- 1Department of Surgery and Anatomy, Ribeirão Preto Medical School, University of São Paulo (USP), São Paulo, Brazil
- 2Molecular Oncology Research Center, Barretos Cancer Hospital, Barretos, São Paulo, Brazil
- 3Department of Clinical, Toxicological and Bromatological Analysis, University of São Paulo (USP), Faculty of Pharmaceutical Sciences of Ribeirão Preto, São Paulo, Brazil
- 4Department of Neurosurgery, Brigham and Women’s Hospital and Harvard Medical School, Boston, MA, United States
- 5Department of Neuroscience and Physiology, SUNY Upstate Medical University, Syracuse, NY, United States
- 6Department of Pathology, School of Medicine, UNESP– Univ. Estadual Paulista, Botucatu, Brazil
- 7Barretos School of Health Sciences, Dr. Paulo Prata – FACISB, Barretos, Brazil
- 8Department of Neurology, Medical School, University of São Paulo (USP), São Paulo, Brazil
- 9Department of Neurology, Faculty of Medicine, Federal University of São Paulo (UNIFESP), São Paulo, Brazil
- 10Department of Internal Medicine, Faculty of Medicine, University of São Paulo (USP), São Paulo, Brazil
- 11Department of Human Genetics, McGill University, Montreal, QC, Canada
Integrins are heterodimeric transmembrane glycoproteins resulting from the non-covalent association of an α and β chain. The major integrin receptor for collagen/laminin, α2β1 is expressed on a wide variety of cell types and plays an essential role in the adhesion of normal and tumor cells to the extracellular matrix. Integrin-triggered signaling pathways promote the invasion and survival of glioma cells by modifying the brain microenvironment. In this study, we investigated the association of a specific genetic polymorphism of integrin α2β1 with the incidence of diffusely infiltrating astrocytoma and the progression of these tumors. Single-nucleotide polymorphism in intron 7 of the integrin ITGA2 gene was examined in 158 patients and 162 controls using polymerase chain reaction and restriction enzyme analysis. The ITGA2 genotype +/+ (with a BglII restriction site in both alleles) exhibited higher frequency in grade II astrocytoma compared to control (P = 0.02) whereas the genotype -/- (lacking the BglII site) correlated with the poorest survival rate (P = 0.04). In addition, in silico analyses of ITGA2 expression from low-grade gliomas (LGG, n = 515) and glioblastomas (GBM, n = 159) indicated that the higher expression of ITGA2 in LGG was associated with poor overall survival (P < 0.0001). However, the distribution of integrin ITGA2 BglII genotypes (+/+, +/-, -/-) was not significantly different between astrocytoma subgroups III and IV (P = 0.65, 0.24 and 0.33; 0.29, 0.48, 0.25, respectively) compared to control. These results suggest a narrow association between the presence of this SNP and indicate that further studies with larger samples are warranted to analyze the relation between tumor grade and overall survival, highlighting the importance of determining these polymorphisms for prognosis of astrocytomas.
Introduction
Gliomas constitute the most common primary brain tumors and include several histologically subtypes, most of them malignant and highly invasive (1). High-grade gliomas including glioblastoma (GB) are considered among the most devastating cancers due to their markedly short post-diagnosis survival time (2), accounting for approximately 15% of all intracranial neoplasms and 60%–75% of astrocytic tumors (3, 4).
A hallmark of the severity of astrocytomas is the ability of tumor cells to invade and infiltrate the brain parenchyma (5). Although the molecular mechanisms underlying these events remain to be better elucidated, the first step encompasses the adhesion of tumor cells to the host’s extracellular matrix (ECM), which is mediated primarily by integrins on the tumor cell surface (6). Aggregation of integrin receptors followed by association of cytoskeletal proteins and tyrosine kinase-mediated phosphorylation are key events responsible for diverse cell responses such as cell migration and differentiation, tissue remodeling, cell proliferation, angiogenesis, and tumor cell invasion, metastasis, and survival (7–11).
Several integrin subunits are significantly upregulated in GB compared to normal brain tissue (12) including α2, α3, α4, α5, α6, and β1 integrins (11, 13–15). Integrin expression and ECM production are directly correlated with the tumor grade and have been found to promote human glioma progression and reduce survival (15–17).
Integrins are transmembrane glycoproteins with non-covalently associated and chains, which mediate the interaction of tumor cells with their microenvironment (17–19). The association of distinctive and subunits determines the functional specificity of receptors (11). Eight different β chains and 18 α chains have been described, whereas only 24 different heterodimers have been observed (7, 19, 20). Among these, α2β1 integrin is a major collagen receptor that plays an essential role in the adhesion of normal and tumor cells to the extracellular matrix (20–23).
The gene encoding integrin α2 chain (ITGA2) is described in the occurrence and progression of multiple cancers, including colorectal cancer, lung cancer, and breast cancer (24, 25). ITGA2 mediated adhesion to type I collagen and are expressed on cancer cells, immune cells, stroma cells, and endothelial cells (9, 21, 26). The high expression in tumor tissue identified ITGA2 as a potential therapeutic target to treatment of cancer. In addition, ITGA2 have been used as a novel molecular target to treat GBM; nevertheless, its role in diffuse glioma still remains to be elucidated (27). However, changes in ITGA2 expression may affect therapeutic targets, immune microenvironment, and the immunogenicity of glioma tumors (27, 28).
ITGA2 has several single-nucleotide polymorphisms (SNPs) including the BglII polymorphism (29, 30). A/G SNP in intron 7 creates a restriction site for the enzyme BglII (sequence AGATCT, BglII +/-) (31). This polymorphism is in linkage disequilibrium, and the BglII (+) allele is linked to the 807T/873A allele. BglII (-) is linked to the 807C/873G allele. The BglII(+) allele has been associated with a high level of ITGA2, and BglII (-) was associated with a low level (29–31). Additionally, the ITGA2 BglII polymorphism has been associated with increased risk for higher disease stages of breast cancer (32), positive history for oral cancer (33), and prevalence of diabetic retinopathy, myocardial infarction, and stroke (34–36).
Here, we hypothesize that the functional ITGA2 BglII polymorphism of integrin α2β1 can also correlate with the incidence or progression of glioma tumors. To test this hypothesis, the prevalence of this polymorphism was evaluated in patients with diffuse infiltrating astrocytomas (malignant grades II to IV) compared to a healthy control group. Our results indicate that BglII polymorphisms of ITGA2 have increased frequency in grade II astrocytoma cases and suggests a protective effect on the risk to LGG; however, more studies are necessary to correlate the polymorphism with tumor progression and overall survival.
Materials and Methods
Subject ́ Recruitment
The study involved 320 unrelated individuals with similar ethnic backgrounds, 158 patients with astrocytomas, and 162 age- and sex-matched controls without histories of cancer or other major disease. Individuals were recruited at the Clinical Hospital of Ribeirão Preto Medical School, University of São Paulo (HC-FMRPUSP), Clinical Hospital of the Medical School, University of São Paulo (HC-FMUSP), and São Paulo Hospital, Federal University of São Paulo (UNIFESP) during the Cancer Clinical Genome Project (FAPESP 01/13716-7, 03/00960-2, 04/12133-6).
Tumor cases and controls were distributed as 97 men/61 women and 97 men/65 women, respectively. The distribution of the 158 patients with astrocytoma was as follows: 28 with grade II, 26 with grade III, and 104 grade with IV astrocytoma (WHO 2016). The control group consisted of individuals who lived in the study areas for at least 1 year with any known history of cancer or chronic disease in a self-reported questionnaire. On the basis of phenotype characteristics and family history, 120 patients and 113 controls were identified as white (European descendants); 27 patients and 27 controls as mulatto, and 8 patients and 13 control as black (African descendants); 2 and 8 were oriental descendants; and 1 and 1 were classified as others. Epidemiological data from the study population were obtained by a standard interviewer-administered questionnaire, including data on social habits, health problems, and ancestry. The human subject protocol was approved by the local Institutional Review Boards of the participating institutions; written informed consent was obtained from all subjects or their parents.
