- 1Department of Biotechnological and Applied Clinical Sciences, University of L’Aquila, L’Aquila, Italy
- 2Center for Molecular Diagnostics and Advanced Therapies, University of L’Aquila, L’Aquila, Italy
Extracellular vesicles (EVs), defined as intercellular messengers that carry their cargos between cells, are involved in several physiological and pathological processes. These small membranous vesicles are released by most cells and contain biological molecules, including nucleic acids, proteins and lipids, which can modulate signaling pathways of nearby or distant recipient cells. Exosomes, one the most characterized classes of EVs, include, among others, microRNAs (miRNAs), small non-coding RNAs able to regulate the expression of several genes at post-transcriptional level. In cancer, exosomal miRNAs have been shown to influence tumor behavior and reshape tumor microenvironment. Furthermore, their possible involvement in drug resistance mechanisms has become evident in recent years. Hepatocellular carcinoma (HCC) is the major type of liver cancer, accounting for 75-85% of all liver tumors. Although the improvement in HCC treatment approaches, low therapeutic efficacy in patients with intermediate-advanced HCC is mainly related to the development of tumor metastases, high risk of recurrence and drug resistance. Exosomes have been shown to be involved in pathogenesis and progression of HCC, as well as in drug resistance, by regulating processes such as cell proliferation, epithelial-mesenchymal transition and immune response. Herein, we summarize the current knowledge about the involvement of exosomal miRNAs in HCC therapy, highlighting their role as modulators of therapeutic response, particularly chemotherapy and immunotherapy, as well as possible therapeutic tools.
Introduction
Liver tumor is one of the most common types of cancers (1), displaying the 7th highest age-adjusted incidence rate in the world. Hepatocellular carcinoma (HCC) is considered the most frequent liver neoplasm (1) and the third most common cause of cancer-related death worldwide (2), predominantly in Asian countries, due to late diagnosis and lack of effective surveillance programs for high-risk people. In this context, several non-invasive diagnostic biomarkers are considered, but, unfortunately, they do not reach necessary sensitivity and specificity, especially for early stage-HCC (3). On the other hand, liver biopsy is a limited procedure due to its invasiveness, and imaging methods (e.g. ultrasonography, magnetic resonance and/or computed tomography) are usually utilized for diagnosis, even though small tumors can be often missed (4, 5).
HCC initiation, development and progression are dependent both on intrinsic (e.g. gene mutations) and extrinsic (e.g. viral infections, western type diet intake, alcohol consumption) factors able to induce in liver cells the typical responses of malignant transformation, leading to apoptosis evasion, cell proliferation and survival, neovascularization (4). In particular, non-alcoholic fatty liver disease (NAFLD) is now considered the most important liver chronic disease (6) and it has been shown that, among HCC predisposing factors, not only high-fat (7), but also high-carbohydrate/western type diet can induce disease progression up to tumor formation in a NAFLD/NASH mouse model (8–10). The progression of the disease includes a passage through a cirrhotic stage in a large majority of HCC cases (up to 90%) (11). Oxidative damage (12), inflammation (13), hepatocyte compensatory regeneration (14), with consequent accumulation of gene mutations, are typical HCC features. Mutational HCC landscape includes many genes with different mutation frequency, such as TP53 (30%), CTNNB1/β-catenin (26%), ARID1A (8%), ARID2 (6%), AXIN (6%) (15). Several pathways, such TGF-β, Wnt/β-catenin, Hedgehog, Notch, EGF, HGF, VEFG, JAK/STAT, Hippo, and HIF are dysregulated and play a crucial role in HCC, leading to uncontrolled cell division and metastasis. For some of them, small promising molecules for therapeutic approaches are under investigation (16).
To date, the main approach in HCC management is radiofrequency ablation (RFA), surgical resection or liver transplantation, if feasible, and outcome of patients untreatable with resection curative methods is dependent on the response to the currently available therapies. Novel treatments, principally based on sorafenib and regorafenib, two tyrosine kinases inhibitors (TKIs) seem, however, to induce a moderate increase of HCC patients’ survival (17). MicroRNAs (miRNAs) are short non-coding RNA molecules able to regulate gene expression at the post-transcriptional level (18), playing a pivotal role in high-impact disorders, including neurodegenerative (19), cardiovascular (20) diseases and cancer (21, 22). They work by fine-tuning key physiological and pathophysiological processes, such as proliferation, survival, apoptosis, invasion, angiogenesis, epithelial-mesenchymal transition (EMT), metastasis and resistance to therapeutic treatments as well (23, 24). MiRNAs dysregulated expression levels are described both in tumor tissues and serum/plasma, where they are included in macromolecular protein complexes (25–27) or encapsulated in microvesicles/exosomes (28, 29) to be protected from endogenous RNase, being so easily detectable and quantifiable. Such properties allowed the identification of miRNAs as potential diagnostic, prognostic and predictive cancer biomarkers. Furthermore, aberrant miRNA expression in cancer led to the characterization of oncomiRs and tumor-suppressor miRs, playing a role in promoting or suppressing oncogenesis, respectively. The possibility to synthesize and obtain molecules able to specifically repristinate physiological conditions (i.e. antagomiRs inhibiting oncomiRs, mimics replacing tumor suppressor miRs) made these molecules of great interest for innovative cancer therapeutic strategies (30). Furthermore, several miRNAs were described as biomarkers for therapy response and disease-free survival/clinical progression in HCC patients (31–34).
In this review, we report recent advances on exosomal miRNAs in HCC, by focusing on their involvement and role in therapeutic responses.
MiRNA biogenesis
In the canonical pathway, microRNAs are transcribed, from intergenic or intragenic genomic regions, by Polymerase II in the nucleus, thus originating long double stranded-hairpin primary transcripts (pri-miR). Subsequently, RNase III enzyme Drosha, associated to RNA binding protein (RBP) DGCR8 (DiGeorge critical region 8), cleaves pri-miRNAs to generate pre-miRNAs (60-100 nucleotides in length hairpin precursors) which are subsequently transferred to the cytoplasm by Exportin 5 through a Ran (Ras-related nuclear protein)-GTPase-dependent mechanism. There, pre-miRNAs are cleaved again by RNase enzyme Dicer, linked to the trans-activation-responsive RNA-binding protein (TRBP), to produce mature double-stranded miRNAs. Mature miRNAs associate to a member of Argonaute family (Ago1-2-3-4 paralogs in mammals) thus generating the ribonucleoprotein miRNA-induced silencing complex (miRISC). Two main mechanisms for miRNA-mediated regulation are described through the interaction between the seed region and specific partially or perfectly complementary microRNA responsive elements (MREs), mainly located at the level of target mRNA 3’-UTR, with consequent translation repression or mRNA decay by deadenylation followed by decapping, respectively (18, 35, 36). A DICER-independent mechanism has been described as well for pre-miR-451, involved in erythropoiesis. This is due to the stem-loop structure, too short to be processed by DICER. In this case, miRNA’s maturation requires direct loading into Ago2 and subsequent cleavage by its catalytic centre (37).
Exosomes
Cells can secrete different types of extracellular vesicles (EVs). This feature is conserved from bacteria up to higher organisms (38, 39), and it was originally intended to discard unwanted or unnecessary molecules (40). However, it is known that EVs are involved also in exchanging nucleic acids, lipids and proteins among cells; moreover, they play a role in favouring intercellular communication, at the level of both physiological and pathophysiological processes (41). Three main types of EVs have been described: exosomes, microvesicles and apoptotic bodies which differ based on their biogenesis and release mechanisms, content, size and role (42, 43),.
