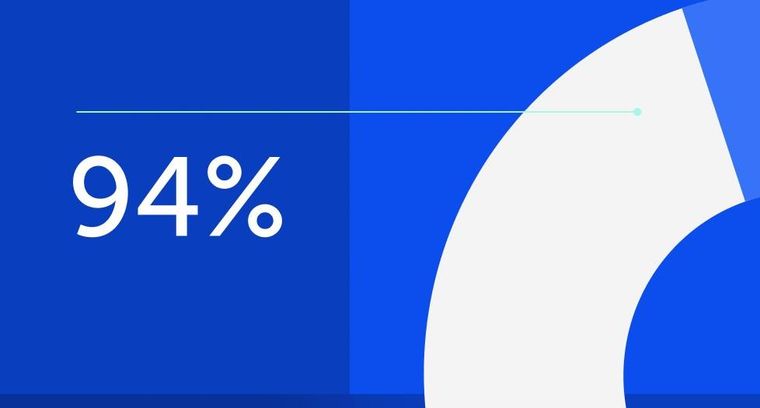
94% of researchers rate our articles as excellent or good
Learn more about the work of our research integrity team to safeguard the quality of each article we publish.
Find out more
REVIEW article
Front. Oncol., 02 September 2022
Sec. Cancer Molecular Targets and Therapeutics
Volume 12 - 2022 | https://doi.org/10.3389/fonc.2022.957370
This article is part of the Research Topic365 Days of Progress In Cancer Molecular Targets and TherapeuticsView all 23 articles
Acute myeloid leukemia (AML) is a malignant proliferative clonal disease, characterized by a wide spectrum of molecular alterations. Although targeted therapy for AML has resulted in pronounced achievements in the past decade, clinical resistance caused by mutations in targeted oncogenes has been observed. A subset of patients with AML still requires the treatment of refractory and relapsed diseases. The field of targeted therapy in AML still presents many challenges. More studies are needed to overcome those obstacles. Through the CiteSpace tool, we can gain a systematic understanding of the current achievements in targeted therapy in AML, and we can predict hot research topics. Relevant publications from the Web of Science Core Collection were retrieved, and the acquired data were analyzed by CiteSpace to identify and predict trends and research hotspots in this field. We found that research on AML had focused on venetoclax resistance, novel therapies (such as targeting epigenetic modification), and FLT3 mutation in the past decade. The clustering of keywords suggested interest in cell-targeted therapy (chimeric antigen receptor T cells and natural killer cells), signaling pathways (mTORC1), epigenetic therapies, and leukemic stem cells (LSCs). Drug resistance causing failure and relapse of treatment has raised increasing concern, and LSCs play a key role in relapsed AML, which represent an important direction for identifying targets for AML treatment in the future.
Acute myeloid leukemia (AML) is a heterogeneous disease caused by various gene mutations and cytogenetic abnormalities that affect the differentiation and proliferation of myeloid lineage cells (1). Advances in genomic investigations over the past 10 years have dramatically improved our understanding of mutated genes in AML and have allowed the tailoring of therapeutic strategies targeting mutated genes (2).
Several genes are recurrently mutated in AML, such as internal tandem duplication (ITD) of FMS-like tyrosine kinase 3 (FLT3-ITD), and mutations in isocitrate dehydrogenase (IDH1/2), nucleophosmin (NPM1), and/or CCAAT/enhancer binding protein alpha (CEBPA). As predictive biomarkers, these are indicators of prognosis and, in turn, can guide targeted therapeutic strategies, because patients harboring these specific mutations do not benefit from traditional chemotherapy (3, 4). Fortunately, tremendous progress has been made in deciphering the molecular pathogenesis of AML, enabling the development of target drugs, such as the introduction of small-molecule inhibitors of FLT3, IDH1/IDH2, and BCL-2. For example, the presence of ITDs in the FLT3 receptor tyrosine kinase gene has long been known to confer a poor prognosis in patients with AML (5). The approval of multikinase FLT3 inhibitor (FLT3i) midostaurin with induction therapy for newly diagnosed FLT3mut AML, and a more specific and potent FLT3i gilteritinib as monotherapy for relapsed/refractory (R/R) FLT3mut AML, has improved outcomes in patients with FLT3-mutated AML (4, 5).
However, targeted therapy in AML still faces challenges (6). Clinical resistance caused by mutations in the targeted oncogene has been observed (7–9). Furthermore, most cases of AML are without a targetable mutation (10). Many efforts have been made to explore new targets and develop the corresponding therapies. Numerous novel approaches that aim to achieve a new goal are currently being actively pursued.
To grasp the scope of research in this area, bibliometric analysis was applied to analyze the progress of targeted therapy in AML. Bibliometric analysis is a method used to analyze enormous amounts of heterogeneous literature. It can be applied to estimate the impact of research areas and to identify emerging trends (11). CiteSpace is a bibliometric tool, which aimed to analyze and visualize trends and patterns in the scientific literature, and presents the structure and distribution of current scientific knowledge (12). CiteSpace can be applied to visualize research frontiers, the knowledge base, and time spans, as well as the literature that has played a key role in research evolution. It focuses on finding critical points in the development of a field or a domain, especially intellectual turning points and pivotal points (13). The visual analytic tools applied in this review supplement traditional review, and survey articles and the findings are valuable for identifying critical developments from a vast number of published studies. In this review, we examine the evolution of targeted therapy in AML and provide a critical perspective on the clinical development of a variety of targeted treatments.
The publications were obtained from the Web of Science Core Collection database (WoSCC). The strategy used during the search was Topic search #1 = (“acute myeloid leukemia” OR “AML”) AND Topic search #2 = (“targeted therapy*”), and then, the results were refined by [Document Types = (Articles or Review) Timespan: 2012-01-01 to 2021-12-31]. A total of 4,205 results were found. After data cleaning, duplicate publications were removed by CiteSpace v.5.8.R3; and ultimately, 4,150 total unique records were used in the final analysis. All records were then imported into CiteSpace v.5.8.R3.
The time slices for analysis were set at 1 year, and the sources selected included all publication details—title, abstract, supplementary keywords and author keywords, selected keywords, author, institution, country for the node, selected g-index for the threshold, and the labels of clusters chosen by the log-likelihood ratio (LRR) test method algorithm—were used in the subsequent analysis. Pathfinder was selected for fine-tuning the setting to highlight key points. Betweenness centrality (BC) >0.1 was considered as pivot nodes. Modularity (Q) >0.3 was considered reasonable for the group. Silhouette >0.5 was considered homogeneity clusters. BC can be used to partially assess the influence of each node in the network (14). Usually, a node with a BC >0.1 is displayed as a purple ring, whose size is associated with the transformative potential of a scientific contribution (15). In addition, cluster analysis was used as another important approach to easily analyze knowledge networks in CiteSpace. More specifically, terms are classified according to their similarity and scored by specific algorithms, and then, the term with the highest score of each cluster is selected as the label of the cluster (16, 17).
