- 1Department of Pulmonary Medicine, Xijing Hospital, Air Force Medical University, Xi’an, China
- 2Department of Anus and Intestine Surgery, The 942th Hospital of Joint Logistics Support Force, Yinchuan, China
- 3Department of Hemato-Oncology, The 942th Hospital of Joint Logistics Support Force, Yinchuan, China
SCFFBXW7 E3 ubiquitin ligase complex is a crucial enzyme of the ubiquitin proteasome system that participates in variant activities of cell process, and its component FBXW7 (F-box and WD repeat domain–containing 7) is responsible for recognizing and binding to substrates. The expression of FBXW7 is controlled by multiple pathways at different levels. FBXW7 facilitates the maturity and function maintenance of immune cells via functioning as a mediator of ubiquitination-dependent degradation of substrate proteins. FBXW7 deficiency or mutation results in the growth disturbance and dysfunction of immune cell, leads to the resistance against immunotherapy, and participates in multiple illnesses. It is likely that FBXW7 coordinating with its regulators and substrates could offer potential targets to improve the sensitivity and effects of immunotherapy. Here, we review the mechanisms of the regulation on FBXW7 and its tumor suppression role in immune filed among various diseases (mostly cancers) to explore novel immune targets and treatments.
1. Introduction
Degradation is one of most significant bioprocesses of metabolism in almost all forms of life. For most eukaryotic cells, three pathways were discovered to degrade multiple proteins: (1) lysosomal pathway, (2) caspase pathway, and (3) ubiquitin proteasome pathway. Among them, the ubiquitin-proteasome pathway is the most irreplaceable specific protein degradation pathway, which can participate in various biological processes including cell proliferation, division, differentiation, and apoptosis, by promoting protein degradation (1, 2). The ubiquitin proteasome system (UPS) performs its protein-degrading function by three enzymes: the ubiquitin-activating enzyme (E1), the ubiquitin-conjugating enzyme (E2), and the ubiquitin ligase (E3). Among them, E3 ubiquitin ligase is the most critical component of UPS that can specifically recognize proteins to complete their ubiquitination. Emerging evidence exhibits that E3 shows a tendency weighing more in tumor suppressing than that in activating (3, 4). Skp1–Cullin1–F-box (SCF)FBXW7 consisting of Skp1, CUL1, F-box, and RBX1, is a well-learned category of E3 ligase in Really Interesting New Gene (RING) family (5). FBXW7 (F-box and WD repeat domain–containing 7) is usually deemed as a negative regulator of human cancers and is the most crucial F-box protein in E3 ligase so far (6).
Studies have indicated that FBXW7 is also involved in the regulation of immunity (7–11). Theories have explained the correlation between immunity and tumorigenesis, including tumor immunosurveillance and tumor immunoediting theory (12). Tumor immunoediting theory is a refinement of tumor immunosurveillance to explain the immune evsion of mutated cells and the progression of tumors. According to this theory, tumors develop in three stages. The first step is the elimination phase—the healthy body detects and eliminates mutated cells through immune surveillance; the second is the equilibrum phase—the immune system is so vulnerable that is uncapable of clearing out all of the mutated cells and leaves a significant number of the tumor cells remaining inside the body; and the final step is the escape phase—the tumor cells take a dominant position and become resistant to the attack launched by the immune system with the emergence of clinical signs and symptoms. Because immunity shows a close correlation with tumorigenesis, immunotherapy has been explored to fight against multiple cancers (13). Multiple immunotherapies have been developed, such as tumor vaccine, Bacille Calmette-Guerin (BCG), chimeric antigen receptor–engineered T lymphocytes, adoptive cellular therapy, and immune checkpoint inhibitors (ICIs). Among them, ICIs (including anti–PD-1/anti–PD-L1 and anti–CTLA-4) are mostly applied into the clinic, especially anti–PD-1/anti–PD-L1. Three immune patterns were characterized according to the anti-tumor reaction to PD-L1/PD-1 treatment (14). The first is the immune-inflamed phenotype featured by the existence of CD4- and CD8-positive T cells in the tumor parenchyma. The second is the immune-excluded phenotype featured by plentiful immune cells existing in the stroma without the ability of invading tumor parenchyma. The last one is the immune-desert phenotype featured by the absence of T cells in either parenchyma or stroma. A lot of research studies have found that SCFFBXW7 acting as a tumor suppressor is involved in tumorigenesis and resistance to immunotherapy by maintaining immune evasion (9, 15, 16).
This review mainly focuses on the FBXW7 functions in different cancers immunotherapy based on its structure and regulations in different levels, its involvement in multiple biological processes, as well its effect on immune cells and cytokines, which eventually draws a blueprint of targeting FBXW7 in immunotherapy.
2. Skp1–Cullin1–F-Box Protein Complex
The UPS plays a significant role in multiple bioprocess including cell propagation, division, and differentiation to decide the destiny of cell (7). It consists of three functionally interconnected enzymes: E1, E2, and E3. RING finger–type proteins and Homologous to the E6-AP Carboxyl Terminus (HECT) domain–type proteins are the largest two families of E3 ligase. In the RING family, E3 ligases transfer ubiquitin molecules directly from the E2 ubiquitin complex to the substrate without binding to ubiquitin molecules. The SCF ubiquitin ligase complex is a crucial member mainly composed of four units: Skp1 (composed of 163 residues), CUL1 (composed of 776 residues), F-box (composed of ~430 to >1,000 residues), and RBX1 (also called ROC1, composed of 108 residues) (17–20). CUL1 functions as a skeleton protein to interplay with the remaining three subunits (20). CUL1 interacts with RBX1via C terminus while it interacts with SKP1 via its N terminus. Moreover, F-box interacts with Skp1 via the F-box motif (21). SCF complex is deemed as one of the critical controllers of the mechanism in cell cycle for they regulate pivotal proteins progressing cell cycle (22). The F-box is the part of SCF complex responsible for recognizing and binding substrates. So far, nearly 70 F-box proteins have been found in humans as well as in other species (23). These proteins are further divided into three categories: Those rich in leucine repeats are called FBXL; the domain containing WD40 is called FBXW; the others are FBXO (with another or without motif except F-box protein) (24). FBXW7 (F-box with 7 tandem WD40 repeats) is the most famous FBXW protein for its significance in cellular processes including cell proliferation, division, and differentiation and is also known as FBW7, hAgo, hCDC4, and Sel10 (7).
3. FBXW7—The Most Critical F-Box Protein in the RING Family of E3 Ubiquitin Ligase
3.1. Structure and Locations
FBXW7 is the most widely researched F-box protein for its tumor suppression role up to now. It was first identified in budding yeast in 1973 by Hartwell et al. and then named CDC4 (25). This gene is highly conserved in multiple species. During the research on regulation of SEL-10 to LIN-12, the conserved gene CDC4 was also found in human cells and was named FBXW7 in terms of its structure, attracting increasing attention from then (26–28). FBXW7 gene that consists of 13 coding exons and 4 non-coding exons is located in the 4q31q.3 region of the human chromosome (a region frequently associated with deletion mutations in human tumors), and the gene length of FBXW7 is approximately 210 kb (6, 29). According to the difference between the 5′ untranslated region (5’UTR) and N-terminal coding region, the spliced variants of FBXW7 are divided into three subtypes: FBXW7α, FBXW7β, and FBXW7γ. Same gene as it comes from, the corresponding proteins translated by the three subtypes are located differently in subcellular regions: FBXW7α is located in the cytoplasm; FBXW7β is located in the cytoplasm; FBXW7γ is located in the nucleolus (30a). Different localization of FBXW7 can regulate their respective functions, which may be related to different pathways to bind to substrates. The three subtypes of FBXW7 share the following important conserved sequences: (1) the F-box domain, performing the function of recruiting SCF complexes through Skp1; (2) D domain, promoting the formation of FBXW7 dimer; and (3) WD40 domain, responsible for substrate recognition. Apart from the distinguishment in intracellular localization, three subtypes of FBXW7 also expressed discrepantly in different tissue types. A study in 2002 found that FBXW7α is widely expressed in human tissues, whereas FBXW7β and FBXW7γ are highly expressed mainly in the heart, brain, and skeletal muscle (29). Four years later, another mice model study came to similar conclusions (31). Currently, research studies lay emphasis mainly on the function of FBXW7α, whereas the other two subtypes of FBXW7-related biological studies are relatively rare.
3.2. Regulation of FBXW7
3.2.1. FBXW7 Regulation at Transcriptional Level
Since we have known the structure and locations of FBXW7, we want to figure out the mechanisms on how it is regulated. Regulation of FBXW7 at transcriptional level represents a tendency to negatively regulating the expression of FBXW7. RAN-binding protein 10 (RANBP10) promotes the stabilization of c-myc via binding to the region P4 of FBW7 promotor and inhibiting its transcription, which induces the progression of glioblastoma (32). A complex of PHF1/PRMT5–WDR77/CRL4B represses transcription of FBWX7 by taking up its promotor, which leads to the progression of cancer (33). C/EBPδ functions as an inhibitor binding to the promoter of FBW7α and decreases the abundance of FBXW7α mRNA, contributing to improved activity of HIF-1 via stabilizing mTOR (34). Furthermore, a feedback loop consisting of FBXW7, Hes5, and NOTCH intracellular domain (NICD) is involved in the inactivation of FBXW7 mRNA (35). In this loop, Hes5 binds to the N-box in the promotor of FBXW7 to suppress its transcription, which can affect the fate of intestinal and neural stem cells. Moreover, the inactivation of FBXW7 mediated by Hes5 participates in the inhibition of TGF-β pathway as well (36). TRIP13 confers the carcinogenicity of glioblastoma via binding to the promoter near FBXW7 gene and further stabilizing c-myc by suppressing expression of FBXW7 (37). Intriguingly, P53 activates the transcription of FBXW7β by binding to a site in exon 1b, acting as a resistance against genotoxic pressure from UV radiation and adriamycin (38).
3.2.2. Epigenetic Regulation of FBXW7
3.2.2.1. Methylation and Demethylation Modification
Several studies have uncovered the correlation of DNA methylation with FBXW7 expression. In the study of Akhoondi et al., they found that the ratio of methylation of the FBXW7β promoter in cancer cell line is 43%, wherereas the number in primary breast cancer is 51% (39). Data suggested that FBXW7β of methylated group was downregulated both in cancer cell line and in primary breast cancer compared with the unmethylated group. They also found that although methylation was connected with advanced tumors, the hazard ratio (HR) for patients’ death with FBXW7β methylation on the opposite showed a downtrend. Gu et al. discovered that, with the methylation of the CpG sequence in FBXW7β’s promoter, a significant decline of the expression of FBXW7β was observed (40a). The methylation in FBXW7β promoter was found to be positively associated with thymoma histological subtype that presents a positive correlation with prognosis of patients (41b). In addition, the methylation level of FBXW7 gene 5′ upstream areas of p53-mutated samples was significantly higher than that of wild-type samples, which may due to the suppression of FBXW7 expression by p53 mutations through the hypermethylation in designated areas (42). More epigenetic silencing of FBXW7 was exhibited in human papillomavirus-IMM (HPV-IMM) than HPV16-KRT, which may work in the stratification of cervical squamous cell carcinoma (CSCC) affected by HPV16 to provide reasonable treatment for patients (43). Furthermore, lysine demethylase 5B (KDM5B), a histone demethylase of FBXW7, was unveiled to suppress FBXW7 expression via demethylation of H3K4me3 at promoter region (44). The mechanism of FBXW7 epigenetic modulation has been applied into clinical treatment for patient of lung cancer as decitabine is able to demethylate the epigenetically silenced FBXW7 gene and reactivate it (45)
3.2.2.2. Histone Acetylation
In addition to DNA methylation, histone acetylation is also implicated in regulation of FBXW7. A research to detect the DNA methylation, histone methylation, histone acetylation, and chromatin remodeling uncovered that H3K27 acetylation suppressed by the mutation or knockdown in CREBBP or EP300 in B-lymphoma cells weakened the expression of FBXW7, leading to the activation of NOTCH pathway and thereby caused the tumor-associated macrophage (TAM) polarizing to M2 phenotype and proliferation of tumor cells (46). Histone acetylation could also work synergistically with DNA methylation. Data acquired from The Cancer Genome Atlas (TCGA) revealed that high DNA methylation of the FBXW7 was accompanied with high KDM5c (a histone demethylase) expression, which might ascribe to the recruitment of DNMT3b induced by interaction of KDM5c and H3K4me3 of FBXW7 downstream so that the CpG of FBXW7 could be methylated, followed with inhibited FBXW7 expression (47a).
3.2.2.3. Chromatin Remodeling
Chromatin remodeling is involved in the mediation of FBXW7 as well. An experiment conducted by Masayuki Hagiwara disclosed that the expression of FBXW7 was restrained by the overexpression of MUC1-1C, which activates the components, including MBD3, MTA1, and CHD4, of the nucleosome remodeling and deacetylation (NuRD) complex to facilitate Interferon Gamma Receptor 1 (IFNGR1) expression (48, 49). Another study demonstrated that FBXW7 has an intimated relation with chromatin remodeling through whole-exome sequencing of 57 cancers (50). Although the direct relationship between FBXW7 and chromatin remodeling was not demonstrated, we speculate that FBXW7 expression could be inhibited by means of chromatin remodeling. However, more work remains to be done to explore the deeper mechanisms. In this way, the substrate of FBXW7, IFNGR1, showed a trend of increasing expression and further promoted the tumorigenesis and metastasis of cancer (51).
Collectively, epigenetic modification regulates FBXW7 in three ways: methylation and demethylation modification, Histone acetylation and Chromatin remodeling.
3.2.2.4. RNA Epitranscriptomic Modification of FBXW7
A previous research conducted by our team revealed that N6-methyladenosine (m6A) is involved in the methylated modification of FBW7 mRNA (52). M6A refers to the methylation of N6-adenosine in eukaryotic mRNA controlled by writers—methyltransferases, erasers—demethylases, and readers—binding proteins, which is a universal modification of mRNA and affects various pathphysiological processes including tumorgensis (53, 54). METTL3 is one of the methyltransferases which methylates m6A of FBXW7 mRNA and facilitates its translational efficiency to repress lung adenocarcinoma (52). Interestingly, not only is FBXW7 regulated through m6A, but it also regulates the m6A of other mRNAs. FBXW7 targets YTHDF2 protein, the m6A reader of BMF mRNA, rescuing the YTHDF2-mediated inactivation of BMF mRNA and repressing the growth and progression of ovarian cancer (55).
3.2.3. Regulation of FBXW7 Mediated by Non-Coding RNA
3.2.3.1. MicroRNA Regulation of FBXW7
Non-coding RNA also functions in regulation of FBXW7. MicroRNA (miRNA) is a kind of non-coding RNA that can suppress the mRNA and intervene the subsequent protein synthesis via binding to the 3′UTR. Plenty of miRNAs have been uncovered to bind to the 3′UTR of FBXW7 and inhibit the protein translation. Overexpression of miR-223 plays roles in different situations by the counteraction of FBXW7. MiR-223 functions in gastric cancer for carcinostasis and drug resistance (56, 57), gives rise to physiologic cardiac hypertrophy (58), and protects CRC cells against apoptosis and promotes its proliferation as well (59). Moreover, a KLF5/mi-R27a/FBXW7 axis was reported to enhance migration and invasion of ccRCC when FBXW7 was reduced by mi-R27a (60). Apart from involvement in cancer, downregulation of FBXW7 by miR-322 demonstrates a possible curing method to protect myocardium from ischemia/reperfusion injury. Collectively, there are still other miRNAs, such as miR129 (61), miR-92a-3p (62), miR182 (63), miR-27a-3p (64, 65), miR-212/132 (66), miR-223-3p (67), miR-103a-3p (68), miR-25 (69), miR−25−3p (70), miR-144 (71), miR-101 (72), miR-188-5p (73), and miR-500a-3p (74), functioning discrepantly to mediate different cell phenotypes but via the identical mechanism of targeting FBXW7.
