- 1Key Laboratory of Cancer and Microbiome, State Key Laboratory of Molecular Oncology, National Cancer Center/National Clinical Research Center for Cancer/Cancer Hospital, Chinese Academy of Medical Sciences and Peking Union Medical College, Beijing, China
- 2Beijing Key Laboratory of Cancer Invasion and Metastasis Research, Department of Biochemistry and Molecular Biology, School of Basic Medical Sciences, Capital Medical University, Beijing, China
Hepatobiliary tumors, which include cholangiocarcinoma, hepatocellular carcinoma (HCC), and gallbladder cancer, are common cancers that have high morbidity and mortality rates and poor survival outcomes. In humans, the microbiota is comprised of symbiotic microbial cells (10-100 trillion) that belong to the bacterial ecosystem mainly residing in the gut. The gut microbiota is a complicated group that can largely be found in the intestine and has a dual role in cancer occurrence and progression. Previous research has focused on the crucial functions of the intestinal microflora as the main pathophysiological mechanism in HCC development. Intestinal bacteria produce a broad range of metabolites that exhibit a variety of pro- and anticarcinogenic effects on HCC. Therefore, probiotic alteration of the gut microflora could promote gut flora balance and help prevent the occurrence of HCC. Recent evidence from clinical and translational studies suggests that fecal microbiota transplant is one of the most successful therapies to correct intestinal bacterial imbalance. We review the literature describing the effects and mechanisms of the microbiome in the gut in the context of HCC, including gut bacterial metabolites, probiotics, antibiotics, and the transplantation of fecal microbiota, and discuss the potential influence of the microbiome environment on cholangiocarcinoma and gallbladder cancer. Our findings are expected to reveal therapeutic targets for the prevention of hepatobiliary tumors, and the development of clinical treatment strategies, by emphasizing the function of the gut microbiota.
Introduction
Hepatobiliary tumors are malignant space-occupying lesions that occur in hepatocytes, the gallbladder, and intrahepatic bile ducts. They are associated with a poor survival rate and an aggressive phenotype (1). Primary liver cancer is the second leading cause of cancer-related death worldwide. Approximately 75% of patients with primary liver cancer have hepatocellular carcinoma (HCC) (2), and it was estimated that, in 2030, more than one million patients will die from liver cancer (3). The major risk factors for HCC are alcohol intake, viral hepatitis, and nonalcoholic steatohepatitis (NASH), which occurs in chronic liver disease patients (4, 5). HCC is invasive and has a high level of metastasis; however, as it is asymptomatic in its early stages, it is frequently diagnosed by imaging at an advanced stage (6). Serum α-fetoprotein is commonly used as a screening biomarker for patients with HCC in developing countries (7, 8). However, there is controversy regarding the use of α-fetoprotein due to its poor specificity and sensitivity, limiting its application in clinical practice (9). There is thus an urgent need to investigate and identify combinations of tumor markers that have a high level of prediction.
The human body consists of trillions of microbes that mainly live in the digestive system, and these include bacteria, viruses, fungi, and archaea (10). The ratio of microbial cells to human cells is roughly equal and the total bacterial count in the colon, the organ with the most microorganisms, is approximately 3.8 × 1013 (11). The human gut microbiota has a close relationship with the homeostasis and health maintenance of the host (12, 13). Many probiotics exhibit an antitumor activity through apoptotic signaling pathway activation or immune response enhancement, and they can help improve intestinal health and maintain intestinal flora (14). At the same time, probiotic metabolites may regulate various cellular and molecular processes and the composition of the gut microbiome (15), which plays a critical role in cancer prevention and supports anticancer therapies. Bacterial fermentation of carbohydrates and proteins produces a range of metabolites that have been shown to influence human health and are associated with many diseases either directly or indirectly (16). These metabolites interact with numerous important targets in several metabolic pathways and the molecular processes of malignant tumors.
The largest proportion of bacteria is located in the colon and direct bacterial actions and metabolites promote colorectal cancer initiation and development (17). The hepatic portal vein transports venous blood to the liver, which is subjected continually to bacterial products derived from the gut (18). The gut-liver axis forms a reciprocal interaction between the intestine and the liver that involves the intestinal microbiome and hepatic immune system, which mediates hepatobiliary disorders (19–21). An increasing number of studies have recently shown that the microbiota of the gut, intestinal commensal bacterial species, and their metabolites and products, have a vital function in the pathogenesis of or protection against the development of HCC (22–24). Antibiotic treatments are connected to decreased commensal intestinal bacterial diversity, which, in combination with anticancer therapies, affects the outcome of patients with HCC (25, 26). The promising strategy of fecal microbiota transplantation (FMT) for improving the immune response against cancer has been examined (27), and the enhanced effectiveness of inhibitors of the immune checkpoints with FMT may be a potential strategy to prevent HCC.
Here, we summarize the current progress in our comprehension of the gut microbiota in the treatment of hepatobiliary tumors, including HCC, cholangiocarcinoma (CCA), and gallbladder cancer (GBC). The fundamental mechanisms of the effects of metabolites derived from the gut microbiota on the occurrence of HCC are also discussed. These potential effective therapeutic or preventive strategies, including probiotics, antibiotics, and FMT, may lead to new noninvasive and cost-effective approaches for preventing and treating HCC in the future.
Bacterial Metabolism and HCC Promotion
The gut-liver axis is an anatomical and physiological connection between the liver and gut. The gut microbiota, including metabolites, helps to maintain normal liver functions. Exposure of the liver with chronic lesions to bacterial metabolites results in liver damage and ultimately to the development of HCC (28). Synthesis of cholic acid, primary bile acids (PBAs), and chenodeoxycholic acid takes place via liver cholesterol, and several studies have examined the function of bile acid metabolism disorders in relation to the growth of HCC. Bile acids are categorized as PBAs and secondary bile acids (SBAs). SBAs are derived from PBAs that are converted by gut bacteria and microbial bile acid products and could, thus, be promoters of liver cancer (29). The SBA deoxycholic acid (DCA) is a byproduct of intestinal bacterial metabolism that promotes hepatocarcinogenesis, and the reduction of DCA levels prevents the formation of liver cancer in obese mice (30). The STHD-01 model of steatohepatitis induced by high-fat diet (HFD), which promotes HCC without the administration of chemical carcinogens, shows that the hepatic accumulation of SBAs, such as DCA, is regulated by the gut microbiota and could promote hepatocarcinogenesis by activating the pathway of mammalian target of the rapamycin in hepatocytes (31). The prevalence of nonalcoholic fatty liver disease (NAFLD) is mainly increased by obesity and type 2 diabetes mellitus, and high-fat or high-cholesterol diets are closely linked to NAFLD-associated HCC, which animal experiments and clinical research have confirmed (32, 33). The pathological mechanism associated with gut microbiota dysbiosis involves an increase in the gut bacterial metabolite taurocholic acid and a decrease in 3-indolepropionic acid, as observed in the metabolomic profiling of the serum in high-fat/high-cholesterol diet-fed mice (34). The underlying molecular mechanisms involved in HCC progression include the gut microbial dysbiosis that can lead to enhanced serum taurocholic acid and reduced 3-indolepropionic acid levels that can promote cell propagation and lipid accretion, leading to NAFLD-associated HCC (34). In addition, obesity-associated tumorigenesis develops via NAFLD, continuing to NASH and eventually to HCC (35). The constituent of gram-positive bacterial cell walls, lipoteichoic acid, cooperates with DCA to promote NASH-associated HCC development in the tumor microenvironment via the microbiota-driven cyclo-oxygenase 2 pathway under the gut-liver axis (36). This implies that the abundance of the PBAs and SBAs, which is influenced by changes in the gut microbiota, shows significant associations between risk factors and effective strategies for the prevention of HCC.
The key metabolites produced by gut microbial fermentation of dietary fiber are short-chain fatty acids (SCFAs), which have a vital function in maintaining intestinal homeostasis. An epidemiological study of 125,455 participants from two cohorts in the USA suggested that the increased dietary fiber and whole grains intake was linked to lower HCC development risk (37). Treatment with SCFAs significantly decreased the HCC-positive mouse numbers and prevented the chronic liver disease progression to HCC (McBrearty et al., 2021). The molecular mechanism behind this was that SCFAs upregulated tumor suppressor disabled homolog 2 expression and partially blocked the activity of hepatitis B X antigen (HBx) (38, 39). These results were consistent with those from an in vivo model of HBx transgenic mice in which all the mice developed hepatitis and steatosis, which progressed to dysplastic nodules and noticeable HCC by 1 year (40). Propionate is a health-promoting SCFA that is produced by gut bacterial fermentation of indigestible fiber and is a principal metabolite that maintains intestinal homeostasis (41). Kobayashi et al. found that propionate enhanced the sensitivity of cisplatin and cisplatin-induced apoptosis of HCC cell lines through a GPR41-dependent pathway (42). The above findings demonstrate that SCFAs could be a possible treatment target to delay the development of HCC (Table 1).
A cross-sectional case-control study involving patients with primary liver cancer (n = 671) and 671 controls was conducted to examine the relationship between the levels of metabolites dependent on the gut microbiota, trimethylamine N-oxide and choline, and risk of primary liver cancer. Serum trimethylamine N-oxide level was positively related to primary liver cancer development, particularly in patients with lower levels of choline in the serum (44). Several studies have examined the concentration of ammonia during end-stage liver disease, including NASH and cirrhosis, and hyperammonia is linked with liver disease severity while scavenging ammonia prevents the progression of fibrosis in NAFLD (45, 46). Ammonia is generated by gram-negative anaerobes or gram-positive non-sporing anaerobes in the gut. It can enter the bloodstream and activate hepatic stellate cells (HSCs) and contribute to HCC occurrence and development (47).
