- 1Department of Surgery, Kindai University Faculty of Medicine, Osaka-Sayama, Japan
- 2Department of Medical Oncology, Kindai University Faculty of Medicine, Osaka-Sayama, Japan
- 3Department of Otolaryngology–Head and Neck Surgery, Kyoto Prefectural University of Medicine, Kyoto, Japan
- 4Clinical Research Center, Kindai University Hospital, Osaka-Sayama, Japan
- 5Department of Pathology, Kindai University Faculty of Medicine, Osaka-Sayama, Japan
Matrix metalloproteinase 14 (MMP14) expression is implicated in progression of colorectal cancer, but its role in the tumor microenvironment (TME) has been unclear. The relevance of MMP14 to colorectal cancer progression was explored by analysis of transcriptomic data for colorectal adenocarcinoma patients (n = 592) in The Cancer Genome Atlas. The role of MMP14 in the TME was investigated in a retrospective analysis of tumor samples from 86 individuals with stage III colorectal cancer by single cell–based spatial profiling of MMP14 expression as performed by 12-color multiplex immunohistochemistry (mIHC). Analysis of gene expression data revealed that high MMP14 expression was associated with tumor progression and implicated both cancer-associated fibroblasts (CAFs) and tumor-associated macrophages in such progression. Spatial profiling by mIHC revealed that a higher percentage of MMP14+ cells among intratumoral CAFs (MMP14+ CAF/CAF ratio) was associated with poorer relapse-free survival. Multivariable analysis including key clinical factors identified the MMP14+ CAF/CAF ratio as an independent poor prognostic factor. Moreover, the patient subset with both a high MMP14+ CAF/CAF ratio and a low tumor-infiltrating lymphocyte density showed the worst prognosis. Our results suggest that MMP14+ CAFs play an important role in progression of stage III colorectal cancer and may therefore be a promising therapeutic target.
Introduction
Colorectal cancer is the second most common cause of cancer-related deaths worldwide (1). Stage III colorectal cancer, which accounts for one-third of all colorectal cancer cases at diagnosis, is generally characterized by local peritoneal invasion or lymph node metastasis and thus has a high recurrence rate of >30% even after complete tumor resection (2, 3). Although 5-fluorouracil (5-FU)–based adjuvant chemotherapy is administered to mitigate this poor prognosis in individuals with stage III colorectal cancer, no substantial advances in treatment have been achieved in recent decades and treatment outcome remains unsatisfactory (4–6). Optimization of treatment strategies for stage III colorectal cancer, including better stratification of patients, is therefore an important clinical goal.
Increasing evidence has suggested that the tumor microenvironment (TME), including immune cells and cancer-associated fibroblasts (CAFs), plays an important role in cancer fate including therapeutic response and clinical outcome (7–14). An Immunoscore based on peritumoral and intratumoral populations of T cells including CD3+ and CD8+ T cells has been found to classify stage III colorectal cancer into recurrent and nonrecurrent subgroups (15–19). In addition, transcriptome-based phenotyping allows categorization of colorectal cancer into four distinct subgroups, designated Consensus Molecular Subtypes (CMSs). Similar to the Immunoscore, the CMS classification has revealed that a gene expression profile suggestive of intratumoral immune infiltration (CMS1) predicts a better relapse-free survival (RFS), whereas poorly immunogenic profiles including a canonical subtype characterized by WNT and MYC pathway activation (CMS2) and a metabolic subtype characterized by metabolic dysregulation (CMS3) tend to be associated with recurrence after complete tumor resection. A mesenchymal phenotype characterized by enrichment of transcriptomes associated with stromal components and angiogenesis (CMS4) shows the worst RFS, even though the tumors manifest moderate immune-related gene expression (20). CAFs constitute one such stromal component and are key players in the TME (21). These cells are defined as activated fibroblasts present specifically in the TME (22), and they are thought to interact with immune cells such as tumor-associated macrophages (TAMs) and myeloid-derived suppressor cells (MDSCs) (22–29). These findings suggest that further investigation of the TME, including both immune cells and mesenchymal or other stromal components, is required to better characterize the biology of colorectal cancer and to inform the development of new treatment strategies based on a better stratification of stage III colorectal cancer.
Matrix metalloproteinase 14 (MMP14) is a transmembrane proteolytic enzyme (30) that plays a key role in establishment of a desmoplastic TME in colorectal cancer (31). Indeed, recent clinical studies have shown that colorectal tumors with a relatively high MMP14 expression level tend to have a mesenchymal phenotype such as CMS4 (20, 32). In addition, a study based on data in The Cancer Genome Atlas (TCGA) found that a high MMP14 expression level was associated with poor prognosis in stage I–III colorectal cancer (33). Furthermore, a preclinical study of genetically engineered or syngeneic mouse models revealed that the expression of MMP14 in colorectal tumors gave rise to 5-FU resistance through activation of CAFs (32). These various observations suggest that MMP14 expression in tumors may contribute to the recurrence of colorectal cancer in a manner dependent on the TME, and that clarification of this role of MMP14 may lead to improvement in the survival of individuals with stage III colorectal cancer.
We have now conducted a retrospective biomarker analysis of surgically resected tumor specimens from patients with stage III colorectal cancer attending Kindai University Hospital as well as an analysis of TCGA transcriptomic data in order to clarify this role of MMP14. We performed 12-color multiplex immunohistochemistry (mIHC) to evaluate the relation of MMP14 protein expression in multiple cell subsets including intratumoral immune cells to colorectal cancer progression. Our digital pathology platform thus allowed a single cell–based quantitative spatial profiling of MMP14 expression in various cell lineages in the stage III colorectal tumors. We found that CAFs are an important source of MMP14 and that MMP14+ CAFs may act in collaboration with TAMs to promote the progression of colorectal cancer. Our results suggest a novel risk stratification of stage III colorectal cancer based on MMP14 expression in CAFs.
Materials and methods
Patients
We consecutively reviewed the medical records of patients with stage III colorectal cancer who underwent definitive surgery followed by adjuvant chemotherapy at Kindai University Hospital between January 2013 and December 2017. Patients who received adjuvant chemotherapy for <3 months and those without sufficient tumor tissue available for our study were excluded. Colorectal cancer was staged according to the eighth edition of the Union for International Cancer Control (UICC) TNM Classification of Malignant Tumors (34). From this review, we identified 86 patients who received 5-FU–based adjuvant chemotherapy (Supplementary Figure S1). Colorectal cancer for which the primary tumor was located proximal to the splenic flexure was defined as right-sided. RFS was defined as the time from surgery to the date that clinical evidence of recurrent or metastatic disease was obtained or to the date of last follow-up. The cutoff date for follow-up was 13 May 2021.
mIHC staining protocol
mIHC staining was performed as described previously (35, 36). In brief, sections (thickness, 4 µm) of formalin-fixed, paraffin-embedded (FFPE) tissue were depleted of paraffin, stained with hematoxylin (S3301, Dako), and subjected to whole-tissue scanning with a NanoZoomer instrument (Hamamatsu Photonics) at 20× magnification to detect the nucleus of each cell. Peroxidase activity was blocked by exposure of the sections to 0.6% hydrogen peroxide in phosphate-buffered saline (PBS) for 15 min, and antigen retrieval was performed by exposure to microwave radiation (to achieve a temperature of 95°C for 15 min) in Antigen Retrieval Citra Solution (B-HK0809K, BioGenex). After exposure to 5.0% goat serum and 2.5% bovine serum albumin (37) in PBS to block nonspecific sites, the tissue was incubated with primary antibodies, anti-mouse or anti-rabbit Histofine Simple Stain MAX PO horseradish peroxidase–conjugated polymer (Nichirei Biosciences), and the alcohol-soluble peroxidase substrate 3-aminomethyl carbazolezole as shown in Supplementary Table S1. Chromogenic destaining and antibody stripping were performed between sequential staining steps in the order indicated (Supplementary Table S1). Representative images of staining for each antigen are shown in Supplementary Figure S2A.
Digital analysis
Representative fields of 1884 by 1884 μm for two distinct tumor areas, the invasive front (IF) and center of the tumor (CT), were randomly selected from the whole digital slides with the use of Aperio ImageScope v.12 software (Leica Biosystems). The IF was defined as the area within 300 µm external to the boundary separating the tumor cell area from the surrounding connective tissue (Supplementary Figure S2B), as described previously (38). The CT was defined as an intratumoral area and was further categorized into a tumor cell nest (TN) area and a non–tumor cell area comprising mostly intratumoral stromal tissue (ISA) (Supplementary Figure S2B) with the use of a mathematical morphological approach performed with the Tissue Segmentation platform introduced in our previous study (39). Between two and five fields of view were evaluated depending on the size of the tumor (average of 3.64 fields of view for CT and 4.37 for IF). The CT field could not be determined because of the small size of the tumor in one case. Image processing and subsequent computational analysis were performed with ImageJ/Fiji version 1.51 (National Institutes of Health), CellProfiler version 2.2.0 (Broad Institute), Aperio ImageScope, and FCS Express 7 Image Cytometry v.7.04.0014 (De Novo Software) as described previously (35, 36). In brief, the serially scanned images were coregistered by ImageJ/Fiji and CellProfiler. The coregistered images were then converted to single-marker images by ImageJ. Pseudocoloring of the images was performed in Aperio ImageScope. Quantitative assessment of the images was performed with FCS Express 7 Image Cytometry after single-cell segmentation and quantification of staining intensity by CellProfiler. For quantification of individual immune cell subsets by FCS Express 7 Image Cytometry, fluorescence-minus-one controls were used to determine true positive cells. The final value for each cell number was calculated as the average of values from the multiple fields of view.
Immunoscore
The Immunoscore was determined as described previously (16). In brief, the densities of CD3+ and CD8+ T cells in both the CT or ISA and the IF regions were calculated, and tumors were then categorized into two groups based on the median values of the densities of each T cell subset in each region. Values lower than or equal to the median and those higher than the median were scored as 0 or 1, respectively. The sum of all scores (CD3+ cells in CT or ISA, CD3+ cells in IF, CD8+ cells in CT or ISA, and CD8+ cells in IF) was calculated as the final Immunoscore. A final score based on CT and IF regions was determined as the conventional Immunoscore. In addition, an IS-Immunoscore based on scores in IF and ISA regions was provided by our Tissue Segmentation platform. Patients with final scores of ≥2 or <2 were classified as having a high or low Immunoscore, respectively.
