- 1Department of Pharmacy, Affiliated Cancer Hospital of Zhengzhou University & Henan Cancer Hospital, Zhengzhou University, Zhengzhou, China
- 2Department of Pharmacy, First Affiliated Hospital of Zhengzhou University, Zhengzhou, China
- 3Affiliated Cancer Hospital of Zhengzhou University & Henan Cancer Hospital, Artificial Intelligence and IoT Smart Medical Engineering Research Center of Henan Province, Zhengzhou, China
- 4Children’s Hospital Affiliated to Zhengzhou University, Henan Children’s Hospital, Zhengzhou Children’s Hospital, Zhengzhou, China
- 5Key Laboratory of Advanced Drug Preparation Technologies, Ministry of Education, School of Pharmaceutical Sciences, Zhengzhou University, State Key Laboratory of Esophageal Cancer Prevention and Treatment, Zhengzhou University, Zhengzhou, China
Despite improved methods of diagnosis and the development of different treatments, mortality from lung cancer remains surprisingly high. Non-small cell lung cancer (NSCLC) accounts for the large majority of lung cancer cases. Therefore, it is important to review current methods of diagnosis and treatments of NSCLC in the clinic and preclinic. In this review, we describe, as a guide for clinicians, current diagnostic methods and therapies (such as chemotherapy, chemoradiotherapy, targeted therapy, antiangiogenic therapy, immunotherapy, and combination therapy) for NSCLC.
1 Introduction
Lung cancer, as a common malignant cancer, presents a serious threat to human life. Lung cancers can be divided into NSCLC and small cell lung cancer (SCLC), based on differences in histology and origin (1). NSCLC predominates, accounting for almost 85%, of lung cancer cases. NSCLC is further subdivided into two main subtypes: lung adenocarcinoma (LUAD) and lung squamous cell carcinoma (LUSC). The two types have different gene expression profiles, especially of NECTIN1, a cadherin biomarker (2). In addition, LUSC proliferates faster than LUAD (3).
The causes of lung cancer are diverse, but smoking is considered to be the primary reason. In some lung cancer patients with no smoking history, the disease can be attributed to exposure to radon (222Rn), usually from building materials (4). The incidence of lung cancer is also related to genetics and demographic characteristics (5). The link with demographic characteristics may be attributable to differences in health care systems in different countries. For example, differences in the physical examination of patients may affect the stage at which lung cancer is diagnosed (the development of NSCLC can be divided into four stages: I, II, III, and IV) (6). The main reason for the high mortality rate among lung cancer patients is that only 15% of patients are diagnosed at an early stage (7), and in most patients (70%) the disease is not diagnosed until it is at an advanced stage, perhaps because symptoms are relatively slight in the early stages, and patients may ignore them.
It appears that NSCLC does not metastasize in the early stages and, therefore, surgery could extend the life of patients provided the disease is diagnosed at this stage (8). However, surgery will not benefit those patients, the majority, in whom the disease is diagnosed at an advanced stage. Therefore, the low rate of diagnosis of NSCLC in the early stages remains a problem.
The use of positron emission tomography (PET) could increase the proportion of patients in whom lung cancer is diagnosed in the early stages and thereby reduce lung cancer mortality. The problem is how to increase the number of patients who undergo PET. Common symptoms of lung cancer, such as coughing, chest pain, and wheezing, are often ignored by patients, and hemoptysis, although more likely to be worrying to patients, is experienced by only 20% of lung cancer patients (9). As a result, many patients miss out on the opportunity for early diagnosis and effective treatment.
Treatments for lung cancer include chemotherapy, chemoradiotherapy, targeted therapy, antiangiogenic therapy, immunotherapy, and combination therapy. Treatment of stage II–IV disease also involves adjuvant therapy and neoadjuvant therapy, in addition to the therapies mentioned above. In some cases, these therapies can be used to confirm the success or otherwise of surgery or combined with surgery to give better results. Besides, surgery is the main treatment for stage I disease. In this review, we describe the biological features of lung cancer, diagnostic methods, and drugs or other compounds currently used in chemotherapy, chemoradiotherapy, targeted therapy, antiangiogenic therapy, immunotherapy, and combination therapy (Figure 1). We hope that this review will act as guidance for the clinical treatment of lung cancer.
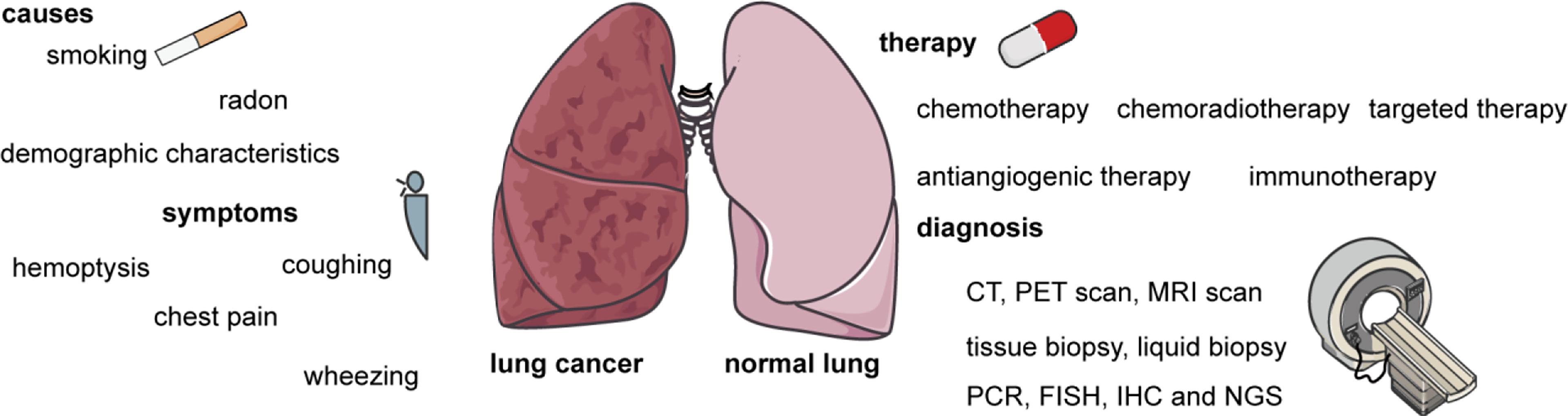
Figure 1 The main causes and symptoms of lung cancer, as well as methods of diagnosis and therapies. The causes of lung cancer include smoking, radon, genetics, and demographic characteristics. The symptoms of lung cancer including hemoptysis, coughing, chest pain, and wheezing. Therapies include chemotherapy, chemoradiotherapy, targeted therapy, antiangiogenic therapy, immunotherapy, and combination therapy. Diagnostic methods include computed tomography (CT), positron emission tomography (PET), magnetic resonance imaging (MRI), tissue biopsy, liquid biopsy, polymerase chain reaction (PCR), fluorescence in situ hybridization (FISH), immunohistochemistry (IHC), and next-generation sequencing (NGS).
2 The biological features of lung cancer
Lung cancer is a heterogeneous cancer, which means that the tumor contains different subpopulations of cells. Heterogeneity is correlated with chemoresistance and the probability of metastasis (10). Diagnostic methods, therapeutic methods, and the identification of novel biomarkers would also benefit from the further study of lung cancer biology. It is therefore important to summarize the biological features of lung cancer.
2.1 Oncogene mutations in NSCLC patients
Oncogene mutations are found in most NSCLC patients and, therefore, targeted drugs are associated with fewer side effects, higher response rates (RRs), and longer progression-free survival (PFS) than cytotoxic drugs. A mutation in the gene coding for epidermal growth factor receptor (EGFR) is common in NSCLC patients (found in 10%–30% of patients), and downstream signaling pathways such as MAPK/ERK, PI3K/AKT and Bax/Bcl-2 are also potential targets (11). Almost 90% of EGFR mutations in NSCLC patients are exon 19 deletions or L858R substitutions in exon 21. In addition, mutation of the T790M gene occurs in 50%–60% of NSCLC patients with the EGFR mutation, and this mutation is associated with acquired resistance (12). Acquired resistance to the EGFR tyrosine kinase inhibitor (TKI) in NSCLC patients is correlated with overexpression of osteopontin (OPN), upregulation of integrin αVβ3, and activation of downstream signaling pathways such as FAK/AKT and ERK (13). Activation of the PI3K/AKT/mTOR signaling pathway is also associated with acquired resistance to EGFR TKIs in NSCLC patients (14). The PI3K/AKT/mTOR signaling pathway is linked to the proliferation and invasion of cancer cells, affecting the likelihood of success of chemotherapy.
Rearranged during transfection (RET) rearrangements are found in 1%–2% of NSCLC patients, and the downstream signaling pathways of RET, such as PI3K/AKT, JAK-STAT, and RAS/MAPK, are associated with cell proliferation, invasion, and migration (15, 16). MET mutations could result in the abnormal expression of MET axis, and the MET/HGF (hepatocyte growth factor) signal pathway play an important role in the MET axis, and this signal pathway leads to tumor cell migration, invasion, and metastasis (17) and are associated with resistance to treatment with EGFR and vascular endothelial growth factor receptor (VEGFR) inhibitor. Mutations in exon 14 are the most common MET mutations found in NSCLC patients (18). The majority of MET exon 14 mutations are point mutations, but indels, insertions, and deletions are also found (19).
Rearrangement of the anaplastic lymphoma kinase gene (ALK) has been identified in 5%–6% of younger NSCLC patients (20). Overexpression of ALK in A549 cells can induce epithelial–mesenchymal transition (EMT), and increase migration and invasion, phenomena that are correlated with the upregulation of signal transducer and activator of transcriptions 3 (STAT3) (21). Many NSCLC patients with an ALK mutation develop drug resistance after taking drugs for a few years. In the case of ALK inhibitors, the most common mutation associated with acquired resistance is F1174L (22). In addition, some studies have confirmed that drug resistance in NSCLC is associated with signal transducer and activator of transcriptions (STATs), especially the STAT3/ZEB1 signaling pathway (23). These findings are a reminder that combination therapies targeting both ALK and STAT3 could perhaps overcome the resistance associated with the use of ALK inhibitors.
Mutations in the gene encoding human epidermal growth factor receptor 2 (HER2) is the mutation of exon 20, and these mutations are found in 2%–4% of NSCLC patients, especially women, besides, the patients with HER2 mutations easily appear brain metastases (24). Activation of HER2 induces the phosphorylation of tyrosine residues, leading to the activation of downstream signaling pathways such as MEK/ERK and PI3K/AKT, which in turn increases the migration and proliferation of lung cancer cells (25). Around 4% of NSCLC patients have a mutation in the B-Raf proto-oncogene (BRAF), but the V600E mutation is present in only half of such patients, who as a result are resistant to BRAF inhibitors (the V600E mutation is associated with a better response to BRAF-targeted therapy) (26). BRAFV600E mutation is usually accompanied by MAPK signaling pathway activation, and, therefore, combination therapy with two different drugs, one targeting BRAF and the other targeting MEK (27), may give better results.
c-Ros oncogene 1 (ROS1) rearrangement is found in around 1%–2% of NSCLC patients (28). There are several different ROS1 rearrangements, including CD74-ROS1, SLC34A2-ROS1, YWHAE-ROS1, TFG-ROS1, and CEP85L-ROS1, but CD74-ROS1 (44%) is the most common ROS1 rearrangement found in NSCLC patients (29). ROS1 is a kind of tyrosine kinase; its ligand is neural epidermal growth factor-like 2 neural EGFL-like 2 (30). Just as the other oncogene we mentioned above, such as HER2, BRAF, when ROS1 is activated by its ligands, downstream signaling pathways such as the PI3K/AKT/mTOR, JAK/STAT, and MAPK/ERK signaling pathways are also activated, leading to the proliferation of lung cancer cells and tumor invasion (31).
Among NSCLC patients tested, 13% were found to have the Kirsten rat sarcoma viral oncogene (KRAS) p.G12C mutation (32). KRAS mutations, like mutations of other oncogenes, are associated with drug resistance and poorer outcomes in NSCLC (33).
KRAS mutations are also known to be present in 90% of smokers. KRAS is related to inflammation, and KRAS mutation is found in the most smokers, therefore, it may be some sort of inflammatory reaction in lung cells by smoking (34). The drug resistance induced by KRAS mutations is usually intrinsic. However, KRAS mutations are heterogeneous, i.e., there is more than one type, and different KRAS mutations lead to activation of different downstream signaling pathways. KRAS mutations do not result in changes in the phosphorylation of the AKT signaling pathway (35).
Fusion of the neurotrophic tropomyosin receptor kinase (NTRK) gene is a relatively rare oncogene mutation, which occurs in less than 1% of NSCLC patients. The detection of NTRK fusions relies on RNA-based next-generation sequencing (NGS) (36). The downstream signaling pathways include the MEK/ERK and PI3K/AKT signaling pathways. As mentioned above, these signaling pathways are related to cancer cell proliferation and migration, and the PI3K/AKT signaling pathway is also involved in apoptosis, which is induced by chemotherapy (36). In early-stage NSCLC with NTRK gene fusions (Figure 2), patients have a high RR to TKIs (37).
