- 1Centre for Human Drug Research, Leiden, Netherlands
- 2Leiden University Medical Center, Leiden, Netherlands
- 3Division of BioTherapeutics, Leiden Academic Centre for Drug Research, Leiden University, Leiden, Netherlands
Aim: Traditionally, early phase clinical trials in oncology have been performed in patients based on safety risk-benefit assessment. Therapeutic transition to immuno-oncology may open new opportunities for studies in healthy volunteers, which are conducted faster and are less susceptible to confounders. Aim of this study was to investigate to what extent this approach is utilized and whether pharmacodynamic endpoints are evaluated in these early phase trials. We conducted a comprehensive review of clinical trials with healthy volunteers using immunotherapies potentially relevant for oncology.
Methods: Literature searches according to PRISMA guidelines and after registration in PROSPERO were conducted in PubMed, Embase, Web of Science and Cochrane databases with the cut-off date 20 October 2020, using search terms of relevant targets in immuno-oncology. Articles describing clinical trials with immunotherapeutics in healthy volunteers with a mechanism relevant for oncology were included. “Immunotherapeutic” was defined as compounds exhibiting effects through immunological targets. Data including study design and endpoints were extracted, with specific attention to pharmacodynamic endpoints and safety.
Results: In total, we found 38 relevant immunotherapeutic compounds tested in HVs, with 86% of studies investigating safety, 82% investigating the pharmacokinetics (PK) and 57% including at least one pharmacodynamic (PD) endpoint. Most of the observed adverse events (AEs) were Grade 1 and 2, consisting mostly of gastrointestinal, cutaneous and flu-like symptoms. Severe AEs were leukopenia, asthenia, syncope, headache, flu-like reaction and liver enzymes increase. PD endpoints investigated comprised of cytokines, immune and inflammatory biomarkers, cell counts, phenotyping circulating immune cells and ex vivo challenge assays.
Discussion: Healthy volunteer studies with immuno-oncology compounds have been performed, although not to a large extent. The integration of healthy volunteers in well-designed proof-of-mechanism oriented drug development programs has advantages and could be pursued more in the future, since integrative clinical trial protocols may facilitate early dose selection and prevent cancer patients to be exposed to non-therapeutic dosing regimens.
Systematic Review Registration: https://www.crd.york.ac.uk/prospero/display_record.php?RecordID=210861, identifier CRD42020210861
Introduction
The field of oncology is rapidly changing, with a major shift from broad-acting cytotoxic chemotherapy to drugs targeting specific molecular and immunological mechanisms (1–4). This is reflected by an ongoing increase in number of immuno-oncological agents in development, even during the COVID-19 pandemic (5). Where traditionally early phase clinical trials with oncological drugs were designed to find a maximum tolerated dose, today’s oncological drugs require a clinical development program based on pharmacologically active dose (PAD) or minimal anticipated biological effect level (MABEL), preferably guided by monitoring of the pharmacological activity (6). Since these drugs have a well-defined molecular target, target engagement and functional downstream effects can be quantified by state-of-the-art molecular and cellular techniques (7). Such an approach enables the evaluation of the relationship between pharmacokinetic (PK) and pharmacodynamic (PD) effects, and the selection of the biologically active dose for subsequent studies. Ideally, this is already done at the earliest clinical stages of drug development, in healthy volunteers (HVs) (8).
Traditionally, early phase clinical trials with non-specific oncological compounds were performed in patients (9). The mechanism of action of these broad-acting cytotoxic compounds did not support evaluation of drug effects in HVs for the obvious reason that the benefit-risk ratio was not acceptable. However, for (certain members of) the new class of targeted immunotherapies pharmacological activity can be evaluated in HVs (9–11). An initial pharmacological evaluation of a novel immuno-modulatory drug in HVs rather than in cancer patients avoids interference of concomitant medication, altered immune status or co-morbidities. Identification of the pharmacologically active dose in HVs would facilitate initial patient studies at selected dose levels and regimens that may translate into clinically desired effects. As such, complicated, inefficient, and time-consuming dose-finding studies in cancer patients could be avoided.
Of course, the benefit-risk assessment for certain immunomodulatory oncology drugs could be negative for HVs. Checkpoint inhibitors, for example CTLA-4 and PD-1 blockers, release the brakes that block the action of the immune system against the tumor. Unfortunately, these compounds also bear the risk for development of immune-related adverse events such as dermatologic, gastrointestinal, endocrine, or hepatic autoimmune reactions. Therefore, this class of compounds is commonly not evaluated in HVs. An alternative approach to enhance the action of the adaptive immune system against malignancies is via targeted stimulation of components of the innate immune system, since a fully functional antigen-specific response is dependent on efficient support by innate immune cells and cytokines. This can be reached by specific challenges of innate immune receptors and pathways, for example via interleukin receptors or toll-like receptors (TLRs). Whereas checkpoint inhibition theoretically may lead to wide-spread inflammation, targeted stimulation of specific innate immune pathways may result in desirable and well-controllable immune enhancement, which could be evaluated in a safe manner in HVs. We decided to review early phase clinical pharmacology studies with immunomodulatory compounds for oncological conditions addressing the following specific questions: which drug classes have been studied in HVs, did these studies only evaluate safety/tolerability and pharmacokinetics, or also pharmacodynamics, and if so, which type of biomarkers were used to evaluate the pharmacological activity. As a starting point, we selected relevant modes of action based on previously published literature (1, 2), and using the Landscape of Immuno-Oncology Drug Development tool (12).
Methods
We limited our evaluation to oncological compounds with an immunomodulatory mode of action, defined as modulation of a molecular/cellular immunological target. Relevant modes of action/targets were selected based on the recent drug overviews (1, 2), and by using the Landscape of Immuno-Oncology Drug Development tool (version 2020) (12). Drug targets selected are presented in Table 1, grouped by mechanism.
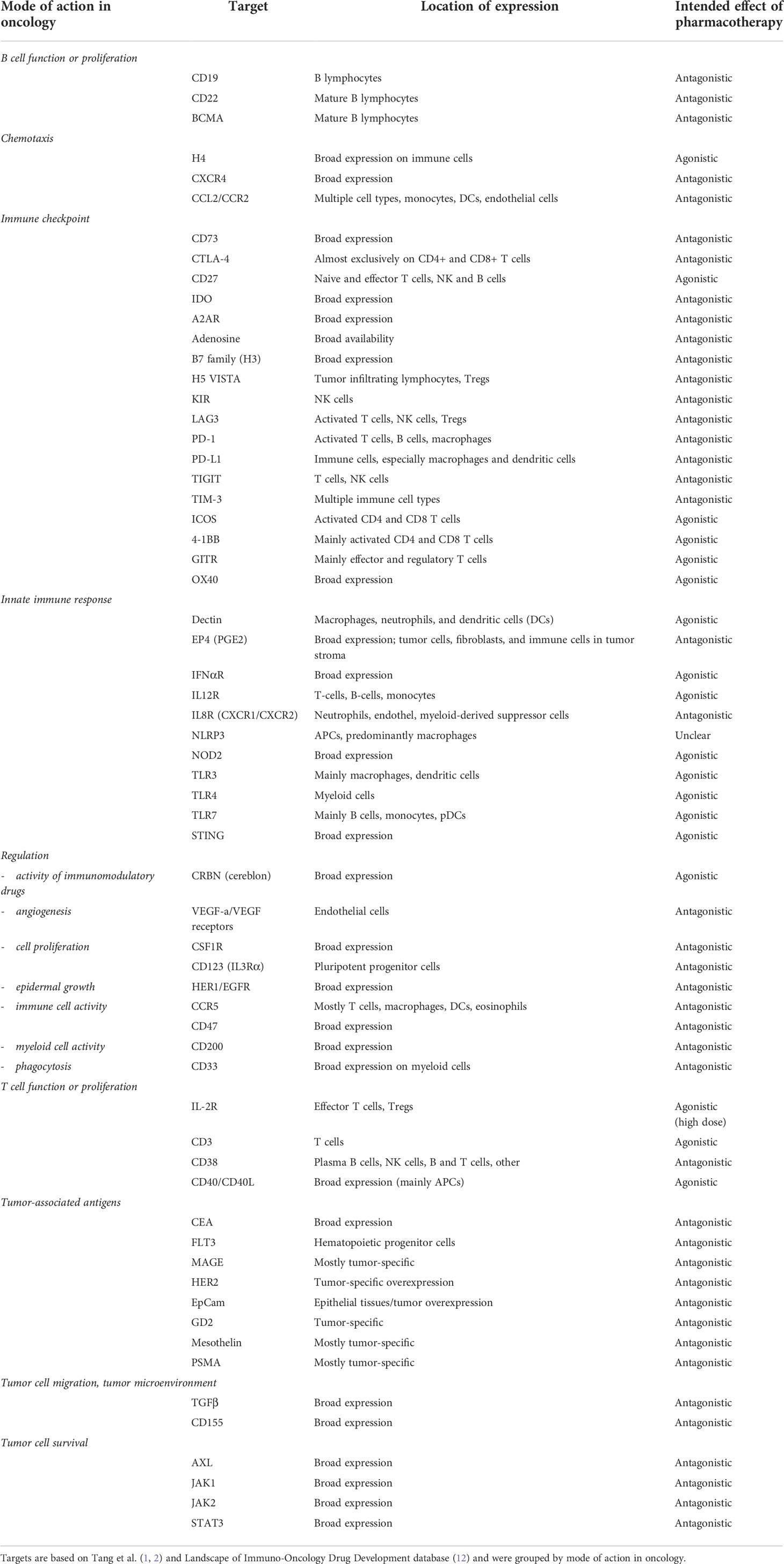
Table 1 Overview of the relevant oncology search targets, with their location of expression and intended effect of pharmacotherapy.