Genotyping
Genomic DNA was extracted from peripheral blood lymphocytes by the conventional phenol-chloroform method. Isolated DNA was resuspended in Tris–EDTA buffer (pH 8.0) and stored at -20°C until use. Polymerase chain reaction-restriction fragment length polymorphism (PCR-RFLP) to determine the ITGA2 genotype using BglII (New England Biolabs, Beverly, MA) was carried out as previously described [26]. The PCR primers used were as follows (5′–3′): forward, GATTTAACTTTCCCGACTGCCTTC (nucleotide number 2789-2812); reverse, CATAGGTTTTTGGGGAACAGGTGG (nucleotide number 3346-3369, GenBank accession number AF035968). Restriction fragments were separated by electrophoresis using 2% agarose gels. The PCR products containing a BglII (+) site yielded fragments of 200 and 400 bp upon enzymatic digestion, as shown in Figure 1.
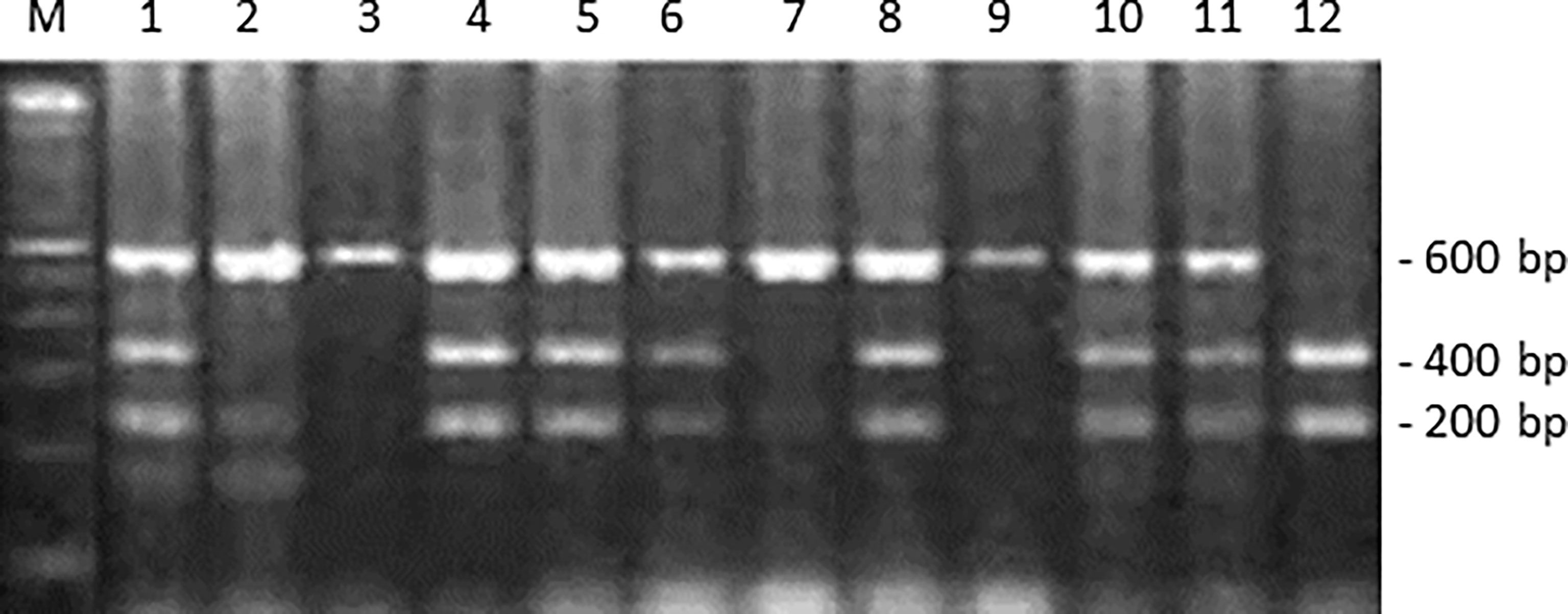
Figure 1 ITGA2 polymorphism genotyping by PCR-RFLP assay. This panel shows the BglII digestion of ITGA2 gene PCR products. Lane M: 100-bp molecular marker. Lanes 2, 3, 7, and 9 are representative of a homozygote -/- (absence of BglII site); lanes 1, 4, 5, 6, 8, 10, and 11 of a heterozygote -/+; and lane 12 of a homozygote polymorphic +/+ (presence of BglII site).
In Silico Analysis of ITGA2 Expression in TCGA Dataset
There were obtained normalized RNASeq and clinicopathological data from The Cancer Genome Atlas (TCGA) from low-grade gliomas (LGG, n = 515) and glioblastomas (GBM, n = 1) using the RTCGA Toolbox package (37) on R. The number of reads of ITGA2 was log10-transformed. Then, patients were distributed into two groups according to the median. Those with the number of reads equal or higher to the median were considered with a high expression. Otherwise, the patients were considered with a low expression.
In LGG, the expression of ITGA2 was associated with histology (astrocytoma, oligoastrocytoma, or oligodendroglioma), grade (2 or 3), IDH, TERT, ATRX mutation status, MGMT promoter methylation status, and subtype. Moreover, in GBM, the expression of ITGA2 was associated with IDH, TERT, ATRX mutation status, MGMT promoter methylation status, and subtype. The expression of ITGA2 was analyzed according to Noushmehr et al. and Cecarelli et al. (38, 39). Additionally, overall survival was analyzed through Kaplan–Meier curves, using the log-rank test to compare the groups based on ITGA2 expression. Finally, hazard ratio and 95% confidence interval were determined using Cox proportional hazards in LGG and GBM using ITGA2 expression as contrast groups and death as endpoint.
Statistical Analysis
Differences between groups were compared by chi-square test or Fisher´s exact test. The 95% confidence intervals (95% CI) of the percentage were calculated by assuming a binomial distribution. Sex-age-adjusted odds ratio (ORs) and 95% confidence interval (95% CI) were calculated according to an unconditional logistic model. Goodness of fit of genotype distribution was analyzed by the Hardy–Weinberg equilibrium. Values of P < 0.05 were considered statistically significant. All statistical analyses were performed using the statistical package SPSS.
Results
The genotype and allele frequency for ITGA2 BglII polymorphism in the tumor and control groups are shown in Table 1. The frequencies for the three possible genotype frequencies (+/+, +/-, and -/-) were in agreement with those predicted by the Hardy–Weinberg equilibrium. Genotype and allelic frequency were compared between tumor cases and controls as well as within the subgroups of astrocytomas (WHO grade II, III, and IV) (Table 2 and Supplementary Tables S1, S2).