Probiotics in HCC
Probiotics are living microbes that confer beneficial effects to the host and help to maintain the stability of an organism as a whole (48). The well-known probiotic strains, e.g., Lactobacillus and Bifidobacterium, are involved in preserving gut microbiota homeostasis. Several beneficial strains for human health including Faecalibacterium prausnitzii, Akkermansia muciniphila, and other SCFA-producing bacteria require further investigation (48–51). Recent studies have revealed that various probiotic strains exert antiproliferative and proapoptotic effects on carcinoma cells and tumor-bearing or tumor-induced animal models (52, 53). The derangement of the gut flora has an important function in a variety of liver diseases, which includes HCC (54). Kumar et al. showed that probiotic fermented milk prepared with probiotic lactic acid bacteria Lactobacillus rhamnosus GG (LGG) and Lactobacillus casei strain Shirota could decrease the risk of HCC by regulating lower genotoxicity or oncogene expression and DNA damage levels (55). Furthermore, Prohep reduced subcutaneous HCC in a tumor model in mice, as there was a 40% reduction in tumor weight and size in comparison with that of the control group (56). Prohep is a novel probiotic blend that comprises three ingredients, LGG, heat-inactivated VSL#3, and viable Escherichia coli Nissle 1917. The possible mechanism of action of Prohep in preventing the development of HCC is the induction and emission of anti-inflammatory interleukin (IL)-10 and suppression of the differentiation of Th17 cells to regulate the gut microbiota and weaken tumor growth and angiogenesis (56). There have been several in vitro investigations of the effects of probiotics against fibrogenesis. Liver fibrosis is caused by an increase in extracellular interstitial protein levels, which leads to the cirrhosis and HCC development. Kanmani et al. reported that the probiotics (Weissella cibaria, Lactobacillus brevis, and Lactiplantibacillus plantarum) might reduce HSC activation and reduce collagen deposition in human LX-2 cell lines by modifying the signaling of transforming growth factor (TGF)-β/SMAD and inducing autophagy and apoptotic pathways (57). This study reported the beneficial role of probiotics in the inhibition of liver fibrogenesis and the prevention of liver cancer. Another study has shown the protective effects of LGG against patulin-induced apoptosis in HepG2 cells by regulating the levels of proapoptotic BCL2 proteins p53 upregulated modulator of apoptosis and BH3-interacting domain death agonist in vitro (58).
Several chemical-induced HCC mouse models have been used to investigate the effects of gut probiotics on healthy individuals. Diethylnitrosamine (DEN) is the most extensively used toxic chemical that induces human liver fibrosis and cirrhosis resulting in HCC development (59). Recent studies have reported that chronic DEN treatment of rats increases the number of cecal E. coli and Atopobium while inducing significant inhibition of the Lactobacillus spp., Bifidobacterium spp., Enterococcus spp., and Bacteroides fragilis groups. Probiotics alleviated hepatocarcinogenesis induced by DEN by re-establishing homeostasis of the gut and improving intestinal and hepatic inflammation. Administration of probiotics could inhibited liver cirrhosis progression to HCC through endogenous damage-associated molecular pattern and exogenous pathogen-associated molecular patterns inflammatory signaling pathways (60). Another study reported excessive E. coli growth in the guts of patients with HCC, which contributed to the hepatocarcinogenesis process (61). A rat model of DEN-induced hepatocarcinogenesis showed that probiotics could potentially be an effective treatment for HCC (62). Indeed, exopolysaccharides are highly viscous microorganism-produced and secreted carbohydrate polymers (63) that have immune, antitumor, and potent antioxidant properties. Lactobacillus acidophilus ATCC 4356 exopolysaccharides exhibit a preventive and therapeutic effect against DEN-induced HCC through the regulation of inflammatory biomarkers IL-17 and TGF-β1 and TLR2/STAT-3/P38-MAPK signaling pathways (62). This suggests that the potential mechanism of the effect of the gut microbiota on HCC includes changes in the intestinal microbiome composition that affects the function of hepatic immune cells. Several studies have shown that the gut microbiota modulates the immune system in patients with HCC by reducing CD8+ T cells (23), stimulating inflammatory response (64), and modulating immunosurveillance (22). Xie et al. explored the dynamic alterations in the microbiota of the gut in HCC development and progression using a mouse model of streptozotocin/HFD-induced NASH-HCC. Their work identified that the abundance of major bacterial species, including Bacteroides spp. and Desulfovibrio spp., were amplified in the group given streptozotocin/HFD, while the abundance of Barnesiella spp., Odoribacter spp., and Paraprevotella spp. was significantly decreased (65). The increased abundance of bacterial species correlated positively with lipopolysaccharide levels and the host pathophysiological features.
Clinical trials examining the connection between gut bacterial microbiota and HCC via the gut-liver axis are underway. Alterations in the populations of live bacteria in the intestines are linked to liver function, implying that changes in the gut microbiome serve as biomarkers for early detection of HCC (Table 2). A recent study reported that the presence of qualitative and quantitative differences between the microbiota of the gut in patients with HCC related to NAFLD when compared with that of the healthy controls. The numbers of Bacteroidales, Bacteroidaceae, Bacteroides, Gemellaceae, and Enterococcaceae identified in the fecal samples from cirrhotic patients with HCC were increased, while there was a decline in the numbers of Bifidobacteriaceae, Verrucomicrobiaceae, Akkermansia, Adlercreutzia, and Dialister when compared to those in the controls (66). In patients with HCC, Bifidobacterium was depleted and its abundance was correlated inversely with the concentration of calprotectin, thereby showing a link with intestinal inflammation. The results suggested that systemic inflammation and the profile of the gut microbiota correlate significantly in NAFLD-related cirrhosis and contribute to HCC development. Interestingly, in a large cohort study from three regions in China with a total of 486 fecal samples from 135 healthy controls, 311 patients with hepatic tumor and 40 patients with cirrhosis were used to clarify how the gut microbiome might be used to noninvasively identify biomarkers of HCC (67). In the study, 419 samples underwent 16S rRNA sequencing with MiSeq to construct an early HCC diagnostic model. The results indicated that the diversity of the gut microbes was increased from cirrhosis to early HCC. There was a decrease in the abundance of bacteria that produce butyrate, such as Oscillibacter, Ruminococcus, Clostridium cluster IV, Faecalibacterium, and Coprococcus, while there was an increase in lipopolysaccharide-producing bacteria, including Haemophilus and Klebsiella, in early HCC when compared with those of the healthy controls (67). In addition, it has recently been demonstrated via high-throughput amplicon sequencing of the 16S rRNA gene that patients with primary HCC exhibited an increased degree of dysbiosis, calculated based on abundance of probiotic and harmful bacterial genera, in comparison to the healthy controls. The patients’ fecal samples showed that the relative abundance of Desulfococcus, Enterobacter, Prevotella, and Veillonella was enhanced at all stages, while the patients with primary HCC (stages I-III) had reduced Cetobacterium (68). In line with this, several studies have noted a correlation between intestinal dysbiosis and chronic liver disease (69, 70) and have proposed a novel integrated index degree for dysbiosis (Ddys) as a tool for evaluating the discrepancies between the microbiota of the gut and the development of HCC.
Antibiotics in HCC
Antibiotic treatments are related to decreased diversity of commensal bacteria, and the early use of antibiotics when treating malignancies is considered to be a disadvantage as it reduces the abundance of Bifidobacteria, Akkermansia, and Ruminococcus in the gut microbiota and increases that of the Bacteroides (71, 72). Clinical studies have also shown that antibiotic treatments are linked with a worse overall survival in patients with advanced HCC who received treatment with first-line sorafenib therapy. Antibiotics can cause changes in gut microbiota that influence reactions in systemic therapy (26). Prospective studies should focus on elucidating the mechanism behind the failure of the multitargeted tyrosine kinase inhibitors for HCC that caused by antibiotics which lead to gut microbiota dysbiosis. Moreover, immune checkpoint inhibitors (ICIs) that target programmed cell death-1 and its ligand are new promising therapies that extend the survival of patients with HCC. The humanized monoclonal antibodies target inhibitory receptors to provoke an antitumor immune response and modulate T-cell activation by interrupting the delivery of inhibitory signals (73). A retrospective cohort study investigated the impacts of antibiotics on the clinical trial results of ICIs for treating patients with HCC (74). Antibiotics against anaerobes were linked with a higher risk of HCC-related death during ICI therapy when compared with antibiotics against aerobes. In a retrospective study, 59 patients with advanced HCC from two academic centers were exposed to antibiotics while receiving ICI nivolumab treatment. Patients who received antibiotics had a shorter median overall survival than those who did not (P = 0.04). This suggests that patients with HCC receiving nivolumab have worse survival rates if they receive antibiotics, and that antibiotics should be used carefully during ICI treatments (75). In some other tumor types, multiple clinical trials have also demonstrated that early exposure to antibiotics was associated with poorer outcomes and reduced clinical benefits following ICI treatment, such as worse overall survival and increased disease refractory risk (76, 77). Future studies should include stool sampling, as well as biopsies and blood sampling, to focus on elucidating the mechanisms of the effect of antibiotics on the gut microbiota of patients with HCC treated with ICIs.
Some studies have assessed the effects of antibiotics in combination with anticancer therapies on the outcomes of patients with HCC. In an international multicenter cohort of 450 ICI recipients, early exposure to antibiotics was not disadvantageous to the therapeutic outcomes of immunotherapy in patients with HCC (78). Antibiotic exposure 30 days before or after ICI initiation during early immunotherapy showed no association with overall survival but was significantly associated with prolonged progression-free survival. The efficacy of ICI therapy, mainly anti-PD-1 monotherapy, in 414 patients from 11 centers, suggests that antibiotics (beta-lactams or quinolones) administered 30 days pre- or post-ICI initiation in HCC are linked with extended progression-free survival (79). It is recommend that patients with HCC should be given broad-spectrum antibiotics before surgery. Furthermore, Wang et al. used a meta-analysis to assess the influence of prophylactic antibiotic treatments on infections and complications in transarterially treated patients with HCC (80). They showed that antibiotic prophylaxis may not be routinely necessary for postoperative infectious complications in patients with HCC who have been treated with transarterial therapy. However, in patients with HCC who have undergone biliary tract disease or biliary reconstruction surgery and enterobiliary anastomosis, the prophylactic use of antibiotics could reduce the risk of infection. In a spontaneous HCC model using MYC transgenic mice, a cocktail treatment with three antibiotics was invented to remove gram-positive bacteria to provoke hepatic natural killer (NK) T cell accretion and prevent liver tumor growth (81). They also found that vancomycin or cefoperazone alone was sufficient to increase hepatic NKT cells, while the effect of neomycin was weakened. Abdel-Hamid et al. examined the anticancer activity of some macrolide antibiotics including clarithromycin, azithromycin, and erythromycin on HCC development both in vitro and in vivo (82). Treatment with clarithromycin inhibited the proliferation of HepG2 cells with an IC50 of 3.13 μg/mL and induced the apoptosis pathway to suppress HCC in a DEN-induced rat model. The basic experimental research should include the mechanisms of antibiotic-mediated intestinal dysbiosis and anticancer immune response drivers.