Search strategy
We conducted a comprehensive, electronic search to identify articles indexed in PubMed, Embase, Web of Science and Cochrane Library. The protocol was registered in the international register of systematic reviews (PROSPERO), in accordance with PRISMA guidelines (PROSPERO CRD42020210861) (13). Studies up to 20 October 2020 were extracted. We searched for “healthy volunteers”, “healthy subjects” and at least one of the drug targets as presented in Table 1, or alternative synonyms in titles and abstracts. Targets were grouped by their mode of action in oncology. Inclusion criteria were: 1) articles reporting the results of at least one clinical trial; 2) clinical trials conducted in healthy volunteers; 3) articles reporting the clinical evaluation of an immunotherapeutic agent, and the immunotherapeutic agent had a mode of action relevant for an oncological indication (considered relevant if confirmed by a journal publication, in which the possibility of the target in question was investigated or hypothesized), and 4) articles in English. Exclusion criteria were: 1) (systematic) reviews and metanalyses, or population PK studies; 2) articles reporting the results of studies in patients; 3) articles reporting the clinical evaluation of therapies not primarily acting through modulation of the immune system (e.g., tyrosine kinase inhibitor or antibodies such as trastuzumab; 4) articles without full-text availability. Although studies in HVs are primarily conducted during early phase (phase 1a) clinical research, we did not limit our search to only such studies, in order to conduct a more comprehensive review of the literature.
Data extraction
Relevant data were extracted from the included studies, including treatment, target, study design, study objectives, pharmacodynamic endpoints, number of enrolled subjects, safety/adverse events. Data were grouped and summarized per therapeutic category.
Results
Literature search
A total of 1593 unique entries were identified. Out of those, 158 articles passed the screening and were included for a full-text review. Finally, 73 articles fulfilled the inclusion/exclusion criteria and were included in the review. Figure 1 shows the PRISMA flow diagram with number of articles in each stage and reasons for exclusion.
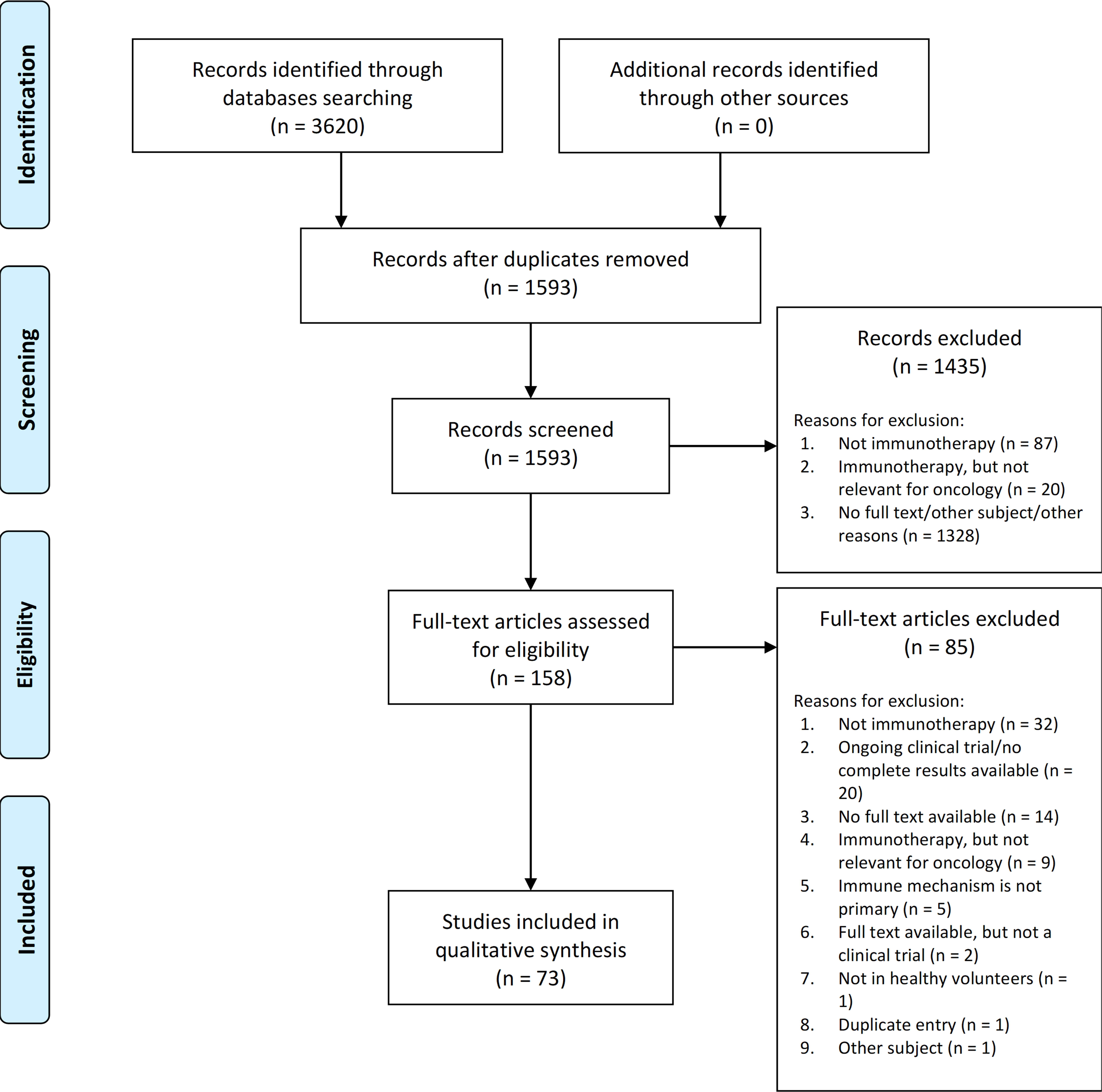
Figure 1 PRISMA diagram showing the total number of studies found by the search, screened, excluded (with reasons for exclusion at screening and full-text review) and included.
Compounds tested in healthy volunteers
A total of 38 different relevant compounds were evaluated in HV studies in 2352 HVs, based on our search. Studies and compounds are presented in Table 2, grouped by target mode of action in oncology and compound’s target/mechanism of action.
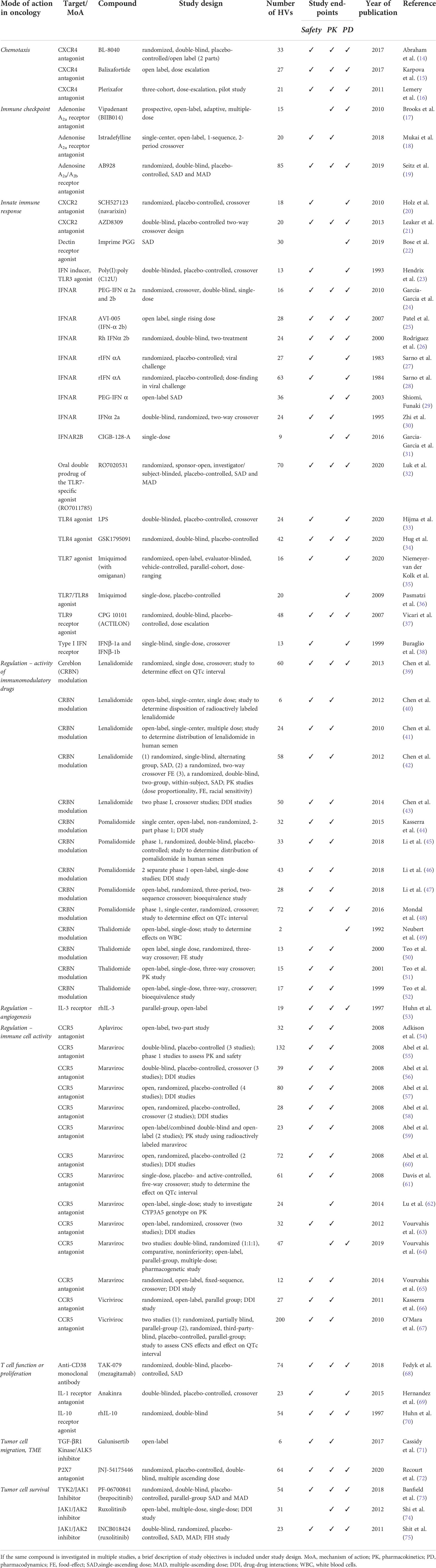
Table 2 Overview of the included clinical studies conducted in healthy volunteers (HVs) with a compound possibly relevant for immuno-oncology, with their corresponding study design and study endpoints, grouped by potential mode of action in oncology.
In terms of study endpoints, 86% of studies investigated the safety, 82% investigated the compound pharmacokinetics and 57% included evaluation of the pharmacodynamic endpoints in the study design. A full overview of the study design and endpoints can also be found in Table 2.
Most studies investigated compounds acting on the innate immune system (19 studies) (20–38), followed by compounds with immunoregulatory activity, classified into immunomodulatory (cereblon [CRBN] modulators; 14 studies) (39–44, 46–52) and mediators of immune cell functions (CCR5 antagonists; 14 studies) (54–59, 61–63, 65–67, 76). All the other compounds were investigated in only one or two HV studies. Overall, the studies included single doses, single ascending doses (SAD) and multiple ascending doses (MAD). Most studies were randomized controlled trials, although a substantial percentage (29%) of articles described a non-randomized trial.
Safety and tolerability in healthy volunteer studies
An overview of the safety findings in HV studies is provided in Table 3. Most of the observed adverse events (AE) were Grade 1 and 2, which included gastro-intestinal side effects (nausea, diarrhea, vomiting, constipation), flu-like symptoms (headache, fever, malaise) and cutaneous side effects (pruritus, erythema, dry skin).