3.2.3.2. Long Non-Coding RNA Regulation of FBXW7
Long non-coding RNAs (lncRNAs) are non-coding RNAs with more than 200 nucleotides in length and without the function of coding proteins (75). LncRNAs take part in various biological activities by interplaying with DNAs, RNAs, and proteins (76, 77), which means lncRNAs regulate FBXW7 at different levels directly or indirectly. A lncRNA-associated-feedback loop was explored by Pengfei Zhang et al. demostrated that lncRNA-MIF (c-myc inhibitory factor) functioning as a competing endogenous RNA (ceRNA) for miR586 weakened the suppression of miR-586 on FBXW7 (78). Therefore, the substrate of FBXW7, c-myc, was repressed subsequently as FBXW7 was upregulated by the lncRNA-MIF–associated feedback loop and the aerobic glycolysis and pro-oncogenicity triggered by c-myc was eliminated. As c-myc was repressed by FBXW7, its induction to lncRNA-MIF was attenuated, which in turn results in the reduction of lncRNA-MIF abundance. Moreover, lncRNAs of similar roles served as miRNA “sponges” include MALAT1 (79), CASC2 (80), TINCR (81), MT1JP (82), FER1L4 (83), MIR22HG (84), TTN-AS1 (85), LINC00173 (86), and PADNA (87). Other mechanisms of lncRNAs regulating the expression of FBXW7 are displayed as well. A research carried out by Lianzhi Wu et al. revealed the pathway that FBXW7 directly recruited by MALAT1 contributed to the degradation of CRY2 (88). LncRNA BDNF-AS was capable of recruiting WDR5 to contribute to CpG island methylation of FBXW7, by which FBXW7 was downregulated and the ubiquitination of its substrate VDAC3 was diminished (89a). Another study verified that lncRNA SEMA3B-AS1 integrates with HMGB1, a transcription factor of FBXW7, and then facilitates the expression of FBXW7, resulting in the enhanced ubiquitination-mediated degradation (90). In addition, lncRNA TUG1 facilitates the expression of FBXW7 at protein level instead of mRNA level to destabilize SIRT1, leading to the abrogation of neuronal mitophagy (91).
3.2.3.3. Circular RNA Regulation of FBXW7
Circular RNA (circRNA) is distinguished by its structure of covalently closed loop without polyadenylated tail or 5′ to 3′ polarity (92). Parallel to lncRNAs, most circRNAs regulate FBXW7 as miRNA “sponges”. For instance, hsa_circ_11780 (93), circFBXW4 (94), Hsa_circ_001988 (95), circ_CLASP2 (96), circBRAF (97), circ_0000094 (98), hsa_circ_0001306 (99), hsa_circ_0022742 (100), circPSD3 (101), and circKL (102) were confirmed to sponge different miRNAs to impact the expression of FBXW7, respectively. Intriguingly, although the majority of circRNAs are deemed unable to code proteins as non-coding RNAs, a special circRNA associated with FBXW7 has been confirmed the function of coding protein. Circ-FBXW7 was a product of the circulation of cyclization of exons 3 and 4 in the FBXW7 gene that can code a neo-protein named FBXW7-185aa (103, 104). One study revealed that the FBXW7-185aa coded by circ-FBXW7 regulating FBXW7 protein via competitively binding to USP28 in glioma (104). Another study discovered two pathways in triple-negative breast cancer to control the expression of FBXW7: One displayed that circ-FBXW7 sponges miR-197-3p to promote the expression of FBXW7. The other demonstrated a similar pathway to that in glioma—FBXW7-185aa competitively binding to USP28 to protect the function of FBXW 7 (103).
In brief, three kinds of non-coding RNAs, including miRNAs, lncRNAs, and circRNAs, regulate the expression of FBXW7 in their own ways. In addition, some miRNAs work synergistically with lncRNAs or circRNAs to fulfill the function together.
3.2.4 Dimerization of FBXW7
Although the protein translation process has been completed, the abundance of FBXW7 can also been influenced. Dimerization is not only a widely phenomenon for all F-box proteins but also a critical regulatory mechanism for FBW7-mediated ubiquitination to substrates as well (105–108). FBXW7 owns three shared domains among all different isoforms as depicted, and the D domain mainly mediates FBXW7′ dimerization (107). Dimerization of FBXW7 may exert the following effects: (1) impacting the location of different isoforms; (2) raising the possibility for several substrates to be regulated by FBXW7; (3) capable of regulating the autoubiquitination of FBXW7; (4) functioning as a buffer to endure mutations that impair the FBXW7 substrate and hinder the substrate degradation mediated by monomeric FBXW7; (5) and increasing ubiquitination rate and processivity (5, 105, 108). However, take the examples of c-myc and cyclin E (108), widely seen as it is, not all substrates ubiquitination need dimerization of FBXW7. Intriguingly, a latest research revealed that LSD1, often regarded as a demethylase of histone, directly bound to FBXW7 to disturb the formation of dimerization to facilitate autoubiquitination rather than activate the demethylation of FBXW7, which might offer a new target for cancer treatment (109).
3.2.5 Phosphorylation of FBXW7
Phosphorylation of FBXW7 also occurs after FBXW7 translation. Activation of ERK1/2 kinase occurs in many drug-resistant tumor cells (110, 111), and FBXW7 is phosphorylated at Thr205 and further degraded by UPS (112). The instability of FBXW7 caused by phosphorylation has been verified in many experiments. Mun et al. investigated that Erk1/2 kinase participated in the inhibition of FBXW7 expression in drug-resistant cells (A549-Taxolr cells and T47D-Doxr cells), which led to an increase in heat-shock factor 1 (HSF1) and promoted transcriptional activation of MDR1 (113). This phosphorylation-dependent regulation of FBXW7 by ERK1/2 was also confirmed by other studies. Shu et al. found that ERK1/2-mediated phosphorylation of FBXW7 was involved in transcriptional regulation of FOS-like 1 (Fra-1) by Neuregulin 1 via stabilizing downstream c-myc that could bind to the promoter of Fra-1, thereby promoting metastasis of triple negative breast cancer (114). Another example of FBXW7 phosphorylation by ERK kinase at the T205 residue was verified likely to affect the ferroptosis of pancreatic carcinoma cells (115). In addition, FBXW7-myc-PLK1 forms a regulatory loop that controlled neuroblastoma tumor progression, in which FBXW7 was phosphorylated at Thr284 and Ser58 by PLK1 (116). Similar regulatory loop was also discovered in medulloblastoma (117). Phosphorylation of FBXW7 by related kinases not only contributes to the degradation of FBXW7 but also improves the catalytic activity of FBXW7 to downstream substrates. The process of FBXW7 phosphorylation at Ser227 mediated by serum and glucocorticoidregulated kinase 1 (SGK1) or Phosphoinositide 3-kinase (PI3K) enhances the ubiquitination ability of FBXW7 (118, 119). Furthermore, phosphorylation of FBXW7α at Ser10/Ser18 mediated by protein kinase (PK) C was found both in vitro and in mammals, and phosphorylation of Ser10 had been validated to affect nuclear localization of FBXW7α (120). Other results had been obtained both in human and Xenopus eggs in regard to PKC phosphorylation of FBXW7 that FBXW7α phosphorylated by PKC at Ser18 residues occurred during mitosis, which stabilized FBXW7 itself but interfered with ubiquitination of downstream cyclin E (121).
3.2.6 Autoubiquitination of FBXW7
Not only can FBXW7 degrade its substrates in the ubiquitination dependent way, but it is also competent for its autoubiquitination. It is a universal phenomenon that F-box is ubiquitinated for its necessity to strike the functional balance of SCF complex via the autoubiquitination (122, 123). F-box is more apt to be degraded compared with other components in SCF complex for its instability. The process of F-box ubiquitination takes place within the SCF complex itself without the assistance of other F-box proteins as the ubiquitination is required to be complete (124). Ubiquitin binding to FBXW7 was reported to facilitate ubiquitination and degradation of FBXW7, for which was predominant in the competition against the substrates of the FBXW7 (125). Moreover, FBXW7 autoubiquitination is also promoted through its dimerization (105). Another investigation revealed that CSN6 increased neddylation of Cullin-1 to contribute to FBXW7 autoubiquitination as a K48 linkage (126). In contrast, PML promotes FBXW7 expression via inhibiting ubiquitination and degradation of FBXW7 in the K48-linked way to enhance antiviral immunoreaction against influenza virus (127).
3.2.7 Biomechanical Regulation of FBXW7
What we have mentioned above are biological pathways to regulate FBXW7 expression. Interestingly, a biomechanical pathway that controls FBXW7 was unveiled recently (128). The investigation conducted by Haiyan Zhang suggested that mechanical overloading downregulated FBXW7 in chondrocyte of patients with osteoarthritis. The critical step that they found contributing to FBXW7 suppression occurred in the synthesis of mRNA. As a result of FBXW7 downregulation, MKK7–JNK pathway was activated and further facilitated the senility of chondrocyte. It offers us a promising curing target on treatment osteoarthritis and a fire-new viewpoint to explore the undiscovered pathways of the regulation of FBXW7.
3.3. The Pattern of FBXW7 Binding to Its Substrates
3.3.1. CPDs of Substrates
Previous studies found that a large proportion of substrates of FBXW7 was unable to bind FBXW7 E3 ligase until the CDC4 phospho-degrons (CPDs) of substrates were phosphorylated by protein kinase (129–131). Optimal sequences refer to CPDs sequences interacting with FBXW7 that contain essential residues. Specifically, there should be at least one hydrophobic amino acid at the sites −5, −3, −2, and −1; it is usually threonine or serine that takes up site zero; the +1 and +2 sites are generally proline; the +4 site may be one of serine, threonine, glutamate, and aspartic acid (7). Only when the 0 and +4 amino acids are phosphorylated by kinases can the substrate be discerned by the WD40 domain and participate in the UPS pathway for degradation. Intriguingly, not all CPDs of substrates follow this rule. CPDs with unfavorable residues of some proteins partially different from the optimal sequence are called semi-optimal CPDs. However, a portion of substrates CPDs remains undiscovered (7). In addition, some substrates possess more than one CPD sequence. KLF5 was reported to be degraded by FBXW7 with any of three CPDs phosphorylated by GSK-3β, which result in its repressed bio-activity in cell propagation (129). FBXW7 dimerization is likely to play a role in its interaction with the CPDs of protein as it was surveyed that the dimer structure can interact with the two CPDs of cyclin E to enhance the binding ability of cyclin E and promote its ubiquitination degradation (105, 107).
3.3.2. UPS-Dependent Degradation of Substrates
Multifarious substrates of FBXW7 have been previously reported before (7). E3 ligase recognizes the substrates once the CPDs of substrates are phosphorylated and then transfer ubiquitin molecules to the substrates (generally to a lysine side chain) to facilitate the ubiquitination of substrates (132). More than one poly-ubiquitin chain or multiple mono-ubiquitins are required to initiate the degradation (133). When substrates acquire enough ubiquitin chains, they will be captured and cut into peptides in an Adenosine Triphosphate (ATP)-dependent manner by the 26S proteosome (134). The UPS-dependent degradation of substrates mediated by SCFFBXW7 is shown in (Figure 1).
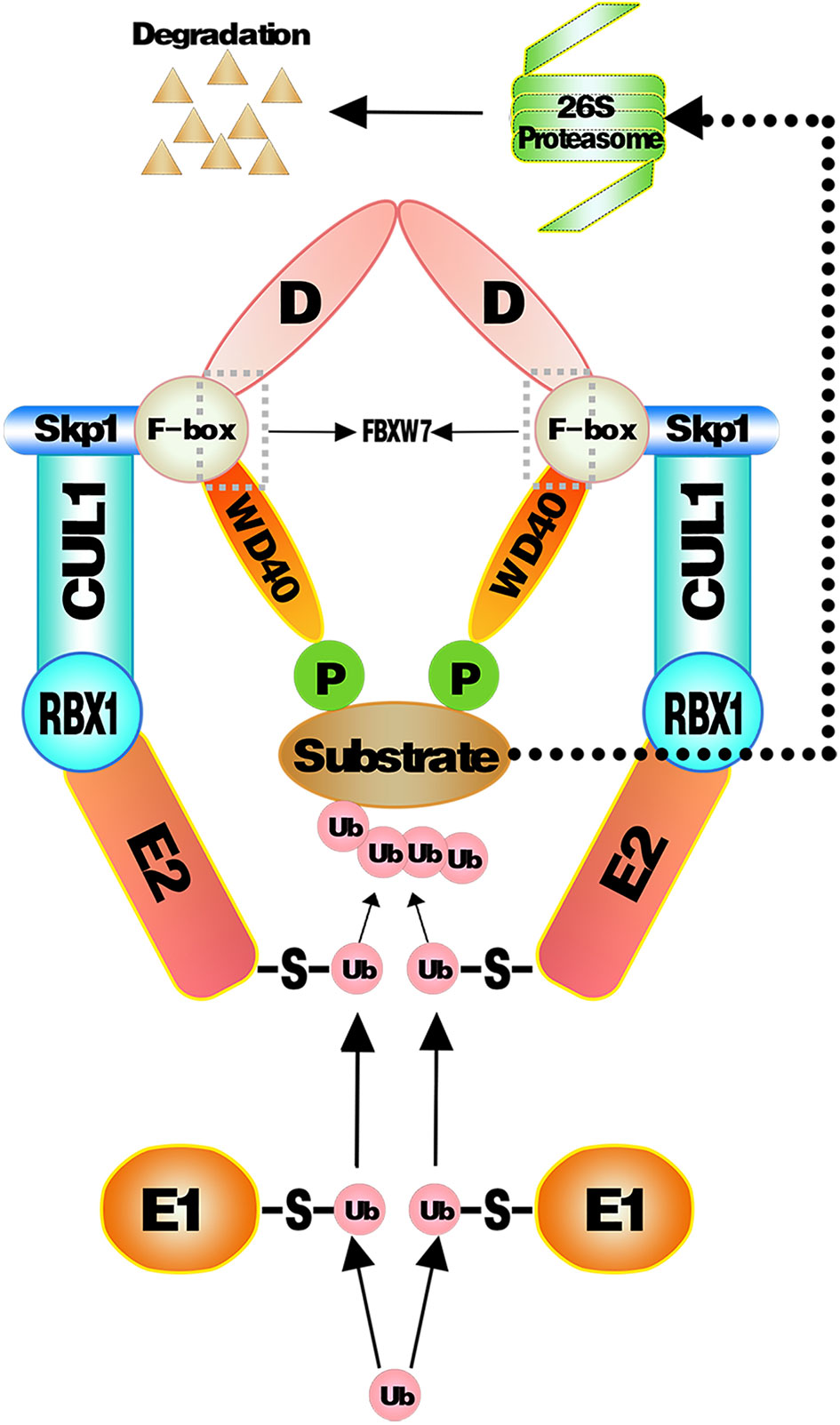
Figure 1 Substrates proteins targeted and ubiquitinated by SCFFBXW7 complex. Ubiquitin molecules are activated by E1 and then transferred to E2. E3 recognizes phosphorylated substrates and combined to E2 ubiquitin to transfer ubiquitin from E2 to substrates. As sufficient ubiquitin chains form onto substrates, they will be captured and cut into peptides in an ATP-dependent way by the 26S proteosome.
3.4. Interaction of Deubiquitinating Enzymes and FBXW7
Generally, as proteins are captured by 26S proteosome, deubiquitinating enzymes (DUBs) directly cut off ubiquitin from ubiquitin-binding proteins in various cell bioprocesses (135). Specifically, here, we introduce unusual pathways for DUBs regulating protein. USP28 (one of DUBs) could counteract the effect of FBXW7α degrading myc in the nucleus by forming a special complex with myc and FBW7α (136a; 137). Similarly, USP28 could stabilize HIF-1α by antagonizing FBXW7 rather than interacting with HIF-1α, which impacts the cancer cell activity and capillary formation (138). Analogous mechanism was observed on the regulation of NICD1 and c-jun in intestinal homeostasis and tumorigenesis (139). Interestingly, another study found that partial reduction of USP28 stabilizes FBXW7 and facilitates degradation of its substrates, whereas the absence of USP28 can facilitate FBXW7 ubiquitination, and overexpression of USP28 preferentially counteracts autocatalytic ubiquitination of FBXW7, leading to the increased stability of both FBXW7 and of its substrates simultaneously (140). USP36 is another DUB antagonizing the degradation of c-myc mediated by FBXW7γ to maintain the stability of c-myc in nucleolus (141–143). Moreover, USP9X interacts with FBXW7 and protects it from autoubiquitination, which results in the suppression on substrates of FBXW7 and maintain intestinal homeostasis via controlling c-myc and NOTCH1 (144). In short, DUBs not only interact with proteins to cut off ubiquitins but also interact with FBXW7 to influence its function on substrates.
4. FBXW7 in Immunity
4.1 FBXW7-Mediated Immune Evasion Promotes Carcinogenicity
Both the regulations and degradation functions of FBXW7 have been reviewed above, and we want to further explore whether it is involved in immunity for immunity has a crucial connection with most diseases including cancers. Immunity has an intimated correlation with the health of mammals from birth to death in controlling the equilibrium state of bodies and causing harmful response under unbalanced immune homeostasis. Chances are that immune evasion happens when immunity is suppressed. Studies have demonstrated that FBXW7 is involved in the regulation of immune evasion occurring both in anti-virus and anti-tumor immunoreaction. Porcine epidemic diarrhea virus (PEDV) was found to promote FBXW7 degradation in a UPS-dependent way to attenuate the antiviral reaction (145). Nonstructural protein (nsp2) encoded by PEDV was identified to be the component interplaying with FBXW7 and contributing to degradation of FBXW7 via disturbing the stability of retinoic acid-inducible gene I (RIG-I), which results in reduced production of IFN I (145, 146). The involvement of FBXW7 in immune evasion was also reported in cancer cells (15). Eyes absent homolog 2 (EYA2) could be recognized by SCFFBXW7 and further accept the final destiny of being degraded. FBXO7, one of the F-box proteins, is capable of binding and stabilizing EYA2 in an SCF-independent way to protect EYA2 against FBXW7-mediated degradation so as to facilitate AXL-mediated immune evasion (15).