FMT in HCC
FMT involves transferring healthy donor stool to the gut of a recipient with a physical illness (83). FMT is a safe and effective alternative to treat a recurrent Clostridium difficile infection (84). Multiple randomized clinical trials have confirmed that FMT had a success rate of nearly 92% when used as a second-line treatment of recurrent Clostridium difficile infection (85, 86). Preliminary studies have proposed that FMT is a potential treatment for a variety of other conditions, which include obesity, functional gastrointestinal disorders, inflammatory bowel disease, and metabolic syndromes (87). According to several randomized clinical trials, healthy donor FMT could possibly heal liver disease (88). FMT administered via an oral capsule was safe in patients with recurrent hepatic encephalopathy and cirrhosis (NCT03152188, Phase 1). There was increased abundance of Ruminococcaceae and Bifidobacteriaceae while that of Streptococcaceae and Veillonellaceae was decreased in the post-FMT microbiota, which is associated with improved duodenal mucosal diversity and hepatic encephalopathy (89). A characteristic of NAFLD is excess accumulation of fat in the hepatocytes and it is estimated to be present in 10-40% of adults worldwide (90). NAFLD is a risk factor of cirrhotic and HCC progression (91, 92). Craven et al. reported that patients with NAFLD demonstrating elevated permeability of the small intestinal at baseline reduced significantly 6 weeks after allogenic FMT (NCT02496390, Phase 1 and 2). However, there were no significant changes hepatic proton density fat fraction (allogeneic or autologous) or resistance to insulin (93). In most cases, the cause of HCC is the underlying liver damage due to chronic hepatitis, and in some developed countries, the mortality rate of HCC associated with cirrhosis is increasing (94). Previous researchers have evaluated the alterations in the gut microbiota using metagenomic analysis and found a decreased expression of the antibiotic resistance gene in cirrhosis patients who underwent FMT via capsule or enema. These genes could be potential targets with healthy donor FMT (NCT03152188, Phase 1) (95). Moreover, many clinical trials have investigated the preliminary benefits of FMT in various liver diseases, such as: the effect of consecutive FMT on NAFLD with 20 participants through a randomized-controlled trial (NCT04465032, Phase 4); FMT using stool from five lean donors to determine the efficacy and safety for patients with NASH through single group assignments of interventional clinical trial (NCT02469272, Phase 1); and FMT versus standard therapy in NASH related cirrhosis with 112 participants through a randomized control trial (NCT02721264) (Table 3).
Data from animal studies have suggested that FMT alleviates steatohepatitis induced by HFD and has a beneficial outcome because of its alterations to the composition of the gut microbiota (96). In male C57BL/6 mice, FMT reverses gut microbiota dysbiosis and regulates SCFA levels by increasing the butyrate concentration in the cecum to correct the ratio of T-cell subsets that are beneficial to immunological balance. Bacteroidetes abundance was decreased whereas that of Firmicutes was increased after FMT when related with the HFD group (96). The direct effects of FMT on HCC growth and development have not been studied yet. The possible mechanism is that FMT regulates intestinal flora disorders and reduces cytotoxin production to inhibit the incidence of HCC in patients with hepatitis B cirrhosis (97). In addition, FMT can modulate the gut microbiome to abrogate ICI-associated colitis and toxicity (98), which implying the other mechanisms such as immune microenvironment could be involved in the effect of FMT on HCC. Thus, further investigations are needed to prove the safety of FMT in patients with HCC.
Microbiome and CCA
After HCC, CCA is the most common malignant tumor and epithelial cell malignancy that accounts for almost 15% of all primary liver cancers. More than two-thirds of patients with CCA are diagnosed at an advanced disease stage with poor prognosis, as there is an absence of obvious symptoms to facilitate earlier diagnosis (99). Based on the anatomical sites of origin, CCA is categorized into three subtypes: intrahepatic, perihilar, and distal (100). Microbiota dysbiosis is closely linked with CCA progression from precancerous diseases (101). Gut microbiota can be used as a potential biomarker for CCA. In a case-control study, Zhang et al. performed high-throughput sequencing of the 16S rRNA from the fecal samples from 53 patients with CCA, 47 patients with cholelithiasis, and 40 healthy controls, and proposed a predictive model based on four enriched genera (Bacteroides, Muribaculaceae_unclassified, Muribaculum, and Alistipes) for the better diagnosis of CCA (102). Similarly, a classification model consisting of eight genera was constructed to classify CCA, HCC, and healthy controls (103).
In healthy people, the gut microbiota is ecologically balanced and meanwhile the link between gut microbiota and the pathogenesis of CCA is attracting more attention (104, 105). Biliary obstruction caused by CCA leads to cholestasis, which provides a culture medium for bacteria. Decreased bile secretion, low immunity of patients with CCA, and surgical trauma can promote overgrowth of the gut microbiota, which can result in the overgrowth of small intestinal bacteria, increased abundance of pathogenic bacteria, and change in microbiota abundance (105, 106). The overgrowth of gut microbiota can damage the intestinal mucosal barrier, which can lead to increased permeability of the intestinal mucosa; thus, a large amount of endotoxin and pathogenic bacteria permeate into the blood, resulting in intestinal endotoxemia and movement of the microbiota (107). This process leads to the inflammatory mediator release such as IL-1, TNF-α, and IL-6 which induces a series of inflammatory immune responses (108, 109). Moreover, long-term low-grade chronic inflammation is related to cancer incidence and development. At the same time, the gut microbiota can enter the biliary tract through the blood, lymph, or in a retrograde manner leading to a biliary infection (110).
Bile duct stones are a risk factor for intrahepatic CCA development, as a systematic review reported that there was a strong correlation between hepatolithiasis and intrahepatic CCA (111). Liver flukes are linked to bile duct infection and proinflammatory cytokine production which was consistent with the inflammatory phenotype in CCA development (112–114). Chng et al. reported that the Stenotrophomonas species abundance was significantly increased in tumor tissues of non-fluke-related CCA (115). The pathogenesis of CCA may involve the microbial community, as reported by Saab et al., who found that Streptococcus, Enterococcus, Klebsiella, Bacteroides, and Pyramidobacter were the most abundant in the bile of patients with extrahepatic CCA. The bile of patients with CCA had lower Firmicutes abundance and higher Bacteroidetes abundance than those of benign biliary pathology (116). The microbiota of the bile from patients with distal CCA was highly enriched with Proteobacteria, Firmicutes, Bacteroidetes, and Actinobacteria. Moreover, the abundance of phyla Nitrospirae, Gemmatimonadetes, Latescibacteria, Chloroflexi, and Planctomycetes were significantly increased in patients with distal CCA when compared with patients with cholelithiasis (117). However, when compared with HCC, liver cirrhosis, and healthy controls, Jia et al. reported that patients with intrahepatic CCA demonstrated the highest levels of species richness, with four highly enriched genera in the gut microbiota, Lactobacillus, Actinomyces, Peptostreptococcaceae, and Alloscardovia (109). The Ruminococcaceae family is also enriched in patients with intrahepatic CCA who have vascular invasion. Patients with intrahepatic CCA have high plasma-stool ratios of tauroursodeoxycholic acid and glycoursodeoxycholic acid, which can be used as biomarkers to distinguish intrahepatic CCA from HCC (109). These studies indicate the microbiome diversity in patients with CCA and the heterogeneity among individuals.
A potential risk in CCA development is choledocholithiasis (CDL), which is a consequence of bacterial infection. Dangtakot et al. performed a sequencing study of the 16S rRNA gene, including samples of bile from 30 patients with CDL and 30 with CCA. Results showed higher abundance of Enterobacter, Pseudomonas, and Stenotrophomonas species in CCA when related with CDL (P < 0.05), while the Pyramidobacter, Cetobacterium, and Streptococcus species were less abundant in CCA when compared with CDL (P < 0.05) (118). The chronic liver disease, primary sclerosing cholangitis is accompanied by inflammation and scarring and is an important risk factor in CCA (119). It was recently reported that the gut microbiome directs hepatocytes through myeloid cell accumulation to control CCA; and when treated with neomycin to eliminate gram-negative gut bacteria, CCA inhibition could be achieved in the bile duct ligation-modeled mice (120). The bacteria that commonly exist in the environment although not in humans, such as Nesterenkonia, Methylophilaceae, and Mesorhizobium, were found in the microbiota of patients with extrahepatic CCA and benign biliary pathology. In a multicenter case-control study, the abundance of Actinomyces, Novosphingobium, Prevotella, and Fusobacterium were significantly increased in extrahepatic CCA, while that of Nesterenkonia and Rothia were reduced (121). Serra et al. found in bile samples that Pseudomonas was a significant positive prognosticator for CCA (122), and Lee et al. reported that Pseudomonas was a significant negative biomarker for CCA using plasma samples (123). These contrasting results might be due to the differences in sample types and study objects. When compared with bile samples, the blood microbiome of patients with CCA requires further investigation to explore its conformation and mechanisms.
The functional mechanisms of the microbiota of the gut in CCA have been inadequately studied. The gut microbiome directs hepatocytes and promotes CXCL1 expression in a TLR4-dependent manner to recruit myeloid-derived suppressor cells and promote CCA (120). Using large-scale proteogenomics, Dong et al. yielded insights into intrahepatic CCA etiology and taxonomy. They identified four intrahepatic CCA subgroups that were associated with different molecular and clinical features and selected HKDC1 and SLC16A3 as prognostic biomarkers (124).
Microbiome and GBC
GBC is a common malignant tumor with poor prognosis and almost all types are adenocarcinomas that are usually diagnosed at an advanced stage (125). GBC is recognized as a highly lethal disease due to a lack of predictive radiological features and accurate identification, which leads to only 10% of patients presenting at a stage suitable for surgical resection (126). Multiple investigations have demonstrated that bacterial infections are related to higher GBC risk. Choi et al. reported the bacterial species present in the bile microbiome, through metagenomic sequencing from patients with normal gallbladder, chronic cholecystitis, and GBC. They showed significantly increased Escherichia, Enterobacter, and Klebsiella in GBC samples when compared with that in normal bile samples. Klebsiella abundance between patients with chronic cholecystitis and GBC was significantly different (127), which indicates a link between microbiological disorders and GB carcinogenesis. Another study clarified the microbial communities between patients with GBC and cholelithiasis using next-generation-based 16S rRNA sequencing. Enterobacter sp. and Fusobacterium nucleatum were predominant and identified in the GBC samples, while Salmonella sp. and Enterococcus gallinarum were prevalent in the bile of cholelithiasis patients (128). A chronic Salmonella carrier state is related to GB carcinogenesis, especially in areas with high typhoid endemicity (129). This is consistent with the finding that Salmonella typhi in the gallbladder of gallstone patients may be a cause for the development of GBC (130). A recent study investigated if Salmonella infection is a risk factor for GBC in genetically susceptible mice through the regulation of AKT and MAPK pathways during infection (131). The results indicated that Salmonella enterica can promote carcinogenesis and is a causative agent of GBC. Thus, eradicating bacterial infection via immunotherapy, anti-inflammatory therapy, and hygienic practices might help in reducing the incidence of GBC.