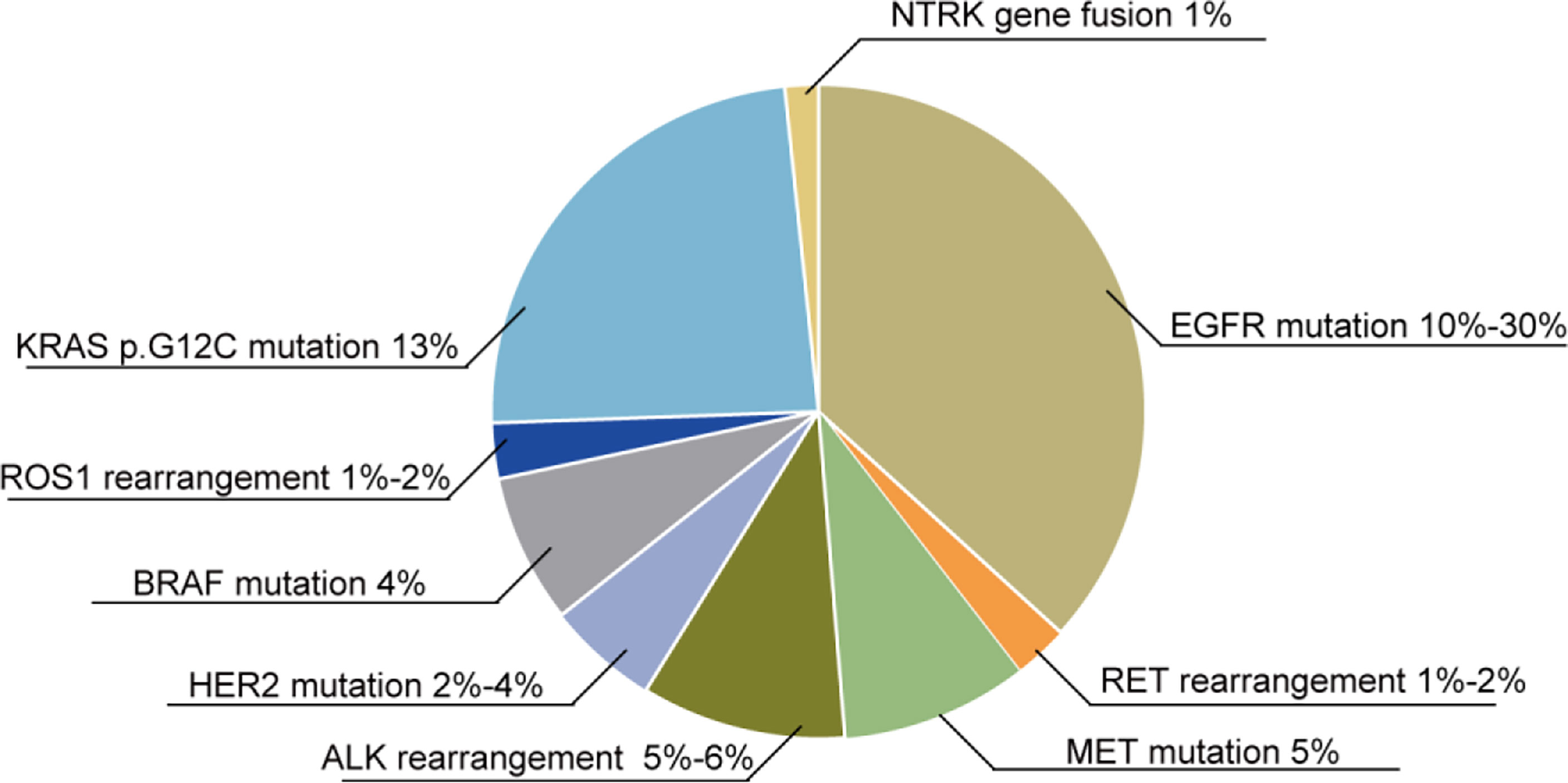
Figure 2 Oncogene mutations in NSCLC patients. Various oncogene mutations are found in NSCLC patients: 10%–30% of NSCLC patients exhibit EGFR mutations, 1%–2% have RET rearrangements, 5% have a MET mutation, 5%–6% have an ALK rearrangement, 2%–4% have a HER2 mutation, 4% have a BRAF mutation, 1%–2% have ROS1 rearrangements, 13% have the KRAS p.G12C mutation, and 1% have NTRK gene fusions.
Moreover, the immune checkpoint development also benefits NSCLC patients. If the mutation in patients does not concern the mutations above, then the programmed death ligand 1 (PD-L1) mutation maybe a better choice, but there are still some limits, for example, the mutation of PD-L1 at least appears 50% mutation in the lung cancer patients (38). The combination of programmed death 1 (PD-1) and PD-L1 would decrease immune response; therefore, the tumor cells will escape the surveillance of immune cells such as T cells. There are also some studies reported that the EGFR mutation in NSCLC could increase the expression of PD-L1 protein, and TKIs could reduce the amount of PD-L1 protein, the signaling pathways referring to this phenomenon are PI3K-AKT, STAT3, NF-κB, and MEK-ERK signaling pathways (39). Furthermore, ALK and KRAS mutations could improve the expression of PD-L1; therefore, if the patients are harboring PD-L1 and EGFR or ALK or KRAS at the same time, patients will have a higher RR when the interaction between PD-1 and PD-L1 is blocked (40). However, there is still no study that can verify the results for NSCLC patients harboring several mutations at the same time. The high PD-L1 expression is also associated with smoking, and PD-L1 usually appears in the early stage of NSCLC, and could become a biomarker in the diagnosis of lung cancer (41).
Apart from the targets mentioned above, there are also some signaling pathways abnormally expressed in NSCLC that could become new biomarkers in diagnosis and therapy, but these signaling pathways still stand in the preclinical stage.
2.2 long non-coding RNAs, microRNAs, and abnormal proteins in NSCLC
The long non-coding RNAs (lncRNAs) and microRNAs (miRNAs) are non-coding RNAs existing in the cells, and these non-coding RNAs are correlated with tumor progression and tumor features, for example, its proliferation, migration, invasion, resistance, and recurrence. In NSCLC, these non-coding RNAs also show a more important role, and some results in preclinical studies could give rise to new biomarkers or targets in the diagnosis and treatment of NSCLC.
2.2.1 lncRNAs and NSCLC
lncRNA H19 and miRNA-21 overexpress in the NSCLC tumor, and these could become biomarkers in the diagnosis and treatment of NSCLC (42). Circular RNAs (circRNAs) hsa_circ_0058357 overexpress in NSCLC, and the abnormal expression of hsa_circ_0058357 is associated with migration, proliferation, and apoptosis through increasing AVL9 accompanied by the inhibition of miR-24-3p (43). LncRNA SNHG14 is a cancer-promoting lncRNA, and it is upregulated in the lung cancer tissue; lncRNA SNHG14 could promote the migration, proliferation, and invasion of NSCLC cells; and lncRNA SNHG14 could inhibit the miR-206 expression; therefore, the downstream targets of miR-206 such as G6PD are upregulated (44). lncRNA ABHD11-AS1 is overexpressed in NSCLC, and it could upgrade the Warburg effect and proliferation of NSCLC. There is m6 A methyltransferase-like 3 (METTL3) in the upstream of lncRNA ABHD11-AS1, which could promote the expression of ABHD11-AS1, and the prognosis for NSCLC patients will get worse (45). lncRNA DUXAP8, an oncogenic lncRNA, could induce the proliferation, EMT, and aerobic glycolysis in lung cancer cells. Its effects will be studied further. Moreover, the overexpression of lncRNA DUXAP8 in NSCLC patients is correlated with the poor prognosis. The mechanisms here are diverse including transcriptional, post-transcriptional, and epigenetic regulation (46). The overexpression of lncRNA CCDC144NL-AS1 in NSCLC patients could promote the proliferation, migration, and invasion of NSCLC cells (H1299, A549, NCI-H650, and HCC827 cells). Mechanically, lncRNA CCDC144NL-AS1 could directly bind to miR-490-3p (47). There are also some other examples showing that lncRNA could be a biomarker in NSCLC, one is LncRNA HOTAIR that could promote the proliferation, invasion, and migration in NSCLC cells by regulating the CCL22 signaling pathway (48). lncRNA UFC1 could promote the progression of NSCLC by downregulating the expression of PTEN through zeste homolog 2 (EZH2) (49). LncRNA WTAPP1 could promote the invasion and migration of NSCLC cells by suppressing the expression of lncRNA HAND2-AS1 (50).
In addition, there are also some lncRNAs that play an inhibitor role in the progression of NSCLC. LncRNA NBR2 is downregulated in NSCLC patients, and the overexpression of lncRNA NBR2 could inhibit the migration of lung cancer cells (SPC-A1 cells) and the Notch signaling pathways are also suppressed, and the EMT-related genes are also reduced (51). lncRNA LINC00261 is downregulated in the lung cancer tissues, and the overexpression of lncRNA LINC00261 in A549 and SPC-A1 cells would inhibit metastasis in vitro and in vivo through regulating the miR-1269a/FOXO1 signaling pathway (52). There is a novel lncRNA BRCAT54 that is overexpressed in the lung cancer tissue, but this lncRNA benefits the patients, and its knockdown could promote the migration, proliferation, and apoptosis inhibition of lung cancer cells, which concern the regulation of JAK-STAT and calcium-related signaling pathways (53).
2.2.2 microRNAs and NSCLC
The microRNA functions in NSCLC are different. Some microRNAs show promotion in the progression of lung cancer, and others show inhibition in the progression of lung cancer.
Radiotherapy is useful for most NSCLC patients in the early stage, but radiotherapy is usually accompanied by acquired resistance. Acquired resistance has been proven to be correlated with the overexpression of miR-410 in NSCLC. Mechanically, miR-410 could induce EMT and target the PTEN/PI3K/mTOR signaling pathway (54). miR-10b aberrantly expresses in multiple malignant cancers, such as breast cancer, esophageal cancer, pancreatic cancer, and lung cancer, and it is related to proliferation and invasion (55). miRNA-21 is overexpressed in NSCLC and is related to the poor survival and prognosis of patients, especially with miRNA-21 being correlated with the radiation resistance of NSCLC. Therefore, the inhibition of miRNA-21 in NSCLC cells (A549 cells) could suppress proliferation and improve sensitivity to radiation through increasing apoptosis (56). miR-142-3p, on the one hand, could improve the sensitivity of NSCLC by downregulating the high-mobility group box-1 (HMGB1) protein and inhibiting autophagy. On the other hand, it could also play as an oncogene, and its overexpression is correlated with the poor outcome of NSCLC patients in clinical treatment, and promotes the migration and proliferation of NSCLC cells by downregulating TGFβR1 (57).
In the NSCLC tissues, miR-936 is at a low expression, and the overexpression of miR-936 could block the cell cycle, and inhibit the proliferation and invasion of NSCLC cells. At the same time, the downstream target E2F transcription factor 2 (E2F2) that could promote the invasion of NSCLC is downregulated (58). The overexpression of miR-221–3p could decrease the resistance of paclitaxel by inducing apoptosis accompanied by the inhibition of MDM2/p53 signaling pathway (59). miR-340 is at a lower expression of NSCLC tissues, and its overexpression could inhibit the migration and invasion of NSCLC cells through targeting RAB27B. In addition, the overexpression of miR-340 could suppress proliferation and induce apoptosis through regulating p27 (60). The level of miRNA-597 in the NSCLC tissues is lower than the normal tissue, and the downregulated miRNA-597 is related to the stage and poor prognosis of NSCLC patients. The overexpression of miRNA-597 could inhibit progression by regulating CDK2 (61). miR-4732-5p expression is inhibited in NSCLC; its downregulation is related to metastasis, late stage, and poor outcome of NSCLC patients. Its overexpression could suppress the proliferation, migration, and invasion of NSCLC cells (A549, HCC827, H23, and H1975 cells) by regulating TSPAN13 (also known as NET-6 and TM4SF13) that has been proven to inhibit proliferation and invasion in breast cancer (62, 63).
2.2.3 Abnormal proteins and NSCLC
There are also some proteins that overexpress in the NSCLC patients, which could become new targets in clinical trials. Fibulin2 (FBLN2) is decreased in the lung cancer cell lines, and the overexpression of FBLN2 would inhibit the activation of MAPK/ERK and AKT/mTOR signaling pathways, accompanied by the decreased migration and invasion of cells (64). This means that FBLN2 could be a potential biomarker for detecting NSCLC in the clinic. The abnormal expression of nuclear factor kappa B (NF-κb) is correlated with chemoresistance and radio-resistance in lung cancer therapy, and the inhibition of NF-κβ signaling pathway will decrease the resistance given by chemotherapy and radiotherapy (65). NF-κb is related to multi-signaling pathways such as apoptosis, angiogenesis, and inflammation; therefore, NF-κb is a relatively difficult oncogenic mutation compared with other oncogene mutations such as EGFR and KRAS (66). Nuclear factor erythroid 2-related factor 2 (Nrf2) is increased and Keap1 in cytoplasmic is decreased, and these changes in Nrf2 and Keap1 are correlated with the poor outcome of NSCLC patients, and increased Nrf2 may contribute to chemoresistance when using platinum-related chemotherapy (67). Almost 25% of patients with NSCLC appear to have brain metastases, and there are several aberrant proteins arising in this process. NFATc1 and NFATc3 are listed in these biomarkers, and the expression of these two proteins is decreased in patients with brain metastases, at the same time, the downstream targets such as IL-11 (correlated with JAK-STAT3 signaling pathways), CDH5 (correlated with metastasis), and CCL2 (correlated with proliferation and apoptosis) are also regulated by NFATc1 and NFATc3 (68). Tripartite motif (TRIM) protein is a type of protein correlated with multiple malignant cancers including lung cancer, and takes part in various signaling pathways regulation including p53, NF-κB, and PI3K/AKT. In NSCLC, TRIM could play as an oncogene or suppressor. As disintegrins and metalloproteinases with thrombospondin motifs (ADAMTS8) are downregulated in NSCLC cells (H460 and A549 cells), the overexpression of ADAMTS8 could inhibit proliferation and induce apoptosis of lung cancer cells. Mechanically, the vascular endothelial growth factor A (VEGFA) and CD31 are suppressed (69). Neurexophilin 4 (NXPH4) is overexpressed in NSCLC tissues, and its knockdown could suppress the proliferation and migration of NSCLC cells (A549, H226, H2106, and HCC827 cell line), and trigger cell cycle arrest in phase S1. EZH2 was in the upstream of NXPH4, and could activate the expression of NXPH4; then, the activated NXPH4 could downregulate the expression of CDKN2A, and the downregulated CDKN2A could regulate the cyclinD-CDK4/6-pRB-E2F signaling pathway resulting in the cell cycle activation and the promotion of proliferation and migration of lung cancer cells (70).
2.3 CSCs and lung cancer
Cancer stem cells (CSCs) are considered to be the root of cancer, and evidence confirm that CSCs are related to chemoresistance and recurrence and the survival of lung cancer patients. Therefore, there are many compounds targeting CSCs in preclinical or clinical trials. There are also other strategies that inhibit the stemness of cancer cells. More specifically, targeting signaling pathways such as Wnt, hippo, and notch could inhibit the stemness of cancer cells or the biomarkers correlated with CSCs (71). CSCs also exist in NSCLC, and lung cancers also have the feature of stemness; therefore, these facts confirm that targeting CSCs in NSCLC is crucial (72, 73).