The R programming language (version 4.1.0) was used for data visualization (packages ggplot2, tidyverse, ggthemes, ggsci, maps, patchwork, and RColorBrewer).
The annual number of published articles is a significant indicator for studying research trends in the field and reflects the pace of subject knowledge (18). We found a total of 129,587 citations for AML, with 83,287 articles cited, and an h-index of 142 in the field of targeted therapy in AML. The proportion studies investigating targeted therapies published annually in overall AML treatment are visually displayed in Figure 1. The number of targeted therapy documents in AML has increased annually between 2012 and 2021. Furthermore, the proportion of targeted therapy in total treatment has gradually increased, with the number of articles growing much faster.
Figure 1 Annual publishing and proportion trends. (A) Trends in the number of publications per year on general research on AML treatment. (B) Proportion of publications about research for different AML treatment in 2012. (C) Proportion of publications about research into different AML treatment in 2021.
Visualized knowledge maps and institutional networks can provide information on the cooperative relationship between different research teams and countries (19). An analysis of the country distribution of these publications indicates that the United States, China, and Germany ranked the top three in the number of citations to articles. The United States (BC = 0.19) and Germany (BC = 0.14) were the countries that are most frequently associated with co-authored articles due to the highest BC (Figures 2A, B).
Figure 2 Country and institution analysis of targeted therapy in AML. (A) Top 10 countries for publications on the world map. (B) The network of countries for co-authored articles on targeted therapy in AML. Nodes represent publications from countries. The more frequently the publications of countries are cited, the larger is the size of the node. Links between nodes describe a co-occurrence or a co-citation between these nodes, and their thickness indicates the strength of these correlations: the thicker the line, the closer is the connection between them. The node color and link color indicate different clusters; the nodes that are of the same color belong to the same closely related cluster. (C) The network of institutions.
The network of institutions participating in publications is visualized in Figure 2C. The MD Anderson Cancer Center of the University of Texas, Memorial Sloan Kettering Cancer Center, Dana-Farber Cancer Institute, Harvard Medical School, and Harvard University ranked the top five institutions for the number of articles published. High BC values were obtained from the Dana-Farber Cancer Institute (BC = 0.12) and Harvard Medical School (BC = 0.10), which were also in different clusters.
BC measures the extent to which a node plays a bridging role or influence in a network. Specifically, BC measures the extent that the node falls within the shortest path between other pairs of nodes in a network. If the author plays a bridge that makes connections with other authors, then author’s BC value will high. The more an A depends on B to make connections with other people, the higher that B’s BC value (20). In particular, Lars Bullinger (BC = 0.29) and Ross L. Levine (BC = 0.22) had extensive contact with other researchers. Moreover, Ross L. Levine, Farhad Ravandi, and Guillermo Garcia-Manero were included the top three categories of citations (Figure 3A). In Figure 3B, cluster groups can be observed. The connection density between these groups was higher than that between the other nodes. The nodes within each group were uniform, and the number of publications and BC of co-occurring subject categories in AML are shown in Figure 3C.
Figure 3 Co-author and category analysis. (A) Co-author network. The circle in (A) indicates the author; the larger the size of the circle, the higher the number of citations of the author. (B) Clusters of the co-author network. (C) Top 10 topics for publications. The right axis is the publication, and the left axis is the BC value.
As a highly condensed summary of the paper’s content, keywords can be used to summarize the topics of the paper simply and directly. When the co-occurrence network of the keywords was dense, we applied Pruning functions to select important connections to highlight the key aspects of the network. The co-occurrence of targeted therapy keywords in AML and related research can be visualized in Figure 4A. Figure 4B shows the top 14 keyword clusters based on the LLR algorithm. This information, which consisted of highly cited and representative terms, in each group is summarized in Table 1. Burst detection is also a feature of CiteSpace, and a positive node after burst detection indicates a sharp change in its frequency of citations over a short period of time (15). Such nodes usually suggest a shift in a certain field of research and are indicated in red on the knowledge map. The identification of a research focus was based on the keyword co-occurrence network. The top 25 keywords with the strongest citation bursts relative to targeted therapy in AML in the last 10 years are shown in Figure 4C.
Figure 4 Co-occurrence and clustering of keywords. (A) Network of main keywords in publications. The circle in (A) indicates the keyword; the larger the size of the circle, the higher the frequency of the keyword. Purple circles represent the node of BC > 0.1: purple circles are thicker and the BC is higher. (B) Timeline view of keywords. Each horizontal line represents a cluster; the circular nodes on the line represent the top three keywords with the highest frequency of occurrence in this time slice. The timeline is shown at the top of the figure, and the year corresponding to the node is its publication time. The link between nodes represents the co-citation relationship. (C) The 25 keywords with the strongest citation bursts. The blue line denotes the time axis, whereas the red segment on the blue time axis shows the burst detection, indicating the start year, end year, and burst duration.
The identification of core literature in a certain field depends on the frequency of citations, and references with the highest cited frequency, namely, high-impact publications, are usually the focus of researchers. The top 10 cited references with the highest number of citations are shown in Table 2. The themes of references with the highest frequency of citations are divided into three parts: guidelines (diagnosis and management of 2017 ELN recommendations, and 2016 WHO classification revision from 2016 WHO); reviews of prognostic relevance of genomic classification and epigenetic landscapes (DNMT3A mutations) in AML; and targeted drugs for AML (midostaurin plus chemotherapy for FLT3 mutation, and enasidenib for relapsed or refractory AML).
Figures 5A, B shows the co-citation network and the timeline view of reference sources on targeted therapy in AML and related studies. The timeline view is a visualization method that combines clustering and time-slicing techniques. Items are ranked according to their early or late appearance after clustering, displaying both the topic distribution in the field and illustrating the trends and interconnections of research topics over time. The references with the highest BC were Wang F 2013 (BC = 0.15), followed by Papaemmanuil E 2016 (BC = 0.12), Dombret H 2015 (BC = 0.10), Patel JP, 2012 (BC = 0.10), and Welch JS, 2016, (BC = 0.10), namely, these are the critical research authors driving research on the development of targeted therapy in AML. In Figure 5B, a straight line at the same horizontal position indicates all the references belonging to the group, with the group label located at the right-hand end of the line. Each cluster represents the fundamental knowledge of the underlying specialty.
Figure 5 Co-citation network and timeline view of references. (A) Reference co-citation network. The circles in Figure 4A indicate references. (B) Timeline view of references. The circular nodes on the line represent the three most cited references in this time slice.
