 3 LncPVT1 regulates chemoresistance and radioresistance through autophagy, apoptosis, EMT, CSC, DNA damage repair, hypoxia, and other mechanisms.
In recent years, several preclinical studies have explored the mechanisms underlying lncPVT1-induced chemoresistance and radioresistance in malignant tumor models (Figures 4, 5). Among them, lncPVT1 can act as a molecular sponge of ceRNAs to inhibit miRNA expression, as a skeleton to bind proteins to specific targeted sequences, or bind proteins to form specific modules. In addition, lncPVT1 can encode miR-1204, miR-1205, miR-1206, miR-1207-5p, miR-1207-3p, and miR-1208 (149). Subsequently, downstream apoptosis and DNA damage repair pathways can be regulated to induce chemoresistance and radioresistance in cancer. Previous reviews have shown that lncPVT1 regulated various signaling pathways through miRNAs to influence cancer progression and chemoresistance (150). However, the types of miRNAs involved in the current study were limited. The interaction between lncPVT1 and other miRNAs needs to be revealed in a more comprehensive study of mechanisms related to chemoresistance and radioresistance. Many studies have only identified phenotypes in which lncPVT1 regulates chemoresistance or radioresistance in certain cancer cells, but a detailed analysis of the underlying regulatory mechanisms still needs to be performed. Hence, further research is required to address these gaps. Most therapeutic agents involved in the studies regarding lncPVT1 and chemoresistance are first-line treatments. Second- or third-line therapies are also commonly used in clinical oncology; therefore, it is necessary to investigate the effect of lncPVT1 expression on the efficacy of these therapeutic agents (151). So far, compared with chemoresistance, there have been few studies associating lncPVT1 and radioresistance, and the types of tumors involved are relatively few. Therefore, further research is needed on lncPVT1 and radiotherapy resistance.
Severe acute respiratory syndrome coronavirus 2 (SARS-CoV-2), as a new human coronavirus, has caused the coronavirus disease 2019 (COVID-19) pandemic, bringing significant harm and challenges to all parts of the world (152). In recent years, research on the link between COVID-19 and cancer has also increased. Patients with cancer, especially hematological malignancies, are at higher risk for breakthrough infections and severe consequences. The COVID-19 mRNA vaccine significantly reduces the risk of breakthrough infection in cancer patients (153). In addition, lncPVT1 has been reported to be involved in regulating SARS-CoV-2 infection (154). Therefore, the potential roles of lncPVT1 in cancer patients with COVID-19 is expected to be further investigated.
In conclusion, targeting lncPVT1 has broad therapeutic prospects in oncology. LncPVT1 inhibitors are expected to be developed in the future, which can be used in combination with radiotherapy and chemotherapy to reduce the incidence of chemoresistance and radioresistance and further improve the efficacy of these treatments in patients with malignancies. The detailed molecular mechanisms underlying the lncPVT1-induced regulation of chemoresistance and radioresistance require further study.
Author contributions
All authors listed have made a substantial, direct, and intellectual contribution to the work, and approved it for publication.
Funding
This study was supported in part by grants from the National Natural Science Foundation of China (82003236, to HZ), Zhejiang Provincial Nature Science Foundation of China (Grant number: LGF20H160030 to LG), and Zhejiang Health Science and Technology Project (Grant number: 2019KY280, to LG; 2022KY596, to HZ).
Conflict of interest
The authors declare that the research was conducted in the absence of any commercial or financial relationships that could be construed as a potential conflict of interest.
Publisher’s note
All claims expressed in this article are solely those of the authors and do not necessarily represent those of their affiliated organizations, or those of the publisher, the editors and the reviewers. Any product that may be evaluated in this article, or claim that may be made by its manufacturer, is not guaranteed or endorsed by the publisher.
References
1. Mercer TR, Dinger ME, Mattick JS. Long non-coding rnas: Insights into functions. Nat Rev Genet (2009) 10(3):155–9. doi: 10.1038/nrg2521
2. Cory S, Graham M, Webb E, Corcoran L, Adams JM. Variant (6;15)Translocations in murine plasmacytomas involve a chromosome 15 locus at least 72 kb from the c-myc oncogene. EMBO J (1985) 4(3):675–81. doi: 10.1002/j.1460-2075.1985.tb03682.x
3. Tseng YY, Moriarity BS, Gong W, Akiyama R, Tiwari A, Kawakami H, et al. Pvt1 dependence in cancer with myc copy-number increase. Nature (2014) 512(7512):82–6. doi: 10.1038/nature13311
4. Jin K, Wang S, Zhang Y, Xia M, Mo Y, Li X, et al. Long non-coding rna Pvt1 interacts with myc and its downstream molecules to synergistically promote tumorigenesis. Cell Mol Life Sci (2019) 76(21):4275–89. doi: 10.1007/s00018-019-03222-1
5. Boloix A, Masanas M, Jiménez C, Antonelli R, Soriano A, Roma J, et al. Long non-coding rna Pvt1 as a prognostic and therapeutic target in pediatric cancer. Front Oncol (2019) 9:1173. doi: 10.3389/fonc.2019.01173
6. Martínez-Barriocanal Á, Arango D, Dopeso H. Pvt1 long non-coding rna in gastrointestinal cancer. Front Oncol (2020) 10:38. doi: 10.3389/fonc.2020.00038
7. Ghafouri-Fard S, Omrani MD, Taheri M. Long noncoding rna Pvt1: A highly dysregulated gene in malignancy. J Cell Physiol (2020) 235(2):818–35. doi: 10.1002/jcp.29060