Transcriptomic data analysis
Normalized gene expression data (RNA Seq v2) for the colorectal adenocarcinoma cohort (n = 592) of the TCGA database were downloaded via the cBioportal website (http://www.cbioportal.org) in August 2021. The downloaded value (x) was converted to x + 1 for subsequent differential gene expression analysis. Genes expressed at a low level (those for which >50% of samples showed an expression value below the minimum threshold defined as 1.5) were filtered out. Gene set enrichment analysis (GSEA) (http://www.broadinstitute.org/gsea/index.jsp) was performed with hallmark gene sets v7.4 and GSEA software version 4.0.3 (Broad Institute) (40). The extent of immune cell infiltration was determined as the absolute score of the LM22 signature with the CIBERSORTx tool (41) (https://cibersortx.stanford.edu). Correlation coefficients for gene expression levels were estimated with the cBioportal website platform.
Statistical analysis
Differences between survival curves constructed by the Kaplan-Meier method were assessed with the log-rank test. The hazard ratio (HR) and its 95% confidence interval (CI) were calculated according to the Cox proportional hazards model. Correlations were examined with the Spearman rank correlation test. The Benjamini-Hochberg method was applied to calculate the false discovery rate (FDR) for multiple testing unless specified otherwise. The Wilcoxon rank-sum test was adopted to compare continuous variables, with the Bonferroni correction being applied to adjust P values in multiple tests. Multivariable analysis was performed with the Cox proportional hazards regression model. Age, sex, tumor location, stage, histology, and adjuvant chemotherapy were chosen as covariates because they were identified as clinically important factors in previous studies (42–45). All P values were based on a two-sided hypothesis, and all statistical analysis was performed with JMP software version 15.1.0 (SAS Institute) or GraphPad Prism version 9.1.1 (GraphPad Software).
Study approval
This retrospective study was approved by the Institutional Review Board (R02-070) and the Ethics Committee of Kindai University Faculty of Medicine. The Institutional Review Board waived the need to obtain written consent.
Results
Association of MMP14-related fibrotic tissue with immunosuppressive TAM infiltration into tumors suggested by TCGA data
To explore the clinical relevance of MMP14 in colorectal cancer, we first analyzed publicly available gene expression data for tumor tissue of the colorectal adenocarcinoma data set in TCGA. A high level of MMP14 expression in tumors was associated with a poor survival outcome in this cohort (Figure 1A). To investigate the character of tumors expressing MMP14 at a high level, we searched for genes whose expression level was correlated with that of MMP14 (Figure 1B). This analysis showed that MMP14 expression was strongly correlated with that of genes related to stromal cells (including PDGFRB and VIM) as well as that of many collagen genes (46), suggesting that CAFs might contribute to tumor aggressiveness associated with MMP14. Of note, however, the expression level of MRC2, an M2-type immunosuppressive and tumorigenic macrophage–related gene (47), was most strongly correlated with that of MMP14, also implicating M2-TAMs in MMP14-related tumor progression. In addition, we generated a list of differentially expressed genes for tumors with high versus low levels of MMP14 expression by calculating log2 of the fold change in expression level and the FDR (q) value. This list included M2-TAM–related genes such as MARCO (48) and SPP1 (49, 50) in the top 20 genes associated with high MMP14 expression (Figure 1C). MRC2 was also a gene associated with high MMP14 expression in this list (ranked 143 out of 16,795 genes, log2[fold change] = 1.729, q value = 3.999 × 10–87). MMP14 expression was also associated with that of other M2-TAM genes such as CD163 (ranked 103, log2[fold change] = 1.815, q value = 2.501 × 10–40) and CD206 (ranked 327, log2[fold change] = 1.475, q value = 4.332 × 10–27).
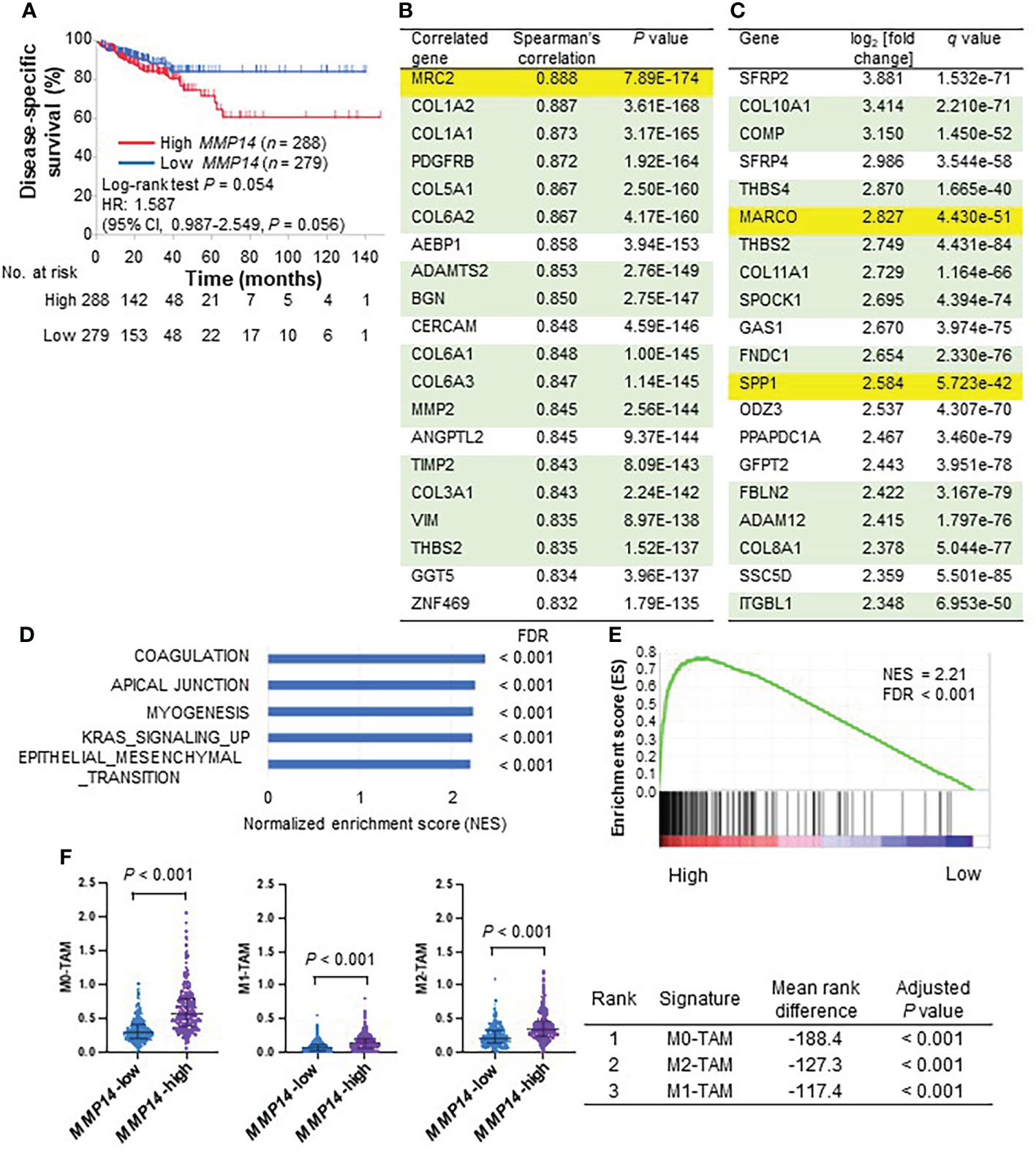
Figure 1 Analysis of the colorectal adenocarcinoma cohort (n = 592) of the TCGA database according to MMP14 expression. (A) Kaplan-Meier curves for disease-specific survival in patients divided according to the median value of MMP14 expression level. Vertical bars denote censoring. (B) The top 20 genes whose expression level was correlated with that of MMP14 ranked according to Spearman’s correlation coefficient. Genes related to stromal tissue or M2-TAMs are highlighted in green and yellow, respectively. (C) The top 20 genes whose expression was associated with that of MMP14 ranked according to log2 of the fold change in normalized expression value for MMP14-high relative to MMP14-low tumors. FDR q values were also calculated. Genes are highlighted as in (B). (D) The top five hallmark gene sets whose expression was up-regulated in the MMP14-high group as revealed by GSEA. FDR q values were calculated with GSEA software. (E) GSEA plot of enrichment for the gene signature related to epithelial-mesenchymal transition for MMP14-high versus MMP14-low tumors. (F) The top three immune cell signatures associated with MMP14 expression by CIBERSORTx analysis. In the dot plots for the immune cell signatures (top), each dot represents one patient and the median value and interquartile range are indicated. The mean rank difference values and adjusted P values calculated by multiple Wilcoxon rank-sum tests with Bonferroni correction are also shown (bottom).
We next performed gene expression profiling of MMP14-expressing colorectal tumors with the use of GSEA and CIBERSORTx analysis. GSEA revealed that a signature enriched in fibroblast-related genes (HALLMARK_EPITHELIAL_MESENCHYMAL_TRANSITION) was in the top five gene sets that were significantly up-regulated in tumors expressing MMP14 at a high level (Figures 1D, E). CIBERSORTx analysis of the LM22 signature matrix suggested that MMP14 expression was associated with the accumulation of TAMs including M2-TAMs in colorectal tumors, with the top three immune cell subsets identified by this analysis being M0-, M2-, and M1-TAMs, respectively (Figure 1F). Overall, these results suggested that MMP14 expression, in association with CAFs and M2-TAM infiltration into tumor tissue, contributes to poor survival outcome in patients with colorectal cancer.
Contribution of MMP14 expression in CAFs to colorectal cancer recurrence as revealed by spatial profiling with mIHC
To examine further how MMP14 expression in tumors might contribute to poor survival outcome in colorectal cancer, we performed 12-color mIHC analysis of tumor tissue surgically removed from patients with stage III colorectal cancer at Kindai University Hospital. A consecutive review of medical records from 2013 to 2017 identified 86 individuals who underwent curative resection for stage III colorectal cancer followed by 5-FU–based adjuvant chemotherapy for inclusion in our study (Table 1). Most cases were stage IIIB (71%), and all were adenocarcinoma, including 13 cases with a histology known to be prognostic for an unfavorable survival outcome (poorly differentiated, mucinous, and signet ring cell). Postoperative adjuvant chemotherapy regimens included 5-FU administered either alone or together with oxaliplatin in 45 (52%) and 41 (48%) patients, respectively. Twenty-five patients (29%) experienced disease recurrence during follow-up with a median of 60.1 months.