Discussion
There is growing proof for the profound impact of gut homeostasis on HCC development, and consequently, gut microflora may embody a novel target for prevention of primary HCC. Due to its anatomical position, the liver is subjected to enteric bacterial components, meaning intestinal bacterial products could spread to the liver through the portal vein (132). Many have examined the alterations that occur in the microbiomes of patients with CCA and GBC, and it was found that dysbiosis of the microbiome could promote tumorigenesis and GBC and CCA development (101, 128). In this review, we have summarized the data regarding the bacteria that are altered in the microbiome, such as Enterobacter and Klebsiella and the gram-negative bacteria that co-occur in cirrhotic patients with HCC using samples from patients with GBC (Figure 1). There was a significant increase in Enterobacter in GBC samples when compared with the normal bile samples, and its abundance was enhanced in the fecal samples of patients with HCC at all disease stages (68, 127). It has been reported that Enterobacter is related to a proinflammatory response (133). The above phenotype was consistent with an investigation, which found that inflammation is central to GBC development and that reducing inflammation-related pathways was associated with increased survival during GBC outcomes (134). Inflammation is a critical component of HCC progression and anti-inflammatory drugs are reported to enhance the prognosis of patients with HCC (135). There was increased Bacteroides, Prevotella, Klebsiella, and Enterobacter in the stool samples from cirrhotic patients with HCC and the biliary microbiota of patients with CCA as compared with the control groups (66, 116). Gram-negative Bacteroides are correlated with chronic intestinal inflammation and tissue injury, which are colorectal cancer risk factors (136). Chronic and unresolved inflammation is regarded as the main carcinogenic mechanism promoting GBC development (137), as it provides a possible underlying mechanism, in which Bacteroides have essential roles in HCC and GBC incidence through gut-derived inflammation. In this context, human bacteria, such as Enterobacter and Bacteroides, coexist with their host and facilitate multiple types of carcinogenesis. Other studies have also reported that augmented Actinomyces abundance in the bile ducts of patients with extrahepatic CCA may have originated from the intestine (109).
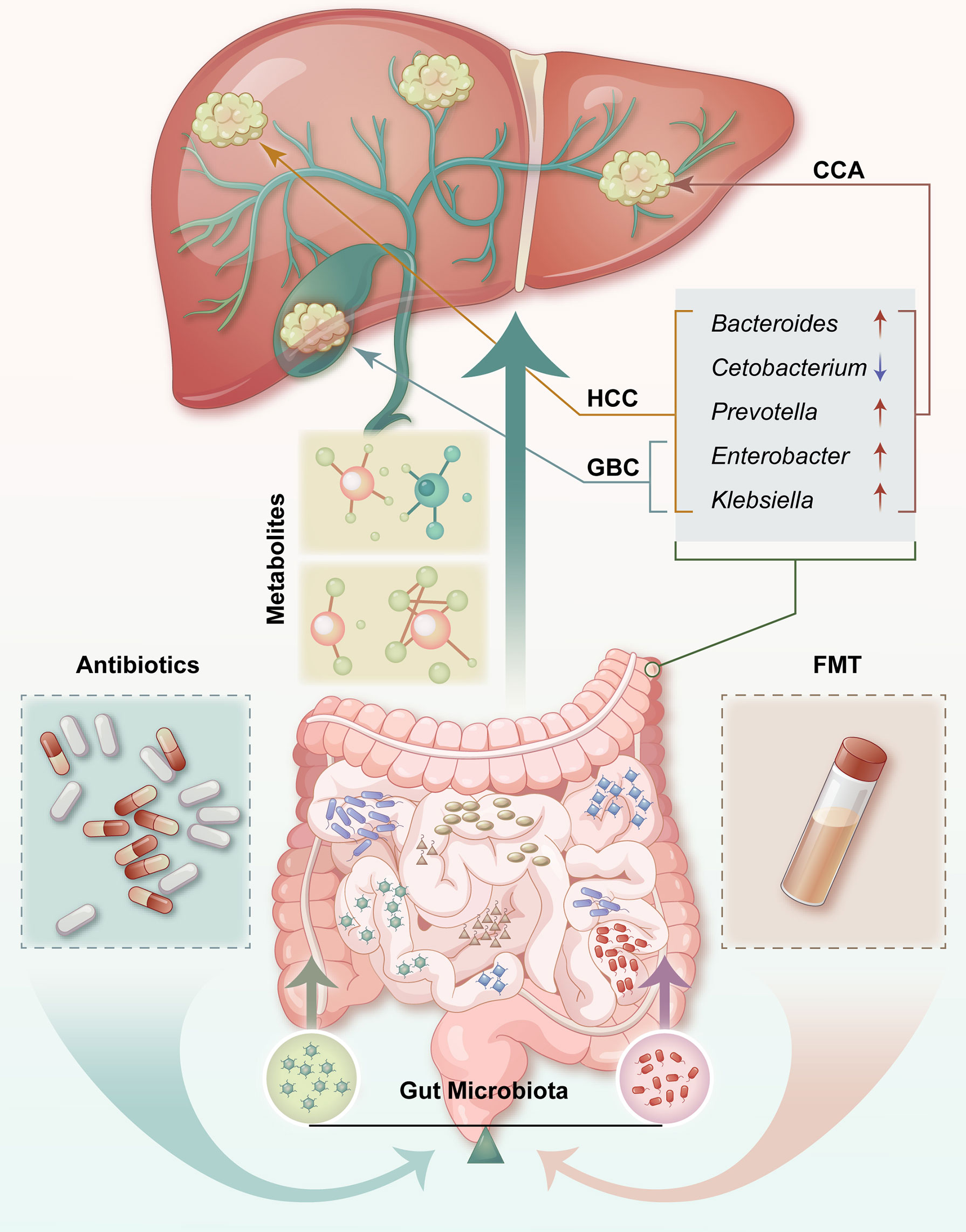
Figure 1 Contributions of the microbiota of the gut to hepatobiliary tumors. There is an anatomical and physiological connection between the liver and the intestinal tract. Potential effective treatments or preventive strategies for HCC, including probiotics, antibiotics, and FMT, may be cost-effective. HCC, hepatocellular carcinoma; CCA, cholangiocarcinoma; GBC, gallbladder cancer; FMT, fecal microbiota transplant; ↑ = upregulated; ↓ = downregulated.
Probiotics are used to modulate the intestinal microflora balance and confer health benefits to the host. LGG is a widely used probiotic strain that has been evaluated for the treatment of diarrhea (138), antiproliferative effects on the cells of colon adenocarcinoma (139), suppressive effects on inflammation during Helicobacter infection in gastric adenocarcinoma cells (140), and high-fructose diet-induced NAFLD (141). In the present review, we found that probiotic LGG can decrease the risk of HCC through multiple pathways, including DNA damage (55), inflammatory and cell differentiation (56), and human hepatoma cell apoptotic regulation (58). Probiotics interact with the host and the microbiome via molecular effectors as metabolic products through the gastrointestinal microenvironment and mediate health benefits (142). Intestinal dysbiosis influences HCC development partly via microbial and gut metabolites and products conveyed through the portal vein to the liver, which could be a key regulator of tumorigenicity and HCC aggressiveness (143). This was consistent with a previous study that explored the molecular mechanisms of gut dysbiosis on gut barrier and inflammation in relation to carcinogenesis, such as immune responses, toxic metabolites, and signaling pathway intervention (144). The metabolic byproduct of intestinal bacteria, DCA, could contribute to HCC via multiple signaling pathways such as the activation of mammalian targets of rapamycin signaling or the gut microbiota-driven cyclo-oxygenase 2 pathway (31, 36). It was recently reported that DCA induced human HSC malignant behavior of HCC by inducing senescence-associated secretory phenotype factors, e.g., TGF-β and IL-8 (145). The above investigations suggest that multiple actions of gut metabolites could control cancer growth and progression in different HCC models.
In conclusion, we have summarized the role of microbiota of the gut in hepatobiliary tumor incidence and development, including HCC, CCA, and GBC. The potential effectiveness of the treatments and representative novel strategies, including probiotics, antibiotics, and FMT, focus on fecal microbiome modulation, which provides alternative therapeutic methods for HCC. There are still many questions regarding microbiota function in the occurrence of HCC that remain unclear. There are limitations to the gut microbiome as a biomarker or therapeutic target for hepatobiliary tumors, since the gut microbiome is easily disturbed by several confounding factors, such as diet, medication (especially probiotics and antibiotics), mental health, and environmental factors, which make this type of biomarker not robust or reliable. In addition, interruption of the balance of the gut microbiome could lead to a series of problems, thus a global view should be taken before using the gut microbiome as a therapeutic target. The gut microbiome and hepatobiliary tumors are not directly linked spatially, which means that the gut microbiome does not reflect hepatobiliary tumors straightforwardly. The gut microbiome is involved in hepatobiliary tumor development through complex mechanisms, and there is an urgent need for more laboratory investigations and extensive human clinical trials to assess the gut microbiota abundance and screen for beneficial strains.
Author Contributions
YW designed the content of the review with input from all the co-authors. HQ and BY drafted the manuscript. YW and WH revised the manuscript. All authors contributed to the article and approved the submitted version.
Funding
This study was supported by grants from The National Natural Science Foundation of China (42125707, 41931291, 81902960), the Non-profit Central Research Institute Fund of Chinese Academy of Medical Sciences (2019PT310027), the Chinese Academy of Medical Sciences Innovation Fund for Medical Sciences (2019-I2M-1-003, 2021-1-I2M-018, 2021-RC310-018).
Conflict of Interest
The authors declare that the research was conducted in the absence of any commercial or financial relationships that could be construed as a potential conflict of interest.
Publisher’s Note
All claims expressed in this article are solely those of the authors and do not necessarily represent those of their affiliated organizations, or those of the publisher, the editors and the reviewers. Any product that may be evaluated in this article, or claim that may be made by its manufacturer, is not guaranteed or endorsed by the publisher.
Abbreviations
HCC, hepatocellular carcinoma; FMT, fecal microbiota transplantation; CCA, cholangiocarcinoma; GBC, gallbladder cancer; PBA, primary bile acids; SBA, secondary bile acid; DCA, deoxycholic acid; NAFLD, nonalcoholic fatty liver disease; NASH, nonalcoholic steatohepatitis; SCFA, short-chain fatty acid; HSC, hepatic stellate cell; LGG, Lactobacillus rhamnosus GG; TGF, forming growth factor; DEN, diethylnitrosamine; ICI, immune checkpoint inhibitors; CDL, choledocholithiasis.