8. Derderian C, Orunmuyi AT, Olapade-Olaopa EO, Ogunwobi OO. Pvt1 signaling is a mediator of cancer progression. Front Oncol (2019) 9:502. doi: 10.3389/fonc.2019.00502
9. Ogunwobi OO, Segura MF. Editorial: Pvt1 in cancer. Front Oncol (2020) 10:588786. doi: 10.3389/fonc.2020.588786
10. Lai SW, Chen MY, Bamodu OA, Hsieh MS, Huang TY, Yeh CT, et al. Exosomal lncrna Pvt1/Vegfa axis promotes colon cancer metastasis and stemness by downregulation of tumor suppressor mir-152-3p. Oxid Med Cell Longev (2021) 2021:9959807. doi: 10.1155/2021/9959807
11. Zhao J, Du P, Cui P, Qin Y, Hu C, Wu J, et al. Lncrna Pvt1 promotes angiogenesis Via activating the Stat3/Vegfa axis in gastric cancer. Oncogene (2018) 37(30):4094–109. doi: 10.1038/s41388-018-0250-z
12. Sun C, Wang P, Dong W, Liu H, Sun J, Zhao L. Lncrna Pvt1 promotes exosome secretion through Ykt6, Rab7, and Vamp3 in pancreatic cancer. Aging (Albany NY) (2020) 12(11):10427–40. doi: 10.18632/aging.103268
13. Wu BQ, Jiang Y, Zhu F, Sun DL, He XZ. Long noncoding rna Pvt1 promotes emt and cell proliferation and migration through downregulating P21 in pancreatic cancer cells. Technol Cancer Res Treat (2017) 16(6):819–27. doi: 10.1177/1533034617700559
14. Zhu Y, Wu F, Gui W, Zhang N, Matro E, Zhu L, et al. A positive feedback regulatory loop involving the lncrna Pvt1 and hif-1α in pancreatic cancer. J Mol Cell Biol (2021) 13(9):676–89. doi: 10.1093/jmcb/mjab042
15. Wei G, Wang Y, Yang G, Wang Y, Ju R. Recent progress in nanomedicine for enhanced cancer chemotherapy. Theranostics (2021) 11(13):6370–92. doi: 10.7150/thno.57828
16. Zhang X, Xie K, Zhou H, Wu Y, Li C, Liu Y, et al. Role of non-coding rnas and rna modifiers in cancer therapy resistance. Mol Cancer (2020) 19(1):47. doi: 10.1186/s12943-020-01171-z
17. Zheng HC. The molecular mechanisms of chemoresistance in cancers. Oncotarget (2017) 8(35):59950–64. doi: 10.18632/oncotarget.19048
18. De Ruysscher D, Niedermann G, Burnet NG, Siva S, Lee AWM, Hegi-Johnson F. Radiotherapy toxicity. Nat Rev Dis Primers (2019) 5(1):13. doi: 10.1038/s41572-019-0064-5
19. Olivares-Urbano MA, Griñán-Lisón C, Marchal JA, Núñez MI. Csc radioresistance: A therapeutic challenge to improve radiotherapy effectiveness in cancer. Cells (2020) 9(7):1651. doi: 10.3390/cells9071651
20. Baskar R, Lee KA, Yeo R, Yeoh KW. Cancer and radiation therapy: Current advances and future directions. Int J Med Sci (2012) 9(3):193–9. doi: 10.7150/ijms.3635
21. Ouellette MM, Zhou S, Yan Y. Cell signaling pathways that promote radioresistance of cancer cells. Diagnostics (Basel) (2022) 12(3):656. doi: 10.3390/diagnostics12030656
22. Suwa T, Kobayashi M, Nam JM, Harada H. Tumor microenvironment and radioresistance. Exp Mol Med (2021) 53(6):1029–35. doi: 10.1038/s12276-021-00640-9
23. Bouleftour W, Rowinski E, Louati S, Sotton S, Wozny AS, Moreno-Acosta P, et al. A review of the role of hypoxia in radioresistance in cancer therapy. Med Sci Monit (2021) 27:e934116. doi: 10.12659/msm.934116
24. Zhou C, Yi C, Yi Y, Qin W, Yan Y, Dong X, et al. Lncrna Pvt1 promotes gemcitabine resistance of pancreatic cancer Via activating Wnt/β-catenin and autophagy pathway through modulating the mir-619-5p/Pygo2 and mir-619-5p/Atg14 axes. Mol Cancer (2020) 19(1):118. doi: 10.1186/s12943-020-01237-y
25. Shi Y, Wang Y, Qian J, Yan X, Han Y, Yao N, et al. Mgmt expression affects the gemcitabine resistance of pancreatic cancer cells. Life Sci (2020) 259:118148. doi: 10.1016/j.lfs.2020.118148
26. Liu YF, Luo D, Li X, Li ZQ, Yu X, Zhu HW. Pvt1 knockdown inhibits autophagy and improves gemcitabine sensitivity by regulating the mir-143/Hif-1α/Vmp1 axis in pancreatic cancer. Pancreas (2021) 50(2):227–34. doi: 10.1097/mpa.0000000000001747
27. Yoshida K, Toden S, Ravindranathan P, Han H, Goel A. Curcumin sensitizes pancreatic cancer cells to gemcitabine by attenuating Prc2 subunit Ezh2, and the lncrna Pvt1 expression. Carcinogenesis (2017) 38(10):1036–46. doi: 10.1093/carcin/bgx065
28. Sun Y, Ren D, Zhou Y, Shen J, Wu H, Jin X. Histone acetyltransferase 1 promotes gemcitabine resistance by regulating the Pvt1/Ezh2 complex in pancreatic cancer. Cell Death Dis (2021) 12(10):878. doi: 10.1038/s41419-021-04118-4
29. You L, Wang H, Yang G, Zhao F, Zhang J, Liu Z, et al. Gemcitabine exhibits a suppressive effect on pancreatic cancer cell growth by regulating processing of Pvt1 to Mir1207. Mol Oncol (2018) 12(12):2147–64. doi: 10.1002/1878-0261.12393
30. Zhang XW, Bu P, Liu L, Zhang XZ, Li J. Overexpression of long non-coding rna Pvt1 in gastric cancer cells promotes the development of multidrug resistance. Biochem Biophys Res Commun (2015) 462(3):227–32. doi: 10.1016/j.bbrc.2015.04.121
31. Zhang XW, Liu L, Zhang XZ, Bo P. Kanglaite inhibits the expression of drug resistance genes through suppressing Pvt1 in cisplatin-resistant gastric cancer cells. Exp Ther Med (2017) 14(2):1789–94. doi: 10.3892/etm.2017.4650
32. Wu C, Hu Y, Ning Y, Zhao A, Zhang G, Yan L. Long noncoding rna plasmacytoma variant translocation 1 regulates cisplatin resistance Via mir-3619-5p/Tbl1xr1 axis in gastric cancer. Cancer Biother Radiopharm (2020) 35(10):741–52. doi: 10.1089/cbr.2019.3342
33. Du P, Hu C, Qin Y, Zhao J, Patel R, Fu Y, et al. Lncrna Pvt1 mediates antiapoptosis and 5-fluorouracil resistance Via increasing Bcl2 expression in gastric cancer. J Oncol (2019) 2019:9325407. doi: 10.1155/2019/9325407
34. Liu E, Liu Z, Zhou Y, Mi R, Wang D. Overexpression of long non-coding rna Pvt1 in ovarian cancer cells promotes cisplatin resistance by regulating apoptotic pathways. Int J Clin Exp Med (2015) 8(11):20565–72.
