- 1Shanxi Province Cancer Hospital/Shanxi Hospital Affiliated to Cancer Hospital, Chinese Academy of Medical Sciences/Cancer Hospital Affiliated to Shanxi Medical University, Taiyuan, China
- 2Department of Pharmacology, School of Medicine, Southern University of Science and Technology, Shenzhen, China
Breast cancer (BC) remains the most prevalent malignancy due to its incidence rate, recurrence, and metastasis in women. Conventional strategies of cancer detection– mammography and tissue biopsy lack the capacity to detect the complete cancer genomic landscape. Besides, they often give false- positive or negative results. The presence of this and other disadvantages such as invasiveness, high-cost, and side effects necessitates developing new strategies to overcome the BC burden. Liquid biopsy (LB) has been brought to the fore owing to its early detection, screening, prognosis, simplicity of the technique, and efficient monitoring. Remarkably, microRNAs (miRNAs)– gene expression regulators seem to play a major role as biomarkers detected in the samples of LB. Particularly, miR-21 and miR-155 among other possible candidates seem to serve as favorable biomarkers in the diagnosis and prognosis of BC. Hence, this review will assess the potential utility of miRNAs as biomarkers and will highlight certain promising candidates for the LB approach in the diagnosis and management of BC that may optimize the patient outcome.
Introduction
Breast cancer (BC) is the most malignant and emergent tumor among women cancer patients (1, 2). Millions of women are diagnosed with BC and over half a million deaths are reported annually (3, 4). There are four main molecular subtypes of BC– luminal A, luminal B, human epidermal growth factor receptor 2 (Her2)-enriched, and basal-like (5). The first two subtypes usually have a more favorable prognosis. The most common subtype- luminal A is characterized by the expression of estrogen and progesterone receptors (ER/PR) while the luminal B subtype is additionally characterized by the absence of Her2. In the Her2-positive subtype, the Her2 gene is highly expressed and the cell proliferation rate is also high. Basal-like subtype does not express any of the mentioned markers and accounts for up to 20%. Additionally, there is one more subtype– normal-like subtype which represents the rarest BC and accounts for only up to 10% of all BCs. The normal-like subtype is characterized by the expression of ER, PR, and Her2 and clinically it is between basal-like and luminal A subtypes (6). The early diagnosis and advancements in treatment are the main focus of BC research. Mammography and tissue biopsy remain the standard screening methods until now (7). However, a number of disadvantages exist. E.g., the false-positive result of mammography requires additional analysis that may lead to potential side effects (8) while the eventual result may be negative. Except for the mentioned, mammography is related to exposure to ionizing radiation (9). Mammography imaging often is not sufficient for evaluation and requires further analysis. It is often performed along with tissue biopsy. This requires the imaging to be done before and after the biopsy to ensure the accuracy of sampling and biopsy marker placement. This technique is advantageous for its specificity to the suspicious tissue which is detected on a mammogram. Nonetheless, the disadvantages include a painful, long time of the procedure, invasiveness, and high-cost (10). Moreover, it is not adequately comprehensive to obtain the complete landscape of BC (11). Fortunately, a relatively new approach liquid biopsy (LB) that is a non-invasive and simple technique compared with surgical biopsies enables obtaining the important information of tumor via simple body fluids-based samples, mainly blood. The other advantages comprise the short time of the procedure, precise and real-time results, serial sampling, and monitoring. This enhances its application for the early diagnosis that enables better management of BC, better outcome, and, most importantly, less mortality (11). Indeed, the application of LBs has revolutionized the existing standard clinical approach and may play a critical role in diagnosing and monitoring the tumor as well as the response to the treatment. LB next-generation sequencing (NGS)-based FoundationOne Liquid CDx test was approved by Food and Drug Administration (FDA) in 2020 for application for diagnosis via detecting multiple tumor biomarkers in plasma (12). LB is undoubtedly considered one of the most perspective detection approaches for many cancers including BC. Indeed, LB allows the detection of circulating tumor components such as cancer cells, RNA, or circulating tumor DNA (ctDNA) in liquid specimens (13). Despite their low concentrations in the liquid samples, they still can be used as indicators and biomarkers of cancer. This characteristic makes LB a sensitive, advanced, alternative, reliable, and cost-effective approach for the diagnosis and screening of BC (14). Remarkably microRNAs (miRNAs) detected in LB specimens seem to be promising measurable indicators of BC. The change in their levels reflects the various conditions of the body. miRNAs are small non-coding RNAs that serve as gene-regulator molecules in the body. As miRNAs are implicated in various signaling pathways, alteration of their levels indicates certain conditions, e.g., various cancers (11, 15–17). Indeed, proof-of-concept studies demonstrated that the composition of miRNAs and their abundance in the blood are altered in cancer patients (11, 18, 19). Additionally, Li et al. have studied miRNAs in BC patients and demonstrated that 13 miRNAs were found to be differentially expressed in patients with metastatic BC (20). Kim et al. successfully monitored the expression levels of miR-21 and miR-155 in the urinary samples of mice injected with BC cells (21). Hence, in this review, we summarize recent and relevant data on circulating miRNAs (c-miRNAs) for their potential role in the diagnostics of BC to become a part of the LB approach in clinics. A schematic illustration of the potential utility of miRNAs detected in body fluids as biomarkers for BC diagnosis and prognosis is given in Figure 1.
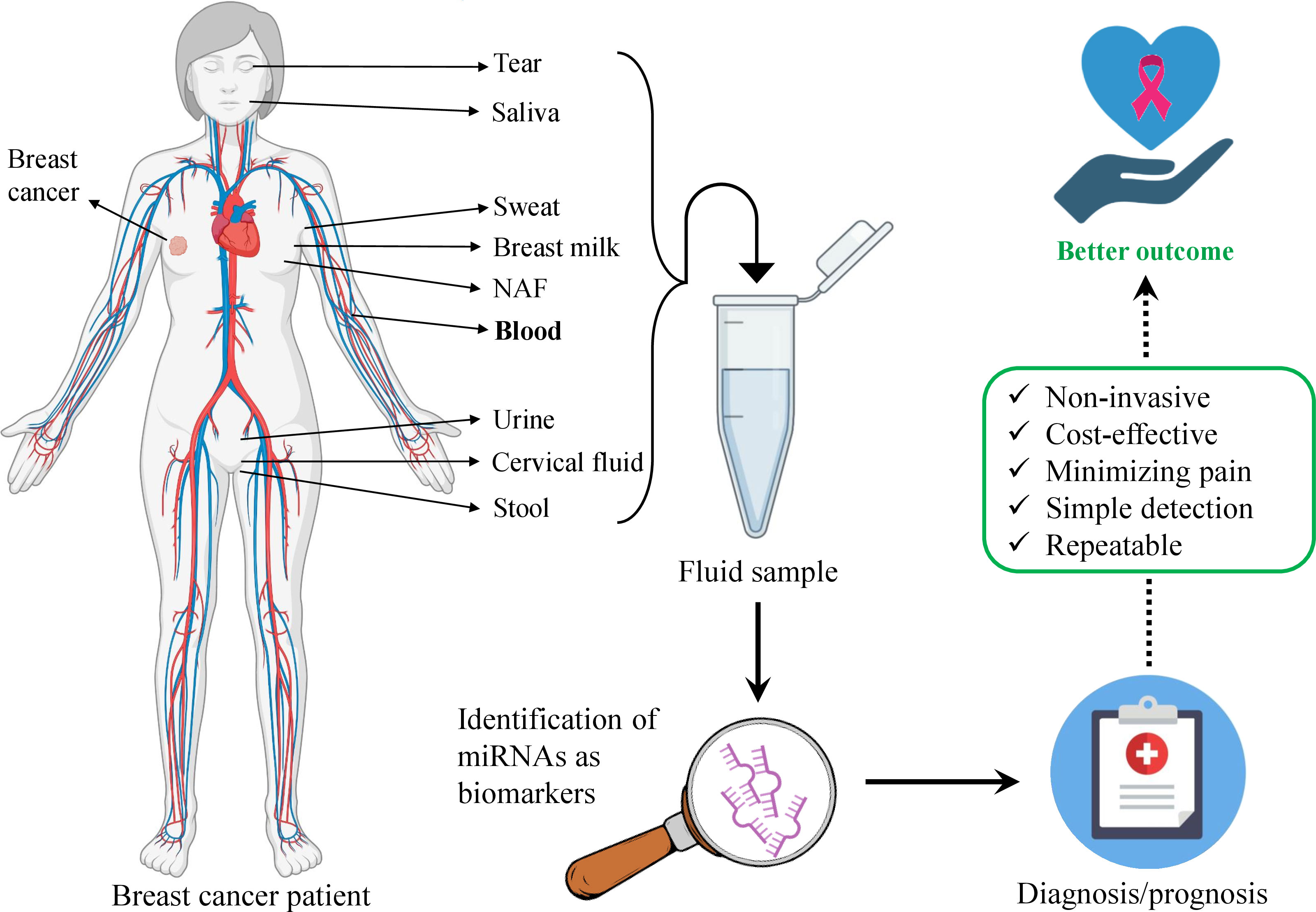
Figure 1 Schematic illustration of body fluids-based miRNA utility as biomarkers in breast cancer diagnosis and prognosis. The body fluids are the source of miRNAs that can be obtained via liquid biopsy. Bold-type indicates the most promising and studied body fluid for detecting miRNAs in breast cancer. Several listed biofluids have not yet been studied for miRNA detection as biomarkers in breast cancer, still, they represent potential liquid biopsy candidates.
miRNA role in cancers
Since the identification of miRNA in 1993, it is gaining growing attention. Currently, over 3000 miRNAs are found in almost all human body fluids. These miRNAs are implicated in various processes and play a significant role in the progression or regression of a number of diseases (22–26). miRNAs vary in their function as they have a strong capacity of regulating multiple gene expression. miRNAs have a significant impact on tumorigenesis, apoptosis, embryogenesis, cell proliferation, and differentiation among many other biological processes (27). miRNAs regulate gene expression via the post-transcriptional mechanism (6). The biogenesis of miRNA consists of several steps and takes place in the nucleus and cytoplasm of the cell. First, the primary miRNA (pri-miRNA) is transcribed mostly from introns into the nucleus and cleaved by endonuclease Drosha which results in the formation of precursor miRNA (pre-miRNA). Pre-miRNA is then translocated into the cytoplasm by Exportin 5 (XPO5) where it undergoes further processing and the mature miRNA duplex is formed. Argonaute (AGO) protein family recognizes miRNA and leads to the loading of miRNA guide strand into the RNA-induced silencing complex (RISC). The passenger strand is degraded and approximately 22 nucleotide-length miRNA containing seed region is ready to bind the specific region of the target messenger RNA (mRNA) molecule on the 3’untranslated region (3’UTR) that consequently leads to the silencing of the target gene (22, 28). Remarkably, miRNAs are implicated in the regulation of key pathways that are usually genetically altered in various cancers. As cancer represents a disease when the normal cells become abnormal via acquiring the ability of uncontrollable growth and division, miRNAs that are regulators of cell proliferation, differentiation, and apoptosis, play a crucial role in this condition. Indeed, several signaling pathways have been identified to be actively involved in cancers– RTK/RAS/MAP pathway, phosphatidylinositol 3-kinase (PI3K)/protein kinase B (AKT) (29), Wingless (WNT)/β-catenin (30), etc.
OncomiRs, tumor suppressors
According to the role in cancer, miRNAs elicit either oncogenic or tumor-suppressive functions via silencing target genes. Therefore, they may be considered oncomiRs or tumor suppressor miRNAs (6). miRNAs that are associated with cancer due to their upregulated levels are called oncomiRs (31, 32). Interestingly, tumor angiogenesis is the formation of new blood vessels that helps the tumor to grow via supplying with the blood (33) and plays a key role in cancer development. It is a complex process and comprises activation, migration, proliferation, and differentiation of the endothelial cell. There are numerous factors that control each of these steps positively or negatively (34). A number of miRNAs may act as pro-angiogenic or anti-angiogenic factors. Evidently, upregulation of miR-155 increases proliferation, invasion, migration, and tube formation in triple-negative BC (TNBC) and other cancers via targeting tumor suppressor von Hippel-Lindau (VHL) (35). TNBC is a type of BC with no commonly found BC receptors– PR, ER, and Her2. Elevated miR-210 expression increases the endothelial cell migration and its levels in BC represent a prognostic factor (34, 36). miR-182 promotes invasion and proliferation via inducing vascular endothelial growth factor A (VEGF-A) in BC (37). miR-10b is the first miRNA with oncogenic action that was discovered in metastatic BC cells. Its expression levels are positively correlated with metastasis and invasion as the action of miR-10b induces overexpression of RAS homolog family member C (RHOC) via inhibiting homeobox D10 (HOXD10) mRNA translation (38). Another miRNA miR-21 also influences the oncogenic mechanism via inhibiting tumor suppressor genes. It promotes the growth and metastasis of tumor cells. Most importantly, miR-21 is the most commonly found oncomiR in BC. Its upregulation is directly correlated with the poor prognosis of BC (38, 39) and angiogenesis (34, 40). The significance of miR-21 is conditioned by a number of target genes that are affected by miR-21 (38). On the contrary, certain miRNAs act like tumor suppressors inhibiting tumor cell growth and preventing metastasis. miR-335 is one of the tumor suppressor miRNAs that inhibits tumor growth via inducing apoptosis (41). miR-16, miR-26a, and miR-101, among a number of other miRNAs, are reported to elicit tumor-suppressive properties in BC (15).