As it is known, immune evasion is one of the mechanisms of tumorigenesis. Therefore, FBXW7 might be involved in immunity-related tumorigenesis and immunotherapy resistance.
4.2 FBXW7 Influences the Cancer Progression Through Its Effect on Immune Cells
4.2.1. Macrophages
Macrophages are involved both in innate immune response and adaptive immune response. FBXW7 participates in the polarization of tumor-associated macrophages via different pathways (9, 46). The CCAAT/enhancer-binding protein-δ (C/EBPδ, Cebpd), an inflammation related gene regulated by NF-κB and ATF3, functions to amplify innate immune response via identifying the status of Toll-like receptor 4 (TLR4)–induced signals (TLR4 could induce the activation of macrophages) (16). FBXW7 was revealed to weaken the inflammatory pathway by targeting C/EBPδ as it was phosphorylated to further negatively regulate TLR4 and its reaction to ligand lipopolysaccharide (LPS) (147). Another research found that MiR-223 downregulated FBXW7 and TLR4 expression in macrophages, which modulated the inflammatory reaction of macrophage to LPS (148). However, when FBXW7 was repressed by estrogen receptor α (Erα) in breast cancer, high expression of C/EBPδ was revealed to attenuate the carcinogenicity of cancer cells through suppressing expression of the SNAI2, which is different from a previous research correlated with the role of C/EBPδ in breast cancer (34, 149). Mice lacking in FBXW7 show improved expression of chemokine C-C Motif Chemokine Ligand 2 (CCL2) in serum, which contributed to the recruitment of macrophages and monocytic myeloid-derived cells and then led to the metastasis of tumor (11). The similar negative relationship between FBXW7 and CCL2 was observed in serum of human later (150). By contrast, the deficiency of FBXW7 in CX3CR1hi macrophages enhanced the abundance of CCL2/CCL7 to induce intestinal inflammation (151). In the study of Zhang et al., FBXW7 inhibited by calcium/calmodulin-dependent protein kinase IV (CaMKIV) promoted the upregulation of mTOR in macrophages, resulting in the LPS-induced autophagy of macrophages subsequently (152). FBXW7 deficiency in myeloid cells promotes the recruitment of monocyte-macrophages in pulmonary tissue, facilitating the collagen deposition induced by bleomycin and finally developing into progressive pulmonary fibrosis (153). Moreover, the suppression of MCL-1 by FBXW7 in M2 macrophages demonstrated an improved apoptosis and repressed EMT phenotype of colon cancer cells (8). Data from timer 2.0 show that the expression of FBXW7 displays a positive correlation with macrophages infiltration level in colon adenocarcinoma (COAD) (Figure 2). A metabolism-related pathway of FBXW7 deficiency in myeloid results in the loss of substrate flux via pentose phosphate pathway, which causes a decreasing production of equivalents [nicotinamide adenine dinucleotide phosphate (NADPH) and Glutathione (GSH)] and then increases the reactive oxygen species in macrophages to promote the inflammation (154).
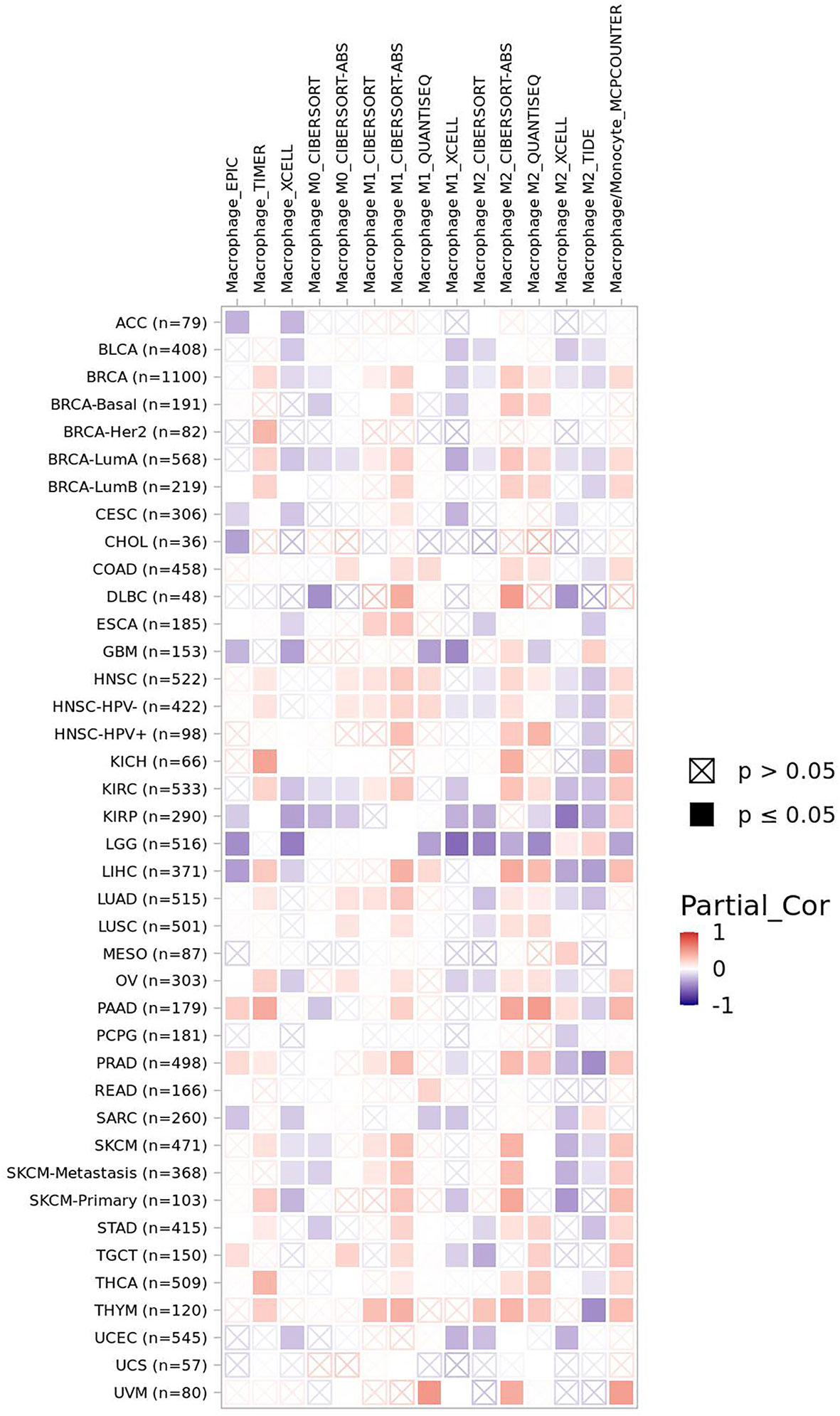
Figure 2 The correlation of FBXW7 expression with macrophages infiltration level in diverse cancer types (data from timer 2.0).
4.2.2. NK Cells
EYA2 mentioned above can be degraded by SCFFBXW7-mediated ubiquitination. Downregulation of EYA2 leads to weakened mesenchymal phenotypes, improved immunogenicity of cancer cells, decreased carcinogenicity including tumor growth and metastasis, increased infiltration level of natural killer cells (NK cells), and cytotoxic T cells. As a result, a favorable anti–PD-L1 therapy occurs in mice tumor models (15). The correlation between FBXW7 and NK cells demonstrates a positive interaction for curing tumors. Infiltration level of NK cells basically is positively associated with FBXW7 expression in different cancer (Figure 3).
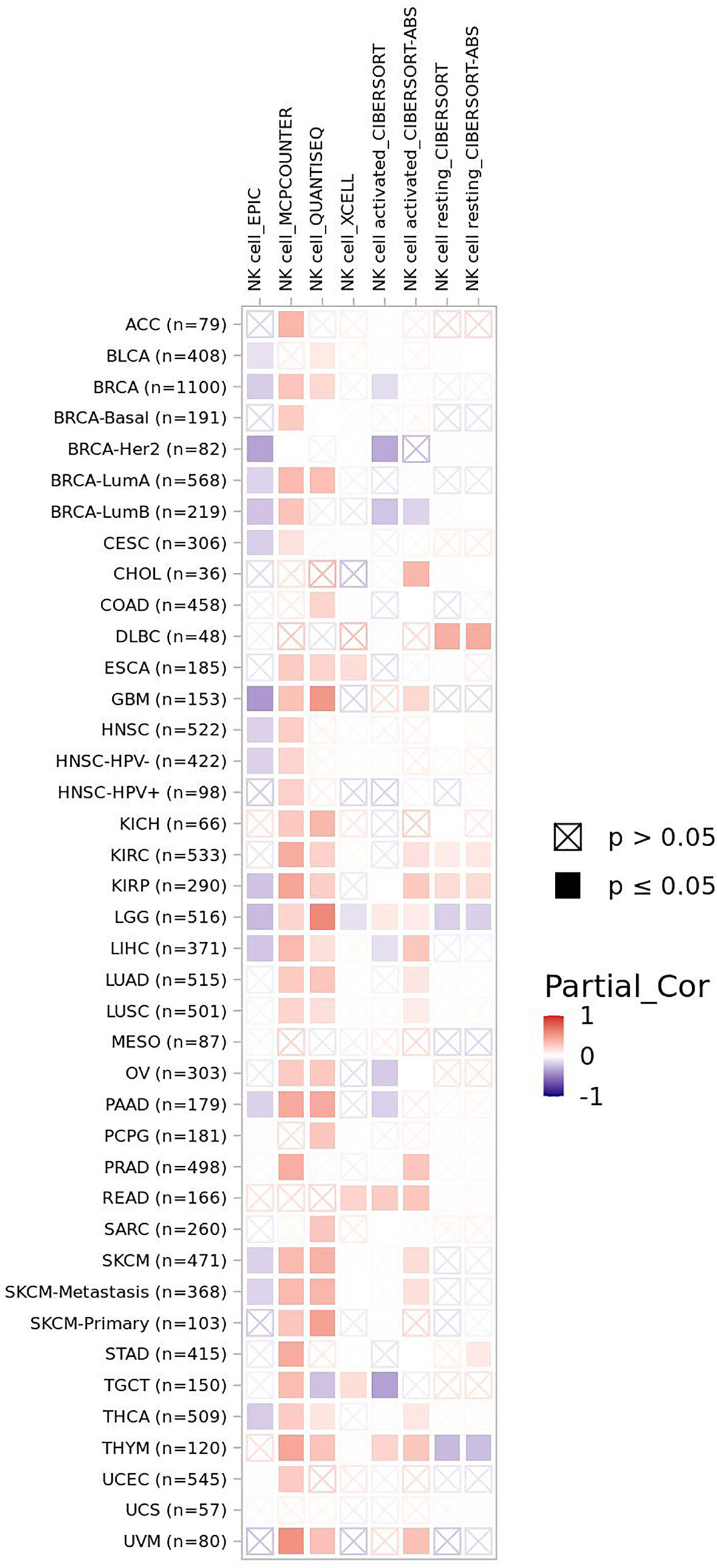
Figure 3 The correlation of FBXW7 expression with NK cells infiltration level in diverse cancer types (data from timer 2.0).
4.2.3. Lymphocytes
FBXW7 is a critical regulator to maintain the quiescence and self-renewal of hematopoietic stem cells (HSCs) via regulating four critical genes, including Ccnd1, Evi1, Pbx3, and Meis1, participating in differentiation of HSCs. On regulation of lymphocytes, FBXW7 deficiency of progenitors in bone marrow lose the ability to colonize the thymus, resulting in a significant shortage of T lymphocyte progenitors and an obvious decline of all B lymphocytes, and FBXW7 is considered as a driver gene for CLL ascribe to its mutations and degradation of NOTCH1 (155, 156).
4.2.3.1. B Lymphocytes
B lymphocyte plays an important role in keeping of immunity and immune tolerance (157). FBXW7 is of critical significance to maintain the mature B lymphocytes populations in mice. B-cell receptors (BCRs) stimulation of B cells lead to their apoptosis and stasis of proliferation and growth, owing to FBXW7 deficiency in mice (10). The volume of FBXW7-deficeient B cells is smaller that of normal group after accepting the stimulation of anti-IgM, displaying that FBXW7 matters a lot to BCR-mediated proliferation and survival of B cells (157). On condition that FBXW7 is absent in B cells, Ig class-switching is destroyed, functions of GC (germinal center) including CSR (class switch recombination) and affinity maturation of antibody are badly impaired, and memory antibody response is whittled, which might be the result of FBXW7 affecting BCL6, a protein of great importance to initiate and maintain the GC reaction (157). As shown in the mice model case of collagen-induced arthritis, a slower disease induction stage, a later disease onset, a lighter disease severity, a lower disease incidence, and a gentler joint destruction occur in FBXW7-deleted mice compared with control group, in accordance with the parallelly decreased anti-CII autoantibodies (157). Collectively, a latent treatment related to FBXW7 to cure GC-connected and autoantibody-induced autoimmune diseases is offered. FBXW7 expression presents a positive correlation of B-cell infiltration level in most cancers (Figure 4).
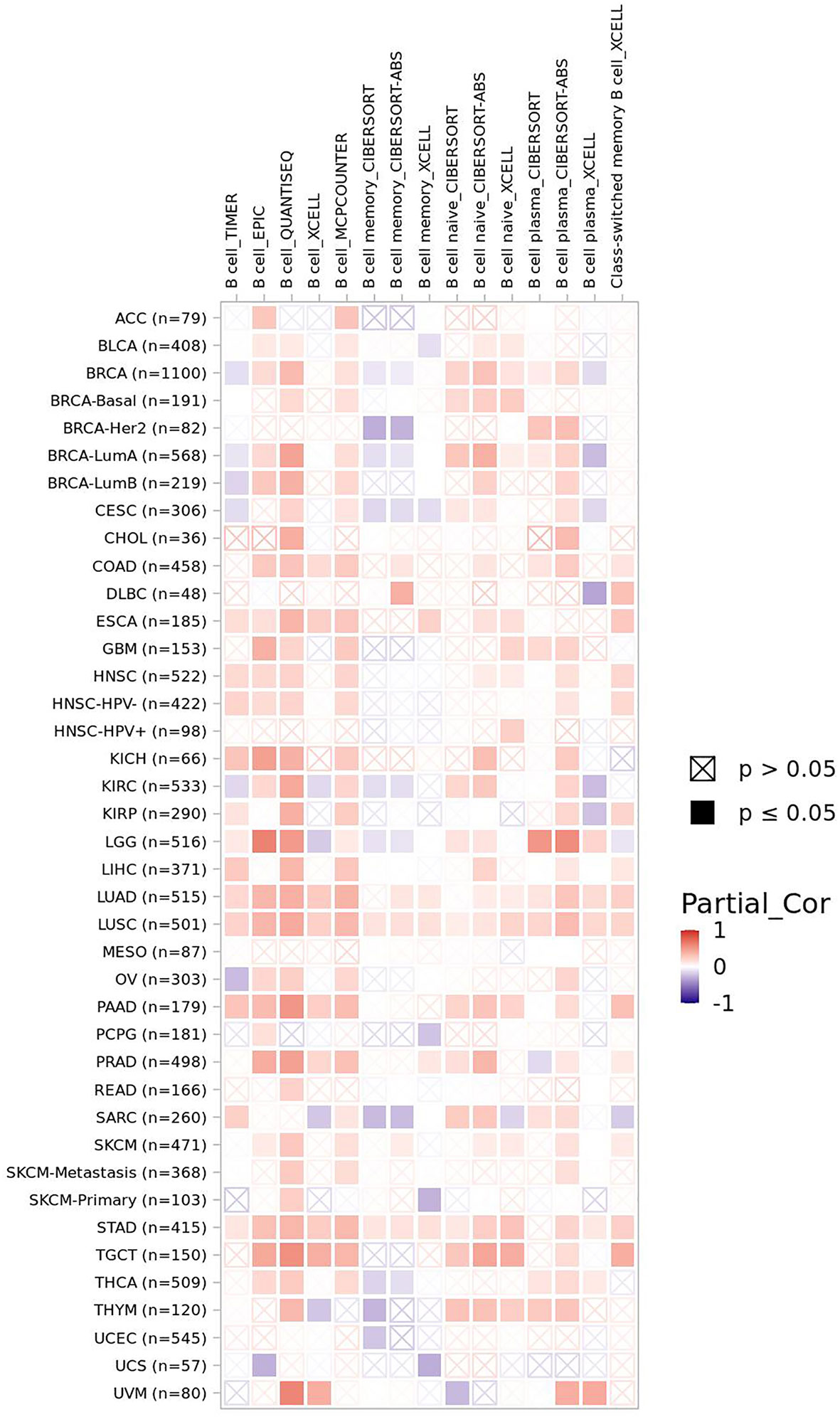
Figure 4 The correlation of FBXW7 expression with B-cell infiltration level in diverse cancer types (data from timer 2.0).
