- 1Emory College, Emory University, Atlanta, GA, United States
- 2Department of Radiation Oncology, Winship Cancer Institute, School of Medicine, Emory University, Atlanta, GA, United States
- 3Nell Hodgson Woodruff School of Nursing, Emory University, Atlanta, GA, United States
The human skin hosts millions of bacteria, fungi, archaea, and viruses. These skin microbes play a crucial role in human immunological and physiological functions, as well as the development of skin diseases, including cancer when the balance between skin commensals and pathogens is interrupted. Due to the linkages between inflammation processes and skin microbes, and viral links to skin cancer, new theories have supported the role a dysbiotic skin microbiome plays in the development of cancer and cancer treatment-related skin toxicities. This review focuses on the skin microbiome and its role in cancer treatment-related skin toxicities, particularly from chemotherapy, radiation therapy, and immunotherapy. The current literature found changes in the diversity and abundance of the skin microbiome during cancer treatments such as radiation therapy, including lower diversity of the skin microbiome, an increased Proteobacteria/Firmicutes ratio, and a higher abundance of pathogenic Staphylococcus aureus. These changes may be associated with the development and severity of treatment-related skin toxicities, such as acute radiation dermatitis, hand-foot syndrome in chemotherapy, and immunotherapy-induced rash. Several clinical guidelines have issued potential interventions (e.g., use of topical corticosteroids, phototherapy, and non-pharmaceutical skin care products) to prevent and treat skin toxicities. The effectiveness of these promising interventions in alleviating treatment-related skin toxicities should be further tested among cancer patients.
Introduction
The human skin microbiome is composed of millions of bacteria, fungi, archaea, and viruses, which function cooperatively to protect against invasive pathogens, acquire immunity, and metabolize natural products (1–3). The skin microbiome plays a crucial role in balancing human immunological and physiological functions; disruption to the balance between skin commensals and pathogens can lead to the development of skin diseases with varying severity (4, 5). Changes in human health conditions (e.g., chronic illness, use of antibiotics, and compromised immune status) can alter the skin microbiome (1, 6). The composition of the skin microbiome is highly dependent on the physiology of the skin site, which is characterized by the microenvironment, distinguished as sebaceous, oily, moist, or dry (1, 2). Dermatological research has provided emerging insights on the composition of skin microbiome based on these sites: sebaceous sites (e.g., glabella, alar crease, back, and face) are dominated by lipophilic Cutibacterium species; moist areas (e.g., umbilicus, gluteal crease, and plantar heel) are dominated by Staphylococcus (S.) and Corynebacterium, and Cutibacterium (C.); Corynebacterium, and various Streptococcus strains have been discovered in dry areas (e.g., buttock, volar forearm, and hypothenar palm) (1, 5).
Recent research has been conducted to understand the relationship of the human gut microbiome (7–9) and vaginal microbiome (10–12) with human health and disease, including its importance as a biomarker of cancer diagnosis, treatment toxicities, and prognosis (1). However, less is known about the relationship of the skin microbiome with disease, such as cancer and cancer treatment-related toxicities. Due to the linkages between processes of inflammation and skin microbes, as well as discoveries on non-oncogenic viral interference linked to reduced cancer risks, new theories have emerged about the role of a dysbiotic skin microbiome in cancer development (13, 14), cancer treatments, and oncologic outcomes (15). Sherwani and colleagues demonstrated the effect of skin cancer on the human skin microbiome, indicating that the skin microbiome of skin cancer patients was less diverse than that of healthy controls (15). Additionally, the microbial taxa thriving within the tumor environment have been found to be tumor-specific, and they direct regulators of cancer initiation, progression, and response to chemotherapy and immunotherapies (15, 16).
Cancer treatment including chemotherapy, radiation therapy [RT], and immunotherapy have significantly improved cancer survival, but treatment-related skin toxicities are common (6). Skin toxicities differ in severity as reported in various treatments (17). Cancer treatment-related skin toxicities can be distressing as skin toxicities can alter one’s appearance and serve as a constant reminder of their ailment. Toxicities affecting quality of life (QOL) can limit patients’ daily functionality, force changes in their therapy schedules, and even lead to treatment termination (17). With RT, radiation dermatitis is a common problem affecting up to 90% of patients with RT (18–20); the severity of radiation dermatitis ranges from mild erythema to moist desquamation and can manifest as dramatic acute skin reactions (18, 21) and/or chronic skin alterations that might have a significant negative impact on patients’ QOL (18, 22, 23). Commonly reported skin toxicities include epidermal growth factor receptor inhibitor (EGFRI) rash, hand-foot skin reaction, hand-foot syndrome or palmar-plantar erythroderma, and chemotherapy-induced alopecia (17, 24).
Few studies have characterized the skin microbiome in cancer patients receiving oncologic therapy. In this mini-review, we reviewed current literature on the skin microbiome and its impact on cancer treatment-related skin toxicities. Specifically, this study reviewed: 1) cancer treatment-related skin toxicities; 2) the skin microbiome and its potential role in treatment-related skin toxicities; and 3) potential interventions for preventing and treating skin toxicities among patients with cancer. We hypothesize a decreasing diversity of the skin microbiome and an increasing pathogenic skin microbes (e.g., S. aureus) with the emergence of cancer and employment of some form of cancer therapy. Additionally, we hypothesize that chronic inflammation plays a promising mechanistic role in the skin microbiome-related treatment toxicities.
Skin Microbiome in Cancer
Advances in microbial research have illuminated understanding of immune and inflammatory pathways in the tumor microenvironment, as well as pathogenesis and cancer progression (25). Given that chronic inflammation is known to create a pro-cancer environment and microbial dysbiosis is associated with mechanisms of inflammation, the abundance of certain microbes is linked to the development of specific cancer types, including skin cancer. Current literature has primarily focused on preclinical models (e.g., piglets and mice). A study comparing the composition and diversity of microbiota in healthy skin vs. melanoma in a pig-model found that Fusobacterium nucleatum promoted proliferation, binding to tumors to prevent immune cell attack and inhibit natural killer cell cytotoxicity (26, 27). More evidence showed that Lactobacillus, Clostridium sensu stricto 1 and Corynebacterium 1 were primarily dominated genera in the healthy skin, while Fusobacterium, Trueperella, Staphylococcus, Streptococcus, and Bacteroides were discriminately abundant in melanoma tissue (28). Healthy skin is primarily characterized with Firmicutes, in which Staphylococcus, Streptococcus, and Lactobacillus are dominant (26). Although the mechanism of bacteria-inducing tumor proliferation is not well understood, there are several contributing processes, such as the production of toxins and inflammation, which leads to DNA damage and induce a pro-inflammatory environment in the skin (25, 29–32).