Lung cancer stem cells (LCSCs) with high chemo-resistance were obtained from the NSCLC patients; the subpopulation of LCSCs show self-renewal, resistance, invasion, and tumorigenic potential in the in vitro experiments, and the CDKN1A, ITGA6, and SNAI1that were selected by different expression levels between LCSCs and the adherent-cultured cells could become biomarkers for indicating the different stages of lung cancer in patients (74). The LCSC biomarkers in humans include CD133+, CD90+, CD44+, CD87+, ABCG2, SP, and ALDH (75). Forkhead box C1 (FOXC1) is correlated with the CSC features, and is elevated in NSCLC. The knockdown of FOXC1 could decrease the subpopulation of CD133+ cells, and the associated genes, such as NANOG, ABCG2, SOX2, and Oct4, are also downregulated, and the chemo-sensitivity for cisplatin, docetaxel, and gefitinib is also increased (76). m6A demethylase ALKBH5 is upregulated in LCSCs, and its knockdown could contribute to the E-cadherin upregulation and stem markers such as NANOG and Oct4 are downregulated. Mechanically, there is a positive relationship between ALKBH5 and p53, and the knockdown of p53 would make ALKBH5 downregulate, and the tumor formation ability and invasion are also suppressed (77). Nerve injury-induced protein 1 (Ninj1) is upregulated in NSCLC cells and tissues; the subpopulation of Ninj1high LCSCs exhibits the CSC-related features such as the increase of ALDH+ subpopulation, sphere-forming ability, and stemness markers; and the downstream signaling pathway Wnt/β-Catenin is also activated by Frizzled2-LRP6 assembly (78). Histamine N-methyltransferase (HNMT) is overexpressed in NSCLC tissues as found in clinical trials, and is related to a poor prognosis for patients. Moreover, HNMT has a positive relationship with HER2 that could improve the features of CSCs. The knockdown of HNMT could decrease the tumorsphere formation ability, and reduce the expression of CSC markers such as NANOG, CD133, OCT4, and KLF4 through the Nrf2/HO-1/HER2 signaling pathway increasing the accumulation of reactive oxygen species (ROS) (79). The stemness markers ALDH and CD133 are well-verified in LCSCs; p53 is a cancer suppressor, the mutation which is found in 47% of NSCLC cases, and the knockdown of the three genes could reduce the CSC characteristics and prolong the survival of NSCLC patients (80). This study is a reminder that the stemness markers may have some therapeutic effect in NSCLC patients. Heat shock protein 90 (hsp90) inhibitors show better results in clinical use, but in therapy, there is resistance that maybe correlated with CSCs in lung cancer. However, there is a new Hsp90 inhibitor named NCT-80 that could reverse CSCs resulting to resistance by regulating STAT3/Wnt/β-catenin signaling pathways (81). RNF168, a E3 ubiquitin ligase, is downregulated in lung adenocarcinoma, but upregulated in squamous cell carcinoma; the overexpression of RNF168 could inhibit the CSC features (such as sphere-formation ability, stemness markers ALDH) of NSCLC cells. Mechanically, the RNF168 could ubiquitylate RhoC and cause its degradation (82). Non-muscle myosin heavy chain 9 (MYH9) is upregulated in lung cancer, and correlated with the worst prognosis in NSCLC patients, and the overexpression of MYH9 in lung cancer cells could improve the expression of stemness markers (such as SOX2, OCT4, Nanog, CD133, and CD44) and sphere-formation ability by regulating the mTOR signaling pathway (83). The Orai3 channel is a calcium channel related to the chemoresistance of lung cancer, and the overexpression of Orai3 could improve metastasis in NSCLC. LCSCs, derived from NSCLC cells with cisplatin resistance, has a higher expression of Orai3, and the silence of Orai3 could worsen metastasis, accompanied by a sensitivity to cisplatin. Moreover, stemness markers such as Sox2 reduced through regulating the PI3K/AKT signaling pathway (84).
Overall, there are still many stemness markers of NSCLC studied in the preclinical and clinical trials, and the development of small molecular markers could become the new targets or diagnostic markers for the different stages of lung cancer.
3 The diagnosis of lung cancer
Except for the symptom of coughing appearing in the early stage of lung cancer, most lung cancer patients are asymptomatic in the early stage; therefore, early diagnosis and treatment could be missed. The development of technology in diagnosis could save majority of patients and could prolong their lives. Diagnostic methods mainly include image test, biopsy test, and biomarker test.
3.1 Image test
Image tests, such as computed tomography (CT), PET scan, and magnetic resonance imaging (MRI) scan, play an important role in the diagnosis of lung cancer. CT is the most common diagnostic means in lung cancer, which could determine tumor size (≥ 6 mm) and the number of nodules in lung cancer patients. It also could test the metastases, especially the mediastinal lymph nodes in the lung cancer patients (85–87). CT could also detect if the nodules are benign or malignant, but for further determination, biopsy is still needed (88). PET has more sensitivity and specificity than CT because the PET scan uses fluorine-18 fluorodeoxyglucose (F-18 FDG) as the biomarkers. It could locate in the malignant lesions with aberrant glucose metabolism (89). PET could also test if the lesions are benign or malignant, and it also differentiates the different types and staging (especially the distant metastases) of lung cancer by the uptake degree of FDG (90, 91). MRI scan has been used in NSCLC patients with brain and bone metastases because the dye used in MRI scan is not suitable for tissues that can move. With the development of high-performance gradient systems, phased-array receiver coil, and optimized imaging sequences, MRI could also detect nodules in lung tissues; the lowest size of nodules that can be detected is 3 mm (92).
3.1.2 Biopsy test
Furthermore, the identification of lung cancer also needs biopsy (93), which could be tissue or liquid biopsy. Tissue biopsy is a type of invasive mean, and liquid biopsy is a non- invasive mean. Tissue biopsy is the gold standard to test lung cancer in the clinic. The determination of different histological types of lung cancer relies on tissue biopsy (94). Tissue biopsy could also test the mutations in lung cancer, but lung biopsy usually has complications (95). With the limitations of liquid biopsy, its application is restricted. In liquid biopsy, the sample used is the peripheral blood of the NSCLC patients, and the common testing indicators are circulating tumor DNA (ctDNA), circulating tumor cells (CTCS), and exosomes (96). In addition, it could also detect miRNA, circRNAs, circulating tumor vascular endothelial cells (CTECs), and tumor-educated blood platelets (TEPs) (97). Compared with tissue biopsy, liquid biopsy is more sensitive, effective, practical, and acceptable, and it could provide different mutations in the tumor (98).
3.1.3 Biomarker test
Regarding the development of targeted therapy in NSCLC, if patients are diagnosed with NSCLC, then they are advised to take molecular testing to verify possible mutations. The methods are diverse. For example, polymerase chain reaction (PCR) could identify signal gene mutation, mostly used in determining the mutation of EGFR in the clinic (99). Fluorescence in situ hybridization (FISH) was approved by the food and drug administration (FDA) to test ALK rearrangements by fixing the tissue in formalin and embedding in paraffin (20). FISH could also diagnose the aberrant expression of ROS1, RET, HER2, and MET (100). Immunohistochemistry (IHC) analysis is suitable for testing the mutations of PD-L1 (approved by FDA), ROS1, EGFR, BRAF-V600E, and RET (101). Moreover, IHC could be used in testing the mutation of ALK (approved by FDA) (102). NGS is suitable for almost all of mutations appearing in the NSCLC, such as EGFR, RET, MET, ALK, HER2, BRAF, ROS1, KRAS, and NTRK, also including some new biomarkers such as PIK3CA (103). The NGS efficiency is high, the needed sample is small, and the cost is relatively low; therefore, there are more applications of NGS in the clinic.
The development of diagnostic methods in lung cancer (Table 1) could help most patients diagnosed in the early stage; therefore, the treatments for lung cancer could work.
4 Treatments for lung cancer
4.1 Chemotherapy and chemoradiotherapy
4.1.1 Chemotherapy
Before targeted therapy, chemotherapy dominated the clinical treatment for lung cancer. After the gene types of NSCLC have been identified in the clinic, chemotherapy was gradually replaced by targeted therapy, but chemotherapy also concerns cisplatin combination therapy. Currently, chemotherapy in NSCLC mostly involves cisplatin and carboplatin plus gemcitabine, taxanes, and pemetrexed plus some targeted therapy drugs such as VEGFR inhibitor (bevacizumab) or EGFR inhibitor (erlotinib) (104). The mechanism of chemotherapy is diverse. Cisplatin, carboplatin, and gemcitabine could disturb the DNA repair system, create DNA damage, and induce apoptosis in the cancer cell (105, 106). Taxanes could interfere with microtubule dynamics, trigger cell cycle arrest, and induce apoptosis (107, 108). Pemetrexed, an antifolate drug, could cause cell cycle arrest in the S phase (109).
The limitations of chemotherapy in lung cancer treatment mainly involve intrinsic resistance even though the compounds could have some effects at the first early treatment, but the tumor can acquire resistance rapidly (110). This disturbs the process of chemotherapy in the lung cancer treatment. There are various mechanisms of resistance in lung cancer. CSCs are correlated with the resistance of chemotherapy and radiation therapy as some compounds directly targeting CSCs could reduce the resistance in lung cancer therapy and improve the outcome of chemotherapy and radiosensitivity. The compounds target CSCs, mostly targeting the representative signaling pathways in the CSCs, such as Notch, MYC. RO4929097 (an inhibitor of Notch signaling pathway, γ-secretase inhibitor) combined with erlotinib could improve the efficiency of erlotinib in advanced NSCLC with chemoresistance and the PFS was up to 5 years (NCT01193881 (first posted: 2 September 2010), NCT01193868 (first posted: 2 September 2010)). In preclinical research, sulforaphane could inhibit the properties of LCSCs, such as sphere-forming ability, biomarkers of LCSCs, which could combine with cisplatin and doxorubicin to reduce the chemoresistance of NSCLC (111). Additionally, there are also some signaling pathways related to the resistance of lung cancer, which could provide a combined strategy for chemotherapy to overcome the resistance further. For example, Acetyl-11-keto-β-boswellic acid (AKBA) could improve the sensitivity of cisplatin in NSCLC through targeting P21, which maybe correlated with the increase of apoptosis and the inhibition of autophagy (112). This study reminds us that AKBA could become a new combination therapy in the clinic, even though it is still in preclinical research. The regulation of cell death such as autophagy, apoptosis, and ferroptosis could provide a new perspective to reducing resistance in chemotherapy (71).
Moreover, chemotherapy and radiotherapy have a function in neoadjuvant or adjuvant therapy in stage III NSCLC patients. Chemotherapy could help ensure that surgery goes well and could also serve as supplement after surgery (113). For example, patients with nodal metastases after surgery could benefit from adjuvant cisplatin-based therapy, and induction therapy could serve as a precondition for surgery (114, 115).
4.1.2 Chemoradiotherapy
Radiotherapy is usually used in the local control of different stages of lung cancer, especially stage III unresectable NSCLC, which accounts for 30% in NSCLC patients (104). Moreover because of the development of four-dimensional computed tomography (4DCT), stereotactic body radiotherapy (SBRT), and intensity-modulated radiotherapy (IMRT), the side effects of radiotherapy are reduced (116). However, even though radiotherapy is the standard therapy for stage III NSCLC patients, the survival rate of patients has not improved. After the application of sequential radiotherapy to patients, the overall survival (OS) improved, but elderly patients still have not benefited from it. Therefore, combination therapy with radiotherapy may be of benefit to diverse patients with different states of health (117).
The mechanism of radiotherapy is mainly the damage of DNA, and damaged DNA could induce immune responses in the lung cancer; therefore, the combination therapy of radiotherapy and immunotherapy could produce a better result in the treatment of lung cancer (118). This combination has been verified by clinical trials. For example, in a phase III trial (NCT02125461 (first posted: 29 April 2014)), the conventional chemoradiotherapy (platinum-based chemotherapy and radiotherapy) plus durvalumab (an immune checkpoint inhibitor of PD-L1) could significantly prolong OS (up to 4 years) in stage III NSCLC patients compared with chemoradiotherapy alone, and the PFS of patients was also up to 3 years (119).
Chemoradiotherapy (CRT) mostly adjusts to the limited-stage SCLC. In addition, CRT also offers benefit for the lung cancer without metastasis. The chemotherapy in chemoradiotherapy generally includes cisplatin–etoposide (120) and carboplatin plus etoposide (121).
4.2 Targeted therapy
The lung cancer is driven by mutation of multiple oncogenes, the targetable alterations in the clinic provide probability for targeted therapy (122). In order to conduct targeted therapy in lung cancer patients, the molecular mutations in the tumor must be confirmed by diagnostic assays (123). The development of NGS provides a method to test the mutations appearing in lung cancer patients, which could help them get precision and personalized treatment in the clinic (124).
4.2.1 Drugs approved by FDA
The targets that have drugs approved by FDA include EGFR (gefitinib (brand name: Iressa, company: ASTRAZENECA,London, the UK), erlotinib (brand name: Tarceva, company: OSI PHARMS, Ardsley, the USA), afatinib (brand name: Gilotrif, company: BOEHRINGER INGELHEIM, southwest Washington, the USA), dacomitinib (brand name: Vizimpro, company: PFIZER, New York City, the USA) and osimertinib (brand name: Tagrisso, company: ASTRAZENECA,London, the UK)), ALK (crizotinib (brand name: Xalkori, company: PF PRISM CV, Netherlands), alectinib (brand name: Alecensa, company: HOFFMANN-LA ROCHE, Basel, Switzerland), brigatinib (brand name: Alunbrig, company: TAKEDA PHARMS USA, Lexington, the USA), ceritinib (brand name: Zykadia, company: NOVARTIS, Basel, Switzerland), and lorlatinib (brand name: Lorbrena, location and company: PFIZER, New York City, the USA), ROS1 (crizotinib (brand name: Xalkori, company: PF PRISM CV, Netherlands), lorlatinib (brand name: Lorbrena, company: PFIZER, New York City, the USA), entrectinib (brand name: Rozlytrek, company: GENENTECH INC, Pennsylvania, the US) and brigatinib (brand name: Alunbrig, company: TAKEDA PHARMS USA, the USA), RET (pralsetinib (brand name: Gavreto, company: GENENTECH INC, Pennsylvania, the US) and selpercatinib (brand name: Retevmo, company: LOXO ONCOLOGY INC, Massachusetts, the USA)) (123, 125–127). Some targets such as HER2, KRAS, BRAF, NTRK, and MET in the clinical trials benefit from the development of genomic profiling (128). The drugs target HER2 mainly including TKIs (pyrotinib and tucatinib), mono-antibody (trastuzumab), and antibody–drug conjugates (trastuzumab deruxtecan) (129, 130), target KRAS contain adagrasib (MRTX849) and sotorasib (AMG510) (122), target BRAF (dabrafenib plus trametinib) (NCT04452877 (first posted: 1 July 2020)), target NTRK (larotrectinib and entrectinib) (NCT02576431 (first posted: 15 October 2015), NCT02568267 (first posted: 5 October 2015)), and target MET (crizotinib) (NCT04084717 (first posted: 10 September 2019)). The drugs approved by FDA significantly improved the OS of patients, such as gefitinib that improved the median PFS (mPFS) by almost 10.8 months, erlotinib increased mPFS by nearly 14 months, afatinib improved PFS by approximately 48 months, and dacomitinib increased mPFS up to 14.7 months (131–133). The mPFS of patients after taking osimertinib increased 18 months (134). The mPFS of patients with ALK-positive or ROS-1-positive NSCLC was increased 8.2 months after taking crizotinib and the OS was up to 114 months after taking lorlatinib (135, 136). The mPFS of ALK-positive metastatic NSCLC patients improved by 34.8 months after taking alectinib, and 7.8 months for ceritinib (135, 137). Brigatinib for NSCLC patients with ALK-positive, ROS-1-positive, or EGFR mutation-positive could also improve PFS by almost 11.0 months (138). Pralsetinib and selpercatinib for NSCLC patients with metastatic RET fusion- positive could also improve mPFS by almost 17.1 months and 16.5 months, respectively (139).
4.2.2 Drugs still in preclinical and clinical trials
There are some drugs that are still in clinical trials, but also show significant effects on prolonging the OS of NSCLC patients. These drugs could give more hope to patients. For example, pyrotinib for advanced NSCLC with HER2 mutation was proved to prolong the PFS of patients for 6.9 months and the median OS for 14.4 months in clinical trial (NCT02834936 (first posted: 15 July 2016)). Moreover, the new biomarkers found in the preclinical stage also provide targets for the treatment of lung cancer, for example, the mutations of the PIK3CA gene (140) and overexpression of VEGF in lung cancer driven by smoking (141).