Role of miRNAs in breast cancer
A number of miRNAs have been reported to have a regulatory function in the pathways implicated in the development of BC. Indeed, certain expression levels of certain miRNAs, e.g., miR-222, are increased in the serum of patients with BC (6). Hannafon et al. have demonstrated remarkably high concentrations of miR-21 and miR-1246 in exosomes obtained from plasma of BC patients compared with healthy controls (42). Si et al. have assessed the impact of miR-21 on tumorigenesis via transfecting anti-miR-21 antagomiR into the MCF-7 BC cells and demonstrated that the cell growth was inhibited via augmented apoptosis in MCF-7 cells as well as in xenograft mouse model. Interestingly, the decreased cell proliferation was associated with the downregulation of B-cell lymphoma 2 (Bcl-2) protein (43). Zhao et al. observed a similar outcome after miR-21 knockdown via antagomiR-21 in a mouse breast tumor model. Moreover, the results revealed that anti-miR-21 suppressed angiogenesis via inhibiting HIF-1A/VEGF/VEGFR2-associated signaling pathway (44). Wang et al. have demonstrated the augmented levels of miR-21 in BC patients compared with patients with benign breast tumors and healthy subjects while significantly reduced levels of miR-21 were found in the patients after surgery. Moreover, inhibition of this miRNA impeded tumor progression in BC. Remarkably, leucine zipper transcription factor-like 1 (LZTFL1) was found to be a direct target of miR-21 (45). Therefore, miR-21 may be considered an important pro-angiogenic miRNA (46). miRNAs such as the cluster miR-17-92 are evidenced to be implicated in BC angiogenesis and are called oncomiR-1 (33). miRNAs involved in the angiogenesis of cancers are listed in Table 1. There is a number of other miRNAs implicated in various stages of BC metastasis. These miRNAs include miR-9, miR-10a, miR-10b, miR-93, miR-125b, miR-155, miR-181d-5p, miR-191, miR-200, miR-205, miR-221/222, miR-374a, miR-375, miR-378e, etc. (31). There are anti-angiogenic miRNAs that act as tumor suppressors, e.g., miR-7, miR-29b, miR34a, miR124, miR153, miR141, miR-148a, miR-152-3p, miR-205, miR-497, etc. (55). miR-155 is found to be abnormally overexpressed in BC tissues and is associated with advanced tumor stages as well as metastasis (56). On the contrary, miR-497 is found to diminish the tumor development and formation of endothelial cell tube in BC and it was found to be downregulated in BC (57). miR-497 could be considered anti-angiogenic miRNA. Hence, a number of miRNAs are important components of BC angiogenesis which also makes them potential therapeutic targets (55). miRNAs induce tumor progression or suppression via regulating gene expression implicated in certain signaling pathways. For instance, miRNAs of the miR-200a-c family represent tumor suppressor miRNAs. miR-200a is found to be under-expressed in BC while its increased levels interfere with WNT/β-catenin signaling which is one of the leading pathways implicated in tumor cell proliferation (58). Pathways including PI3K/AKT, GAS6/MERTK, RTK/RAS/MAP-kinase, and TGF-β signaling are being affected by certain miRNAs that leads to the regulation of tumor progression or suppression (58). According to the abovementioned, there are a number of miRNAs that can be used as potential therapeutic targets for BC. miRNAs involved in inhibiting angiogenesis via targeting pro-angiogenic genes are listed in Table 2.
Liquid biopsy in breast cancer
The main barrier to advancing BC treatment is the complex heterogeneity of breast tumors (66). For BC diagnosis, a solid biopsy is a well-established technique in clinics that provides information about tumor histology and subtype. Nonetheless, mishaps in the detection of alternative biomarkers, insufficient tissue obtention, or incompatibility with long-term monitoring, among other limitations still exist (5). Compared with solid biopsy, LB is a more comprehensive diagnostic test that uses blood, urine (67), saliva, and other biological fluids (68, 69). After the sampling circulating tumor nucleic acids and cells are extracted and further analyzed (68, 70). The major tumor component that is usually extracted for the identification of tumor characteristics is circulating tumor cells (CTCs) (71). Except for the CTCs, cell-free DNA (cfDNA), ctDNA (72, 73), and c-miRNAs among other circulating non-coding RNAs (74) can be used as LB markers (13). The advancement of NGS and digital genomic approaches made biomarkers such as c-RNAs, circulating extracellular vesicles, tumor-educated platelets, proteins, etc. possible to detect in biofluids (71, 75, 76). Detection and identification of specific biomarkers are essential for the early diagnosis of BC and other cancers as they can provide additional insight into diagnosis, prognosis, and the response to treatment. In order to achieve this, LB represents a favorable approach. Additionally, in LB, invasiveness and residual disease are minimized that also enables and simplifies the longitudinal monitoring of BC patients. LB potentiates obtaining the complete molecular picture of the tumor and, thus, enhances more precise clinical decisions (5, 77). Indeed, a number of studies successfully consolidate the significance of LB in BC (78–81) and other cancers too (82, 83). Biomarkers detected via LB in biofluid samples provide an attractive alternative to biomarkers identified in tissues. The number of body fluids and the existence of all the mentioned potential biomarkers in it as tumor components warrant their promising utility in BC diagnosis, prognosis, and discrimination of different BCs. E.g., serum biomarkers are especially attractive because of the simplicity of sample collection (13). The schematic illustration of the importance and feasibility of LB in BC diagnosis and prognosis is given in Figure 2.
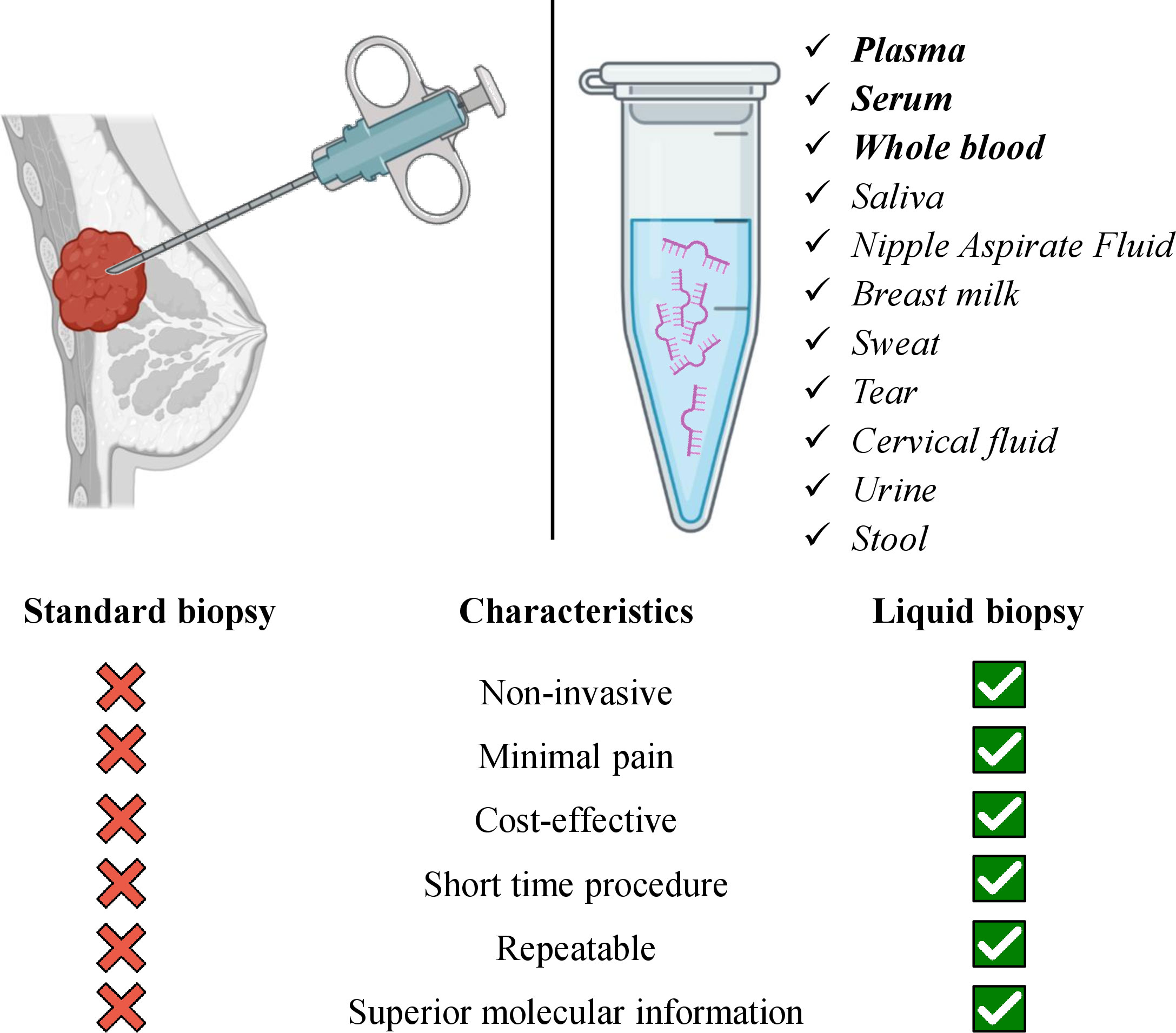
Figure 2 Advantages of liquid biopsy utility in breast cancer management via using various samples of body fluids. The biofluids written in bold indicate the most studied samples for miRNA detection in breast cancer. Several listed biofluids have not yet been studied for miRNA detection as biomarkers in breast cancer, still, they represent potential liquid biopsy candidates.
Diagnostic and prognostic function of miRNAs in breast cancer
Current biomarkers for BC such as CA15.3 and BR27.29 lack enough sensitivity which necessitates seeking the new biomarkers (84). miR-19a, miR-21, miR-24, miR-155, and miR-181b, are found to be overexpressed in patients with BC and downregulated after the surgery or treatment. This also exacerbates the concept of miRNAs’ application as a biomarker for BC diagnosis (45, 84). Henaghan et al. have studied the miRNA expression in the serum of patients with various cancers. The study revealed that certain miRNAs were specific to certain cancer types. E.g., miR-195 was abnormally increased in patients with BC (85). The downregulation of miR-329 in serum and tissue of patients with BC is associated with metastasis to lymph nodes while upregulation of miR-200 is correlated with metastasis to the brain, liver, and lung (84). Indeed, there are a number of studies that demonstrate the potency of certain miRNA utilization as BC biomarkers in various samples (56, 86–93). Apparently, miRNAs maintain the healthy balance of signaling pathways for the normal function of the body. The dysregulation of their normal expression may lead to the progression of the BC. This makes the c-miRNAs attractive biomarker candidates. Application of miRNAs as non-invasive biomarkers in BC allows not only timely diagnosis and successful monitoring of the disease (38), but it also has a discriminatory capacity for, e.g., metastatic and non-metastatic cancers (75). Moreover, in some cases, the miRNA panel demonstrates better discrimination (75, 94). Many individual miRNAs or miRNA combinations associated with certain tumors have been successfully detected in various biofluids such as uterine (95) and cervical (96, 97) fluids, serum, bile (98, 99), etc. Song et al. have screened breast milk for cfDNA and miRNAs and demonstrated that both of these molecules are stably present in breast milk. As they are derived from breast cells they might serve as biomarkers sampled non-invasively (100). Interestingly, levels of miR-155 which is strongly associated with BC were increased in urine samples of BC patients while the expression levels of other miRNAs– miR-21, miR-125b, and miR-451 were significantly lower in the urine samples compared with healthy controls (101). Interestingly, nipple aspirate fluid (NAF) as one of the best samples for detecting miRNAs deserves attention. NAF is an intraductal mammary physiological fluid and represents a source of many biomarkers including miRNAs (102). A number of advantages including its obtainability in the majority of women, the origin of BC, simplicity, and non-invasiveness of sampling, make it a valuable source of miRNAs and LB target in BC diagnosis (102, 103). There are other body fluids where miRNAs have been detected and associated with certain cancers or disorders– saliva (104–106), sweat (107), tear (108–111), and stool (112, 113). Besides, fingernails are also found to be a promising sample for the detection of miRNAs (114). Importantly, the characteristics such as the area under curve (AUC), sensitivity, and specificity should be considered while selecting the most suitable biomarker for BC diagnosis. AUC evaluates the diagnostic capability of studied candidate miRNA in BC detection. When the AUC is close to one, the diagnostic value of miRNA is higher as there are substantial differences between BC and negative control samples. Additionally, while assessing the miRNA diagnostic potential, sensitivity, and specificity that indicate the accuracy of biomarkers also need to be considered (115, 116). E.g., a study revealed that four plasma miRNA panel comprising miR-24, miR-206, miR-373, and miR-1246 could discriminate BC patients from healthy subjects with 96% specificity, 98% sensitivity, and 97% accuracy (117, 118) while the combination of miR-142-5p and miR-320a could distinguish luminal A subtype from healthy individuals with 100% sensitivity, 93.80% specificity, and AUC 0.94 (117). The abovementioned information indicates that c-miRNAs found in various body fluids are strongly correlated with the health state and may serve as attractive biomarker candidates for the diagnosis and prognosis of BC (116) (Figure 3). Broad information on c-miRNAs with a significant role in BC is combined in Table 3. Apparently, the technique of detecting miRNAs for liquid biopsy is based on the following: after obtaining the liquid sample of a BC patient, total RNA is extracted, cDNA is synthesized, and RT-qPCR is performed to detect the relative expression of target miRNAs.