As can be seen in Figure 5B, research on AML-targeted therapy focused on MLL-rearranged leukemia (cluster #1), TET2 mutations (cluster #9), and DNA methyltransferase cluster #11) from 2007 to 2013. Research on targeted therapy in AML shifted to venetoclax resistance (cluster #0), novel therapy (cluster #3), and FLT3 mutations (cluster #4) from 2013 to 2021. The largest cluster focused on venetoclax resistance. The information, which consisted of highly cited and representative terms, in each group is shown in Table 3.
Targeted therapy in AML has received increasing attention. In the past decade, the number of publications in this field has increased rapidly. Through the co-authored network analysis of countries and institutions, we found that the United States and Germany were the representative countries with the highest BC and were found in different clusters, surrounded by other countries. The MD Anderson Cancer Center of the University of Texas, the Memorial Sloan Kettering Cancer Center, the Dana-Farber Cancer Institute, and Harvard Medical School, and Harvard University ranked the top five in the number of articles published.
Ross L. Levine, Farhad Ravandi, and Guillermo Garcia-Manero were the top three most cited authors. We observed that Lars Bullinger and Ross L. Levine had extensive contact with other researchers. Farhad Ravandi and Guillermo Garcia-Manero collaborated closely and specialized in state-of-the-art treatments for leukemia at The University of Texas MD Anderson Cancer Center (21). The research field of the co-author could be determined from the clusters of label names. Related fields involved the following topics: leukemic transformation, clonal hematopoiesis (CH), myelodysplastic syndrome (MDS), and FLT3 study. Of these, the year of CH clustering was the most recent. We also noticed that the following categories were involved in driving the development of the field: biochemistry and molecular biology, pharmacology and pharmacy, immunology, applied microbiology and biotechnology, and chemistry. There are various degrees of interrelatedness between targeted therapy in AML and different categories, namely, the field of targeted therapy in AML is innovative and interdisciplinary.
We can discover topic distribution in the field and trends of research topics over time. Through the co-citation timeline, we found that research on targeted therapy in AML had changed to venetoclax resistance (cluster #0), novel therapy (cluster #3), and FLT3 mutation (cluster #4) over the past decade. The clustering of keywords focused on cell-targeted therapy [chimeric antigen receptor T cells (CAR-T) and natural kill cells], signaling pathways (mTORC1), epigenetic therapies, and leukemic stem cells (LSCs). Combined with clustering of keywords and cited references, it is obvious that LSCs appeared not only in clustering of keywords but also in clustering of cited references, such as the largest cluster representing venetoclax resistance.
The key term “FLT3 inhibitor” was present in a cluster of co-references and in the keywords having the strongest citation burst, respectively. Up to 2017, the European Leukemia-Net (ELN) adjusted and updated cytogenetic and genomic mutations to the classification based on relative evidence. ELN categories were developed to correlate with genetic abnormalities having a clinical prognostic impact. The categories of these guidelines are divided into favorable, intermediate, and adverse. The adverse categories included FLT3-ITD with a high allelic ratio (22). FLT3 mutations associated with an adverse prognosis have been identified in approximately one-third of patients with AML and represent an attractive therapeutic target.
The clinical development of FLT3 inhibitors is one of the most active fields in AML (23). The standard first-line treatment for AML had not changed for more than 45 years (24). With the advent of FLT3 inhibitors, the treatment armamentarium for FLT3-mutated AML has begun to expand. First-generation agents, such as midostaurin, sorafenib, and lestaurtinib, are broad-spectrum tyrosine kinase inhibitors. Midostaurin became the first targeted therapy approved by the United States Food and Drug Administration (FDA) for FLT3-mutated AML in 2017 (25, 26). In the international randomized phase III RATIFY trial, the multikinase inhibitor midostaurin significantly improved overall and event-free survival in patients aged 18 to 59 years of age with FLT3-mutated AML (27). Data from a phase II single-arm trial have provided evidence that midostaurin also improves the outcome of patients of 60 to 70 years of age with FLT3-ITD positive AML (28). The National Comprehensive Cancer Network Clinical Practice Guidelines recommend clinic trails for patients harboring molecular mutations. When a complete remission (CR) is observed, enrollment in a clinical trial is recommended for patients. Other recommendations include intermediate dose cytarabine and midostaurin for patients with FLT3-mutation–positive AML (28, 29). In addition, next-generation FLT3 inhibitors, such as gilteritinib, quizartinib, and crenolanib, are more selective and have shown promising activity as single agents in early phase trials (30).
Although considerable progress has been made in the treatment of AML by targeting FLT3, many challenges still remain. Many resistance mechanisms have been identified with FLT3 inhibitors, but not all have been fully elucidated. These resistance patterns render FLT3-targeted molecules ineffective in many ways, making them difficult to overcome. Furthermore, although several FLT3 inhibitor studies have included maintenance therapy (31), additional studies are still needed to confirm these findings.
Aberrations of DNA methylation also rank among the most frequent alterations observed in patients with AML. Recurrent somatic alterations in myeloid malignancies of key proteins involved in DNA methylation have highlighted the importance of epigenetic regulation of gene expression in the initiation and maintenance of various malignancies (32).
DNMT3A is one of several epigenetic modifiers identified as recurrently mutated in AML. DNMT3A mutations have also been identified in patients with MDSs (31) and myeloproliferative neoplasms (33) and are associated with a greater likelihood of progression to AML. In fact, in some studies, the same DNMT3A mutation as the antecedent hematologic disorder is identified in secondary AML, suggesting that these mutations may be an early event in malignant clonal evolution (34). These observations are further reinforced by recent findings demonstrating that recurrent DNMT3A mutations are frequently present in a pool of preleukemic clonal hematopoietic stem cells (HSCs) from which AML develops (35). These HSCs have a competitive multilineage repopulation advantage over wild-type HSCs and also have been demonstrated to persist after chemotherapy, thus acting as a reservoir for therapeutic resistance (36). Epigenetic alterations result in changes in gene expression in the absence of modifications of the relevant DNA sequence. Such alterations have the potential to promote stem cell renewal and alter progenitor cell differentiation (4, 37).
In other citations, TP53 mutations and decitabine treatment have a high BC value in AML and MDS. With more and more researchers focusing on the role of DNA methylation in AML, decitabine (5-aza-2-deoxycytidine), a strong specific inhibitor of DNA methylation, is commonly used as a single agent to treat patients with MDS and older aged patients with AML (38). However, response rates are low. Extended treatment exposure to decitabine (administered on days 1 through 10 of the 28-day cycles instead of on days 1 through 5) shows an improved response rate (ranging from 40% to 64%). Patients with AML and MDS presenting cytogenetic abnormalities associated with unfavorable risk, TP53 mutations, or both had favorable clinical responses and robust (but incomplete) mutation clearance after receiving serial 10-day courses of decitabine. They achieved overall survival rates similar to those among patients with AML who had an intermediate-risk cytogenetic profile and who also received serial 10-day courses of decitabine (39).