Even though targeted therapy could produce high RR and improve the OS of patients, the special targets, such as EGFR, ALK, and ROS1, only account for a very small part (<20%) in the lung cancer patients (142). Hence, there is an urgency to develop more nonspecific therapies so they can be used to treat more lung cancer patients. The high cost of targeted therapy in the clinical treatment of lung cancer still limits its usage (143). Additionally, there are also some questions such as chemo-resistance in clinical therapy with the wide use of targeted drugs. The mechanism of acquired resistance in NSCLC after treatment with EGFR TKIs for several months mainly includes the hepatocyte growth-factor receptor amplification. Currently, deoxypodophyllotoxin (DPT) has been reported to reduce the resistance of HCC827GR cells by targeting EGFR and the hepatocyte growth-factor receptor, and induce apoptosis. This study could provide a combination therapy for the use of EGFR TKIs to reduce acquired resistance in the clinic (144). Furthermore, there are other therapies combined with targeted drugs that are in clinical trial.
The combination of erlotinib (an EGFR inhibitor) and bevacizumab (a monoclonal antibody targeting VEGF) could prolong the PFS of NSCLC patients (NCT02759614 (first posted: 3 May 2016)) (145). This reveals the probability of VEGF and EGFR double inhibition in the untreated metastatic EGFR-mutated NSCLC. Apatinib (a VEGFR inhibitor) plus gefitinib (a first-generation EGFR TKI) could prolong the mPFS for 19.2 months in advanced NSCLC with EGFR mutation, but this combination therapy also has some side effects and the quality of life (QoL) did not change (NCT02824458 (first posted: 6 July 2016) (146). The use of osimertinib (a third generation of EGFR TKI) is usually accompanied by chemo-resistance in the terminal treatment of advanced EGFR-mutated NSCLC patients; the reason maybe because the second-site mutations appear in the EGFR. Therefore, osimertinib plus dacomitinib (a pan-HER inhibitor) could reduce drug resistance appearing in therapy, in a phase I/II trial (NCT03810807 (first posted: 22 January 2019)) (147). Moreover, the combination of osimertinib and navitoclax (an inhibitor of BCL-2 that could increase apoptosis and reduce chemo-resistance) was feasible in patients with EGFR-mutated NSCLC in a phase IB trial (NCT02520778 (first posted: 13 August 2015)) (148). The inhibitors targeting KRAS mostly through targeting KRAS p. G12c, for example, AMG510 and MRTX849 are still in the clinical study (149). AMG15 was used to treat patients with advanced metastatic NSCLC patients with KRAS p. G12c mutation in a phase 3 study (NCT04303780, first posted: 11 March 2020). MRTX849 showed better results in NSCLC patients, but had more side effects compared with AMG510. However, for clinical studies such as NCT04613596 (first posted: 3 November 2020), NCT04685135 (first posted: 28 December 2020), and NCT04330664 (first posted: 1 April 2020) results are yet to be obtained. AMG510 had already been approved by FDA. ARS-1620, an inhibitor of KRAS p. G12c is still in the preclinical stage but shows better anti-cancer ability in NSCLC through targeting his95 amino acid on KRAS p. G12c (150).
However, there are also some combination therapies that did not reach the expected results. For example, the combination of binimetinib (a MEK inhibitor), cisplatin, and pemetrexed did not improve anti-tumor activity compared with the chemotherapy of cisplatin and pemetrexed in advanced NSCLC with KRAS mutation (151). In a phase II study (NCT03133546 (first posted: 28 April 2017)), the combination of osimertinib (an EGFR TKI) and bevacizumab (a monoclonal antibody targeting VEGF) did not prolong the PFS in patients with advanced NSCLC with EGFR and T790M mutations; instead, the side effects increased (152). However, these trials also provide a guidance for clinical therapy (Table 2).
4.3 Antiangiogenic therapy
The abnormal growth of tumor is always accompanied by angiogenesis to supply nutrition for the cancer (153). Molecular markers such as hypoxia-inducible factor (HIF), vascular endothelial growth factor (VEGF), and VEGF receptor (VEGFR) play an important role in this process, and the most used targets are VEGF and VEGFR in cancer therapy (154). In addition, VEGF in the tumor microenvironment (TME) could inhibit the immune reaction of the immune cells. Therefore, VEGF inhibitors could also increase the capacity of immune cells (155). This reminds us that antiangiogenic therapy could combine with immunotherapy to benefit cancer patients. In clinical therapy, using antiangiogenic strategy usually involves two ways, namely, using the antibody to block the reaction between VEGF and VEGFR and using TKIs to inhibit the VEGFR and corresponding signaling pathways (156).
Bevacizumab (brand names: Avastin, Mvasi, Zirabev, company: GENENTECH, AMGEN INC, PFIZER INC), a monoclonal antibody targeting VEGF, has been approved by FDA and could play a role in the NSCLC treatment. The most widely explored use of bevacizumab is in combination therapy. Bevacizumab could increase the PFS (up for 4.4 months) and median OS compared with chemotherapy, but there is no difference of OS between the two therapies (NCT00318136 (first posted: 26 April 2006), NCT00806923 (first posted: 11 December 2008)), and the combination therapy of antiangiogenic therapy plus chemotherapy (bevacizumab plus cisplatin and gemcitabine) could prolong the median OS more than 13 months (157). Bevacizumab and atezolizumab are confirmed to be a potential therapy for the non-squamous NSCLC patients with higher PD-L1 expression (≥50%) but without EGFR/ALK/ROS1 mutations, in a phase II study (NCT03836066 (first posted: 11 February 2019)) (158). In a phase III trial (NCT02366143 (first posted: 19 February 2015)), bevacizumab combined with immunotherapy atezolizumab and chemotherapy (carboplatin and paclitaxel) could act as the first-line treatment in NSCLC patients with KRAS and STK11 mutations and/or STK11, KEAP1, TP53 mutations and/or high PD-L1 expression (≥50%) (159), and the PFS of patients was up to 29 months and the OS of patients was prolonged by almost 53 months. Moreover, the biosimilars of bevacizumab, such as FKB238 and LY01008 have also shown the same efficiency and safety in non-squamous NSCLC patients, and the patients’ PFS and OS were almost 30 months after taking these drugs. These trials were in the phase III (NCT02810457 (first posted: 23 June 2016), NCT03533127 (first posted: 22 May 2018)) (160, 161).
VEGFR includes VEGFR1, VEGFR2, and VEGFR3. Even though VEGFR1 and VEGFR2 correlated with angiogenesis, the affinity between VEGFR1 and VEGF is relatively weak. In addition, VEGFR3 regulates lymphangiogenesis (162, 163). Therefore, the target used in anti-angiogenesis in the clinic is usually VEGFR2. Apatinib, a VEGFR2 TKI, has been confirmed to significantly increase the PFS in advanced NSCLC patients with EGFR mutation combined with gefitinib, but the QoL did not change (NCT02824458 (first posted: 6 July 2016)) (146). In a phase IB clinical trial (NCT04670107 (first posted: 17 December 2020)), anlotinib, a multitarget receptor of TKI, plus PD-1 inhibitor camrelizumab showed some efficiency in advanced NSCLC patients who are resistant to the first-line therapy (164).
4.4 Immunotherapy
Immunotherapy in NSCLC usually uses some antibodies to block the recognize between the antigens in immunocytes and ligands in tumor cells (165). Immune checkpoint inhibitors (ICIs) are usually used in advanced and metastatic NSCLC (166). The most widely used targets in NSCLC include cytotoxic T-lymphocyte-associated protein 4 (CTLA-4), programmed death receptor 1 (PD-1), and programmed death-ligand 1 (PD-L1) (167).
The corresponding monoclonal antibodies that are well-developed include anti-CTLA-4 antibody (ipilimumab (brand names: Yervoy, company: BRISTOL MYERS SQUIBB)), anti-PD-1 antibodies (pembrolizumab (brand names: Keytruda, company: MERCK SHARP DOHME), and nivolumab (brand names: Opdivo, company: BRISTOL MYERS SQUIBB)), and anti-PD-L1 antibodies (atezolizumab (brand names: Tecentriq, company: GENENTECH INC), durvalumab (brand names: Imfinzi, company: ASTRAZENECA UK LTD), and avelumab (brand names: Bavencio, company: EMD SERONO INC)) (168). Recently, immunotherapy in NSCLC has been further developed and plays an even more important role in NSCLC. The drugs approved by FDA in immunotherapy could improve the survival of patients. For example, ipilimumab could improve the patients’ PFS up to 0.84 years, and these are patients normally with PD-L1 overexpression and no EFGR or ALK mutation (169). Patients with metastatic NSCLC with high PD-L1 expression (≥50%) and without EGF) or ALK mutation could improve mPFS for 10.3 months and median OS for 15.5 months after taking pembrolizumab and atezolizumab (170, 171). Patients with metastatic NSCLC with EGFR- or ALK-positive mutation could acquire a better mPFS (4.2 months) and median OS (14.4 months) (172). Avelumab could improve the PFS almost 907 days in patients with PD-L1 positive and after failure of a platinum-based doublet (NCT02395172 (first posted: 20 March 2015)). Durvalumab was proved to increase the PFS up to 907 days and OS up to 1,420 days after chemotherapy and radiotherapy failed for patients with unresectable stage III NSCLC in a phase III trial (NCT02395172 (first posted: 20 March 2015)). Sugemalimab, an anti-PD-L1 monoclonal antibody, was used in stage IV NSCLC (NCT03789604 (first posted: 28 December 2018)) (173). In a phase III trial, sugemalimab had the same OS and better PFS compared with durvalumab (174). Toripalimab, an anti-PD-1 antibody, was reported to play a role in the limited-stage small cell lung cancer, which has no reaction to the current chemotherapy (NCT04418648 (first posted: 5 June 2020)). In a phase II study (NCT04304248 (first posted: 11 March 2020)), toripalimab combined with platinum-based doublet chemotherapy could produce higher MPR/pCR rates in stage III NSCLC (175).
Other immunotherapies for NSCLC usually takes combination therapy and not limited to the monoclonal antibody alone. The combination therapy including immunotherapy plus chemotherapy (chemo-immunotherapy), immunotherapy plus radiotherapy, chemo-immunotherapy and radiotherapy. In a phase III trial (NCT02492568 (first posted: 8 July 2015), NCT02444741 (first posted: 14 May 2015)), pembrolizumab (an anti-PD-1 antibody) with radiotherapy could significantly increase the outcome of metastatic NSCLC patients (176). In a phase III trial (NCT02477826 (first posted: 23 June 2015)), nivolumab (an anti-PD-1 antibody) plus ipilimumab has a long-term efficacy in patients who have advanced NSCLC (177), but this combination could not prolong the OS in extensive-disease SCLC patients, in a phase III trial (NCT02538666 (first posted: 2 September 2015)) (178). Furthermore, nivolumab plus ipilimumab combined with chemotherapy such as platinum doublet (179) or two cycles of chemotherapy (180) could extend the OS of patients in advanced stages compared with chemotherapy alone. Durvalumab, an anti-PD-L1 antibody, also combined with other monoclonal antibodies, chemotherapy or radiotherapy, has a better outcome compared with durvalumab alone. The most common combination is durvalumab and tremelimumab (an anti-CTLA-4 antibody) plus radiotherapy or chemotherapy. In a phase II study (NCT03373760 (first posted: 14 December 2017)), the combination of durvalumab and tremelimumab has some activity in patients with advanced NSCLC with resistance to PD-(L)1 therapy, and the OS of patients was 7 months (181). Durvalumab and tremelimumab plus chemotherapy such as platinum had no marked improvement on the OS of patients with advanced NSCLC (182). Furthermore, durvalumab and/or tremelimumab plus radiotherapy improves the efficacy and tolerance of NSCLC patients who are not suited for chemotherapy (NCT05000710 (first posted: 11August 2021)). Therefore, the optimum combination with durvalumab still needs more research to explore. However, current research also provides an option for the patients. Camrelizumab is an investigational PD-L1 inhibitor. The combination therapy involving camrelizumab has also been a research interest. In a phase III trial (NCT03668496 (first posted: 12 September 2018)), camrelizumab plus chemotherapy such as carboplatin and paclitaxel could dramatically extend the PFS (9.1 months) and median OS (18.2 months) in patients with advanced NSCLC (183). The same result was also found in another phase III trial (NCT03134872 (first posted: 1 May 2017)). The combination of camrelizumab and chemotherapy including carboplatin and pemetrexed could also ameliorate the mPFS (11 months) of NSCLC patients without EGFR and ALK mutations (184). More interestingly, in a phase Ib/II study (NCT03268057 (first posted: 31 August 2017)), pepinemab that mainly treats Alzheimer’s disease and Huntington’s disease in combination with avelumab (an anti-PD-L1 antibody) was proved well-tolerated in NSCLC patients (185). Even though the patients’ mPFS was only 8.4 weeks in this trial (Table 3), this clinical study provides a new option for the treatment of NSCLC (Figure 3).
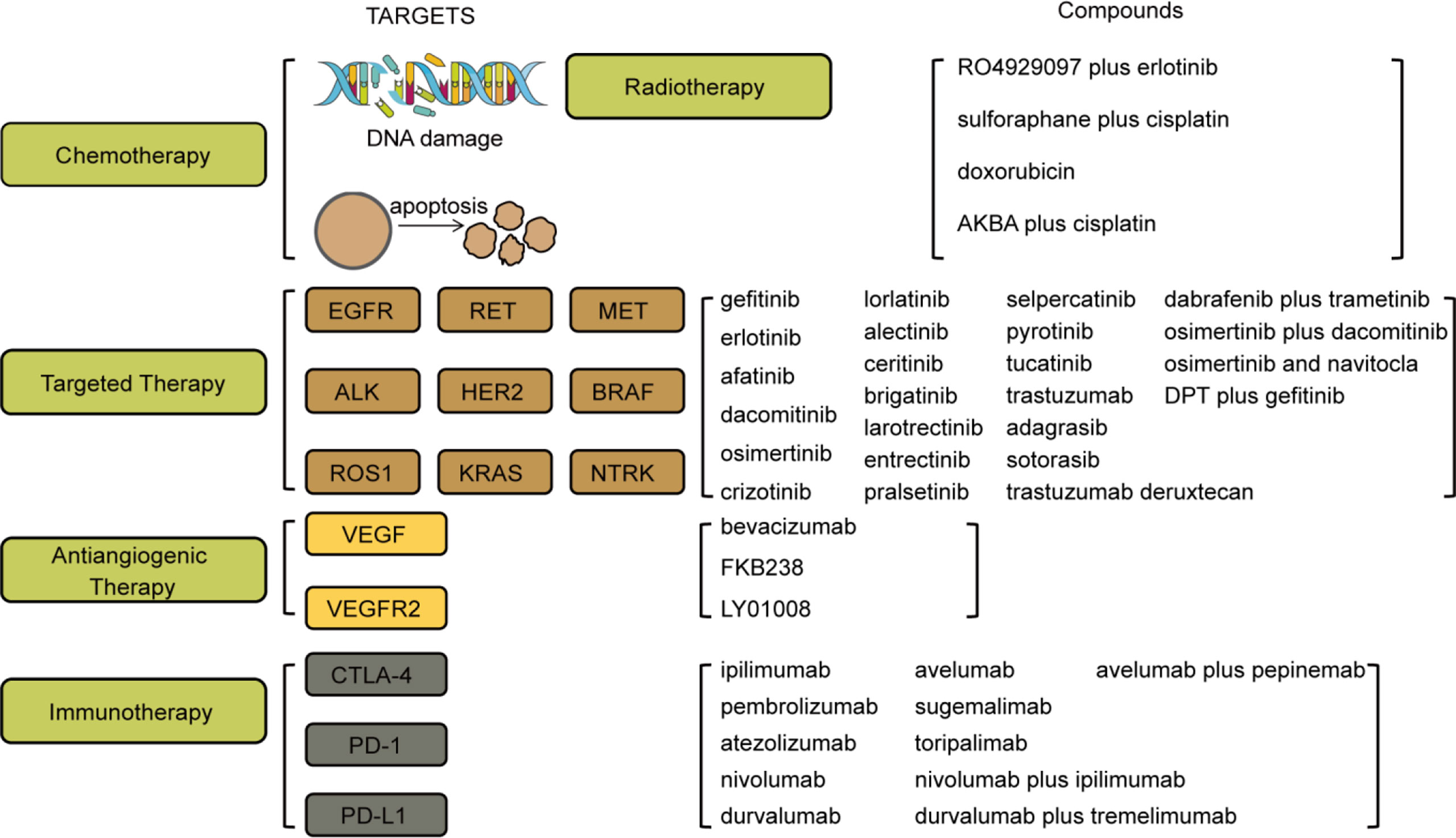
Figure 3 Targets and compounds in the treatment of NSCLC. Therapies in use are chemotherapy, targeted therapy, antiangiogenic therapy, and immunotherapy. Targets in chemotherapy include DNA damage and apoptosis. Targets in targeted therapy are EGFR, RET, MET, ALK, HER2, BRAF, ROS1, KRAS, and NTRK. Targets in antiangiogenic therapy are VEGF and VEGFR2. Targets in immunotherapy are CTLA-4, PD-1, and PD-L1. The corresponding drugs or compounds are listed on the right.