Exosomes are nano-sized biovesicles (diameter 30-150 nm) secreted by all cell types. They can be detected in most of body fluids and are delimited by a lipid bilayer membrane which protects and aids to deliver cargos to recipient cells (44). Exosome biogenesis occurs as a part of membrane-trafficking processes: cargos are insourced and distributed into early endosomes at the level of endosomal system. Subsequently, late endosomes/multivesicular bodies (MVBs), containing intraluminal vesicles (ILVs), are generated from early endosomes. ILVs can sequester lipids, proteins and other cargos from cytosolic compartments and Golgi apparatus. After, MVBs containing cargos are driven at the level of the plasma membrane, where they merge with it, so that ILVs are released, as exosomes as an outward budding (43, 45, 46) (Figure 1).
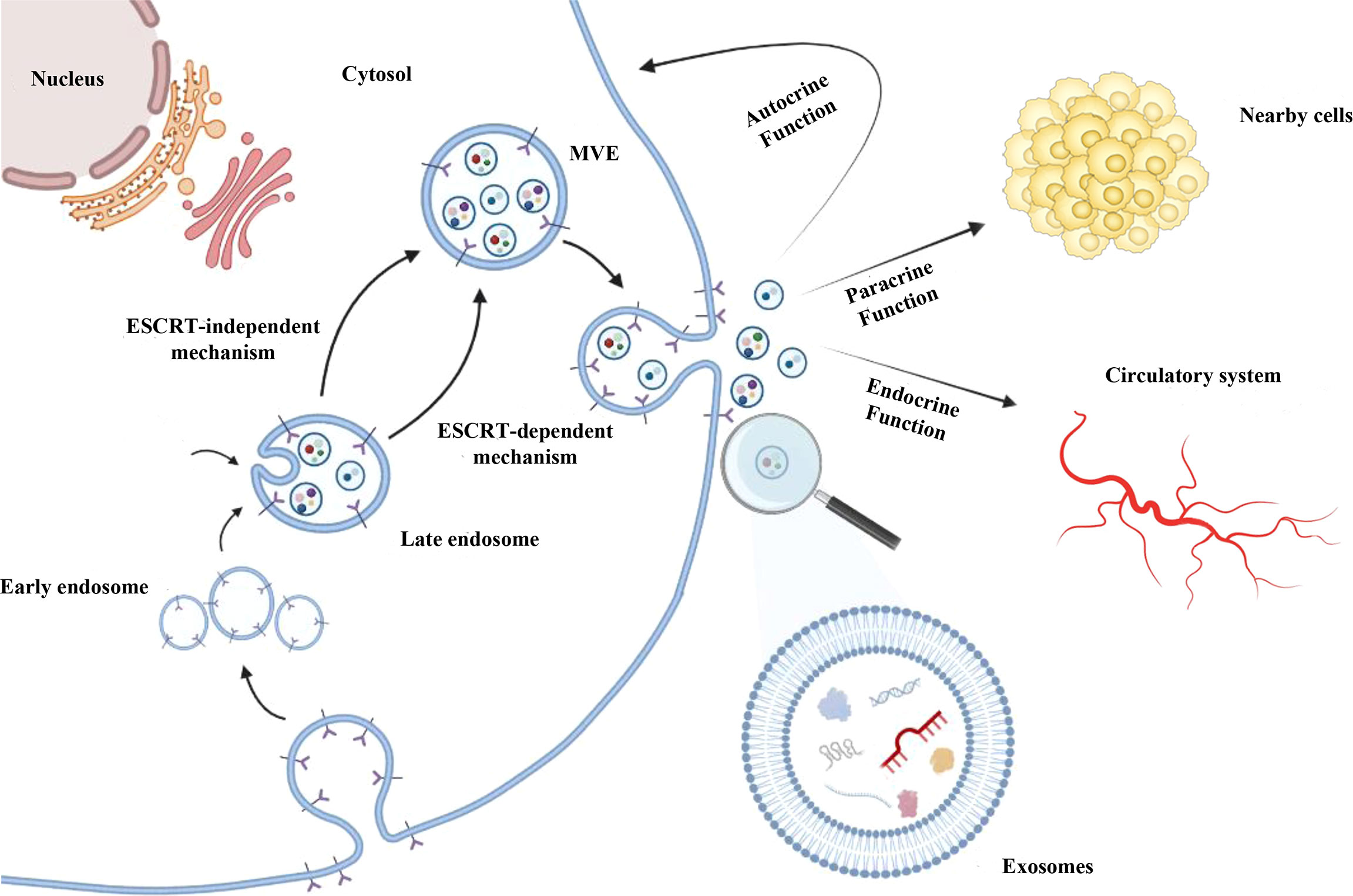
Figure 1 Exosomes biogenesis. After being generated by endocytosis at the level of endosomal system, exosomes are included as invagination within the multivesicular endosomes (MVEs) lumen and released as an outward budding upon the fusion of MVEs with the cell membrane. MVEs formation can occur mainly by endosomal sorting complex required for transport (ESCRT) pathway-dependent mechanism; ESCRT-independent mechanisms were also described. Exosomes can contain different cargos, such as proteins, lipids, DNA, mRNAs, lnRNAs and miRNAs. Exosomes are uptake by recipient cells thus favouring intercellular communication through their autocrine, paracrine and/or endocrine function.
The MVBs formation seems to be dependent also on stimulation by growth factors (47) and can occur mainly by endosomal sorting complex required for transport (ESCRT) pathway-dependent mechanisms (48). In addition, ESCRT-independent mechanisms have been described for MVBs formation (49–51) since it was demonstrated that MVBs can still form after depletion of ESCRT components (52). ESCRT (ESCRT-0/I/II/III) and accessory (e.g. Alix, TSG101, HSC70, HSP70) proteins are contained in exosomes following ESCRT-dependent MVEs generation, irrespective of the cell types, and for this reason they are considered as exosomal markers (42, 43, 53). On the other hand, in ESCRT-independent mechanism, other molecules (e.g. tetraspanins, CD63, CD9, CD81) are commonly found in exosomes, but also detected in other types of vesicles, such as MVs (54, 55). Based on information reported by some databases, exosomes can contain more than 8,000 proteins and 190 lipids. Integrins, tetraspanins, MHC-II complex proteins are described in the exosomal membrane, whereas other (CD55, trombospondin, ALIX, lactadherin) are included into exosomes during the biogenesis (56).
Exosome can load different and tissue-specific cargos, such as proteins, nucleic acids, lipids and metabolites, depending on the type of cell from which they are produced. Following their uptake by recipient cells, via exosomal fusion or endocytosis, they provide autocrine, paracrine and endocrine functions, thus favouring intercellular communications (55, 57) (Figure 1), participating in cell homeostasis (58), stimulating immune responses (59), promoting tissue repairing (60), cell survival (61), and modulating angiogenesis (62). For these reasons, they are considered as crucial modulators of intercellular cross-talks at the base of several high-impact diseases (63–65), including cancer.
Exosomal miRNAs in HCC
Exosomal miRNAs have been shown to regulate tumor behavior and reshape tumor microenvironment (TME). Increasing evidence indicates that exosomal miRNAs contribute to HCC pathogenesis and progression by regulating processes such as cell proliferation, metastasis and immune response (66), thus showing considerable potential as diagnostic, predictive and prognostic biomarkers as well as new therapeutic tools (67). Table 1 shows a comprehensive overview of exosomal miRNAs and related functions in HCC development and progression, based on the most recent literature. The utility of exosomes as vectors of biological therapeutic agents, such as miRNAs, has been actively explored in HCC (67). In the following sections, we will discuss, among those reported in Table 1 the most relevant miRNAs described as mediators of therapeutic response and/or putative treatment tools in HCC.