On the basis of our analysis of TCGA gene expression data, we spatially evaluated multiple cell compartments including CAFs, M1- and M2-TAMs, and tumor cells in addition to MMP14 expression in individual FFPE tumor tissue sections by 12-color mIHC. Tumor-infiltrating lymphocytes (TILs) were also evaluated in the same sections, given that previous studies based on the Immunoscore have implicated these cells in colorectal cancer progression (16–19). In addition, we included MDSCs in this analysis because these cells have been associated with TAMs and CAFs in the TME (51–53). Each cell lineage as defined by marker expression was appropriately distinguished by our 12-color mIHC platform (Figures 2A, B). Image cytometry–based analysis of cell populations allowed quantitative evaluation of the cell subsets as cell number per area (Figure 2C). In addition, we applied our recently developed Tissue Segmentation method (39) to quantify cell number according to different tumor areas including IF, ISA, and TN (Supplementary Figure S2B), thus allowing detailed evaluation of the clinical relevance of each cell subset. Given that α smooth muscle actin (αSMA), a marker for CAFs in our mIHC panel, was expressed in both smooth muscle cells and fibroblasts in the IF, this region was excluded from subsequent analysis of the association of CAFs with immune cells. We detected CD45+ immune cells in both ISA and TN regions of CT, whereas CAFs and tumor cells were identified almost exclusively in ISA and TN, respectively (Supplementary Figure S2C), supporting the ability of our Tissue Segmentation method to distinguish between these two regions of the CT area.
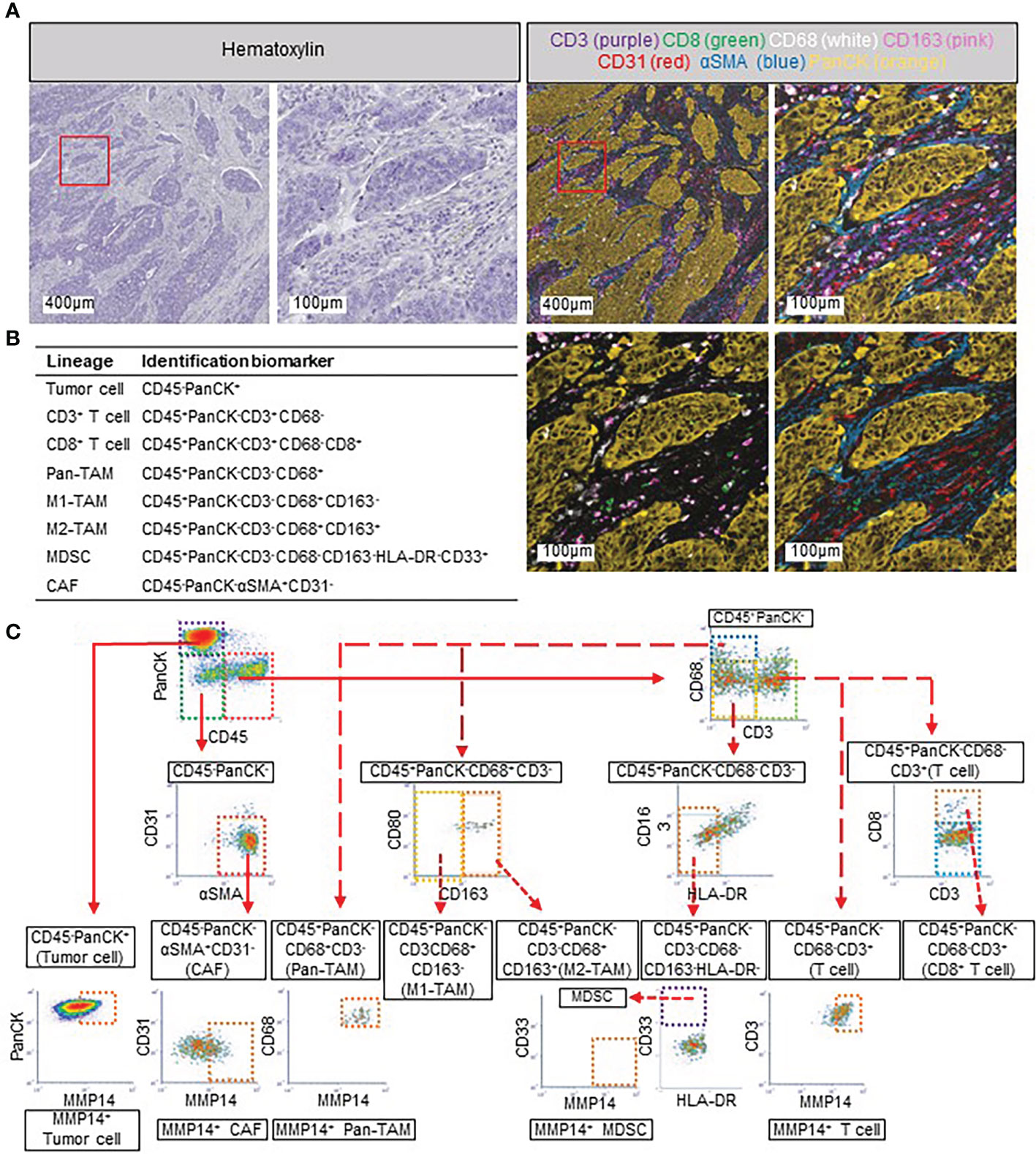
Figure 2 Multiplex immunohistochemistry (mIHC) and strategy for quantitative evaluation of cell populations. (A) Representative images of mIHC. Images for hematoxylin staining (upper) and multicolor images (lower) are shown. The area within the red box is shown at higher magnification in the other corresponding images. Seven markers are shown in the top two multicolor images, with CD8 (green), CD68 (white), CD163 (pink), and pan-cytokeratin (panCK, orange) being shown in the lower left image and CD8 (green), CD31 (red), αSMA (blue), and panCK (orange) in the lower right. (B) Definition of cell lineages according to marker expression in the present study. (C) Gating strategy for mIHC-based single-cell population analysis.
To determine the cell lineages responsible for MMP14-related tumor recurrence, we evaluated the relation of RFS to MMP14 expression in different cell types. MMP14 expression was detected predominantly in tumor cells and CAFs, although it was also apparent in TAMs and TILs (Figure 3A). A higher percentage of MMP14-expressing cells among CAFs (MMP14+ CAF/CAF ratio) was associated with a poorer RFS (HR of 1.936, with a 95% CI of 0.855–4.384), whereas a similar trend was not apparent for MMP14 expression in tumor cells, TAMs, or TILs (Figure 3B and Supplementary Figure S3A). Overall CAF density was not correlated with the MMP14+ CAF/CAF ratio (Supplementary Figure S3B) and was not associated with RFS (Supplementary Figure S3C). Collectively, our spatial profiling data thus suggested that intratumoral CAFs might contribute to tumor progression in stage III colorectal cancer by expressing MMP14.
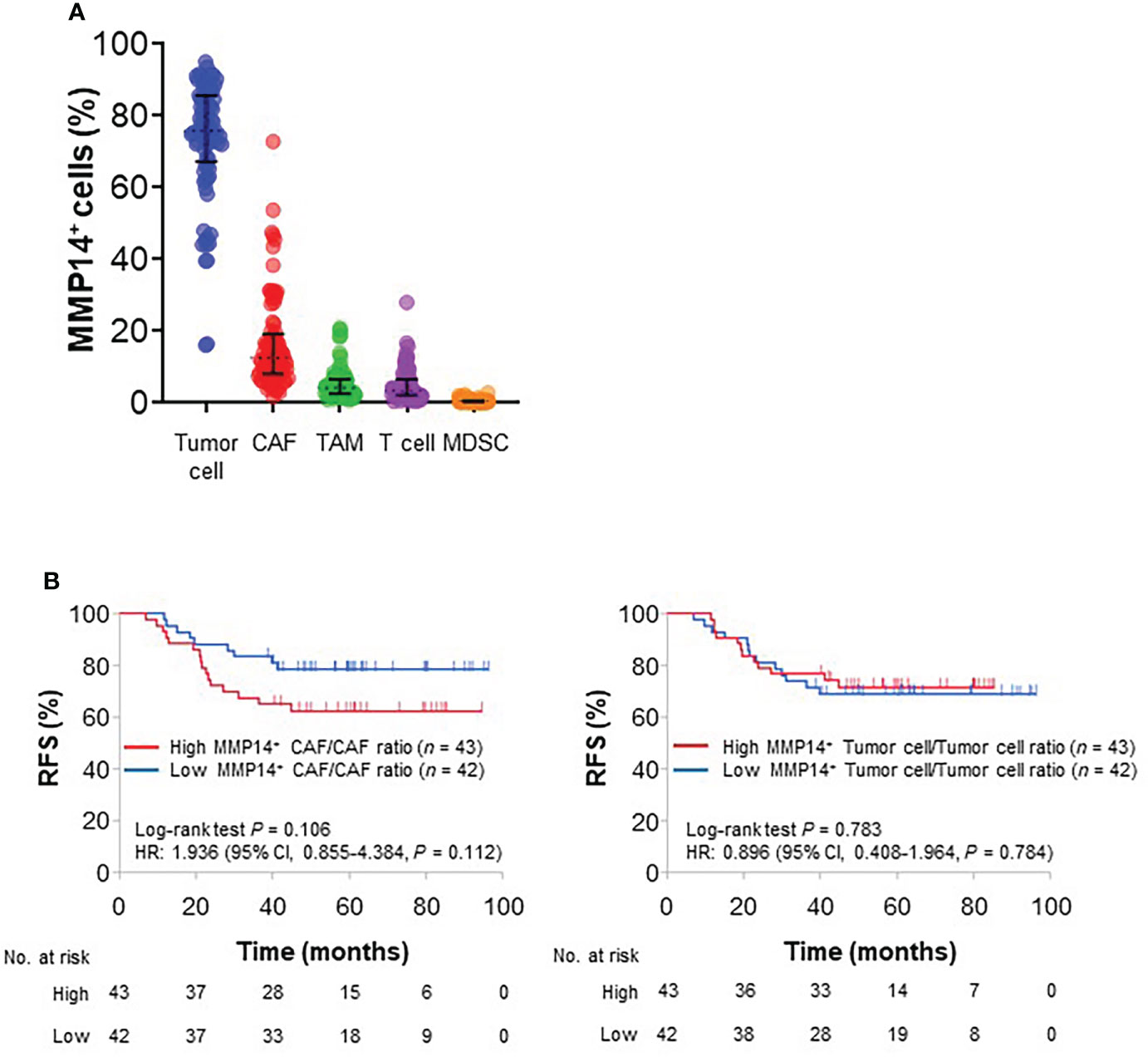
Figure 3 Prognostic impact of MMP14-expressing cells. (A) Percentage of MMP14-expressing cells among cell subsets as determined by mIHC analysis. Each dot represents one patient, and the median value and interquartile range are shown for each plot. (B) Kaplan-Meier curves for RFS according to the median values of the MMP14+ CAF/CAF ratio (left) or the MMP14+ tumor cell/tumor cell ratio (right).