Limited but promising clinical work similarly investigated the role of skin microbiome in cancer development. Voigt et al. characterized the skin microbiome in squamous cell carcinoma (SCC), its precursor, actinic keratoses as compared to healthy controls (33). Voigt et al. discovered Cutibacterium to be associated with healthy skin, while Staphylococcus was associated with actinic keratoses and SCC. Considering the antagonistic properties of C. acnes and S. aureus, researchers hypothesized that malignant tissue’s loss of its sebaceous properties may prevent growth of C. acnes, promoting a pro-inflammatory environment susceptible to S. aureus colonization (33, 34). Moreover, Corynebacterium genus was found associated with patients with advanced (stage III/IV) melanoma, in which IL-17 promotes the proliferation of melanoma cells through upregulation of IL-6 and signal transducer and activator of transcription 3 (35, 36). Besides skin cancer, recent preliminary work showed a high abundance of some skin Staphylococcus species linked with breast cancer and metastases, in which microbial transfer to underlying tissue is proposed, including retrograde transfer via ductal systems, skin barrier breakdown, and migration through nipple-aspirate fluid (37). As a summary, skin morphology is significantly changed during carcinogenesis and consequently the microbial communities are altered, inhabiting potentially pro-tumorigenic microbes (29). To fully evaluate the skin microbiome, we encourage future researchers to further confirm alterations in microbial profiles across the continuum of cancer care trajectory.
Cancer Treatment-Related Skin Toxicities
Chemotherapy
Chemotherapy-related toxicities frequently occur from systemic damage of bone marrow, hair follicles, mouth, digestive tract, and reproductive system (38, 39). Pyrimidine antagonists and anthracycline chemotherapy agents interfere with synthesis of biological molecules and ultimately block cell division, resulting in a variety of skin toxicities, including hand-foot syndrome (HFS) and chemotherapy-induced alopecia (17, 40, 41). HFS is a well-studied cutaneous adverse reaction of chemotherapy agents, such as capecitabine, 5-fluorouracil, docetaxel, and pegylated liposomal doxorubicin. HFS is not typically life-threatening; however, debilitating discomfort of the palms and soles can significantly affect patient’s QOL as well as impact treatment compliance (40). In extremely severe cases, HFS has shown to lead to tissue necrosis, requiring amputation; immunocompromised patients are also at risk for infection, making patients prone to bacterial sepsis, which could be fatal (17, 41). Chemotherapy-induced alopecia affects about 65% of patients receiving cytotoxic drugs and has a broad range of incidence depending on the therapeutic agent, dosage, administration, and other patient-related factors, such as age, comorbidities, nutritional and hormonal status (42, 43). Alopecia often raises negative attitudes towards body image and self-esteem, as it is seen as a stigmatizing reminder of patients’ disease (17, 44). Some patients even choose to forgo physician’s recommendations because of alopecia, and its impact on patients’ QOL is greatly underestimated by the medical community. Interviews of women being treated for early-stage breast cancer found most of them were greatly troubled by their appearance (17), despite alopecia being mostly reversible after treatment completion with possible complications in color, texture, and complete regrowth (44).
Radiation Therapy
RT is part of definitive treatment for many cancers, but it can cause acute and late toxicities to healthy tissue (45, 46). An inflammatory response occurs in the initial period of RT, caused by pro-inflammatory cytokines (e.g., interleukin [IL]-1, IL-3, IL-5, IL-6, IL-8, tumor necrosis factor [TNF]-α) (47). These factors create a local inflammatory response leading to skin tissue damage and loss of protective barriers (48). Using mice models, Janko et al. found that mice lacking IL-1 or the IL-1 receptor developed less inflammation and suffered lower levels of radiation dermatitis (48). These findings signify the important role of IL-1 in the development of RT-induced skin toxicities, indicating that cytokine pathways (e.g., IL-1) have potential for precisely targeted therapy, especially considering their previous approvals for use in humans to block this cytokine (49, 50).
Acute radiation dermatitis includes symptoms, such as mild erythema and desquamation, ranging from dry desquamation to severe, confluent moist desquamation. When the skin basal cells are destroyed, the balance of normal cell production is disrupted. As the total RT dose accumulates with treatment, the protective skin barrier becomes impaired and dysfunctional (51). The human skin consists of trillions of rapidly proliferating and maturing cells, thus the skin experiences high levels of radiosensitivity and can have dramatic toxicities. While the exact mechanism is unknown, some proposed theories include basal cell proliferation, endothelial cell damage, alterations in membrane permeability, and release of inflammatory cytokines (51–56). A supportive skin care regimen is important in maintaining the integrity of the epidermal barrier, and thus treating irritating symptoms, such as desquamation, xerosis, erythema, pruritus, and hyperpigmentation (51).
Immunotherapy
Immunotherapy targets immune checkpoint pathways through a class of negative key regulators of T cell activation: cytotoxic T-lymphocyte-associated protein-4 (CTLA-4) and programmed cell death protein-1 (PD-1). The primary biological function for these immune checkpoint inhibitors (ICIs) is to induce a pro-inflammatory state in the tumor microenvironment, modulating the cellular immune response to specific tumor antigens and killing tumor cells. However, lack of specificity of immune activation and mediation of ICIs has led to several different skin immune-related adverse events (irAEs). Specifically, patients with advanced melanoma, who were treated with anti-CTLA-4 (ipilimumab) or anti-PD-1 (nivolumab and pembrolizumab), reported immune side effects, such as skin rash, pruritus, and vitiligo early on during ICIs treatment, approximately 2-8 weeks after initiation. IrAEs can be even more common during combined therapies (anti-CTLA-4 and anti-PD-1) (57).
Researchers have defined irAEs as different than damage to single dermatologic units. The most prevalent irCAE is maculopapular rash, which manifests as multiple pruritic erythematous macules and papules on the trunk and extensor surfaces of the extremities. PD-1 inhibitors are known to cause a lichenoid eruption, which is characterized by papules, pruritic hypertrophic plaques, or patches (57).