Exosomal miRNAs as modulators of therapeutic response
Several studies have described the ability of exosomal miRNAs to modulate HCC therapeutic response to different drugs, such as sorafenib, 5-fluorouracil (5-FU) and doxorubicin, or immunotherapy (107, 108). Experimental evidence suggests the involvement of exosomal miRNAs both in drug resistance mechanisms and in drug sensitivity improvement.
Lou et al. observed that miR-122-enriched exosomes, obtained from adipose tissue-derived mesenchymal stem cells (AMSC) after transfection with miR-122 expression plasmids, inhibited HCC cell proliferation and increased sensitivity to 5-FU and sorafenib both in vitro and in xenograft mouse models, by targeting and downregulating the expression of CCNG1, ADAM10 and IGF1R, genes involved in tumorigenesis and drug sensitivity in several cancer types. Thus, the authors highlighted the potential use of exosomal miR-122 to improve therapeutic response and revert drug resistance (71).
Similarly, exosomal miR-744 was able to rescue sorafenib sensitivity in resistant HepG2 cancer cells, by targeting PAX2, involved in the regulation of chemotherapy response in several cancers (88). Decreased level of miR-744 was found in HCC tissues, in exosomes from patients’ sera and HCC cells resistant to sorafenib: the restoration of miR-744 expression in HepG2 cancer cells and the subsequent release of miR-744-enriched exosomes led to decreased miR-744-induced cell proliferation and resistance to therapy (88).
On the contrary, miR-32-5p was found to contribute to multidrug resistance in HCC cells (94). The authors observed that exosomes from multidrug-resistant Bel/5-FU cells were able to deliver miR-32-5p into sensitive Bel7402 cells, thus inducing angiogenesis, EMT and drug resistance through PI3K/AKT pathway activation. Increased levels of exosomal miR-32-5p were also found in sera from HCC patients, associated with poor prognosis (94).
Resistance to chemotherapy, is one of the main factors responsible for high mortality rate in HCC patients, and the identification of mechanisms underlying chemoresistance as well as the enhancement of therapeutic options is of great clinical interest. In this context, the possible role of the exosomal tumor suppressor miR-199a-3p was recently explored in two studies (78, 79).
Lou et al. showed that exosome-mediated crosstalk between adipose tissue-derived mesenchymal stem cells (AMSCs) hyper-expressing miR-199a-3p and HCC cells increased the tumor sensitivity to doxorubicin by targeting mTOR signaling pathway. In vivo experiments, based on AMSC-Exo-199a injection into a HCC mouse model, confirmed increased doxorubicin anti-tumor effect in HCC (78).
Likewise, Zhang et al. observed that exo-miR-199a-3p restored sensitivity to cisplatin (DDP) and decreased tumor growth in chemo-resistant HCC cells. Due to the ability of exo-miR-199a-3p to overturn DDP resistance, the authors highlighted its great potential as an alternative therapeutic option in DDP-refractory HCC (79).
In a study aimed at elucidating the possible mechanism by which hepatitis B core antigen (HBc) promotes doxorubicin resistance in HCC, Wei et al. suggested that HBc led to upregulation of exosomal miR-135a-5p inducing cell proliferation, anti-apoptotic effects, and drug resistance. VAMP2 was identified as a novel miR-135a target, and its level decrease was linked to cell proliferation, apoptosis escape and drug resistance, thus identifying the miR-135a-5p/VAMP2 axis as a key regulatory chemo-resistance mechanism in HCC (99).
Exosomal miR-451a acts as tumor suppressor miRNA and its expression is down-regulated in HCC (84). Xu et al. used human umbilical cord mesenchymal stem cells (hucMSCs) derived exosomes to treat Hep3B cells and assess paclitaxel resistance. The authors demonstrated that exosomal miR-451 slowed EMT progression and reduced proliferation, migration and resistance to paclitaxel by suppressing ADAM10 in HCC cells, thus acting as a chemosensitivity-inducing factor and promoting HCC cell apoptosis (85).
Semaan et al. reported that the use of exosomal miR-214 from human cerebral endothelial cell-derived exosomes (hCEC-Exo-214) in combination with oxaliplatin or sorafenib could effectively reduce cancer cell viability and invasion of HepG2 and Hep3B cells compared to monotherapy. At the molecular level, this effect seems to be mediated by the glycoprotein P-gp and the splicing factor SF3B3 (81).
Inhibiting the release of exosomal oncomiRs, or increasing the effect of tumor suppressor miRNAs, could also play a synergic role in immunotherapy: for example, it has been shown that the inhibition of miR-23a-3p in HCC cancer cells could prevent their exosomal release and the consequent expression of PD-L1 in macrophages (92).
Similarly, it has been observed that high VEGF expression, in response to hypoxia, in TME can have immunosuppressive effect in tumors, resulting in decreased efficacy of PD-L1 and PD-1 inhibitor drugs (109, 110). Therefore, inhibition of VEGF and other hypoxia-induced factors, such as exosomal miRNAs involved in the regulation of angiogenesis in TME, could improve the efficacy of current immunotherapies (111). In this context, other studies led to hypothesize that targeting specific exosomal miRNAs, released from HCC cells and able to stimulate angiogenesis and HCC proliferation, such as miR-210 and miR-155, could therefore interfere with cellular crosstalk that promotes angiogenesis, further improving therapy (100, 102).
Exosomal miRNAs as main therapeutic tools
Exosomes naturally act as carriers of nucleic acids, proteins and lipids from donor to recipient cells. They are characterized by high biocompatibility, low immunogenicity, low toxicity and ability to cross the blood-brain barrier. These features make them promising vehicles for the delivery of chemical and biological drugs (107). In this section, we focus on exosomal miRNAs described for their potential application as main biological therapeutic agents in HCC.
Zhang et al. observed that exosomes released from cancer-associated fibroblasts (CAFs) overexpressing miR-320a were able to transfer this miRNA into HCC cells and suppressed HCC cell proliferation and metastasis both in vitro and in vivo by targeting PBX3 (82).
Through the same experimental approach, Wang et al. demonstrated that CAFs-derived miR-335-5p-enriched exosomes could inhibit HCC cell proliferation and invasion, by regulating genes including CDC42, CDK2, CSNK1G2, EIF2C2, EIF5, LIMaK1, NRG1, PLK2, RGS19, TCF3, THBS1, YBX1, and ZMYND8 (83).
Xiong et al. showed that stimulation of mast cell with hepatitis C virus E2 envelope glycoprotein (HCV-E2) resulted in miR-490 expression increase in mast cells as well as in secreted exosomes. Furthermore, the delivery of Exo-miR-490 to HCC recipient cells inhibited the ERK1/2 pathway, thereby suppressing cell migration and metastasis (86).
MiR-21 is a well characterized oncomiR, involved in cell proliferation and metastases through the inhibition of genes such as PTEN (112), PDD4, RECK, and SULF-1 (113). The ability of miR-21 to confer resistance to chemotherapy in cancer cells has also been reported (112, 114).