35. Yi K, Hou M, Yuan J, Yang L, Zeng X, Xi M, et al. Lncrna Pvt1 epigenetically stabilizes and post-transcriptionally regulates Foxm1 by acting as a microrna sponge and thus promotes malignant behaviors of ovarian cancer cells. Am J Transl Res (2020) 12(6):2860–74.
36. Chen Y, Li F, Li D, Liu W, Zhang L. Atezolizumab and blockade of lncrna Pvt1 attenuate cisplatin resistant ovarian cancer cells progression synergistically Via Jak2/Stat3/Pd-L1 pathway. Clin Immunol (2021) 227:108728. doi: 10.1016/j.clim.2021.108728
37. Ping G, Xiong W, Zhang L, Li Y, Zhang Y, Zhao Y. Silencing long noncoding rna Pvt1 inhibits tumorigenesis and cisplatin resistance of colorectal cancer. Am J Transl Res (2018) 10(1):138–49.
38. Fan H, Zhu JH, Yao XQ. Knockdown of long Non−Coding rna Pvt1 reverses multidrug resistance in colorectal cancer cells. Mol Med Rep (2018) 17(6):8309–15. doi: 10.3892/mmr.2018.8907
39. Liu Z, Zhang H. Lncrna plasmacytoma variant translocation 1 is an oncogene in bladder urothelial carcinoma. Oncotarget (2017) 8(38):64273–82. doi: 10.18632/oncotarget.19604
40. Jiang X, Li H, Fang Y, Xu C. Lncrna Pvt1 contributes to invasion and doxorubicin resistance of bladder cancer cells through promoting Mdm2 expression and aurkb-mediated P53 ubiquitination. Environ Toxicol (2022) 37(6):1495–508. doi: 10.1002/tox.23501
41. Chen L, Han X, Hu Z, Chen L. The Pvt1/Mir-216b/Beclin-1 regulates cisplatin sensitivity of nsclc cells Via modulating autophagy and apoptosis. Cancer Chemother Pharmacol (2019) 83(5):921–31. doi: 10.1007/s00280-019-03808-3
42. Wang J, Dong Z, Sheng Z, Cai Y. Hypoxia-induced Pvt1 promotes lung cancer chemoresistance to cisplatin by autophagy Via Pvt1/Mir-140-3p/Atg5 axis. Cell Death Discovery (2022) 8(1):104. doi: 10.1038/s41420-022-00886-w
43. Luo Y, Zhang W, Xu L, Chen Y, Xu Y, Yuan L. Long non-coding rna Pvt1 regulates the resistance of the breast cancer cell line mda-Mb-231 to doxorubicin Via Nrf2. Technol Cancer Res Treat (2020) 19:1533033820980763. doi: 10.1177/1533033820980763
44. Sun ZY, Jian YK, Zhu HY, Li B. Lncrnapvt1 targets mir-152 to enhance chemoresistance of osteosarcoma to gemcitabine through activating c-Met/Pi3k/Akt pathway. Pathol Res Pract (2019) 215(3):555–63. doi: 10.1016/j.prp.2018.12.013
45. Song T, Yan L, Cai K, Zhao T, Xu M. Downregulation of long noncoding rna Pvt1 attenuates paclitaxel resistance in glioma cells. Cancer biomark (2018) 23(3):447–53. doi: 10.3233/cbm-181573
46. Gong R, Li ZQ, Fu K, Ma C, Wang W, Chen JC. Long noncoding rna Pvt1 promotes stemness and temozolomide resistance through mir-365/Elf4/Sox2 axis in glioma. Exp Neurobiol (2021) 30(3):244–55. doi: 10.5607/en20060
47. Shen CJ, Cheng YM, Wang CL. Lncrna Pvt1 epigenetically silences mir-195 and modulates emt and chemoresistance in cervical cancer cells. J Drug Target (2017) 25(7):637–44. doi: 10.1080/1061186x.2017.1307379
48. Yang S, Yuan ZJ, Zhu YH, Chen X, Wang W. Lncrna Pvt1 promotes cetuximab resistance of head and neck squamous cell carcinoma cells by inhibiting mir-124-3p. Head Neck (2021) 43(9):2712–23. doi: 10.1002/hed.26742
49. Pal G, Huaman J, Levine F, Orunmuyi A, Olapade-Olaopa EO, Onagoruwa OT, et al. Long noncoding rna from Pvt1 exon 9 is overexpressed in prostate cancer and induces malignant transformation and castration resistance in prostate epithelial cells. Genes (Basel) (2019) 10(12):964. doi: 10.3390/genes10120964
50. He Y, Jing Y, Wei F, Tang Y, Yang L, Luo J, et al. Long non-coding rna Pvt1 predicts poor prognosis and induces radioresistance by regulating DNA repair and cell apoptosis in nasopharyngeal carcinoma. Cell Death Dis (2018) 9(2):235. doi: 10.1038/s41419-018-0265-y
51. Wang Y, Chen W, Lian J, Zhang H, Yu B, Zhang M, et al. The lncrna Pvt1 regulates nasopharyngeal carcinoma cell proliferation Via activating the Kat2a acetyltransferase and stabilizing hif-1α. Cell Death Differ (2020) 27(2):695–710. doi: 10.1038/s41418-019-0381-y
52. Han Y, Li F, Xie J, Wang Y, Zhang H. Pvt1 mediates cell proliferation, apoptosis and radioresistance in nasopharyngeal carcinoma through regulating mir-515-5p/Pik3ca axis. Cancer Manag Res (2020) 12:10077–90. doi: 10.2147/cmar.S257583
53. Wu D, Li Y, Zhang H, Hu X. Knockdown of lncrna Pvt1 enhances radiosensitivity in non-small cell lung cancer by sponging mir-195. Cell Physiol Biochem (2017) 42(6):2453–66. doi: 10.1159/000480209