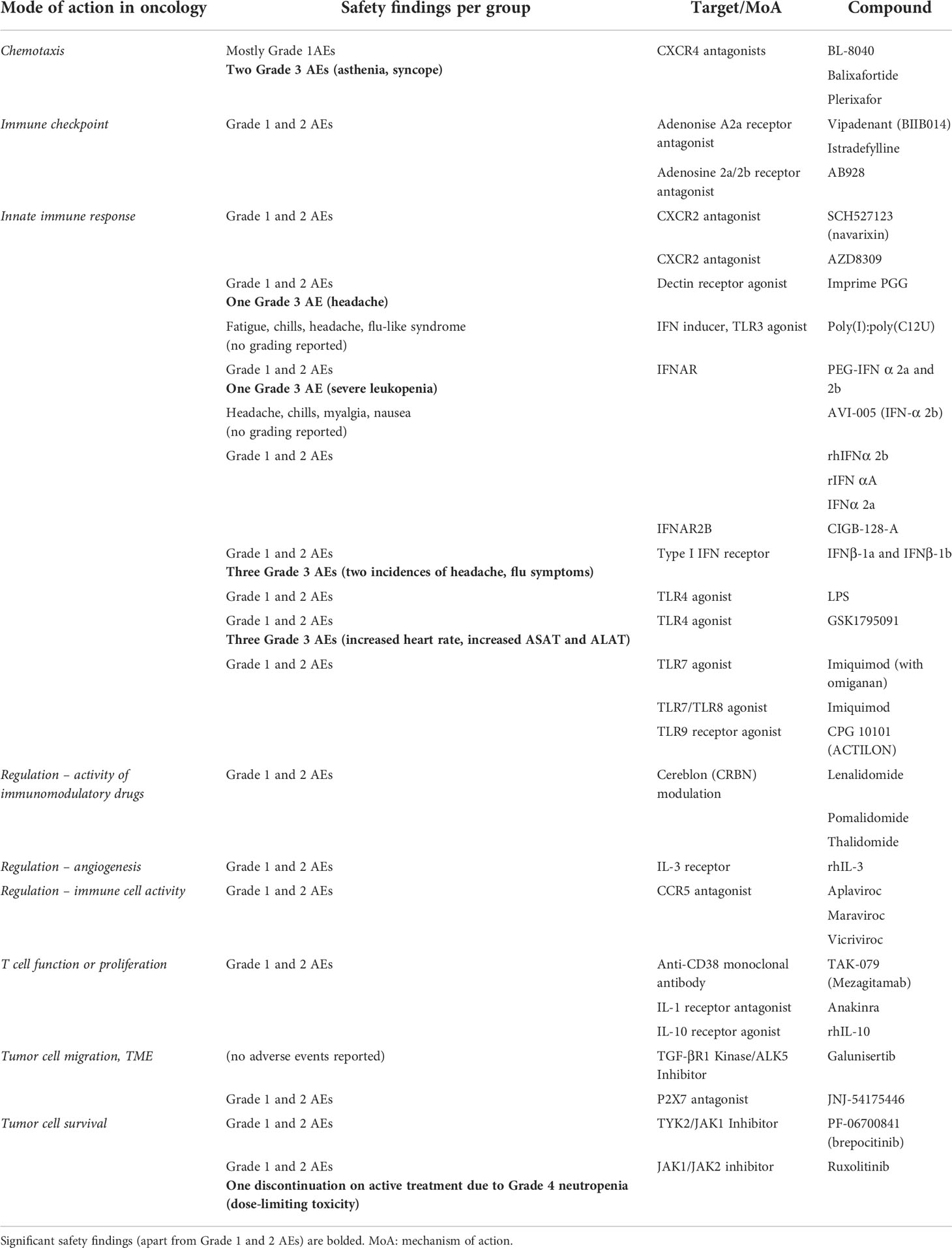
Table 3 Overview of safety findings in healthy volunteer studies of compounds with proposed mode of action for immuno-oncology.
Overall, there were no serious adverse events (SAE) which were assessed to be related to the study drug. There was a single case of dose-limiting Grade 4 leukopenia occurring in JAK1/JAK2 inhibitor ruxolitinib (75). Severe AEs were observed in the chemotaxis category (asthenia and syncope with a CXCR4 antagonist) and with compounds eliciting innate immune response (severe headache, flu-like symptoms and leukopenia with interferons; increased heart rate, increased ASAT and ALAT with TLR agonists; severe headache with dectin receptor agonist Imprime PGG). There were no severe adverse events observed in other categories, including immune checkpoint inhibitor, drugs with regulatory/immunomodulatory activity, drugs acting on T cell function or proliferation and drugs with presumed effect on tumor cell migration and tumor microenvironment.
Pharmacodynamic effect evaluation in healthy volunteer studies
Pharmacodynamic endpoints evaluated in studies with compounds possibly relevant for immuno-oncology were categorized by mechanism of action and summarized in Table 4. In total, there were 27 compounds for which at least one PD endpoint was investigated. All compounds except imiquimod were administered systemically. An overview of the studies evaluating PD endpoints per target group is presented in the earlier discussed Figure 2. The majority of HV studies with compounds targeting the innate immune response (consisting of CXCR2 antagonists, dectin receptor antagonist, interferons TLR agonists and P2X7 antagonist) included at least one PD endpoint (18 out of 19 studies) (20–38). Overall, most studies aimed to evaluate the effect of the investigational compound on circulating cytokine/chemokine levels, immune and inflammatory parameters and biomarkers in blood, cell counts, immunophenotype of circulating immune cells, and on the response to an ex vivo immune challenge.
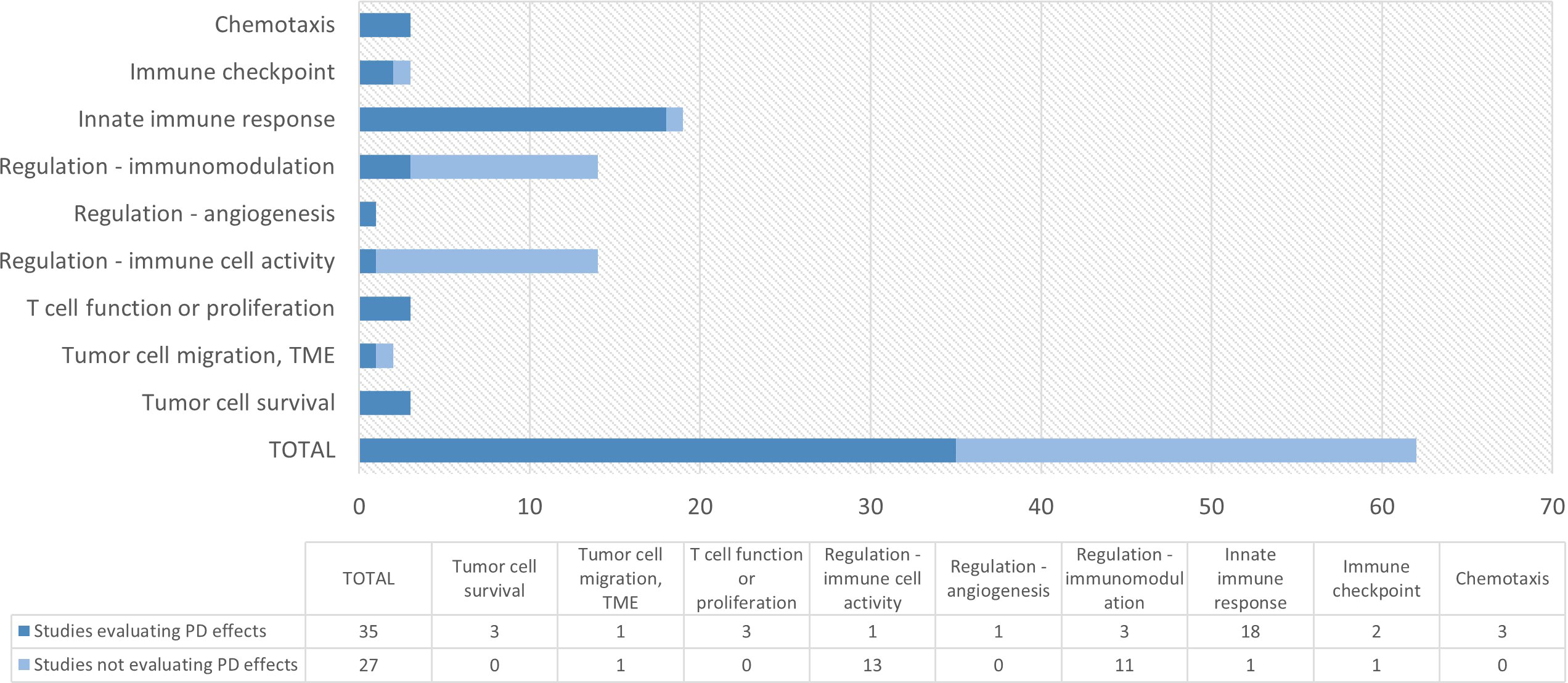
Figure 2 Overview of number of studies with at least one pharmacodynamic (PD) endpoint per target group. Targets included in each category are 1) chemotaxis: CXC4 antagonists; 2) immune checkpoint: A2a and A2a/A2b antagonists; 3) innate immune response: CXCR2 antagonist, dectin receptor agonist, TLR3/4/7/8/9 agonists, IFN; 4) Regulation – immunomodulation: CRBN modulators; 5) Regulation – angiogenesis: IL-3; 6) Regulation – immune cell activity: CCR5 antagonists; 7) T cell function or proliferation: anti-CD38 mAb, IL-1R antagonist, IL-10 agonist; 8) Tumor cell migration: TGF-βR1 Kinase/ALK5 inhibitor, P2X7 antagonist; 9) Tumor cell survival: TYK2/JAK1 inhibitor, JAK1/JAK2 inhibitor; PD, pharmacodynamic; TME: tumor microenvironment.
All three studies with anti-chemotaxis agents (CXCR4 antagonists) (14–16) included PD markers, such as the mobilization of immune cell subsets including CD34+ hematopoietic stem cells, and receptor and surface marker expression (i.e., surface markers of mature immune cell subsets such as T, B and NK cells, T cell subpopulations, monocytes and plasmacytoid dendritic cell progenitors). For the immune checkpoint compounds (adenosine antagonists), positron emission tomography (PET) was used to investigate adenosine A2a receptor occupancy (17). In another study target engagement by a double adenosine A2a and A2b receptor antagonist was determined by ex vivo challenge with a synthetic adenosine agonist (5’-N-ethylcarboxamidoadenosine; NECA) and subsequent evaluation of the levels of the phosphorylated cyclic AMP (cAMP) response element binding protein (CREB) in CD8+ cells (19).
In the category of compounds affecting the tumor microenvironment (TME), one study was identified investigating a P2X7 antagonist. The compound’s peripheral target engagement was demonstrated by an ex vivo immune challenge, evaluating the LPS/BzATP-induced IL-1β release in peripheral blood mononuclear cell (PBMC) cultures (72).
Studies with compounds targeting the tumor cell survival pathways included JAK1/JAK2 and TYK/JAK1 inhibitors. One study measured the levels of phosphorylated STAT3 (pSTAT3) after ex vivo cell stimulation with IL-6 (75), whereas in the other study markers downstream from JAK1 were evaluated (circulating IP-10 and hsCRP levels and neutrophil and lymphocyte count) (73).
Finally, of note was the observable lack of pharmacodynamic endpoints in HV studies which investigated the immunomodulatory drug thalidomide (and analogues) and CCR5 antagonist maraviroc (and analogues), where almost all of the studies only assessed the safety and pharmacokinetics of the compounds.