Relation of MMP14 expression in CAFs to the infiltration of M2-TAMs into the TN
We next investigated further the relation between TAMs and MMP14+ CAFs in stage III colorectal cancer suggested by our analysis of TCGA transcriptomic data. The MMP14+ CAF/CAF ratio was positively correlated with M2-TAM density in both the TN and ISA, whereas it was not correlated with the density of M1-TAMs, TILs, or MDSCs (Supplementary Tables S2, S3). In contrast, overall CAF density was not positively correlated with M2-TAM density in TN or ISA regions (Supplementary Figures S3D, E). These data suggested that M2-TAMs might infiltrate into the TN via an intratumoral stromal space enriched in MMP14 expressed by CAFs (Figure 4A). We also examined the impact of these tumor-infiltrating M2-TAMs on colorectal cancer recurrence. Whereas the density of M2-TAMs in the ISA was not associated with colorectal cancer recurrence (HR of 0.719, with a 95% CI of 0.326–1.586), a high M2-TAM density in the TN tended to be associated with a poor RFS (HR of 1.582, with a 95% CI of 0.710–3.524) (Figure 4B). Together, these results suggested that MMP14-related colorectal cancer aggressiveness might be explained, at least in part, by the promotion of M2-TAM infiltration into the TN by MMP14+ CAFs.
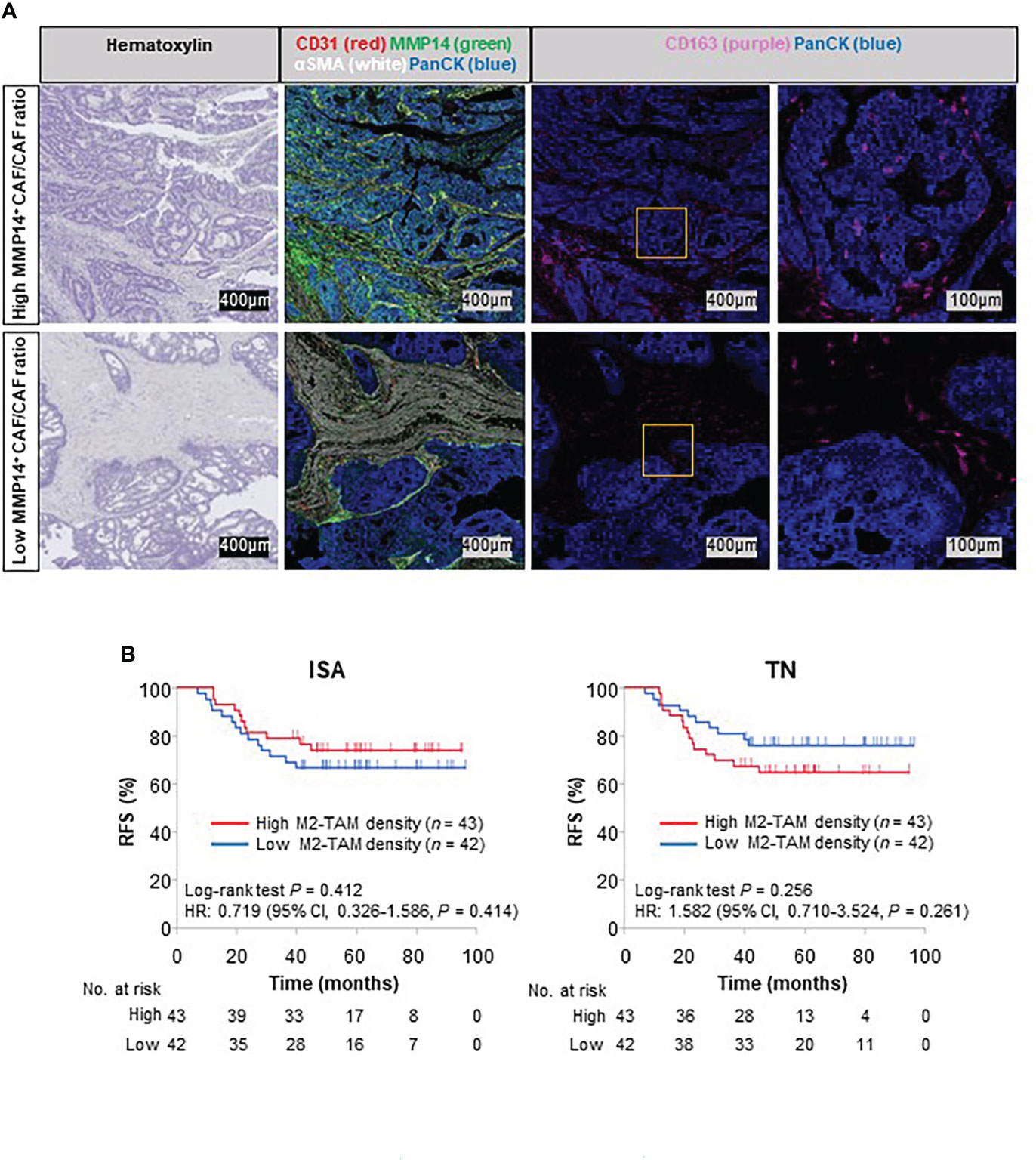
Figure 4 Association of M2-TAM distribution in tumors with MMP14 expression in CAFs and survival outcome. (A) Representative mIHC images showing the relation between MMP14-expressing CAFs and the spatial distribution of M2-TAMs. The regions within the yellow squares are shown at higher magnification in the corresponding images to the right. The upper and lower sets of images correspond to patients with a high or low MMP14+ CAF/CAF ratio, respectively. (B) Kaplan-Meier curves for RFS according to the median values of M2-TAM density in ISA (left) or TN (right) regions.
Recurrence of stage III colorectal cancer with a high MMP14+ CAF/CAF ratio is exacerbated by a low lymphocyte density in peri- and intratumoral stromal areas
To clarify the clinical importance of MMP14+ CAFs in stage III colorectal cancer, we performed a further detailed analysis taking into account both peritumoral and intratumoral lymphocytes, given that the prognostic relevance of these immune cells has been well-validated by the Immunoscore or similar scores in previous studies (15–19). Indeed, a higher conventional Immunoscore was associated with a longer RFS in our study cohort (HR of 0.504, with a 95% CI of 0.210–1.207) (Supplementary Figure S4A). However, our Tissue Segmentation method indicated that TILs in the TN were not relevant to tumor recurrence, whereas a higher density of those in stromal areas including the IF and ISA was associated with a longer RFS (Supplementary Figures S4B, C), possibly because lymphocytes were distributed mainly in these peritumoral and intratumoral stromal areas rather than in the TN (Supplementary Figure S4D). We therefore developed a modified Immunoscore based on CD3+ and CD8+ cell density only in IF and ISA regions, excluding the TN region that is included in the conventional Immunoscore. This IS-Immunoscore (IF-ISA–Immunoscore) was more clearly prognostic (HR of 0.304, with a 95% CI of 0.134–0.689) (Supplementary Figure S4E) than was the conventional Immunoscore (Supplementary Figure S4A).
We next performed multivariable analysis including clinically important baseline characteristics as well as the IS-Immunoscore as covariates to test further the prognostic relevance of MMP14+ CAFs. This analysis revealed that a high MMP14+ CAF/CAF ratio was independently associated with a worse RFS (HR of 2.926, with a 95% CI of 1.167–7.334), whereas a high IS-Immunoscore was an independent prognostic factor for a better RFS (HR of 0.277, with a 95% CI of 0.117–0.652) (Table 2). We therefore divided our stage III colorectal cancer patients into four groups according to these two independent biomarkers (IS-Immunoscore and MMP14+ CAF/CAF ratio). Among these four groups (high IS-Immunoscore and high MMP14+ CAF/CAF ratio, group A; high IS-Immunoscore and low MMP14+ CAF/CAF ratio, group B; low IS-Immunoscore and high MMP14+ CAF/CAF ratio, group C; low IS-Immunoscore and low MMP14+ CAF/CAF ratio, group D), group C showed the worst prognosis whereas the other three groups each showed a similarly better prognosis (Figure 5). Comparison of group C with the other three groups combined yielded a HR of 7.382 (95% CI, 3.309–16.469), indicative of the higher prognostic relevance of the combination of the IS-Immunoscore and MMP14+ CAF/CAF ratio.