References
1. Siegel RL, Miller KD, Fuchs HE, Jemal A. Cancer Statistic. CA Cancer J Clin (2022) 72:7–33. doi: 10.3322/caac.21708
2. Petrick JL, Florio AA, Znaor A, Ruggieri D, Laversanne M, Alvarez CS, et al. International Trends in Hepatocellular Carcinoma Incidenc –2012. Int J Cancer (2020) 147:317–30. doi: 10.1002/ijc.32723
3. Villanueva A. Hepatocellular Carcinoma. N Engl J Med (2019) 380:1450–62. doi: 10.1056/NEJMra1713263
4. Van Meer S, De Man RA, Siersema PD, Van Erpecum KJ. Surveillance for Hepatocellular Carcinoma in Chronic Liver Disease: Evidence and Controversies. World J Gastroenterol (2013) 19:6744–56. doi: 10.3748/wjg.v19.i40.6744
5. Llovet JM, Zucman-Rossi J, Pikarsky E, Sangro B, Schwartz M, Sherman M, et al. Hepatocellular Carcinoma. Nat Rev Dis Primers (2016) 2:16018. doi: 10.1038/nrdp.2016.18
6. Ayuso C, Rimola J, Vilana R, Burrel M, Darnell A, García-Criado Á, et al. Diagnosis and Staging of Hepatocellular Carcinoma (HCC): Current Guidelines. Eur J Radiol (2018) 101:72–81. doi: 10.1016/j.ejrad.2018.01.025
7. Kaur H, Bhalla S, Raghava GPS. Classification of Early and Late Stage Liver Hepatocellular Carcinoma Patients From Their Genomics and Epigenomics Profiles. PloS One (2019) 14:e0221476. doi: 10.1371/journal.pone.0221476
8. Jasirwan COM, Fahira A, Siregar L, Loho I. The Alpha-Fetoprotein Serum is Still Reliable as a Biomarker for the Surveillance of Hepatocellular Carcinoma in Indonesia. BMC Gastroenterol (2020) 20:215. doi: 10.1186/s12876-020-01365-1
9. Jang ES, Jeong SH, Kim JW, Choi YS, Leissner P, Brechot C. Diagnostic Performance of Alpha-Fetoprotein, Protein Induced by Vitamin K Absence, Osteopontin, Dickkopf-1 and Its Combinations for Hepatocellular Carcinoma. PloS One (2016) 11:e0151069. doi: 10.1371/journal.pone.0151069
10. Bonde A, Daly S, Kirsten J, Kondapaneni S, Mellnick V, Menias CO, et al. Human Gut Microbiota-Associated Gastrointestinal Malignancies: A Comprehensive Review. Radiographics (2021) 41:1103–22. doi: 10.1148/rg.2021200168
11. Kho ZY, Lal SK. The Human Gut Microbiome – A Potential Controller of Wellness and Disease. Front Microbiol (2018) 9. doi: 10.3389/fmicb.2018.01835
12. Zhang P, Meng X, Li D, Calderone R, Mao D, Sui B. Commensal Homeostasis of Gut Microbiota-Host for the Impact of Obesity. Front Physiol (2018) 8. doi: 10.3389/fphys.2017.01122
13. Visconti A, Le Roy CI, Rosa F, Rossi N, Martin TC, Mohney RP, et al. Interplay Between the Human Gut Microbiome and Host Metabolism. Nat Commun (2019) 10:4505. doi: 10.1038/s41467-019-12476-z
14. Vivarelli S, Salemi R, Candido S, Falzone L, Santagati M, Stefani S, et al. Gut Microbiota and Cancer: From Pathogenesis to Therapy. Cancers (Basel) (2019) 11:38–63. doi: 10.3390/cancers11010038
15. Kumar M, Nagpal R, Verma V, Kumar A, Kaur N, Hemalatha R, et al. Probiotic Metabolites as Epigenetic Targets in the Prevention of Colon Cancer. Nutr Rev (2013) 71:23–34. doi: 10.1111/j.1753-4887.2012.00542.x
16. Guo Y, Bian X, Liu J, Zhu M, Li L, Yao T, et al. Dietary Components, Microbial Metabolites and Human Health: Reading Between the Lines. Foods (2020) 9:1045. doi: 10.3390/foods9081045
17. Raskov H, Burcharth J, Pommergaard HC. Linking Gut Microbiota to Colorectal Cancer. J Cancer (2017) 8:3378–95. doi: 10.7150/jca.20497
18. Tao X, Wang N, Qin W. Gut Microbiota and Hepatocellular Carcinoma. Gastrointest Tumors (2015) 2:33–40. doi: 10.1159/000380895
19. Jiang JW, Chen XH, Ren Z, Zheng SS. Gut Microbial Dysbiosis Associates Hepatocellular Carcinoma via the Gut-Liver Axis. Hepatobil Pancreat Dis Int (2019) 18:19–27. doi: 10.1016/j.hbpd.2018.11.002
20. Guo X, Jiao Y, Song J, Wang F-S. Gut Microbiota Alteration in Hepatobiliary Diseases: Cause-and-Effect Relationship. Hepatol Int (2021) 15:1305–8. doi: 10.1007/s12072-021-10260-0
21. Ohtani N, Hara E. Gut-Liver Axis-Mediated Mechanism of Liver Cancer: A Special Focus on the Role of Gut Microbiota. Cancer Sci (2021) 112:4433–43. doi: 10.1111/cas.15142
22. Schwabe RF, Greten TF. Gut Microbiome in HCC - Mechanisms, Diagnosis and Therapy. J Hepatol (2020) 72:230–8. doi: 10.1016/j.jhep.2019.08.016
23. Behary J, Amorim N, Jiang X-T, Raposo A, Gong L, Mcgovern E, et al. Gut Microbiota Impact on the Peripheral Immune Response in Non-Alcoholic Fatty Liver Disease Related Hepatocellular Carcinoma. Nat Commun (2021) 12:187. doi: 10.1038/s41467-020-20422-7
24. Kang Y, Cai Y, Yang Y. The Gut Microbiome and Hepatocellular Carcinoma: Implications for Early Diagnostic Biomarkers and Novel Therapies. Liver Cancer (2021) 11:113–25. doi: 10.1159/000521358
25. Lee Y-M, Chang W-C, Lei F-J, Kor C-T, Lai H-C, Chen Y-L, et al. Early Exposure to Gut Microbiome Reduces Hepatocellular Carcinoma Risks in Mice. BioMed Res Int (2020) 2020:9807379. doi: 10.1155/2020/9807379
26. Pomej K, Balcar L, Scheiner B, Semmler G, Meischl T, Mandorfer M, et al. Antibiotic Therapy is Associated With Worse Outcome in Patients With Hepatocellular Carcinoma Treated With Sorafenib. J Hepatocell Carcinoma (2021) 8:1485–93. doi: 10.2147/JHC.S317957
27. Delaune V, Orci LA, Lacotte S, Peloso A, Schrenzel J, Lazarevic V, et al. Fecal Microbiota Transplantation: A Promising Strategy in Preventing the Progression of Non-Alcoholic Steatohepatitis and Improving the Anti-Cancer Immune Response. Expert Opin Biol Ther (2018) 18:1061–71. doi: 10.1080/14712598.2018.1518424
28. Bi C, Xiao G, Liu C, Yan J, Chen J, Si W, et al. Molecular Immune Mechanism of Intestinal Microbiota and Their Metabolites in the Occurrence and Development of Liver Cancer. Front Cell Dev Biol (2021) 9. doi: 10.3389/fcell.2021.702414
29. Ridlon JM, Kang DJ, Hylemon PB, Bajaj JS. Bile Acids and the Gut Microbiome. Curr Opin Gastroenterol (2014) 30:332–8. doi: 10.1097/MOG.0000000000000057
30. Yoshimoto S, Loo TM, Atarashi K, Kanda H, Sato S, Oyadomari S, et al. Obesity-Induced Gut Microbial Metabolite Promotes Liver Cancer Through Senescence Secretome. Nature (2013) 499:97–101. doi: 10.1038/nature12347
31. Yamada S, Takashina Y, Watanabe M, Nagamine R, Saito Y, Kamada N, et al. Bile Acid Metabolism Regulated by the Gut Microbiota Promotes Non-Alcoholic Steatohepatitis-Associated Hepatocellular Carcinoma in Mice. Oncotarget (2018) 9:9925–39. doi: 10.18632/oncotarget.24066
32. Yokohama K, Fukunishi S, Ii M, Nakamura K, Ohama H, Tsuchimoto Y, et al. Rosuvastatin as a Potential Preventive Drug for the Development of Hepatocellular Carcinoma Associated With Non-Alcoholic Fatty Liver Disease in Mice. Int J Mol Med (2016) 38:1499–506. doi: 10.3892/ijmm.2016.2766
33. Thompson SM, Garg I, Ehman EC, Sheedy SP, Bookwalter CA, Carter RE, et al. Non-Alcoholic Fatty Liver Disease-Associated Hepatocellular Carcinoma: Effect of Hepatic Steatosis on Major Hepatocellular Carcinoma Features at MRI. Br J Radiol (2018) 91:20180345. doi: 10.1259/bjr.20180345
34. Zhang X, Coker OO, Chu ES, Fu K, Lau HCH, Wang YX, et al. Dietary Cholesterol Drives Fatty Liver-Associated Liver Cancer by Modulating Gut Microbiota and Metabolites. Gut (2021) 70:761–74. doi: 10.1136/gutjnl-2019-319664
35. Rajesh Y, Sarkar D. Molecular Mechanisms Regulating Obesity-Associated Hepatocellular Carcinoma. Cancers (Basel) (2020) 12:1290–318. doi: 10.3390/cancers12051290
36. Loo TM, Kamachi F, Watanabe Y, Yoshimoto S, Kanda H, Arai Y, et al. Gut Microbiota Promotes Obesity-Associated Liver Cancer Through PGE(2)-Mediated Suppression of Antitumor Immunity. Cancer Discovery (2017) 7:522–38. doi: 10.1158/2159-8290.CD-16-0932
37. Yang W, Ma Y, Liu Y, Smith-Warner SA, Simon TG, Chong DQ, et al. Association of Intake of Whole Grains and Dietary Fiber With Risk of Hepatocellular Carcinoma in US Adults. JAMA Oncol (2019) 5:879–86. doi: 10.1001/jamaoncol.2018.7159