4.2.3.2. T Lymphocytes
FBXW7 deletion in T cells results in enhanced cell proliferation, which expresses both CD4 and CD8 (double-positive, DP); however, the anticipation improvement of single-positive (SP) T cells does not appear as expected, instead DP cells may develop into lymphoma due to uncontrollable cell cycle caused by accumulated c-myc at last (158). It demonstrates that either DP cells are uncapable of performing positive selection or the proliferative and survival ability of SP T cell is injured due to the loss of FBXW7. The former explanation was excluded because positive selection does not take part in cell circle progression, and the latter explanation was remained for it is consistent with the result of experiment. GATA3, a T-cell differentiation regulator, was revealed to be a substrate of FBXW7 taking an effect on the development and differentiation of T cells at the DN (CD4/CD8 double-negative) phase (159). Sox12 is able to promote the degradation of GATA3 mediated by FBXW7 in Th2 cells to inhibit the differentiation of Th2 cells and subsequently weaken allergic inflammation (160). The loss of FBXW7 was also reported to lead to T-ALL (161). NOTCH1/FBXW7 mutation shows a positive correlation with prednisone response against T-ALL (162, 163). The reason patients with FBXW7 mutations demonstrate an incline of prednisone response might lie in the glucocorticoid receptor α (GRα) serving as one of the targets of FBXW7 (164). All mutations of FBXW7 gene are limited within exons 9 and 10, which are the regions functioning to encode the C-terminal binding site to bind substrates (163). Moreover, enhancer of zeste homolog 2 (EZH2) is reported to activate the expression of T-cell multifunctional cytokines and facilitate its survival owing to inhibiting NUMB and FBXW7 that target NOTCH for degradation (165). Here, we show the correlation of FBXW7 expression with CD4+ T cells, CD8+ T cells, and Tregs infiltration level in multiple cancer types (Figure 5).
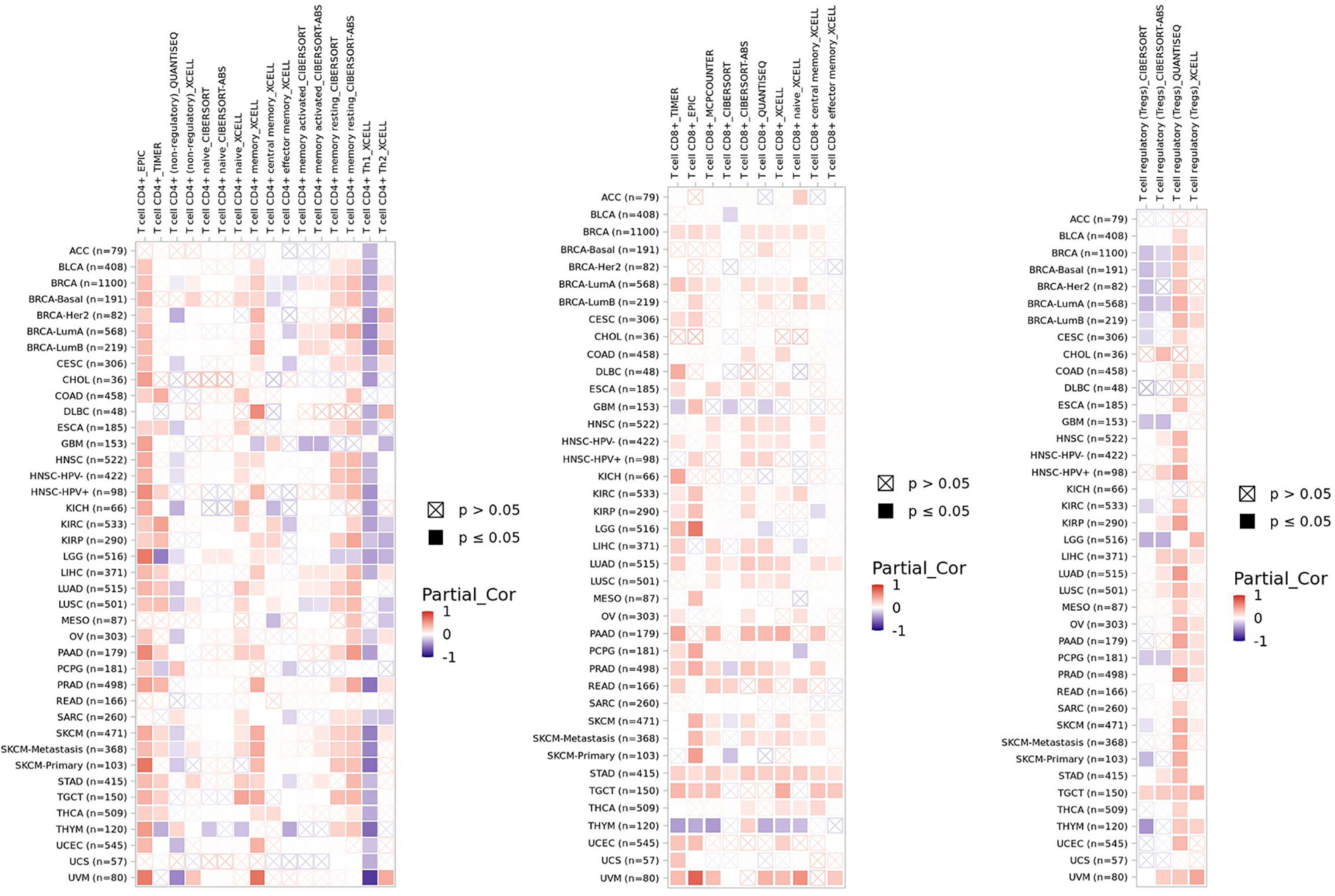
Figure 5 The correlation of FBXW7 expression with CD4+ T cells, CD8+ T cells, and Tregs infiltration level in diverse cancer types (data from timer 2.0).
To summarize, four kinds of immune cells including macrophages, NK cells, B lymphocytes, and T lymphocytes areregulated by FBXW7. Because FBXW7 functions as a regulator of immune cells, we want to figure out how it works in immunotherapy.
4.3. FBXW7 Is Involved in Immunotherapy in Multiple Cancers
4.3.1. Renal Cell Cancer
FBXW7 mutation has been discovered in plentiful human cancers including renal cell cancer (Figure 6), and it is one the 10 most frequently mutated genes in metastatic tissues of renal cancer (166). NFAT1 is one of the critical factors of activated T-cell (NFAT) family participating in innate and adaptive immunoreaction (167, 168). NFAT1 improves the expression of PD-L1 by means of boosting TNF abundance in renal cancer to promote the proliferation of renal cancer cells and regulate immunoreaction via multiple signaling pathways (169). The expression of PD-L1 regulated by RRM2–ANXA1–AKT axis affects sensitivity to sunitinib and ICIs to cancer cells in renal cell cancer (170). FBXW7 induces the degradation of NFAT1 that is phosphorylated by PI3K/AKT/GSK-3β in a UPS-mediated pathway. Downregulation of the expression of NFAT1 follows with downregulation of PD-L1, which facilitates the tumor cytotoxicity of PD-1 antibodies and infiltration of T lymphocytes (169).
4.3.2 Prostate Cancer
MUC1-C, which could facilitate the expression of IFNGR1 via inhibiting FBXW7 expression, drives dedifferentiation of castrate-resistant prostate cancer (CRPC) cell in a chromatin remodeling-dependent way (48). The enhanced stability of IFNGR1 leads to stimulation of the type II interferon-gamma (IFN-γ) inflammatory reaction pathway in prostate carcinomas. In return, the silencing of MUC1-C improves the expression of FBXW7 both at the level of mRNA and proteins. IFN-γ stimulating the IFNGR1 receptor complex induces the transcription factors including Signal Transducer and Activator of Transcription 1 (STAT1) and Interferon Regulatory Factor 1 (IRF1) to actuate the type II IFN response genes and chronic inflammatory reaction in prostate carcinoma cells (48).
4.3.3 Glioblastoma
As a specific inhibitor for NEDD8-activating enzyme, Pevonedistat (also known as MLN4924) is capable of suppressing the degradation function of FBXW7 E3 ligase and leading to the improved stability of c-myc, a critical transcriptional activation factor of PD-L1 binding to the promoter region of PD-L1 gene (171), to promote the expression of PD-L1 (172). In this way, the killing effect of T cells was weakened via PD-L1 improvement. However, the inactivation of the cytotoxic effect of T cells induced by Pevonedistat was rescued by PD-L1 blockade. Then, an interesting phenomenon occurs; the treatment of Glioblastoma acquired a better effect through the integration of pevonedistat and anti–PD-L1 drugs than that of each drug alone. In short, the integration of pevonedistat and anti–PD-L1 drugs offers a novel method to cure glioblastoma.
4.3.4 Breast Cancer
HSF1 phosphorylated by gsk3β and ERK1 at Ser303 and Ser307 is revealed as one of the substrates of FBXW7 (173). Nevertheless, the phosphorylation at another amino acid residue, Thr120, by PIM2 protects it from being degrading by FBXW7 and results in the accumulation of HSF1, which subsequently induces the expression of PD-L1 in breast cancer and enhances growth of breast cancer (174). This may offer a potential target for anti–PD-L1 drugs treatment.
In addition, the investigation by Singh et al. illustrates that E74-like transcription factor 5 (Elf5) facilitates the expression of FBXW7 through binding to the enhancer region of FBXW7 (51). The deficiency of Elf5 downregulates the expression of FBXW7 and confers the accumulation of IFNGR1 as a result of the deleted ubiquitination of IFNGR1 in breast cancer cells. The IFN-γ signaling pathway therewith is promoted via the elevation of IFNGR1 abundance, which facilitates the propagation of neutrophils as well as the potential proliferation and metastasis of breast cancer. The abundance of PD-L1 is improved due to elevation of IFNGR1. As anticipated, the carcinogenicity induced by IFNGR1 could be blocked by PD1 and PD-L1 inhibitors.
4.3.5 Colorectal Cancer
Phoaphoinositide 3-kinase γ (PI3Kγ) is an isotype of PI3K that elicits an effect on the regulation of metabolic pathways in inflammation and oncogenicity (175). PI3Kγ expresses lavishly in macrophages but has no expression in cancer cells (176). As discussed above, an axis concerning FBXW7–MCL-1 is associated with the features of macrophages in colorectal cancer, and suppression of PI3Kγ elicits the reversion of cancer progression in a FBXW7–MCL-1–dependent way (8). PI3Kγ alters the function of macrophages between the status of immunological tolerance and immune surveillance by affecting abundance of cytokines (pro-inflammatory factors: IL-1α, IL-1β, CXCL10, IL-8, and IL-12β; anti-inflammatory factors: TGF-β and IL-10) (8). Hence, PI3Kγ of macrophage is likely to fulfill a function for immunotherapy in colon cancer.
4.3.6 Melanoma
FBXW7 mutation functions as a driver to initiate melanoma by activating NOTCH1 (177). In addition, EZH2 improves the abundance of NOTCH via suppressing the inhibitors (NUMB and FBXW7) of NOTCH to attenuate the activation of Bcl-2 and to weaken the polyfunctionality and survival of effector T cells (165). Gstalder et al. found that dysfunction of FBXW7 caused by mutation has a correlation with the resistance to pembrolizumab in melanoma patients (178). To uncover the sealed mechanism, they constructed a FBXW7 deficiency melanoma model in mice and obtained similar consequence as patients. Absence of FBXW7 remolds tumor immune microenvironment into an inclination of weaker response to anti-virus and anti-tumors by means of decreasing IFN-γ–related genes, which are with respect to type I interferon stimulation or viral sensing and the amount of multiple immune cells in tumors. In contrast, the presence of FBXW7 maintaining the stability of Rig-I and melanoma differentiation-associated protein 5 (Mda5) facilitates the dsRNA sensing to further enhance interferon pathway and then boost the sensitivity of anti–PD-1 against tumors. Moreover, the viral sensing pathway could be restored by overexpressing mitochondrial antiviral-signaling protein (Mavs) or interferon regulatory factor 1 (Irf1). Nevertheless, the only fly in the ointment is that the mechanism of FBXW7 affecting Rig-1 and Mda5 still needs further exploration.
4.3.7 Lung Cancer
FBXW7 mutation is associated with unfavorable response to patients with Lung Squamous Cell Carcinoma (LUSC) treated with adjuvant therapy (179), which is conversed to its effects in chemotherapy as a tumor suppression gene. Zhong et al. revealed an evident augment of M2-like TAM and aggressive tumor progression via inoculating subcutaneously with Lewis lung cancer cells (LLCs) into mice without myeloid FBXW7 (9). The mechanisms that M2-like TAMs facilitate the propagation and metastasis of LLCs may be as follows. FBXW7 induces degradation of c-myc in a UPS-dependent pathway at the post-translational level. Consequently, the deficiency of FBXW7 results in the accumulation of c-myc, which ulteriorly improves the expression of M2-related genes both at the level of mRNA and proteins. Then, the polarization of M2 macrophages occurs and polarized M2 macrophages facilitate the expression of pro-tumor factors to motivate the progression of LLCs. In this way, chances are that novel targets for tumor immunotherapy are found out. In comparison, a clinical research (180) of non–small cell lung cancer unveiled that patients with mutation of FBXW7 profit more from immunotherapy than those of without mutations, which might be on account of improved infiltration level of M1 macrophages and CD8 T cells as well as the enhanced immunogenicity associated with FBXW7 mutation.
4.3.8 Hematological Malignancies
T-cell receptor (TCR) gene therapy serves as an unconventional immunotherapy that isolates TCR genes from antigen-specific T lymphocytes and then transfer TCR gene into T lymphocytes of patients to amplify abundant antigen-specific lymphocytes (181). Then, antigen-specific lymphocytes are adoptively transferred into patients to exert the function of anti-tumor. As mentioned, FBXW7 is often mutated in hematological malignancies. The mutation of FBXW7 is used to isolate CD8 T cells from healthy donor. CD8 T cells specific for an HLA-A*11:01–presented mutant FBXW7(mFBXW7) peptide were triumphantly isolated, which are capable of recognizing targeting cells edited to express mFBXW7. The recurrent mutation of pR465H in FBXW7 was found to encode an HLA-A*11:01–presented neoepitope, which could be applied into the treatment of hematological malignancies via TCR gene therapy.
4.3.9 Coronavirus Disease 2019
Coronavirus disease 2019 (COVID-19), which is caused by severe acute respiratory syndrome coronavirus 2 (SARS-CoV-2), is still a severe ongoing contagious disease causing thousands of millions of deaths worldwide. In human lung cells, RIG-I suppresses the replication of SARS-CoV-2 without the participation of type I/III IFN (182). The 3′UTR of viral RNA is recognized by RIG-I via its helicase domains rather than the C-terminal domain. FBW7 is capable of maintaining the stability of RIG-I (178). Therefore, it is possible that FBW7 is capable of interfering with the viral RNA synthesis in the early stage of SARS-CoV-2 invasion via stabling RIG-I. Moreover, the expression of PD-1and PD-L1 was reported to increase in patients with severe COVID-19 (183). The abundance of PD-1 demonstrates a closed correlation with the severity of the disease (184). It is rational that ICIs could serve as a promising treatment against COVID-19 in this way. However, patients treating with PD-1/PD-L1 blockade show neither advantages nor disadvantages to their recovery (183).
4.3.10 Others
Furthermore, the mutation of FBXW7 is reported to be associated with immunotherapy resistance in endometrial and pancreatic cancer (185) (details are unknown).
All in all, FBXW7 functions discrepantly in immunotherapy of different cancers. However, it fails to work in COVID-19. More work should be done to investigate the function of FBXW7 in various cancers for so many proteins can be ubiquitinated by SCFFBXW7.