Zhou et al. showed the ability of HCC-derived exosomal miR-21 to convert hepatocyte stellate cells (HSCs) to CAFs, resulting in angiogenesis promotion through increased secretion of VEGF, MMP2, MMP9, bFGF and TGF-β by CAFs. Results of this study provided further insight into the crosstalk between cancer cells and their microenvironment during tumor progression, providing new information of potential clinical utility in HCC (90).
Interestingly, Liang et al. used nanoparticles loaded with small interfering RNA (siRNA) to downregulate the expression of the pro-oncogenic factor Sphk2 in HCC cells to reduce exosomal miR-21, thus decreasing tumor cell migration and exosome-mediated tumorigenic function. The anti-tumor effect of Sphk2 siRNA was also demonstrated in a xenograft mouse model resulting in reduced HCC tumor progression. Therefore, targeting exosomal oncomiR secretion could represent a new therapeutic strategy (91).
Moh-Moh-Aung et al. reported that the downregulation of exosomal miR-200b-3p in HCC cells led to the promotion of angiogenesis through endothelial ERG expression increase, thus providing new insights into possible targetable mechanisms to improve the efficacy of anti-angiogenic therapies (80).
Immune cells play a key role in tumorigenic process, therefore the collection and possible engineering of exosomes from these cell types might represent an anti-tumor strategy that requires further investigation.
It has been shown that exosomal miR-142 and miR-223, transferred from tumor associated macrophages (TAMs) to HCC cells, can suppress cancer cell proliferation through the modulation of genes involved in cell cycle regulation, such as the miR-223 target gene STMN1 (73).
Furthermore, the use of the intravenous anaesthetic propofol, induced the secretion of miR-142-3p-enriched exosomes from TAMs, and the internalization of these vesicles into HCC cells led to the inhibition of cell invasion in vitro and tumor growth in vivo through down-regulation of miR-142-3p target gene RAC1 (74).
Liang et al. described an alternative approach to deliver antioncomiRs-enriched exosomes to HCC cells. In their study, HEK293T cells were engineered to secrete exosomes actively loaded with miR-26a by electroporation. These exosomes were able to selectively target HepG2 cells, thus decreasing cancer cell migration and proliferation in vitro though the inhibition of key cell cycle regulators such as CCND2, CCNE2, CDK6 (69).
The same approach was described by Pomatto et al. who used renal carcinoma endothelial instead of HEK293T cells, loaded with tumor suppressor miR-31 and miR-451a able to induce chemosensitivity (70). Overall, results of these two studies highlight the interesting possibility of using engineered exosomes as therapeutic agents.
Conclusion
MiRNAs are considered pivotal modulators of intercellular crosstalk and miRNA transfer via exosomes has been described as one of the possible strategies by which resistant HCC cells can share their resistance with neighbouring cells, thus hindering therapies.
In this context, based on the results principally obtained from in vitro and in vivo models, exosomes can be also considered as promising vehicles of miRNAs for therapeutic purposes, representing a great resource for the design of new treatment strategies with potential efficacy, especially in combination with chemotherapy, TKIs or immunotherapy, currently considered as a cutting-edge cancer treatment.
In addition to exosomal miRNAs directly targeting HCC cells, an interesting alternative could also be HCC tumor microenvironment targeting, in terms of CAFs, immune cells or tumor endothelial cells, on which exosomal miRNAs could induce desirable responses.
Furthermore, technological advances, focused for example on vesicles and donor cells engineering, offer an unprecedented opportunity to improve and provide novel tools for potential therapeutic applications of exosomal miRNAs in HCC and other types of cancers.
Overall, although more in-depth studies to elucidate the exact biological role and possible applicability of exosomal miRNAs in HCC treatment are required, these mediators can represent promising factors of potential therapeutic utility in HCC patients.
Author contributions
VZ, CC, and AT: original draft preparation; VZ, CC, RC, and AC: Bibliographic information; MDVN and FZ: reading and editing manuscript; EA and AT: conceptualization, review and editing manuscript. All authors contributed to the article and approved the submitted version.
Conflict of interest
The authors declare that the research was conducted in the absence of any commercial or financial relationships that could be construed as a potential conflict of interest.
Publisher’s note
All claims expressed in this article are solely those of the authors and do not necessarily represent those of their affiliated organizations, or those of the publisher, the editors and the reviewers. Any product that may be evaluated in this article, or claim that may be made by its manufacturer, is not guaranteed or endorsed by the publisher.
Acknowledgments
VZ is supported by PON-AIM 2014–2020 Research and Innovation funding and by grants from Carispaq Foundation L’Aquila 2020 and intramural “DISCAB GRANT 2022 code 07_DG_2022_25” awarded by the Department of Biotechnological and Applied Clinical Sciences, University of L’Aquila. AC is supported by PON 2014–2020 Research and Innovation PhD program.
References
1. Stuver S, Trichopoulos D. Cancer of the liver and biliary tract. In: Adami HO, Hunter D, Trichopoulos D, editors. Textbook of cancer epidemiology, 2nd. New York:Oxford University Press (2008).
2. World Health Organization, I.A.f.R.o.C. Estimated cancer incidence, mortality and prevalence worldwide in 2012. Available at: http://www.globocan.iarc.fr/Pages/fact_sheets_population.aspx.
3. Pan Y, Chen H, Yu J. Biomarkers in hepatocellular carcinoma: Current status and future perspectives. Biomedicines (2020) 8(12):576. doi: 10.3390/biomedicines8120576
4. Forner A, Reig M, Bruix J. Hepatocellular carcinoma. Lancet (2018) 391(10127):1301–14. doi: 10.1016/S0140-6736(18)30010-2
5. Schraml C, Kaufmann S, Rempp H, Syha R, Ketelsen D, Notohamiprodjo M, et al. Imaging of HCC-current state of the art. Diagn (Basel) (2015) 5(4):513–45. doi: 10.3390/diagnostics5040513
6. Ge X, Zheng L, Wang M, Du Y, Jiang J. Prevalence trends in non-alcoholic fatty liver disease at the global, regional and national levels, 1990-2017: a population-based observational study. BMJ Open (2020) 10(8):e036663. doi: 10.1136/bmjopen-2019-036663
7. Pedersen KB, Pulliam CF, Patel A, Del Piero F, Watanabe TTN, Wankhade UD, et al. Liver tumorigenesis is promoted by a high saturated fat diet specifically in male mice and is associated with hepatic expression of the proto-oncogene Agap2 and enrichment of the intestinal microbiome with coprococcus. Carcinogenesis (2019) 40(2):349–59. doi: 10.1093/carcin/bgy141