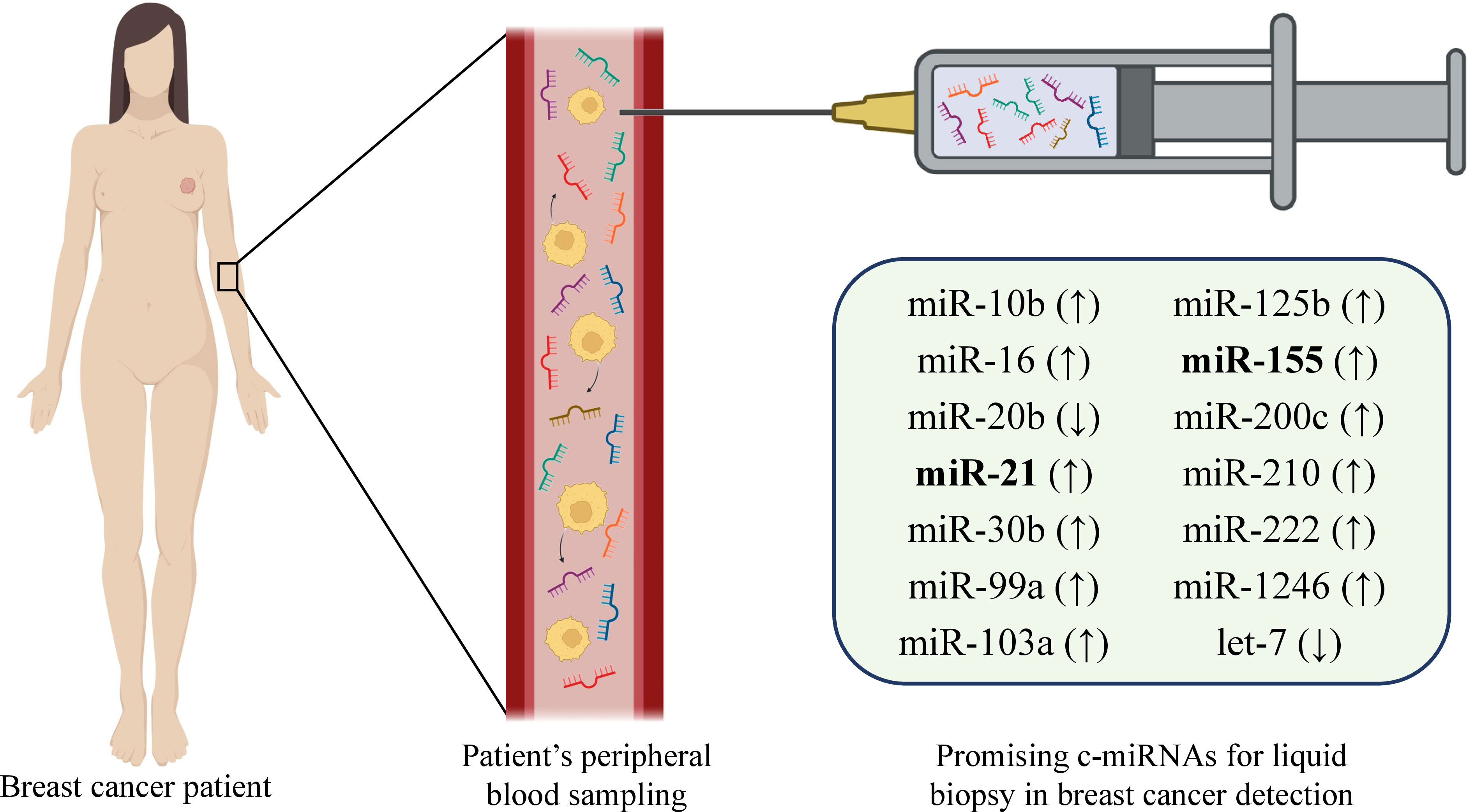
Figure 3 Representative miRNA candidates with the potential of biomarker function in breast cancer diagnosis and prognosis.

Table 3 Summary of recent studies since 2015 on altered levels of circulating miRNAs in breast cancer.
miR-10b
miR-10b via binding to the mRNA of HOXD10 gene in 3’UTR inhibits its translation and gives rise to the upregulation levels of RHOC, therefore, invasion, and metastasis (38), as well as angiogenesis, are promoted in BC (48). Indeed, a number of studies have demonstrated that the levels of miR-10b are overexpressed in the serum of BC patients which makes this particular miRNA a promising target for developing a non-invasive and cost-efficient BC LB detection approach (129, 144, 209, 212). Ibrahim et al. have studied the expression levels of certain miRNAs in plasma during different steps– diagnosis, chemotherapy, and after the tumor resection in BC patients. According to their finding, it is suggested that the upregulation of miR-10b might be the diagnostic marker of BC (144). Mangolini et al. have also demonstrated the significance of miR-10b as a biomarker of tumor aggressiveness. Particularly, the study showed that serum levels of miR-10b-5p were increased significantly along with the tumor stage while there was no substantial difference observed between the miR-10b-5p expression levels in stage I and control subjects (209). Despite the evidence, there are more studies needed to strengthen the idea of miR-10b utility as a BC biomarker via the LB approach.
miR-16
miR-16 is evidenced to be overexpressed in the blood of BC patients (127, 186). Indeed, Kim et al. have demonstrated the upregulation of miR-16 along with several more miRNAs in EVs of early-stage BC patients compared with the healthy subjects which indicates the involvement of miR-16 in tumor initiation. Their results suggested the promising outcome of the tested miRNA signatures for BC early diagnosis (127). On the contrary, Hu et al. showed no difference in the expression levels of miR-16 in BC patients and healthy subjects (177). The current evidence warrants the need for more and comprehensive studies on this miRNA to validate its role as a biomarker for LB in BC patients.
miR-17
Despite a number of studies focusing on c-miR-17 expression regulation, there is no consistency in its expression levels in BC patients (176, 187, 194, 195). Hesari et al. have studied the changes in the levels of miR-17 in the serum of BC patients compared with control subjects and found that its upregulation is associated with augmented cell proliferation and poor survival time in patients (176). Swellam et al. have also revealed the concordance of the increased miR-17-5p serum levels and clinical stages that indicates its implication in cancer progression. Furthermore, the detection technique of miR-17-5p along with miR-155 and miR-222 with RT-PCR was superior to the tumor markers such as carcinoembryonic antigen (CEA) and Cancer antigen 15-3 (CA15.3) for BC early diagnosis (187). Conversely, Jurkovicova et al. have shown decreased levels of plasma miR-17 in BC patients compared with healthy controls (194). Hence, according to the current evidence, it is difficult to postulate whether this particular miRNA can serve as a biomarker candidate for the diagnosis and prognosis of BC.
miR-20b
It is suggested that miR-20b plays a critical role in BC tumorigenesis. miR-20b is shown to have pro-angiogenic functions via inhibiting HIF-1A, STAT3 (49), and PTEN (50). Besides, it is also evidenced that its circulating levels are downregulated in BC patients (174, 195). This makes miR-20b-5p an interesting biomarker target for LB in BC.
miR-21
Noteworthily, miR-21 is abundantly found oncomiR in BC and its augmented level is strongly associated with the poor prognosis of BC (38, 39). Based on recent studies miR-21 represents a key target for most scientists in terms of its dysregulated circulating levels in BC. Indeed, a number of studies have demonstrated that c-miR-21 levels are found to be significantly augmented in BC patients compared with the healthy controls (90, 123, 127, 144, 145, 150, 162, 167, 169, 173, 177, 185, 186, 191, 201, 204, 207, 213). Interestingly, both– miR-21-3p and miR-21-5p are found to be upregulated in BC (177). Moreover, miR-21 seems to promote tumor progression via inducing cell growth, invasion, and metastasis (40). Therefore, miR-21 is indeed suggested to be one of the most attractive candidates for LB approach development in BC. According to the abovementioned information miR-21 also represents a pro-angiogenic molecule (46). Indeed, the study demonstrated that the miR-21 knockdown with anti-miR-21 resulted in the suppression of angiogenesis and cell proliferation via inhibiting the HIF-1A/VEGF/VEGFR2-associated signaling pathway in mice with implanted 4T1 murine BC cells (44). Yu et al. have also validated the advantages of miR-21-3p and miR-21-5p as potential biomarkers for BC early detection (173). Similarly, Matamala et al. have hypothesized miR-21-5p as a biomarker candidate for non-invasive BC detection (207). Subsequently, miR-21 is the most studied oncomiR in BC and the results of various studies are consistent. Thus, miR-21 deserves more attention that may change the standards in BC detection and become the first approved c-miRNA-based biomarker for BC detection.
miR-30b
The circulating levels of miR-30b are also noticed to be dysregulated in patients with BC. Particularly, its levels are reported to be augmented (120, 130, 161, 199). Indeed, Adam-Artigues et al. investigated the plasma levels of miR-30b and found it to be a valuable non-invasive diagnostic biomarker for BC. miR-30b may aid in the discrimination of stage I BC without clinical manifestation from the plasma samples which are obtained from healthy subjects (120). Zhang et al. have also found the upregulated miR-30b-5p to be a promising biomarker candidate for the diagnosis of BC in patients with very early-stage BC (199). Hence, miR-39b is suggested to be one of the candidates for LB approach development in BC detection, although, more studies are required for the complete validation of this hypothesis.
miR-99a
c-miR-99a is reported to be significantly upregulated in BC patients (120, 125, 143, 147). However, Yu et al. identified a three-miRNA panel that might be a biomarker for early BC detection, and the levels of serum miR-99a-5p were found to be downregulated in BC (173) which causes discrepancy and requires validation with more studies before it is considered as a biomarker candidate for the diagnosis of BC via LB approach.
miR-103a
miR-103a is suggested to be a biomarker for BC detection as its circulating levels are reported to be increased in patients with BC (86, 210). Liu et al. have investigated the circulating levels of miR-103a-3p in patients with BC and found them to be significantly upregulated when compared with the healthy subjects. Moreover, its expression levels in patients with positive Her2 status were higher than in subjects with Her2-negative status and patients with metastases exhibited higher levels of miR-103a-3p compared with the subjects without metastasis (86). Sahlberg et al. have suggested miR-103a-3p along with other miRNAs as a minimally invasive biomarker for tumor relapse in patients with TNBC (210). However, the studies are not sufficient enough until now and more cohorts are vital to validate its application in LB for BC detection and overall management.
miR-125b
Despite the lack of studies on c-miR-125b-5p, there is no inconsistency in its levels in BC patients. It is reported that miR-125b-5p levels are upregulated in the plasma of BC (8, 168, 207). Incoronato et al. have investigated the BC-associated miRNAs and found miR-125b-5p to be upregulated in the plasma of BC patients and could distinguish BC patients from healthy controls (168). miR-125b-5p provides information about prognosis and, therefore, the potential use of this miRNA is suggested as a non-invasive BC biomarker after further validation with more studies is performed.
miR-155
Evidently, miR-155 plays an important role in BC development. Via targeting the VHL gene it exhibits a pro-angiogenic role in BC tumorigeneses. miR-155 is shown to be upregulated in the blood of BC patients by a number of studies (149, 186, 187, 197, 212, 216). Anwar et al. studied the dynamic changes of c-miR-155 expression levels in BC patients and according to the results they concluded that women with BC expressed significantly higher levels of c-miR-155 compared with the healthy subjects while after the surgery and chemotherapy, its levels were found to be downregulated. Moreover, the expression levels were higher in patients with larger-size tumors (149). Fan et al. demonstrated that the serum level of c-miR-155 may serve as a biomarker for BC early diagnosis as well as discrimination of BC molecular subtypes (186). The same results were shown by Hagrass and colleagues who also concluded the potential of miR-155 utility as BC biomarkers (212). Kong et al. have demonstrated that except for promoting the angiogenesis, the upregulation of miR-155 promotes BC metastasis (35). Gao et al. suggested that the augmented levels of miR-155 seem to be associated with the initiation of the tumor but not the metastasis and that miR-155 may serve as a non-invasive biomarker for early-stage BC detection (197). Accordingly, miR-155 undoubtedly deserves further attention for its role in BC non-invasive detection via the LB technique.
miR-200c
There is evidence demonstrating that circulating levels of miR-200 are augmented in BC patients (169, 185, 196), which makes it an attractive biomarker candidate for BC diagnosis/prognosis. Niedźwiecki et al. have investigated the serum levels of miR-200c and its association with TNCB. They compared the expression levels of c-miR-200c between TNBC and ER/PR-positive group and demonstrated that its circulating level was lower in patients with TNBC (179). As there are still discrepancies regarding its levels in BC patients, more studies are warranted for the validation of its utility as a biomarker molecule.
miR-210
Interestingly, miR-210 targets the EFNA3 gene which makes it pro-angiogenic miRNA in BC tumorigenesis (54). Besides, a number of studies also demonstrate that the circulating levels of miR-210 are increased in BC patients (89, 138, 160). Qattan et al. have revealed that the significantly augmented levels of miR-210-3p which is implicated in certain signaling pathways are specific to the TNBC subtype of BC (138). The consistent results of the reported studies support the miR-210 utility as a promising biomarker molecule for the non-invasive detection of BC.
miR-222
It has been observed that the levels of miR-222 are increased in the blood of patients with BC (133, 162, 181, 182). A study has revealed the significantly increased plasma levels of miR-222-3p in inflammatory BC patients (133). Similar results were shown in other studies (162, 181). Zhu et al. have observed the overexpression of miR-222 in BC and demonstrated its potential role as a predictive biomarker of the response to neoadjuvant chemotherapy in HR-positive/Her2-negative BC (182). Evidently, no controversy on the levels of this miRNA in different studies has been noticed. However, in order to strengthen this claim and make miR-222 a reliable biomarker candidate, more studies are required.
miR-1246
Based on the evidence, miR-1246 is also suggested to exhibit a biomarker role in BC detection, thus, it may represent one of the key targets of LB development in BC. miR-1246 is shown to be upregulated in the blood of BC patients (122, 178, 189, 203). However, not all the studies are consistent (118). Thus, more cohorts are required to confirm the reliability of miR-1246 as a potential biomarker candidate for LB development in BC.
let-7
miRNA let-7 (lethal-7) was discovered in C. elegans and, evidently, the abnormal expression levels and dysregulation of c-let-7 are observed in BC patients. Nevertheless, it is noteworthy that these studies are not consistent about the levels of c-let-7 in BC patients as some evidence its upregulation (193) while some show the downregulation (191). Elghoroury et al. have revealed that the downregulation of let-7 expression level is associated with the risk of metastasis in BC patients and, thus, let-7 is a promising biomarker of the BC prognosis and progression (191). Importantly, more studies have demonstrated its decreased levels (133, 144, 150, 191, 208) in the blood of BC patients which makes it an attractive biomarker candidate for LB.