Targeted epigenetic therapies are effective therapies in advanced preclinical and early clinical development (40). Isocitrate dehydrogenases (IDHs) are present in 15%–30% of AML patients (41). If mutations occur in IDH1 and IDH2, then it would leads to gene hypermethylation, resulting in cellular proliferation, aberrant gene expression, and the inhibition of myeloid differentiation (42). It is reported that co-occurrence of IDH and TET methylcytosine dioxygenase 2 (TET2) mutations leads to DNA hypermethylation, contributing to leukemogenesis (41, 43, 44). IDH1/2 inhibitor interrupts epigenetic changes. Recently (45), IDH inhibitors have been approved by the FDA: the IDH1 inhibitor ivosidenib (AG-120) and the IDH2 inhibitor enasidenib (AG-221) (43).
Advances in genomics, epigenetics, and drug discovery have led to the development of several potential novel therapeutic agents, many of which are being investigated in ongoing clinical trials. Additional studies will be necessary to determine how best to incorporate these novel agents into the routine clinical treatment of AML (10). The epigenetic therapies for AML might be one of the most important future treatment options (46, 47).
Mixed lineage leukemia (MLL) family, also named the human KMT2 family, due to the role of the first member KMT2A was found in this disease. Recent exome sequencing studies have revealed that the KMT2 genes are among the most frequently mutated genes in many types of human cancers (48). Rearrangements in the MLL gene cause aggressive AML leukemias that follow an aggressive clinical course with poor response to conventional chemotherapy and frequent early relapse (49).
A new menin-MLL inhibitor (VTP-50469) appears to promote leukemia cell differentiation through direct effects on the HOX cofactor MEIS1 [HOX expression has been shown to be essential to maintain the leukemic phenotype of MLLr (50)]. The MEIS1 HOX cofactor plays a key role in maintaining a functional HOX network in AML (51)), paving the way for clinical trials (10). VTP-50469 not only induced loss of Meis1 expression and significant differentiation of cytosolic NPM1(NPM1c) leukemic cells but also prevented long-term engraftment and subsequent transformation of NPM1c-GMPs into leukemic cells after secondary transplantation in mice. Furthermore, the inhibitor was identified to suppress growth and induced differentiation in human NPM1c AML cells in patient-derived xenograft models (52).
In addition, there are other promising novel drugs: the DOT1L inhibitor pinometostat is activated in KMT2A-rearranged AML, KO-539, and SNDX-5613 (both are menin inhibitors) useful for KMT2Ar and NPM1-mutated AML (10). For example, in a phase I trial, KO-539 may be active in patients with AML. The agent induced CRs in two patients with relapsed/refractory disease and showed signs of activity in several patients (53).
The increasing availability of high-throughput genomic technologies in clinical settings allows a more accurate diagnosis of MLL-rearranged leukemia, which may provide an individual therapeutic strategy in time. The menin-MLL inhibitor may become a promising preclinical drug and worth investigating in the future, which will benefit patients with poor response to conventional chemotherapy and frequent early relapse.
Recently, research has focused on LSCs in AML (54). Mounting evidence shows that LSCs are the key drivers of relapse in AML (55).
Relapse is a major problem in AML. Although chemotherapy can achieve CR in most patients with AML, approximately two-thirds of patients relapse within 18 months (56). The underlying reason for treatment failure is increasingly attributed to the presence of a drug-resistant subpopulation of AML cells, especially leukemia-initiating cells or LSCs (57). Most HSCs are usually in a state of quiescence. A subpopulation of AML cells is the same as HSCs, which means that LSCs also acquire quiescence. Quiescence is an important contributor to LSC drug resistance, as conventional chemotherapeutics target dividing cells (58).
The clonal representation of AML at the time of disease relapse reflects the continued evolution of LSC in many patients during remission. At relapse, minor clones present at diagnosis can emerge as dominant ones, or founder clones can re-emerge with new subclonal structures (59, 60). Even in rare cases of late relapse, residual LSCs from founder clones are the usual cause (61). In many reported cases, new mutations, particularly transversions, are present in relapsed clones (59, 60), suggesting that chemotherapy itself induces DNA damage that accelerates LSC clonal (62).
Treatment regimens targeting the quiescent LSC population or targeting niche-driven drug resistance are beginning to emerge. For example, combination drug treatments where the first treatment aims to activate quiescent LSCs or remove them from the quiescence driving niche, such as CXCR4 antagonists or E-selectin inhibitors that potentially block CD44 activation, combined with a cytotoxic drug, hold promise (63, 64). The differences between LSC and HSC quiescence are an important direction for the identification of targets for AML treatment in the future.
The number of gene mutations increases with age. Strong evidence in AML points to the origins of LSCs in preleukemic cells that arise through the sequential accumulation of somatic DNA mutations in HSCs (65, 66). Among those mutations, one may arise that confers a fitness advantage for a cell. When this process occurs in the hematopoietic system, a substantial proportion of circulating blood cells can derive from a single mutated stem cell, which is called “clonal hematopoiesis” or CH (56, 67).
Early mutations enhance or acquire self-renewal potential and differentiation impairment (68, 69), both of which can lead to variably expanded clonal populations of preleukemic HSCs in patients (60, 70). Late mutations in molecules within signaling pathways (for example, FLT3) promote proliferation, impose a full differentiation block, and drive the development of AML (71).
Previous research based on large samples found that CH is largely the result of mutations in fixed “early” mutational events: The genes that encode epigenetic modifiers, DNMT3A and TET2, are the two most common mutations; the third most commonly mutated gene was ASXL1, whereas mutations in splicing factors (SF3B1, SRSF2, PRPF8, and U2AF1) were also frequent (72).
Cancer-free individuals with somatic mutations in a cancer-associated variant (with a variant allele fraction equal or greater than 2%) should be considered to have CH of indeterminate potential (CHIP) (56). The most common mutations in CHIP are also recurrent drivers of AML. People with CHIP would be likely to develop AML, because they have the “first hit” needed for malignant transformation. Mutations in DNMT3A, ASXL1, IDH1/2, and TET2 are often acquired at the early stage of the “two-hit hypothesis” (73–75). To refine the risk estimates for developing AML associated with CH, two groups have conducted studies with a large population having several years of follow-up (76, 77). The results indicated that individuals with antecedent CH had an approximately ~3- to 5-fold increased risk of developing AML in subsequent years (72).
The research into CH may be a promising field and worth focusing on. Such research could provide a theory for screening for people at especially high risk of transformation in the future. Additional studies are needed to understand and discover CHIP. Most importantly, it is necessary to find ways to reverse the pathogenic effects of CHIP. Ideally, drugs that can suppress mutant clones in the future should be identified, which could block malignant transformation in the “first hit” state to some extent.