5. Conclusion
In general, FBXW7 functions as a suppressor of tumor by means of promoting the degradation of proteins correlated with carcinogenicity, such as c-myc, cyclin E, NOTCH1, and HIF1α (186–191). The mechanism of FBXW7 recognizing proteins and inducing the UPS-dependent degradation has been almost elucidated. Therefore, here, we lay emphasis on its own regulation by various pathways, such as epigenetic regulation, miRNA, circRNA, lncRNA, dimerization, phosphorylation, and autoubiquitination to offer neo-targets for exploring the novel methods of cancer or other diseases treatment via regulating the expression of FBXW7. Moreover, previous studies have attached more importance to the effect of FBXW7 on carcinostasis and chemoradiotherapy (64, 117, 192–194) and targeted therapies (195–197), whereas its influence on immunotherapy is ignored to a certain extent. In consequence, we summarize the role of FBXW7 principally in immune cells and in immunotherapy. Here, we demonstrate the relative substrates of FBXW7 functioning in immunity in different cancers (Figure 7). In accordance with its role as tumor suppressor, mutation or downregulation of FBXW7 is more likely to contribute to resistance of immunotherapy rather than the opposite. Nevertheless, increasing efforts still need to be taken in unveiling the mysterious regulations, such as how FBXW7 regulating Rig-1 and Mda5 (178). In summary, FBXW7 could be utilized as a target to improve the sensitivity of immunotherapy or that with the combination of other treatment to benefit patients suffering from cancers.
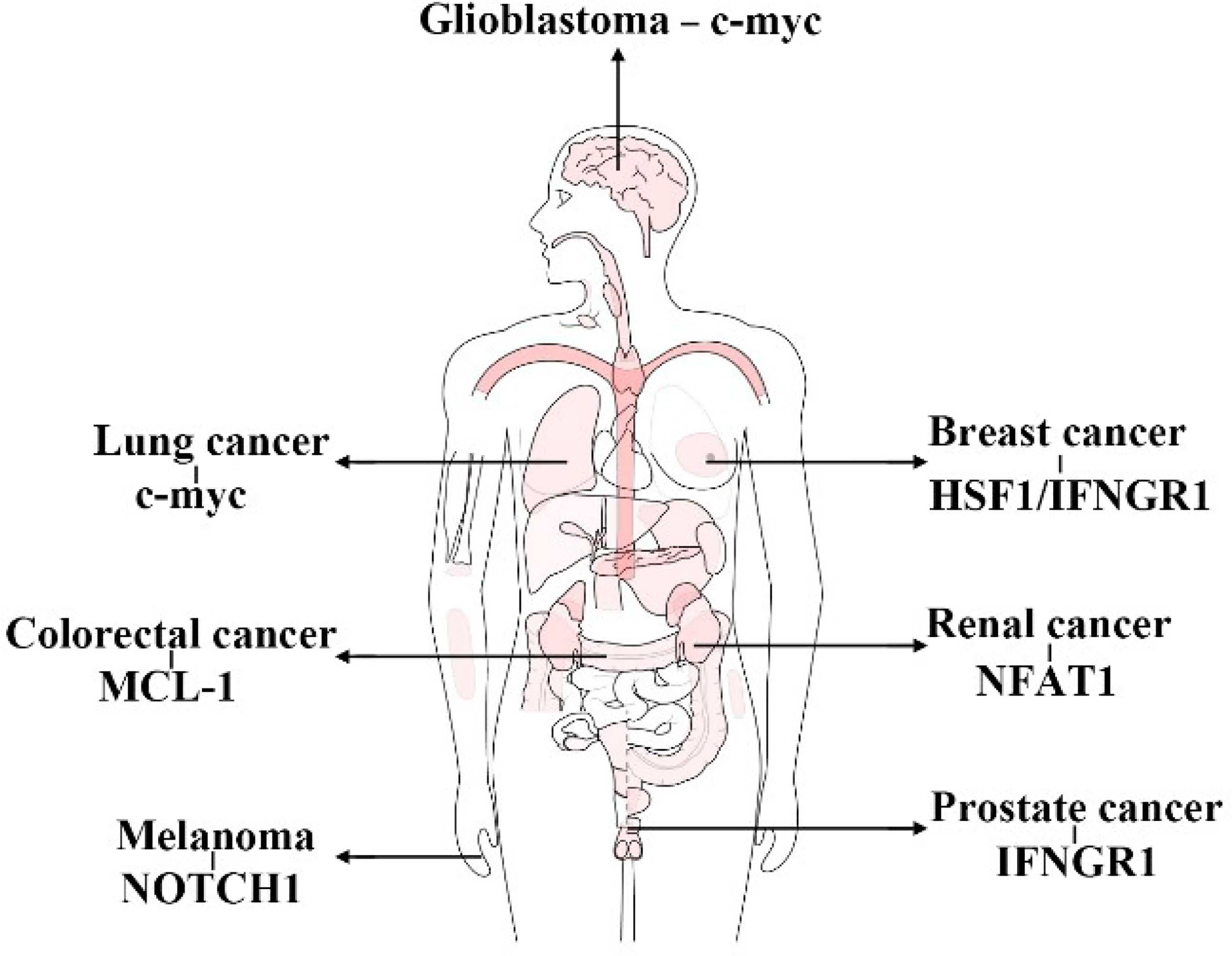
Figure 7 Substrates of FBXW7 functioning in immunity in different cancers. (This figure takes GEPIA as a template).
Author Contributions
JZ and FZ designed this review. YZ and Yinggang Che searched the references. LLX and LX wrote the manuscript. MW and DQ revised the manuscript. YS and HY drew the figures. All authors contributed to the article and approved the submitted version.
Funding
This work was supported by National Natural Science Foundation of China (81773153).
Conflict of Interest
The authors declare that the research was conducted in the absence of any commercial or financial relationships that could be construed as a potential conflict of interest.
Publisher’s Note
All claims expressed in this article are solely those of the authors and do not necessarily represent those of their affiliated organizations, or those of the publisher, the editors and the reviewers. Any product that may be evaluated in this article, or claim that may be made by its manufacturer, is not guaranteed or endorsed by the publisher.
Acknowledgments
The authors would like to thank all the researchers of references cited in this review.
References
1. Nakajima T, Morita K, Ohi N, Arai T, Nozaki N, Kikuchi A, et al. Degradation of Topoisomerase IIalpha During Adenovirus E1A-Induced Apoptosis is Mediated by the Activation of the Ubiquitin Proteolysis System. J Biol Chem (1996) 271(40):24842–9. doi: 10.1074/jbc.271.40.24842
2. Hochstrasser M. Ubiquitin, Proteasomes, and the Regulation of Intracellular Protein Degradation. Curr Opin Cell Biol (1995) 7(2):215–23. doi: 10.1016/0955-0674(95)80031-x
3. Díaz VM, de Herreros AG. F-Box Proteins: Keeping the Epithelial-to-Mesenchymal Transition (EMT) in Check. Semin. Cancer Biol (2016) 36:71–9. doi: 10.1016/j.semcancer.2015.10.003
4. Zheng N, Zhou Q, Wang Z, Wei W. Recent Advances in SCF Ubiquitin Ligase Complex: Clinical Implications. Biochim Biophys Acta (2016) 1866(1):12–22. doi: 10.1016/j.bbcan.2016.05.001
5. Hao B, Oehlmann S, Sowa ME, Harper JW, Pavletich NP. Structure of a Fbw7-Skp1-Cyclin E Complex: Multisite-Phosphorylated Substrate Recognition by SCF Ubiquitin Ligases. Mol Cell (2007) 26(1):131–43. doi: 10.1016/j.molcel.2007.02.022
6. Yeh CH, Bellon M, Nicot C. FBXW7: A Critical Tumor Suppressor of Human Cancers. Mol Cancer (2018) 17(1):115. doi: 10.1186/s12943-018-0857-2
7. Yumimoto K, Nakayama KI. Recent Insight Into the Role of FBXW7 as a Tumor Suppressor. Semin. Cancer Biol (2020) 67(Pt 2):1–15. doi: 10.1016/j.semcancer.2020.02.017
8. Lee YS, Song SJ, Hong HK, Oh BY, Lee WY, Cho YB. The FBW7-MCL-1 Axis is Key in M1 and M2 Macrophage-Related Colon Cancer Cell Progression: Validating the Immunotherapeutic Value of Targeting PI3Kγ. Exp Mol Med (2020) 52(5):815–31. doi: 10.1038/s12276-020-0436-7
9. Zhong L, Zhang Y, Li M, Song Y, Liu D, Yang X, et al. E3 Ligase FBXW7 Restricts M2-Like Tumor-Associated Macrophage Polarization by Targeting C-Myc. Aging (Albany NY) (2020) 12(23):24394–423. doi: 10.18632/aging.202293
10. Ramezani-Rad P, Leung CR, Apgar JR, Rickert RC. E3 Ubiquitin Ligase Fbw7 Regulates the Survival of Mature B Cells. J Immunol (Baltimore Md 1950) (2020) 204(6):1535–42. doi: 10.4049/jimmunol.1901156
11. Yumimoto K, Akiyoshi S, Ueo H, Sagara Y, Onoyama I, Ueo H, et al. F-Box Protein FBXW7 Inhibits Cancer Metastasis in a non-Cell-Autonomous Manner. J Clin Invest (2015) 125(2):621–35. doi: 10.1172/JCI78782
12. Dunn GP, Bruce AT, Ikeda H, Old LJ, Schreiber RD. Cancer Immunoediting: From Immunosurveillance to Tumor Escape. Nat Immunol (2002) 3(11):991–8. doi: 10.1038/ni1102-991
13. Varadé J, Magadán S, González-Fernández Á. Human Immunology and Immunotherapy: Main Achievements and Challenges. Cell Mol Immunol (2021) 18(4):805–28. doi: 10.1038/s41423-020-00530-6
14. Chen DS, Mellman I. Elements of Cancer Immunity and the Cancer–Immune Set Point. Nature (2017) 541(7637):321–30. doi: 10.1038/nature21349
15. Shen JZ, Qiu Z, Wu Q, Zhang G, Harris R, Sun D, et al. A FBXO7/EYA2-SCFFBXW7 Axis Promotes AXL-Mediated Maintenance of Mesenchymal and Immune Evasion Phenotypes of Cancer Cells. Mol Cell (2022) 82(6):1123–1139.e8. doi: 10.1016/j.molcel.2022.01.022
16. Litvak V, Ramsey SA, Rust AG, Zak DE, Kennedy KA, Lampano AE, et al. Function of C/EBPdelta in a Regulatory Circuit That Discriminates Between Transient and Persistent TLR4-Induced Signals. Nat Immunol (2009) 10(4):437–43. doi: 10.1038/ni.1721
17. Craig KL, Tyers M. The F-Box: A New Motif for Ubiquitin Dependent Proteolysis in Cell Cycle Regulation and Signal Transduction. Prog Biophys Mol Biol (1999) 72(3):299–328. doi: 10.1016/s0079-6107(99)00010-3
18. Krek W. Proteolysis and the G1-S Transition: The SCF Connection. Curr Opin Genet Dev (1998) 8(1):36–42. doi: 10.1016/s0959-437x(98)80059-2
19. Skowyra D, Craig KL, Tyers M, Elledge SJ, Harper JW. F-Box Proteins are Receptors That Recruit Phosphorylated Substrates to the SCF Ubiquitin-Ligase Complex. Cell (1997) 91(2):209–19. doi: 10.1016/s0092-8674(00)80403-1
20. Zheng N, Schulman BA, Song L, Miller JJ, Jeffrey PD, Wang P, et al. Structure of the Cul1–Rbx1–Skp1–F Boxskp2 SCF Ubiquitin Ligase Complex. Nature (2002) 416(6882):703–9. doi: 10.1038/416703a
21. Bai C, Sen P, Hofmann K, Ma L, Goebl M, Harper JW, et al. SKP1 Connects Cell Cycle Regulators to the Ubiquitin Proteolysis Machinery Through a Novel Motif, the F-Box. Cell (1996) 86(2):263–74. doi: 10.1016/s0092-8674(00)80098-7
22. Wang Z, Liu P, Inuzuka H, Wei W. Roles of F-Box Proteins in Cancer. Nat Rev Cancer (2014) 14(4):233–47. doi: 10.1038/nrc3700
23. Jin J, Cardozo T, Lovering RC, Elledge SJ, Pagano M, Harper JW. Systematic Analysis and Nomenclature of Mammalian F-Box Proteins. Genes Dev (2004) 18(21):2573–80. doi: 10.1101/gad.1255304
24. Kipreos ET, Pagano M. The F-Box Protein Family. Genome Biol (2000) 1(5):REVIEWS3002–REVIEWS3002. doi: 10.1186/gb-2000-1-5-reviews3002
25. Hartwell LH, Culotti J, Reid B. Genetic Control of the Cell-Division Cycle in Yeast. I. Detection of Mutants. Proc Natl Acad Sci U.S.A. (1970) 66(2):352–9. doi: 10.1073/pnas.66.2.352
26. Gupta-Rossi N, Le Bail O, Gonen H, Brou C, Logeat F, Six E, et al. Functional Interaction Between SEL-10, an F-Box Protein, and the Nuclear Form of Activated Notch1 Receptor*. J Biol Chem (2001) 276(37):34371–8. doi: 10.1074/jbc.M101343200
27. Wu G, Lyapina S, Das I, Li J, Gurney M, Pauley A, et al. SEL-10 is an Inhibitor of Notch Signaling That Targets Notch for Ubiquitin-Mediated Protein Degradation. Mol Cell Biol (2001) 21(21):7403–15. doi: 10.1128/MCB.21.21.7403-7415.2001
28. öberg C, Li J, Pauley A, Wolf E, Gurney M, Lendahl U. The Notch Intracellular Domain Is Ubiquitinated and Negatively Regulated by the Mammalian Sel-10 Homolog*. J Biol Chem (2001) 276(38):35847–53. doi: 10.1074/jbc.M103992200
29. Spruck CH, Strohmaier H, Sangfelt O, Müller HM, Hubalek M, Müller-Holzner E, et al. Hcdc4 Gene Mutations in Endometrial Cancer1. Cancer Res (2002) 62(16):4535–9.