Challenges of liquid biopsy in breast cancer
Despite the number of advantages of LB in BC compared with tissue biopsy (217), some challenges of this approach still exist. E.g., because of the low concentration in the blood, the isolation and detection of CTCs are technically complicated which necessitate developing a specific method for it (75). In case of cfDNA application as a diagnostic biomarker, the major obstacle is the need for knowledge about tumor-specific variants (218). As to the ctDNA, its low concentration in the early stages of cancer makes the detection technically difficult while, on the other hand, the somatic mutations in blood stem cells become the source of the background noise and often lead to inaccurate interpretation of LB results (219). The main challenge for the application of c-miRNAs as biomarkers in BC diagnosis and prognosis is the detection and accurate quantification difficulties that are conditioned by their low abundance and small size in biofluids (220). Other than blood samples, there are insufficient studies on miRNAs detected in various biofluids (NAF, breast milk, saliva, urine, cervical fluid, etc.) for BC detection. Besides, more sensitive detection methods are needed for facilitating LB application in clinical settings. Additionally, the biology of each particular type of BC needs to be considered as different miRNAs exhibit different expression levels depending on the tumor types (116). This makes their use more challenging. Moreover, considering the huge number of miRNAs potentially and actually implicated in different BCs along with their complexity, there still is a lack of evidence to finally implement their utility as LB-detected biomarkers. Although a number of pre-clinical and clinical studies (Table 4) have been performed on c-miRNA dysregulation in BC for LB approach advancement, the most suitable miRNAs or miRNA panels that could be employed in clinics remain controversial. There are conflicting studies on certain c-miRNAs in BC, e.g., there is inconsistent evidence regarding the levels of miR-145. Some studies demonstrate its upregulated levels (126, 141, 170) while the others report its downregulation in BC (144, 148). This makes miR-145 a nonreliable target for the LB approach in BC diagnosis and prognosis. As well, miR-205-5p together with miR-194-5p is evidenced to exhibit a biomarker role in the detection of BC brain metastasis. miR-205-5p expression level is upregulated in 4T1-injected mice plasma compared with the control (124). However, there is no consistency in miR-205 levels in BC detection (215, 216). According to Zhang et al., miR-205 expression levels in the serum of control subjects are higher than in subjects with stage I and II (215) while Shaker et al. demonstrated significantly augmented serum levels of miR-205 in BC (216). Nevertheless, despite the mentioned shortcomings, single as well as panel miRNAs remain remarkably promising biomarker candidates for the early diagnosis and prognosis of BC.
Summary
The current review aimed to collect the most relevant scientific findings regarding the LB technique in BC detection and discussed the potential attractive miRNAs for developing this non-invasive approach. In order to save time, minimize pain, increase efficiency, and overcome the rest of the obstacles which exist in the current screening methods, the development of LB for the early diagnosis of BC is critical. miRNAs, except for their function– post-transcriptional gene regulation, may also accomplish a biomarker role for the diagnosis and prognosis of BC via detecting their levels in various biofluids. Apparently, single miRNAs or miRNA panels have a great potential of improving the accuracy of clinical diagnosis and prognosis that will undoubtedly have a positive impact on BC management and treatment. Remarkably, some miRNAs including miR-21 and miR-155 have been demonstrated to play a major role in BC progression among other miRNAs which makes them special in BC management. Except for the evidence of increased blood levels of miR-21 and miR-155 in BC patients, it is shown that the urinary levels are also upregulated compared to healthy subjects. This makes these two miRNAs even more attractive targets for developing the LB approach in BC. Although a number of pre-clinical and observational/interventional clinical studies are focused on c-miRNAs for their utility for LB in BC, the consensus about the most suitable miRNA candidate or panel still remains a debate. Currently, there is no miRNA-based LB detection method employed in clinics yet. Therefore, in order to facilitate the development of the LB approach based on miRNAs in BC, more robust and well-designed studies including a large number of subjects are essential. Moreover, the utility of the LB approach is advantageous with its feasibility– minimal invasiveness, minimal pain, short procedure, simplicity, optimal for longitudinal monitoring, and cost-effectiveness. There is a considerable evidence that miRNA signatures may be employed successfully for LB in BC.
Author contributions
The Authors DP, XLiu, and ZW contributed to the study conception, YL, XC, JL, ZhiL, LH, and ZheL contributed in data collection, DP and NR wrote the sections and prepared the original draft of the manuscript, DP worked on the visualization of the manuscript, NR reviewed and edited the manuscript, XLu and JM supervised. All authors contributed to manuscript revision, read, and approved the submitted version.
Funding
This work was supported by the National Natural Science Foundation of China (82002820 and 82072740).
Acknowledgments
Parts of the figures were created with BioRender.com.
Conflict of interest
The authors declare that the research was conducted in the absence of any commercial or financial relationships that could be construed as a potential conflict of interest.
Publisher’s note
All claims expressed in this article are solely those of the authors and do not necessarily represent those of their affiliated organizations, or those of the publisher, the editors and the reviewers. Any product that may be evaluated in this article, or claim that may be made by its manufacturer, is not guaranteed or endorsed by the publisher.
References
1. Cayrefourcq L, Alix-Panabières C. Clinical relevance of liquid biopsy in breast cancer: update in 2020. Expert Rev Mol Diagn (2020) 20:913–20. doi: 10.1080/14737159.2020.1816828
2. Desantis CE. Breast cancer statistics, 2017, racial disparity in mortality by state. CA Cancer J Clin (2017) 67:439–48. doi: 10.3322/caac.21412
3. Siegel RL, Miller KD, Fuchs HE, Jemal A. Cancer statistics, 2021. CA Cancer J Clin (2021) 71:7–33. doi: 10.3322/caac.21654
4. World Health Organization (WHO). Breast Cancer. (2021). Available at: https://www.who.int/news-room/fact-sheets/detail/breast-cancer
5. Godoy-Ortiz A, Alba-Bernal A, Pascual J, Alba E. Unveiling the potential of liquid biopsy in HER2-positive breast cancer management. Cancers (Basel) (2022) 14:587. doi: 10.3390/cancers14030587
6. Singh R, Mo Y. Role of microRNAs in breast cancer. Cancer Biol Ther (2013) 14:201–12. doi: 10.4161/cbt.23296
7. Russell A, Reeves TK. Mammography. Treasure Island (FL: StatPearls Publishing (2021) p. 3–5. Available at: https://www.ncbi.nlm.nih.gov/books/NBK559310/.
8. Zou R, Loke SY, Tang YC, Too H, Zhou L, Lee ASG, et al. Development and validation of a circulating microRNA panel for the early detection of breast cancer. Br J Cancer (2022) 126:472–81. doi: 10.1038/s41416-021-01593-6
9. Heywang-Köbrunner SH, Hacker A, Sedlacek S. Advantages and disadvantages of mammography screening. Breast Care (2011) 6:199–207. doi: 10.1159/000329005
10. Versaggi SL, De Leucio A. Breast biopsy. In: StatPearls. Treasure Island (FL: StatPearls Publishing (2022). doi: 10.1016/b978-1-4377-1720-4.00362-9
11. Alimirzaie S, Bagherzadeh M, Akbari MR. Liquid biopsy in breast cancer: A comprehensive review. Clin Genet (2019) 95:643–60. doi: 10.1111/cge.13514
12. Food and Drug Administration (FDA). FDA Approves liquid biopsy NGS companion diagnostic test for multiple cancers and biomarkers. (2020). p. 200016. Available at: https://www.fda.gov/drugs/resources-information-approved-drugs/fda-approves-liquid-biopsy-ngs-companion-diagnostic-test-multiple-cancers-and-biomarkers#:~:text=FDA%20approves%20liquid%20biopsy%20NGS%20companion%20diagnostic%20test%20for%20multiple%20cancers%20and%20biomarkers,-Share&text=On%20October%2026%20and%20November,(Foundation%20Medicine%2C%20Inc.)
13. Seale KN, Tkaczuk KHR. Circulating biomarkers in breast cancer. Clin Breast Cancer (2022) 22:e319–31. doi: 10.1016/j.clbc.2021.09.006
14. Mann RM, Hooley R, Barr RG, Moy L. Novel approaches to screening for breast cancer. Radiology (2020) 297:266–85. doi: 10.1148/RADIOL.2020200172
15. Loh HY, Norman BP, Lai KS, Rahman NMANA, Alitheen NBM, Osman MA. The regulatory role of microRNAs in breast cancer. Int J Mol Sci (2019) 20:1–27. doi: 10.3390/ijms20194940
16. Jackson BL, Grabowska A, Ratan HL. MicroRNA in prostate cancer: Functional importance and potential as circulating biomarkers. BMC Cancer (2014) 14:930. doi: 10.1186/1471-2407-14-930
17. Ono S, Lam S, Nagahara M, Hoon DSB. Circulating microRNA biomarkers as liquid biopsy for cancer patients: Pros and cons of current assays. J Clin Med (2015) 4:1890–907. doi: 10.3390/jcm4101890
18. Jansson MD, Lund AH. MicroRNA and cancer. Mol Oncol (2012) 6:590–610. doi: 10.1016/j.molonc.2012.09.006
19. Trejo-Becerril C, Pérez-Cárdenas E, Taja-Chayeb L, Anker P, Herrera-Goepfert R, Medina-Velázquez LA, et al. Cancer progression mediated by horizontal gene transfer in an in vivo model. PloS One (2012) 7:e52754. doi: 10.1371/journal.pone.0052754
20. Li H, Liu J, Chen J, Wang H, Yang L, Chen F, et al. A serum microRNA signature predicts trastuzumab benefit in HER2-positive metastatic breast cancer patients. Nat Commun (2018) 9:1614. doi: 10.1038/s41467-018-03537-w
21. Kim J, Park J, Ki J, Rho HW, Huh YM, Kim E, et al. Simultaneous dual-targeted monitoring of breast cancer circulating miRNA via surface-enhanced raman spectroscopy. Biosens Bioelectron (2022) 207:114143. doi: 10.1016/j.bios.2022.114143
22. Papukashvili D, Rcheulishvili N, Liu C, Xie F, Tyagi D, He Y, et al. Perspectives on miRNAs targeting DKK1 for developing hair regeneration therapy. Cells (2021) 10:2957. doi: 10.3390/cells10112957
23. Gazerani P. Current evidence on potential uses of MicroRNA biomarkers for Migraine: From diagnosis to treatment. Mol Diagn Ther (2019) 23:681–94. doi: 10.1007/s40291-019-00428-8
24. Ahmed SP, Castresana JS, Shahi MH. Glioblastoma and MiRNAs. Cancers (Basel) (2021) 13:1581. doi: 10.3390/cancers13071581
25. Bouchie A. First microRNA mimic enters clinic. Nat Biotechnol (2013) 31:577. doi: 10.1038/nbt0713-577
26. Chakraborty C, Sharma AR, Sharma G, Lee SS. Therapeutic advances of miRNAs: A preclinical and clinical update. J Adv Res (2021) 28:127–38. doi: 10.1016/j.jare.2020.08.012
27. Wojciechowska A, Braniewska A, Kozar-kaminska K. MicroRNA in cardiovascular biology and disease. Adv Clin Exp Med (2017) 26:865–74. doi: 10.17219/acem/62915
28. Brien JO, Hayder H, Zayed Y, Peng C. Overview of microRNA biogenesis, mechanisms of actions, and circulation. Front Endocrinol (Lausanne) (2018) 9:402. doi: 10.3389/fendo.2018.00402
29. Sanchez-Vega F, Mina M, Armenia J, Chatila WK, Luna A, La KC, et al. Oncogenic signaling pathways in the cancer genome atlas. Cell (2018) 173:321–337.e10. doi: 10.1016/j.cell.2018.03.035
30. Jung YS, Park J. Wnt signaling in cancer: therapeutic targeting of wnt signaling beyond β-catenin and the destruction complex. Exp Mol Med (2020) 52:183–91. doi: 10.1038/s12276-020-0380-6
31. Teo AYT, Xiang X, Le MTN, Wong ALA, Zeng Q, Wang L, et al. Tiny mirnas play a big role in the treatment of breast cancer metastasis. Cancers (Basel) (2021) 13:337. doi: 10.3390/cancers13020337
32. Aftab M, Poojary SS, Seshan V, Kumar S, Agarwal P, Tandon S, et al. Urine miRNA signature as a potential non − invasive diagnostic and prognostic biomarker in cervical cancer. Sci Rep (2021) 11:10323. doi: 10.1038/s41598-021-89388-w
33. Luengo-gil G, Gonzalez-billalabeitia E, Perez-henarejos SA. Angiogenic role of miR-20a in breast cancer. PloS One (2018) 13:e0194638. doi: 10.1371/journal.pone.0194638
34. Leone P, Buonavoglia A, Fasano R, Solimando AG, De Re V, Cicco S, et al. Insights into the regulation of tumor angiogenesis by micro-rnas. J Clin Med (2019) 8:2030. doi: 10.3390/jcm8122030
35. Kong W, He L, Richards EJ, Challa S, Xu CX, Permuth-Wey J, et al. Upregulation of miRNA-155 promotes tumour angiogenesis by targeting VHL and is associated with poor prognosis and triple-negative breast cancer. Oncogene (2014) 33:679–89. doi: 10.1038/onc.2012.636
36. Camps C, Buffa FM, Colella S, Moore J, Sotiriou C, Sheldon H, et al. Hsa-miR-210 is induced by hypoxia and is an independent prognostic factor in breast cancer. Clin Cancer Res (2008) 14:1340–8. doi: 10.1158/1078-0432.CCR-07-1755
37. Chiang CH, Chu PY, Hou MF, Hung WC. MiR-182 promotes proliferation and invasion and elevates the HIF-1α-VEGF-A axis in breast cancer cells by targeting FBXW7. Am J Cancer Res (2016) 6:1785–98.