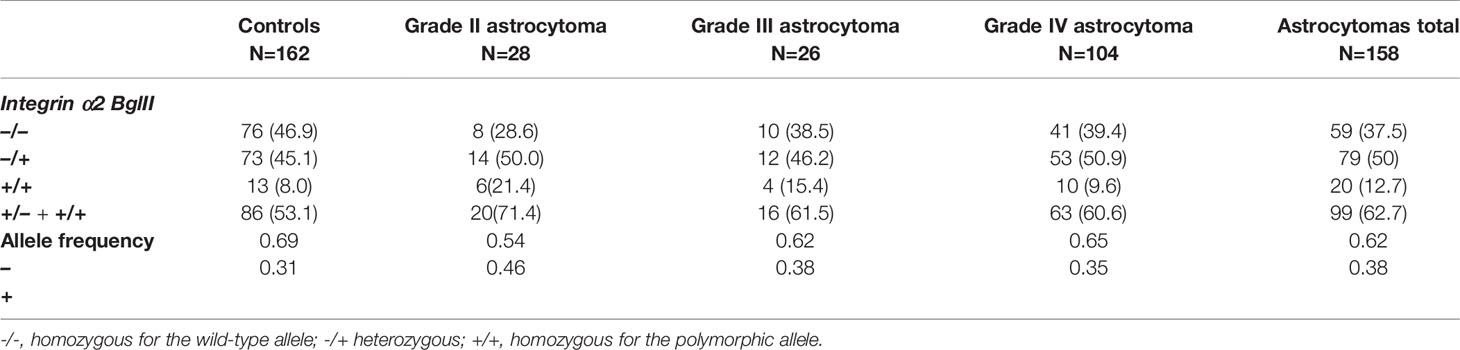
Table 1 Distribution of allele and genotype frequencies of polymorphisms and statistical data obtained from the analysis of integrin α2 BglII polymorphisms in astrocytoma grades II, III, and IV and control.
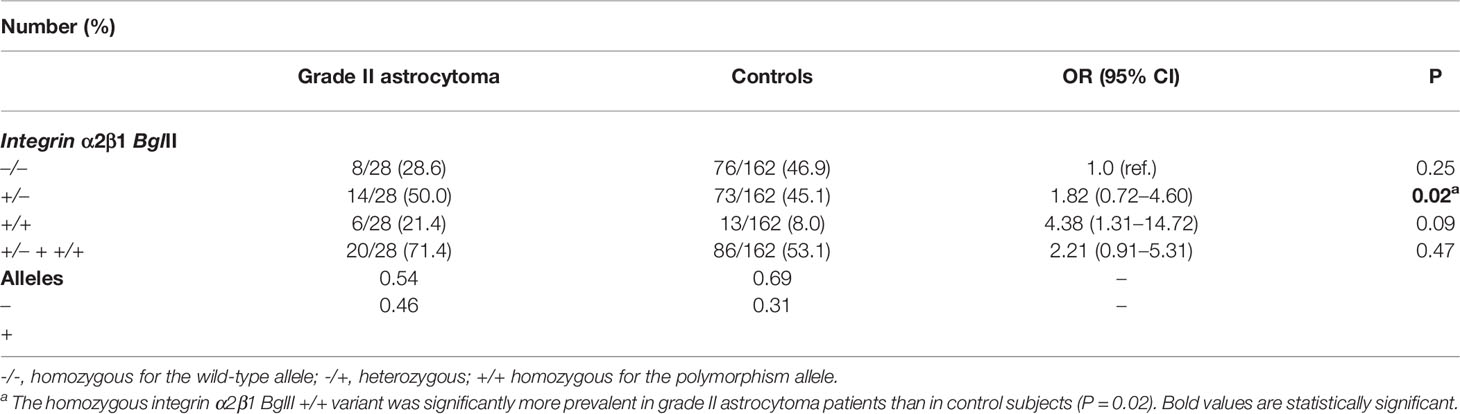
Table 2 Distribution of allele and genotype frequencies of polymorphisms and statistical data obtained from the analysis of integrin α2 BglII polymorphisms in the astrocytoma grade II and control.
The frequencies of ITGA2 BglII polymorphism observed in control subjects were in agreement with those reported for other populations (Table 1) (36, 40). Multivariate analysis did not reveal an association of this polymorphism with age, race, or gender as co-variable (Supplementary Tables S3, S4). In contrast, male patients with astrocytoma showed higher frequencies than women for the ITGA2 BglII +/+ and +/- genotypes; however, these differences were not statistically significant.
Based on TCGA database, we analyzed the expression of ITGA2 on gliomas. The analyses demonstrated that, in LGG, oligodendrogliomas were associated with low ITGA2 expression as well as lower-grade tumors (Grade 2). Additionally, low ITGA2 expression was associated with mutation in IDH, methylation in MGMT and subtype IDHmut-codel or codel, and mesenchymal-like tumors (Supplementary Table S5). Similarly, in GBM, low ITGA2 expression was associated with mutation in IDH. Moreover, low ITGA2 expression was associated with absence of mutation in TERT and G-CIMP and proneural tumors (Supplementary Table S6). Finally, a poor overall survival was found in LGG patients with high expression of ITGA2 (Figure 2, P < 0.0001 in log rank test), presenting a hazard ratio of 2.361 (95CI 1.633–3.412, P < 0.001 in Cox proportional hazards test, Supplementary Table S7). No difference was found regarding the overall survival of GBM patients considering ITGA2 expression (Figure 2 and Supplementary Table S7).
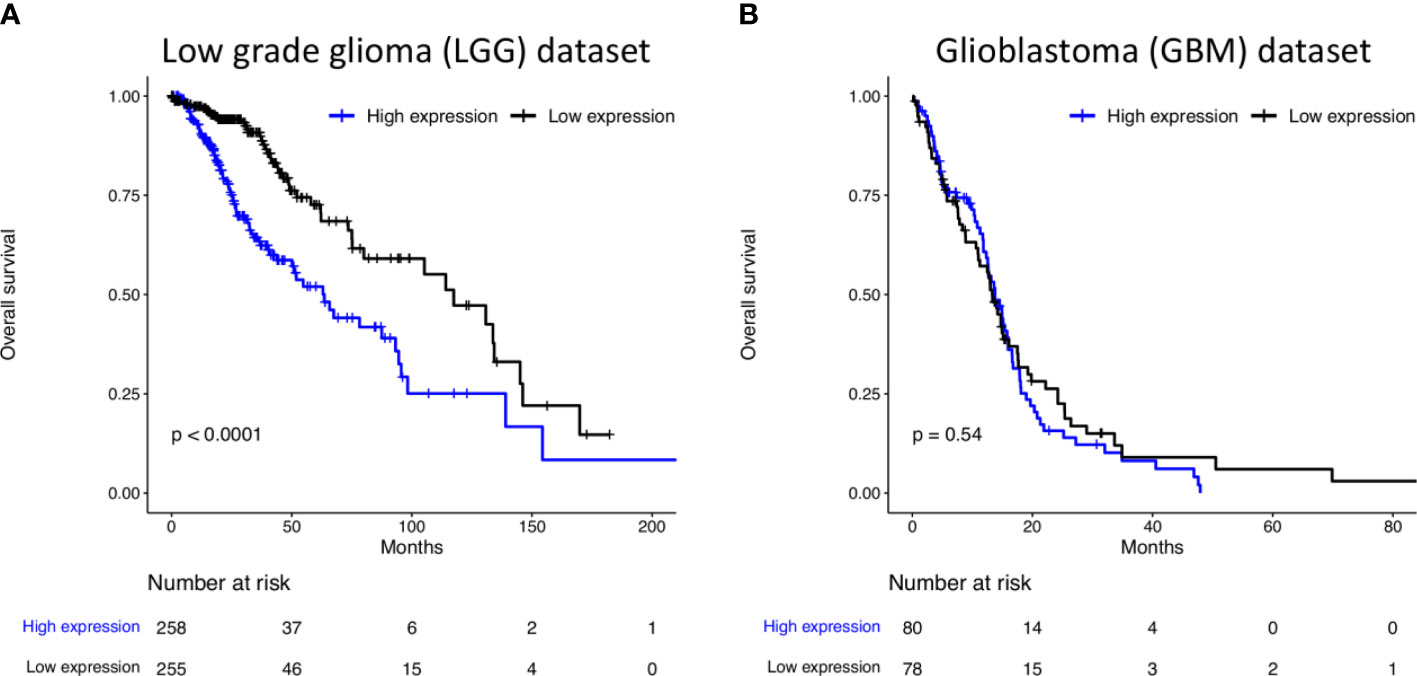
Figure 2 Kaplan–Meier curves of survival related to ITGA2 in (A) 515 patients LGG. LGG patients with high expression of ITGA2 had a poorer overall survival compared with patients with low expression and (B) 159 patients with GBM. The expression of ITGA2 in GBM was not statistically significant when compared with patients with low and high expressions and overall survival.