Skin Microbiome in Skin Toxicities
Most skin diseases or infections are associated with skin microbiome dysbiosis, a term that describes a disruption or imbalance in microbiota homeostasis (1, 4, 5). When the stability of the skin microbiome is threatened, the individual’s skin sites can become populated with pathogenic bacteria, such as S. aureus, presenting significant risks of infection (1, 2, 58). The skin microbiome serves as a modulator between symbiotic commensals that provide a wide variety of skin niches to protect against invasion of pathogenic microorganisms. Chemotherapy-induced damage to skin and hair follicle cells alters the skin’s microbial environment. In particularly, a significant increase in microbial diversity (i.e., Shannon) and decrease of S. aureus proportion were observed with eczema treatment by topical corticosteroid (58). A knowledge gap still exists regarding the biological mechanism of skin microbiome dysbiosis leading to chemotherapy-induced skin toxicities, such as alopecia and hand-foot syndrome. Understanding the skin microbiome and its associations with chemotherapy-related skin toxicities can aid in the development of strategic planning and therapeutic interventions that increase patients’ QOL.
Characterization of the skin microbiome profiles associated with RT-induced dermatitis could help elucidate the mechanisms of pro-inflammatory cytokines and possibly identify targets to decrease RT-induced skin toxicities. However, the relationship between the skin microbiome and immune system is not well-understood. In contrast, atopic dermatitis, or eczema, is a chronic allergic skin disease that has been extensively studied regarding its relationship to the skin microbiome (56, 59). With flareups of atopic dermatitis, healthy skin microbial flora approach a diseased state as defective genes lead to Th2-mediated immunological disruptions in the skin barrier, thereby accelerating susceptibility to infection (56, 60). Physical irritation, such as excoriation of dry and inflamed skin, can further exacerbate microbiome dysbiosis (59). Microbial homeostasis is mediated by Th2 cytokines, which suppress keratinocyte induction of antimicrobial peptides, including human beta-defensin-3 and cathelicidins that prevent colonization of pathogenic organisms (61–63). Increased rates of pathogenic S. aureus and decreased diversity of other microorganisms are consistently reported in atopic dermatitis lesions in comparison to the healthy skin (64–67). As reported by Ramadan and colleagues (68), cancer patients with RT-induced dermatitis had significant reduction in bacterial diversity (Shannon, Chao1, and observed species) comparison to healthy controls. The delayed recovery or tendency toward the permanence of RT-induced dermatitis were associated with a raised Proteobacteria/Firmicutes ratio as well as the dermotype with overrepresentation of Pseudomonas, Staphylococcus, and Stenotrophomonas. With limited evidence, these findings need to be further confirmed in patients with cancer receiving RT.
The diversity and stability of the skin microbiome differs across locations and is of particular interest to understanding regulation of the immune response, as well as the progression of chronic skin disease, such as atopic dermatitis and psoriasis. Research has proposed that a lack of cutaneous microbial diversity and greater density of S. aureus communities are associated with increase inflammation and disease pathogenesis (65–67, 69, 70). Psoriasis is a chronic inflammatory skin disease that appears as raised, scaly, erythematous lesions, known to be triggered by disruptions in the immune system (71, 72). While previous research has shown a decrease in commensal diversity in psoriasis, there have been conflicting reports of the level of abundance in Firmicutes and Actinobacteria, as well as in the major species, including Corynebacterium, Cutibacterium, Staphylococcus, and Streptococcus (73–76). These studies demonstrated how the nature of the skin microbiome stimulates the innate and adaptive immune response. However, the pathways and microbial communities significant to immunotherapy-related skin toxicities are still unknown.
A healthy skin microbiome protects against pathogenic organisms, whereas disruptions in the microenvironment can introduce skin irritations, including acute dermatitis and psoriasis, as well as skin toxicities caused by cancer treatment. Future studies will likely utilize whole genome sequencing to approach the direct mechanism between microbes and the host to evaluate therapeutic targeting potentials of the skin microbiome in irritated skin. By assessing the key patterns in microbial dysbiosis, we can address specific QOL concerns with earlier diagnosis and improved treatment strategies.
Clinical Guidelines and Interventions for Skin Toxicities
Management of cancer treatment-related skin toxicities composes several different facets, including patient education, early prevention, dosage regulation, and symptom assessment and management. Patient’s QOL guidelines have been developed to determine the necessity and scale of treatment suspension, dose modification, and treatment options for skin toxicities. Incorporating the management guidelines related to skin toxicities into clinical practice and testing promising interventions can potentially address the skin toxicities early, reduce patients’ skin symptom burden, and improve patients’ QOL (Figure 1) (17).