Recently, with the development of immunotherapy, CAR-T therapy targeting AML cells is undergoing active development (78). The treatment strategy for AML is shifting from being limited to two selections (cytotoxic chemotherapy followed by hematopoietic stem cell transplant or hypomethylating agent) to the availability of various novel target therapies (45).
After the great success in B cell malignancies, scientists turn to focus on CAR products that could target AML; however, there has been hindered by several obstacles. Numerous antigens are being investigated to find the ideal AML target, one that is expressed solely by the AML cell, including the LSCs, and that is a driver for AML proliferation. The majority of CAR T or NK cell antigens are cell surface antigens, which are commonly expressed by normal HSCs. Targeting intracellular antigens with CAR T or NK cells is a much more laborious task that involves expression of the intracellular antigen, or segments of the antigen, on the cell surface. FLT3, transmembrane receptor tyrosine kinase, has a vital role in maintaining normal HSC and progenitor cell function, including proliferation and differentiation. Wang et al. described potent in vitro cytotoxicity of FLT3 CAR Ts in AML cell lines, especially in cells harboring the FLT3-ITD mutation (79). Surprisingly, the growth of normal CD34+ HSC was not inhibited by the CARs. Administration of the anti-FLT3 CAR also prolonged survival of mice in a human FLT3+ AML xenograft mouse model.
As we can observe, the largest cluster in the co-citation network of references is venetoclax resistance. Drug resistance causing treatment failure and relapse raises increasing concern. Despite the discovery of multiple molecular and targeted therapeutic targets and the ongoing development of various targeted therapies, there are still major challenges for resistance to targeted drugs.
Because of the genomic and/or epigenetic complexity of AML, with clonal heterogeneity and multiple lesions already present at diagnosis and subsequent further evolution throughout the course of the disease that often leads to the emergence of additional subclones with different resistance mechanisms, new targeted therapies are likely to have only moderate activity. When venetoclax is used as a single agent, therapeutic resistance inevitably evolves, typically within weeks or months.
The true anti-leukaemic potential of venetoclax was revealed only in combination with other agents, resulting in its approval in combination with hypomethylating agents or low-dose cytarabine, in elderly AML, but not as a monotherapy (80, 81). We have learned that the efficacy is markedly enhanced and might become evident only when the use of these agents is combined with other therapies, such as standard of care chemotherapies or HMA. Therefore, how to overcome drug resistance will become a new topic in the future.
There were some limitations in this study. First, our analysis was based on articles from the WoSCC database. Because of the restriction of the program, we only included Web of Science data. However, had more databases been included, a broader coverage of studies would have been provided. Second, because of the restriction of the program, the study interval was not extensive, with the extracted articles published from 2012 to 2021.
This review has revealed that targeted therapy for AML has developed rapidly over the last decade. Through analysis of clusters (co-author, keywords, and cited references), we defined the topic distribution in the field and the trends in research topics over time. The research of targeted therapy in AML has shifted to venetoclax resistance, novel therapy (such as targeting epigenetic modification), and FLT3 mutation during the past decade. The clustering of keywords focused on cell-targeted therapy (CAR-T and natural killer cells), signaling pathways (mTORC1), epigenomic and epigenetic targeted therapies, and LSCs.
The advances in FLT3 inhibitors and the discovery of epigenetic therapies (such as IDH1/2 inhibitors) have led to the development of many potential novel therapeutic agents, many of which are being investigated in ongoing clinical trials. With the development of immunotherapy, CAR-T therapy targeting AML cells is rapidly developing. Therapy combination may give us possibilities for various treatments.
As we can observe, the largest cluster in the co-citation network of references was venetoclax resistance. Drug resistance that causes failure and relapse of treatment raises growing concern. LSCs play a key role in relapsed AML, which would be a challenge but important direction for identification of targets for AML treatment in future. Furthermore, as a pre-leukemic event, CH has also received attention in the field of targeted therapy in AML. Ideally, drugs that can suppress mutant clones in the future will be found, which could block malignant transformation in the “first hit” state to some extent.
Through bibliometric analysis, our study provides insight into the process of developing targeted therapy in AML and provides a perspective on the clinical development of a variety of precision treatment approaches. We hope that our study will provide researchers with a deeper understanding of AML pathogenesis and treatment.
All authors listed have made a substantial, direct, and intellectual contribution to the work, and approved it for publication.
The manuscript was supported by the Project of Key Medical Specialty and Treatment Center of Pudong Hospital of Fudan University (Grant No. zdzk2020-06).
The authors are deeply indebted to ZL and GS for their support and effort in this study.
The authors declare that the research was conducted in the absence of any commercial or financial relationships that could be construed as a potential conflict of interest.
All claims expressed in this article are solely those of the authors and do not necessarily represent those of their affiliated organizations, or those of the publisher, the editors and the reviewers. Any product that may be evaluated in this article, or claim that may be made by its manufacturer, is not guaranteed or endorsed by the publisher.
1. Gebru MT, Wang HG. Therapeutic targeting of FLT3 and associated drug resistance in acute myeloid leukemia. J Hematol Oncol (2020) 13(1):155. doi: 10.1186/s13045-020-00992-1
2. Hahn WC, Bader JS, Braun TP, Califano A, Clemons PA, Druker BJ, et al. An expanded universe of cancer targets. Cell (2021) 184(5):1142–55. doi: 10.1016/j.cell.2021.02.020
3. Coombs CC, Tallman MS, Levine RL. Molecular therapy for acute myeloid leukaemia. Nat Rev Clin Oncol (2016) 13(5):305–18. doi: 10.1038/nrclinonc.2015.210
4. Dohner H, Wei AH, Lowenberg B. Towards precision medicine for AML. Nat Rev Clin Oncol (2021) 18(9):577–90. doi: 10.1038/s41571-021-00509-w
5. Daver N, Venugopal S, Ravandi F. FLT3 mutated acute myeloid leukemia: 2021 treatment algorithm. Blood Cancer J (2021) 11(5):104. doi: 10.1038/s41408-021-00495-3
6. Issa GC, Ravandi F, DiNardo CD, Jabbour E, Kantarjian HM, Andreeff M. Therapeutic implications of menin inhibition in acute leukemias. Leukemia (2021) 35(9):2482–95. doi: 10.1038/s41375-021-01309-y
7. Coates A, Hu Y, Bax R, Page C. The future challenges facing the development of new antimicrobial drugs. Nat Rev Drug Discovery (2002) 1(11):895–910. doi: 10.1038/nrd940
8. Walsh C. Molecular mechanisms that confer antibacterial drug resistance. Nature (2000) 406(6797):775–81. doi: 10.1038/35021219
9. Daub H, Specht K, Ullrich A. Strategies to overcome resistance to targeted protein kinase inhibitors. Nat Rev Drug Discovery (2004) 3(12):1001–10. doi: 10.1038/nrd1579
10. Grieselhuber NR, Mims AS. Novel targeted therapeutics in acute myeloid leukemia: an embarrassment of riches. Curr Hematol Malig Rep (2021) 16(2):192–206. doi: 10.1007/s11899-021-00621-9
11. Price DJ. Networks of scientific papers. Science (1965) 149(3683):510–5. doi: 10.1126/science.149.3683.510
12. Chen C, Song M. Visualizing a field of research: A methodology of systematic scientometric reviews. PloS One (2019) 14(10):e0223994. doi: 10.1371/journal.pone.0223994
13. CiteSpace CC II. Detecting and visualizing emerging trends and transient patterns in scientific literature. J Am Soc Inf Sci Technol (2006) 57(3):359–77. doi: 10.1002/asi.20317
14. Ouyang W, Wang Y, Lin C, He M, Hao F, Liu H, et al. Heavy metal loss from agricultural watershed to aquatic system: A scientometrics review. Sci Total Environ (2018) 637-638:208–20. doi: 10.1016/j.scitotenv.2018.04.434
15. Chen C, Hu Z, Liu S, Tseng H. Emerging trends in regenerative medicine: a scientometric analysis in CiteSpace. Expert Opin Biol Ther (2012) 12(5):593–608. doi: 10.1517/14712598.2012.674507
16. C CM. The CiteSpace manual. Available at: http://cluster.ischool.drexel.edu/~cchen/citespace/CiteSpaceManual.pdf (Accessed December 20, 2020).