Our results revealed that the ITGA2 BglII +/+ genotype was significantly overexpressed in patients with grade II astrocytoma compared to controls, OR (95% CI) 4.38 (1.31–14.72) and P = 0.02 (Table 2). However, the overrepresentation of this genotype did not extend to the astrocytoma subgroups III and IV (P = 0.65, 0.24, and 0.53; 0.29, 0.48, 0.25, respectively), suggesting a narrow association between the presence of this SNP and tumor grade (Table 1 and Supplementary Table S1). Also, there were no significant differences in the frequencies of these genotypes when comparing each tumor grade against the other grades (Supplementary Table S2). Although the ITGA2 BglII +/+ polymorphism was overrepresented in grade II astrocytomas, it was associated with increased survival profile in this group. Survival curves for the three ITGA2 genotypes were significantly different for ITGA2 BglII +/+ and +/+,+/- genotypes (P = 0.04 and P = 0.01, respectively) (Figures 3A, B). However, the ITGA2 BglII polymorphism did not show a statistically significant correlation with survival of higher-grade astrocytomas (P > 0.05).
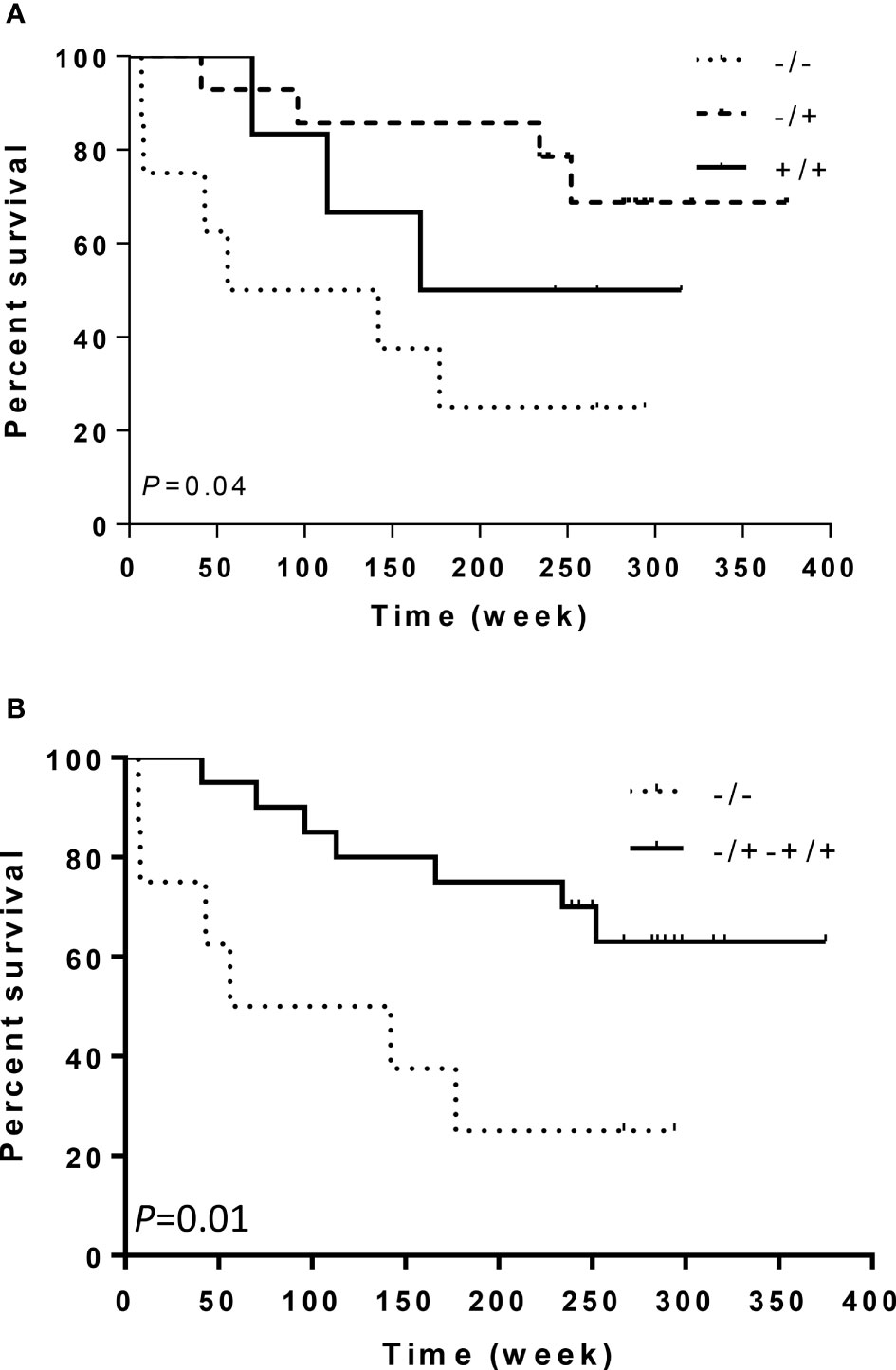
Figure 3 Kaplan–Meier curves of survival related to ITGA2 polymorphisms in 28 patients with astrocytoma grade II. Patients with ITGA2 -/- had a poorer prognosis than patients with (A) ITGA2 +/+ or (B) ITGA2 +/+,+/-.
Discussion
Adhesive molecules of the integrin family, including integrin α2β1, integrin α2β3, and GP Ibα (specially integrins), are critical for adhesion of tumor cells and participate in the cellular mechanisms of tumoral progression (41–44). The subunit of integrin ITGA2 was found to be upregulated in several types of cancer and has been associated with tumor cell proliferation, migration, invasion, and angiogenesis (45–47). In breast cancer, ITGA2 has been linked to tumor aggressiveness, cancer progression (via EMT—epithelial–mesenchymal transition), and metastatic ability (47). Interestingly, Guo et al. described that in human GBM cells, ITGA2 expression is higher than epidermal growth factor receptor (EGFR), an important target in GBM. In addition, they described that ITGA2 was less expressed on normal glial cells, then they suggested ITGA2 as a novel GBM-specific target (27). Nonetheless, the role of ITGA2 in glioma tumor is still not well understood. Furthermore, the high expression in several carcinoma cells of epithelial origin indicates that ITGA2 is an important key pathway in cancer pathogenesis (48–50). The abnormal expression of ITGA2 has been correlated with unfavorable prognoses in multiple types of cancer. The nucleotide polymorphisms affecting adhesive molecules have been associated with the risk and prognosis of astrocytoma tumors (51).
In this study, we have found that a specific polymorphism in the gene coding for a chain of integrin α2β1 (corresponding to the ITGA2 BglII site) was associated with differences in survival of patients with low-grade astrocytoma, with one specific variant (ITGA2 BglII +/+) being overrepresented in grade II astrocytoma cases compared to control individuals. To our knowledge, this is the first study investigating the possible involvement of the integrin α2β1 genomic variant in the prevalence and overall survival of gliomas. Integrin α2 has been investigated in platelets, where it mediates the adhesion of these cells to collagen and regulates platelet function (11, 52, 53). To other solid tumors, alpha chain integrins are sufficient to mediate adhesion to the luminal side of blood vessels and promote extravasation and angiogenesis (54, 55). In pancreatic cancer, the integrin β1 subunit mediates the tumor cell interaction with the tumor microenvironment and ECM proteins, such as types I and IV collagen, laminin, and fibronectin (56); in breast cancer, colorectal cancer, and lung and hepatocellular carcinoma, integrin has been linked to tumor evolution and aggressiveness (24, 25, 28, 57). The aggressiveness with enhancement of metastasis and stemness of colorectal cancer cells have been associated with the interaction of α2β1 that activates the PI3K/Akt/Snail signaling pathway (19). In addition, the expression levels of integrin α2β1, α2β3, and glycoprotein Ibα are associated with invasiveness of tumor cells (58, 59) and could be relevant to explain the correlation between integrin α2β1 polymorphism and glioma grade (60).