5 Conclusion
Lung cancer is already becoming a worldwide threat to human life. NSCLC is a major type of lung cancer. In this review, we described the causes, biological features, (especially the mutations (EGFR mutation, T790M mutation, RET rearrangements, MET mutation, ALK rearrangement, HER2 mutation, BRAF mutation, ROS1 rearrangement, KRAS mutation, NTRK fusions, and PD-L1 mutation)), abnormal signaling pathways (MAPK/ERK, Bax/Bcl-2, FAK/AKT, ERK, PI3K/AKT/mTOR, JAK-STAT, RAS/MAPK, MDM2/p53, PTEN/PI3K/mTOR, MAPK/ERK, and NF-κβ signaling pathways), diagnostic methods (such as CT, PET scan, MRI scan, tissue biopsy, liquid biopsy, PCR, FISH, IHC, and NGS), and therapies for lung cancer, such as chemotherapy, chemoradiotherapy, targeted therapy, antiangiogenic therapy, immunotherapy, and some combination therapy. More specifically, we reviewed current drugs used in the clinic, including chemotherapy (RO4929097 plus erlotinib, sulforaphane plus cisplatin and doxorubicin, AKBA plus cisplatin), targeted therapy (gefitinib, erlotinib, afatinib, dacomitinib, osimertinib, crizotinib, lorlatinib, alectinib, ceritinib, brigatinib, dabrafenib plus trametinib, larotrectinib, entrectinib, pralsetinib, selpercatinib, pyrotinib, tucatinib, trastuzumab, trastuzumab deruxtecan, adagrasib, sotorasib, DPT plus gefitinib, osimertinib plus dacomitinib, osimertinib, and navitoclax), antiangiogenic therapy (bevacizumab, FKB238, LY01008), immunotherapy (ipilimumab, pembrolizumab, atezolizumab, nivolumab, durvalumab, avelumab, sugemalimab, toripalimab, nivolumab plus ipilimumab, durvalumab plus tremelimumab, avelumab plus pepinemab), combination therapy, such as chemoradiotherapy plus immunotherapy (conventional chemoradiotherapy (platinum-based chemotherapy add radiotherapy) plus durvalumab, toripalimab plus platinum-based doublet chemotherapy, camrelizumab plus chemotherapy such as carboplatin and paclitaxel, camrelizumab and chemotherapy including carboplatin and pemetrexed), targeted therapy plus antiangiogenic therapy (erlotinib plus bevacizumab, gefitinib plus apatinib), antiangiogenic therapy plus chemotherapy (bevacizumab plus carboplatin and paclitaxel, bevacizumab plus cisplatin and gemcitabine), antiangiogenic therapy plus immunotherapy (bevacizumab and atezolizumab, anlotinib plus camrelizumab), antiangiogenic therapy plus immunotherapy and chemotherapy (bevacizumab combined with atezolizumab and chemotherapy (carboplatin and paclitaxel)), and immunotherapy plus radiotherapy (pembrolizumab plus radiotherapy, durvalumab and/or tremelimumab plus radiotherapy). These diagnostic methods may also undergo further development accompanied by the application of deep learning artificial intelligence (AI) (187). From the drugs used in clinical treatment, we could find that combination therapy and targeted therapy or immunotherapy play an even more important role in the treatment of lung cancer. In addition, with increasing understanding of the pathogenesis of lung cancer and the development of sequencing, the novel targets in lung cancer could be found, and take a role in clinical drug development. Moreover, combination therapy with multi-types of treatment will benefit more patients with lung cancer.
Author contributions
QG, LL, YF, YZ, and ZY reviewed the literature and drafted the article. ZC organized the figures and tables. QG, LL, ZY, and WZ finalized the paper and provided suggestions for improvement. All authors participated in designing the concept of this manuscript. All authors contributed to the article and approved the submitted version.
Funding
The Medical Science and Technology Research Project of Henan Province (no. SBGJ202003010); the Medical Science and Technology Research Project of Henan Province (no. LHGJ20190675); the Doctoral Research Start-up Foundation of Henan Cancer Hospital.
Conflict of interest
The authors declare that the research was conducted in the absence of any commercial or financial relationships that could be construed as a potential conflict of interest.
Publisher’s note
All claims expressed in this article are solely those of the authors and do not necessarily represent those of their affiliated organizations, or those of the publisher, the editors and the reviewers. Any product that may be evaluated in this article, or claim that may be made by its manufacturer, is not guaranteed or endorsed by the publisher.
References
1. Yang D, Ma X, Song P. A prognostic model of non small cell lung cancer based on TCGA and ImmPort databases. Sci Rep (2022) 12(1):437–. doi: 10.1038/s41598-021-04268-7
2. Chen JW, Dhahbi J. Lung adenocarcinoma and lung squamous cell carcinoma cancer classification, biomarker identification, and gene expression analysis using overlapping feature selection methods. Sci Rep (2021) 11(1):13323. doi: 10.1038/s41598-021-92725-8
3. Chen M, Liu X, Du J, Wang XJ, Xia L. Differentiated regulation of immune-response related genes between LUAD and LUSC subtypes of lung cancers. Oncotarget (2017) 8(1):133–44. doi: 10.18632/oncotarget.13346
4. Autsavapromporn N, Klunklin P, Chitapanarux I, Jaikang C, Chewaskulyong B, Sripan P, et al. A potential serum biomarker for screening lung cancer risk in high level environmental radon areas: A pilot study. Life (Basel Switzerland) (2021) 11(11):1273–83. doi: 10.3390/life11111273
5. Raman V, Yong V, Erkmen CP, Tong BC. Social disparities in lung cancer risk and screening. Thorac Surg Clinics (2022) 32(1):23–31. doi: 10.1016/j.thorsurg.2021.09.011
6. Lövgren M, Leveälahti H, Tishelman C, Runesdotter S, Hamberg K. Time spans from first symptom to treatment in patients with lung cancer–the influence of symptoms and demographic characteristics. Acta Oncol (Stockholm Sweden) (2008) 47(3):397–405. doi: 10.1080/02841860701592392
7. Revels SL, Lee JM. Anti-angiogenic therapy in nonsquamous non-small cell lung cancer (NSCLC) with tyrosine kinase inhibition (TKI) that targets the VEGF receptor (VEGFR): perspective on phase III clinical trials. J Thorac Dis (2018) 10(2):617–20. doi: 10.21037/jtd.2018.01.105
8. Ahern E, Solomon BJ, Hui R, Pavlakis N, O'Byrne K, Hughes BGM. Neoadjuvant immunotherapy for non-small cell lung cancer: right drugs, right patient, right time? J Immunother Cancer (2021) 9(6):1–9. doi: 10.1136/jitc-2020-002248
9. Gershman E, Guthrie R, Swiatek K, Shojaee S. Management of hemoptysis in patients with lung cancer. Ann Trans Med (2019) 7(15):358. doi: 10.21037/atm.2019.04.91
10. de Sousa VML, Carvalho L. Heterogeneity in lung cancer. Pathobiology (2018) 85(1-2):96–107. doi: 10.1159/000487440
11. Alam M, Alam S, Shamsi A, Adnan M, Elasbali AM, Al-Soud WA, et al. Bax/Bcl-2 cascade is regulated by the EGFR pathway: Therapeutic targeting of non-small cell lung cancer. Front Oncol (2022) 12:869672. doi: 10.3389/fonc.2022.869672
12. Tanaka H, Sakagami H, Kaneko N, Konagai S, Yamamoto H, Matsuya T, et al. Mutant-selective irreversible EGFR inhibitor, naquotinib, inhibits tumor growth in NSCLC models with EGFR-activating mutations, T790M mutation, and AXL overexpression. Mol Cancer Ther (2019) 18(8):1366–73. doi: 10.1158/1535-7163.MCT-18-0976
13. Fu Y, Zhang Y, Lei Z, Liu T, Cai T, Wang A, et al. Abnormally activated OPN/integrin αVβ3/FAK signalling is responsible for EGFR-TKI resistance in EGFR mutant non-small-cell lung cancer. J Hematol Oncol (2020) 13(1):169–. doi: 10.1186/s13045-020-01009-7
14. Fumarola C, Bonelli MA, Petronini PG, Alfieri RR. Targeting PI3K/AKT/mTOR pathway in non small cell lung cancer. Biochem Pharmacol (2014) 90(3):197–207. doi: 10.1016/j.bcp.2014.05.011
15. Cascetta P, Sforza V, Manzo A, Carillio G, Palumbo G, Esposito G, et al. RET inhibitors in non-Small-Cell lung cancer. Cancers (2021) 13(17):4415. doi: 10.3390/cancers13174415
16. Li Y, Shan Z, Liu C, Yang D, Wu J, Men C, et al. MicroRNA-294 promotes cellular proliferation and motility through the PI3K/AKT and JAK/STAT pathways by upregulation of NRAS in bladder cancer. Biochem Biokhim (2017) 82(4):474–82. doi: 10.1134/S0006297917040095
17. Landi L, Minuti G, D'Incecco A, Salvini J, Cappuzzo F. MET overexpression and gene amplification in NSCLC: a clinical perspective. Lung Cancer (Auckland NZ) (2013) 4:15–25. doi: 10.2147/LCTT.S35168
18. Dong Y, Xu J, Sun B, Wang J, Wang Z. MET-targeted therapies and clinical outcomes: A systematic literature review. Mol Diagn Ther (2022) 26(2):203–27. doi: 10.1007/s40291-021-00568-w
19. Fujino T, Suda K, Mitsudomi T. Lung cancer with MET exon 14 skipping mutation: Genetic feature, current treatments, and future challenges. Lung Cancer (Auckland NZ) (2021) 12:35–50. doi: 10.2147/LCTT.S269307
20. Du X, Shao Y, Qin H-F, Tai Y-H, Gao H-J. ALK-rearrangement in non-small-cell lung cancer (NSCLC). Thorac Cancer (2018) 9(4):423–30. doi: 10.1111/1759-7714.12613
21. Shen J, Meng Y, Wang K, Gao M, Du J, Wang J, et al. EML4-ALK G1202R mutation induces EMT and confers resistance to ceritinib in NSCLC cells via activation of STAT3/Slug signaling. Cell Signal (2022) 92:110264. doi: 10.1016/j.cellsig.2022.110264
22. Awad MM, Shaw AT. ALK inhibitors in non-small cell lung cancer: crizotinib and beyond. Clin Adv Hematol Oncol H&O (2014) 12(7):429–39.