17. Yuan G, Shi J, Jia Q, Shi S, Zhu X, Zhou Y, et al. Cardiac rehabilitation: A bibliometric review from 2001 to 2020. Front Cardiovasc Med (2021) 8:672913. doi: 10.3389/fcvm.2021.672913
18. Qin Y, Zhang Q, Liu Y. Analysis of knowledge bases and research focuses of cerebral ischemia-reperfusion from the perspective of mapping knowledge domain. Brain Res Bull (2020) 156:15–24. doi: 10.1016/j.brainresbull.2019.12.004
19. Liang YD, Li Y, Zhao J, Wang XY, Zhu HZ, Chen XH. Study of acupuncture for low back pain in recent 20 years: a bibliometric analysis via CiteSpace. J Pain Res (2017) 10:951–64. doi: 10.2147/JPR.S132808
20. Derek L, Hansen BS, Marc A, Itai Himelboim S. Analyzing social media networks with NodeXL insights from a connected world. Second Edition. (2020). doi: 10.1016/C2018-0-01348-1
21. Wei AH, Dohner H, Pocock C, Montesinos P, Afanasyev B, Dombret H, et al. Oral azacitidine maintenance therapy for acute myeloid leukemia in first remission. N Engl J Med (2020) 383(26):2526–37. doi: 10.1056/NEJMoa2004444
22. Dohner H, Estey E, Grimwade D, Amadori S, Appelbaum FR, Buchner T, et al. Diagnosis and management of AML in adults: 2017 ELN recommendations from an international expert panel. Blood (2017) 129(4):424–47. doi: 10.1182/blood-2016-08-733196
23. Smith CC. The growing landscape of FLT3 inhibition in AML. Hematol Am Soc Hematol Educ Program (2019) 2019(1):539–47. doi: 10.1182/hematology.2019000058
24. Estey E, Dohner H. Acute myeloid leukaemia. Lancet (2006) 368(9550):1894–907. doi: 10.1016/S0140-6736(06)69780-8
25. Weis TM, Marini BL, Bixby DL, Perissinotti AJ. Clinical considerations for the use of FLT3 inhibitors in acute myeloid leukemia. Crit Rev Oncol Hematol (2019) 141:125–38. doi: 10.1016/j.critrevonc.2019.06.011
26. Garcia JS, Stone RM. The development of FLT3 inhibitors in acute myeloid leukemia. Hematol Oncol Clin North Am (2017) 31(4):663–80. doi: 10.1016/j.hoc.2017.03.002
27. Schmalbrock LK, Dolnik A, Cocciardi S, Strang E, Theis F, Jahn N, et al. Clonal evolution of acute myeloid leukemia with FLT3-ITD mutation under treatment with midostaurin. Blood (2021) 137(22):3093–104. doi: 10.1182/blood.2020007626
28. Stone RM, Mandrekar SJ, Sanford BL, Laumann K, Geyer S, Bloomfield CD, et al. Midostaurin plus chemotherapy for acute myeloid leukemia with a FLT3 mutation. N Engl J Med (2017) 377(5):454–64. doi: 10.1056/NEJMoa1614359
29. Roboz GJ, Strickland SA, Litzow MR, Dalovisio A, Perl AE, Bonifacio G, et al. Updated safety of midostaurin plus chemotherapy in newly diagnosed FLT3 mutation-positive acute myeloid leukemia: the RADIUS-X expanded access program. Leuk Lymphoma (2020) 61(13):3146–53. doi: 10.1080/10428194.2020.1805109
30. Perl AE, Altman JK, Cortes J, Smith C, Litzow M, Baer MR, et al. Selective inhibition of FLT3 by gilteritinib in relapsed or refractory acute myeloid leukaemia: a multicentre, first-in-human, open-label, phase 1-2 study. Lancet Oncol (2017) 18(8):1061–75. doi: 10.1016/S1470-2045(17)30416-3
31. Onishi C, Mori-Kimachi S, Hirade T, Abe M, Taketani T, Suzumiya J, et al. Internal tandem duplication mutations in FLT3 gene augment chemotaxis to Cxcl12 protein by blocking the down-regulation of rho-associated kinase via the Cxcl12/Cxcr4 signaling axis. J Biol Chem (2015) 290(47):28356. doi: 10.1074/jbc.A114.568287
32. Schoofs T, Muller-Tidow C. DNA Methylation as a pathogenic event and as a therapeutic target in AML. Cancer Treat Rev (2011) 37 Suppl 1:S13–8. doi: 10.1016/j.ctrv.2011.04.013
33. Yang X, Sexauer A, Levis M. Bone marrow stroma-mediated resistance to FLT3 inhibitors in FLT3-ITD AML is mediated by persistent activation of extracellular regulated kinase. Br J Haematol (2014) 164(1):61–72. doi: 10.1111/bjh.12599
34. Parmar A, Marz S, Rushton S, Holzwarth C, Lind K, Kayser S, et al. Stromal niche cells protect early leukemic FLT3-ITD+ progenitor cells against first-generation FLT3 tyrosine kinase inhibitors. Cancer Res (2011) 71(13):4696–706. doi: 10.1158/0008-5472.CAN-10-4136
35. Lindblad O, Cordero E, Puissant A, Macaulay L, Ramos A, Kabir NN, et al. Aberrant activation of the PI3K/mTOR pathway promotes resistance to sorafenib in AML. Oncogene (2016) 35(39):5119–31. doi: 10.1038/onc.2016.41
36. Short NJ, Konopleva M, Kadia TM, Borthakur G, Ravandi F, DiNardo CD, et al. Advances in the treatment of acute myeloid leukemia: New drugs and new challenges. Cancer Discovery (2020) 10(4):506–25. doi: 10.1158/2159-8290.CD-19-1011
37. Levin M, Stark M, Ofran Y, Assaraf YG. Deciphering molecular mechanisms underlying chemoresistance in relapsed AML patients: towards precision medicine overcoming drug resistance. Cancer Cell Int (2021) 21(1):53. doi: 10.1186/s12935-021-01746-w
38. Malik P, Cashen AF. Decitabine in the treatment of acute myeloid leukemia in elderly patients. Cancer Manag. Res (2014) 6:53–61. doi: 10.2147/CMAR.S40600
39. Welch JS, Petti AA, Miller CA, Fronick CC, O’Laughlin M, Fulton RS, et al. TP53 and decitabine in acute myeloid leukemia and myelodysplastic syndromes. N Engl J Med (2016) 375(21):2023–36. doi: 10.1056/NEJMoa1605949