Histological analysis of high-grade glioma indicates that integrins α2β1, α5β1, and α6β1 are strongly expressed compared to normal brain tissue (16, 61). All these integrins share the β1 subunit, which is involved in the adhesion, motility, and invasion of glioma cells in vitro (62) as well as tumor dispersion in the brain (63). These data suggest that β1 subunit expression might be related to the clinically invasive phenotype of gliomas. Therefore, the polymorphic genetic variation affecting the expression or function of these adhesive receptors could modify the risk of development and the biological aggressiveness of astrocytomas.
We found that the genotypes of ITGA2 BglII for gliomas and control individuals were distributed in accordance with the Hardy–Weinberg equilibrium and in agreement with those previously published to Caucasian subjects. In control subjects, the frequencies of ITGA2 BglII polymorphisms for genotypes -/-, -/+, and +/+ were, respectively, 46.9, 45.1, and 8.0 matching prior reports (36) in diabetic retinopathy and in diabetic patients (40).
The total distribution of ITGA2 BglII genotypes was not significantly different between astrocytomas and controls. Similar results have been described to other tumors and population and failed to find or increased the risk for tumor progression with BglII (+) polymorphism (64–67). However, future investigation in larger cohorts of patients, and among various racial groups, will be necessary to further define the role of the BglII genotype in the pathogenesis of GBM. Regarding grade II astrocytoma, the ITGA2 genotype (+/+ and +/-) exhibited higher frequency and, in contrast, increased median survival compared to the homozygous -/- genotype. These results suggest that the BglII polymorphism in the gene which codes for the α2 chain of integrin α2β1 may have a protective effect on the risk to low-grade astrocytomas. Furthermore, we analyzed the potential impact of ITGA2 on the overall survival of glioma grade II, III, and IV patients using the same database (TCGA). The correlation between overall survival and ITGA2 expression was not statistically significant to grade III and IV of astrocytomas. In contrast, in a recent study the ITGA2 expression was significantly associated with decreased GBM patient survival (27). The difference in the results may be due to the number of patients analyzed in the two studies and highlight the relevance of future investigation in a large cohort of patients.
The poor prognosis for patients with LGG shows the diversity of this malignant glioma; therefore, new treatment strategies are needed to continuously improve the prognosis of LGG patients. Similar results to LGG have been described in a series of systematic analysis (68). In another study, the high expression of ITGA2 was associated with reduced survival rate of solid cancer (28). The high expression of ITGA2 has been associated with worse prognosis (68), and studies have shown that multiple epigenetic mechanisms regulate ITGA2 expression in solid tumors including altered promoter methylation (50, 69, 70). In our results, low ITGA2 expression indicates an association with mutation in IDH, methylation in MGMT in mesenchymal-like tumors, and absence of mutation in TERT and G-CIMP and proneural tumors. Tumor ITGA2 expressions have been correlated with hypermethylation in prostate cancer and hypomethylation in breast cancer (71). In the breast cancer subtype, the high expression of ITGA2 was variable and was associated with metastases and poor survival (71). In addition, the high expression of ITGA2 in tumor tissue indicates ITGA2 as an important clinical biomarker of poor prognosis in patients (19).
ITGA2 has been targeted by small molecules and antibodies as potential cancer therapies and is in clinical trials (27, 45). Recently, it has been reported that the ITGA-2 antibody inhibits cell migration, impede actin organization, and mediate cell apoptosis (27, 45). The anti-integrin therapeutics for GBM has been described as antiangiogenic, anti-invasion, and antitumor strategies (72). In immune therapy, the inhibition of ITGA2 increased the ratio of tumor-killing lymphocytes and decreased the proportion of immunosuppression-related cells in tumors (28). In another study, the authors reported that ITGA2 increased the PD-L1 expression in multiple types of cancer cells and improved the antitumor efficacy of immune therapy (27). Furthermore, immune checkpoint treatment has become a new method to treat cancer and ITGA2 can bring new insights into targeted immunotherapy and, for patient with LGG, may represent a potential molecular marker for targeted therapy (68). Therefore, it is very pivotal to find a new prognostic biomarker to enhance the treatment of glioma and increase the understanding of glioma treatment.
Our data revealed that the BglII polymorphism was significantly associated with better overall survival of LGG patient. The overrepresentation of the ITGA2 +/+ polymorphism in grade II, but not in higher-grade astrocytomas, could be explained by the hypothesis that this polymorphism could have a lower tendency to evolve to higher grades or due to the lower percentage of secondary glioblastoma cases (5%) (1), hampering the identification of genetic abnormalities. Hence, a longer and larger follow-up study would be necessary. Additionally, it remains unclear whether this polymorphism affects the synthesis or function of the integrin α2 chain and how these changes would be involved in the development or progression of low-grade astrocytomas. This correlation will be tested in future gene functional experiments.
In conclusion, our findings suggest that the BglII polymorphism in the gene which codes for the α2 chain of integrin α2β1 may have a role in the pathogenesis of low-grade astrocytomas and could help the molecular prognosis of these tumors. In addition, the increased frequency of the ITGA2 BglII polymorphism in grade II astrocytoma suggests a protective effect on the risk factor to LGG; however, more studies are necessary to correlate the polymorphism with tumor progression and overall survival. We expect that these results will provide a foundation for future research into the association between integrin α2β1 and low-grade glioma and astrocytoma progression in other populations.
Data Availability Statement
The original contributions presented in the study are included in the article/Supplementary Material. Further inquiries can be directed to the corresponding author.
Ethics Statement
The studies involving human participants were reviewed and approved by Institutional Ethics Committee of Clinical Hospital of Ribeirão Preto Medical School, University of São Paulo. The patients/participants provided their written informed consent to participate in this study.
Author Contributions
ST and RB designed the study and participated in the sample acquisition. SKNM, SMFM, SO-S, and CC contributed with patient samples and clinical information. ST and RB performed the analysis and interpretation of data and wrote the initial draft of the manuscript. LB performed the in silico analysis. MV and AA did the critical revisions and wrote sections of the manuscript. All the authors reviewed and approved the final manuscript.
Funding
This research was funded by FAPESP (Process n04/12133-6; 03/00960-2), FAEPA, CAPES, and LICR grants. Barretos Cancer Hospital, Pio XII Foundation.
Conflict of Interest
The authors declare that the research was conducted in the absence of any commercial or financial relationships that could be construed as a potential conflict of interest.
Publisher’s Note
All claims expressed in this article are solely those of the authors and do not necessarily represent those of their affiliated organizations, or those of the publisher, the editors and the reviewers. Any product that may be evaluated in this article, or claim that may be made by its manufacturer, is not guaranteed or endorsed by the publisher.
Acknowledgments
The authors are grateful to Amelia G. de Arauíjo and Julia M.Y. Komoto for the technical assistance.