38. Aggarwal V, Priyanka K, Singh H. Emergence of circulating MicroRNAs in breast cancer as diagnostic and therapeutic efficacy biomarkers. Mol Diagn Ther (2020) 24:153–73. doi: 10.1007/s40291-020-00447-w
39. Frankel LB, Christoffersen NR, Jacobsen A, Lindow M, Krogh A, Lund AH. Programmed cell death 4 (PDCD4) is an important functional target of the microRNA miR-21 in breast cancer cells. J Biol Chem (2008) 283:1026–33. doi: 10.1074/jbc.M707224200
40. Qian B, Katsaros D, Lu L, Preti M, Durando A, Arisio R, et al. High miR-21 expression in breast cancer associated with poor disease-free survival in early stage disease and high TGF-β1. Breast Cancer Res Treat (2009) 117:131–40. doi: 10.1007/s10549-008-0219-7
41. Heyn H, Engelmann M, Schreek S, Ahrens P, Lehmann U, Kreipe H, et al. MicroRNA miR-335 is crucial for the BRCA1 regulatory cascade in breast cancer development. Int J Cancer (2011) 129:2797–806. doi: 10.1002/ijc.25962
42. Hannafon BN, Trigoso YD, Calloway CL, Zhao YD, Lum DH, Welm AL, et al. Plasma exosome microRNAs are indicative of breast cancer. Breast Cancer Res (2016) 18:90. doi: 10.1186/s13058-016-0753-x
43. Si ML, Zhu S, Wu H, Lu Z, Wu F, Mo YY. miR-21-mediated tumor growth. Oncogene (2007) 26:2799–803. doi: 10.1038/sj.onc.1210083
44. Zhao D, Tu Y, Wan L, Bu L, Huang T, Sun X, et al. In vivo monitoring of angiogenesis inhibition via down-regulation of mir-21 in a VEGFR2-luc murine breast cancer model using bioluminescent imaging. PloS One (2013) 8:1–11. doi: 10.1371/journal.pone.0071472
45. Wang H, Tan Z, Hu H, Liu H, Wu T, Zheng C, et al. microRNA-21 promotes breast cancer proliferation and metastasis by targeting LZTFL1. BMC Cancer (2019) 19:738. doi: 10.1186/s12885-019-5951-3
46. Liu L, Li C, Chen Q, Jing Y, Carpenter R, Jiang Y, et al. MiR-21 induced angiogenesis through AKT and ERK activation and HIF-1a expression. PloS One (2011) 6:e19139. doi: 10.1371/journal.pone.0019139
47. Ma L, Young J, Prabhala H, Pan E, Mestdagh P, Muth D, et al. MiR-9, a MYC/MYCN-activated microRNA, regulates e-cadherin and cancer metastasis. Nat Cell Biol (2010) 12:247–56. doi: 10.1038/ncb2024
48. Shen X, Fang J, Lv X, Pei Z, Wang Y, Jiang S, et al. Heparin impairs angiogenesis through inhibition of MicroRNA-10b*. J Biol Chem (2011) 286:26616–27. doi: 10.1074/jbc.M111.224212
49. Cascio S, Andrea AD, Ferla R, Surmacz EVA, Gulotta E, Amodeo V, et al. miR-20b modulates VEGF expression by targeting HIF-1 a and STAT3 in MCF-7 breast cancer cells. J Cell Physiol (2010) 224:242–9. doi: 10.1002/jcp.22126
50. Zhou W, Shi G, Zhang Q, Wu Q, Li B, Zhang Z. MicroRNA-20b promotes cell growth of breast cancer cells partly via targeting phosphatase and tensin homologue (PTEN). Cell Biosci (2014) 4:62. doi: 10.1186/2045-3701-4-62
51. Guttilla IK, White BA. Coordinate regulation of FOXO1 by miR-27a, miR-96, and miR-182 in breast cancer cells. J Biol Chem (2009) 284:23204–16. doi: 10.1074/jbc.M109.031427
52. Fang L, Du WW, Yang W, Rutnam ZJ, Peng C, Li H, et al. MiR-93 enhances angiogenesis and metastasis by targeting LATS2. Cell Cycle (2012) 11:4352–65. doi: 10.4161/cc.22670
53. Paul U, Banerjee S. The functional significance and cross-talk of non-coding RNAs in triple negative and quadruple negative breast cancer. Mol Biol Rep (2022) 49:6899–918. doi: 10.1007/s11033-022-07288-2
54. Jung KO, Youn H, Lee C, Kang KW. Visualization of exosome-mediated miR-210 transfer from hypoxic tumor cells. Oncotarget (2017) 8:9899–910. doi: 10.18632/oncotarget.14247
55. Hussen BM, Abdullah ST, Rasul MF, Salihi A, Ghafouri-fard S, Hidayat HJ, et al. MicroRNAs: Important players in breast cancer angiogenesis and therapeutic targets. Front Mol Biosci (2021) 8:764025. doi: 10.3389/fmolb.2021.764025
56. Chen J, Wang B, Tang J-T. Clinical significance of MicoRNA-155 expression in human breast cancer patients and tissue samples. J Surg Oncol (2011) 106:260–6. doi: 10.1002/jso.22153
57. Wu Z, Cai X, Huang C, Xu J, Liu A. miR-497 suppresses angiogenesis in breast carcinoma by targeting HIF-1 α. Oncol Rep (2016) 35:1696–702. doi: 10.3892/or.2015.4529
58. Jabbarzadeh P, Rahmat A, Ismail P, Ling K. MicroRNA-based therapy and breast cancer: A comprehensive review of novel therapeutic strategies from diagnosis to treatment. Pharmacol Res (2015) 97:104–21. doi: 10.1016/j.phrs.2015.04.015
59. Li Y, Cai B, Shen L, Dong Y, Lu Q, Sun S, et al. MiRNA-29b suppresses tumor growth through simultaneously inhibiting angiogenesis and tumorigenesis by targeting Akt3. Cancer Lett (2017) 397:111–9. doi: 10.1016/j.canlet.2017.03.032
60. Kim H, Ko Y, Park H, Zhang H, Jeong Y, Kim Y, et al. MicroRNA-148a/b-3p regulates angiogenesis by targeting neuropilin-1 in endothelial cells. Exp Mol Med (2019) 51:134. doi: 10.1038/s12276-019-0344-x
61. Sun G. Overexpression of microRNA-190 inhibits migration, invasion, epithelial-mesenchymal transition, and angiogenesis through suppression of protein kinase b-extracellular signal-regulated kinase signaling pathway via binding to stanniocalicin 2 in breast canc. J Cell Physiol (2019) 234:17824–38. doi: 10.1002/jcp.28409
62. Lin X, Qiu W, Xiao Y, Ma J, Xu F, Zhang K, et al. MiR-199b-5p suppresses tumor angiogenesis mediated by vascular endothelial cells in breast cancer by targeting ALK1. Front Genet (2020) 10:1397. doi: 10.3389/fgene.2019.01397
63. He T, Qi F, Jia L, Wang S, Song N, Guo L, et al. MicroRNA-542-3p inhibits tumour angiogenesis by targeting. J Pathol (2014) 232:499–508. doi: 10.1002/path.4324
64. Li W, Kong L, Li J, Guo Z, Xue Q, Yang T, et al. MiR-568 inhibits the activation and function of CD4+ T cells and treg cells by targeting NFAT5. Int Immunol (2013) 26:269–81. doi: 10.1093/intimm/dxt065
65. Li S, Mai H, Zhu Y, Li G, Sun J, Li G, et al. MicroRNA-4500 inhibits migration, invasion, and angiogenesis of breast cancer cells via RRM2-dependent MAPK signaling pathway. Mol Ther Nucleic Acid (2020) 21:278–89. doi: 10.1016/j.omtn.2020.04.018
66. Fumagalli C, Barberis M. Breast cancer heterogeneity. Diagnostics (2021) 11:1555. doi: 10.3390/diagnostics11091555
67. Oshi M, Murthy V, Takahashi H, Huyser M, Okano M, Tokumaru Y, et al. Urine as a source of liquid biopsy for cancer. Cancers (Basel) (2021) 13:2652. doi: 10.3390/cancers13112652
68. Alba-Bernal A, Lavado-Valenzuela R, Domínguez-Recio ME, Jiménez-Rodriguez B, Queipo-Ortuño MI, Alba E, et al. Challenges and achievements of liquid biopsy technologies employed in early breast cancer. EBioMedicine (2020) 62:103100. doi: 10.1016/j.ebiom.2020.103100
69. Wijewardene AA, Chehade M, Gild ML, Clifton-bligh RJ, Bullock M. Translational utility of liquid biopsies in thyroid cancer management. Cancers (Basel) (2021) 13:3443. doi: 10.3390/cancers13143443
70. Banys-paluchowski M, Fehm N, Grimm-glang D, Rodi A, Krawczyk N. Liquid biopsy in metastatic breast cancer: Current role of circulating tumor cells and circulating tumor DNA. Oncol Res Treat (2022) 45:4–11. doi: 10.1159/000520561
71. Amico PD, Corvaja C, Gerratana L, Reduzzi C, Curigliano G. The use of liquid biopsy in early breast cancer: Clinical evidence and future perspectives. J Cancer Metastasis Treat (2021) 7:3. doi: 10.20517/2394-4722.2020.93
72. Sant M, Bernat-peguera A, Felip E. Role of ctDNA in breast cancer. Cancers (Basel) (2022) 14:310. doi: 10.3390/cancers14020310
73. Shoukry M, Broccard S, Kaplan J, Gabriel E. The emerging role of circulating tumor dna in the management of breast cancer. Cancers (Basel) (2021) 13:3813. doi: 10.3390/cancers13153813
74. Powrózek T, Ochieng Otieno M. Blood circulating non-coding RNAs for the clinical management of triple-negative breast cancer. Cancers (Basel) (2022) 14:803. doi: 10.3390/cancers14030803
75. Wu H-J, Chu P-Y. Current and developing liquid biopsy techniques for breast cancer. Cancers (Basel) (2022) 14:2052. doi: 10.3390/cancers14092052
76. Duque G, Manterola C, Otzen T, Arias C, Galindo B, Mora M, et al. Clinical utility of liquid biopsy in breast cancer: A systematic review. Clin Genet (2022) 101:285–95. doi: 10.1111/cge.14077
77. Palacín-Aliana I, García-Romero N, Asensi-Puig A, Carrión-Navarro J, González-Rumayor V, Ayuso-Sacido Á. Clinical utility of liquid biopsy-based actionable mutations detected via ddPCR. Biomedicines (2021) 9:906. doi: 10.3390/biomedicines9080906
78. Nakai M, Yamada T, Sekiya K, Sato A, Hankyo M, Kuriyama S, et al. Use of liquid biopsy to detect PIK3CA mutation in metastatic breast cancer. J Nippon Med Sch (2022) 89:66–71. doi: 10.1272/jnms.JNMS.2022_89-107
79. Tay TKY, Tan PH. Liquid biopsy in breast cancer: A focused review. Arch Pathol Lab Med (2019) 145:678–86. doi: 10.5858/arpa.2019-0559-RA
80. Halvaei S, Daryani S, Eslami-s Z, Samadi T, Jafarbeik-iravani N, Bakhshayesh TO, et al. Exosomes in cancer liquid biopsy: A focus on breast cancer. Mol Ther Nucleic Acid (2018) 10:131–41. doi: 10.1016/j.omtn.2017.11.014
81. Kujala J, Hartikainen JM, Tengström M, Sironen R, Auvinen P, Kosma V, et al. Circulating cell-free DNA reflects the clonal evolution of breast cancer tumors. Cancers (Basel) (2022) 14:1332. doi: 10.3390/cancers14051332
82. Qi Z, Xu H, Zhang S, Xu J, Li S, Gao H, et al. The significance of liquid biopsy in pancreatic cancer. J Cancer (2018) 9:3417–26. doi: 10.7150/jca.24591
83. Hofman P. Detecting resistance to therapeutic ALK inhibitors in tumor tissue and liquid biopsy Markers: An update to a clinical routine practice. Cells (2021) 10:168. doi: 10.3390/cells10010168
84. Quirico L, Orso F. The power of microRNAs as diagnostic and prognostic biomarkers in liquid biopsies. Cancer Drug Resist (2020) 3:117–39. doi: 10.20517/cdr.2019.103
85. Heneghan HM, Miller N, Kelly R, Newell J, Kerin MJ. Systemic miRNA-195 differentiates breast cancer from other malignancies and is a potential biomarker for detecting noninvasive and early stage disease. Oncologist (2010) 15:673–82. doi: 10.1634/theoncologist.2010-0103
86. Liu H, Bian QZ, Zhang W, Cui H. Circulating microRNA−103a−3p could be a diagnostic and prognostic biomarker for breast cancer. Oncol Lett (2022) 23:38. doi: 10.3892/ol.2021.13156
87. Zhang K, Wang Y, Xu Y, Zhang L, Zhu J, Si P, et al. A two-miRNA signature of upregulated miR-185-5p and miR-362-5p as a blood biomarker for breast cancer. Pathol - Res Pract (2021) 222:153458. doi: 10.1016/j.prp.2021.153458
88. Fischer C, Deutsch TM, Feisst M, Rippinger N, Riedel F, Hartkopf AD, et al. Circulating miR−200 family as predictive markers during systemic therapy of metastatic breast cancer. Arch Gynecol Obstet (2022) 5 (50):06Z. doi: 10.1007/s00404-022-06442-2
89. Nashtahosseini Z, Aghamaali MR, Heydari N, Parsian H. Circulating status of microRNAs 660-5p and 210-3p in breast cancer patients. J Gene Med (2021) 23:e3320. doi: 10.1002/jgm.3320