23. Liu Z, Ma L, Sun Y, Yu W, Wang X. Targeting STAT3 signaling overcomes gefitinib resistance in non-small cell lung cancer. Cell Death Dis (2021) 12(6):561. doi: 10.1038/s41419-021-03844-z
24. Yu X, Ji X, Su C. HER2-altered non-small cell lung cancer: Biology, clinicopathologic features, and emerging therapies. Front Oncol (2022) 12:860313–. doi: 10.3389/fonc.2022.860313
25. Zhao J, Xia Y. Targeting HER2 alterations in non–Small-Cell lung cancer: A comprehensive review. JCO Precis Oncol (2020) 4):411–25. doi: 10.1200/PO.19.00333
26. Cardarella S, Ogino A, Nishino M, Butaney M, Shen J, Lydon C, et al. Clinical, pathologic, and biologic features associated with BRAF mutations in non-small cell lung cancer. Clin Cancer Res (2013) 19(16):4532–40. doi: 10.1158/1078-0432.CCR-13-0657
27. O'Leary CG, Andelkovic V, Ladwa R, Pavlakis N, Zhou C, Hirsch F, et al. Targeting BRAF mutations in non-small cell lung cancer. Transl Lung Cancer Res (2019) 8(6):1119–24. doi: 10.21037/tlcr.2019.10.22
28. Roskoski R Jr. ROS1 protein-tyrosine kinase inhibitors in the treatment of ROS1 fusion protein-driven non-small cell lung cancers. Pharmacol Res (2017) 121:202–12. doi: 10.1016/j.phrs.2017.04.022
29. Liu M, Dai J, Wei M, Pan Q, Zhu W. An updated patent review of small-molecule ROS1 kinase inhibitors (2015-2021). Expert Opin Ther Patents (2022) 32(6):1–17. doi: 10.1080/13543776.2022.2058872
30. Kiyozumi D, Noda T, Yamaguchi R, Tobita T, Matsumura T, Shimada K, et al. NELL2-mediated lumicrine signaling through OVCH2 is required for male fertility. Sci (New York NY). (2020) 368(6495):1132–5. doi: 10.1126/science.aay5134
31. Guaitoli G, Bertolini F, Bettelli S, Manfredini S, Maur M, Trudu L, et al. Deepening the knowledge of ROS1 rearrangements in non-small cell lung cancer: Diagnosis, treatment, resistance and concomitant alterations. Int J Mol Sci (2021) 22(23):12867. doi: 10.3390/ijms222312867
32. Nacchio M, Sgariglia R, Gristina V, Pisapia P, Pepe F, De Luca C, et al. KRAS mutations testing in non-small cell lung cancer: the role of Liquid biopsy in the basal setting. Journal of thoracic disease (2020) 12(7):3836–43. doi: 10.21037/jtd.2020.01.19
33. Hong DS, Fakih MG, Strickler JH, Desai J, Durm GA, Shapiro GI, et al. KRAS(G12C) inhibition with sotorasib in advanced solid tumors. New Engl J Med (2020) 383(13):1207–17. doi: 10.1056/NEJMoa1917239
34. Kitajima S, Thummalapalli R, Barbie DA. Inflammation as a driver and vulnerability of KRAS mediated oncogenesis. Semin Cell Dev Biol (2016) 58:127–35. doi: 10.1016/j.semcdb.2016.06.009
35. Addeo A, Banna GL, Friedlaender A. KRAS G12C mutations in NSCLC: From target to resistance. Cancers (Basel) (2021) 13(11):2541. doi: 10.3390/cancers13112541
36. Liu F, Wei Y, Zhang H, Jiang J, Zhang P, Chu Q. NTRK fusion in non-small cell lung cancer: Diagnosis, therapy, and TRK inhibitor resistance. Front Oncol (2022) 12:864666–. doi: 10.3389/fonc.2022.864666
37. Farago AF, Taylor MS, Doebele RC, Zhu VW, Kummar S, Spira AI, et al. Clinicopathologic features of non-Small-Cell lung cancer harboring an NTRK gene fusion. JCO Precis Oncol (2018) 2018:1–12. doi: 10.1200/PO.18.00037
38. Cefalì M, Epistolio S, Ramelli G, Mangan D, Molinari F, Martin V, et al. Correlation of KRAS G12C mutation and high PD-L1 expression with clinical outcome in NSCLC patients treated with anti-PD1 immunotherapy. J Clin Med (2022) 11(6):1627. doi: 10.3390/jcm11061627
39. Zhang M, Li G, Wang Y, Wang Y, Zhao S, Haihong P, et al. PD-L1 expression in lung cancer and its correlation with driver mutations: a meta-analysis. Sci Rep (2017) 7(1):10255–. doi: 10.1038/s41598-017-10925-7
40. Lan B, Ma C, Zhang C, Chai S, Wang P, Ding L, et al. Association between PD-L1 expression and driver gene status in non-small-cell lung cancer: a meta-analysis. Oncotarget (2018) 9(7):7684–99. doi: 10.18632/oncotarget.23969
41. Scheel AH, Ansén S, Schultheis AM, Scheffler M, Fischer RN, Michels S, et al. PD-L1 expression in non-small cell lung cancer: Correlations with genetic alterations. Oncoimmunology (2016) 5(5):e1131379. doi: 10.1080/2162402X.2015.1131379
42. Zhou Y, Sheng B, Xia Q, Guan X, Zhang Y. Association of long non-coding RNA H19 and microRNA-21 expression with the biological features and prognosis of non-small cell lung cancer. Cancer Gene Ther (2017) 24(8):317–24. doi: 10.1038/cgt.2017.20
43. Wei D, Sun L, Feng W. hsa_circ_0058357 acts as a ceRNA to promote non−small cell lung cancer progression via the hsa−miR−24−3p/AVL9 axis. Mol Med Rep (2021) 23(6):1–15. doi: 10.3892/mmr.2021.12109
44. Zhao L, Zhang X, Shi Y, Teng T. LncRNA SNHG14 contributes to the progression of NSCLC through miR-206/G6PD pathway. Thorac Cancer (2020) 11(5):1202–10. doi: 10.1111/1759-7714.13374
45. Xue L, Li J, Lin Y, Liu D, Yang Q, Jian J, et al. m(6) a transferase METTL3-induced lncRNA ABHD11-AS1 promotes the warburg effect of non-small-cell lung cancer. J Cell Physiol (2021) 236(4):2649–58. doi: 10.1002/jcp.30023
46. Wu C, Song W, Wang Z, Wang B. Functions of lncRNA DUXAP8 in non-small cell lung cancer. Mol Biol Rep (2022) 49(3):2531–42. doi: 10.1007/s11033-021-07066-6
47. Zhang L, Chi B, Chai J, Qin L, Zhang G, Hua P, et al. LncRNA CCDC144NL-AS1 serves as a prognosis biomarker for non-small cell lung cancer and promotes cellular function by targeting miR-490-3p. Mol Biotechnol (2021) 63(10):933–40. doi: 10.1007/s12033-021-00351-6
48. Liang H, Peng J. LncRNA HOTAIR promotes proliferation, invasion and migration in NSCLC cells via the CCL22 signaling pathway. PloS One (2022) 17(2):e0263997–e. doi: 10.1371/journal.pone.0263997
49. Zang X, Gu J, Zhang J, Shi H, Hou S, Xu X, et al. Exosome-transmitted lncRNA UFC1 promotes non-small-cell lung cancer progression by EZH2-mediated epigenetic silencing of PTEN expression. Cell Death Dis (2020) 11(4):215. doi: 10.1038/s41419-020-2409-0
50. Zhang L, Jin C, Yang G, Wang B, Hua P, Zhang Y. LncRNA WTAPP1 promotes cancer cell invasion and migration in NSCLC by downregulating lncRNA HAND2-AS1. BMC Pulmonary Med (2020) 20(1):153. doi: 10.1186/s12890-020-01180-0
51. Gao YP, Li Y, Li HJ, Zhao B. LncRNA NBR2 inhibits EMT progression by regulating Notch1 pathway in NSCLC. Eur Rev Med Pharmacol Sci (2019) 23(18):7950–8. doi: 10.26355/eurrev_201909_19011
52. Guo C, Shi H, Shang Y, Zhang Y, Cui J, Yu H. LncRNA LINC00261 overexpression suppresses the growth and metastasis of lung cancer via regulating miR-1269a/FOXO1 axis. Cancer Cell Int (2020) 20:275. doi: 10.1186/s12935-020-01332-6
53. Yang W, Qian Y, Gao K, Zheng W, Wu G, He Q, et al. LncRNA BRCAT54 inhibits the tumorigenesis of non-small cell lung cancer by binding to RPS9 to transcriptionally regulate JAK-STAT and calcium pathway genes. Carcinogenesis (2021) 42(1):80–92. doi: 10.1093/carcin/bgaa051
54. Yuan Y, Liao H, Pu Q, Ke X, Hu X, Ma Y, et al. miR-410 induces both epithelial-mesenchymal transition and radioresistance through activation of the PI3K/mTOR pathway in non-small cell lung cancer. Signal Transduct Target Ther (2020) 5(1):85. doi: 10.1038/s41392-020-0182-2
55. Liu Y, Li M, Zhang G, Pang Z. MicroRNA-10b overexpression promotes non-small cell lung cancer cell proliferation and invasion. Eur J Med Res (2013) 18(1):41. doi: 10.1186/2047-783X-18-41
56. Wang XC, Wang W, Zhang ZB, Zhao J, Tan XG, Luo JC. Overexpression of miRNA-21 promotes radiation-resistance of non-small cell lung cancer. Radiat Oncol (London England) (2013) 8:146. doi: 10.1186/1748-717X-8-146
57. Chen Y, Zhou X, Qiao J, Bao A. MiR-142-3p overexpression increases chemo-sensitivity of NSCLC by inhibiting HMGB1-mediated autophagy. Cell Physiol Biochem (2017) 41(4):1370–82. doi: 10.1159/000467896
58. Zhou X, Tao H. Overexpression of microRNA-936 suppresses non-small cell lung cancer cell proliferation and invasion via targeting E2F2. Exp Ther Med (2018) 16(3):2696–702. doi: 10.3892/etm.2018.6490
59. Ni L, Xu J, Zhao F, Dai X, Tao J, Pan J, et al. MiR-221-3p-mediated downregulation of MDM2 reverses the paclitaxel resistance of non-small cell lung cancer in vitro and in vivo. Eur J Pharmacol (2021) 899:174054. doi: 10.1016/j.ejphar.2021.174054
60. Zhu X, Tian G, Quan J, He P, Liu J. Effects of miR−340 overexpression and knockdown on the proliferation and metastasis of NSCLC cell lines. Int J Mol Med (2019) 44(2):643–51. doi: 10.3892/ijmm.2019.4213
61. Yu DJ, Li YH, Zhong M. MicroRNA-597 inhibits NSCLC progression through negatively regulating CDK2 expression. Eur Rev Med Pharmacol Sci (2020) 24(8):4288–97. doi: 10.26355/eurrev_202004_21009
62. Tang X, Liu S, Cui Y, Zhao Y. MicroRNA-4732 is downregulated in non-small cell lung cancer and inhibits tumor cell proliferation, migration, and invasion. Respir Med Res (2021) 80:100865. doi: 10.1016/j.resmer.2021.100865
63. Hemler ME. Targeting of tetraspanin proteins–potential benefits and strategies. Nat Rev Drug Discov (2008) 7(9):747–58. doi: 10.1038/nrd2659
64. Ma Y, Nenkov M, Schröder DC, Abubrig M, Gassler N, Chen Y. Fibulin 2 is hypermethylated and suppresses tumor cell proliferation through inhibition of cell adhesion and extracellular matrix genes in non-small cell lung cancer. Int J Mol Sci (2021) 22(21):11834. doi: 10.3390/ijms222111834
65. Li M, Liu P, Wang B, Zhou J, Yang J. Inhibition of nuclear factor kappa b as a therapeutic target for lung cancer. Altern Therap Health Med (2022) 28(1):44–51.
66. Suryavanshi SV, Kulkarni YA. NF-κβ: A potential target in the management of vascular complications of diabetes. Front Pharmacol (2017) 8:798. doi: 10.3389/fphar.2017.00798
67. Solis LM, Behrens C, Dong W, Suraokar M, Ozburn NC, Moran CA, et al. Nrf2 and Keap1 abnormalities in non-small cell lung carcinoma and association with clinicopathologic features. Clin Cancer Res (2010) 16(14):3743–53. doi: 10.1158/1078-0432.CCR-09-3352
68. Peng L, Tao Y, Wu R, Su J, Sun M, Cheng Y, et al. NFAT as a biomarker and therapeutic target in non-small cell lung cancer-related brain metastasis. Front Oncol (2021) 11:781150. doi: 10.3389/fonc.2021.781150
69. Zhang Y, Hu K, Qu Z, Xie Z, Tian F. ADAMTS8 inhibited lung cancer progression through suppressing VEGFA. Biochem Biophys Res Commun (2022) 598:1–8. doi: 10.1016/j.bbrc.2022.01.110
70. Yang Z, Wei B, Qiao A, Yang P, Chen W, Zhen D, et al. A novel EZH2/NXPH4/CDKN2A axis is involved in regulating the proliferation and migration of non-small cell lung cancer cells. Biosci Biotechnol Biochem (2022) 86(3):340–50. doi: 10.1093/bbb/zbab217
71. Yang Y, Li X, Wang T, Guo Q, Xi T, Zheng L. Emerging agents that target signaling pathways in cancer stem cells. J Hematol Oncol (2020) 13(1):60. doi: 10.1186/s13045-020-00901-6
72. Raniszewska A, Vroman H, Dumoulin D, Cornelissen R, Aerts J, Domagała-Kulawik J. PD-L1(+) lung cancer stem cells modify the metastatic lymph-node immunomicroenvironment in nsclc patients. Cancer Immunol Immunother CII (2021) 70(2):453–61. doi: 10.1007/s00262-020-02648-y
73. Parakh S, Ernst M, Poh AR. Multicellular effects of STAT3 in non-small cell lung cancer: Mechanistic insights and therapeutic opportunities. Cancers (Basel) (2021) 13(24):6228. doi: 10.3390/cancers13246228
74. Herreros-Pomares A, de-Maya-Girones JD, Calabuig-Fariñas S, Lucas R, Martínez A, Pardo-Sánchez JM, et al. Lung tumorspheres reveal cancer stem cell-like properties and a score with prognostic impact in resected non-small-cell lung cancer. Cell Death Dis (2019) 10(9):660. doi: 10.1038/s41419-019-1898-1
75. Phi LTH, Sari IN, Yang Y-G, Lee S-H, Jun N, Kim KS, et al. Cancer stem cells (CSCs) in drug resistance and their therapeutic implications in cancer treatment. Stem Cells Int (2018) 2018:5416923–. doi: 10.1155/2018/5416923
76. Cao S, Wang Z, Gao X, He W, Cai Y, Chen H, et al. FOXC1 induces cancer stem cell-like properties through upregulation of beta-catenin in NSCLC. J Exp Clin Cancer Res (2018) 37(1):220. doi: 10.1186/s13046-018-0894-0
77. Liu X, Wang Z, Yang Q, Hu X, Fu Q, Zhang X, et al. RNA Demethylase ALKBH5 prevents lung cancer progression by regulating EMT and stemness via regulating p53. Front Oncol (2022) 12:858694. doi: 10.3389/fonc.2022.858694
78. Hyun SY, Min HY, Lee HJ, Cho J, Boo HJ, Noh M, et al. Ninjurin1 drives lung tumor formation and progression by potentiating wnt/β-catenin signaling through Frizzled2-LRP6 assembly. J Exp Clin Cancer Res (2022) 41(1):133. doi: 10.1186/s13046-022-02323-3
79. Kuo KT, Lin CH, Wang CH, Pikatan NW, Yadav VK, Fong IH, et al. HNMT upregulation induces cancer stem cell formation and confers protection against oxidative stress through interaction with HER2 in non-Small-Cell lung cancer. Int J Mol Sci (2022) 23(3):1663. doi: 10.3390/ijms23031663
80. Yamashita N, So T, Miyata T, Yoshimatsu T, Nakano R, Oyama T, et al. Triple-negative expression (ALDH1A1-/CD133-/mutant p53-) cases in lung adenocarcinoma had a good prognosis. Sci Rep (2022) 12(1):1473. doi: 10.1038/s41598-022-05176-0
81. Lee HJ, Min HY, Yong YS, Ann J, Nguyen CT, La MT, et al. A novel c-terminal heat shock protein 90 inhibitor that overcomes STAT3-wnt-β-catenin signaling-mediated drug resistance and adverse effects. Theranostics (2022) 12(1):105–25. doi: 10.7150/thno.63788