40. Fong CY, Morison J, Dawson MA. Epigenetics in the hematologic malignancies. Haematologica. (2014) 99(12):1772–83. doi: 10.3324/haematol.2013.092007
41. Florean C, Schnekenburger M, Grandjenette C, Dicato M, Diederich M. Epigenomics of leukemia: from mechanisms to therapeutic applications. Epigenomics (2011) 3(5):581–609. doi: 10.2217/epi.11.73
42. Stein EM, Tallman MS. Emerging therapeutic drugs for AML. Blood (2016) 127(1):71–8. doi: 10.1182/blood-2015-07-604538
43. Medeiros BC, Fathi AT, DiNardo CD, Pollyea DA, Chan SM, Swords R. Isocitrate dehydrogenase mutations in myeloid malignancies. Leukemia (2017) 31(2):272–81. doi: 10.1038/leu.2016.275
44. Figueroa ME, Abdel-Wahab O, Lu C, Ward PS, Patel J, Shih A, et al. Leukemic IDH1 and IDH2 mutations result in a hypermethylation phenotype, disrupt TET2 function, and impair hematopoietic differentiation. Cancer Cell (2010) 18(6):553–67. doi: 10.1016/j.ccr.2010.11.015
45. Mehdipour P, Santoro F, Minucci S. Epigenetic alterations in acute myeloid leukemias. FEBS J (2015) 282(9):1786–800. doi: 10.1111/febs.13142
46. Lee E, Koh Y, Hong J, Eom HS, Yoon SS. Recent clinical update of acute myeloid leukemia: Focus on epigenetic therapies. J Korean Med Sci (2021) 36(13):e85. doi: 10.3346/jkms.2021.36.e85
47. Thomas X, Elhamri M, Heiblig M. Emerging pharmacotherapies for elderly acute myeloid leukemia patients. Expert Rev Hematol (2020) 13(6):619–43. doi: 10.1080/17474086.2020.1758058
48. Rao RC, Dou Y. Hijacked in cancer: the KMT2 (MLL) family of methyltransferases. Nat Rev Cancer. (2015) 15(6):334–46. doi: 10.1038/nrc3929
49. Milne TA. Mouse models of MLL leukemia: recapitulating the human disease. Blood (2017) 129(16):2217–23. doi: 10.1182/blood-2016-10-691428
50. Yokoyama A, Somervaille TC, Smith KS, Rozenblatt-Rosen O, Meyerson M, Cleary ML. The menin tumor suppressor protein is an essential oncogenic cofactor for MLL-associated leukemogenesis. Cell (2005) 123(2):207–18. doi: 10.1016/j.cell.2005.09.025
51. Uckelmann HJ, Kim SM, Wong EM, Hatton C, Giovinazzo H, Gadrey JY, et al. Therapeutic targeting of preleukemia cells in a mouse model of NPM1 mutant acute myeloid leukemia. Science (2020) 367(6477):586–90. doi: 10.1126/science.aax5863
52. Bai H, Zhang SQ, Lei H, Wang F, Ma M, Xin M. Menin-MLL protein-protein interaction inhibitors: a patent review (2014-2021). Expert Opin Ther Pat. (2022) 32(5):507–22. doi: 10.1080/13543776.2022.2045947
53. Lei H., Zhang S. Q., Xin M. H.. Recent Progress of Small Molecule Menin-MLL Interaction Inhibitors as Therapeutic Agents for Acute Leukemia Journal of Medicinal Chemistry (2021) 64(21):15519–33. doi: 10.1158/2159-8290.CD-NB2020-120
54. Vetrie D, Helgason GV, Copland M. The leukaemia stem cell: similarities, differences and clinical prospects in CML and AML. Nat Rev Cancer. (2020) 20(3):158–73. doi: 10.1038/s41568-019-0230-9
55. O’Reilly E, Zeinabad HA, Szegezdi E. Hematopoietic versus leukemic stem cell quiescence: Challenges and therapeutic opportunities. Blood Rev (2021) 50:100850. doi: 10.1016/j.blre.2021.100850
56. Rowley JD. Letter: A new consistent chromosomal abnormality in chronic myelogenous leukaemia identified by quinacrine fluorescence and giemsa staining. Nature (1973) 243(5405):290–3. doi: 10.1038/243290a0
57. Heisterkamp N, Stephenson JR, Groffen J, Hansen PF, de Klein A, Bartram CR, et al. Localization of the c-ab1 oncogene adjacent to a translocation break point in chronic myelocytic leukaemia. Nature (1983) 306(5940):239–42. doi: 10.1038/306239a0
58. Hochhaus A, Larson RA, Guilhot F, Radich JP, Branford S, Hughes TP, et al. Long-term outcomes of imatinib treatment for chronic myeloid leukemia. N Engl J Med (2017) 376(10):917–27. doi: 10.1056/NEJMoa1609324
59. Shlush LI, Mitchell A, Heisler L, Abelson S, Ng SWK, Trotman-Grant A, et al. Tracing the origins of relapse in acute myeloid leukaemia to stem cells. Nature (2017) 547(7661):104–8. doi: 10.1038/nature22993
60. Ding L, Ley TJ, Larson DE, Miller CA, Koboldt DC, Welch JS, et al. Clonal evolution in relapsed acute myeloid leukaemia revealed by whole-genome sequencing. Nature (2012) 481(7382):506–10. doi: 10.1038/nature10738
61. Yilmaz M, Wang F, Loghavi S, Bueso-Ramos C, Gumbs C, Little L, et al. Late relapse in acute myeloid leukemia (AML): clonal evolution or therapy-related leukemia? Blood Cancer J (2019) 9(2):7. doi: 10.1038/s41408-019-0170-3
62. Branford S, Wang P, Yeung DT, Thomson D, Purins A, Wadham C, et al. Integrative genomic analysis reveals cancer-associated mutations at diagnosis of CML in patients with high-risk disease. Blood (2018) 132(9):948–61. doi: 10.1182/blood-2018-02-832253
63. Lim S, Saw TY, Zhang M, Janes MR, Nacro K, Hill J, et al. Targeting of the MNK-eIF4E axis in blast crisis chronic myeloid leukemia inhibits leukemia stem cell function. Proc Natl Acad Sci U.S.A. (2013) 110(25):E2298–307. doi: 10.1073/pnas.1301838110
64. Essers MA, Offner S, Blanco-Bose WE, Waibler Z, Kalinke U, Duchosal MA, et al. IFNalpha activates dormant haematopoietic stem cells in vivo. Nature (2009) 458(7240):904–8. doi: 10.1038/nature07815