Discussion
A review of literature on published early phase clinical studies using immuno-oncology compounds in healthy volunteers following PRISMA guidelines and PROSPECT registration was presented in this article. In total, we have found 73 published articles and included 38 different potential immunotherapeutic compounds that have been conducted in HVs.
The majority of the studies investigated immunomodulatory compounds such as interferons, TLR agonists and drugs targeting chemokine receptors. Studies evaluating oncolytic viruses and T-cell based therapies were excluded from our review, since the primary mechanism of action of these compounds is based on an antigen-specific pharmacological activity and not a general immunomodulatory effect. Noteworthy was the lack of studies investigating immune checkpoint inhibitors (other than adenosine antagonists) in HVs, which might be explained by the potential immune-related adverse events of such compounds, typically with a delayed onset and prolonged duration, resulting in an unfavorable benefit/risk ratio for a HV study (98). For comparison, almost all the innate immune system targets mentioned in Table 1 were investigated in HV studies, while at the same time only one immune checkpoint target was identified.
Thalidomide and analogues were investigated in 14 HV studies (Table 2), but only one study included a relevant PD endpoint investigating immunophenotype of circulating immune cells (Table 4) (49). Thalidomide is a drug with troublesome history but remarkable revival decades later as an anti-myeloma drug (99), and it has been discovered that thalidomide and its newer analogues lenalidomide and pomalidomide elicit multiple direct and indirect immune-related anti-myeloma effects, among others by modulating the ubiquitin E3 ligase cereblon (CRBN) (89, 100, 101). Although their indirect immunomodulatory properties in multiple myeloma have been clearly demonstrated (102, 103), previous research might have been more focused on their direct anti-tumor mechanism, requiring the drug effects to be investigated mostly in patients. Similarly, the difference is also significant when looking at CCR5 antagonist maraviroc and its analogues, with 14 HV studies in total and no studies investigating relevant PD, since these compounds are developed and approved as anti-HIV drugs, and their importance for immuno-oncology has only recently been uncovered (104).
Safety perspective
Overall, the adverse event profiles for the compounds evaluated in HVs were acceptable and within the normal range for HV studies, when compared to the available literature. One such published review reported that among 475 phase 1 studies in 27185 HVs, 33% of studies reported at least one severe AE, which is significantly more than what was captured in our review, which was 6 (8%) of the included studies (105). Although we did not directly compare the safety findings in HV studies to the studies with same compounds in patients, safety is expected to be comparable between two populations with regards to drug-related adverse events.
From a safety perspective, drugs targeting proteins that are widely present in healthy tissues inherently carry a higher risk for (auto-immune) toxicity. Safety findings in the identified studies were overall well acceptable, although there were some expected higher-grade toxicities observed in studies with compounds targeting the dectin receptor, CXC4 receptor, JAK1/JAK2 and some specific components of the innate immune pathways. The majority of the severe adverse events of the latter subgroup mainly relate to their inherent ability to boost the (innate) immune response, but also to the immunosuppressive effects of interferon, which can lead to interferon-induced neutropenia (106). Severe neutropenia observed with ruxolitinib has been previously reported (107), which can be explained by the drug’s mechanism of action: its anti-JAK1/JAK2 activity decreases T cell activation and neutrophil activity.
Notably, there were no severe adverse events observed in the immune checkpoint group, where adenosine antagonists were well tolerated up to the highest dose tested, while demonstrating a robust target engagement (19). This points to the possibility of investigating other immune checkpoint modulators in early proof-of-concept clinical trials in HVs. Obviously, a reason to remain cautious is the risk of inducing late-onset immune-related adverse events (irAEs) and autoimmunity in HVs. However, future testing of such compounds in HV trials should not be categorically ruled out, especially when compounds with more controllable immune-mediated mode of actions and favorable immune-related toxicity profiles can be developed.
Pros and cons of healthy volunteer trials
There are numerous advantages of performing early phase clinical trials in HVs before studies in patients are initiated. This is a relatively homogenous population, void of any confounders such as comorbidities or concomitant medications. Patient pre-selection and strict inclusion criteria in early oncology trials may lead to a selection bias, preventing the extrapolation of the results to a general population (108). Practically, recruiting HVs for early phase trials is easier, faster and less expensive, with significantly lower drop-out rates and better compliance which eventually leads to better data quality. Importantly, a HV-based study including PD endpoints can assist in selecting a pharmacologically active dose for the first phase 1B trial, which avoids inefficient dose finding studies in the target population and inclusion of patients in studies with pharmacologically inactive doses (3). Specifically for immunomodulatory compounds, the comparison of immunocompetent HVs with immunosuppressed cancer patients in an integrative study design may be advantageous. Our review shows that testing selected immuno-oncological compounds in early phase clinical trials integrating HVs is feasible from a safety perspective. Furthermore, based on our findings, relevant PD effects were evaluated in 57% of the identified studies, with studies testing compounds targeting the innate immune system being more likely to include at least one PD endpoint. With lack of efficacy as the primary source of failure in later stage clinical research (109), it is of paramount importance to demonstrate pharmacological activity of a new compound early in clinical development in double-blind randomized controlled trials with clear PD endpoints, prior to moving to the more expensive and significantly lengthier patient trials with clinical endpoints (110).
On the other hand, the critical point-of-attention for evaluation of oncology drugs in HVs is the benefit/risk ratio, with is obviously different between cancer patients and HVs. Moreover, for certain compounds evaluation of effects in HVs is not relevant because of low or absent target expression, which is for example the case for tumor-associated antigens. For the presented classes of immunomodulatory compounds this does not represent a problem: these drugs have targets that are expressed in healthy cells or tissues, and consequently there is a possibility to study drug concentration versus effect in HVs. HV trials evaluating JAK1 tyrosine kinase inhibitors (73–75) or an adenosine receptor antagonist (19) included evaluation of cell-based target engagement. Adenosine has been identified as one of the key immunosuppressive molecules reducing effector immune cell activity in TME, which subsequently led to development of inhibitors of the adenosine pathway (78). An example of a successful early phase program in HVs with a compound targeting an immune checkpoint is that of the double adenosine receptor antagonist AB928 (etrumadenant) which is currently undergoing phase 1b/2 trial in cancer patients (ClinicalTrials.gov identifier: NCT04660812) (111), after PK/PD profiling and efficient dose selection in a phase 1 HV study (19). Challenges to investigating immune checkpoint inhibitors in HVs comes from their biological characteristics – they are mostly constructed as IgG monoclonal antibodies (mAbs). This has an impact on the absorption, distribution and metabolism of these compounds, introducing a significant interindividual variability to the PK profiles. Furthermore, target-mediated drug disposition (TMDD) of the mAbs may be one of the main culprits for the complex PK profiles observed with mAbs, considering the availability of the drug molecular target(s) changes with disease state (or absence of disease). These aspects make it particularly challenging to investigate mAb-based checkpoint blockade in HV trials (112, 113).
Since a drug’s effective concentration depends on the clinical context and the desired extent of activity on the specific cellular pathways in a particular condition (114), the PK/PD relationship assessed in HVs does not necessarily translate 1:1 to the targeted patient population. This may represent a significant challenge for immunotherapeutic compounds, such as CXCR2, CXCR4 and CD38 antagonists.
The main function of the chemokine receptor CXCR2 is to regulate the migration and efflux of neutrophils from the bone marrow and it also plays a role in controlling the migration of myeloid derived suppressor cells (MDSCs) to TME in patients. Increased CXCR2 signaling leads to increased levels of neutrophils and MDSCs in TME, which has been associated with abrogated anti-tumor effects of immunotherapy and poorer clinical outcomes. Depletion of neutrophils and MDSCs by CXCR2 antagonists has been shown to increase the numbers and activity of tumor-infiltrating CD8+ T cells, preventing tumor growth and metastasis (115). Of significance for early phase clinical studies could be the ability to investigate the proof-of-concept of CXCR2 engaging compounds to address targeting of CXCR2 already expressed in immune cells of HVs.
In malignancies, the chemokine receptor CXCR4 has been shown to be overexpressed in various tumor cell populations, causing tumor cell migration, angiogenesis, and tumor progression. Blocking this pathway may therefore be an attractive strategy in tumor immunotherapy (77). CXCR4 antagonists work by disrupting the CXCL12/CXCR4 pathway, thereby inducing the mobilization of stem cells to the periphery, making them valuable in the context of harvesting CD34+ cells from both HVs and patients for hematopoietic stem cell transplantation (14).
CD38 is a glycoprotein overexpressed in certain autoimmune conditions (68), and multiple myeloma, where CD38 antagonism by anti-CD38 mAbs can directly deplete CD38+ myeloma cells (94). Nonetheless, anti-CD38 mAbs have been also shown to successfully deplete the MDSCs and regulatory T cells, thereby reverting the tumor-induced immunosuppression and restoring the anti-myeloma effector T cell functions (94). Such indirect cellular immune mechanisms might already be investigated in the context of proof-of-concept HV trials. Thus, in an integrative clinical study design for immunotherapeutic compounds such as CXCR2, CXCR4 and CD38 antagonists, the variability of target expression in HVs compared to cancer patients should be considered when investigating the PK/PD relationship in HVs for translation into the patient setting.
As outlined in a recent review, there are several additional obstacles that should be taken into account when designing early phase oncology trials in HVs, ranging from more stringent requirements for the pre-clinical pharmacology experiments to alternative study designs, to starting dose selection (below the pharmacologically active dose in HV studies, different than for patients), and maximum exposure (with the difficulty to justify dose escalation above the no observed adverse effects level, NOAEL, in HVs) (116). Obviously, the challenge for future early phase clinical design in oncology will be to further integrate HVs using more sophisticated methodology to measure PD endpoints, and to combine HVs and patients in an integrative clinical trial design.