90. Anwar SL, Nur D, Sari I, Kartika AI, Fitria S, Tanjung DS, et al. Upregulation of circulating MiR-21 expression as a potential biomarker for therapeutic monitoring and clinical outcome in breast cancer. Asian Pac J Cancer Prev (2019) 20:1223–8. doi: 10.31557/APJCP.2019.20.4.1223
91. Todorova VK, Byrum SD, Gies AJ, Haynie C, Smith H, Reyna NS, et al. Circulating exosomal microRNAs as predictive biomarkers of neoadjuvant chemotherapy response in breast cancer. Curr Oncol (2022) 29:613–30. doi: 10.3390/curroncol29020055
92. Tian B, Hou M, Zhou K, Qiu X, Du Y, Gu Y, et al. A novel TCGA-validated, MiRNA-based signature for prediction of breast cancer prognosis and survival. Front Cell Dev Biol (2021) 9:717462. doi: 10.3389/fcell.2021.717462
93. Tang Y, Zhou X, Ji J, Chen L, Cao J, Luo J, et al. High expression levels of miR-21 and miR-210 predict unfavorable survival in breast cancer: A systemic review and meta-analysis. Int J Biol Markers (2015) 30:e347–358. doi: 10.5301/jbm.5000160
94. Madhavan D, Peng C, Wallwiener M, Zucknick M, Nees J, Schott S, et al. Circulating miRNAs with prognostic value in metastatic breast cancer and for early detection of metastasis. Carcinogenesis (2016) 37:461–70. doi: 10.1093/carcin/bgw008
95. Von Grothusen C, Frisendahl C, Modhukur V, Lalitkumar PG, Peters M, Faridani OR, et al. Uterine fluid microRNAs are dysregulated in women with recurrent implantation failure. Hum Reprod (2022) 37:734–46. doi: 10.1093/humrep/deac019
96. Wu Y, Wang X, Meng L, Li W, Li C, Li P, et al. Changes of miRNA expression profiles from cervical-vaginal fluid-derived exosomes in response to HPV16 infection. BioMed Res Int (2020) 2020:7046894. doi: 10.1155/2020/7046894
97. Kawai S, Fujii T, Kukimoto I, Yamada H, Yamamoto N, Kuroda M, et al. Identification of miRNAs in cervical mucus as a novel diagnostic marker for cervical neoplasia. Sci Rep (2018) 8:7070. doi: 10.1038/s41598-018-25310-1
98. Puik JR, Meijer LL, Le Large TY, Prado MM, Frampton AE, Kazemier G, et al. miRNA profiling for diagnosis, prognosis and stratification of cancer treatment in cholangiocarcinoma. Pharmacogenomics (2017) 18:1343–58. doi: 10.2217/pgs-2017-0010
99. Voigtländer T, Gupta SK, Thum S, Fendrich J, Manns MP, Lankisch TO, et al. MicroRNAs in serum and bile of patients with primary sclerosing cholangitis and/or cholangiocarcinoma. PloS One (2015) 10:e0139305. doi: 10.1371/journal.pone.0139305
100. Song Q, Zhang Y, Liu H, Du Y. Potential of using cell-free DNA and miRNA in breast milk to screen early breast cancer. BioMed Res Int (2020) 2020:8126176. doi: 10.1155/2020/8126176 Research
101. Erbes T, Hirschfeld M, Rücker G, Jaeger M, Boas J, Iborra S, et al. Feasibility of urinary microRNA detection in breast cancer patients and its potential as an innovative non-invasive biomarker. BMC Cancer (2015) 15:193. doi: 10.1186/s12885-015-1190-4
102. Patuleia SIS, Suijkerbuijk KPM, Van Der Wall E, Van Diest PJ, Moelans CB. Nipple aspirate fluid at a glance. Cancers (Basel) (2022) 14:159. doi: 10.3390/cancers14010159
103. Moelans CB, Patuleia SIS, Van Gils CH, Van Der Wall E, Van Diest PJ. Application of nipple aspirate fluid miRNA profiles for early breast cancer detection and management. Int J Mol Med (2019) 20:5814. doi: 10.3390/ijms20225814
104. Fadhil RS, Wei MQ, Nikolarakos D, Good D, Nair RG. Salivary microRNA miR-let-7a-5p and miR- 3928 could be used as potential diagnostic bio-markers for head and neck squamous cell carcinoma. PloS One (2020) 15:e0221779. doi: 10.1371/journal.pone.0221779
105. Rapado-González Ó, Majem B, Muinelo-Romay L, Álvarez-Castro A, Santamaría A, Gil-Moreno A, et al. Human salivary microRNAs in cancer. J Cancer (2018) 9:638–49. doi: 10.7150/jca.21180
106. Setti G, Pezzi ME, Viani MV, Pertinhez TA, Cassi D, Magnoni C, et al. Salivary MicroRNA for diagnosis of cancer and systemic diseases: A systematic review. Int J Mol Sci (2020) 21:907. doi: 10.3390/ijms21030907
107. Karvinen S, Sievänen T, Karppinen JE, Hautasaari P, Bart G, Samoylenko A, et al. MicroRNAs in extracellular vesicles in sweat change in response to endurance exercise. Front Physiol (2020) 11:676. doi: 10.3389/fphys.2020.00676
108. Chan HW, Yang B, Wong W, Blakeley P, Seah I, Shu Q, et al. A pilot study on MicroRNA profile in tear fluid to predict response to anti-VEGF treatments for diabetic macular edema. J Clin Med (2020) 9:2920. doi: 10.3390/jcm9092920
109. Kakan SS, Edman MC, Yao A, Okamoto CT, Nguyen A, Hjelm BE, et al. Tear miRNAs identified in a murine model of sjögren’s syndrome as potential diagnostic biomarkers and indicators of disease mechanism. Front Immunol (2022) 13:833254. doi: 10.3389/fimmu.2022.833254
110. Kim YJ, Yeon Y, Lee WJ, Shin YU, Cho H, Sung Y, et al. Comparison of MicroRNA expression in tears of normal subjects and sjögren syndrome patients. Investig Opthalmol Vis Sci (2019) 60:4889–95. doi: 10.1167/iovs.19-27062
111. Nakagawa A, Nakajima T, Azuma M. Tear miRNA expression analysis reveals miR-203 as a potential regulator of corneal epithelial cells. BMC Ophthalmol (2021) 21:377. doi: 10.1186/s12886-021-02141-9
112. Rashid H, Hossain B, Siddiqua T, Kabir M, Noor Z, Losurdo G, et al. Fecal MicroRNAs as potential biomarkers for screening and diagnosis of intestinal diseases. Front Mol Biosci (2020) 7:181. doi: 10.3389/fmolb.2020.00181
113. Link A, Balaguer F, Shen Y, Nagasaka T, Lozano JJ, Boland CR, et al. Fecal MicroRNAs as novel biomarkers for colon cancer screening. Cancer Epidemiol Biomarkers Prev (2010) 19:1766–75. doi: 10.1158/1055-9965.EPI-10-0027
114. Wang Z, Jinnin M, Harada M, Kudo H, Inoue K, Nakayama W, et al. Diagnosis of nail psoriasis: evaluation of nail-derived microRNAs as potential novel biomarkers. Eur J Dermatol (2017) 27:20–7. doi: 10.1684/ejd.2016.2906
115. Sadeghi H, Kamal A, Ahmadi M, Najafi H, Sharifi A, Haddad P, et al. A novel panel of blood-based microRNAs capable of discrimination between benign breast disease and breast cancer at early stages. RNA Biol (2021) 18:747–56. doi: 10.1080/15476286.2021.1989218
116. Ozawa PMM, Jucoski TS, Vieira E, Carvalho TM, Malheiros D, Ribeiro EM de SF. Liquid biopsy for breast cancer using extracellular vesicles and cell-free microRNAs as biomarkers. Transl Res (2020) 223:40–60. doi: 10.1016/j.trsl.2020.04.002
117. Palanca-ballester C, Rodriguez-casanova A, Torres S, Calabuig-fariñas S, Jantus-lewintre E, Diaz-lagares A, et al. Cancer epigenetic biomarkers in liquid biopsy for high incidence malignancies. Cancers (Basel) (2021) 13:3016. doi: 10.3390/cancers13123016
118. Jang JY, Kim YS, Kang KN, Kim KH, Park YJ, Kim CW. Multiple microRNAs as biomarkers for early breast cancer diagnosis. Mol Clin Oncol (2021) 14:31. doi: 10.3892/mco.2020.2193
119. Gahlawat AW, Fahed L, Witte T, Schott S. Total circulating microRNA level as an independent prognostic marker for risk strati fi cation in breast cancer. Br J Cancer (2022) 127:156–62. doi: 10.1038/s41416-022-01756-z
120. Adam-Artigues A, Garrido-Cano I, Carbonell-Asins JA, Lameirinhas A, Simón S, Ortega-Morillo B, et al. Identification of a two-microrna signature in plasma as a novel biomarker for very early diagnosis of breast cancer. Cancers (Basel) (2021) 13:2848. doi: 10.3390/cancers13112848
121. Bao S, Hu T, Liu J, Su J, Sun J, Ming Y, et al. Genomic instability-derived plasma extracellular vesicle-microRNA signature as a minimally invasive predictor of risk and unfavorable prognosis in breast cancer. J Nanobiotechnol (2021) 19:22. doi: 10.1186/s12951-020-00767-3
122. Chen Y, Zhai LY, Zhang LM, Ma XS, Liu Z, Li MM, et al. Breast cancer plasma biopsy by in situ determination of exosomal microRNA-1246 with a molecular beacon. Analyst (2021) 146:2264–76. doi: 10.1039/d0an02224a
123. Diansyah MN, Prayogo AA, Sedana MP, Savitri M, Romadhon PZ, Amrita PNA, et al. Early detection breast cancer: Role of circulating plasma miRNA-21 expression as a potential screening biomarker. Turkish J Med Sci (2021) 51:562–9. doi: 10.3906/sag-2005-138
124. Figueira I, Godinho-Pereira J, Galego S, Maia J, Haskó J, Molnár K, et al. Micrornas and extracellular vesicles as distinctive biomarkers of precocious and advanced stages of breast cancer brain metastases development. Int J Mol Sci (2021) 22:5214. doi: 10.3390/ijms22105214
125. Garrido-Cano I, Pla L, Santiago-Felipe S, Simón S, Ortega B, Bermejo B, et al. Nanoporous anodic alumina-based sensor for miR-99a-5p detection as an effective early breast cancer diagnostic tool. ACS Sensors (2021) 6:1022–9. doi: 10.1021/acssensors.0c02222
126. Jusoh AR, Mohan SV, Ping TL, Tengku Din TADAAB, Haron J, Romli RC, et al. Plasma circulating mirnas profiling for identification of potential breast cancer early detection biomarkers. Asian Pac J Cancer Prev (2021) 22:1375–81. doi: 10.31557/APJCP.2021.22.5.1375
127. Kim MW, Park S, Lee H, Gwak H, Hyun KA, Kim JY, et al. Multi-miRNA panel of tumor-derived extracellular vesicles as promising diagnostic biomarkers of early-stage breast cancer. Cancer Sci (2021) 112:5078–87. doi: 10.1111/cas.15155
128. Liu D, Li B, Shi X, Zhang J, Chen AM, Xu J, et al. Cross-platform genomic identification and clinical validation of breast cancer diagnostic biomarkers. Aging (Albany NY) (2021) 13:4258–73. doi: 10.18632/aging.202388
129. Dwedar FI, Shams-Eldin RS, Nayer Mohamed S, Mohammed AF, Gomaa SH. Potential value of circulatory microRNA10b gene expression and its target e-cadherin as a prognostic and metastatic prediction marker for breast cancer. J Clin Lab Anal (2021) 35:e23887. doi: 10.1002/jcla.23887
130. Simón S, Ortega B, Moragón S, Lameirinhas A, Constâncio V, Salta S. Circulating miR-30b-5p levels in plasma as a novel potential biomarker for early detection of breast cancer. ESMO Open (2021) 6:100039. doi: 10.1016/j.esmoop.2020.100039
131. Xun J, Du L, Gao R, Shen L, Wang D, Kang L, et al. Theranostics cancer-derived exosomal miR-138-5p modulates polarization of tumor-associated macrophages through inhibition of KDM6B. Theranostics (2021) 11:6847–59. doi: 10.7150/thno.51864
132. Liu X, Chang Q, Wang H, Qian H, Jiang Y. Discovery and function exploration of microRNA−155 as a molecular biomarker for early detection of breast cancer. Breast Cancer (2021) 28:806–21. doi: 10.1007/s12282-021-01215-2
133. Ahmed SHA, Espinoza-Sanchez NA, El-Damen A, Sarah Fahim A, Badawy MA, Greve B, et al. Small extracellular vesicle-encapsulated miR-181b-5p, miR-222-3p and let-7a-5p: Next generation plasma biopsy-based diagnostic biomarkers for inflammatory breast cancer. PloS One (2021) 16:e0250642. doi: 10.1371/journal.pone.0250642
134. Wang X, Qian T, Bao S, Zhao H, Chen H, Xing Z, et al. Circulating exosomal miR-363-5p inhibits lymph node metastasis by downregulating PDGFB and serves as a potential noninvasive biomarker for breast cancer. Mol Oncol (2021) 15:2466–79. doi: 10.1002/1878-0261.13029