54. Wang D, Hu Y. Long non-coding rna Pvt1 competitively binds microrna-424-5p to regulate Carm1 in radiosensitivity of non-Small-Cell lung cancer. Mol Ther Nucleic Acids (2019) 16:130–40. doi: 10.1016/j.omtn.2018.12.006
55. Rawla P, Sunkara T, Gaduputi V. Epidemiology of pancreatic cancer: Global trends, etiology and risk factors. World J Oncol (2019) 10(1):10–27. doi: 10.14740/wjon1166
56. Binenbaum Y, Na'ara S, Gil Z. Gemcitabine resistance in pancreatic ductal adenocarcinoma. Drug Resist Update (2015) 23:55–68. doi: 10.1016/j.drup.2015.10.002
57. Xie W, Chu M, Song G, Zuo Z, Han Z, Chen C, et al. Emerging roles of long noncoding rnas in chemoresistance of pancreatic cancer. Semin Cancer Biol (2020) 83:303–18. doi: 10.1016/j.semcancer.2020.11.004
58. You L, Chang D, Du HZ, Zhao YP. Genome-wide screen identifies Pvt1 as a regulator of gemcitabine sensitivity in human pancreatic cancer cells. Biochem Biophys Res Commun (2011) 407(1):1–6. doi: 10.1016/j.bbrc.2011.02.027
59. Huang M, Zhang D, Wu JY, Xing K, Yeo E, Li C, et al. Wnt-mediated endothelial transformation into mesenchymal stem cell-like cells induces chemoresistance in glioblastoma. Sci Transl Med (2020) 12(532):eaay7552. doi: 10.1126/scitranslmed.aay7522
60. White E, Mehnert JM, Chan CS. Autophagy, metabolism, and cancer. Clin Cancer Res (2015) 21(22):5037–46. doi: 10.1158/1078-0432.Ccr-15-0490
61. Wickström M, Dyberg C, Milosevic J, Einvik C, Calero R, Sveinbjörnsson B, et al. Wnt/β-catenin pathway regulates mgmt gene expression in cancer and inhibition of wnt signalling prevents chemoresistance. Nat Commun (2015) 6:8904. doi: 10.1038/ncomms9904
62. Liang Y, Yang L, Xie J. The role of the hedgehog pathway in chemoresistance of gastrointestinal cancers. Cells (2021) 10(8):2030. doi: 10.3390/cells10082030
63. Zeng X, Ju D. Hedgehog signaling pathway and autophagy in cancer. Int J Mol Sci (2018) 19(8):2279. doi: 10.3390/ijms19082279
64. Ruiz i Altaba A, Mas C, Stecca B. The gli code: An information nexus regulating cell fate, stemness and cancer. Trends Cell Biol (2007) 17(9):438–47. doi: 10.1016/j.tcb.2007.06.007
65. Wang K, Chen D, Qian Z, Cui D, Gao L, Lou M. Hedgehog/Gli1 signaling pathway regulates mgmt expression and chemoresistance to temozolomide in human glioblastoma. Cancer Cell Int (2017) 17:117. doi: 10.1186/s12935-017-0491-x
66. Rodríguez ME, Catrinacio C, Ropolo A, Rivarola VA, Vaccaro MI. A novel hif-1α/Vmp1-Autophagic pathway induces resistance to photodynamic therapy in colon cancer cells. Photochem Photobiol Sci (2017) 16(11):1631–42. doi: 10.1039/c7pp00161d
67. Sergeant G, Vankelecom H, Gremeaux L, Topal B. Role of cancer stem cells in pancreatic ductal adenocarcinoma. Nat Rev Clin Oncol (2009) 6(10):580–6. doi: 10.1038/nrclinonc.2009.127
68. Xu W, Li B, Xu M, Yang T, Hao X. Traditional Chinese medicine for precancerous lesions of gastric cancer: A review. BioMed Pharmacother (2022) 146:112542. doi: 10.1016/j.biopha.2021.112542
69. Digklia A, Wagner AD. Advanced gastric cancer: Current treatment landscape and future perspectives. World J Gastroenterol (2016) 22(8):2403–14. doi: 10.3748/wjg.v22.i8.2403
70. Huang WJ, Ruan S, Wen F, Lu XN, Gu SP, Chen XX, et al. Multidrug resistance of gastric cancer: The mechanisms and Chinese medicine reversal agents. Cancer Manag Res (2020) 12:12385–94. doi: 10.2147/cmar.S274599
71. Yuan CL, Li H, Zhu L, Liu Z, Zhou J, Shu Y. Aberrant expression of long noncoding rna Pvt1 and its diagnostic and prognostic significance in patients with gastric cancer. Neoplasma (2016) 63(3):442–9. doi: 10.4149/314_150825n45
72. Xu MD, Wang Y, Weng W, Wei P, Qi P, Zhang Q, et al. A positive feedback loop of lncrna-Pvt1 and Foxm1 facilitates gastric cancer growth and invasion. Clin Cancer Res (2017) 23(8):2071–80. doi: 10.1158/1078-0432.Ccr-16-0742
73. Waghray D, Zhang Q. Inhibit or evade multidrug resistance p-glycoprotein in cancer treatment. J Med Chem (2018) 61(12):5108–21. doi: 10.1021/acs.jmedchem.7b01457
74. Lu Y, Li CS, Dong Q. Chinese Herb related molecules of cancer-Cell-Apoptosis: A minireview of progress between kanglaite injection and related genes. J Exp Clin Cancer Res (2008) 27(1):31. doi: 10.1186/1756-9966-27-31
75. Zhan YP, Huang XE, Cao J, Lu YY, Wu XY, Liu J, et al. Clinical safety and efficacy of kanglaite® (Coix seed oil) injection combined with chemotherapy in treating patients with gastric cancer. Asian Pac J Cancer Prev (2012) 13(10):5319–21. doi: 10.7314/apjcp.2012.13.10.5319