38. Moeini A, Cornellà H, Villanueva A. Emerging Signaling Pathways in Hepatocellular Carcinoma. Liver Cancer (2012) 1:83–93. doi: 10.1159/000342405
39. Li L, Zhao GD, Shi Z, Qi LL, Zhou LY, Fu ZX. The Ras/Raf/MEK/ERK Signaling Pathway and its Role in the Occurrence and Development of HCC. Oncol Lett (2016) 12:3045–50. doi: 10.3892/ol.2016.5110
40. Arzumanyan A, Sambandam V, Clayton MM, Choi SS, Xie G, Diehl AM, et al. Hedgehog Signaling Blockade Delays Hepatocarcinogenesis Induced by Hepatitis B Virus X Protein. Cancer Res (2012) 72:5912–20. doi: 10.1158/0008-5472.CAN-12-2329
41. Parada Venegas D, de la Fuente MK, Landskron G, González MJ, Quera R, Dijkstra G, et al. Short Chain Fatty Acids (SCFAs)-Mediated Gut Epithelial and Immune Regulation and Its Relevance for Inflammatory Bowel Diseases. Front Immunol (2019) 10. doi: 10.3389/fimmu.2019.01486
42. Kobayashi M, Mikami D, Uwada J, Yazawa T, Kamiyama K, Kimura H, et al. A Short-Chain Fatty Acid, Propionate, Enhances the Cytotoxic Effect of Cisplatin by Modulating GPR41 Signaling Pathways in HepG2 Cells. Oncotarget (2018) 9:31342–54. doi: 10.18632/oncotarget.25809
43. Mcbrearty N, Arzumanyan A, Bichenkov E, Merali S, Merali C, Feitelson M. Short Chain Fatty Acids Delay the Development of Hepatocellular Carcinoma in HBx Transgenic Mice. Neoplasia (2021) 23:529–38. doi: 10.1016/j.neo.2021.04.004
44. Liu ZY, Tan XY, Li QJ, Liao GC, Fang AP, Zhang DM, et al. Trimethylamine N-Oxide, a Gut Microbiota-Dependent Metabolite of Choline, is Positively Associated With the Risk of Primary Liver Cancer: A Case-Control Study. Nutr Metab (Lond) (2018) 15:81. doi: 10.1186/s12986-018-0319-2
45. De Chiara F, Heebøll S, Marrone G, Montoliu C, Hamilton-Dutoit S, Ferrandez A, et al. Urea Cycle Dysregulation in Non-Alcoholic Fatty Liver Disease. J Hepatol (2018) 69:905–15. doi: 10.1016/j.jhep.2018.06.023
46. De Chiara F, Thomsen KL, Habtesion A, Jones H, Davies N, Gracia-Sancho J, et al. Ammonia Scavenging Prevents Progression of Fibrosis in Experimental Nonalcoholic Fatty Liver Disease. Hepatology (2020) 71:874–92. doi: 10.1002/hep.30890
47. Zhou J, Tripathi M, Sinha RA, Singh BK, Yen PM. Gut Microbiota and Their Metabolites in the Progression of Non-Alcoholic Fatty Liver Disease. Hepatoma Res (2021) 7:11. doi: 10.20517/2394-5079.2020.134
48. Hill C, Guarner F, Reid G, Gibson GR, Merenstein DJ, Pot B, et al. Expert Consensus Document. The International Scientific Association for Probiotics and Prebiotics Consensus Statement on the Scope and Appropriate Use of the Term Probiotic. Nat Rev Gastroenterol Hepatol (2014) 11:506–14. doi: 10.1038/nrgastro.2014.66
49. Van Baarlen P, Wells JM, Kleerebezem M. Regulation of Intestinal Homeostasis and Immunity With Probiotic Lactobacilli. Trends Immunol (2013) 34:208–15. doi: 10.1016/j.it.2013.01.005
50. Ruiz L, Delgado S, Ruas-Madiedo P, Sánchez B, Margolles A. Bifidobacteria and Their Molecular Communication With the Immune System. Front Microbiol (2017) 8:2345. doi: 10.3389/fmicb.2017.02345
51. Lu K, Dong S, Wu X, Jin R, Chen H. Probiotics in Cancer. Front Oncol (2021) 11. doi: 10.3389/fonc.2021.638148
52. Legesse Bedada T, Feto TK, Awoke KS, Garedew AD, Yifat FT, Birri DJ. Probiotics for Cancer Alternative Prevention and Treatment. BioMed Pharmacother (2020) 129:110409. doi: 10.1016/j.biopha.2020.110409
53. Śliżewska K, Markowiak-Kopeć P, Śliżewska W. The Role of Probiotics in Cancer Prevention. Cancers (Basel) (2020) 13:20–41. doi: 10.3390/cancers13010020
54. Wan MLY, El-Nezami H. Targeting Gut Microbiota in Hepatocellular Carcinoma: Probiotics as a Novel Therapy. Hepatobil Surg Nutr (2018) 7:11–20. doi: 10.21037/hbsn.2017.12.07
55. Kumar M, Verma V, Nagpal R, Kumar A, Gautam SK, Behare PV, et al. Effect of Probiotic Fermented Milk and Chlorophyllin on Gene Expressions and Genotoxicity During AFB₁-Induced Hepatocellular Carcinoma. Gene (2011) 490:54–9. doi: 10.1016/j.gene.2011.09.003
56. Li J, Sung CY, Lee N, Ni Y, Pihlajamäki J, Panagiotou G, et al. Probiotics Modulated Gut Microbiota Suppresses Hepatocellular Carcinoma Growth in Mice. Proc Natl Acad Sci U.S.A. (2016) 113:E1306–1315. doi: 10.1073/pnas.1518189113
57. Kanmani P, Kim H. Probiotics Counteract the Expression of Hepatic Profibrotic Genes via the Attenuation of TGF-β/SMAD Signaling and Autophagy in Hepatic Stellate Cells. PloS One (2022) 17:e0262767. doi: 10.1371/journal.pone.0262767
58. Karlton-Senaye BD, Bansode RR, Randolph PD, Williams LL. Lactobacillus Rhamnosus GG Inhibits BID Dependent-Apoptosis in Human Hepatocellular Carcinoma Cells Exposed to Patulin. J Field Robotics (2017) 6:1. doi: 10.5539/jfr.v6n3p1
59. Kurma K, Manches O, Chuffart F, Sturm N, Gharzeddine K, Zhang J, et al. DEN-Induced Rat Model Reproduces Key Features of Human Hepatocellular Carcinoma. Cancers (Basel) (2021) 13:4981–95. doi: 10.3390/cancers13194981
60. Zhang HL, Yu LX, Yang W, Tang L, Lin Y, Wu H, et al. Profound Impact of Gut Homeostasis on Chemically-Induced Pro-Tumorigenic Inflammation and Hepatocarcinogenesis in Rats. J Hepatol (2012) 57:803–12. doi: 10.1016/j.jhep.2012.06.011
61. Grąt M, Wronka KM, Krasnodębski M, Masior Ł, Lewandowski Z, Kosińska I, et al. Profile of Gut Microbiota Associated With the Presence of Hepatocellular Cancer in Patients With Liver Cirrhosis. Transplant Proc (2016) 48:1687–91. doi: 10.1016/j.transproceed.2016.01.077
62. Khedr OMS, El-Sonbaty SM, Moawed FSM, Kandil EI, Abdel-Maksoud BE. Lactobacillus Acidophilus ATCC 4356 Exopolysaccharides Suppresses Mediators of Inflammation Through the Inhibition of TLR2/STAT-3/P38-MAPK Pathway in DEN-Induced Hepatocarcinogenesis in Rats. Nutr Cancer (2021) 74:1037–47. doi: 10.1080/01635581.2021.1934490
63. Moscovici M. Present and Future Medical Applications of Microbial Exopolysaccharides. Front Microbiol (2015) 6:1012. doi: 10.3389/fmicb.2015.01012
64. Zhang N, Gou Y, Liang S, Chen N, Liu Y, He Q, et al. Dysbiosis of Gut Microbiota Promotes Hepatocellular Carcinoma Progression by Regulating the Immune Response. J Immunol Res (2021) 2021:4973589. doi: 10.1155/2021/4973589
65. Xie G, Wang X, Liu P, Wei R, Chen W, Rajani C, et al. Distinctly Altered Gut Microbiota in the Progression of Liver Disease. Oncotarget (2016) 7:19355–66. doi: 10.18632/oncotarget.8466
66. Ponziani FR, Bhoori S, Castelli C, Putignani L, Rivoltini L, Del Chierico F, et al. Hepatocellular Carcinoma Is Associated With Gut Microbiota Profile and Inflammation in Nonalcoholic Fatty Liver Disease. Hepatology (2019) 69:107–20. doi: 10.1002/hep.30036
67. Ren Z, Li A, Jiang J, Zhou L, Yu Z, Lu H, et al. Gut Microbiome Analysis as a Tool Towards Targeted Non-Invasive Biomarkers for Early Hepatocellular Carcinoma. Gut (2019) 68:1014–23. doi: 10.1136/gutjnl-2017-315084
68. Ni J, Huang R, Zhou H, Xu X, Li Y, Cao P, et al. Analysis of the Relationship Between the Degree of Dysbiosis in Gut Microbiota and Prognosis at Different Stages of Primary Hepatocellular Carcinoma. Front Microbiol (2019) 10. doi: 10.3389/fmicb.2019.01458
69. Chen Y, Yang F, Lu H, Wang B, Chen Y, Lei D, et al. Characterization of Fecal Microbial Communities in Patients With Liver Cirrhosis. Hepatology (2011) 54:562–72. doi: 10.1002/hep.24423
70. Wong VW, Tse CH, Lam TT, Wong GL, Chim AM, Chu WC, et al. Molecular Characterization of the Fecal Microbiota in Patients With Nonalcoholic Steatohepatitis–a Longitudinal Study. PloS One (2013) 8:e62885. doi: 10.1371/journal.pone.0062885
71. Gopalakrishnan V, Spencer CN, Nezi L, Reuben A, Andrews MC, Karpinets TV, et al. Gut Microbiome Modulates Response to Anti-PD-1 Immunotherapy in Melanoma Patients. Science (2018) 359:97–103.