Limitations of the study
The findings of this systematic review must be observed in light of some additional considerations. The interpretation of the primary immune-related mechanism of action of a compound is potentially ambiguous. The exclusion of several compounds (listed in Figure 1) deserves a separate justification. Although direct tumor-targeting drugs such as trastuzumab, sunitinib and lapatinib were intentionally not included in this review, we are aware that evidence exists that the activity of these and similar compounds may be partly attributed to the activation of the innate and adaptive immune responses, mainly by induction of CD8+ T-cell responses or inhibition of immunosuppressive Treg cells (117, 118). However, they are typically not considered direct immunotherapeutic compounds. Furthermore, calcineurin inhibitor cyclosporine A and protein kinase C inhibitor sotrastaurin, together with vaccines against hepatitis B and human papillomavirus (viruses known to cause malignancies) were not included in immunological targets presented in Table 1, even though strictly fulfilling our definition of immunotherapeutic agents (119–122). The first two were not included in the original search due to not (yet) being recognized as relevant targets in in immuno-oncology, meaning the possible use in immuno-oncology was not confirmed by literature, although that might change in the future. Although several HV studies with compounds targeting tumor-associated antigens (TAAs) were identified, we decided to omit those studies, since expression of TAAs in HVs is either absent or low, making the relevance of PD endpoints less obvious in HVs. More specifically, FLT3 tyrosine-kinase inhibitors aimed against acute myeloid leukemia (AML) cells and BCR-ABL-derived peptide vaccine aimed against chronic myeloid leukemia (CML) cells were investigated in HVs (123–126). Importantly, the assessment whether a target could be relevant for oncology was also based on the review by Tang et al. (1, 2) and the Landscape of Immuno-Oncology Drug Development tool (12). Obviously, the clinical relevance as oncological targets remains to be proven for many of them and insights are quickly changing. We did not aim to give a complete overview, but rather an indication of the current state of immuno-oncology drug development studies that integrate HVs in early phase clinical trial protocols.
Conclusion
In conclusion, the findings of our systematic review show the potential value of HV studies for investigational oncology compounds with an immunomodulatory mechanism of action. For all identified drug classes, the observed safety profiles in HV were favorable, and for many compounds the drug concentration versus activity relationship could be evaluated based on incorporated PD endpoints. As such, the obtained insights can guide selection of a safe and pharmacologically active dose for the phase 1B/2A trial in patients. Based on a thorough benefit/risk assessment, the integration of HVs in early phase drug development programs for immuno-oncological compounds can be considered on a case-by-case basis and may have significant advantages for the later clinical development program.
Data availability statement
The original contributions presented in the study are included in the article/supplementary material. Further inquiries can be directed to the corresponding author.
Author contributions
IR, EB, and NK conceived the idea, designed the study protocol and devised the search strategy. IR conducted the screening and full-review of the articles based on systematic search strategy, and extracted data per protocol. EB checked the included articles and extracted data for final decisions. IR wrote the first draft, managed the review process and finalized the manuscript based on co-authors’ feedback. MM, RR, and GG contributed to the review and interpretation of the results. JB supervised the process, edited the manuscript and provided final input. All authors discussed the results and provided feedback to the manuscript. All authors contributed to the article and approved the submitted version.
Acknowledgments
The authors would like to thank Jan W. Schoones (Leiden University Medical Centre) for help with preparing the final search strategy.
Conflict of interest
The authors declare that the research was conducted in the absence of any commercial or financial relationships that could be construed as a potential conflict of interest.
Publisher’s note
All claims expressed in this article are solely those of the authors and do not necessarily represent those of their affiliated organizations, or those of the publisher, the editors and the reviewers. Any product that may be evaluated in this article, or claim that may be made by its manufacturer, is not guaranteed or endorsed by the publisher.
References
1. Tang J, Shalabi A, Hubbard-Lucey VM. Comprehensive analysis of the clinical immuno-oncology landscape. Ann Oncol (2018) 29:84–91. doi: 10.1093/annonc/mdx755
2. Tang J, Pearce L, O’Donnell-Tormey J, Hubbard-Lucey VM. Trends in the global immuno-oncology landscape. Nat Rev Drug Discovery (2018) 17:783–4. doi: 10.1038/nrd.2018.167
3. Ahmed MA, Patel C, Drezner N, Helms W, Tan W, Stypinski D. Pivotal considerations for optimal deployment of healthy volunteers in oncology drug development. Clin Transl Sci (2020) 13:31–40. doi: 10.1111/cts.12703
4. Wong KM, Capasso A, Eckhardt SG. The changing landscape of phase I trials in oncology. Nat Rev Clin Oncol (2016) 13:106–17. doi: 10.1038/nrclinonc.2015.194
5. Upadhaya S, Hubbard-Lucey VM, Yu JX. Immuno-oncology drug development forges on despite COVID-19. Nat Rev Drug Discovery (2020) 19:751–2. doi: 10.1038/d41573-020-00166-1
6. Saber H, Gudi R, Manning M, Wearne E, Leighton JK. An FDA oncology analysis of immune activating products and first-in-human dose selection. Regul Toxicol Pharmacol (2016) 81:448–56. doi: 10.1016/j.yrtph.2016.10.002
7. Peskov K, Azarov I, Chu L, Voronova V, Kosinsky Y, Helmlinger G. Quantitative mechanistic modeling in support of pharmacological therapeutics development in immuno-oncology. Front Immunol (2019) 10:924. doi: 10.3389/fimmu.2019.00924
8. Emens LA, Butterfield LH, Hodi FS, Marincola FM, Kaufman HL. Cancer immunotherapy trials: Leading a paradigm shift in drug development. J Immunother Cancer (2016) 4:1–8. doi: 10.1186/s40425-016-0146-9
9. Karakunnel JJ, Bui N, Palaniappan L, Schmidt KT, Mahaffey KW, Morrison B, et al. Reviewing the role of healthy volunteer studies in drug development. J Transl Med (2018) 16:1–15. doi: 10.1186/s12967-018-1710-5
10. Gupta P, Gupta V, Gupta YK. Phase i clinical trials of anticancer drugs in healthy volunteers: Need for critical consideration. Indian J Pharmacol (2012) 44:540–2. doi: 10.4103/0253-7613.99350
11. Iwamoto M, Iannone R, Wagner JA. Use of healthy volunteers drives clinical oncology drug development decision making. Clin Pharmacol Ther (2012) 92:571–4. doi: 10.1038/clpt.2012.157
12. Cancer research institute. landscape of immuno-oncology drug development - cancer research institute (CRI). Cancer Res Inst (2020).
13. Radanovic I, Klarenbeek N, Moerland M, Rissmann R, Groeneveld GJ, van Brummelen E. The role of healthy volunteers in early phase cancer immunotherapy clinical trials: a systematic review. PROSPERO: Int prospective register systematic Rev (2020), CRD42020210861.
14. Abraham M, Pereg Y, Bulvik B, Klein S, Mishalian I, Wald H, et al. Single dose of the cxcr4 antagonist bl-8040 induces rapid mobilization for the collection of human cd34+ cells in healthy volunteers. Clin Cancer Res (2017) 23:6790–801. doi: 10.1158/1078-0432.CCR-16-2919
15. Karpova D, Bräuninger S, Wiercinska E, Krämer A, Stock B, Graff J, et al. Mobilization of hematopoietic stem cells with the novel CXCR4 antagonist POL6326 (balixafortide) in healthy volunteers-results of a dose escalation trial. J Transl Med (2017) 15:1–12. doi: 10.1186/s12967-016-1107-2
16. Lemery SJ, Hsieh MM, Smith A, Rao S, Khuu HM, Theresa D, et al. A pilot study evaluating the safety and CD34+ cell mobilizing activity of escalating doses of plerixafor in healthy volunteers. Br J Haematol (2011) 153:66–75. doi: 10.1111/j.1365-2141.2010.08547.x
17. Brooks DJ, Papapetropoulos S, Vandenhende F, Tomic D, He P, Coppell A, et al. An open-label, positron emission tomography study to assess adenosine A2A brain receptor occupancy of vipadenant (BIIB014) at steady-state levels in healthy male volunteers. Clin Neuropharmacol (2010) 33:55–60. doi: 10.1097/WNF.0b013e3181d137d2
18. Mukai M, Uchimura T, Zhang X, Greene D, Vergeire M, Cantillon M. Effects of rifampin on the pharmacokinetics of a single dose of istradefylline in healthy subjects. J Clin Pharmacol (2018) 58:193–201. doi: 10.1002/jcph.1003
19. Seitz L, Jin L, Leleti M, Ashok D, Jeffrey J, Rieger A, et al. Safety, tolerability, and pharmacology of AB928, a novel dual adenosine receptor antagonist, in a randomized, phase 1 study in healthy volunteers. Invest New Drugs (2019) 37:711–21. doi: 10.1007/s10637-018-0706-6
20. Holz O, Khalilieh S, Ludwig-Sengpiel A, Watz H, Stryszak P, Soni P, et al. SCH527123, a novel CXCR2 antagonist, inhibits ozone-induced neutrophilia in healthy subjects. Eur Respir J (2010) 35:564–70. doi: 10.1183/09031936.00048509
21. Leaker BR, Barnes PJ, O’Connor B. Inhibition of LPS-induced airway neutrophilic inflammation in healthy volunteers with an oral CXCR2 antagonist. Respir Res (2013) 14:1–9. doi: 10.1186/1465-9921-14-137
22. Bose N, Ottoson NR, Qiu X, Harrison B, Lowe JR, Uhlik MT, et al. Immune pharmacodynamic responses of the novel cancer immunotherapeutic imprime PGG in healthy volunteers. J Immunol (2019) 202:2945–56. doi: 10.4049/jimmunol.1801533
23. Hendrix CW, Margolick JB, Petty BG, Markham RB, Nerhood L, Farzadegan H, et al. Biologic effects after a single dose of poly(I):poly(C12U) in healthy volunteers. Antimicrob Agents Chemother (1993) 37:429–35. doi: 10.1128/AAC.37.3.429
24. García-García I, González-Delgado CA, Valenzuela-Silva CM, Díaz-Machado A, Cruz-Díaz M, Nodarse-Cuní H, et al. Pharmacokinetic and pharmacodynamic comparison of two “pegylated” interferon alpha-2 formulations in healthy male volunteers: A randomized, crossover, double-blind study. BMC Pharmacol (2010) 10:15. doi: 10.1186/1471-2210-10-15
25. Patel TB, Pequignot E, Parker SH, Leavitt MC, Greenberg HE, Kraft WK. Transgenic avian-derived recombinant human interferon-a2b (AVI-005) in healthy subjects: an open-label, single-dose, controlled study. Int J Clin Pharmacol Ther (2007) 45:161–8. doi: 10.5414/CPP45161
26. Rodriguez JL, Valenzuela C, Marin N, Ferrero J, Duconge J, Castillo R, et al. Comparative pharmacokinetics and pharmacodynamics of two recombinant human interferon alpha-2b formulations administered intramuscularly in healthy male volunteers. Biotecnol Apl (2000) 17:166–70.