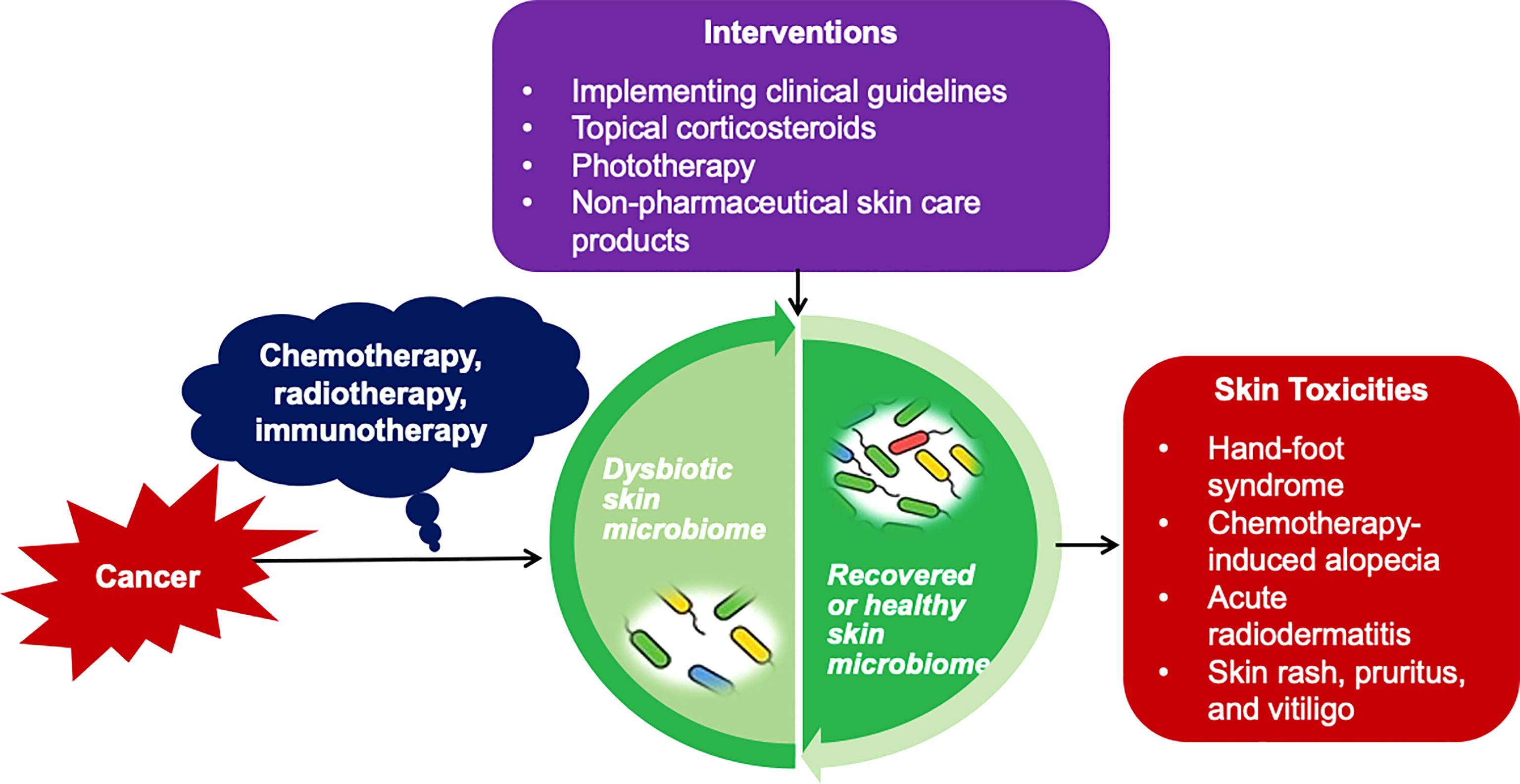
Figure 1 Impact of Skin Microbiome on Cancer Treatment-related Skin Toxicities and Potential Interventions. Based on current knowledge of the bacterial microbiome in cancer treatment-related toxicities, implementing clinical guidelines, topical corticosteroids, phototherapy, and non-pharmaceutical skin care products are suggested approaches for the early prevention and management of cancer treatment-related skin toxicities via adjusting the skin microbiome, and eventually improve patients' quality of life.
Clinical Guidelines
Clinical practice guidelines have been developed to support patients and clinicians in decisions regarding management of treatment-related skin toxicities. The Multinational Association of Supportive Care in Cancer (MASCC) in 2011 published practice guidelines for the prevention and treatment of EGFRI-associated dermatologic toxicities (77). Recently, the Oncology Nursing Society (ONS) Guidelines™ detailed specific recommendations on early prevention and treatment of EGFRI rash, hand-foot skin reaction, HFS, and chemotherapy-induced alopecia (17). For the prevention of EGFRI rash, both ONS and MASCC guidelines recommended topical hydrocortisone 1% cream with moisturizer, twice-daily sunscreen application, and oral antibiotics; to treat the EGFRI rash, both guidelines recommend topical steroids and oral antibiotics (17, 77). Both guidelines further recommended topical minoxidil, a class of drugs called vasodilators, for chemotherapy-induced alopecia (17, 77).
Topical Corticosteroids
For chemotherapy-induced skin reactions, topical steroids in combination with vasoconstrictors are effective in preventing permanent alopecia by inhibiting damage to hair follicle stem cells (58, 61). Acute RT-induced skin reactions are inflammatory reactions that are often treated with topical corticosteroids to vasoconstrict blood vessels, reduce capillary permeability, and inhibit leukocyte proliferation (61). Mometasone furoate is a highly potent corticosteroid that has been shown to significant decrease the pro-inflammatory mediators during breast RT and decrease acute radiation dermatitis (61).
Treatment for irAEs From Immunotherapy
With immunotherapy, treatment options for irAEs are based on dose and grade of skin toxicities. For example, a low-grade maculopapular rash can be managed with mid- to high-potency topical corticosteroids; more severe skin reactions require systemic corticosteroids and can even lead to early ICI termination (44). One study showed that a full recovery from Sweet’s syndrome (i.e., a class of neutrophilic dermatoses) was achieved via oral and intravenous corticosteroids. Continuation of management of high doses of steroids and other immune mediators for irAEs may be hampered as it often counters the therapeutic potency of ICIs. To avoid discontinuation of immunotherapy, other agents to symptomatically treat irAEs are often introduced, including oral retinoids with phototherapy. Other third line therapy options include cyclosporine, methotrexate, and anti-TNF-α. Acitretin, apremilast, and methotrexate are recommended prior to systemic corticosteroids, and biological drugs, including anti-TNF-α, anti-IL-17, and anti-IL-23, can be effective in treating psoriatic lesions and have been used to treat psoriasiform rash from ICIs (51).
Phototherapy
Atopic dermatitis and psoriasis are chronic inflammatory skin diseases that closely intertwine with changes in the skin microbiome (58–62). S. aureus plays an important role in the disease’s development, and its colonization of the skin microbiome is closely related to disease severity (58, 59). Narrowband ultraviolet B radiation has become a common treatment option for patients with differing levels of these adverse skin reactions because it signals the release of antimicrobial peptides, thereby reducing the S. aureus count and ultimately affecting the innate immune system (74, 76). Narrowband ultraviolet B phototherapy paired with corticosteroids could positively affect the skin microbiome by increasing microbial diversity and decreasing the proportion of S. aureus. Utilizing skin swabs and high-throughput sequencing of 16S ribosomal RNA genes could help further our understanding of the skin microbiome and skin toxicities from cancer treatment.
Non-pharmaceutical Skin Care Products
Commercially available, non-pharmaceutical skin care products have been used to reduce radiation dermatitis. Berger et al. reported that patients with breast cancer undergoing RT who were provided with a kit of skin products, including thermal water spray, emollient, cleanser, wound healing cream, and sunscreen, had significantly fewer skin reactions compared to patients who did not receive the kit (51). Proactive skincare is recommended by physicians for patients undergoing RT as these products aid in minimizing skin reactions by maintaining the epidermal barrier and possibly stabilizing microbial homeostasis. With the use of prophylactic skin care, the microenvironment is kept intact and better suited for a healthy skin microbiome, resulting in less severe skin toxicities among patients with cancer (51).