82. Rong G, Pan Z, Ding M, Wang L. RNF168 suppresses the cancer stem cell-like traits of nonsmall cell lung cancer cells by mediating RhoC ubiquitination. Environ Toxicol (2022) 37(3):603–11. doi: 10.1002/tox.23428
83. Chen M, Sun LX, Yu L, Liu J, Sun LC, Yang ZH, et al. MYH9 is crucial for stem cell-like properties in non-small cell lung cancer by activating mTOR signaling. Cell Death Discov (2021) 7(1):282. doi: 10.1038/s41420-021-00681-z
84. Daya HA, Kouba S, Ouled-Haddou H, Benzerdjeb N, Telliez MS, Dayen C, et al. Orai3-mediates cisplatin-resistance in non-small cell lung cancer cells by enriching cancer stem cell population through PI3K/AKT pathway. Cancers (Basel) (2021) 13(10):2314. doi: 10.3390/cancers13102314
85. Ettinger DS, Wood DE, Aisner DL, Akerley W, Bauman JR, Bharat A, et al. NCCN guidelines insights: Non-small cell lung cancer, version 2.2021. J Natl Compr Cancer Netw JNCCN (2021) 19(3):254–66. doi: 10.6004/jnccn.2021.0013
86. Aberle DR, Adams AM, Berg CD, Black WC, Clapp JD, Fagerstrom RM, et al. Reduced lung-cancer mortality with low-dose computed tomographic screening. New Engl J Med (2011) 365(5):395–409. doi: 10.1056/NEJMoa1102873
87. Heitzman ER. The role of computed tomography in the diagnosis and management of lung cancer. Overview Chest (1986) 89(4 Suppl):237s–41s. doi: 10.1378/chest.89.4.237S
88. Xiao YD, Lv FJ, Li WJ, Fu BJ, Lin RY, Chu ZG. Solitary pulmonary inflammatory nodule: CT features and pathological findings. J Inflammation Res (2021) 14:2741–51. doi: 10.2147/JIR.S304431
89. Treglia G, Sadeghi R, Annunziata S, Caldarella C, Bertagna F, Giovanella L. Diagnostic performance of fluorine-18-fluorodeoxyglucose positron emission tomography in the postchemotherapy management of patients with seminoma: systematic review and meta-analysis. BioMed Res Int (2014) 2014:852681. doi: 10.1155/2014/852681
90. Hicks RJ, Lau E, Alam NZ, Chen RY. Imaging in the diagnosis and treatment of non-small cell lung cancer. Respirol (Carlton Vic). (2007) 12(2):165–72. doi: 10.1111/j.1440-1843.2006.01012.x
91. Volpi S, Ali JM, Tasker A, Peryt A, Aresu G, Coonar AS. The role of positron emission tomography in the diagnosis, staging and response assessment of non-small cell lung cancer. Ann Trans Med (2018) 6(5):95. doi: 10.21037/atm.2018.01.25
92. Wang Y-XJ, Lo GG, Yuan J, Larson PEZ, Zhang X. Magnetic resonance imaging for lung cancer screen. J Thorac Dis (2014) 6(9):1340–8. doi: 10.3978/j.issn.2072-1439.2014.08.43
93. Duma N, Santana-Davila R, Molina JR. Non-small cell lung cancer: Epidemiology, screening, diagnosis, and treatment. Mayo Clinic Proc (2019) 94(8):1623–40. doi: 10.1016/j.mayocp.2019.01.013
94. Liam CK, Mallawathantri S, Fong KM. Is tissue still the issue in detecting molecular alterations in lung cancer? Respirol (Carlton Vic) (2020) 25(9):933–43. doi: 10.1111/resp.13823
95. Esagian SM, Grigoriadou G, Nikas IP, Boikou V, Sadow PM, Won JK, et al. Comparison of liquid-based to tissue-based biopsy analysis by targeted next generation sequencing in advanced non-small cell lung cancer: a comprehensive systematic review. J Cancer Res Clin Oncol (2020) 146(8):2051–66. doi: 10.1007/s00432-020-03267-x
96. Trombetta D, Sparaneo A, Fabrizio FP, Muscarella LA. Liquid biopsy and NSCLC. Lung Cancer manage (2016) 5(2):91–104. doi: 10.2217/lmt-2016-0006
97. Li W, Liu JB, Hou LK, Yu F, Zhang J, Wu W, et al. Liquid biopsy in lung cancer: significance in diagnostics, prediction, and treatment monitoring. Mol Cancer (2022) 21(1):25. doi: 10.1186/s12943-021-01462-z
98. Tang JH, Chia D. Liquid biopsies in the screening of oncogenic mutations in NSCLC and its application in targeted therapy. Crit Rev oncogenesis (2015) 20(5-6):357–71. doi: 10.1615/CritRevOncog.v20.i5-6.90
99. Matsubara T, Nakajima E, Namikawa H, Ono S, Takada I, Ohira T, et al. Investigation of EGFR mutations in non-small cell lung cancer usually undetectable by PCR methods. Mol Clin Oncol (2022) 16(1):15. doi: 10.3892/mco.2021.2447
100. Hieggelke L, Schultheis AM. [Application of FISH in the diagnosis of lung cancer]. Der Pathol (2020) 41(6):582–8. doi: 10.1007/s00292-020-00831-7
101. Hung YP, Sholl LM. Diagnostic and predictive immunohistochemistry for non-small cell lung carcinomas. Adv Anat Pathol (2018) 25(6):374–86. doi: 10.1097/PAP.0000000000000206
102. Ibrahim M, Parry S, Wilkinson D, Bilbe N, Allen D, Forrest S, et al. ALK immunohistochemistry in NSCLC: Discordant staining can impact patient treatment regimen. J Thorac Oncol (2016) 11(12):2241–7. doi: 10.1016/j.jtho.2016.07.012
103. Cainap C, Balacescu O, Cainap SS, Pop LA. Next generation sequencing technology in lung cancer diagnosis. Biology (2021) 10(9):864. doi: 10.3390/biology10090864
104. Ramalingam S, Belani C. Systemic chemotherapy for advanced non-small cell lung cancer: recent advances and future directions. Oncologist (2008) 13 Suppl 1:5–13. doi: 10.1634/theoncologist.13-S1-5
105. Dasari S, Tchounwou PB. Cisplatin in cancer therapy: molecular mechanisms of action. Eur J Pharmacol (2014) 740:364–78. doi: 10.1016/j.ejphar.2014.07.025
106. Mini E, Nobili S, Caciagli B, Landini I, Mazzei T. Cellular pharmacology of gemcitabine. Ann Oncol (2006) 17 Suppl 5:v7–12. doi: 10.1093/annonc/mdj941
107. Tan N, Malek M, Zha J, Yue P, Kassees R, Berry L, et al. Navitoclax enhances the efficacy of taxanes in non-small cell lung cancer models. Clin Cancer Res (2011) 17(6):1394–404. doi: 10.1158/1078-0432.CCR-10-2353
108. Zhao J, Kim JE, Reed E, Li QQ. Molecular mechanism of antitumor activity of taxanes in lung cancer (Review). Int J Oncol (2005) 27(1):247–56. doi: 10.3892/ijo.27.1.247
109. Dubey S, Schiller JH. Three emerging new drugs for NSCLC: pemetrexed, bortezomib, and cetuximab. Oncologist (2005) 10(4):282–91. doi: 10.1634/theoncologist.10-4-282
110. Kim ES. Chemotherapy resistance in lung cancer. Adv Exp Med Biol (2016) 893:189–209. doi: 10.1007/978-3-319-24223-1_10
111. Heng WS, Gosens R, Kruyt FAE. Lung cancer stem cells: origin, features, maintenance mechanisms and therapeutic targeting. Biochem Pharmacol (2019) 160:121–33. doi: 10.1016/j.bcp.2018.12.010
112. Lv M, Zhuang X, Zhang Q, Cheng Y, Wu D, Wang X, et al. Acetyl-11-keto-β-boswellic acid enhances the cisplatin sensitivity of non-small cell lung cancer cells through cell cycle arrest, apoptosis induction, and autophagy suppression via p21-dependent signaling pathway. Cell Biol Toxicol (2021) 37(2):209–28. doi: 10.1007/s10565-020-09541-5
113. McElnay P, Lim E. Adjuvant or neoadjuvant chemotherapy for NSCLC. J Thorac Dis (2014) 6 Suppl 2(Suppl 2):S224–7. doi: 10.3978/j.issn.2072-1439.2014.04.26
114. Watanabe SI, Nakagawa K, Suzuki K, Takamochi K, Ito H, Okami J, et al. Neoadjuvant and adjuvant therapy for stage III non-small cell lung cancer. Jpn J Clin Oncol (2017) 47(12):1112–8. doi: 10.1093/jjco/hyx147
115. Pezzetta E, Stupp R, Zouhair A, Guillou L, Taffé P, von Briel C, et al. Comparison of neoadjuvant cisplatin-based chemotherapy versus radiochemotherapy followed by resection for stage III (N2) NSCLC. Eur J Cardio-thoracic Surg (2005) 27(6):1092–8. doi: 10.1016/j.ejcts.2005.02.035
116. Vinod SK, Hau E. Radiotherapy treatment for lung cancer: Current status and future directions. Respirol (Carlton Vic). (2020) 25 Suppl 2:61–71. doi: 10.1111/resp.13870
117. Rallis KS, Lai Yau TH, Sideris M. Chemoradiotherapy in cancer treatment: Rationale and clinical applications. Anticancer Res (2021) 41(1):1–7. doi: 10.21873/anticanres.14746
118. Miyasaka Y, Sato H, Okano N, Kubo N, Kawamura H, Ohno T. A promising treatment strategy for lung cancer: A combination of radiotherapy and immunotherapy. Cancers (Basel) (2021) 14(1):203. doi: 10.3390/cancers14010203
119. Antonia SJ, Villegas A, Daniel D, Vicente D, Murakami S, Hui R, et al. Overall survival with durvalumab after chemoradiotherapy in stage III NSCLC. New Engl J Med (2018) 379(24):2342–50. doi: 10.1056/NEJMoa1809697
120. Faivre-Finn C, Snee M, Ashcroft L, Appel W, Barlesi F, Bhatnagar A, et al. Concurrent once-daily versus twice-daily chemoradiotherapy in patients with limited-stage small-cell lung cancer (CONVERT): an open-label, phase 3, randomised, superiority trial. Lancet Oncol (2017) 18(8):1116–25. doi: 10.1016/S1470-2045(17)30318-2
121. Peters S, Pujol JL, Dafni U, Dómine M, Popat S, Reck M, et al. Consolidation nivolumab and ipilimumab versus observation in limited-disease small-cell lung cancer after chemo-radiotherapy - results from the randomised phase II ETOP/IFCT 4-12 STIMULI trial. Ann Oncol (2022) 33(1):67–79. doi: 10.1016/j.annonc.2021.09.011
122. Tan AC, Tan DSW. Targeted therapies for lung cancer patients with oncogenic driver molecular alterations. J Clin Oncol (2022) 40(6):611–25. doi: 10.1200/JCO.21.01626
123. Cheng Y, Zhang T, Xu Q. Therapeutic advances in non-small cell lung cancer: Focus on clinical development of targeted therapy and immunotherapy. MedComm (2020) (2021) 2(4):692–729. doi: 10.1002/mco2.105
124. Garcia-Robledo JE, Rosell R, Ruíz-Patiño A, Sotelo C, Arrieta O, Zatarain-Barrón L, et al. KRAS and MET in non-small-cell lung cancer: two of the new kids on the 'drivers' block. Ther Adv Respir Dis (2022) 16:17534666211066064–. doi: 10.1177/17534666211066064
125. Chevallier M, Borgeaud M, Addeo A, Friedlaender A. Oncogenic driver mutations in non-small cell lung cancer: Past, present and future. World J Clin Oncol (2021) 12(4):217–37. doi: 10.5306/wjco.v12.i4.217
126. Klug LR, Khosroyani HM, Kent JD, Heinrich MC. New treatment strategies for advanced-stage gastrointestinal stromal tumours. Nat Rev Clin Oncol (2022) 19(5):328–41. doi: 10.1038/s41571-022-00606-4
127. Melosky B, Wheatley-Price P, Juergens RA, Sacher A, Leighl NB, Tsao MS, et al. The rapidly evolving landscape of novel targeted therapies in advanced non-small cell lung cancer. Lung Cancer (Amsterdam Netherlands) (2021) 160:136–51. doi: 10.1016/j.lungcan.2021.06.002
128. Nadler E, Vasudevan A, Wang Y, Ogale S. Real-world patterns of biomarker testing and targeted therapy in de novo metastatic non-small cell lung cancer patients in the US oncology network. Cancer Treat Res Commun (2022) 31:100522. doi: 10.1016/j.ctarc.2022.100522
129. Boolell V, Alamgeer M, Watkins DN, Ganju V. The evolution of therapies in non-small cell lung cancer. Cancers (2015) 7(3):1815–46. doi: 10.3390/cancers7030864
130. Li BT, Smit EF, Goto Y, Nakagawa K, Udagawa H, Mazières J, et al. Trastuzumab deruxtecan in HER2-mutant non-Small-Cell lung cancer. New Engl J Med (2022) 386(3):241–51. doi: 10.1056/NEJMoa2112431
131. Maemondo M, Inoue A, Kobayashi K, Sugawara S, Oizumi S, Isobe H, et al. Gefitinib or chemotherapy for non-small-cell lung cancer with mutated EGFR. New Engl J Med (2010) 362(25):2380–8. doi: 10.1056/NEJMoa0909530
132. Masuda C, Yanagisawa M, Yorozu K, Kurasawa M, Furugaki K, Ishikura N, et al. Bevacizumab counteracts VEGF-dependent resistance to erlotinib in an EGFR-mutated NSCLC xenograft model. Int J Oncol (2017) 51(2):425–34. doi: 10.3892/ijo.2017.4036
133. Nagano T, Tachihara M, Nishimura Y. Dacomitinib, a second-generation irreversible epidermal growth factor receptor tyrosine kinase inhibitor (EGFR-TKI) to treat non-small cell lung cancer. Drugs Today (Barcelona Spain 1998) (2019) 55(4):231–6. doi: 10.1358/dot.2019.55.4.2965337
134. Stirrups R. Osimertinib improves progression-free survival in NSCLC. Lancet Oncol (2018) 19(1):e10. doi: 10.1016/S1470-2045(17)30893-8
135. Gainor JF, Tan DS, De Pas T, Solomon BJ, Ahmad A, Lazzari C, et al. Progression-free and overall survival in ALK-positive NSCLC patients treated with sequential crizotinib and ceritinib. Clin Cancer Res (2015) 21(12):2745–52. doi: 10.1158/1078-0432.CCR-14-3009
136. Ma X, Yang S, Zhang K, Xu J, Lv P, Gao H, et al. Efficacy of different sequential patterns after crizotinib progression in advanced anaplastic lymphoma kinase-positive non-small cell lung cancer. Thorac Cancer (2022) 13(12):1788–94. doi: 10.1111/1759-7714.14455
137. Mok T, Camidge DR, Gadgeel SM, Rosell R, Dziadziuszko R, Kim DW, et al. Updated overall survival and final progression-free survival data for patients with treatment-naive advanced ALK-positive non-small-cell lung cancer in the ALEX study. Ann Oncol (2020) 31(8):1056–64. doi: 10.1016/j.annonc.2020.04.478
138. Camidge DR, Kim HR, Ahn MJ, Yang JCH, Han JY, Hochmair MJ, et al. Brigatinib versus crizotinib in advanced ALK inhibitor-naive ALK-positive non-small cell lung cancer: Second interim analysis of the phase III ALTA-1L trial. J Clin Oncol (2020) 38(31):3592–603. doi: 10.1200/JCO.20.00505
139. Gainor JF, Curigliano G, Kim DW, Lee DH, Besse B, Baik CS, et al. Pralsetinib for RET fusion-positive non-small-cell lung cancer (ARROW): a multi-cohort, open-label, phase 1/2 study. Lancet Oncol (2021) 22(7):959–69. doi: 10.1016/S1470-2045(21)00247-3
140. Villalobos P, Wistuba II. Lung cancer biomarkers. Hematol Oncol Clinics North Am (2017) 31(1):13–29. doi: 10.1016/j.hoc.2016.08.006
141. Giatromanolaki A. Prognostic role of angiogenesis in non-small cell lung cancer. Anticancer Res (2001) 21(6b):4373–82.