27. Sarno TC, Greenberg SB, Couch RB, Quarles J, Johnson PE, Hook S, et al. Efficacy and tolerance of intranasally applied recombinant leukocyte a interferon in normal volunteers. J Infect Dis (1983) 148:535–42. doi: 10.1093/infdis/148.3.535
28. Sarno TC, Greenberg SB, Palmer JM, Couch RB, Harmon MW, Johnson PE. Intranasally applied recombinant leukocyte a interferon in normal volunteers. ii. determination of minimal effective and tolerable dose. J Infect Dis (1984) 150:181–8. doi: 10.1093/infdis/150.2.181
29. Shiomi M, Funaki T. Pharmacokinetic/pharmacodynamic model analysis of pegylated interferon α-2a in healthy subjects. Japanese J Clin Pharmacol Ther (2003) 34:177–85. doi: 10.3999/jscpt.34.4_177
30. Zhi J, Teller SB, Satoh H, Koss-Twardy SG, Luke DR. Influence of human serum albumin content in formulations on the bioequivalency of interferon Alfa-2a given by subcutaneous injection in healthy Male volunteers. J Clin Pharmacol (1995) 35:281–4. doi: 10.1002/j.1552-4604.1995.tb04059.x
31. García-García I, Hernández-González I, Díaz-Machado A, González-Delgado CA, Pérez-Rodríguez S, García-Vega Y, et al. Pharmacokinetic and pharmacodynamic characterization of a novel formulation containing co-formulated interferons alpha-2b and gamma in healthy male volunteers. BMC Pharmacol Toxicol (2016) 17:1–11. doi: 10.1186/s40360-016-0103-8
32. Luk A, Jiang Q, Glavini K, Triyatni M, Zhao N, Racek T, et al. A single and multiple ascending dose study of toll-like receptor 7 agonist (RO7020531) in Chinese healthy volunteers. Clin Transl Sci (2020) 13:985–93. doi: 10.1111/cts.12791
33. Hijma HJ, Moss LM, Gal P, Ziagkos D, de Kam ML, Moerland M, et al. Challenging the challenge: A randomized controlled trial evaluating the inflammatory response and pain perception of healthy volunteers after single-dose LPS administration, as a potential model for inflammatory pain in early-phase drug development. Brain Behav Immun (2020) 88:515–28. doi: 10.1016/j.bbi.2020.04.033
34. Hug BA, Matheny CJ, Burns O, Struemper H, Wang X, Washburn ML. Safety, pharmacokinetics, and pharmacodynamics of the TLR4 agonist GSK1795091 in healthy individuals: Results from a randomized, double-blind, placebo-controlled, ascending dose study. Clin Ther (2020) 42:1519–34.e33. doi: 10.1016/j.clinthera.2020.05.022
35. Niemeyer-van der Kolk T, Assil S, Buters TP, Rijsbergen M, Klaassen ES, Feiss G, et al. Omiganan enhances imiquimod-induced inflammatory responses in skin of healthy volunteers. Clin Transl Sci (2020) 13:573–9. doi: 10.1111/cts.12741
36. Pasmatzi E, Chaidaroglou A, Sakkis T, Monastirli A, Georgiou S, Sagriotis A, et al. Topical application of imiquimod induces alternations in peripheral blood lymphocytes in healthy individuals. Acta Derm Venereol (2009) 89:134–9. doi: 10.2340/00015555-0597
37. Vicari AP, Schmalbach T, Lekstrom-Himes J, Morris ML, Al-Adhami MJ, Laframboise C, et al. Safety, pharmacokinetics and immune effects in normal volunteers of CPG 10101 (ACTILON™), an investigational synthetic toll-like receptor 9 agonist. Antivir Ther (2007) 12:741–51.
38. Buraglio M, Trinchard-Lugan I, Munafo A, Macnamee M. Recombinant human interferon-β-1a (Rebif®) vs recombinant interferon-β-1b (Betaseron®) in healthy volunteers. a pharmacodynamic and tolerability study. Clin Drug Investig (1999) 18:27–34. doi: 10.2165/00044011-199918010-00004
39. Chen N, Ye Y, Liu L, Reyes J, Assaf MS, Kasserra C, et al. Lenalidomide at therapeutic and supratherapeutic doses does not prolong QTc intervals in the thorough QTc study conducted in healthy men. Basic Clin Pharmacol Toxicol (2013) 113:179–86. doi: 10.1111/bcpt.12081
40. Chen N, Wen L, Lau H, Surapaneni S, Kumar G. Pharmacokinetics, metabolism and excretion of [14C]-lenalidomide following oral administration in healthy male subjects. Cancer Chemother Pharmacol (2012) 69:789–97. doi: 10.1007/s00280-011-1760-3
41. Chen N, Lau H, Choudhury S, Wang X, Assaf M, Laskin OL. Distribution of lenalidomide into semen of healthy men after multiple oral doses. J Clin Pharmacol (2010) 50:767–74. doi: 10.1177/0091270009355157
42. Chen N, Kasserra C, Reyes J, Liu L, Lau H. Single-dose pharmacokinetics of lenalidomide in healthy volunteers: Dose proportionality, food effect, and racial sensitivity. Cancer Chemother Pharmacol (2012) 70:717–25. doi: 10.1007/s00280-012-1966-z
43. Chen N, Weiss D, Reyes J, Liu L, Kasserra C, Wang X, et al. No clinically significant drug interactions between lenalidomide and p-glycoprotein substrates and inhibitors: Results from controlled phase I studies in healthy volunteers. Cancer Chemother Pharmacol (2014) 73:1031–9. doi: 10.1007/s00280-014-2438-4
44. Kasserra C, Assaf M, Hoffmann M, Li Y, Liu L, Wang X, et al. Pomalidomide: Evaluation of cytochrome P450 and transporter-mediated drug-drug interaction potential in vitro and in healthy subjects. J Clin Pharmacol (2015) 55:168–78. doi: 10.1002/jcph.384
45. Li Y, Wang X, Liu L, Reyes J, Palmisano M, Zhou S. Distribution of pomalidomide into semen of healthy male subjects after multiple doses. Clin Pharmacol Adv Appl (2018) 10:53–62. doi: 10.2147/CPAA.S167017
46. Li Y, Liu L, Wang X, Zhang C, Reyes J, Hoffmann M, et al. In vivo assessment of the effect of CYP1A2 inhibition and induction on pomalidomide pharmacokinetics in healthy subjects. J Clin Pharmacol (2018) 58:1295–304. doi: 10.1002/jcph.1145
47. Li Y, Liu L, Huang L, Wang X, Hoffmann M, Reyes J, et al. Open-label, randomized, crossover study in healthy subjects to evaluate the bioavailability of, and the food effect on, a pomalidomide oral liquid suspension. Clin Pharmacol Adv Appl (2018) 10:89–99. doi: 10.2147/CPAA.S171735
48. Mondal SA, Assaf M, Liu L, O’Mara E. A phase 1, double-blind, 4-period crossover study to investigate the effects of pomalidomide on QT interval in healthy male subjects. Cancer Chemother Pharmacol (2016) 77:251–8. doi: 10.1007/s00280-015-2912-7
49. Neubert R, Nogueira AC, Neubert D. Thalidomide and the immune system 2. changes in receptors on blood cells of a healthy volunteer. Life Sci (1992) 51:2107–16. doi: 10.1016/0024-3205(92)90162-I
50. Teo SK, Scheffler MR, Kook KA, Tracewell WG, Colburn WA, Stirling DI, et al. Effect of a high-fat meal on thalidomide pharmacokinetics and the relative bioavailability of oral formulations in healthy men and women. Biopharm Drug Dispos (2000) 21:33–40. doi: 10.1002/1099-081X(200001)21:1<33::AID-BDD213>3.0.CO;2-R
51. Teo SK, Scheffler MR, Kook KA. Thalidomide dose proportionality assessment following single doses to healthy subjects. J Clin Pharmacol (2001) 41:662–7. doi: 10.1177/00912700122010555
52. Teo SK, Colburn WA, Thomas SD. Single-dose oral pharmacokinetics of three formulations of thalidomide in healthy male volunteers. J Clin Pharmacol (1999) 39:1162–8. doi: 10.1177/009127009903901108
53. Huhn RD, Yurkow EJ, Kuhn JG, Clarke L, Gunn H, Resta D, et al. Pharmacodynamics of daily subcutaneous recombinant human interleukin-3 in normal volunteers. Clin Pharmacol Ther (1995) 57:32–41. doi: 10.1016/0009-9236(95)90263-5
54. Adkison KK, Shachoy-Clark A, Fang L, Lou Y, Otto VR, Berrey MM, et al. The effects of ritonavir and lopinavir/ritonavir on the pharmacokinetics of a novel CCR5 antagonist, aplaviroc, in healthy subjects. Br J Clin Pharmacol (2006) 62:336–44. doi: 10.1111/j.1365-2125.2006.02661.x
55. Abel S, van der Ryst E, Rosario MC, Ridgway CE, Medhurst CG, Taylor-Worth RJ, et al. Assessment of the pharmacokinetics, safety and tolerability of maraviroc, a novel CCR5 antagonist, in healthy volunteers. Br J Clin Pharmacol (2008) 65:5–18. doi: 10.1111/j.1365-2125.2008.03130.x
56. Abel S, Russell D, Whitlock LA, Ridgway CE, Muirhead GJ. Effect of maraviroc on the pharmacokinetics of midazolam, lamivudine/zidovudine, and ethinyloestradiol/levonorgestrel in healthy volunteers. Br J Clin Pharmacol (2008) 65:19–26. doi: 10.1111/j.1365-2125.2008.03132.x
57. Abel S, Russell D, Taylor-Worth RJ, Ridgway CE, Muirhead GJ. Effects of CYP3A4 inhibitors on the pharmacokinetics of maraviroc in healthy volunteers. Br J Clin Pharmacol (2008) 65:27–37. doi: 10.1111/j.1365-2125.2008.03133.x
58. Abel S, Russell D, Whitlock LA, Ridgway CE, Muirhead GJ. The effects of cotrimoxazole or tenofovir co-administration on the pharmacokinetics of maraviroc in healthy volunteers. Br J Clin Pharmacol (2008) 65:47–53. doi: 10.1111/j.1365-2125.2008.03135.x