72. Zheng Y, Wang T, Tu X, Huang Y, Zhang H, Tan D, et al. Gut Microbiome Affects the Response to Anti-PD-1 Immunotherapy in Patients With Hepatocellular Carcinoma. J Immunother Cancer (2019) 7:193. doi: 10.1186/s40425-019-0650-9
73. Franzin R, Netti GS, Spadaccino F, Porta C, Gesualdo L, Stallone G, et al. The Use of Immune Checkpoint Inhibitors in Oncology and the Occurrence of AKI: Where Do We Stand? Front Immunol (2020) 11. doi: 10.3389/fimmu.2020.574271
74. Cheung KS, Lam LK, Seto WK, Leung WK. Use of Antibiotics During Immune Checkpoint Inhibitor Treatment Is Associated With Lower Survival in Hepatocellular Carcinoma. Liver Cancer (2021) 10:606–14. doi: 10.1159/000518090
75. Alshammari K, Alsugheir F, Aldawoud M, Alolayan A, Algarni MA, Sabatin F, et al. Association Between Antibiotic Exposure and Survival in Patients With Hepatocellular Carcinoma Treated With Nivolumab. J Clin Oncol (2021) 39:e16186–6. doi: 10.1200/JCO.2021.39.15_suppl.e16186
76. Derosa L, Hellmann MD, Spaziano M, Halpenny D, Fidelle M, Rizvi H, et al. Negative Association of Antibiotics on Clinical Activity of Immune Checkpoint Inhibitors in Patients With Advanced Renal Cell and Non-Small-Cell Lung Cancer. Ann Oncol (2018) 29:1437–44. doi: 10.1093/annonc/mdy103
77. Pinato DJ, Howlett S, Ottaviani D, Urus H, Patel A, Mineo T, et al. Association of Prior Antibiotic Treatment With Survival and Response to Immune Checkpoint Inhibitor Therapy in Patients With Cancer. JAMA Oncol (2019) 5:1774–8. doi: 10.1001/jamaoncol.2019.2785
78. Fessas P, Naeem M, Pinter M, Marron TU, Szafron D, Balcar L, et al. Early Antibiotic Exposure Is Not Detrimental to Therapeutic Effect From Immunotherapy in Hepatocellular Carcinoma. Liver Cancer (2021) 10:583–92. doi: 10.1159/000519108
79. Fessas P, Naeem M, Marron TU, Szafron D, Sharon E, Saeed A, et al. Abstract 485: Early Antibiotic Exposure Delays Disease Progression Following Immune Checkpoint Inhibitor Therapy for Hepatocellular Carcinoma: Evidence From an Observational Study. Cancer Res (2021) 81:485–5. doi: 10.1158/1538-7445.AM2021-485
80. Wang J, He XD, Zhang YC. Antibiotic Prophylaxis in Transarterial Therapy of Hepatocellular Carcinoma: A Meta-Analysis. Can J Gastroenterol (2012) 26:85–91. doi: 10.1155/2012/375956
81. Ma C, Han M, Heinrich B, Fu Q, Zhang Q, Sandhu M, et al. Gut Microbiome-Mediated Bile Acid Metabolism Regulates Liver Cancer via NKT Cells. Science (2018) 360. doi: 10.1126/science.aan5931
82. Abdel-Hamid NI, El-Azab MF, Moustafa YM. Macrolide Antibiotics Differentially Influence Human HepG2 Cytotoxicity and Modulate Intrinsic/Extrinsic Apoptotic Pathways in Rat Hepatocellular Carcinoma Model. Naunyn Schmiedebergs Arch Pharmacol (2017) 390:379–95. doi: 10.1007/s00210-016-1337-0
83. Sbahi H, Di Palma JA. Faecal Microbiota Transplantation: Applications and Limitations in Treating Gastrointestinal Disorders. BMJ Open Gastroenterol (2016) 3:e000087. doi: 10.1136/bmjgast-2016-000087
84. Gulati M, Singh SK, Corrie L, Chandwani L, Singh A, Kapoor B, et al. Fecal Microbiota Transplant: Latest Addition to Arsenal Against Recurrent Clostridium Difficile Infection. Recent Pat Antiinfect Drug Discovery (2020) 16:2–12. doi: 10.2174/1574891X15666200925092354
85. Van Nood E, Vrieze A, Nieuwdorp M, Fuentes S, Zoetendal EG, De Vos WM, et al. Duodenal Infusion of Donor Feces for Recurrent Clostridium Difficile. New Engl J Med (2013) 368:407–15. doi: 10.1056/NEJMoa1205037
86. Kelly CR, Khoruts A, Staley C, Sadowsky MJ, Abd M, Alani M, et al. Effect of Fecal Microbiota Transplantation on Recurrence in Multiply Recurrent Clostridium Difficile Infection: A Randomized Trial. Ann Intern Med (2016) 165:609–16. doi: 10.7326/M16-0271
87. Gupta S, Allen-Vercoe E, Petrof EO. Fecal Microbiota Transplantation: In Perspective. Therap Adv Gastroenterol (2016) 9:229–39. doi: 10.1177/1756283X15607414
88. Shasthry SM. Fecal Microbiota Transplantation in Alcohol Related Liver Diseases. Clin Mol Hepatol (2020) 26:294–301. doi: 10.3350/cmh.2020.0057
89. Bajaj JS, Salzman NH, Acharya C, Sterling RK, White MB, Gavis EA, et al. Fecal Microbial Transplant Capsules Are Safe in Hepatic Encephalopathy: A Phase 1, Randomized, Placebo-Controlled Trial. Hepatology (2019) 70:1690–703. doi: 10.1002/hep.30690
90. Brunt EM, Wong VWS, Nobili V, Day CP, Sookoian S, Maher JJ, et al. Nonalcoholic Fatty Liver Disease. Nat Rev Dis Primers (2015) 1:15080. doi: 10.1038/nrdp.2015.80
91. Calzadilla Bertot L, Adams LA. The Natural Course of Non-Alcoholic Fatty Liver Disease. Int J Mol Sci (2016) 17:774–85. doi: 10.3390/ijms17050774
92. Benedict M, Zhang X. Non-Alcoholic Fatty Liver Disease: An Expanded Review. World J Hepatol (2017) 9:715–32. doi: 10.4254/wjh.v9.i16.715
93. Craven L, Rahman A, Nair Parvathy S, Beaton M, Silverman J, Qumosani K, et al. Allogenic Fecal Microbiota Transplantation in Patients With Nonalcoholic Fatty Liver Disease Improves Abnormal Small Intestinal Permeability: A Randomized Control Trial. Am J Gastroenterol (2020) 115:1055–65. doi: 10.14309/ajg.0000000000000661
94. Fattovich G, Stroffolini T, Zagni I, Donato F. Hepatocellular Carcinoma in Cirrhosis: Incidence and Risk Factors. Gastroenterology (2004) 127:S35–50. doi: 10.1053/j.gastro.2004.09.014
95. Bajaj JS, Shamsaddini A, Fagan A, Sterling RK, Gavis E, Khoruts A, et al. Fecal Microbiota Transplant in Cirrhosis Reduces Gut Microbial Antibiotic Resistance Genes: Analysis of Two Trials. Hepatol Commun (2021) 5:258–71. doi: 10.1002/hep4.1639
96. Zhou D, Pan Q, Shen F, Cao H-X, Ding W-J, Chen Y-W, et al. Total Fecal Microbiota Transplantation Alleviates High-Fat Diet-Induced Steatohepatitis in Mice via Beneficial Regulation of Gut Microbiota. Sci Rep (2017) 7:1529. doi: 10.1038/s41598-017-01751-y
97. Yu Q, Wu L, Ji J, Feng J, Dai W, Li J, et al. Gut Microbiota, Peroxisome Proliferator-Activated Receptors, and Hepatocellular Carcinoma. J Hepatocell Carcinoma (2020) 7:271–88. doi: 10.2147/JHC.S277870
98. Wang Y, Wiesnoski DH, Helmink BA, Gopalakrishnan V, Choi K, Dupont HL, et al. Fecal Microbiota Transplantation for Refractory Immune Checkpoint Inhibitor-Associated Colitis. Nat Med (2018) 24:1804–8. doi: 10.1038/s41591-018-0238-9
99. Khan SA, Tavolari S, Brandi G. Cholangiocarcinoma: Epidemiology and Risk Factors. Liver Int (2019) 39 Suppl 1:19–31. doi: 10.1111/liv.14095
100. Banales JM, Marin JJG, Lamarca A, Rodrigues PM, Khan SA, Roberts LR, et al. Cholangiocarcinoma 2020: The Next Horizon in Mechanisms and Management. Nat Rev Gastroenterol Hepatol (2020) 17:557–88. doi: 10.1038/s41575-020-0310-z
101. Rao B, Ren T, Wang X, Wang H, Zou Y, Sun Y, et al. Dysbiosis in the Human Microbiome of Cholangiocarcinoma. Front Physiol (2021) 12. doi: 10.3389/fphys.2021.715536
102. Zhang T, Zhang S, Jin C, Lin Z, Deng T, Xie X, et al. A Predictive Model Based on the Gut Microbiota Improves the Diagnostic Effect in Patients With Cholangiocarcinoma. Front Cell Infect Microbiol (2021) 11:751795. doi: 10.3389/fcimb.2021.751795
103. Deng T, Li J, He B, Chen B, Liu F, Chen Z, et al. Gut Microbiome Alteration as a Diagnostic Tool and Associated With Inflammatory Response Marker in Primary Liver Cancer. Hepatol Int (2022) 16:99–111. doi: 10.1007/s12072-021-10279-3
104. Ketpueak T, Thiennimitr P, Apaijai N, Chattipakorn SC, Chattipakorn N. Association of Chronic Opisthorchis Infestation and Microbiota Alteration on Tumorigenesis in Cholangiocarcinoma. Clin Transl Gastroenterol (2020) 12:e00292. doi: 10.14309/ctg.0000000000000292
105. Wheatley RC, Kilgour E, Jacobs T, Lamarca A, Hubner RA, Valle JW, et al. Potential Influence of the Microbiome Environment in Patients With Biliary Tract Cancer and Implications for Therapy. Br J Cancer (2022) 126:693–705. doi: 10.1038/s41416-021-01583-8
106. Wu R, Zhang Y, Cheng Q, Wu J, Zhu Y, Shi X, et al. The Effect of Biliary Obstruction, Biliary Drainage and Bile Reinfusion on Bile Acid Metabolism and Gut Microbiota in Mice. Liver Int (2022) 42:135–48. doi: 10.1111/liv.15047
107. Chen Y, Pu W, Maswikiti EP, Tao P, Li X, Wang D, et al. Intestinal Congestion and Reperfusion Injury: Damage Caused to the Intestinal Tract and Distal Organs. Biosci Rep (2021) 41:1–11. doi: 10.1042/BSR20211560
108. Shen YZ, Peng XH, Bai Y, Xiong B, Che P, Jiang DQ. Clinical Observation of the Efficacy of Endoscopic Retrograde Cholangiopancreatography on Elder Choledocholithiasis and Its Effects on the Levels of TNF-α, IL-1, and IL-6. Rev Assoc Med Bras (1992) (2018) 64:1012–6. doi: 10.1590/1806-9282.64.11.1012
109. Jia X, Lu S, Zeng Z, Liu Q, Dong Z, Chen Y, et al. Characterization of Gut Microbiota, Bile Acid Metabolism, and Cytokines in Intrahepatic Cholangiocarcinoma. Hepatology (2020) 71:893–906. doi: 10.1002/hep.30852
110. Kochar R, Banerjee S. Infections of the Biliary Tract. Gastrointest Endosc Clin N Am (2013) 23:199–218. doi: 10.1016/j.giec.2012.12.008
111. Cai H, Kong W-T, Chen C-B, Shi G-M, Huang C, Shen Y-H, et al. Cholelithiasis and the Risk of Intrahepatic Cholangiocarcinoma: A Meta-Analysis of Observational Studies. BMC Cancer (2015) 15:831. doi: 10.1186/s12885-015-1870-0