135. Bakr NM, Mahmoud MS, Nabil R, Boushnak H, Swellam M. Impact of circulating miRNA-373 on breast cancer diagnosis through targeting VEGF and cyclin D1 genes. J Genet Eng Biotechnol (2021) 19:84. doi: 10.1186/s43141-021-00174-7
136. Hashimoto K, Inada M, Yamamoto Y, Ochiya T. Heliyon preliminary evaluation of miR-1307-3p in human serum for detection of 13 types of solid cancer using microRNA chip. Heliyon (2021) 7:e07919. doi: 10.1016/j.heliyon.2021.e07919
137. Mohmmed EA, Shousha WG, El-saiid AS, Ramadan SS. A clinical evaluation of circulating MiR-106a and raf-1 as breast cancer diagnostic and prognostic markers. Asian Pac J Cancer Prev (2022) 22:3513–20. doi: 10.31557/APJCP.2021.22.11.3513
138. Qattan A, Al-Tweigeri T, Alkhayal W, Suleman K, Tulbah A, Amer S. Clinical identification of dysregulated circulating microRNAs and their implication in drug response in triple negative breast cancer (TNBC) by target gene network and meta-analysis. Genes (Basel) (2021) 12:549. doi: 10.3390/genes12040549
139. Uyisenga JP, Debit A, Poulet C, Frères P, Poncin A, Thiry J, et al. Differences in plasma microRNA content impair microRNA−based signature for breast cancer diagnosis in cohorts recruited from heterogeneous environmental sites. Sci Rep (2021) 11:11698. doi: 10.1038/s41598-021-91278-0
140. Zou R, Loke SY, Tan VK, Quek ST, Jagmohan P, Tang YC, et al. Development of a microRNA panel for classification of abnormal mammograms for breast cancer. Cancers (Basel) (2021) 13:2130. doi: 10.3390/cancers13092130
141. Ashirbekov Y, Abaildayev A, Omarbayeva N, Botbayev D, Belkozhayev A, Askandirova A, et al. Combination of circulating miR-145-5p / miR-191-5p as biomarker for breast cancer detection. PeerJ (2020) 8:e10494. doi: 10.7717/peerj.10494
142. Yadi W, Shurui C, Tong Z, Suxian C, Qing T, Dongning H. Bioinformatic analysis of peripheral blood miRNA of breast cancer patients in relation with anthracycline cardiotoxicity. BMC Cardiovasc Disord (2020) 20:43. doi: 10.1186/s12872-020-01346-y
143. Holubekova V, Kolkova Z, Grendar M, Brany D, Dvorska D, Stastny I, et al. Pathway analysis of selected circulating miRNAs in plasma of breast cancer patients: A preliminary study. Int J Mol Sci (2020) 21:7288. doi: 10.3390/ijms21197288
144. Ibrahim AM, Said MM, Hilal AM, Medhat AM, Abd Elsalam IM. Candidate circulating microRNAs as potential diagnostic and predictive biomarkers for the monitoring of locally advanced breast cancer patients. Tumor Biol (2020) 42:1–13. doi: 10.1177/1010428320963811
145. Koi Y, Tsutani Y, Nishiyama Y, Ueda D, Ibuki Y, Sasada S, et al. Predicting the presence of breast cancer using circulating small RNAs, including those in the extracellular vesicles. Cancer Sci (2020) 111:2104–15. doi: 10.1111/cas.14393
146. Ahmed EA, El-basit SAA, Mohamed MA. Clinical role of MiRNA 29a and MiRNA 335 on breast cancer management: their relevance to MMP2 protein level. Arch Physiol Biochem (2020) 1:8. doi: 10.1080/13813455.2020.1749085
147. Garrido-Cano I, Constâncio V, Adam-Artigues A, Lameirinhas A, Simón S, Ortega B, et al. Circulating mir-99a-5p expression in plasma: A potential biomarker for early diagnosis of breast cancer. Int J Mol Sci (2020) 21:7427. doi: 10.3390/ijms21197427
148. García-Magallanes N, Beltran-Ontiveros SA, Leal-León EA, Luque-Ortega F, Romero-Quintana JG, Osuna-Ramirez I, et al. Underexpression of circulating miR-145-5p and miR-133a-3p are associated with breast cancer and immunohistochemical markers. J Cancer Res Ther (2020) 16:1223–8. doi: 10.4103/jcrt.JCRT_1111_19
149. Anwar SL, Tanjung DS, Fitria MS, Kartika AI, Nur D, Sari I, et al. Dynamic changes of circulating mir-155 expression and the potential application as a non-invasive biomarker in breast cancer. Asian Pac J Cancer Prev (2020) 21:491–7. doi: 10.31557/APJCP.2020.21.2.491
150. Aksan H, Kundaktepe BP, Sayili U, Velidedeoglu M, Simsek G, Koksal S, et al. Circulating miR-155, let-7c, miR-21, and PTEN levels in differential diagnosis and prognosis of idiopathic granulomatous mastitis and breast cancer. Biofactors (2020) 46:955–62. doi: 10.1002/biof.1676
151. Wang M, Zhang H, Shen B. miR-188-5p suppresses cellular proliferation and migration via IL6ST: A potential noninvasive diagnostic biomarker for breast cancer. J Cell Physiol (2020) 235:4890–901. doi: 10.1002/jcp.29367
152. Kim J, Park S, Hwang D, Il KS, Lee H. Diagnostic value of circulating miR-202 in early-stage breast cancer in South Korea. Med (B Aires) (2020) 56:340. doi: 10.3390/medicina56070340
153. Zou X, Li M, Huang Z, Zhou X, Liu Q, Xia T, et al. Circulating miR-532-502 cluster derived from chromosome X as biomarkers for diagnosis of breast cancer. Gene (2020) 722:144104. doi: 10.1016/j.gene.2019.144104
154. Wang B, Mao J, Wang B, Wang L, Wen H, Xu L, et al. Exosomal miR-1910-3p promotes proliferation, metastasis, and autophagy of breast cancer cells by targeting MTMR3 and activating the NF-κB signaling pathway. Cancer Lett (2020) 489:87–99. doi: 10.1016/j.canlet.2020.05.038
155. Moln K, Medina SJ, Reisz Z, Malh R, Sereno M, Booth SA, et al. Downregulation of circulating miR 802-5p and miR 194-5p and upregulation of brain MEF2C along breast cancer brain metastasization. Mol Oncol (2020) 14:520–38. doi: 10.1002/1878-0261.12632
156. Yan C, Hu J, Yang Y, Hu H, Zhou D, Ma MIN, et al. Plasma extracellular vesicle − packaged microRNAs as candidate diagnostic biomarkers for early − stage breast cancer. Mol Med Rep (2019) 20:3991–4002. doi: 10.3892/mmr.2019.10669
157. Souza KCB, Evangelista AF, Leal LF, Souza CP, Vieira RA, Causin RL, et al. Identification of cell-free circulating MicroRNAs for the detection of early breast cancer and molecular subtyping. J Oncol (2019) 2019:8393769. doi: 10.1155/2019/8393769
158. Raheem AR, Abdul-rasheed OF, Al-naqqash MA. The diagnostic power of circulating micro ribonucleic acid 34a in combination with cancer antigen 15-3 as a potential biomarker of breast cancer. Saudi Med J (2019) 40:1218–26. doi: 10.15537/smj.2019.12.24712
159. Shiino S, Matsuzaki J, Shimomura A, Kawauchi J, Takizawa S, Sakamoto H, et al. Serum miRNA–based prediction of axillary lymph node metastasis in breast cancer. Clin Cancer Res (2019) 25:1817–27. doi: 10.1158/1078-0432.CCR-18-1414
160. Li M, Wang S. A five-miRNA panel in plasma was identified for breast cancer diagnosis. Cancer Med (2019) 8:7006–17. doi: 10.1002/cam4.2572
161. Pereira E, Med JT, Pereira HE, Lobo J, Salta S, Amorim M, et al. Overexpression of circulating MiR−30b−5p identifies advanced breast cancer. J Transl Med (2019) 17:435. doi: 10.1186/s12967-019-02193-y
162. Rodríguez-martínez A, De Miguel-pérez D, Ortega FG, García-puche JL, Robles-fernández I, Exposito J, et al. Exosomal miRNA profile as complementary tool in the diagnostic and prediction of treatment response in localized breast cancer under neoadjuvant chemotherapy. Breast Cancer Res (2019) 21:21. doi: 10.1186/s13058-019-1109-0
163. Tan W, Liang G, Xie X, Jiang W, Tan L, Sanders AJ, et al. Incorporating MicroRNA into molecular phenotypes of circulating tumor cells enhances the prognostic accuracy for patients with metastatic breast cancer. Oncologist (2019) 24:e1044–54. doi: 10.1634/theoncologist.2018-0697
164. Swellam M, Zahran RFK, Ghonem SA, Abdel-Malak C. Serum MiRNA-27a as potential diagnostic nucleic marker for breast cancer. Arch Physiol Biochem (2019) 127:90–6. doi: 10.1080/13813455.2019.1616765
165. Mcanena P, Tanriverdi K, Curran C, Gilligan K, Freedman JE, Brown JAL, et al. Circulating microRNAs miR-331 and miR- 195 differentiate local luminal a from metastatic breast cancer. BMC Cancer (2019) 19:436. doi: 10.1186/s12885-019-5636-y
166. Saleh AA, Soliman SE, Habib MSE, Gohar SF, Abo−Zeid GS. Potential value of circulatory microRNA122 gene expression as a prognostic and metastatic prediction marker for breast cancer. Mol Biol Rep (2019) 46:2809–18. doi: 10.1007/s11033-019-04727-5
167. Abdulhussain MM, Hasan NA, Hussain AG. Interrelation of the circulating and tissue MicroRNA-21 with tissue PDCD4 expression and the invasiveness of Iraqi female breast tumors. Indian J Clin Biochem (2019) 34:26–38. doi: 10.1007/s12291-017-0710-1
168. Incoronato M, Grimaldi AM, Mirabelli P, Cavaliere C, Parente CA, Franzese M, et al. Circulating miRNAs in untreated breast cancer: An exploratory multimodality morpho-functional study. Cancers (Basel) (2019) 11:876. doi: 10.3390/cancers11060876
169. Papadaki C, Stoupis G, Tsalikis L, Monastirioti A. Circulating miRNAs as a marker of metastatic disease and prognostic factor in metastatic breast cancer. Oncotarget (2019) 10:966–81. doi: 10.18632/oncotarget.26629
170. Arabkari V, Clancy E, Dwyer RM, Kerin MJ, Kalinina O, Holian E, et al. Relative and absolute expression analysis of MicroRNAs associated with luminal a breast cancer– a comparison. Pathol Oncol Res (2020) 26:833–44. doi: 10.1007/s12253-019-00627-y
171. Di Cosimo S, Appierto V, Pizzamiglio S, Tiberio P, Iorio MV, Hilbers F, et al. Plasma miRNA levels for predicting therapeutic response to neoadjuvant treatment in HER2-positive breast cancer: Results from the NeoALTTO trial. Clin Cancer Res (2019) 25:3887–95. doi: 10.1158/1078-0432.CCR-18-2507
172. Swellam M, Mahmoud MS, Hashim M, Hassan NM, Sobeih ME, Nageeb AM. Clinical aspects of circulating miRNA-335 in breast cancer patients: A prospective study. J Cell Biochem (2019) 120:8975–82. doi: 10.1002/jcb.28168
173. Yu X, Liang J, Xu J, Li X, Xing S, Li H, et al. Breast cancer identification and validation of circulating MicroRNA signatures for breast cancer early detection based on Large scale tissue-derived data. J Breast Cancer (2018) 21:363–70. doi: 10.4048/jbc.2018.21.e56
174. Li M, Zhou Y, Xia T, Zhou X, Huang Z, Zhang H, et al. Circulating microRNAs from the miR-106a–363 cluster on chromosome X as novel diagnostic biomarkers for breast cancer. Breast Cancer Res Treat (2018) 170:257–70. doi: 10.1007/s10549-018-4757-3
175. Zhang Q, Wang Q, Sun W, Gao F, Liu L, Cheng L, et al. Change of circulating and tissue-based miR-20a in human cancers and associated prognostic implication: A systematic review and meta-analysis. BioMed Res Int (2018) 2018:6124927. doi: 10.1155/2018/6124927
176. Hesari AR, Azizian M, Darabi H, Nesaei A, Hosseini SA, Salarinia R, et al. Expression of circulating miR-17, miR-25, and miR-133 in breast cancer patients. J Cell Biochem (2019) 120:7109–14. doi: 10.1002/jcb.27984
177. Hu X, Fan J, Duan B, Zhang H, He Y, Duan P, et al. Single-molecule catalytic hairpin assembly for rapid and direct quantification of circulating miRNA biomarkers. Anal Chim Acta (2018) 1042:109–15. doi: 10.1016/j.aca.2018.08.037
178. Zhai L, Li M, Pan W, Chen Y, Li M, Pang J, et al. In situ detection of plasma exosomal MicroRNA-1246 for breast cancer diagnostics by a au nanoflare probe. ACS Appl Mater Interfaces (2018) 10:39478–86. doi: 10.1021/acsami.8b12725