8. Basaranoglu M, Basaranoglu G, Bugianesi E. Carbohydrate intake and nonalcoholic fatty liver disease: fructose as a weapon of mass destruction. Hepatobil Surg Nutr (2015) 4(2):109–16. doi: 10.3978/j.issn.2304-3881.2014.11.05
9. Tessitore A, Mastroiaco V, Vetuschi A, Sferra R, Pompili S, Cicciarelli G, et al. Development of hepatocellular cancer induced by long term low fat-high carbohydrate diet in a NAFLD/NASH mouse model. Oncotarget (2017) 8(32):53482–94. doi: 10.18632/oncotarget.18585
10. Pompili S, Vetuschi A, Gaudio E, Tessitore A, Capelli R, Alesse E, et al. Long-term abuse of a high-carbohydrate diet is as harmful as a high-fat diet for development and progression of liver injury in a mouse model of NAFLD/NASH. Nutrition (2020) 110782:75–6. doi: 10.1016/j.nut.2020.110782
11. Ghouri YA, Mian I, Rowe JH. Review of hepatocellular carcinoma: Epidemiology, etiology, and carcinogenesis. J Carcinog (2017) 16:1. doi: 10.4103/jcar.JCar_9_16
12. Brahma MK, Gilglioni EH, Zhou L, Trépo E, Chen P, Gurzov EN. Oxidative stress in obesity-associated hepatocellular carcinoma: sources, signaling and therapeutic challenges. Oncogene (2021) 40(33):5155–67. doi: 10.1038/s41388-021-01950-y
13. Yu LX, Ling Y, Wang HY. Role of nonresolving inflammation in hepatocellular carcinoma development and progression. NPJ Precis Oncol (2018) 2(1):6. doi: 10.1038/s41698-018-0048-z
14. Holczbauer Á, Wangensteen KJ, Shin S. Cellular origins of regenerating liver and hepatocellular carcinoma. JHEP Rep (2021) 4(4):100416. doi: 10.1016/j.jhepr.2021.100416
15. Yim SY, Lee JS. An overview of the genomic characterization of hepatocellular carcinoma. J Hepatocell Carcinoma (2021) 8:1077–88. doi: 10.2147/JHC.S270533
16. Farzaneh Z, Vosough M, Agarwal T, Farzaneh M. Critical signaling pathways governing hepatocellular carcinoma behavior; small molecule-based approaches. Cancer Cell Int (2021) 21(1):208. doi: 10.1186/s12935-021-01924-w
17. Marin JJG, Macias RIR, Monte MJ, Romero MR, Asensio M, Sanchez-Martin A, et al. Molecular bases of drug resistance in hepatocellular carcinoma. Cancers (Basel) (2020) 12(6):1663. doi: 10.3390/cancers12061663
18. O’Brien J, Hayder H, Zayed Y, Peng C. Overview of MicroRNA biogenesis, mechanisms of actions, and circulation. Front Endocrinol (Lausanne) (2018) 9:402. doi: 10.3389/fendo.2018.00402
19. Juźwik CA, Drake S S, Zhang Y, Paradis-Isler N, Sylvester A, Amar-Zifkin A, et al. microRNA dysregulation in neurodegenerative diseases: A systematic review. Prog Neurobiol (2019) 182:101664. doi: 10.1016/j.pneurobio.2019.101664
20. Zhou SS, Jin JP, Wang JQ, Zhang ZG, Freedman JH, Zheng Y, et al. miRNAS in cardiovascular diseases: potential biomarkers, therapeutic targets and challenges. Acta Pharmacol Sin (2018) 39(7):1073–84. doi: 10.1038/aps.2018.30
21. Peng Y, Croce CM. The role of MicroRNAs in human cancer. Signal Transduct Target Ther (2016) 1:15004. doi: 10.1038/sigtrans.2015.4
22. Xu X, Tao Y, Shan L, Chen R, Jiang H, Qian Z, et al. The role of MicroRNAs in hepatocellular carcinoma. J Cancer (2018) 9(19):3557–69. doi: 10.7150/jca.26350
23. Hanna J, Hossain GS, Kocerha J. The potential for microRNA therapeutics and clinical research. Front Genet (2019) 10:478. doi: 10.3389/fgene.2019.00478
24. Liu QW, He Y, Xu WW. Molecular functions and therapeutic applications of exosomal noncoding RNAs in cancer. Exp Mol Med (2022) 54(3):216–25. doi: 10.1038/s12276-022-00744-w
25. Arroyo JD, Chevillet JR, Kroh EM, Ruf IK, Pritchard CC, Gibson DF, et al. Argonaute2 complexes carry a population of circulating microRNAs independent of vesicles in human plasma. Proc Natl Acad Sci U.S.A. (2011) 108(12):5003–8. doi: 10.1073/pnas.1019055108
26. Li L, Zhu D, Huang L, Zhang J, Bian Z, Chen X, et al. Argonaute 2 complexes selectively protect the circulating microRNAs in cell-secreted microvesicles. PloS One (2012) 7(10):e46957. doi: 10.1371/journal.pone.0046957
27. Vickers KC, Palmisano BT, Shoucri BM, Shamburek RD, Remaley AT. MicroRNAs are transported in plasma and delivered to recipient cells by high-density lipoproteins. Nat Cell Biol (2011) 13(4):423–33. doi: 10.1038/ncb2210
28. Sun Z, Shi K, Yang S, Liu J, Zhou Q, Wang G, et al. Effect of exosomal miRNA on cancer biology and clinical applications. Mol Cancer (2018) 17(1):147. doi: 10.1186/s12943-018-0897-7
29. Ghosh S, Bhowmik S, Majumdar S, Goswami A, Chakraborty J, Gupta S, et al. The exosome encapsulated microRNAs as circulating diagnostic marker for hepatocellular carcinoma with low alpha-fetoprotein. Int J Cancer (2020) 147(10):2934–47. doi: 10.1002/ijc.33111
30. Shah MY, Ferrajoli A, Sood AK, Lopez-Berestein G, Calin GA. microRNA therapeutics in cancer - an emerging concept. EBioMedicine (2016) 12:34–42. doi: 10.1016/j.ebiom.2016.09.017
31. Vaira V, Roncalli M, Carnaghi C, Faversani A, Maggioni M, Augello C, et al. MicroRNA-425-3p predicts response to sorafenib therapy in patients with hepatocellular carcinoma. Liver Int (2015) 35(3):1077–86. doi: 10.1111/liv.12636
32. Kohno T, Morishita A, Iwama H, Fujita K, Tani J, Takuma K, et al. Comprehensive analysis of circulating microRNAs as predictive biomarkers for sorafenib therapy outcome in hepatocellular carcinoma. Oncol Lett (2020) 20(2):1727–33. doi: 10.3892/ol.2020.11696
33. Pratama MY, Visintin A, Crocè LS, Tiribelli C, Pascut D. Circulatory miRNA as a biomarker for therapy response and disease-free survival in hepatocellular carcinoma. Cancers (Basel) (2020) 12(10):2810. doi: 10.3390/cancers12102810
34. Ratnasari N, Lestari P, Renovaldi D, Ningsih JR, Qoriansas N, Wardana T, et al. Potential plasma biomarkers: miRNA-29c, miRNA-21, and miRNA-155 in clinical progression of hepatocellular carcinoma patients. PloS One (2022) 17(2):e0263298. doi: 10.1371/journal.pone.0263298
35. Gu S, Kay MA. How do miRNAs mediate translational repression? Silence (2010) 1(1):11. doi: 10.1186/1758-907X-1-11
36. Frédérick PM, Simard MJ. Regulation and different functions of the animal microRNA-induced silencing complex. Wiley Interdiscip Rev RNA (2021):e1701. doi: 10.1002/wrna.1701
37. Cheloufi S, Dos Santos CO, Chong MM, Hannon GJ. A dicer-independent miRNA biogenesis pathway that requires ago catalysis. Nature (2010) 465(7298):584–9. doi: 10.1038/nature09092
38. Toyofuku M, Nomura N, Eberl L. Types and origins of bacterial membrane vesicles. Nat Rev Microbiol (2019) 17(1):13–24. doi: 10.1038/s41579-018-0112-2
39. Tartaglia NR, Nicolas A, Rodovalho VR, Luz BSRD, Briard-Bion V, Krupova Z, et al. Extracellular vesicles produced by human and animal staphylococcus aureus strains share a highly conserved core proteome. Sci Rep (2020) 10(1):8467. doi: 10.1038/s41598-020-64952-y
40. Johnstone RM, Adam M, Hammond JR, Orr L, Turbide C. Vesicle formation during reticulocyte maturation. association of plasma membrane activities with released vesicles (exosomes). J Biol Chem (1987) 262(19):9412–20.