Supplementary Material
The Supplementary Material for this article can be found online at: https://www.frontiersin.org/articles/10.3389/fonc.2022.914156/full#supplementary-material
References
1. Ohgaki H, Kleihues P. The Definition of Primary and Secondary Glioblastoma. Clin Cancer Res (2013) 19:764–72. doi: 10.1158/1078–0432.CCR–12–3002
2. Stupp R, Roila F. Malignant Glioma: ESMO Clinical Recommendations for Diagnosis, Treatment and Follow–Up. Ann Oncol (2009) 20:iv126–8. doi: 10.1093/annonc/mdp151
3. Zhang X, Zhang W, Cao WD, Cheng G, Zhang YQ. Glioblastoma Multiforme: Molecular Characterization and Current Treatment Strategy (Review). Exp Ther Med (2012) 3:9–14. doi: 10.3892/etm.2011.367
4. Louis DN, Perry A, Reifenberger G, von Deimling A, Figarella–Branger D, Cavenee WK, et al. The 2016 World Health Organization Classification of Tumors of the Central Nervous System: A Summary. Acta Neuropathol (2016) 131:803–20. doi: 10.1007/s00401–016–1545–1
5. Ruiz–Ontanãon P, Orgaz JL, Aldaz B, Elosegui–Artola A, Martino J, Berciano MT, Montero JA, et al. Cellular Plasticity Confers Migratory and Invasive Advantages to a Population of Glioblastoma–Initiating Cells That Infiltrate Peritumoral Tissue. Stem Cells (2013) 31:1075–85. doi: 10.1002/stem.1349
6. Hynes RO. Integrins: Versatility, Modulation, and Signaling in Cell Adhesion. Cell (1992) 69:11–25. doi: 10.1016/0092–8674(92)90115–S
7. Ringer P, Colo G, Faüssler R, Grashoff C. Sensing the Mechano–Chemical Properties of the Extracellular Matrix. Matrix Biol (2017) 64:6–16. doi: 10.1016/j.matbio.2017.03.004
8. Uhm HJ. The Role of Integrins in the Malignant Phenotype of Gliomas. Front Biosci (1999) 4:d188. doi: 10.2741/Uhm
9. Uematsu T, Konishi C, Hoshino D, Han X, Tomari T, Egawa N, et al. Identification of Proteins That Associate With Integrin α2 by Proteomic Analysis in Human Fibrosarcoma HT–1080 Cells. J Cell Physiol (2012) 227:3072–9. doi: 10.1002/jcp.23054
10. Danen EHJ. Integrin Signaling as a Cancer Drug Target. ISRN Cell Biol (2013) 2013:1–14. doi: 10.1155/2013/135164
11. Ellert–Miklaszewska A, Poleszak K, Pasierbinska M, Kaminska B. Integrin Signaling in Glioma Pathogenesis: From Biology to Therapy. Int J Mol Sci (2020) 21:888. doi: 10.3390/ijms21030888
12. Friedlander D, Zagzag D, Shiff B, Cohen H, Allen J, Kelly P, et al. Migration of Brain Tumor Cells on Extracellular Matrix Proteins In Vitro Correlates With Tumor Type and Grade and Involves alphaV and Beta1 Integrins. Cancer Res (1996) 56:1939–47.
13. Quader S, Liu X, Chen Y, Mi P, Chida T, Ishii T, et al. cRGD Peptide–Installed Epirubicin–Loaded Polymeric Micelles for Effective Targeted Therapy Against Brain Tumors. J Controlled Release (2017) 258:56–66. doi: 10.1016/j.jconrel.2017.04.033
14. Wadajkar AS, Dancy JG, Hersh DS, Anastasiadis P, Tran NL, Woodworth GF, et al. Tumor–targeted Nanotherapeutics: Overcoming Treatment Barriers for Glioblastoma. WIREs Nanomedicine Nanobiotechnology (2017) 9(4):e1439. doi: 10.1002/wnan.1439
15. Delamarre E, Taboubi S, Mathieu S, Beírenguer C, Rigot V, Lissitzky JC, et al. Expression of Integrin α6β1 Enhances Tumorigenesis in Glioma Cells. Am J Pathol (2009) 175:844–55. doi: 10.2353/ajpath.2009.080920
16. Gingras Mc, Roussel E, Bruner JM, Branch CD, Moser P. Comparison of Cell Adhesion Molecule Expression Between Glioblastoma Multiforme and Autologous Normal Brain Tissue. J Neuroimmunol (1995) 57:143–53. doi: 10.1016/0165–5728(94)00178–Q
17. Paolillo M, Serra M, Schinelli S. Integrins in Glioblastoma: Still an Attractive Target? Pharmacol Res (2016) 113:55–61. doi: 10.1016/j.phrs.2016.08.004
18. Meldolesi J. Pharmacology of the Cell/Matrix Form of Adhesion. Pharmacol Res (2016) 107:430–6. doi: 10.1016/j.phrs.2015.10.019
19. Su C, Li J, Zhang L, Wang H, Wang F, Tao Y, et al. The Biological Functions and Clinical Applications of Integrins in Cancers. Front Pharmacol (2020) 11:579068. doi: 10.3389/fphar.2020.579068
20. Teodorczyk M, Martin–Villalba A. Sensing Invasion: Cell Surface Receptors Driving Spread– Ing of Glioblastoma. J Cell Physiol (2010) 222:1–10. doi: 10.1002/jcp.21901
21. Friedl P, Wolf K. Proteolytic and non–Proteolytic Migration of Tumour Cells and Leucocytes. Biochem Soc Symp (2003) 70:277–85. doi: 10.1042/bss0700277
22. Selistre–de Araujo H, Cominetti M, Terruggi C, Mariano–Oliveira A, De Freitas M, Crepin M, et al. Alternagin–C, a Disintegrin–Like Protein From the Venom of Bothrops Alternatus, Modulates Alpha2ß1 Integrin–Mediated Cell Adhesion, Migration and Proliferation. Braz J Med Biol Res (2005) 38:1505–11. doi: 10.1590/S0100–879X2005001000007
23. Van de Walle GR, Vanhoorelbeke K, Majer Z, Illyeís E, Baert J, Pareyn I, et al. Two Functional Active Conformations of the Integrin α2β1, Depending on Activation Condition and Cell Type. J Biol Chem (2005) 280:36873–82. doi: 10.1074/jbc.M508148200
24. Wang L, Gao Y, Zhao X, Guo C, Wang X, Yang Y, et al. HOXD3 was Negatively Regulated by YY1 Recruiting HDAC1 to Suppress Progression of Hepatocellular Carcinoma Cells via ITGA2 Pathway. Cell Prolif (2020) 53(8):e12835. doi: 10.1111/cpr.12835
25. Ding W, Fan XL, Xu X, Huang JZ, Xu SH, Geng Q, et al. Epigenetic Silencing of ITGA2 by MiR–373 Promotes Cell Migration in Breast Cancer. PLoS One (2015) 10(8):e0135128. doi: 10.1371/journal.pone.0135128
26. Lei X, Lei Y, Li JK, Du WX, Li RG, Yang J, et al. Immune Cells Within the Tumor Microenvironment: Biological Functions and Roles in Cancer Immunotherapy. Cancer Lett (2020) 470:126–33. doi: 10.1016/j.canlet.2019.11.009
27. Guo P, Moses–Gardner A, Huang J, Smith ER, Moses MA. ITGA2 as a Potential Nanotherapeutic Target for Glioblastoma. Sci Rep (2019) 9(1):6195. doi: 10.1038/s41598–019–42643–7
28. Ren D, Zhao J, Sun Y, Li D, Meng Z, Wang B, et al. Overexpressed ITGA2 Promotes Malignant Tumor Aggression by Up–Regulating PD–L1 Expression Through the Activation of the STAT3 Signaling Pathway. J Exp Clin Cancer Res (2019) 38(1):485. doi: 10.1186/s13046–019–1496–1