76. Smyth EC, Nilsson M, Grabsch HI, van Grieken NC, Lordick F. Gastric cancer. Lancet (2020) 396(10251):635–48. doi: 10.1016/s0140-6736(20)31288-5
77. Liu HD, Xia BR, Jin MZ, Lou G. Organoid of ovarian cancer: Genomic analysis and drug screening. Clin Transl Oncol (2020) 22(8):1240–51. doi: 10.1007/s12094-019-02276-8
78. Zou MF, Ling J, Wu QY, Zhang CX. Long non-coding rna Pvt1 functions as an oncogene in ovarian cancer Via upregulating Sox2. Eur Rev Med Pharmacol Sci (2020) 24(14):7571. doi: 10.26355/eurrev_202007_22238
79. Yang Q, Yu Y, Sun Z, Pan Y. Long non-coding rna Pvt1 promotes cell proliferation and invasion through regulating mir-133a in ovarian cancer. BioMed Pharmacother (2018) 106:61–7. doi: 10.1016/j.biopha.2018.06.112
80. El-Khazragy N, Mohammed HF, Yassin M, Elghoneimy KK, Bayoumy W, Hewety A, et al. Tissue-based long non-coding rnas "Pvt1, Tug1 and Meg3" signature predicts cisplatin resistance in ovarian cancer. Genomics (2020) 112(6):4640–6. doi: 10.1016/j.ygeno.2020.08.005
81. Cancer Genome Atlas Research Network. Integrated genomic analyses of ovarian carcinoma. Nature (2011) 474(7353):609–15. doi: 10.1038/nature10166
82. Nowak M, Klink M. The role of tumor-associated macrophages in the progression and chemoresistance of ovarian cancer. Cells (2020) 9(5):1299. doi: 10.3390/cells9051299
83. Gustavsson B, Carlsson G, Machover D, Petrelli N, Roth A, Schmoll HJ, et al. A review of the evolution of systemic chemotherapy in the management of colorectal cancer. Clin Colorectal Cancer (2015) 14(1):1–10. doi: 10.1016/j.clcc.2014.11.002
84. Wu H, Wei M, Jiang X, Tan J, Xu W, Fan X, et al. Lncrna Pvt1 promotes tumorigenesis of colorectal cancer by stabilizing mir-16-5p and interacting with the Vegfa/Vegfr1/Akt axis. Mol Ther Nucleic Acids (2020) 20:438–50. doi: 10.1016/j.omtn.2020.03.006
85. Liu F, Wu R, Guan L, Tang X. Knockdown of Pvt1 suppresses colorectal cancer progression by regulating mir-106b-5p/Fjx1 axis. Cancer Manag Res (2020) 12:8773–85. doi: 10.2147/cmar.S260537
86. He H, Yi L, Zhang B, Yan B, Xiao M, Ren J, et al. Usp24-gsdmb complex promotes bladder cancer proliferation Via activation of the Stat3 pathway. Int J Biol Sci (2021) 17(10):2417–29. doi: 10.7150/ijbs.54442
87. Chen M, Zhang R, Lu L, Du J, Chen C, Ding K, et al. Lncrna Pvt1 accelerates malignant phenotypes of bladder cancer cells by modulating mir-194-5p/Bclaf1 axis as a cerna. Aging (Albany NY) (2020) 12(21):22291–312. doi: 10.18632/aging.202203
88. Tian Z, Cao S, Li C, Xu M, Wei H, Yang H, et al. Lncrna Pvt1 regulates growth, migration, and invasion of bladder cancer by mir-31/ Cdk1. J Cell Physiol (2019) 234(4):4799–811. doi: 10.1002/jcp.27279
89. De Ruysscher D, Faivre-Finn C, Nackaerts K, Jordan K, Arends J, Douillard JY, et al. Recommendation for supportive care in patients receiving concurrent chemotherapy and radiotherapy for lung cancer. Ann Oncol (2020) 31(1):41–9. doi: 10.1016/j.annonc.2019.10.003
90. Lv P, Man S, Xie L, Ma L, Gao W. Pathogenesis and therapeutic strategy in platinum resistance lung cancer. Biochim Biophys Acta Rev Cancer (2021) 1876(1):188577. doi: 10.1016/j.bbcan.2021.188577
91. Xi Y, Shen W, Jin C, Wang L, Yu B. Pvt1 promotes the proliferation and migration of non-small cell lung cancer Via regulating mir-148/Rab34 signal axis. Onco Targets Ther (2020) 13:1819–32. doi: 10.2147/ott.S222898
92. Wang X, Cheng Z, Dai L, Jiang T, Li P, Jia L, et al. Lncrna Pvt1 facilitates proliferation, migration and invasion of nsclc cells Via mir-551b/Fgfr1 axis. Onco Targets Ther (2021) 14:3555–65. doi: 10.2147/ott.S273794
93. Qi G, Li L. Long non-coding rna Pvt1 contributes to cell growth and metastasis in non-Small-Cell lung cancer by regulating mir-361-3p/Sox9 axis and activating Wnt/β-catenin signaling pathway. BioMed Pharmacother (2020) 126:110100. doi: 10.1016/j.biopha.2020.110100
94. Mao Z, Xu B, He L, Zhang G. Pvt1 promotes angiogenesis by regulating mir-29c/Vascular endothelial growth factor (Vegf) signaling pathway in non-Small-Cell lung cancer (Nsclc). Med Sci Monit (2019) 25:5418–25. doi: 10.12659/msm.917601
95. Wang X, Shao QH, Zhou H, Wu JL, Quan WQ, Ji P, et al. Ginkgolide b inhibits lung cancer cells promotion Via beclin-1-Dependent autophagy. BMC Complement Med Ther (2020) 20(1):194. doi: 10.1186/s12906-020-02980-x