Conclusions
The human skin microbiome has proven to have a profound relationship with the innate and adaptive host immune system in particular skin disorders, such as atopic dermatitis and psoriasis. The skin microbial communities help maintain the skin microenvironment through stimulation and inhibition of the gene expression of host-produced immune factors and pro-inflammatory cytokines. Cancer treatments including chemotherapy, RT, and immunotherapy can cause prominent adverse skin toxicities, such as dermatitis, rash, alopecia, among other irritating skin reactions. Although few studies have characterized the skin microbial profiles associated with skin toxicities, some extrapolation from pro-inflammatory skin disorders like atopic dermatitis and psoriasis can be pursued. Well-developed clinical studies investigating the role of the skin microbiome in cancer treatment-related skin toxicities are needed.
Author Contributions
Conception of the study (BR, JL, ZB, and JB), developing the search strategy (BR and JB), conducting the literature search and summary (BR), drafting the article (BR), editing the article (JL, ZB, and JB), and funding acquisition (JL and JB). All authors contributed to the article and approved the submitted version.
Funding
This work was partially supported by the Emory University Woodruff Health Science Center Synergy Award (PIs: J Bai and J Lin) and National Institutes of Health/National Institute of Nursing Research (1K99NR017897-01 and 4R00NR017897-03, PI: J Bai).
Conflict of Interest
The authors declare that the research was conducted in the absence of any commercial or financial relationships that could be construed as a potential conflict of interest.
Publisher’s Note
All claims expressed in this article are solely those of the authors and do not necessarily represent those of their affiliated organizations, or those of the publisher, the editors and the reviewers. Any product that may be evaluated in this article, or claim that may be made by its manufacturer, is not guaranteed or endorsed by the publisher.
Acknowledgments
The authors acknowledge the support from the Emory University Woodruff Health Science Library in assisting with the literature search.
Abbreviations
Cutibacterium acnes C. acnes, CTLA-4, cytotoxic T-lymphocyte-associated protein-4; EGFRI, epidermal growth factor receptor inhibitor; HFS, hand-foot syndrome; irAEs, immune-related adverse events; IL, Interleukin; ICIs, immune checkpoint inhibitors; PD-1, programmed cell death protein-1; QOL, quality of life; RT, radiation therapy;Staphylococcus aureus, S. aureus;
SCC, squamous cell carcinoma; TNF, tumor necrosis factor.
References
1. Byrd AL, Belkaid Y, Segre JA. The Human Skin Microbiome. Nat Rev Microbiol (2018) 16(3):143–55. doi: 10.1038/nrmicro.2017.157
2. Grice EA, Segre JA. The Human Microbiome: Our Second Genome. Annu Rev Genomics Hum Genet (2012) 13:151–70. doi: 10.1146/annurev-genom-090711-163814
3. Kong HH. Skin Microbiome: Genomics-Based Insights Into the Diversity and Role of Skin Microbes. Trends Mol Med (2011) 17(6):320–8. doi: 10.1016/j.molmed.2011.01.013
4. Findley K, Grice EA. The Skin Microbiome: A Focus on Pathogens and Their Association With Skin Disease. PloS Pathogens (2014) 10(10):e1004436. doi: 10.1371/journal.ppat.1004436
5. Flowers L, Grice EA. The Skin Microbiota: Balancing Risk and Reward. Cell Host Microbe (2020) 28(2):190–200. doi: 10.1016/j.chom.2020.06.017
6. Jo J-H, Harkins CP, Schwardt NH, Portillo JA, Zimmerman MD, Carter CL, et al. Alterations of Human Skin Microbiome and Expansion of Antimicrobial Resistance After Systemic Antibiotics. Sci Transl Med (2021) 13(625):eabd8077. doi: 10.1126/scitranslmed.abd8077
7. Bai J, Barandouzi ZA, Rowcliffe C, Meador R, Tsementzi D, Bruner DW. Gut Microbiome and Its Associations With Acute and Chronic Gastrointestinal Toxicities in Cancer Patients With Pelvic Radiation Therapy: A Systematic Review. Front Oncol (2021) 11:745262. doi: 10.3389/fonc.2021.745262
8. Bai J, Bruner DW, Fedirko V, Beitler JJ, Zhou C, Gu J, et al. Gut Microbiome Associated With the Psychoneurological Symptom Cluster in Patients With Head and Neck Cancers. Cancers (Basel) (2020) 12(9):2531. doi: 10.3390/cancers12092531
9. Sims TT, El Alam MB, Karpinets TV, Dorta-Estremera S, Hegde VL, Nookala S, et al. Gut Microbiome Diversity Is an Independent Predictor of Survival in Cervical Cancer Patients Receiving Chemoradiation. Commun Biol (2021) 4(1):237. doi: 10.1038/s42003-021-01741-x
10. Tsementzi D, Pena-Gonzalez A, Bai J, Hu YJ, Patel P, Shelton J, et al. Comparison of Vaginal Microbiota in Gynecologic Cancer Patients Pre- and Post-Radiation Therapy and Healthy Women. Cancer Med (2020) 9(11):3714–24. doi: 10.1002/cam4.3027
11. Muls A, Andreyev J, Lalondrelle S, Taylor A, Norton C, Hart A. Systematic Review: The Impact of Cancer Treatment on the Gut and Vaginal Microbiome in Women With a Gynecological Malignancy. Int J Gynecological Cancer (2017) 27(7):1550–9. doi: 10.1097/igc.0000000000000999
12. Kindinger LM, MacIntyre DA, Lee YS, Marchesi JR, Smith A, McDonald JA, et al. Relationship Between Vaginal Microbial Dysbiosis, Inflammation, and Pregnancy Outcomes in Cervical Cerclage. Sci Transl Med (2016) 8(350):350ra102. doi: 10.1126/scitranslmed.aag1026