41. Yuana Y, Sturk A, Nieuwland R. Extracellular vesicles in physiological and pathological conditions. Blood Rev (2013) 27(1):31–9. doi: 10.1016/j.blre.2012.12.002
42. van Niel G, D’Angelo G, Raposo G. Shedding light on the cell biology of extracellular vesicles. Nat Rev Mol Cell Biol (2018) 19(4):213–28. doi: 10.1038/nrm.2017.125
43. Doyle LM, Wang MZ. Overview of extracellular vesicles, their origin, composition, purpose, and methods for exosome isolation and analysis. Cells (2019) 8(7):727. doi: 10.3390/cells8070727
44. Sato YT, Umezaki K, Sawada S, Mukai SA, Sasaki Y, Harada N, et al. Engineering hybrid exosomes by membrane fusion with liposomes. Sci Rep (2016) 6:21933. doi: 10.1038/srep21933
45. Gurung S, Perocheau D, Touramanidou L, Baruteau J. The exosome journey: from biogenesis to uptake and intracellular signalling. Cell Commun Signal (2021) 19(1):47. doi: 10.1186/s12964-021-00730-1
46. Zhang Y, Liu Y, Liu H, Tang WH. Exosomes: biogenesis, biologic function and clinical potential. Cell Biosci (2019) 9:19. doi: 10.1186/s13578-019-0282-2
47. White IJ, Bailey LM, Aghakhani MR, Moss SE, Futter CE. EGF stimulates annexin 1-dependent inward vesiculation in a multivesicular endosome subpopulation. EMBO J (2006) 25(1):1–12. doi: 10.1038/sj.emboj.7600759
48. Wollert T, Hurley JH. Molecular mechanism of multivesicular body biogenesis by ESCRT complexes. Nature (2010) 464(7290):864–9. doi: 10.1038/nature08849
49. Woodman PG, Futter CE. Multivesicular bodies: co-ordinated progression to maturity. Curr Opin Cell Biol (2008) 20(4):408–14. doi: 10.1016/j.ceb.2008.04.001
50. Babst M. MVB vesicle formation: ESCRT-dependent, ESCRT-independent and everything in between. Curr Opin Cell Biol (2011) 23(4):452–7. doi: 10.1016/j.ceb.2011.04.008
51. Stoorvogel W. Resolving sorting mechanisms into exosomes. Cell Res (2015) 25(5):531–2. doi: 10.1038/cr.2015.39
52. Stuffers S, Sem Wegner C, Stenmark H, Brech A. Multivesicular endosome biogenesis in the absence of ESCRTs. Traffic (2009) 10(7):925–37. doi: 10.1111/j.1600-0854.2009.00920.x
53. Mallia A, Gianazza E, Zoanni B, Brioschi M, Barbieri SS, Banfi C. Proteomics of extracellular vesicles: Update on their composition, biological roles and potential use as diagnostic tools in atherosclerotic cardiovascular diseases. Diagn (Basel) (2020) 10(10):843. doi: 10.3390/diagnostics10100843
54. Willms E, Cabañas C, Mäger I, Wood MJA, Vader P. Extracellular vesicle heterogeneity: Subpopulations, isolation techniques, and diverse functions in cancer progression. Front Immunol (2018) 9:738. doi: 10.3389/fimmu.2018.00738
55. Wei H, Chen Q, Lin L, Sha C, Li T, Liu Y, et al. Regulation of exosome production and cargo sorting. Int J Biol Sci (2021) 17(1):163–77. doi: 10.7150/ijbs.53671
56. Sorop A, Constantinescu D, Cojocaru F, Dinischiotu A, Cucu D, Dima SO. Exosomal microRNAs as biomarkers and therapeutic targets for hepatocellular carcinoma. Int J Mol Sci (2021) 22(9):4997. doi: 10.3390/ijms22094997
57. Meldolesi J. Exosomes and ectosomes in intercellular communication. Curr Biol (2018) 28(8):R435–44. doi: 10.1016/j.cub.2018.01.059
58. Takahashi A, Okada R, Nagao K, Kawamata Y, Hanyu A, Yoshimoto S, et al. Exosomes maintain cellular homeostasis by excreting harmful DNA from cells. Nat Commun (2017) 8:15287. doi: 10.1038/ncomms15287
59. Baquir B, Hancock RE. Exosomes, your body’s answer to immune health. Ann Transl Med (2017) 5(4):81. doi: 10.21037/atm.2017.01.50
60. Basu J, Ludlow JW. Exosomes for repair, regeneration and rejuvenation. Expert Opin Biol Ther (2016) 16(4):489–506. doi: 10.1517/14712598.2016.1131976
61. Kreger BT, Johansen ER, Cerione RA, Antonyak MA. The enrichment of survivin in exosomes from breast cancer cells treated with paclitaxel promotes cell survival and chemoresistance. Cancers (Basel) (2016) 8(12):111. doi: 10.3390/cancers8120111
62. Ribeiro MF, Zhu H, Millard RW, Fan GC. Exosomes function in pro- and anti-angiogenesis. Curr Angiogenes (2013) 2(1):54–9. doi: 10.2174/22115528113020020001
63. Rastogi S, Sharma V, Bharti PS, Rani K, Modi GP, Nikolajeff F, et al. The evolving landscape of exosomes in neurodegenerative diseases: Exosomes characteristics and a promising role in early diagnosis. Int J Mol Sci (2021) 22(1):440. doi: 10.3390/ijms22010440
64. Tan L, Wu H, Liu Y, Zhao M, Li D, Lu Q. Recent advances of exosomes in immune modulation and autoimmune diseases. Autoimmunity (2016) 49(6):357–65. doi: 10.1080/08916934.2016.1191477
65. Sharma S, Sharma U. Exosomes in cardiovascular diseases: a blessing or a sin for the mankind. Mol Cell Biochem (2022) 477(3):833–47. doi: 10.1007/s11010-021-04328-6
66. Li S, Yao J, Xie M, Liu Y, Zheng M. Exosomal miRNAs in hepatocellular carcinoma development and clinical responses. J Hematol Oncol (2018) 11(1):54. doi: 10.1186/s13045-018-0579-3
67. Jun L, Yang G, Zhisu L. The utility of serum exosomal microRNAs in hepatocellular carcinoma. BioMed Pharmacother (2019) 111:1221–7. doi: 10.1016/j.biopha.2018.12.131
68. Tang J, Li Y, Liu K, Zhu Q, Yang WH, Xiong LK, et al. Exosomal miR-9-3p suppresses HBGF-5 expression and is a functional biomarker in hepatocellular carcinoma. Minerva Med (2018) 109(1):15–23. doi: 10.23736/S0026-4806.17.05167-9
69. Liang G, Kan S, Zhu Y, Feng S, Feng W, Gao S. Engineered exosome-mediated delivery of functionally active miR-26a and its enhanced suppression effect in HepG2 cells. Int J Nanomed (2018) 13:585–99. doi: 10.2147/IJN.S154458
70. Pomatto MAC, Bussolati B, D’Antico S, Ghiotto S, Tetta C, Brizzi MF, et al. Improved loading of plasma-derived extracellular vesicles to encapsulate antitumor miRNAs. Mol Ther Methods Clin Dev (2019) 13:133–44. doi: 10.1016/j.omtm.2019.01.001
71. Lou G, Song X, Yang F, Wu S, Wang J, Chen Z, et al. Exosomes derived from miR-122-modified adipose tissue-derived MSCs increase chemosensitivity of hepatocellular carcinoma. J Hematol Oncol (2015) 8:122. doi: 10.1186/s13045-015-0220-7