96. Feng W, He Y, Xu J, Zhang H, Si Y, Xu J, et al. A meta-analysis of the effect and safety of platinum-based neoadjuvant chemotherapy in treatment of resectable triple-negative breast cancer. Anticancer Drugs (2022) 33(1):e52–60. doi: 10.1097/cad.0000000000001196
97. Wang H, Huang Y, Yang Y. Lncrna Pvt1 regulates Trps1 expression in breast cancer by sponging mir-543. Cancer Manag Res (2020) 12:7993–8004. doi: 10.2147/cmar.S263383
98. Wang L, Wang R, Ye Z, Wang Y, Li X, Chen W, et al. Pvt1 affects emt and cell proliferation and migration Via regulating P21 in triple-negative breast cancer cells cultured with mature adipogenic medium. Acta Biochim Biophys Sin (Shanghai) (2018) 50(12):1211–8. doi: 10.1093/abbs/gmy129
99. Li HL, Wang CP, Zhang Y, Du JX, Han LH, Yang HY. Long non-coding rna Pvt1 facilitates cell migration and invasion by regulating mir-148a-3p and Rock1 in breast cancer. Clin Transl Oncol (2022) 24(5):882–91. doi: 10.1007/s12094-021-02736-0
100. Ge W, Zhao K, Wang X, Li H, Yu M, He M, et al. Iaspp is an antioxidative factor and drives cancer growth and drug resistance by competing with Nrf2 for Keap1 binding. Cancer Cell (2017) 32(5):561–73.e6. doi: 10.1016/j.ccell.2017.09.008
101. Leinonen HM, Kansanen E, Pölönen P, Heinäniemi M, Levonen AL. Role of the Keap1-Nrf2 pathway in cancer. Adv Cancer Res (2014) 122:281–320. doi: 10.1016/b978-0-12-420117-0.00008-6
102. Ritter J, Bielack SS. Osteosarcoma. Ann Oncol (2010) 21 Suppl 7:vii320–5. doi: 10.1093/annonc/mdq276
103. Harrison DJ, Geller DS, Gill JD, Lewis VO, Gorlick R. Current and future therapeutic approaches for osteosarcoma. Expert Rev Anticancer Ther (2018) 18(1):39–50. doi: 10.1080/14737140.2018.1413939
104. Chen C, Xie L, Ren T, Huang Y, Xu J, Guo W. Immunotherapy for osteosarcoma: Fundamental mechanism, rationale, and recent breakthroughs. Cancer Lett (2021) 500:1–10. doi: 10.1016/j.canlet.2020.12.024
105. Song J, Wu X, Liu F, Li M, Sun Y, Wang Y, et al. Long non-coding rna Pvt1 promotes glycolysis and tumor progression by regulating mir-497/Hk2 axis in osteosarcoma. Biochem Biophys Res Commun (2017) 490(2):217–24. doi: 10.1016/j.bbrc.2017.06.024
106. Zhao W, Qin P, Zhang D, Cui X, Gao J, Yu Z, et al. Long non-coding rna Pvt1 encapsulated in bone marrow mesenchymal stem cell-derived exosomes promotes osteosarcoma growth and metastasis by stabilizing erg and sponging mir-183-5p. Aging (Albany NY) (2019) 11(21):9581–96. doi: 10.18632/aging.102406
107. Song XJ, Bi MC, Zhu QS, Liu XL. The emerging role of lncrnas in the regulation of osteosarcoma stem cells. Eur Rev Med Pharmacol Sci (2022) 26(3):966–74. doi: 10.26355/eurrev_202202_28006
108. Park KC, Richardson DR. The c-met oncoprotein: Function, mechanisms of degradation and its targeting by novel anti-cancer agents. Biochim Biophys Acta Gen Subj (2020) 1864(10):129650. doi: 10.1016/j.bbagen.2020.129650
109. Wang K, Zhuang Y, Liu C, Li Y. Inhibition of c-met activation sensitizes osteosarcoma cells to cisplatin Via suppression of the Pi3k-akt signaling. Arch Biochem Biophys (2012) 526(1):38–43. doi: 10.1016/j.abb.2012.07.003
110. Ou A, Yung WKA, Majd N. Molecular mechanisms of treatment resistance in glioblastoma. Int J Mol Sci (2020) 22(1):351. doi: 10.3390/ijms22010351
111. Bi Y, Ji J, Zhou Y. Lncrna-Pvt1 indicates a poor prognosis and promotes angiogenesis Via activating the Hnf1b/Emt axis in glioma. J Cancer (2021) 12(19):5732–44. doi: 10.7150/jca.60257
112. Fu C, Li D, Zhang X, Liu N, Chi G, Jin X. Lncrna Pvt1 facilitates tumorigenesis and progression of glioma Via regulation of mir-128-3p/Grem1 axis and bmp signaling pathway. Neurotherapeutics (2018) 15(4):1139–57. doi: 10.1007/s13311-018-0649-9
113. Li Z, Li M, Xia P, Wang L, Lu Z. Targeting long non-coding rna Pvt1/Tgf-β/Smad by P53 prevents glioma progression. Cancer Biol Ther (2022) 23(1):225–33. doi: 10.1080/15384047.2022.2042160
114. Bazzoli E, Pulvirenti T, Oberstadt MC, Perna F, Wee B, Schultz N, et al. Mef promotes stemness in the pathogenesis of gliomas. Cell Stem Cell (2012) 11(6):836–44. doi: 10.1016/j.stem.2012.09.012
115. Crosbie EJ, Einstein MH, Franceschi S, Kitchener HC. Human papillomavirus and cervical cancer. Lancet (2013) 382(9895):889–99. doi: 10.1016/s0140-6736(13)60022-7
116. Yang JP, Yang XJ, Xiao L, Wang Y. Long noncoding rna Pvt1 as a novel serum biomarker for detection of cervical cancer. Eur Rev Med Pharmacol Sci (2016) 20(19):3980–6.