30. Welcker M, Orian A, Grim JA, Eisenman RN, Clurman BE. A Nucleolar Isoform of the Fbw7 Ubiquitin Ligase Regulates C-Myc and Cell Size. Curr Biol (2004) 14(20):1852–7. doi: 10.1016/j.cub.2004.09.083
31. Matsumoto A, Onoyama I, Nakayama KI. Expression of Mouse Fbxw7 Isoforms is Regulated in a Cell Cycle- or P53-Dependent Manner. Biochem Bioph Res Co (2006) 350(1):114–9. doi: 10.1016/j.bbrc.2006.09.003
32. Hou J, Liu Y, Huang P, Wang Y, Pei D, Tan R, et al. RANBP10 Promotes Glioblastoma Progression by Regulating the FBXW7/c-Myc Pathway. Cell Death Dis (2021) 12(11):967–7. doi: 10.1038/s41419-021-04207-4
33. Liu R, Gao J, Yang Y, Qiu R, Zheng Y, Huang W, et al. PHD Finger Protein 1 (PHF1) is a Novel Reader for Histone H4R3 Symmetric Dimethylation and Coordinates With PRMT5-WDR77/CRL4B Complex to Promote Tumorigenesis. Nucleic Acids Res (2018) 46(13):6608–26. doi: 10.1093/nar/gky461
34. Balamurugan K, Wang J, Tsai H, Sharan S, Anver M, Leighty R, et al. The Tumour Suppressor C/Ebpδ Inhibits FBXW7 Expression and Promotes Mammary Tumour Metastasis. EMBO J (2010) 29(24):4106–17. doi: 10.1038/emboj.2010.280
35. Sancho R, Blake SM, Tendeng C, Clurman BE, Lewis J, Behrens A. Fbw7 Repression by Hes5 Creates a Feedback Loop That Modulates Notch-Mediated Intestinal and Neural Stem Cell Fate Decisions. PloS Biol (2013) 11(6):e1001586–e1001586. doi: 10.1371/journal.pbio.1001586
36. Chen L, Hu B, Han Z, Liu W, Zhu J, Chen X, et al. Repression of FBXW7 by HES5 Contributes to Inactivation of the TGF-β Signaling Pathway and Alleviation of Endometriosis. FASEB J (2021) 35(2):e20938. doi: 10.1096/fj.202000438RRR
37. Zhang G, Zhu Q, Fu G, Hou J, Hu X, Cao J, et al. TRIP13 Promotes the Cell Proliferation, Migration and Invasion of Glioblastoma Through the FBXW7/c-MYC Axis. Br J Cancer (2019) 121(12):1069–78. doi: 10.1038/s41416-019-0633-0
38. Kimura T, Gotoh M, Nakamura Y, Arakawa H. Hcdc4b, a Regulator of Cyclin E, as a Direct Transcriptional Target of P53. Cancer Sci (2003) 94(5):431–6. doi: 10.1111/j.1349-7006.2003.tb01460.x
39. Akhoondi S, Lindström L, Widschwendter M, Corcoran M, Bergh J, Spruck C, et al. Inactivation of FBXW7/hCDC4-β Expression by Promoter Hypermethylation is Associated With Favorable Prognosis in Primary Breast Cancer. Breast Cancer Res (2010) 12(6):R105. doi: 10.1186/bcr2788
40. Gu Z, Inomata K, Mitsui H, Horii A. Promoter Hypermethylation is Not the Major Mechanism for Inactivation of the FBXW7 Beta-Form in Human Gliomas. Genes Genet Syst (2008) 83(4):347–52. doi: 10.1266/ggs.83.347
41. Gu Z, Mitsui H, Inomata K, Honda M, Endo C, Sakurada A, et al. The Methylation Status of FBXW7 Beta-Form Correlates With Histological Subtype in Human Thymoma. Biochem Biophys Res Commun (2008) 377(2):685–8. doi: 10.1016/j.bbrc.2008.10.047
42. Kitade S, Onoyama I, Kobayashi H, Yagi H, Yoshida S, Kato M, et al. FBXW7 is Involved in the Acquisition of the Malignant Phenotype in Epithelial Ovarian Tumors. Cancer Sci (2016) 107(10):1399–405. doi: 10.1111/cas.13026
43. Lu X, Jiang L, Zhang L, Zhu Y, Hu W, Wang J, et al. Immune Signature-Based Subtypes of Cervical Squamous Cell Carcinoma Tightly Associated With Human Papillomavirus Type 16 Expression, Molecular Features, and Clinical Outcome. Neoplasia (2019) 21(6):591–601. doi: 10.1016/j.neo.2019.04.003
44. Chen B, Chen H, Lu S, Zhu X, Que Y, Zhang Y, et al. KDM5B Promotes Tumorigenesis of Ewing Sarcoma via FBXW7/CCNE1 Axis. Cell Death Dis (2022) 13(4):354. doi: 10.1038/s41419-022-04800-1
45. Kim MJ, Chen G, Sica GL, Deng X. Epigenetic Modulation of FBW7/Mcl-1 Pathway for Lung Cancer Therapy. Cancer Biol Ther (2021) 22(1):55–65. doi: 10.1080/15384047.2020.1856756
46. Huang YH, Cai K, Xu PP, Wang L, Huang CX, Fang Y, et al. CREBBP/EP300 Mutations Promoted Tumor Progression in Diffuse Large B-Cell Lymphoma Through Altering Tumor-Associated Macrophage Polarization via FBXW7-NOTCH-CCL2/CSF1 Axis. Signal Transd Target Ther (2021) 6(1):10. doi: 10.1038/s41392-020-00437-8
47. Lin H, Ma N, Zhao L, Yang G, Cao B. KDM5c Promotes Colon Cancer Cell Proliferation Through the FBXW7-C-Jun Regulatory Axis. Front Oncol (2020) 10:535449. doi: 10.3389/fonc.2020.535449
48. Hagiwara M, Fushimi A, Bhattacharya A, Yamashita N, Morimoto Y, Oya M, et al. MUC1-C Integrates Type II Interferon and Chromatin Remodeling Pathways in Immunosuppression of Prostate Cancer. Oncoimmunology (2022) 11(1):2029298–2029298. doi: 10.1080/2162402X.2022.2029298
49. Hata T, Rajabi H, Takahashi H, Yasumizu Y, Li W, Jin C, et al. MUC1-C Activates the NuRD Complex to Drive Dedifferentiation of Triple-Negative Breast Cancer Cells. Cancer Res (2019) 79(22):5711–22. doi: 10.1158/0008-5472.CAN-19-1034
50. Zhao S, Choi M, Overton JD, Bellone S, Roque DM, Cocco E, et al. Landscape of Somatic Single-Nucleotide and Copy-Number Mutations in Uterine Serous Carcinoma. P Natl Acad Sci USA (2013) 110(8):2916–21. doi: 10.1073/pnas.1222577110
51. Singh S, Kumar S, Srivastava RK, Nandi A, Thacker G, Murali H, et al. Loss of ELF5-FBXW7 Stabilizes IFNGR1 to Promote the Growth and Metastasis of Triple-Negative Breast Cancer Through Interferon-γ Signalling. Nat Cell Biol (2020) 22(5):591–602. doi: 10.1038/s41556-020-0495-y
52. Wu Y, Chang N, Zhang Y, Zhang X, Xu L, Che Y, et al. METTL3-Mediated M(6)A mRNA Modification of FBXW7 Suppresses Lung Adenocarcinoma. J Exp Clin Cancer Res (2021) 40(1):90. doi: 10.1186/s13046-021-01880-3
53. Chong W, Shang L, Liu J, Fang Z, Du F, Wu H, et al. M(6)A Regulator-Based Methylation Modification Patterns Characterized by Distinct Tumor Microenvironment Immune Profiles in Colon Cancer. Theranostics (2021) 11(5):2201–17. doi: 10.7150/thno.52717
54. Bokar JA, Shambaugh ME, Polayes D, Matera AG, Rottman FM. Purification and cDNA Cloning of the AdoMet-Binding Subunit of the Human mRNA (N6-Adenosine)-Methyltransferase. RNA (1997) 3(11):1233–47.
55. Xu F, Li J, Ni M, Cheng J, Zhao H, Wang S, et al. FBW7 Suppresses Ovarian Cancer Development by Targeting the N(6)-Methyladenosine Binding Protein YTHDF2. Mol Cancer (2021) 20(1):45. doi: 10.1186/s12943-021-01340-8
56. Gao H, Ma J, Cheng Y, Zheng P. Exosomal Transfer of Macrophage-Derived miR-223 Confers Doxorubicin Resistance in Gastric Cancer. Onco Targets Ther (2020) 13:12169–79. doi: 10.2147/OTT.S283542
57. Li J, Guo Y, Liang X, Sun M, Wang G, De W, et al. MicroRNA-223 Functions as an Oncogene in Human Gastric Cancer by Targeting FBXW7/Hcdc4. J Cancer Res Clin Oncol (2012) 138(5):763–74. doi: 10.1007/s00432-012-1154-x
58. Yang L, Li Y, Wang X, Mu X, Qin D, Huang W, et al. Overexpression of miR-223 Tips the Balance of Pro- and Anti-Hypertrophic Signaling Cascades Toward Physiologic Cardiac Hypertrophy. J Biol Chem (2016) 291(30):15700–13. doi: 10.1074/jbc.M116.715805
59. Liu L, Tao T, Liu S, Yang X, Chen X, Liang J, et al. An RFC4/Notch1 Signaling Feedback Loop Promotes NSCLC Metastasis and Stemness. Nat Commun (2021) 12(1):2693–3. doi: 10.1038/s41467-021-22971-x
60. Liu Z, Liu X, Liu S, Cao Q. Cholesterol Promotes the Migration and Invasion of Renal Carcinoma Cells by Regulating the KLF5/miR-27a/FBXW7 Pathway. Biochem Biophys Res Commun (2018) 502(1):69–75. doi: 10.1016/j.bbrc.2018.05.122
61. Meng Q, Wu W, Pei T, Xue J, Xiao P, Sun L, et al. miRNA-129/FBW7/NF-κb, a Novel Regulatory Pathway in Inflammatory Bowel Disease. Mol Ther Nucleic Acids (2020) 19:731–40. doi: 10.1016/j.omtn.2019.10.048
62. Zeng R, Huang J, Sun Y, Luo J. Cell Proliferation is Induced in Renal Cell Carcinoma Through miR-92a-3p Upregulation by Targeting FBXW7. Oncol Lett (2020) 19(4):3258–68. doi: 10.3892/ol.2020.11443
63. Liu S, Liu H, Deng M, Wang H. MiR-182 Promotes Glioma Progression by Targeting FBXW7. J Neurol Sci (2020) 411:116689. doi: 10.1016/j.jns.2020.116689
64. Lu B, Feng Z, Fan B, Shi Y. Blocking miR-27a-3p Sensitises Taxol Resistant Osteosarcoma Cells Through Targeting Fbxw7. Bull Cancer (2021) 108(6):596–604. doi: 10.1016/j.bulcan.2021.01.006
65. Ben W, Zhang G, Huang Y, Sun Y. MiR-27a-3p Regulated the Aggressive Phenotypes of Cervical Cancer by Targeting Fbxw7. Cancer Manag Res (2020) 12:2925–35. doi: 10.2147/CMAR.S234897
66. Bai C, Ren Q, Liu H, Li X, Guan W, Gao Y. miR-212/132-Enriched Extracellular Vesicles Promote Differentiation of Induced Pluripotent Stem Cells Into Pancreatic Beta Cells. Front Cell Dev Biol (2021) 9:673231. doi: 10.3389/fcell.2021.673231
67. Wang Y, Shi S, Wang Y, Zhang X, Liu X, Li J, et al. miR-223-3p Targets FBXW7 to Promote Epithelial-Mesenchymal Transition and Metastasis In Breast Cancer. Thorac Cancer (2022) 13(3):474–82. doi: 10.1111/1759-7714.14284
68. Ren L, Yang J, Meng X, Zhang J, Zhang Y. The Promotional Effect of microRNA-103a-3p in Cervical Cancer Cells by Regulating The Ubiquitin Ligase FBXW7 Function. Hum Cell (2022) 35(2):472–85. doi: 10.1007/s13577-021-00649-2
69. Feng X, Zou B, Nan T, Zheng X, Zheng L, Lan J, et al. MiR-25 Enhances Autophagy and Promotes Sorafenib Resistance of Hepatocellular Carcinoma via Targeting FBXW7. Int J Med Sci (2022) 19(2):257–66. doi: 10.7150/ijms.67352
70. Wang J, Li T, Wang B. Exosomal Transfer of Mir−25−3p Promotes the Proliferation and Temozolomide Resistance of Glioblastoma Cells by Targeting FBXW7. Int J Oncol (2021) 59(2):64. doi: 10.3892/ijo.2021.5244
71. Tian X, Liu Y, Wang Z, Wu S. miR-144 Delivered by Nasopharyngeal Carcinoma-Derived EVs Stimulates Angiogenesis Through the FBXW7/HIF-1α/VEGF-A Axis. Mol Ther Nucleic Acids (2021) 24:1000–11. doi: 10.1016/j.omtn.2021.03.016
72. Li Y, Wang J, Ma Y, Du W, Feng K, Wang S. miR-101-Loaded Exosomes Secreted by Bone Marrow Mesenchymal Stem Cells Requires the FBXW7/HIF1α/FOXP3 Axis, Facilitating Osteogenic Differentiation. J Cell Physiol (2021) 236(6):4258–72. doi: 10.1002/jcp.30027
73. Yi X, Lou L, Wang J, Xiong J, Zhou S. Honokiol Antagonizes Doxorubicin Resistance in Human Breast Cancer via miR-188-5p/FBXW7/c-Myc Pathway. Cancer Chemother Pharmacol (2021) 87(5):647–56. doi: 10.1007/s00280-021-04238-w
74. Lin H, Zhang L, Zhang C, Liu P. Exosomal MiR-500a-3p Promotes Cisplatin Resistance and Stemness via Negatively Regulating FBXW7 in Gastric Cancer. J Cell Mol Med (2020) 24(16):8930–41. doi: 10.1111/jcmm.15524
75. Schmitz SU, Grote P, Herrmann BG. Mechanisms of Long Noncoding RNA Function in Development and Disease. Cell Mol Life Sci (2016) 73(13):2491–509. doi: 10.1007/s00018-016-2174-5
76. Ponting CP, Oliver PL, Reik W. Evolution and Functions of Long Noncoding RNAs. Cell (2009) 136(4):629–41. doi: 10.1016/j.cell.2009.02.006
77. Wang KC, Chang HY. Molecular Mechanisms of Long Noncoding RNAs. Mol Cell (2011) 43(6):904–14. doi: 10.1016/j.molcel.2011.08.018
78. Zhang P, Cao L, Fan P, Mei Y, Wu M. LncRNA-MIF, a C-Myc-Activated Long non-Coding RNA, Suppresses Glycolysis by Promoting Fbxw7-Mediated C-Myc Degradation. EMBO Rep (2016) 17(8):1204–20. doi: 10.15252/embr.201642067
79. Cao S, Wang Y, Li J, Lv M, Niu H, Tian Y. Tumor-Suppressive Function of Long Noncoding RNA MALAT1 in Glioma Cells by Suppressing miR-155 Expression and Activating FBXW7 Function. Am J Cancer Res (2016) 6(11):2561–74.