59. Abel S, Russell D, Whitlock LA, Ridgway CE, Nedderman ANR, Walker DK. Assessment of the absorption, metabolism and absolute bioavailability of maraviroc in healthy male subjects. Br J Clin Pharmacol (2008) 65:60–7. doi: 10.1111/j.1365-2125.2008.03137.x
60. Abel S, Jenkins TM, Whitlock LA, Ridgway CE, Muirhead GJ. Effects of CYP3A4 inducers with and without CYP3A4 inhibitors on the pharmacokinetics of maraviroc in healthy volunteers. Br J Clin Pharmacol (2008) 65:38–46. doi: 10.1111/j.1365-2125.2008.03134.x
61. Davis JD, Hackman F, Layton G, Higgins T, Sudworth D, Weissgerber G. Effect of single doses of maraviroc on the QT/QTc interval in healthy subjects. Br J Clin Pharmacol (2008) 65:68–75. doi: 10.1111/j.1365-2125.2008.03138.x
62. Lu Y, Fuchs EJ, Hendrix CW, Bumpus NN. CYP3A5 genotype impacts maraviroc concentrations in healthy volunteers. Drug Metab Dispos (2014) 42:1796–802. doi: 10.1124/dmd.114.060194
63. Vourvahis M, Langdon G, LaBadie RR, Layton G, Ndongo MN, Banerjee S, et al. Pharmacokinetic effects of coadministration of lersivirine with raltegravir or maraviroc in healthy subjects. Antimicrob Agents Chemother (2012) 56:887–92. doi: 10.1128/AAC.00572-11
64. Vourvahis M, McFadyen L, Nepal S, Valluri SR, Fang A, Fate GD, et al. No clinical impact of CYP3A5 gene polymorphisms on the pharmacokinetics and/or efficacy of maraviroc in healthy volunteers and HIV-1–infected subjects. J Clin Pharmacol (2019) 59:139–52. doi: 10.1002/jcph.1306
65. Vourvahis M, Fang J, Choo HW, Heera J. The effect of maraviroc on the pharmacokinetics of digoxin in healthy volunteers. Clin Pharmacol Drug Dev (2014) 3:202–6. doi: 10.1002/cpdd.91
66. Kasserra C, Li J, March B, O’Mara E. Effect of vicriviroc with or without ritonavir on oral contraceptive pharmacokinetics: a randomized, open-label, parallel-group, fixed-sequence crossover trial in healthy women. Clin Ther (2011) 33:1503–14. doi: 10.1016/j.clinthera.2011.08.012
67. O’Mara E, Kasserra C, Huddlestone JR, Wan Y, Soni P, Caceres M, et al. Effect of vicriviroc on the QT/corrected QT interval and central nervous system in healthy subjects. Antimicrob Agents Chemother (2010) 54:2448–54. doi: 10.1128/AAC.01447-09
68. Fedyk ER, Zhao L, Koch A, Smithson G, Estevam J, Chen G, et al. Safety, tolerability, pharmacokinetics and pharmacodynamics of the anti-CD38 cytolytic antibody TAK-079 in healthy subjects. Br J Clin Pharmacol (2020) 86:1314–25. doi: 10.1111/bcp.14241
69. Hernandez ML, Mills K, Almond M, Todoric K, Aleman MM, Zhang H, et al. IL-1 receptor antagonist reduces endotoxin-induced airway inflammation in healthy volunteers. J Allergy Clin Immunol (2015) 135:379–85. doi: 10.1016/j.jaci.2014.07.039
70. Huhn RD, Radwanski E, Gallo J, Affrime MB, Sabo R, Gonyo G, et al. Pharmacodynamics of subcutaneous recombinant human interleukin-10 in healthy volunteers. Clin Pharmacol Ther (1997) 62:171–80. doi: 10.1016/S0009-9236(97)90065-5
71. Cassidy KC, Gueorguieva I, Miles C, Rehmel J, Yi P, Ehlhardt WJ. Disposition and metabolism of [14 c]-galunisertib, a TGF- β RI kinase/ALK5 inhibitor, following oral administration in healthy subjects and mechanistic prediction of the effect of itraconazole on galunisertib pharmacokinetics. Xenobiotica (2018) 48:382–99. doi: 10.1080/00498254.2017.1323137
72. Recourt K, van der Aart J, Jacobs G, de Kam M, Drevets W, van Nueten L, et al. Characterisation of the pharmacodynamic effects of the P2X7 receptor antagonist JNJ-54175446 using an oral dexamphetamine challenge model in healthy males in a randomised, double-blind, placebo-controlled, multiple ascending dose trial. J Psychopharmacol (2020) 34:1030–42. doi: 10.1177/0269881120914206
73. Banfield C, Scaramozza M, Zhang W, Kieras E, Page KM, Fensome A, et al. The safety, tolerability, pharmacokinetics, and pharmacodynamics of a tyk2/jak1 inhibitor (pf-06700841) in healthy subjects and patients with plaque psoriasis. J Clin Pharmacol (2018) 58:434–47. doi: 10.1002/jcph.1046
74. Shi JG, Chen X, Emm T, Scherle PA, McGee RF, Lo Y, et al. The effect of CYP3A4 inhibition or induction on the pharmacokinetics and pharmacodynamics of orally administered ruxolitinib (INCB018424 phosphate) in healthy volunteers. J Clin Pharmacol (2012) 52:809–18. doi: 10.1177/0091270011405663
75. Shi JG, Chen X, McGee RF, Landman RR, Emm T, Lo Y, et al. The pharmacokinetics, pharmacodynamics, and safety of orally dosed INCB018424 phosphate in healthy volunteers. J Clin Pharmacol (2011) 51:1644–54. doi: 10.1177/0091270010389469
76. Kumar A, Bunnell E, Lynn M, Anel R, Habet K, Neumann A, et al. Experimental human endotoxemia is associated with depression of load-independent contractility indices: prevention by the lipid a analogue e5531. Chest (2004) 126:860–7. doi: 10.1378/CHEST.126.3.860
77. Zhou W, Guo S, Liu M, Burow ME, Wang G. Targeting CXCL12/CXCR4 axis in tumor immunotherapy. Curr Med Chem (2017) 26:3026–41. doi: 10.2174/0929867324666170830111531
78. Leone RD, Emens LA. Targeting adenosine for cancer immunotherapy. J Immunother Cancer (2018) 6:1–9. doi: 10.1186/s40425-018-0360-8
79. Steele CW, Karim SA, Leach JDG, Bailey P, Upstill-Goddard R, Rishi L, et al. CXCR2 inhibition profoundly suppresses metastases and augments immunotherapy in pancreatic ductal adenocarcinoma. Cancer Cell (2016) 29:832–45. doi: 10.1016/j.ccell.2016.04.014
80. Zhao Y, Chu X, Chen J, Wang Y, Gao S, Jiang Y, et al. Dectin-1-activated dendritic cells trigger potent antitumour immunity through the induction of Th9 cells. Nat Commun (2016) 7:1–12. doi: 10.1038/ncomms12368
81. Belardelli F, Ferrantini M, Proietti E, Kirkwood JM. Interferon-alpha in tumor immunity and immunotherapy. Cytokine Growth Factor Rev (2002) 13:119–34. doi: 10.1016/S1359-6101(01)00022-3
82. Qu J, Hou Z, Han Q, Jiang W, Zhang C, Tian Z, et al. Intracellular poly(I:C) initiated gastric adenocarcinoma cell apoptosis and subsequently ameliorated NK cell functions. J Interf Cytokine Res (2014) 34:52–9. doi: 10.1089/jir.2012.0118
83. Ni L, Lu J. Interferon gamma in cancer immunotherapy. Cancer Med (2018) 7:4509–16. doi: 10.1002/cam4.1700
84. Shetab Boushehri MA, Lamprecht A. TLR4-based immunotherapeutics in cancer: A review of the achievements and shortcomings. Mol Pharm (2018) 15:4777–800. doi: 10.1021/acs.molpharmaceut.8b00691
85. Urban-Wojciuk Z, Khan MM, Oyler BL, Fåhraeus R, Marek-Trzonkowska N, Nita-Lazar A, et al. The role of TLRs in anti-cancer immunity and tumor rejection. Front Immunol (2019) 10:2388. doi: 10.3389/fimmu.2019.02388
86. Appleman JR, Webber SE. Abstract 582: Selection of a novel toll-like receptor 7 (TLR7) agonist PRX034 for immunotherapy of cancer. Cancer Res Am Assoc Cancer Res (AACR) (2020) 80:5822. doi: 10.1158/1538-7445.am2020-582
87. Mullins SR, Vasilakos JP, Deschler K, Grigsby I, Gillis P, John J, et al. Intratumoral immunotherapy with TLR7/8 agonist MEDI9197 modulates the tumor microenvironment leading to enhanced activity when combined with other immunotherapies. J Immunother Cancer (2019) 7:244. doi: 10.1186/s40425-019-0724-8
88. Chuang YC, Tseng JC, Huang LR, Huang CM, Huang CYF, Chuang TH. Adjuvant effect of toll-like receptor 9 activation on cancer immunotherapy using checkpoint blockade. Front Immunol (2020) 11:1075. doi: 10.3389/fimmu.2020.01075
89. Zhu YX, Kortuem KM, Stewart AK. Molecular mechanism of action of immune-modulatory drugs thalidomide, lenalidomide and pomalidomide in multiple myeloma. Leuk Lymphoma (2013) 54:683–7. doi: 10.3109/10428194.2012.728597
90. Fink EC, Ebert BL. The novel mechanism of lenalidomide activity. Blood (2015) 126:2366–9. doi: 10.1182/blood-2015-07-567958
91. Dentelli P, Rosso A, Olgasi C, Camussi G, Brizzi MF. IL-3 is a novel target to interfere with tumor vasculature. Oncogene (2011) 30:4930–40. doi: 10.1038/onc.2011.204
92. Litmanovich A, Khazim K, Cohen I. The role of interleukin-1 in the pathogenesis of cancer and its potential as a therapeutic target in clinical practice. Oncol Ther (2018) 6:109–27. doi: 10.1007/s40487-018-0089-z
93. Zhang H, Wang Y, Hwang ES, He Y-W. Interleukin-10: An immune-activating cytokine in cancer immunotherapy. J Clin Oncol (2016) 34:3576–8. doi: 10.1200/JCO.2016.69.6435
94. Morandi F, Horenstein AL, Costa F, Giuliani N, Pistoia V, Malavasi F. CD38: A target for immunotherapeutic approaches in multiple myeloma. Front Immunol (2018) 9:2722. doi: 10.3389/fimmu.2018.02722