117. Gao YL, Zhao ZS, Zhang MY, Han LJ, Dong YJ, Xu B. Long noncoding rna Pvt1 facilitates cervical cancer progression Via negative regulating of mir-424. Oncol Res (2017) 25(8):1391–8. doi: 10.3727/096504017x14881559833562
118. Chang ET, Ye W, Zeng YX, Adami HO. The evolving epidemiology of nasopharyngeal carcinoma. Cancer Epidemiol Biomarkers Prev (2021) 30(6):1035–47. doi: 10.1158/1055-9965.Epi-20-1702
119. Tsang CM, Tsao SW. The role of Epstein-Barr virus infection in the pathogenesis of nasopharyngeal carcinoma. Virol Sin (2015) 30(2):107–21. doi: 10.1007/s12250-015-3592-5
120. Sun XS, Li XY, Chen QY, Tang LQ, Mai HQ. Future of radiotherapy in nasopharyngeal carcinoma. Br J Radiol (2019) 92(1102):20190209. doi: 10.1259/bjr.20190209
121. Sun Z, Wang X, Wang J, Wang J, Liu X, Huang R, et al. Key radioresistance regulation models and marker genes identified by integrated transcriptome analysis in nasopharyngeal carcinoma. Cancer Med (2021) 10(20):7404–17. doi: 10.1002/cam4.4228
122. Guo Z, Wang YH, Xu H, Yuan CS, Zhou HH, Huang WH, et al. Lncrna Linc00312 suppresses radiotherapy resistance by targeting DNA-pkcs and impairing DNA damage repair in nasopharyngeal carcinoma. Cell Death Dis (2021) 12(1):69. doi: 10.1038/s41419-020-03302-2
123. Cui M, Chang Y, Fang QG, Du W, Wu JF, Wang JH, et al. Non-coding rna Pvt1 promotes cancer stem cell-like traits in nasopharyngeal cancer Via inhibiting mir-1207. Pathol Oncol Res (2019) 25(4):1411–22. doi: 10.1007/s12253-018-0453-1
124. Aryankalayil MJ, Chopra S, Levin J, Eke I, Makinde A, Das S, et al. Radiation-induced long noncoding rnas in a mouse model after whole-body irradiation. Radiat Res (2018) 189(3):251–63. doi: 10.1667/rr14891.1
125. Köcher S, Rieckmann T, Rohaly G, Mansour WY, Dikomey E, Dornreiter I, et al. Radiation-induced double-strand breaks require atm but not Artemis for homologous recombination during s-phase. Nucleic Acids Res (2012) 40(17):8336–47. doi: 10.1093/nar/gks604
126. Brentnall M, Rodriguez-Menocal L, De Guevara RL, Cepero E, Boise LH. Caspase-9, caspase-3 and caspase-7 have distinct roles during intrinsic apoptosis. BMC Cell Biol (2013) 14:32. doi: 10.1186/1471-2121-14-32
127. Kabakov AE, Yakimova AO. Hypoxia-induced cancer cell responses driving radioresistance of hypoxic tumors: Approaches to targeting and radiosensitizing. Cancers (Basel) (2021) 13(5):1102. doi: 10.3390/cancers13051102
128. Hong B, Lui VW, Hashiguchi M, Hui EP, Chan AT. Targeting tumor hypoxia in nasopharyngeal carcinoma. Head Neck (2013) 35(1):133–45. doi: 10.1002/hed.21877
129. Tomioka A, Tanaka M, De Velasco MA, Anai S, Takada S, Kushibiki T, et al. Delivery of pten Via a novel gene microcapsule sensitizes prostate cancer cells to irradiation. Mol Cancer Ther (2008) 7(7):1864–70. doi: 10.1158/1535-7163.Mct-07-2198
130. Sonnenblick A, Venet D, Brohée S, Pondé N, Sotiriou C. Pakt pathway activation is associated with Pik3ca mutations and good prognosis in luminal breast cancer in contrast to p-mtor pathway activation. NPJ Breast Cancer (2019) 5:7. doi: 10.1038/s41523-019-0102-1
131. Delaney GP, Barton MB. Evidence-based estimates of the demand for radiotherapy. Clin Oncol (R Coll Radiol) (2015) 27(2):70–6. doi: 10.1016/j.clon.2014.10.005
132. Vinod SK, Hau E. Radiotherapy treatment for lung cancer: Current status and future directions. Respirology (2020) 25 Suppl 2:61–71. doi: 10.1111/resp.13870
133. Huang Y, Yang X, Lu Y, Zhao Y, Meng R, Zhang S, et al. Ube2o targets Mxi1 for ubiquitination and degradation to promote lung cancer progression and radioresistance. Cell Death Differ (2021) 28(2):671–84. doi: 10.1038/s41418-020-00616-8
134. Elakoum R, Gauchotte G, Oussalah A, Wissler MP, Clément-Duchêne C, Vignaud JM, et al. Carm1 and Prmt1 are dysregulated in lung cancer without hierarchical features. Biochimie (2014) 97:210–8. doi: 10.1016/j.biochi.2013.10.021
135. Zhang S, Wang W, Wu X, Liu W, Ding F. Mir-16-5p modulates the radiosensitivity of cervical cancer cells Via regulating coactivator-associated arginine methyltransferase 1. Pathol Int (2020) 70(1):12–20. doi: 10.1111/pin.12867
136. Zheng L, Chen J, Zhou Z, He Z. Mir-195 enhances the radiosensitivity of colorectal cancer cells by suppressing Carm1. Onco Targets Ther (2017) 10:1027–38. doi: 10.2147/ott.S125067
137. Marur S, Forastiere AA. Head and neck squamous cell carcinoma: Update on epidemiology, diagnosis, and treatment. Mayo Clin Proc (2016) 91(3):386–96. doi: 10.1016/j.mayocp.2015.12.017