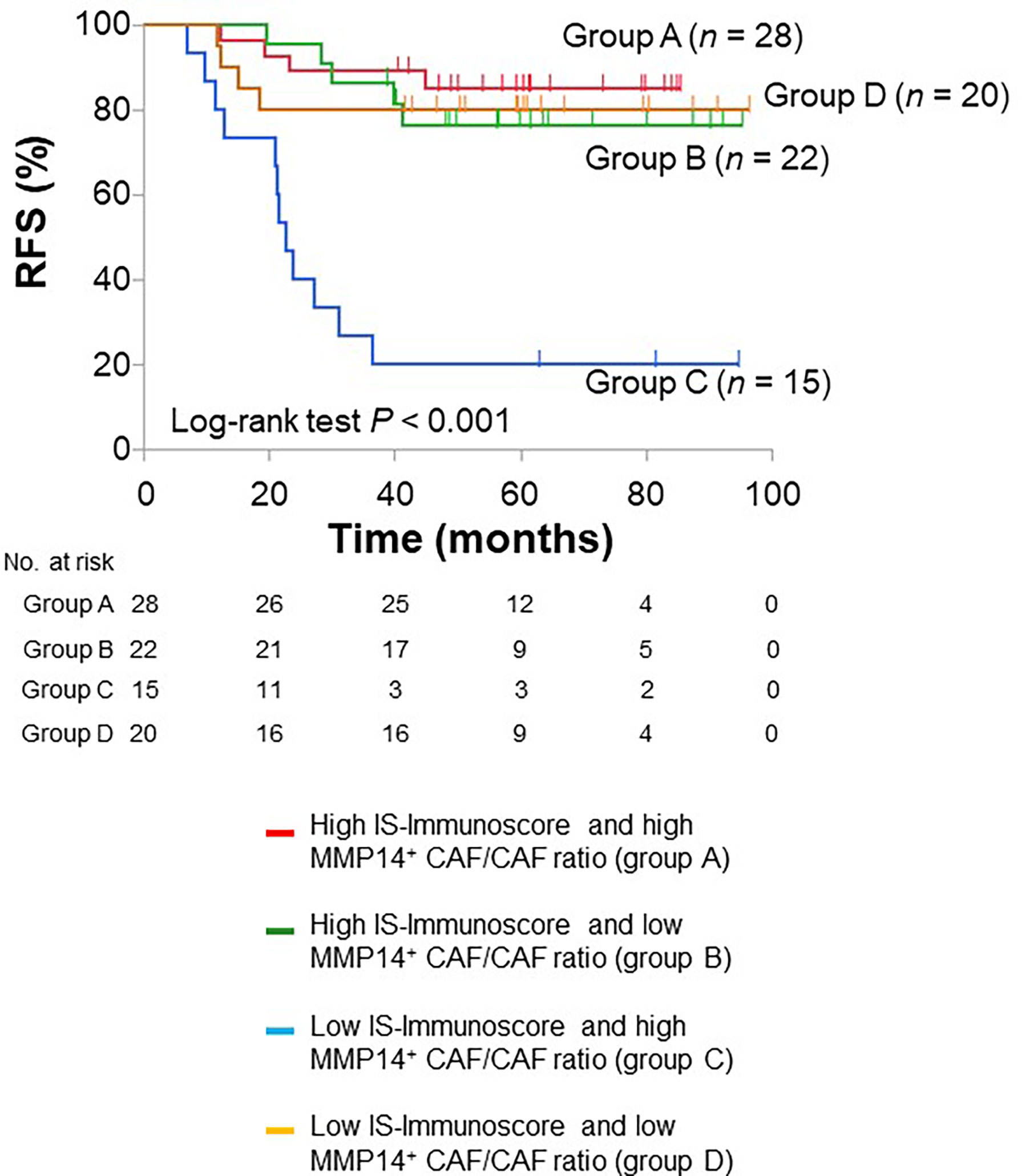
Figure 5 Kaplan-Meier curves for RFS based on the combination of the IS-Immunoscore and MMP14+ CAF/CAF ratio. The median value was used as the cutoff for the ratio.
A previous preclinical study suggested that MMP14 is associated with resistance to 5-FU treatment in colorectal cancer (32). We therefore analyzed RFS in patients with a high MMP14+ CAF/CAF ratio according to adjuvant chemotherapy regimen in order to investigate whether oxaliplatin is able to overcome the poor clinical outcome associated with MMP14+ CAFs. There was no significant difference in RFS between patients treated with 5-FU alone and those treated with both oxaliplatin and 5-FU (HR of 0.946, with a 95% CI of 0.355–2.524), however (Supplementary Figure S5), indicating that the addition of oxaliplatin to 5-FU did not improve RFS in these patients.
Discussion
Numerous studies have revealed an important role for nontumor cells in disease progression of solid cancers, but how these cells contribute to tumor recurrence has remained unclear for colorectal cancer. Our study now suggests that CAFs are a key player in postoperative recurrence of stage III colorectal cancer as a result of their expression of MMP14. In addition to our bioinformatics analysis of publicly available transcriptomic data suggesting the clinical relevance of MMP14 in association with tumorigenic cell lineages such as CAFs and TAMs to tumor recurrence, our single cell–based spatial profiling of the TME by mIHC analysis with a digital pathology platform allowed us to clarify that MMP14+ CAFs are a determinant of poor survival outcome in stage III colorectal cancer. This mIHC-based spatial profiling also suggested that the tumor aggressiveness conferred by MMP14+ CAFs might by explained, at least in part, by promotion of the infiltration of M2-TAMs into the TN by these cells. Of note, our simultaneous evaluation of the Immunoscore revealed that the prognostic role of MMP14+ CAFs was independent of that of TILs. We therefore propose a new and improved stratification of stage III colorectal cancer based on both MMP14+ CAFs and the Immunoscore.
We found that poor survival was related to MMP14 expression only in CAFs among the various cell types that express this protein. This observation is consistent with previous preclinical findings that expression and activation of MMP14 were detected predominantly in stromal cells (54), with MMP14 expressed in tumor cells remaining largely inactive (54), and that MMP14+ CAFs facilitate tumor progression (12, 37). Specific CAF phenotypes have been implicated in tumor progression (7, 55–58). CAFs with a myofibroblastic phenotype (myoCAFs) characterized by a contractile morphology and high αSMA expression were thus found to possess the highest protumorigenic activity, which was driven by endogenous transforming growth factor–β (TGF-β) expression (55). Given that CAFs were defined as αSMA-positive cells in our mIHC analysis, the association of MMP14+ CAFs with colorectal cancer progression might be explained by production of the active form of TGF-β mediated by the proteolytic activity of MMP14. MMP14 has also been shown to function as a key collagenase enzyme (59, 60), with ablation of MMP14 in adult mouse fibroblasts giving rise to a marked skin phenotype characterized by increased dermal thickness and tissue stiffness (59). Loss of MMP14 in fibroblasts was shown to affect melanoma growth by altering the composition of the peritumoral extracellular matrix (37, 61). Although we did not examine matrix components such as collagen and laminin, changes in the peritumoral matrix composition may have contributed to the modulation of tumor progression by MMP14. A preclinical study with a breast carcinoma cell line showed that TAMs also express MMP14 (62), consistent with our findings. Of interest, however, MMP14-expressing TAMs were not associated with tumor recurrence in our stage III colorectal cancer cohort. These observations collectively suggest that it is important to focus on specific cell types with regard to the relevance of MMP14 expression to tumor progression. The application of 12-color mIHC as in the present study may thus provide important information regarding the nature of colorectal cancer that complements transcriptome-based findings such as those underlying the CMS categorization.
Evidence has suggested a relation between CAFs and M2-TAMs (63). We here showed that MMP14 expression in CAFs was associated with the infiltration of M2-TAMs into the TN, suggesting that such expression may be a determinant of intratumoral M2-TAM activity in stage III colorectal cancer. The mechanism by which MMP14+ CAFs might contribute to M2-TAM accumulation remains unclear, but several previous studies support our current findings. Indeed, MMP14 was shown to increase the amount of the active form of TGF-β through cleavage of the latent form of this growth factor (64, 65), and the active form of TGF-β was found to promote the polarization of TAMs from the antitumor M1 phenotype to the protumorigenic M2 phenotype (66). In addition, a preclinical study showed that inhibition of MMP14 activity resulted in suppression of M2-TAMs and tumor regression (67). Of interest, we found that only M2-TAM infiltration into the TN, not that into the ISA, tended to be associated with a poor prognosis in stage III colorectal cancer, which is consistent with the previous finding that direct physical contact of M2-TAMs with tumor cells was important for the immunosuppressive and protumorigenic activity of M2-TAMs in human gastric cancer (68). In the current study, we adopted CD163 as an M2-TAM marker, although other molecules including CD206 (MRC1) and MRC2 are also expressed on these cells (69, 70) and are indicative of different M2-TAM phenotypes (47, 68, 71). Further research is therefore needed to determine how MMP14 contributes to colorectal cancer progression through regulation of M2-TAM activity.
Our spatial profiling analysis also indicated that T cells in stromal areas, but not those in the TN, play an important role in prevention of tumor recurrence in stage III colorectal cancer, leading us to propose the definition of a modified Immunoscore, the IS-Immunoscore. The reason why T cells in the TN were not associated with survival outcome remains unclear. A reduced tendency of T cells to distribute to the TN is one possible explanation, although some specimens did show substantial infiltration of T cells in this region. The potential mechanisms by which intratumoral T cells might be inactivated in the TN therefore warrant further investigation.
There are several limitations to our study. First, it was retrospective in design and the original cohort was from a single university hospital, with our results thus requiring external validation. However, the results of our independent analysis of transcriptomic data in the TCGA database were consistent with our mIHC findings. Second, we were not able to evaluate CMS categories or genomic alterations such as microsatellite instability and KRAS and BRAF mutations that are important for precise characterization of colorectal cancer. Further investigations of the role of MMP14+ CAFs in the TME should thus include simultaneous transcriptomic and genomic profiling of colorectal tumors. Third, the TME consists of a complex meshwork of extracellular matrix macromolecules and a variety of interspersed cell types (72) including CAFs, blood vessel–associated smooth muscle cells, pericytes, endothelial cells, mesenchymal stem cells, and various kinds of immune cell (73), all of which potentially interact with each other. However, not all immune cell subsets that have been implicated in the survival outcome of colorectal cancer, such as regulatory T cells, were evaluated in our study because of the limited number of markers that could be examined by mIHC (74, 75). Fourth, MMP14 exists as active and inactive forms, with only the former being thought to contribute to the control of tumor growth and cancer cell invasion (76–81). However, immunocytochemistry does not discriminate between these active and inactive forms of MMP14, and our mIHC analysis thus did not allow tracing of cellular MMP14 activity (82). And fifth, several proteins have been identified as markers for CAFs, including αSMA, fibroblast-specific protein 1 (FSP1), vimentin, desmin, fibroblast activation protein (FAP), and platelet-derived growth factor receptor (PDGFR) (22, 83). Although CAFs in CRC were shown to express αSMA at a higher level compared with other fibroblasts (84), we examined only αSMA in our study. Other CAF subsets should thus be examined in the future.
In summary, our study has suggested that MMP14+ CAFs play an important role in tumor progression and are therefore a potential therapeutic target in stage III colorectal cancer. Our single cell–based spatial profiling analysis by 12-color mIHC and a digital pathology platform allowed us to identify MMP14+ CAFs among the various cellular components of the TME including T cells and TAMs as a determinant of tumorigenic activity. Further studies are warranted to develop new treatment strategies related to the role of MMP14+ CAFs in the progression of colorectal cancer.
Data availability statement
The original contributions presented in the study are included in the article/Supplementary Material. Further inquiries can be directed to the corresponding authors.
Ethics statement
This study was approved by the Institutional Review Board (R02-070) and the Ethics Committee of Kindai University Faculty of Medicine. Written informed consent for participation was not required for this study in accordance with the national legislation and the institutional requirements.