65. Blokzijl F, de Ligt J, Jager M, Sasselli V, Roerink S, Sasaki N, et al. Tissue-specific mutation accumulation in human adult stem cells during life. Nature (2016) 538(7624):260–4. doi: 10.1038/nature19768
66. Hoang ML, Kinde I, Tomasetti C, McMahon KW, Rosenquist TA, Grollman AP, et al. Genome-wide quantification of rare somatic mutations in normal human tissues using massively parallel sequencing. Proc Natl Acad Sci U.S.A. (2016) 113(35):9846–51. doi: 10.1073/pnas.1607794113
67. Fey MF, Liechti-Gallati S, von Rohr A, Borisch B, Theilkas L, Schneider V, et al. Clonality and X-inactivation patterns in hematopoietic cell populations detected by the highly informative M27 beta DNA probe. Blood (1994) 83(4):931–8. doi: 10.1182/blood.V83.4.931.931
68. Lu C, Ward PS, Kapoor GS, Rohle D, Turcan S, Abdel-Wahab O, et al. IDH mutation impairs histone demethylation and results in a block to cell differentiation. Nature (2012) 483(7390):474–8. doi: 10.1038/nature10860
69. Corces MR, Buenrostro JD, Wu B, Greenside PG, Chan SM, Koenig JL, et al. Lineage-specific and single-cell chromatin accessibility charts human hematopoiesis and leukemia evolution. Nat Genet (2016) 48(10):1193–203. doi: 10.1038/ng.3646
70. Jan M, Snyder TM, Corces-Zimmerman MR, Vyas P, Weissman IL, Quake SR, et al. Clonal evolution of preleukemic hematopoietic stem cells precedes human acute myeloid leukemia. Sci Transl Med (2012) 4(149):149ra18. doi: 10.1126/scitranslmed.3004315
71. Gilliland DG, Griffin JD. The roles of FLT3 in hematopoiesis and leukemia. Blood (2002) 100(5):1532–42. doi: 10.1182/blood-2002-02-0492
72. Jaiswal S, Ebert BL. Clonal hematopoiesis in human aging and disease. Science (2019) 366(6465):586. doi: 10.1126/science.aan4673
73. McKerrell T, Park N, Moreno T, Grove CS, Ponstingl H, Stephens J, et al. Leukemia-associated somatic mutations drive distinct patterns of age-related clonal hemopoiesis. Cell Rep (2015) 10(8):1239–45. doi: 10.1016/j.celrep.2015.02.005
74. Genovese G, Kahler AK, Handsaker RE, Lindberg J, Rose SA, Bakhoum SF, et al. Clonal hematopoiesis and blood-cancer risk inferred from blood DNA sequence. N Engl J Med (2014) 371(26):2477–87. doi: 10.1056/NEJMoa1409405
75. Busque L, Patel JP, Figueroa ME, Vasanthakumar A, Provost S, Hamilou Z, et al. Recurrent somatic TET2 mutations in normal elderly individuals with clonal hematopoiesis. Nat Genet (2012) 44(11):1179–81. doi: 10.1038/ng.2413
76. Abelson S, Collord G, Ng SWK, Weissbrod O, Mendelson Cohen N, Niemeyer E, et al. Prediction of acute myeloid leukaemia risk in healthy individuals. Nature (2018) 559(7714):400–4. doi: 10.1038/s41586-018-0317-6
77. Desai P, Mencia-Trinchant N, Savenkov O, Simon MS, Cheang G, Lee S, et al. Somatic mutations precede acute myeloid leukemia years before diagnosis. Nat Med (2018) 24(7):1015–23. doi: 10.1038/s41591-018-0081-z
78. Leick MB, Silva H, Scarfo I, Larson R, Choi BD, Bouffard AA, et al. Non-cleavable hinge enhances avidity and expansion of CAR-T cells for acute myeloid leukemia. Cancer Cell (2022) 40(5):494–508.e5. doi: 10.1016/j.ccell.2022.04.001
79. Wang Y, Xu Y, Li S, Liu J, Xing Y, Xing H, et al. Targeting FLT3 in acute myeloid leukemia using ligand-based chimeric antigen receptor-engineered T cells. J Hematol Oncol (2018) 11(1):60. doi: 10.1186/s13045-018-0603-7
80. Deeks ED. Venetoclax: First global approval. Drugs (2016) 76(9):979–87. doi: 10.1007/s40265-016-0596-x
Keywords: acute myeloid leukemia, aml, targeted therapy, CiteSpace, bibliometric analysis
Citation: Huang W, Sun G, Wang Q and Long Z (2022) The research progress of targeted therapy in acute myeloid leukemia based on bibliometric analysis. Front. Oncol. 12:957370. doi: 10.3389/fonc.2022.957370
Received: 31 May 2022; Accepted: 11 August 2022;
Published: 02 September 2022.
Edited by:
Giuseppe Giaccone, Cornell University, United StatesReviewed by:
Alisa Damnernsawad, Mahidol University, ThailandCopyright © 2022 Huang, Sun, Wang and Long. This is an open-access article distributed under the terms of the Creative Commons Attribution License (CC BY). The use, distribution or reproduction in other forums is permitted, provided the original author(s) and the copyright owner(s) are credited and that the original publication in this journal is cited, in accordance with accepted academic practice. No use, distribution or reproduction is permitted which does not comply with these terms.
*Correspondence: Zhiguo Long, WmdMb25nMTk3NkAxMjYuY29t
Disclaimer: All claims expressed in this article are solely those of the authors and do not necessarily represent those of their affiliated organizations, or those of the publisher, the editors and the reviewers. Any product that may be evaluated in this article or claim that may be made by its manufacturer is not guaranteed or endorsed by the publisher.
Research integrity at Frontiers
Learn more about the work of our research integrity team to safeguard the quality of each article we publish.