13. Parida S, Sharma D. The Microbiome and Cancer: Creating Friendly Neighborhoods and Removing the Foes Within. Cancer Res (2021) 81(4):790–800. doi: 10.1158/0008-5472.Can-20-2629
14. Yu Y, Champer J, Beynet D, Kim J, Friedman AJ. The Role of the Cutaneous Microbiome in Skin Cancer: Lessons Learned From the Gut. J Drugs Dermatol (2015) 14(5):461–5. doi: 10.1016/j.jaad.2015.02.628
15. Sherwani MA, Tufail S, Muzaffar AF, Yusuf N. The Skin Microbiome and Immune System: Potential Target for Chemoprevention? Photodermatol Photoimmunol Photomed. (2018) 34(1):25–34. doi: 10.1111/phpp.12334
16. Wong-Rolle A, Wei HK, Zhao C, Jin C. Unexpected Guests in the Tumor Microenvironment: Microbiome in Cancer. Protein Cell (2021) 12(5):426–35. doi: 10.1007/s13238-020-00813-8
17. Williams LA, Ginex PK, Ebanks GL Jr., Ganstwig K, Ciccolini K, Kwong BK, et al. ONS Guidelines™ for Cancer Treatment-Related Skin Toxicity. Oncol Nurs Forum (2020) 47(5):539–56. doi: 10.1188/20.Onf.539-556
18. Salvo N, Barnes E, van Draanen J, Stacey E, Mitera G, Breen D, et al. Prophylaxis and Management of Acute Radiation-Induced Skin Reactions: A Systematic Review of the Literature. Curr Oncol (2010) 17(4):94–112. doi: 10.3747/co.v17i4.493
19. McQuestion M. Evidence-Based Skin Care Management in Radiation Therapy: Clinical Update. Semin Oncol Nurs (2011) 27(2):e1–17. doi: 10.1016/j.soncn.2011.02.009
20. Ryan JL. Ionizing Radiation: The Good, the Bad, and the Ugly. J Invest Dermatol (2012) 132(3 Pt 2):985–93. doi: 10.1038/jid.2011.411
21. Hymes SR, Strom EA, Fife C. Radiation Dermatitis: Clinical Presentation, Pathophysiology, and Treatment 2006. J Am Acad Dermatol (2006) 54(1):28–46. doi: 10.1016/j.jaad.2005.08.054
22. Brown KR, Rzucidlo E. Acute and Chronic Radiation Injury. J Vasc Surg (2011) 53(1 Suppl):15s–21s. doi: 10.1016/j.jvs.2010.06.175
23. Salzmann M, Marmé F, Hassel JC. Prophylaxis and Management of Skin Toxicities. Breast Care (Basel). (2019) 14(2):72–7. doi: 10.1159/000497232
24. Wiley K, Ebanks GL Jr., Shelton G, Strelo J, Ciccolini K. Skin Toxicity: Clinical Summary of the ONS Guidelines™ for Cancer Treatment-Related Skin Toxicity. Clin J Oncol Nursing (2020) 24(5):561–5. doi: 10.1188/20.Cjon.561-565
25. Woo YR, Cho SH, Lee JD, Kim HS. The Human Microbiota and Skin Cancer. Int J Mol Sci (2022) 23(3):1813. doi: 10.3390/ijms23031813
26. Mrázek J, Mekadim C, Kučerová P, Švejstil R, Salmonová H, Vlasáková J, et al. Melanoma-Related Changes in Skin Microbiome. Folia Microbiol (2019) 64:435–42. doi: 10.1007/s12223-018-00670-3
27. Gur C, Ibrahim Y, Isaacson B, Yamin R, Abed J, Gamliel M, et al. Binding of the Fap2 Protein of Fusobacterium Nucleatum to Human Inhibitory Receptor TIGIT Protects Tumors From Immune Cell Attack. Immunity. (2015) 42:344–55. doi: 10.1016/j.immuni.2015.01.010
28. Mekadim C, Skalnikova HK, Cizkova J, Cizkova V, Palanova A, Horak V, et al. Dysbiosis of Skin Microbiome and Gut Microbiome in Melanoma Progression. BMC Microbiol (2022) 22(1):63. doi: 10.1186/s12866-022-02458-5
29. Krueger A, Zaugg J, Chisholm S, Linedale R, Lachner N, Teoh SM, et al. Secreted Toxins From Staphylococcus Aureus Strains Isolated From Keratinocyte Skin Cancers Mediate Pro-Tumorigenic Inflammatory Responses in the Skin. Front Microbiol (2022) 12:789042. doi: 10.3389/fmicb.2021.789042
30. Madhusudhan N, Pausan MR, Halwachs B, Durdević M, Windisch M, Kehrmann J, et al. Molecular Profiling of Keratinocyte Skin Tumors Links Staphylococcus Aureus Overabundance and Increased Human β-Defensin-2 Expression to Growth Promotion of Squamous Cell Carcinoma. Cancers. (2020) 12(3):541. doi: 10.3390/cancers12030541
31. Schwabe RF, Jobin C. The Microbiome and Cancer. Nat Rev Cancer. (2013) 13:800–12. doi: 10.1038/nrc3610
32. Bottomley MJ, Thomson J, Harwood C, Leigh I. The Role of the Immune System in Cutaneous Squamous Cell Carcinoma. Int J Mol Sci (2019) 20:2009. doi: 10.3390/ijms20082009
33. Voigt AY, Emiola A, Johnson JS, Fleming ES, Nguyen H, Zhou W, et al. Skin Microbiome Variation With Cancer Progression in Human Cutaneous Squamous Cell Carcinoma. J Invest Dermatol 2022:1–35. doi: 10.1016/j.jid.2022.03.017
34. Kullander J, Forslund O, Dillner J. Staphylococcus Aureus and Squamous Cell Carcinoma of the Skin. Cancer Epidemiol Biomarkers Prev (2009) 18(2):472–8. doi: 10.1158/1055-9965.EPI-08-0905
35. Mizuhashi S, Kajihara I, Sawamura S, Kanemaru H, Makino K, Aoi J, et al. Skin Microbiome in Acral Melanoma: Corynebacterium is Associated With Advanced Melanoma. J Dermatol (2021) 48(1):e15–6. doi: 10.1111/1346-8138.15633
36. Wang L, Yi T, Kortylewski M, Pardoll DM, Zeng D, Yu H. IL-17 can Promote Tumor Growth Through an IL-6-Stat3 Signaling Pathway. J Exp Med (2009) 206(7):1457–64. doi: 10.1084/jem.20090207
37. Wang K, Nakano K, Naderi N, Bajaj-Elliott M, Mosahebi A. Is the Skin Microbiota a Modifiable Risk Factor for Breast Disease? A Systematic Review. Breast. (2021) 59:279–85. doi: 10.1016/j.breast.2021.07.014
38. Toale KM, Johnson TN, Ma MQ. . Chemotherapy-Induced Toxicities. In: Todd KH, Thomas JCR, editors. Oncologic Emergency Medicine: Principles and Practice. Cham, Switzerland: Springer International Publishing (2016). p. 381–406.