29. Kamata T, Puzon W, Takada Y. Identification of Putative Ligand Binding Sites Within I Domain of Integrin Alpha 2 Beta 1 (VLA–2, CD49b/Cd29). J Biol Chem (1994) 269:9659–63. doi: 10.1016/S0021–9258(17)36932–6
30. Takada Y, Elices MJ, Crouse C, Hemler ME. The Primary Structure of the Alpha 4 Subunit of VLA–4: Homology to Other Integrins and a Possible Cell–Cell Adhesion Function. EMBO J (1989) 8:1361–8. doi: 10.1002/j.1460–2075.1989.tb03516.x
31. Kritzik M, Savage B, Nugent DJ, Santoso S, Ruggeri ZM, Kunicki TJ. Nucleotide Polymorphisms in the α2 Gene Define Multiple Alleles That are Associated With Differences in Platelet α2β1 Density. Blood (1998) 92:2382–8. doi: 10.1182/blood.V92.7.2382
32. Ayala F, Corral J, Gonzaílez–Conejero R, Saínchez I, Mariía Moraleda J, Vicente V. Genetic Polymorphisms of Platelet Adhesive Molecules: Association With Breast Cancer Risk and Clinical Presentation. Breast Cancer Res Treat (2003) 80:145–54. doi: 10.1023/A:1024598732178
33. Vairaktaris E, Yapijakis C, Derka S, Vassilio S, Serefoglou Z, Vylliotis A, et al. Association of Platelet Glycoprotein Ia Polymorphism With Minor Increase of Risk for Oral Cancer. Eur J Surg Oncol (EJSO) (2006) 32:455–7. doi: 10.1016/j.ejso.2005.12.005
34. Carlsson LE, Santoso S, Spitzer C, Kessler C, Greinacher A. The Alpha2 Gene Coding Sequence T807/A873 of the Platelet Collagen Receptor Integrin Alpha2beta1 Might be a Genetic Risk Factor for the Development of Stroke in Younger Patients. Blood (1999) 93:3583–6. doi: 10.1182/blood.V93.11.3583
35. Moshfegh K, Wuillemin WA, Redondo M, Laümmle B, Beer JH, Liechti–Gallati S, et al. Association of Two Silent Polymorphisms of Platelet Glycoprotein La/Lla Receptor With Risk of Myocardial Infarction: A Case–Control Study. Lancet (1999) 35:351–4. doi: 10.1016/S0140–6736(98)06448–4
36. Matsubara Y, Murata M, Maruyama T, Handa M, Yamagata N, Watanabe G, et al. Association Between Diabetic Retinopathy and Genetic Variations in Alpha2beta1 Integrin, a Platelet Receptor for Collagen. Blood (2000) 95:1560–4. doi: 10.1182/blood.V95.5.1560.005k43_1560_1564
37. Samur MK. RTCGAToolbox: A New Tool for Exporting TCGA Firehose Data. PloS One (2014) 9(9):e106397. doi: 10.1371/journal.pone.0106397
38. Noushmehr H, Weisenberger DJ, Diefes K, Phillips HS, Pujara K, Berman BP, et al. Cancer Genome Atlas Research Network. Identification of a CpG Island Methylator Phenotype That Defines a Distinct Subgroup of Glioma. Cancer Cell (2010) 17(5):510–22. doi: 10.1016/j.ccr.2010.03.017
39. Ceccarelli M, Barthel FP, Malta TM, Sabedot TS, Salama SR, Murray BA, et al. Molecular Profiling Reveals Biologically Discrete Subsets and Pathways of Progression in Diffuse Glioma. Cell (2016) 3):550–63. doi: 10.1016/j.cell.2015.12.028
40. Poglajen G, Kirbis J, Milutinovic í A. The –455g/A Polymorphism of the Beta Fibrinogen Gene and the Bgl II Polymorphism of the Alpha2beta1 Integrin Gene and Myocardial Infarction in Patients With Type 2 Diabetes. Folia Biologica (2004) 5:203–4.
41. Ihnatko R, Kubes M, Takacova M, Sedlakova O, Sedlak J, Pastorek J, et al. Extracellular Acidosis Elevates Carbonic Anhydrase IX in Human Glioblastoma Cells via Transcriptional Modulation That Does Not Depend on Hypoxia. Int J Oncol (2006) 19(4):1025-33. doi: 10.3892/ijo.29.4.1025
42. Huang CW, Li Z, Conti PS. In Vivo Near–Infrared Fluorescence Imaging of Integrin α2β1 in Prostate Cancer With Cell–Penetrating–Peptide–Conjugated DGEA Probe. J Nucl Med (2011) 52:1979–86. doi: 10.2967/jnumed.111.091256
43. Girotti MR, Fernaíndez M, Loípez JA, Camafeita E, Fernaíndez EA, Albar JP, et al. SPARC Promotes Cathepsin B–mediated Melanoma Invasiveness Through a Collagen I/ α2β1 Integrin Axis. J Invest Dermatol (2011) 131:2438–47. doi: 10.1038/jid.2011.239
44. Tran T, Barlow B, O’Rear L, Jarvis B, Li Z, Dickeson K, et al. Loss of the α2β1 Integrin Alters Human Papilloma Virus–Induced Squamous Carcinoma Progression In Vivo and In Vitro. PLoS One (2011) 6:e26858. doi: 10.1371/journal.pone.0026858
45. Chuang YC, Wu HY, Lin YL, Tzou SC, Chuang CH, Jian TY, et al. Blockade of ITGA2 Induces Apoptosis and Inhibits Cell Migration in Gastric Cancer. Biol Proced Online (2018) 20:10. doi: 10.1186/s12575–018–0073–x
46. Ojalill M, Parikainen M, Rappu P, Aalto E, Jokinen J, Virtanen N, et al. Integrin α2β1 Decelerates Proliferation, But Promotes Survival and Invasion of Prostate Cancer Cells. Oncotarget (2018) 9(65):32435–47. doi: 10.18632/oncotarget.25945
47. Salemi Z, Azizi R, Fallahian F, Aghaei M. Integrin α2β1 Inhibition Attenuates Prostate Cancer Cell Proliferation by Cell Cycle Arrest, Promoting Apoptosis and Reducing Epithelial–Mesenchymal Transition. J Cell Physiol (2021) 236(7):4954–65. doi: 10.1002/jcp.30202
48. Madamanchi A, Santoro SA, Zutter MM. α2β1 Integrin. Adv Exp Med Biol (2014) 819:41–60. doi: 10.1007/978–94–017–9153–3_3
49. Naci D, Vuori K, Aoudjit F. Alpha2beta1 Integrin in Cancer Development and Chemoresistance. Semin Cancer Biol (2015) 35:145–53. doi: 10.1016/j.semcancer.2015.08.004
50. Verhoeff TJ, Holloway AF, Dickinson JL. A Novel Long non–Coding RNA Regulates the Integrin, ITGA2 in Breast Cancer. Breast Cancer Res Treat (2022) 192(1):89–100. doi: 10.1007/s10549–021–06496–x
51. Yilmaz U, Zeybek U, Kahraman OT, Kafadar AM, Toptas B, Yamak N, et al. Investigation of ICAM–1 and 3 Integrin Gene Variations in Patients With Brain Tumors. Asian Pacific J Cancer Prev (2013) 14:5929–34. doi: 10.7314/APJCP.2013.14.10.5929
52. Kunicki TJ, Kritzik M, Annis DS, Nugent DJ. Hereditary Variation in Platelet Integrin Alpha 2 Beta 1 Density Is Associated With Two Silent Polymorphisms in the Alpha 2 Gene Coding Sequence. Blood (1997) 89:1939–43. doi: 10.1182/blood.V89.6.1939
53. Liu CZ, Wang YC, Wang JH, Shaw CK, Lin GL. Differential Expression of Platelet Glycoprotein Ia/IIa in Taiwan Chinese Corresponds to Glycoprotein Ia Gene Polymorphisms. J Pharmacol Sci (2006) 101:103–6. doi: 10.1254/jphs.SC0050174
54. Nierodzik ML, Klepfish A, Karpatkin S. Role of Platelets, Thrombin, Integrin IIb–IIIa, Fibronectin and Von Willebrand Factor on Tumor Adhesion In Vitro and Metastasis In Vivo. Thromb Haemostasis (1995) 74:282–90.