Author contributions
Conception and design, YM, KH, HK, and KN. Development of methodology, YM, KH, KY, and TT. Acquisition of data (such as management of patients and provision of facilities), YM, KH, HK, and JK. Analysis and interpretation of data (such as statistical analysis, biostatistics, and computational analysis), YM, KH, HK, AI, YC, and KN. Writing, review, and/or revision of the manuscript, YM, KH, and HK. Administrative, technical, or material support (such as reporting or organization of data and database construction), YM, KH, HK, KY, and TT. Study supervision, KH, HK, AI, JK, and KN. Funding, JK, and KN. All authors contributed to the article and approved the submitted version.
Acknowledgments
We thank Kyoko Itoh of the Department of Pathology and Applied Neurobiology, Kyoto Prefectural University of Medicine, as well as Hiroshi Ogi of SCREEN Holdings Co. Ltd. for technical support.
Conflict of interest
HK has received consulting fees from Bristol-Myers Squibb Co. Ltd., Eli Lilly Japan K.K., MSD K.K., Ono Pharmaceutical Co. Ltd., Daiichi-Sankyo Co. Ltd., and Taiho Pharmaceutical Co. Ltd.YC honoraria from Bristol-Myers Squibb Co. Ltd., Bayer Yakuhin Ltd., Eli Lilly Japan K.K., MSD K.K., Ono Pharmaceutical Co. Ltd., Chugai Pharmaceutical Co. Ltd., Daiichi Sankyo Co. Ltd., Merck Biopharma Co. Ltd., Takeda Pharmaceutical Co. Ltd., Yakult Pharmaceutical Industry, Teijin Pharma Ltd., and Taiho Pharmaceutical Co. Ltd.YC lecture fees from Glaxo Smith Kline K.K. and Otsuka Pharmaceutical Co. Ltd.YC and research funding from Chugai Pharmaceutical Co. Ltd., Taiho Pharmaceutical Co. Ltd., Kobayashi Pharmaceutical Co. Ltd., and Eisai Co. Ltd. TT has received speaker fees from MSD K.K., Ono Pharmaceutical Co. Ltd., and Bristol-Myers Squibb Co. Ltd. YC has received honoraria from Chugai Pharmaceutical Co. Ltd. KH has received lecture fees from AS ONE Corp., AstraZeneca K.K., Bristol-Myers Squibb Co. Ltd., MSD K.K., and Ono Pharmaceutical Co. Ltd. as well as research funding from AstraZeneca K.K. and MSD K.K. KN has received honoraria from Astellas Pharma Inc., Takeda Pharmaceutical Co. Ltd., Nanzando Co. Ltd., AstraZeneca K.K., Chugai Pharmaceutical Co. Ltd., Roche Diagnostics K.K., MSD K.K., Eli Lilly Japan K.K., Nippon Kayaku Co. Ltd., Daiichi Sankyo Co. Ltd., Novartis Pharma K.K., Kyowa Kirin Co. Ltd., Taiho Pharmaceutical Co. Ltd., Pfizer Japan Inc., AbbVie Inc., Bristol-Myers Squibb Co. Ltd., CareNet Inc., Amgen Inc., Medical Review Co. Ltd., Yodosha Co. Ltd., 3H Clinical Trial Inc., Thermo Fisher Scientific K.K., Hisamitsu Pharmaceutical Co. Inc., Nichi-Iko Pharmaceutical Co. Ltd., Kyorin Pharmaceutical Co. Ltd., Medicus Shuppan Publishers Co. Ltd., Nippon Boehringer Ingelheim Co. Ltd., Nikkei Business Publications Inc., Yomiuri Telecasting Corp., and Medical Mobile Communications Co. Ltd.YC research funding from MSD K.K., AstraZeneca K.K., Pfizer Japan Inc., ICON Japan K.K., Astellas Pharma Inc., Bayer Yakuhin Ltd., Takeda Pharmaceutical Co. Ltd., Novartis Pharma K.K., Otsuka Pharmaceutical Co. Ltd., Eli Lilly Japan K.K., EPS International Co. Ltd., Bristol Myers Squibb Co. Ltd., CMIC Shift Zero K.K., PRA Health Sciences, Taiho Pharmaceutical Co. Ltd., Eisai Co. Ltd., Merck Biopharma Co. Ltd., Parexel International Corp., Mochida Pharmaceutical Co. Ltd., Covance Japan Inc., Ono Pharmaceutical Co. Ltd., Kissei Pharmaceutical Co. Ltd., Medical Research Support, Sysmex Corp., GlaxoSmithKline K.K., Sanofi K.K., A2 Healthcare Corp., Kyowa Hakko Kirin Co. Ltd., Syneos Health, AbbVie Inc., EPS Corp., Pfizer R&D Japan G.K., Chugai Pharmaceutical Co. Ltd., Daiichi Sankyo Co. Ltd., PPD-SNBL K.K., Nippon Boehringer Ingelheim Co. Ltd., IQVIA Services Japan K.K./Quintiles Inc., Japan Clinical Research Operations, and SymBio Pharmaceuticals Ltd.YC and consulting fees from Astellas Pharma Inc., Takeda Pharmaceutical Co. Ltd., Eli Lilly Japan K.K., Pfizer Japan Inc., Kyorin Pharmaceutical Co. Ltd., and Ono Pharmaceutical Co. Ltd.
The remaining authors declare that the research was conducted in the absence of any commercial or financial relationships that could be construed as a potential conflict of interest.
Publisher’s note
All claims expressed in this article are solely those of the authors and do not necessarily represent those of their affiliated organizations, or those of the publisher, the editors and the reviewers. Any product that may be evaluated in this article, or claim that may be made by its manufacturer, is not guaranteed or endorsed by the publisher.
Supplementary material
The Supplementary Material for this article can be found online at: https://www.frontiersin.org/articles/10.3389/fonc.2022.956270/full#supplementary-material
References
1. Keum N, Giovannucci E. Global burden of colorectal cancer: Emerging trends, risk factors and prevention strategies. Nat Rev Gastroenterol Hepatol (2019) 16(12):713–32. doi: 10.1038/s41575-019-0189-8
2. Van Cutsem E, Cervantes A, Adam R, Sobrero A, Van Krieken JH, Aderka D, et al. Esmo consensus guidelines for the management of patients with metastatic colorectal cancer. Ann Oncol (2016) 27(8):1386–422. doi: 10.1093/annonc/mdw235
3. Carlomagno C, De Stefano A, Rosanova M, De Falco S, Attademo L, Fiore G, et al. Multiple treatment lines and prognosis in metastatic colorectal cancer patients. Cancer Metastasis Rev (2019) 38(1-2):307–13. doi: 10.1007/s10555-018-9748-7
4. Piawah S, Venook AP. Targeted therapy for colorectal cancer metastases: A review of current methods of molecularly targeted therapy and the use of tumor biomarkers in the treatment of metastatic colorectal cancer. Cancer (2019) 125(23):4139–47. doi: 10.1002/cncr.32163
5. Zhu GX, Gao D, Shao ZZ, Chen L, Ding WJ, Yu QF. Wnt/Betacatenin signaling: Causes and treatment targets of drug resistance in colorectal cancer (Review). Mol Med Rep (2021) 23(2);105. doi: 10.3892/mmr.2020.11744
6. Grothey A, Fakih M, Tabernero J. Management of braf-mutant metastatic colorectal cancer: A review of treatment options and evidence-based guidelines. Ann Oncol (2021) 32(8):959–67. doi: 10.1016/j.annonc.2021.03.206
7. Nurmik M, Ullmann P, Rodriguez F, Haan S, Letellier E. In search of definitions: Cancer-associated fibroblasts and their markers. Int J Cancer (2020) 146(4):895–905. doi: 10.1002/ijc.32193
8. Becker LM, LeBleu VS. Endoglin targeting in colorectal tumor microenvironment. Clin Cancer Res (2018) 24(24):6110–1. doi: 10.1158/1078-0432.CCR-18-2023
9. Wu T, Dai Y. Tumor microenvironment and therapeutic response. Cancer Lett (2017) 387:61–8. doi: 10.1016/j.canlet.2016.01.043
10. Senthebane DA, Rowe A, Thomford NE, Shipanga H, Munro D, Mazeedi M, et al. The role of tumor microenvironment in chemoresistance: To survive, keep your enemies closer. Int J Mol Sci (2017) 18(7):1586. doi: 10.3390/ijms18071586
11. Mao X, Xu J, Wang W, Liang C, Hua J, Liu J, et al. Crosstalk between cancer-associated fibroblasts and immune cells in the tumor microenvironment: New findings and future perspectives. Mol Cancer (2021) 20(1):131. doi: 10.1186/s12943-021-01428-1
12. Winkler J, Abisoye-Ogunniyan A, Metcalf KJ, Werb Z. Concepts of extracellular matrix remodelling in tumour progression and metastasis. Nat Commun (2020) 11(1):5120. doi: 10.1038/s41467-020-18794-x
13. Soldevilla B, Carretero-Puche C, Gomez-Lopez G, Al-Shahrour F, Riesco MC, Gil-Calderon B, et al. The correlation between immune subtypes and consensus molecular subtypes in colorectal cancer identifies novel tumour microenvironment profiles, with prognostic and therapeutic implications. Eur J Cancer (2019) 123:118–29. doi: 10.1016/j.ejca.2019.09.008
14. Kalluri R. The biology and function of fibroblasts in cancer. Nat Rev Cancer (2016) 16(9):582–98. doi: 10.1038/nrc.2016.73
15. Pagès F, Mlecnik B, Marliot F, Bindea G, Ou F-S, Bifulco C, et al. International validation of the consensus immunoscore for the classification of colon cancer: A prognostic and accuracy study. Lancet (2018) 391(10135):2128–39. doi: 10.1016/s0140-6736(18)30789-x
16. Guo G, Wang Y, Zhou Y, Quan Q, Zhang Y, Wang H, et al. Immune cell concentrations among the primary tumor microenvironment in colorectal cancer patients predicted by clinicopathologic characteristics and blood indexes. J Immunother Cancer (2019) 7(1):179. doi: 10.1186/s40425-019-0656-3