72. Basu S, Bhattacharyya SN. Insulin-like growth factor-1 prevents miR-122 production in neighbouring cells to curtail its intercellular transfer to ensure proliferation of human hepatoma cells. Nucleic Acids Res (2014) 42(11):7170–85. doi: 10.1093/nar/gku346
73. Aucher A, Rudnicka D, Davis DM. MicroRNAs transfer from human macrophages to hepato-carcinoma cells and inhibit proliferation. J Immunol (2013) 191(12):6250–60. doi: 10.4049/jimmunol.1301728
74. Zhang J, Shan WF, Jin TT, Wu GQ, Xiong XX, Jin HY, et al. Propofol exerts anti-hepatocellular carcinoma by microvesicle-mediated transfer of miR-142-3p from macrophage to cancer cells. J Transl Med (2014) 12:279. doi: 10.1186/s12967-014-0279-x
75. Gai X, Tang B, Liu F, Wu Y, Wang F, Jing Y, et al. mTOR/miR-145-regulated exosomal GOLM1 promotes hepatocellular carcinoma through augmented GSK-3β/MMPs. J Genet Genomics (2019) 46(5):235–45. doi: 10.1016/j.jgg.2019.03.013
76. Han Q, Zhao H, Jiang Y, Yin C, Zhang J. HCC-derived exosomes: Critical player and target for cancer immune escape. Cells (2019) 8(6):558. doi: 10.3390/cells8060558
77. Yugawa K, Yoshizumi T, Mano Y, Itoh S, Harada N, Ikegami T, et al. Cancer-associated fibroblasts promote hepatocellular carcinoma progression through downregulation of exosomal miR-150-3p. Eur J Surg Oncol (2021) 47(2):384–93. doi: 10.1016/j.ejso.2020.08.002
78. Lou G, Chen L, Xia C, Wang W, Qi J, Li A, et al. MiR-199a-modified exosomes from adipose tissue-derived mesenchymal stem cells improve hepatocellular carcinoma chemosensitivity through mTOR pathway. J Exp Clin Cancer Res (2020) 39(1):4. doi: 10.1186/s13046-019-1512-5
79. Zhang K, Shao CX, Zhu JD, Lv XL, Tu CY, Jiang C, et al. Exosomes function as nanoparticles to transfer miR-199a-3p to reverse chemoresistance to cisplatin in hepatocellular carcinoma. Biosci Rep (2020) 40(7):BSR20194026. doi: 10.1042/BSR20194026
80. Moh-Moh-Aung A, Fujisawa M, Ito S, Katayama H, Ohara T, Ota Y, et al. Decreased miR-200b-3p in cancer cells leads to angiogenesis in HCC by enhancing endothelial ERG expression. Sci Rep (2020) 10(1):10418. doi: 10.1038/s41598-020-67425-4
81. Semaan L, Zeng Q, Lu Y, Zhang Y, Zreik MM, Chamseddine MB, et al. MicroRNA-214 enriched exosomes from human cerebral endothelial cells (hCEC) sensitize hepatocellular carcinoma to anti-cancer drugs. Oncotarget (2021) 12(3):185–98. doi: 10.18632/oncotarget.27879
82. Zhang Z, Li X, Sun W, Yue S, Yang J, Li J, et al. Loss of exosomal miR-320a from cancer-associated fibroblasts contributes to HCC proliferation and metastasis. Cancer Lett (2017) 397:33–42. doi: 10.1016/j.canlet.2017.03.004
83. Wang F, Li L, Piontek K, Sakaguchi M, Selaru FM. Exosome miR-335 as a novel therapeutic strategy in hepatocellular carcinoma. Hepatology (2018) 67(3):940–54. doi: 10.1002/hep.29586
84. Zhao S, Li J, Zhang G, Wang Q, Wu C, Zhang Q, et al. Exosomal miR-451a functions as a tumor suppressor in hepatocellular carcinoma by targeting LPIN1. Cell Physiol Biochem (2019) 53(1):19–35. doi: 10.33594/000000118
85. Xu Y, Lai Y, Cao L, Li Y, Chen G, Chen L, et al. Human umbilical cord mesenchymal stem cells-derived exosomal microRNA-451a represses epithelial-mesenchymal transition of hepatocellular carcinoma cells by inhibiting ADAM10. RNA Biol (2021) 18(10):1408–23. doi: 10.1080/15476286.2020.1851540
86. Xiong L, Zhen S, Yu Q, Gong Z. HCV-E2 inhibits hepatocellular carcinoma metastasis by stimulating mast cells to secrete exosomal shuttle microRNAs. Oncol Lett (2017) 14(2):2141–6. doi: 10.3892/ol.2017.6433
87. Sugimachi K, Matsumura T, Hirata H, Uchi R, Ueda M, Ueo H, et al. Identification of a bona fide microRNA biomarker in serum exosomes that predicts hepatocellular carcinoma recurrence after liver transplantation. Br J Cancer (2015) 112(3):532–8. doi: 10.1038/bjc.2014.621
88. Wang G, Zhao W, Wang H, Qiu G, Jiang Z, Wei G, et al. Exosomal MiR-744 inhibits proliferation and sorafenib chemoresistance in hepatocellular carcinoma by targeting PAX2. Med Sci Monit (2019) 25:7209–17. doi: 10.12659/MSM.919219
89. Tian XP, Wang CY, Jin XH, Li M, Wang FW, Huang WJ, et al. Acidic microenvironment up-regulates exosomal miR-21 and miR-10b in early-stage hepatocellular carcinoma to promote cancer cell proliferation and metastasis. Theranostics (2019) 9(7):1965–79. doi: 10.7150/thno.30958
90. Zhou Y, Ren H, Dai B, Li J, Shang L, Huang J, et al. Hepatocellular carcinoma-derived exosomal miRNA-21 contributes to tumor progression by converting hepatocyte stellate cells to cancer-associated fibroblasts. J Exp Clin Cancer Res (2018) 37(1):324. doi: 10.1186/s13046-018-0965-2
91. Liang J, Zhang X, He S, Miao Y, Wu N, Li J, et al. Sphk2 RNAi nanoparticles suppress tumor growth via downregulating cancer cell derived exosomal microRNA. J Control Release (2018) 286:348–57. doi: 10.1016/j.jconrel.2018.07.039
92. Liu J, Fan L, Yu H, Zhang J, He Y, Feng D, et al. Endoplasmic reticulum stress causes liver cancer cells to release exosomal miR-23a-3p and up-regulate programmed death ligand 1 expression in macrophages. Hepatology (2019) 70(1):241–58. doi: 10.1002/hep.30607
93. Liu H, Chen W, Zhi X, Chen EJ, Wei T, Zhang J, et al. Tumor-derived exosomes promote tumor self-seeding in hepatocellular carcinoma by transferring miRNA-25-5p to enhance cell motility. Oncogene (2018) 37(36):4964–78. doi: 10.1038/s41388-018-0309-x
94. Fu X, Liu M, Qu S, Ma J, Zhang Y, Shi T, et al. Exosomal microRNA-32-5p induces multidrug resistance in hepatocellular carcinoma via the PI3K/Akt pathway. J Exp Clin Cancer Res (2018) 37(1):52. doi: 10.1186/s13046-018-0677-7