55. Dardik R, Kaufmann Y, Savion N, Rosenberg N, Shenkman B, Varon D. Platelets Mediate Tumor Cell Adhesion to the Subendothelium Under Flow Conditions: Involvement of Platelet GPIIb–IIIa and Tumor Cell Integrins. Int J Cancer (1997) 70:201–7. doi: 10.1002/(SICI)1097-0215(19970117)70:2<201::AID-IJC11>3.0.CO;2-
56. Grzesiak JJ, Bouvet M. The α2β1 Integrin Mediates the Malignant Phenotype on Type I Collagen in Pancreatic Cancer Cell Lines. Br J Cancer (2006) 94:1311–9. doi: 10.1038/sj.bjc.6603088
57. Ferraro A, Boni T, Pintzas A. EZH2 Regulates Cofilin Activity and Colon Cancer Cell Migration by Targeting ITGA2 Gene. PLoS One (2014) 9(12):e115276. doi: 10.1371/journal.pone.0115276
58. Grossi IM, Hatfield JS, Fitzgerald LA, Newcombe M, Taylor JD, Honn KV. Role of Tumor Cell Glycoproteins Immunologically Related to Glycoproteins Ib and IIb/IIIa in Tumor Cell–Platelet and Tumor Cell–Matrix Interactions. FASEB J (1988) 2:2385–95. doi: 10.1096/fasebj.2.8.2452113
59. Gui G, Puddefoot J, Vinson G, Wells C, Carpenter R. Altered Cell–Matrix Contact: A Prerequisite for Breast Cancer Metastasis? Br J Cancer (1997) 75:623–33. doi: 10.1038/bjc.1997.113
60. Miyake K, Kimura S, Nakanishi M, Hisada A, Hasegawa M, Nagao S, et al. Transforming Growth Factor–1 Stimulates Contraction of Human Glioblastoma Cell–Mediated Collagen Lattice Through Enhanced α2 Integrin Expression. J Neuropathol Exp Neurol (2000) 59:18–28. doi: 10.1093/jnen/59.1.18
61. Mahesparan R, Read TA, Lund–Johansen M, Skaftnesmo K, Bjerkvig R, Engebraaten O. Expression of Extracellular Matrix Components in a Highly Infiltrative In Vivo Glioma Model. Acta Neuropathol (2003) 105:49–57. doi: 10.1007/s00401–002–0610–0
62. Rooprai HK, Vanmeter T, Panou C, Schnüll S, Trillo–Pazos G, Davies D, et al. The Role of Integrin Receptors in Aspects of Glioma Invasion In Vitro. Int J Dev Neurosci (1999) 17:613–23. doi: 10.1016/S0736–5748(99)00051–9
63. Paulus W, Baur I, Beutler AS, Reeves SA. Diffuse Brain Invasion of Glioma Cells Requires Beta 1 Integrins. Lab Investigation J Tech Methods Pathol (1996) 75:819–26.
64. Di Paola J, Jugessur A, Goldman T, Reiland J, Tallman D, Sayago C, et al. Platelet Glycoprotein I(b)alpha and Integrin Alpha2 Beta1 Polymorphisms: Gene Frequencies and Linkage Disequilibrium in a Population Diversity Panel. J Thromb Haemost (2005) 3(7):1511–21. doi: 10.1111/j.1538–7836.2005.01273.x
65. Casorelli I, De Stefano V, Leone AM, Chiusolo P, Burzotta F, Paciaroni K, et al. The C807T/G873A Polymorphism in the Platelet Glycoprotein Ia Gene and the Risk of Acute Coronary Syndrome in the Italian Population. Br J Haematol (2001) 114(1):150–4. doi: 10.1046/j.1365–2141.2001.02868.x
66. Corral J, González–Conejero R, Rivera J, Ortuño F, Aparicio P, Vicente V. Role of the 807 C/T Polymorphism of the Alpha2 Gene in Platelet GP Ia Collagen Receptor Expression and Function–Effect in Thromboembolic Diseases. Thromb Haemost (1999) 81(6):951–6.
67. Arsène S, Pouplard C, Perrodeau E, Delbachian I, Giraudeau B, Maillot F, et al. No Association Between the ITGA2 807t Allele and Retinopathy in French Patients With Type 2 Diabetes. Thromb Res (2011) 128(3):293–5. doi: 10.1016/j.thromres.2011.05.009
68. Lin L, Huang K, Tu Z, Zhu X, Li J, Lei K, et al. Integrin Alpha–2 as a Potential Prognostic and Predictive Biomarker for Patients With Lower–Grade Glioma. Front Oncol (2021) 11:738651. doi: 10.3389/fonc.2021.738651
69. Chin SP, Marthick JR, West AC, Short AK, Chuckowree J, Polanowski AM, et al. Regulation of the ITGA2 Gene by Epigenetic Mechanisms in Prostate Cancer. Prostate (2015) 75(7):723–34. doi: 10.1002/pros.22954
70. Wafai R, Williams ED, de Souza E, Simpson PT, McCart Reed AE, Kutasovic JR, et al. Integrin Alpha–2 and Beta–1 Expression Increases Through Multiple Generations of the EDW01 Patient–Derived Xenograft Model of Breast Cancer–Insight Into Their Role in Epithelial Mesenchymal Transition In Vivo Gained From an In Vitro Model System. Breast Cancer Res (2020) 22(1):136. doi: 10.1186/s13058–020–01366–8
71. Adorno–Cruz V, Hoffmann AD, Liu X, Dashzeveg NK, Taftaf R, Wray B, et al. ITGA2 Promotes Expression of ACLY and CCND1 in Enhancing Breast Cancer Stemness and Metastasis. Genes Dis (2020) 8(4):493–508. doi: 10.1016/j.gendis.2020.01.015
Keywords: single nucleotide polymorphism, extracellular matrix, brain microenvironment, tumor progression, low grade glioma, invasion, ITGA2
Citation: Teixeira SA, Burim RV, Viapiano MS, Bidinotto LT, Nagashi Marie SK, Fleury Malheiros SM, Oba-Shinjo SM, Andrade AF and Carlotti CG (2022) Alpha2beta1 Integrin Polymorphism in Diffuse Astrocytoma Patients. Front. Oncol. 12:914156. doi: 10.3389/fonc.2022.914156
Received: 06 April 2022; Accepted: 24 June 2022;
Published: 22 July 2022.
Edited by:
Giovana Tardin Torrezan, A.C.Camargo Cancer Center, BrazilReviewed by:
Aloukick Kumar Singh, Center for Stem Cell Research (CSCR), IndiaJuliano Andreoli Miyake, Federal University of Santa Catarina, Brazil
Copyright © 2022 Teixeira, Burim, Viapiano, Bidinotto, Nagashi Marie, Fleury Malheiros, Oba-Shinjo, Andrade and Carlotti. This is an open-access article distributed under the terms of the Creative Commons Attribution License (CC BY). The use, distribution or reproduction in other forums is permitted, provided the original author(s) and the copyright owner(s) are credited and that the original publication in this journal is cited, in accordance with accepted academic practice. No use, distribution or reproduction is permitted which does not comply with these terms.
*Correspondence: Silvia A. Teixeira, c2F0ZWl4ZWlyYUBhbHVtbmkudXNwLmJy; c3RlaXhlaXJhLmhjYkBnbWFpbC5jb20=