179. Niedźwiecki S, Piekarski J, Szymańska B, Pawłowska Z, Jeziorski A. Serum levels of circulating miRNA-21, miRNA-10b and miRNA-200c in triple-negative breast cancer patients. Ginekol Pol (2018) 89:415–20. doi: 10.5603/GP.a2018.0071
180. Ali OS, Shabayek MI, Seleem MM, Abdelaziz HG, Makhlouf DO. MicroRNAs 182 and 375 sera expression as prognostic biochemical markers in breast cancer. Clin Breast Cancer (2018) 18:e1373–9. doi: 10.1016/j.clbc.2018.07.020
181. Wang Y, Yin W, Lin Y, Yin K, Zhou L, Du Y, et al. Downregulated circulating microRNAs after surgery: Potential noninvasive biomarkers for diagnosis and prognosis of early breast cancer. Cell Death Discov (2019) 5:21. doi: 10.1038/s41420-018-0089-7
182. Zhu W, Liu M, Fan Y, Ma F, Xu N, Xu B. Dynamics of circulating microRNAs as a novel indicator of clinical response to neoadjuvant chemotherapy in breast cancer. Cancer Med (2018) 7:4420–33. doi: 10.1002/cam4.1723
183. Masuda T, Shinden Y, Noda M, Ueo H, Hu Q, Yoshikawa Y, et al. Circulating pre-microRNA-488 in peripheral blood is a potential biomarker for predicting. Anticancer Res (2018) 4523:4515–23. doi: 10.21873/anticanres.12755
184. Guo J, Liu C, Wang W, Liu Y, He H, Chen C, et al. Identification of serum miR-1915-3p and miR-455-3p as biomarkers for breast cancer. PloS One (2018) 13:e0200716. doi: 10.1371/journal.pone.0200716
185. Papadaki C, Stratigos M, Markakis G, Spiliotaki M, Mastrostamatis G, Nikolaou C, et al. Circulating microRNAs in the early prediction of disease recurrence in primary breast cancer. Breast Cancer Res (2018) 20:72. doi: 10.1186/s13058-018-1001-3
186. Fan T, Mao Y, Sun Q, Liu F, Lin JS, Liu Y, et al. Branched rolling circle amplification method for measuring serum circulating microRNA levels for early breast cancer detection. Cancer Sci (2018) 109:2897–906. doi: 10.1111/cas.13725
187. Swellam M, Zahran RFK, Abo El-Sadat Taha H, El-Khazragy N, Abdel-Malak C. Role of some circulating MiRNAs on breast cancer diagnosis. Arch Physiol Biochem (2019) 125:456–64. doi: 10.1080/13813455.2018.1482355
188. Swellam M, El Magdoub HM, Hassan NM, Hefny MM, Sobeih ME. Potential diagnostic role of circulating MiRNAs in breast cancer: Implications on clinicopathological characters. Clin Biochem (2018) 56:47–54. doi: 10.1016/j.clinbiochem.2018.04.013
189. Cui X, Li Z, Zhao Y, Song A, Shi Y, Hai X, et al. Breast cancer identification via modeling of peripherally circulating miRNAs. PeerJ (2018) 26:e4551. doi: 10.7717/peerj.4551
190. Alunni-fabbroni M, Majunke L, Trapp EK, Tzschaschel M, Mahner S, Fasching PA, et al. Whole blood microRNAs as potential biomarkers in post-operative early breast cancer patients. BMC Cancer (2018) 18:141. doi: 10.1186/s12885-018-4020-7
191. Elghoroury EA, Eldine HG, Kamel SA, Abdelrahman AH, Mohammed A, Kamel MM, et al. Evaluation of miRNA-21 and miRNA let-7 as prognostic markers in patients with breast cancer. Clin Breast Cancer (2018) 18:e721–6. doi: 10.1016/j.clbc.2017.11.022
192. Li HY, Liang JL, Kuo YL, Lee HH, Calkins MJ, Chang HT, et al. miR-105/93-3p promotes chemoresistance and circulating miR-105/93-3p acts as a diagnostic biomarker for triple negative breast cancer. Breast Cancer Res (2017) 19:133. doi: 10.1186/s13058-017-0918-2
193. Qattan A, Intabli H, Alkhayal W, Eltabache C, Tweigieri T, Amer SB. Robust expression of tumor suppressor miRNA’s let-7 and miR-195 detected in plasma of Saudi female breast cancer patients. BMC Cancer (2017) 17:799. doi: 10.1186/s12885-017-3776-5
194. Jurkovicova D, Smolkova B, Magyerkova M, Sestakova Z, Kajabova VH, Kulcar L, et al. Down-regulation of traditional oncomiRs in plasma of breast cancer patients. Oncotarget (2017) 8:77369–84. doi: 10.18632/oncotarget.20484
195. Sueta A, Yamamoto Y, Tomiguchi M, Takeshita T, Yamamoto-Ibusuki M, Iwase H. Differential expression of exosomal miRNAs between breast cancer patients with and without recurrence. Oncotarget (2017) 8:69934–44. doi: 10.18632/oncotarget.19482
196. Zhang G, Zhang W, Li B, Stringer-reasor E, Chu C, Sun L, et al. MicroRNA-200c and microRNA- 141 are regulated by a FOXP3-KAT2B axis and associated with tumor metastasis in breast cancer. Breast Cancer Res (2017) 19:73. doi: 10.1186/s13058-017-0858-x
197. Gao S, Wang Y, Wang M, Li Z, Zhao Z, Raymond X, et al. MicroRNA-155, induced by FOXP3 through transcriptional repression of BRCA1, is associated with tumor initiation in human breast cancer. Oncotarget (2017) 8:41451–64. doi: 10.18632/oncotarget.17816
198. Zeng Z, Chen X, Zhu D, Luo Z, Yang M. Low expression of circulating microRNA-34c is associated with poor prognosis in triple-negative breast cancer. Yonsei Med J (2017) 58:697–702. doi: 10.3349/ymj.2017.58.4.697
199. Zhang K, Wang Y, Wang Y, Song Y, Zhu J, Si P, et al. Identi fi cation of microRNA biomarkers in the blood of breast cancer patients based on microRNA pro fi ling. Gene (2017) 619:10–20. doi: 10.1016/j.gene.2017.03.038
200. Shao Y, Gu W, Ning Z, Song X, Pei H, Jiang J. Evaluating the prognostic value of microRNA-203 in solid tumors based on a meta-analysis and the cancer genome atlas (TCGA) datasets. Cell Physiol Biochem (2017) 41:1468–80. doi: 10.1159/000470649
201. Jinling W, Sijing S, Jie Z, Guinian W. Prognostic value of circulating microRNA-21 for breast cancer: A systematic review and meta-analysis. Artif Cells Nanomed Biotechnol (2016) 45:1–6. doi: 10.1080/21691401.2016.1216856
202. Hamam R, Ali AM, Alsaleh KA, Kassem M, Alfayez M. microRNA expression profiling on individual breast cancer patients identifies novel panel of circulating microRNA for early detection. Sci Rep (2016) 6:25997. doi: 10.1038/srep25997
203. Shimomura A, Shiino S, Kawauchi J, Takizawa S, Sakamoto H, Matsuzaki J, et al. Novel combination of serum microRNA for detecting breast cancer in the early stage. Cancer Sci (2016) 107:326–34. doi: 10.1111/cas.12880
204. Huo D, Clayton WM, Yoshimatsu TF, Chen J, Olopade OI. Identification of a circulating MicroRNA signature to distinguish recurrence in breast cancer patients. Oncotarget (2016) 7:55231–48. doi: 10.18632/oncotarget.10485
205. Mihelich BL, Dambal S, Lin S, Nonn L. miR-182, of the miR-183 cluster family, is packaged in exosomes and is detected in human exosomes from serum, breast cells and prostate cells. Oncol Lett (2016) 12:1197–203. doi: 10.3892/ol.2016.4710
206. Hamdi K, Blancato J, Goerlitz D, Neili B, Abidi A, Gati A, et al. Circulating cell-free miRNA expression and its association with clinicopathologic features in inflammatory and non- inflammatory breast cancer. Asian (2016) 17:1801–10. doi: 10.7314/apjcp.2016.17.4.1801
207. Matamala N, Vargas MT, González-Cámpora R, Miñambres R, Arias JI, Menéndez P, et al. Tumor MicroRNA expression profiling identifies circulating MicroRNAs for early breast cancer detection. Clin Chem (2015) 61:1098–106. doi: 10.1373/clinchem.2015.238691
208. Li X-X, Gao S-Y, Wang P-Y, Zhou X, Li Y-J, Yu Y, et al. Reduced expression levels of let−7c in human breast cancer patients. Oncol Lett (2015) 9:1207–12. doi: 10.3892/ol.2015.2877
209. Mangolini A, Ferracin M, Zanzi MV, Saccenti E, Ebnaof SO, Poma VV, et al. Diagnostic and prognostic microRNAs in the serum of breast cancer patients measured by droplet digital PCR. biomark Res (2015) 3:12. doi: 10.1186/s40364-015-0037-0
210. Sahlberg KK, Bottai G, Naume B, Burwinkel B, Calin GA, Santarpia L. A serum MicroRNA signature predicts tumor relapse and survival in triple-negative breast cancer patients. Clin Cancer Res (2015) 21:1207–14. doi: 10.1158/1078-0432.CCR-14-2011
211. Ferracin M, Lupini L, Salamon I, Saccenti E, Vittoria M, Rocchi A, et al. Absolute quantification of cell-free microRNAs in cancer patients. Oncotarget (2015) 6:14545–55. doi: 10.18632/oncotarget.3859
212. Hagrass HA, Sharaf S, Pasha HF, Tantawy EA, Mohamed RH, Kassem R. Circulating microRNAs - A new horizon in molecular diagnosis of breast cancer. Genes Cancer (2015) 6:281–7. doi: 10.18632/genesandcancer.66
213. Toraih EA, Mohammed EA, Farrag S, Ramsis N. Pilot study of serum MicroRNA-21 as a diagnostic and prognostic biomarker in Egyptian breast cancer patients. Mol Diagn Ther (2015) 19:179–90. doi: 10.1007/s40291-015-0143-6
214. Zhang L, Xu Y, Jin X, Wang Z, Wu Y, Zhao D. A circulating miRNA signature as a diagnostic biomarker for non-invasive early detection of breast cancer. Breast Cancer Res Treat (2015) 154:423–34. doi: 10.1007/s10549-015-3591-0
215. Zhang H, Li B, Zhao H, Chang J. The expression and clinical significance of serum miR-205 for breast cancer and its role in detection of human cancers. Int J Clin Exp Med (2015) 8:3034–43.
216. Shaker O, Maher M, Nassar Y, Morcos G, Gad Z. Role of microRNAs -29b-2, –155, –197 and –205 as diagnostic biomarkers in serum of breast cancer females. Gene (2015) 560:77–82. doi: 10.1016/j.gene.2015.01.062
217. Magbanua MJM, Gumusay O, Kurzrock R, van ‘t Veer LJ, Rugo HS. Immunotherapy in breast cancer and the potential role of liquid biopsy. Front Oncol (2022) 12:802579. doi: 10.3389/fonc.2022.802579
218. Leary RJ, Sausen M, Kinde I, Papadopoulos N, John D, Craig D, et al. Detection of chromosomal alterations in the circulation of cancer patients with whole-genome sequencing. Sci Transl Med (2013) 4:162ra154. doi: 10.1126/scitranslmed.3004742
219. Chan HT, Chin YM, Nakamura Y, Low S-K. Clonal hematopoiesis in liquid biopsy: From biological noise to valuable clinical implications. Cancers (Basel) (2020) 12:2277. doi: 10.3390/cancers12082277
Keywords: liquid biopsy, breast cancer, miRNA, biomarker, biofluids
Citation: Liu X, Papukashvili D, Wang Z, Liu Y, Chen X, Li J, Li Z, Hu L, Li Z, Rcheulishvili N, Lu X and Ma J (2022) Potential utility of miRNAs for liquid biopsy in breast cancer. Front. Oncol. 12:940314. doi: 10.3389/fonc.2022.940314
Received: 10 May 2022; Accepted: 04 July 2022;
Published: 04 August 2022.
Edited by:
Elisa Frullanti, University of Siena, ItalyReviewed by:
Viola Bianca Serio, University of Siena, ItalyElena Lastraioli, University of Florence, Italy
Vasyl Chekhun, National Academy of Sciences of Ukraine (NAN Ukraine), Ukraine
Copyright © 2022 Liu, Papukashvili, Wang, Liu, Chen, Li, Li, Hu, Li, Rcheulishvili, Lu and Ma. This is an open-access article distributed under the terms of the Creative Commons Attribution License (CC BY). The use, distribution or reproduction in other forums is permitted, provided the original author(s) and the copyright owner(s) are credited and that the original publication in this journal is cited, in accordance with accepted academic practice. No use, distribution or reproduction is permitted which does not comply with these terms.
*Correspondence: Xiaoqing Lu, luxiaoqing860227@163.com; Jinfeng Ma, mjinfeng99@163.com
†These authors have contributed equally to this work and share first authorship