95. Yang B, Feng X, Liu H, Tong R, Wu J, Li C, et al. High-metastatic cancer cells derived exosomal miR92a-3p promotes epithelial-mesenchymal transition and metastasis of low-metastatic cancer cells by regulating PTEN/Akt pathway in hepatocellular carcinoma. Oncogene (2020) 39(42):6529–43. doi: 10.1038/s41388-020-01450-5
96. Nakano T, Chen IH, Wang CC, Chen PJ, Tseng HP, Huang KT, et al. Circulating exosomal miR-92b: Its role for cancer immunoediting and clinical value for prediction of posttransplant hepatocellular carcinoma recurrence. Am J Transplant (2019) 19(12):3250–62. doi: 10.1111/ajt.15490
97. Xue X, Wang X, Zhao Y, Hu R, Qin L. Exosomal miR-93 promotes proliferation and invasion in hepatocellular carcinoma by directly inhibiting TIMP2/TP53INP1/CDKN1A. Biochem Biophys Res Commun (2018) 502(4):515–21. doi: 10.1016/j.bbrc.2018.05.208
98. Fang JH, Zhang ZJ, Shang LR, Luo YW, Lin YF, Yuan Y, et al. Hepatoma cell-secreted exosomal microRNA-103 increases vascular permeability and promotes metastasis by targeting junction proteins. Hepatology (2018) 68(4):1459–75. doi: 10.1002/hep.29920
99. Wei XC, Xia YR, Zhou P, Xue X, Ding S, Liu LJ, et al. Hepatitis b core antigen modulates exosomal miR-135a to target vesicle-associated membrane protein 2 promoting chemoresistance in hepatocellular carcinoma. World J Gastroenterol (2021) 27(48):8302–22. doi: 10.3748/wjg.v27.i48.8302
100. Matsuura Y, Wada H, Eguchi H, Gotoh K, Kobayashi S, Kinoshita M, et al. Exosomal miR-155 derived from hepatocellular carcinoma cells under hypoxia promotes angiogenesis in endothelial cells. Dig Dis Sci (2019) 64(3):792–802. doi: 10.1007/s10620-018-5380-1
101. Sun JF, Zhang D, Gao CJ, Zhang YW, Dai QS. Exosome-mediated MiR-155 transfer contributes to hepatocellular carcinoma cell proliferation by targeting PTEN. Med Sci Monit Basic Res (2019) 25:218–28. doi: 10.12659/MSMBR.918134
102. Lin XJ, Fang JH, Yang XJ, Zhang C, Yuan Y, Zheng L, et al. Hepatocellular carcinoma cell-secreted exosomal MicroRNA-210 promotes angiogenesis in vitro and in vivo. Mol Ther Nucleic Acids (2018) 11:243–52. doi: 10.1016/j.omtn.2018.02.014
103. Cui Y, Xu HF, Liu MY, Xu YJ, He JC, Zhou Y, et al. Mechanism of exosomal microRNA-224 in development of hepatocellular carcinoma and its diagnostic and prognostic value. World J Gastroenterol (2019) 25(15):1890–8. doi: 10.3748/wjg.v25.i15.1890
104. Qu Z, Wu J, Wu J, Ji A, Qiang G, Jiang Y, et al. Exosomal miR-665 as a novel minimally invasive biomarker for hepatocellular carcinoma diagnosis and prognosis. Oncotarget (2017) 8(46):80666–78. doi: 10.18632/oncotarget.20881
105. Fang T, Lv H, Lv G, Li T, Wang C, Han Q, et al. Tumor-derived exosomal miR-1247-3p induces cancer-associated fibroblast activation to foster lung metastasis of liver cancer. Nat Commun (2018) 9(1):191. doi: 10.1038/s41467-017-02583-0
106. Yu Y, Min Z, Zhihang Z, Linhong M, Tao R, Yan L, et al. Hypoxia-induced exosomes promote hepatocellular carcinoma proliferation and metastasis via miR-1273f transfer. Exp Cell Res (2019) 385(1):111649. doi: 10.1016/j.yexcr.2019.111649
107. Chen R, Xu X, Tao Y, Qian Z, Yu Y. Exosomes in hepatocellular carcinoma: a new horizon. Cell Commun Signal (2019) 17(1):1. doi: 10.1186/s12964-018-0315-1
108. Pascut D, Pratama MY, Vo NVT, Masadah R, Tiribelli C. The crosstalk between tumor cells and the microenvironment in hepatocellular carcinoma: The role of exosomal microRNAs and their clinical implications. Cancers (Basel) (2020) 12(4):823. doi: 10.3390/cancers12040823
109. Georganaki M, van Hooren L, Dimberg A. Vascular targeting to increase the efficiency of immune checkpoint blockade in cancer. Front Immunol (2018) 9:3081. doi: 10.3389/fimmu.2018.03081
110. Pinato DJ, Black JR, Trousil S, Dina RE, Trivedi P, Mauri FA, et al. Programmed cell death ligands expression in phaeochromocytomas and paragangliomas: Relationship with the hypoxic response, immune evasion and malignant behavior. Oncoimmunology (2017) 6(11):e1358332. doi: 10.1080/2162402X.2017.1358332
111. Faivre S, Rimassa L, Finn RS. Molecular therapies for HCC: Looking outside the box. J Hepatol (2020) 72(2):342–52. doi: 10.1016/j.jhep.2019.09.010
112. Tomimaru Y, Eguchi H, Nagano H, Wada H, Tomokuni A, Kobayashi S, et al. MicroRNA-21 induces resistance to the anti-tumour effect of interferon-α/5-fluorouracil in hepatocellular carcinoma cells. Br J Cancer (2010) 103(10):1617–26. doi: 10.1038/sj.bjc.6605958
113. Bao L, Yan Y, Xu C, Ji W, Shen S, Xu G, et al. MicroRNA-21 suppresses PTEN and hSulf-1 expression and promotes hepatocellular carcinoma progression through AKT/ERK pathways. Cancer Lett (2013) 337(2):226–36. doi: 10.1016/j.canlet.2013.05.007
Keywords: exosomes, miRNAs, hepatocellular carcinoma, therapy, drug resistance
Citation: Zelli V, Compagnoni C, Capelli R, Corrente A, Di Vito Nolfi M, Zazzeroni F, Alesse E and Tessitore A (2022) Role of exosomal microRNAs in cancer therapy and drug resistance mechanisms: focus on hepatocellular carcinoma. Front. Oncol. 12:940056. doi: 10.3389/fonc.2022.940056
Received: 09 May 2022; Accepted: 27 June 2022;
Published: 15 July 2022.
Edited by:
Antonio Giordano, Temple University, United StatesReviewed by:
Maria Carmen Ragosta, University of Siena, ItalyReyes Benot Dominguez, Temple University, United States
Copyright © 2022 Zelli, Compagnoni, Capelli, Corrente, Di Vito Nolfi, Zazzeroni, Alesse and Tessitore. This is an open-access article distributed under the terms of the Creative Commons Attribution License (CC BY). The use, distribution or reproduction in other forums is permitted, provided the original author(s) and the copyright owner(s) are credited and that the original publication in this journal is cited, in accordance with accepted academic practice. No use, distribution or reproduction is permitted which does not comply with these terms.
*Correspondence: Alessandra Tessitore, YWxlc3NhbmRyYS50ZXNzaXRvcmVAdW5pdmFxLml0
†These authors have contributed equally to this work