95. Lara R, Adinolfi E, Harwood CA, Philpott M, Barden JA, Di Virgilio F, et al. P2X7 in cancer: From molecular mechanisms to therapeutics. Front Pharmacol (2020) 11:793. doi: 10.3389/fphar.2020.00793
96. Lu C, Talukder A, Savage NM, Singh N, Liu K. JAK-STAT-mediated chronic inflammation impairs cytotoxic T lymphocyte activation to decrease anti-PD-1 immunotherapy efficacy in pancreatic cancer. Oncoimmunology (2017) 6:e1291106. doi: 10.1080/2162402X.2017.1291106
97. Wöss K, Simonović N, Strobl B, Macho-Maschler S, Müller M. Tyk2: An upstream kinase of stats in cancer. Cancers (Basel) (2019) 11:1–18. doi: 10.3390/cancers11111728
98. Ramos-Casals M, Brahmer JR, Callahan MK, Flores-Chávez A, Keegan N, Khamashta MA, et al. Immune-related adverse events of checkpoint inhibitors. Nat Rev Dis Prim (2020) 6:1–21. doi: 10.1038/s41572-020-0160-6
99. Rehman W, Arfons LM, Lazarus HM. The rise, fall and subsequent triumph of thalidomide: Lessons learned in drug development. Ther Adv Hematol (2011) 2:291–308. doi: 10.1177/2040620711413165
100. Fink EC, Ebert BL. The novel mechanism of lenalidomide activity. Blood (2015) 126:2366–9. doi: 10.1182/blood-2015-07-567958
101. Lopez-Girona A, Mendy D, Ito T, Miller K, Gandhi AK, Kang J, et al. Cereblon is a direct protein target for immunomodulatory and antiproliferative activities of lenalidomide and pomalidomide. Leukemia (2012) 26:2326–35. doi: 10.1038/leu.2012.119
102. Krämer I, Engelhardt M, Fichtner S, Neuber B, Medenhoff S, Bertsch U, et al. Lenalidomide enhances myeloma-specific T-cell responses in vivo and in vitro. Oncoimmunology (2016) 5:e1139662. doi: 10.1080/2162402X.2016.1139662
103. Busch A, Zeh D, Janzen V, Mügge L-O, Wolf D, Fingerhut L, et al. Treatment with lenalidomide induces immunoactivating and counter-regulatory immunosuppressive changes in myeloma patients. Clin Exp Immunol (2014) 177:439–53. doi: 10.1111/cei.12343
104. Hemmatazad H, Berger MD. CCR5 is a potential therapeutic target for cancer. Expert Opin Ther Targets (2021) 25:311–27. doi: 10.1080/14728222.2021.1902505
105. Johnson RA, Rid A, Emanuel E, Wendler D. Risks of phase I research with healthy participants: A systematic review. Clin Trials (2016) 13:149–60. doi: 10.1177/1740774515602868
106. Kowdley KV. Hematologic side effects of interferon and ribavirin therapy. J Clin Gastroenterol (2005) 39:S3–S8. doi: 10.1097/01.MCG.0000145494.76305.11
107. Manduzio P. Ruxolitinib in myelofibrosis: to be or not to be an immune disruptor. Ther Clin Risk Manag (2017) 13:169. doi: 10.2147/TCRM.S121683
108. Kosov M, Riefler J, Belotserkovskiy M. Patient selection bias in oncology clinical trials. J Clin Stud (2016) 8:26–8.
109. Hwang TJ, Carpenter D, Lauffenburger JC, Wang B, Franklin JM, Kesselheim AS. Failure of investigational drugs in late-stage clinical development and publication of trial results. JAMA Intern Med (2016) 176:1826–33. doi: 10.1001/jamainternmed.2016.6008
110. Kummar S, Kinders R, Rubinstein L, Parchment RE, Murgo AJ, Collins J, et al. Compressing drug development timelines in oncology using phase “0” trials. Nat Rev Cancer (2007) 7:131–9. doi: 10.1038/nrc2066
111. ClinicalTrials.gov [Internet]. NCT04660812L: An open label study evaluating the efficacy and safety of etrumadenant (AB928) based treatment combinations in participants with metastatic colorectal cancer. Bethesda (MD: National Library of Medicine (US (2020).
112. Centanni M, Moes DJAR, Trocóniz IF, Ciccolini J, van Hasselt JGC. Clinical pharmacokinetics and pharmacodynamics of immune checkpoint inhibitors. Clin Pharmacokinet (2019) 58:835–57. doi: 10.1007/s40262-019-00748-2
113. Postel-Vinay S, Aspeslagh S, Lanoy E, Robert C, Soria JC, Marabelle A. Challenges of phase 1 clinical trials evaluating immune checkpoint-targeted antibodies. Ann Oncol (2016) 27:214–24. doi: 10.1093/annonc/mdv550
114. Radanovic I, Likic R, Groeneveld GJ. Spotlight commentary: Importance of dose redefining in the process of drug repurposing. Br J Clin Pharmacol (2021) 87:1705–7. doi: 10.1111/bcp.14562
115. Cheng Y, Ma X, Wei Y, Wei X-W. Potential roles and targeted therapy of the CXCLs/CXCR2 axis in cancer and inflammatory diseases. Biochim Biophys Acta - Rev Cancer (2019) 1871:289–312. doi: 10.1016/j.bbcan.2019.01.005
116. de las Heras B, Bouyoucef-Cherchalli D, Reeve L, Reichl A, Mandarino D, Flach S, et al. Healthy volunteers in first-in-human oncology drug development for small molecules. Br J Clin Pharmacol (2021) 88:1773–84. doi: 10.1111/bcp.15092
117. Hannesdóttir L, Tymoszuk P, Parajuli N, Wasmer MH, Philipp S, Daschil N, et al. Lapatinib and doxorubicin enhance the Stat1-dependent antitumor immune response. Eur J Immunol (2013) 43:2718–29. doi: 10.1002/eji.201242505
118. Costa RLB, Czerniecki BJ. Clinical development of immunotherapies for HER2+ breast cancer: a review of HER2-directed monoclonal antibodies and beyond. NPJ Breast Cancer (2020) 6:10. doi: 10.1038/s41523-020-0153-3
119. Lai L, Ault K, Rouphael N, Beck A, Domjahn B, Xu Y, et al. Duration of cellular and humoral responses after quadrivalent human papillomavirus vaccination in healthy female adults with or without prior type 16 and/or 18 exposure. Vaccines (2020) 8:348. doi: 10.3390/vaccines8030348
120. Lin C, Zhu J, Zheng Y, Chen Y, Wu Z, Chong Y, et al. Effect of GM-CSF in combination with hepatitis b vaccine on revacination of healthy adult non-responders. J Infect (2010) 60:264–70. doi: 10.1016/j.jinf.2010.01.011
121. Mohseni AH, Sedigheh Taghinezhad S, Keyvani H. The first clinical use of a recombinant lactococcus lactis expressing human papillomavirus type 16 e7 oncogene oral vaccine: a phase i safety and immunogenicity trial in healthy women volunteers. Mol Cancer Ther (2020) 19:717–27. doi: 10.1158/1535-7163.MCT-19-0375
122. Yokomine M, Matsueda S, Kawano K, Sasada T, Fukui A, Yamashita T, et al. Enhancement of humoral and cell mediated immune response to HPV16 L1-derived peptides subsequent to vaccination with prophylactic bivalent HPV L1 virus-like particle vaccine in healthy females. Exp Ther Med (2017) 13:1500–5. doi: 10.3892/etm.2017.4150
123. Sanga M, James J, Marini J, Gammon G, Hale C, Li J. An open-label, single-dose, phase 1 study of the absorption, metabolism and excretion of quizartinib, a highly selective and potent FLT3 tyrosine kinase inhibitor, in healthy male subjects, for the treatment of acute myeloid leukemia. Xenobiotica (2017) 47:856–69. doi: 10.1080/00498254.2016.1217100
124. Del Corral A, Dutreix C, Huntsman-Labed A, Lorenzo S, Morganroth J, Harrell R, et al. Midostaurin does not prolong cardiac repolarization defined in a thorough electrocardiogram trial in healthy volunteers. Cancer Chemother Pharmacol (2012) 69:1255–63. doi: 10.1007/s00280-012-1825-y
125. Rojas JM, Knight K, Watmough S, Bell J, Wang L, Callaghan T, et al. BCR-ABL peptide vaccination in healthy subjects: Immunological responses are equivalent to those in chronic myeloid leukaemia patients. Leuk Res (2011) 35:369–72. doi: 10.1016/j.leukres.2010.05.028
Keywords: phase I, oncology, immunotherapy, healthy volunteers, pharmacology, clinical trials
Citation: Radanovic I, Klarenbeek N, Rissmann R, Groeneveld GJ, van Brummelen EMJ, Moerland M and Bosch JJ (2022) Integration of healthy volunteers in early phase clinical trials with immuno-oncological compounds. Front. Oncol. 12:954806. doi: 10.3389/fonc.2022.954806
Received: 27 May 2022; Accepted: 09 August 2022;
Published: 29 August 2022.
Edited by:
Yuanliang Yan, Xiangya Hospital, Central South University, ChinaReviewed by:
Meng Shen, Tianjin Medical University Cancer Institute and Hospital, ChinaCarolina Ines Domaica, Consejo Nacional de Investigaciones Científicas y Técnicas (CONICET), Argentina
Copyright © 2022 Radanovic, Klarenbeek, Rissmann, Groeneveld, van Brummelen, Moerland and Bosch. This is an open-access article distributed under the terms of the Creative Commons Attribution License (CC BY). The use, distribution or reproduction in other forums is permitted, provided the original author(s) and the copyright owner(s) are credited and that the original publication in this journal is cited, in accordance with accepted academic practice. No use, distribution or reproduction is permitted which does not comply with these terms.
*Correspondence: Jacobus J. Bosch, amJvc2NoQGNoZHIubmw=