142. Raphael J, Chan K, Karim S, Kerbel R, Lam H, Santos KD, et al. Antiangiogenic therapy in advanced non-small-cell lung cancer: A meta-analysis of phase III randomized trials. Clin Lung Cancer (2017) 18(4):345–53.e5. doi: 10.1016/j.cllc.2017.01.004
143. Aguilar A, Mas L, Enríquez D, Vallejos C, Gutarra R, Flores CJ. Impact of targeted therapy on the survival of patients with advanced-stage non-small cell lung cancer in oncosalud - AUNA. Cancer Control (2022) 29:10732748211068637. doi: 10.1177/10732748211068637
144. Kim HS, Oh HN, Kwak AW, Kim E, Lee MH, Seo JH, et al. Deoxypodophyllotoxin inhibits cell growth and induces apoptosis by blocking EGFR and MET in gefitinib-resistant non-small cell lung cancer. J Microbiol Biotechnol (2021) 31(4):559–69. doi: 10.4014/jmb.2101.01029
145. Zhou Q, Xu C-R, Cheng Y, Liu Y-P, Chen G-Y, Cui J-W, et al. Bevacizumab plus erlotinib in Chinese patients with untreated, EGFR-mutated, advanced NSCLC (ARTEMIS-CTONG1509): A multicenter phase 3 study. Cancer Cell (2021) 39(9):1279–91.e3. doi: 10.1016/j.ccell.2021.07.005
146. Zhao H, Yao W, Min X, Gu K, Yu G, Zhang Z, et al. Apatinib plus gefitinib as first-line treatment in advanced EGFR-mutant NSCLC: The phase III ACTIVE study (CTONG1706). J Thorac Oncol (2021) 16(9):1533–46. doi: 10.1016/j.jtho.2021.05.006
147. Poels KE, Schoenfeld AJ, Makhnin A, Tobi Y, Wang Y, Frisco-Cabanos H, et al. Identification of optimal dosing schedules of dacomitinib and osimertinib for a phase I/II trial in advanced EGFR-mutant non-small cell lung cancer. Nat Commun (2021) 12(1):3697–. doi: 10.1038/s41467-021-23912-4
148. Bertino EM, Gentzler RD, Clifford S, Kolesar J, Muzikansky A, Haura EB, et al. Phase IB study of osimertinib in combination with navitoclax in EGFR-mutant NSCLC following resistance to initial EGFR therapy (ETCTN 9903). Clin Cancer Res (2021) 27(6):1604–11. doi: 10.1158/1078-0432.CCR-20-4084
149. Xie M, Xu X, Fan Y. KRAS-mutant non-small cell lung cancer: An emerging promisingly treatable subgroup. Front Oncol (2021) 11:672612–. doi: 10.3389/fonc.2021.672612
150. Palma G, Khurshid F, Lu K, Woodward B, Husain H. Selective KRAS G12C inhibitors in non-small cell lung cancer: chemistry, concurrent pathway alterations, and clinical outcomes. NPJ Precis Oncol (2021) 5(1):98. doi: 10.1038/s41698-021-00237-5
151. Froesch P, Mark M, Rothschild SI, Li Q, Godar G, Rusterholz C, et al. Binimetinib, pemetrexed and cisplatin, followed by maintenance of binimetinib and pemetrexed in patients with advanced non-small cell lung cancer (NSCLC) and KRAS mutations. the phase 1B SAKK 19/16 trial. Lung Cancer (Amsterdam Netherlands) (2021) 156:91–9. doi: 10.1016/j.lungcan.2021.04.002
152. Soo RA, Han JY, Dafni U, Cho BC, Yeo CM, Nadal E, et al. A randomised phase II study of osimertinib and bevacizumab versus osimertinib alone as second-line targeted treatment in advanced NSCLC with confirmed EGFR and acquired T790M mutations: the European thoracic oncology platform (ETOP 10-16) BOOSTER trial. Ann Oncol (2022) 33(2):181–92. doi: 10.1016/j.annonc.2021.11.010
153. Chen W, Shen L, Jiang J, Zhang L, Zhang Z, Pan J, et al. Antiangiogenic therapy reverses the immunosuppressive breast cancer microenvironment. biomark Res (2021) 9(1):59. doi: 10.1186/s40364-021-00312-w
154. Jayson GC, Kerbel R, Ellis LM, Harris AL. Antiangiogenic therapy in oncology: current status and future directions. Lancet (2016) 388(10043):518–29. doi: 10.1016/S0140-6736(15)01088-0
155. Ohm JE, Carbone DP. VEGF as a mediator of tumor-associated immunodeficiency. Immunol Res (2001) 23(2-3):263–72. doi: 10.1385/IR:23:2-3:263
156. Imai K, Takaoka A. Comparing antibody and small-molecule therapies for cancer. Nat Rev Cancer (2006) 6(9):714–27. doi: 10.1038/nrc1913
157. Reck M, von Pawel J, Zatloukal P, Ramlau R, Gorbounova V, Hirsh V, et al. Phase III trial of cisplatin plus gemcitabine with either placebo or bevacizumab as first-line therapy for nonsquamous non-small-cell lung cancer: AVAil. J Clin Oncol (2009) 27(8):1227–34. doi: 10.1200/JCO.2007.14.5466
158. Seto T, Nosaki K, Shimokawa M, Toyozawa R, Sugawara S, Hayashi H, et al. Phase II study of atezolizumab with bevacizumab for non-squamous non-small cell lung cancer with high PD-L1 expression (@Be study). J Immunother Cancer (2022) 10(2):1–9. doi: 10.1136/jitc-2021-004025
159. West HJ, McCleland M, Cappuzzo F, Reck M, Mok TS, Jotte RM, et al. Clinical efficacy of atezolizumab plus bevacizumab and chemotherapy in KRAS-mutated non-small cell lung cancer with STK11, KEAP1, or TP53 comutations: subgroup results from the phase III IMpower150 trial. J Immunother Cancer (2022) 10(2):1–12. doi: 10.1136/jitc-2021-003027
160. Shi Y, Lei K, Jia Y, Ni B, He Z, Bi M, et al. Bevacizumab biosimilar LY01008 compared with bevacizumab (Avastin) as first-line treatment for Chinese patients with unresectable, metastatic, or recurrent non-squamous non-small-cell lung cancer: A multicenter, randomized, double-blinded, phase III trial. Cancer Commun (London England) (2021) 41(9):889–903. doi: 10.1002/cac2.12179
161. Syrigos K, Abert I, Andric Z, Bondarenko IN, Dvorkin M, Galic K, et al. Efficacy and safety of bevacizumab biosimilar FKB238 versus originator bevacizumab: Results from AVANA, a phase III trial in patients with non-squamous non-Small-Cell lung cancer (non-sq-NSCLC). BioDrugs (2021) 35(4):417–28. doi: 10.1007/s40259-021-00489-4
162. Shibuya M. Vascular endothelial growth factor (VEGF) and its receptor (VEGFR) signaling in angiogenesis: A crucial target for anti- and pro-angiogenic therapies. Genes Cancer (2011) 2(12):1097–105. doi: 10.1177/1947601911423031
163. Ferrara N, Kerbel RS. Angiogenesis as a therapeutic target. Nature (2005) 438(7070):967–74. doi: 10.1038/nature04483
164. Zhou N, Jiang M, Li T, Zhu J, Liu K, Hou H, et al. Anlotinib combined with anti-PD-1 antibody, camrelizumab for advanced NSCLCs after multiple lines treatment: An open-label, dose escalation and expansion study. Lung Cancer (Amsterdam Netherlands) (2021) 160:111–7. doi: 10.1016/j.lungcan.2021.08.006
165. Mielgo-Rubio X, Uribelarrea EA, Cortés LQ, Moyano MS. Immunotherapy in non-small cell lung cancer: Update and new insights. J Clin Trans Res (2021) 7(1):1–21.
166. Roller JF, Veeramachaneni NK, Zhang J. Exploring the evolving scope of neoadjuvant immunotherapy in NSCLC. Cancers (Basel) (2022) 14(3):741. doi: 10.3390/cancers14030741
167. Chen Y, Zhou Y, Tang L, Peng X, Jiang H, Wang G, et al. Immune-checkpoint inhibitors as the first line treatment of advanced non-small cell lung cancer: A meta-analysis of randomized controlled trials. J Cancer (2019) 10(25):6261–8. doi: 10.7150/jca.34677
168. Nasser NJ, Gorenberg M, Agbarya A. First line immunotherapy for non-small cell lung cancer. Pharm (Basel) (2020) 13(11):373. doi: 10.3390/ph13110373
169. Wang J, Chmielowski B, Pellissier J, Xu R, Stevinson K, Liu FX. Cost-effectiveness of pembrolizumab versus ipilimumab in ipilimumab-naïve patients with advanced melanoma in the united states. J Manag Care Specialty pharmacy (2017) 23(2):184–94. doi: 10.18553/jmcp.2017.23.2.184
170. Santini FC, Rudin CM. Atezolizumab for the treatment of non-small cell lung cancer. Expert Rev Clin Pharmacol (2017) 10(9):935–45. doi: 10.1080/17512433.2017.1356717
171. Lim SH, Sun JM, Lee SH, Ahn JS, Park K, Ahn MJ. Pembrolizumab for the treatment of non-small cell lung cancer. Expert Opin Biol Ther (2016) 16(3):397–406. doi: 10.1517/14712598.2016.1145652
172. Peters S, Felip E, Dafni U, Tufman A, Guckenberger M, Álvarez R, et al. Progression-free and overall survival for concurrent nivolumab with standard concurrent chemoradiotherapy in locally advanced stage IIIA-b NSCLC: Results from the European thoracic oncology platform NICOLAS phase II trial (European thoracic oncology platform 6-14). J Thorac Oncol (2021) 16(2):278–88. doi: 10.1016/j.jtho.2020.10.129
173. Zhou C, Wang Z, Sun Y, Cao L, Ma Z, Wu R, et al. Sugemalimab versus placebo, in combination with platinum-based chemotherapy, as first-line treatment of metastatic non-small-cell lung cancer (GEMSTONE-302): interim and final analyses of a double-blind, randomised, phase 3 clinical trial. Lancet Oncol (2022) 23(2):220–33. doi: 10.1016/S1470-2045(21)00650-1
174. Rosell R, Cao P. Promising outlook with sugemalimab in non-small-cell lung cancer. Lancet Oncol (2022) 23(2):186–8. doi: 10.1016/S1470-2045(21)00698-7
175. Zhao ZR, Yang CP, Chen S, Yu H, Lin YB, Lin YB, et al. Phase 2 trial of neoadjuvant toripalimab with chemotherapy for resectable stage III non-small-cell lung cancer. Oncoimmunology (2021) 10(1):1996000. doi: 10.1080/2162402X.2021.1996000
176. Theelen W, Chen D, Verma V, Hobbs BP, Peulen HMU, Aerts J, et al. Pembrolizumab with or without radiotherapy for metastatic non-small-cell lung cancer: a pooled analysis of two randomised trials. Lancet Respir Med (2021) 9(5):467–75. doi: 10.1016/S2213-2600(20)30391-X
177. Paz-Ares LG, Ramalingam SS, Ciuleanu TE, Lee JS, Urban L, Caro RB, et al. First-line nivolumab plus ipilimumab in advanced NSCLC: 4-year outcomes from the randomized, open-label, phase 3 CheckMate 227 part 1 trial. J Thorac Oncol (2022) 17(2):289–308. doi: 10.1016/j.jtho.2021.09.010
178. Owonikoko TK, Park K, Govindan R, Ready N, Reck M, Peters S, et al. Nivolumab and ipilimumab as maintenance therapy in extensive-disease small-cell lung cancer: CheckMate 451. J Clin Oncol (2021) 39(12):1349–59. doi: 10.1200/JCO.20.02212
179. Gettinger SN, Redman MW, Bazhenova L, Hirsch FR, Mack PC, Schwartz LH, et al. Nivolumab plus ipilimumab vs nivolumab for previously treated patients with stage IV squamous cell lung cancer: The lung-MAP S1400I phase 3 randomized clinical trial. JAMA Oncol (2021) 7(9):1368–77. doi: 10.1001/jamaoncol.2021.2209
180. Reck M, Ciuleanu TE, Cobo M, Schenker M, Zurawski B, Menezes J, et al. First-line nivolumab plus ipilimumab with two cycles of chemotherapy versus chemotherapy alone (four cycles) in advanced non-small-cell lung cancer: CheckMate 9LA 2-year update. ESMO Open (2021) 6(5):100273. doi: 10.1016/j.esmoop.2021.100273
181. Leighl NB, Redman MW, Rizvi N, Hirsch FR, Mack PC, Schwartz LH, et al. Phase II study of durvalumab plus tremelimumab as therapy for patients with previously treated anti-PD-1/PD-L1 resistant stage IV squamous cell lung cancer (Lung-MAP substudy S1400F, NCT03373760). J Immunother Cancer (2021) 9(8):1–9. doi: 10.1136/jitc-2021-002973
182. Leighl NB, Laurie SA, Goss GD, Hughes BGM, Stockler M, Tsao MS, et al. CCTG BR34: A randomized phase 2 trial of durvalumab and tremelimumab with or without platinum-based chemotherapy in patients with metastatic NSCLC. J Thorac Oncol (2022) 17(3):434–45. doi: 10.1016/j.jtho.2021.10.023
183. Ren S, Chen J, Xu X, Jiang T, Cheng Y, Chen G, et al. Camrelizumab plus carboplatin and paclitaxel as first-line treatment for advanced squamous NSCLC (CameL-sq): A phase 3 trial. J Thorac Oncol (2021) 17(4):544–57. doi: 10.1016/j.jtho.2021.11.018
184. Zhou C, Chen G, Huang Y, Zhou J, Lin L, Feng J, et al. Camrelizumab plus carboplatin and pemetrexed versus chemotherapy alone in chemotherapy-naive patients with advanced non-squamous non-small-cell lung cancer (CameL): a randomised, open-label, multicentre, phase 3 trial. Lancet Respir Med (2021) 9(3):305–14. doi: 10.1016/S2213-2600(20)30365-9
185. Shafique MR, Fisher TL, Evans EE, Leonard JE, Pastore DRE, Mallow CL, et al. A phase Ib/II study of pepinemab in combination with avelumab in advanced non-small cell lung cancer. Clin Cancer Res (2021) 27(13):3630–40. doi: 10.1158/1078-0432.CCR-20-4792
186. Malkki H. Bevacizumab prolongs progression-free survival but not overall survival in newly diagnosed glioblastoma. Nat Rev Neurol (2014) 10(4):179–. doi: 10.1038/nrneurol.2014.47
Keywords: NSCLC, diagnosis, chemotherapy, chemoradiotherapy, targeted therapy, antiangiogenic therapy, immunotherapy
Citation: Guo Q, Liu L, Chen Z, Fan Y, Zhou Y, Yuan Z and Zhang W (2022) Current treatments for non-small cell lung cancer. Front. Oncol. 12:945102. doi: 10.3389/fonc.2022.945102
Received: 16 May 2022; Accepted: 06 July 2022;
Published: 11 August 2022.
Edited by:
Muhammad Abbas, Riphah International University, PakistanReviewed by:
Dayong Zheng, North China University of Science and Technology, ChinaXiaohui Wei, Anhui Medical University, China
Copyright © 2022 Guo, Liu, Chen, Fan, Zhou, Yuan and Zhang. This is an open-access article distributed under the terms of the Creative Commons Attribution License (CC BY). The use, distribution or reproduction in other forums is permitted, provided the original author(s) and the copyright owner(s) are credited and that the original publication in this journal is cited, in accordance with accepted academic practice. No use, distribution or reproduction is permitted which does not comply with these terms.
*Correspondence: Wenzhou Zhang, aG56enp3enhAc2luYS5jb20=; Ziqiao Yuan, ZmlnYXJveXpxQDE2My5jb20=