17. Mlecnik B, Bifulco C, Bindea G, Marliot F, Lugli A, Lee JJ, et al. Multicenter international society for immunotherapy of cancer study of the consensus immunoscore for the prediction of survival and response to chemotherapy in stage iii colon cancer. J Clin Oncol (2020) 38(31):3638–51. doi: 10.1200/JCO.19.03205
18. Malka D, Lievre A, Andre T, Taieb J, Ducreux M, Bibeau F. Immune scores in colorectal cancer: Where are we? Eur J Cancer (2020) 140:105–18. doi: 10.1016/j.ejca.2020.08.024
19. Angell HK, Bruni D, Barrett JC, Herbst R, Galon J. The immunoscore: Colon cancer and beyond. Clin Cancer Res (2020) 26(2):332–9. doi: 10.1158/1078-0432.CCR-18-1851
20. Guinney J, Dienstmann R, Wang X, de Reynies A, Schlicker A, Soneson C, et al. The consensus molecular subtypes of colorectal cancer. Nat Med (2015) 21(11):1350–6. doi: 10.1038/nm.3967
21. Costa A, Kieffer Y, Scholer-Dahirel A, Pelon F, Bourachot B, Cardon M, et al. Fibroblast heterogeneity and immunosuppressive environment in human breast cancer. Cancer Cell (2018) 33(3):463–79 e10. doi: 10.1016/j.ccell.2018.01.011
22. Gunaydin G. Cafs interacting with tams in tumor microenvironment to enhance tumorigenesis and immune evasion. Front Oncol (2021) 11:668349. doi: 10.3389/fonc.2021.668349
23. Monteran L, Erez N. The dark side of fibroblasts: Cancer-associated fibroblasts as mediators of immunosuppression in the tumor microenvironment. Front Immunol (2019) 10:1835. doi: 10.3389/fimmu.2019.01835
24. Chen Y, McAndrews KM, Kalluri R. Clinical and therapeutic relevance of cancer-associated fibroblasts. Nat Rev Clin Oncol (2021) 18(12):792–804. doi: 10.1038/s41571-021-00546-5
25. Erez N, Truitt M, Olson P, Arron ST, Hanahan D. Cancer-associated fibroblasts are activated in incipient neoplasia to orchestrate tumor-promoting inflammation in an nf-Kappab-Dependent manner. Cancer Cell (2010) 17(2):135–47. doi: 10.1016/j.ccr.2009.12.041
26. Augsten M, Sjoberg E, Frings O, Vorrink SU, Frijhoff J, Olsson E, et al. Cancer-associated fibroblasts expressing Cxcl14 rely upon Nos1-derived nitric oxide signaling for their tumor-supporting properties. Cancer Res (2014) 74(11):2999–3010. doi: 10.1158/0008-5472.CAN-13-2740
27. Cohen N, Shani O, Raz Y, Sharon Y, Hoffman D, Abramovitz L, et al. Fibroblasts drive an immunosuppressive and growth-promoting microenvironment in breast cancer Via secretion of chitinase 3-like 1. Oncogene (2017) 36(31):4457–68. doi: 10.1038/onc.2017.65
28. Sun X, He X, Zhang Y, Hosaka K, Andersson P, Wu J, et al. Inflammatory cell-derived Cxcl3 promotes pancreatic cancer metastasis through a novel myofibroblast-hijacked cancer escape mechanism. Gut (2021) 71(1):129–47. doi: 10.1136/gutjnl-2020-322744
29. Zhao Q, Huang L, Qin G, Qiao Y, Ren F, Shen C, et al. Cancer-associated fibroblasts induce monocytic myeloid-derived suppressor cell generation Via il-6/Exosomal mir-21-Activated Stat3 signaling to promote cisplatin resistance in esophageal squamous cell carcinoma. Cancer Lett (2021) 518:35–48. doi: 10.1016/j.canlet.2021.06.009
30. Li Y, Kuscu C, Banach A, Zhang Q, Pulkoski-Gross A, Kim D, et al. Mir-181a-5p inhibits cancer cell migration and angiogenesis Via downregulation of matrix metalloproteinase-14. Cancer Res (2015) 75(13):2674–85. doi: 10.1158/0008-5472.CAN-14-2875
31. Afik R, Zigmond E, Vugman M, Klepfish M, Shimshoni E, Pasmanik-Chor M, et al. Tumor macrophages are pivotal constructors of tumor collagenous matrix. J Exp Med (2016) 213(11):2315–31. doi: 10.1084/jem.20151193
32. Ragusa S, Prat-Luri B, González-Loyola A, Nassiri S, Squadrito ML, Guichard A, et al. Antiangiogenic immunotherapy suppresses desmoplastic and chemoresistant intestinal tumors in mice. J Clin Invest (2020) 130(3):1199–216. doi: 10.1172/jci129558
33. Cui G, Cai F, Ding Z, Gao L. Mmp14 predicts a poor prognosis in patients with colorectal cancer. Hum Pathol (2019) 83:36–42. doi: 10.1016/j.humpath.2018.03.030
34. Brierley J, Gospodarowicz M, Wittekind C. Tnm classification of malignant tumours. (2017) Wiley: Chichester.
35. Tsujikawa T, Kumar S, Borkar RN, Azimi V, Thibault G, Chang YH, et al. Quantitative multiplex immunohistochemistry reveals myeloid-inflamed tumor-immune complexity associated with poor prognosis. Cell Rep (2017) 19(1):203–17. doi: 10.1016/j.celrep.2017.03.037
36. Tsujikawa T, Crocenzi T, Durham JN, Sugar EA, Wu AA, Onners B, et al. Evaluation of Cyclophosphamide/Gvax pancreas followed by listeria-mesothelin (Crs-207) with or without nivolumab in patients with pancreatic cancer. Clin Cancer Res (2020) 26(14):3578–88. doi: 10.1158/1078-0432.CCR-19-3978
37. Pach E, Brinckmann J, Rubsam M, Kumper M, Mauch C, Zigrino P. Fibroblast Mmp14-dependent collagen processing is necessary for melanoma growth. Cancers (Basel) (2021) 13(8):1984. doi: 10.3390/cancers13081984
38. Reichling C, Taieb J, Derangere V, Klopfenstein Q, Le Malicot K, Gornet JM, et al. Artificial intelligence-guided tissue analysis combined with immune infiltrate assessment predicts stage iii colon cancer outcomes in Petacc08 study. Gut (2020) 69(4):681–90. doi: 10.1136/gutjnl-2019-319292
39. Yoshimura K, Tsujikawa T, Mitsuda J, Ogi H, Saburi S, Ohmura G, et al. Spatial profiles of intratumoral pd-1(+) helper T cells predict prognosis in head and neck squamous cell carcinoma. Front Immunol (2021) 12:769534. doi: 10.3389/fimmu.2021.769534
40. Subramanian A, Tamayo P, Mootha VK, Mukherjee S, Ebert BL, Gillette MA, et al. Gene set enrichment analysis: A knowledge-based approach for interpreting genome-wide expression profiles. Proc Natl Acad Sci U.S.A. (2005) 102(43):15545–50. doi: 10.1073/pnas.0506580102
41. Newman AM, Steen CB, Liu CL, Gentles AJ, Chaudhuri AA, Scherer F, et al. Determining cell type abundance and expression from bulk tissues with digital cytometry. Nat Biotechnol (2019) 37(7):773–82. doi: 10.1038/s41587-019-0114-2
42. Allen WL, Dunne PD, McDade S, Scanlon E, Loughrey M, Coleman H, et al. Transcriptional subtyping and Cd8 immunohistochemistry identifies poor prognosis stage Ii/Iii colorectal cancer patients who benefit from adjuvant chemotherapy. JCO Precis Oncol (2018) 2018:PO.17.00241. doi: 10.1200/PO.17.00241
43. Danielsen HE, Hveem TS, Domingo E, Pradhan M, Kleppe A, Syvertsen RA, et al. Prognostic markers for colorectal cancer: Estimating ploidy and stroma. Ann Oncol (2018) 29(3):616–23. doi: 10.1093/annonc/mdx794
44. Simillis C, Singh H, Afxentiou T, Mills S, Warren OJ, Smith JJ, et al. Postoperative chemotherapy improves survival in patients with resected high-risk stage ii colorectal cancer: Results of a systematic review and meta-analysis. Colorectal Dis (2020) 22(10):1231–44. doi: 10.1111/codi.14994
45. Parent P, Cohen R, Rassy E, Svrcek M, Taieb J, Andre T, et al. A comprehensive overview of promising biomarkers in stage ii colorectal cancer. Cancer Treat Rev (2020) 88:102059. doi: 10.1016/j.ctrv.2020.102059
46. Lee H, Sodek KL, Hwang Q, Brown TJ, Ringuette M, Sodek J. Phagocytosis of collagen by fibroblasts and invasive cancer cells is mediated by Mt1-mmp. Biochem Soc Trans (2007) 35(Pt 4):704–6. doi: 10.1042/BST0350704
47. Ji J, Upadhyay S, Xiong X, Malmlof M, Sandstrom T, Gerde P, et al. Multi-cellular human bronchial models exposed to diesel exhaust particles: Assessment of inflammation, oxidative stress and macrophage polarization. Part Fibre Toxicol (2018) 15(1):19. doi: 10.1186/s12989-018-0256-2
48. Georgoudaki AM, Prokopec KE, Boura VF, Hellqvist E, Sohn S, Ostling J, et al. Reprogramming tumor-associated macrophages by antibody targeting inhibits cancer progression and metastasis. Cell Rep (2016) 15(9):2000–11. doi: 10.1016/j.celrep.2016.04.084
49. Zhang Y, Du W, Chen Z, Xiang C. Upregulation of pd-L1 by Spp1 mediates macrophage polarization and facilitates immune escape in lung adenocarcinoma. Exp Cell Res (2017) 359(2):449–57. doi: 10.1016/j.yexcr.2017.08.028
50. Dong B, Wu C, Huang L, Qi Y. Macrophage-related Spp1 as a potential biomarker for early lymph node metastasis in lung adenocarcinoma. Front Cell Dev Biol (2021) 9:739358. doi: 10.3389/fcell.2021.739358
51. Tcyganov E, Mastio J, Chen E, Gabrilovich DI. Plasticity of myeloid-derived suppressor cells in cancer. Curr Opin Immunol (2018) 51:76–82. doi: 10.1016/j.coi.2018.03.009
52. Gabrilovich DI. Myeloid-derived suppressor cells. Cancer Immunol Res (2017) 5(1):3–8. doi: 10.1158/2326-6066.Cir-16-0297
53. Kumar V, Donthireddy L, Marvel D, Condamine T, Wang F, Lavilla-Alonso S, et al. Cancer-associated fibroblasts neutralize the anti-tumor effect of Csf1 receptor blockade by inducing pmn-mdsc infiltration of tumors. Cancer Cell (2017) 32(5):654–68 e5. doi: 10.1016/j.ccell.2017.10.005