138. Concu R, Cordeiro M. Cetuximab and the head and neck squamous cell cancer. Curr Top Med Chem (2018) 18(3):192–8. doi: 10.2174/1568026618666180112162412
139. Zheng X, Zhao K, Liu T, Liu L, Zhou C, Xu M. Long noncoding rna Pvt1 promotes laryngeal squamous cell carcinoma development by acting as a molecular sponge to regulate mir-519d-3p. J Cell Biochem (2019) 120(3):3911–21. doi: 10.1002/jcb.27673
140. Li X, Ren H. Long noncoding rna Pvt1 promotes tumor cell proliferation, invasion, migration and inhibits apoptosis in oral squamous cell carcinoma by regulating Mir−150−5p/Glut−1. Oncol Rep (2020) 44(4):1524–38. doi: 10.3892/or.2020.7706
141. Teng M, Zhou S, Cai C, Lupien M, He HH. Pioneer of prostate cancer: Past, present and the future of Foxa1. Protein Cell (2021) 12(1):29–38. doi: 10.1007/s13238-020-00786-8
142. Liu J, Li Y, Zhang Q, Lv C, Wang M, Jiao Y, et al. Pvt1 expression is a predictor for poor survival of prostate cancer patients. Technol Cancer Res Treat (2021) 20:1533033820971610. doi: 10.1177/1533033820971610
143. Chang Z, Cui J, Song Y. Long noncoding rna Pvt1 promotes emt Via mediating microrna-186 targeting of Twist1 in prostate cancer. Gene (2018) 654:36–42. doi: 10.1016/j.gene.2018.02.036
144. Sun F, Wu K, Yao Z, Mu X, Zheng Z, Sun M, et al. Long noncoding rna Pvt1 promotes prostate cancer metastasis by increasing Nop2 expression Via targeting tumor suppressor micrornas. Onco Targets Ther (2020) 13:6755–65. doi: 10.2147/ott.S242441
145. Liu HT, Fang L, Cheng YX, Sun Q. Lncrna Pvt1 regulates prostate cancer cell growth by inducing the methylation of mir-146a. Cancer Med (2016) 5(12):3512–9. doi: 10.1002/cam4.900
146. Weaver BA, Cleveland DW. Decoding the links between mitosis, cancer, and chemotherapy: The mitotic checkpoint, adaptation, and cell death. Cancer Cell (2005) 8(1):7–12. doi: 10.1016/j.ccr.2005.06.011
147. Li MY, Tang XH, Fu Y, Wang TJ, Zhu JM. Regulatory mechanisms and clinical applications of the long non-coding rna Pvt1 in cancer treatment. Front Oncol (2019) 9:787. doi: 10.3389/fonc.2019.00787
148. Onagoruwa OT, Pal G, Ochu C, Ogunwobi OO. Oncogenic role of Pvt1 and therapeutic implications. Front Oncol (2020) 10:17. doi: 10.3389/fonc.2020.00017
149. Beck-Engeser GB, Lum AM, Huppi K, Caplen NJ, Wang BB, Wabl M. Pvt1-encoded micrornas in oncogenesis. Retrovirology (2008) 5:4. doi: 10.1186/1742-4690-5-4
150. Wang W, Zhou R, Wu Y, Liu Y, Su W, Xiong W, et al. Pvt1 promotes cancer progression Via micrornas. Front Oncol (2019) 9:609. doi: 10.3389/fonc.2019.00609
151. Ogunwobi OO, Kumar A. Chemoresistance mediated by cerna networks associated with the Pvt1 locus. Front Oncol (2019) 9:834. doi: 10.3389/fonc.2019.00834
152. Gao Z, Xu Y, Sun C, Wang X, Guo Y, Qiu S, et al. A systematic review of asymptomatic infections with covid-19. J Microbiol Immunol Infect (2021) 54(1):12–6. doi: 10.1016/j.jmii.2020.05.001
153. Song Q, Bates B, Shao YR, Hsu FC, Liu F, Madhira V, et al. Risk and outcome of breakthrough covid-19 infections in vaccinated patients with cancer: Real-world evidence from the national covid cohort collaborative. J Clin Oncol (2022) 40(13):1414–27. doi: 10.1200/jco.21.02419
Keywords: PVT1, lncRNA, chemoresistance, radioresistance, cancer
Citation: Yao W, Li S, Liu R, Jiang M, Gao L, Lu Y, Liang X and Zhang H (2022) Long non-coding RNA PVT1: A promising chemotherapy and radiotherapy sensitizer. Front. Oncol. 12:959208. doi: 10.3389/fonc.2022.959208
Received: 01 June 2022; Accepted: 30 June 2022;
Published: 29 July 2022.
Edited by:
Aamir Ahmad, University of Alabama at Birmingham, United StatesReviewed by:
Qianqian Song, Wake Forest School of Medicine, United StatesRanran Zhang, Penn Medicine, United States
Copyright © 2022 Yao, Li, Liu, Jiang, Gao, Lu, Liang and Zhang. This is an open-access article distributed under the terms of the Creative Commons Attribution License (CC BY). The use, distribution or reproduction in other forums is permitted, provided the original author(s) and the copyright owner(s) are credited and that the original publication in this journal is cited, in accordance with accepted academic practice. No use, distribution or reproduction is permitted which does not comply with these terms.
*Correspondence: Haibo Zhang, emhiZG9jdG9yIEAxNjMuY29t; Xiaodong Liang, bHhkY3RvcG9uZUBzaW5hLmNvbQ==
†These authors have contributed equally to this work