80. Wang Y, Liu Z, Yao B, Li Q, Wang L, Wang C, et al. Long non-Coding RNA CASC2 Suppresses Epithelial-Mesenchymal Transition of Hepatocellular Carcinoma Cells Through CASC2/miR-367/FBXW7 Axis. Mol Cancer (2017) 16(1):123. doi: 10.1186/s12943-017-0702-z
81. Liu X, Ma J, Xu F, Li L. TINCR Suppresses Proliferation and Invasion Through Regulating miR-544a/FBXW7 Axis In Lung Cancer. Biomed Pharmacother (2018) 99:9–17. doi: 10.1016/j.biopha.2018.01.049
82. Zhang G, Li S, Lu J, Ge Y, Wang Q, Ma G, et al. LncRNA MT1JP Functions as a ceRNA in Regulating FBXW7 Through Competitively Binding To miR-92a-3p in Gastric Cancer. Mol Cancer (2018) 17(1):87. doi: 10.1186/s12943-018-0829-6
83. Huo W, Qi F, Wang K. Long non-Coding RNA FER1L4 Inhibits Prostate Cancer Progression via Sponging miR-92a-3p and Upregulation of FBXW7. Cancer Cell Int (2020) 20:64. doi: 10.1186/s12935-020-1143-0
84. Chen H, Ali M, Ruben A, Stelmakh D, Pak M. E2F6-Mediated Downregulation of MIR22HG Facilitates the Progression of Laryngocarcinoma by Targeting the miR-5000-3p/FBXW7 Axis. Mol Cell Biol (2020) 40(10):e00496–19. doi: 10.1128/MCB.00496-19
85. Miao S, Wang J, Xuan L, Liu X. LncRNA TTN-AS1 Acts as Sponge for miR-15b-5p to Regulate FBXW7 Expression in Ovarian Cancer. Biofactors (2020) 46(4):600–7. doi: 10.1002/biof.1622
86. Zhang J, Zhou M, Zhao X, Wang G, Li J. Long Noncoding RNA LINC00173 is Downregulated in Cervical Cancer and Inhibits Cell Proliferation and Invasion by Modulating the miR-182-5p/FBXW7 Axis. Pathol Res Pract (2020) 216(8):152994. doi: 10.1016/j.prp.2020.152994
87. Yuning F, Liang C, Tenghuan W, Zhenhua N, Shengkai G. Knockdown of lincRNA PADNA Promotes Bupivacaine-Induced Neurotoxicity by miR-194/FBXW7 Axis. Mol Med (2020) 26(1):79. doi: 10.1186/s10020-020-00209-8
88. Wu L, Liu Q, Fan C, Yi X, Cheng B. MALAT1 Recruited the E3 Ubiquitin Ligase FBXW7 to Induce CRY2 Ubiquitin-Mediated Degradation and Participated in Trophoblast Migration and Invasion. J Cell Physiol (2021) 236(3):2169–77. doi: 10.1002/jcp.30003
89. Huang G, Xiang Z, Wu H, He Q, Dou R, Lin Z, et al. The lncRNA BDNF-AS/WDR5/FBXW7 Axis Mediates Ferroptosis in Gastric Cancer Peritoneal Metastasis by Regulating VDAC3 Ubiquitination. Int J Biol Sci (2022) 18(4):1415–33. doi: 10.7150/ijbs.69454
90. Huang G, Xiang Z, Wu H, He Q, Dou R, Yang C, et al. The lncRNA SEMA3B-AS1/HMGB1/FBXW7 Axis Mediates the Peritoneal Metastasis of Gastric Cancer by Regulating BGN Protein Ubiquitination. Oxid Med Cell Longev (2022) 2022:5055684–5055684. doi: 10.1155/2022/5055684
91. Xue L, Chen S, Xue S, Liu P, Liu H. LncRNA TUG1 Compromised Neuronal Mitophagy in Cerebral Ischemia/Reperfusion Injury by Targeting Sirtuin 1. Cell Biol Toxicol (2022). doi: 10.1007/s10565-022-09700-w
92. Chen LL, Yang L. Regulation of circRNA Biogenesis. RNA Biol (2015) 12(4):381–8. doi: 10.1080/15476286.2015.1020271
93. Liu Y, Yang C, Cao C, Li Q, Jin X, Shi H. Hsa_circ_RNA_0011780 Represses the Proliferation and Metastasis of Non-Small Cell Lung Cancer by Decreasing FBXW7 via Targeting miR-544a. Onco Targets Ther (2020) 13:745–55. doi: 10.2147/OTT.S236162
94. Chen X, Li HD, Bu FT, Li XF, Chen Y, Zhu S, et al. Circular RNA Circfbxw4 Suppresses Hepatic Fibrosis via Targeting the miR-18b-3p/FBXW7 Axis. Theranostics (2020) 10(11):4851–70. doi: 10.7150/thno.42423
95. Sun D, Wang G, Xiao C, Xin Y. Hsa_circ_001988 Attenuates GC Progression In Vitro and In Vivo via Sponging miR-197-3p. J Cell Physiol (2021) 236(1):612–24. doi: 10.1002/jcp.29888
96. Zhang Q, Long J, Li N, Ma X, Zheng L. Circ_CLASP2 Regulates High Glucose-Induced Dysfunction of Human Endothelial Cells Through Targeting miR-140-5p/FBXW7 Axis. Front Pharmacol (2021) 12:594793. doi: 10.3389/fphar.2021.594793
97. Zhang J, Chen Z, Liu X, Yang C, Xie D. Gain of circBRAF Represses Glioma Progression by Regulating miR-1290/FBXW7 Axis. Neurochem Res (2021) 46(5):1203–13. doi: 10.1007/s11064-021-03259-4
98. Hou Y, Sun J, Huang J, Yao F, Chen X, Zhu B, et al. Circular RNA circRNA_0000094 Sponges microRNA-223-3p and Up-Regulate F-Box and WD Repeat Domain Containing 7 to Restrain T Cell Acute Lymphoblastic Leukemia Progression. Hum Cell (2021) 34(3):977–89. doi: 10.1007/s13577-021-00504-4
99. Wu Y, Fan T, Zhao Y, Hu R, Yan D, Sun D, et al. Circular RNA Hsa_Circ_0001306 Functions as a Competing Endogenous RNA to Regulate FBXW7 Expression by Sponging miR-527 in Hepatocellular Carcinoma. J Cancer (2021) 12(21):6531–42. doi: 10.7150/jca.61381
100. Liu S, Wang L, Wu X, Wu J, Liu D, Yu H. Overexpression of Hsa_Circ_0022742 Suppressed Hyperglycemia-Induced Endothelial Dysfunction by Targeting the miR-503-5p/FBXW7 Axis. Microvasc Res (2022) 139:104249. doi: 10.1016/j.mvr.2021.104249
101. Xie X, Li H, Gao C, Lai Y, Liang J, Chen Z, et al. Downregulation of Circular RNA Circpsd3 Promotes Metastasis by Modulating FBXW7 Expression in Clear Cell Renal Cell Carcinoma. J Oncol (2022) 2022:5084631. doi: 10.1155/2022/5084631
102. Cao J, Yu U, Li L, Yuan X, Chen S, Xu H, et al. circKL Inhibits the Growth and Metastasis of Kidney Cancer by Sponging Mir−182−5p and Upregulating FBXW7. Oncol Rep (2022) 47(4):75. doi: 10.3892/or.2022.8286
103. Ye F, Gao G, Zou Y, Zheng S, Zhang L, Ou X, et al. Circfbxw7 Inhibits Malignant Progression by Sponging miR-197-3p and Encoding a 185-Aa Protein in Triple-Negative Breast Cancer. Mol Ther Nucleic Acids (2019) 18:88–98. doi: 10.1016/j.omtn.2019.07.023
104. Yang Y, Gao X, Zhang M, Yan S, Sun C, Xiao F, et al. Novel Role of FBXW7 Circular RNA in Repressing Glioma Tumorigenesis. J Natl Cancer Institute (2018) 110(3):304–15. doi: 10.1093/jnci/djx166
105. Welcker M, Larimore EA, Swanger J, Bengoechea-Alonso MT, Grim JE, Ericsson J, et al. Fbw7 Dimerization Determines the Specificity and Robustness of Substrate Degradation. Genes Dev (2013) 27(23):2531–6. doi: 10.1101/gad.229195.113
106. Welcker M, Clurman BE. FBW7 Ubiquitin Ligase: A Tumour Suppressor at the Crossroads of Cell Division, Growth and Differentiation. Nat Rev Cancer (2008) 8(2):83–93. doi: 10.1038/nrc2290
107. Tang X, Orlicky S, Lin Z, Willems A, Neculai D, Ceccarelli D, et al. Suprafacial Orientation of the SCFCdc4 Dimer Accommodates Multiple Geometries for Substrate Ubiquitination. Cell (2007) 129(6):1165–76. doi: 10.1016/j.cell.2007.04.042
108. Welcker M, Clurman BE. Fbw7/hCDC4 Dimerization Regulates its Substrate Interactions. Cell Div (2007) 2:7. doi: 10.1186/1747-1028-2-7
109. Lan H, Tan M, Zhang Q, Yang F, Wang S, Li H, et al. LSD1 Destabilizes FBXW7 and Abrogates FBXW7 Functions Independent of its Demethylase Activity. Proc Natl Acad Sci U.S.A. (2019) 116(25):12311–20. doi: 10.1073/pnas.1902012116
110. Mirmohammadsadegh A, Mota R, Gustrau A, Hassan M, Nambiar S, Marini A, et al. ERK1/2 is Highly Phosphorylated in Melanoma Metastases and Protects Melanoma Cells From Cisplatin-Mediated Apoptosis. J Invest Dermatol (2007) 127(9):2207–15. doi: 10.1038/sj.jid.5700870
111. Jeong EK, Lee SY, Jeon HM, Ju MK, Kim CH, Kang HS. Role of Extracellular Signal-Regulated Kinase (ERK)1/2 in Multicellular Resistance To Docetaxel in MCF-7 Cells. Int J Oncol (2010) 37(3):655–61. doi: 10.3892/ijo_00000714
112. Ji S, Qin Y, Shi S, Liu X, Hu H, Zhou H, et al. ERK Kinase Phosphorylates and Destabilizes the Tumor Suppressor FBW7 in Pancreatic Cancer. Cell Res (2015) 25(5):561–73. doi: 10.1038/cr.2015.30
113. Mun GI, Choi E, Lee Y, Lee YS. Decreased Expression of FBXW7 by ERK1/2 Activation in Drug-Resistant Cancer Cells Confers Transcriptional Activation of MDR1 by Suppression of Ubiquitin Degradation Of HSF1. Cell Death Dis (2020) 11(5):395. doi: 10.1038/s41419-020-2600-3
114. Shu L, Chen A, Li L, Yao L, He Y, Xu J, et al. (2022). NRG1 Regulates Fra-1 Transcription and Metastasis of Triple-Negative Breast Cancer Cells via the C-Myc Ubiquitination as Manipulated by ERK1/2-Mediated Fbxw7 Phosphorylation. Oncogene (2022) 41(6):907–19. doi: 10.1038/s41388-021-02142-4
115. Ye Z, Zhuo Q, Hu Q, Xu X, Mengqi L, Zhang Z, et al. FBW7-NRA41-SCD1 Axis Synchronously Regulates Apoptosis and Ferroptosis in Pancreatic Cancer Cells. Redox Biol (2021) 38:101807–7. doi: 10.1016/j.redox.2020.101807
116. Xiao D, Yue M, Su H, Ren P, Jiang J, Li F, et al. Polo-Like Kinase-1 Regulates Myc Stabilization and Activates a Feedforward Circuit Promoting Tumor Cell Survival. Mol. Cell (2016) 64(3):493–506. doi: 10.1016/j.molcel.2016.09.016
117. Wang D, Pierce A, Veo B, Fosmire S, Danis E, Donson A, et al. A Regulatory Loop of FBXW7-MYC-PLK1 Controls Tumorigenesis of MYC-Driven Medulloblastoma. Cancers (Basel) (2021) 13(3):387. doi: 10.3390/cancers13030387
118. Schülein C, Eilers M, Popov N. PI3K-Dependent Phosphorylation of Fbw7 Modulates Substrate Degradation and Activity. FEBS Lett (2011) 585(14):2151–7. doi: 10.1016/j.febslet.2011.05.036
119. Mo JS, Ann EJ, Yoon JH, Jung J, Choi YH, Kim HY, et al. Serum- and Glucocorticoid-Inducible Kinase 1 (SGK1) Controls Notch1 Signaling by Downregulation of Protein Stability Through Fbw7 Ubiquitin Ligase. J Cell Sci (2011) 124(Pt 1):100–12. doi: 10.1242/jcs.073924
120. Durgan J, Parker PJ. Regulation of the Tumour Suppressor Fbw7α by PKC-Dependent Phosphorylation and Cancer-Associated Mutations. Biochem J (2010) 432(1):77–87. doi: 10.1042/BJ20100799
121. Zitouni S, Méchali F, Papin C, Choquet A, Roche D, Baldin V, et al. The Stability of Fbw7α in M-Phase Requires its Phosphorylation by PKC. PloS One (2017) 12(8):e0183500–e0183500. doi: 10.1371/journal.pone.0183500
122. Kus BM, Caldon CE, Andorn-Broza R, Edwards AM. Functional Interaction of 13 Yeast SCF Complexes With a Set of Yeast E2 Enzymes In Vitro. Proteins: Structure Function Bioinf (2004) 54(3):455–67. doi: 10.1002/prot.10620
123. Zhou P, Howley PM. Ubiquitination and Degradation of the Substrate Recognition Subunits of SCF Ubiquitin-Protein Ligases. Mol Cell (1998) 2(5):571–80. doi: 10.1016/s1097-2765(00)80156-2
124. Galan JM, Peter M. Ubiquitin-Dependent Degradation of Multiple F-Box Proteins by an Autocatalytic Mechanism. P Natl Acad Sci USA (1999) 96(16):9124–9. doi: 10.1073/pnas.96.16.9124
125. Pashkova N, Gakhar L, Winistorfer SC, Yu L, Ramaswamy S, Piper RC. WD40 Repeat Propellers Define a Ubiquitin-Binding Domain That Regulates Turnover of F Box Proteins. Mol Cell (2010) 40(3):433–43. doi: 10.1016/j.molcel.2010.10.018
126. Chen J, Shin J, Zhao R, Phan L, Wang H, Xue Y, et al. CSN6 Drives Carcinogenesis by Positively Regulating Myc Stability. Nat Commun (2014) 5:5384–4. doi: 10.1038/ncomms6384
127. Yan H, Wang H, Zhong M, Wu S, Yang L, Li K, et al. PML Suppresses Influenza Virus Replication by Promoting FBXW7 Expression. Virol Sin (2021) 36(5):1154–64. doi: 10.1007/s12250-021-00399-3
128. Zhang H, Shao Y, Yao Z, Liu L, Zhang H, Yin J, et al. Mechanical Overloading Promotes Chondrocyte Senescence and Osteoarthritis Development Through Downregulating FBXW7. Ann Rheumatol Dis (2022) 81(5):676–86. doi: 10.1136/annrheumdis-2021-221513
129. Liu N, Li H, Li S, Shen M, Xiao N, Chen Y, et al. The Fbw7/human CDC4 Tumor Suppressor Targets Proproliferative Factor KLF5 for Ubiquitination and Degradation Through Multiple Phosphodegron Motifs. J Biol Chem (2010) 285(24):18858–67. doi: 10.1074/jbc.M109.099440
130. Orlicky S, Tang X, Willems A, Tyers M, Sicheri F. Structural Basis for Phosphodependent Substrate Selection and Orientation by the SCFCdc4 Ubiquitin Ligase. Cell (2003) 112(2):243–56. doi: 10.1016/S0092-8674(03)00034-5
131. Nash P, Tang X, Orlicky S, Chen Q, Gertler FB, Mendenhall MD, et al. Multisite Phosphorylation of a CDK Inhibitor Sets a Threshold for the Onset of DNA Replication. Nature (2001) 414(6863):514–21. doi: 10.1038/35107009
132. Deshaies RJ, Joazeiro CA. RING Domain E3 Ubiquitin Ligases. Annu Rev Biochem (2009) 78:399–434. doi: 10.1146/annurev.biochem.78.101807.093809
133. Xu P, Duong DM, Seyfried NT, Cheng D, Xie Y, Robert J, et al. Quantitative Proteomics Reveals the Function of Unconventional Ubiquitin Chains in Proteasomal Degradation. Cell (2009) 137(1):133–45. doi: 10.1016/j.cell.2009.01.041
134. Ravid T, Hochstrasser M. Diversity of Degradation Signals in the Ubiquitin-Proteasome System. Nat Rev Mol Cell Biol (2008) 9(9):679–90. doi: 10.1038/nrm2468
135. Chung CH, Baek SH. Deubiquitinating Enzymes: Their Diversity and Emerging Roles. Biochem Biophys Res Commun (1999) 266(3):633–40. doi: 10.1006/bbrc.1999.1880
136. Popov N, Herold S, Llamazares M, Schülein C, Eilers M. Fbw7 and Usp28 Regulate Myc Protein Stability in Response to DNA Damage. Cell Cycle (2007) 6(19):2327–31. doi: 10.4161/cc.6.19.4804
137. Popov N, Wanzel M, Madiredjo M, Zhang D, Beijersbergen R, Bernards R, et al. The Ubiquitin-Specific Protease USP28 is Required for MYC Stability. Nat Cell Biol (2007) 9(7):765–74. doi: 10.1038/ncb1601
138. Flügel D, Görlach A, Kietzmann T. GSK-3β Regulates Cell Growth, Migration, and Angiogenesis via Fbw7 and USP28-Dependent Degradation of HIF-1α. Blood (2012) 119(5):1292–301. doi: 10.1182/blood-2011-08-375014
139. Diefenbacher ME, Popov N, Blake SM, Schülein-Völk C, Nye E, Spencer-Dene B, et al. The Deubiquitinase USP28 Controls Intestinal Homeostasis and Promotes Colorectal Cancer. J Clin Invest (2014) 124(8):3407–18. doi: 10.1172/JCI73733
140. Schülein-Völk C, Wolf E, Zhu J, Xu W, Taranets L, Hellmann A, et al. Dual Regulation of Fbw7 Function and Oncogenic Transformation by Usp28. Cell Rep (2014) 9(3):1099–109. doi: 10.1016/j.celrep.2014.09.057
141. Zhang J, Ren P, Xu D, Liu X, Liu Z, Zhang C, et al. Human UTP14a Promotes Colorectal Cancer Progression by Forming a Positive Regulation Loop With C-Myc. Cancer Lett (2019) 440-441:106–15. doi: 10.1016/j.canlet.2018.10.010
142. Sun XX, Sears RC, Dai MS. Deubiquitinating C-Myc: USP36 Steps Up in the Nucleolus. Cell Cycle (2015) 14(24):3786–93. doi: 10.1080/15384101.2015.1093713
143. Sun X, He X, Yin L, Komada M, Sears RC, Dai M. The Nucleolar Ubiquitin-Specific Protease USP36 Deubiquitinates and Stabilizes C-Myc. P Natl Acad Sci USA (2015) 112(12):3734–9. doi: 10.1073/pnas.1411713112