54. Amara N, Tholen M, Bogyo M. Chemical tools for selective activity profiling of endogenously expressed mmp-14 in multicellular models. ACS Chem Biol (2018) 13(9):2645–54. doi: 10.1021/acschembio.8b00562
55. Sahai E, Astsaturov I, Cukierman E, DeNardo DG, Egeblad M, Evans RM, et al. A framework for advancing our understanding of cancer-associated fibroblasts. Nat Rev Cancer (2020) 20(3):174–86. doi: 10.1038/s41568-019-0238-1
56. Fridman WH, Miller I, Sautes-Fridman C, Byrne AT. Therapeutic targeting of the colorectal tumor stroma. Gastroenterology (2020) 158(2):303–21. doi: 10.1053/j.gastro.2019.09.045
57. Yoshida GJ. Regulation of heterogeneous cancer-associated fibroblasts: The molecular pathology of activated signaling pathways. J Exp Clin Cancer Res (2020) 39(1):112. doi: 10.1186/s13046-020-01611-0
58. Gok Yavuz B, Gunaydin G, Gedik ME, Kosemehmetoglu K, Karakoc D, Ozgur F, et al. Cancer associated fibroblasts sculpt tumour microenvironment by recruiting monocytes and inducing immunosuppressive pd-1(+) tams. Sci Rep (2019) 9(1):3172. doi: 10.1038/s41598-019-39553-z
59. Zigrino P, Brinckmann J, Niehoff A, Lu Y, Giebeler N, Eckes B, et al. Fibroblast-derived mmp-14 regulates collagen homeostasis in adult skin. J Invest Dermatol (2016) 136(8):1575–83. doi: 10.1016/j.jid.2016.03.036
60. Fields GB. Interstitial collagen catabolism. J Biol Chem (2013) 288(13):8785–93. doi: 10.1074/jbc.R113.451211
61. Pach E, Kumper M, Fromme JE, Zamek J, Metzen F, Koch M, et al. Extracellular matrix remodeling by fibroblast-Mmp14 regulates melanoma growth. Int J Mol Sci (2021) 22(22):12276. doi: 10.3390/ijms222212276
62. Ager EI, Kozin SV, Kirkpatrick ND, Seano G, Kodack DP, Askoxylakis V, et al. Blockade of Mmp14 activity in murine breast carcinomas: Implications for macrophages, vessels, and radiotherapy. J Natl Cancer Inst (2015) 107(4):djv017. doi: 10.1093/jnci/djv017
63. Cho H, Seo Y, Loke KM, Kim SW, Oh SM, Kim JH, et al. Cancer-stimulated cafs enhance monocyte differentiation and protumoral Tam activation Via Il6 and gm-csf secretion. Clin Cancer Res (2018) 24(21):5407–21. doi: 10.1158/1078-0432.CCR-18-0125
64. Karsdal MA, Larsen L, Engsig MT, Lou H, Ferreras M, Lochter A, et al. Matrix metalloproteinase-dependent activation of latent transforming growth factor-beta controls the conversion of osteoblasts into osteocytes by blocking osteoblast apoptosis. J Biol Chem (2002) 277(46):44061–7. doi: 10.1074/jbc.M207205200
65. Nguyen HL, Kadam P, Helkin A, Cao K, Wu S, Samara GJ, et al. Mt1-mmp activation of tgf-beta signaling enables intercellular activation of an epithelial-mesenchymal transition program in cancer. Curr Cancer Drug Targets (2016) 16(7):618–30. doi: 10.2174/1568009616666160216125634
66. Zhang F, Wang H, Wang X, Jiang G, Liu H, Zhang G, et al. Tgf-beta induces M2-like macrophage polarization Via snail-mediated suppression of a pro-inflammatory phenotype. Oncotarget (2016) 7(32):52294–306. doi: 10.18632/oncotarget.10561
67. Winer A, Adams S, Mignatti P. Matrix metalloproteinase inhibitors in cancer therapy: Turning past failures into future successes. Mol Cancer Ther (2018) 17(6):1147–55. doi: 10.1158/1535-7163.MCT-17-0646
68. Huang YK, Wang M, Sun Y, Di Costanzo N, Mitchell C, Achuthan A, et al. Macrophage spatial heterogeneity in gastric cancer defined by multiplex immunohistochemistry. Nat Commun (2019) 10(1):3928. doi: 10.1038/s41467-019-11788-4
69. Vayrynen JP, Haruki K, Lau MC, Vayrynen SA, Zhong R, Dias Costa A, et al. The prognostic role of macrophage polarization in the colorectal cancer microenvironment. Cancer Immunol Res (2021) 9(1):8–19. doi: 10.1158/2326-6066.CIR-20-0527
70. Yang C, Wei C, Wang S, Shi D, Zhang C, Lin X, et al. Elevated Cd163(+)/Cd68(+) ratio at tumor invasive front is closely associated with aggressive phenotype and poor prognosis in colorectal cancer. Int J Biol Sci (2019) 15(5):984–98. doi: 10.7150/ijbs.29836
71. Nielsen MC, Hvidbjerg Gantzel R, Claria J, Trebicka J, Moller HJ, Gronbaek H. Macrophage activation markers, Cd163 and Cd206, in acute-on-Chronic liver failure. Cells (2020) 9(5):1175. doi: 10.3390/cells9051175
72. Zeltz C, Primac I, Erusappan P, Alam J, Noel A, Gullberg D. Cancer-associated fibroblasts in desmoplastic tumors: Emerging role of integrins. Semin Cancer Biol (2020) 62:166–81. doi: 10.1016/j.semcancer.2019.08.004
73. Taube JM, Akturk G, Angelo M, Engle EL, Gnjatic S, Greenbaum S, et al. The society for immunotherapy of cancer statement on best practices for multiplex immunohistochemistry (Ihc) and immunofluorescence (If) staining and validation. J Immunother Cancer (2020) 8(1):e000155. doi: 10.1136/jitc-2019-000155
74. Zhang L, Yu X, Zheng L, Zhang Y, Li Y, Fang Q, et al. Lineage tracking reveals dynamic relationships of T cells in colorectal cancer. Nature (2018) 564(7735):268–72. doi: 10.1038/s41586-018-0694-x
75. Saito T, Nishikawa H, Wada H, Nagano Y, Sugiyama D, Atarashi K, et al. Two Foxp3(+)Cd4(+) T cell subpopulations distinctly control the prognosis of colorectal cancers. Nat Med (2016) 22(6):679–84. doi: 10.1038/nm.4086
76. Hotary K, Allen E, Punturieri A, Yana I, Weiss SJ. Regulation of cell invasion and morphogenesis in a three-dimensional type I collagen matrix by membrane-type matrix metalloproteinases 1, 2, and 3. J Cell Biol (2000) 149(6):1309–23. doi: 10.1083/jcb.149.6.1309
77. Hotary K, Li XY, Allen E, Stevens SL, Weiss SJ. A cancer cell metalloprotease triad regulates the basement membrane transmigration program. Genes Dev (2006) 20(19):2673–86. doi: 10.1101/gad.1451806
78. Hotary KB, Allen ED, Brooks PC, Datta NS, Long MW, Weiss SJ. Membrane type I matrix metalloproteinase usurps tumor growth control imposed by the three-dimensional extracellular matrix. Cell (2003) 114(1):33–45. doi: 10.1016/s0092-8674(03)00513-0
79. Hotary KB, Yana I, Sabeh F, Li XY, Holmbeck K, Birkedal-Hansen H, et al. Matrix metalloproteinases (Mmps) regulate fibrin-invasive activity Via Mt1-Mmp-Dependent and -independent processes. J Exp Med (2002) 195(3):295–308. doi: 10.1084/jem.20010815
80. Itoh Y, Seiki M. Mt1-mmp: A potent modifier of pericellular microenvironment. J Cell Physiol (2006) 206(1):1–8. doi: 10.1002/jcp.20431
81. Zhai Y, Hotary KB, Nan B, Bosch FX, Munoz N, Weiss SJ, et al. Expression of membrane type 1 matrix metalloproteinase is associated with cervical carcinoma progression and invasion. Cancer Res (2005) 65(15):6543–50. doi: 10.1158/0008-5472.CAN-05-0231
82. Remacle AG, Shiryaev SA, Golubkov VS, Freskos JN, Brown MA, Karwa AS, et al. Non-destructive and selective imaging of the functionally active, pro- invasive membrane type-1 matrix metalloproteinase (Mt1-mmp) enzyme in cancer cells. J Biol Chem (2013) 288(28):20568–80. doi: 10.1074/jbc.M113.471508
83. Han C, Liu T, Yin R. Biomarkers for cancer-associated fibroblasts. biomark Res (2020) 8(1):64. doi: 10.1186/s40364-020-00245-w
Keywords: colorectal cancer, matrix metalloproteinase 14 (MMP14), multiplex immunohistochemistry (mIHC), cancer-associated fibroblast (CAF), M2 tumor-associated macrophages (M2-TAMs)
Citation: Makutani Y, Kawakami H, Tsujikawa T, Yoshimura K, Chiba Y, Ito A, Kawamura J, Haratani K and Nakagawa K (2022) Contribution of MMP14-expressing cancer-associated fibroblasts in the tumor immune microenvironment to progression of colorectal cancer. Front. Oncol. 12:956270. doi: 10.3389/fonc.2022.956270
Received: 30 May 2022; Accepted: 28 July 2022;
Published: 16 August 2022.
Edited by:
Aditi Banerjee, University of Maryland, Baltimore, United StatesReviewed by:
Gurcan Gunaydin, Hacettepe University, TurkeyKevin Dzobo, University of Cape Town, South Africa
Copyright © 2022 Makutani, Kawakami, Tsujikawa, Yoshimura, Chiba, Ito, Kawamura, Haratani and Nakagawa. This is an open-access article distributed under the terms of the Creative Commons Attribution License (CC BY). The use, distribution or reproduction in other forums is permitted, provided the original author(s) and the copyright owner(s) are credited and that the original publication in this journal is cited, in accordance with accepted academic practice. No use, distribution or reproduction is permitted which does not comply with these terms.
*Correspondence: Hisato Kawakami, a2F3YWthbWlfaEBtZWQua2luZGFpLmFjLmpw; Koji Haratani, aGFyYXRhbmlfa0BtZWQua2luZGFpLmFjLmpw