39. Peters BG. An Overview of Chemotherapy Toxicities. Topics in Hospital Pharmacy Management. Aspen Syst Corporation. (1994) 14(2):59–88.
40. Kwakman JJM, Elshot YS, Punt CJA, Koopman M. Management of Cytotoxic Chemotherapy-Induced Hand-Foot Syndrome. Oncol Rev (2020) 14(1):442. doi: 10.4081/oncol.2020.442
41. Nikolaou V, Syrigos K, Saif MW. Incidence and Implications of Chemotherapy Related Hand-Foot Syndrome. Expert Opin Drug Saf. (2016) 15(12):1625–33. doi: 10.1080/14740338.2016.1238067
42. Rossi A, Caro G, Fortuna MC, Pigliacelli F, D'Arino A, Carlesimo M. Prevention and Treatment of Chemotherapy-Induced Alopecia. Dermatol Pract Concept. (2020) 10(3):e2020074–e. doi: 10.5826/dpc.1003a74
43. Paus R, Haslam IS, Sharov AA, Botchkarev VA. Pathobiology of Chemotherapy-Induced Hair Loss. Lancet Oncol (2013) 14(2):e50–e9. doi: 10.1016/S1470-2045(12)70553-3
44. Rossi A, Fortuna MC, Caro G, Pranteda G, Garelli V, Pompili U, et al. Chemotherapy-Induced Alopecia Management: Clinical Experience and Practical Advice. J Cosmet Dermatol (2017) 16(4):537–41. doi: 10.1111/jocd.12308
45. Majeed H, Gupta V. Adverse Effects of Radiation Therapy. StatPearls. Treasure Island (FL: StatPearls Publishing, StatPearls Publishing LLC (2022).
46. Berkey FJ. Managing the Adverse Effects of Radiation Therapy. Am Family physician. (2010) 82(4):381–8, 94.
47. Wei J, Meng L, Hou X, Qu C, Wang B, Xin Y, et al. Radiation-Induced Skin Reactions: Mechanism and Treatment. Cancer Manage Res (2019) 11:167–77. doi: 10.2147/cmar.S188655
48. Janko M, Ontiveros F, Fitzgerald TJ, Deng A, DeCicco M, Rock KL. IL-1 Generated Subsequent to Radiation-Induced Tissue Injury Contributes to the Pathogenesis of Radiodermatitis. Radiat Res (2012) 178(3):166–72. doi: 10.1667/rr3097.1
49. Braddock M, Quinn A. Targeting IL-1 in Inflammatory Disease: New Opportunities for Therapeutic Intervention. Nat Rev Drug Discovery (2004) 3(4):330–9. doi: 10.1038/nrd1342
50. Dinarello CA. Blocking IL-1 in Systemic Inflammation. J Exp Med (2005) 201(9):1355–9. doi: 10.1084/jem.20050640
51. Berger A, Regueiro C, Hijal T, Pasquier D, de la Fuente C, Le Tinier F, et al. Interest of Supportive and Barrier Protective Skin Care Products in the Daily Prevention and Treatment of Cutaneous Toxicity During Radiotherapy for Breast Cancer. Breast Cancer (Auckl). (2018) 12:1178223417752772. doi: 10.1177/1178223417752772
52. Gallet P, Phulpin B, Merlin JL, Leroux A, Bravetti P, Mecellem H, et al. Long-Term Alterations of Cytokines and Growth Factors Expression in Irradiated Tissues and Relation With Histological Severity Scoring. PloS One (2011) 6(12):e29399. doi: 10.1371/journal.pone.0029399
53. Hu JJ, Urbanic JJ, Case LD, Takita C, Wright JL, Brown DR, et al. Association Between Inflammatory Biomarker C-Reactive Protein and Radiotherapy-Induced Early Adverse Skin Reactions in a Multiracial/Ethnic Breast Cancer Population. J Clin Oncol (2018) 36(24):2473–82. doi: 10.1200/jco.2017.77.1790
54. Miller RC, Schwartz DJ, Sloan JA, Griffin PC, Deming RL, Anders JC, et al. Mometasone Furoate Effect on Acute Skin Toxicity in Breast Cancer Patients Receiving Radiotherapy: A Phase III Double-Blind, Randomized Trial From the North Central Cancer Treatment Group N06c4. Int J Radiat oncology biology physics. (2011) 79(5):1460–6. doi: 10.1016/j.ijrobp.2010.01.031
55. Rodriguez-Gil JL, Takita C, Wright J, Reis IM, Zhao W, Lally BE, et al. Inflammatory Biomarker C-Reactive Protein and Radiotherapy-Induced Early Adverse Skin Reactions in Patients With Breast Cancer. Cancer epidemiol Biomarkers prevention: Publ Am Assoc Cancer Research cosponsored by Am Soc Prev Oncol (2014) 23(9):1873–83. doi: 10.1158/1055-9965.Epi-14-0263
56. Ellis SR, Nguyen M, Vaughn AR, Notay M, Burney WA, Sandhu S, et al. The Skin and Gut Microbiome and Its Role in Common Dermatologic Conditions. Microorganisms. (2019) 7(11):550. doi: 10.3390/microorganisms7110550
57. Sollena P, Cappilli S, Federico F, Schinzari G, Tortora G, Peris K. Skin Rashes" and Immunotherapy in Melanoma: Distinct Dermatologic Adverse Events and Implications for Therapeutic Management. Hum Vaccin Immunother. (2022) 18(3):e1889449. doi: 10.1080/21645515.2021.1889449
58. Kwon S, Choi JY, Shin JW, Huh CH, Park KC, Du MH, et al. Changes in Lesional and Non-Lesional Skin Microbiome During Treatment of Atopic Dermatitis. Acta Derm Venereol. (2019) 99(3):284–90. doi: 10.2340/00015555-3089
59. Williams MR, Gallo RL. The Role of the Skin Microbiome in Atopic Dermatitis. Curr Allergy Asthma Rep (2015) 15(11):65. doi: 10.1007/s11882-015-0567-4
60. Ong PY, Ohtake T, Brandt C, Strickland I, Boguniewicz M, Ganz T, et al. Endogenous Antimicrobial Peptides and Skin Infections in Atopic Dermatitis. New Engl J Med (2002) 347(15):1151–60. doi: 10.1056/NEJMoa021481