144. Khan OM, Carvalho J, Spencer-Dene B, Mitter R, Frith D, Snijders AP, et al. The Deubiquitinase USP9X Regulates FBW7 Stability and Suppresses Colorectal Cancer. J Clin Invest (2018) 128(4):1326–37. doi: 10.1172/JCI97325
145. Li M, Wu Y, Chen J, Shi H, Ji Z, Zhang X, et al. Innate Immune Evasion of Porcine Epidemic Diarrhea Virus Through Degradation of the FBXW7 Protein via the Ubiquitin-Proteasome Pathway. J Virol (2022) 96(5):e0088921. doi: 10.1128/JVI.00889-21
146. Song Y, Lai L, Chong Z, He J, Zhang Y, Xue Y, et al. E3 Ligase FBXW7 is Critical for RIG-I Stabilization During Antiviral Responses. Nat Commun (2017) 8:14654–4. doi: 10.1038/ncomms14654
147. Balamurugan K, Sharan S, Klarmann KD, Zhang Y, Coppola V, Summers GH, et al. Fbxw7α Attenuates Inflammatory Signalling by Downregulating C/Ebpδ and its Target Gene Tlr4. Nat. Commun (2013) 4:1662. doi: 10.1038/ncomms2677
148. Deiuliis JA, Syed R, Duggineni D, Rutsky J, Rengasamy P, Zhang J, et al. Visceral Adipose MicroRNA 223 Is Upregulated in Human and Murine Obesity and Modulates the Inflammatory Phenotype of Macrophages. PLoS One (2016) 11(11):e0165962–e0165962. doi: 10.1371/journal.pone.0165962
149. Mendoza-Villanueva D, Balamurugan K, Ali HR, Kim S, Sharan S, Johnson RC, et al. The C/Ebpδ Protein is Stabilized by Estrogen Receptor α Activity, Inhibits SNAI2 Expression and Associates With Good Prognosis in Breast Cancer. Oncogene (2016) 35(48):6166–76. doi: 10.1038/onc.2016.156
150. Masuda T, Noda M, Kogawa T, Kitagawa D, Hayashi N, Jomori T, et al. Phase I Dose-Escalation Trial to Repurpose Propagermanium, an Oral CCL2 Inhibitor, in Patients With Breast Cancer. Cancer Sci (2020) 111(3):924–31. doi: 10.1111/cas.14306
151. He J, Song Y, Li G, Xiao P, Liu Y, Xue Y, et al. Fbxw7 Increases CCL2/7 in CX3CR1hi Macrophages to Promote Intestinal Inflammation. J Clin Invest (2019) 129(9):3877–93. doi: 10.1172/JCI123374
152. Zhang X, Howell GM, Guo L, Collage RD, Loughran PA, Zuckerbraun BS, et al. CaMKIV-Dependent Preservation of mTOR Expression is Required for Autophagy During Lipopolysaccharide-Induced Inflammation and Acute Kidney Injury. J Immunol (Baltimore Md 1950) (2014) 193(5):2405–15. doi: 10.4049/jimmunol.1302798
153. He J, Du Y, Li G, Xiao P, Sun X, Song W, et al. Myeloid Fbxw7 Prevents Pulmonary Fibrosis by Suppressing TGF-β Production. Front Immunol (2021) 12:760138. doi: 10.3389/fimmu.2021.760138
154. Wang C, Chao Y, Xu W, Liu Z, Wang H, Huang K. Myeloid FBW7 Deficiency Disrupts Redox Homeostasis and Aggravates Dietary-Induced Insulin Resistance. Redox Biol (2020) 37:101688–8. doi: 10.1016/j.redox.2020.101688
155. Close V, Close W, Kugler SJ, Reichenzeller M, Yosifov DY, Bloehdorn J, et al. FBXW7 Mutations Reduce Binding of NOTCH1, Leading to Cleaved NOTCH1 Accumulation and Target Gene Activation in CLL. Blood (2019) 133(8):830–9. doi: 10.1182/blood-2018-09-874529
156. Thompson BJ, Jankovic V, Gao J, Buonamici S, Vest A, Lee JM, et al. Control of Hematopoietic Stem Cell Quiescence by the E3 Ubiquitin Ligase Fbw7. J Exp Med (2008) 205(6):1395–408. doi: 10.1084/jem.20080277
157. Feng C, Li L, Zhou L, Li D, Liu M, Han S, et al. Critical Roles of the E3 Ubiquitin Ligase FBW7 in B-Cell Response and the Pathogenesis of Experimental Autoimmune Arthritis. Immunology (2021) 164(3):617–36. doi: 10.1111/imm.13398
158. Onoyama I, Tsunematsu R, Matsumoto A, Kimura T, de Alborán IM, Nakayama K, et al. Conditional Inactivation of Fbxw7 Impairs Cell-Cycle Exit During T Cell Differentiation and Results in Lymphomatogenesis. J Exp Med (2007) 204(12):2875–88. doi: 10.1084/jem.20062299
159. Kitagawa K, Shibata K, Matsumoto A, Matsumoto M, Ohhata T, Nakayama KI, et al. Fbw7 Targets GATA3 Through Cyclin-Dependent Kinase 2-Dependent Proteolysis and Contributes to Regulation of T-Cell Development. Mol Cell Biol (2014) 34(14):2732–44. doi: 10.1128/MCB.01549-13
160. Suehiro KI, Suto A, Suga K, Furuya H, Iwata A, Iwamoto T, et al. Sox12 Enhances Fbw7-Mediated Ubiquitination and Degradation of GATA3 in Th2 Cells. Cell Mol Immunol (2021) 18(7):1729–38. doi: 10.1038/s41423-020-0384-0
161. Matsuoka S, Oike Y, Onoyama I, Iwama A, Arai F, Takubo K, et al. ). Fbxw7 Acts as a Critical Fail-Safe Against Premature Loss of Hematopoietic Stem Cells and Development of T-ALL. Gene Dev (2008) 22(8):986–91. doi: 10.1101/gad.1621808
162. Zuurbier L, Homminga I, Calvert V, Winkel MT, Buijs-Gladdines JGCA, Kooi C, et al. NOTCH1 and/or FBXW7 Mutations Predict for Initial Good Prednisone Response But Not for Improved Outcome in Pediatric T-Cell Acute Lymphoblastic Leukemia Patients Treated on DCOG or COALL Protocols. Leukemia (2010) 24(12):2014–22. doi: 10.1038/leu.2010.204
163. Kox C, Zimmermann M, Stanulla M, Leible S, Schrappe M, Ludwig W, et al. The Favorable Effect of Activating NOTCH1 Receptor Mutations on Long-Term Outcome in T-ALL Patients Treated on the ALL-BFM 2000 Protocol can be Separated From FBXW7 Loss of Function. Leukemia (2010) 24(12):2005–13. doi: 10.1038/leu.2010.203
164. Malyukova A, Brown S, Papa R, O'Brien R, Giles J, Trahair TN, et al. FBXW7 Regulates Glucocorticoid Response in T-Cell Acute Lymphoblastic Leukaemia by Targeting the Glucocorticoid Receptor for Degradation. Leukemia (2013) 27(5):1053–62. doi: 10.1038/leu.2012.361
165. Zhao E, Maj T, Kryczek I, Li W, Wu K, Zhao L, et al. Cancer Mediates Effector T Cell Dysfunction by Targeting microRNAs and EZH2 via Glycolysis Restriction. Nat Immunol (2016) 17(1):95–103. doi: 10.1038/ni.3313
166. van der Mijn JC, Eng KW, Chandra P, Fernandez E, Ramazanoglu S, Sigaras A, et al. The Genomic Landscape of Metastatic Clear Cell Renal Cell Carcinoma After Systemic Therapy. Mol Oncol (2022). doi: 10.1002/1878-0261.13204
167. Qin JJ, Nag S, Wang W, Zhou J, Zhang WD, Wang H, et al. NFAT as Cancer Target: Mission Possible? Biochim Biophys Acta (2014) 1846(2):297–311. doi: 10.1016/j.bbcan.2014.07.009
168. Xanthoudakis S, Viola JP, Shaw KT, Luo C, Wallace JD, Bozza PT, et al. An Enhanced Immune Response in Mice Lacking the Transcription Factor NFAT1. Science (1996) 272(5263):892–5. doi: 10.1126/science.272.5263.892
169. Liu W, Ren D, Xiong W, Jin X, Zhu L. A Novel FBW7/NFAT1 Axis Regulates Cancer Immunity in Sunitinib-Resistant Renal Cancer by Inducing PD-L1 Expression. J Exp Clin Cancer Res CR (2022) 41(1):38–8. doi: 10.1186/s13046-022-02253-0
170. Xiong W, Zhang B, Yu H, Zhu L, Yi L, Jin X. RRM2 Regulates Sensitivity to Sunitinib and PD-1 Blockade in Renal Cancer by Stabilizing ANXA1 and Activating the AKT Pathway. Adv Sci (Weinh) (2021) 8(18):e2100881. doi: 10.1002/advs.202100881
171. Casey SC, Tong L, Li Y, Do R, Walz S, Fitzgerald KN, et al. MYC Regulates the Antitumor Immune Response Through CD47 and PD-L1. Science (2016) 352(6282):227–31. doi: 10.1126/science.aac9935
172. Zhou S, Zhao X, Yang Z, Yang R, Chen C, Zhao K, et al. Neddylation Inhibition Upregulates PD-L1 Expression and Enhances the Efficacy of Immune Checkpoint Blockade in Glioblastoma. Int J Cancer (2019) 145(3):763–74. doi: 10.1002/ijc.32379
173. Kourtis N, Moubarak RS, Aranda-Orgilles B, Lui K, Aydin IT, Trimarchi T, et al. FBXW7 Modulates Cellular Stress Response and Metastatic Potential Through HSF1 Post-Translational Modification. Nat Cell Biol (2015) 17(3):322–32. doi: 10.1038/ncb3121
174. Yang T, Ren C, Lu C, Qiao P, Han X, Wang L, et al. Phosphorylation of HSF1 by PIM2 Induces PD-L1 Expression and Promotes Tumor Growth in Breast Cancer. Cancer Res (2019) 79(20):5233–44. doi: 10.1158/0008-5472.CAN-19-0063
175. Vanhaesebroeck B, Stephens L, Hawkins P. PI3K Signalling: The Path to Discovery and Understanding. Nat Rev Mol Cell Biol (2012) 13(3):195–203. doi: 10.1038/nrm3290
176. Martin EL, Souza DG, Fagundes CT, Amaral FA, Assenzio B, Puntorieri V, et al. Phosphoinositide-3 Kinase Gamma Activity Contributes to Sepsis and Organ Damage by Altering Neutrophil Recruitment. Am J Respir Crit Care Med (2010) 182(6):762–73. doi: 10.1164/rccm.201001-0088OC
177. Aydin IT, Melamed RD, Adams SJ, Castillo-Martin M, Demir A, Bryk D, et al. FBXW7 Mutations in Melanoma and a New Therapeutic Paradigm. J Natl Cancer Inst (2014) 106(6):dju107. doi: 10.1093/jnci/dju107
178. Gstalder C, Liu D, Miao D, Lutterbach B, Devine AL, Lin C, et al. Inactivation of Fbxw7 Impairs dsRNA Sensing and Confers Resistance to PD-1 Blockade. Cancer Discovery (2020) 10(9):1296–311. doi: 10.1158/2159-8290.CD-19-1416
179. Choi M, Kadara H, Zhang J, Parra ER, Rodriguez-Canales J, Gaffney SG, et al. Mutation Profiles in Early-Stage Lung Squamous Cell Carcinoma With Clinical Follow-Up and Correlation With Markers of Immune Function. Ann Oncol (2017) 28(1):83–9. doi: 10.1093/annonc/mdw437
180. Liu XY, Cui YN, Li J, Zhang Z, Guo RH. Effect of FBXW7 Gene Mutation on the Prognosis of Immunotherapy in Patients With non-Small Cell Lung Cancer. Zhonghua Yi Xue Za Zhi (2022) 102(13):914–21. doi: 10.3760/cma.j.cn112137-20211021-02332
181. Xue S, Gillmore R, Downs A, Tsallios A, Holler A, Gao L, et al. Exploiting T Cell Receptor Genes for Cancer Immunotherapy. Clin Exp Immunol (2005) 139(2):167–72. doi: 10.1111/j.1365-2249.2005.02715.x
182. Yamada T, Sato S, Sotoyama Y, Orba Y, Sawa H, Yamauchi H, et al. RIG-I Triggers a Signaling-Abortive Anti-SARS-CoV-2 Defense in Human Lung Cells. Nat Immunol (2021) 22(7):820–8. doi: 10.1038/s41590-021-00942-0
183. Aygun H. Vitamin D can Reduce Severity in COVID-19 Through Regulation of PD-L1. Naunyn-Schmiedeberg's Arch Pharmacol (2022) 395(4):487–94. doi: 10.1007/s00210-022-02210-w
184. Diao B, Wang C, Tan Y, Chen X, Liu Y, Ning L, et al. Reduction and Functional Exhaustion of T Cells in Patients With Coronavirus Disease 2019 (COVID-19). Front Immunol (2020) 11:827. doi: 10.3389/fimmu.2020.00827
185. Lin PC, Yeh YM, Hsu HP, Chan RH, Lin BW, Chen PC, et al. Comprehensively Exploring the Mutational Landscape and Patterns of Genomic Evolution in Hypermutated Cancers. Cancers (Basel) (2021) 13(17):4317. doi: 10.3390/cancers13174317
186. Cassavaugh JM, Hale SA, Wellman TL, Howe AK, Wong C, Lounsbury KM. Negative Regulation of HIF-1α by an FBW7-Mediated Degradation Pathway During Hypoxia. J Cell Biochem (2011) 112(12):3882–90. doi: 10.1002/jcb.23321
187. O'Neil J, Grim J, Strack P, Rao S, Tibbitts D, Winter C, et al. FBW7 Mutations in Leukemic Cells Mediate NOTCH Pathway Activation and Resistance to Gamma-Secretase Inhibitors. J Exp Med (2007) 204(8):1813–24. doi: 10.1084/jem.20070876
188. Thompson BJ, Buonamici S, Sulis ML, Palomero T, Vilimas T, Basso G, et al. The SCFFBW7 Ubiquitin Ligase Complex as a Tumor Suppressor in T Cell Leukemia. J Exp Med (2007) 204(8):1825–35. doi: 10.1084/jem.20070872
189. Yada M, Hatakeyama S, Kamura T, Nishiyama M, Tsunematsu R, Imaki H, et al. Phosphorylation-Dependent Degradation of C-Myc is Mediated by the F-Box Protein Fbw7. EMBO J (2004) 23(10):2116–25. doi: 10.1038/sj.emboj.7600217
190. Welcker M, Orian A, Jin J, Grim JE, Harper JW, Eisenman RN, et al. The Fbw7 Tumor Suppressor Regulates Glycogen Synthase Kinase 3 Phosphorylation-Dependent C-Myc Protein Degradation. Proc Natl Acad Sci U.S.A. (2004) 101(24):9085–90. doi: 10.1073/pnas.0402770101
191. Welcker M, Singer J, Loeb KR, Grim J, Bloecher A, Gurien-West M, et al. Multisite Phosphorylation by Cdk2 and GSK3 Controls Cyclin E Degradation. Mol Cell (2003) 12(2):381–92. doi: 10.1016/s1097-2765(03)00287-9
192. Liu Z, Ma T, Duan J, Liu X, Liu L. MicroRNA−223−induced Inhibition of the FBXW7 Gene Affects the Proliferation and Apoptosis of Colorectal Cancer Cells via the Notch and Akt/mTOR Pathways. Mol Med Rep (2021) 23(2):154. doi: 10.3892/mmr.2020.11793
193. Zhang P, Shao Y, Quan F, Liu L, Yang J. FBP1 Enhances the Radiosensitivity by Suppressing Glycolysis via the FBXW7/mTOR Axis In Nasopharyngeal Carcinoma Cells. Life Sci (2021) 283:119840. doi: 10.1016/j.lfs.2021.119840
194. Gombodorj N, Yokobori T, Tanaka N, Suzuki S, Kuriyama K, Kumakura Y, et al. Correlation Between High FBXW7 Expression in Pretreatment Biopsy Specimens and Good Response to Chemoradiation Therapy in Patients With Locally Advanced Esophageal Cancer: A Retrospective Study. J Surg Oncol (2018) 118(1):101–8. doi: 10.1002/jso.25127
195. Chen Z, Yu D, Owonikoko TK, Ramalingam SS, Sun SY. Induction of SREBP1 Degradation Coupled With Suppression of SREBP1-Mediated Lipogenesis Impacts the Response of EGFR Mutant NSCLC Cells to Osimertinib. Oncogene (2021) 40(49):6653–65. doi: 10.1038/s41388-021-02057-0
196. Song X, Shen L, Tong J, Kuang C, Zeng S, Schoen RE, et al. Mcl-1 Inhibition Overcomes Intrinsic and Acquired Regorafenib Resistance in Colorectal Cancer. Theranostics (2020) 10(18):8098–110. doi: 10.7150/thno.45363
Keywords: FBXW7, ubiquitination, epigenetic regulation, immunity, immunotherapy
Citation: Xing L, Xu L, Zhang Y, Che Y, Wang M, Shao Y, Qiu D, Yu H, Zhao F and Zhang J (2022) Recent Insight on Regulations of FBXW7 and Its Role in Immunotherapy. Front. Oncol. 12:925041. doi: 10.3389/fonc.2022.925041
Received: 21 April 2022; Accepted: 24 May 2022;
Published: 24 June 2022.
Edited by:
Xuebing Li, Tianjin Medical University General Hospital, ChinaReviewed by:
Lin Lin, Tianjin Medical University General Hospital, ChinaFengjie Guo, South China University of Technology, China
Yue Zhen Deng, Central South University, China
Copyright © 2022 Xing, Xu, Zhang, Che, Wang, Shao, Qiu, Yu, Zhao and Zhang. This is an open-access article distributed under the terms of the Creative Commons Attribution License (CC BY). The use, distribution or reproduction in other forums is permitted, provided the original author(s) and the copyright owner(s) are credited and that the original publication in this journal is cited, in accordance with accepted academic practice. No use, distribution or reproduction is permitted which does not comply with these terms.
*Correspondence: Jian Zhang, 13991802890@163.com; Feng Zhao, xjzhaof@fmmu.edu.cn
†These authors have contributed equally to this work