112. Sia D, Hoshida Y, Villanueva A, Roayaie S, Ferrer J, Tabak B, et al. Integrative Molecular Analysis of Intrahepatic Cholangiocarcinoma Reveals 2 Classes That Have Different Outcomes. Gastroenterology (2013) 144:829–40. doi: 10.1053/j.gastro.2013.01.001
113. Pérez PN, Ramírez MA, Fernández JA, Ladrón De Guevara L. A Patient Presenting With Cholangitis Due to Stenotrophomonas Maltophilia and Pseudomonas Aeruginosa Successfully Treated With Intrabiliary Colistine. Infect Dis Rep (2014) 6:5147. doi: 10.4081/idr.2014.5147
114. Roscetto E, Vitiello L, Muoio R, Soriano AA, Iula VD, Vollaro A, et al. In Vitro Interaction of Stenotrophomonas Maltophilia With Human Monocyte-Derived Dendritic Cells. Front Microbiol (2015) 6. doi: 10.3389/fmicb.2015.00723
115. Chng KR, Chan SH, Ng AHQ, Li C, Jusakul A, Bertrand D, et al. Tissue Microbiome Profiling Identifies an Enrichment of Specific Enteric Bacteria in Opisthorchis Viverrini Associated Cholangiocarcinoma. EBioMedicine (2016) 8:195–202. doi: 10.1016/j.ebiom.2016.04.034
116. Saab M, Mestivier D, Sohrabi M, Rodriguez C, Khonsari MR, Faraji A, et al. Characterization of Biliary Microbiota Dysbiosis in Extrahepatic Cholangiocarcinoma. PloS One (2021) 16:e0247798. doi: 10.1371/journal.pone.0247798
117. Chen B, Fu SW, Lu L, Zhao H. A Preliminary Study of Biliary Microbiota in Patients With Bile Duct Stones or Distal Cholangiocarcinoma. BioMed Res Int (2019) 2019:1092563. doi: 10.1155/2019/1092563
118. Dangtakot R, Intuyod K, Ahooja A, Wongwiwatchai J, Hanpanich P, Lulitanond A, et al. Profiling of Bile Microbiome Identifies District Microbial Population Between Choledocholithiasis and Cholangiocarcinoma Patients. Asian Pac J Cancer Prev (2021) 22:233–40. doi: 10.31557/APJCP.2021.22.1.233
119. Dyson JK, Beuers U, Jones DEJ, Lohse AW, Hudson M. Primary Sclerosing Cholangitis. Lancet (2018) 391:2547–59. doi: 10.1016/S0140-6736(18)30300-3
120. Zhang Q, Ma C, Duan Y, Heinrich B, Rosato U, Diggs LP, et al. Gut Microbiome Directs Hepatocytes to Recruit MDSCs and Promote Cholangiocarcinoma. Cancer Discovery (2021) 11:1248–67. doi: 10.1158/2159-8290.CD-20-0304
121. Avilés-Jiménez F, Guitron A, Segura-López F, Méndez-Tenorio A, Iwai S, Hernández-Guerrero A, et al. Microbiota Studies in the Bile Duct Strongly Suggest a Role for Helicobacter Pylori in Extrahepatic Cholangiocarcinoma. Clin Microbiol Infect (2016) 22:178.e111–178.e122. doi: 10.1016/j.cmi.2015.10.008
122. Serra N, Di Carlo P, Gulotta G, D’ Arpa F, Giammanco A, Colomba C, et al. Bactibilia in Women Affected With Diseases of the Biliary Tract and Pancreas. A STROBE Guidelines-Adherent Cross-Sectional Study in Southern Italy. J Med Microbiol (2018) 67:1090–5. doi: 10.1099/jmm.0.000787
123. Lee H, Lee HK, Min SK, Lee WH. 16s rDNA Microbiome Composition Pattern Analysis as a Diagnostic Biomarker for Biliary Tract Cancer. World J Surg Oncol (2020) 18:19. doi: 10.1186/s12957-020-1793-3
124. Dong L, Lu D, Chen R, Lin Y, Zhu H, Zhang Z, et al. Proteogenomic Characterization Identifies Clinically Relevant Subgroups of Intrahepatic Cholangiocarcinoma. Cancer Cell (2022) 40:70–87.e15. doi: 10.1016/j.ccell.2021.12.006
125. Nemunaitis JM, Brown-Glabeman U, Soares H, Belmonte J, Liem B, Nir I, et al. Gallbladder Cancer: Review of a Rare Orphan Gastrointestinal Cancer With a Focus on Populations of New Mexico. BMC Cancer (2018) 18:665. doi: 10.1186/s12885-018-4575-3
126. Kanthan R, Senger J-L, Ahmed S, Kanthan SC. Gallbladder Cancer in the 21st Century. J Oncol (2015) 2015:967472. doi: 10.1155/2015/967472
127. Choi SJ, Kim Y, Jeon J, Gwak HJ, Kim M, Kang K, et al. Association of Microbial Dysbiosis With Gallbladder Diseases Identified by Bile Microbiome Profiling. J Korean Med Sci (2021) 36:e189. doi: 10.3346/jkms.2021.36.e189
128. Tsuchiya Y, Loza E, Villa-Gomez G, Trujillo CC, Baez S, Asai T, et al. Metagenomics of Microbial Communities in Gallbladder Bile From Patients With Gallbladder Cancer or Cholelithiasis. Asian Pac J Cancer Prev (2018) 19:961–7.
129. Koshiol J, Wozniak A, Cook P, Adaniel C, Acevedo J, Azócar L, et al. Salmonella Enterica Serovar Typhi and Gallbladder Cancer: A Case-Control Study and Meta-Analysis. Cancer Med (2016) 5:3310–235. doi: 10.1002/cam4.915
130. Walawalkar YD, Gaind R, Nayak V. Study on Salmonella Typhi Occurrence in Gallbladder of Patients Suffering From Chronic Cholelithiasis-a Predisposing Factor for Carcinoma of Gallbladder. Diagn Microbiol Infect Dis (2013) 77:69–73. doi: 10.1016/j.diagmicrobio.2013.05.014
131. Scanu T, Spaapen RM, Bakker JM, Pratap CB, Wu LE, Hofland I, et al. Salmonella Manipulation of Host Signaling Pathways Provokes Cellular Transformation Associated With Gallbladder Carcinoma. Cell Host Microbe (2015) 17:763–74. doi: 10.1016/j.chom.2015.05.002
132. Giuffrè M, Campigotto M, Campisciano G, Comar M, Crocè LS. A Story of Liver and Gut Microbes: How Does the Intestinal Flora Affect Liver Disease? A Review of the Literature. Am J Physiol Gastrointest Liver Physiol (2020) 318:G889–906. doi: 10.1152/ajpgi.00161.2019
133. Menezes-Garcia Z, Do Nascimento Arifa RD, Acúrcio L, Brito CB, Gouvea JO, Lima RL, et al. Colonization by Enterobacteriaceae Is Crucial for Acute Inflammatory Responses in Murine Small Intestine via Regulation of Corticosterone Production. Gut Microbes (2020) 11:1531–46. doi: 10.1080/19490976.2020.1765946
134. Liu Z, Kemp TJ, Gao Y-T, Corbel A, Mcgee EE, Roa JC, et al. Circulating Levels of Inflammatory Proteins and Survival in Patients With Gallbladder Cancer. Sci Rep (2018) 8:5671. doi: 10.1038/s41598-018-23848-8
135. Chen H-J, Hu M-H, Xu F-G, Xu H-J, She J-J, Xia H-P. Understanding the Inflammation-Cancer Transformation in the Development of Primary Liver Cancer. Hepatoma Res (2018) 4:29. doi: 10.20517/2394-5079.2018.18
136. Goodwin AC, Destefano Shields CE, Wu S, Huso DL, Wu X, Murray-Stewart TR, et al. Polyamine Catabolism Contributes to Enterotoxigenic Bacteroides Fragilis-Induced Colon Tumorigenesis. Proc Natl Acad Sci U.S.A. (2011) 108:15354–9. doi: 10.1073/pnas.1010203108
137. Espinoza JA, Bizama C, García P, Ferreccio C, Javle M, Miquel JF, et al. The Inflammatory Inception of Gallbladder Cancer. Biochim Biophys Acta (2016) 1865:245–54. doi: 10.1016/j.bbcan.2016.03.004
138. Szajewska H, Kołodziej M. Systematic Review With Meta-Analysis: Lactobacillus Rhamnosus GG in the Prevention of Antibiotic-Associated Diarrhoea in Children and Adults. Aliment Pharmacol Ther (2015) 42:1149–57. doi: 10.1111/apt.13404
139. Orlando A, Linsalata M, Russo F. Antiproliferative Effects on Colon Adenocarcinoma Cells Induced by Co-Administration of Vitamin K1 and Lactobacillus Rhamnosus GG. Int J Oncol (2016) 48:2629–38. doi: 10.3892/ijo.2016.3463
140. Rokka S, Myllykangas S, Joutsjoki V. Effect of Specific Colostral Antibodies and Selected Lactobacilli on the Adhesion of Helicobacter Pylori on AGS Cells and the Helicobacter-Induced IL-8 Production. Scand J Immunol (2008) 68:280–6. doi: 10.1111/j.1365-3083.2008.02138.x
141. Ritze Y, Bárdos G, Claus A, Ehrmann V, Bergheim I, Schwiertz A, et al. Lactobacillus Rhamnosus GG Protects Against Non-Alcoholic Fatty Liver Disease in Mice. PloS One (2014) 9:e80169. doi: 10.1371/journal.pone.0080169
142. Cunningham M, Azcarate-Peril MA, Barnard A, Benoit V, Grimaldi R, Guyonnet D, et al. Shaping the Future of Probiotics and Prebiotics. Trends Microbiol (2021) 29:667–85. doi: 10.1016/j.tim.2021.01.003
143. Liu J, Geng W, Sun H, Liu C, Huang F, Cao J, et al. Integrative Metabolomic Characterisation Identifies Altered Portal Vein Serum Metabolome Contributing to Human Hepatocellular Carcinoma. Gut (2021) 71:1203–13. doi: 10.1136/gutjnl-2021-325189
144. Vimal J, Himal I, Kannan S. Role of Microbial Dysbiosis in Carcinogenesis & Cancer Therapies. Indian J Med Res (2020) 152:553–61. doi: 10.4103/ijmr.IJMR_1026_18
Keywords: hepatobiliary tumors, gut microbiota, metabolites, probiotics, antibiotics, fecal microbial transplantation
Citation: Qin H, Yuan B, Huang W and Wang Y (2022) Utilizing Gut Microbiota to Improve Hepatobiliary Tumor Treatments: Recent Advances. Front. Oncol. 12:924696. doi: 10.3389/fonc.2022.924696
Received: 20 April 2022; Accepted: 20 June 2022;
Published: 18 July 2022.
Edited by:
Yunfei Xu, Shandong University, ChinaReviewed by:
Peter Suenaert, A-Mansia Biotech, BelgiumLutao Du, The Second Hospital of Shandong University, China
Di Zhang, Peking University, China
Copyright © 2022 Qin, Yuan, Huang and Wang. This is an open-access article distributed under the terms of the Creative Commons Attribution License (CC BY). The use, distribution or reproduction in other forums is permitted, provided the original author(s) and the copyright owner(s) are credited and that the original publication in this journal is cited, in accordance with accepted academic practice. No use, distribution or reproduction is permitted which does not comply with these terms.
*Correspondence: Wei Huang, d2VpaHVhbmdAY2NtdS5lZHUuY24=; Yan Wang, eWFud2FuZ0BjaWNhbXMuYWMuY24=
†These authors have contributed equally to this work and share first authorship