61. Harder J, Bartels J, Christophers E, Schroder JM. Isolation and Characterization of Human Beta -Defensin-3, a Novel Human Inducible Peptide Antibiotic. J Biol Chem (2001) 276(8):5707–13. doi: 10.1074/jbc.M008557200
62. Howell MD. The Role of Human Beta Defensins and Cathelicidins in Atopic Dermatitis. Curr Opin Allergy Clin Immunol (2007) 7(5):413–7. doi: 10.1097/ACI.0b013e3282a64343
63. Grice EA, Kong HH, Conlan S, Deming CB, Davis J, Young AC, et al. Topographical and Temporal Diversity of the Human Skin Microbiome. Science (2009) 324(5931):1190–2. doi: 10.1126/science.1171700
64. Breuer K, HA S, Kapp A, Werfel T. Staphylococcus Aureus: Colonizing Features and Influence of an Antibacterial Treatment in Adults With Atopic Dermatitis. Br J Dermatol (2002) 147(1):55–61. doi: 10.1046/j.1365-2133.2002.04872.x
65. Goh CL, Wong JS, Giam YC. Skin Colonization of Staphylococcus Aureus in Atopic Dermatitis Patients Seen at the National Skin Centre, Singapore. Int J Dermatol (1997) 36(9):653–7. doi: 10.1046/j.1365-4362.1997.00290.x
66. Gong JQ, Lin L, Lin T, Hao F, Zeng FQ, Bi ZG, et al. Skin Colonization by Staphylococcus Aureus in Patients With Eczema and Atopic Dermatitis and Relevant Combined Topical Therapy: A Double-Blind Multicentre Randomized Controlled Trial. Br J Dermatol (2006) 155(4):680–7. doi: 10.1111/j.1365-2133.2006.07410.x
67. Masenga J, Garbe C, Wagner J, Orfanos CE. Staphylococcus Aureus in Atopic Dermatitis and in Nonatopic Dermatitis. Int J Dermatol (1990) 29(8):579–82. doi: 10.1111/j.1365-4362.1990.tb03473.x
68. Ramadan M, Hetta HF, Saleh MM, Ali ME, Ahmed AA, Salah M. Alterations in Skin Microbiome Mediated by Radiotherapy and Their Potential Roles in the Prognosis of Radiotherapy-Induced Dermatitis: A Pilot Study. Sci Rep (2021) 11(1):5179. doi: 10.1038/s41598-021-84529-7
69. Ogawa T, Katsuoka K, Kawano K, Nishiyama S. Comparative Study of Staphylococcal Flora on the Skin Surface of Atopic Dermatitis Patients and Healthy Subjects. J Dermatol (1994) 21(7):453–60. doi: 10.1111/j.1346-8138.1994.tb01774.x
70. Tauber M, Balica S, Hsu CY, Jean-Decoster C, Lauze C, Redoules D, et al. Staphylococcus Aureus Density on Lesional and Nonlesional Skin is Strongly Associated With Disease Severity in Atopic Dermatitis. J Allergy Clin Immunol (2016) 137(4):1272–4.e3. doi: 10.1016/j.jaci.2015.07.052
71. Pariser DM, Bagel J, Gelfand JM, Korman NJ, Ritchlin CT, Strober BE, et al. National Psoriasis Foundation Clinical Consensus on Disease Severity. Arch Dermatol (2007) 143(2):239–42. doi: 10.1001/archderm.143.2.239
72. Xu X, Zhang HY. The Immunogenetics of Psoriasis and Implications for Drug Repositioning. Int J Mol Sci (2017) 18(12):2650. doi: 10.3390/ijms18122650
73. Gao Z, Tseng CH, Strober BE, Pei Z, Blaser MJ. Substantial Alterations of the Cutaneous Bacterial Biota in Psoriatic Lesions. PloS One (2008) 3(7):e2719. doi: 10.1371/journal.pone.0002719
74. Assarsson M, Duvetorp A, Dienus O, Söderman J, Seifert O. Significant Changes in the Skin Microbiome in Patients With Chronic Plaque Psoriasis After Treatment With Narrowband Ultraviolet B. Acta Derm Venereol. (2018) 98(4):428–36. doi: 10.2340/00015555-2859
75. Nangia J, Wang T, Osborne C, Niravath P, Otte K, Papish S, et al. Effect of a Scalp Cooling Device on Alopecia in Women Undergoing Chemotherapy for Breast Cancer: The SCALP Randomized Clinical Trial. Jama. (2017) 317(6):596–605. doi: 10.1001/jama.2016.20939
76. Martin R, Henley JB, Sarrazin P, Seité S. Skin Microbiome in Patients With Psoriasis Before and After Balneotherapy at the Thermal Care Center of La Roche-Posay. J Drugs Dermatol (2015) 14(12):1400–5. doi: 10.1016/j.jaad.2016.02.1062
Keywords: cancer, chemotherapy, immunotherapy, radiation therapy, skin microbiome, adverse event, skin toxicity, 16S rRNA
Citation: Richardson BN, Lin J, Buchwald ZS and Bai J (2022) Skin Microbiome and Treatment-Related Skin Toxicities in Patients With Cancer: A Mini-Review. Front. Oncol. 12:924849. doi: 10.3389/fonc.2022.924849
Received: 20 April 2022; Accepted: 23 June 2022;
Published: 15 July 2022.
Edited by:
Ilaria Grazia Zizzari, Sapienza University of Rome, ItalyReviewed by:
Shigefumi Okamoto, Kanazawa University, JapanAnita Y. Voigt, Jackson Laboratory for Genomic Medicine, United States
Copyright © 2022 Richardson, Lin, Buchwald and Bai. This is an open-access article distributed under the terms of the Creative Commons Attribution License (CC BY). The use, distribution or reproduction in other forums is permitted, provided the original author(s) and the copyright owner(s) are credited and that the original publication in this journal is cited, in accordance with accepted academic practice. No use, distribution or reproduction is permitted which does not comply with these terms.
*Correspondence: Jinbing Bai, amluYmluZy5iYWlAZW1vcnkuZWR1